- 1Centre For Advanced Formulation and Technology, Delhi Pharmaceutical Sciences and Research University, New Delhi, India
- 2Department of Pharmaceutics, College of Pharmacy, Jazan University, Jazan, Saudi Arabia
- 3Department of Pharmaceutical Chemistry, College of Pharmacy, Jazan University, Jazan, Saudi Arabia
- 4Department of Clinical Pharmacy, College of Pharmacy, Jazan University, Jazan, Saudi Arabia
Breast cancer being one of the most frequent cancers in women accounts for almost a quarter of all cancer cases. Early and late-stage breast cancer outcomes have improved dramatically, with considerable gains in overall survival rate and disease-free state. However, the current therapy of breast cancer suffers from drug resistance leading to relapse and recurrence of the disease. Also, the currently used synthetic and natural agents have bioavailability issues which limit their use. Recently, nanocarriers-assisted delivery of synthetic and natural anticancer drugs has been introduced to the breast cancer therapy which alienates the limitations associated with the current therapy to a great extent. Significant progress has lately been made in the realm of nanotechnology, which proved to be vital in the fight against drug resistance. Nanotechnology has been successfully applied in the effective and improved therapy of different forms of breast cancer including invasive, non-invasive as well as triple negative breast cancer (TNBC), etc. This review presents a comprehensive overview of various nanoformulations prepared for the improved delivery of synthetic and natural anticancer drugs alone or in combination showing better efficacy and pharmacokinetics. In addition to this, various ongoing and completed clinical studies and patents granted on nanotechnology-based breast cancer drug delivery are also reviewed.
1 Introduction
Breast cancer is the most common form of cancers amongst all types with 2.3 million cases diagnosed annually (Figueroa et al., 2021). Breast cancer-related mortality in women in the United States alone accounts for about 41,000 per year, or 15% of all cancer-related deaths (Liang et al., 2020). Early detection and extensive treatment techniques has decreased the cancer mortality in the last 2 decades improving the prognosis of breast cancer patients (Ganz and Goodwin, 2015). Considerable advancements have been made in the screening, diagnosis and treatment options for breast cancer (Fahad Ullah, 2019). Breast cancer starts with physiologically and molecularly diverse conditions in the breast, risk factors varying according to the type and genetic predisposition. Most notably mutations in the BRCA1 or BRCA2 genes remain significant component (Feng et al., 2018).
Treating breast cancer using estrogen receptor (ER) antagonists is the most established molecular targeted therapy approach as compared to other receptors (Sharma et al., 2018; Damodaran and Hortobagyi, 2021). Furthermore, the widespread use of tamoxifen, a selective ER modulator in treating breast cancer has been attributed with considerable advances in cure rates, quality of life, and preventing disease over the past quarter-century. Alternative therapies are also available for estrogen-dependent breast cancer which includes the use of aromatase inhibitors (AI) (anastrozole, letrozole, and exemestane) that impede the biosynthesis of androgens in tumor by down-regulating the aromatase enzyme (Chumsri et al., 2011). This results in decreased estrogen levels in tumor cells. Combination of receptor targets with chemotherapy at the molecular level have also been a significant step forward in the breast cancer treatment. Some of these combination targets include human epidermal growth factor receptor-2 (HER2) and the vascular endothelial growth factor (VEGF), which are used in tandem with chemotherapy drugs such as trastuzumab and bevacizumab (Munagala et al., 2011; Toss and Cristofanilli, 2015). In addition, inhibitors of downstream pathways such as PI3K/AKT/mTOR and RAS/MEK/ERK have the potential to be used therapeutically in some cases. There have also been reports of other tyrosine kinases being involved in cancer cell cancer progression and metastasis, such as insulin-like growth factor receptor (IGFR), poly-ADP ribose polymerase (PARP), and matrix metalloproteinases (MMP) inhibitors (Masoud and Pagès, 2017a). Several of these targeted medications showed significant improvements in the survival and prognosis of breast cancer patients. The MMP inhibitors work by blocking the activity of MMPs, a family of enzymes involved in the breakdown of extracellular matrix (ECM) proteins. ECM breakdown is an important step in cancer invasion and metastasis. By inhibiting MMPs, MMP inhibitors may prevent cancer cells from invading and spreading to other parts of the body. These MMP inhibitors include Batimastat, Marimastat, Prinomastat, BAY 12–9,566, Tanomastat, etc., The PARP inhibitors work by blocking the activity of poly (ADP-ribose)polymerase (PARP), an enzyme involved in DNA repair. PARP inhibitors specifically target cancer cells that have mutations in the BRCA1 and BRCA2 genes, which are involved in DNA repair. By inhibiting PARP, these cells are unable to repair DNA damage, leading to cell death. Some examples of drugs acting as PARP inhibitors are Olaparib, Rucaparib, Niraparib, Talazoparib, Veliparib, etc.
Significant advancements were made recently in the research aimed at targeting the molecular pathways of breast cancer, which have helped in understanding the targets at molecular level and developing targeted therapies to tackle it. Identifying and blocking the pathways that promote or perpetuate the proliferation and invasion of breast carcinoma cells is required for long-term efficacy in treating breast cancer. Over the past 2 decades, various monoclonal antibodies and small-molecule inhibitors have been proposed in clinical trials for their anticancer properties (Schlotter et al., 2008). Breast cancer has several molecular target pathways, including the EGFR Family, the vascular endothelial growth factor (VEGF) family, the RAS/MEK/ERK Pathway, cell cycle and apoptosis, estrogen receptor (ER) antagonist, targeting invasion and metastasis, insulin-like growth factor inhibitors, and the steroid hormone receptor.
However, the existing breast cancer therapy suffers from various issues including recurrence and relapse. The recurrence and relapse of breast cancer is mainly due to the resistance to conventional chemotherapeutic drugs and it is the leading cause of mortality despite significant research breakthroughs in the breast cancer therapy (Gote et al., 2021). Pharmacokinetics and metabolism of the tumor changes by the drug resistance and tumor protein P53, ATP-binding cassette (ABC), microtubules (MT), permeability glycoprotein (P-gp), HER2, topoisomerase, and breast cancer type 1 (BRAC1) lead to drug resistance in various ways. It also affects the mitochondrial metabolism, fatty acid synthesis, redox metabolism, and glycolysis in the breast cancer cells. All these resistance pathways are induced by the drugs including lapatinib, trastuzumab, adriamycin, paclitaxel, and tamoxifen (Gonzalez-Angulo et al., 2007). Owing to the fact that tumors contain distinct cancer-causing genes which frequently undergo several mutations, modified treatments can be achieved by identifying innovative multi-target agents and combining them. Chemotherapeutic drugs given intravenously result in widespread systemic dispersion and drug toxicity in cancer cells (Vasan et al., 2019). As a result, therapeutic medications that target non-cancerous cells and tumor locations are unable to provide adequate drug dose, resulting in poor therapy. Furthermore, the existing treatment is beset by low solubility and bioavailability of drugs at cancer locations. Numerous techniques are being adopted in order to overcome the drug resistance including application of nanotechnology, development of novel synthetic analogues of currently used drugs, repurposing drugs, combination of drugs, immunotherapy, patient monitoring, and synthetic lethality. Among these techniques, application of nanotechnology in preparing the nanoformulations of existing synthetic and natural anticancer molecules has garnered much attention and significant advancements have been made in this field.
Nanotechnology is one of the fastest growing fields of science and technology in the world; and it is frequently applied in breast cancer theranostics. As time progresses, nanotechnology aids in the discovery of novel research methodologies in oncology, even at the molecular level. Nanoparticles are unique in different ways and they can be used as nanomedicine in therapeutic applications. This review focuses on the recent studies aimed to enhance the targeted delivery of anticancer molecules of synthetic and natural origin using nanotechnology and its role in combating the drug resistance. Moreover, this article includes a research study based on the selection of an anticancer dose using combination index since drug combinations can have synergistic, antagonistic, or additive effects. Using the combination index, the dose of a combination of natural and synthetic drug was determined on two breast cancer cell lines, MCF-7 and T47D.
2 Nanomaterials to combat drug resistance
Drug resistance has become a significant concern in cancer therapy as the number of cancer treatment options is growing day by day. Cancer progression and a bad prognosis are caused by multidrug resistance, which results in the failure of cancer therapy. The mechanisms underlying drug resistance are well understood now. Interstitial fluid pressure, overexpression of the ATP binding cassette (ABC) efflux transporters, faulty apoptotic machinery, and a hypoxic or acidic tumor microenvironment are some of the factors implicated in medication resistance (Yao et al., 2020). Nanotechnology-based drug delivery devices play a crucial role in the treatment of cancer patients who developed drug resistance to conventional treatments. Nanoparticles that target the processes involved in drug resistance may be able to improve their effectiveness (Mba and Nweze, 2021). Targeting efflux transporters, which are the members of ABC transporter family, also play a significant role in the development of drug resistance. This transporter causes the drug to be effluxed out of the cell, reducing the therapeutic effect. P-glycoprotein (P-gp) is the most important efflux transporter and is overexpressed in some drug-resistant cancers (Waghray and Zhang, 2018; Robinson and Tiriveedhi, 2020). Nanoparticles can assist in bypassing the medication through the efflux transporter in these circumstances and polymer based nanoparticles can inhibit P-gp and change the regulation of drug release (Peng et al., 2020).
It has been reported that the nanoparticles containing COX-2 inhibitors along with doxorubicin were able to combat the multidrug resistance of breast cancer cells (Zhang et al., 2019). Furthermore, by suppressing the ABC transporters expression, co-delivery of anticancer agents with P-gp-targeted si-RNA using nanoparticles were found to aid in the treatment of drug-resistant tumors (Mirzaei et al., 2022). It was revealed that the lipid-based nanoparticles containing surfactant and co-surfactant helped in the prevention of drug from being effluxed by P-gp (Mangla et al., 2020). Cancer cells that have malfunctioned apoptotic machinery are able to circumvent apoptosis and improve their survival leading to drug resistance. Discrepancies in the apoptotic pathway are frequently produced by de-regulation of the nuclear factor-kappa B (NF-κB) and the anti-apoptotic protein Bcl-2; both of which are overexpressed in many tumors (Rinkenbaugh and Baldwin, 2016; Singh et al., 2019a). The nanoparticle-based delivery system exhibited improved cytotoxicity by causing apoptosis via downregulation of NF-κB and Bcl-2 expression along with the suppression of efflux transporter expression, thereby promoting apoptosis (Haider et al., 2022). The upregulation of drug efflux proteins has been shown to be mediated by hypoxia. Hypoxia creates a oxygen gradient inside the tumor which increases the heterogeneity of tumor and promotes more aggressive phenotype in the tumor cell itself. A hypoxia-inducible factor (HIF-1) has been identified to be overexpressed in a number of human cancer forms and plays a key role in treatment of drug resistance (Jun et al., 2017). Another therapeutic technique to overcome drug resistance is to target HIF-1 and nanoparticles such as PEGylated or non-PEGylated liposomes carrying HIF-1 siRNA were found to assist in treating hypoxia and drug resistance in cancer cells (Hajizadeh et al., 2020).
