- 1Department of Gynecology, Hospital of Chengdu University of Traditional Chinese Medicine, Chengdu, Sichuan, China
- 2Department of Stomatology, Hospital of Chengdu University of Traditional Chinese Medicine, Chengdu, Sichuan, China
Lipid metabolism disorders (LMD) can cause a series of metabolic diseases, including hyperlipidemia, obesity, non-alcoholic fatty liver disease (NAFLD) and atherosclerosis (AS). Its development is caused by more pathogenic factors, among which intestinal flora dysbiosis is considered to be an important pathogenic mechanism of LMD. In recent years, the research on intestinal flora has made great progress, opening up new perspectives on the occurrence and therapeutic effects of diseases. With its complex composition and wide range of targets, traditional Chinese medicine (TCM) is widely used to prevent and treat LMD. This review takes intestinal flora as a target, elaborates on the scientific connotation of TCM in the treatment of LMD, updates the therapeutic thinking of LMD, and provides a reference for clinical diagnosis and treatment.
1 Introduction
With the development of society and the improvement of living conditions, the incidence of lipid metabolism disorders (LMDs) is increasing year by year and showing a trend of rejuvenation, which seriously threatens human health. Abnormal lipid metabolism is a pathological process of elevated blood lipid levels and ectopic lipid deposition caused by genetic or acquired factors, and is an important risk factor for many metabolic diseases. Lipid metabolism is central to this process, and when lipid biosynthesis and degradation are abnormal, or when lipoprotein synthesis, metabolism, and transport are impaired, this can lead to disorders of lipid metabolism, contributing to hyperlipoproteinemia (Karr, 2017), obesity (Aron-Wisnewsky et al., 2021), non-alcoholic liver disease (NAFLD) (Katsiki et al., 2016), and atherosclerosis (Zhang et al., 2022b). Furthermore, dyslipidemia also has an effect on other target organs such as the brain and kidneys (Ross, 1999). Therefore, it is crucial to investigate the biological mechanisms underlying the development of LMD and to seek effective therapeutic targets for effective regulation of lipid metabolism to prevent and treat LMD.
The intestinal flora is an essential component of the intestinal micro-ecosystem and controls numerous metabolic processes with the host immune system, such as energy balance, glucose and lipid metabolism (Sonnenburg and Backhed, 2016). In recent years, numerous studies have further elucidated the connection between intestinal flora and its metabolites and LMD (Woting and Blaut, 2016; Jonsson and Backhed, 2017; Duttaroy, 2021), finding that dysregulation of intestinal flora and its metabolites contribute to the development of LMD (Ridaura et al., 2013; Matey-Hernandez et al., 2018; Jia et al., 2021). LMD can also be improved by altering the intestinal flora by fecal transplantation or probiotic administration (Falcinelli et al., 2018; Allegretti et al., 2020; Witjes et al., 2020). In addition, LMD can affect intestinal flora homeostasis and have a negative impact on the organism’s health. Numerous studies have shown that the intestinal flora structure of animals with high-fat diet (HFD) -induced LMD is altered, as evidenced by a decrease in intestinal flora diversity, a reduction in Bacteroidetes, and an increase in Firmicutes, Proteobacteria, and Verrucomicrobia (Tomas et al., 2016), or a significant decrease in the abundance of beneficial bacteria like Bifidobacterium and Lactobacillus (Wang B. et al., 2020). Meanwhile, some key intestinal metabolites such as lipopolysaccharides (LPS) (Cani et al., 2007b), bile acids (BAs) (Gillard et al., 2022), short-chain fatty acids (SCFAs) (Wang G. et al., 2021), branched-chain amino acids (BCAAs) (Nie et al., 2018) and trimethylamine-N-oxide (TMAO) (Janeiro et al., 2018) were discovered to play a role in the host’s lipid metabolism function. Therefore, intestinal flora dysbiosis may take a significant part in the progression of LMD, and reshaping the structure of intestinal flora can contribute to correcting LMD.
Studies have found that Lipid-lowering drugs can treat LMD by altering the intestinal flora. Simvastatin can alter the abundance and diversity of intestinal flora from phylum to genus level, modulate the downstream metabolic pathways of intestinal flora and ultimately exert a hypolipidemic effect (Zhang S. et al., 2020). In another research investigating the influence of intestinal flora on the atorvastatin’s hypolipidemic effect, it was found that atorvastatin significantly reduced serum levels of total cholesterol (TC) and low-density lipoprotein (LDL) in mice with intact intestinal flora but did not have the same effects in mice with depleted intestinal flora. Besides, we observed changes in the abundance of several sphingolipids after treatment with atorvastatin in mice with intact intestinal flora, which was not present in mice with depleted intestinal flora, suggesting that the lipid-lowering efficacy of atorvastatin also depends on the composition of intestinal flora before treatment (Zimmermann et al., 2020). However, in addition to the financial burden, long-term use of statins may trigger side effects such as myopathy (muscle pain or muscle weakness), hyperglycemia and liver enzyme abnormalities (Bellosta and Corsini, 2018), limiting the treatment options for patients. Therefore, it is crucial to provide patients with therapies that are effective and have few side effects.
TCM has been practiced for several thousand years in China, and now its use has expanded globally. TCM has a significant role in improving human subhealth, controlling metabolism and preventing major diseases. For a long time, TCM has been playing a significant role in improving metabolic disorders due to its multi-component and multi-target properties (Zhang et al., 2014; Zhang H. Y. et al., 2021). Research has revealed that TCM (natural medicine extracts and Chinese herbal formulas) can significantly improve the components and metabolite function of intestinal flora, as well as help to maintain of intestinal flora homeostasis, thereby regulating lipid metabolism. For example, baicalin improved HFD-induced abnormalities in glucose and lipid metabolism via enhancing the number of bacteria that produce SCFA (Ju et al., 2019). Poria cocos (Schw.) Wolf [Polyporaceae; Poria] water insoluble polysaccharide (WIP) improved markedly glucose and lipid metabolism in ob/ob mice, and the underlying mechanism may be associated with increased numbers of butyrate-producing bacteria Lachnospiracea and Clostridium, elevated butyrate levels in the intestine, improved intestinal barrier function, and activated the intestinal peroxisome proliferator-activated receptor γ (PPAR-γ) pathway (Sun et al., 2019). Si Miao Formula, a classic TCM formula, played an anti-NAFLD role by altering the composition of intestinal flora, especially by upping the proportion of Akkermansia muciniphila and down-regulating the production of pro-inflammatory proteins (Han et al., 2021). Resveratrol (RSV) inhibited TMAO production by remodeling intestinal flora, depressed the enterohepatic farnesoid X receptor/fibroblast growth factor 15 (FXR/FGF15) pathway, enhanced BA hydrolase activity, and promoted the synthesis of hepatic BAs, resulting in its anti-AS effects (Chen et al., 2016). On the other hand, intestinal flora may convert effective ingredients of TCM in a variety of ways to generate secondary metabolites, thus exerting the therapeutic effects of TCM in regulating lipid metabolism-related pathways and gene expression. It was found that treatment with Dingxin Recipes (DXR) IV resulted in increased levels of metabolites related to fatty acid metabolism, such as acetate and butyrate, downregulation of the Liver X Receptor α/sterol regulatory element-binding protein 1 (LXR-α/SREBP1) axis, decreased blood lipid levels, as well as suppression of excessive cholesterol deposition in the aorta in HFD-fed mice (Zhang et al., 2021c). Notably, lack of Blautia greatly reduced the cholesterol-lowering efficacy of berberine (BBR), revealing that intestinal flora plays a crucial part in BBR’s hypolipidemic impact (Wu et al., 2022).
In this review, we aimed to elucidate the role of intestinal flora and its metabolites in the occurrence and prevention of LMDs, and to systematically evaluate the multiple mechanisms involved in the regulation of intestinal flora in the improvement of LMDs in TCM, with the aim of providing new approaches for the prevention and treatment of LMDs in TCM and evidence for the development of novel anti-LMD drugs.
2 Relationship between intestinal flora and LMD
A growing majority of research have revealed an interaction between lipid metabolism and intestinal flora (Matey-Hernandez et al., 2018; Aron-Wisnewsky et al., 2021). Dysbiosis of the intestinal flora can disrupt lipid metabolism, and dyslipidemia can in turn cause imbalances in intestinal flora, but the exact mechanisms between the two remain to be investigated in depth. In recent years, researchers have also identified intestinal flora metabolites that may also be involved in regulating lipid metabolism, including LPS, SCAFAs, BAs, TMAO (Liang et al., 2020; Zhou X. et al., 2021; Jia et al., 2021). These metabolites derived from the intestinal flora can act not only on the host local tissues, but also affect the host endocrine and organ functions the in various ways (Figure 1).
