- 1Center for Evidence-Based Medicine and Clinical Research, Taihe Hospital, Hubei University of Medicine, Shiyan, Hubei, China
- 2Department of Emergency, Renmin Hospital, Hubei University of Medicine, Shiyan, Hubei, China
- 3Department of Stomatology, Taihe Hospital, Hubei University of Medicine, Shiyan, Hubei, China
Background: Even 3 years into the COVID-19 pandemic, questions remain about how to safely and effectively vaccinate vulnerable populations. A systematic analysis of the safety and efficacy of the COVID-19 vaccine in at-risk groups has not been conducted to date.
Methods: This study involved a comprehensive search of PubMed, EMBASE, and Cochrane Central Controlled Trial Registry data through 12 July 2022. Post-vaccination outcomes included the number of humoral and cellular immune responders in vulnerable and healthy populations, antibody levels in humoral immune responders, and adverse events.
Results: A total of 23 articles assessing 32 studies, were included. The levels of IgG (SMD = −1.82, 95% CI [−2.28, −1.35]), IgA (SMD = −0.37, 95% CI [−0.70, −0.03]), IgM (SMD = −0.94, 95% CI [−1.38, −0.51]), neutralizing antibodies (SMD = −1.37, 95% CI [−2.62, −0.11]), and T cells (SMD = −1.98, 95% CI [−3.44, −0.53]) were significantly lower in vulnerable than in healthy populations. The positive detection rates of IgG (OR = 0.05, 95% CI [0.02, 0.14]) and IgA (OR = 0.03, 95% CI [0.01, 0.11]) antibodies and the cellular immune response rates (OR = 0.20, 95% CI [0.09, 0.45]) were also lower in the vulnerable populations. There were no statistically significant differences in fever (OR = 2.53, 95% CI [0.11, 60.86]), chills (OR = 2.03, 95% CI [0.08, 53.85]), myalgia (OR = 10.31, 95% CI [0.56, 191.08]), local pain at the injection site (OR = 17.83, 95% CI [0.32, 989.06]), headache (OR = 53.57, 95% CI [3.21, 892.79]), tenderness (OR = 2.68, 95% CI [0.49, 14.73]), and fatigue (OR = 22.89, 95% CI [0.45, 1164.22]) between the vulnerable and healthy populations.
Conclusion: Seroconversion rates after COVID-19 vaccination were generally worse in the vulnerable than healthy populations, but there was no difference in adverse events. Patients with hematological cancers had the lowest IgG antibody levels of all the vulnerable populations, so closer attention to these patients is recommended. Subjects who received the combined vaccine had higher antibody levels than those who received the single vaccine.
1 Introduction
Coronavirus disease 2019 (COVID-19), a highly transmissible viral illness caused by severe acute respiratory syndrome coronavirus 2 (SARS-CoV-2), quickly spread worldwide and became a significant global health crisis (Chan et al., 2020). While SARS-CoV-2 infection can have a debilitating long-term effect on daily activities, no drugs are available to safely and effectively treat COVID-19 (Huang et al., 2020). As a result, timely vaccination is the most effective preventive measure to minimize the negative impact of this infection at both the individual and community level (Majid et al., 2021).
Deployment of the COVID-19 vaccine cannot be standardized across the entire population because particular subgroups carry different risks of exposure to SARS-CoV-2 (Grassly et al., 2015; Koff and Williams, 2020). Some individuals are at higher risk of becoming infected and/or developing severe disease or may not have access to timely and effective treatment (Koff et al., 2021). Thus, strengthening prevention efforts among vulnerable populations, including ensuring adequate COVID-19 vaccination coverage, can help reduce the risk of a large-scale outbreak (Lipsitch and Dean, 2020). Populations considered vulnerable to COVID-19 include the elderly, pregnant women, children, people with underlying medical conditions, and those with limited access to primary medical care (Agrati et al., 2021).
The safest and most effective method of vaccinating vulnerable populations against COVID-19 remains unclear, and particular subgroups are still hesitant to receive the vaccine (Grassly et al., 2015). One study found that the infection rate of kidney transplant patients who received the same vaccination regimen as healthy patients at the peak of the pandemic remained unchanged, suggesting that this at-risk population requires an individualized vaccination protocol (Swai et al., 2022). Few studies have assessed the impact of COVID-19 vaccination in elderly populations, so evidence is lacking on how best to support the development of a vaccine initiative for this age group (Veronese et al., 2021). Seroconversion following COVID-19 vaccination is often lower in vulnerable than healthy populations (Thuluvath et al., 2021). Systematic analysis of available data is needed to explore options for increasing seroconversion and assessing the safety of COVID-19 vaccination of at-risk populations.
The current study evaluated humoral and cellular immune responses to the COVID-19 vaccine and the adverse effects (AEs) of vaccination in vulnerable and healthy populations. Meta-analysis and subgroup analyses were performed to comprehensively assess vaccine efficacy and safety in at-risk populations. The findings should inform the development of a protocol for COVID-19 vaccination of different vulnerable populations and help to alleviate vaccine hesitation.
2 Methods
This study was performed according to the Preferred Reporting Initiative for Systematic Assessment and Meta-Analysis (PRISMA) guidelines (Page et al., 2021).
2.1 Search strategy
This study conducted a meta-analysis of reports in PubMed, EMbase, and the Cochrane Central Register of Controlled Trials (CENTRAL) through 12 July 2022. Mesh terms including “vulnerable populations,” “chronic disease,” “underserved populations,” “sensitive populations,” “immunocompromised patients,” “aged,” “organ transplantation,” “neoplasms,” “tumor,” “cancer,” and “COVID-19 vaccines,” were used to search the three databases. A manual search of references from the included studies was also performed. The search strategies are described in Supplementary Method S1.
2.2 Inclusion and exclusion criteria
The subjects included in this meta-analysis were vulnerable and healthy recipients of the COVID-19 vaccine. The exposure group, defined as vulnerable, included elderly patients, immunocompromised patients, organ transplant recipients, and cancer patients. Elderly subjects were defined as those ≥60 years of age. Immunocompromised patients included AIDS patients, maintenance hemodialysis patients, and those receiving immunosuppressive drugs. Organ transplant recipients included common kidney and liver transplant patients. Cancer patients included those with solid cancers or hematological malignancies. The non-exposure group was defined as healthy. None of the subjects were previously infected with COVID-19. Outcomes included the number of subjects in each group with a measurable humoral and cellular immune response, the levels of antibodies in humoral immune responders after vaccination, and vaccination-related AEs [including fever, chills, myalgia, local pain at the site of injection, headache, tenderness, fatigue, gastrointestinal disturbances, arthralgia, local reddening, local swelling, lymph node swelling, malaise, flu-like symptoms, and a need for non-steroidal anti-inflammatory drugs (NSAIDs)]. Subjects with detectable serum levels of SARS-CoV-2 immunoglobulins (including IgG, IgA, and IgM) were defined as humoral immune responders. A measurable cellular immune response was defined as the presence of T cells that were activated in response to SARS-CoV-2 antigen. The primary outcomes included serum IgG antibody levels after vaccination and the number of virus-specific antibodies. Secondary outcomes included the number of patients with serum IgA and neutralizing antibody levels, IgA and IgM antibody levels, the cellular immune response rate, and the incidence of post-vaccine AEs.
Studies involving subjects who were children, pregnant women, received only one dose of the COVID-19 vaccine or lacked sufficient data were excluded, along with non-English publications, and those that replicated a prior study.
2.3 Study selection and data extraction
Studies were screened by two independent reviewers (Hui-Jun Li and Yang-Yang Yao). A third reviewer (Chao Zhang) was consulted when there was uncertainty about including a study. The following data were extracted from studies that met the inclusion criteria: name of the first author, year of publication, subject demographic information (mean age and gender), type of vaccine administered, dose of vaccine administered, time between vaccine dosages, the time between vaccination and serologic diagnosis, the number of occurrences of humoral immune responses and antibody levels, the number of occurrences of cellular immune responses, the number of post-vaccine AEs, and the number of people with post-vaccine AEs. Studies with multiple time points for serological diagnosis or more than one type of vulnerable population were defined as multiple studies during data extraction. If the continuous variable was shown as the median and interquartile range (IQR), data were extracted by estimating the sample mean and standard deviation based on the approximate formula for optimal weights (Shi et al., 2020).