3 Nanomaterials from synthetic molecules
The drug therapy of breast cancer is still suboptimal owing to various issues affecting the efficacies of the currently used synthetic drugs. Major challenges associated with the existing therapy include insufficient biodistribution of drugs in the body which further decreases in the tumor tissues (Senapati et al., 2018). Drugs are more distributed to the healthy tissues causing toxicity and less into the tumor causing decreased efficacy. The microenvironment of tumor and drug resistance is other reason responsible for problem in the conventional therapy (Mansoori et al., 2017). Nanotechnology can be applied to overcome some of these limitations as they offer larger surface area-to-volume ratio providing good opportunity to manipulate the surface properties of drugs (Navya et al., 2019).
The fundamental goals of nanomedicine are to accurately diagnose and treat patients with minimal adverse effects; as well as to evaluate the efficiency of non-invasive therapeutic techniques (Longo et al., 2021). Nanomaterials used as carriers for the anticancer drugs utilized in the breast cancer treatment are broadly classified into two categories: organic and inorganic. Liposomes, micelles, dendrimers, and cyclodextrin are examples of organic materials; whereas inorganic materials include iron oxide, gold nanoparticles, and mesoporous silica nanoparticles (Gupta et al., 2021) (Figure 1).
Nanotechnology can help achieve improved treatment in many ways including cancer targeting, increased endocytosis and extended circulation time which improves the access of anticancer drugs to the tumor sites (Figure 2). Moreover, encapsulating the drugs into the nanocarrier improves their solubility, stability and controlled release. Using nanocarriers, even the combination of drugs can be co-delivered to the target site in order to achieve synergistic anticancer activities. The magnetic nanoparticles on the other hand can also be utilized for the guided therapy, imaging as well as in drug delivery using external magnetic filed.
Numerous studies have been conducted on nanoparticles to assess their applications in breast cancer research. Some of the important nanocarriers used in breast cancer treatment as well as the dose and mode of administration are summarized in Table 1. These nanoformulations shown including NLCs, liposomes, phospholipid complexes, transferrin conjugates, solid lipid nanoparticles, β-cyclodextrin inclusion complexes, PEGylated liposomes, albumin-coated nanocrystals, cannabidiol-loaded microparticles, and chitosan-folate-coated mesoporous silica particles, have shown prominent effects against breast cancer cells. Synthetic drugs including exemestane, sulforaphane, tamoxifen, vitamin D3, etoposide and their combinations have been delivered using the nanoparticulate systems for effective breast cancer therapy.
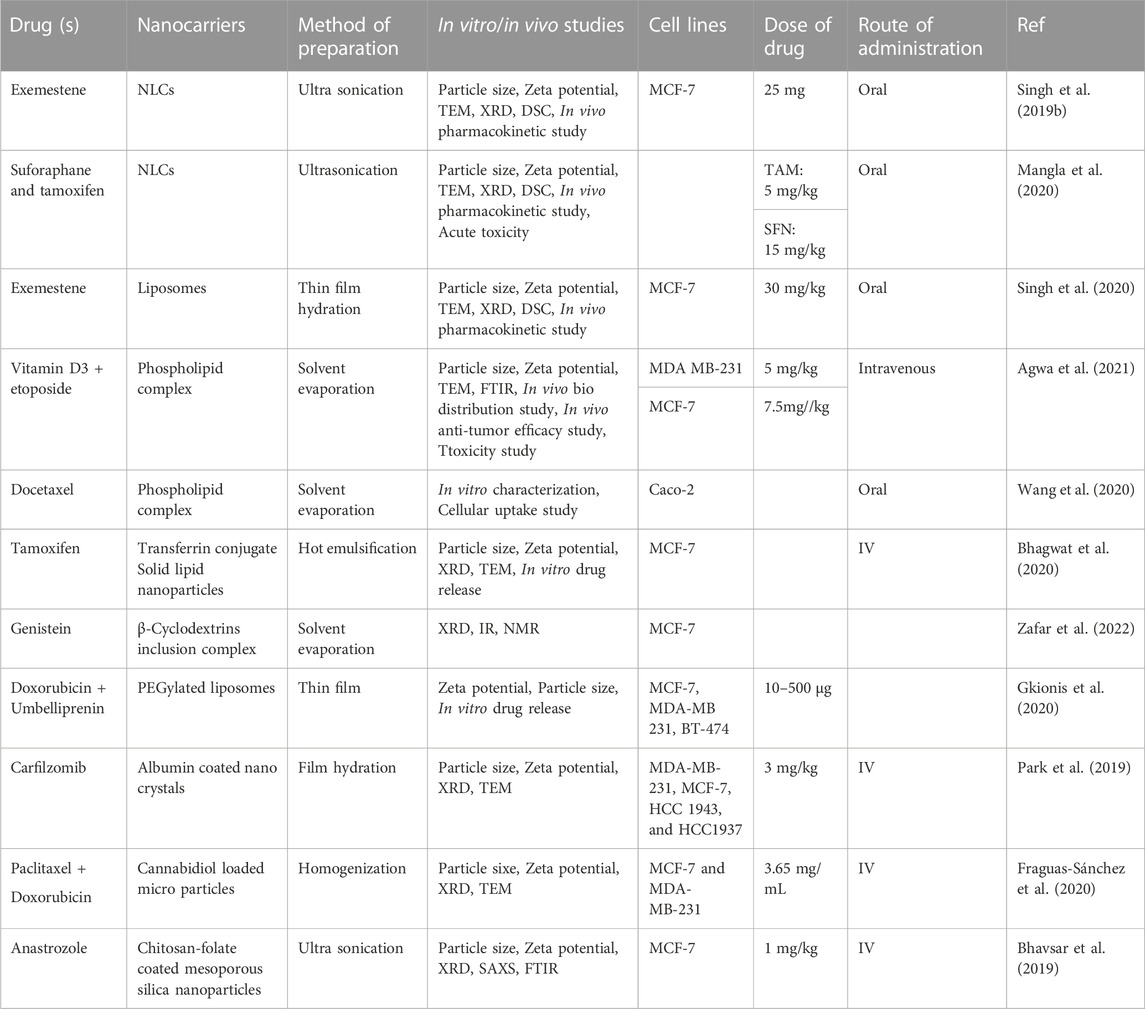
TABLE 1. Synthetic drugs and their combinations delivered using nanotechnology for the treatment of breast cancer.
One of the synthetic drugs, Exemestane (EXE) which is a steroidal aromatase inhibitor and has been approved for the breast cancer therapy, was incorporated into NLCs as the drug encounters from the problem of limited water solubility and therefore, lesser oral bioavailability (Singh et al., 2019b). The EXE-NLCs showed extended release of the drug for upto 24 h in vitro and an augmented bioavailability by 3.9 folds was observed. Also, the permeation across the gut wall was shown to be improved as compared to the plain drug suspension. In an effort to explore the effects of combination of synthetic and natural molecules, tamoxifen (TAM) was loaded with sulforaphane (SFN) into a NLC system with the aim to enhance their oral delivery and to reduce the toxicity of TAM using SFN (Mangla et al., 2020). Formulation into NLCs was shown to enhance the intestinal permeability of TAM and SFN resulting into augmented oral bioavailability of TAM and SFN by 5.2 and 4.8 folds, respectively. Liposomes have been proved to be one of the most successful nanocarriers for the oral delivery of anticancer drugs; however, the harsh GI environment poses a challenge for effective drug delivery using liposomes. PEGylation has been shown to protect these liposomes for getting degraded in the GI and improves the oral drug delivery of drugs. In one such study, EXE was loaded into PEGylated liposomes and the stability in simulated gastric fluid was assessed and compared with conventional liposomes (Singh et al., 2020). PEGylation was reported to protect the liposomes from acidic degradation thereby improving the intracellular uptake of EXE as compared to the plain liposomes.
Similarly, the synergistic combination of vitamin D3/phospholipid complex and the anticancer drug etoposide (ETP) was explored by preparing vitaminD3/phospholipid decorated ETP-loaded nanomicelles (Agwa et al., 2021). This study aimed to target the drug into breast cancer cell lines using vit. D3 receptors which are overexpressed on breast cancer cells. The results showed improved anticancer activity of ETP on the tested cell lines without any toxicity to the lung fibroblasts demonstrating good selectivity of the prepared decorated nanomicelles. The use of docetaxel (DTX), one of the very useful drugs for breast cancer, is limited owing to its low solubility and permeability; therefore, DTX-phospholipid complex (DTX@PLC) was prepared and loaded into a self-microemulsifying drug delivery system (SMEDDS) (Wang et al., 2020). The DTX@PLC-SME increased the surface area and therefore the dissolution and permeability of DTX owing to the formation of a microemulsion in the gastrointestinal fluid. The action of drug TAM citrate was targeted to breast cancer cells in a strategy where TAM-loaded transferrin-conjugated-solid lipid nanoparticles (SLNs) were prepared with the aim to reduce its toxicity to uterus and other vital organs (Bhagwat et al., 2020). Results revealed improved cytotoxicity of TAM in a time- and concentration-dependent manner on MCF-7 cells as compared to the plain TAM solution.
A ternary inclusion complex of Genistein (GT) was prepared using β-cylcodextrin (β-CD) as complexing agent, while D-α-tocopherol-polyethylene glycol 1,000 succinate (TPGS) as ternary substance (Zafar et al., 2022). The prepared complex showed remarkable solubility enhancement as well as increased antioxidant and cell viability activity in vitro against human breast cancer cell lines MCF-7 in comparison to the pure GT. Similarly, the combination of doxorubicin (DOX) and umbelliprenin was loaded to PEGylated DSPC liposomes using microfluidic and thin-film methods and compared (Gkionis et al., 2020). The former technique produced particles with more homogeneous shape and size and an improved cytotoxicity against breast cancer cell lines. The poor aqueous solubility and stability of carfilzomib (CFZ) was addressed by preparing its nanocrystals which were subsequently coated with albumin to achive enhanced formulation stability and targeted drug delivery (Park et al., 2019). Improved anticancer activity of the developed formulation was observed with enhanced metabolic stability and improved cellular uptake. Therefore, encapsulation into the nanoparticles has proved to be an effective strategy in order to improve the biopharmaceutical properties of drugs and their combinations; and a sustained release of drug molecules was obtained over a long period of time.
4 Nanomaterials from natural molecules
Traditional medicines play an important role in many healthcare systems across the world, particularly in developing countries. According to a World Health Organization (WHO) estimate, traditional medicine covers the essential health requirements of around 80% of the population in impoverished countries, which is increasing day by day (World Health Organization, 2013). Natural anticancer medications are in increasing demand because they are efficient cancer cell inhibitors. Herb refers to a plant or their derived component that is used for its scent, flavor, and/or therapeutic properties, as well as for other purposes (El-Sayed and Youssef, 2019). Conventional medicinal herbs are naturally occurring plant-derived medications that are used to treat various types of diseases including cancer in local or regional healing traditions for hundreds of years with little or no chemical change to their formulation (Mahomoodally, 2013; Li et al., 2018). However, many natural molecules suffer from biopharmaceutical issues including poor solubility, low bioavailability, instability and poor permeability (Patra et al., 2018; Kesharwani and Bhat, 2020). Numerous novel drug delivery systems have been developed during the last 2 decades to function as carriers for a variety of bioactive chemicals or herbs; with the primary aim of increasing their bioavailability, reducing adverse effects, and avoiding drug degradation (Patra et al., 2018; Yap et al., 2021; Chavda et al., 2022). Moreover, these delivery systems improve the therapeutic efficacy, enhance stability and target the drugs to specific site and prevent first pass metabolism.