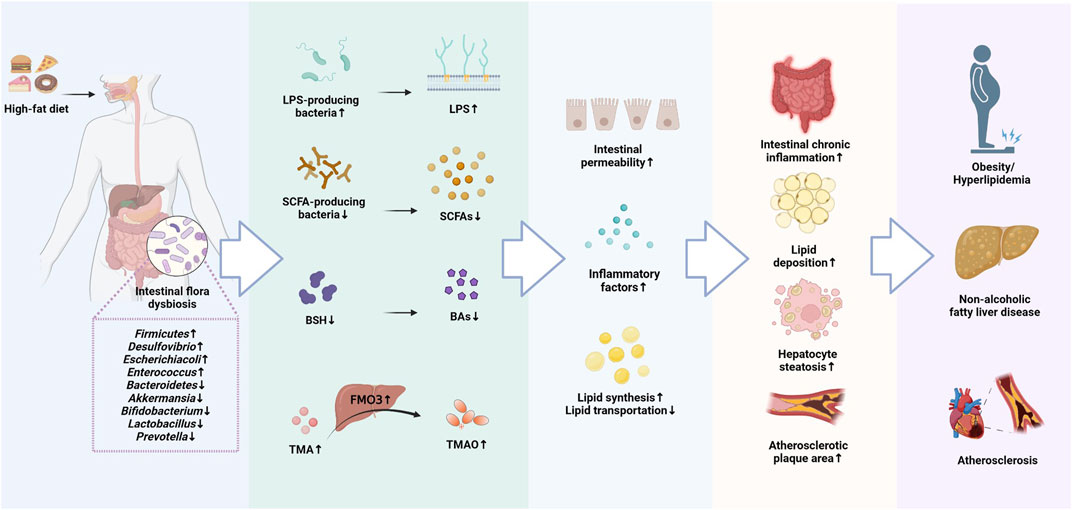
FIGURE 1. The molecular mechanisms of intestinal flora involved in the development of Lipid metabolism disorders (LMD).
2.1 The role of intestinal flora structure in the development of LMD
Intestinal flora is an important influence on energy absorption and metabolism, and a decrease in intestinal flora diversity is associated with an increased relative risk of LMD (Rastelli et al., 2018). Studies have shown that the α diversity of intestinal flora in high-fat rats is significantly reduced, as demonstrated through a drop in the intestinal flora abundance and a decrease in the structural stability of intestinal flora (Zhao J. et al., 2022). This phenomenon is consistent with the findings on intestinal flora of patients with LMD (Mu et al., 2020), but the specific composition of intestinal flora in healthy and obese people is still debated (Kasai et al., 2015). The human intestinal flora is mainly composed of Firmicutes and Bacteroidetes, which account for more than 98% of intestinal flora abundance and are involved in lipid and BA metabolism to maintain energy balance in the host. Among them, Firmicutes can induce hepatic steatosis via regulating fatty acid inflow and lipogenesis (Chen Y. H. et al., 2019), while Bacteroidetes can suppress obesity by promoting the catabolism of BCAAs in brown adipose tissue (BAT) (Yoshida et al., 2021). In addition, Bacteroidetes also produce bile salt hydrolase (BSH), which hydrolyzes conjugated BAs and regulates BAs-mediated lipid metabolism (Tian et al., 2019). Therefore, Firmicutes are hypothesized to be positively correlated with LMD, and Bacteroidetes are negatively correlated with LMD. A lower Firmicutes/Bacteroidetes (F/B) ratio represents a healthier gut microbial environment, and the risk of obesity and LMD may be increased by an elevated F/B ratio (Magne et al., 2020). Firmicutes were increased in the intestinal tract of obese individuals compared to healthy individuals, and F/B ratio decreased with an increase in Bacteroidetes and a decrease in Firmicutes following weight loss treatment (Damms-Machado et al., 2015). Akkermansia is a mucus-degrading bacteria that reverses HFD-induced increase in adiposity, metabolic endotoxemia, and adipose tissue inflammation (Everard et al., 2013). Bifidobacterium also promotes changes in lipid metabolism and glucose homeostasis (Salazar et al., 2019). Long-term HFD-induced changes in the structure of intestinal flora in animal models, mainly in the form of a significant reduction in the content of beneficial bacteria like Akkermansia, Bifidobacterium, Lactobacillus, Blautia, and Faecalibaculum, which have the ability to alleviate LMD (Shin et al., 2014; Wang B. et al., 2020). In another study, a substantial growth in the abundance of Bacteroides and Parabacteroides was found in hyperlipidemic rats (Zhao J. et al., 2022). Bacteroides and Parabacteroides contain a large number of conditionally pathogenic bacteria that can increase the risk of infection. They can also produce acetic acid, which promotes appetite and increases food intake by activating the parasympathetic nervous system and promoting the release of insulin and ghrelin, leading to elevated TG levels, liver and muscle fat accumulation and the development of obesity-related metabolic diseases (Perry et al., 2016). LMD influenced by changes in the intestinal flora have been found extensively in animals and are also largely reflected in humans (Maruvada et al., 2017). Therefore, the management of LMD focused on the modulation of intestinal flora structure is crucial.
2.2 The role of LPS in the development of LMD
A normal intestinal mucosal barrier is fundamental for maintaining intestinal function. One study indicates that the pathophysiological root of LMD is a compromised intestinal barrier (Schulz et al., 2014). Damage to the tight junction proteins between intestinal epithelial cells, such as ZO-1, Occludin and Claudin1, which are essential for the intestinal mucosal barrier, leads to a rise in intercellular permeability and the admission of bacteria, endotoxins, as well as macromolecules into other tissues, organs or body circulation via the cellular bypass pathway, resulting in metabolic endotoxemia, inflammation and metabolic disorders (Zheng et al., 2022). LPS is the central aspect of Gram-negative (G-) bacteria’s outermost layer of cell wall, and is an important inflammatory stimulus that can cause severe damage to the intestinal barrier and contribute to a chronic inflammatory response in the body, leading to the development and progression of metabolic diseases (Zhang-Sun et al., 2015). It has been found that a HFD disrupts the structure of intestinal flora and increases the abundance of LPS-producing bacteria, such as Desulfovibrio, which further enhances intestinal permeability and contributes to large amounts of LPS to enter the circulation from the intestine, triggering metabolic endotoxemia (Cani et al., 2007a), which is linked to the development of LMDs including dyslipidemia, obesity, NAFLD and cardiovascular disease (Manco et al., 2010). LPS can exacerbate lipid metabolism by binding to Toll-like receptor 4 (TLR4), a receptor expressed on macrophages, hepatocytes and adipocytes, and inducing the secretion of pro-inflammatory factors like interleukin 6 (IL-6) and monocyte chemotactic protein 1 (MCP-1). Secretion, exacerbating lipid metabolic disorders (Robbins et al., 2014). Furthermore, LPS increases intestinal permeability through chronic inflammation mediated by MAPK or NF-κB pathways (Zhou et al., 2018), triggering circulating metabolic endotoxemia and leading to lipid dysfunction (Sultana et al., 2016). Myeloid differentiation factor 88 (MyD88), a central adapter molecule for most TLRs, regulates fat storage and inflammatory responses (Everard et al., 2014), influences the activity of transcription factors related to lipid metabolism and BA profiles, and is involved in regulating the body’s inflammatory response and lipid metabolism (Duparc et al., 2017). Additionally, LPS forms a triple complex with CD14 and LPS-binding protein (LBP), which activates the MyD88/NF-B pathway and increases the production of numerous inflammatory proteins. Moreover, cluster of differentiation 36 (CD36) is an important lipid absorption regulator in the intestine, and its expression is mediated by derivatives of Desulfovibrio and Clostridium. Loss of CD36 may lead to excessive lipid accumulation and activation of inflammatory factors, triggering the risk of LMD (Plociennikowska et al., 2015; Petersen et al., 2019). Thus, chronic low-level inflammation in the organism may be a key link in the pathogenesis of LMD, and disorders of intestinal flora may also contribute to chronic inflammation.
2.3 The role of SCFAs in the development of LMD
SCFAs are metabolites derived from the fermentation of undigested carbohydrates or proteins by intestinal flora and include butyrate and acetate, propionate. Among them, butyrate is primarily performed by Firmicutes, while acetate and propionate are mostly generated by bacteria belonging to Bacteroidetes (Walker et al., 2005), and they can play a crucial part in controlling host appetite, lipid metabolism, inflammatory response, and maintenance of energy balance through various pathways (Sanchez et al., 2020). SCFAs, represented by butyric acid and propionic acid, not only stimulate the release of leptin from adipose tissue, suppressing hunger and reducing host feeding activity (Naraoka et al., 2018), but also inhibit hepatic FAS activity and reduce serum lipid (TG and TC) levels (Larkin et al., 2009). As key regulators of host lipid metabolism, about 95% of SCFAs are absorbed in the intestine or utilized by intestinal flora, acting as important regulators of host lipid metabolism. These molecules not only providing the host energy, but also acting as signal transduction molecules to initiate G protein-coupled receptors 43 and 41 (GPR43 and GPR41) in intestinal epithelial cells. Activated GPR43 and GPR41 promote the secretion of peptide tyrosine-tyrosine (PYY) and glucagon-like peptide-1 (GLP-1) from enteroendocrine L cells, which reduce appetite, increase energy release and inhibit adipocyte synthesis through the “brain-gut axis” (Eslick et al., 2022). SCFAs also promote beige lipogenesis, leading to enhanced fat oxidation and energy expenditure, a process associated with the activation of GPR43 or GPR41 (Lu et al., 2016). And butyric acid also improves serum lipid metabolism by increasing fatty acid oxidation in brown adipose tissue (BAT) through thermogenesis, significantly reducing serum TG levels (Li Z. et al., 2018). In addition, SCFAs may also be involved in lipid metabolism by regulating related pathways and gene expression. For example, butyric acid downregulates the expression of proliferator-activated receptor γ (PPARγ), upregulates the expression of uncoupling protein 2 (UCP2), promotes mitochondrial proton efflux, which in turn activates the AMPK pathway, decreases lipid synthesis, and increases lipid oxidation. Acetic acid decreases SREBP-1 expression and ATP citrate lyase (ATP-CL) mRNA levels, decreases acetyl coenzyme A supply, and contributes to a decrease in cholesterol and fatty acid synthesis; it also enhances acyl-CoA oxidase (AOX) gene expression, promotes fatty acid β-oxidation, and increases energy expenditure (Fushimi et al., 2006). While another study speculated that acetate regulation of lipid metabolism may be associated with an increase in the AMPK/PGC-1α/PPARα axis (Araujo et al., 2020). Additionally, SCFAs can regulate the intestinal barrier and inflammatory levels and are indirectly implicated in lipid metabolism regulation. According to studies, SCFAs can elevate intestinal trans-epithelial resistance and reduce LPS production, thereby upregulating tight junction protein expression and mucin secretion, reducing intestinal mucosal permeability and alleviating intestinal barrier disorders (Zhao et al., 2018). Meanwhile, SCFAs inhibit the expression of TNF-α/NF-κB inflammation-related genes, reduce the secretion of pro-inflammatory molecules like IL-6 and IL-12, as well as increase the secretion of anti-inflammatory molecules like IL-10 and IL-4, alleviating chronic inflammatory response and avoiding the formation of hyperlipidemia (Chang et al., 2014; Yuan et al., 2019).
