- 1Innovative Institute of Chinese Medicine and Pharmacy, Shandong University of Traditional Chinese Medicine, Jinan, China
- 2Experimental Center, Shandong University of Traditional Chinese Medicine, Jinan, China
- 3Department of Scientific Research, Shandong University of Traditional Chinese Medicine, Jinan, China
- 4Shandong Tai’an Central Hospital, Tai’an, China
- 5Key Laboratory of Traditional Chinese Medicine Classical Theory, Ministry of Education, Shandong University of Traditional Chinese Medicine, Jinan, China
- 6Shandong Provincial Key Laboratory of Traditional Chinese Medicine for Basic Research, Shandong University of Traditional Chinese Medicine, Jinan, China
- 7Daiyue District Maternal and Child Health Hospital, Tai’an, Shandong, China
- 8School of Pharmacy, Shandong University of Traditional Chinese Medicine, Jinan, China
Non-small cell lung cancer (NSCLC) is a common pathological type of lung cancer, which has a serious impact on human life, health, psychology and life. At present, chemotherapy, targeted therapy and other methods commonly used in clinic are prone to drug resistance and toxic side effects. Natural extracts of traditional Chinese medicine (TCM) have attracted wide attention in cancer treatment because of their small toxic and side effects. Kaempferol is a flavonoid from natural plants, which has been proved to have anticancer properties in many cancers such as lung cancer, but the exact molecular mechanism is still unclear. Therefore, on the basis of in vitro experiments, we used network pharmacology and molecular docking methods to study the potential mechanism of kaempferol in the treatment of non-small cell lung cancer. The target of kaempferol was obtained from the public database (PharmMapper, Swiss target prediction), and the target of non-small cell lung cancer was obtained from the disease database (Genecards and TTD). At the same time, we collected gene chips GSE32863 and GSE75037 in conjunction with GEO database to obtain differential genes. By drawing Venn diagram, we get the intersection target of kaempferol and NSCLC. Through enrichment analysis, PI3K/AKT is identified as the possible key signal pathway. PIK3R1, AKT1, EGFR and IGF1R were selected as key targets by topological analysis and molecular docking, and the four key genes were further verified by analyzing the gene and protein expression of key targets. These findings provide a direction for further research of kaempferol in the treatment of NSCLC.
1 Introduction
Non-small cell lung cancer (NSCLC) is the leading cause of cancer death worldwide (Kumar, Yadavilli, & Kannan, 2021). Non-small cell lung cancer (NSCLC) accounts for 85% of lung cancer. NSCLC includes many cancer types, such as adenocarcinoma (LUAD), squamous cell carcinoma (LUSC) and large cell carcinoma. LUADs and LUSCs are the largest subsets of NSCLC (Relli, Trerotola, Guerra, & Alberti, 2019). Generally, NSCLC is treated by surgery in the early stage, but most NSCLC is found in the late stage. Therefore, at present, the treatment methods of NSCLC mainly include radiotherapy, chemotherapy, targeted therapy and immunotherapy, etc. However, radiotherapy, chemotherapy and targeted therapy will produce toxic side effects and drug resistance, and immunotherapy will also face the challenge of low response rate (C. Li, Qiu, & Zhang, 2022). Therefore, it is very important to find a natural extract with few side effects and clarify its molecular mechanism. These polyphenol compounds, such as kaempferol, curcumin, quercetin and resveratrol, which are natural plant extracts, have powerful anti-tumor effects. They can reverse epigenetic changes of oncogene activation and tumor suppressor gene inactivation, and are powerful anticancer agents (Rajendran et al., 2022). Kaempferol is a flavonoid, which exists in many vegetables, fruits, ginkgo biloba and so on (Rajendran et al., 2014). There are reports in the literature, Kaempferol can inhibit cell proliferation, motivation and invasion, and stimulate apoptosis and autophagy, consensus with modifications in coding and non-coding genes’ expression (Budisan et al., 2019). Studies have proved that kaempferol may induce NSCLC cell apoptosis through antioxidant pathway (Leung et al., 2007). Although kaempferol has a good application prospect in tumor therapy, there is little research on the molecular mechanism of kaempferol against non-small cell lung cancer, and there is a lack of systematic and complete understanding. In order to better understand the molecular mechanism of drug anti-tumor, more and more scholars use network pharmacology for research. Pharmacology of the network is the cross synthesis of pharmacology, system biology, bioinformatics, network science and other disciplines, which can systematically explain the mechanism of action of drugs (X. Wang, Hu, Zhou, & Li, 2022). With the development of bioinformatics, some high-throughput platforms are usually used to analyze gene expression during tumorigenesis, which is of great significance in revealing the molecular mechanism of diseases (L. Wang et al., 2020). On the basis of experimental verification, with the help of network pharmacology, molecular docking and bioinformatics, this study predicted the molecular mechanism of kaempferol’s intervention in non-small cell lung cancer.
2 Materials and methods
2.1 Cell culture and cell viability detection
At first, CCK-8 kit was used to evaluate the effect of kaempferol on the viability of BEAS-2B cells. BEAS-2B cells were seeded in 96-well plates at 5 * 10 ^ 3 cells per well and cultured in 37°C and 5% CO2 incubator for 24 h. Complete culture medium (blank control), 0.1% DMSO (negative control),10 μg/mL cisplatin (DDP positive control) and KA (10 μM, 50μM and100 μM) were given respectively, and the culture continued for 48 h. Then, the culture medium was removed, and 100 μL of fresh culture medium containing 10%CCK-8 was added to each well and cultured for 2 h. OD value at 450 nm was measured by microplate reader. Three independent experiments were conducted. Next, CCK-8 kit was used to evaluate the effect of kaempferol on the viability of A549 cells. Three independent experiments were conducted.
2.2 Scratch experiment
Lung cancer A549 cells were inoculated into 24-well plates with 2 * 10 4 cells per well, and incubated overnight in 37°C and 5% CO2 incubator. Scribe vertically with 200 μL pipette tip, and then wash with PBS for 3 times. The cells were treated with complete culture medium (blank control), 0.1% DMSO (negative control), 10 μg/mL cisplatin (DDP positive control) and 50 μM kaempferol, and each group had three accessory holes, and continued to be cultured for 48 h. The migration distance of cells was measured and recorded by microscope. Three independent experiments were conducted.
2.3 Transwell invasion experiment
Serum-free medium was used to dilute the matrix glue at the ratio of 1:8, and 100 μL was added to the upper chamber, which was placed in an incubator at 37°C. When the matrix glue solidified, 200 μL of cell suspensions treated with different drugs (complete medium, 0.1% DMSO, 10 μg/mL cisplatin, 50 μM kaempferol) were added to the upper chamber, with 2 * 10^4 cells per well. Add 600 μL complete medium (containing 10% FBS) into the lower chamber. Lung cancer A549 cells were cultured in an incubator for 48 h. Then suck out the culture medium in the upper chamber, clean the remaining cells in the upper chamber with cotton swabs, and wash them twice with PBS. Soak in 4% paraformaldehyde for 20 min, air dry, dye with 1% crystal violet solution for 15 min, and wash with PBS for 3 times. The adherent cells were observed and counted by microscope. Three independent experiments were conducted.