Treatment of breast cancer is undergoing extensive progress majorly with targeted therapies bypassing the severe side-effects of conventional chemotherapy (Masoud and Pagès, 2017b; Bukhari, 2022). The effectiveness of chemotherapy is restricted by the cancer cells’ resistance. As alternatives, phytochemicals and other natural molecules provide promising moieties for breast cancer prevention, treatment and therapy of difficult-to-treat mammary malignancies (Israel et al., 2018; Gregoriou et al., 2021). Phytochemicals significantly decrease the risk of cancer spread by direct tumor growth inhibition, apoptosis induction, and inhibition of tumor metastasis. Smart therapeutic approaches are the need of the hour for the treatment of various cancer types (Senapati et al., 2018). Numerous plant-derived phytochemicals have been found to have anticancer potentials and nanotechnological approaches of bioavailability enhancement are gaining rapid interest due to their tremendous potentials (Khan and Gurav, 2018; Dhupal and Chowdhury, 2020; More et al., 2021).
Niosomes, solid lipid nanoparticles, silver nanoparticles, gold nanoparticles, polymeric nanoparticles, liposomes, nanocapsules, and nanospheres are some of the delivery systems available for delivery of plant-based drugs including curcumin, cordycepin, balanocarpol, diallyl disulphite, epigallocatechin, gallic acid, sulphoraphane, resveratrol, punicalagin, ursolic acid, transferrin, bleomycin, and noscapine. Important phytochemicals showing anti-breast cancer activity and their corresponding developed nanoformulations are given in Table 2. These phytochemicals are found to have good efficacy in the treatment of breast cancer through various mechanisms. They are reported to mediate several physiological processes such as cellular uptake, pro-apoptotic activity, cytotoxicity, intracellular ROS generation, Bad, Bax, Caspase 3 and 9 protein level, nuclear shrinkage, stability, morphological change, cell migration, antioxidant activity, growth inhibitory activity, MMP-9 gene expression, etc. In contrast, they also decrease organ toxicities, Bcl-2 protein level, cell migration, P13K, pAKT level, pmTOR protein, cell viability, etc.
Various phytochemicals and plant extracts showing promising activities against breast cancer cell lines were further incorporated into nano-carriers in order to improve their activity and to combat their bioavailability issues. Metal-based nanoparticles (NPs) have shown considerable potential and a number of studies were reported where silver and gold nanoparticles of phytochemical compounds and plant extracts were prepared and tested in vitro against breast cancer cell lines. Silver and gold nanoparticles are noble nanoparticles with optimized shapes and sizes which were found to be effective as carriers in the cancer therapy.
Previously, silver nanoparticles (Ag-NPs) of Agrimoniae herba extract exhibited strong antineoplastic effect on A549 cells, indicating better antitumor effects than the extract alone (Qu et al., 2014). Similarly, the Ag-NPs of Beta vulgaris extract were also prepared and tested against human breast cancer (MCF-7), pharynx (Hep-2) and lung (A549) cancer cell lines (Venugopal et al., 2017a) and the results showed improved permeability and efficacy. In a similar study, Ag-NPs of Syzygium aromaticum extract showed in vitro cytotoxic activity against Hep-2 and MCF-7 cells and the results suggested its use as cancer therapeutics (Venugopal et al., 2017b). In yet another study, green tea, turmeric and garlic extracts-mediated Ag-NPs were prepared and characterized. Results revealed that the Ag-NPs prepared from turmeric extracts exhibited superior antioxidant and cytotoxic activity in comparison to other extracts on all four tested cancer cell lines including HeLa, MCF-7, A549 and Hep-2, in vitro (Arumai Selvan et al., 2018). Ag-NPs of Jasminum officinale L. leaves extract also showed high cytotoxicity against MCF-7 and -5,637 cells and an IC50 values of 13.09 μg/mL and 9.3 μg/mL, respectively were obtained, proving it to be an effective approach (Elhawary et al., 2020). Ag-NPs of Cuminum cyminum L. seed extract were also reported to posses anticancer activities when tested on MCF-7 and AU565 (human breast adenocarcinoma metastatic) cell lines (Dinparvar et al., 2020).
Similarly, gold NPs (Au-NPs) are gold particles of nanosized range (1–100 nm) which are being used in many studies as carriers for delivering anticancer agents of natural origin (Singh et al., 2021). In one such study, Au-NPs of Nerium oleander were prepared, characterized and anticancer potential was tested against MCF-7 cells. Results showed that the prepared Au-NPs induced apoptosis in the tested cell lines selectively (Barai et al., 2018). Recently, Au-NPs of phytochemicals of nano-ayurvedic drugs also called as “Nano Swarna Bhasma” were reported for the treatment of metastatic breast cancer (Khoobchandani et al., 2020). The characteristic features of Au-NPs of being biogenic and easy to synthesize, resulted in diverse research and applications. The anticancer phytocompound Withanolide-A conjugated with Au-NPs was also reported where the prepared nanoconjugate significantly induced SKBR3 cell growth blockage at half of the maximal-active concentration in comparison to the Withanolide-A alone (Tabassam et al., 2020).
Various other nanoformulations of phytochemicals were also prepared and screened against the human breast cancer cell lines such as niosomes, nanoemulsions, and nanovesicles. The nanoniosomes (a non-ionic surfactant based nanovesicles) of the phytochemical lawsone were fabricated by film-hydration method using non-ionic surfactants and cholesterol. The in vitro study exhibited significant antitumor efficacy of the nanoformulation against the MCF-7 cell lines in comparison to the Lawsone solution (Barani et al., 2018). In another study, diosgenin was loaded into niosomes in order to improve its solubility profile. The method adopted to prepare the niosomes was thin-film hydration technique and the cytotoxicity screening was performed on HepG2 cell line. The prepared niosomes showed good loading efficiency (89%) and the in vitro findings revealed that the niosomes exhibited enhanced anticancer effects 28.32% higher than the free diosgenin (Hajizadeh et al., 2019). Curcumin-loaded deformable nanovesicles were obtained by thin-film hydration technique followed by extrusion. Cytotoxicity studies were performed on MCF-7 cell lines using MTT assay technique. The prepared nanovesicles showed an IC50 of 20 μg/mL suggesting them to be successful candidates for the breast cancer therapy (Abdel-Hafez et al., 2018).
Resveratrol, a polyphenolic phytoalexin has significant pharmacological properties; however, it exhibits poor aqueous solubility issues. In lieu of its poor bioavailability issues, nanocarrier of resveratrol loaded NPs were synthesized by the emulsification method using Pluronic-F127 block copolymer and D-α-tocopheryl polyethylene glycol 1,000 succinate (Vitamin E-TPGS). The prepared NPs showed spherical shape, nanosize (179 ± 22 nm), good encapsulation efficiency (73% ± 0.9%) and optimum drug loading (6.2% ± 0.1%) and were reported to have remarkable efficacy against breast cancer cell lines (Gregoriou et al., 2021). The restricted use of anticancer phytochemical sulforaphane was successfully modified with the help of a reliable micellar drug delivery system using monomethoxy-poly (ethylene glycol)-poly (ε-caprolactone) (mPEG-PC) polymer. The anticancer efficacy studied on MCF-7 cells suggested their better efficacy against the breast cancer cell lines in comparison to pure sulforaphane (Danafar et al., 2017b). The nanoemulsion of Zataria essential oil with citrus-pectin was formulated and assessed for its anticancer potential. The resulting nanoemulsion enhanced the viability suppression of drug resistant breast cancer cell lines, MCF-7 and MDA-MB-231 as well as spheroids making it a viable and promising anti-proliferative candidate against breast cancer (Salehi et al., 2020a; Salehi et al., 2020b).
The phytosterol, Stigmasterol (STS)-loaded CD44 receptor targeted PEGylated nanohybrid phytoliposomes were prepared for synergistic chemotherapy along with doxorubicin (DOX) against the metastasis and proliferation of breast cancer cells. The nanocarriers were fabricated by thin-film hydration method and were tested both in vivo and in vitro against the breast cancer cell lines expressing various CD44 receptor levels. The PEGylated DOX-STS liposomes modified using hyaluronic acid (HA) were found to be promising carrier system towards CD44-overexpressing tumors (Gautam et al., 2020). In one such study, Thymoquinone (TQ)-loaded HA-conjugated Pluronic®-P123 and -F127 copolymeric NPs were prepared as drug loading vehicles for the delivery of anticancer phytochemical, TQ to TNBC cells. The TNBC is known to be the most non-responsive subtypes of all breast cancers towards the available therapeutic options (Bhattacharya et al., 2020).
On similar grounds, herbal anticancer phytochemicals can also be incorporated into novel nanocarrier systems such as a polymerosome system to achieve controlled release of natural anticancer agents with improved drug loading efficiency and controlled release from the nanocarriers (Nosrati et al., 2019). It was reported that the plant polyphenols or flavonoids exhibited properties that are important for the therapy of breast cancer. This can be attributed to their antioxidant and anticarcinogenic potential that assists in cell cycle arrest as well as suppression of the uncontrolled cell division in cancer cells, and the activity can further be enhanced by incorporating them into nanocarriers (Sindhu et al., 2021). The essential oils obtained from plants are also a big source of phytochemicals with myriad biological activities including anticancer. However, their use is still beset because of low bioavailability, and rapid degradation profile which can be improved using nanocarriers.
5 Nanomaterials targeting triple negative breast cancer (TNBC)
The term “triple negative breast cancer” (TNBC) refers to a form of breast cancer in which none of the breast cancer’s expression receptors—human epidermal growth factor receptor (EGFR), progesterone receptor (PR), and estrogen receptor (ER) are present. As per the gene expression profile analysis, TNBC is classified as the subtype of basal-like breast cancer (BLBC). The TNBC constitutes about 15%–25% of all breast cancers and has a more aggressive biology, including early stage metastatic disease, visceral organ metastases, highly progressing disease, extremely limited responses to existing treatment regimens, and overall shorter survival rate compared with other major breast cancer subtypes and the mortality rate is 40% within the first 5 years post diagnosis (Yin et al., 2020). In TNBC patients, the relapse period remains between 19 and 40 months, as compared to 35 and 67 months in case of non-TNBC patients. Approximately 75% of TNBC patients die within 3 months of the recurrence (Lyons, 2019). Also, it was reported that the risk of TNBC increased as the use of oral contraceptives increased in duration (Howard and Olopade, 2021).
