- 1School of Pharmacy, Chengdu Medical College, Chengdu, China
- 2Department of Pharmacy, The Third People’s Hospital of Yibin, Yibin, China
- 3Department of Pharmacy, The First Affiliated Hospital of Chengdu Medical College, Chengdu Medical College, Chengdu, China
The antimicrobial resistance of Acinetobacter baumannii (A. baumannii) clinical isolates has emerged as a great threat to public health. Quorum sensing (QS) is one of the resistance mechanisms for drug-resistant A. baumannii. Interfering with QS is a promising strategy to combat infections caused by drug-resistant bacteria. This study explored the QS inhibition ability of thirty-four traditional Chinese medicine monomers (TCMMs) and assessed the effect of QS inhibitors (QSIs) on the virulence factors of twelve extensively drug-resistant A. baumannii (XDRAB) strains. Nine traditional Chinese medicine monomers, such as caffeic acid, cinnamic acid, and myricetin, were found to be able to inhibit the bacterial QS. Then, at 1/8 of the minimal inhibitory concentration, we found that these QSIs inhibited extensively drug-resistant A. baumannii adhesion and biofilm formation and downregulated the expression levels of virulence-associated genes (abaI, abaR, csuE, pgaA, and bap). In conclusion, nine traditional Chinese medicine monomers have QS inhibitory activity and may downregulate QS genes to interfere with the QS system, which could inhibit the expression of extensively drug-resistant A. baumannii virulence factors. These results suggest that traditional Chinese medicine monomers could develop as novel anti-virulence compounds to control extensively drug-resistant A. baumannii infections.
1 Introduction
Extensively drug-resistant A. baumannii (XDRAB) is a superbug-bacteria responsible for nosocomial infections in hospitals through strong intrinsic and acquired resistance mechanisms (Lee et al., 2017), which has been listed as one of the highest-grade resistant pathogens of the ESKAPE family by the World Health Organization (Mulani et al., 2019). In addition, the ability of XDRAB to form biofilms increased colonization and persistence in environments, leading to aggravating drug resistance and bacterial pathogenicity (Yan and Bassler, 2019; Mohammed et al., 2020). Therefore, it is of great necessity to search for novel therapeutic strategies to combat XDRAB infections. Recent studies have revealed the key roles of drug resistance and biofilm formation in A. baumannii, which are regulated by the quorum sensing (QS) system (Shaaban et al., 2019; PauL et al., 2020).
QS system is a process of communication between microbial cells. Bacteria coordinate their behaviors in a bacterial cell density-dependent manner through this process (Abisado et al., 2018). In A. baumannii, QS is regulated by the two-component system abaI/abaR, which is the luxI and luxR homologs of QS in Gram-negative bacteria (Oh and Han, 2022). The abaI gene is responsible for the synthesis of N-acyl-homoserine lactone (AHL) signaling molecules (Kalia, V.C., 2013), and then the QS system senses changes in bacterial cell density by signaling molecules. When the density reaches the threshold, the synthesized signaling molecules interact with the receptor abaR, resulting in a coordinated change in gene expression, which plays an important role in the production of virulence factors and antibiotic resistance (Wu et al., 2020). Thus, interfering with the QS system is an attractive treatment against XDRAB infections by inhibiting bacterial virulence hence effectively reducing bacteria pathogenicity without inducing selective pressure resistance.
It has been reported that some synthetic chemical quorum sensing inhibitors (QSIs) like furanones and pyrrolidones, could successfully inhibit the virulence of pathogens (de Nys et al., 2006). However, the majority of these inhibitors are not only toxic to humans but also expensive. Hence, there is an urgent need to find economic, safe, and effective QSIs. Traditional Chinese medicine (TCM) has been used to treat infectious diseases since ancient times, and due to their low toxicity, many monomers isolated from TCM are now used as anti-virulence drugs or antibiotic adjuvants to improve therapeutic effects and safety (Guzzo et al., 2020; Gao et al., 2021). Therefore, it may be a better choice to search for effective QSIs from traditional Chinese medicine monomers (TCMMs).
In this study, we sought to investigate the QS inhibition ability of thirty-four TCMMs, as well as the effect of TCMMs on virulence factors of XDRAB measured by adhesion ability, biofilm formation ability and the expression of virulence factor-related genes.
2 Materials and methods
2.1 Bacterial strains
Twelve isolates of XDRAB were derived from the bacterial specimens of patients from the critical care units, internal medicine, and emergency departments at the First Affiliated Hospital of Chengdu Medical College from 2018 to 2020 (Chengdu, China). Standard laboratory methods and ATB New (BioMérieux, France) were used to identify these isolates, which were also confirmed by PCR of the genes 16S rRNA and blaOXA-51 (Chang et al., 2015); Chromobacterium violaceum 026 (CV026) was purchased from Biobw (Beijing, China); A. baumannii ATCC 17978 and Escherichia coli DH5a were preserved in our laboratory.
