- 1Acupuncture and Tuina School, Chengdu University of Traditional Chinese Medicine, Chengdu, Sichuan, China
- 2Acupuncture and Chronobiology Key Laboratory of Sichuan Province, Chengdu, Sichuan, China
Ischemic stroke has been a prominent focus of scientific investigation owing to its high prevalence, complex pathogenesis, and difficulties in treatment. Mitochondria play an important role in cellular energy homeostasis and are involved in neuronal death following ischemic stroke. Hence, maintaining mitochondrial function is critical for neuronal survival and neurological improvement in ischemic stroke, and mitochondria are key therapeutic targets in cerebral stroke research. With the benefits of high efficacy, low cost, and high safety, traditional Chinese medicine (TCM) has great advantages in preventing and treating ischemic stroke. Accumulating studies have explored the effect of TCM in preventing and treating ischemic stroke from the perspective of regulating mitochondrial structure and function. In this review, we discuss the molecular mechanisms by which mitochondria are involved in ischemic stroke. Furthermore, we summarized the current advances in TCM in preventing and treating ischemic stroke by modulating mitochondria. We aimed to provide a new perspective and enlightenment for TCM in the prevention and treatment of ischemic stroke by modulating mitochondria.
1 Introduction
Stroke is a devastating disease with high disability and mortality rates worldwide (Ajoolabady et al., 2021). Approximately 7,95,000 people suffer from either new or recurrent strokes annually, increasing the economic burden on the family and society (Virani et al., 2020). Most strokes are ischemic, accounting for approximately 87% of all strokes. Ischemic stroke causes brain tissue necrosis due to narrowing or occlusion of the blood supply arteries (carotid and vertebral arteries) and insufficient blood supply to the brain (Shao et al., 2020).
In patients with ischemic stroke, a significant decline in focal cerebral blood flow causes glucose and oxygen deprivation (OGD). Mitochondrial dysfunction is an early and initiating event in OGD following ischemia. It is increasingly evident that it plays a critical role in the onset, development, and pathology of ischemic stroke (Song et al., 2022). During ischemic stroke, OGD causes adenosine triphosphate (ATP) consumption and Na+/K+ ATPase pump failure, resulting in neuronal membrane depolarization and excessive glutamate release. Excessive Ca2+ influx can cause reactive oxygen species (ROS) production and mitochondrial dysfunction, such as an imbalance in mitochondrial dynamics, mitochondrial-induced apoptosis, mitochondrial biogenesis dysfunction, and mitophagy over-activation. These cellular processes eventually lead to neuronal cell death.
The most effective treatment for acute ischemic stroke is reperfusion therapy, which aims to restore blood flow and oxygen levels before neuronal damage occurs. Tissue plasminogen activator (tPA) is the only thrombolytic agent approved by the U.S. Food and Drug Administration for patients with acute ischemic stroke (Alkahtani et al., 2023). However, the narrow treatment window and the risk of complications limit its clinical application (Zhang et al., 2022; Li et al., 2022c). Additionally, tPA can cause mitochondria to produce excessive ROS, exacerbating cell damage (Giorgi et al., 2018). New therapeutic agents are required to address the paucity of stroke management approaches. Increasing evidence suggests that maintaining mitochondrial function is critical for neuronal survival and neurological improvement in ischemic stroke, and mitochondria are the key therapeutic targets in cerebral stroke research (Andrabi et al., 2020; Zhou et al., 2021; Zhong et al., 2022). Therefore, a promising treatment option for ischemic stroke that targets mitochondria is needed.
Chinese herbs and acupuncture are essential components of traditional Chinese medicine (TCM). In clinical trials and basic research, Chinese herbs and acupuncture have demonstrated therapeutic effects in preventing and treating ischemic stroke (Zhang et al., 2021; Song et al., 2022). Further studies reported that Chinese herbs and acupuncture could prevent and relieve cerebral ischemia injury in vivo and in vitro and have neuroprotective effects by modulating the mitochondrial respiratory chain (Zhong et al., 2009), increasing mitochondrial biogenesis (Sun et al., 2021), inhibiting the mitochondrial apoptotic pathway (Bai et al., 2020) and attenuating excessive mitophagy (Ting et al., 2017). However, only a few studies have comprehensively reviewed these studies hindering the elucidation of the mechanism of action of TCM and the development of clinical applications. Moreover, the existing review, published 2 years ago, only summarized the effects of Chinese herbs on mitochondrial permeability transition pore (mPTP) overopening-induced ischemic neuron apoptosis. Nonetheless, the regulatory effects of TCM on mitochondria in the treatment of ischemic stroke are multifaceted and acupuncture, an integral aspect of TCM, appears to be overlooked in the treatment of ischemic stroke by restoring mitochondrial function.
Understanding the molecular mechanisms of the mitochondria involved in ischemic stroke is crucial to identify potential interventional targets. Thus, we first discuss the role of mitochondria in ischemic stroke. We subsequently summarized the recent advances in TCM in preventing and treating ischemic stroke by regulating mitochondria. We aimed to provide a new perspective and insight into the use of TCM in treating ischemic stroke by improving mitochondrial structure and function.
2 The role of mitochondria in ischemic stroke
2.1 Ischemic stroke cascade involves mitochondrial function and structure changes
2.1.1 Ischemic stroke cascade involves mitochondrial function changes
Mitochondria produce the majority of ATP via the mitochondrial respiratory chain and oxidative phosphorylation to meet the high-energy demands of neurons in the brain that are extremely sensitive to ischemia and hypoxia. Within minutes of the onset of cerebral ischemia, ATP depletion deactivates the Na+/K+ ATPase pump, causing excessive glutamate release into the extracellular fluid (Sarmah et al., 2020). Overactivation of glutamate receptors, such as N-methyl-D-aspartate-receptor, α-amino-3-hydroxy-5-methyl-4-isox-azolepropionic acid receptor, and kainic acid receptor, results in Ca2+ influx and accumulation into cells (Hu et al., 2018; Engin and Engin, 2021; Guo and Ma, 2021). A large Ca2+ influx leads to a series of events ranging from mPTP opening and dissipation of mitochondrial membrane potential (MMP) to the release of cytochrome c (Cyt-c) or apoptosis-inducing factor (AIF), thus activating effector caspases and eventually causing neuronal death (Anzell et al., 2018; Li et al., 2020). Concomitantly, decreased ATP depletes nicotinamide adenine dinucleotide (NAD+), and the reduced NAD+ drives mitochondria to the vicinity of the endoplasmic reticulum to form mitochondria-associated endoplasmic reticulum membranes (MAMs). Moreover, certain MAM-related proteins join mPTP to regulate its opening, an important marker of cerebral cell death during ischemia/reperfusion (I/R).
In addition to energy production, mitochondria are the primary producers of intracellular ROS and are sites of eukaryotic oxidative metabolism. Disrupting mitochondrial electron transport increases ROS generated during cerebral ischemia, particularly during reperfusion (He et al., 2020). Further, this excess ROS affects mitochondrial function and promotes neuroinflammation and neuronal apoptosis after oxygen-glucose deprivation/reoxygenation (OGD/R) (Yang et al., 2021).
2.1.2 Ischemic stroke cascade involves mitochondrial structure changes
In addition to the function of mitochondria, their structure also plays an important role in the pathophysiological process of ischemic stroke. Mitochondria are highly dynamic cellular organelles that can change the shape, size, position, and integrity of mitochondrial DNA (mtDNA) through highly coordinated fission, fusion, and transport to tactical locations. The imbalance of mitochondrial fission and fusion after stroke may increase mitochondrial fragmentation, cause aberrant mitochondrial morphology, and disrupt mitochondrial homeostasis, leading to mitochondrial dysfunction and ultimately triggering neuronal death (Li et al., 2022). Additionally, mutation of gene-encoded subunits in mtDNA results in increased ROS generation, which makes mtDNA more susceptible to mutations than nuclear DNA (Zhang et al., 2022). Researchers have reported that the frequency of mtDNA mutations was significantly higher in the brains of patients with ischemic stroke (Luan et al., 2021). In summary, the ischemic stroke cascade involves changes in mitochondrial function and structure, indicating that mitochondrial structure and function play a critical role in the pathogenesis of ischemic stroke.
2.2 Mitochondrial biogenesis in ischemic stroke
Mitochondrial biogenesis is a multifaceted process involving the coordinated regulation of mitochondrial and nuclear transcription factors. Peroxisome proliferator-activated receptor γ coactivator-1α (PGC-1α) is a major regulator of mitochondrial biogenesis. During ischemic stroke, PGC-1α is first activated by upstream AMP-activated protein kinase (AMPK) phosphorylation and sirtuin 1 (SIRT1) acetylation (Kaarniranta et al., 2018), which then interacts with downstream nuclear respiratory factor 1/2 (NRF1/2), taking part in the expression of nuclear and mitochondrial respiratory factors. The binding of NRF1 to the promoter of the mitochondrial transcription factor A (TFAM) gene is enhanced under oxidative stress. Activated TFAM promotes mtDNA copying, transcription, and related protein synthesis, ultimately inducing mitochondrial biogenesis (Ryoo and Kwak, 2018). Additionally, two mitochondrial proteins, uncoupling protein 2 and superoxide dismutase 2, both regulated by PGC1-α, play a pivotal role in counteracting the damaging effects elicited by excessive oxidative stress in ischemic stroke (Chen et al., 2011). Peroxisome proliferator-activated receptor gamma agonists can upregulate PGC-1α, NRF1, TFAM, and cytochrome c oxidase subunits I and IV and enhance mitochondrial biogenesis in ischemic stroke (Yang et al., 2018). This indicated that mitochondrial biogenesis exerted a protective effect by enhancing the signal transduction pathways upstream of mitochondrial biogenesis.
Generally, mitochondrial biogenesis plays an important role as an endogenous protective mechanism in ischemic stroke. Therefore, boosting the signal transduction pathways upstream of mitochondrial biogenesis, such as the PGC-1α signaling cascade, may become a novel therapeutic strategy against ischemic brain damage.
2.3 Mitochondrial dynamics in ischemic stroke
Mitochondrial dynamics include fission and fusion. Mitochondrial fission allows damaged mitochondria to separate, leading to their subsequent elimination by mitophagy. The production of one or more daughter mitochondria is highly dependent on dynamin-related protein 1 (Drp1). Mitochondrial fusion facilitates the complementation of neighboring mitochondria, enabling the survival of damaged mitochondria (Zhou et al., 2021). It is a two-step process that requires the fusion of outer and inner mitochondrial membranes, mediated by mitofusins-1/mitofusins-2 (Mfn1/2) and optic atrophy 1 (Opa1), respectively.
The interaction between calcium overload, ROS production, and mPTP increases mitochondrial fission and decreases mitochondrial fusion in ischemic stroke (Zhou et al., 2021). Although increased mitochondrial fission during hypoxia may increase mitochondrial energy production, which is beneficial for maintaining neural function after stroke (Quintana et al., 2019), inducing excessive mitochondrial fission is harmful to neurons (Zhang et al., 2020). Excessive mitochondrial fission affects intracellular calcium homeostasis, exacerbates excitotoxicity, and accelerates neuronal death after ischemic stroke (Zhou et al., 2021). Researchers have observed increased levels of Drp1 in mice subjected to cerebral ischemia and reperfusion injury. After the knockdown of Drp1, oxidative stress, mitochondrial ROS production, and infarct volume decrease, contributing to the survival of neurons in cerebral ischemia (He et al., 2020). Mitochondrial fusion can repair damaged mitochondria and produce additional energy by upregulating the activity of ATP synthase through mitochondrial cristae remodeling (Cohen and Tareste, 2018). The levels of mitochondrial fusion proteins, such as Mfn-1/Mfn-2 and Opa1, decrease after cerebral ischemia (Rutkai et al., 2019). However, hypoxia-induced apoptosis improved when Mfn-2 was restored (Zhou et al., 2022).
In summary, inhibiting excessive mitochondrial fission, promoting mitochondrial fusion, and restoring the balance of mitochondrial dynamics are beneficial for ischemic stroke recovery. Maintaining this balance can serve as a target for treating ischemic stroke.
2.4 Mitophagy in ischemic stroke
Mitophagy is a type of selective autophagy in which damaged or dysfunctional mitochondria are removed. In ischemic stroke, mitophagy could be predominantly mediated by the PINK1/Parkin pathway, Bcl-2/E1B-19 KD-interacting protein 3 (BNIP3), NIP3-like protein X (NIX, also known as BNIP3L), and FUN14 domain containing 1 (FUNDC1). Shen et al. demonstrated that mitophagy could protect brain cells from ischemic injury during the ischemic phase of stroke (Shen et al., 2021). In contrast, mitophagy serves as a double-edged sword when the brain suffers from reperfusion injury. Activating mitophagy to clear excessively aggregated and damaged mitochondria reduces neuronal damage caused by cerebral I/R injury (Li et al., 2018; Wang and Xu, 2020; Wu et al., 2021). However, some studies have shown that inhibiting excessive mitophagy can protect against cerebral I/R injury in middle cerebral artery occlusion (MCAO) rats (Lan et al., 2018; Jakic et al., 2019). Inhibition of excessive mitophagy could exert neuroprotective effects against neuronal death caused by chronic cerebral hypoperfusion (Su et al., 2018).
Mitophagy is important for the pathogenesis of cerebral I/R damage. Regulation of mitophagy could exert neuroprotective effects in ischemic stroke, although some issues regarding its role in ischemic stroke remain unclear. It would be meaningful to explore the role of mitophagy in treating I/R.
2.5 Proteins associated with mitochondria-dependent apoptosis in ischemic stroke
Apoptosis is a planned or controlled cell death triggered by mitochondrial malfunction through intrinsic and extrinsic pathways. Mitochondria are associated with many apoptosis-related proteins, suggesting that they are crucial for cell death following I/R (Yang et al., 2018). Many studies have revealed that B cell lymphoma (BCL-2) family proteins regulate neuronal death in cerebral ischemic stroke (Ader et al., 2019). After I/R, apoptotic members of the Bcl-2 protein family (e.g., Bax and Bak) are inserted into the outer mitochondrial membranes, and MMP is significantly downregulated. Another decisive step in the apoptotic cascade is related to the mPTP. Transient opening of the mPTP in the mitochondrial inner membrane after I/R causes MMP collapse. Several apoptosis-related proteins (e.g., AIF, Cyt-c, endonuclease G [Endo G], the second mitochondrion-derived activator of caspase/direct inhibitor of apoptosis-binding protein with low pI [Smac/Diablo]) originating in the mitochondria are released into the cytoplasmic matrix (Zhou et al., 2021). After migration to the cytoplasmic matrix, Cyt-c interacts with apoptosis-activating factor-1 (Apaf-1), deoxyadenosine triphosphate (dATP), and procaspase-9 to form the apoptosome, which then activates procaspase-9 and follows with caspase-9 to cleave and activates caspase-3 (Wang et al., 2020). Smac binds to and inhibits inhibitor-of-apoptosis proteins (IAPs), which normally inhibit procaspase activation and caspases activity (Zhao et al., 2020). AIF can trigger caspase-independent chromatin condensation and large-scale DNA breakage (Yang et al., 2017) and functions as a mitochondrial effector of apoptotic cell death following translocation from mitochondria to the nucleus (Guida et al., 2019).
Overall, modulating the expression of apoptotic members of the Bcl-2 protein family and preventing translocation of AIF, Cyt-c, and Smac from the mitochondria into the cytoplasmic matrix can serve as targets for the treatment of ischemic stroke.