In comparison to other cancer types, TNBC has limited treatment options. Therefore, chemotherapy has become the main approach of TNBC using the drugs Cisplatin, Docetaxel, Doxorubicin, Adriamycin, Taxan, Anthracycline, 5-Flurouracil and EGFR inhibitors. FDA has recently approved new drugs acting as PARP inhibitors which can destroy TNBC cancer cells (Barchiesi et al., 2021). Although, these chemotherapies are effective but are associated with critical biopharmaceutical issues. Therefore, to overcome these issues, use of nanocarriers was found to be a better alternative for delivering the chemotherapeutic drugs. In addition to serving as a delivery system, nanocarriers were found to be a highly successful therapeutic approach for TNBC (Nahvi et al., 2022). The circulation of drugs in blood is increased by nanoparticles, which also decreases the phagocytosis and reticuloendothelial system (RES) absorption. Moreover, it reduces the side effects associated with chemotherapy. Drug repurposing is currently in vogue along with chemotherapy for the treatment of TNBC. Repurposed drug include evofosfamide, azadiradione, niclosamide, diindolylmethane derivatives, ginsenosides, triptorelin, etc (Ávalos-Moreno et al., 2020). These repurposed medications are currently being administered for the TNBC treatment using a variety of nanocarrier systems. Different types of nanocarriers which showed effectiveness in TNBC include liposomes, lipid nanocarriers, micelle, dendrimers, carbon nanotubes, polymeric nanoparticles and gold nanoparticles. Divergent methods are utilized for the preparation of these nanocarriers which include solvent dispersion method, film hydration method, high speed homogenization, dissolution method, emulsification-solvent evaporation, high-pressure homogenizer, gel electrophoresis, chemical vapor deposition, self -assembled polymersomes and adhesion method (Krishnamoorthy and Mahalingam, 2015). Various nanocarriers used for the delivery of anticancer drugs used in chemotherapy targeting TNBC are presented in Table 3.
6 Challenges associated with nanomaterials
There are several challenges in nanoparticulate developments that need to be addressed before these materials can be widely used in medicine. One of the main challenges is the potential toxicity of nanomaterials. Due to their tiny size, nanoparticles can enter cells and tissues, potentially causing harm. It is crucial to thoroughly assess the toxicity associated with nanoparticles and understand their effects on biological systems before they can be introduced to clinical settings. Another challenge is the complexity of nanoparticle manufacturing. The production of nanoparticles can be a complicated and expensive process, requiring specialized equipment and expertise.
The reproducibility and scalability of nanoparticle manufacturing also need to be addressed to ensure that they can be prepared at a larger scale for widespread use. The shelf-life and stability of nanoparticles can also be a challenge. Nanoparticles can be prone to aggregation, which can affect their properties and efficacy. Additionally, the long-term stability of nanoparticles needs to be evaluated to ensure that they maintain their properties over time. Finally, there are regulatory challenges associated with the use of nanomaterials in medicine. The regulatory landscape for nanoparticles is still evolving, and there are currently no specific guidelines for their use in clinical applications. This can create uncertainty for researchers and companies developing nanoparticulate therapies and may slow down their development and approval processes.
7 Advantages of nanomaterials over traditional therapies
The use of nanomaterials emerged as a potential approach in the field of drug delivery owing to their unique chemical, physical and biological properties. They offer several advantages over traditional therapies based on pharmacokinetics (PK), pharmacodynamics (PD), and therapeutic benefits as shown in Table 4.
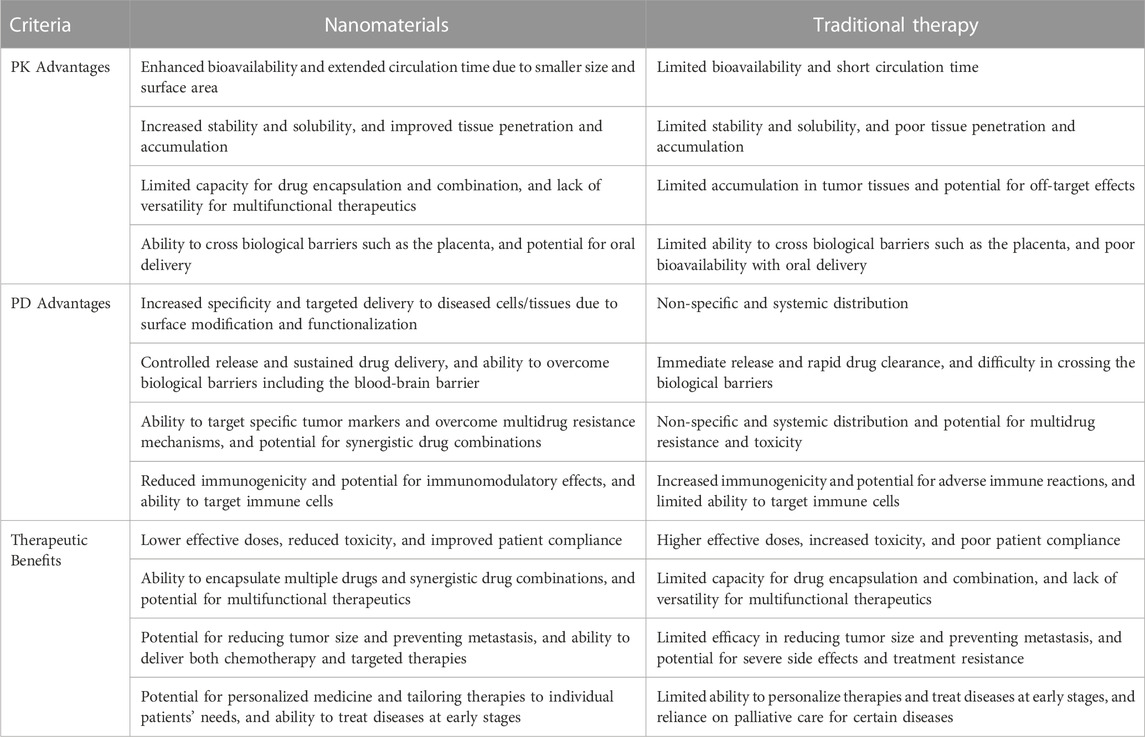
TABLE 4. Advantages of nanomaterials over traditional therapy on the basis of PK, PD and therapeutic benefits.
8 PHOTOIMMUNONANOTHERAPY (PINT)
PhotoImmunoNanoTherapy (PINT) is a type of cancer treatment that combines three different approaches: phototherapy, immunotherapy, and nanotechnology. It involves the use of nanoparticles that are designed to target cancer cells and then activate them with light to destroy them. The nanoparticles are usually coated with antibodies that specifically bind to cancer cells, making them a highly targeted approach to treat cancer. Once the nanoparticles have been taken up by the cancer cells, light is used to activate them, causing the nanoparticles to release reactive oxygen species (ROS) that kill the cancer cells. In addition to the direct effects of the phototherapy, PINT also harnesses the power of the immune system to fight cancer. The ROS generated by the nanoparticles can also trigger an immune response that targets the cancer cells, further enhancing the effectiveness of the treatment. PINT is still in the early stages of development, but it has shown promising potential in preclinical studies and holds great potential as a new cancer therapy.
PINT offers several advantages in the treatment of breast cancer. It can be highly targeted, meaning that the nanoparticles can be designed to specifically bind to breast cancer cells only and spare the healthy cells. Secondly, PINT can be combined with other treatment approaches including chemotherapy or radiation therapy, to enhance their effectiveness. Third, PINT can activate the immune system to fight the cancer cells, providing a systemic response that can help prevent the cancer from spreading. Several preclinical studies have shown promising results for PINT in breast cancer. For example, in one study, researchers used PINT to target breast cancer cells in mice and found that the treatment was highly effective at reducing the size of tumors and preventing metastasis. Another study showed that PINT combined with immunotherapy was able to eliminate breast cancer cells in mice without causing any significant side effects.
9 Selection criteria for a nanoparticulate-based therapy
Nanoparticulate-based therapy is an upcoming field of medicine that involves the use of tiny particles for the targeted delivery of drugs or therapeutic agents to specific cells or tissues in the body. The selection of appropriate nanoparticles for therapy is crucial for the success of the treatment. The selection criteria for nanoparticulate-based therapy involve numerous factors such as size, surface charge, composition, and biocompatibility of the nanoparticles. The size of the nanoparticles is an important consideration because it determines their ability to penetrate biological barriers and reach the targeted cells or tissues. The ideal size for nanoparticles used in therapy is typically between 10 and 200 nm. Additionally, the surface charge of nanoparticles can also influence their ability to target specific cells or tissues. Particles with a neutral or slightly negative surface charge tend to be more stable and have longer circulation times in the bloodstream, which is critical for the delivery of drugs to target cells. The composition of nanoparticles also plays a crucial role in determining their suitability for therapy. For example, nanoparticles made of biodegradable or biocompatible materials such as lipids, proteins, or polysaccharides tend to have fewer adverse effects and can be easily metabolized and eliminated from the body.
Moreover, nanoparticles can be functionalized with specific ligands or targeting molecules that enable them to specifically bind to the target cells or tissues, improving their specificity and efficacy. Finally, the biocompatibility of nanoparticles is also essential for successful therapy. Nanoparticles should not trigger an immune response or cause toxicity or inflammation in the body. Hence, it is critical to carefully assess the toxicity and biocompatibility of nanoparticles in preclinical studies before using them in clinical trials. Overall, the selection of appropriate nanoparticles for therapy involves a careful balance between the size, surface charge, composition, and biocompatibility of the nanoparticles to ensure their safety and efficacy for therapeutic use.
10 Recent patents on nanomaterials for anticancer therapy
To comprehend the ongoing research into the breast cancer treatment using nanocarriers and nanotechnology, an effective search was conducted using the databases ‘Patentscope’, ‘Espacenet’, ‘USPTO public pair’, ‘Google patents’ and ‘InPASS’, to identify the relevant patents in this domain, which yielded a total of 3,239 results. To attain an accurate sequence of ongoing research work, the results obtained were further restricted to recently granted patents in the year 2021 and 2022. Consequently, some of the relevant inventions were studied, and the most pertinent results were included in the current review to facilitate comprehension of the research work. These studies should provide a conceptual framework for conducting more programmed and effective breast cancer research and implementing nanotechnology-based strategies. Table 5 provided below summarizes some of the selected inventions.
11 Clinical studies on anticancer nanomaterials
Chemotherapy, surgery, hormone therapy, radiation, and targeted therapy are the cornerstones of breast cancer treatment. However, scientists continue to investigate new treatments and medications, as well as novel combinations of existing treatments. Participants in clinical trials have the opportunity to receive a new, potentially superior treatment and to contribute to cancer research that may improve the breast cancer treatment for others. However, there is a new emphasis on adding targeted therapies to hormone therapy for HR-positive cancers that are advanced or metastatic. These treatments could delay the need for chemotherapy and ideally, increase survival. The United States Food and Drug Administration (USFDA) approved the use of palbociclib, ribociclib, and everolimus in conjunction with hormone therapy to treat advanced or metastatic breast cancer. It has been demonstrated that ribociclib prolongs the lives of patients with metastatic breast cancer. Abemaciclib can be used with or after hormone therapy to treat HR-positive, HER2-negative advanced or metastatic breast cancer. table 6 showed list of drugs approved by the USFDA from 2020 to 2022.
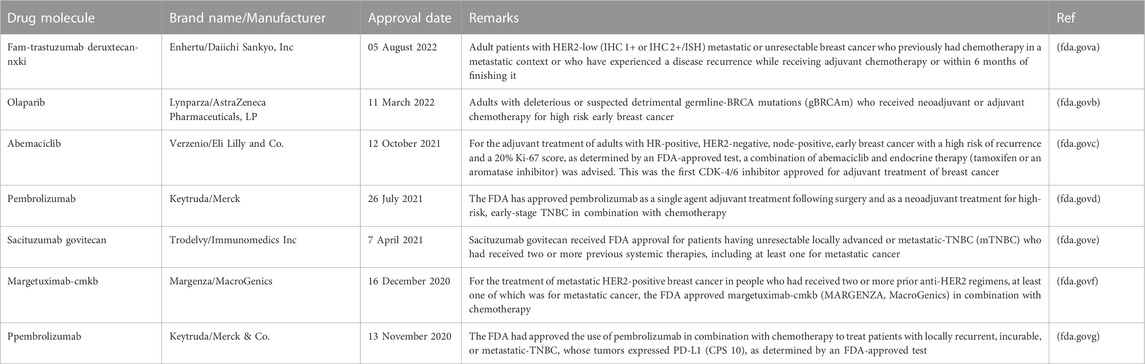
TABLE 6. List of the drugs approved by USFDA from 2020–2022 for advanced or metastatic breast cancer.