2.2 Drugs and reagents
Berberine, ferulic acid, baicalin, baicalein, ursolic acid, naringenin, hesperidin, gallic acid, malic acid, resveratrol, myricetin, vanillin, phloretin, capsaicin, osthole, andrographolide, parthenolide, magnolol, and ellagic acid were purchased from Meilun Biological (Dalian, China). Meanwhile, ampicillin, piperacillin, ampicillin sulbactam (2:1), cefoperazone sulbactam (2:1), efotaxime, ceftazidime, tetracycline, doxycycline, amikacin, gentamicin, ciprofloxacin, levofloxacin, imipenem, meropenem, tigecycline and polymyxin B were purchased from Meilun Biological (Dalian, China); Linoleic acid, kaempferol, 4-terpineol, coumarin, linalool, naringin, matrine, and cinnamaldehyde were purchased from Macklin Biochemical (Shanghai, China); Eugenol, citral, vanillin, cinnamic acid, hordenine, and nootkatone were purchased from Yuanye Biochemical (Shanghai, China); Furanone C30 and C6-HSL were purchased from Sigma-Aldrich (Taufkirchen, Germany); RNA isolation kit, cDNA synthesis kit, and fluorescent dye SYBR Color qPCR Master Mix were purchased from Vazyme Biological (Nanjing, China). Cation-adjusted Muller-Hinton broth (CAMHB) (Comprised of beef extract, caseins hydrolyzates, starch and calcium chloride), Luria Bertani (LB) broth medium (Comprised of tryptone, yeast extract, sodium chloride and glucose), LB agar medium (Comprised of tryptone, yeast extract, sodium chloride and agar) and tryptone soy broth (TSB) medium (Comprised of tryptone, soya peptone, sodium chloride, glucose and dipotassium hydrogen phosphate) were purchased from Haibo Biotechnology (Qingdao, China). Dimethyl sulfoxide (DMSO) and phosphate buffer saline (PBS) were purchased from Kelong Chemical (Chengdu, China).
2.3 Minimum inhibitory concentration test
The minimal inhibitory concentration (MIC) of thirty-four TCMMs for CV026 and XDRAB were determined by using the broth microdilution method. Briefly, CV026 and XDRAB were inoculated in LB agar medium at 30°C and 37°C for 16–20 h, respectively. And then resuspended in saline (0.9% sodium chloride) to produce a 0.5 McFarland turbidity standard, followed by a 20-fold dilution. TCMMs were initially dissolved in dimethyl sulfoxide (DMSO) and diluted with PBS into a series of solutions at different concentrations. CAMHB medium, bacterial suspension and TCMMs at different concentrations were added into 96-well cell culture plates in groups. The CV026 and XDRAB were incubated at 30°C and 37°C for 16–20 h, respectively. Less than OD600 of 0.1 were regarded as no bacterial growth, and colored drugs were observed macroscopically and data were recorded (Peng et al., 2020; Humphries et al., 2021).
2.4 Quorum sensing inhibition activity assay
CV026 is used as a biosensor strain to detect anti-QS activity. A culture-activated CV026 strain (5 mL) was added to 50 mL of LB nutrient agar medium containing kanamycin and C6-HSL (final concentration of 20 μg/mL), mixed and poured well, then punched and subinhibitory TCMM concentrations were added into the wells. After 24 h of incubation at 30°C, plates were observed for the inhibition of violacein. At the same time, the culture-activated CV026 bacterial solution was added to a 6-well cell culture plate, and then LB broth medium containing kanamycin, C6-HSL, and TCMM solutions with subinhibitory concentrations were added. 0.9% normal saline was used as the blank control, and furanone C30 was used as the positive control. After 24 h of incubation at 30°C, 1 mL of the bacterial solution from each well was centrifuged (13,000 g, 5 min) and DMSO was added to the cell pellets to dissolve violacein pigment. Supernatants (containing dissolved violacein) were transferred to a 96-well cell culture plate, and the absorbances were measured at 585 nm (Lou et al., 2019).
2.5 Growth curve test
Taking the 96-well cell culture plate, 170 μL TSB medium was added into each well, and then diluted bacterial solution and TCMMs solution with the different concentrations (1 MIC, 1/2 MIC, 1/4 MIC, and 1/8 MIC) were added. The value of OD570 was measured after incubation at 37°C every 6 h. The growth curves of XDRAB within 24 h were plotted, respectively. Concentrations that did not affect bacterial growth were finally selected as subsequent working concentrations (Aranda et al., 2011).
2.6 Effect on adhesion
Twelve activated XDRAB strains were diluted 1:1,000 and added into confocal dishes, then 1 mL of working concentration TCMMs were added to each well and incubated at 37°C for 4 h. After rinsing twice with PBS and sonicating for 10 min, PBS was added again to detach the bacteria adhering to the culture dish. 100 μL of detached bacteria were diluted 10-fold and spread on LB plates and cultured at 37°C for 24 h before counting. The relative value method (lg10 (N×10 × 1,000)) was used to assess the effect of TCMMs on XDRAB adhesion (Fan et al., 2018).
2.7 Effect on biofilm formation
We performed biofilm formation assays using crystal violet. Briefly, using the 96-well cell culture plate, 170 μL of TSB medium and 10 μL of PBS (control group) or TCMM solution at the working concentration were added into each well, followed by the inoculation of 20 μL of bacterial suspension (OD600 of 0.12). Escherichia coli DH5a was used as a negative control and six replicate wells were set for each strain. The plates were incubated at 37°C for 24 h, then the wells were voided and washed three times with PBS. After complete dryness, 0.1% crystal violet was added for staining for 20 min, then PBS was washed three times, dried in air for 30 min, dissolved in 95% ethanol for 15 min, and finally, the absorbance at 570 nm was measured (Farshadzadeh et al., 2018).