2.4 Quality assessment
Two reviewers (Hui-Jun Li and Cheng-Yang Huang) independently used the Cochrane Risk of Bias 2.0 (RoB-2.0) to assess the quality of the included literature. The selected items included confounding bias, subject selection bias, intervention classification bias, bias in deviation from established interventions, missing data bias, endpoint measurement bias, and selective reporting bias. Responses to each question were selected from “Yes,” “Probably Yes,” “No,” “Probably No,” “No Information” and “Not Applicable.” Any disagreement between the two reviewers was resolved by a third reviewer (Shao-Juan Chen).
2.5 Statistical analysis
The effect size for outcomes that belonged to dichotomous data was presented as the odds ratio (OR) calculated using the Mantel-Haenszel method, with a corresponding 95% confidence interval (CI). For continuous data on outcomes, the effect size of the standard mean difference (SMD) was used with a 95% CI. Heterogeneity was assessed by calculating the I2 and p values. Data were analyzed using a random-effects model When p < 0.1 and I2 >40%, the data were analyzed using a random-effects model, and when p > 0.1 and I2 <40%, the data were analyzed using a fixed-effects model. According to Cochrane’s handbook, an I2 value ≤ 40% represents mild heterogeneity and a value > 40% represents significant heterogeneity. To further examine heterogeneity, various confounding factors were introduced into the subgroup analysis. IgG antibody levels were analysed in subgroups by type of disease [hemodialysis, organ transplant (kidney or liver), solid cancer, hematological malignancies (multiple myeloma, myeloproliferative neoplasms, and other haematological cancers)], elderly (yes/no), vaccination subtype (BNT162b2 or BNT162b2 mixed with other vaccines), vaccination dose (first and second), the time between doses (≤21 days or >21 days), number of days from vaccination to COVID-19 serologic test (≤21 days after the first vaccination dose, >21 days after the first vaccination dose, ≤21 days after the second vaccination dose, and >21 days after the second vaccination dose), antibody detection method [enzyme-linked immunosorbent assay (ELISA) and LIAISON], type of antibodies (anti-spike, anti-S1, anti-N, anti-S, and anti-RBD) and COVID-19 infection status (absence of infection, infection after vaccination, uncertain about infection). RevMan 5.4 was used for all statistical analyses.
3 Results
3.1 Study selection
A total of 5,340 articles were selected for the initial screening and after reading the titles and abstracts, 5,317 that did not meet the inclusion criteria were excluded. A total of 23 articles (Grupper et al., 2021a; Palich et al., 2021a; Grupper et al., 2021b; Palich et al., 2021b; Geisen et al., 2021; Goupil et al., 2021; Herzog Tzarfati et al., 2021; Korth et al., 2021; Levy et al., 2021; Massarweh et al., 2021; Monin et al., 2021; Moor et al., 2021; Pimpinelli et al., 2021; Rabinowich et al., 2021; Sattler et al., 2021; Speer et al., 2021; Stumpf et al., 2021; Tzioufas et al., 2021; Waissengrin et al., 2021; Waldhorn et al., 2021; Yanay et al., 2021; Ligumsky et al., 2022; Shem-Tov et al., 2022) covering 32 observational studies were finally included. The specific screening process is shown in Figure 1.
3.2 Study characteristics
The characteristics of the 32 observational studies (Grupper et al., 2021a; Palich et al., 2021a; Grupper et al., 2021b; Palich et al., 2021b; Geisen et al., 2021; Goupil et al., 2021; Herzog Tzarfati et al., 2021; Korth et al., 2021; Levy et al., 2021; Massarweh et al., 2021; Monin et al., 2021; Moor et al., 2021; Pimpinelli et al., 2021; Rabinowich et al., 2021; Sattler et al., 2021; Speer et al., 2021; Stumpf et al., 2021; Tzioufas et al., 2021; Waissengrin et al., 2021; Waldhorn et al., 2021; Yanay et al., 2021; Ligumsky et al., 2022; Shem-Tov et al., 2022), which included 4,875 vulnerable subjects and 2,285 healthy subjects, are shown in Table 1. The study subjects included cancer patients receiving immune checkpoint inhibitors, immunosuppressive therapy for chronic inflammatory diseases, maintenance hemodialysis, kidney and liver transplants, as well as those with solid cancers, hematologic malignancies, a history of CD20 B-cell depletion therapy, myeloproliferative tumors, human immunodeficiency virus (HIV) infection, autoimmune rheumatism, recipients of hematopoietic stem cell transplantation, and systemic autoimmune diseases. The subjects in this study were all ≥18 years of age and none had been infected with COVID-19 prior to vaccination. Most subjects received the BNT162b2 vaccination. The interval between the first and second vaccine dose was 3 weeks in most cases, and the interval between vaccination and serological diagnosis was 1–8 weeks. Of the 23 included studies, one (Speer et al., 2021) explicitly excluded those infected with COVID-19 after vaccination, seven (Palich et al., 2021a; Massarweh et al., 2021; Rabinowich et al., 2021; Sattler et al., 2021; Stumpf et al., 2021; Tzioufas et al., 2021; Shem-Tov et al., 2022) used nucleic acid testing to ensure that the included subjects were not infected with COVID-19 prior to vaccination, five (Grupper et al., 2021b; Goupil et al., 2021; Monin et al., 2021; Yanay et al., 2021; Ligumsky et al., 2022) documented subjects with asymptomatic or symptomatic infection after vaccination, and nine (Grupper et al., 2021a; Palich et al., 2021b; Geisen et al., 2021; Korth et al., 2021; Levy et al., 2021; Moor et al., 2021; Tzioufas et al., 2021; Waissengrin et al., 2021; Waldhorn et al., 2021) included a statement that the included subjects did not have COVID-19 prior to vaccination. Most studies measured the post-vaccine humoral immune response using an enzyme-linked immunosorbent assay (ELISA) or LIAISON. Individual studies assessed cellular immune responses using an interferon- γ release assay (IGRA) or fluorescent spot analysis of interferon-gamma (IFN-γ)-producing and interleukin-2 (IL-2)-producing SARS-CoV-2 reactive T cells.
3.3 Quality evaluation
The results of a qualitative evaluation of this study are shown in Table 2. None of the 24 studies had a potential confounding effect on the intervention. The selection of study subjects was not based on individual characteristics observed after the start of the intervention, and the intervention and follow-up did not coincide for most subjects. While the intervention groups were clearly defined, information used to characterize the intervention groups was not recorded at the start of the intervention, and the delineation of the intervention groups was not affected by knowledge or risks associated with the outcome. Significant concomitant interventions were likely balanced between intervention groups, and most subjects successfully implemented and were compliant with the assigned interventions. Knowledge about the intervention did not affect the outcome measurements, the outcome assessor was aware of the intervention the subject received, and there was no systematic error in the outcome measure associated with receiving the intervention. Outcomes were available for all subjects in all studies, and there was no selective reporting of multiple measures for specific outcomes or the effects of multiple analyses of the intervention-outcome relationship.
3.4 Humoral immune responses
3.4.1 IgG
A total of 13 articles describing 22 studies (Grupper et al., 2021a; Palich et al., 2021a; Grupper et al., 2021b; Geisen et al., 2021; Goupil et al., 2021; Herzog Tzarfati et al., 2021; Korth et al., 2021; Massarweh et al., 2021; Moor et al., 2021; Pimpinelli et al., 2021; Rabinowich et al., 2021; Speer et al., 2021; Yanay et al., 2021) that included 1,303 vulnerable subjects and 720 healthy subjects reported serum anti-SARS-CoV-2 IgG antibody levels. The enrolled vulnerable populations included patients receiving immunosuppressive therapy for chronic inflammatory diseases, hemodialysis, and kidney or liver transplants, as well as those with solid cancers, a history of CD20 B-cell depletion therapy, and hematologic malignancy. Anti-spiking IgG antibodies were significantly lower in the vulnerable than in the healthy populations (SMD = −1.82, 95% CI [−2.28, −1.35]) (Figure 2).
