- 1Endocrine Research Center, Tabriz University of Medical Sciences, Tabriz, Iran
- 2Student Research Committee, Tabriz University of Medical Sciences, Tabriz, Iran
- 3Students’ Scientific Research Center, Tehran University of Medical Sciences, Tehran, Iran
- 4Department of Clinical Nutrition, School of Nutritional Sciences and Dietetics, Tehran University of Medical Sciences, Tehran, Iran
- 5Nutrition Research Center, Tabriz University of Medical Sciences, Tabriz, Iran
Background: Oxidative stress is considered a major factor in the pathophysiology of non-alcoholic liver disease (NAFLD). A growing body of evidence indicates that oleoylethanolamide (OEA), a bioactive lipid mediator, has anti-inflammatory and antioxidant properties. This trial investigated the effects of OEA administration on inflammatory markers, oxidative stress and antioxidant parameters of patients with NAFLD.
Methods: The present randomized controlled trial was conducted on 60 obese patients with NAFLD. The patients were treated with OEA (250 mg/day) or placebo along with a low-calorie diet for 12 weeks. Inflammatory markers and oxidative stress and antioxidant parameters were evaluated pre-and post-intervention.
Results: At the end of the study, neither the between-group changes, nor the within-group differences were significant for serum levels of high-sensitivity C-reactive protein (hs-CRP), interleukin-1 beta (IL-1β), IL-6, IL-10, and tumor necrosis-factor α (TNF-α). Serum levels of total antioxidant capacity (TAC) and superoxide dismutase (SOD) significantly increased and serum concentrations of malondialdehyde (MDA) and oxidized-low density lipoprotein (ox-LDL) significantly decreased in the OEA group compared to placebo at study endpoint (p = 0.039, 0.018, 0.003 and 0.001, respectively). Although, no significant between-group alterations were found in glutathione peroxidase and catalase. There were significant correlations between percent of changes in serum oxidative stress and antioxidant parameters with percent of changes in some anthropometric indices in the intervention group.
Conclusion: OEA supplementation could improve some oxidative stress/antioxidant biomarkers without any significant effect on inflammation in NAFLD patients. Further clinical trials with longer follow-up periods are demanded to verify profitable effects of OEA in these patients.
Clinical Trial Registration: www.irct.ir, Iranian Registry of Clinical Trials IRCT20090609002017N32.
Introduction
Non-alcoholic fatty liver disease (NAFLD) is recognized as the most frequent chronic liver disease globally (Abd El-Kader and El-Den Ashmawy, 2015). Various estimates of NAFLD prevalence have been reported in the general population (Estes et al., 2020). In 2030, the prevalence of NAFLD is estimated at 33.5% among the adult population and 28.4% among all ages (Estes et al., 2018). The disease spectrum ranges from simple steatosis to non-alcoholic steatohepatitis (NASH), characterized by hepatocyte ballooning and/or lobular inflammation, which may lead to advanced fibrosis, cirrhosis and fatal liver failure (Benedict and Zhang, 2017). Patients with NAFLD, especially those with NASH, often have one or more components of the metabolic syndrome: obesity, dyslipidemia, hypertension, and elevated fasting plasma glucose concentrations or overt type 2 diabetes mellitus (T2DM). Recently, it has been proposed that to rename NAFLD as metabolic dysfunction-associated fatty liver disease (MAFLD) (Tutunchi et al., 2020a; Singh et al., 2021).
Oxidative stress is regarded as the leading cause of various liver diseases (Rolo et al., 2012; Li et al., 2015a). According to the multiple-hit hypothesis, the widely accepted theory in NAFLD pathogenesis, oxidative stress is one of the major mechanisms responsible for the development of liver disorders by stimulating Kupffer cells, hepatic stellate cells, and hepatocytes (Takaki et al., 2013; Ma et al., 2021; Wang et al., 2021). Moreover, accumulating evidence indicates that inflammatory pathways play pivotal roles in the pathogeneses of NAFLD, and continuous inflammation (e.g., pro-inflammatory markers and recruitment of inflammatory cells) stimulates the progression of NASH (Stojsavljević et al., 2014; Kwon et al., 2016). Evidence demonstrates that the severity of NAFLD may be related to increased serum concentrations of inflammatory mediators as well as decreased levels of anti-inflammatory cytokines (Braunersreuther et al., 2012; Paredes-Turrubiarte et al., 2016).
To date, there is no Food and Drug Administration (FDA)-approved pharmacological treatment for NAFLD. Adherence to a calorie-restricted diet and regular physical activity are the backbone of therapy for patients with NAFLD. However, these goals are difficult to implement due to poor adherence. Therefore, adjuvant therapy is proposed as a potential treatment strategy in these patients (Mahjoubin-Tehran et al., 2021). As peroxisome proliferator-activated receptor (PPAR) agonists can improve metabolic dysfunctions, inflammation, and oxidative stress related to liver dysfunctions, agonists of PPARs have gained much attention from the NAFLD and NASH research community (Gross et al., 2017; Fougerat et al., 2020). Oleoylethanolamide (OEA), a potent endogenous PPAR-α agonist, is a bioactive lipid mediator that belongs to the family of endogenous acylethanolamides. OEA acts via PPAR-α and has been proposed to exert multiple biological activities such as anti-obesity, anti-inflammatory, and antioxidant effects (Tutunchi et al., 2019; Akbari et al., 2022). OEA administration has been demonstrated to reduce food intake (Fu et al., 2003; Oveisi et al., 2004) and increase across-meal satiety (Fu et al., 2008) through gut-brain communication. Furthermore, OEA induces fatty acid uptake and lipolysis in the adipose tissue and liver and reduces body weight gain in mice and rats (Guzmán et al., 2004; Tutunchi et al., 2019).