2.8 Effect on the expression of genes related to QS and virulence factors
The effect of TCMMs on XDRAB QS-regulated genes and virulence factor-related genes abaR, abaI, csuE, bap, and pgaA was investigated by quantitative reverse transcription-polymerase chain reaction (qRT-PCR), the gene primer sequences are presented in Table 1. The 16S rRNA gene was used for the internal standard of mRNA quantification, and the expression levels of virulence factor genes were detected using the fluorescent dye SYBR Color qPCR Master Mix. According to the kit instructions, RNA was extracted and reverse transcribed into cDNA, followed by a 20 μL system used for the reaction. The cycling conditions of qRT-PCR were as follows: pre-denaturation at 95°C for 3 min, followed by 40 cycles of 95°C for 15 s and 60°C for 5 s. The expression level of each gene was normalized and the relative expression was calculated as 2−ΔΔCT. Fold changes in gene expression from TCMM-treated cells were compared to untreated cells that were propagated under the same conditions (Luo et al., 2015; Navidifar et al., 2022).
2.9 Statistical analysis
The mean and standard deviation of the mean were calculated using SPSS 26.0. GraphPad Prism 8.0 was used for mapping. The statistical analysis of the data was performed using the Student’s t-test to compare the treated group with the control group. Data was considered significant when p < 0.05.
3 Results
3.1 Anti-bacterial activity of TCMMs
The MIC of thirty-four TCMMs against strain CV026 ranged from 16 to 1,024 μg/mL (Table 2). Cinnamic aldehyde, carvacrol, and resveratrol showed obvious antibacterial activity, and the MICs were 32 μg/mL, 32 μg/mL and 16 μg/mL, respectively. The MIC of furanone C30 was 64 μg/mL. Twelve isolates of A. baumannii were susceptible to polymyxin B or tigecycline, but resistant to other antimicrobial agents, including aminoglycosides, carbapenems, cephalosporins, fluoroquinolones, and tetracyclines (Table 3). Such resistance profiles classified these isolates as XDRAB.
3.2 Quorum sensing inhibition activity of TCMMs
Thirty-four TCMMs showed different effects on the QS activity of CV026 (Table 4). Twenty-one TCMMs, including coumarin, 4-terpineol, and hordenine, formed turbid inhibition circles on purple plates and showed different degrees of QS inhibition effects, which belonged to QS activity inhibitors. In contrast, the remaining thirteen TCMMs and blank controls did not form turbid or only transparent diaphragms, indicating no QS inhibitory activity. Thus, twenty-one of thirty-four TCMMs showed different degrees of QS inhibitory activity.
Nine of twenty-one TCMMs could inhibit violacein production to varying degrees at sub-inhibitory concentrations and decrease in a concentration-dependent manner (Figure 1). Caffeic acid, vanillin, cinnamic acid, matrine, hordenine, kaempferol, coumarin, 4-terpineol, and myricetin showed relatively obvious QS inhibitory activity, with significant differences at the concentrations of both 1/4 MIC and 1/8 MIC (p < 0.05), and the inhibitory effects were better than furanone C30. In addition, caffeic acid, vanillin, kaempferol, myricetin, and coumarin also showed inhibitory effects at a concentration of 1/16 MIC (p < 0.05). Thus, nine TCMMs, including caffeic acid, vanillin, and cinnamic acid, have inhibitory effects on the QS system without affecting the normal growth of the strains, which can be regarded as potential QSIs.
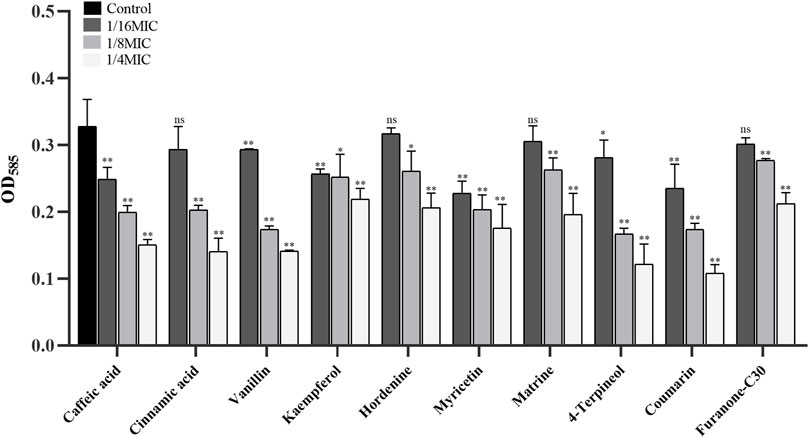
FIGURE 1. Effect of 9 TCMMs on the violacein production of CV026 at 1/4, 1/8 and 1/16MIC. TCMMs, traditional Chinese medicine monomers; MIC, minimal inhibitory concentration; CV026, Chromobacterium violaceum 026; Furanone C30, drug control; **, p < 0.01; *, p < 0.05; ns, p > 0.05.