12. Conclusions and future Directions
As the molecular biology and etiology of breast cancer is understood well, significant progress have been achieved in the recent years in the diagnosis and treatment of this disease. Various molecular targets have been identified and several drugs are developed showing good potential in managing the disease. The limitations of currently used drugs have also been successfully overcome by developing the nanomedicines using the nanotechnology. Incorporating the drugs and their combinations in nanocarriers has shown better potential as far as the biopharmaceutical issues of the drugs are concerned. Nanoparticles have emerged as versatile drug carrier systems which allow delivery of multiple anticancer agents with the ability to target more than one type of cancer leading to advancements in both diagnosis and treatment of cancer. However, adopting these advanced strategies should be exercised with caution owing to the complexity of the nanoformulations which might lead to immunogenicity and toxicity. Also, the higher production cost of nanomedicines and limited upscalability are crucial factors with the manufacturing of nanomedicines which need to be addressed. Nanocarriers are also associated with some drawbacks including the possible immunogenicity, toxicity and fast excretion. The solid-lipid nanoparticles (SLNs) have low drug loading capacities and contain other complex colloidal structures. The cationic lipids present in liposomes can cause toxicity and also suffer from rapid degradation in body. Till date, only few nanomedicines have received the approval from USFDA out of which Doxil and Abraxane are the two most successful ones for the breast cancer treatment in clinical settings. Several other promising nanocarriers-assisted targeted delivery of synthetic and natural molecules are being investigated in recent years and a number of other formulations are in clinical trial stage.
Author contributions
PK, BM, and GA: conceptualization, methodology, writing of the original draft; SA, DS, and GA: project administration, supervision; SJ, WA, and PM: data collection, feedback, reviewing and making substantive changes; SA and DS: funding acquisition. All authors listed have made a substantial, direct, and intellectual contribution to the work and approved it for publication.
Funding
This work was funded by the Deputyship for Research & Innovation, Ministry of Education in Saudi Arabia through the project number ISP22-9.
Acknowledgments
The authors extend their appreciation to the Deputyship for Research & Innovation, Ministry of Education in Saudi Arabia for funding this research work through the project number ISP22-9.
Conflict of interest
The authors declare that the research was conducted in the absence of any commercial or financial relationships that could be construed as a potential conflict of interest.
Publisher’s note
All claims expressed in this article are solely those of the authors and do not necessarily represent those of their affiliated organizations, or those of the publisher, the editors and the reviewers. Any product that may be evaluated in this article, or claim that may be made by its manufacturer, is not guaranteed or endorsed by the publisher.
References
Abdel-Hafez, S. M., Hathout, R. M., and Sammour, O. A. (2018). Curcumin-loaded ultradeformable nanovesicles as a potential delivery system for breast cancer therapy. Colloids Surf. B Biointerfaces 167, 63–72. doi:10.1016/j.colsurfb.2018.03.051
Agwa, M. M., Abu-Serie, M. M., Abdelmonsif, D. A., Moussa, N., Elsayed, H., Khattab, S. N., et al. (2021). Vitamin D3/phospholipid complex decorated caseinate nanomicelles for targeted delivery of synergistic combination therapy in breast cancer. Int. J. Pharm 607, 120965. doi:10.1016/j.ijpharm.2021.120965
Andey, T., Sudhakar, G., Marepally, S., Patel, A., Banerjee, R., and Singh, M. (2015). Lipid nanocarriers of a lipid-conjugated estrogenic derivative inhibit tumor growth and enhance cisplatin activity against triple-negative breast cancer: Pharmacokinetic and efficacy evaluation. Mol. Pharm. 12 (4), 1105–1120. doi:10.1021/mp5008629
Arumai Selvan, D., Mahendiran, D., Senthil Kumar, R., and Kalilur Rahiman, A. (2018). Garlic, green tea and turmeric extracts-mediated green synthesis of silver nanoparticles: Phytochemical, antioxidant and in vitro cytotoxicity studies. J. Photochem Photobiol. B 180, 243–252. doi:10.1016/j.jphotobiol.2018.02.014
Ávalos-Moreno, M., López-Tejada, A., Blaya-Cánovas, J. L., Cara-Lupiañez, F. E., González-González, A., Lorente, J. A., et al. (2020). Drug repurposing for triple-negative breast cancer. J. Pers. Med. 10 (4), 200. doi:10.3390/jpm10040200
Barai, A. C., Paul, K., Dey, A., Manna, S., Roy, S., Bag, B. G., et al. (2018). Green synthesis of Nerium oleander-conjugated gold nanoparticles and study of its in vitro anticancer activity on MCF-7 cell lines and catalytic activity. Nano Converg. 5 (1), 10. doi:10.1186/s40580-018-0142-5
Barani, M., Mirzaei, M., Torkzadeh-Mahani, M., and Nematollahi, M. H. (2018). Lawsone-loaded niosome and its antitumor activity in MCF-7 breast cancer cell line: A nano-herbal treatment for cancer. Daru 26 (1), 11–17. doi:10.1007/s40199-018-0207-3
Barchiesi, G., Roberto, M., Verrico, M., Vici, P., Tomao, S., and Tomao, F. (2021). Emerging role of PARP inhibitors in metastatic triple negative breast cancer. Current scenario and future perspectives. Front. Oncol. 11, 769280. doi:10.3389/fonc.2021.769280
Benqing, Z., and Jinxing, L. PEG-MAL modification-based two-dimensional black phosphorus hybrid nano-drug targeting HER2 positive breast cancer. Chinese Patent number CN114306628A, 2021. Available at: https://patents.google.com/patent/CN114306628A/en?oq=CN114306628.
Bhagwat, G. S., Athawale, R. B., Gude, R. P., Alhakamy, N. A., Fahmy, U. A., and Kesharwani, P. (2020). Formulation and development of transferrin targeted solid lipid nanoparticles for breast cancer therapy. Front. Pharmacol. 11, 614290. doi:10.3389/fphar.2020.614290
Bhattacharya, S., Ghosh, A., Maiti, S., Ahir, M., Debnath, G. H., Gupta, P., et al. (2020). Delivery of thymoquinone through hyaluronic acid-decorated mixed Pluronic® nanoparticles to attenuate angiogenesis and metastasis of triple-negative breast cancer. J. Control Release 322, 357–374. doi:10.1016/j.jconrel.2020.03.033
Bhavsar, D., Gajjar, J., and Sawant, K. (2019). Formulation and development of smart pH responsive mesoporous silica nanoparticles for breast cancer targeted delivery of anastrozole: In vitro and in vivo characterizations. Microporous Mesoporous Mater 279, 107–116. doi:10.1016/J.MICROMESO.2018.12.026
Brisander, M., Demirbuker, M., Derand, H., Jesson, G., and Malmsten, M. A pharmaceutical composition comprising stable, amorphous hybrid nanoparticles of at least one protein kinase inhibitor and at least one polymeric stabilizing and matrix-forming component. Australian Patent number AU2021269331A1, 2021. Available at: https://patents.google.com/patent/AU2021269331A1/en?oq=AU+2021269331.
Bukhari, S. N. A. (2022). Emerging nanotherapeutic approaches to overcome drug resistance in cancers with update on clinical trials. Pharmaceutics 14 (4), 866. doi:10.3390/pharmaceutics14040866
Caldeira de Araújo Lopes, S., Vinícius Melo Novais, M., Salviano Teixeira, C., Honorato-Sampaio, K., Tadeu Pereira, M., Ferreira, L. A., et al. (2013). Preparation, physicochemical characterization, and cell viability evaluation of long-circulating and pH-sensitive liposomes containing ursolic acid. Biomed. Res. Int. 2013, 467147. doi:10.1155/2013/467147
Chavda, V. P., Patel, A. B., Mistry, K. J., Suthar, S. F., Wu, Z. X., Chen, Z. S., et al. (2022). Nano-drug delivery systems entrapping natural bioactive compounds for cancer: Recent progress and future challenges. Front. Oncol. 12, 867655. doi:10.3389/fonc.2022.867655
Chen, R., Ni, S., Chen, W., Liu, M., Feng, J., and Hu, K. (2021). Improved anti-triple negative breast cancer effects of docetaxel by RGD-modified lipid-core micelles. Int. J. Nanomedicine 16, 5265–5279. doi:10.2147/IJN.S313166
Chumsri, S., Howes, T., Bao, T., Sabnis, G., and Brodie, A. (2011). Aromatase, aromatase inhibitors, and breast cancer. J. Steroid Biochem. Mol. Biol. 125 (1-2), 13–22. doi:10.1016/j.jsbmb.2011.02.001
Conghui, C. Co-loaded nano preparation based on antigen and active polysaccharide and preparation method and application thereof. Chinese Patent number CN114099656A. Available at: https://patents.google.com/patent/CN114099656A/en?oq=CN114099656.
Damodaran, S., and Hortobagyi, G. N. (2021). Estrogen receptor: A paradigm for targeted therapy. Cancer Res. 81 (21), 5396–5398. doi:10.1158/0008-5472.CAN-21-3200
Danafar, H., Sharafi, A., Askarlou, S., and Manjili, H. K. (2017). Preparation and characterization of PEGylated iron oxide-gold nanoparticles for delivery of sulforaphane and curcumin. Drug Res. 67 (12), 698–704. doi:10.1055/s-0043-115905
Danafar, H., Sharafi, A., Kheiri Manjili, H., and Andalib, S. (2017). Sulforaphane delivery using mPEG-PCL co-polymer nanoparticles to breast cancer cells. Pharm. Dev. Technol. 22 (5), 642–651. doi:10.3109/10837450.2016.1146296
Desai, N. P. Biomarkers for nanoparticle compositions. Australian Patent number AU2021290200A1, 2022. Available at: https://patents.google.com/patent/AU2021290200A1/en?oq=AU+2021290200.