2.4 Collect kaempferol targets
Pubchem (https://pubchem.ncbi.nlm.nih.gov/) the kaempferol Canonical SMILES obtained are imported into SwissTargetPrediction (https://www.swisstargetprediction.ch/), to obtain the target with p > 0; The target of kaempferol was obtained by Pharm Mapper (https://www.lilab-ecust.cn/pharmmapper/). The acquired target gene set was imported into UniprotKB (https://www.uniprot.gov/) database for standardization.
2.5 Acquisition of NSCLC target gene
Search target genes of non-small cell lung cancer from GeneCards database (https://www.genecards.org/) and TTD database (https://db.idrblab.net/ttd/). The target gene was integrated and de-duplicated. Selected and downloaded the data of GSE32863 and GSE75037 chips from the GEO database (https://www.ncbi.nlm.nih.gov/). Gene chip data came from the same annotation platform, and the sample species were Homo sapiens, including tumor group and control group. The differential genes were screened by Limma package in R software, with ∣Log2FC∣≥1 and correction p < 0.05; Using pheatmap and ggplot2 packages to draw the heat map and volcano map of different genes.
2.6 Screening of core targets of kaempferol in the treatment of non-small cell lung cancer
In order to determine the interaction target of kaempferol in the treatment of NSCLC, we imported kaempferol prediction target gene and non-small cell lung cancer target gene into Venny2.1.0 (https://bioinfogp.cnb.csic.es/tools/venny/) online website to obtain the intersection gene and draw the Wayne diagram. Introducing intersection targets into STRING online platform to construct protein interaction network. Set the species source as H. sapiens, and the confidence level as medium confidence level. The results were imported into Cytoscape3.9.0 software to build PPI network, and the core targets were screened out by CytoNCA plug-in. Select degree centrality, closeness centrality and betweenness centrality in CytoNCA plugin to screen core targets.
2.7 Enrichment analysis of GO and KEGG
The intersection targets were imported into DAVID database (https://david.ncifcrf.gov/) for GO and KEGG enrichment analysis respectively, and some results were visualized by drawing bubble chart and chord chart with bioinformatics online tool (https://vip.sangerbox.com/login.html).
2.8 Molecular docking between kaempferol and core targets
In order to understand the mechanism of action between kaempferol and core targets, molecular docking was carried out. The 2D structure of kaempferol downloaded from PubChem database was imported into Chem Bio3D Ultra 14.0 for correction, and stored in mol2 format. Download the core target protein from PDB database. SYBYL was used to dehydrate and hydrogenate the core target protein, and then it was molecular docked with kaempferol, and some results were visualized.
2.9 Expression level of core target genes
We used GEPIA (https://gepia.cancer-pku.cn/) database to analyze the differential expression of core targets between tumor tissues and normal tissues. The data comes from TCGA and GTEx databases.
2.10 Protein expression level of core target
HPA (https://www.proteinatlas.org/) Database uses transcriptomics and protein omics techniques to study protein expression in different human tissues and organs at RNA and protein levels. According to the staining intensity and the percentage of stained cells in tissues, we compared the protein expression levels of core genes in NSCLC tissues and normal lung tissues, and selected representative immunohistochemical staining pictures from HPA database.
2.11 Survival analysis
Kaplan-Meier Plotter Database (https://kmplot.com/analysis/) is an online analysis database related to the prognosis of malignant tumors, which can be used to explore the relationship between target gene expression and the prognosis of related tumors. Therefore, we use this database to draw the survival curve of key genes, and analyze the influence of the expression of key genes in NSCLC on the overall survival of patients.
3 Results
3.1 The effect of kaempferol on A549 cell viability
CCK-8 assay was used to detect the cell viability of BEAS-2B cells treated with complete medium, 0.1% DMSO, 10 μg/mL cisplatin and KA (10 μM, 50 μM, and 100 μM) for 48 h. The experimental results showed that (Figure 1), when the concentration was 10 μM and 50 μM, KA had less toxicity to cells. Therefore, we chose 50 μM to act on A549 cells for 48 h. The experimental results showed that (Figure 1), compared with the blank control group and the negative control group, kaempferol had a significant inhibitory effect on the proliferation of lung cancer A549 cells.
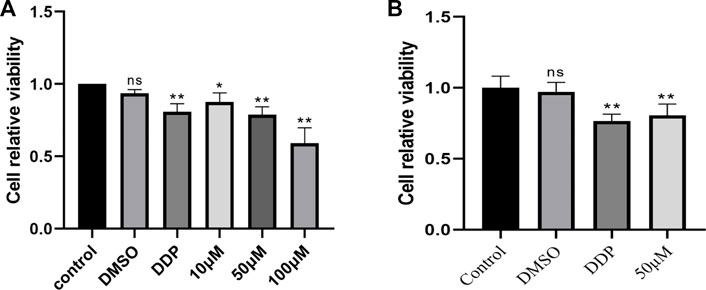
FIGURE 1. (A) Cell viability of BEAS-2B cells treated with complete medium, 0.1% DMSO, 10 μg/mL cisplatin and KA (10 μM, 50 μM and 100 μM) for 48 h was measured by CCK-8. (B) Cell viability of A549 cells treated with complete medium, 0.1% DMSO, 10 μg/mL cisplatin and KA50 μM for 48 h was measured by CCK-8. (* Compared with the control group, p < 0.05, * * compared with the control group, p < 0.01).
3.2 Effect of kaempferol on migration and invasion of A549 cells
To study the effect of kaempferol on the migration and invasion of A549 cells, scratch test and invasion test were carried out. The results showed that compared with the control group, kaempferol could significantly inhibit the migration of A549 cells, and the effect was slightly better than that of the positive control group. In the invasion experiment, compared with the control group, kaempferol can significantly inhibit the invasion of lung cancer A549 cells. (Figure 2).