3.3 Effect of TCMMs on the growth of XDRAB
The MICs of nine TCMMs against twelve XDRAB strains ranged from 256 to 2048 μg/mL. We found that myricetin, cinnamic acid, caffeic acid, vanillin, hordenine, kaempferol, matrine, coumarin, 4-terpineol, and furanone C30 did not affect the growth of bacterial cells at the 1/8 MIC concentration of 32, 256, 256, 256, 128, 64, 256, 32, 64, and 4 μg/mL, respectively. The growth curve of XDRAB by TCMMs is shown in Figure 2.
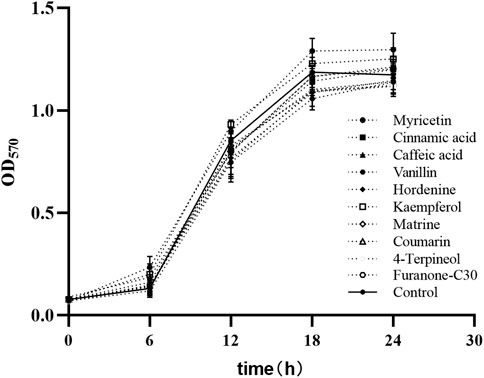
FIGURE 2. Bacterial growth curves of 12 XDRAB in the presence of 9 TCMMs at sub-MIC. Control, no TCMM; Myricetin (1/8 MIC = 32 μg/mL), cinnamic acid (1/8 MIC = 256 μg/mL), caffeic acid (1/8 MIC = 256 μg/mL), vanillin (1/8 MIC = 256 μg/mL), hordenine (1/8 MIC = 128 μg/mL), kaempferol (1/8 MIC = 64 μg/mL), matrine (1/8 MIC = 256 μg/mL), coumarin (1/8 MIC = 32 μg/mL), 4-terpineol (1/8 MIC = 64 μg/mL) and furanone C30 (1/8 MIC = 4 μg/mL). TCMMs, traditional Chinese medicine monomers; XDRAB, extensively drug-resistant A. baumannii; MIC, minimal inhibitory concentration.
3.4 Effect of TCMMs on the adhesion of XDRAB
Nine TCMMs could inhibit the adhesion of twelve XDRAB strains to confocal dishes at a concentration of 1/8 MIC and significantly reduce the number of colonies adhering to the culture dishes (p < 0.01). After 1/8 MIC of kaempferol and 4-terpineol, the relative numbers of bacteria adhering to the culture dishes were 3.52 ± 0.09 and 3.70 ± 0.17, respectively, compared with 6.99 ± 0.02 in the blank control group (Figure 3).
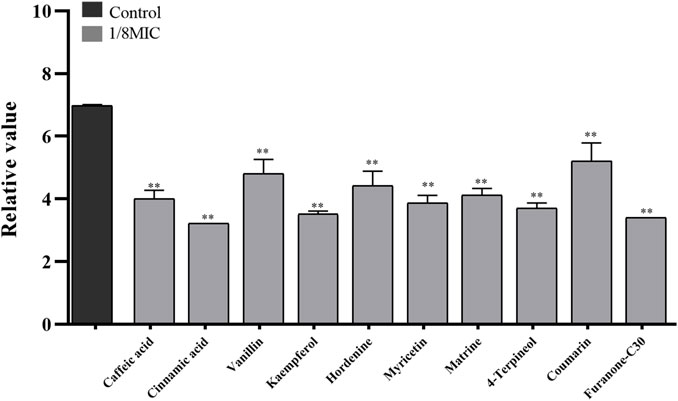
FIGURE 3. Effect of 9 TCMMs on the adhesion ability of XDRAB strains at 1/8 MIC (x ± s, n = 12). TCMMs, traditional Chinese medicine monomers; MIC, minimal inhibitory concentration; XDRAB, extensively drug-resistant A. baumannii; **, p < 0.01.
3.5 Effect of TCMMs on the biofilm formation of XDRAB
Nine TCMMs could inhibit the biofilm formation of XDRAB to different extents. At a concentration of 1/8 MIC, 4-terpineol, coumarin, hordenine, caffeic acid, matrine, kaempferol, and vanillin could significantly inhibit the biofilm formation of XDRAB (p < 0.05). Myricetin, cinnamic acid, and furanone C30 had relatively weak inhibitory effects (p > 0.05). Overall, TCMMs showed stronger anti-biofilm effects compared with furanone C30 (Table 5).
3.6 Effect of TCMMs on the expression of XDRAB virulence factor-related genes
The expression levels of QS-regulated genes abaI, abaR and virulence factor-related genes csuE, bap, and pgaA in biofilm strongly positive strains were analyzed by qRT-PCR at a concentration of 1/8 MIC (Table 6). Compared with the control group, the expression levels of QS genes abaI and abaR, pilus regulatory gene csuE, and biofilm-related genes bap and pgaA were significantly decreased by the eight TCMMs at the concentration of 1/8 MIC, except coumarin, which showed no effect on the biofilm-related gene bap. Therefore, nine TCMMs downregulated the QS-related genes abaI, abaR and the virulence factor-associated genes csuE, bap, and pgaA, except coumarin, which upregulated the gene bap.