Dhupal, M., and Chowdhury, D. (2020). Phytochemical-based nanomedicine for advanced cancer theranostics: Perspectives on clinical trials to clinical use. Int. J. Nanomedicine 15, 9125–9157. doi:10.2147/IJN.S259628
Ding, Y., Yang, R., Yu, W., Hu, C., Zhang, Z., Liu, D., et al. (2021). Chitosan oligosaccharide decorated liposomes combined with TH302 for photodynamic therapy in triple negative breast cancer. J. nanobiotechnology 19 (1), 147–217. doi:10.1186/s12951-021-00891-8
Dinparvar, S., Bagirova, M., Allahverdiyev, A. M., Abamor, E. S., Safarov, T., Aydogdu, M., et al. (2020). A nanotechnology-based new approach in the treatment of breast cancer: Biosynthesized silver nanoparticles using Cuminum cyminum L. seed extract. J. Photochem Photobiol. B 208, 111902. doi:10.1016/j.jphotobiol.2020.111902
El-Sayed, S. M., and Youssef, A. M. (2019). Potential application of herbs and spices and their effects in functional dairy products. Heliyon 5 (6), e01989. doi:10.1016/j.heliyon.2019.e01989
El-Senduny, F. F., Altouhamy, M., Zayed, G., Harsha, C., Jalaja, R., Somappa, S. B., et al. (2021). Azadiradione-loaded liposomes with improved bioavailability and anticancer efficacy against triple negative breast cancer. J. Drug Deliv. Sci. Technol. 65, 102665. doi:10.1016/j.jddst.2021.102665
Elhawary, S., El-Hefnawy, H., Mokhtar, F. A., Sobeh, M., Mostafa, E., Osman, S., et al. (2020). Green synthesis of silver nanoparticles using extract of Jasminum officinal L. Leaves and evaluation of cytotoxic activity towards bladder (5637) and breast cancer (MCF-7) cell lines. Int. J. Nanomedicine 15, 9771–9781. doi:10.2147/IJN.S269880
Fahad Ullah, M. (2019). Breast cancer: Current perspectives on the disease status. Adv. Exp. Med. Biol. 1152, 51–64. doi:10.1007/978-3-030-20301-6_4
Farhangi, B., Alizadeh, A. M., Khodayari, H., Khodayari, S., Dehghan, M. J., Khori, V., et al. (2015). Protective effects of dendrosomal curcumin on an animal metastatic breast tumor. Eur. J. Pharmacol. 758, 188–196. doi:10.1016/j.ejphar.2015.03.076
fda.gov. Available at: https://www.fda.gov/drugs/resources-information-approved-drugs/fda-approves-fam-trastuzumab-deruxtecan-nxki-her2-low-breast-cancer.
fda.gov. Available at: https://www.fda.gov/drugs/resources-information-approved-drugs/fda-approves-olaparib-adjuvant-treatment-high-risk-early-breast-cancer.
fda.gov. Available at: https://www.fda.gov/drugs/resources-information-approved-drugs/fda-approves-abemaciclib-endocrine-therapy-early-breast-cancer.
fda.gov. Available at: https://www.fda.gov/drugs/resources-information-approved-drugs/fda-approves-pembrolizumab-high-risk-early-stage-triple-negative-breast-cancer.
fda.gov. Available at: https://www.fda.gov/drugs/resources-information-approved-drugs/fda-grants-regular-approval-sacituzumab-govitecan-triple-negative-breast-cancer.
fda.gov. Available at: https://www.fda.gov/drugs/resources-information-approved-drugs/fda-approves-margetuximab-metastatic-her2-positive-breast-cancer.
fda.gov. Available at: https://www.fda.gov/drugs/resources-information-approved-drugs/fda-grants-accelerated-approval-pembrolizumab-locally-recurrent-unresectable-or-metastatic-triple.
Feng, Y., Spezia, M., Huang, S., Yuan, C., Zeng, Z., Zhang, L., et al. (2018). Breast cancer development and progression: Risk factors, cancer stem cells, signaling pathways, genomics, and molecular pathogenesis. Genes Dis. 5 (2), 77–106. doi:10.1016/j.gendis.2018.05.001
Figueroa, J. D., Gray, E., Pashayan, N., Deandrea, S., Karch, A., Vale, D. B., et al. (2021). The impact of the Covid-19 pandemic on breast cancer early detection and screening. Prev. Med. 151, 106585. doi:10.1016/j.ypmed.2021.106585
Fraguas-Sánchez, A. I., Fernández-Carballido, A., Simancas-Herbada, R., Martin-Sabroso, C., and Torres-Suárez, A. I. (2020). CBD loaded microparticles as a potential formulation to improve paclitaxel and doxorubicin-based chemotherapy in breast cancer. Int. J. Pharm. 574, 118916. doi:10.1016/J.IJPHARM.2019.118916
Gang, W., Yang, W., and Wenya, D. Intestinal SGLT1 targeted docetaxel-curcumin solid lipid nanoparticle and preparation method and application thereof. Chinese Patent number CN114191549A, 2022. Available at: https://patents.google.com/patent/CN114191549A/en?oq=CN114191549.
Ganz, P. A., and Goodwin, P. J. (2015). Breast cancer survivorship: Where are we today? Adv. Exp. Med. Biol. 862, 1–8. doi:10.1007/978-3-319-16366-6_1
Gautam, M., Thapa, R. K., Gupta, B., Soe, Z. C., Ou, W., Poudel, K., et al. (2020). Phytosterol-loaded CD44 receptor-targeted PEGylated nano-hybrid phyto-liposomes for synergistic chemotherapy. Expert Opin. Drug Deliv. 17 (3), 423–434. doi:10.1080/17425247.2020.1727442
Gkionis, L., Campbell, R. A., Aojula, H., Harris, L. K., and Tirella, A. (2020). Manufacturing drug co-loaded liposomal formulations targeting breast cancer: Influence of preparative method on liposomes characteristics and in vitro toxicity. Int. J. Pharm. 590, 119926. doi:10.1016/J.IJPHARM.2020.119926
Godugu, C., Doddapaneni, R., Safe, S. H., and Singh, M. (2016). Novel diindolylmethane derivatives based NLC formulations to improve the oral bioavailability and anticancer effects in triple negative breast cancer. Eur. J. Pharm. Biopharm. 108, 168–179. doi:10.1016/j.ejpb.2016.08.006
Gonzalez-Angulo, A. M., Morales-Vasquez, F., and Hortobagyi, G. N. (2007). Overview of resistance to systemic therapy in patients with breast cancer. Adv. Exp. Med. Biol. 608, 1–22. doi:10.1007/978-0-387-74039-3_1
Gote, V., Nookala, A. R., Bolla, P. K., and Pal, D. (2021). Drug resistance in metastatic breast cancer: Tumor targeted nanomedicine to the rescue. Int. J. Mol. Sci. 22 (9), 4673. doi:10.3390/ijms22094673
Gregoriou, Y., Gregoriou, G., Yilmaz, V., Kapnisis, K., Prokopi, M., Anayiotos, A., et al. (2021). Resveratrol loaded polymeric micelles for theranostic targeting of breast cancer cells. Nanotheranostics 5 (1), 113–124. doi:10.7150/ntno.51955
Gupta, P., Neupane, Y. R., Parvez, S., and Kohli, K. (2021). Recent advances in targeted nanotherapeutic approaches for breast cancer management. Nanomedicine 16 (29), 2605–2631. doi:10.2217/NNM-2021-0281
Haider, M., Elsherbeny, A., Pittalà, V., Consoli, V., Alghamdi, M. A., Hussain, Z., et al. (2022). Nanomedicine strategies for management of drug resistance in lung cancer. Int. J. Mol. Sci. 23 (3), 1853. doi:10.3390/ijms23031853
Hajizadeh, F., Moghadaszadeh Ardebili, S., Baghi Moornani, M., Masjedi, A., Atyabi, F., Kiani, M., et al. (2020). Silencing of HIF-1α/CD73 axis by siRNA-loaded TAT-chitosan-spion nanoparticles robustly blocks cancer cell progression. Eur. J. Pharmacol. 882, 173235. doi:10.1016/j.ejphar.2020.173235
Hajizadeh, M. R., Parvaz, N., Barani, M., Khoshdel, A., Fahmidehkar, M. A., Mahmoodi, M., et al. (2019). Diosgenin-loaded niosome as an effective phytochemical nanocarrier: Physicochemical characterization, loading efficiency, and cytotoxicity assay. Daru 27 (1), 329–339. doi:10.1007/s40199-019-00277-0
Hasan, M., Belhaj, N., Benachour, H., Barberi-Heyob, M., Kahn, C. J. F., Jabbari, E., et al. (2014). Liposome encapsulation of curcumin: Physico-chemical characterizations and effects on MCF7 cancer cell proliferation. Int. J. Pharm. 461 (1–2), 519–528. doi:10.1016/j.ijpharm.2013.12.007
Hasan, M., Elkhoury, K., Belhaj, N., Kahn, C., Tamayol, A., Barberi-Heyob, M., et al. (2020). Growth-inhibitory effect of chitosan-coated liposomes encapsulating curcumin on MCF-7 breast cancer cells. Mar. Drugs 18 (4), 217. doi:10.3390/md18040217
Hassani, A., Azarian, M. M. S., Ibrahim, W. N., and Hussain, S. A. (2020). Preparation, characterization and therapeutic properties of gum Arabic-stabilized gallic acid nanoparticles. Sci. Rep. 10 (1), 17808. doi:10.1038/s41598-020-71175-8
Howard, F. M., and Olopade, O. I. (2021). Epidemiology of triple-negative breast cancer: A review. Cancer J. 27 (1), 8–16. doi:10.1097/PPO.0000000000000500
Hussein, Y., Loutfy, S. A., Kamoun, E. A., El-Moslamy, S. H., Radwan, E. M., and Elbehairi, S. E. I. (2021). Enhanced anti-cancer activity by localized delivery of curcumin form PVA/CNCs hydrogel membranes: Preparation and in vitro bioevaluation. Int. J. Biol. Macromol. 170, 107–122. doi:10.1016/j.ijbiomac.2020.12.133
Israel, B. B., Tilghman, S. L., Parker-Lemieux, K., and Phytochemicals, P.-S. F. (2018). Phytochemicals: Current strategies for treating breast cancer. Oncol. Lett. 15 (5), 7471–7478. doi:10.3892/ol.2018.8304
Jain, A., Mahira, S., Majoral, J. P., Bryszewska, M., Khan, W., and Ionov, M. (2019). Dendrimer mediated targeting of siRNA against polo-like kinase for the treatment of triple negative breast cancer. J. Biomed. Mater. Res. Part A 107 (9), 1933–1944. doi:10.1002/jbm.a.36701
Jun, J. C., Rathore, A., Younas, H., Gilkes, D., and Polotsky, V. Y. (2017). Hypoxia-inducible factors and cancer. Curr. Sleep. Med. Rep. 3 (1), 1–10. doi:10.1007/s40675-017-0062-7
Kesharwani, S. S., and Bhat, G. J. (2020). Formulation and nanotechnology-based approaches for solubility and bioavailability enhancement of zerumbone. Med. Kaunas. 56 (11), 557. doi:10.3390/medicina56110557
Khan, T., and Gurav, P. (2018). PhytoNanotechnology: Enhancing delivery of plant based anti-cancer drugs. Front. Pharmacol. 8, 1002. doi:10.3389/fphar.2017.01002
Khoobchandani, M., Katti, K. K., Karikachery, A. R., Thipe, V. C., Srisrimal, D., Dhurvas Mohandoss, D. K., et al. (2020). New approaches in breast cancer therapy through green nanotechnology and nano-ayurvedic medicine - pre-clinical and pilot human clinical investigations. Int. J. Nanomedicine 15, 181–197. doi:10.2147/IJN.S219042
Kim, Y. K. Nanovesicles derived from micrococcus bacteria and use thereof. Japanese Patent number JP2022058656A, 2022. Available at: https://patents.google.com/patent/JP2022058656A/en?oq=JP2022058656.
Krishnamoorthy, K., and Mahalingam, M. (2015). Selection of a suitable method for the preparation of polymeric nanoparticles: Multi-criteria decision making approach. Adv. Pharm. Bull. 5 (1), 57–67. doi:10.5681/apb.2015.008
Kwon, Y. J., Mohammad, S. N., Kim, I., and Baek, S. Nucleic acid-mediated delivery of therapeutics. Worldwide Patent number WO2021046480, 2021. Available at: https://patentscope.wipo.int/search/en/detail.jsf?docId=WO2021046480&_cid=P11-L32LD2-04742-1.