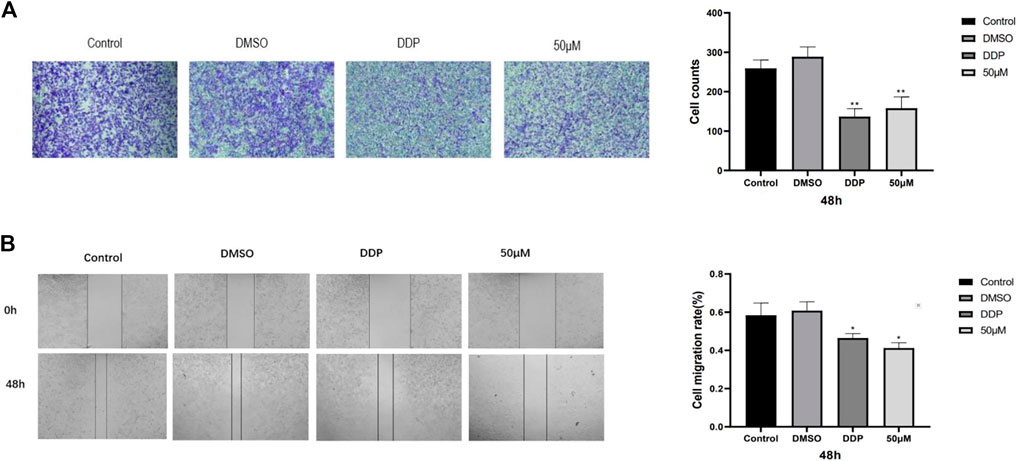
FIGURE 2. (A) Image of cell migration process in scratch experiment and 48-h cell migration rate. (B) Cell invasion experiment. Statistics of cell invasion images and cell invasion counts after 48 h of treatment in different drug groups. (* Compared with the control group, p < 0.05, * * compared with the control group, p < 0.01).
3.3 Kaempferol and NSCLC targets
According to the screening conditions, we got 113 predicted targets of kaempferol. After de-duplication of two disease databases, we got 5818 targets of non-small cell lung cancer. 956 and 2856 differential genes were obtained from gene chips GSE32863 and GSE75037, respectively. There are 339 upregulated genes and 617 downregulated genes in GSE32863, and 1313 upregulated genes and 1543 downregulated genes in GSE75037. According to the two data sets, a volcano map is constructed (Figure 3). We selected the first 40 differential genes to make a gene heat map (Figure 3).
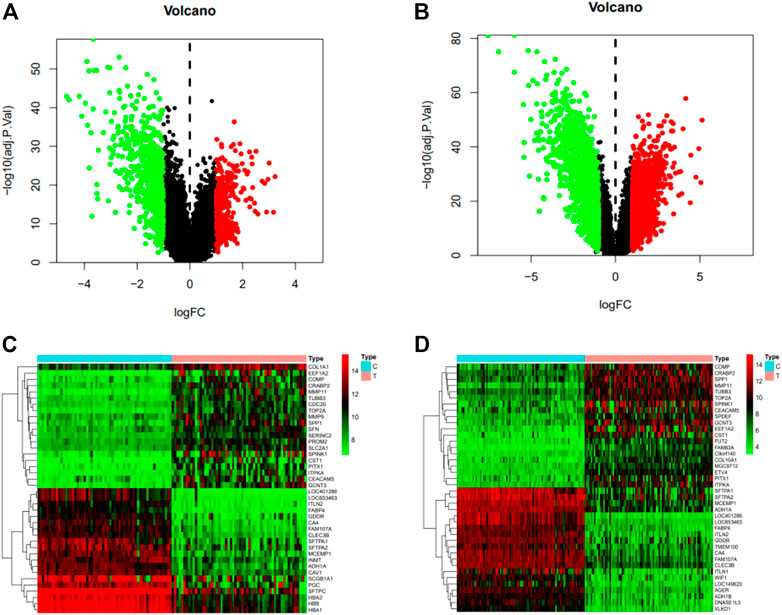
FIGURE 3. (A) Volcano map of GSE32863 differential genes, with red representing upregulated genes (logFC > 0), green representing downregulated genes (logFC < 0) and black representing no significant difference. (B) Volcano map of GSE75037 differential genes, with red representing upregulated genes (logFC > 0), green representing downregulated genes (logFC < 0) and black representing no significant difference. (C) The heat map of the first 40 differentially expressed genes in GSE32863, with red indicating upregulation, green indicating downregulation, and black indicating no significant difference. (D) Heat map of the first 40 differentially expressed genes of GSE75037.
3.4 Kaempferol is the core target of NSCLC
By drawing the Wayne diagram (Figure 4), we got 83 intersecting targets of kaempferol and NSCLC, and identified them as potential candidate targets of kaempferol in the treatment of NSCLC. Then, we import the candidate targets into the STRING database to obtain the protein-protein interaction network. We imported the PPI network into Cytoscape for visualization, and determined AKT1, ESR1, SRC, EGFR, and MMP9 as targets for further analysis according to the degree, closeness and betweenness value in the CytoNCA plug-in (Figure 4).
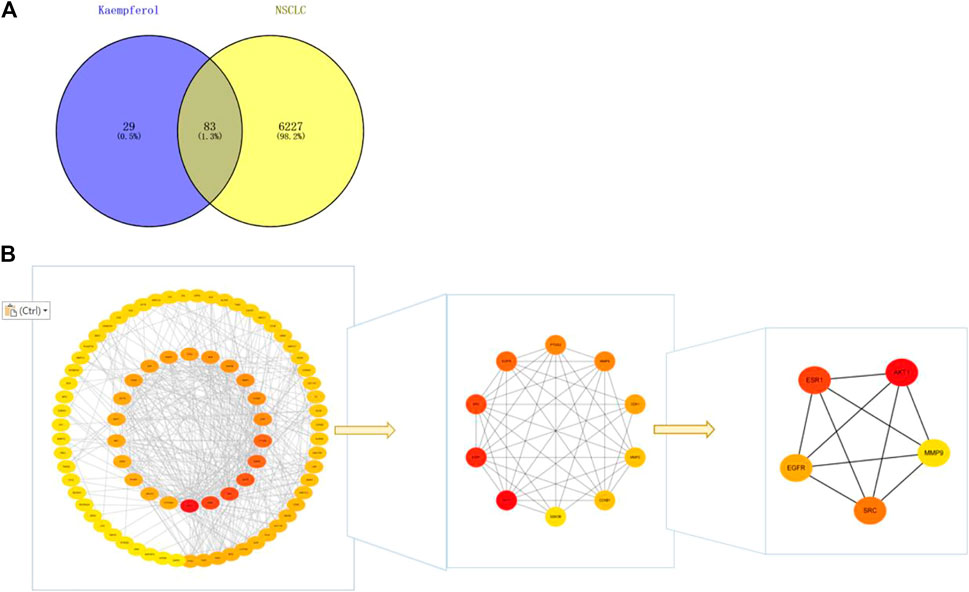
FIGURE 4. (A) Wayne diagram of kaempferol target and NSCLC target, with a total of 83 intersecting targets. (B) Get the top five core targets from PPI network by using cytohubba plug-in.