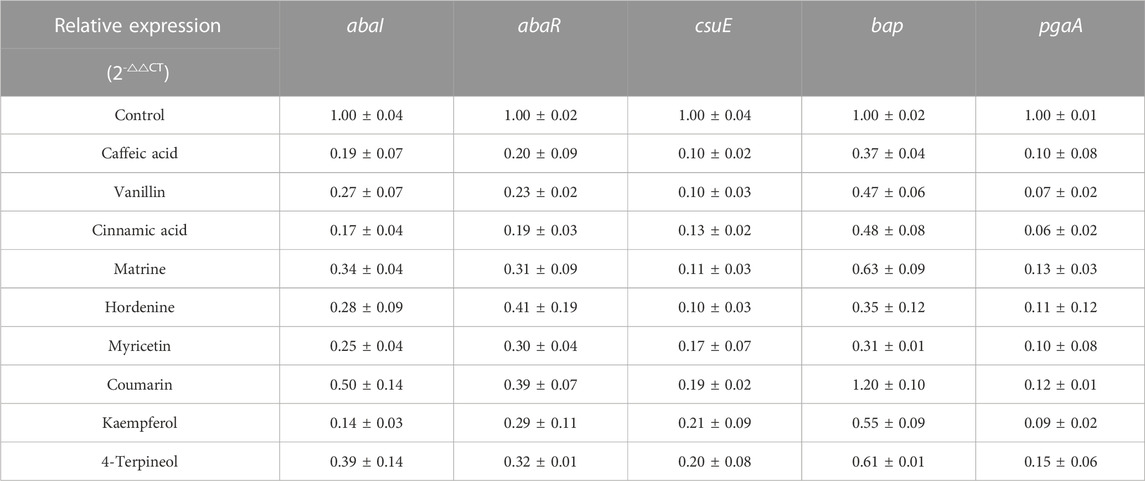
TABLE 6. Effect of 9 TCMMs on the virulence factor-related gene expression of XDRAB at 1/8 MIC (x ± s, n = 3).
4 Discussion
Biofilm formation in XDRAB is closely associated with bacterial virulence and resistance (Ayoub and Hammoudi, 2020). It has been found that biofilm formation is a dynamic process regulated by various factors, in which QS plays a crucial role (Carradori et al., 2020). QSI makes bacteria more susceptible to clearance by reducing biofilm formation, thus decreasing the virulence and drug resistance of pathogens (Warrier et al., 2021). Hence, QS is a critical target for the design of novel antimicrobial therapeutics. Some TCMMs have been shown to inhibit QS in addition to their antimicrobial activity (Lee et al., 2014; Chen et al., 2016; Zhao et al., 2020). In this study, the QS inhibitory activity of thirty-four TCMMs was investigated by biosensor CV026. The results revealed that twenty-one TCMMs showed different degrees of QS inhibitory activity, among which nine TCMMs, such as caffeic acid, cinnamic acid, and vanillin, had relatively obvious QS inhibitory activity. Similar to our findings, coumarin and caffeic acid have been found to interfere with the violacein production of CV026 (D’almeida et al., 2017; Pejin et al., 2015).
The effect of nine TCMMs on XDRAB adhesion and biofilm formation was investigated in this study. At the concentration of 1/8 MIC, all the nine TCMMs reduced the adhesion and biofilm formation of XDRAB: adhesion ability was inhibited in a range of 25.46%–53.79%; biofilm formation ability was inhibited in a range of 12.40%–47.37%. The QS system is an important way for information exchange between bacteria and involves different stages of bacterial biofilm formation (Ahmed et al., 2020). It has been reported that coumarin affects the production of violacein from CV026 at a concentration of 100 μg/mL, followed by inhibiting the formation of biofilm in Pseudomonas aeruginosa (D’almeida et al., 2017). Similarly, kaempferol, 4-terpineol and hordenine, also showed QS and biofilm inhibition activity against other common clinical pathogenic bacteria (Rekha et al., 2017; Zhou et al., 2018; Bose et al., 2020). The results depicted that the nine TCMMs represented by 4- terpineol could inhibit the adhesion and biofilm formation of XDRAB, and these drugs might have broad anti-virulence activity. Biofilm is a component of the XDRAB resistance mechanisms (Mirghani et al., 2022). So, the susceptibility of antimicrobial agents to bacteria can be increased by reducing biofilm formation. These results suggested that the nine TCMMs could be used as antibacterial adjuvants to increase the bactericidal effect of antibacterial drugs. Alves also found linalool to be a QSI that reduced the expression of A. baumannii virulence factors (Alves et al., 2016). Therefore, QS is closely related to bacterial virulence, which is consistent with our research results.
We also used qRT-PCR to investigate the effect of nine TCMMs on virulence factor-related gene expression of XDRAB with strong biofilm formation ability. It has been shown that QS genes are critical regulators of biofilm formation, and various virulence factors (Elnegery et al., 2021). QSI inhibits the expression of virulence factor-related genes in pathogens by down-regulating QS key genes (Wang et al., 2021). The results indicated that the nine TCMMs downregulated QS-related genes abaI, abaR and virulence factor-related genes csuE, bap, and pgaA except coumarin, which upregulated the bap gene. The abaI expression was reduced by 80.97%, 83.18%, 73.30%, and 86.22% in cultures treated with 1/8 MICs of caffeic acid, cinnamic acid, vanillin, and kaempferol, respectively. In addition, treatment with myricetin and 4-terpineol revealed a significant decrease in the expression of pgaA and bap by 90.10%, 84.94%, and 68.77%, 39.07%, respectively. However, coumarin upregulated the expression of bap by 19.80%. Therefore, by down-regulating QS genes abaI and abaR, the nine TCMMs affected the expression of csuE, which prevented bacteria from transforming from plankton to biofilm (de Breij et al., 2009), and inhibited the expression of bap and pgaA, which reduced bacterial adhesion and destroyed biofilm formation, followed by reducing bacterial virulence (Choi et al., 2009; Farshadzadeh et al., 2018).