2.4 The role of BAs in the development of LMD
The synthesis of BAs is the main pathway of cholesterol degradation and metabolism in humans, which is regulated by intestinal flora. The majority of primary BAs are converted from cholesterol by the enzymatic action of three cholesterol hydroxylases, CYP7A1, CYP8B1 and CYP27A1 in the liver (classical pathway), and a small proportion is processed by CYP27A1 and CYP7B1 in extrahepatic sites (alternative pathway). Primary BAs, which including cholic acid (CA) and chenodeoxycholic acid (CDCA), can bind to glycine or taurine to form conjugated BAs like glycocholic acid (GCA), glycochenodeoxycholic acid (GCDCA), taurocholic acid (TCA) and taurochenodeoxycholic acid (TCDCA). The bile salt export pump (BSEP) or ATP-binding cassette subfamily G member 5 (ABCG5)/ABCG8 subsequently actively transfers these conjugated BAs into the bile, where they contribute to lipid emulsification when the food enters the intestine (Jia et al., 2018). The apical sodium-dependent bile acid transporter (ASBT) reabsorbs around 95% of BAs from the intestine; The apical sodium-dependent bile acid transporter (ASBT) reabsorbs around 95% of BAs from the intestine; these are subsequently released into the portal circulation by the organic solute transporter α/β (OST α/β), which finally transports them back to the liver (Vourakis et al., 2021). Intestinal flora can reduce cholesterol levels by producing BSH and CYP7A1, which decouple conjugated BAs into unconjugated BAs including deoxycholic acid (DCA) and lithocholic acid (LCA), preventing their reabsorption through ASBT and promoting BAs excretion through the feces (Wahlström et al., 2016). It is known that BSH-producing bacteria include Bacteroides, Bifidobacterium, Clostridium, Eubacterium and Lactobacillus, while Clostridium and Eubacterium also possess 7α-dehydroxylation activity (Vourakis et al., 2021). BAs can also act as signaling molecules, such as conjugated BA T-βMCA, unconjugated BAs CDCA and LCA, which regulate lipid metabolism by interacting to the BA receptors like FXR and Takeda G protein-coupled receptor 5 (TGR5) to regulate BA self-synthesis, transport, as well as lipid digestion and absorption (Schoeler and Caesar, 2019). In summary, BAs play an important part in lipid metabolism homeostasis. Increasing intestinal BA production can block the reverse cycle from BA to cholesterol; it can also accelerate the transformation of cholesterol to BA, lowering serum TC levels. It is worth noting that the synthesis and excretion of BAs are related to the regulation of intestinal flora.
2.5 The role of TMAO in the development of LMD
Trimethylamine oxide (TMAO) is an intestinal flora-dependent metabolite formed by the oxidation of trimethylamine (TMA) in the liver by flavin-containing monooxygenase (FMO), especially FMO3 (Chen Y. et al., 2019). Studies have shown that serum TMAO levels are determined by genetics, diet and intestinal flora, and may be a biomarker of AS (Randrianarisoa et al., 2016; Canyelles et al., 2018). Another observational study found that TMAO levels were positively and significantly related with body mass index (BMI), fatty liver index and visceral obesity index, and could be used as a predictor of NAFLD and metabolic syndrome (Barrea et al., 2018). Additionally, 8 consecutive weeks of supplementation with a high TMAO diet may lead to elevated the levels of plasma TC, TG and LDL-C and induce hyperlipidemia (Koeth et al., 2013). Knockdown of FMO3 may downregulate circulating TMAO levels and acts as a preventive measure against hyperlipidemia (Miao et al., 2015). As a result, intestinal flora metabolite TMAO is essential for lipid metabolism. The underlying mechanism of TMAO-induced disruption of lipid metabolism is unknown; however, it may be associated with host cholesterol and BA metabolism. It has been investigated that TMAO reduces the expression of CYP7A1 and CYP27A1, two key enzymes necessary for the synthesis of BAs, and various BA transport proteins, decreases BA biosynthesis, inhibits the reverse cholesterol transport (RCT) pathway, and affects BA metabolism and cholesterol homeostasis in the hepatic-intestinal tract (Koeth et al., 2019). In turn, BAs can also lead to an increase in serum TMAO by mediating the upregulation of FMO3 expression by FXR (Bennett et al., 2013). Moreover, TMAO is able to induce CD36 and scavenger receptor A1 (SR-A1), which are involved in stimulating macrophages to bind ox-LDL, promoting macrophage foaminess and causing intracellular cholesterol accumulation (Wang et al., 2011). This process may also be mediated through MAPK and NF-κB signaling pathways that promote vascular inflammation, one of the earliest cellular signals in the atherosclerotic process (Ma et al., 2017; Geng et al., 2018).
2.6 The role of intestinal flora and its metabolites in the clinical monitoring and treatment of LMD
Obesity, NAFLD and AS are frequently associated with LMD. Therefore, for the efficient implementation of atherosclerotic cardiovascular disease (ASCVD) preventive and treatment methods, early diagnosis of dyslipidemia and monitoring changes in its levels are key foundations.
Routine lipid testing to identify the “hidden” high-risk groups will not only facilitate better treatment decisions, but more importantly, it will provide early intervention for these high-risk individuals or patients with LMD, so that they can have more cardiovascular benefit. The basic items of clinical lipid monitoring are TC, TG, LDL-C and HDL-C. It is noteworthy that LDL-C is an important causative factor of ASCVD and has an important significance in lipid monitoring. More and more clinical laboratories are using ApoAⅠ, ApoB, and Lp(a) as routine lipid testing items. In addition, the clinical testing items of oxLDL, FFA and non-HDL-C are also receiving increasing attention. In recent years, assays for intestinal flora and its metabolites have also been increasingly used to assist in monitoring lipid levels. Usually healthy people have low F/B ratio, while patients with LMD tend to have high F/B ratio (Human Microbiome Project, 2012). The main core of LMD such as hyperlipidemia is chronic low-level inflammation in the body, which is also caused by intestinal flora, often manifested as elevated serum LPS levels (Dai and Fu, 2019). SCFA is inversely correlated with lipid levels and can reduce serum TG and TC levels by inhibiting FAS activity (Larkin et al., 2009). BA is produced by cholesterol conversion, and its increased synthesis and impaired transport frequently lead to the development of LMD (Haeusler et al., 2016). Serum TMAO levels predict the risk of cardiovascular disease and are closely related to the regulation of cholesterol (Chen et al., 2019). Therefore, the detection of intestinal flora and its metabolites can also be used as a reference item for clinical lipid monitoring, but it still needs to be combined with the items of lipid testing for comprehensive assessment, so it is not yet popular in the clinic.