3.5 Enrichment analysis of Go and KEGG
The results of GO analysis (Figure 5) clarify the biological process, molecular function and cell components. The enrichment analysis showed that the biological processes mainly included peptidyl-serine phosphorylation, protein autophosphorylation, protein phosphorylation, negative regulation of apoptotic process, positive regulation of protein kinase B signaling and other processes related to cell apoptosis. The molecular functions were related to cell activity, such as protein kinase activity, ATP binding, protein serine/threonine kinase activity, carbonate dehydratase activity, enzyme binding. The cell components were related to the cyclin-dependent protein kinase enzyme complex, cytosol, macromolecular complex, nucleus and plasma membrane. The results of KEGG enrichment analysis showed that there were 54 pathways with p < 0.01, and the first 30 pathways were selected to draw chords for visualization (Figure 5). Among them, the main gene-rich pathways include Endocrine resistance, Nitrogen metabolism, EGFR tyrosine kinase inhibitor resistance, Pathways in cancer, Steroid hormone biosynthesis and so on, and we found that these signal pathways are closely connected with PI3K/Akt. We also found that the core targets were closely related to the PI3K/Akt signaling pathway. To verify this finding, we made a “pathway-target” network diagram. In the “pathway-target” (Figure 5) network diagram, the genes associated with the most pathways are PIK3R1, AKT1, EGFR, IGF1R and SRC, and the signaling pathway associated with the most targets is PI3K/Akt. Based on the above results, we speculate that the key targets of kaempferol in NSCLC may be PIK3R1, AKT1, EGFR, IGF1R and SRC, and the possible signaling pathway is PI3K/Akt.
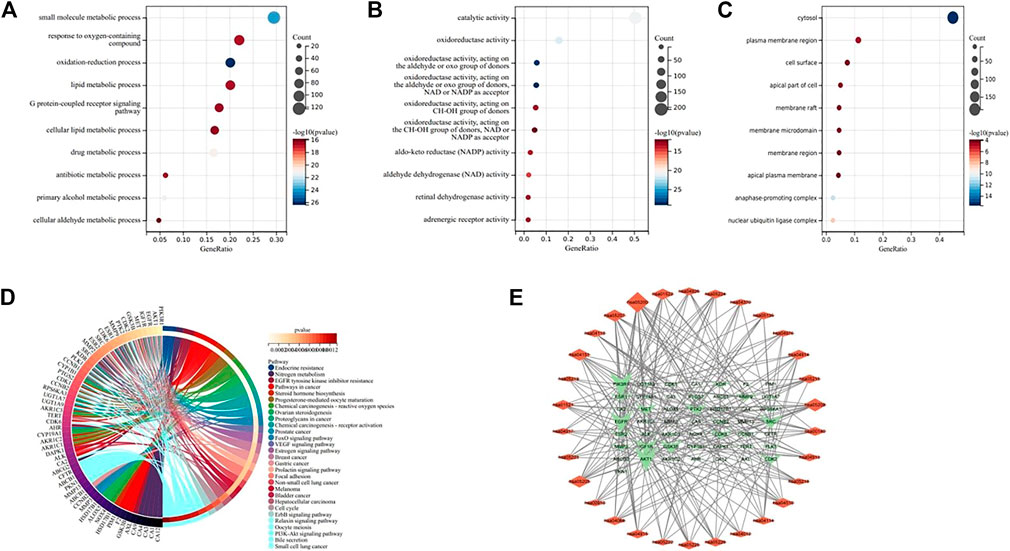
FIGURE 5. (A–C) Bubble diagram of GO enrichment analysis, indicating BP, MF, and CC respectively. (D) KEGG enrichment analysis chord diagram. (E) “Pathway-Target” network diagram. The network shows the relationship between the first 30 pathways and 50 genes, and the size of the graph shows the number of connected pathways and genes.
3.6 Molecular docking
In order to verify the reliability of kaempferol acting on core targets and interfering with key signaling pathways, we performed molecular docking on targets screened by CytoNCA and targets screened by “target-pathway”network diagram. In general, if the docking score > 5, it indicates that the molecules and proteins have good docking activity ; if the docking result > 7, it is considered to have strong docking activity (Jain, 2003). The molecular docking results show (Table 1) that among the seven targets, only PIK3R1, AKT1, EGFR and IGF1R have good docking activity with kaempferol (Figure 6), so we identified them as key core targets and further analyzed them.
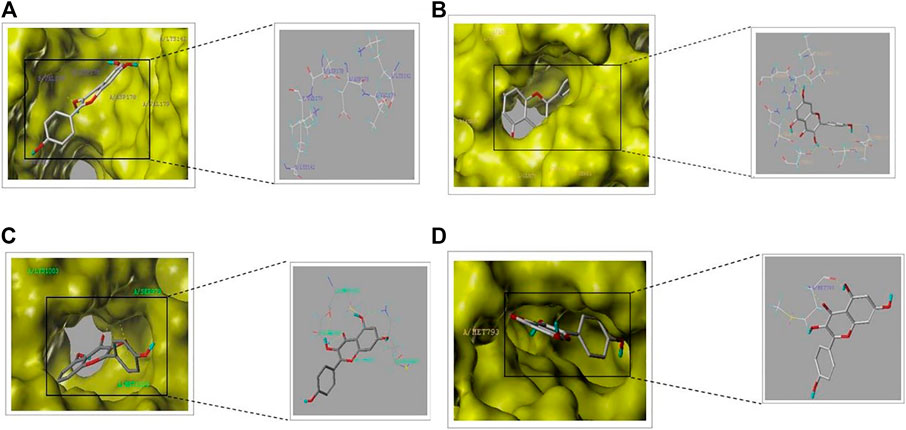
FIGURE 6. Schematic diagram of molecular docking part: (A) PIK3R1-kaempferol. (B) AKT1-kaempferol (C) IGF1R-kaempferol (D) EGFR-kaempferol.
3.7 Differential expression level of core target genes
We used GEPIA online web analytics to detect the differential expression of key core target genes between NSCLC tissues and normal lung tissues (Figure 7). The results showed that the mRNA expressions of AKT1, EGFR and IGF1R were upregulated in lung cancer tissues, while the expression of PI3KR1 was downregulated compared with normal lung tissues. These results suggest that the gene expression levels of these four core targets may be related to the progress of NSCLC.