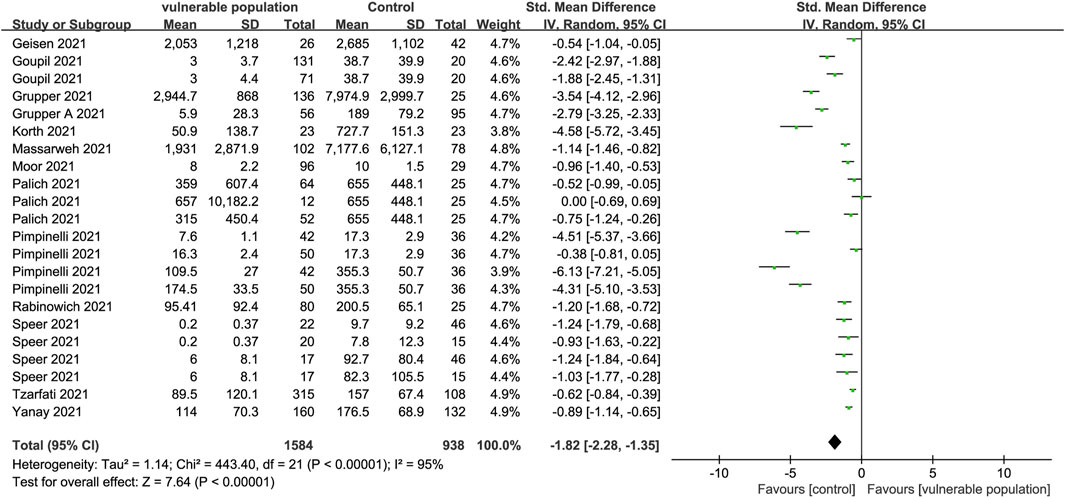
FIGURE 2. Forest plot showing the levels of IgG antibodies in sera of vulnerable and healthy populations.
A total of 17 studies (Palich et al., 2021a; Levy et al., 2021; Monin et al., 2021; Moor et al., 2021; Stumpf et al., 2021; Tzioufas et al., 2021; Waldhorn et al., 2021; Ligumsky et al., 2022; Shem-Tov et al., 2022) involving 3,100 vulnerable subjects and 1,259 healthy participants reported the proportion of individuals with positive IgG antibody test results. The vulnerable subjects could be classified into eight groups including patients with solid cancers, hematological illnesses, renal transplant, a history of CD20 B-cell depletion therapy, HIV, autoinflammatory rheumatic diseases, hematopoietic stem cell transplantation, and systemic autoimmune diseases. A positive antibody reaction was reported as follows: serum samples at a concentration of 50% of maximal effect (EC50) value using GraphPad Prism at a 1:25 dilution or an optical density (OD) ≥25 at 405 nm measured after four further dilutions (Levy et al., 2021); samples with an OD ratio >1 at 450–620 nm to the OD of the calibrator (Ligumsky et al., 2022); an anti-N IgG assay value ≥ 0.8 UA/mL and an anti-s IgG assay value ≥ 50 UA/mL (Monin et al., 2021); new antibodies at the T1 or T2 phase (seroconversion) (Stumpf et al., 2021). Serological diagnostic time points were set selectively at 1–8 weeks post-vaccination in each study according to their respective conditions. Anti-S IgG antibody levels were lower in the vulnerable than in the healthy populations (OR = 0.05, 95% CI [0.02, 0.14]) (Figure 3).
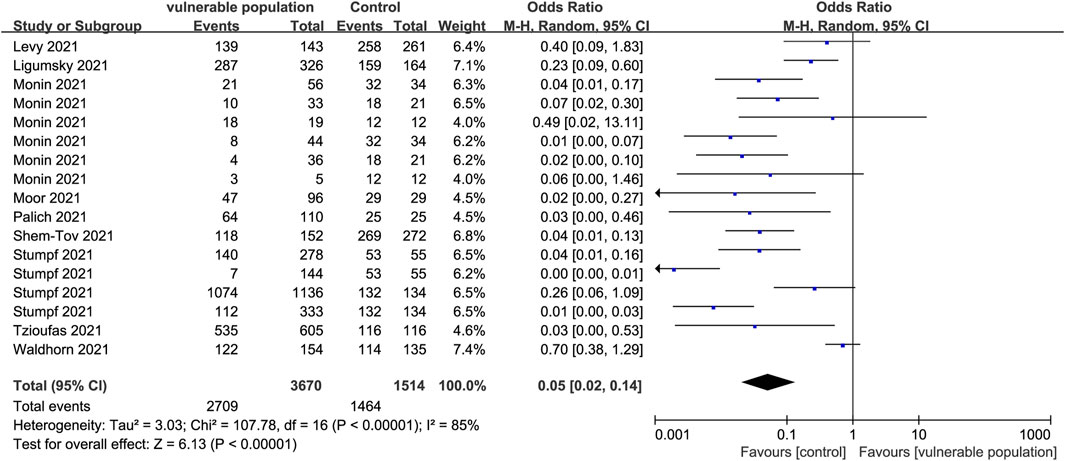
FIGURE 3. Forest plot showing the detection rate of positive IgG antibodies in sera of vulnerable and healthy populations.
3.4.2 IgA
Two studies (Geisen et al., 2021; Moor et al., 2021) examined IgA antibody levels in the serum of 122 vulnerable patients (those receiving immunosuppressive therapy for chronic inflammatory diseases and those with a history of CD20 b-cell depletion therapy) and 71 healthy subjects. Vulnerable subjects had significantly lower IgA antibody levels than healthy subjects (SMD = −0.37, 95% CI [−0.70, −0.03]) (Figure 4).

FIGURE 4. Forest plot showing the levels of IgA antibodies in sera of vulnerable and healthy populations.
Two studies (Sattler et al., 2021; Stumpf et al., 2021) reported the number of subjects with positive IgA antibody responses, determined by OD ratios ≥1.1, and the presence of new antibodies at T1 or T2 (seroconversion). They included 1,403 subjects from vulnerable populations (hemodialysis and renal transplant recipients) and 328 subjects from healthy populations. The proportion of IgA-positive subjects was lower in the vulnerable than in the healthy groups (OR = 0.03, 95% CI [0.01, 0.11]) (Figure 5).
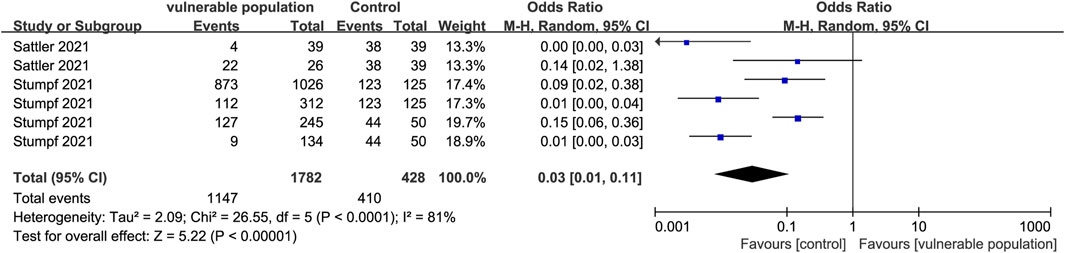
FIGURE 5. Forest plot showing the positive detection rate of IgG antibodies in sera of vulnerable and healthy populations.
3.4.3 IgM
Two studies (Goupil et al., 2021; Moor et al., 2021) enrolling 227 vulnerable subjects (hemodialysis patients and those with a history of CD20 b-cell depletion therapy) and 49 healthy subjects reported serum IgM antibody levels. The vulnerable subjects had significantly lower levels of IgM antibodies than the healthy subjects (SMD = −0.94, 95% CI [−1.38, −0.51]) (Figure 6).

FIGURE 6. Forest plot showing the levels of IgM antibodies in the sera of vulnerable and healthy populations.
3.4.4 Neutralizing antibody
Two studies (Geisen et al., 2021; Speer et al., 2021) including 48 vulnerable individuals (those receiving hemodialysis and those with chronic inflammatory diseases) and 88 healthy subjects, reported serum levels of neutralizing antibodies. Antibody levels were lower in the vulnerable groups than in the healthy groups (SMD = −1.37, 95% CI [−2.62, −0.11]) (Figure 7). One study (Grupper et al., 2021a) reported that kidney transplant recipients had relatively lower levels of serum antibodies than healthy subjects.

FIGURE 7. Forest plot showing the levels of neutralizing antibodies in sera of vulnerable and healthy populations.
3.5 Cellular immune responses
One study (Moor et al., 2021) examined CD3, CD4, and CD19 expression in the plasma of patients with a history of CD20 b-cell depletion therapy and healthy individuals. There were fewer CD3 (SMD = −1.14, 95% CI [−1.58, −0.70]), CD4 (SMD = −1.14, 95% CI [−1.58, −0.70]), and CD19 (SMD = −3.72, 95% CI [−4.34, −3.09]) expressing cells in the plasma of the vulnerable patients than the healthy subjects (Figure 8).