The therapeutic effects of OEA in the modulation of various liver disorders have also been reported in several studies. For instance, it has been demonstrated that OEA can decrease lipid synthesis and lipoprotein secretion in the liver (Pan et al., 2018) and improve liver steatosis in rats (Li et al., 2015b) and humans (Tutunchi et al., 2020b; Tutunchi et al., 2020c; Tutunchi et al., 2021). OEA attenuated liver fibrosis through a PPAR-α dependent mechanism (Chen et al., 2015). Misto et al. (2019) have found that OEA enhances fasting-induced liver ketogenesis by activating PPAR-α. Moreover, treatment with OEA significantly alleviated acute liver injury in mice by decreasing plasma alanine aminotransferase (ALT) and aspartate aminotransferase (AST) concentrations, and reducing the histopathological changes. Furthermore, administration of OEA attenuated hepatic apoptosis, inhibited the expression of oxidative stress biomarkers, and elevated the activity of antioxidant enzymes. On the other hand, OEA significantly prevented the expression of pro-inflammatory mediators and suppressed NLRP3 inflammasome in mice (Hu et al., 2020). Therefore, due to the profitable effects of OEA on liver diseases on the one hand and the limitation of clinical trials on antioxidant and anti-inflammatory effects of OEA in NAFLD patients on the other hand, the present trial, for the fist time, assessed the effects of OEA on inflammatory markers, oxidative stress and antioxidant parameters of patients with NAFLD on a calorie-restricted diet.
Methods
Trial design and subjects
The methods have been published previously (Tutunchi et al., 2020c; Tutunchi et al., 2021). The present investigation was a randomized, triple-blind, parallel-controlled trial conducted between 2019-February and 2019-July. The design was approved by the ethics committee of Tabriz University of Medical Sciences (IR.TBZMED.REC.1401.425). This trial was registered in the Iranian Registry of Clinical Trials (IRCT20090609002017N32). All of the participants provided written informed consent.
By posting advertisements, subjects willing to participate were recruited from hospitals and clinics in the north-west of Iran. The participants of the current study were obese men and women with NAFLD, who met the following inclusion criteria: age of 20–50 years, body mass index (BMI) of 30–40 kg/m2, with a diagnosis of NAFLD (grade I or II) based on ultrasonographic findings.
To assess the severity of fatty liver, ultrasonographic scoring system developed by Hamaguchi et al (Hamaguchi et al., 2007) was applied. Hepatorenal echo contrast, bright liver, deep attenuation, and vessel blurring were applied to classify steatosis into three grades including Mild (grade I), Moderate (grade II) and Severe (grade III). As the most severe form of NAFLD patients (grade III) took specific medications for live damage, they were not eligible to enroll to the study (according to defined inclusion criteria) as well as ethical problems.
Exclusion criteria included the use of weight-lowering diets or agents in the last 6 months, pregnancy, lactation, menopause, smoking, being professional athlete, addiction to alcohol (>10 g/d for women and >20 g/d for men), any evidence of chronic infections, diabetes, or autoimmune diseases, high blood pressure, kidney or thyroid dysfunction, polycystic ovary syndrome, cardiovascular, gastrointestinal, cancer, or hepatocellular diseases, the use of anti-diabetic, antioxidant, or multivitamin-mineral supplements in the last 3 months, the use of any medication or dietary supplements that affect glucose and lipids metabolism during the last 3 months or attending another clinical trial. In addition, the individual characteristics and medical history of each patient were collected.
Sample size
The sample size was determined according to mean and standard deviation (SD) of TNF-α on the basis of previous trial by Payahoo et al. (Payahoo et al., 2018). We calculated that a sample size of 30 subjects was required for each group of the trial considering a power of 80% and 95% significance level and an drop-out rate of 20%.
Randomization and intervention
Using the block randomization method in blocks stratified by sex and grading of NAFLD, the participants were randomly allocated to either the intervention group (n = 30) or the control group (n = 30) for 12 weeks. Until the end of the analysis, patients and researchers as well as statistical consultant were blind to the group assignments of the trial. Synthesis of OEA was conducted at Nutrition Research Center of Tabriz University of Medical Sciences and has been described in detail in previous clinical trial (Laleh et al., 2018; Tutunchi et al., 2020c). The daily OEA dose received by each patient was considered at 250 mg based on the previous study on obese patients (Laleh et al., 2018). The intervention group was treated with OEA (125 mg), twice a day, half an hour before lunch and dinner meal, and the placebo group received two capsules of 125 mg starch for 12 weeks. The capsules of both groups (in the same shape, size, and color) were delivered to patients monthly.
Individual calorie-restricted diet (−500 kcal of total energy expenditure) was designed for participants of each group. Macronutrient distribution range for fat, protein, and carbohydrates was respectively set at 30%, 15%, and 55% of daily total energy intake.
To increase compliance, all patients received brief daily cell phone reminders to take the supplements and emphasis physical activity and weight-loss dietary plans given to them. Besides, every 2 weeks, participants were returned to clinic to assess diet based on data obtained from 3- day food records. Participants were also directly asked for adverse events. Assessment of supplement adherence was conducted using unused capsule counts of each patient (patients consuming >90% of the capsules delivered during the study were considered as high adherence to the study intervention). Data on calorie intake, obtained from the 3-day food record, was applied for assessing adherence to the dietary plans given to the participants.
Dietary intake and physical activity assessment
Dietary intake information was collected based on 3-day food record (two non-consecutive days and one weekend day per individual) and analyzed by the Nutritionist IV software (First Databank, San Bruno, CA, United States) at the onset and end of the study. International Physical Activity Questionnaire-Short Form (IPAQ-SF) was also applied to evaluate physical activity, based on metabolic equivalent task minutes per week (MET, min/week) (Craig et al., 2003).
Anthropometric and biochemical measurements
Fasting body weight (kilograms) and height were assessed on a calibrated Seca balance beam scale with height rod while patients were barefoot and wearing light clothe. Other anthropometric indices, including waist circumference (WC) and hip circumference (HC) were evaluated using the standard methods. BMI, waist-to-hip ratio (WHR) and waist-to-height ratio (WHtR) were also computed.