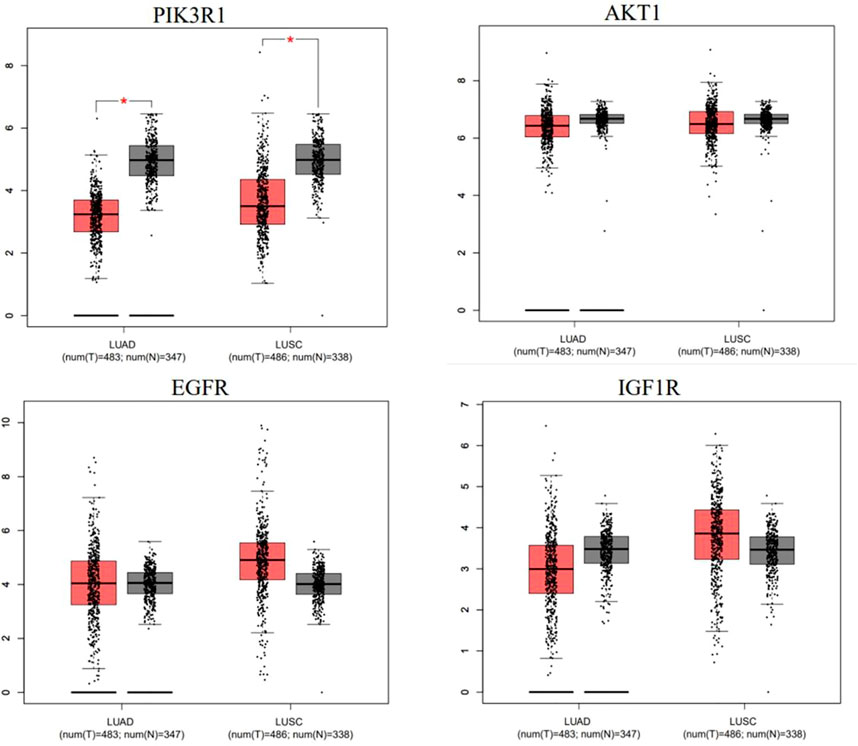
FIGURE 7. mRNA expression levels of hub genes in cancer genome map (TCGA) and genotypic tissue expression (GTEx) databases. Red represents lung cancer tissue, and black represents normal lung tissue.
3.8 Expression level of core target protein
We obtained immunohistochemical staining images of core targets in lung tumor tissues and normal lung tissues from HPA database to observe the protein expression level of core targets. As shown in the results (Figure 8), the expression level of all the core proteins in lung tumor tissues was moderate. It is suggested that the protein expression levels of these four core targets may be related to the progress of NSCLC.
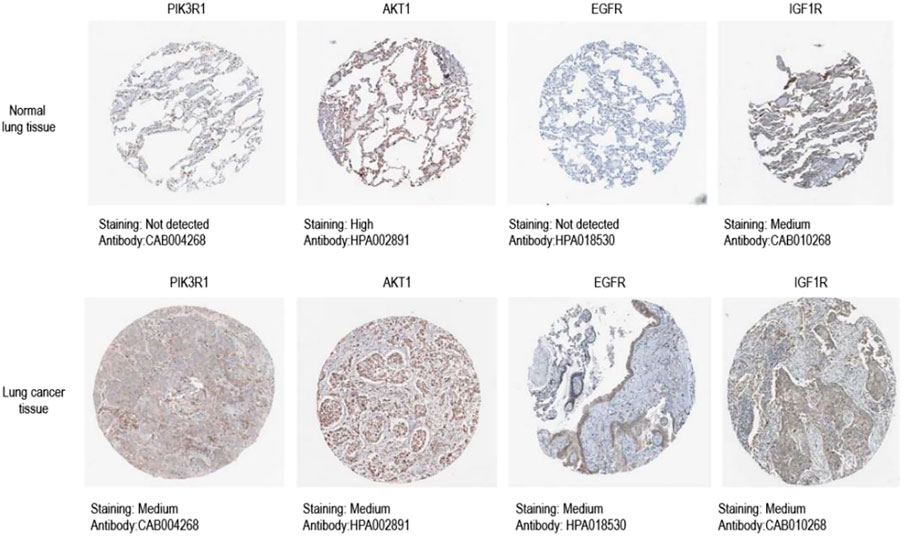
FIGURE 8. Immunohistochemical image of core target protein expression level in HPA database.Kaplan-Meier analysis of four key genes, PIK3R1, AKT, EGFR and IGF1R, in lung cancer patients.
3.9 Survival analysis
Survival analysis of four key genes, PIK3R1, AKT, EGFR and IGF1R (Figure 9), showed that the expression of these four key genes was significantly correlated with the prognosis of patients (p < 0.05).
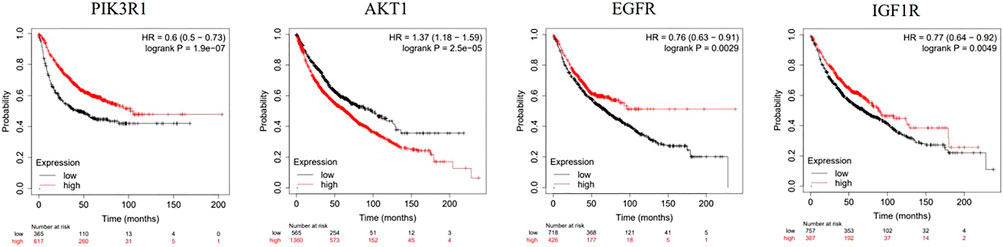
FIGURE 9. Kaplan-Meier analysis of four key genes, PIK3R1, AKT, EGFR and IGF1R, in lung cancer patients.
4 Discussion
According to the global cancer statistics in 2020, at present, lung cancer is the second most common malignant tumor among new malignant tumor cases in the world, accounting for 11.4% of all cases. It is also the “number one killer” of cancer deaths, accounting for 18.0% of the total cancer deaths 18.0% (Sung et al., 2021). NSCLC, as the most common type of lung cancer, has a high metastasis rate and mortality, which poses a serious threat to the survival of the patients (Ahlawat, Phutela, Bal, Singh, & Sharma, 2022). Therefore, it is of great significance to study the molecular mechanism of the occurrence and development of NSCLC and explore effective therapeutic drugs and schemes for preventing the occurrence of NSCLC and improving the prognosis of NSCLC patients. As a natural plant extract, kaempferol is a flavonoid, which has been proved to exert anticancer properties in various cancers through different modes of action (Chen & Chen, 2013; Han, Yu, Yang, Shen, & Wang, 2017; Zhu & Xue, 2019) And compared with standard chemotherapy drugs, the toxicity to normal cells is much smaller (Zhang, Chen, Li, Chen, & Yao, 2008). At the same time, some literatures have pointed out that kaempferol can inhibit the growth of lung cancer A549 cells, induce cell apoptosis, and limit cell migration and invasion (Imran et al., 2019). In this study, the potential molecular mechanism of kaempferol against NSCLC was preliminarily discussed based on network pharmacology, molecular docking, and bioinformatics, on the basis of verifying the efficacy in vitro. First, we verified that kaempferol can effectively inhibit the proliferation, migration and invasion of lung cancer A549 cells through CCK-8 experiment, scratch experiment and invasion experiment. Then, we predicted and screened kaempferol targets with SwissTargetPrediction and PharmMapper database, obtained NSCLC targets with GeneCards, TTD and GEO database, and obtained cross targets with Wayne platform. Then, we build PPI network, visualize it through Cytoscape3.9.1, and use its plug-in SytoHbba to screen and identify the targets for further analysis AKT1, ESR1, SRC, EGFR, MMP9; Next, Go and KEGG enrichment analysis was performed on the cross targets to construct a “pathway-target” target network. The genes related to the most pathways were PIK3R1, AKT1, EGFR, IGF1R and SRC, and the signal pathway related to the most targets was PI3K/Akt. Seven targets AKT1, PIK3R1, ESR1, SRC, EGFR, MMP9 and IGF1R were verified by SYBYL software, and the targets AKT1, PIK3R1, EGFR and IGF1R with good docking effect were obtained. The gene differential expression analysis, protein expression analysis and survival analysis of these four core targets were carried out. The results showed that, The expression levels of genes and proteins of these four core targets may be related to the progress of NSCLC, and they are also involved in the whole survival process of NSCLC patients, and have reliable correlation, which may prove to be potential biomarkers for clinical detection and treatment of NSCLC. Therefore, we predict that kaempferol may regulate PI3K/AKT signaling pathway by targeting PIK3R1, AKT1, EGFR and IGF1R genes, and inhibit the proliferation, invasion and migration of NSCLC cells.