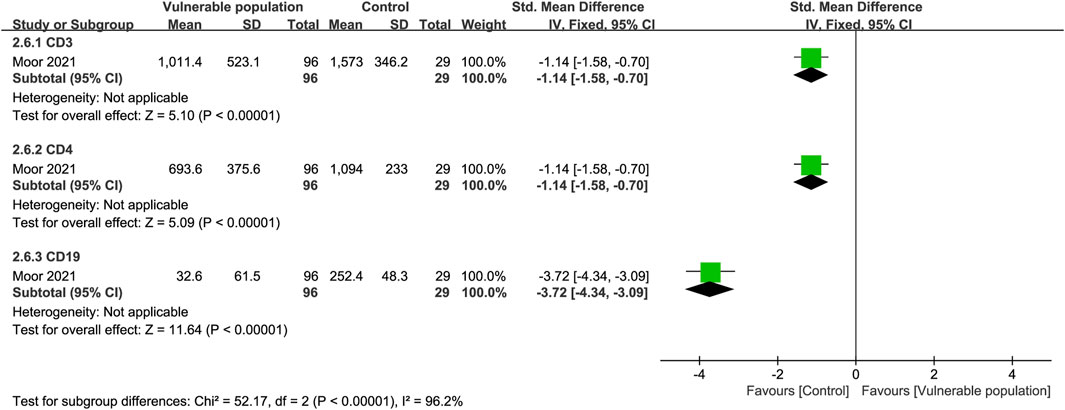
FIGURE 8. Forest plot showing the levels of CD3, CD4 and CD19 cells in the sera of vulnerable and healthy populations.
Two studies (Monin et al., 2021; Stumpf et al., 2021) enrolling 328 vulnerable people (hemodialysis patients and renal transplant recipients) and 106 healthy people, reported the number of subjects who developed a cellular immune response. The criteria for determining a positive antibody reaction were reported as follows: an EC50 that did not reach 1:25; an OD at 405 nm that was 4-fold above background and assigned a value of 25; IFN-γ release ≥100 MIU/mL. Vulnerable subjects had lower cellular immune responses than healthy subjects (OR = 0.20, 95% CI [0.09, 0.45]) (Figure 9).
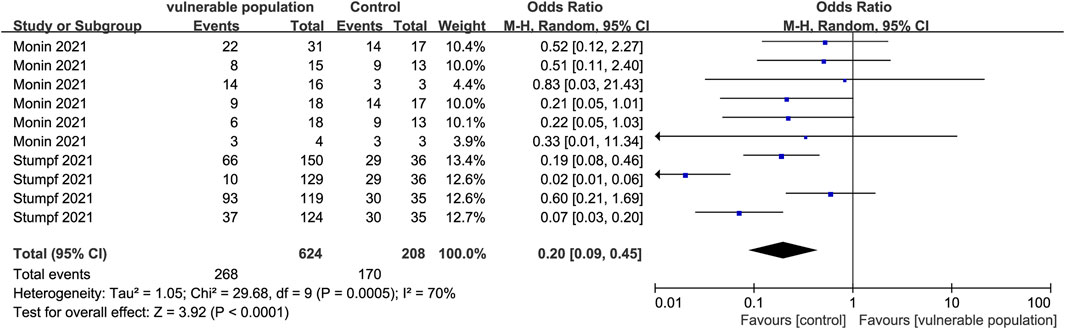
FIGURE 9. Forest plot showing the prevalence of positive cellular immune responses in vulnerable and healthy populations.
3.6 Adverse events
3.6.1 Fever
Three studies (Geisen et al., 2021; Pimpinelli et al., 2021; Waissengrin et al., 2021), including 255 vulnerable subjects and 215 healthy subjects, reported fever as an AE. Vulnerable populations included those receiving immunosuppressive treatment for chronic inflammatory diseases, hematologic malignancy patients, and cancer patients receiving immune checkpoint inhibitors. There was no statistical difference between the vulnerable and healthy groups (OR = 2.53, 95% CI [0.11, 60.86]).
3.6.2 Chills
Three trials (Geisen et al., 2021; Pimpinelli et al., 2021; Waissengrin et al., 2021) including 255 vulnerable subjects and 215 healthy subjects reported chills as AEs. Vulnerable subjects included patients receiving immunosuppressive therapies for chronic inflammatory diseases, hematologic malignancy patients, and cancer patients receiving immune checkpoint inhibitors. There was no statistical difference between the vulnerable and healthy groups (OR = 2.03, 95% CI [0.08, 53.85]).
3.6.3 Myalgia
AEs of myalgia occurred in four trials (Geisen et al., 2021; Pimpinelli et al., 2021; Waissengrin et al., 2021; Ligumsky et al., 2022) including 581 vulnerable subjects and 379 healthy subjects. Enrolled vulnerable subjects included patients receiving immunosuppressive therapies for chronic inflammatory diseases, hematologic malignancy patients, cancer patients receiving immune checkpoint inhibitors, and solid cancer patients. There was no statistical difference between the vulnerable and healthy groups (OR = 10.31, 95% CI [0.56, 191.08]).
3.6.4 Local pain at the site of injection
AEs of local pain at the injection site were reported by three studies (Grupper et al., 2021a; Geisen et al., 2021; Ligumsky et al., 2022) including 488 vulnerable subjects and 231 healthy subjects. Vulnerable populations included patients who received immunosuppressive therapy for chronic inflammatory diseases, renal transplant recipients, and solid cancer patients. There was no statistical difference between the vulnerable and healthy groups (OR = 17.83, 95% CI [0.32, 989.06]).
3.6.5 Headache
Headache AEs occurred in four studies (Geisen et al., 2021; Pimpinelli et al., 2021; Waissengrin et al., 2021; Ligumsky et al., 2022) of 673 vulnerable subjects and 415 healthy subjects. Vulnerable subjects included patients receiving immunosuppressive therapies for chronic inflammatory diseases, hematologic malignancy patients, solid cancer patients, and cancer patients receiving immune checkpoint inhibitors. Only one patient with multiple myeloma and myeloproliferative neoplasm experienced a headache during the first dose of BNT162b2, and two patients experienced a headache during the second dose of BNT162b2. There was no statistical difference between the vulnerable and healthy groups (OR = 4.80, 95% CI [0.75, 30.71]).
3.6.6 Tenderness
Injection-related tenderness AEs were reported in one study (Pimpinelli et al., 2021), including 92 vulnerable and 36 healthy subjects. Vulnerable subjects included patients receiving immunosuppressive therapy for chronic inflammatory diseases and renal transplant recipients. There was no statistical difference between the vulnerable and healthy groups (OR = 7.30, 95% CI [0.95, 55.91]).
3.6.7 Fatigue
AEs of fatigue were observed in three studies (Geisen et al., 2021; Waissengrin et al., 2021; Ligumsky et al., 2022) including 489 vulnerable and 343 healthy subjects. Vulnerable subjects included patients treated with immune checkpoint inhibitors, patients receiving immunosuppressive therapy for chronic inflammatory diseases, and solid cancer patients. There was no statistical difference between the vulnerable and healthy groups (OR = 22.89, 95% CI [0.45, 1164.22]).
3.6.8 Other adverse events
One study (Waissengrin et al., 2021) reported flu-like symptoms in three cancer patients receiving immune checkpoint inhibitors. Five patients (Pimpinelli et al., 2021) with multiple myeloma and myeloproliferative neoplasms, who received a single dose of BNT162b2, developed malaise. According to one study (Geisen et al., 2021), four of 26 immunosuppressed patients with chronic inflammatory diseases had arthralgia, two had local erythema, four had local swelling, three had swollen lymph nodes, nine required NSAIDs, and five had other AEs (not specified in the original article). Meanwhile, six of 42 subjects in the healthy group had arthralgia, two had local erythema, four had local swelling, four had swollen lymph nodes, ten required NSAIDs, and seven had other AEs (also not specified in the original article).