All the biochemical measurements were performed at pre-and post-intervention. A 5 ml venous blood sample was collected from each patient after a 12-h overnight fasting and frozen at −80°C immediately after collection. Serum concentration of high-sensitivity C-reactive protein (hs-CRP) was assessed using the immunoturbidimetry method (Pars Azmoon Co., Tehran, Iran). Serum levels of interleukin-1 beta (IL-1β), IL-6, IL-10, tumor necrosis-factor α (TNF-α) were measured by commercial enzyme-linked immunosorbent assay (ELISA) kits (Bioassay Technology Laboratory, Shanghai City, China). Total antioxidant capacity (TAC) was assessed through the colorimetric method by Randox total antioxidant status kit (Randox Laboratories, United Kingdom). The levels of glutathione peroxidase (GSH-Px) and superoxide dismutase (SOD) were evaluated by spectrophotometric method using Ransel and Ransod kits, respectively (Randox Laboratories, United Kingdom). The spectrophotometric thiobarbituric acid reactive substances (TBARS) method was used to determine serum level of malondialdehyde (MDA). The Aebi’s method was used for measuring catalase activity. Serum oxidized-low density lipoprotein (ox-LDL) was detected by ELISA (Mercodia AB, Uppsala, Sweden), according to manufacturers’ specifications. Serum concentrations of total cholesterol (TC), high-density lipoprotein cholesterol (HDL-C), low-density lipoprotein cholesterol (LDL-C) and triglyceride were determined by commercial kits (Pars-Azmoon Co., Tehran, Iran).
Statistical analysis
An intention-to-treat approach was used in all analyses. The Shapiro-Wilk test and histograms were applied to check the normality of variables. Continuous variables with normal distribution were reported as mean (SD) and non-normal variables were presented as median (25th and 75th percentiles). The qualitative variables were represented with frequency (percentage). Comparison of baseline characteristics of patients between study groups was performed using the independent samples t-test or Mann–Whitney U test. To test within-group differences, Paired samples t-test or Wilcoxon signed-rank test were applied. Fisher’s exact test run for analyzing between-group changes of qualitative data. To compare the two groups at the end of the trial adjusting for baseline values and potential confounders (i.e., age, alterations in physical activity, energy intake, and BMI), analysis of covariance (ANCOVA) was run. The correlation of serum oxidative stress and antioxidant parameters with anthropometric indices and metabolic parameters was analyzed using Pearson’s correlation coefficient test. Two-tailed p-value <0.05 was regarded to be statistically significant. All statistical analyses were done using SPSS, version 23.0 (SPSS Inc., Chicago, IL, United States).
Results
The CONSORT flowchart of the trial is exhibited in Figure 1. A total of 58 participants completed the trial; one patient in each arm was lost to follow-up due to non-adherence to dietary recommendations. No side effects were reported by those who ended the trial. There were no significant alterations between the study groups in the baseline characteristics of patients including demographic characteristics, physical activity levels and the severity of fatty liver (Table 1).
At baseline, there were no significant differences between the two groups, for anthropometric indices, dietary intakes and physical activity (Table 2). Physical activity of the participants did not change significantly throughout the trial (p = 0.206; data not shown).
As presented in Table 3, there were no significant differences in inflammatory biomarkers as well as oxidative stress/antioxidant parameters between study groups at baseline. In terms of inflammatory markers, hs-CRP decreased significantly in both study groups, no significant between-group alteration was observed for this parameter at the end of the study, though. Neither the between-group changes, nor the within-group differences reached statistical significance for other markers of inflammation including IL-1β, IL-6, IL-10, and TNF-α. Serum levels of TAC and SOD significantly increased in both groups. Greater increase in TAC and SOD levels was found in the OEA group compared to the control at endpoint of the study (p = 0.039 and 0.018, respectively) after adjusting for potential confounders including baseline values, age, changes in physical activity, energy intake, and BMI. Moreover, serum MDA and ox-LDL concentrations decreased in the OEA group compared to the control at study endpoint (p = 0.003 and 0.001, respectively). However, between-group alterations adjusted for above-mentioned confounding variables revealed no meaningful differences between the study groups in terms of GSH-Px and catalase.
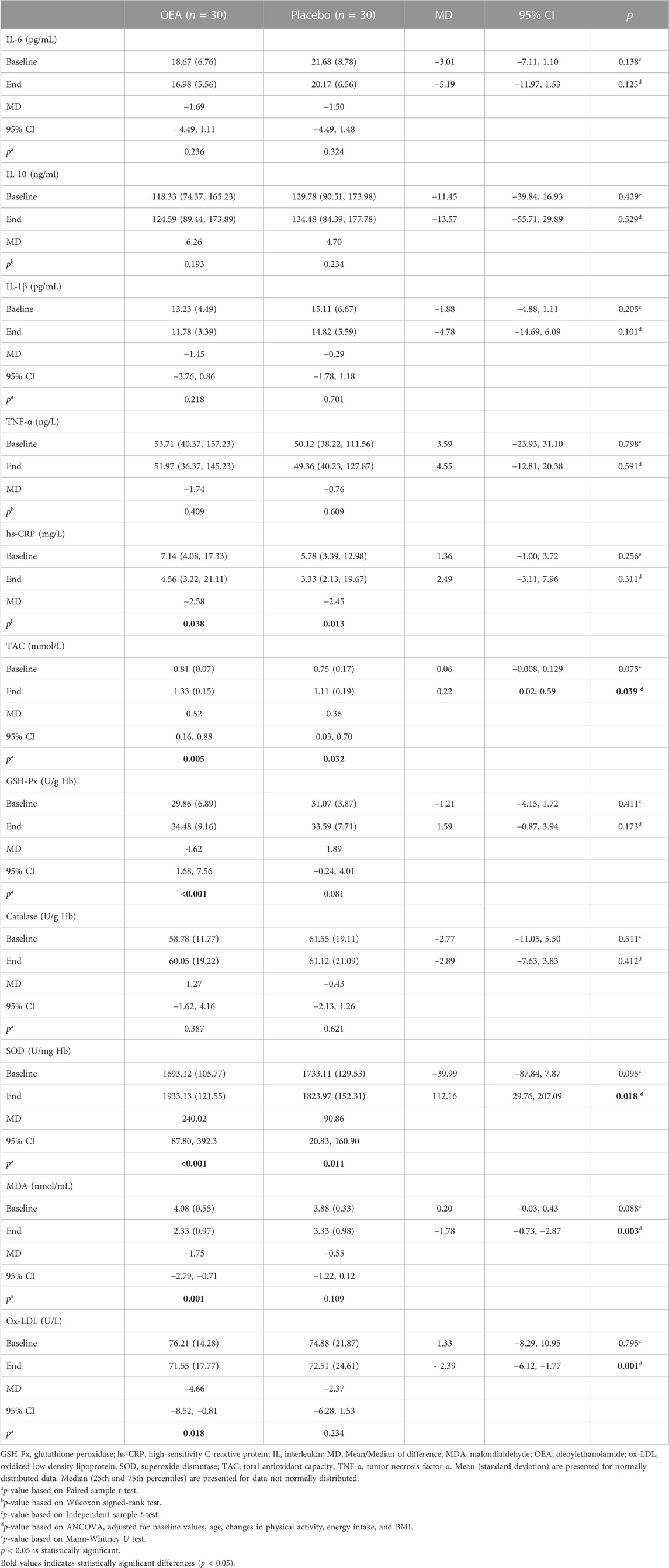
TABLE 3. Inflammatory biomarkers and oxidative stress/antioxidant parameters of the patients throughout study.
Correlation coefficients of serum oxidative stress and antioxidant parameters with anthropometric indices and metabolic parameters are presented in Table 4. A significant inverse relationship was detected between percent of changes in TAC with percentage of changes in BMI (p = 0.016) and WHR (p = 0.027) as well as an inverse relationship between percent of changes in serum SOD level with percent of changes in BMI (p = 0.018) and WC (p = 0.001) in the intervention group. However, we found a positive correlation between percent of changes in MDA with percent of changes in BMI (p = 0.022) and WHR (p = 0.041) as well as a positive relationship between percent of changes in serum ox-LDL level with percent of changes in BMI (p = 0.012) and WC (p = 0.001) in the intervention group. No meaningful relationships were detected between serum oxidative stress and antioxidant parameters with other anthropometric indices as well as metabolic parameters in the study groups.
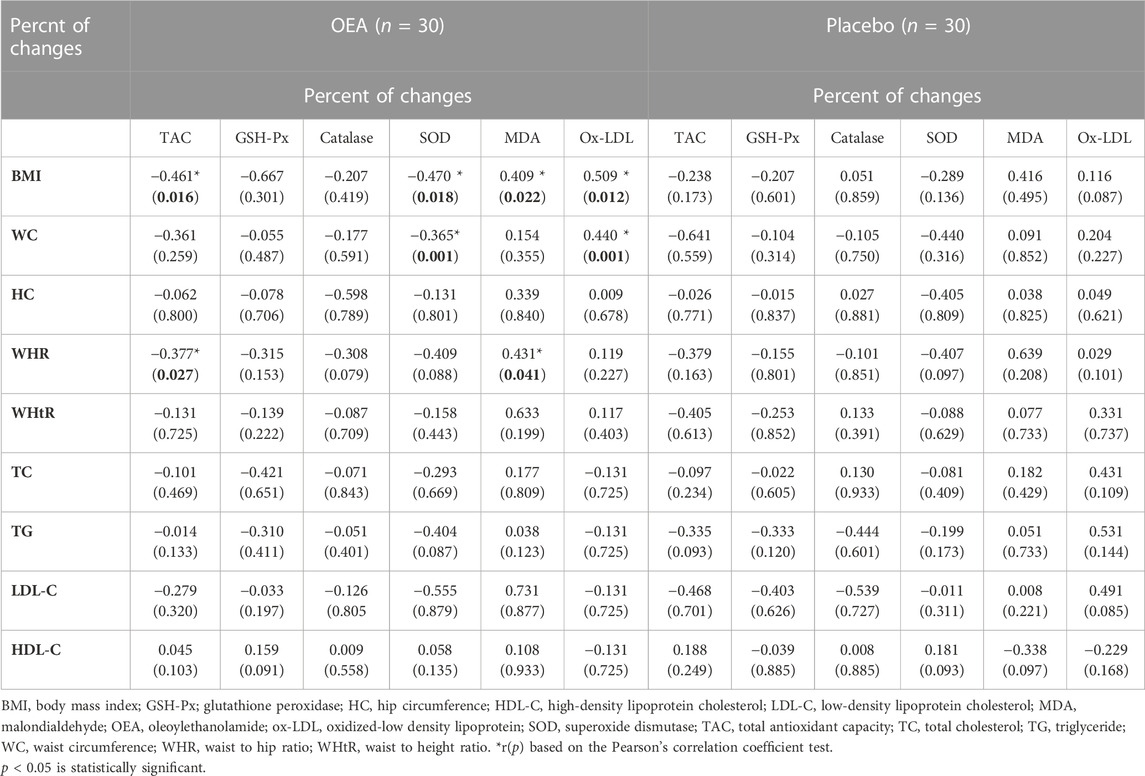
TABLE 4. Correlation coefficients among serum oxidative stress and antioxidant parameters and anthropometric indices and metabolic parameters.
Discussion
Little has been investigated about the effects of OEA on inflammation and oxidative stress, main pathological factors contributing to the initiation and progression of various liver diseases, in patients with NAFLD. To our knowledge, the present clinical trial is the first study to assess the effects of OEA supplementation on inflammatory indices, oxidative stress and antioxidant parameters in patients with NAFLD. Based on the obtained results, after adjusting for possible confounders, OEA supplementation caused a remarkable increase in TAC and SOD levels in obese patients with NAFLD on a calorie-restricted diet. However, treatment with OEA did not have a significant effect on inflammatory biomarkers including hs-CRP, IL-1β, IL-6, IL-10, and TNF-α. Furthermore, a significant decrease in serum MDA and ox-LDL concentrations were found by OEA supplementation. We also found significant correlations between percentage of changes in serum oxidative stress and antioxidant parameters with percentage of changes in some anthropometric indices in the intervention group.