3 Progress in ischemic stroke prevention and treatment using TCM that regulates mitochondria
Based on the above summary, we identified several targets for treating ischemic stroke from the mitochondrial perspective. In clinical and experimental studies, TCM has demonstrated significant efficacy in preventing and treating ischemic stroke. The mechanisms of action of TCM have also been gradually revealed in recent years. Many studies have revealed that TCM exerts therapeutic effects on ischemic stroke by regulating the mitochondria. Therefore, we summarized the literature on acupuncture, herbal extracts, effective TCM compounds, and TCM prescriptions in preventing and treating ischemic stroke and attempted to further clarify the molecular mechanisms of TCM in improving ischemic stroke from the perspective of regulating mitochondria.
3.1 Acupuncture and its molecular mechanisms for regulating mitochondria in ischemic stroke
3.1.1 Acupuncture pretreatment for regulating mitochondria in ischemic stroke
The MCAO group exhibited apparent mitochondrial structural abnormalities, including a reduction in mitochondrial volume and number, swelling, vacuolization, formation of autophagosomes and lysosomes, and broken/irregular/disappeared inner membranes and cristae. However, 5–7 consecutive days of electroacupuncture (EA) pretreatment reduced mitochondrial abnormalities, including an increase in mitochondrial volume and number (Sun et al., 2021), less swelling (Tian et al., 2022), a relatively integrated membrane and cristae (Zhang et al., 2018), and a reduction in the number of autolysosomes (Tian et al., 2022). Elevated radical generation (Sun et al., 2021), attenuated MMP levels (Mao et al., 2020; Sun et al., 2021; Tian et al., 2022), and reduced citrate synthase (Sun et al., 2021) were detected in the MCAO group 24 h after reperfusion, compared with those in the control group. These trends could be reversed by EA pretreatment. Additionally, researchers reported that five consecutive days of EA pretreatment at the Baihui (DU20) acupoint induced neuronal protection by inhibiting the expression (Zhang et al., 2017; Zhang et al., 2018) and translocation (Zhang et al., 2018) of mitochondrial Drp1 in rats with focal cerebral IR injury. Meanwhile, EA pretreatment at the DU20 and Shuigou (DU26) acupoints for 5 days was applied to treat cerebral I/R injury in rats and exerted neuroprotective effects by inhibiting the autophagy-related p-ULK1/FUNDC1 pathway (Mao et al., 2020; Tian et al., 2022). EA pretreatment at the DU20 acupoint induced cerebral ischemic tolerance, increased the expression of NRF-1, TFAM, and mtDNA levels, and further promoted mitochondrial biogenesis by activating CB1R-dependent PGC-1α (Sun et al., 2021). Sun et al. found that the release of Cyt-c in the cytoplasm (Cyto-Cyt-c) was reduced in the EA group 24 h after reperfusion compared with that in the I/R mice group induced by MCAO (Sun et al., 2021). Their findings were consistent with another previous study that also found a significant decrease in Cyto-Cyt-c levels in the EA group compared with the IR group at 6, 24, and 48 h after reperfusion (Zhang et al., 2018).
In summary, EA pretreatment promoted mitochondrial biogenesis 4 h after reperfusion. At 6, 24, and 48 h after reperfusion, EA pretreatment inhibited mitochondrial fission and apoptosis by decreasing mitochondrial Drp1 and Cyto-Cyt-c levels, respectively. Moreover, after 24 h of reperfusion, EA pretreatment reversed mitochondrial structural abnormalities, inhibited the autophagy-related p-ULK1/FUNDC1 pathway, attenuated radical generation, elevated MMP levels, and increased mitochondrial energy metabolism. Specific mechanisms are shown in Table 1; Figure 1A.
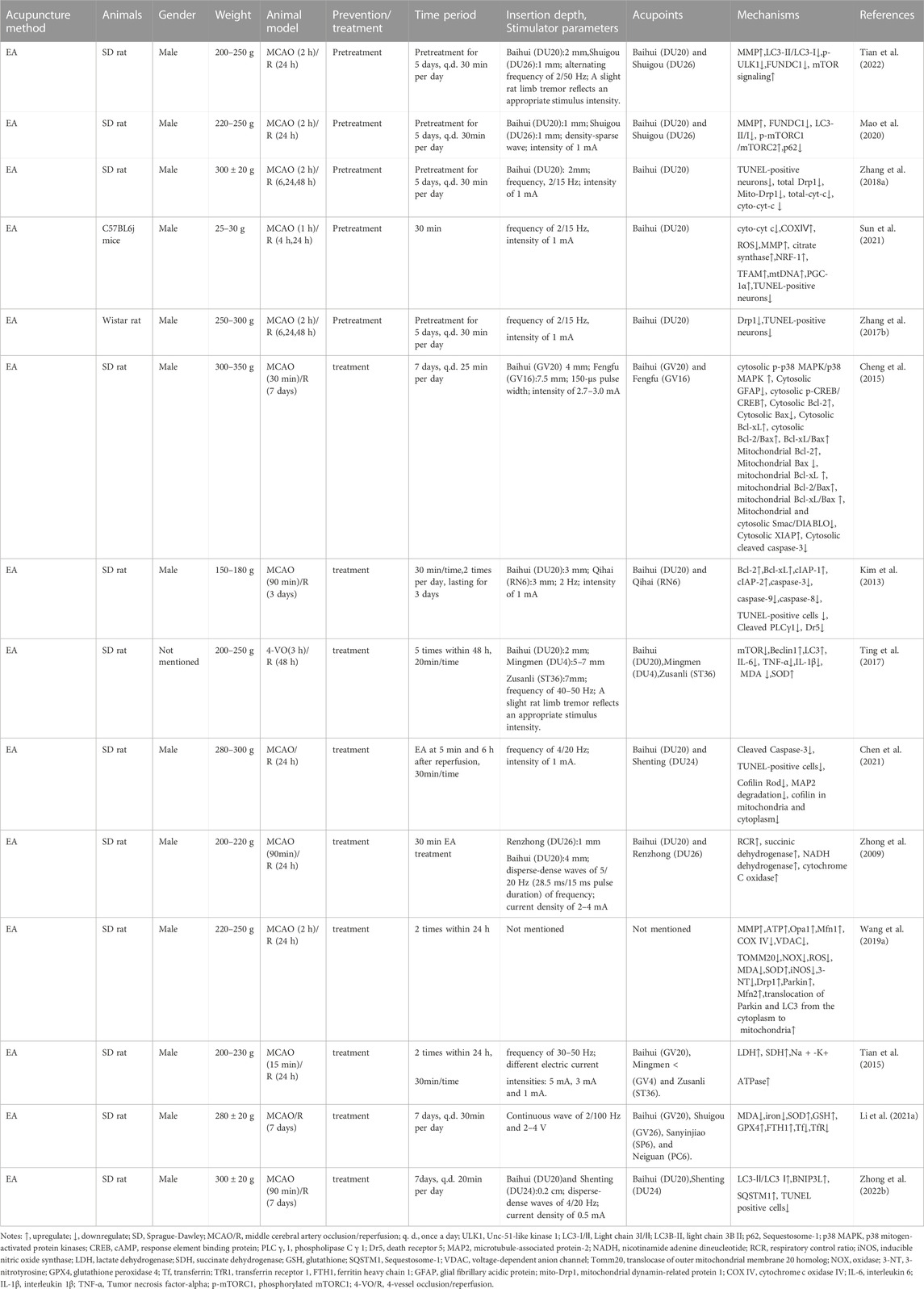
TABLE 1. The molecular mechanism of acupuncture in the treatment of ischemic stroke by targeting mitochondria.
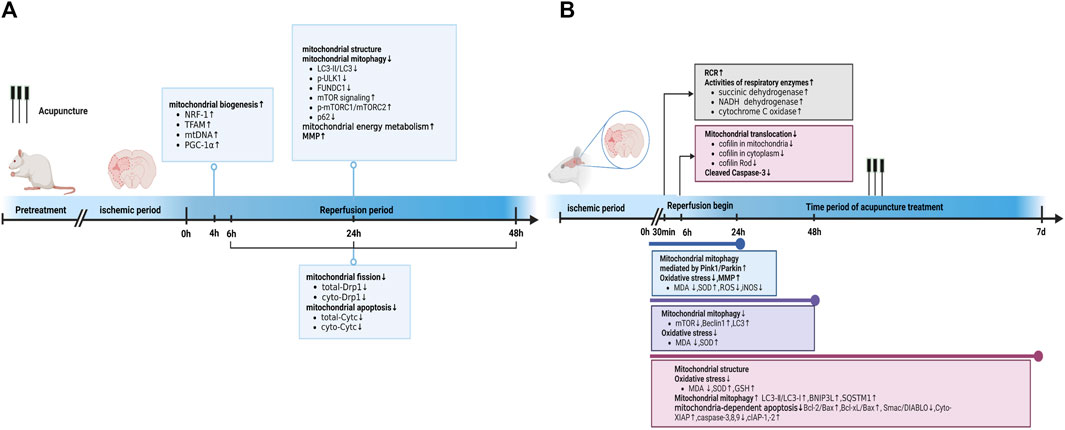
FIGURE 1. Acupuncture prevented and treated ischemic stroke by regulating mitochondria. (A) Acupuncture prevented ischemic stroke by regulating mitochondria at different time point in reperfusion stage. (B) Acupuncture treated ischemic stroke by regulating mitochondria at different time point of acupuncture treatment. Abbreviations: RCR, respiratory control ratio; LC3-I/II, Light chain 3I/II; GSH, glutathione; SQSTM1, Sequestosome-1; iNOS, inducible nitric oxide synthase.
3.1.2 The effect of acupuncture after ischemic stroke in regulating mitochondria
In the MCAO group, the neuronal mitochondria became swollen, the mitochondrial cristae and outer membrane were broken, and EA alleviated the mitochondrial structure abnormalities within 24 h after reperfusion (Li et al., 2021). Acupuncture can alleviate cerebral I/R injury by increasing MMP levels and inhibiting nitro/oxidative stress by downregulating oxidase, ROS, and malondialdehyde (MDA) levels and upregulating superoxide dismutase (SOD) (Ting et al., 2017; Wang et al., 2019). In addition, EA at DU20 and DU26 for 30 min decreased the neurological deficit score, improved the respiratory control ratio, and promoted the activities of respiratory enzymes, including succinic dehydrogenase, NADH dehydrogenase, and cytochrome C oxidase, in MCAO rats (Zhong et al., 2009). These findings are consistent with those reported by Tian et al. (Tian et al., 2015). Tian et al. further pointed out that 3 mA EA could more effectively elevate the activities of succinic dehydrogenase and lactate dehydrogenase compared to 1 mA EA and 5 mA EA in the brain tissue of rats with I/R injury (Tian et al., 2015). Zuo et al. noticed that EA at ZuSanLi (ST36), DU20, and Mingmen (DU4) five times within 48 h after reperfusion could improve cerebral I/R by inhibiting excessive autophagy in neurons (Ting et al., 2017). However, Zhong et al. conducted 7 days of EA treatment at DU20 and Shenting (DU24) after reperfusion and reported that EA could alleviate cerebral I/R injury and improve neural function by promoting BNIP3L mediated autophagic clearance (Zhong et al., 2022). Another study also demonstrated that EA within 24 h after reperfusion decreased the accumulation of damaged mitochondria by increasing Pink1/Parkin-mediated mitophagy clearance to protect cells against neuronal injury in cerebral I/R (Wang et al., 2019). Furthermore, within 24 h after reperfusion, EA at DU20 and Fengfu (DU16) increased the expression of anti-apoptotic Bcl-2, Bcl-Xl, and cellular inhibitor of apoptosis- 1,-2 (cIAP-1, -2), and decreased the activities of caspase-3, -8, and -9 compared with the untreated rats with MCAO (Kim et al., 2013). Similarly, EA at DU20 and DU16 for 7 consecutive days activated p38 MAPK-mediated anti-apoptotic signaling pathways, which ultimately contributed to the prevention of Smac/DIABLO translocation and subsequent restoration of the X-linked inhibitor of apoptosis protein (XIAP) suppression of caspase-3 in the cortical peri-infarct area (Cheng et al., 2015). Another study also found that EA treatment within 6 h of ischemic stroke could attenuate ischemic brain injury and cellular apoptosis by inhibiting mitochondrial translocation of cofilin and caspase-3 cleavage (Chen et al., 2021) (Figure 1B).
In summary, acupuncture treatment and pretreatment could both restore mitochondrial morphology, improve MMP levels, further upregulate mitochondrial energy metabolism, attenuate mitochondrial autophagy, and inhibit mitochondrial-dependent apoptosis. Acupuncture pretreatment promoted mitochondrial biogenesis and inhibited mitochondrial fission. Additionally, acupuncture treatment inhibited oxidative stress, cofilin translocation, and activated mitochondrial autophagy. The detailed mechanisms are shown in Table 1 and Figures 1A,B.
3.2 Herbal extract and its molecular mechanisms by regulating mitochondria in treating ischemic stroke
3.2.1 Herbal extract pretreatment in regulating mitochondria of ischemic stroke
Although the clinical treatment of ischemic stroke with a single herb is rare, in recent years, researchers have reported that the individual application of certain herbs has the potential to treat diseases. Mitochondrial ultrastructure injury was partially improved in cerebral I/R rats after pretreatment with in vitro cultured Bos taurus domesticus Gmelin or Chrysanthemum morifolium Ramat. extracts (Lin et al., 2010; Lu et al., 2020). Pretreatment with herbal extracts (e.g., Astragalus membranaceus (Fisch.) Bge. combined with Panax notoginseng (Burk.) F.H.Chen, Astragalus membranaceus (Fisch.) Bge., and Gardenia jasminoides (Ellis) alleviated nerve injury after cerebral I/R by improving mitochondrial respiration function and energy metabolism (Huang et al., 2012; Huang et al., 2017; Wang et al., 2021). Previous studies have noted that herbal extracts (including Pinellia ternata (Thunb.) Breit., Rosa laevigata Michx., Curcuma Longa L., C. morifolium Ramat., and Lavandula angustifolia Mill.) could play a neuroprotective role in the pretreatment of animal models of MCAO by increasing MMP levels and inhibiting mitochondrial oxidative stress (by upregulating SOD, glutathione, glutathione peroxidase catalase, and downregulating MDA, NO, ROS, and peroxynitrite) (Dohare et al., 2008b; Lin et al., 2010; Wang et al., 2012; Zhang et al., 2013; Ye et al., 2016). Recent in vitro studies have also shown that Scrophularia ningpoensis Hemsl., Aglaia odorata Lour., Spatholobus suberectus Dunn, and Arctium lappa L. roots exert neuroprotective effects by increasing MMP levels and inhibiting mitochondrial oxidative stress in preconditioned OGD/R cell models (Meng et al., 2018; Park et al., 2018; Wang K. et al., 2020; Yang et al., 2021). Lycium barbarum L. polysaccharide pretreatment decreased cerebral I/R injury in MCAO rats by maintaining mitochondrial fission and fusion balance (upregulating Opa1 and downregulating Drp1) (Liu et al., 2017). Similarly, Arctium lappa L. roots ameliorated OGD/R-induced injury by suppressing AMPK/mammalian target of rapamycin (mTOR)-mediated autophagy (Yang et al., 2021). Herbal extracts (such as P. ternata (Thunb.) Breit., R. laevigata Michx, Curcuma Longa L., S. ningpoensis Hemsl., L. barbarum L. polysaccharides, Astragalus membranaceus (Fisch.) Bge., in vitro cultured B. taurus domesticus Gmelin and Angelica sinensis (Oliv.) (Diels) prevented cerebral I/R injury in MCAO animal models by inhibiting the mitochondria-dependent apoptosis pathway. These herbal extracts upregulated the expression of Bcl-2, mitochondrial Cyt-C (Mito-Cyt-c), cytosolic phospho-Bad (p-Bad)/Bad ratios, and mitochondrial p-Bad/Bad. Additionally, they downregulated the expression of Bax, p53, Apaf1, Bax, Bid, Cyt-c, cleaved PARP-1, and active caspase-3, -9, and -8 (Dohare et al., 2008b; Huang et al., 2012; Zhang et al., 2013; Wang et al., 2014; Ye et al., 2016; Cheng et al., 2017; Meng et al., 2018; Lu et al., 2020). Evidence from in vitro experiments has demonstrated that A. odorata Lour. and Arctium lappa L. roots showed a significant protective effect in OGD/R cell models by inhibiting the mitochondria-dependent apoptotic pathway (Wang et al., 2020; Yang et al., 2021).