Currently, the guidelines recommend three main classes of lipid-lowering drugs (Du, 2022): 1) Statins, which reduce cholesterol synthesis in hepatocytes mainly through competitive inhibition of endogenous cholesterol synthesis rate-limiting enzymes, leading to a significant decrease in blood cholesterol levels, and are the cornerstone of primary prevention of ASCVD. 2) Ezetimibe, which reduces cholesterol by inhibiting the absorption of cholesterol in the intestinal tract. 3) Proprotein convertase subtilisin/kexin type 9 (PCSK9) inhibitors, which lower cholesterol levels by inhibiting the binding of PCSK9 to LDL receptors and preventing LDL receptor degradation, are the most potent cholesterol-lowering drugs available and have a good safety profile, offering another option for high-risk patients who are statin intolerant (Koren et al., 2019). The intestinal flora and its metabolites serve as a key link in lipid metabolism, and the therapeutic effect of lipid-lowering drugs may be related to the regulation of intestinal flora. Statins were found to modulate the abundance of SCFA, SBA, TMAO and LPS-producing intestinal flora, which in turn affected AMPK-SREBP, FXR, PXR, FFAR2 and TLR4-Myd88 signaling pathways, acting to regulate cholesterol metabolism and LDL-C levels (Sun et al., 2022). In addition, different statins, such as atorvastatin, simvastatin and resevastatin, have different effects on different intestinal flora. Desulfurization was negatively correlated with TG and HDL-C levels, and desulfurization was significantly reduced after ezetimibe intervention (Jin et al., 2022). Desulfovibrio was considerably decreased following ezetimibe treatment and had a negative correlation with TG and HDL-C levels (Jin et al., 2022). PCSK9 inhibitors can promote the clearance of LDL-C and LPS from the blood, regulate intestinal microecology, alleviate intestinal inflammation and endotoxemia, and reduce the risk of cardiovascular disease (Morelli et al., 2019). Furthermore, probiotic supplementation alone was able to significantly reduce plaque area in the full-length aorta and aortic sinus, lower plasma TMAO and cecum TMA levels, improve lipid disorders, reduce serum oxLDL and inflammatory factor levels in mice, increase cecum acetate and butyric acid levels, and reduce inflammatory responses in the aorta and liver of mice by inhibiting the levels of key proteins in the TLR4/MyD88/NF-kB/NLRP3 pathway (Wang, 2022). In contrast, mice on diets supplemented with choline or TMAO showed increased cholesterol levels in peritoneal macrophages, elevated TMAO plasma levels, and enhanced aortic atherosclerotic plaques (Wang et al., 2011). Thus, intestinal flora and its metabolites also play an important role in the treatment of LMD, but further studies are needed to validate this in order to maximize the clinical benefits of drugs.
3 Mechanisms of regulating intestinal flora in TCM for LMD
TCM is widely utilized to treat metabolic diseases in China. The effective components of TCM can directly interact with the intestinal flora once they enter the intestinal system by oral administration and produce therapeutic effects. Studies have shown that TCM (natural medicine extracts, Chinese herbal formulas and proprietary Chinese medicines) can effectively modify the host intestinal flora structure and its metabolite levels, repair the intestinal mucosal barrier, reduce inflammatory infiltration, as well as regulate lipid metabolism-related pathways and genes expression to correct LMD (Dai and Fu, 2019). Therefore, we systematically summarized the possible mechanisms involved in TCM to modulate intestinal flora and its metabolites in order to prevent and treat LMD (obesity, hyperlipidemia, NAFLD, and AS) (Figure 2).
3.1 TCM remodels the structure of intestinal flora to improve LMD
TCM treatment encourages the development of beneficial intestinal bacteria by increasing the diversity of intestinal flora, while inhibiting the growth of harmful bacteria (Ding K. et al., 2019; Ling et al., 2022). It was found that intervention with Zexie Tang (ZXT) (Xu et al., 2017), Shenlingbaizhu Powder (SLBZ) (Hong, 2021), Ganoderma lucidum polysaccharide (GLP) (Sang et al., 2021), G. lucidum polysaccharide and chitosan (PC) (Tong et al., 2020), Pueraria lobata starch (PLS) (Yang Y. et al., 2022), Luteolin (Liu, 2022), Naringin (Wang F. et al., 2020), Inulin (Bao, 2021; Cui et al., 2022), Epigallocatechin gallate (EGCG) (Zuo, 2020), RSV (Chen et al., 2016) greatly enriched the abundance of Bifidobacterium in the intestinal tract; Modified Xiongdan yinchen granules (MXYG) (Wu et al., 2021), Tian Huang Formula (THF) (Pang, 2021), Tea seed saponins (Lin et al., 2020), Kaempferol (Wang T. et al., 2020), Green Brick Tea (Zhou T. T. et al., 2021) were able to upregulate the relative abundance of Lactobacillus; the intervention of Yunpi Huazhuo granules (YPHZ) (Kou et al., 2022), Qinggan Qushi Huoxie prescription (QGQSHX) (Zhang X. Y. et al., 2022), Jiangzhi Ligan Decoction (JZLG) (Tang et al., 2016), Modfied Yinchen Wuling San (MYCWL) (Xu et al., 2019), Purple yam (Dioscorea alata L.) resistant starch (PYRS) (Li T. et al., 2019) and PLS (Yang Y. et al., 2022) also increased the abundance of intestinal Bifidobacterium and Lactobacillus, resulting in an increase in the proportion of beneficial bacteria and a corresponding increase in its metabolites, such as acetate and lactate, thus lowering the PH in the intestine and greatly inhibiting the development of harmful bacteria such as Parabacteroides, Desulfovibrio, Escherichiacoli, Enterococcus and Helicobacter. Interestingly, we also found that Alistipes had a negatively relationship with serum lipid profile, while PC (Tong et al., 2020) and Porphyran (Wang X. et al., 2022) were able to reduce lipid levels by upregulating the abundance of Alistipes. Blautia was negatively correlated with visceral fat area, and the number of Blautia was noticeably increased by Ilex pubescens triterpenoid saponins (IPTS) (Ba et al., 2021) treatment. Akkermansia was negatively correlated with inflammation levels, while Alisma orientale extract (Li L. S. et al., 2019), Jaboticaba peel and seed powder (JPSP) (Soares et al., 2021) were able to elevate the relative abundance of Akkermansia and reduce inflammation levels and lipid accumulation. Faecalibaculum, a SCFAs-producing bacteria, while Burdock inulin (Wang, 2020) and Yinchenhao Decoction (Li Z. H. et al., 2019) can upregulate the relative abundance of Faecalibaculum to promote the production of SCFAs and alleviate inflammation to improve lipid metabolism. In contrast, the opposite result was observed after Caffeic acid (Mu et al., 2021) treatment, which showed a decrease in the abundance of Faecalibaculum. This suggests that it may be the role of multiple intestinal flora in regulating lipid metabolism and inflammation levels, and that Faecalibaculum may not be a core flora.
In addition, the effectiveness of TCM in reversing the elevated F/B ratio in animals with a HFD has been experimentally confirmed. It was found that some natural medicine extracts, proprietary Chinese medicines and Chinese herbal formulas reduce F/B ratio by decreasing Firmicutes abundance and increasing Bacteroidetes abundance, such as Guizhi Tang (GZT) (Yuan et al., 2021), Naoxintong Capsule (NXT) (Lu et al., 2022), Huanglian Jiedu Decoction (HLJD) (Jiang et al., 2021), Quyu Huatan Tongmai Prescription (QYHTTM) (Miao et al., 2022), Danggui Shaoyao San (DGSY) (Yu et al., 2021), Laminaria japonica polysaccharide (LJP) (Zhang et al., 2021d), Porphyran-derived oligosaccharides (PYOs) (Wang X. et al., 2021), Ethyl Acetate Extract of Eleutherococcus senticosus (Rupr. & Maxim.) Maxim. [Araliaceae, Acanthopanacis senticosi radix et rhizoma seu caulis] (Jia et al., 2022) and Lonicera caerulea L. berry polyphenols (LCBP) (Wu et al., 2018). In contrast, the abundance of Firmicutes and Bacteroidetes were not mentioned after applying interventions such as Jieyu Qutan Huazhuo Prescription (JYQTHZ) (Li N. et al., 2021; Li N. et al., 2022), Shenerjiangzhi formulation (SEJZ) (Zhang et al., 2022c), Jian Pi Tiao Gan Yin (JPTGY) (Dong et al., 2022), Shanmei (SM) Capsule (Du et al., 2022), Tongxinluo (TXL) Capsule (Qi et al., 2022), Hugan Qingzhi Tablet (HGQZ) (Tang et al., 2018; Tang, 2019), Compound Danshen Dripping Pills (Zhang Y. Y., 2020), Jiangan Jiangzhi Pill (JGJZ) (Zhao Z. et al., 2022), Jiangzhi Granules (JZG) (Wang R. R. et al., 2021), Procyanidin B2 (PB2) (Xing et al., 2019), Resistant starch (RS) (Shou, 2021), Tea polyphenol (TP) (Wang et al., 2018) and Gynostemma pentaphyllum (Thunb.) Makino [Cucurbitaceae; Gynostemmatis herba] (GP) (Huang et al., 2018), and only a decrease in F/B ratio was reported. It has been suggested that one of the pathways in which TCM regulates LMDs is through a relative decrease in the F/B ratio of the intestine. However, it remains to be further investigated exactly by which bacteria are regulated, or which of the many genera affected by TCM have the greatest effect on F/B ratio by alteration.