3.7 Stratification analysis
3.7.1 Vulnerable populations
Serum IgG antibody levels were lower in patients receiving hemodialysis (Grupper et al., 2021b; Goupil et al., 2021; Speer et al., 2021; Yanay et al., 2021) (SMD = −1.88, 95% CI [−3.03, −0.72]) or organ transplants (Grupper et al., 2021a; Korth et al., 2021; Rabinowich et al., 2021) (SMD = −2.78, 95% CI [−4.34, −1.22]) and those with hematological malignancies (Herzog Tzarfati et al., 2021; Pimpinelli et al., 2021) (SMD = −3.66, 95% CI [−7.15, −0.16]) or solid cancers (Palich et al., 2021b; Massarweh et al., 2021) (SMD = −0.85, 95% CI [−1.47, −0.24]) than in healthy individuals. Vulnerable patients with an average age >60 years were classified as elderly. A total of 1,116 vulnerable elderly subjects (Grupper et al., 2021b; Palich et al., 2021b; Goupil et al., 2021; Herzog Tzarfati et al., 2021; Massarweh et al., 2021; Moor et al., 2021; Pimpinelli et al., 2021; Rabinowich et al., 2021; Yanay et al., 2021) had lower IgG antibody levels than their healthy counterparts (SMD = −2.01, 95% CI [−2.68, −1.34]) (Table 3).
3.7.2 Vaccination type
In the 12 studies (Grupper et al., 2021a; Grupper et al., 2021b; Palich et al., 2021b; Goupil et al., 2021; Herzog Tzarfati et al., 2021; Korth et al., 2021; Massarweh et al., 2021; Pimpinelli et al., 2021; Rabinowich et al., 2021; Speer et al., 2021; Yanay et al., 2021) that used the BNT162b2 vaccine alone, IgG antibody levels were lower in vulnerable than in healthy individuals (SMD = −2.30, 95% CI [−2.99, −1.62]). In the two studies (Geisen et al., 2021; Moor et al., 2021) in which subjects, including 122 vulnerable and 71 healthy individuals, received either the BNT162b2 or the Moderna mRNA-1273 vaccine, IgG antibody levels were also lower in vulnerable than healthy individuals (SMD = −0.77, 95% CI [−1.18, −0.36]) (Table 3).
3.7.3 Vaccine doses
In five studies (Palich et al., 2021b; Goupil et al., 2021; Pimpinelli et al., 2021; Speer et al., 2021), including 249 vulnerable and 163 healthy subjects, in which only one vaccine dose was administered, the vulnerable subjects had weaker IgG antibody levels than the healthy individuals (SMD = −1.66, 95% CI [−2.79, −0.53]). In 12 studies (Grupper et al., 2021a; Grupper et al., 2021b; Geisen et al., 2021; Korth et al., 2021; Massarweh et al., 2021; Moor et al., 2021; Pimpinelli et al., 2021; Rabinowich et al., 2021; Speer et al., 2021; Tzioufas et al., 2021; Yanay et al., 2021) in which two vaccine doses were administered, IgG antibody levels were also lower in the vulnerable than in the healthy population (SMD = −2.22, 95% CI [−2.89, −1.56]) (Table 3).
3.7.4 Interval time between vaccine doses
Of the studies in which two vaccine doses were administered, four studies (Grupper et al., 2021a; Grupper et al., 2021b; Pimpinelli et al., 2021), including 284 vulnerable and 192 healthy subjects, had an interval of ≤21 days between the two doses. IgG antibody levels were lower in the vulnerable than in the healthy subjects (SMD = −4.11, 95% CI [−5.27, −2.95]). Only three studies (Geisen et al., 2021; Korth et al., 2021; Speer et al., 2021) had an interval of >21 days between the two doses and the IgG antibody levels were lower in the vulnerable than in the healthy group (SMD = −2.04, 95% CI [−3.82, −0.26]) (Table 3).
3.7.5 Time from vaccination to COVID-19 Ab testing
IgG antibody levels were lower in the vulnerable than healthy subjects (SMD = −1.62, 95% CI [−3.00, −0.23]) included in four studies (Palich et al., 2021b; Pimpinelli et al., 2021; Speer et al., 2021) with ≤21 days between the first vaccine dose and COVID-19 Ab testing, as well as in one study (Goupil et al., 2021), including 71 vulnerable and 20 healthy subjects, with >21 days between the first vaccine and testing (SMD = −1.88, 95% CI [−2.45, −1.31]). IgG antibody levels were also lower in vulnerable than healthy subjects included in nine studies (Grupper et al., 2021a; Grupper et al., 2021b; Geisen et al., 2021; Herzog Tzarfati et al., 2021; Korth et al., 2021; Pimpinelli et al., 2021; Rabinowich et al., 2021; Speer et al., 2021) in which ≤21 days elapsed between the second vaccine dose and COVID-19 Ab testing (SMD = −2.71, 95% CI [−3.77, −1.65]). Similar results were seen in three studies (Massarweh et al., 2021; Moor et al., 2021; Yanay et al., 2021), including 358 vulnerable and 239 healthy subjects with >21 days between the second vaccine dose and Ab testing (SMD = −0.98, 95% CI [−1.16, −0.81]) (Table 3).
3.7.6 Antibody detection methods
Four studies (Geisen et al., 2021; Goupil et al., 2021; Moor et al., 2021; Speer et al., 2021), including 210 vulnerable and 137 healthy subjects, used ELISA to measure the humoral immune response. IgG antibody levels were lower in the vulnerable than in the healthy subjects (SMD = −1.14, 95% CI [−1.67, −0.61]). Ten studies (Grupper et al., 2021a; Grupper et al., 2021b; Palich et al., 2021b; Herzog Tzarfati et al., 2021; Korth et al., 2021; Massarweh et al., 2021; Pimpinelli et al., 2021; Rabinowich et al., 2021; Yanay et al., 2021), including 1,028 vulnerable and 583 healthy subjects, used LIAISON to test antibody levels and the vulnerable subjects had lower IgG antibody levels than the healthy subjects (SMD = −2.47, 95% CI [−3.25, −1.68]) (Table 3).
3.7.7 Antibody type
Nineteen experiments were performed to measure serum anti-S, anti-S1, and anti-RBD IgG antibody levels. Eleven datasets (Grupper et al., 2021a; Palich et al., 2021b; Geisen et al., 2021; Herzog Tzarfati et al., 2021; Korth et al., 2021; Massarweh et al., 2021; Moor et al., 2021; Pimpinelli et al., 2021; Rabinowich et al., 2021; Yanay et al., 2021) with anti-S antibody data (SMD = −2.01, 95% CI [−2.66, −1.37]) and three datasets (Grupper et al., 2021b; Palich et al., 2021b; Speer et al., 2021) with anti-S1 antibody data (SMD = −1.84, 95% CI [−3.53, −0.15]) had lower antibody levels in the vulnerable than in the healthy group. In one experiment (Goupil et al., 2021) measuring anti-RBD antibodies, including 71 vulnerable and 20 healthy people, IgG antibody levels were lower in the vulnerable than in the healthy group (SMD = −1.88, 95% CI [−2.45, −1.31]) (Table 3).
3.7.8 COVID-19 infection after vaccination
Vulnerable individuals who were uninfected with COVID-19 (SMD = −1.30, 95% CI [−1.91, −0.70]) (Palich et al., 2021a; Geisen et al., 2021; Massarweh et al., 2021; Pimpinelli et al., 2021; Rabinowich et al., 2021; Tzioufas et al., 2021), had infection after vaccination (SMD = −1.84, 95% CI [−3.09–0.60]) (Grupper et al., 2021b; Goupil et al., 2021; Yanay et al., 2021), or were uncertain about infection (SMD = −2.99, 95% CI [−5.16, −0.82]) (Grupper et al., 2021a; Korth et al., 2021; Moor et al., 2021), had lower IgG antibody levels than healthy individuals.
4 Discussion
Vulnerable populations are often more prone to severe symptoms or adverse outcomes of COVID-19 infection and have lower seroconversion rates after COVID-19 vaccination than healthy populations due to a depressed immune response. Thus, more attention is needed to prevent COVID-19 prevention in these populations using tailored vaccination regimens. This study conducted a meta-analysis of virus-specific antibody measurements and the proportion of vulnerable and healthy individuals with serum SARS-CoV-2 antibodies following COVID-19 vaccination was assessed to evaluate the effectiveness and safety of the vaccine in at-risk populations. The findings indicated that vulnerable individuals had lower virus-specific antibody levels after vaccination than healthy individuals. However, both groups had a similar incidence of adverse events, and antibody-positive subjects in either group were less likely to develop severe COVID-19 infection. Thus, while vulnerable populations were less likely to seroconvert after COVID-19 vaccination, the vaccine remained effective and safe and there was no association between vaccination and the development of adverse reactions in at-risk individuals.