PI3K/AKT signaling pathway is an important carcinogenesis pathway. The activation of this pathway can promote the growth, survival, migration and invasion of tumor cells by affecting tumor cell cycle, inhibiting tumor cell apoptosis, autophagy, promoting tumor angiogenesis and chemotherapy resistance, etc. The activation of this pathway is often found in NSCLC (Chin et al., 2018; Liao et al., 2019). Kaempferol can inhibit tumor angiogenesis by regulating PI3K/AKT, MEK and ERK (Chin et al., 2018). Kaempferol can also affect the growth of NSCLC, colorectal cancer and cervical cancer cells by regulating PI3K/AKT signaling pathway (Imran et al., 2019; Kashafi, Moradzadeh, Mohamadkhani, & Erfanian, 2017; Q; Li et al., 2019). PIK3R1 is the regulatory subunit of phosphoinositide 3 kinase (PI3K), which participates in the biological processes of various human malignant tumors (Yuan & Cantley, 2008). It is a potential upregulated oncogene in NSCLC, and plays an important role in the inhibition of the growth of NSCLC cells by miR-486-5p (Tian et al., 2019) AKT is a serine/threonine protein kinase, which is an important downstream effector of PI3K signal. Overactivation of PI3K leads to the accumulation of AKT, and AKT is sensitive to the level of epidermal growth factor (EGFR) (Nomura et al., 2003; Chin et al., 2018). AKT1, as a subtype of AKT, may play an important role in the occurrence and development of non-small cell lung cancer (Zhou et al., 2019). Kaempferol Suppresses Transforming Growth Factor-β1–Induced Epithelial-to-Mesenchymal Transition and Migration of A549 Lung Cancer Cells by Inhibiting Akt1-Mediated Phosphorylation of Smad3 at Threonine-179 (Jo, Park, Choi, Jeon, & Kim, 2015). Epidermal growth factor receptor (EGFR) is a 170 kDa receptor tyrosine kinase (RTK). Phosphorylation of EGFR can activate phosphatidylinositol 3 kinase (PI3K), which activates downstream signal molecules in the pathway, thus regulating tumor cell proliferation, invasion, migration and apoptosis (Y. Liu et al., 2020). Inhibition of EGFR/PI3K/Akt signaling pathway can inhibit the proliferation, invasion and migration of NSCLC cells (Sato et al., 2018). Shihua Yao et al. pointed out that kaempferol has a direct effect on the activity of epidermal growth factor receptor (EGFR), while inhibiting EGFR, its downstream signaling pathway is also obviously inhibited. And confirmed kaempferol inhibitions cell proliferation and glycilysis in esophagus squamus cell carcinogena via targeting EGFR signaling pathway (Yao et al., 2016). Insulin-like growth factor 1 receptor (IGF1R) is a transmembrane receptor tyrosine kinase receptor and the upstream regulator of Akt. It is highly expressed in a variety of cancers such as lung adenocarcinoma, pancreatic cancer and breast cancer (Baserga, Peruzzi, & Reiss, 2003; Allen et al., 2007). Generally speaking, IGF1R will be activated by a specific ligand in the extracellular space (for example, IGF-I, IGF-II) (Durai, Yang, Gupta, Seifalian, & Winslet, 2005; Kim, Cho, Paik, & Kim, 2012) Then it will activate downstream PI3K/AKT signaling pathways, and play a vital role in cell proliferation and differentiation and anti-apoptosis (Bertrand et al., 2006; C. H; Liu et al., 2013). Hyun Sook Lee et al. proved that Kaempferol down regulations activation of PI3K/Akt and ERK-1/2 pathways by inhibiting IGF-IR and erbb3 signaling in HT-29 cells (Lee, Cho, Kwon, & Park, 2014).
In a word, EGFR, IGF1R, PIK3R1 and AKT are all expressed in different degrees in NSCLC. Kaempferol may regulate EGFR/PI3K/AKT and IGF1R/PI3K/AKT signaling pathways by targeting EGFR, IGF1R, PIK3R1 and Akt, and then play a role in the treatment of non-small cell lung cancer.
5 Conclusion
To sum up, this study verified that kaempferol can inhibit the proliferation, migration and invasion of lung cancer A549 cells based on in vitro experiments, and verified the pharmacological characteristics of kaempferol with multiple targets and multiple pathways based on network pharmacology, molecular docking and bioinformatics. It was predicted that Kaempferol may regulate EGFR/PI3K/AKT and IGF1R/PI3K/AKT signaling pathways by targeting EGFR, IGF1R, PIK3R1 and Akt1, which has certain guiding significance for the following experimental research and clinical research. Although there are some limitations in network pharmacology and molecular docking, it points out the direction for our subsequent experimental mechanism research.
Data availability statement
Publicly available datasets were analyzed in this study. This data can be found here: Pubchem (https://pubchem.ncbi.nlm.nih.gov/) Swiss Target Prediction (https://www.swisstargetprediction.ch/) Pharm Mapper (https://www.lilab-ecust.cn/pharmmapper/). UniprotKB (https://www.uniprot.gov/) Gene Cards database and TTD database, GEO database, GEPIA (https://gepia.cancer-pku.cn/), HPA (https://www.proteinatlas.org/), Kaplan-Meier Plotter Database (https://kmplot.com/analysis/).
Author contributions
QZ, PS, JZ, GZ, and XL conceived and designed the study; JW, CL, ZL, and HW, performed the study; LD, KC, and MS analyzed the data; JZ, GZ, and XL wrote the paper. All authors read and approved the final article. All authors listed have made a substantial, direct, and intellectual contribution to the work and approved it for publication.