In brief, evidence from in vivo and in vitro studies indicated that herbal extract pretreatment could ameliorate cerebral ischemia by improving mitochondrial ultrastructure, increasing MMP levels, mitochondrial respiration function, and energy metabolism, maintaining mitochondrial dynamic balance, inhibiting mitochondria-related oxidative stress, autophagy, and mitochondria-dependent apoptosis (Figure 2).
3.2.2 The effect of herbal extract after ischemic stroke in regulating mitochondria
Not only herbal extract pretreatment can alleviate mitochondrial structural abnormalities, but also herbal extract treatment can mitigate these abnormalities. Seven days of Dengzhanxixin injection treatment can improve decreased and unclear mitochondrial cristae observed in the MCAO rat model (An et al., 2021) while Ganoderma lucidum (Leyss.ex Fr.) Karst. polysaccharides can alleviate swollen and vacuolized mitochondria observed in OGD/R primary cortical neuronal cells (Zhou et al., 2010). Cordyceps sinensis (BerK.) Sacc. extract improved ATP levels and mitochondrial complexes I-IV in MCAO rats. Cordyceps sinensis (BerK.) Sacc., Curcuma Longa L., and polysaccharides from A. sinensis (Oliv.) Diels decreased oxygen free radicals, NO, ROS, peroxynitrite, glutathione peroxidase, SOD, and Ca2+ and increased MMP and MDA levels in MCAO rats (Dohare et al., 2008a; Lei et al., 2014; Bai et al., 2020). These findings are consistent with in vitro studies of C. sinensis (BerK.) Sacc. extract, L. barbarum L. polysaccharides, and polysaccharides from A. sinensis (Oliv.) Diels in alleviating OGD/R injury (Lei et al., 2014; Shi et al., 2017; Zhao et al., 2017; Bai et al., 2020). Additionally, combining Panax ginseng C.A. Mey. and A. sinensis (Oliv.) Diels partially attenuated cerebral injury by ameliorating Drp1-mediated mitochondrial fission (downregulating Drp1) in vivo and in vitro (Hu et al., 2020). However, Ginkgo biloba L. extract upregulated Drp1 and Opa1 in vivo (Li et al., 2019). Researchers found that G. biloba L. extract induced autophagy by activating the AMPK/mTOR pathway (Li et al., 2019). Both in vivo and in vitro experiments, including C. sinensis (BerK.) Sacc., Curcuma Longa L., L. barbarum L. polysaccharides, G. lucidum (Leyss.ex Fr.) Karst. polysaccharides, and extract of G. biloba L., exhibited obvious neuroprotective effects in MCAO rats, and the OGD/R cell model by inhibiting mitochondrial-dependent apoptosis (Dohare et al., 2008a; Zhou et al., 2010; Shi et al., 2017; Zhao et al., 2017; Li et al., 2019; Bai et al., 2020) (Figure 2).
Overall, herbal extract pretreatment and treatment could alleviate abnormal mitochondrial structure; improve MMP, mitochondrial energy metabolism, mitochondrial respiration function, and mitochondrial fusion; and inhibit oxidative stress, mitochondrial fission, and mitochondrial-dependent apoptosis. Furthermore, herbal extract pretreatment suppressed AMPK/mTOR-mediated mitophagy, whereas herbal extract treatment induced autophagy by activating the AMPK/mTOR pathway and promoting mitochondrial fission. Specific mechanisms are shown in Table 2; Figure 3.
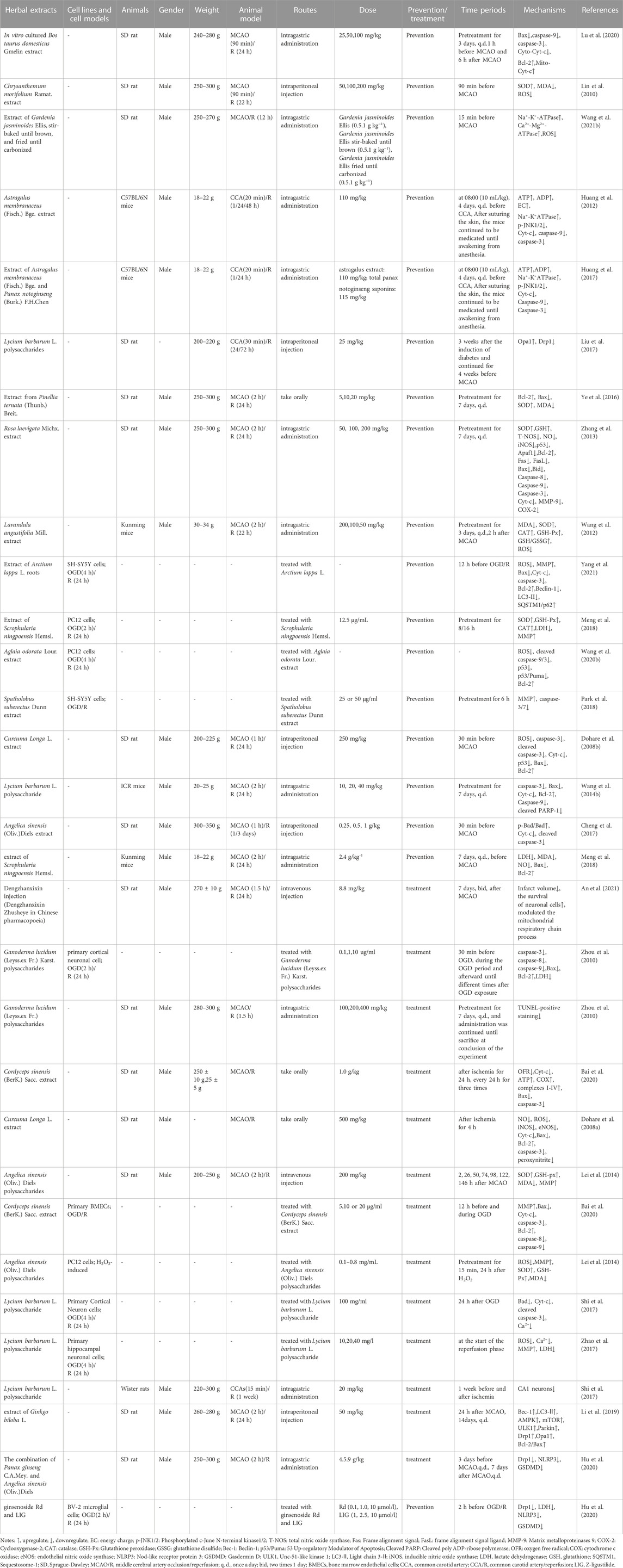
TABLE 2. The molecular mechanism of herbal extracts in the treatment of ischemic stroke by targeting mitochondria.
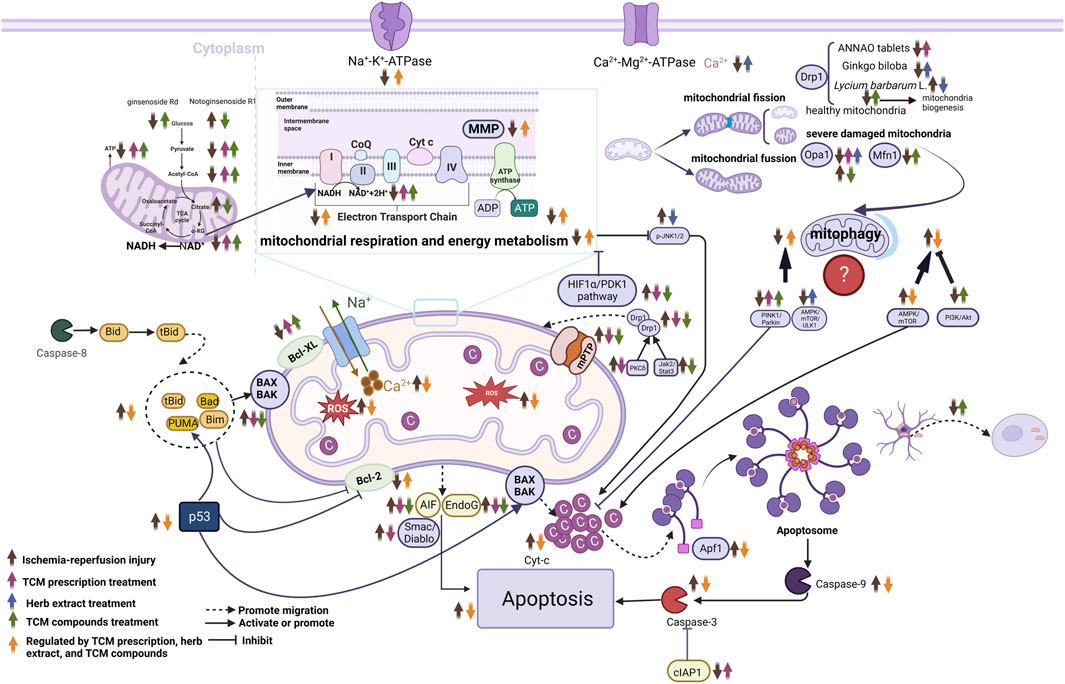
FIGURE 3. Molecular mechanisms of herbal extract, TCM compounds and TCM prescriptions in treating ischemic stroke from the prospective of mitochondria. ↑, upregulate; ↓, downregulate; Abbreviations: NAD+, nicotinamide adenine dinucleotide; Endo G, endonuclease G; Apaf-1, apoptosis activating factor-1; AIF, apoptosis-related proteins; Mfn1, mitofusins-1; Opa1, optic atrophy 1; Smac/Diablo, the second mitochondrion-derived activator of caspase/direct inhibitor of apoptosis-binding protein with low pI; cIAP-1, cellular inhibitor of apoptosis- 1; Cyt-c, cytochrome C; mPTP, mitochondrial permeability transition pore; MMP, mitochondrial membrane potential; AMPK, AMP-activated protein kinase; mTOR, mammalian target of rapamycin; Drp1, dynamin-related protein 1; HIF1α/PDK1, Hypoxia-Inducible Factor 1-Alpha/Pyruvate Dehydrogenase Kinase 1; NADH, Nicotinamide adenine dineucleotide; JNK, c-Jun N-terminal kinase; ROS, reactive oxygen species; TCA, tricarboxylic acid.
3.3 TCM compounds and their molecular mechanisms by regulating mitochondria in treating ischemic stroke
3.3.1 TCM compound pretreatment in regulating mitochondria of ischemic stroke
Herbal medications have yielded many active compounds for treating ischemic stroke, and this number is increasing as research progresses. Based on published literature, we analyzed 34 TCM compounds and their molecular mechanisms in regulating mitochondria in ischemic stroke. Mitochondria appeared swollen with irregular, disrupted membranes and poorly defined cristae in an MCAO rat model. However, these mitochondrial abnormalities were prevented by piperine pretreatment (Kaushik et al., 2021). In vitro, OGD/R induced mitochondrial fragmentation, mitochondrial enlargement, mitochondrial number reduction, and mitochondrial swelling, which could be alleviated by pretreatment with notoginsenoside R1 (Zhu et al., 2021; Liu et al., 2022), hydroxysafflor yellow A (Huang et al., 2021), and calenduloside E (Li et al., 2022b). Ginsenoside Rd and piperine pretreatment improved mitochondrial energy metabolism after cerebral I/R injury (Ye et al., 2011; Kaushik et al., 2021) whereas notoginsenoside R1 and notoginseng leaf triterpene pretreatment improved mitochondrial energy metabolism after OGD/R injury (Xie et al., 2020; Zhu et al., 2021; Liu et al., 2022). In vivo (Ye et al., 2011; Mukherjee et al., 2019; Zhang et al., 2019; Huang et al., 2021; Kaushik et al., 2021) and in vitro (Li et al., 2017; Wu et al., 2017; Zhou et al., 2017; Huang et al., 2020; Xie et al., 2020; Li et al., 2021; Huang et al., 2021; Li et al., 2022b; Ni et al., 2022; Peng et al., 2022) studies have reported that TCM compounds (e.g., piperine, ginsenoside Rd, hydroxysafflor yellow A, β-patchoulene, curcumin, ginsenoside Rb1, artemether, notoginseng leaf triterpenes, ginkgolide k, ginsenoside monomer compound k, tanshinone IIA, artemisinin, and kaempferol) inhibited oxidative stress and mPTP and upregulated MMP levels. In vitro studies show that atractylenolide III, ginkgolide K, calenduloside E, and kaempferol decreased Drp1 translocation from the cytosol to the outer mitochondrial membrane, reduced its phosphorylation at Ser616, and enhanced its phosphorylation at Ser637 (Wu et al., 2017; Zhou et al., 2017; Zhou et al., 2019; Li et al., 2022b). In addition, ginsenoside Rb1 inhibits astrocyte activation and promotes the transfer of astrocytic mitochondria to neurons against ischemic stroke in vitro (Ni et al., 2022). Chrysophanol and ginsenoside monomer compound K decreased the level of mitochondrial autophagy in MCAO mice after I/R injury and in neurons after OGD/R injury, respectively (Huang et al., 2020; Cui et al., 2022) by inhibiting the AMPK/mTOR pathway (Huang et al., 2020). In contrast, kaempferol potentiated autophagy in primary neurons after OGD/R injury (Wu et al., 2017). Much evidence in vivo (Ye et al., 2011; Mukherjee et al., 2019; Zhang et al., 2019; Kaushik et al., 2021) and in vitro (Chen et al., 2017; Li et al., 2017; Zhou et al., 2017; Huang et al., 2020; Huang et al., 2021; Li et al., 2022b; Peng et al., 2022) suggests that TCM compounds (e.g., piperine, ginsenoside Rd, β-patchoulene, curcumin, hydroxysafflor yellow A, ginkgolide K, ginsenoside monomer compound K, tanshinone IIA, calenduloside E, artemisinin, and paeoniflorin) have protective effects against cerebral I/R injury or OGD/R injury by inhibiting mitochondria-mediated apoptosis.
In summary, TCM compounds can alleviate abnormal mitochondrial structure, improve mitochondrial energy metabolism, decrease the expression and translocation of Drp1, reduce oxidative stress, mPTP, and mitochondria-dependent apoptosis, upregulate MMP, and promote the transfer of astrocytic mitochondria to neurons to prevent ischemic stroke. However, mitophagy results remain controversial and require further investigation (Figure 4).