Li, Q., Li, H. J., Xu, T., Du, H., Huan Gang, C. L., Fan, G., et al. (2018). Natural medicines used in the traditional Tibetan medical system for the treatment of liver diseases. Front. Pharmacol. 9, 29. doi:10.3389/fphar.2018.00029
Liang, Y., Zhang, H., Song, X., and Yang, Q. (2020). Metastatic heterogeneity of breast cancer: Molecular mechanism and potential therapeutic targets. Semin. Cancer Biol. 60, 14–27. doi:10.1016/j.semcancer.2019.08.012
Liu, X., Meng, H., and Nel, A. E. Mesoporous silica nanoparticules with lipid bilayer coating for cargo delivery. Australian Patent number AU2022200881A1, 2022. Available at: https://patents.google.com/patent/AU2022200881A1/en?oq=AU2022200881.
Longo, J. P., Muehlmann, L. A., Calderón, M., Stockmann, C., and Azevedo, R. B. (2021). Nanomedicine in cancer targeting and therapy. Front. Oncol. 11, 4393. doi:10.3389/FONC.2021.788210/BIBTEX
Luo, X., Wang, H., and Ji, D. (2021). Carbon nanotubes (CNT)-loaded ginsenosides Rb3 suppresses the PD-1/PD-L1 pathway in triple-negative breast cancer. Aging (Albany NY) 13 (13), 17177–17189. doi:10.18632/aging.203131
Lyons, T. G. (2019). Targeted therapies for triple-negative breast cancer. Curr. Treat. options Oncol. 20 (11), 82–13. doi:10.1007/s11864-019-0682-x
Mahomoodally, M. F. (2013). Traditional medicines in africa: An appraisal of ten potent african medicinal plants. Evid. Based Complement. Altern. Med. 2013, 617459. doi:10.1155/2013/617459
Mamnoon, B., Loganathan, J., Confeld, M. I., De Fonseka, N., Feng, L., Froberg, J., et al. (2020). Targeted polymeric nanoparticles for drug delivery to hypoxic, triple-negative breast tumors. ACS Appl. bio Mater. 4 (2), 1450–1460. doi:10.1021/acsabm.0c01336
Mangla, B., Neupane, Y. R., Singh, A., Kumar, P., Shafi, S., and Kohli, K. (2020). Lipid-nanopotentiated combinatorial delivery of tamoxifen and sulforaphane: Ex vivo, in vivo and toxicity studies. Nanomedicine (Lond). 15 (26), 2563–2583. doi:10.2217/nnm-2020-0277
Mansoori, B., Mohammadi, A., Davudian, S., Shirjang, S., and Baradaran, B. (2017). The different mechanisms of cancer drug resistance: A brief review. Adv. Pharm. Bull. 7 (3), 339–348. doi:10.15171/apb.2017.041
Marslin, G., Khandelwal, V., and Franklin, G. (2020). Cordycepin nanoencapsulated in poly(lactic-co-glycolic acid) exhibits better cytotoxicity and lower hemotoxicity than free drug. Nanotechnol. Sci. Appl. 13, 37–45. doi:10.2147/NSA.S254770
Masoud, V., and Pagès, G. (2017). Targeted therapies in breast cancer: New challenges to fight against resistance. Oncol 8 (2), 120–134. doi:10.5306/WJCO.V8.I2.120
Masoud, V., and Pagès, G. (2017). Targeted therapies in breast cancer: New challenges to fight against resistance. World J. Clin. Oncol. 8 (2), 120–134. doi:10.5306/wjco.v8.i2.120
Mba, I. E., and Nweze, E. I. (2021). Nanoparticles as therapeutic options for treating multidrug-resistant bacteria: Research progress, challenges, and prospects. World J. Microbiol. Biotechnol. 37 (6), 108. doi:10.1007/s11274-021-03070-x
Mirzaei, S., Gholami, M. H., Hashemi, F., Zabolian, A., Farahani, M. V., Hushmandi, K., et al. (2022). Advances in understanding the role of P-gp in doxorubicin resistance: Molecular pathways, therapeutic strategies, and prospects. Drug Discov. Today 27 (2), 436–455. doi:10.1016/j.drudis.2021.09.020
More, M. P., Pardeshi, S. R., Pardeshi, C. V., Sonawane, G. A., Shinde, M. N., Deshmukh, P. K., et al. (2021). Recent advances in phytochemical-based Nano-formulation for drug-resistant Cancer. Med. Drug Discov. 10, 100082. doi:10.1016/j.medidd.2021.100082
Mughees, M., Wajid, S., and Samim, M. (2020). Cytotoxic potential of Artemisia absinthium extract loaded polymeric nanoparticles against breast cancer cells: Insight into the protein targets. Int. J. Pharm. 586, 119583. doi:10.1016/j.ijpharm.2020.119583
Munagala, R., Aqil, F., and Gupta, R. C. (2011). Promising molecular targeted therapies in breast cancer. J. Pharmacol. 43 (3), 236–245. doi:10.4103/0253-7613.81497
Nagy, J. O., Triche, T. J., and Kang, H. G. Targeted polymerized nanoparticles for cancer treatment, United States Patent number US20220117894A1, 2022. Available at: https://patents.google.com/patent/US20220117894A1/en?oq=US20220117894.
Nahvi, I., Belkahla, S., Biswas, S., and Chakraborty, S. (2022). A review on nanocarrier mediated treatment and management of triple negative breast cancer: A Saudi arabian scenario. Front. Oncol. 12, 953865. doi:10.3389/fonc.2022.953865
Navya, P. N., Kaphle, A., Srinivas, S. P., Bhargava, S. K., Rotello, V. M., and Daima, H. K. (2019). Current trends and challenges in cancer management and therapy using designer nanomaterials. Nano Converg. 6 (1), 23. doi:10.1186/s40580-019-0193-2
Nordin, N., Yeap, S. K., Rahman, H. S., Zamberi, N. R., Mohamad, N. E., Abu, N., et al. (2020). Antitumor and anti-metastatic effects of citral-loaded nanostructured lipid carrier in 4T1-induced breast cancer mouse model. Molecules 25 (11), 2670. doi:10.3390/molecules25112670
Nosrati, H., Adinehvand, R., Manjili, H. K., Rostamizadeh, K., and Danafar, H. (2019). Synthesis, characterization, and kinetic release study of methotrexate loaded mPEG-PCL polymersomes for inhibition of MCF-7 breast cancer cell line. Pharm. Dev. Technol. 24 (1), 89–98. doi:10.1080/10837450.2018.1425433
Obeid, M. A., Gany, S. A. S., Gray, A. I., Young, L., Igoli, J. O., and Ferro, V. A. (2020). Niosome-encapsulated balanocarpol: Compound isolation, characterisation, and cytotoxicity evaluation against human breast and ovarian cancer cell lines. Nanotechnology 31 (19), 195101. doi:10.1088/1361-6528/ab6d9c
Park, J. E., Park, J., Jun, Y., Oh, Y., Ryoo, G., Jeong, Y. S., et al. (2019). Expanding therapeutic utility of carfilzomib for breast cancer therapy by novel albumin-coated nanocrystal formulation. J. Control Release 302, 148–159. doi:10.1016/j.jconrel.2019.04.006
Patra, J. K., Das, G., Fraceto, L. F., Campos, E. V. R., Rodriguez-Torres, M. D. P., Acosta-Torres, L. S., et al. (2018). Nano based drug delivery systems: Recent developments and future prospects. J. Nanobiotechnology 16 (1), 71. doi:10.1186/s12951-018-0392-8
Peng, Y., Chen, L., Ye, S., Kang, Y., Liu, J., Zeng, S., et al. (2020). Research and development of drug delivery systems based on drug transporter and nano-formulation. Asian J. Pharm. Sci. 15 (2), 220–236. doi:10.1016/j.ajps.2020.02.004
Pindiprolu, S. K. S., Chintamaneni, P. K., Krishnamurthy, P. T., and Ratna Sree Ganapathineedi, K. (2019). Formulation-optimization of solid lipid nanocarrier system of STAT3 inhibitor to improve its activity in triple negative breast cancer cells. Drug Dev. industrial Pharm. 45 (2), 304–313. doi:10.1080/03639045.2018.1539496
Qingpo, L., Xindong, J., and Chunqi, Z. Nano-lipid microsphere of chemical anti-tumor drug and combined application of nano-lipid microsphere and tumor immunotherapy drug. Chinese Patent number CN114272209A, 2021. Available at: https://patents.google.com/patent/CN114272209A/en?oq=CN114272209.
Qu, D., Sun, W., Chen, Y., Zhou, J., and Liu, C. (2014). Synthesis and in vitro antineoplastic evaluation of silver nanoparticles mediated by Agrimoniae herba extract. Int. J. Nanomedicine 9, 1871–1882. doi:10.2147/IJN.S58732
Radhakrishnan, R., Kulhari, H., Pooja, D., Gudem, S., Bhargava, S., Shukla, R., et al. (2016). Encapsulation of biophenolic phytochemical EGCG within lipid nanoparticles enhances its stability and cytotoxicity against cancer. Chem. Phys. Lipids 198, 51–60. doi:10.1016/j.chemphyslip.2016.05.006
Radhakrishnan, R., Pooja, D., Kulhari, H., Gudem, S., Ravuri, H. G., Bhargava, S., et al. (2019). Bombesin conjugated solid lipid nanoparticles for improved delivery of epigallocatechin gallate for breast cancer treatment. Chem. Phys. Lipids 224, 104770. doi:10.1016/j.chemphyslip.2019.04.005
Rinkenbaugh, A. L., and Baldwin, A. S. (2016). The NF-κB pathway and cancer stem cells. Cells 5 (2), 16. doi:10.3390/cells5020016
Robinson, K., and Tiriveedhi, V. (2020). Perplexing role of P-glycoprotein in tumor microenvironment. Front. Oncol. 10, 265. doi:10.3389/fonc.2020.00265
Salehi, F., Behboudi, H., Kavoosi, G., and Ardestani, S. K. (2020). Incorporation of Zataria multiflora essential oil into chitosan biopolymer nanoparticles: A nanoemulsion based delivery system to improve the in-vitro efficacy, stability and anticancer activity of zeo against breast cancer cells. Int. J. Biol. Macromol. 143, 382–392. doi:10.1016/j.ijbiomac.2019.12.058
Salehi, F., Jamali, T., Kavoosi, G., Ardestani, S. K., and Vahdati, S. N. (2020). Stabilization of Zataria essential oil with pectin-based nanoemulsion for enhanced cytotoxicity in monolayer and spheroid drug-resistant breast cancer cell cultures and deciphering its binding mode with gDNA. Int. J. Biol. Macromol. 164, 3645–3655. doi:10.1016/j.ijbiomac.2020.08.084
Schlotter, C. M., Vogt, U., Allgayer, H., and Brandt, B. (2008). Molecular targeted therapies for breast cancer treatment. Breast Cancer Res. 10 (4), 211–212. doi:10.1186/bcr2112
Sebak, S., Mirzaei, M., Malhotra, M., Kulamarva, A., and Prakash, S. (2010). Human serum albumin nanoparticles as an efficient noscapine drug delivery system for potential use in breast cancer: Preparation and in vitro analysis. Int. J. Nanomedicine 5, 525–532. doi:10.2147/ijn.s10443
Senapati, S., Mahanta, A. K., Kumar, S., and Maiti, P. (2018). Controlled drug delivery vehicles for cancer treatment and their performance. Signal Transduct. Target Ther. 3, 7. doi:10.1038/s41392-017-0004-3
Sharma, D., Kumar, S., and Narasimhan, B. (2018). Estrogen alpha receptor antagonists for the treatment of breast cancer: A review. Chem. Cent. J. 12 (1), 107. doi:10.1186/s13065-018-0472-8
Shen, G., Juanjuan, L., and Miyaharuai, Z. H. Nano delivery system capable of promoting penetration, relieving tumor hypoxia and targeting tumor cells, and preparation method and application thereof. Chinese Patent number CN114259477A, 2022. Available at: https://patents.google.com/patent/CN114259477A/en?oq=CN114259477.