3.2 TCM restores intestinal barrier and alleviates LPS-induced inflammation to improve LMD
Studies have found that TCMs are effective in improving the inflammatory response related to dyslipidemia, possibly by maintaining intestinal barrier and reducing the release of inflammatory factors. Some natural medicine extracts, proprietary Chinese medicines and Chinese herbal formulas, such as Xiexin Decoction (XXD) (Chen, 2021), Biejia Jian Wan (BJJW) (Qiu et al., 2017), RSV (Yao, 2017) increased occludin mRNA expression; Dengzhan Shengmai Capsules (DZSM) (Guo et al., 2022), Senna tora (L.) Roxb. [Fabaceae; Cassiae semen] (ST) (Luo et al., 2021), Astragalus mongholicus polysaccharides (mAPS) (Zhong M. et al., 2022), Lycium barbarum polysaccharide (LBPs) (Gao et al., 2021), Inulin (Perez-Monter et al., 2022), EGCG (Zuo, 2020), Usnea diffracta Vain. [Usneaceae; U. diffracta] (UD) (Zhang L. D. et al., 2021) and Momordica charantia L. [Cucurbitaceae; Fructus momordicae] (MC) (Bai, 2019) also significantly enhanced occludin and ZO-1 expression, thus significantly enhancing intestinal barrier integrity. Huayu Qutan Formula (HYQT) (Zheng et al., 2022), Gegen Qinlian Decoction (GGQL) (Liu, 2019), Diammonium glycyrrhizinate (DG) (Li Y. et al., 2018) and GLP (Sang et al., 2021) significantly upregulated Claudin-1, Occludin and ZO-1 expression, which repaired intestinal mucosal barrier and prevented the development of chronic inflammation and LMD. Moreover, WIP (Sun et al., 2019) and JZG (Wang R. R. et al., 2021) elevated the expression of mucosal integrity protein Muc5 in addition to ZO-1 and occluding has also been reported to increase the expression of Muc2 and Muc4, maintain intestinal mucosal integrity, and improve intestinal inflammation (Li W. et al., 2019). Akkermansia, a mucin-degrading bacteria present in the mucosal layer, it can produce certain enzymes that affect the expression of mucins and tight junction proteins to regulate the intestinal barrier function. RSV (Chen, 2019; Chen et al., 2020), BBR (Zhu et al., 2018) and Rosa roxburghii Tratt polysaccharide (RTFP) (Zhang p. et al., 2022) were able to enrich the abundance of Akkermansia, and increase the colonic mucus layer thickness and intestinal tight junction proteins expression, thus reducing intestinal hypometabolic endotoxemia. Therefore, all of these TCMs can enhance intestinal barrier integrity and reduce intestinal permeability, thereby improving intestinal inflammation and abnormal lipid metabolism.
The abundance of Desulfovibrio, a producer of LPS, was positively correlated with inflammation and LMD. Danlou Tablet (DLT) (Sun et al., 2020), DZSM (Guo et al., 2022), GQD (Liu, 2019), SLBZ (Zhang et al., 2018), DG (Li Y. et al., 2018), Chitooligosaccharide (COSM) (Feng et al., 2022), Rhizoma Coptidis (RC) alkaloids (He et al., 2016), Baicalin (Liu, 2016), Quercetin (Porras et al., 2017) and BBR (Xu, 2013) might ameliorate LMD by decreasing the abundance of Desulfovibrio to reduce LPS into the blood and inflammatory factors secretion. Notably, DLT (Sun et al., 2020), Gastrodin (Liu F. Y. et al., 2021) could downregulate the levels of inflammatory markers IL-1β, ICAM-1 and TNF-α, favoring the reversal of periaortic inflammation and reduction of plaque area in AS mice. By reducing LPS levels, EGCG (Li, 2020; Zuo, 2020), Citrus Peel Powder extract (CPP) (Wang H. et al., 2022), HGQZ (Tang et al., 2018), Si Ni San (SNS) (Zhu et al., 2019), Rosa Laevigata Michx. Fruits Polysaccharides (RLPs) (Zhang X. J., 2020), Honokiol (Ding Y. et al., 2019), Fucoidan (Huang J. L., 2021) and ST (Luo et al., 2021) were found to inhibit the excessive production of inflammatory cytokines (mainly TNF-α, IL-6, IL-1β and MCP-1). In contrast, Alisma orientalis Beverage (AOB) (Zhu, 2021), Chaihu Shugan san (CHSG) (Xie et al., 2021), RSV (Chen, 2019), ST (Luo et al., 2021), Zhibitai Capsule (ZBT) (Pan et al., 2020), Xiaoyao San (XYS) (Zhou, 2020), JGJZ (Zhao Z. et al., 2022), Qinghua Fang (QHF) (Wang Y. et al., 2021), RTFP (Zhang p. et al., 2022), LBPs (Gao et al., 2021), Noni fruit polysaccharide (NFP) (Yang et al., 2020), Inulin (Bao, 2021; Wang L. J. et al., 2021), Quercetin (Wu et al., 2019), BBR (Wu et al., 2020), GP (Shen et al., 2020), CPP (Hu et al., 2021), Paeonol (Pae) (Jiang, 2019) and Blackberry leaf and fruit extracts (BLF) (Park et al., 2019) had effective antagonistic effects on the secretion of pro-inflammatory factors (e.g., IL-6, IL-1β and TNF-α) although the effects on LPS levels were not mentioned. In addition to this, some natural medicine extracts also have a promoting effect on anti-inflammatory cytokines IL-10, such as RSV, LBPs, NFP, COSM, BBR and Inulin.
Numerous experimental studies have demonstrated that TCMs achieve improvement of LMD by down-regulating inflammation-related signaling pathways (Li, 2015). Huazhi-Rougan formula (HZRG) (Li C. et al., 2022), DZSM (Guo et al., 2022), mAPS (Zhong M. et al., 2022), Luteolin (Liu, 2022) and Myricetin (Sun et al., 2021) markedly reduced metabolic endotoxemia and chronic hypo-inflammation associated with LPS, downregulated TLR4 mRNA and protein expression, inhibited IKKβ phosphorylation, and prevented p65 NF-κB translocation to the nucleus by modulating the TLR4/NF-κB pathway. CA (Mu et al., 2021) decreased LPS/TLR4, an LPS-mediated inflammatory pathway, and reduced TNF-α, IL-6 levels, thereby inhibiting dysregulation of lipid metabolism. LBPs (Gao et al., 2021) and COSM (Feng et al., 2022) inhibited indicators related to hepatic LPS/TLR4/NF-κB signaling pathway and attenuated the level of inflammation. HQT (Tang, 2019) and Compound Danshen Dripping Pills (Zhang Y. Y., 2020) selectively targeted TLR4 and inhibited LPS-induced inflammatory mediator production by attenuating the LPS/TLR4/MyD88 pathway. SLBZ (Zhang et al., 2018) and polyphenol-rich loquat fruit extract (LFP) (Li W. et al., 2019) also exhibited downregulation of the TLR4/MyD88 pathway and its downstream molecules, thereby inhibiting the overproduction of serum lipids. While, Qiang Gan formula extract (QGE) (Li et al., 2020), GLP (Sang et al., 2021) and EGCG (Zuo, 2020) regulated lipid metabolism-related processes by reducing the expression of LPS, LBP, and CD14, inhibiting TLR4/MyD88/NF-κB signaling pathway, reducing inflammatory factors secretion, and improving endotoxemia. Si Miao Formula (SMF) (Han et al., 2021), TXL (Qi et al., 2022) and Pae (Liu, 2021) inhibited the expression of NLRP3 inflammatory vesicles and their downstream factors including IL-18, IL-1β and caspase-1, alleviated the inflammatory response and reduced lipid levels. Furthermore, MC also inhibited LPS intestinal leakage, elevated intestinal tight junction protein expression, and protected intestinal mucosal integrity, which was associated with inhibition of the intestinal NF-κB/JNK/MAPKs pathway (Bai, 2019). Notably, Luffa cylindrica (L.) Roem [Cucurbitaceae; Luffa aegyptiaca Miller] (LC) (Zhang et al., 2019) and Berberrubine (BRB) (Yang S. et al., 2022) resulted in genes associated with hepatic lipid metabolism expression reduction, including fatty acid synthase (FAS), fatty acid synthesis protein (FABP), fatty acid transport protein (FATP), SREBP-1c and CD36, thus acting to reduce lipid levels.