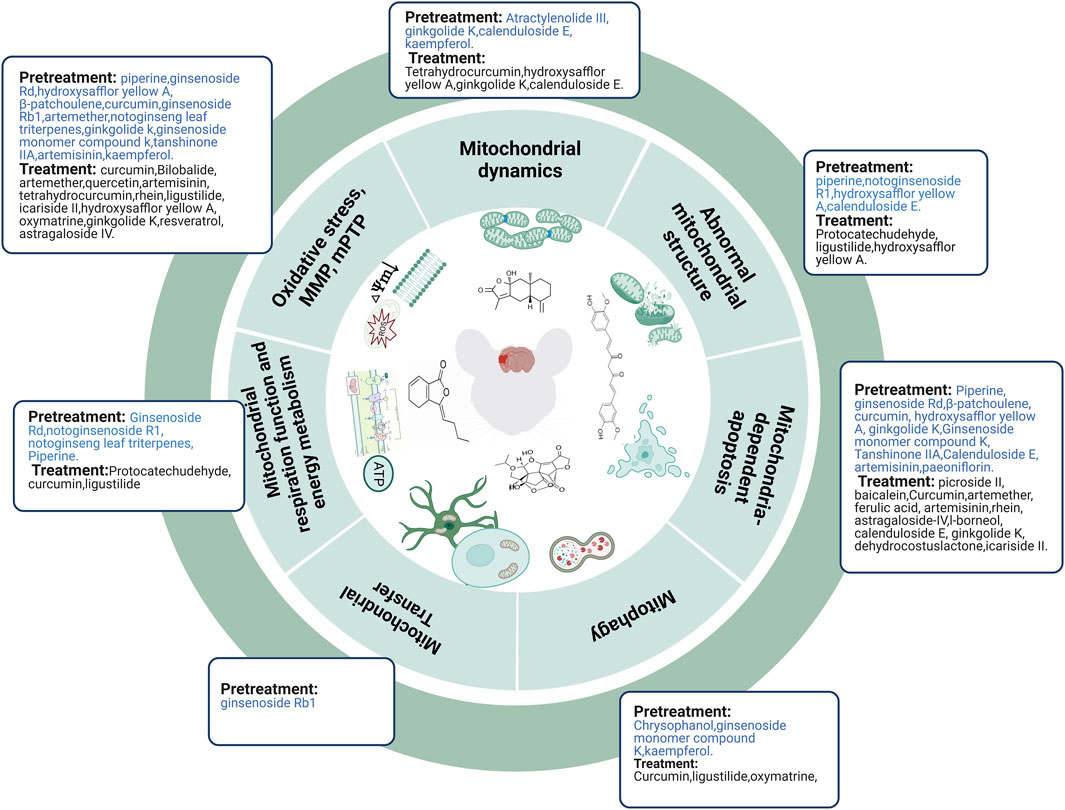
FIGURE 4. TCM compounds prevented and treated ischemic stroke by regulating mitochondria. Abbreviations: mPTP, mitochondrial permeability transition pore; MMP, mitochondrial membrane potential.
3.3.2 The effect of TCM compounds after ischemic stroke in regulating mitochondria
Protocatechudehyde and ligustilide improved mitochondrial morphology after cerebral I/R injury in vivo (Zeng et al., 2021a; Mao et al., 2022) whereas hydroxysafflor yellow A maintained mitochondrial morphology after OGD injury in vitro (Chen et al., 2019). Protocatechudehyde, curcumin, and ligustilide protect against cerebral ischemic injury by improving mitochondrial energy metabolism (Wang and Xu, 2020; Zeng et al., 2021a; Mao et al., 2022). Curcumin could also alleviate OGD injury by improving mitochondrial energy metabolism (Wang and Xu, 2020). Evidence from in vivo studies (Zhang et al., 2017; Zhao et al., 2018b; Mondal et al., 2019; Wang and Xu, 2020; Li et al., 2021; Cen et al., 2022; Mao et al., 2022; Peng et al., 2022) and in vitro studies (Feng et al., 2018; Liu et al., 2018; Chen et al., 2019; Xiang et al., 2019; Xue et al., 2019; Wang and Xu, 2020; Wei et al., 2021; Ye et al., 2021; Mao et al., 2022) demonstrated that, following cerebral I/R injury or OGD injury, TCM compounds (e.g., curcumin, bilobalide, artemether, quercetin, artemisinin, tetrahydrocurcumin, rhein, ligustilide, icariside II, hydroxysafflor yellow A, oxymatrine, ginkgolide K, resveratrol, and astragaloside IV) alleviated oxidative stress, inhibited mPTP, and upregulated MMP levels. Mfn-1 and Drp-1 downregulation after cerebral I/R injury was restored by tetrahydrocurcumin treatment (Mondal et al., 2019) whereas Drp-1 downregulation and Opa1 upregulation after OGD injury were restored by hydroxysafflor yellow A treatment (Chen et al., 2019). However, treating mice with ginkgolide K and calenduloside E prevents Drp1 translocation to the mitochondria and attenuates mitochondrial dysfunction after MCAO (Zhou et al., 2017; Li et al., 2022b). Curcumin and ligustilide enhanced cerebral I/R- or OGD-induced mitophagy (upregulating PINK1, Parkin, the colocalization of LC3B and mitochondrial markers, and the ratio of LC3-II to LC3-I) in vivo and in vitro (Wang and Xu, 2020; Mao et al., 2022). However, oxymatrine attenuates excessive autophagy (downregulating LC3 and Beclin-1) in vivo and in vitro by activating the PI3k/Akt pathway (Wei et al., 2021). Additionally, picroside II attenuated cerebral I/R injury by inhibiting EndoG release from the mitochondria into the cytoplasm (Li et al., 2018) Similarly, baicalein treatment decreased cerebral I/R injury by inhibiting nuclear translocation of AIF in cerebral I/R rats (Li et al., 2020). Many in vivo (Zhang et al., 2017; Zhao et al., 2018b; Cheng et al., 2019; Yin et al., 2020; Li et al., 2021; Zhang et al., 2021; Li et al., 2022b; Peng et al., 2022) and in vitro (Zhao et al., 2018a; Feng et al., 2018; Liu et al., 2018) studies have shown that TCM compounds (e.g., curcumin, artemether, ferulic acid, artemisinin, rhein, astragaloside IV, l-borneol, calenduloside E, ginkgolide K, dehydrocostus lactone, and icariside II) exert protective effects in MCAO animal models or OGD/R cell models by inhibiting BAX/BCL2, caspase-9, caspase-3, cleaved caspase-3, caspase-8, Cyt-c, Bid, Apaf-1, Bad, and p53 (Figure 4).
Through in-depth comparative analysis, TCM compounds provided during pretreatment and treatment could alleviate abnormal mitochondrial structure; inhibit oxidative stress, mPTP opening, and Drp1 translocation to the mitochondria; and improve mitochondrial energy metabolism and MMP. TCM compounds provided pretreatment could promote the transfer of astrocytic mitochondria to neurons and potentiate autophagy and also decrease the level of mitochondrial autophagy by inhibiting the AMPK/mTOR pathway. Meanwhile, TCM compounds during treatment could maintain the dynamic balance between mitochondrial fission and fusion, inhibit mitochondrial autophagy by activating the PI3k/Akt pathway, promote mitophagy by activating PINK1/Parkin, inhibit Endo G and AIF release from mitochondria into the cytoplasm, and further attenuate mitochondria-mediated apoptosis. The specific mechanisms of these compounds in vivo and in vitro are shown in Table 3; Figure 3.
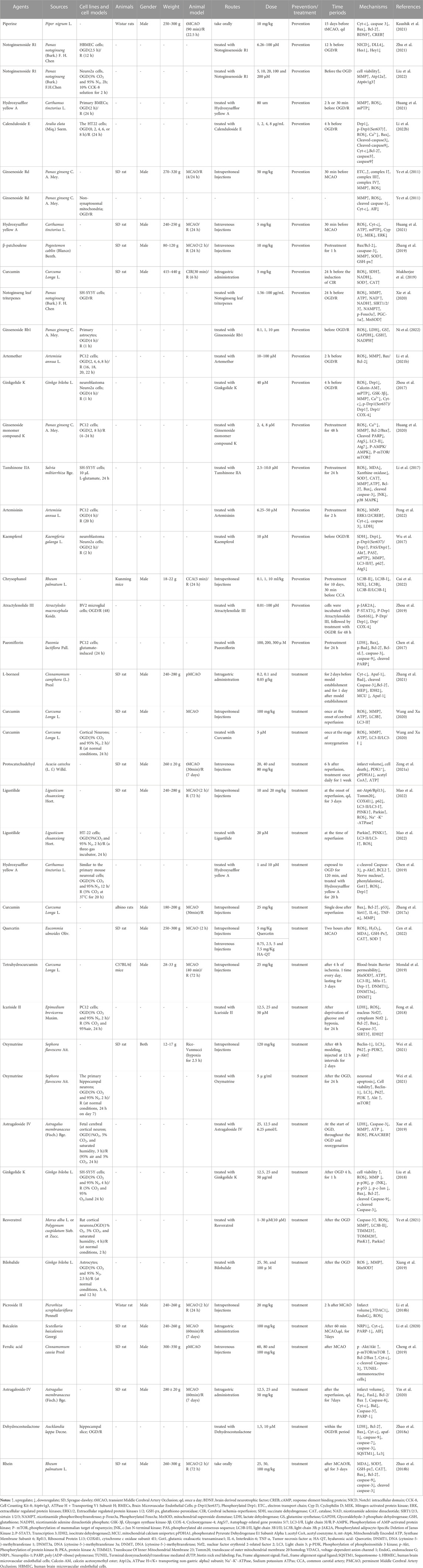
TABLE 3. The molecular mechanism of TCM compounds in the treatment of ischemic stroke by targeting mitochondria.
3.4 TCM prescription and its molecular mechanisms by regulating mitochondria in treating ischemic stroke
3.4.1 TCM prescription pretreatment for regulating mitochondria in ischemic stroke
Ischemic strokes are usually treated using TCM prescriptions owing to TCM’s overall concept of TCM and syndrome differentiation-based treatment. In MCAO rats, pretreatment with Xiao-Xu-Ming decoction improved the abnormal mitochondrial ultrastructure (Lan et al., 2018). Pretreatment with Buyang Huanwu decoction prevents H2O2-induced ultrastructural disruption of mitochondria in human umbilical vein endothelial cells, whereas Guhong injection preconditioning preserves mitochondrial morphology during OGD injury (Shen et al., 2016; Zhou et al., 2021). In human umbilical vein endothelial cells and primary cultured cortical neurons, H2O2 decreased ATP production, MDA levels, and MMP levels while increasing ROS and SOD levels, which could be reversed by pretreatment with Buyang Huanwu Decoction, Zhenbao pill, and YiQiFuMai Powder injection (Shen et al., 2016; Xu et al., 2017; Jia et al., 2021). In brain microvascular endothelial cells and primary cortical neurons, OGD/R induced MMP loss and oxidative stress injury, which could be alleviated by pretreatment with Guhong injection, Xingxiong injection, and Naoxintong capsules (Wang et al., 2021; Zhou et al., 2021; Zhu et al., 2022). Danhong injection pretreatment improved mitochondrial energy metabolism after OGD/R injury (Orgah et al., 2019). Xu et al. demonstrated that pretreatment with YiQiFuMai powder ameliorated H2O2-induced neuronal apoptosis by inhibiting mitochondrial dysfunction and PKCδ/Drp1-mediated excessive mitochondrial fission (Xu et al., 2017). Pretreatment with Xiao-Xu-Ming decoction and Zhenbao pill-containing serum exerted neuroprotective effects in MCAO rats and H2O2-induced vascular endothelial cells, respectively, by inhibiting mitophagy (Lan et al., 2018; Jia et al., 2021). In addition, Zhenbao pill-containing serum represses cell apoptosis by inhibiting autophagy (Jia et al., 2021). Xiao-Xu-Ming decoction inhibited the translocation of Smac/Diablo from the mitochondria to the nucleus, increased the level of cytoplasmic c-IAP1, and further inhibited ischemia-induced neuronal apoptosis (Lan et al., 2014). To date, many in vivo studies (e.g., Ershiwei Chenxiang pills, Pien-Tze-Huang and Xiao-Xu-Ming decoction) (Lan et al., 2014; Zhang et al., 2018; Hou et al., 2020) and in vitro studies (e.g., Buyang Huanwu decoction, Guhong injection, Xingxiong injection, YiQiFuMai powder injection, and Zhenbao pill) (Shen et al., 2016; Zhou et al., 2021; Zhu et al., 2022) have revealed that traditional Chinese prescriptions protect mitochondria from ischemic injury and inhibit the mitochondria-dependent apoptosis pathway.
In brief, TCM pretreatment improved abnormal mitochondrial structure, inhibited oxidative stress and mitophagy, improved mitochondrial energy metabolism and MMP, and maintained the dynamic balance of mitochondrial fission and fusion. TCM prescriptions inhibited Smac/Diablo release from the mitochondria into the cytoplasm and further attenuated mitochondria-mediated apoptosis (Figure 5).
3.4.2 The effect of TCM prescription after ischemic stroke in regulating mitochondria
Taohong Siwu decoction treatment for seven consecutive days decreased damage to mitochondrial structures following cerebral I/R injury (Ji et al., 2022). Treatment with Huang-Lian-Jie-Du decoction, Danhong injection, and Baoyuan capsule eliminated the inhibitory effect of cerebral I/R on mitochondrial metabolism in MCAO models (Wang et al., 2014; Zeng et al., 2021b; Du et al., 2021). In vitro studies have also shown that Baoyuan capsule and Qing Nao Yi Zhi Fang improved mitochondrial energy metabolism after glutamate exposure or OGD/R injury (Zhang et al., 2000; Du et al., 2021). Many in vivo (e.g., Danhong injection (Zeng et al., 2021b), Huang-Lian-Jie-Du-Decoction (Wang et al., 2014) and in vitro studies (e.g., Naoxintong (Ma et al., 2016), Shuxuetong injection (Sun et al., 2019), Mu-Xiang-You-Fang (Ma et al., 2020) and Tong Luo Jiu Nao injection (Li et al., 2014)) could downregulate mitochondrial oxidative stress levels and upregulate MMP levels in the MCAO rat and OGD/R cell models, respectively. YiQiFuMai powder injection inhibited the expression, phosphorylation, and translocation of Drp1 in oxidative stress-induced primary neurons and cerebral ischemia-injured rats, resulting in a significant improvement in cerebral infarction and neurological scores (Xu et al., 2017). Taohong Siwu decoction, Danhong injection, and ANNAO tablets upregulated the expression of autophagy markers (LC3-II/LC3-I and Beclin1) (Ji et al., 2022) and mitochondrial autophagy markers (Parkin (Orgah et al., 2019) and PINK1 (Zhang et al., 2020)) after cerebral I/R injury. Mu-Xiang-You-Fang inhibits autophagy after OGD/R-induced PC12 cell injury through the AMPK-mTOR pathway (Ma et al., 2020). Nan et al. reported that Gualou Guizhi decoction exerted its neuroprotective effects by inhibiting poly (ADP-ribose) translocation into mitochondria, thereby reducing the release and inhibiting the translocation of AIF and Endo G from mitochondria to the nucleus, which further inhibits ischemia-induced neuronal apoptosis (Nan et al., 2020). TCM prescriptions (e.g., Danhong injection (Feng et al., 2020), Guhong injection (Zhou et al., 2021), YiQiFuMai powder injection (Xu et al., 2017), ANNAO tablets (Zhang et al., 2020), and Huang-Lian-Jie-Du decoction (Wang et al., 2019)) could downregulate pro-apoptotic factors (Cyt-c, cleaved-caspase-3, cleaved-caspase-9, Bad, Bax, and Bim) and upregulate anti-apoptotic factors (Bcl-2) after cerebral I/R injury in MCAO rats. Further, TCM prescriptions (e.g., Shuxuetong injection (Sun et al., 2019) and Naoxintong (Ma et al., 2016)) also downregulated pro-apoptotic factors (e.g., Cyt-c, cleaved-caspase-3, cleaved-caspase-9, and Bax) and upregulated anti-apoptotic factors (e.g., Bcl-2) after OGD/R injury (Figure 5).