In conclusion, nine TCMMs like caffeic acid, cinnamic acid, and vanillin showed QS inhibitory activity. They might interfere with the QS system and inhibit the expression of XDRAB virulence factors by inhibiting QS and virulence factor-related genes, thus reversing bacterial drug resistance and reducing pathogenicity. This could develop as novel anti-virulence compounds to control XDRAB infections.
Data availability statement
The original contributions presented in the study are included in the article/Supplementary Materials, further inquiries can be directed to the corresponding author.
Ethics statement
This study uses strains derived from bacteria specimens (endotracheal aspirate or respiratory lavage fluid) of patients from the critical care units, internal medicine and emergency department at the First Affiliated Hospital of Chengdu Medical College in 2018 to 2020. The Biomedical Ethics Committee of Chengdu Medical College did not require the study to be reviewed or approved by an ethics committee because this was an entirely in-vitro study of bacteria ethical review is not required.
Author contributions
LZ designed and performed the experiments, and drafted the manuscript; FL contributed to the study design and revising the manuscript; BL initiated and supervised the study, and revised the manuscript. All authors reviewed the manuscript.
Funding
This work was supported by the National Natural Science Foundation of China (Grant No. 81373454).
Conflict of interest
The authors declare that the research was conducted in the absence of any commercial or financial relationships that could be construed as a potential conflict of interest.
Publisher’s note
All claims expressed in this article are solely those of the authors and do not necessarily represent those of their affiliated organizations, or those of the publisher, the editors and the reviewers. Any product that may be evaluated in this article, or claim that may be made by its manufacturer, is not guaranteed or endorsed by the publisher.
Supplementary material
The Supplementary Material for this article can be found online at: https://www.frontiersin.org/articles/10.3389/fphar.2023.1135180/full#supplementary-material
References
Abisado, R. G., Benomar, S., Klaus, J. R., Dandekar, A. A., and Chandler, J. R. (2018). Bacterial quorum sensing and microbial community interactions. mBio 9 (3), e02331. doi:10.1128/mBio.02331-17
Ahmed, T., Pattnaik, S., Khan, M. B., Ampasala, D. R., Busi, S., and Sarma, V. V. (2020). Inhibition of quorum sensing-associated virulence factors and biofilm formation in Pseudomonas aeruginosa PAO1 by Mycoleptodiscus indicus PUTY1. Braz J. Microbiol. 51 (2), 467–487. doi:10.1007/s42770-020-00235-y
Alves, S., Duarte, A., Sousa, S., and Domingues, F. C. (2016). Study of the major essential oil compounds of Coriandrum sativum against Acinetobacter baumannii and the effect of linalool on adhesion, biofilms and quorum sensing. Biofouling 32 (2), 155–165. doi:10.1080/08927014.2015.1133810
Aranda, J., Bardina, C., Beceiro, A., Rumbo, S., Cabral, M. P., Barbé, J., et al. (2011). Acinetobacter baumannii RecA protein in repair of DNA damage, antimicrobial resistance, general stress response, and virulence. J. Bacteriol. 193 (15), 3740–3747. doi:10.1128/JB.00389-11
Ayoub, M. C., and Hammoudi, H. D. (2020). Insights into acinetobacter baumannii: A review of microbiological, virulence, and resistance traits in a threatening nosocomial pathogen. Antibiot. (Basel) 9 (3), 119. doi:10.3390/antibiotics9030119
Bose, S. K., Chauhan, M., Dhingra, N., Chhibber, S., and Harjai, K. (2020). Terpinen-4-ol attenuates quorum sensing regulated virulence factors and biofilm formation in pseudomonas aeruginosa. Future Microbiol. 15 (2), 127–142. doi:10.2217/fmb-2019-0204
Carradori, S., Di, G. N., Lobefalo, M., Luisi, G., Campestre, C., and Sisto, F. (2020). Biofilm and quorum sensing inhibitors: The road so far. Expert Opin. Ther. Pat. 30 (12), 917–930. doi:10.1080/13543776.2020.1830059
Chang, Y., Luan, G., Xu, Y., Wang, Y., Shen, M., Zhang, C., et al. (2015). Characterization of carbapenem-resistant Acinetobacter baumannii isolates in a Chinese teaching hospital. Front. Microbiol. 6, 910. doi:10.3389/fmicb.2015.00910
Chen, Y., Liu, T., Wang, K., Hou, C., Cai, S., Huang, Y., et al. (2016). Baicalein inhibits Staphylococcus aureus biofilm formation and the quorum sensing system in vitro. PLoS One 11 (4), e0153468. doi:10.1371/journal.pone.0153468