Funding
This work was supported by the National Natural Science Foundation of China (82170085, 81970064, 81870001, 81670064, and 31670365), the Natural Science Foundation of Shandong Province (ZR2020MH009 and ZR2020MH010), the National Natural Science Foundation of China (NSFC, Nos. 81874419, 81673719, and 81303074), National Health Commission of the People’s Republic of China (Nos 2017ZX09301064 and 2017ZX09301064002), Natural Science Foundation of Shandong Province (Nos ZR2020MB108 and ZR2019MH063), Central Government Guided Local Science and Technology Development Project (22-1-3-11-zyyd-nsh-3), Shandong Major Innovation Project (2022CXGC020514) and the Youth Research and Innovation Team of TCM-Based Neuroimmune Pharmacology, University of Shandong Traditional Chinese Medicine (No. 22202112).
Conflict of interest
The authors declare that the research was conducted in the absence of any commercial or financial relationships that could be construed as a potential conflict of interest.
Publisher’s note
All claims expressed in this article are solely those of the authors and do not necessarily represent those of their affiliated organizations, or those of the publisher, the editors and the reviewers. Any product that may be evaluated in this article, or claim that may be made by its manufacturer, is not guaranteed or endorsed by the publisher.
Supplementary material
The Supplementary Material for this article can be found online at: https://www.frontiersin.org/articles/10.3389/fphar.2023.1148171/full#supplementary-material
References
Ahlawat, P., Phutela, K., Bal, A., Singh, N., and Sharma, S. (2022). Therapeutic potential of human serum albumin nanoparticles encapsulated actinonin in murine model of lung adenocarcinoma. Drug Deliv. 29 (1), 2403–2413. doi:10.1080/10717544.2022.2067600
Allen, G. W., Saba, C., Armstrong, E. A., Huang, S. M., Benavente, S., Ludwig, D. L., et al. (2007). Insulin-like growth factor-I receptor signaling blockade combined with radiation. Cancer Res. 67 (3), 1155–1162. doi:10.1158/0008-5472.CAN-06-2000
Baserga, R., Peruzzi, F., and Reiss, K. (2003). The IGF-1 receptor in cancer biology. Int. J. Cancer 107 (6), 873–877. doi:10.1002/ijc.11487
Bertrand, F. E., Steelman, L. S., Chappell, W. H., Abrams, S. L., Shelton, J. G., White, E. R., et al. (2006). Synergy between an IGF-1R antibody and Raf/MEK/ERK and PI3K/Akt/mTOR pathway inhibitors in suppressing IGF-1R-mediated growth in hematopoietic cells. Leukemia 20 (7), 1254–1260. doi:10.1038/sj.leu.2404217
Budisan, L., Gulei, D., Jurj, A., Braicu, C., Zanoaga, O., Cojocneanu, R., et al. (2019). Inhibitory effect of CAPE and kaempferol in colon cancer cell lines-possible implications in new therapeutic strategies. Int. J. Mol. Sci. 20 (5), 1199. doi:10.3390/ijms20051199
Chen, A. Y., and Chen, Y. C. (2013). A review of the dietary flavonoid, kaempferol on human health and cancer chemoprevention. Food Chem. 138 (4), 2099–2107. doi:10.1016/j.foodchem.2012.11.139
Chin, H. K., Horng, C. T., Liu, Y. S., Lu, C. C., Su, C. Y., Chen, P. S., et al. (2018). Kaempferol inhibits angiogenic ability by targeting VEGF receptor-2 and downregulating the PI3K/AKT, MEK and ERK pathways in VEGF-stimulated human umbilical vein endothelial cells. Oncol. Rep. 39 (5), 2351–2357. doi:10.3892/or.2018.6312
Durai, R., Yang, W., Gupta, S., Seifalian, A. M., and Winslet, M. C. (2005). The role of the insulin-like growth factor system in colorectal cancer: Review of current knowledge. Int. J. Colorectal Dis. 20 (3), 203–220. doi:10.1007/s00384-004-0675-4
Han, B., Yu, Y. Q., Yang, Q. L., Shen, C. Y., and Wang, X. J. (2017). Kaempferol induces autophagic cell death of hepatocellular carcinoma cells via activating AMPK signaling. Oncotarget 8 (49), 86227–86239. doi:10.18632/oncotarget.21043
Imran, M., Salehi, B., Sharifi-Rad, J., Aslam Gondal, T., Saeed, F., Imran, A., et al. (2019). Kaempferol: A key emphasis to its anticancer potential. Molecules 24 (12), 2277. doi:10.3390/molecules24122277
Jain, A. N. (2003). Surflex: fully automatic flexible molecular docking using a molecular similarity-based search engine. J. Med. Chem. 46 (4), 499–511. doi:10.1021/jm020406h
Jo, E., Park, S. J., Choi, Y. S., Jeon, W. K., and Kim, B. C. (2015). Kaempferol suppresses transforming growth factor-β1-induced epithelial-to-mesenchymal transition and migration of A549 lung cancer cells by inhibiting akt1-mediated phosphorylation of Smad3 at threonine-179. Neoplasia 17 (7), 525–537. doi:10.1016/j.neo.2015.06.004
Kashafi, E., Moradzadeh, M., Mohamadkhani, A., and Erfanian, S. (2017). Kaempferol increases apoptosis in human cervical cancer HeLa cells via PI3K/AKT and telomerase pathways. Biomed. Pharmacother. 89, 573–577. doi:10.1016/j.biopha.2017.02.061
Kim, T. R., Cho, E. W., Paik, S. G., and Kim, I. G. (2012). Hypoxia-induced SM22α in A549 cells activates the IGF1R/PI3K/Akt pathway, conferring cellular resistance against chemo- and radiation therapy. FEBS Lett. 586 (4), 303–309. doi:10.1016/j.febslet.2011.12.036
Kumar, V., Yadavilli, S., and Kannan, R. (2021). A review on RNAi therapy for NSCLC: Opportunities and challenges. Wiley Interdiscip. Rev. Nanomed Nanobiotechnol 13 (2), e1677. doi:10.1002/wnan.1677
Lee, H. S., Cho, H. J., Kwon, G. T., and Park, J. H. (2014). Kaempferol downregulates insulin-like growth factor-I receptor and ErbB3 signaling in HT-29 human colon cancer cells. J. Cancer Prev. 19 (3), 161–169. doi:10.15430/JCP.2014.19.2.161
Leung, H. W., Lin, C. J., Hour, M. J., Yang, W. H., Wang, M. Y., and Lee, H. Z. (2007). Kaempferol induces apoptosis in human lung non-small carcinoma cells accompanied by an induction of antioxidant enzymes. Food Chem. Toxicol. 45 (10), 2005–2013. doi:10.1016/j.fct.2007.04.023