In summary, TCM prescription pretreatment and treatment could improve the abnormal mitochondrial structure, mitochondrial energy metabolism, and MMP, and inhibit oxidative stress, mitochondrial fission, and mitophagy. Moreover, TCM prescription pretreatment inhibited Smac/Diablo release from mitochondria into the cytoplasm and further attenuated mitochondria-mediated apoptosis. In contrast, TCM prescription treatment promoted mitophagy by activating PINK1/Parkin and inhibiting mitochondria-mediated apoptosis by attenuating AIF and Endo G release from mitochondria into the cytoplasm. The specific mechanisms of the TCM prescriptions in vivo and in vitro are shown in Table 4; Figure 3.
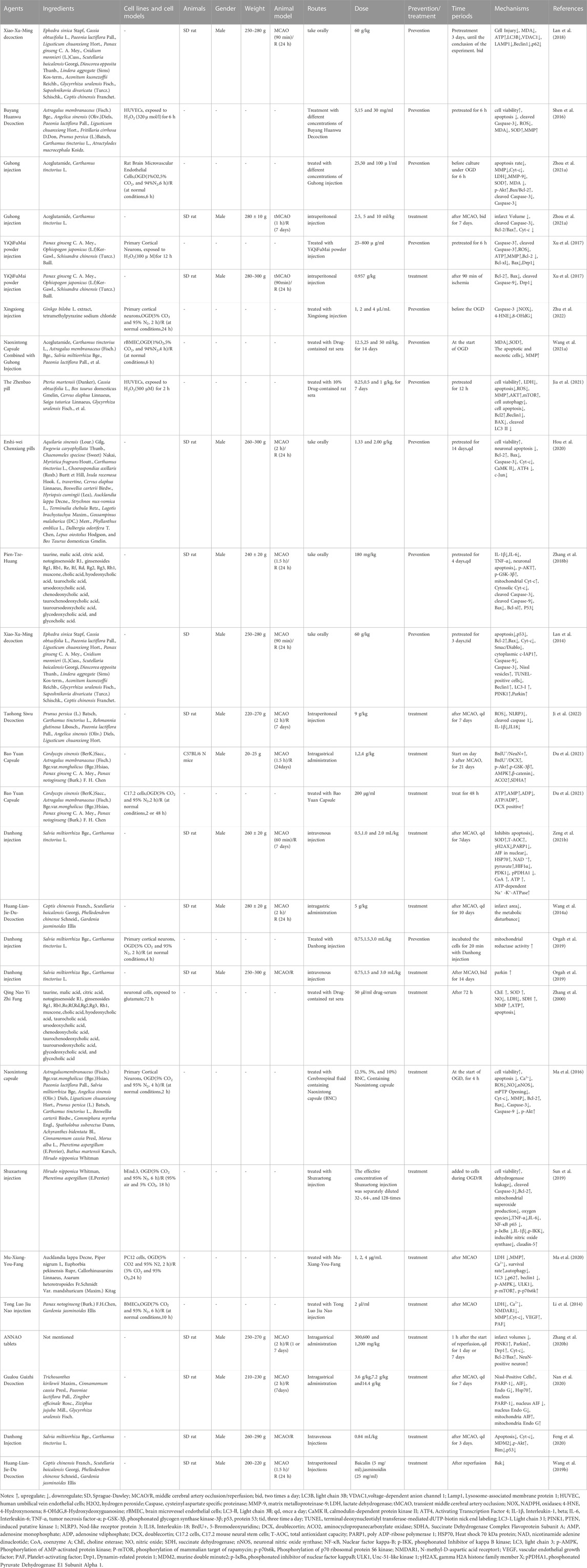
TABLE 4. The molecular mechanism of TCM prescription in the treatment of ischemic stroke by targeting mitochondria.
3.5 The specific molecular mechanism among acupuncture, herbal extracts, TCM compounds, and TCM prescriptions in treating ischemic stroke
Acupuncture, herbal medicine, TCM compounds, and TCM prescriptions prevent and treat ischemic stroke by improving abnormal mitochondrial structure, increasing MMP levels, mitochondrial respiration function, and mitochondrial energy metabolism, decreasing oxidative stress, maintaining mitochondrial fission and fusion dynamics, promoting mitochondrial biogenesis, regulating mitophagy, and inhibiting mitochondrial-dependent apoptosis. However, the specific molecular mechanism differs among acupuncture, herbal extracts, TCM compounds, and TCM prescriptions in treating ischemic stroke. Acupuncture, herbal extract, TCM compounds, and TCM prescriptions could alleviate mitochondrial respiration function and energy metabolism by improving the electron transport chain. Acupuncture, TCM compounds, and TCM prescriptions can improve tricarboxylic acid cycle dysfunction. Additionally, TCM compounds and TCM prescriptions can improve mitochondrial respiration and energy metabolism by inhibiting the hypoxia-inducible factor 1-alpha/pyruvate dehydrogenase kinase 1(HIF1α/PDK1) pathway. Acupuncture inhibited mitochondrial fission, whereas herbal extracts and TCM prescriptions promoted mitochondrial fusion. However, the results regarding mitochondrial fission have been inconsistent in studies of herbal extracts, TCM compounds, and TCM prescriptions. We noted that TCM compounds decreased Drp1 translocation from the cytosol to the outer mitochondrial membrane by inhibiting Jak2/Stat3. In contrast, TCM prescriptions decreased Drp1 translocation from the cytosol to the outer mitochondrial membrane by inhibiting PKCδ. Acupuncture, TCM compounds, and TCM prescriptions promoted mitophagy by activating the PINK1/Parkin pathway. On the other hand, herbal extracts, TCM compounds, and TCM prescriptions suppressed mitophagy by inhibiting the AMPK/mTOR pathway.
Acupuncture could attenuate mitophagy by inhibiting the p-ULK1/FUNDC1 pathway, whereas TCM compounds could inhibit mitophagy by activating the PI3k/Akt pathway. Acupuncture, herbal extracts, TCM compounds, and TCM prescriptions can upregulate the expression of anti-apoptotic proteins in the BCL-2 family and downregulate the expression of pro-apoptotic proteins in the BCL-2 family. In addition, acupuncture prevented Smac/DIABLO and cofilin translocation from the mitochondria into the cytoplasm, whereas TCM compounds inhibited Endo G and AIF release from the mitochondria into the cytoplasm. Moreover, TCM prescriptions could inhibit Smac/DIABLO, Endo G, and AIF release from the mitochondria into the cytoplasm. Generally, the above evidence demonstrated that the specific molecular mechanism differed among acupuncture, herbal extract, TCM compounds, and TCM prescriptions in treating ischemic stroke (Figures 1, 3).
Interestingly, only TCM compounds have been reported to promote the transfer of astrocytic mitochondria to neurons in response to ischemic stroke. Emerging evidence suggests that mitochondria could serve as “help-me” signaling in response to various external stimuli and recruit neighboring cells to rescue injured cells. Removing damaged mitochondria and replacing them with healthy ones is a potential treatment for hypoxia and ischemia-related disorders, especially in the central nervous system, where mitochondria are abundant in the distal axonal synapses and dendritic protrusions. More studies can be conducted exploring the underlying therapeutic mechanism of TCM in treating ischemic stroke from the perspective of mitochondrial transfer.
4 Conclusion and prospects
In this review, we summarize the molecular mechanisms underlying the involvement of mitochondria in ischemic stroke. Mitochondrial function and structure play important roles in ischemic stroke, serving as crucial targets for TCM in alleviating ischemic stroke, and we have identified some key proteins and signaling pathways, as mentioned above. In addition, some issues require further clarification and improvement in future research. First, the precise molecular mechanisms underlying the effects of TCM on mitochondria in cellular and rat models of ischemic stroke remain incompletely understood. Further research is needed to elucidate the underlying mechanisms of TCM’s effects on mitochondrial structure and function in ischemic stroke, using molecular, cellular, and biochemical approaches. Second, the lack of standardized experimental designs and methods may affect the reproducibility and comparability of the results. Standardization of experimental designs and methods, including the quality control of TCM preparations, should be established to ensure the scientific rigor and reliability of future studies. Third, in the field of EA therapy for ischemic stroke, research from the perspective of mitochondria is scarce in comparison to studies on herbal extracts, compounds, and prescriptions. More rigorous and well-designed studies are urgently needed. In addition, it is critical to establish standardized EA stimulation parameters (e.g., frequency, duration, and intensity) to investigate the dose-response relationship and corresponding mechanisms from the perspective of mitochondria in future studies. Fourth, the results of mitophagy, mitochondrial fission, and mitochondrial fusion have been inconsistent among studies. These controversial results might be associated with different experimental models, different stages of ischemic stroke, and intervention modes. Further investigations are required to elucidate this. Fifth, most of the studies summarized above are based on OGD/R or MCAO ischemia models, and most MCAO ischemia models are conducted on young rats or mice. Few studies have used aged animals or models that closely mimic clinical patients who often have hypertension, hyperglycemia, or other disorders. Therefore, it is necessary to investigate the neuroprotective benefits of TCM against ischemic stroke using pseudo-clinical models (e.g., complicated models of multiple coexisting disorders), which will provide a reliable foundation for TCM’s clinical application of TCM. Sixth, most in vitro studies mentioned above were limited to a particular type of nerve cells. Neurovascular dysfunction induced by ischemic stroke demonstrates a combined action of multiple nerves in the brain, and investigating only one type of nerve cell is insufficient. Thus, ischemic stroke can be better understood using a cell co-culture model of neurons, microglia, and astrocytes in vitro. Finally, since Chinese herbs and prescriptions contain various chemical components, the precise underlying mechanisms remain unknown. Further research is required into the molecular targets and active components that contribute to the bioactivity of Chinese herbs and prescriptions in preventing and treating ischemic stroke. Furthermore, we should examine the synergic effects of the constituents and their metabolites, as well as the targeted signaling pathways in post-ischemic brains.
In conclusion, this review summarizes the recent experimental evidence of TCM in preventing and treating ischemic stroke by modulating mitochondria and identifies areas that future research should focus on. In addition, TCM has few side effects and is highly effective and specific; therefore, with adequate research, it will be widely available for ischemic stroke treatment.
Author contributions
LL conceived the study. LL, DC, ZZ, JY, MS,YC, MZ, YL,SS and JC reviewed and summarized the literatures. LL wrote the manuscript and drew all the figures. DC, ZZ and JC created the tables. LZ and JC supervised and revised the study and gave final approval of the version to be published. The final version of the manuscript was read and approved by all authors.
Funding
This study was supported by the National Natural Science Foundation of China (Grant No. 82274664), Department of Science and Technology of Sichuan Province (2023NSFSC1822), Sichuan Provincial central guiding local science and technology development special project (Grant No. 2021ZYD0103), and Innovation Team and Talents Cultivation Program of National Administration of Traditional Chinese Medicine (Grant No. ZYYCXTD-D-202003).
Acknowledgments
We acknowledge the assistance of Editage (www.editage.cn) for their professional editing services, which have significantly improved the English language quality of our manuscript. Moreover, we would like to extend our appreciation to Biorender for their essential role in the creation of Figure 1, Figure 2, Figure 3, Figure 4, and Figure 5.
Conflict of interest
The authors declare that the research was conducted in the absence of any commercial or financial relationships that could be construed as a potential conflict of interest.
Publisher’s note
All claims expressed in this article are solely those of the authors and do not necessarily represent those of their affiliated organizations, or those of the publisher, the editors and the reviewers. Any product that may be evaluated in this article, or claim that may be made by its manufacturer, is not guaranteed or endorsed by the publisher.