Choi, A. H., Slamti, L., Avci, F. Y., Pier, G. B., and Maira-Litrán, T. (2009). ThepgaABCD locus of acinetobacter baumannii encodes the production of poly-β-1-6-N-acetylglucosamine, which is critical for biofilm formation. J. Bacteriol. 191 (19), 5953–5963. doi:10.1128/JB.00647-09
D'Almeida, R. E., Molina, R. D. I., Viola, C. M., Luciardi, M. C., Nieto, P. C., Bardón, A., et al. (2017). Comparison of seven structurally related coumarins on the inhibition of Quorum sensing of Pseudomonas aeruginosa and Chromobacterium violaceum. Bioorg. Chem. 73, 37–42. doi:10.1016/j.bioorg.2017.05.011
de Breij, A., Gaddy, J., van der Meer, J., Koning, R., Koster, A., van den Broek, P., et al. (2009). CsuA/BABCDE-dependent pili are not involved in the adherence of Acinetobacter baumannii ATCC19606T to human airway epithelial cells and their inflammatory response. Res. Microbiol. 160 (3), 213–218. doi:10.1016/j.resmic.2009.01.002
de Nys, R., Givskov, M., Kumar, N., Kjelleberg, S., and Steinberg, P. D. (2006). Furanones. Prog. Mol. Subcell. Biol. 42, 55–86. doi:10.1007/3-540-30016-3_2
Elnegery, A. A., Mowafy, W. K., Zahra, T. A., and Abou El-Khier, N. T. (2021). Study of quorum-sensing LasR and RhlR genes and their dependent virulence factors in Pseudomonas aeruginosa isolates from infected burn wounds. Access Microbiol. 3 (3), 000211. doi:10.1099/acmi.0.000211
Fan, L., Li, Q. D., Tian, J., Sun, F. J., Feng, W., and Zhou, S. W. (2018). Imipenem with sub-inhibitory concentration enhances biofilm formation of drug-resistant Acinetobacter baumannii by affecting type ⅳ pili. J. third Mil. Med. Univ. 40 (21), 1967–1972. doi:10.16016/j.1000-5404.201808141
Farshadzadeh, Z., Taheri, B., Rahimi, S., Shoja, S., Pourhajibagher, M., Haghighi, M. A., et al. (2018). Growth rate and biofilm formation ability of clinical and laboratory-evolved colistin-resistant strains of acinetobacter baumannii. Front. Microbiol. 9, 153. doi:10.3389/fmicb.2018.00153
Gao, S., Zhang, S., and Zhang, S. (2021). Enhancedin vitro antimicrobial activity of amphotericin B with berberine against dual-species biofilms of candida albicans and Staphylococcus aureus. J. Appl. Microbiol. 130 (4), 1154–1172. doi:10.1111/jam.14872
Guzzo, F., Scognamiglio, M., Fiorentino, A., Buommino, E., and D'Abrosca, B. (2020). Plant derived natural products against Pseudomonas aeruginosa and Staphylococcus aureus: Antibiofilm activity and molecular mechanisms. Molecules 25 (21), 5024. doi:10.3390/molecules25215024
Humphries, R., Bobenchik, A. M., Hindler, J. A., and Schuetz, A. N. (2021). Overview of changes to the clinical and laboratory standards institute performance standards for antimicrobial susceptibility testing, M100, 31st edition. J. Clin. Microbiol. 18, e0021321. doi:10.1128/JCM.00213-21
Kalia, V. C. (2013). Quorum sensing inhibitors: An overview. Biotechnol. Adv. 31 (2), 224–245. doi:10.1016/j.biotechadv.2012.10.004
Lee, C. R., Lee, J. H., Park, M., Park, K. S., Bae, I. K., Kim, Y. B., et al. (2017). Biology of acinetobacter baumannii: Pathogenesis, antibiotic resistance mechanisms, and prospective treatment options. Front. Cell. Infect. Microbiol. 7, 55. doi:10.3389/fcimb.2017.00055
Lee, J. H., Kim, Y. G., Ryu, S. Y., Cho, M. H., and Lee, J. (2014). Ginkgolic acids and Ginkgo biloba extract inhibit Escherichia coli O157:H7 and Staphylococcus aureus biofilm formation. Int. J. Food Microbiol. 174, 47–55. doi:10.1016/j.ijfoodmicro.2013.12.030
Lou, Z., Letsididi, K. S., Yu, F., Pei, Z., Wang, H., and Letsididi, R. (2019). Inhibitive effect of Eugenol and its nanoemulsion on quorum sensing–mediated virulence factors and biofilm formation by Pseudomonas aeruginosa. J. Food Prot. 82 (3), 379–389. doi:10.4315/0362-028X.JFP-18-196
Luo, L. M., Wu, L. J., Xiao, Y. L., Zhao, D., Chen, Z. X., Kang, M., et al. (2015). Enhancing pili assembly and biofilm formation in Acinetobacter baumannii ATCC19606 using non-native acyl-homoserine lactones. BMC Microbiol. 15 (1), 62. doi:10.1186/s12866-015-0397-5
Mirghani, R., Saba, T., Khaliq, H., Mitchell, J., Do, L., Chambi, L., et al. (2022). Biofilms: Formation, drug resistance and alternatives to conventional approaches. AIMS Microbiol. 8 (3), 239–277. doi:10.3934/microbiol.2022019
Mohammed, M. A., Ahmed, M. T., Anwer, B. E., Aboshanab, K. M., and Aboulwafa, M. M. (2020). Propranolol, chlorpromazine and diclofenac restore susceptibility of extensively drug-resistant (XDR) Acinetobacter baumannii to fluoroquinolones. PLoS One 15 (8), e0238195. doi:10.1371/journal.pone.0238195