3.3 TCM modulates the level of SCFAs to improve LMD
Although the strong connection between intestinal flora, SCFAs and LMDs are still unclear, an increasing number of studies have shown that LMDs are directly correlated with both the number of SCFA-producing bacteria and the level of SCFAs. To play its part in treating LMDs, TCM can control the prevalence of bacteria that produce SCFAs and encourage the generation of SCFAs. LC (Zhang et al., 2019), DLT (Sun et al., 2020), NXT (Lu et al., 2022), IPTS (Ba et al., 2022), LJP (Zhang et al., 2021d), LBPs (Gao et al., 2021), Zanthoxylum bungeanum Maxim. [Rutaceae; Zanthoxyli pericarpium] (ZB) (You, 2016), Ginkgo biloba extract (GbE) (Wang Y. et al., 2022), Inulin (Bao, 2021; Wang L. J. et al., 2021), NFP (Yang et al., 2020), TP (Wang et al., 2018), Bilberry anthocyanins (Nakano et al., 2020) and COSM (Feng et al., 2022) significantly improved serum lipid levels (TC, TG) as well as reducing lipid accumulation, which may be associated with modifying intestinal flora and increasing SCFAs levels. In-depth research revealed that Compound Danshen Dripping Pills (Zhang Y. Y., 2020) and JZG (Wang R. R. et al., 2021) intervention elevated the abundance of Lachnospiraceae; Qingxin Jieyu Granule (QXJY) (Wang, 2019) and water insoluble polysaccharide WIP (Sun et al., 2019) intervention increased Clostridium abundance; Macroalgae Laminaria japonica (MLJ) (Zhang Q. et al., 2020), Quercetin (Porras et al., 2017), Myricetin (Sun et al., 2021), MC (Bai, 2019) and GLP (Sang et al., 2021) administration improved the abundance of Allobaculum; Erchen decoction (ECD) (Liu H. et al., 2021) and EGCG (Zuo, 2020) administration enhanced the abundance of Roseburia; HGQZ (Tang, 2019) and DG (Li Y. et al., 2018) treatment increased the abundance of Ruminococcaceae; SLBZ (Hong, 2021) and DZSM (Guo et al., 2022) administration enriched the abundance of Bifidobacterium, Lactobacillus; Guanxinning Tablet (GXNT) (Yang Q. et al., 2022), Black tea polyphenols (BTP) (Henning et al., 2018) and RLPs (Zhang X. J., 2020) treatment all increased the relative abundance of Prevotella; G. pentaphyllum saponins (GPS) (Zhong F. W. et al., 2022) administration enriched the abundance of Bacteroides. The above mentioned Ruminococcaceae, Lachnospiraceae, Clostridium, Allobaculum, Bifidobacterium, Lactobacillus, Prevotella, Roseburia, Bacteroides are all SCFAs-producing bacteria. In addition, TCM can promote the synthesis of key synthetic enzymes of SCFAs to elevate the level of SCFAs, or mediate SCFAs to regulate energy metabolism and inflammation-related pathways to improve LMD. GXNT treatment was also found to promote the production of butyrate kinase, propionate kinase, the key synthetic enzymes of SCFAs, thereby increasing the levels of butyric acid and propionic acid (Yang Q. et al., 2022). BTP treatment increased the level of SCFAs and activated the AMPK signaling pathway to increase energy expenditure, thus exerting a fat-lowering and weight-reducing effect (Henning et al., 2018). WIP treatment enhanced intestinal butyric acid levels, improved intestinal mucosal integrity, and regulated PPAR-γ pathway in the intestine (Sun et al., 2019). Dingxin Recipe IV (DXR) intervention promoted acetate, butyrate, propionate production, inhibited LXR-α/SREBP1 pathway and improved lipid metabolism (Zhang et al., 2021c). The mechanism through which GLP intervention ameliorated LMD may be indirectly engaged in regulating lipid metabolism by promoting the production of acetate and butyrate, activating the GPR43 receptor and modulating the intestinal barrier and inflammatory response (Sang et al., 2021).
3.4 TCM regulates BAs metabolism to improve LMD
Studies have shown that GXNT (Yang Q. et al., 2022) and Quercetin (Nie et al., 2019) can reduce serum or intestinal BAs levels. HYQT (Sui et al., 2021b), HZRG (Li C. et al., 2022), Proanthocyanidin (Fu et al., 2013), Theabrownins (Huang et al., 2019), Radix scutellariae water extract (Zhao et al., 2021), ZB (You, 2016), GbE (Wang Y. et al., 2022), QGE (Li et al., 2020) and Pae (He, 2021) promoted the conversion of cholesterol to BA and significantly increased the excretion of BA in feces. In-depth studies have revealed that the hypolipidemic effects of TCM are closely related to the modulation of BA synthesis and transport pathways. Among them, HYQT can increase BA synthesis-related genes expression, upregulate hepatic CYP7A1 expression, promote BA biosynthesis, and reduce serum lipid levels (Sui et al., 2021b). XXD may be achieved hypolipidemic effects by upregulating CYP8B1 expression (Lei, 2020), and Baicalein exhibited the same effects (Li P. et al., 2022). HZRG aimed to reduce serum TC and TG levels mainly by decreasing the expression of BA transporter-related genes ASBT and OSTβ mRNA and reducing the reabsorption of harmful BA such as LCA, DCA and HCA (Li C. et al., 2022). QGE promoted BA transport in the liver by increasing BSEP expression and hepatic TGR5 receptor expression to attenuate the inflammatory response and regulate lipid metabolism in the liver (Li et al., 2020). RC alkaloids reduced lipid and TBA levels involving multiple BA receptor pathways, and were associated with reduced ASBT expression and elevated CYP7A1 and TGR5 expression (He et al., 2016). NXT decreased BSH enzyme activity, modulated the BA profile in the intestine, and reduced lipid deposition, but the exact mechanism has not been elucidated (Lu et al., 2022). It was found that treatment with THF (Yang L. et al., 2022), WESB (Zhao et al., 2021), HQF (Sui et al., 2021b), TB (Huang et al., 2019), Pae (He, 2021), Naringin (Wang F. et al., 2020) and Penthorum chinense Pursh. (PCP) extract (Li X. et al., 2022) decreased the abundance of intestinal flora that produces BSH and BSH enzyme activity, leading to a weakened hydrolysis of BAs, increased the level of FXR antagonist T-βMCA, decreased FXR agonist CDCA and LCA levels, inhibited FXR/FGF15 axis in the intestine, activated CYP7A1 and CYP7B1 expression in the liver, and promoted hepatic BA synthesis, thereby reducing serum and hepatic TC levels. In addition, RSV enhanced BSH enzyme activity via boosting the number of BSH-producing bacteria, including Bifidobacterium and Lactobacillus, to promote BA catabolism and fecal excretion in the intestine, downregulated OSTα, OSTβ expression to reduce BA reabsorption transport, and reduced CDCA content to suppress enterohepatic FXR/FGF15 axis in order to promote hepatic BA synthesis (Chen et al., 2016).
3.5 TCM adjusts TMAO production to improve LMD
It was found that serum lipid and TMAO levels were significantly decreased in HFD-induced AS model animals after treatment with Ginkgolide B (GB) (Lv et al., 2021), Eucommia ulmoides extract (Sun, 2020), HYQT (Sui et al., 2021a) and AOB (Zhu, 2021). The possible mechanism is to reduce TMA production, decrease hepatic FMO3 expression and inhibit the oxidation of TMA to TMAO by adjusting intestinal flora, which is a process consistent with the study of Xiangsha Liujunzi Decoction (XSLJZ) for the treatment of hyperlipidemia model rats (Wang J. et al., 2022). BBA reduces TMAO biosynthesis by decreasing the abundance of TMAO-related enzymes, such as carnitine oxygenase (CntA), choline-trimethylamine lyase (CutC), FMO and betaine reductase, effectively reducing serum TMAO levels (Wu et al., 2020; Li X. et al., 2021; Ma et al., 2022). The content of TMAO in the blood was shown to be associated to the severity of AS. Treatment with Tongmai Zhuyu decoction (TMZY) (Ji et al., 2020) or Tanyutongzhi Formula (TYTZ) (Wang, 2016) led to the reduction of TMAO levels as well as the decrease of atherosclerotic plaque area in AS model animals, and its anti-AS effect may be connected with TMAO reduction and the reduction of endothelial damage and inflammation levels. Another study showed that GXNT treatment was effective in reducing the production of TMAO, a TMA metabolite, lowering ox-LDL levels and inhibiting macrophage foaminess, while reducing NF-κB expression in AS plaques and alleviating the inflammatory response, thus preventing and preventing the formation of AS, which was correlated with reduced Escherichia abundance (Yang Q. et al., 2022). Furthermore, Jianpi Huazhuo Tiaozhi Granule (JPHZTZ) (Huang Q., 2021) and RSV (Chen et al., 2016) also exerted significant efficacy in anti-AS by a mechanism that may be achieved by inhibiting the enterohepatic FXR/FGF15 pathway, upregulating hepatic CYP7A1 expression, promoting BA biosynthesis, reducing TMAO production, and maintaining cholesterol metabolic homeostasis.
4 Discussion
Lipid metabolism, which is an essential component of an organism’s fundamental metabolism, and LMD is linked inextricably to numerous illnesses and their consequences. With the rapid development of sequencing technology, significant advancements in our study of intestinal flora have been achieved, and it is also clear that lipid metabolism is closely related to intestinal flora. When LMD occur, the structure and composition of intestinal flora are abnormal, while dysbiosis of intestinal flora can further aggravate LMD. Therefore, the relationship between intestinal flora metabolites and LMD (hyperlipidemia, obesity, NAFLD and AS) can provide new perspectives for understanding LMD, and promote the development of research and treatment methods of metabolism disorders.