IgG appears earlier and is more sensitive and easily detected than IgM after COVID-19 infection. As a result, most studies evaluate seroconversion by detecting IgG positivity (Lee et al., 2020). However, one study (Chen et al., 2021) found that the most accurate test for determining whether a subject is COVID-19 positive is assessing IgM or IgG positivity. Based on all serological results, measuring IgA antibody production and positive detection rates were superior to those of the other tests. Yu et al. (Yu et al., 2020) found that IgA positivity has high sensitivity but poor specificity and can only be used as a reference for positive patients, indicating the need for additional evaluation. Lee et al. (Chen et al., 2021) suggested that the most effective vaccine regimen for vulnerable populations should be developed by combining IgG, IgM, and IgA positivity rates.
To identify the potential factors that influence IgG antibody production, subgroup analyses were performed. All vulnerable populations had lower IgG antibody production than healthy populations after COVID-19 vaccination, with solid cancer patients having the highest IgG antibody levels of all vulnerable populations, hematological cancer patients having the lowest, and hemodialysis patients having higher IgG antibody levels than hematological cancer patients. Low antibody production in hematological cancer patients may be explained by the strong suppression of humoral and cellular immune capacity caused by both the treatment and underlying disease (Espi et al., 2022). The use of monoclonal antibodies in hematological cancer patients and hemodialysis patients could be used to supplement the relatively low seroconversion levels observed after vaccination.
In subjects who received BNT162b2 alone or multiple vaccine types (Pfizer-BioNTech BNT162b2 and Moderna mRNA-1273), IgG antibody levels were weaker in the vulnerable population. There was moderate variation in antibody production, reflecting the different responses elicited by a combination vaccine versus a single mRNA vaccine in vulnerable patients. However, the measurement of a single seroconversion rate is not sufficient to indicate that the combined vaccine is superior to a single vaccine. More data are required to recommend the use of a combined vaccine to improve seroconversion in vulnerable populations. After either one or two COVID-19 vaccine doses, the vulnerable population had lower IgG antibody levels than the healthy population. This pattern of poorer vaccination outcomes in vulnerable populations is also seen following other types of vaccine, likely because of the inferior immune capacity of these patients (Osmanodja et al., 2022). However, some studies (Lisboa Bastos et al., 2020; Gounant et al., 2022) indicate that more vaccine doses can increase antibody production in cancer patients and should be considered to achieve seroconversion levels comparable to healthy individuals. IgG antibody levels in vulnerable populations were more varied when the interval between COVID-19 vaccine doses was ≤21 days and were elevated when the interval was >21 days. This may be because the immune system is able to produce more antibodies in response to vaccination at intervals >21 days. More data are needed to determine the optimal interval between vaccine doses in vulnerable populations.
Post-vaccination blood testing using either an ELISA or LIAISON showed that the vulnerable population had lower IgG antibody levels than the healthy population. IgG antibody levels were elevated when serological testing was performed ≥21 days after both doses. This may be due to a delayed immune response to the vaccine in vulnerable populations, with increased antibody production occurring after 21 days. Thus, testing for antibodies 21 days after vaccination may be considered for future testing of vulnerable populations. An ELISA was able to detect higher antibody levels than LIAISON. This may be due to the greater sensitivity of ELISA for anti-RBD antibodies (Lisboa Bastos et al., 2020) and suggests that ELISA testing for anti-RBD antibodies should be considered for future testing of vulnerable populations. Vulnerable populations had lower levels of anti-S, anti-S1, and anti-RBD IgG antibodies than healthy populations. Anti-RBD antibodies cause greater virus neutralization than anti-spike-in antibodies, however, more studies are needed to assess the effectiveness of COVID-19 vaccination in vulnerable populations by detecting anti-RBD antibodies.
Due to the high infectivity of SARS-CoV-2, subjects may become infected after receiving the COVID-19 vaccine. Studies indicate that post-vaccination infection can lead to higher serum IgG antibody levels. Individuals who become infected from the vaccine may artificially lead to high IgG antibody levels. The current study found that IgG antibody levels in vulnerable patients were lower than in healthy subjects after vaccination, regardless of the presence of disease. While IgG antibody levels were elevated in a subgroup of subjects with COVID-19 infection after vaccination, they remained low compared to the healthy population. These findings suggested that COVID-19 infection after vaccination has a limited impact on the evaluation of vaccine efficacy.
Serum antibody levels are lower after COVID-19 vaccination than after infection with the virus (Rastawicki and Płaza, 2021). This suggests that antibody production may not be sufficient to completely protect healthy people from COVID-19 and indicates why the low serum antibody levels in vulnerable individuals often fail to protect them from COVID-19. These findings suggest that vulnerable populations must maintain a level of physical protection against SARS-CoV-2 after vaccination. However, vaccination can reduce the rate of COVID-19 infection and effectively lower the occurrence of severe respiratory symptoms in infected individuals. While vaccination is not likely to prevent COVID-19 infection in vulnerable individuals, it can prevent the occurrence of acute and severe respiratory diseases, thereby reducing mortality.
In this study, the seroconversion rate was lower in the vulnerable than in the healthy population, regardless of whether the subject was infected with COVID-19 after vaccination, a finding consistent with other studies. A meta-analysis (Del Bello et al., 2022) found that while patients with organ transplants only had a 34% seroconversion rate after the second COVID-19 vaccine dose, patients receiving a third dose had a seroconversion rate of 66%. However, those who did not seroconvert after the second dose continued to have a negative seroconversion rate after the third dose. Studies suggest improving vaccination regimens for organ transplant patients using monoclonal antibodies to supplement or replace those produced by vaccination. Other studies (Piechotta et al., 2022) have shown that the post-vaccination humoral response is lower in patients with hematological cancers than in healthy individuals, with chronic lymphocytic leukemia patients having the lowest response. Patients with impaired B lymphocytes have poor immunogenicity even after a third booster dose. Thus, depressed seroconversion rates after COVID-19 vaccination are unavoidable in vulnerable populations and a third booster dose can have a mitigating though somewhat limited impact on antibody production. For patients who continue to have depressed seroconversion rates after a third booster dose, monoclonal antibodies are recommended as a supplement. The use of monoclonal antibodies instead of a third booster dose could also be considered, however, the efficacy of this regimen requires further study.
This study has some limitations. First, no studies have been conducted to date on subjects vaccinated against each SARS-CoV-2 subtype. Since the virus has a very quick mutation rate and each subtype has a different level of virulence and infectivity, protection of the COVID-19 vaccine against vulnerable populations may differ. Second, most subjects included in this meta-analysis received the BNT162b2 COVID-19 vaccine and, to a lesser extent, the Moderna mRNA-1273 vaccine. Current data on vulnerable populations vaccinated with AD26.COV2.S, AZD-1222/ChAdOx1 nCoV-19, or other approved vaccines that met the inclusion criteria for this meta-analysis are scarce. Multiple future trials are required to further assess the efficacy and safety of these vaccines in vulnerable populations. Third, most of the studies included in this meta-analysis focused on the serum antibody levels of the subjects after vaccination and paid comparatively little attention to the cellular immune responses. To obtain more accurate results, further study of the post-vaccination cellular immune response in vulnerable populations is needed.
5 Conclusion
This meta-analysis found that post-COVID-19 vaccination seroconversion rates were generally poorer in vulnerable than in healthy populations, but there was no difference in adverse events. Patients with hematological cancers had the lowest IgG antibody levels of all vulnerable populations, and so more focus on these patients is recommended. Subjects who received the combined vaccine had higher antibody levels than those who received the single vaccine. Additional studies are needed to inform the development of more tailored vaccination protocols for vulnerable populations.
Data availability statement
The original contributions presented in the study are included in the article/Supplementary Material, further inquiries can be directed to the corresponding authors.
Author contributions
S-JC and CZ conceptualized and coordinated the study. CZ developed the search strategy. H-JL, Y-YY, and C-YH screened citations and assessed studies for eligibility. H-JL and Y-YY extracted data. F-QY and T-TM performed quality assessments. Q-CY, CZ, and H-JL performed statistical analyses. CZ and S-JC provided methodologic expertise in knowledge synthesis and resolved disagreements regarding study eligibility or quality assessments. CZ and S-JC critically reviewed the manuscript for important intellectual content. CZ, C-Y-Y, and Q-CY participated in the revision of the manuscript. All of the authors gave final approval of the version to be published and agreed to be accountable for all aspects of the work.
Funding
This study was supported by the Innovation training program of Hubei University of Medicine (No. X202110929022).
Conflict of interest
The authors declare that the research was conducted in the absence of any commercial or financial relationships that could be construed as a potential conflict of interest.