Mulani, M. S., Kamble, E. E., Kumkar, S. N., Tawre, M. S., and Pardesi, K. R. (2019). Emerging strategies to combat ESKAPE pathogens in the era of antimicrobial resistance: A review. Front. Microbiol. 1 (4), 00539. doi:10.3389/fmicb.2019.00539
Navidifar, T., Amin, M., and Rashno, M. (2022). Effects of sub-inhibitory concentrations of meropenem and tigecycline on the expression of genes regulating pili, efflux pumps and virulence factors involved in biofilm formation by Acinetobacter baumannii. Infect. Drug Resist 12, 1099–1111. doi:10.2147/IDR.S199993
Oh, M. H., and Han, K. (2022). AbaR is a LuxR-type regulator essential for motility and the formation of biofilm and pellicle in Acinetobacter baumannii. Genes and Genomics 42 (11), 1339–1346. doi:10.1007/s13258-020-01005-8
Paul, B. S., Mitra, A., Bhattacharya, A., and Sen, A. (2020). Quorum quenching activity of pentacyclic triterpenoids leads to inhibition of biofilm formation by Acinetobacter baumannii. Biofouling 36 (8), 922–937. doi:10.1080/08927014.2020.1831480
Pejin, B., Ciric, A., Markovic, J. D., Glamoclija, J., Nikolic, M., Stanimirovic, B., et al. (2015). Quercetin potently reduces biofilm formation of the strain Pseudomonas aeruginosa PAO1 in vitro. Curr. Pharm. Biotechnol. 16 (8), 733–737. doi:10.2174/1389201016666150505121951
Peng, Q., Lin, F., and Ling, B. (2020). In vitro activity of biofilm inhibitors in combination with antibacterial drugs against extensively drug-resistant Acinetobacter baumannii. Sci. Rep. 10 (1), 18097. doi:10.1038/s41598-020-75218-y
Rekha, P. D., Vasavi, H. S., Vipin, C., Saptami, K., and Arun, A. B. (2017). A medicinal herbCassia alata attenuates quorum sensing inChromobacterium violaceum and pseudomonas aeruginosa. Lett. Appl. Microbiol. 64 (3), 231–238. doi:10.1111/lam.12710
Shaaban, M., Elgaml, A., and Habib, E. E. (2019). Biotechnological applications of quorum sensing inhibition as novel therapeutic strategies for multidrug resistant pathogens. Microb. Pathog. 127, 138–143. doi:10.1016/j.micpath.2018.11.043
Wang, S., Feng, Y., Han, X., Cai, X., Yang, L., Liu, C., et al. (2021). Inhibition of virulence factors and biofilm formation by wogonin attenuates pathogenicity of Pseudomonas aeruginosa PAO1 via targeting pqs quorum-sensing system. Int. J. Mol. Sci. 22 (23), 12699. doi:10.3390/ijms222312699
Warrier, A., Satyamoorthy, K., and Murali, T. S. (2021). Quorum-sensing regulation of virulence factors in bacterial biofilm. Future Microbiol. 16, 1003–1021. doi:10.2217/fmb-2020-0301
Wu, S., Liu, J., Liu, C., Yang, A., and Qiao, J. (2020). Quorum sensing for population-level control of bacteria and potential therapeutic applications. Cell. Mol. Life Sci. 77 (7), 1319–1343. doi:10.1007/s00018-019-03326-8
Yan, J., and Bassler, B. L. (2019). Surviving as a community: Antibiotic tolerance and persistence in bacterial biofilms. Cell Host Microbe 26 (1), 15–21. doi:10.1016/j.chom.2019.06.002
Zhao, Y., Mei, L., Si, Y., Wu, J., Shao, J., Wang, T., et al. (2020). Sodium New houttuyfonate affects transcriptome and virulence factors of Pseudomonas aeruginosa controlled by quorum sensing. Front. Pharmacol. 11, 572375. doi:10.3389/fphar.2020.572375
Keywords: traditional Chinese medicine monomer, extensively drug-resistant A. baumannii, quorum sensing inhibitor, virulence factor, quorum sensing
Citation: Zeng L, Lin F and Ling B (2023) Effect of traditional Chinese medicine monomers interfering with quorum-sensing on virulence factors of extensively drug-resistant Acinetobacter baumannii. Front. Pharmacol. 14:1135180. doi: 10.3389/fphar.2023.1135180
Received: 31 December 2022; Accepted: 20 March 2023;
Published: 30 March 2023.
Edited by:
Karunakaran Kalesh, Teesside University, United KingdomReviewed by:
Karthikeyan Sivashanmugam, VIT University, IndiaPrasun Kumar, Chungbuk National University, Republic of Korea
Copyright © 2023 Zeng, Lin and Ling. This is an open-access article distributed under the terms of the Creative Commons Attribution License (CC BY). The use, distribution or reproduction in other forums is permitted, provided the original author(s) and the copyright owner(s) are credited and that the original publication in this journal is cited, in accordance with accepted academic practice. No use, distribution or reproduction is permitted which does not comply with these terms.
*Correspondence: Baodong Ling, lingbaodong@cmc.edu.cn