In this review, we systematically analyzed the previous related literature and found that intestinal flora and its metabolites may be major targets of TCM for treating LMD (Tables 1, Supplementary Table S1). The composition of Chinese herbal formulas and proprietary Chinese medicines is shown in the Supplementary Table S2. TCM can reshape the composition and structure of intestinal flora by enhancing the abundance of beneficial intestinal bacteria, for example, increasing the abundance of Akkermansia to repair intestinal barrier function (Park et al., 2019); upregulating Prevotella, a bacteria that produces SCFAs, to improve lipid metabolism of the host and alleviate inflammatory responses (Tong et al., 2020); promoting the growth of Lactobacillus and Bifidobacterium, and enhancing BSH enzyme activity to promote BA catabolism and fecal excretion in the intestine to accelerate cholesterol excretion (Yang L. et al., 2022). At the same time, TCM can also reduce the abundance of harmful bacteria by inhibiting the growth of pathogenic bacteria such as Escherichiacoli and Enterococcus, down-regulating the number of Desulfovibrio, bacteria that produces LPS, alleviating low chronic inflammatory response and metabolic endotoxemia in the intestine, and maintaining intestinal microecological health (Zhang et al., 2018; Kou et al., 2022). Notably, TCM also reduces the risk of abnormal lipid metabolism diseases by lowering the F/B ratio, reducing fat accumulation in the body and accelerating fat metabolism. For example, Luteolin modulated intestinal flora (decreased F/B ratio, elevated Bifidobacterium, Lactobacillus) against liver fatty lesions in rats (Liu, 2022), while BBR successful in boosting Akkermansia abundance, it also enhanced intestinal tight junction protein expression and colonic mucus layer thickness, reduced HFD-induced metabolic endotoxemia and decreased expression of pro-inflammatory factors and chemokines (Zhu et al., 2018). After SLBZ supplementation treatment, the abundance of Desulfovibrio was reduced in NAFLD rats, and LPS production as well as the secretion of inflammatory factors were inhibited, resulting in improvement of LMD (Hong, 2021). NXT reduced BSH enzyme activity in hyperlipidemic rats and modulated the BA profile in the intestine to reduce lipid levels in serum and liver (Lu et al., 2022). RLPs increased the relative abundance of Prevotella, promoted the production of SCFAs such as Propionic acid and butyric acid, and reduced lipid levels in NAFLD mice (Zhang X. J., 2020). In addition, TCM can also modulate intestinal flora metabolites to play a role in correcting LMD, including LPS, SCFAs, BAs and TMAO. Among them, LPS and SCFAs-mediated inflammatory pathways are key aspects of herbal agents for lipid lowering. BAs have a vital part in maintaining lipid metabolism homeostasis, involving lipid synthesis, transport, and excretion. TMAO is positively correlated with visceral obesity index and is not only involved in the biosynthesis of BAs, but also serves as a biomarker for AS (Barrea et al., 2018). Given its multi-component and multi-target of action characteristics, TCM often also affects MAPK or TLR4/NF-κB pathway-mediated chronic inflammatory responses and FXR/FGF15 pathway-mediated metabolism of BAs to regulate lipid metabolism. For instance, Quercetin reduced LPS translocation, promoted SCFA production, increased intestinal Occludin, Claudin-1 expression, repaired intestinal barrier function, and inhibited TLR4 pathway to improve inflammation levels by regulating NAFLD intestinal flora (Porras et al., 2017). Myricetin significantly reduced LPS-induced metabolic endotoxemia and systemic inflammation, corrected dyslipidemia and restored liver function in NAFLD rats by regulating the TLR4/NF-κB signaling pathway (Sun et al., 2021). After treatment with BTP, it can effectively increase the level of SCFAs in obese mice, and at the same time activate AMPK signaling pathway to increase energy consumption for weight loss (Henning et al., 2018). QGE promoted BA transport in the liver by increasing BSEP expression, in addition to upregulating hepatic TGR5 receptor expression, attenuating hepatic inflammatory response and regulating lipid metabolism in NAFLD mice (Li et al., 2020). RSV regulated lipid levels mainly by regulating BA metabolism, which not only promoted the catabolism of BA in the intestine and fecal excretion, but also downregulated OSTα, OSTβ expression and reduced BA reabsorption transport (Chen et al., 2016). HYQT may reduce TMA production, decrease hepatic FMO3 expression and inhibit TMAO synthesis by altering the structure of the intestinal flora; meanwhile, it can inhibit the intestinal and hepatic FXR/FGF15 axis, promote the synthesis and excretion of BAs and maintain the balance of cholesterol metabolism, and finally achieve the purpose of preventing and treating AS (Sui et al., 2021a; Sui et al., 2021b). It can be seen that TCM and its effective ingredients can improve the metabolic indexes of blood lipids directly or indirectly by acting on intestinal flora and its metabolites, thus playing a comprehensive role in preventing and treating LMD.
The study of the interaction between TCM and intestinal microorganisms has an important prospect, and the in-depth study of the interaction between TCM and intestinal flora is conducive to the elucidation of the potential mechanisms of TCM in preventing and treating LMD, as well as to the enrichment of TCM theory. In recent years, a few reviews have been reported on the regulation of intestinal flora by TCM to improve metabolic diseases, but there are shortcomings such as few included studies and few diseases involved (Zhang H. Y. et al., 2021; Li Y. et al., 2021). Based on previous studies, this review enriches the number of included literature and adds the amount of LMD, including hyperlipidemia, obesity, NAFLD and AS. Also, we illustrate potential mechanisms of TCM for regulating intestinal flora to improve LMD by category, which helps to minimize the issue of biased reporting. In this review, we have conducted a full quality assessment of the included original literature, which includes the dosage, periodicity and quality control of the use of TCM prescriptions (Chinese herbal formulas and proprietary Chinese medicines) and natural medicine extracts in animal experiments and clinical trials (Tables 2–4). However, there are some limitations in the included studies. 1) The impact of TCM on LMD based on intestinal flora has gained attention in the scientific community, and the number of related clinical studies is growing. However, there are issues such as small sample sizes, poor quality of single studies, as well as few reports of large-scale clinical trials. 2) There were differences in the specific content of the control group between the included original literature, and although the experimental groups were Chinese herbal formulas or natural medicine extracts that could regulate intestinal flora, the specific groups were different, which was also a source of heterogeneity and may have affected the results of the review assessment. 3) The majority of the original literature that was considered did not mention any allocation concealment, blinding, or other concerns, which made the results less reliable. 4) Despite the extensive search, the currently included literature is mainly in English and Chinese, which may have regional bias and language bias. 5) The current research on the treatment of diseases related to LMD by TCM is mainly focused on obesity, hyperlipidemia and NAFLD, while there are fewer studies on diseases like AS, which may be related to the bioavailability of TCM and to the stage and severity of the disease. 6) The number of research on various intestinal metabolites is significantly skewed. Most studies of TCM against LMD have taken the chronic low levels of inflammation as their entry point, focusing mainly on the regulation of LPS levels and the levels of SCFAs in stool, while fewer studies have been conducted on BAs and TMAO, which are lipid metabolism-related risk factors. 7) Studies on intestinal flora are mostly correlational, while relatively few studies on causality are available. 8) Most of the research is focused on natural medicine extracts, but there is still considerable opportunity for research on proprietary Chinese medicines and Chinese herbal formulas. Therefore, Future research should focus on how the effective ingredients of TCM are bio-transformed by intestinal flora, and whether these biotransformation metabolites have synergistic or antagonistic effects on the treatment of LMD in TCM, which will help discover new favorable metabolites of intestinal flora. Furthermore, it is necessary to conduct more high-quality studies to verify the safety of TCM in modulating intestinal flora to improve LMD, so as to promote clinical application and provide new ways and references for the intervention targets of TCM in preventing and treating LMD.
In summary, intestinal microecology plays a significant part in the development of LMD, and the regulation of intestinal flora and its metabolites is a potential new therapeutic target for LMD. TCM has obtained some achievements in improving lipid metabolism disorder diseases, probably by regulating intestinal flora and its metabolites, but in order to clarify the precise mechanism of action, more extensive research will still be required in the future.
Author contributions
ML, YW, and KW designed the research protocol; WS and YH collected and organized the literature; ML drafted the original manuscript; YW and KW revised the manuscript.
Funding
This study was supported by the National Natural Science Foundation of China (Nos 81973684 and 81873334).
Conflict of interest
The authors declare that the research was conducted in the absence of any commercial or financial relationships that could be construed as a potential conflict of interest.
Publisher’s note
All claims expressed in this article are solely those of the authors and do not necessarily represent those of their affiliated organizations, or those of the publisher, the editors and the reviewers. Any product that may be evaluated in this article, or claim that may be made by its manufacturer, is not guaranteed or endorsed by the publisher.
Supplementary material
The Supplementary Material for this article can be found online at: https://www.frontiersin.org/articles/10.3389/fphar.2023.1134430/full#supplementary-material
References
Allegretti, J. R., Kassam, Z., Mullish, B. H., Chiang, A., Carrellas, M., Hurtado, J., et al. (2020). Effects of fecal microbiota transplantation with oral Capsules in obese patients. Clin. Gastroenterol. Hepatol. 18 (4), 855–863 e852. doi:10.1016/j.cgh.2019.07.006
Araujo, J. R., Tazi, A., Burlen-Defranoux, O., Vichier-Guerre, S., Nigro, G., Licandro, H., et al. (2020). Fermentation products of commensal bacteria alter enterocyte lipid metabolism. Cell. Host Microbe 27 (3), 358–375 e357. doi:10.1016/j.chom.2020.01.028
Aron-Wisnewsky, J., Warmbrunn, M. V., Nieuwdorp, M., and Clement, K. (2021). Metabolism and metabolic disorders and the microbiome: The intestinal microbiota associated with obesity, lipid metabolism, and metabolic health-pathophysiology and therapeutic strategies. Gastroenterology 160 (2), 573–599. doi:10.1053/j.gastro.2020.10.057
Ba, R. Y., Y, H., Chen, F. L., Qiu, J. W., Wang, Y., Chen, B. Y., et al. (2021). Effect of triterpenoid saponins from Ilex pubescens on intestinal flora in atherosclerotic rats. Chin. Traditional Herb. Drugs 52 (20), 6245–6253.
Ba, R. Y., Y, H., Qiu, J. W., Chen, F. L., Wang, Y., Chen, B. Y., et al. (2022). Effects of Ilex pubescens triterpenoid saponins on fecal and urine metabolomics in atherosclerotic rats. Nanjing Univ. Tradit. Chin. Med. 38 (05), 424–431. doi:10.14148/j.issn.1672-0482.2022.0424
Bai, J. (2019). The Correlation and Mechanism between improved Endotoxemia and gut Microbiota by bitter melon intervention in obese rats. [dissertation/master’s thesis]. Zhenjiang: Jiangsu University.
Bao, T. (2021). Inulin ameliorates non-alcoholic fatty liver disease via modulating gut microbiome and inflammation. [dissertation/master’s thesis]. Yinchuan: Ningxia Medical University.
Barrea, L., Annunziata, G., Muscogiuri, G., Di Somma, C., Laudisio, D., Maisto, M., et al. (2018). Trimethylamine-N-oxide (TMAO) as novel potential biomarker of early predictors of metabolic syndrome. Nutrients 10 (12), 1971. doi:10.3390/nu10121971
Bellosta, S., and Corsini, A. (2018). Statin drug interactions and related adverse reactions: An update. Expert Opin. Drug Saf. 17 (1), 25–37. doi:10.1080/14740338.2018.1394455
Bennett, B. J., de Aguiar Vallim, T. Q., Wang, Z., Shih, D. M., Meng, Y., Gregory, J., et al. (2013). Trimethylamine-N-oxide, a metabolite associated with atherosclerosis, exhibits complex genetic and dietary regulation. Cell. Metab. 17 (1), 49–60. doi:10.1016/j.cmet.2012.12.011
Cani, P. D., Amar, J., Iglesias, M. A., Poggi, M., Knauf, C., Bastelica, D., et al. (2007a). Metabolic endotoxemia initiates obesity and insulin resistance. Diabetes 56 (7), 1761–1772. doi:10.2337/db06-1491
Cani, P. D., Neyrinck, A. M., Fava, F., Knauf, C., Burcelin, R. G., Tuohy, K. M., et al. (2007b). Selective increases of bifidobacteria in gut microflora improve high-fat-diet-induced diabetes in mice through a mechanism associated with endotoxaemia. Diabetologia 50 (11), 2374–2383. doi:10.1007/s00125-007-0791-0
Canyelles, M., Tondo, M., Cedo, L., Farras, M., Escola-Gil, J. C., and Blanco-Vaca, F. (2018). Trimethylamine N-oxide: A link among diet, gut microbiota, gene regulation of liver and intestine cholesterol homeostasis and hdl function. Int. J. Mol. Sci. 19 (10), 3228. doi:10.3390/ijms19103228
Chang, P. V., Hao, L., Offermanns, S., and Medzhitov, R. (2014). The microbial metabolite butyrate regulates intestinal macrophage function via histone deacetylase inhibition. Proc. Natl. Acad. Sci. U. S. A. 111 (6), 2247–2252. doi:10.1073/pnas.1322269111
Chen, M. (2021). Exploration on the intervention effect of XieXinTang on atherosclerosis based on gut microbiota and bile acid metabolism. [dissertation/master’s thesis]. Wuhan: Hubei University Of Traditional Chinese Medicine.
Chen, M., Hou, P., Zhou, M., Ren, Q., Wang, X., Huang, L., et al. (2020). Resveratrol attenuates high-fat diet-induced non-alcoholic steatohepatitis by maintaining gut barrier integrity and inhibiting gut inflammation through regulation of the endocannabinoid system. Clin. Nutr. 39 (4), 1264–1275. doi:10.1016/j.clnu.2019.05.020
Chen, M. L., Yi, L., Zhang, Y., Zhou, X., Ran, L., Yang, J., et al. (2016). Resveratrol attenuates trimethylamine-N-oxide (TMAO)-Induced atherosclerosis by regulating TMAO synthesis and bile acid metabolism via remodeling of the gut microbiota. mBio 7 (2), e02210–e02215. doi:10.1128/mBio.02210-15
Chen, M. T. (2019). The role of "gut microbiota-endocannabinoid system" inthe prevention and therpay of non-alcoholic fatty liver disease by resveratrol supplementation. [dissertation/master’s thesis]. Chongqing: Army Medical University.
Chen, Y. H., Chiu, C. C., Hung, S. W., Huang, W. C., Lee, Y. P., Liu, J. Y., et al. (2019b). Gnotobiotic mice inoculated with Firmicutes, but not Bacteroidetes, deteriorate nonalcoholic fatty liver disease severity by modulating hepatic lipid metabolism. Nutr. Res. 69, 20–29. doi:10.1016/j.nutres.2019.07.001
Chen, Y., Weng, Z., Liu, Q., Shao, W., Guo, W., Chen, C., et al. (2019a). FMO3 and its metabolite TMAO contribute to the formation of gallstones. Biochim. Biophys. Acta Mol. Basis Dis. 1865 (10), 2576–2585. doi:10.1016/j.bbadis.2019.06.016
Cui, H. Y., Xu, L., and Liu, Y. (2022). Efficacy of inulin combined with conventional therapy in the treatment of non-alcoholic fatty liver disease and the effect on intestinal flora. Mod. Pract. Med. 34 (01), 74–76.
Dai, Y. N., and Fu, Z. F. (2019). Research progress of effect of traditional Chinese medicine in preventing lipid metabolism disorder diseases by regulating gut microbiota. World Sci. Technology/Modernization Traditional Chin. Med. Materia Medica 21 (06), 1118–1126.
Damms-Machado, A., Mitra, S., Schollenberger, A. E., Kramer, K. M., Meile, T., Konigsrainer, A., et al. (2015). Effects of surgical and dietary weight loss therapy for obesity on gut microbiota composition and nutrient absorption. Biomed. Res. Int. 2015, 806248. doi:10.1155/2015/806248
Ding, K., Le, J. W., Fang, M. S., Zhou, X. J., and Wang, H. (2019a). Study on regulating effect of liqi huatan Quyu decoction on hyperlipidemia hamsters based on intestinal microecology. Chin. J. Mod. Appl. Pharm. 36 (18), 2249–2255. doi:10.13748/j.cnki.issn1007-7693.2019.18.003
Ding, Y., Song, Z., Li, H., Chang, L., Pan, T., Gu, X., et al. (2019b). Honokiol ameliorates high-fat-diet-induced obesity of different sexes of mice by modulating the composition of the gut microbiota. Front. Immunol. 10, 2800. doi:10.3389/fimmu.2019.02800
Dong, W., Mao, Y., Xiang, Z., Zhu, J., Wang, H., Wang, A., et al. (2022). Traditional Chinese medicine formula jian Pi Tiao Gan Yin reduces obesity in mice by modulating the gut microbiota and fecal metabolism. Evid. Based Complement. Altern. Med. 2022, 9727889. doi:10.1155/2022/9727889
Du, L., Wang, Q., Ji, S., Sun, Y., Huang, W., Zhang, Y., et al. (2022). Metabolomic and microbial remodeling by Shanmei Capsule improves hyperlipidemia in high fat food-induced mice. Front. Cell. Infect. Microbiol. 12, 729940. doi:10.3389/fcimb.2022.729940
Du, R. X. (2022). Active lipid management for primary prevention of cardiovascular disease. Chronic Pathematology J. 23 (10), 1441–1444. doi:10.16440/j.Cnki.1674-8166.2022.10.01
Duparc, T., Plovier, H., Marrachelli, V. G., Van Hul, M., Essaghir, A., Stahlman, M., et al. (2017). Hepatocyte MyD88 affects bile acids, gut microbiota and metabolome contributing to regulate glucose and lipid metabolism. Gut 66 (4), 620–632. doi:10.1136/gutjnl-2015-310904
Duttaroy, A. K. (2021). Role of gut microbiota and their metabolites on atherosclerosis, hypertension and human blood platelet function: A review. Nutrients 13 (1), 144. doi:10.3390/nu13010144
Eslick, S., Williams, E. J., Berthon, B. S., Wright, T., Karihaloo, C., Gately, M., et al. (2022). Weight loss and short-chain fatty acids reduce systemic inflammation in monocytes and adipose tissue macrophages from obese subjects. Nutrients 14 (4), 765. doi:10.3390/nu14040765
Everard, A., Belzer, C., Geurts, L., Ouwerkerk, J. P., Druart, C., Bindels, L. B., et al. (2013). Cross-talk between Akkermansia muciniphila and intestinal epithelium controls diet-induced obesity. Proc. Natl. Acad. Sci. U. S. A. 110 (22), 9066–9071. doi:10.1073/pnas.1219451110
Everard, A., Geurts, L., Caesar, R., Van Hul, M., Matamoros, S., Duparc, T., et al. (2014). Intestinal epithelial MyD88 is a sensor switching host metabolism towards obesity according to nutritional status. Nat. Commun. 5, 5648. doi:10.1038/ncomms6648
Falcinelli, S., Rodiles, A., Hatef, A., Picchietti, S., Cossignani, L., Merrifield, D. L., et al. (2018). Influence of probiotics administration on gut microbiota core: A review on the effects on appetite control, glucose, and lipid MetabolismProceedings from the 9th probiotics, prebiotics and new foods, nutraceuticals and botanicals for nutrition and human and microbiota health meeting. J Clin Gastroenterolheld Rome, Italy Sept. 5210 to 12 (1), S50–S56. doi:10.1097/MCG.0000000000001064
Feng, J., Liu, Y., Chen, J., Bai, Y., He, J., Cao, H., et al. (2022). Marine Chitooligosaccharide alters intestinal flora structure and regulates hepatic inflammatory response to influence nonalcoholic fatty liver disease. