- Department of Rheumatology and Immunology, Guangxi Academy of Medical Sciences, The People’s Hospital of Guangxi Zhuang Autonomous Region, Nanning, China
Interstitial lung disease (ILD) is a heterogeneous group of diseases characterized by lung injury caused by lung fibroblast proliferation, interstitial inflammation, and fibrosis. Different cell signal transduction pathways are activated in response to various proinflammatory or fibrotic cytokines, such as IL-6, and these cytokines are increased in different ILDs. The overexpressed cytokines and growth factors in ILD can activate TGF-β/Smad2/3/4, NF-κB, and JAK/STAT signal transduction pathways, promote the activation of immune cells, increase the release of pro-inflammatory and pro-fibrotic factors, differentiate fibroblasts into myofibroblasts, and promote the occurrence and development of ILD. This finding suggests the importance of signal transduction pathways in patients with ILD. Recent evidence suggests that resveratrol (RSV) attenuates excessive inflammation and pulmonary fibrosis by inhibiting the TGF-β/Smad2/3/4, NF-κB, and JAK/STAT signal transduction pathways and overactivation of immune cells. In this review, advances in lung protection and the underlying mechanisms of RSV are summarized, and the potential efficacy of RSV as a promising treatment option for ILD is highlighted.
Introduction
Interstitial lung disease (ILD) includes a group of heterogeneous diseases, which can be derived from a variety of different etiology, such as infection, drug, radiation-induced lung diseases and autoimmune diseases such as rheumatoid arthritis (RA), etc., leading to damage of alveolar epithelium and lung parenchyma, eventually leading to lung inflammation and fibrosis (Shao et al., 2021). ILD was characterized by inflammation or fibrosis of different degrees in the lung parenchyma. For example, in inflammatory diseases, the histological form is characterized by institutional pneumonia or non-specific interstitial pneumonia, while diseases dominated by fibrosis are characterized by common interstitial pneumonia (Mikolasch et al., 2017) (Figure1).
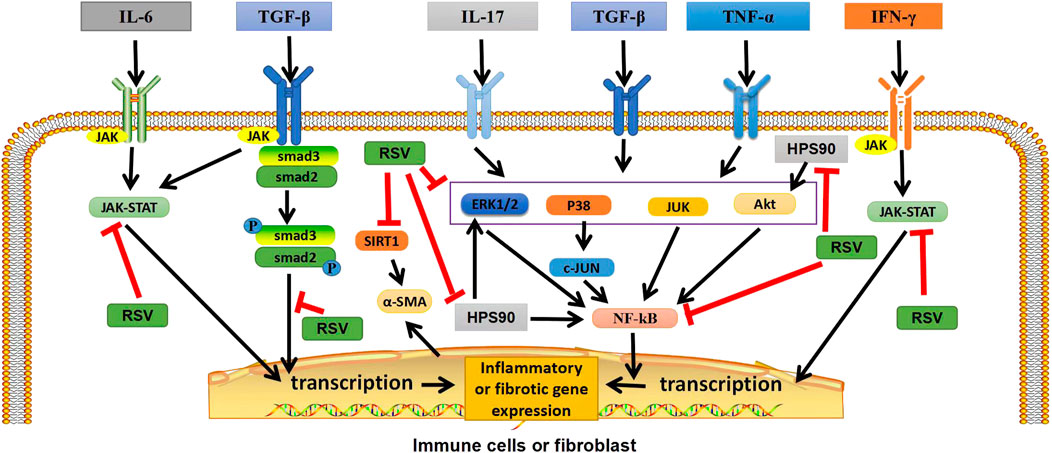
FIGURE 1. Graphical abstract: Potential targets of RSV in ILD. In immune cells or fibroblasts, RSV exerts anti-inflammatory and antifibrotic effects by inhibiting TGF-β/Smad2/3/4, NF-κB, and JAK/STAT pathways, as well as by regulating heat shock protein 90, inhibiting Akt, ERK1/2 and NF-κB pathways. In addition, RSV can also inhibit SIRT1, reduce α-ASM activation, and play an antifibrotic role. TGF-β, transforming growth factor β; IFN-γ, interferon-γ; TNF-α, tumor necrosis factor; P, phosphate; JAK, janus tyrosine kinase; STAT, signal transducers and activators of transcription; ERK1/2,extracellular signal-regulated protein kinases; AKT, serine-threonine kinase; NF-κB, nuclear factor-kappa B; SIRT1, sirtuin1; α-SMA, α-smooth muscle actin. “ê” indicates activation, and “—׀” indicates inhibition.
ILD is one of the most common and serious lung diseases. It was found that the total incidence of ILD was 19.4/100,000, and the most common diagnosis was sarcoidosis (42.6%) (Duchemann et al., 2017). Overactivation of immune cells in ILD patients resulted in the release of pro-inflammatory/pro-fibrotic cytokines and oxidative damage, and abnormal myofibroblast activation was observed with enhanced epithelial-interstitial transformation (EMT) and the secretion of large amounts of collagen for post-injury repair (You et al., 2015; Gouda and Bhandary, 2018). This leads to excessive deposition of extracellular matrix (ECM) and the development of interstitial fibrosis (Pardo and Selman, 2002). Eventually, symptoms such as a dry cough, shortness of breath and fatigue. The management and treatment of ILD remains challenging for clinicians. Currently, common therapeutic strategies include glucocorticoids and immunosuppressants (such as cyclophosphamide) (Shao et al., 2021), but sometimes they are resistant to the above-mentioned drugs and have serious adverse reactions (Huo et al., 2022). Therefore, it is urgent to find a drug with significant efficacy and few side effects.
Resveratrol (RSV) is a natural plant antitoxin, which is abundant in a variety of plants such as grapes and peanuts as well as a variety of commercial products, such as grape juice and red wine (Truong et al., 2018). Resveratrol exists in two isomeric forms, cis-trans and trans-trans, but trans is the main form, which has the most effective therapeutic benefits due to its low steric hindrance of the side chain (Cardile et al., 2007; Weiskirchen and Weiskirchen, 2016; Raj et al., 2021). Trans can be reconstituted from yeast extracts and become more biologically active forms with higher stability (Chen et al., 2007; Camont et al., 2009). It has been reported that RSV can slow down the progression of autoimmune diseases, such as RA, systemic lupus erythematosus (SLE), psoriasis (PsO) and inflammatory bowel disease (IBD), etc. (Oliveira et al., 2017). In addition, studies have shown that RSV has inhibitory effects on inflammation and anti-fibrosis, and thus has a therapeutic prospect for pulmonary diseases and fibrosis diseases (Vargas et al., 2016). At present, more and more studies have reported that RSV can play an anti-fibrosis role by inhibiting the molecules and cells in some signal transduction pathways. In animal model studies, RSV has been shown to improve ILD in mice by inhibiting the expression of Smad and Smad7, inhibiting the over-activation of immune cells, inhibiting inflammation and the proliferation and differentiation of lung fibroblasts, and reducing collagen deposition (Chávez et al., 2008; Liu et al., 2010). Recent studies have found that RSV can also play a beneficial role in the later stage of pulmonary fibrosis by regulating the metabolism of collagen in lung tissue and reducing its deposition in lung interstitium (Wang et al., 2021). In addition, by inhibiting the activation of NF-κB in macrophages and lymphocytes, the expression levels of nitric oxide and tumor necrosis factor-α (TNF-α), interleukin (IL-1β), IL-6, transforming growth factor-β (TGF-β) and TNF in pulmonary fibrosis are reduced to prevent oxidative damage (Yahfoufi et al., 2018; Fu et al., 2020). RSV may inhibit pro-inflammatory factors and down-regulate oxidative stress levels by inhibiting epithel-mesenchymal transformation and down-regulating NF-κB and TGF-β1/smad3 signaling pathways (Wang et al., 2022). At the same time, the autophagy process and the nucleotide-binding domain and leucine-rich repeat protein 3 inflammasome activities are inhibited, and the production of pro-inflammatory factors is reduced, thus improving lung inflammation and fibrosis (Ding et al., 2019). In addition, in the fusion process of autophagosome and lysosome, RSV can relieve their inhibitory state and reverse the destruction of autophagosome—lysosome fusion, thus improving ILD (Bao et al., 2022). These findings suggest that RSV can be used as a potential treatment for ILD (Figure 1).
In this review, we focus on the protective effects and potential mechanisms of RSV against ILD. Meanwhile, this review summarizes the progress of RSV in the treatment of ILD and its related adverse reactions, providing new insights for the treatment of ILD.
Mechanism of ILD formation
Immune cells and ILD
In ILD patients, especially those with autoimmune ILD, immune disorders can activate macrophages, T cells, B cells, and NK cells to damage the alveolar epithelium, and then repeated repair leads to the gradual destruction of functional lung parenchyma, which is replaced by increased deposition of non-functional connective tissue (Chambers and Mercer, 2015), eventually leading to ILD. The mechanism of different types of immune cells in ILD is shown in Table 1.
Macrophages and ILD
Macrophages are immune cells that differentiate from monocytes and play a role in the balance between innate and adaptive immunity. In the process of fibrosis caused by immune imbalance, monocytes in the blood are recruited into the lungs and activated into macrophages under the action of chemokines, and cytokines are secreted to differentiate fibroblasts into myofibroblasts. In addition, macrophages can be polarised into “pro-inflammatory” classical M1 macrophages that secrete pro-inflammatory and/or pro-fibrotic cytokines, such as interleukin (IL) -1β, or “pro-fibrotic” M2a macrophages that secrete pro-fibrotic cytokines (Kolahian et al., 2016; Bellamri et al., 2019), thus promoting the progression of pulmonary fibrosis. Especially in SSc-ILD. In inflammatory environment, promoting nuclear factor (NF) -κB and Janus kinase (JAK)/signal transductor and transcription activator (STAT) pathways in macrophages to induce the production of pro-inflammatory factors, such as tumor necrosis factor (TNF) -α, IL-1, IL-6, etc. (Balachandran and Adams, 2013), can promote the occurrence of ILD.
T cells and ILD
At present, preclinical studies have determined that some T cell subtypes are associated with fibrosis, such as Th2 and Th17, which promote fibrosis. Up-regulation of Pd-1 on CD4+ T cells increases type 1 collagen synthesis and promotes pulmonary fibrosis through STAT 3-mediated IL-17A and TGF-β production (Celada et al., 2018). At present, the most studied ILD are IPF and SSc-ILD. The two diseases share similar common features, such as T cell profiles (Th2, Th17, increased ratio of CD4 to CD8 T cells), T cell cytokine profiles (IL-4, IL-5, IL-10, and IL-17 of IPF, and IL-4, IL-5, IL-6, IL-10, IL-13, and IL-22 in SSc-ILD (Bagnato and Harari, 2015). In RA-ILD, like IPF and SSc-ILD, the expression of IL-17 receptor was up-regulated, indicating that Th17 cell-mediated immunity was involved in the pathogenesis of ILD (Wang et al., 2019; Zhang et al., 2019). Furthermore, the number of CD4+ T cells in lung tissues of RA-ILD patients was significantly higher than that of idiopathic UIP patients, suggesting that immune dysregulation may be more prevalent in RA-ILD patients than in idiopathic UIP patients (Turesson et al., 2005). These results indicated that the imbalance of T cell subsets might be involved in ILD formation.
B cells and ILD
As a result of immune disorders in patients with autoimmune diseases, autoantigens are presented to CD4+ helper T cells by antigen-presenting cells and then further presented to B cells, so that B cells differentiate into plasma cells and produce and secrete autoantibodies. Recently, several studies have pointed to a possible role of B cells in IPF, RA, or ILD associated with connective tissue disease. Some studies have found that, compared with healthy people, the phenotype distribution of B cells in the peripheral blood of IPF patients is abnormal, and the percentage of plasmabytes in IPF patients is negatively correlated with forced vital capacity (Xue et al., 2013). The authors detected focal aggregates of CD20-positive B cells in all IPF lung tissues using immunohistochemistry (Xue et al., 2013). Atkins et al. (2006) compared the lung biopsy specimens of patients with RA-ILD and control subjects and observed obvious follicular B cell hyperplasia in patients with RA-ILD. Recent studies have also found that the total peripheral blood B cell count is higher in patients with RA-ILD, but the frequency of memory B cells is lower. The mechanism may be that some of them selectively migrate to the lung tissue (Shimizu et al., 2021). In some ILDs, B cell production of antibodies may play a key role. For example, there is an association between antibodies, including anti-citrullinated protein antibodies (ACPA) and rheumatoid factor (RF), and the risk of RA-ILD (Katsumata et al., 2019). The presence of Sjogren’s syndrome-related antigen A antibody is a predisposing factor for ILD in patients with Sjogren’s syndrome (Flament et al., 2016). Anti-synthetase antibody or anti-melanoma differentiation-associated protein 5 (MDA5) antibodies are associated with myositis-related ILD, while anti-topoisomerase I (anti-scl70), anti-U11/U12, and anti-eukaryotic initiation factor 2B antibodies are associated with SSc-ILD (Kuwana et al., 2021). These results suggest that B cell-formed autoantibodies may be involved in the formation of ILD.
Natural killer (NK) cells and ILD
Previous studies have shown that NK cells play a key role in the pathogenesis of acute lung injury (Liu et al., 2016). There are two different NK environments in human pulmonary fibrosis: one in the lung and the other in the peripheral blood. In pulmonary fibrosis, NK cells are thought to counteract the fibrotic activity of TGF-β by producing the anti-fibrotic mediator interferon-γ (IFN-γ), thereby inducing anti-fibrotic signals in the lung (Culley, 2009). As for NK cells in the peripheral blood, studies have found that the percentage and absolute number of NK cells in the peripheral blood of 11 IPF patients are both high (Esposito et al., 2005). In addition, a retrospective study found that the absolute and relative counts of CD3−CD56+ NK cells in the peripheral blood of patients with RA-ILD were higher than those of patients with RA, whereas the percentages of T cells and CD4+ T cells were lower, suggesting that the occurrence of RA-ILD may be unbalanced with the lymphocyte subsets of patients. In particular, CD3−CD56+ NK cells are associated with T cell imbalances (Lai et al., 2019). The increase in the percentage and absolute number of CD3−CD56+ NK cells clearly suggests a possible involvement in the pathogenesis of human ILD and suggests a novel approach for studying ILD. These results suggest that NK cells may be involved in the formation of profibrotic, antifibrotic, and ILD conditions; however, further studies are needed.
Cytokine and growth factor-related signal transduction pathways and ILD
Multiple molecular pathways are activated by pro-inflammatory/pro-fibrotic cytokines (e.g., IL-6, IL-17, and TNF-α) and growth factors (e.g., TGF-β), which are increased in different ILD. The above overexpressed cytokines and growth factors in ILD were activated in the corresponding intracellular signal transduction pathways, such as the Smad-dependent and Smad-independent signaling pathways, NF-κB and JAK/STAT signaling pathways, which were closely related to the occurrence and development of ILD (Figure 2).
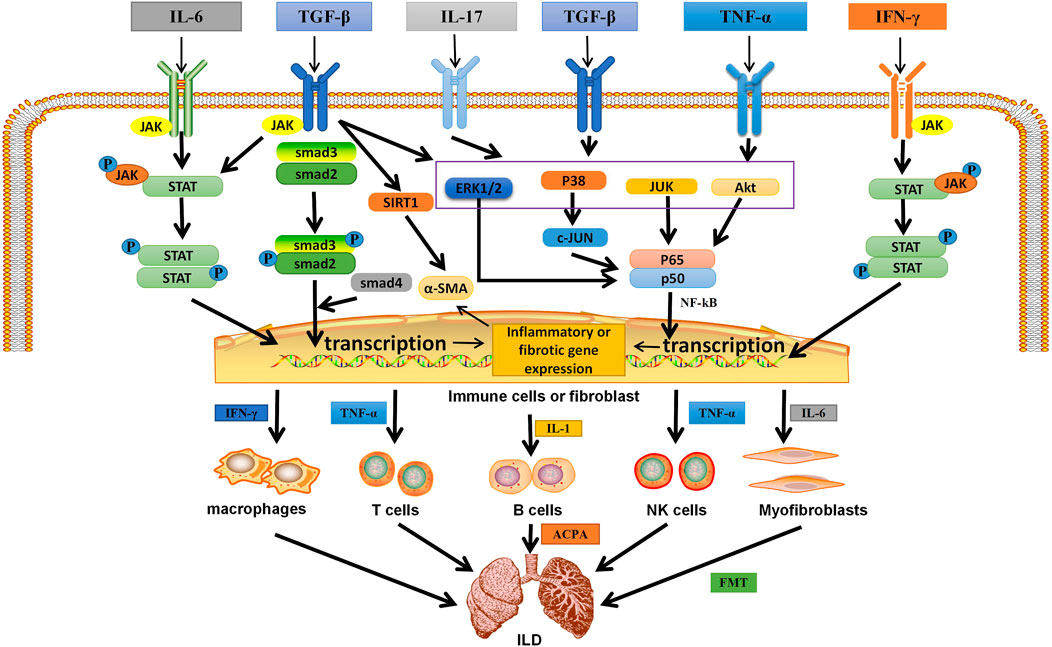
FIGURE 2. The related mechanism of ILD occurrence. Cytokines and growth factors, after binding to their corresponding receptors in immune cells or myofibroblasts, can be activated through different signal transduction pathways. Activated immune cells can secrete a variety of cytokines. They further activate macrophages; T, B, and NK cells; and myofibroblasts. They are over-activated and can secrete pro-inflammatory and pro-fibrotic cytokines, which is the environment for the formation of inflammation and fibrosis in the lung. Meanwhile, in different autoimmune diseases, B cells secrete corresponding antibodies, which are related to pulmonary fibrosis. In addition, IL-6 can activate myofibroblasts, causing them to undergo EMT and form ECM, which is deposited in the lung. Eventually ILD is formed. IL, interleukin; TGF-β, transforming growth factor β; IFN-γ, interferon-γ; TNF-α, tumor necrosis factor; P, phosphate; JAK, janus tyrosine kinase; STAT, signal transducers and activators of transcription; ERK1/2,extracellular signal-regulated protein kinases; AKT, serine-threonine kinase; NF-κB, nuclear factor-kappa B; SIRT1, sirtuin1; α-SMA, α-smooth muscle actin; ACPA, anticitrullinated peptide antibodie; ECM,extracellular matrix; EMT, epithelial-mesenchymal transition; ILD,Interstitial lung disease.
① Activation of a Smad-dependent signaling pathway: after TGF-β induction, the downstream transduction molecule Smad2 binds to Smad3, which is subsequently phosphorylated and activated to form trimers with Smad4 and transmit signals from the cell membrane to the nucleus (Feng and Derynck, 2005).