Shirode, A. B., Bharali, D. J., Nallanthighal, S., Coon, J. K., Mousa, S. A., and Reliene, R. (2015). Nanoencapsulation of pomegranate bioactive compounds for breast cancer chemoprevention. Int. J. Nanomedicine 10, 475–484. doi:10.2147/IJN.S65145
Sindhu, R. K., Verma, R., Salgotra, T., Rahman, M. H., Shah, M., Akter, R., et al. (2021). Impacting the remedial potential of nano delivery-based flavonoids for breast cancer treatment. Molecules 26 (17), 5163. doi:10.3390/molecules26175163
Singh, A., Neupane, Y. R., Mangla, B., and Kohli, K. (2019). Nanostructured lipid carriers for oral bioavailability enhancement of exemestane: Formulation design, in vitro, ex vivo, and in vivo studies. J. Pharm. Sci. 108 (10), 3382–3395. doi:10.1016/j.xphs.2019.06.003
Singh, A., Neupane, Y. R., Shafi, S., Mangla, B., and Kohli, K. (2020). PEGylated liposomes as an emerging therapeutic platform for oral nanomedicine in cancer therapy: In vitro and in vivo assessment. J. Mol. Liq. 303, 112649. doi:10.1016/j.molliq.2020.112649
Singh, N., Das, M. K., Ansari, A., Mohanta, D., and Rajamani, P. (2021). Biogenic nanosized gold particles: Physico-chemical characterization and its anticancer response against breast cancer. Biotechnol. Rep. (Amst). 30, e00612. doi:10.1016/j.btre.2021.e00612
Singh, R., Letai, A., and Sarosiek, K. (2019). Regulation of apoptosis in health and disease: The balancing act of BCL-2 family proteins. Nat. Rev. Mol. Cell Biol. 20 (3), 175–193. doi:10.1038/s41580-018-0089-8
Ss Pindiprolu, S. K., Krishnamurthy, P. T., Ghanta, V. R., and Chintamaneni, P. K. (2020). Phenyl boronic acid-modified lipid nanocarriers of niclosamide for targeting triple-negative breast cancer. Nanomedicine 15 (16), 1551–1565. doi:10.2217/nnm-2020-0003
Tabassam, Q., Mehmood, T., Raza, A. R., Ullah, A., Saeed, F., and Anjum, F. M. (2020). Synthesis, characterization and anti-cancer therapeutic potential of withanolide-A with 20nm sAuNPs conjugates against SKBR3 breast cancer cell line. Int. J. Nanomedicine 15, 6649–6658. doi:10.2147/IJN.S258528
Talluri, S. V., Kuppusamy, G., Karri, V. V. S. R., Yamjala, K., Wadhwani, A., Madhunapantula, S. V., et al. (2016). Application of quality-by-design approach to optimize diallyl disulfide-loaded solid lipid nanoparticles. Artif. Cells, Nanomedicine, Biotechnol. 45 (3), 474–488. doi:10.3109/21691401.2016.1173046
Talluri, S. V., Pindiprolu, S. K. S. S., Chintamaneni, P. K., Tummala, S., and Nandha Kumar, S. (2017). RAGE receptor targeted bioconjuguate lipid nanoparticles of diallyl disulfide for improved apoptotic activity in triple negative breast cancer: In vitro studies. Artif. Cells Nanomedicine Biotechnol. 46 (2), 387–397. doi:10.1080/21691401.2017.1313267
Toss, A., and Cristofanilli, M. (2015). Molecular characterization and targeted therapeutic approaches in breast cancer. Breast Cancer Res. 17 (1), 60–11. doi:10.1186/s13058-015-0560-9
Uzonwanne, V. O., Navabi, A., Obayemi, J. D., Hu, J., Salifu, A. A., Ghahremani, S., et al. (2022). Triptorelin-functionalized PEG-coated biosynthesized gold nanoparticles: Effects of receptor-ligand interactions on adhesion to triple negative breast cancer cells. Biomater. Adv. 136, 212801.doi:10.1016/j.bioadv.2022.212801
Vasan, N., Baselga, J., and Hyman, D. M. (2019). A view on drug resistance in cancer. Nature 575 (7782), 299–309. doi:10.1038/s41586-019-1730-1
Venugopal, K., Ahmad, H., Manikandan, E., Thanigai Arul, K., Kavitha, K., Moodley, M. K., et al. (2017). The impact of anticancer activity upon Beta vulgaris extract mediated biosynthesized silver nanoparticles (ag-NPs) against human breast (MCF-7), lung (A549) and pharynx (Hep-2) cancer cell lines. J. Photochem Photobiol. B 173, 99–107. doi:10.1016/j.jphotobiol.2017.05.031
Venugopal, K., Rather, H. A., Rajagopal, K., Shanthi, M. P., Sheriff, K., Illiyas, M., et al. (2017). Synthesis of silver nanoparticles (Ag NPs) for anticancer activities (MCF 7 breast and A549 lung cell lines) of the crude extract of Syzygium aromaticum. J. Photochem Photobiol. B 167, 282–289. doi:10.1016/j.jphotobiol.2016.12.013
Waghray, D., and Zhang, Q. (2018). Inhibit or evade multidrug resistance P-glycoprotein in cancer treatment. J. Med. Chem. 61 (12), 5108–5121. doi:10.1021/acs.jmedchem.7b01457
Wang, M., You, S. K., Lee, H. K., Han, M. G., Lee, H. M., Pham, T. M., et al. (2020). Development and evaluation of docetaxel-phospholipid complex loaded self-microemulsifying drug delivery system: Optimization and in vitro/ex vivo studies. Pharmaceutics 12 (6), 544–619. doi:10.3390/pharmaceutics12060544
Wang, W., Zhou, M., Xu, Y., Peng, W., Zhang, S., Li, R., et al. (2021). Resveratrol-loaded TPGS-resveratrol-solid lipid nanoparticles for multidrug-resistant therapy of breast cancer: In vivo and in vitro study. Front. Bioeng. Biotechnol. 9, 762489. doi:10.3389/fbioe.2021.762489
World Health Organization (2013). WHO traditional medicine strategy 2014-2023. Geneva, Switzerland: WHO Press. Available at https://apps.who.int/iris/bitstream/handle/10665/92455/9789241506090_eng.pdf (accessed May 15, 2022).
Yang, C., Uertz, J., and Chithrani, D. B. (2016). Colloidal gold-mediated delivery of bleomycin for improved outcome in chemotherapy. Nanomater. (Basel) 6 (3), 48. doi:10.3390/nano6030048
Yantasee, W., Reda, M., Ngamcherdtrakul, W., and Hoang, N. H. Therapeutic constructs for co-delivery of mitotic kinase inhibitor and immune checkpoint inhibitor. Worldwide Patent number WO2021011501, 2021. Available at: https://patentscope.wipo.int/search/en/detail.jsf?docId=WO2021011501&_cid=P11-L32LMK-08384-1.
Yao, Y., Zhou, Y., Liu, L., Xu, Y., Chen, Q., Wang, Y., et al. (2020). Nanoparticle-based drug delivery in cancer therapy and its role in overcoming drug resistance. Front. Mol. Biosci. 7, 193. doi:10.3389/fmolb.2020.00193
Yap, K. M., Sekar, M., Fuloria, S., Wu, Y. S., Gan, S. H., Mat Rani, N. N. I., et al. (2021). Drug delivery of natural products through nanocarriers for effective breast cancer therapy: A comprehensive review of literature. Int. J. Nanomedicine 16, 7891–7941. doi:10.2147/IJN.S328135
Yin, L., Duan, J. J., Bian, X. W., and Yu, S. C. (2020). Triple-negative breast cancer molecular subtyping and treatment progress. Breast Cancer Res. 22 (1), 61–13. doi:10.1186/s13058-020-01296-5
Zafar, A., Alruwaili, N. K., Imam, S. S., Alsaidan, O. A., Alharbi, K. S., Mostafa, E. M., et al. (2022). Formulation of ternary genistein β-cyclodextrin inclusion complex: In vitro characterization and cytotoxicity assessment using breast cancer cell line. J. Drug Deliv. Sci. Technol. 67, 102932. doi:10.1016/J.JDDST.2021.102932
Zhang, S., Guo, N., Wan, G., Zhang, T., Li, C., Wang, Y., et al. (2019). pH and redox dual-responsive nanoparticles based on disulfide-containing poly(β-amino ester) for combining chemotherapy and COX-2 inhibitor to overcome drug resistance in breast cancer. J. Nanobiotechnology 17 (1), 109. doi:10.1186/s12951-019-0540-9
Zhaoqi, Y., ChammondiSenyi, G., and Jian, J. Method for treating triple negative breast cancer by double antisense nucleic acid synergistic photodynamic. Chinese Patent number CN114010786A, 2022. Available at: https://patents.google.com/patent/CN114010786A/en?oq=CN114010786.
Keywords: breast cancer, anticancer drugs, synthetic, phytochemicals, nanocarriers, drug delivery
Citation: Kumar P, Mangla B, Javed S, Ahsan W, Musyuni P, Sivadasan D, Alqahtani SS and Aggarwal G (2023) A review of nanomaterials from synthetic and natural molecules for prospective breast cancer nanotherapy. Front. Pharmacol. 14:1149554. doi: 10.3389/fphar.2023.1149554
Received: 22 January 2023; Accepted: 10 May 2023;
Published: 19 May 2023.
Edited by:
Raghuram Kandimalla, James Graham Brown Cancer Center, United StatesReviewed by:
Indrani Poddar, University of Minnesota Twin Cities, United StatesSanjay Mukherjee, Columbia University, United States
Copyright © 2023 Kumar, Mangla, Javed, Ahsan, Musyuni, Sivadasan, Alqahtani and Aggarwal. This is an open-access article distributed under the terms of the Creative Commons Attribution License (CC BY). The use, distribution or reproduction in other forums is permitted, provided the original author(s) and the copyright owner(s) are credited and that the original publication in this journal is cited, in accordance with accepted academic practice. No use, distribution or reproduction is permitted which does not comply with these terms.
*Correspondence: Geeta Aggarwal, cHJvZi5nZWV0YWFnZ2Fyd2FsQGdtYWlsLmNvbQ==; Shamama Javed, c2phaG1hZEBqYXphbnUuZWR1LnNh; Bharti Mangla, YmhhcnRpcGhhcm1hY3lAZ21haWwuY29t