In contrast with aforementioned results, Payahoo et al (Payahoo et al., 2018) concluded that OEA supplementation (250 mg for 8 weeks) could significantly reduce serum concentrations of IL-6 and TNF-α in healthy obese people. Moreover, a significant reduction in the expression levels of nuclear factor-kappa B (NF-kB) and IL-6 was observed in obese patients with NAFLD after OEA supplementation (Tutunchi et al., 2021). On the other hand, in the recent investigation by Sabahi et al. (2022), OEA treatment (300 mg/day) could improve the short-term inflammatory and oxidative stress status in patients with acute ischemic stroke. A significant reduction in serum levels of IL-1β, monocyte chemoattractant protein-1 (MCP-1), and TNF-α were also found by OEA supplementation (5 mg/kg) in alcohol intoxicated rats (Antón et al., 2017). In addition, an animal study conducted by Sun et al. (2007) demonstrated that administration of 10 mg/kg OEA could significantly prevent the expression of cyclooxygenase-2 (COX-2) in wild-type mice. Anti-inflammatory characteristics of OEA have been attributed to the PPAR-α activation (Yang et al., 2016). OEA as a potent endogenous PPAR-α agonist exerts anti-inflammatory effects through increasing the expression of IkB as NF-kB inhibitory protein, inhibiting lipopolysaccharide (LPS)-induced NF-kB activation, suppressing the expression of COX-2 as a NF-kB regulated protein, reducing the expression of vascular cell adhesion molecule-1 (VCAM-1) and intracellular adhesion molecule 1 (ICAM-1) as molecules involved in the inflammatory response, interfering with the extracellular-signal-regulated kinase (ERK)1/2-dependent signaling cascade, and attenuating the expression of genes coding for inflammatory biomarkers including IL-6, IL-1β, and TNF-α (Yang et al., 2016; Payahoo et al., 2018; Tutunchi et al., 2021). On the other hand, as OEA is an endogenous ligand for hypoxia-inducible factor 1 (HIF-α), it might alleviate inflammation (Diao et al., 2022). HIF-1α inhibition could be a potential strategy for limiting exacerbated inflammatory responses because it caused sustained inflammation by activating NF-kB pathway (Palazon et al., 2014). It is important to note that discrepancies between our conclusions and above-mentioned findings might be explained by different nature and design of the investigations as well as supplementation with various dosages of OEA for dissimilar periods of time. In addition, different methods and various ELISA kits to measure serum concentrations of inflammatory biomarkers may account for the apparent discordant results and conclusions. Moreover, follow-up period may not be sufficient enough to affect serum inflammatory biomarkers in the present study.
In line with our results, a recent animal study by Giudetti et al. (2021) revealed a meaningful reduction in the amounts of MDA and carbonylated proteins after OEA treatment. In the same study, OEA significantly increased the activity of antioxidant enzymes including SOD, catalase, and GSH-Px (Giudetti et al., 2021). Additionally, oral administration of 10 mg/kg/day OEA for 8 weeks attenuated oxidative stress, endothelial cell damage, and early atherosclerotic plaque formation in atherosclerotic mice (Ma et al., 2015). Moreover, in plasma samples of healthy subjects, copper-induced lipid peroxidation was reduced by OEA incubation (1 µM for 7 h) (Zolese et al., 2008). The suspected mechanisms responsible for the effects of OEA on oxidative stress and antioxidant parameters are not yet fully understood. OEA acts as a potent antioxidant through enhancing PPAR-α signaling, inhibiting LPS-induced oxidative/nitrosative stress, decreasing the expression of apoptosis-related proteins, preventing lipid peroxidation, attenuating the expression of nuclear factor erythroid 2-related factor 2 (Nrf-2) as a key transcription factor involved in inflammation and antioxidant responses, and reducing the production of reactive oxygen species (ROS) (Fan et al., 2014; Sayd et al., 2014; Payahoo et al., 2018; Giudetti et al., 2021). We found significant correlation between the percentage of changes in serum oxidative stress and antioxidant parameters and the percentage of changes in some anthropometric indices in the OEA group. In the interpretation of these findings, it should be considered that improvement in oxidative stress and antioxidant factors by OEA supplementation might be attributed to improvement in anthropometric parameters. It is documented that obesity can induce systemic oxidative stress (Manna and Jain, 2015). OEA could improve obesity by regulating energy hemostasis, modulating lipid and glucose metabolism, stimulating the expression of genes associated with fatty acid oxidation and lipolysis, and controlling appetite and food intake through induction of satiety-related genes expression (Laleh et al., 2018). Therefore, improvement in anthropometric parameters by OEA supplementation may contribute to the reduction of oxidative stress through lowering tissue lipid levels and inflammation, alleviating endothelial dysfunction and impaired mitochondrial function, decreasing hyperglycemia, and improving hyperleptinemia (Manna and Jain, 2015).
Strengths and limitations
The inclusion of patients newly diagnosed with NAFLD, perfect adherence to the intervention, using a specified weight-loss diet for all participants, frequent contact with the patients through visit sessions and telephone, robust statistical analysis and consideration of probable confounders were some of the strength points of present trial. However, there are some weaknesses to be considered for data interpretation. First, serum concentration of OEA, the most appropriate method for evaluating the adherence of participants to the intervention, was not measured due to funding restrictions. Second, self-reporting of dietary intake and physical activity may lead to the low or high reporting bias. Third, although ultrasonography is the preferred initial non-invasive and relatively low-cost test for diagnosing NAFLD, it has been shown to be less accurate in diagnosis of mild steatosis. Thus, some cases of mild steatosis might have been missed in this clinical trial. Finally, as it is documented that the biological effects of OEA are mainly mediated through PPAR-α signaling pathway, it was better to measure serum level of PPAR-α. However, funding constraints did not allow for measuring serum concentration of PPAR-α in our patients.
Conclusion
In summary, OEA supplementation (250 mg for 12 weeks) significantly improved oxidative stress and antioxidant parameters of obese patients with NAFLD on a calorie-restricted diet, while no signicant changes were found in terms of inflammatory biomarkers. Although obtained results indicate that OEA might be a promising therapy option for obese patients with NAFLD, further high-quality randomized clinical trials with longer follow-up periods are demanded to verify profitable effects of OEA in these patients.