Li, C., Qiu, Y., and Zhang, Y. (2022). Research progress on therapeutic targeting of cancer-associated fibroblasts to tackle treatment-resistant NSCLC. Pharm. (Basel) 15 (11), 1411. doi:10.3390/ph15111411
Li, Q., Wei, L., Lin, S., Chen, Y., Lin, J., and Peng, J. (2019). Synergistic effect of kaempferol and 5-fluorouracil on the growth of colorectal cancer cells by regulating the PI3K/Akt signaling pathway. Mol. Med. Rep. 20 (1), 728–734. doi:10.3892/mmr.2019.10296
Liao, X. Z., Gao, Y., Huang, S., Chen, Z. Z., Sun, L. L., Liu, J. H., et al. (2019). Tanshinone IIA combined with cisplatin synergistically inhibits non-small-cell lung cancer in vitro and in vivo via down-regulating the phosphatidylinositol 3-kinase/Akt signalling pathway. Phytother. Res. 33 (9), 2298–2309. doi:10.1002/ptr.6392
Liu, C. H., Bao, H. G., Ge, Y. L., Wang, S. K., Shen, Y., and Xu, L. (2013). Celecoxib inhibits insulin-like growth factor 1 induced growth and invasion in non-small cell lung cancer. Oncol. Lett. 5 (6), 1943–1947. doi:10.3892/ol.2013.1277
Liu, Y., Jia, J., Song, B., Qiu, H., Liang, G., Zhang, B., et al. (2020). Serum microRNA-365 suppresses non-small-cell lung cancer metastasis and invasion in patients with bone metastasis of lung cancer. J. Int. Med. Res. 48 (10), 300060520939718. doi:10.1177/0300060520939718
Nomura, M., He, Z., Koyama, I., Ma, W. Y., Miyamoto, K., and Dong, Z. (2003). Involvement of the Akt/mTOR pathway on EGF-induced cell transformation. Mol. Carcinog. 38 (1), 25–32. doi:10.1002/mc.10140
Rajendran, P., Abdelsalam, S. A., Renu, K., Veeraraghavan, V., Ben Ammar, R., and Ahmed, E. A. (2022). Polyphenols as potent epigenetics agents for cancer. Int. J. Mol. Sci. 23 (19), 11712. doi:10.3390/ijms231911712
Rajendran, P., Rengarajan, T., Nandakumar, N., Palaniswami, R., Nishigaki, Y., and Nishigaki, I. (2014). Kaempferol, a potential cytostatic and cure for inflammatory disorders. Eur. J. Med. Chem. 86, 103–112. doi:10.1016/j.ejmech.2014.08.011
Relli, V., Trerotola, M., Guerra, E., and Alberti, S. (2019). Abandoning the notion of non-small cell lung cancer. Trends Mol. Med. 25 (7), 585–594. doi:10.1016/j.molmed.2019.04.012
Sato, H., Yamamoto, H., Sakaguchi, M., Shien, K., Tomida, S., Shien, T., et al. (2018). Combined inhibition of MEK and PI3K pathways overcomes acquired resistance to EGFR-TKIs in non-small cell lung cancer. Cancer Sci. 109 (10), 3183–3196. doi:10.1111/cas.13763
Sung, H., Ferlay, J., Siegel, R. L., Laversanne, M., Soerjomataram, I., Jemal, A., et al. (2021). Global cancer statistics 2020: GLOBOCAN estimates of incidence and mortality worldwide for 36 cancers in 185 countries. CA Cancer J. Clin. 71 (3), 209–249. doi:10.3322/caac.21660
Tian, F., Wang, J., Ouyang, T., Lu, N., Lu, J., Shen, Y., et al. (2019). MiR-486-5p serves as a good biomarker in nonsmall cell lung cancer and suppresses cell growth with the involvement of a target PIK3R1. Front. Genet. 10, 688. doi:10.3389/fgene.2019.00688
Wang, L., Qu, J., Liang, Y., Zhao, D., Rehman, F. U., Qin, K., et al. (2020). Identification and validation of key genes with prognostic value in non-small-cell lung cancer via integrated bioinformatics analysis. Thorac. Cancer 11 (4), 851–866. doi:10.1111/1759-7714.13298
Wang, X., Hu, Y., Zhou, X., and Li, S. (2022). Editorial: Network pharmacology and traditional medicine: Setting the new standards by combining in silico and experimental work. Front. Pharmacol. 13, 1002537. doi:10.3389/fphar.2022.1002537
Yao, S., Wang, X., Li, C., Zhao, T., Jin, H., and Fang, W. (2016). Kaempferol inhibits cell proliferation and glycolysis in esophagus squamous cell carcinoma via targeting EGFR signaling pathway. Tumour Biol. 37 (8), 10247–10256. doi:10.1007/s13277-016-4912-6
Yuan, T. L., and Cantley, L. C. (2008). PI3K pathway alterations in cancer: Variations on a theme. Oncogene 27 (41), 5497–5510. doi:10.1038/onc.2008.245
Zhang, Y., Chen, A. Y., Li, M., Chen, C., and Yao, Q. (2008). Ginkgo biloba extract kaempferol inhibits cell proliferation and induces apoptosis in pancreatic cancer cells. J. Surg. Res. 148 (1), 17–23. doi:10.1016/j.jss.2008.02.036
Zhou, J., Sun, M., Jin, S., Fan, L., Zhu, W., Sui, X., et al. (2019). Combined using of paclitaxel and salinomycin active targeting nanostructured lipid carriers against non-small cell lung cancer and cancer stem cells. Drug Deliv. 26 (1), 281–289. doi:10.1080/10717544.2019.1580799
Keywords: kaempferol (compound CID 5280863), network pharmacology, NSCLC, molecular docking, in vitro experiments
Citation: Zhang J, Liu X, Zhang G, Wu J, Liu Z, Liu C, Wang H, Miao S, Deng L, Cao K, Shang M, Zhu Q and Sun P (2023) To explore the effect of kaempferol on non-small cell lung cancer based on network pharmacology and molecular docking. Front. Pharmacol. 14:1148171. doi: 10.3389/fphar.2023.1148171
Received: 19 January 2023; Accepted: 27 April 2023;
Published: 18 July 2023.
Edited by:
Carmela Spagnuolo, National Research Council (CNR), ItalyReviewed by:
Idolo Tedesco, National Research Council (CNR), ItalyWisnu Ananta Kusuma, IPB University, Indonesia
Copyright © 2023 Zhang, Liu, Zhang, Wu, Liu, Liu, Wang, Miao, Deng, Cao, Shang, Zhu and Sun. This is an open-access article distributed under the terms of the Creative Commons Attribution License (CC BY). The use, distribution or reproduction in other forums is permitted, provided the original author(s) and the copyright owner(s) are credited and that the original publication in this journal is cited, in accordance with accepted academic practice. No use, distribution or reproduction is permitted which does not comply with these terms.
*Correspondence: Qingjun Zhu, zhuqingjuncn@hotmail.com; Peng Sun, sunpeng@sdutcm.edu.cn