Publisher’s note
All claims expressed in this article are solely those of the authors and do not necessarily represent those of their affiliated organizations, or those of the publisher, the editors and the reviewers. Any product that may be evaluated in this article, or claim that may be made by its manufacturer, is not guaranteed or endorsed by the publisher.
Supplementary material
The Supplementary Material for this article can be found online at: https://www.frontiersin.org/articles/10.3389/fphar.2023.1144824/full#supplementary-material
References
Agrati, C., Di Cosimo, S., Fenoglio, D., Apolone, G., Ciceri, F., Ciliberto, G., et al. (2021). COVID-19 vaccination in fragile patients: Current evidence and an harmonized transdisease trial. Front. Immunol. 12, 704110. doi:10.3389/fimmu.2021.704110
Chan, J. F., Yuan, S., Kok, K. H., To, K. K., Chu, H., Yang, J., et al. (2020). A familial cluster of pneumonia associated with the 2019 novel coronavirus indicating person-to-person transmission: A study of a family cluster. Lancet (London, Engl. 395, 514–523. doi:10.1016/S0140-6736(20)30154-9
Chen, M., Qin, R., Jiang, M., Yang, Z., Wen, W., and Li, J. (2021). Clinical applications of detecting IgG, IgM or IgA antibody for the diagnosis of COVID-19: A meta-analysis and systematic review. Int. J. Infect. Dis. IJID 104, 415–422. doi:10.1016/j.ijid.2021.01.016
Del Bello, A., Abravanel, F., Marion, O., Couat, C., Esposito, L., Lavayssière, L., et al. (2022). Efficiency of a boost with a third dose of anti-SARS-CoV-2 messenger RNA-based vaccines in solid organ transplant recipients. Am. J. Transplant. 22, 322–323. doi:10.1111/ajt.16775
Espi, M., Charmetant, X., Barba, T., Mathieu, C., Pelletier, C., Koppe, L., et al. (2022). A prospective observational study for justification, safety, and efficacy of a third dose of mRNA vaccine in patients receiving maintenance hemodialysis. Kidney Int. 101, 390–402. doi:10.1016/j.kint.2021.10.040
Geisen, U. M., Berner, D. K., Tran, F., Sümbül, M., Vullriede, L., Ciripoi, M., et al. (2021). Immunogenicity and safety of anti-SARS-CoV-2 mRNA vaccines in patients with chronic inflammatory conditions and immunosuppressive therapy in a monocentric cohort. Ann. rheumatic Dis. 80, 1306–1311. doi:10.1136/annrheumdis-2021-220272
Gounant, V., Ferré, V. M., Soussi, G., Charpentier, C., Flament, H., Fidouh, N., et al. (2022). Efficacy of severe acute respiratory syndrome coronavirus-2 vaccine in patients with thoracic cancer: A prospective study supporting a third dose in patients with minimal serologic response after two vaccine doses. J. Thorac. Oncol. 17, 239–251. doi:10.1016/j.jtho.2021.10.015
Goupil, R., Benlarbi, M., Beaubien-Souligny, W., Nadeau-Fredette, A. C., Chatterjee, D., Goyette, G., et al. (2021). Short-term antibody response after 1 dose of BNT162b2 vaccine in patients receiving hemodialysis. CMAJ Can. Med. Assoc. J. 193, E793–e800. doi:10.1503/cmaj.210673
Grassly, N. C., Kang, G., and Kampmann, B. (2015). Biological challenges to effective vaccines in the developing world. Philosophical Trans. R. Soc. Lond. Ser. B, Biol. Sci. 370, 20140138. doi:10.1098/rstb.2014.0138
Grupper, A., Rabinowich, L., Schwartz, D., Schwartz, I. F., Ben-Yehoyada, M., Shashar, M., et al. (2021a). Reduced humoral response to mRNA SARS-CoV-2 BNT162b2 vaccine in kidney transplant recipients without prior exposure to the virus. Am. J. Transplant. 21, 2719–2726. doi:10.1111/ajt.16615
Grupper, A., Sharon, N., Finn, T., Cohen, R., Israel, M., Agbaria, A., et al. (2021b). Humoral response to the pfizer BNT162b2 vaccine in patients undergoing maintenance hemodialysis. Clin. J. Am. Soc. Nephrol. CJASN 16, 1037–1042. doi:10.2215/CJN.03500321
Herzog Tzarfati, K., Gutwein, O., Apel, A., Rahimi-Levene, N., Sadovnik, M., Harel, L., et al. (2021). BNT162b2 COVID-19 vaccine is significantly less effective in patients with hematologic malignancies. Am. J. Hematol. 96, 1195–1203. doi:10.1002/ajh.26284
Huang, C., Wang, Y., Li, X., Ren, L., Zhao, J., Hu, Y., et al. (2020). Clinical features of patients infected with 2019 novel coronavirus in Wuhan, China. Lancet (London, Engl. 395, 497–506. doi:10.1016/S0140-6736(20)30183-5
Koff, W. C., Schenkelberg, T., Williams, T., Baric, R. S., McDermott, A., Cameron, C. M., et al. (2021). Development and deployment of COVID-19 vaccines for those most vulnerable. Sci. Transl. Med. 13, eabd1525. doi:10.1126/scitranslmed.abd1525
Koff, W. C., and Williams, M. A. (2020). Covid-19 and immunity in aging populations - a new research agenda. N. Engl. J. Med. 383, 804–805. doi:10.1056/NEJMp2006761
Korth, J., Jahn, M., Dorsch, O., Anastasiou, O. E., Sorge-Hädicke, B., Eisenberger, U., et al. (2021). Impaired humoral response in renal transplant recipients to SARS-CoV-2 vaccination with BNT162b2 (Pfizer-BioNTech). Viruses 13, 756. doi:10.3390/v13050756
Lee, Y. L., Liao, C. H., Liu, P. Y., Cheng, C. Y., Chung, M. Y., Liu, C. E., et al. (2020). Dynamics of anti-SARS-Cov-2 IgM and IgG antibodies among COVID-19 patients. J. Infect. 81, e55–e58. doi:10.1016/j.jinf.2020.04.019
Levy, I., Wieder-Finesod, A., Litchevsky, V., Biber, A., Indenbaum, V., Olmer, L., et al. (2021). Immunogenicity and safety of the BNT162b2 mRNA COVID-19 vaccine in people living with HIV-1. Clin. Microbiol. Infect. 27, 1851–1855. doi:10.1016/j.cmi.2021.07.031
Ligumsky, H., Safadi, E., Etan, T., Vaknin, N., Waller, M., Croll, A., et al. (2022). Immunogenicity and safety of the BNT162b2 mRNA COVID-19 vaccine among actively treated cancer patients. J. Natl. Cancer Inst. 114, 203–209. doi:10.1093/jnci/djab174
Lipsitch, M., and Dean, N. E. (2020). Understanding COVID-19 vaccine efficacy. Sci. (New York, NY) 370, 763–765. doi:10.1126/science.abe5938
Lisboa Bastos, M., Tavaziva, G., Abidi, S. K., Campbell, J. R., Haraoui, L. P., Johnston, J. C., et al. (2020). Diagnostic accuracy of serological tests for Covid-19: Systematic review and meta-analysis. BMJ Clin. Res. ed) 370, m2516. doi:10.1136/bmj.m2516
Majid, S., Khan, M. S., Rashid, S., Niyaz, A., Farooq, R., Bhat, S. A., et al. (2021). COVID-19: Diagnostics, therapeutic advances, and vaccine development. Curr. Clin. Microbiol. Rep. 8, 152–166. doi:10.1007/s40588-021-00157-9
Massarweh, A., Eliakim-Raz, N., Stemmer, A., Levy-Barda, A., Yust-Katz, S., Zer, A., et al. (2021). Evaluation of seropositivity following BNT162b2 messenger RNA vaccination for SARS-CoV-2 in patients undergoing treatment for cancer. JAMA Oncol. 7, 1133–1140. doi:10.1001/jamaoncol.2021.2155
Monin, L., Laing, A. G., Muñoz-Ruiz, M., McKenzie, D. R., Del Molino Del Barrio, I., Alaguthurai, T., et al. (2021). Safety and immunogenicity of one versus two doses of the COVID-19 vaccine BNT162b2 for patients with cancer: Interim analysis of a prospective observational study. Lancet Oncol. 22, 765–778. doi:10.1016/S1470-2045(21)00213-8
Moor, M. B., Suter-Riniker, F., Horn, M. P., Aeberli, D., Amsler, J., Möller, B., et al. (2021). Humoral and cellular responses to mRNA vaccines against SARS-CoV-2 in patients with a history of CD20 B-cell-depleting therapy (RituxiVac): An investigator-initiated, single-centre, open-label study. Lancet Rheumatology 3, e789–e797. doi:10.1016/S2665-9913(21)00251-4
Osmanodja, B., Ronicke, S., Budde, K., Jens, A., Hammett, C., Koch, N., et al. (2022). Serological response to three, four and five doses of SARS-CoV-2 vaccine in kidney transplant recipients. J. Clin. Med. 11, 2565. doi:10.3390/jcm11092565
Page, M. J., Bossuyt, P. M., Boutron, I., Hoffmann, T. C., Mulrow, C. D., Shamseer, L., et al. (2021). The PRISMA 2020 statement: An updated guideline for reporting systematic reviews. BMJ 372, n71. doi:10.1136/bmj.n71
Palich, R., Veyri, M., Marot, S., Vozy, A., Gligorov, J., Maingon, P., et al. (2021a). Weak immunogenicity after a single dose of SARS-CoV-2 mRNA vaccine in treated cancer patients. Ann. Oncol. 32, 1051–1053. doi:10.1016/j.annonc.2021.04.020
Palich, R., Veyri, M., Vozy, A., Marot, S., Gligorov, J., Benderra, M. A., et al. (2021b). High seroconversion rate but low antibody titers after two injections of BNT162b2 (Pfizer-BioNTech) vaccine in patients treated with chemotherapy for solid cancers. Ann. Oncol. 32, 1294–1295. doi:10.1016/j.annonc.2021.06.018
Piechotta, V., Mellinghoff, S. C., Hirsch, C., Brinkmann, A., Iannizzi, C., Kreuzberger, N., et al. (2022). Effectiveness, immunogenicity, and safety of COVID-19 vaccines for individuals with hematological malignancies: A systematic review. Blood cancer J. 12, 86. doi:10.1038/s41408-022-00684-8
Pimpinelli, F., Marchesi, F., Piaggio, G., Giannarelli, D., Papa, E., Falcucci, P., et al. (2021). Fifth-week immunogenicity and safety of anti-SARS-CoV-2 BNT162b2 vaccine in patients with multiple myeloma and myeloproliferative malignancies on active treatment: Preliminary data from a single institution. J. Hematol. Oncol. 14, 81. doi:10.1186/s13045-021-01090-6
Rabinowich, L., Grupper, A., Baruch, R., Ben-Yehoyada, M., Halperin, T., Turner, D., et al. (2021). Low immunogenicity to SARS-CoV-2 vaccination among liver transplant recipients. J. hepatology 75, 435–438. doi:10.1016/j.jhep.2021.04.020
Rastawicki, W., and Płaza, K. (2021). The level of protective post-vaccination antibodies in NIPH-NIH employees after administration of Pfizer vaccine against COVID-19. Przeglad Epidemiol. 75, 3–13. doi:10.32394/pe.75.01
Sattler, A., Schrezenmeier, E., Weber, U. A., Potekhin, A., Bachmann, F., Straub-Hohenbleicher, H., et al. (2021). Impaired humoral and cellular immunity after SARS-CoV-2 BNT162b2 (tozinameran) prime-boost vaccination in kidney transplant recipients. J. Clin. investigation 131, e150175. doi:10.1172/JCI150175
Shem-Tov, N., Yerushalmi, R., Danylesko, I., Litachevsky, V., Levy, I., Olmer, L., et al. (2022). Immunogenicity and safety of the BNT162b2 mRNA COVID-19 vaccine in haematopoietic stem cell transplantation recipients. Br. J. Haematol. 196, 884–891. doi:10.1111/bjh.17918
Shi, J., Luo, D., Weng, H., Zeng, X. T., Lin, L., Chu, H., et al. (2020). Optimally estimating the sample standard deviation from the five-number summary. Res. synthesis methods 11, 641–654. doi:10.1002/jrsm.1429
Speer, C., Göth, D., Benning, L., Buylaert, M., Schaier, M., Grenz, J., et al. (2021). Early humoral responses of hemodialysis patients after COVID-19 vaccination with BNT162b2. Clin. J. Am. Soc. Nephrol. CJASN 16, 1073–1082. doi:10.2215/CJN.03700321
Stumpf, J., Siepmann, T., Lindner, T., Karger, C., Schwöbel, J., Anders, L., et al. (2021). Humoral and cellular immunity to SARS-CoV-2 vaccination in renal transplant versus dialysis patients: A prospective, multicenter observational study using mRNA-1273 or BNT162b2 mRNA vaccine. Lancet regional health Eur. 9, 100178. doi:10.1016/j.lanepe.2021.100178
Swai, J., Gui, M., Long, M., Wei, Z., Hu, Z., and Liu, S. (2022). Humoral and cellular immune response to severe acute respiratory syndrome coronavirus-2 vaccination in haemodialysis and kidney transplant patients. Nephrol. Carlt. Vic. 27, 7–24. doi:10.1111/nep.13974
Thuluvath, P. J., Robarts, P., and Chauhan, M. (2021). Analysis of antibody responses after COVID-19 vaccination in liver transplant recipients and those with chronic liver diseases. J. hepatology 75, 1434–1439. doi:10.1016/j.jhep.2021.08.008
Tzioufas, A. G., Bakasis, A. D., Goules, A. V., Bitzogli, K., Cinoku, I., Chatzis, L. G., et al. (2021). A prospective multicenter study assessing humoral immunogenicity and safety of the mRNA SARS-CoV-2 vaccines in Greek patients with systemic autoimmune and autoinflammatory rheumatic diseases. J. Autoimmun. 125, 102743. doi:10.1016/j.jaut.2021.102743
Veronese, N., Petrovic, M., Benetos, A., Denkinger, M., Gudmundsson, A., Knol, W., et al. (2021). Underrepresentation of older adults in clinical trials on COVID-19 vaccines: A systematic review. Ageing Res. Rev. 71, 101455. doi:10.1016/j.arr.2021.101455
Waissengrin, B., Agbarya, A., Safadi, E., Padova, H., and Wolf, I. (2021). Short-term safety of the BNT162b2 mRNA COVID-19 vaccine in patients with cancer treated with immune checkpoint inhibitors. Lancet Oncol. 22, 581–583. doi:10.1016/S1470-2045(21)00155-8
Waldhorn, I., Holland, R., Goshen-Lago, T., Shirman, Y., Szwarcwort-Cohen, M., Reiner-Benaim, A., et al. (2021). Six-month efficacy and toxicity profile of BNT162b2 vaccine in cancer patients with solid tumors. Cancer Discov. 11, 2430–2435. doi:10.1158/2159-8290.CD-21-1072
Yanay, N. B., Freiman, S., Shapira, M., Wishahi, S., Hamze, M., Elhaj, M., et al. (2021). Experience with SARS-CoV-2 BNT162b2 mRNA vaccine in dialysis patients. Kidney Int. 99, 1496–1498. doi:10.1016/j.kint.2021.04.006
Keywords: vulnerable populations, COVID-19 vaccination, haematological cancers, organ transplant, elderly, meta-analysis
Citation: Li H-J, Yang Q-C, Yao Y-Y, Huang C-Y, Yin F-Q, Xian-Yu C-Y, Zhang C and Chen S-J (2023) COVID-19 vaccination effectiveness and safety in vulnerable populations: a meta-analysis of 33 observational studies. Front. Pharmacol. 14:1144824. doi: 10.3389/fphar.2023.1144824
Received: 15 January 2023; Accepted: 15 June 2023;
Published: 23 June 2023.
Edited by:
Andrea Visentin, University of Padua, ItalyReviewed by:
Francesco Angotzi, University Hospital of Padua, ItalyAlessandro Cellini, University of Padua, Italy
Copyright © 2023 Li, Yang, Yao, Huang, Yin, Xian-Yu, Zhang and Chen. This is an open-access article distributed under the terms of the Creative Commons Attribution License (CC BY). The use, distribution or reproduction in other forums is permitted, provided the original author(s) and the copyright owner(s) are credited and that the original publication in this journal is cited, in accordance with accepted academic practice. No use, distribution or reproduction is permitted which does not comply with these terms.
*Correspondence: Chao Zhang, zhangchao0803@126.com; Shao-Juan Chen, 407220842@qq.com
†These authors have contributed equally to this work