References
Ader, N. R., Hoffmann, P. C., Ganeva, I., Borgeaud, A. C., Wang, C., Youle, R. J., et al. (2019). Molecular and topological reorganizations in mitochondrial architecture interplay during Bax-mediated steps of apoptosis. Elife 8, e40712. doi:10.7554/eLife.40712
Ajoolabady, A., Wang, S., Kroemer, G., Penninger, J. M., Uversky, V. N., Pratico, D., et al. (2021). Targeting autophagy in ischemic stroke: From molecular mechanisms to clinical therapeutics. Pharmacol. Ther. 225, 107848. doi:10.1016/j.pharmthera.2021.107848
Alkahtani, S., Al-Johani, N. S., and Alarifi, S. (2023). Mechanistic insights, treatment paradigms, and clinical progress in neurological disorders: Current and future prospects. Int. J. Mol. Sci. 24 (2), 1340. doi:10.3390/ijms24021340
An, H., Tao, W., Liang, Y., Li, P., Li, M., Zhang, X., et al. (2021). Dengzhanxixin injection ameliorates cognitive impairment through a neuroprotective mechanism based on mitochondrial preservation in patients with acute ischemic stroke. Front. Pharmacol. 12, 712436. doi:10.3389/fphar.2021.712436
Andrabi, S. S., Parvez, S., and Tabassum, H. (2020). Ischemic stroke and mitochondria: Mechanisms and targets. Protoplasma 257 (2), 335–343. doi:10.1007/s00709-019-01439-2
Anzell, A. R., Maizy, R., Przyklenk, K., and Sanderson, T. H. (2018). Mitochondrial quality control and disease: Insights into ischemia-reperfusion injury. Mol. Neurobiol. 55 (3), 2547–2564. doi:10.1007/s12035-017-0503-9
Bai, X., Tan, T. Y., Li, Y. X., Li, Y., Chen, Y. F., Ma, R., et al. (2020). The protective effect of cordyceps sinensis extract on cerebral ischemic injury via modulating the mitochondrial respiratory chain and inhibiting the mitochondrial apoptotic pathway. Biomed. Pharmacother. 124, 109834. doi:10.1016/j.biopha.2020.109834
Cen, J., Zhang, R., Zhao, T., Zhang, X., Zhang, C., Cui, J., et al. (2022). A water-soluble quercetin conjugate with triple targeting exerts neuron-protective effect on cerebral ischemia by mitophagy activation. Adv. Healthc. Mater 11 (22), e2200817. doi:10.1002/adhm.202200817
Chen, A., Wang, H., Zhang, Y., Wang, X., Yu, L., Xu, W., et al. (2017). Paeoniflorin exerts neuroprotective effects against glutamate-induced PC12 cellular cytotoxicity by inhibiting apoptosis. Int. J. Mol. Med. 40 (3), 825–833. doi:10.3892/ijmm.2017.3076
Chen, B., Lin, W. Q., Li, Z. F., Zhong, X. Y., Wang, J., You, X. F., et al. (2021). Electroacupuncture attenuates ischemic brain injury and cellular apoptosis via mitochondrial translocation of cofilin. Chin. J. Integr. Med. 27 (9), 705–712. doi:10.1007/s11655-021-3335-4
Chen, S. D., Yang, D. I., Lin, T. K., Shaw, F. Z., Liou, C. W., and Chuang, Y. C. (2011). Roles of oxidative stress, apoptosis, PGC-1α and mitochondrial biogenesis in cerebral ischemia. Int. J. Mol. Sci. 12 (10), 7199–7215. doi:10.3390/ijms12107199
Chen, S., Sun, M., Zhao, X., Yang, Z., Liu, W., Cao, J., et al. (2019). Neuroprotection of hydroxysafflor yellow A in experimental cerebral ischemia/reperfusion injury via metabolic inhibition of phenylalanine and mitochondrial biogenesis. Mol. Med. Rep. 19 (4), 3009–3020. doi:10.3892/mmr.2019.9959
Cheng, C. Y., Ho, T. Y., Hsiang, C. Y., Tang, N. Y., Hsieh, C. L., Kao, S. T., et al. (2017). Angelica sinensis exerts angiogenic and anti-apoptotic effects against cerebral ischemia-reperfusion injury by activating p38MAPK/HIF-1[Formula: See text]/VEGF-A signaling in rats. Am. J. Chin. Med. 45 (8), 1683–1708. doi:10.1142/s0192415x17500914
Cheng, C. Y., Kao, S. T., and Lee, Y. C. (2019). Ferulic acid ameliorates cerebral infarction by activating Akt/mTOR/4E-BP1/Bcl-2 anti-apoptotic signaling in the penumbral cortex following permanent cerebral ischemia in rats. Mol. Med. Rep. 19 (2), 792–804. doi:10.3892/mmr.2018.9737
Cheng, C. Y., Lin, J. G., Tang, N. Y., Kao, S. T., and Hsieh, C. L. (2015). Electroacupuncture at different frequencies (5Hz and 25Hz) ameliorates cerebral ischemia-reperfusion injury in rats: Possible involvement of p38 MAPK-mediated anti-apoptotic signaling pathways. BMC Complement. Altern. Med. 15, 241. doi:10.1186/s12906-015-0752-y
Cohen, M. M., and Tareste, D. (2018). Recent insights into the structure and function of Mitofusins in mitochondrial fusion. F1000Res 7, F1000. doi:10.12688/f1000research.16629.1
Cui, W. H., Zhang, H. H., Qu, Z. M., Wang, Z., Zhang, D. J., and Wang, S. (2022). Effects of chrysophanol on hippocampal damage and mitochondrial autophagy in mice with cerebral ischemia reperfusion. Int. J. Neurosci. 132 (6), 613–620. doi:10.1080/00207454.2020.1830085
Dohare, P., Garg, P., Sharma, U., Jagannathan, N. R., and Ray, M. (2008a). Neuroprotective efficacy and therapeutic window of curcuma oil: In rat embolic stroke model. BMC Complement. Altern. Med. 8, 55. doi:10.1186/1472-6882-8-55
Dohare, P., Varma, S., and Ray, M. (2008b). Curcuma oil modulates the nitric oxide system response to cerebral ischemia/reperfusion injury. Nitric Oxide 19 (1), 1–11. doi:10.1016/j.niox.2008.04.020
Du, Q., Deng, R., Li, W., Zhang, D., Tsoi, B., and Shen, J. (2021). Baoyuan Capsule promotes neurogenesis and neurological functional recovery through improving mitochondrial function and modulating PI3K/Akt signaling pathway. Phytomedicine 93, 153795. doi:10.1016/j.phymed.2021.153795
Engin, A., and Engin, A. B. (2021). N-Methyl-D-Aspartate receptor signaling-protein kinases crosstalk in cerebral ischemia. Adv. Exp. Med. Biol. 1275, 259–283. doi:10.1007/978-3-030-49844-3_10
Feng, C., Wan, H., Zhang, Y., Yu, L., Shao, C., He, Y., et al. (2020). Neuroprotective effect of Danhong injection on cerebral ischemia-reperfusion injury in rats by activation of the PI3K-Akt pathway. Front. Pharmacol. 11, 298. doi:10.3389/fphar.2020.00298
Feng, L., Gao, J., Liu, Y., Shi, J., and Gong, Q. (2018). Icariside II alleviates oxygen-glucose deprivation and reoxygenation-induced PC12 cell oxidative injury by activating Nrf2/SIRT3 signaling pathway. Biomed. Pharmacother. 103, 9–17. doi:10.1016/j.biopha.2018.04.005
Giorgi, C., Marchi, S., Simoes, I. C. M., Ren, Z., Morciano, G., Perrone, M., et al. (2018). Mitochondria and reactive oxygen species in aging and age-related diseases. Int. Rev. Cell. Mol. Biol. 340, 209–344. doi:10.1016/bs.ircmb.2018.05.006
Guida, M., Zanon, A., Montibeller, L., Lavdas, A. A., Ladurner, J., Pischedda, F., et al. (2019). Parkin interacts with apoptosis-inducing factor and interferes with its translocation to the nucleus in neuronal cells. Int. J. Mol. Sci. 20 (3), 748. doi:10.3390/ijms20030748
Guo, C., and Ma, Y. Y. (2021). Calcium permeable-AMPA receptors and excitotoxicity in neurological disorders. Front. Neural Circuits 15, 711564. doi:10.3389/fncir.2021.711564
He, J., Liu, J., Huang, Y., Zhuo, Y., Chen, W., Duan, D., et al. (2020a). Olfactory mucosa mesenchymal stem cells alleviate cerebral ischemia/reperfusion injury via golgi apparatus secretory pathway Ca(2+) -ATPase Isoform1. Front. Cell. Dev. Biol. 8, 586541. doi:10.3389/fcell.2020.586541
He, M., Ma, Y., Wang, R., Zhang, J., Jing, L., and Li, P. A. (2020b). Deletion of mitochondrial uncoupling protein 2 exacerbates mitochondrial damage in mice subjected to cerebral ischemia and reperfusion injury under both normo- and hyperglycemic conditions. Int. J. Biol. Sci. 16 (15), 2788–2802. doi:10.7150/ijbs.48204
Hou, Y., Qieni, X., Li, N., Bai, J., Li, R., Gongbao, D., et al. (2020). Longzhibu disease and its therapeutic effects by traditional Tibetan medicine: Ershi-wei Chenxiang pills. J. Ethnopharmacol. 249, 112426. doi:10.1016/j.jep.2019.112426
Hu, J., Zeng, C., Wei, J., Duan, F., Liu, S., Zhao, Y., et al. (2020). The combination of Panax ginseng and Angelica sinensis alleviates ischemia brain injury by suppressing NLRP3 inflammasome activation and microglial pyroptosis. Phytomedicine 76, 153251. doi:10.1016/j.phymed.2020.153251
Hu, S., Hu, H., Mak, S., Cui, G., Lee, M., Shan, L., et al. (2018). A novel tetramethylpyrazine derivative prophylactically protects against glutamate-induced excitotoxicity in primary neurons through the blockage of N-Methyl-D-aspartate receptor. Front. Pharmacol. 9, 73. doi:10.3389/fphar.2018.00073
Huang, P., Wu, S. P., Wang, N., Seto, S., and Chang, D. (2021). Hydroxysafflor yellow A alleviates cerebral ischemia reperfusion injury by suppressing apoptosis via mitochondrial permeability transition pore. Phytomedicine 85, 153532. doi:10.1016/j.phymed.2021.153532
Huang, Q., Lou, T., Wang, M., Xue, L., Lu, J., Zhang, H., et al. (2020). Compound K inhibits autophagy-mediated apoptosis induced by oxygen and glucose deprivation/reperfusion via regulating AMPK-mTOR pathway in neurons. Life Sci. 254, 117793. doi:10.1016/j.lfs.2020.117793
Huang, X. P., Tan, H., Chen, B. Y., and Deng, C. Q. (2012). Astragalus extract alleviates nerve injury after cerebral ischemia by improving energy metabolism and inhibiting apoptosis. Biol. Pharm. Bull. 35 (4), 449–454. doi:10.1248/bpb.35.449
Huang, X. P., Tan, H., Chen, B. Y., and Deng, C. Q. (2017). Combination of total Astragalus extract and total Panax notoginseng saponins strengthened the protective effects on brain damage through improving energy metabolism and inhibiting apoptosis after cerebral ischemia-reperfusion in mice. Chin. J. Integr. Med. 23 (6), 445–452. doi:10.1007/s11655-015-1965-0
Jakic, B., Carlsson, M., Buszko, M., Cappellano, G., Ploner, C., Onestingel, E., et al. (2019). The effects of endurance exercise and diet on atherosclerosis in young and aged ApoE-/- and wild-type mice. Gerontology 65 (1), 45–56. doi:10.1159/000492571
Ji, Z. J., Shi, Y., Li, X., Hou, R., Yang, Y., Liu, Z. Q., et al. (2022). Neuroprotective effect of taohong Siwu decoction on cerebral ischemia/reperfusion injury via mitophagy-NLRP3 inflammasome pathway. Front. Pharmacol. 13, 910217. doi:10.3389/fphar.2022.910217
Jia, Y., Chen, X., Chen, Y., Li, H., Ma, X., Xing, W., et al. (2021). Zhenbao pill attenuates hydrogen peroxide-induced apoptosis by inhibiting autophagy in human umbilical vein endothelial cells. J. Ethnopharmacol. 274, 114020. doi:10.1016/j.jep.2021.114020
Kaarniranta, K., Kajdanek, J., Morawiec, J., Pawlowska, E., and Blasiak, J. (2018). PGC-1α protects RPE cells of the aging retina against oxidative stress-induced degeneration through the regulation of senescence and mitochondrial quality control the significance for AMD pathogenesis. Int. J. Mol. Sci. 19 (8), 2317. doi:10.3390/ijms19082317
Kaushik, P., Ali, M., Salman, M., Tabassum, H., and Parvez, S. (2021). Harnessing the mitochondrial integrity for neuroprotection: Therapeutic role of piperine against experimental ischemic stroke. Neurochem. Int. 149, 105138. doi:10.1016/j.neuint.2021.105138
Kim, Y. R., Kim, H. N., Jang, J. Y., Park, C., Lee, J. H., Shin, H. K., et al. (2013). Effects of electroacupuncture on apoptotic pathways in a rat model of focal cerebral ischemia. Int. J. Mol. Med. 32 (6), 1303–1310. doi:10.3892/ijmm.2013.1511
Lan, R., Zhang, Y., Wu, T., Ma, Y. Z., Wang, B. Q., Zheng, H. Z., et al. (2018). Xiao-xu-ming decoction reduced mitophagy activation and improved mitochondrial function in cerebral ischemia and reperfusion injury. Behav. Neurol. 2018, 4147502. doi:10.1155/2018/4147502
Lan, R., Zhang, Y., Xiang, J., Zhang, W., Wang, G. H., Li, W. W., et al. (2014). Xiao-Xu-Ming decoction preserves mitochondrial integrity and reduces apoptosis after focal cerebral ischemia and reperfusion via the mitochondrial p53 pathway. J. Ethnopharmacol. 151 (1), 307–316. doi:10.1016/j.jep.2013.10.042
Lei, T., Li, H., Fang, Z., Lin, J., Wang, S., Xiao, L., et al. (2014). Polysaccharides from Angelica sinensis alleviate neuronal cell injury caused by oxidative stress. Neural Regen. Res. 9 (3), 260–267. doi:10.4103/1673-5374.128218
Li, C., Chen, C., Qin, H., Ao, C., Chen, J., Tan, J., et al. (2022a). The role of mitochondrial dynamin in stroke. Oxid. Med. Cell. Longev. 2022, 2504798. doi:10.1155/2022/2504798
Li, F., Tan, J., Zhou, F., Hu, Z., and Yang, B. (2018a). Heat shock protein B8 (HSPB8) reduces oxygen-glucose deprivation/reperfusion injury via the induction of mitophagy. Cell. Physiol. Biochem. 48 (4), 1492–1504. doi:10.1159/000492259
Li, G., Li, X., Dong, J., and Han, Y. (2021a). Electroacupuncture ameliorates cerebral ischemic injury by inhibiting ferroptosis. Front. Neurol. 12, 619043. doi:10.3389/fneur.2021.619043
Li, H., Han, W., Wang, H., Ding, F., Xiao, L., Shi, R., et al. (2017). Tanshinone IIA inhibits glutamate-induced oxidative toxicity through prevention of mitochondrial dysfunction and suppression of MAPK activation in SH-SY5Y human neuroblastoma cells. Oxid. Med. Cell. Longev. 2017, 4517486. doi:10.1155/2017/4517486
Li, J., Bu, Y., Li, B., Zhang, H., Guo, J., Hu, J., et al. (2022b). Calenduloside E alleviates cerebral ischemia/reperfusion injury by preserving mitochondrial function. J. Mol. Histol. 53 (4), 713–727. doi:10.1007/s10735-022-10087-5
Li, J., Zhao, T., Qiao, H., Li, Y., Xia, M., Wang, X., et al. (2022c). Research progress of natural products for the treatment of ischemic stroke. J. Integr. Neurosci. 21 (1), 14. doi:10.31083/j.jin2101014
Li, S., Peng, T., Zhao, X., Silva, M., Liu, L., Zhou, W., et al. (2021b). Artemether confers neuroprotection on cerebral ischemic injury through stimulation of the Erk1/2-P90rsk-CREB signaling pathway. Redox Biol. 46, 102069. doi:10.1016/j.redox.2021.102069
Li, S., Wang, T., Zhai, L., Ge, K., Zhao, J., Cong, W., et al. (2018b). Picroside II exerts a neuroprotective effect by inhibiting mPTP permeability and EndoG release after cerebral ischemia/reperfusion injury in rats. J. Mol. Neurosci. 64 (1), 144–155. doi:10.1007/s12031-017-1012-z
Li, W. H., Yang, Y. L., Cheng, X., Liu, M., Zhang, S. S., Wang, Y. H., et al. (2020). Baicalein attenuates caspase-independent cells death via inhibiting PARP-1 activation and AIF nuclear translocation in cerebral ischemia/reperfusion rats. Apoptosis 25 (5-6), 354–369. doi:10.1007/s10495-020-01600-w
Li, W., Li, P., Liu, Z., Du, Q., Steinmetz, A., Wang, N., et al. (2014). A Chinese medicine preparation induces neuroprotection by regulating paracrine signaling of brain microvascular endothelial cells. J. Ethnopharmacol. 151 (1), 686–693. doi:10.1016/j.jep.2013.11.035
Li, X., Zhang, D., Bai, Y., Xiao, J., Jiao, H., and He, R. (2019). Ginaton improves neurological function in ischemic stroke rats via inducing autophagy and maintaining mitochondrial homeostasis. Neuropsychiatr. Dis. Treat. 15, 1813–1822. doi:10.2147/ndt.S205612
Lin, G. H., Lin, L., Liang, H. W., Ma, X., Wang, J. Y., Wu, L. P., et al. (2010). Antioxidant action of a Chrysanthemum morifolium extract protects rat brain against ischemia and reperfusion injury. J. Med. Food 13 (2), 306–311. doi:10.1089/jmf.2009.1184
Liu, B., Zhao, T., Li, Y., Han, Y., Xu, Y., Yang, H., et al. (2022). Notoginsenoside R1 ameliorates mitochondrial dysfunction to circumvent neuronal energy failure in acute phase of focal cerebral ischemia. Phytother. Res. 36 (5), 2223–2235. doi:10.1002/ptr.7450
Liu, Q., Li, X., Li, L., Xu, Z., Zhou, J., and Xiao, W. (2018). Ginkgolide K protects SH-SY5Y cells against oxygen-glucose deprivation-induced injury by inhibiting the p38 and JNK signaling pathways. Mol. Med. Rep. 18 (3), 3185–3192. doi:10.3892/mmr.2018.9305
Liu, W. J., Jiang, H. F., Rehman, F. U., Zhang, J. W., Chang, Y., Jing, L., et al. (2017). Lycium barbarum polysaccharides decrease hyperglycemia-aggravated ischemic brain injury through maintaining mitochondrial fission and fusion balance. Int. J. Biol. Sci. 13 (7), 901–910. doi:10.7150/ijbs.18404
Lu, F., Wang, L., Chen, Y., Zhong, X., and Huang, Z. (2020). In vitro cultured calculus bovis attenuates cerebral ischaemia-reperfusion injury by inhibiting neuronal apoptosis and protecting mitochondrial function in rats. J. Ethnopharmacol. 263, 113168. doi:10.1016/j.jep.2020.113168
Luan, Y., Yang, D., Zhang, Z., Bie, X., Zhao, H., Wang, Y., et al. (2021). Association study between genetic variation in whole mitochondrial genome and ischemic stroke. J. Mol. Neurosci. 71 (10), 2152–2162. doi:10.1007/s12031-020-01778-3
Ma, H. X., Hou, F., Chen, A. L., Li, T. T., Zhu, Y. F., and Zhao, Q. P. (2020). Mu-Xiang-You-Fang protects PC12 cells against OGD/R-induced autophagy via the AMPK/mTOR signaling pathway. J. Ethnopharmacol. 252, 112583. doi:10.1016/j.jep.2020.112583
Ma, Y., Zhao, P., Zhu, J., Yan, C., Li, L., Zhang, H., et al. (2016). Naoxintong protects primary neurons from oxygen-glucose deprivation/reoxygenation induced injury through PI3K-Akt signaling pathway. Evid. Based Complement. Altern. Med. 2016, 5815946. doi:10.1155/2016/5815946
Mao, C., Hu, C., Zhou, Y., Zou, R., Li, S., Cui, Y., et al. (2020). Electroacupuncture pretreatment against cerebral ischemia/reperfusion injury through mitophagy. Evid. Based Complement. Altern. Med. 2020, 7486041. doi:10.1155/2020/7486041
Mao, Z., Tian, L., Liu, J., Wu, Q., Wang, N., Wang, G., et al. (2022). Ligustilide ameliorates hippocampal neuronal injury after cerebral ischemia reperfusion through activating PINK1/Parkin-dependent mitophagy. Phytomedicine 101, 154111. doi:10.1016/j.phymed.2022.154111
Meng, X., Xie, W., Xu, Q., Liang, T., Xu, X., Sun, G., et al. (2018). Neuroprotective effects of radix scrophulariae on cerebral ischemia and reperfusion injury via MAPK pathways. Molecules 23 (9), 2401. doi:10.3390/molecules23092401
Mondal, N. K., Behera, J., Kelly, K. E., George, A. K., Tyagi, P. K., and Tyagi, N. (2019). Tetrahydrocurcumin epigenetically mitigates mitochondrial dysfunction in brain vasculature during ischemic stroke. Neurochem. Int. 122, 120–138. doi:10.1016/j.neuint.2018.11.015
Mukherjee, A., Sarkar, S., Jana, S., Swarnakar, S., and Das, N. (2019). Neuro-protective role of nanocapsulated curcumin against cerebral ischemia-reperfusion induced oxidative injury. Brain Res. 1704, 164–173. doi:10.1016/j.brainres.2018.10.016
Nan, L., Xie, Q., Chen, Z., Zhang, Y., Chen, Y., Li, H., et al. (2020). Involvement of PARP-1/AIF signaling pathway in protective effects of Gualou Guizhi decoction against ischemia-reperfusion injury-induced apoptosis. Neurochem. Res. 45 (2), 278–294. doi:10.1007/s11064-019-02912-3
Ni, X. C., Wang, H. F., Cai, Y. Y., Yang, D., Alolga, R. N., Liu, B., et al. (2022). Ginsenoside Rb1 inhibits astrocyte activation and promotes transfer of astrocytic mitochondria to neurons against ischemic stroke. Redox Biol. 54, 102363. doi:10.1016/j.redox.2022.102363
Orgah, J. O., Ren, J., Liu, X., Orgah, E. A., Gao, X. M., and Zhu, Y. (2019). Danhong injection facilitates recovery of post-stroke motion deficit via Parkin-enhanced mitochondrial function. Restor. Neurol. Neurosci. 37 (4), 375–395. doi:10.3233/rnn-180828
Park, H. R., Lee, H., Lee, J. J., Yim, N. H., Gu, M. J., and Ma, J. Y. (2018). Protective effects of spatholobi caulis extract on neuronal damage and focal ischemic stroke/reperfusion injury. Mol. Neurobiol. 55 (6), 4650–4666. doi:10.1007/s12035-017-0652-x
Peng, T., Li, S., Liu, L., Yang, C., Farhan, M., Chen, L., et al. (2022). Artemisinin attenuated ischemic stroke induced cell apoptosis through activation of ERK1/2/CREB/BCL-2 signaling pathway in vitro and in vivo. Int. J. Biol. Sci. 18 (11), 4578–4594. doi:10.7150/ijbs.69892
Quintana, D. D., Garcia, J. A., Sarkar, S. N., Jun, S., Engler-Chiurazzi, E. B., Russell, A. E., et al. (2019). Hypoxia-reoxygenation of primary astrocytes results in a redistribution of mitochondrial size and mitophagy. Mitochondrion 47, 244–255. doi:10.1016/j.mito.2018.12.004
Rutkai, I., Merdzo, I., Wunnava, S. V., Curtin, G. T., Katakam, P. V., and Busija, D. W. (2019). Cerebrovascular function and mitochondrial bioenergetics after ischemia-reperfusion in male rats. J. Cereb. Blood Flow. Metab. 39 (6), 1056–1068. doi:10.1177/0271678x17745028
Ryoo, I. G., and Kwak, M. K. (2018). Regulatory crosstalk between the oxidative stress-related transcription factor Nfe2l2/Nrf2 and mitochondria. Toxicol. Appl. Pharmacol. 359, 24–33. doi:10.1016/j.taap.2018.09.014
Sarmah, D., Datta, A., Raut, S., Sarkar, A., Shah, B., Bohra, M., et al. (2020). The role of inflammasomes in atherosclerosis and stroke pathogenesis. Curr. Pharm. Des. 26 (34), 4234–4245. doi:10.2174/1381612826666200427084949
Shao, Z., Dou, S., Zhu, J., Wang, H., Xu, D., Wang, C., et al. (2020). The role of mitophagy in ischemic stroke. Front. Neurol. 11, 608610. doi:10.3389/fneur.2020.608610
Shen, J., Zhu, Y., Huang, K., Jiang, H., Shi, C., Xiong, X., et al. (2016). Buyang Huanwu Decoction attenuates H2O2-induced apoptosis by inhibiting reactive oxygen species-mediated mitochondrial dysfunction pathway in human umbilical vein endothelial cells. BMC Complement. Altern. Med. 16, 154. doi:10.1186/s12906-016-1152-7
Shen, L., Gan, Q., Yang, Y., Reis, C., Zhang, Z., Xu, S., et al. (2021). Mitophagy in cerebral ischemia and ischemia/reperfusion injury. Front. Aging Neurosci. 13, 687246. doi:10.3389/fnagi.2021.687246
Shi, Z., Zhu, L., Li, T., Tang, X., Xiang, Y., Han, X., et al. (2017). Neuroprotective mechanisms of Lycium barbarum polysaccharides against ischemic insults by regulating NR2B and NR2A containing NMDA receptor signaling pathways. Front. Cell. Neurosci. 11, 288. doi:10.3389/fncel.2017.00288
Song, M., Zhou, Y., and Fan, X. (2022a). Mitochondrial quality and quantity control: Mitophagy is a potential therapeutic target for ischemic stroke. Mol. Neurobiol. 59 (5), 3110–3123. doi:10.1007/s12035-022-02795-6
Song, Z., Huang, Q., Guo, Y., Song, X., Zhang, X., and Xiao, H. (2022b). Xingnao kaiqiao acupuncture method combined with temporal three-needle in the treatment of acute ischemic stroke: A randomized controlled trial. Comput. Intell. Neurosci. 2022, 8145374. doi:10.1155/2022/8145374
Su, S. H., Wu, Y. F., Wang, D. P., and Hai, J. (2018). Inhibition of excessive autophagy and mitophagy mediates neuroprotective effects of URB597 against chronic cerebral hypoperfusion. Cell. Death Dis. 9 (7), 733. doi:10.1038/s41419-018-0755-y
Sun, S., Jiang, T., Duan, N., Wu, M., Yan, C., Li, Y., et al. (2021). Activation of CB1R-dependent PGC-1α is involved in the improved mitochondrial biogenesis induced by electroacupuncture pretreatment. Rejuvenation Res. 24 (2), 104–119. doi:10.1089/rej.2020.2315
Sun, Z. Y., Wang, F. J., Guo, H., Chen, L., Chai, L. J., Li, R. L., et al. (2019). Shuxuetong injection protects cerebral microvascular endothelial cells against oxygen-glucose deprivation reperfusion. Neural Regen. Res. 14 (5), 783–793. doi:10.4103/1673-5374.249226
Tian, W. Q., Peng, Y. G., Cui, S. Y., Yao, F. Z., and Li, B. G. (2015). Effects of electroacupuncture of different intensities on energy metabolism of mitochondria of brain cells in rats with cerebral ischemia-reperfusion injury. Chin. J. Integr. Med. 21 (8), 618–623. doi:10.1007/s11655-013-1512-9
Tian, W., Zhu, M., Zhou, Y., Mao, C., Zou, R., Cui, Y., et al. (2022). Electroacupuncture pretreatment alleviates cerebral ischemia-reperfusion injury by regulating mitophagy via mTOR-ULK1/FUNDC1 Axis in rats. J. Stroke Cerebrovasc. Dis. 31 (1), 106202. doi:10.1016/j.jstrokecerebrovasdis.2021.106202
Ting, Z., Jianbin, Z., and Luqi, H. (2017). Protective effect of electroacupuncture on neurons autophagy in perfusion period of cerebral ischemia. Neurosci. Lett. 661, 41–45. doi:10.1016/j.neulet.2017.06.043
Virani, S. S., Alonso, A., Benjamin, E. J., Bittencourt, M. S., Callaway, C. W., Carson, A. P., et al. (2020). Heart disease and stroke statistics-2020 update: A report from the American heart association. Circulation 141 (9), e139–e596. doi:10.1161/cir.0000000000000757
Wang, D., Yuan, X., Liu, T., Liu, L., Hu, Y., Wang, Z., et al. (2012). Neuroprotective activity of lavender oil on transient focal cerebral ischemia in mice. Molecules 17 (8), 9803–9817. doi:10.3390/molecules17089803
Wang, H., Chen, S., Zhang, Y., Xu, H., and Sun, H. (2019a). Electroacupuncture ameliorates neuronal injury by Pink1/Parkin-mediated mitophagy clearance in cerebral ischemia-reperfusion. Nitric Oxide 91, 23–34. doi:10.1016/j.niox.2019.07.004
Wang, H. Y., Zhou, H. F., He, Y., Yu, L., Li, C., Yang, J. H., et al. (2021a). Protective effect of Naoxintong capsule combined with Guhong injection on rat brain microvascular endothelial cells during cerebral ischemia-reperfusion injury. Chin. J. Integr. Med. 27 (10), 744–751. doi:10.1007/s11655-020-3215-3
Wang, H., Zhu, J., Jiang, L., Shan, B., Xiao, P., Ai, J., et al. (2020a). Mechanism of Heshouwuyin inhibiting the Cyt c/Apaf-1/Caspase-9/Caspase-3 pathway in spermatogenic cell apoptosis. BMC Complement. Med. Ther. 20 (1), 180. doi:10.1186/s12906-020-02904-9
Wang, J. K., Guo, Q., Zhang, X. W., Wang, L. C., Liu, Q., Tu, P. F., et al. (2020b). Aglaia odorata Lour. extract inhibit ischemic neuronal injury potentially via suppressing p53/Puma-mediated mitochondrial apoptosis pathway. J. Ethnopharmacol. 248, 112336. doi:10.1016/j.jep.2019.112336
Wang, P., Dai, L., Zhou, W., Meng, J., Zhang, M., Wu, Y., et al. (2019b). Intermodule coupling analysis of huang-lian-jie-du decoction on stroke. Front. Pharmacol. 10, 1288. doi:10.3389/fphar.2019.01288
Wang, P. R., Wang, J. S., Yang, M. H., and Kong, L. Y. (2014a). Neuroprotective effects of Huang-Lian-Jie-Du-Decoction on ischemic stroke rats revealed by (1)H NMR metabolomics approach. J. Pharm. Biomed. Anal. 88, 106–116. doi:10.1016/j.jpba.2013.08.025
Wang, T., Li, Y., Wang, Y., Zhou, R., Ma, L., Hao, Y., et al. (2014b). Lycium barbarum polysaccharide prevents focal cerebral ischemic injury by inhibiting neuronal apoptosis in mice. PLoS One 9 (3), e90780. doi:10.1371/journal.pone.0090780
Wang, W., and Xu, J. (2020). Curcumin attenuates cerebral ischemia-reperfusion injury through regulating mitophagy and preserving mitochondrial function. Curr. Neurovasc Res. 17 (2), 113–122. doi:10.2174/1567202617666200225122620
Wang, Y., Li, P., Zhang, X., Li, L., Liu, M., Li, X., et al. (2021b). Mitochondrial-respiration-improving effects of three different gardeniae fructus preparations and their components. ACS Omega 6 (50), 34229–34241. doi:10.1021/acsomega.1c03265
Wei, W., Lu, M., Lan, X. B., Liu, N., Su, W. K., Dushkin, A. V., et al. (2021). Neuroprotective effects of oxymatrine on PI3K/Akt/mTOR pathway after hypoxic-ischemic brain damage in neonatal rats. Front. Pharmacol. 12, 642415. doi:10.3389/fphar.2021.642415
Wu, B., Luo, H., Zhou, X., Cheng, C. Y., Lin, L., Liu, B. L., et al. (2017). Succinate-induced neuronal mitochondrial fission and hexokinase II malfunction in ischemic stroke: Therapeutical effects of kaempferol. Biochim. Biophys. Acta Mol. Basis Dis. 1863 (9), 2307–2318. doi:10.1016/j.bbadis.2017.06.011
Wu, M., Lu, G., Lao, Y. Z., Zhang, H., Zheng, D., Zheng, Z. Q., et al. (2021). Garciesculenxanthone B induces PINK1-Parkin-mediated mitophagy and prevents ischemia-reperfusion brain injury in mice. Acta Pharmacol. Sin. 42 (2), 199–208. doi:10.1038/s41401-020-0480-9