Data availability statement
The raw data supporting the conclusion of this article will be made available by the authors, without undue reservation.
Ethics statement
All procedures performed in this study were in accordance with the ethical standards of the Ethics Committee of Tabriz University of Medical Science. In this study all subjects signed a consent form and the study protocol was approved by the ethical committee of Tabriz University of Medical Sciences (Ethics code: TBZMED. REC.1401.425). The patients/participants provided their written informed consent to participate in this study.
Author contributions
The authors' responsibilities were as follows: HT wrote the original paper; MN-S, FN, and RS help in data collection; FZ contributed in patient selection; HT did statistical analysis; FN, SN, and AO contributed to the conception of the article as well as to the final revision of the manuscript. All authors read and approved the final version of the manuscript.
Funding
This research was funded by the Endocrine Research Center of Tabriz University of Medical Sciences [Grant No.70088].
Acknowledgments
We sincerely thank the patients who participated in the present study.
Conflict of interest
The authors declare that the research was conducted in the absence of any commercial or financial relationships that could be construed as a potential conflict of interest.
Publisher’s note
All claims expressed in this article are solely those of the authors and do not necessarily represent those of their affiliated organizations, or those of the publisher, the editors and the reviewers. Any product that may be evaluated in this article, or claim that may be made by its manufacturer, is not guaranteed or endorsed by the publisher.
References
Abd El-Kader, S. M., and El-Den Ashmawy, E. M. (2015). Non-alcoholic fatty liver disease: The diagnosis and management. World J. Hepatol. 7 (6), 846–858. doi:10.4254/wjh.v7.i6.846
Akbari, N., Ostadrahimi, A., Tutunchi, H., Pourmoradian, S., Farrin, N., Najafipour, F., et al. (2022). Possible therapeutic effects of boron citrate and oleoylethanolamide supplementation in patients with COVID-19: A pilot randomized, double-blind, clinical trial. J. trace Elem. Med. Biol. organ Soc. Minerals Trace Elem. (GMS) 71, 126945. doi:10.1016/j.jtemb.2022.126945
Antón, M., Alén, F., Gómez de Heras, R., Serrano, A., Pavón, F. J., Leza, J. C., et al. (2017). Oleoylethanolamide prevents neuroimmune HMGB1/TLR4/NF-kB danger signaling in rat frontal cortex and depressive-like behavior induced by ethanol binge administration. Addict. Biol. 22 (3), 724–741. doi:10.1111/adb.12365
Benedict, M., and Zhang, X. (2017). Non-alcoholic fatty liver disease: An expanded review. World J. Hepatol. 9 (16), 715–732. doi:10.4254/wjh.v9.i16.715
Braunersreuther, V., Viviani, G. L., Mach, F., and Montecucco, F. (2012). Role of cytokines and chemokines in non-alcoholic fatty liver disease. World J. gastroenterology 18 (8), 727–735. doi:10.3748/wjg.v18.i8.727
Chen, L., Li, L., Chen, J., Li, L., Zheng, Z., Ren, J., et al. (2015). Oleoylethanolamide, an endogenous PPAR-α ligand, attenuates liver fibrosis targeting hepatic stellate cells. Oncotarget 6 (40), 42530–42540. doi:10.18632/oncotarget.6466
Craig, C. L., Marshall, A. L., Sjöström, M., Bauman, A. E., Booth, M. L., Ainsworth, B. E., et al. (2003). International physical activity questionnaire: 12-country reliability and validity. Med. Sci. sports Exerc. 35 (8), 1381–1395. doi:10.1249/01.MSS.0000078924.61453.FB
Diao, X., Ye, F., Zhang, M., Ren, X., Tian, X., Lu, J., et al. (2022). Identification of oleoylethanolamide as an endogenous ligand for HIF-3α. Nat. Commun. 13 (1), 2529. doi:10.1038/s41467-022-30338-z
Estes, C., Chan, H. L. Y., Chien, R. N., Chuang, W. L., Fung, J., Goh, G. B., et al. (2020). Modelling NAFLD disease burden in four Asian regions-2019-2030. Aliment. Pharmacol. Ther. 51 (8), 801–811. doi:10.1111/apt.15673
Estes, C., Razavi, H., Loomba, R., Younossi, Z., and Sanyal, A. J. (2018). Modeling the epidemic of nonalcoholic fatty liver disease demonstrates an exponential increase in burden of disease. Hepatol. Baltim. Md) 67 (1), 123–133. doi:10.1002/hep.29466
Fan, A., Wu, X., Wu, H., Li, L., Huang, R., Zhu, Y., et al. (2014). Atheroprotective effect of oleoylethanolamide (OEA) targeting oxidized LDL. PLoS One 9 (1), e85337. doi:10.1371/journal.pone.0085337
Fougerat, A., Montagner, A., Loiseau, N., Guillou, H., and Wahli, W. (2020). Peroxisome proliferator-activated receptors and their novel ligands as candidates for the treatment of non-alcoholic fatty liver disease. Cells 9 (7), 1638. doi:10.3390/cells9071638
Fu, J., Gaetani, S., Oveisi, F., Lo Verme, J., Serrano, A., Rodríguez De Fonseca, F., et al. (2003). Oleylethanolamide regulates feeding and body weight through activation of the nuclear receptor PPAR-alpha. Nature 425 (6953), 90–93. doi:10.1038/nature01921
Fu, J., Kim, J., Oveisi, F., Astarita, G., and Piomelli, D. (2008). Targeted enhancement of oleoylethanolamide production in proximal small intestine induces across-meal satiety in rats. Am. J. physiology Regul. Integr. Comp. physiology 295 (1), R45–R50. doi:10.1152/ajpregu.00126.2008
Giudetti, A. M., Vergara, D., Longo, S., Friuli, M., Eramo, B., Tacconi, S., et al. (2021). Oleoylethanolamide reduces hepatic oxidative stress and endoplasmic reticulum stress in high-fat diet-fed rats. Antioxidants (Basel, Switz. 10 (8), 1289. doi:10.3390/antiox10081289
Gross, B., Pawlak, M., Lefebvre, P., and Staels, B. (2017). PPARs in obesity-induced T2DM, dyslipidaemia and NAFLD. Nat. Rev. Endocrinol. 13 (1), 36–49. doi:10.1038/nrendo.2016.135
Guzmán, M., Lo Verme, J., Fu, J., Oveisi, F., Blázquez, C., and Piomelli, D. (2004). Oleoylethanolamide stimulates lipolysis by activating the nuclear receptor peroxisome proliferator-activated receptor alpha (PPAR-alpha). J. Biol. Chem. 279 (27), 27849–27854. doi:10.1074/jbc.M404087200
Hamaguchi, M., Kojima, T., Itoh, Y., Harano, Y., Fujii, K., Nakajima, T., et al. (2007). The severity of ultrasonographic findings in nonalcoholic fatty liver disease reflects the metabolic syndrome and visceral fat accumulation. Am. J. Gastroenterol. 102 (12), 2708–2715. doi:10.1111/j.1572-0241.2007.01526.x
Hu, J., Zhu, Z., Ying, H., Yao, J., Ma, H., Li, L., et al. (2020). Oleoylethanolamide protects against acute liver injury by regulating nrf-2/HO-1 and NLRP3 pathways in mice. Front. Pharmacol. 11, 605065. doi:10.3389/fphar.2020.605065
Kwon, H., Song, K., Han, C., Chen, W., Wang, Y., Dash, S., et al. (2016). Inhibition of hedgehog signaling ameliorates hepatic inflammation in mice with nonalcoholic fatty liver disease. Hepatol. Baltim. Md) 63 (4), 1155–1169. doi:10.1002/hep.28289
Laleh, P., Yaser, K., Abolfazl, B., Shahriar, A., Mohammad, A. J., Nazila, F., et al. (2018). Oleoylethanolamide increases the expression of PPAR-Α and reduces appetite and body weight in obese people: A clinical trial. Appetite 128, 44–49. doi:10.1016/j.appet.2018.05.129
Li, L., Li, L., Chen, L., Lin, X., Xu, Y., Ren, J., et al. (2015). Effect of oleoylethanolamide on diet-induced nonalcoholic fatty liver in rats. J. Pharmacol. Sci. 127 (3), 244–250. doi:10.1016/j.jphs.2014.12.001
Li, S., Tan, H. Y., Wang, N., Zhang, Z. J., Lao, L., Wong, C. W., et al. (2015). The role of oxidative stress and antioxidants in liver diseases. Int. J. Mol. Sci. 16 (11), 26087–26124. doi:10.3390/ijms161125942
Ma, L., Guo, X., and Chen, W. (2015). Inhibitory effects of oleoylethanolamide (OEA) on H-O-induced human umbilical vein endothelial cell (HUVEC) injury and apolipoprotein E knockout (ApoE-/-) atherosclerotic mice. Int. J. Clin. Exp. pathology 8 (6), 6301–6311.
Ma, Y., Lee, G., Heo, S. Y., and Roh, Y. S. (2021). Oxidative stress is a key modulator in the development of nonalcoholic fatty liver disease. Antioxidants (Basel, Switz. 11 (1), 91. doi:10.3390/antiox11010091
Mahjoubin-Tehran, M., De Vincentis, A., Mikhailidis, D. P., Atkin, S. L., Mantzoros, C. S., Jamialahmadi, T., et al. (2021). Non-alcoholic fatty liver disease and steatohepatitis: State of the art on effective therapeutics based on the gold standard method for diagnosis. Mol. Metab. 50, 101049. doi:10.1016/j.molmet.2020.101049
Manna, P., and Jain, S. K. (2015). Obesity, oxidative stress, adipose tissue dysfunction, and the associated health risks: Causes and therapeutic strategies. Metab. Syndr. Relat. Disord. 13 (10), 423–444. doi:10.1089/met.2015.0095
Misto, A., Provensi, G., Vozella, V., Passani, M. B., and Piomelli, D. (2019). Mast cell-derived histamine regulates liver ketogenesis via oleoylethanolamide signaling. Cell Metab. 29 (1), 91–102.e5. doi:10.1016/j.cmet.2018.09.014
Oveisi, F., Gaetani, S., Eng, K. T., and Piomelli, D. (2004). Oleoylethanolamide inhibits food intake in free-feeding rats after oral administration. Pharmacol. Res. 49 (5), 461–466. doi:10.1016/j.phrs.2003.12.006
Palazon, A., Goldrath, A. W., Nizet, V., and Johnson, R. S. (2014). HIF transcription factors, inflammation, and immunity. Immunity 41 (4), 518–528. doi:10.1016/j.immuni.2014.09.008
Pan, X., Schwartz, G. J., and Hussain, M. M. (2018). Oleoylethanolamide differentially regulates glycerolipid synthesis and lipoprotein secretion in intestine and liver. J. lipid Res. 59 (12), 2349–2359. doi:10.1194/jlr.M089250
Paredes-Turrubiarte, G., González-Chávez, A., Pérez-Tamayo, R., Salazar-Vázquez, B. Y., Hernández, V. S., Garibay-Nieto, N., et al. (2016). Severity of non-alcoholic fatty liver disease is associated with high systemic levels of tumor necrosis factor alpha and low serum interleukin 10 in morbidly obese patients. Clin. Exp. Med. 16 (2), 193–202. doi:10.1007/s10238-015-0347-4
Payahoo, L., Khajebishak, Y., Asghari Jafarabadi, M., and Ostadrahimi, A. (2018). Oleoylethanolamide supplementation reduces inflammation and oxidative stress in obese people: A clinical trial. Adv. Pharm. Bull. 8 (3), 479–487. doi:10.15171/apb.2018.056
Rolo, A. P., Teodoro, J. S., and Palmeira, C. M. (2012). Role of oxidative stress in the pathogenesis of nonalcoholic steatohepatitis. Free Radic. Biol. Med. 52 (1), 59–69. doi:10.1016/j.freeradbiomed.2011.10.003
Sabahi, M., Ahmadi, S. A., Kazemi, A., Mehrpooya, M., Khazaei, M., Ranjbar, A., et al. (2022). The effect of oleoylethanolamide (OEA) add-on treatment on inflammatory, oxidative stress, lipid, and biochemical parameters in the acute ischemic stroke patients: Randomized double-blind placebo-controlled study. Oxid. Med. Cell Longev. 2022, 5721167. doi:10.1155/2022/5721167
Sayd, A., Antón, M., Alén, F., Caso, J. R., Pavón, J., Leza, J. C., et al. (2014). Systemic administration of oleoylethanolamide protects from neuroinflammation and anhedonia induced by LPS in rats. Int. J. Neuropsychopharmacol. 18 (6), pyu111. doi:10.1093/ijnp/pyu111
Singh, S. P., Anirvan, P., Khandelwal, R., and Satapathy, S. K. (2021). Nonalcoholic fatty liver disease (NAFLD) name change: Requiem or reveille? J. Clin. Transl. hepatology 9 (6), 931–938. doi:10.14218/JCTH.2021.00174
Stojsavljević, S., Gomerčić Palčić, M., Virović Jukić, L., Smirčić Duvnjak, L., and Duvnjak, M. (2014). Adipokines and proinflammatory cytokines, the key mediators in the pathogenesis of nonalcoholic fatty liver disease. World J. gastroenterology 20 (48), 18070–18091. doi:10.3748/wjg.v20.i48.18070
Sun, Y., Alexander, S. P., Garle, M. J., Gibson, C. L., Hewitt, K., Murphy, S. P., et al. (2007). Cannabinoid activation of PPAR alpha; a novel neuroprotective mechanism. Br. J. Pharmacol. 152 (5), 734–743. doi:10.1038/sj.bjp.0707478
Takaki, A., Kawai, D., and Yamamoto, K. (2013). Multiple hits, including oxidative stress, as pathogenesis and treatment target in non-alcoholic steatohepatitis (NASH). Int. J. Mol. Sci. 14 (10), 20704–20728. doi:10.3390/ijms141020704
Tutunchi, H., Naeini, F., Saghafi-Asl, M., Farrin, N., Monshikarimi, A., and Ostadrahimi, A. (2020). Effects of oleoylethanolamide supplementation on atherogenic indices and hematological parameters in patients with nonalcoholic fatty liver disease: A clinical trial. Health Promot Perspect. 10, 373–382. doi:10.34172/hpp.2020.56
Tutunchi, H., Ostadrahimi, A., Saghafi-Asl, M., Hosseinzadeh-Attar, M-J., Shakeri, A., Asghari-Jafarabadi, M., et al. (2020). Oleoylethanolamide supplementation in obese patients newly diagnosed with non-alcoholic fatty liver disease: Effects on metabolic parameters, anthropometric indices, and expression of PPAR-α, UCP1, and UCP2 genes. Pharmacol. Res. 156, 104770. doi:10.1016/j.phrs.2020.104770
Tutunchi, H., Ostadrahimi, A., Saghafi-Asl, M., and Maleki, V. (2019). The effects of oleoylethanolamide, an endogenous PPAR-α agonist, on risk factors for NAFLD: A systematic review. Obes. Rev. official J. Int. Assoc. Study Obes. 20 (7), 1057–1069. doi:10.1111/obr.12853
Tutunchi, H., Ostadrahimi, A., Saghafi-Asl, M., Roshanravan, N., Shakeri-Bavil, A., Asghari-Jafarabadi, M., et al. (2021). Expression of NF-κB, IL-6, and IL-10 genes, body composition, and hepatic fibrosis in obese patients with NAFLD-combined effects of oleoylethanolamide supplementation and calorie restriction: A triple-blind randomized controlled clinical trial. J. Cell Physiol. 236 (1), 417–426. doi:10.1002/jcp.29870
Tutunchi, H., Saghafi-Asl, M., Asghari Jafarabadi, M., and Ostadrahimi, A. (2020). The relationship between severity of liver steatosis and metabolic parameters in a sample of Iranian adults. BMC Res. Notes 13, 218. doi:10.1186/s13104-020-05059-5
Wang, H., Mehal, W., Nagy, L. E., and Rotman, Y. (2021). Immunological mechanisms and therapeutic targets of fatty liver diseases. Cell Mol. Immunol. 18 (1), 73–91. doi:10.1038/s41423-020-00579-3
Yang, L., Guo, H., Li, Y., Meng, X., Yan, L., Dan, Z., et al. (2016). Oleoylethanolamide exerts anti-inflammatory effects on LPS-induced THP-1 cells by enhancing PPARα signaling and inhibiting the NF-κB and ERK1/2/AP-1/STAT3 pathways. Sci. Rep. 6, 34611. doi:10.1038/srep34611
Keywords: OEA, inflammation, and NAFLD inflammation, non-alcoholic fatty liver disease, oleoylethanolamide, oxidative stress
Citation: Tutunchi H, Zolrahim F, Nikbaf-Shandiz M, Naeini F, Ostadrahimi A, Naghshi S, Salek R and Najafipour F (2023) Effects of oleoylethanolamide supplementation on inflammatory biomarkers, oxidative stress and antioxidant parameters of obese patients with NAFLD on a calorie-restricted diet: A randomized controlled trial. Front. Pharmacol. 14:1144550. doi: 10.3389/fphar.2023.1144550
Received: 14 January 2023; Accepted: 28 March 2023;
Published: 07 April 2023.
Edited by:
Xiaoying Yang, Xuzhou Medical University, ChinaReviewed by:
Xiaotang Fan, Third Military Medical Univeristy, ChinaHanchen Xu, Longhua Hospital Shanghai University of Traditional Chinese Medicine, China
Copyright © 2023 Tutunchi, Zolrahim, Nikbaf-Shandiz, Naeini, Ostadrahimi, Naghshi, Salek and Najafipour. This is an open-access article distributed under the terms of the Creative Commons Attribution License (CC BY). The use, distribution or reproduction in other forums is permitted, provided the original author(s) and the copyright owner(s) are credited and that the original publication in this journal is cited, in accordance with accepted academic practice. No use, distribution or reproduction is permitted which does not comply with these terms.
*Correspondence: Farzad Najafipour, Farzadnajafipour@gmail.com