② Activation of the Smad independent signaling pathway and the NF-κB pathway: TGF-β receptor complex or TNF-α interacts with tumor necrosis factor receptor-associated protein 6 (TRAF6) to promote its own ubiquitination (Tzavlaki and Moustakas, 2020). TRAF6 activates TGF-β-activated kinase 1 (TAK1) through Lys63-linked polyubiquitination, which in turn is activated by phosphorylating MAP kinase kinases (MKK) MKK4, MKK3, and MKK6. MKKS further activate their downstream kinases JNK and p38, which can then phosphorylate their target transcription factors (TF) and regulate transcription (Tzavlaki and Moustakas, 2020). In addition, TGF-β-induced ShcA tyrosine phosphorylation promotes Ras protein activation. This leads to the sequential activation of Raf, mitogen activated protein kinase, and extracellular signal-regulated protein kinase (ERK1/2). Activated ERK1/2 phosphorylates TF. TGF-β also promotes the activation of rapamycin C2 through phosphatidylinositol 3-kinase (PI3K), which further recruits and phosphorylates serine/threonine kinase (AKT) (Tzavlaki and Moustakas, 2020), thereby activating the NF-kB pathway. Studies have shown that TGF-β can also induce Sirtuin 1 activation and activate α-smooth muscle actin (α-SMA) (Ma and Li, 2020). In addition, IL-17 forms heterodimer complexes with its receptors IL-17RA and IL-17RC to recruit NF-κB activator 1(Act1) through SEFIR domain interactions. Subsequently, Act1 binds to TRAF6 via its TRAF6 binding site, recruiting TRAF6 into the IL-17R complex) (Zhu and Qian, 2012). Act1 acts as E3 ubiquitin ligase to polymerize TRAF6. TRAF6 also acts as an E3 ubiquitin ligase, which may ubiquitinate TAK1 complex and activate PI3K/AKT leading to NF-κB activation (Zhu and Qian, 2012). In addition, heat shock protein (HSP) is also involved in ILD formation. HSP90 has been shown to directly regulate ERK through dissociation of the ERk-HSP90-CDC37 complex (Erazo et al., 2013), thereby activating the ERK pathway. HSP90 also regulates AKT, and activation of AKT pathway has been reported in different cells with different pathological functions for pulmonary fibrosis (Colunga Biancatelli et al., 2020).
③JAK/STAT signaling pathway: Activation of the JAK/STAT pathway begins with the binding of ligands (usually cytokines, growth factors, such as IL-6, TGF-β, and IFN-γ) to their receptors, inducing JAK dimerization. The receptor-associated JAK is then activated and phosphorylates the tyrosine residue in the tail of its receptor cell to form p-JAK. These phosphorylations act as docking sites for STAT and bind to them via its SH2 domain, which phosphorylates STAT and is activated by tyrosine phosphorylation to form p-STAT, which subsequently becomes a dimer and translocations from the cytoplasm to the nucleus, where they act as transcription factors (Montero et al., 2021). Thus, STATs are activated by JAKs and transferred from cytoplasm to nucleus, and then bind to specific DNA sequences to direct gene transcription, resulting in increased expression of pro-inflammatory cytokines TNF-α and IL-1β and pro-fibrotic cytokine IL-6 in the lung (Yang et al., 2019).
In the lung, the above cytokines and growth factors are activated in immune cells through the above signal transduction pathways, resulting in the expression of pro-inflammatory, pro-fibrosis genes and α-SMA (Ma and Li, 2020), thus activating more immune cells (including macrophages, T cells, B cells, and NK cells) and lung fibroblasts (Figure 2). The above pathway can up-regulate the expression of macrophage related polarization markers (such as CD86 and CD206), and cause macrophages to differentiate into “pro-inflammatory” M1 macrophages and release inflammatory factors (such as IFN-γ), and “pro-fibrotic” M2a macrophages and release pro-fibrotic cytokines (IL-6) (Lescoat et al., 2020). T cells and NK cells release pro-inflammatory cytokines (such as IFN-γ, TNF-α, IL-1, and IL-17) and pro-fibrotic cytokines (IL-6) (Figure 2) (Alesci et al., 2022). In addition to releasing cytokines, B cells can also differentiate into plasma cells and secrete ACPA, anti-SCL70 antibodies (Bellamri et al., 2019). Moreover, positive feedback amplifies the above signaling pathways and promotes the occurrence of pulmonary inflammation and fibrosis (Lescoat et al., 2020). Lung fibroblasts can induce self-proliferation or mesenchymal transformation through the pathway, resulting in abnormal expression of α-SMA and EMC components, thus causing fibroblasts to lose differentiation and obtain mesenchymal phenotype of myofibroblasts, ultimately leading to ILD formation (Duchemann et al., 2017) (Figure 2).
Effects of RSV on immune cells
RSV can enter cells by a variety of means (e.g. passive diffusion, endocytosis, or via transporters) and bind to specific receptors, such as the integrin receptor alphavβ3 (Delmas and Lin, 2011; Ho et al., 2020). It helps regulate innate and adaptive immunity, such as macrophages, B cells, T cells, and NK cells, thereby inhibiting overactivation of cells, reducing the production of pro-inflammatory or pro-fibrotic factors, and controlling the progression of ILD. Effects of RSV on immune cells (Table 2).
RSV and autoimmune diseases
The development of autoimmune diseases may result in damage to one or more body tissues or organs. Autoimmune diseases disorders of immune cells in the body, which cause immune cells to overactivate and produce a large number of inflammatory factors, such as TNF-α, IFN-γ, and IL-1β. Overstrong immune response can simultaneously attack different organs or tissues, leading to local or systemic immune response, such as RA, amyotrophic lateral sclerosis (ALS), PsO, SLE, IBD, etc. (Oliveira et al., 2017). RSV is a well-studied substance known for its effects on a large number of chronic diseases and its numerous therapeutic benefits, including anti-regulatory immunity, anti-inflammatory, etc. In autoimmune diseases, RSV can inhibit synovial macrophages, reduce angiogenesis, leukocyte and lymphocyte recruitment, fibroblast proliferation and protease secretion, especially in RA, and thus inhibit cartilage and bone destruction at pus formation sites (Oliveira et al., 2017). It can also inhibit the differentiation of T lymphocytes into Th2 and Th17, and reduce the activation of B cells and the production of autoantibodies (Oliveira et al., 2017). Cytokines are also closely related to autoimmune diseases. For example, macrophages increase the recruitment of neutrophils at the site of inflammation through the activation of NF-κB, and produce related cytokines (IFN-γ, TNF-α, IL-17, IL-21, and IL-23, etc.). In experimental animals, When IL-6, TNF-α and other disorders occur, they are sufficient to cause destructive arthritis (Oliveira et al., 2017). RSV not only inhibited TNF-α and IL-1β-induced NF-κB activation, but also activated SIRT1 and inhibited RelA acetylation in mice with arthritis. In addition, the expression of NF-κB-induced inflammatory factors such as TNF-α, IL-1β, IL-6, metalloproteinase (MMP)-1 and MMP3 is decreased (Yamamoto and Gaynor, 2001). It can also reduce the mRNA expression levels of IL17 and IL19, reduce the thickness of animal skin and improve the damage caused by psoriasis (Malaguarnera, 2019). In addition, RSV reduced mRNA expression levels of IL-6, TNF-α, IFN-γ, JAK, and STAT when treating inflammatory mice (Yang et al., 2019). It is suggested that RSV can inhibit the anti-inflammatory effect of JAK/STAT signaling pathway. Therefore, RSV may have some efficacy in the treatment of autoimmune diseases by regulating the immune system and interfering with multiple cellular and molecular processes (see Table 3). Epithelial interstitial transformation (EMT) is an important factor in the development of pulmonary fibrosis (Tian et al., 2017). Some studies have found that the activation of NF-κB is an effective inducer of EMT activation, suggesting a close relationship between pulmonary fibrosis, inflammation and EMT (Chua et al., 2007; Chen et al., 2018a). In autoimmune diseases, some cytokines, such as IFN-γ, TNF-α, IL-17, and IL-6, are associated with ILD (Montero et al., 2021). In addition, it has been found that JAK-STAT pathway plays an important role in early alveolitis and the development of ILD (Banerjee et al., 2017). In RA-ILD mouse models, intraperitoneal injection of a JAK inhibitor (tofacitinib) improved symptoms and inhibited the progression of ILD (Sendo et al., 2019). RSV can not only reduce the levels of NF-κB and JAK-STAT, but also reduce the levels of IFN-γ, TNF-α, IL-17, and other cytokines, so RSV may have the potential to treat ILD, especially auto-immune-related ILD.
RSV and ILD
ILD is associated with decreased lung function, and complications can lead to rapid deterioration of the clinical course of ILD patients. In the past few years, two anti-fibrosis drugs pirfenidone and Nidanib have been approved for the treatment of ILD patients, but there are associated adverse reactions, including gastrointestinal adverse reactions, fatigue, weight loss, etc. (Morrow et al., 2022). More and more studies confirm that RSV is an ideal treatment for ILD. Fagone et al. (2011) studied the effect of RSV on TGF-β-induced myoblast activation of human lung fibroblasts. They found that RSV inhibited collagen production, lung fibroblast proliferation, and α-SMA protein levels by inhibiting the phosphorylation of ERK1/2 and Akt, and down-regulated TGF-β1 levels by inhibiting TGF-β1/Smad2/3/4 signaling pathways, improving pulmonary fibrosis in mouse models (Fagone et al., 2011). In addition, RSV also down-regulates TGF-β-induced Smad2/3 phosphorylation by reducing the phosphorylation levels of c-Jun, α-SMA, p-Smad2, Smad7, and p38, significantly reducing TGF-β-induced collagen deposition and alleviating symptoms in rats (Wang et al., 2018). Recent results showed that RSV (40 mg/kg) could not only inhibit the expression of TGF-β1 in IPF rats, but also inhibit the phosphorylation of Smad2/3 and ERK1/2, significantly reduce the elevated levels of TNF-α, IL-6, and IL-13 excitability in ILD rats, and inhibit the damage of alveolar wall. Inflammatory cell infiltration, congestion and edema are reduced (Liu et al., 2020). These results indicate that RSV can inhibit TGF-β/Smad2/3/4 and NF-κB signaling pathways to play anti-inflammatory and antifibrotic roles.
In addition, Li et al. found that RSV targeted TAK1, significantly inhibited TAK1 activation, inhibited alveolar macrophages in alveoli, and reduced lung inflammation and pulmonary fibrosis in mice (Li et al., 2017). In bleomycin-induced EMT-related pulmonary fibrosis, SIRT1 expression is decreased, and the expression of type I collagen and α-SMA is increased (Rong et al., 2016). After RSV treatment, SIRT1 expression is increased, which reduces alveolar epithelial cell damage, fibroblast proliferation, collagen deposition and pulmonary fibrosis, and is related to lung protection (Ma and Li, 2020).
A recent study evaluated the effect of RSV on RA-ILD. We treated RA-ILD rats with RSV (10 mg/kg/day) orally for 4 weeks, and found that RSV inhibited the JAK/STAT signaling pathway (i.e. the expression of JAK and STAT were significantly reduced in lung tissue) and decreased the levels of pro-inflammatory cytokines TNF-α, IL-6, and IL-1β. Meanwhile, it can promote the production of anti-inflammatory factor IL-10 and reduce lung inflammation (Colunga Biancatelli et al., 2020). In addition, the authors found that RSV improved pulmonary pathology (reduced inflammatory cell infiltration, reduced collagen deposition, significantly thinner alveolar wall thickness) and reduced fibrosis degree (Colunga Biancatelli et al., 2020). Therefore, RSV plays an anti-inflammatory and antifibrotic role by regulating the JAK/STAT signaling pathway, thereby improving RA-ILD.
In ILD, HSP90 expression was increased, and HSP90 ATPase activity was increased in fibroblasts isolated from fibrotic lung injury (Sontake et al., 2017). HSP90 may also contribute to the development of pulmonary fibrosis through IL-6, as HSP90 mediates activation of the nuclear factor kappa light chain enhancer in the B cell (NF-κB) dependent inflammatory pathway, promoting IL-6 production (Bohonowych et al., 2014; Bellaye et al., 2018). In IPF, alveolar epithelial type II cells promote pulmonary fibrosis through HSP90-AKT signaling (Chen et al., 2021). In SSc-ILD patients, HSP90 was overexpressed, and HSP90 could promote the persistence of myoblasts in pulmonary fibrosis by enhancing TGF-β signal transduction pathway (Štorkánová et al., 2021). In RA-ILD, IFN-γ produced by T cells stimulated by anti-citrullinated HSP90 indicated a TH1 immune response, and thus participated in the development of ILD (Chen et al., 2018b). In addition, inhibition of HSP90 has an effective antifibrotic effect in vitro and in mouse pulmonary fibrosis models (Koh et al., 2015). The use of HSP90 inhibitors showed that inhibition of HSP90 can down-regulate the expression of AKT, ERK, and NF-κB, regulate the stability of TGF-β receptor, and interfere with the Smad and non-Smad (P-ERK) TGF-β signaling cascade. Thus, EMT is reduced and cell proliferation, formation of fibrotic mediators and ECM are reduced (Schumacher et al., 2007). Studies have shown that RSV can inhibit HSP90 (Liu et al., 2014). RSV inhibition of HSP90 can not only down-regulate MEK1/2 and ERK phosphorylation and NF-κB expression, but also reduce IL-2, IFN-γ, and TNF-α levels (Huang et al., 2020). Therefore, RSV may have similar effects as an inhibitor of HSP90. HSP90 may be the target of RSV, which can inhibit signal transduction pathways, reduce cytokine levels and play the role of anti-fibrosis, so as to achieve the purpose of treating ILD. However, there is still a lack of relevant research, and more studies are expected to further confirm. In conclusion, RSV inhibits different signaling pathways and immune cells, and therefore has therapeutic potential for treating ILD.
Potential adverse reactions to RSV
In general, RSV ingestion is generally well tolerated. In animal models, RSV has been shown to be non-irritating and genotoxic to the skin and eyes. After 90 days of subchronic toxicity test, RSV was found to have no adverse effects on the body and no reproductive toxicity at the maximum dose of 700 mg/(kgd) (Williams et al., 2009). This preliminarily proves that RSV is non-toxic and safe. However, some studies have found that RSV has certain side effects. When rats were treated with 1.0 and 3.0 g/(kgd) RSV, different degrees of dehydration, dyspnea, nephrotoxicity and elevated serum liver enzymes were observed in female and male rats (Shaito et al., 2020). This indicates that high dose RSV has certain toxicity (Hebbar et al., 2005). When administered continuously for 4 weeks at doses of 0, 300, 1,000, and 3,000 mg/kg/day, no adverse reactions occurred at doses up to 300 mg/kg/day, whereas 1,000 and 3,000 mg/kg/day caused renal toxicity (Crowell et al., 2004). When RSV was administered simultaneously to rats at (0.3, 1.0, or 3.0 g/kg/day), abnormal expression of liver genes was noted, possibly indicating liver injury (Hebbar et al., 2005). A significant increase in bilirubin levels was observed at 1,000 (mg/kg)/day RSV in rats, but no adverse effects were observed at 200 mg/(kgd) in rats and 600 mg/(kgd) in dogs (Johnson et al., 2011). The toxicity of RSV to target organs remains to be further studied.
In human subjects, a daily RSV dose of 450 mg has been reported to be safe for a 60 kg person (Moon et al., 2004). However, some adverse effects have been reported, and high dose RSV intake appears to have negative effects on metabolic status, endothelial health, inflammation, and cardiovascular markers in human patients (Ramírez-Garza et al., 2018). For example, higher doses of RSV (1,000 mg/day) have recently been shown to increase biomarkers of cardiovascular risk (e.g., oxidized LDL, soluble intercellular adhesion molecule-1, etc.), while lower doses have no effect on the same biomarkers (Mankowski et al., 2020). In a recent meta-analysis of 18 studies included, adverse reactions occurred in two studies (Zhang et al., 2021), one of which initially administered 500 mg, qd, followed by an increase of 500 mg daily every 3 days, with a maximum dose of 3,000 mg daily (1,000 mg, tid). Adverse events occurred in three of the five patients. In the RSV group, one patient with a history of fatty liver disease developed asymptomatic and mild elevated alanine aminotransferase, another patient developed diarrhea and mild hypoglycemia, and one patient developed mild cellulitis at the biopsy site (Goh et al., 2014). In another study, which administered RSV 500 mg once daily plus losartan 12.5 mg, only two patients (one RSV and one placebo) complained of side effects of gastritis, such as mild dyspepsia, throughout the study of 30 patients in the RSV and placebo groups (Sattarinezhad et al., 2019). Therefore, more in vivo studies involving animal models are necessary, and more clinical trials of RSV in humans are needed to verify its efficacy and safety, especially before it can be considered for therapeutic or prophylactic use in humans.
Conclusion
ILD is one of the most important factors that directly affect the quality of life of patients. Long-term use of immunosuppressant and antifibrotic drugs can lead to many inevitable side effects. In addition, current drug treatments are still insufficient to reduce ILD progression and mortality worldwide. Bioactive natural ingredients derived from natural herbs may provide additional benefits in the prevention and treatment of ILD and represent an important source of new drug screening and development.
RSV inhibits TGF-β/Smad, NF-κB, and JAK/STAT pathways and immune cells, reduces the levels of pro-inflammatory and pro-fibrotic cytokines, and has powerful anti-inflammatory and anti-fibrotic effects. Therefore, TGF-β/Smad, NF-κB, and JAK/STAT pathways are potential targets of RSV in the treatment of ILD. It is also a potential and beneficial candidate for combination with other clinical antifibrosis agents. In addition, Piceatannol (PIC), a derivative of RSV, promotes autophagy by inhibiting the TGF-β1-Smad3/ERK/P38 signaling pathway, resulting in a decrease in the number of activated myoblasts, significantly reducing BLM-induced collagen deposition and myoblast accumulation (Sheng et al., 2022; Tieyuan et al., 2022). It also indicated that the related derivatives of RSV and the extracted compounds may also be able to treat ILD. However, the oral bioavailability of RSV is very low, with a maximum oral bioavailability of only 20%, although the total absorption rate in the gut is as high as 70% (Walle et al., 2004). Oral and intravenous radioisotope labeling of 14C RSVS suggests that the stage of biotransformation is a rate-limiting factor in RSV bioavailability. Therefore, targeted delivery of RSV to desired tissues or increased stability of RSV in vivo through the development of slow release systems are critical for improving bioavailability (Santos et al., 2019; Thipe et al., 2019). In addition, RSVS have been reported to have synergistic therapeutic effects when combined with other bioactive ingredients and micronutrients, giving RSVS a more stable chemical structure, higher solubility, and easier absorption in the small intestine (Williamson and Manach, 2005).
The drug dosage of RSV in the treatment of ILD is still unclear, as the dosage used in different studies varies. If clinical studies of RSV dose gradient Settings exist, deeper mechanisms of therapeutic effect may be clearly understood. Future studies should further explore the effects of different drug doses of RSV on treatment and the deeper mechanism, and open a new window for the treatment of ILD. Although the results of most studies on polyphenols have proved promising, further research on animals and humans is warranted. By extensively evaluating the biological activity, efficacy, safety and appropriate dose of RSV, and determining specific molecular targets and structure-activity relationships, it provides a new way for clinicians to treat ILD.
Author contributions
RH and XH wrote the manuscript. YTY and YY collected the references. JL reviewed and revised the manuscript. All authors read and approved the final manuscript.
Conflict of interest
The authors declare that the research was conducted in the absence of any commercial or financial relationships that could be construed as a potential conflict of interest.
Publisher’s note
All claims expressed in this article are solely those of the authors and do not necessarily represent those of their affiliated organizations, or those of the publisher, the editors and the reviewers. Any product that may be evaluated in this article, or claim that may be made by its manufacturer, is not guaranteed or endorsed by the publisher.
References
Alesci, A., Nicosia, N., Fumia, A., Giorgianni, F., Santini, A., and Cicero, N. (2022). Resveratrol and immune cells: A link to improve human health. Molecules 27 (2), 424. doi:10.3390/molecules27020424
Aquino-Galvez, A., Pérez-Rodríguez, M., Camarena, A., Falfan-Valencia, R., Ruiz, V., Montaño, M., et al. (2009). MICA polymorphisms and decreased expression of the MICA receptor NKG2D contribute to idiopathic pulmonary fibrosis susceptibility. Hum. Genet. 125 (5-6), 639–648. doi:10.1007/s00439-009-0666-1
Atkins, S. R., Turesson, C., Myers, J. L., Tazelaar, H. D., Ryu, J. H., Matteson, E. L., et al. (2006). Morphologic and quantitative assessment of CD20+ B cell infiltrates in rheumatoid arthritis-associated nonspecific interstitial pneumonia and usual interstitial pneumonia. Arthritis Rheum. 54 (2), 635–641. doi:10.1002/art.21758
Bagnato, G., and Harari, S. (2015). Cellular interactions in the pathogenesis of interstitial lung diseases. Eur. Respir. Rev. 24 (135), 102–114. doi:10.1183/09059180.00003214
Balachandran, S., and Adams, G. P. (2013). Interferon-γ-induced necrosis: An antitumor biotherapeutic perspective. J. Interferon Cytokine Res. 33 (4), 171–180. doi:10.1089/jir.2012.0087
Banerjee, S., Biehl, A., Gadina, M., Hasni, S., and Schwartz, D. M. (2017). JAK-STAT signaling as a target for inflammatory and autoimmune diseases: Current and future prospects. Drugs 77 (5), 521–546. doi:10.1007/s40265-017-0701-9
Bao, L., Ye, J., Liu, N., Shao, Y., Li, W., Fan, X., et al. (2022). Resveratrol ameliorates fibrosis in rheumatoid arthritis-associated interstitial lung disease via the autophagy-lysosome pathway. Molecules 27 (23), 8475. doi:10.3390/molecules27238475
Bellamri, N., Morzadec, C., Joannes, A., Lecureur, V., Wollin, L., Jouneau, S., et al. (2019). Alteration of human macrophage phenotypes by the anti-fibrotic drug nintedanib. Int. Immunopharmacol. 72, 112–123. doi:10.1016/j.intimp.2019.03.061
Bellaye, P. S., Shimbori, C., Yanagihara, T., Carlson, D. A., Hughes, P., Upagupta, C., et al. (2018). Synergistic role of HSP90α and HSP90β to promote myofibroblast persistence in lung fibrosis. Eur. Respir. J. 51 (2), 1700386. doi:10.1183/13993003.00386-2017
Bohonowych, J. E., Hance, M. W., Nolan, K. D., Defee, M., Parsons, C. H., and Isaacs, J. S. (2014). Extracellular Hsp90 mediates an NF-κB dependent inflammatory stromal program: Implications for the prostate tumor microenvironment. Prostate 74 (4), 395–407. doi:10.1002/pros.22761
Camont, L., Cottart, C. H., Rhayem, Y., Nivet-Antoine, V., Djelidi, R., Collin, F., et al. (2009). Simple spectrophotometric assessment of the trans-/cis-resveratrol ratio in aqueous solutions. Anal. Chim. Acta 634 (1), 121–128. doi:10.1016/j.aca.2008.12.003
Cardile, V., Chillemi, R., Lombardo, L., Sciuto, S., Spatafora, C., and Tringali, C. (2007). Antiproliferative activity of methylated analogues of E- and Z-resveratrol. Z Naturforsch C J. Biosci. 62 (3-4), 189–195. doi:10.1515/znc-2007-3-406
Celada, L. J., Kropski, J. A., Herazo-Maya, J. D., Luo, W., Creecy, A., Abad, A. T., et al. (2018). PD-1 up-regulation on CD4+ T cells promotes pulmonary fibrosis through STAT3-mediated IL-17A and TGF-β1 production. Sci. Transl. Med. 10 (460), eaar8356. doi:10.1126/scitranslmed.aar8356
Chambers, R. C., and Mercer, P. F. (2015). Mechanisms of alveolar epithelial injury, repair, and fibrosis. Ann. Am. Thorac. Soc. 12 (1), S16–S20. doi:10.1513/AnnalsATS.201410-448MG
Chávez, E., Reyes-Gordillo, K., Segovia, J., Shibayama, M., Tsutsumi, V., Vergara, P., et al. (2008). Resveratrol prevents fibrosis, NF-kappaB activation and TGF-beta increases induced by chronic CCl4 treatment in rats. J. Appl. Toxicol. 28 (1), 35–43. doi:10.1002/jat.1249
Chen, H., Chen, Q., Jiang, C. M., Shi, G. Y., Sui, B. W., Zhang, W., et al. (2018). Triptolide suppresses paraquat induced idiopathic pulmonary fibrosis by inhibiting TGFB1-dependent epithelial mesenchymal transition. Toxicol. Lett. 284, 1–9. doi:10.1016/j.toxlet.2017.11.030
Chen, H., He, A., Li, H., Chen, H., Xie, H., Luo, L., et al. (2021). TSSK4 upregulation in alveolar epithelial type-II cells facilitates pulmonary fibrosis through HSP90-AKT signaling restriction and AT-II apoptosis. Cell Death Dis. 12 (10), 938. doi:10.1038/s41419-021-04232-3
Chen, J., Song, S., Liu, Y., Liu, D., Lin, Y., Ge, S., et al. (2018). Autoreactive T cells to citrullinated HSP90 are associated with interstitial lung disease in rheumatoid arthritis. Int. J. Rheum. Dis. 21 (7), 1398–1405. doi:10.1111/1756-185X.13316
Chen, L., and Musa, A. E. (2021). Boosting immune system against cancer by resveratrol. Phytother. Res. 35 (10), 5514–5526. doi:10.1002/ptr.7189
Chen, X., He, H., Wang, G., Yang, B., and Ren, W. (2007). Stereospecific determination of cis- and trans-resveratrol in rat plasma by HPLC: Application to pharmacokinetic studies. Biomed. Chromatogr. 21 (3), 257–265. doi:10.1002/bmc.747
Chen, X., Lu, J., An, M., Ma, Z., Zong, H., and Yang, J. (2014). Anti-inflammatory effect of resveratrol on adjuvant arthritis rats with abnormal immunological function via the reduction of cyclooxygenase-2 and prostaglandin E2. Mol. Med. Rep. 9 (6), 2592–2598. doi:10.3892/mmr.2014.2070
Chua, H. L., Bhat-Nakshatri, P., Clare, S. E., MorimiyA, A., Badve, S., and NaksHatri, H. (2007). NF-kappaB represses E-cadherin expression and enhances epithelial to mesenchymal transition of mammary epithelial cells: Potential involvement of ZEB-1 and ZEB-2. Oncogene 26 (5), 711–724. doi:10.1038/sj.onc.1209808
Colunga Biancatelli, R. M. L., Solopov, P., Gregory, B., and Catravas, J. D. (2020). HSP90 inhibition and modulation of the proteome: Therapeutical implications for idiopathic pulmonary fibrosis (IPF). Int. J. Mol. Sci. 21 (15), 5286. doi:10.3390/ijms21155286
Crowell, J. A., Korytko, P. J., Morrissey, R. L., Booth, T. D., and Levine, B. S. (2004). Resveratrol-associated renal toxicity. Toxicol. Sci. 82 (2), 614–619. doi:10.1093/toxsci/kfh263
Cruz, T., Jia, M., Sembrat, J., Tabib, T., Agostino, N., Bruno, T. C., et al. (2021). Reduced proportion and activity of natural killer cells in the lung of patients with idiopathic pulmonary fibrosis. Am. J. Respir. Crit. Care Med. 204 (5), 608–610. doi:10.1164/rccm.202012-4418LE
Culley, F. J. (2009). Natural killer cells in infection and inflammation of the lung. Immunology 128 (2), 151–163. doi:10.1111/j.1365-2567.2009.03167.x
Delmas, D., and Lin, H. Y. (2011). Role of membrane dynamics processes and exogenous molecules in cellular resveratrol uptake: Consequences in bioavailability and activities. Mol. Nutr. Food Res. 55 (8), 1142–1153. doi:10.1002/mnfr.201100065
Deng, L., Huang, T., and Zhang, L. (2023). T cells in idiopathic pulmonary fibrosis: Crucial but controversial. Cell Death Discov. 9 (1), 62. doi:10.1038/s41420-023-01344-x
Desai, O., Winkler, J., Minasyan, M., and Herzog, E. L. (2018). The role of immune and inflammatory cells in idiopathic pulmonary fibrosis. Front. Med. (Lausanne) 5, 43. doi:10.3389/fmed.2018.00043
Ding, S., Wang, H., Wang, M., Bai, L., Yu, P., and Wu, W. (2019). Resveratrol alleviates chronic "real-world" ambient particulate matter-induced lung inflammation and fibrosis by inhibiting NLRP3 inflammasome activation in mice. Ecotoxicol. Environ. Saf. 182, 109425. doi:10.1016/j.ecoenv.2019.109425
Duchemann, B., Annesi-Maesano, I., Jacobe de Naurois, C., Sanyal, S., Brillet, P. Y., Brauner, M., et al. (2017). Prevalence and incidence of interstitial lung diseases in a multi-ethnic county of Greater Paris. Eur. Respir. J. 50(2), 1602419. doi:10.1183/13993003.02419-2016
Erazo, T., Moreno, A., Ruiz-Babot, G., Rodríguez-Asiain, A., Morrice, N. A., Espadamala, J., et al. (2013). Canonical and kinase activity-independent mechanisms for extracellular signal-regulated kinase 5 (ERK5) nuclear translocation require dissociation of Hsp90 from the ERK5-Cdc37 complex. Mol. Cell Biol. 33 (8), 1671–1686. doi:10.1128/MCB.01246-12
Esposito, I., Perna, F., Ponticiello, A., Gilli, M., and Sanduzzi, A. (2005). Natural killer cells in Bal and peripheral blood of patients with idiopathic pulmonary fibrosis (IPF). Int. J. Immunopathol. Pharmacol. 18 (3), 541–545. doi:10.1177/039463200501800314
Fagone, E., Conte, E., Gili, E., Fruciano, M., Pistorio, M. P., Lo Furno, D., et al. (2011). Resveratrol inhibits transforming growth factor-β-induced proliferation and differentiation of ex vivo human lung fibroblasts into myofibroblasts through ERK/Akt inhibition and PTEN restoration. Exp. Lung Res. 37 (3), 162–174. doi:10.3109/01902148.2010.524722
Feng, X. H., and Derynck, R. (2005). Specificity and versatility in tgf-beta signaling through Smads. Annu. Rev. Cell Dev. Biol. 21, 659–693. doi:10.1146/annurev.cellbio.21.022404.142018
Flament, T., Bigot, A., Chaigne, B., Henique, H., Diot, E., and Marchand-Adam, S. (2016). Pulmonary manifestations of Sjögren's syndrome. Eur. Respir. Rev. 25 (140), 110–123. doi:10.1183/16000617.0011-2016
Fu, Y., Yan, M., Xie, C., Hu, J., Zeng, X., and Hu, Q. (2020). Polydatin relieves paraquat-induced human MRC-5 fibroblast injury through inhibiting the activation of the NLRP3 inflammasome. Ann. Transl. Med. 8 (12), 765. doi:10.21037/atm-20-4570
Goh, K. P., Lee, H. Y., Lau, D. P., Supaat, W., Chan, Y. H., and Koh, A. F. Y. (2014). Effects of resveratrol in patients with type 2 diabetes mellitus on skeletal muscle SIRT1 expression and energy expenditure. Int. J. Sport Nutr. Exerc Metab. 24 (1), 2–13. doi:10.1123/ijsnem.2013-0045
Gouda, M. M., and Bhandary, Y. P. (2018). Curcumin down-regulates IL-17A mediated p53-fibrinolytic system in bleomycin induced acute lung injury in vivo. J. Cell Biochem. 119 (9), 7285–7299. doi:10.1002/jcb.27026
Hebbar, V., Shen, G., Hu, R., Kim, B. R., Chen, C., Korytko, P. J., et al. (2005). Toxicogenomics of resveratrol in rat liver. Life Sci. 76 (20), 2299–2314. doi:10.1016/j.lfs.2004.10.039
Heukels, P., van Hulst, J. A. C., van Nimwegen, M., Boorsma, C. E., Melgert, B. N., von der Thusen, J. H., et al. (2019). Enhanced Bruton's tyrosine kinase in B-cells and autoreactive IgA in patients with idiopathic pulmonary fibrosis. Respir. Res. 20 (1), 232. doi:10.1186/s12931-019-1195-7
Ho, Y., Li, Z. L., Shih, Y. J., Chen, Y. R., Wang, K., Whang-Peng, J., et al. (2020). Integrin αvβ3 in the mediating effects of dihydrotestosterone and resveratrol on breast cancer cell proliferation. Int. J. Mol. Sci. 21 (8), 2906. doi:10.3390/ijms21082906
Huang, H., Liao, D., Zhou, G., Zhu, Z., Cui, Y., and Pu, R. (2020). Antiviral activities of resveratrol against rotavirus in vitro and in vivo. Phytomedicine 77, 153230. doi:10.1016/j.phymed.2020.153230
Huang, Y., Oldham, J. M., Ma, S. F., Unterman, A., Liao, S. Y., Barros, A. J., et al. (2021). Blood transcriptomics predicts progression of pulmonary fibrosis and associated natural killer cells. Am. J. Respir. Crit. Care Med. 204 (2), 197–208. doi:10.1164/rccm.202008-3093OC
Huo, R., Guo, Q., Hu, J., Li, N., Gao, R., Mi, L., et al. (2022). Therapeutic potential of janus kinase inhibitors for the management of interstitial lung disease. Drug Des. Devel Ther. 16, 991–998. doi:10.2147/DDDT.S353494
Johnson, W. D., Morrissey, R. L., Usborne, A. L., KapetanovIc, I., Crowell, J. A., Muzzio, M., et al. (2011). Subchronic oral toxicity and cardiovascular safety pharmacology studies of resveratrol, a naturally occurring polyphenol with cancer preventive activity. Food Chem. Toxicol. 49 (12), 3319–3327. doi:10.1016/j.fct.2011.08.023
Katsumata, M., Hozumi, H., Yasui, H., Suzuki, Y., Kono, M., Karayama, M., et al. (2019). Frequency and clinical relevance of anti-cyclic citrullinated peptide antibody in idiopathic interstitial pneumonias. Respir. Med. 154, 102–108. doi:10.1016/j.rmed.2019.06.016
Kishore, A., and Petrek, M. (2021). Roles of macrophage polarization and macrophage-derived miRNAs in pulmonary fibrosis. Front. Immunol. 12, 678457. doi:10.3389/fimmu.2021.678457
Kjær, T. N., Thorsen, K., Jessen, N., Stenderup, K., and Pedersen, S. B. (2015). Resveratrol ameliorates imiquimod-induced psoriasis-like skin inflammation in mice. PLoS One 10 (5), e0126599. doi:10.1371/journal.pone.0126599
Koh, R. Y., Lim, C. L., Uhal, B. D., Abdullah, M., Vidyadaran, S., Ho, C. C., et al. (2015). Inhibition of transforming growth factor-β via the activin receptor-like kinase-5 inhibitor attenuates pulmonary fibrosis. Mol. Med. Rep. 11 (5), 3808–3813. doi:10.3892/mmr.2015.3193
Kolahian, S., Fernandez, I. E., Eickelberg, O., and Hartl, D. (2016). Immune mechanisms in pulmonary fibrosis. Am. J. Respir. Cell Mol. Biol. 55 (3), 309–322. doi:10.1165/rcmb.2016-0121TR
Krzewski, K., and Strominger, J. L. (2008). The killer's kiss: The many functions of NK cell immunological synapses. Curr. Opin. Cell Biol. 20 (5), 597–605. doi:10.1016/j.ceb.2008.05.006
Kuwana, M., Gil-Vila, A., and Selva-O'Callaghan, A. (2021). Role of autoantibodies in the diagnosis and prognosis of interstitial lung disease in autoimmune rheumatic disorders. Ther. Adv. Musculoskelet. Dis. 13, 1759720X211032457. doi:10.1177/1759720X211032457
Lai, N. L., Jia, W., Wang, X., Luo, J., Liu, G. Y., Gao, C., et al. (2019). Risk factors and changes of peripheral NK and T cells in pulmonary interstitial fibrosis of patients with rheumatoid arthritis. Can. Respir. J. 2019, 7262065. doi:10.1155/2019/7262065
Lee, J. S., Kim, E. J., Lynch, K. L., Elicker, B., Ryerson, C. J., Katsumoto, T. R., et al. (2013). Prevalence and clinical significance of circulating autoantibodies in idiopathic pulmonary fibrosis. Respir. Med. 107 (2), 249–255. doi:10.1016/j.rmed.2012.10.018
Lescoat, A., Lelong, M., Jeljeli, M., Piquet-Pellorce, C., Morzadec, C., Ballerie, A., et al. (2020). Combined anti-fibrotic and anti-inflammatory properties of JAK-inhibitors on macrophages in vitro and in vivo: Perspectives for scleroderma-associated interstitial lung disease. Biochem. Pharmacol. 178, 114103. doi:10.1016/j.bcp.2020.114103
Li, J., Liang, C., Zhang, Z. K., Pan, X., Peng, S., Lee, W. S., et al. (2017). TAK1 inhibition attenuates both inflammation and fibrosis in experimental pneumoconiosis. Cell Discov. 3, 17023. doi:10.1038/celldisc.2017.23
Liu, G., Friggeri, A., Yang, Y., Milosevic, J., Ding, Q., Thannickal, V. J., et al. (2010). miR-21 mediates fibrogenic activation of pulmonary fibroblasts and lung fibrosis. J. Exp. Med. 207 (8), 1589–1597. doi:10.1084/jem.20100035
Liu, L. L., He, J. H., Xie, H. B., Yang, Y. S., Li, J. C., and Zou, Y. (2014). Resveratrol induces antioxidant and heat shock protein mRNA expression in response to heat stress in black-boned chickens. Poult. Sci. 93 (1), 54–62. doi:10.3382/ps.2013-03423
Liu, M., Zeng, X., Wang, J., Fu, Z., and Liu, M. (2016). Immunomodulation by mesenchymal stem cells in treating human autoimmune disease-associated lung fibrosis. Stem Cell Res. Ther. 7 (1), 63. doi:10.1186/s13287-016-0319-y
Liu, Y. L., Chen, B. Y., Nie, J., Zhao, G. H., Zhuo, J. Y., Yuan, J., et al. (2020). Polydatin prevents bleomycin-induced pulmonary fibrosis by inhibiting the TGF-β/Smad/ERK signaling pathway. Exp. Ther. Med. 20 (5), 62. doi:10.3892/etm.2020.9190
Lynde, C. W., Poulin, Y., Vender, R., Bourcier, M., and Khalil, S. (2014). Interleukin 17A: Toward a new understanding of psoriasis pathogenesis. J. Am. Acad. Dermatol 71 (1), 141–150. doi:10.1016/j.jaad.2013.12.036
Ma, B. N., and Li, X. J. (2020). Resveratrol extracted from Chinese herbal medicines: A novel therapeutic strategy for lung diseases. Chin. Herb. Med. 12 (4), 349–358. doi:10.1016/j.chmed.2020.07.003
Malaguarnera, L. (2019). Influence of resveratrol on the immune response. Nutrients 11 (5), 946. doi:10.3390/nu11050946
Mankowski, R. T., You, L., Buford, T. W., Leeuwenburgh, C., Manini, T. M., Schneider, S., et al. (2020). Higher dose of resveratrol elevated cardiovascular disease risk biomarker levels in overweight older adults - a pilot study. Exp. Gerontol. 131, 110821. doi:10.1016/j.exger.2019.110821
Martín, A. R., Villegas, I., La Casa, C., and de la Lastra, C. A. (2004). Resveratrol, a polyphenol found in grapes, suppresses oxidative damage and stimulates apoptosis during early colonic inflammation in rats. Biochem. Pharmacol. 67 (7), 1399–1410. doi:10.1016/j.bcp.2003.12.024
Mikolasch, T. A., Garthwaite, H. S., and Porter, J. C. (2017). Update in diagnosis and management of interstitial lung disease. Clin. Med. (Lond). 17 (2), 146–153. doi:10.7861/clinmedicine.17-2-146
Montero, P., Milara, J., Roger, I., and Cortijo, J. (2021). Role of JAK/STAT in interstitial lung diseases; molecular and cellular mechanisms. Int. J. Mol. Sci. 22 (12), 6211. doi:10.3390/ijms22126211
Moon, R. T., Kohn, A. D., De Ferrari, G. V., and Kaykas, A. (2004). WNT and beta-catenin signalling: Diseases and therapies. Nat. Rev. Genet. 5 (9), 691–701. doi:10.1038/nrg1427
Morrow, L. E., Hilleman, D., and Malesker, M. A. (2022). Management of patients with fibrosing interstitial lung diseases. Am. J. Health Syst. Pharm. 79 (3), 129–139. doi:10.1093/ajhp/zxab375
Neys, S. F. H., Heukels, P., van Hulst, J. A. C., Rip, J., Wijsenbeek, M. S., Hendriks, R. W., et al. (2021). Aberrant B cell receptor signaling in naïve B cells from patients with idiopathic pulmonary fibrosis. Cells 10 (6), 1321. doi:10.3390/cells10061321
Oliveira, A. L. B., Monteiro, V. V. S., Navegantes-Lima, K. C., Reis, J. F., Gomes, R. d. S., Rodrigues, D. V. S., et al. (2017). Resveratrol role in autoimmune disease-A mini-review. Nutrients 9 (12), 1306. doi:10.3390/nu9121306
Pardo, A., and Selman, M. (2002). Idiopathic pulmonary fibrosis: New insights in its pathogenesis. Int. J. Biochem. Cell Biol. 34 (12), 1534–1538. doi:10.1016/s1357-2725(02)00091-2
Poles, J., Karhu, E., McGill, M., McDaniel, H. R., and Lewis, J. E. (2021). The effects of twenty-four nutrients and phytonutrients on immune system function and inflammation: A narrative review. J. Clin. Transl. Res. 7 (3), 333–376.
Raj, P., Thandapilly, S. J., Wigle, J., Zieroth, S., and Netticadan, T. (2021). A comprehensive analysis of the efficacy of resveratrol in atherosclerotic cardiovascular disease, myocardial infarction and heart failure. Molecules 26 (21), 6600. doi:10.3390/molecules26216600
Ramírez-Garza, S. L., Laveriano-Santos, E. P., Marhuenda-Muñoz, M., Storniolo, C. E., Tresserra-Rimbau, A., Vallverdu-Queralt, A., et al. (2018). Health effects of resveratrol: Results from human intervention trials. Nutrients 10 (12), 1892. doi:10.3390/nu10121892
Riveiro-Naveira, R. R., Valcárcel-Ares, M. N., Almonte-Becerril, M., Vaamonde-Garcia, C., Loureiro, J., Hermida-Carballo, L., et al. (2016). Resveratrol lowers synovial hyperplasia, inflammatory markers and oxidative damage in an acute antigen-induced arthritis model. Rheumatol. Oxf. 55 (10), 1889–1900. doi:10.1093/rheumatology/kew255
Roberts, A. B., Russo, A., Felici, A., and Flanders, K. C. (2003). Smad3: A key player in pathogenetic mechanisms dependent on TGF-beta. Ann. N. Y. Acad. Sci. 995, 1–10. doi:10.1111/j.1749-6632.2003.tb03205.x
Roman, J., and Chiba, H. (2021). B cells in idiopathic pulmonary fibrosis: Targeting immune cells with antifibrotic agents. Am. J. Respir. Cell Mol. Biol. 64 (6), 652–654. doi:10.1165/rcmb.2021-0101ED
Rong, L., Wu, J., Wang, W., Zhao, R. P., Xu, X. W., and Hu, D. (2016). Sirt 1 activator attenuates the bleomycin-induced lung fibrosis in mice via inhibiting epithelial-to-mesenchymal transition (EMT). Eur. Rev. Med. Pharmacol. Sci. 20 (10), 2144–2150.
Sánchez-Fidalgo, S., Cárdeno, A., Villegas, I., Talero, E., and de la Lastra, C. A. (2010). Dietary supplementation of resveratrol attenuates chronic colonic inflammation in mice. Eur. J. Pharmacol. 633 (1-3), 78–84. doi:10.1016/j.ejphar.2010.01.025
Santos, A. C., Pereira, I., Magalhães, M., Pereira-Silva, M., Caldas, M., Ferreira, L., et al. (2019). Targeting cancer via resveratrol-loaded nanoparticles administration: Focusing on in vivo evidence. AAPS J. 21 (4), 57. doi:10.1208/s12248-019-0325-y
Sattarinezhad, A., Roozbeh, J., Shirazi Yeganeh, B., and ShaMs, M. (2019). Resveratrol reduces albuminuria in diabetic nephropathy: A randomized double-blind placebo-controlled clinical trial. Diabetes Metab. 45 (1), 53–59. doi:10.1016/j.diabet.2018.05.010
Schumacher, J. A., Crockett, D. K., Elenitoba-Johnson, K. S., and Lim, M. S. (2007). Proteome-wide changes induced by the Hsp90 inhibitor, geldanamycin in anaplastic large cell lymphoma cells. Proteomics 7 (15), 2603–2616. doi:10.1002/pmic.200700108
Sendo, S., Saegusa, J., Yamada, H., Nishimura, K., and Morinobu, A. (2019). Tofacitinib facilitates the expansion of myeloid-derived suppressor cells and ameliorates interstitial lung disease in SKG mice. Arthritis Res. Ther. 21 (1), 184. doi:10.1186/s13075-019-1963-2
Shaito, A., Posadino, A. M., Younes, N., Hasan, H., Halabi, S., Alhababi, D., et al. (2020). Potential adverse effects of resveratrol: A literature review. Int. J. Mol. Sci. 21 (6), 2084. doi:10.3390/ijms21062084
Shao, T., Shi, X., Yang, S., Zhang, W., Li, X., Shu, J., et al. (2021). Interstitial lung disease in connective tissue disease: A common lesion with heterogeneous mechanisms and treatment considerations. Front. Immunol. 12, 684699. doi:10.3389/fimmu.2021.684699
Sheng, H., Lin, G., Zhao, S., Li, W., Zhang, Z., Zhang, W., et al. (2022). Antifibrotic mechanism of Piceatannol in bleomycin-induced pulmonary fibrosis in mice. Front. Pharmacol. 13, 771031. doi:10.3389/fphar.2022.771031
Shimizu, T., Nagafuchi, Y., Harada, H., Tsuchida, Y., Tsuchiya, H., Hanata, N., et al. (2021). Decreased peripheral blood memory B cells are associated with the presence of interstitial lung disease in rheumatoid arthritis: A case-control study. Mod. Rheumatol. 31 (1), 127–132. doi:10.1080/14397595.2020.1719596
Sontake, V., Wang, Y., Kasam, R. K., Sinner, D., Reddy, G. B., Naren, A. P., et al. (2017). Hsp90 regulation of fibroblast activation in pulmonary fibrosis. JCI Insight 2 (4), e91454. doi:10.1172/jci.insight.91454
Štorkánová, H., Oreská, S., Špiritović, M., Heřmánková, B., Bubová, K., Komarc, M., et al. (2021). Plasma Hsp90 levels in patients with systemic sclerosis and relation to lung and skin involvement: A cross-sectional and longitudinal study. Sci. Rep. 11 (1), 1. doi:10.1038/s41598-020-79139-8
Thipe, V. C., Panjtan Amiri, K., Bloebaum, P., Raphael Karikachery, A., Khoobchandani, M., Katti, K. K., et al. (2019). Development of resveratrol-conjugated gold nanoparticles: Interrelationship of increased resveratrol corona on anti-tumor efficacy against breast, pancreatic and prostate cancers. Int. J. Nanomedicine 14, 4413–4428. doi:10.2147/IJN.S204443
Tian, R., Zhu, Y., Yao, J., Meng, X., Wang, J., Xie, H., et al. (2017). NLRP3 participates in the regulation of EMT in bleomycin-induced pulmonary fibrosis. Exp. Cell Res. 357 (2), 328–334. doi:10.1016/j.yexcr.2017.05.028
Tieyuan, Z., Ying, Z., Xinghua, Z., Huimin, W., and Huagang, L. (2022). Piceatannol-mediated JAK2/STAT3 signaling pathway inhibition contributes to the alleviation of oxidative injury and collagen synthesis during pulmonary fibrosis. Int. Immunopharmacol. 111, 109107. doi:10.1016/j.intimp.2022.109107
Truong, V. L., Jun, M., and Jeong, W. S. (2018). Role of resveratrol in regulation of cellular defense systems against oxidative stress. Biofactors 44 (1), 36–49. doi:10.1002/biof.1399
Tsai, M. H., Hsu, L. F., Lee, C. W., Chiang, Y. C., Lee, M. H., How, J. M., et al. (2017). Resveratrol inhibits urban particulate matter-induced COX-2/PGE2 release in human fibroblast-like synoviocytes via the inhibition of activation of NADPH oxidase/ROS/NF-κB. Int. J. Biochem. Cell Biol. 88, 113–123. doi:10.1016/j.biocel.2017.05.015
Turesson, C., Matteson, E. L., Colby, T. V., Vuk-Pavlovic, Z., Vassallo, R., Weyand, C. M., et al. (2005). Increased CD4+ T cell infiltrates in rheumatoid arthritis-associated interstitial pneumonitis compared with idiopathic interstitial pneumonitis. Arthritis Rheum. 52 (1), 73–79. doi:10.1002/art.20765
Tzavlaki, K., and Moustakas, A. (2020). TGF-Β signaling. Biomolecules 10 (3), 487. doi:10.3390/biom10030487
Van Dyken, S. J., and Locksley, R. M. (2013). Interleukin-4- and interleukin-13-mediated alternatively activated macrophages: Roles in homeostasis and disease. Annu. Rev. Immunol. 31, 317–343. doi:10.1146/annurev-immunol-032712-095906
Vargas, J. E., Souto, A. A., Pitrez, P. M., Stein, R. T., and Porto, B. N. (2016). Modulatory potential of resveratrol during lung inflammatory disease. Med. Hypotheses 96, 61–65. doi:10.1016/j.mehy.2016.09.023
Walle, T., Hsieh, F., DeLegge, M. H., Oatis, J. E., and Walle, U. K. (2004). High absorption but very low bioavailability of oral resveratrol in humans. Drug Metab. Dispos. 32 (12), 1377–1382. doi:10.1124/dmd.104.000885
Wang, D., Zhang, J., Lau, J., Wang, S., Taneja, V., Matteson, E. L., et al. (2019). Mechanisms of lung disease development in rheumatoid arthritis. Nat. Rev. Rheumatol. 15 (10), 581–596. doi:10.1038/s41584-019-0275-x
Wang, J., He, F., Chen, L., Li, Q., Jin, S., Zheng, H., et al. (2018). Resveratrol inhibits pulmonary fibrosis by regulating miR-21 through MAPK/AP-1 pathways. Biomed. Pharmacother. 105, 37–44. doi:10.1016/j.biopha.2018.05.104
Wang, J., Xu, L., Xiang, Z., Ren, Y., Zheng, X., Zhao, Q., et al. (2020). Macrophages promote epithelial proliferation following infectious and non-infectious lung injury through a Trefoil factor 2-dependent mechanism. Cell Death Dis. 11 (2), 136. doi:10.1038/s41419-020-2329-z
Wang, L., Shao, M., Jiang, W., and Huang, Y. (2022). Resveratrol alleviates bleomycin-induced pulmonary fibrosis by inhibiting epithelial-mesenchymal transition and down-regulating TLR4/NF-κB and TGF-β1/smad3 signalling pathways in rats. Tissue Cell 79, 101953. doi:10.1016/j.tice.2022.101953
Wang, Z., Li, X., Chen, H., Han, L., Ji, X., Wang, Q., et al. (2021). Resveratrol alleviates bleomycin-induced pulmonary fibrosis via suppressing HIF-1α and NF-κB expression. Aging (Albany NY) 13 (3), 4605–4616. doi:10.18632/aging.202420
Wang, Z. L., Luo, X. F., Li, M. T., Xu, D., Zhou, S., Chen, H. Z., et al. (2014). Resveratrol possesses protective effects in a pristane-induced lupus mouse model. PLoS One 9 (12), e114792. doi:10.1371/journal.pone.0114792
Weiskirchen, S., and Weiskirchen, R. (2016). Resveratrol: How much wine do you have to drink to stay healthy? Adv. Nutr. 7 (4), 706–718. doi:10.3945/an.115.011627
Williams, L. D., Burdock, G. A., and Edwards, J. A. (2009). Safety studies conducted on high-purity trans-resveratrol in experimental animals. Food Chem. Toxicol. 47 (9), 2170–2182. doi:10.1016/j.fct.2009.06.002
Williamson, G., and Manach, C. (2005). Bioavailability and bioefficacy of polyphenols in humans. II. Review of 93 intervention studies. Am. J. Clin. Nutr. 81, 243S–255S. doi:10.1093/ajcn/81.1.243S
Wills-Karp, M., and Finkelman, F. D. (2008). Untangling the complex web of IL-4- and IL-13-mediated signaling pathways. Sci. Signal 1 (51), pe55. doi:10.1126/scisignal.1.51.pe55
Wynn, T. A. (2008). Cellular and molecular mechanisms of fibrosis. J. Pathol. 214 (2), 199–210. doi:10.1002/path.2277
Xue, J., Kass, D. J., Bon, J., Vuga, L., Tan, J., Csizmadia, E., et al. (2013). Plasma B lymphocyte stimulator and B cell differentiation in idiopathic pulmonary fibrosis patients. J. Immunol. 191 (5), 2089–2095. doi:10.4049/jimmunol.1203476
Yahfoufi, N., Alsadi, N., Jambi, M., and Matar, C. (2018). The immunomodulatory and anti-inflammatory role of polyphenols. Nutrients 10 (11), 1618. doi:10.3390/nu10111618
Yamamoto, Y., and Gaynor, R. B. (2001). Therapeutic potential of inhibition of the NF-kappaB pathway in the treatment of inflammation and cancer. J. Clin. Invest. 107 (2), 135–142. doi:10.1172/JCI11914
Yang, C. M., Chen, Y. W., Chi, P. L., Lin, C. C., and Hsiao, L. D. (2017). Resveratrol inhibits BK-induced COX-2 transcription by suppressing acetylation of AP-1 and NF-κB in human rheumatoid arthritis synovial fibroblasts. Biochem. Pharmacol. 132, 77–91. doi:10.1016/j.bcp.2017.03.003
Yang, G., Lyu, L., Wang, X., Bao, L., Lyu, B., and Lin, Z. (2019). Systemic treatment with resveratrol alleviates adjuvant arthritis-interstitial lung disease in rats via modulation of JAK/STAT/RANKL signaling pathway. Pulm. Pharmacol. Ther. 56, 69–74. doi:10.1016/j.pupt.2019.03.011
You, X. Y., Xue, Q., Fang, Y., Liu, Q., Zhang, C. F., Zhao, C., et al. (2015). Preventive effects of Ecliptae Herba extract and its component, ecliptasaponin A, on bleomycin-induced pulmonary fibrosis in mice. J. Ethnopharmacol. 175, 172–180. doi:10.1016/j.jep.2015.08.034
Zhang, J., Wang, D., Wang, L., Wang, S., Roden, A. C., Zhao, H., et al. (2019). Profibrotic effect of IL-17A and elevated IL-17RA in idiopathic pulmonary fibrosis and rheumatoid arthritis-associated lung disease support a direct role for IL-17A/IL-17RA in human fibrotic interstitial lung disease. Am. J. Physiol. Lung Cell Mol. Physiol. 316 (3), L487–L497. doi:10.1152/ajplung.00301.2018
Zhang, T., He, Q., Liu, Y., Chen, Z., and Hu, H. (2021). Efficacy and safety of resveratrol supplements on blood lipid and blood glucose control in patients with type 2 diabetes: A systematic review and meta-analysis of randomized controlled trials. Evid. Based Complement. Altern. Med. 2021, 5644171. doi:10.1155/2021/5644171
Keywords: interstitial lung disease, resveratrol, signal transduction pathway, immune cells, adverse reaction
Citation: Huo R, Huang X, Yang Y, Yang Y and Lin J (2023) Potential of resveratrol in the treatment of interstitial lung disease. Front. Pharmacol. 14:1139460. doi: 10.3389/fphar.2023.1139460
Received: 07 January 2023; Accepted: 09 March 2023;
Published: 06 April 2023.
Edited by:
Rishi Sharma, University of Missouri, United StatesReviewed by:
Pavel Solopov, Old Dominion University, United StatesAyaz Shahid, Western University of Health Sciences, United States
Copyright © 2023 Huo, Huang, Yang, Yang and Lin. This is an open-access article distributed under the terms of the Creative Commons Attribution License (CC BY). The use, distribution or reproduction in other forums is permitted, provided the original author(s) and the copyright owner(s) are credited and that the original publication in this journal is cited, in accordance with accepted academic practice. No use, distribution or reproduction is permitted which does not comply with these terms.
*Correspondence: Jinying Lin, amlueWluZ2xpbkBzaW5hLmNvbQ==
†These authors have contributed equally to this work