Xiang, J., Zhang, J., Cai, X., Yang, F., Zhu, W., Zhang, W., et al. (2019). Bilobalide protects astrocytes from oxygen and glucose deprivation-induced oxidative injury by upregulating manganese superoxide dismutase. Phytother. Res. 33 (9), 2329–2336. doi:10.1002/ptr.6414
Xie, W., Zhu, T., Zhou, P., Xu, H., Meng, X., Ding, T., et al. (2020). Notoginseng leaf triterpenes ameliorates OGD/R-Induced neuronal injury via SIRT1/2/3-foxo3a-MnSOD/PGC-1α signaling pathways mediated by the NAMPT-NAD pathway. Oxid. Med. Cell. Longev. 2020, 7308386. doi:10.1155/2020/7308386
Xu, Y., Wang, Y., Wang, G., Ye, X., Zhang, J., Cao, G., et al. (2017). YiQiFuMai powder injection protects against ischemic stroke via inhibiting neuronal apoptosis and pkcδ/drp1-mediated excessive mitochondrial fission. Oxid. Med. Cell. Longev. 2017, 1832093. doi:10.1155/2017/1832093
Xue, B., Huang, J., Ma, B., Yang, B., Chang, D., and Liu, J. (2019). Astragaloside IV protects primary cerebral cortical neurons from oxygen and glucose deprivation/reoxygenation by activating the PKA/CREB pathway. Neuroscience 404, 326–337. doi:10.1016/j.neuroscience.2019.01.040
Yang, J. L., Mukda, S., and Chen, S. D. (2018). Diverse roles of mitochondria in ischemic stroke. Redox Biol. 16, 263–275. doi:10.1016/j.redox.2018.03.002
Yang, S., Zhao, X., Xu, H., Chen, F., Xu, Y., Li, Z., et al. (2017). AKT2 blocks nucleus translocation of apoptosis-inducing factor (AIF) and endonuclease G (EndoG) while promoting caspase activation during cardiac ischemia. Int. J. Mol. Sci. 18 (3), 565. doi:10.3390/ijms18030565
Yang, Y., Gao, H., Liu, W., Liu, X., Jiang, X., Li, X., et al. (2021). Arctium lappa L. roots ameliorates cerebral ischemia through inhibiting neuronal apoptosis and suppressing AMPK/mTOR-mediated autophagy. Phytomedicine 85, 153526. doi:10.1016/j.phymed.2021.153526
Ye, M., Wu, H., and Li, S. (2021). Resveratrol alleviates oxygen/glucose deprivation/reoxygenation-induced neuronal damage through induction of mitophagy. Mol. Med. Rep. 23 (1), 73. doi:10.3892/mmr.2020.11711
Ye, R., Zhang, X., Kong, X., Han, J., Yang, Q., Zhang, Y., et al. (2011). Ginsenoside Rd attenuates mitochondrial dysfunction and sequential apoptosis after transient focal ischemia. Neuroscience 178, 169–180. doi:10.1016/j.neuroscience.2011.01.007
Ye, Y., Li, J., Cao, X., Chen, Y., Ye, C., and Chen, K. (2016). Protective effect of n-butyl alcohol extracts from Rhizoma Pinelliae Pedatisectae against cerebral ischemia-reperfusion injury in rats. J. Ethnopharmacol. 188, 259–265. doi:10.1016/j.jep.2016.04.046
Yin, F., Zhou, H., Fang, Y., Li, C., He, Y., Yu, L., et al. (2020). Astragaloside IV alleviates ischemia reperfusion-induced apoptosis by inhibiting the activation of key factors in death receptor pathway and mitochondrial pathway. J. Ethnopharmacol. 248, 112319. doi:10.1016/j.jep.2019.112319
Zeng, M., Shao, C., Zhou, H., He, Y., Li, W., Zeng, J., et al. (2021a). Protocatechudehyde improves mitochondrial energy metabolism through the HIF1α/PDK1 signaling pathway to mitigate ischemic stroke-elicited internal capsule injury. J. Ethnopharmacol. 277, 114232. doi:10.1016/j.jep.2021.114232
Zeng, M., Zhou, H., He, Y., Wang, Z., Shao, C., Yin, J., et al. (2021b). Danhong injection alleviates cerebral ischemia/reperfusion injury by improving intracellular energy metabolism coupling in the ischemic penumbra. Biomed. Pharmacother. 140, 111771. doi:10.1016/j.biopha.2021.111771
Zhang, C., Wang, J., Zhu, J., Chen, Y., and Han, X. (2020a). Microcystin-leucine-arginine induced neurotoxicity by initiating mitochondrial fission in hippocampal neurons. Sci. Total Environ. 703, 134702. doi:10.1016/j.scitotenv.2019.134702
Zhang, F. B., Wang, J. P., Zhang, H. X., Fan, G. M., and Cui, X. (2019). Effect of β-patchoulene on cerebral ischemia-reperfusion injury and the TLR4/NF-κB signaling pathway. Exp. Ther. Med. 17 (5), 3335–3342. doi:10.3892/etm.2019.7374
Zhang, G. F., Yang, P., Yin, Z., Chen, H. L., Ma, F. G., Wang, B., et al. (2018a). Electroacupuncture preconditioning protects against focal cerebral ischemia/reperfusion injury via suppression of dynamin-related protein 1. Neural Regen. Res. 13 (1), 86–93. doi:10.4103/1673-5374.224373
Zhang, J., Li, L., Chen, X., Zhang, B., Wang, Y., and Yamamoto, K. (2000). Effects of a traditional Chinese medicine, Qing Nao Yi Zhi Fang, on glutamate excitotoxicity in rat fetal cerebral neuronal cells in primary culture. Neurosci. Lett. 290 (1), 21–24. doi:10.1016/s0304-3940(00)01311-2
Zhang, S., Qi, Y., Xu, Y., Han, X., Peng, J., Liu, K., et al. (2013). Protective effect of flavonoid-rich extract from Rosa laevigata Michx on cerebral ischemia-reperfusion injury through suppression of apoptosis and inflammation. Neurochem. Int. 63 (5), 522–532. doi:10.1016/j.neuint.2013.08.008
Zhang, S., Zhou, Y., Li, R., Chen, Z., and Fan, X. (2022a). Advanced drug delivery system against ischemic stroke. J. Control Release 344, 173–201. doi:10.1016/j.jconrel.2022.02.036
Zhang, W., Wen, J., Jiang, Y., Hu, Q., Wang, J., Wei, S., et al. (2021). l-Borneol ameliorates cerebral ischaemia by downregulating the mitochondrial calcium uniporter-induced apoptosis cascade in pMCAO rats. J. Pharm. Pharmacol. 73 (2), 272–280. doi:10.1093/jpp/rgaa028
Zhang, X., Zeng, W., Zhang, Y., Yu, Q., Zeng, M., Gan, J., et al. (2022b). Focus on the role of mitochondria in NLRP3 inflammasome activation: A prospective target for the treatment of ischemic stroke (review). Int. J. Mol. Med. 49 (6), 74. doi:10.3892/ijmm.2022.5130
Zhang, X., Zhang, Y., Tang, S., Yu, L., Zhao, Y., Ren, Q., et al. (2018b). Pien-Tze-Huang protects cerebral ischemic injury by inhibiting neuronal apoptosis in acute ischemic stroke rats. J. Ethnopharmacol. 219, 117–125. doi:10.1016/j.jep.2018.03.018
Zhang, Y., Cao, M., Wu, Y., Wang, J., Zheng, J., Liu, N., et al. (2020b). Improvement in mitochondrial function underlies the effects of ANNAO tablets on attenuating cerebral ischemia-reperfusion injuries. J. Ethnopharmacol. 246, 112212. doi:10.1016/j.jep.2019.112212
Zhang, Y., Yan, Y., Cao, Y., Yang, Y., Zhao, Q., Jing, R., et al. (2017a). Potential therapeutic and protective effect of curcumin against stroke in the male albino stroke-induced model rats. Life Sci. 183, 45–49. doi:10.1016/j.lfs.2017.06.023
Zhang, Z., Liu, Y., Zhang, G., Shi, F., Chen, H., Yin, Z., et al. (2017b). Effects of electroacupuncture preconditioning on activity of dynamin-related protein 1 in brain tissues during cerebral ischemia-reperfusion in rats. Chin. J. Anesthesiol. 37 (12), 1498–1501. doi:10.3760/cma.j.issn.0254-1416.2017.12.023
Zhao, P., Ma, N. T., Chang, R. Y., Li, Y. X., Hao, Y. J., Yang, W. L., et al. (2017). Mechanism of Lycium barbarum polysaccharides on primary cultured rat hippocampal neurons. Cell. Tissue Res. 369 (3), 455–465. doi:10.1007/s00441-017-2648-2
Zhao, Q., Chen, A., Wang, X., Zhang, Z., Zhao, Y., Huang, Y., et al. (2018a). Protective effects of dehydrocostuslactone on rat hippocampal slice injury induced by oxygen-glucose deprivation/reoxygenation. Int. J. Mol. Med. 42 (2), 1190–1198. doi:10.3892/ijmm.2018.3691
Zhao, Q., Wang, X., Chen, A., Cheng, X., Zhang, G., Sun, J., et al. (2018b). Rhein protects against cerebral ischemic-/reperfusion-induced oxidative stress and apoptosis in rats. Int. J. Mol. Med. 41 (5), 2802–2812. doi:10.3892/ijmm.2018.3488
Zhao, X. Y., Wang, X. Y., Wei, Q. Y., Xu, Y. M., and Lau, A. T. Y. (2020). Potency and selectivity of SMAC/DIABLO mimetics in solid tumor therapy. Cells 9 (4), 1012. doi:10.3390/cells9041012
Zhong, S., Li, Z., Huan, L., and Chen, B. Y. (2009). Neurochemical mechanism of electroacupuncture: Anti-injury effect on cerebral function after focal cerebral ischemia in rats. Evid. Based Complement. Altern. Med. 6 (1), 51–56. doi:10.1093/ecam/nem062
Zhong, W. J., Yang, X. S., Zhou, H., Xie, B. R., Liu, W. W., and Li, Y. (2022a). Role of mitophagy in the pathogenesis of stroke: From mechanism to therapy. Oxid. Med. Cell. Longev. 2022, 6232902. doi:10.1155/2022/6232902
Zhong, X., Li, C., Wang, F., Chen, B., Liang, H., and Ruan, S. (2022b). Mechanism of electroacupuncture at Baihui and Shenting alleviating cerebral ischemia-reperfusion injury via regulating BNIP3L-mediated mitophagy. Rehabil. Med. 32 (01), 32–39. doi:10.3724/sp.j.1329.2022.01006
Zhou, H., He, Y., Zhu, J., Lin, X., Chen, J., Shao, C., et al. (2021a). Guhong injection protects against apoptosis in cerebral ischemia by maintaining cerebral microvasculature and mitochondrial integrity through the PI3K/AKT pathway. Front. Pharmacol. 12, 650983. doi:10.3389/fphar.2021.650983
Zhou, K., Chen, J., Wu, J., Wu, Q., Jia, C., Xu, Y. X. Z., et al. (2019). Atractylenolide III ameliorates cerebral ischemic injury and neuroinflammation associated with inhibiting JAK2/STAT3/Drp1-dependent mitochondrial fission in microglia. Phytomedicine 59, 152922. doi:10.1016/j.phymed.2019.152922
Zhou, X., Chen, H., Wang, L., Lenahan, C., Lian, L., Ou, Y., et al. (2021b). Mitochondrial dynamics: A potential therapeutic target for ischemic stroke. Front. Aging Neurosci. 13, 721428. doi:10.3389/fnagi.2021.721428
Zhou, X., Wang, H. Y., Wu, B., Cheng, C. Y., Xiao, W., Wang, Z. Z., et al. (2017). Ginkgolide K attenuates neuronal injury after ischemic stroke by inhibiting mitochondrial fission and GSK-3β-dependent increases in mitochondrial membrane permeability. Oncotarget 8 (27), 44682–44693. doi:10.18632/oncotarget.17967
Zhou, Z. W., Ren, X., Zheng, L. J., Li, A. P., and Zhou, W. S. (2022). LncRNA NEAT1 ameliorate ischemic stroke via promoting Mfn2 expression through binding to Nova and activates Sirt3. Metab. Brain Dis. 37 (3), 653–664. doi:10.1007/s11011-021-00895-1
Zhou, Z. Y., Tang, Y. P., Xiang, J., Wua, P., Jin, H. M., Wang, Z., et al. (2010). Neuroprotective effects of water-soluble Ganoderma lucidum polysaccharides on cerebral ischemic injury in rats. J. Ethnopharmacol. 131 (1), 154–164. doi:10.1016/j.jep.2010.06.023
Zhu, T., Fang, B. Y., Meng, X. B., Zhang, S. X., Wang, H., Gao, G., et al. (2022). Folium Ginkgo extract and tetramethylpyrazine sodium chloride injection (Xingxiong injection) protects against focal cerebral ischaemia/reperfusion injury via activating the Akt/Nrf2 pathway and inhibiting NLRP3 inflammasome activation. Pharm. Biol. 60 (1), 195–205. doi:10.1080/13880209.2021.2014895
Zhu, T., Xie, W. J., Wang, L., Jin, X. B., Meng, X. B., Sun, G. B., et al. (2021). Notoginsenoside R1 activates the NAMPT-NAD(+)-SIRT1 cascade to promote postischemic angiogenesis by modulating Notch signaling. Biomed. Pharmacother. 140, 111693. doi:10.1016/j.biopha.2021.111693
Glossary
ATP adenosine triphosphate
TCM traditional Chinese medicine
OGD oxygen-glucose deprivation
ROS reactive oxygen species
tPA tissue plasminogen activator
mPTP mitochondrial permeability transition pore
MMP mitochondrial membrane potential
NAD+ nicotinamide adenine dinucleotide
MAMs mitochondria-associated endoplasmic reticulum membranes
I/R ischemia/reperfusion
OGD/R oxygen-glucose deprivation/reoxygenation
mtDNA mitochondrial DNA
PGC-1α peroxisome proliferator-activated receptor γ coactivator-1α
AMPK AMP-activated protein kinase
SIRT1 phosphorylation and sirtuin 1
NRF1/2 nuclear respiratory factors 1/2
TFAM mitochondrial transcription factor A
Drp1 dynamin-related protein 1
Mfn1/2 mitofusins-1/mitofusins-2
Opa1 optic atrophy 1
BNIP3 Bcl-2/E1B-19 KD-interacting protein 3
FUNDC1 FUN14 domain containing 1
MCAO middle cerebral artery occlusion
Endo G endonuclease G
Apaf-1 apoptosis activating factor-1
IAPs inhibitor-of-apoptosis proteins
AIF apoptosis-related proteins
EA electroacupuncture
MDA malondialdehyde
SOD superoxide dismutase
mTOR mammalian target of rapamycin
Smac/Diablo the second mitochondrion-derived activator of caspase/direct inhibitor of apoptosis-binding protein with low pI
Cyto-Cyt-c Cyt-c in the cytoplasm
Mito-Cyt-c mitochondrial Cyt-c
cIAP-1 -2, cellular inhibitor of apoptosis- 1,-2
XIAP X-linked inhibitor of apoptosis protein
p-Bad phospho-Bad
BNIP3L NIP3-like protein X
Cyt-c cytochrome C
HIF1α/PDK1 hypoxia-inducible factor 1-alpha/pyruvate dehydrogenase kinase 1
ROS reactive oxygen species
Keywords: traditional Chinese medicine, mitochondria, molecular mechanism, review, ischemic stroke
Citation: Liu L, Chen D, Zhou Z, Yuan J, Chen Y, Sun M, Zhou M, Liu Y, Sun S, Chen J and Zhao L (2023) Traditional Chinese medicine in treating ischemic stroke by modulating mitochondria: A comprehensive overview of experimental studies. Front. Pharmacol. 14:1138128. doi: 10.3389/fphar.2023.1138128
Received: 05 January 2023; Accepted: 10 March 2023;
Published: 22 March 2023.
Edited by:
Yusof Kamisah, Faculty of Medicine Universiti Kebangaan Malaysia, MalaysiaReviewed by:
Lei Chen, University of Kentucky, United StatesMiao Mingsan, Chinese Pharmacology, China
Copyright © 2023 Liu, Chen, Zhou, Yuan, Chen, Sun, Zhou, Liu, Sun, Chen and Zhao. This is an open-access article distributed under the terms of the Creative Commons Attribution License (CC BY). The use, distribution or reproduction in other forums is permitted, provided the original author(s) and the copyright owner(s) are credited and that the original publication in this journal is cited, in accordance with accepted academic practice. No use, distribution or reproduction is permitted which does not comply with these terms.
*Correspondence: Ling Zhao, emhhb2xpbmdAY2R1dGNtLmVkdS5jbg==; Jiao Chen, Y2hlbmppYW9AY2R1dGNtLmVkdS5jbg==
†These authors have contributed equally to this work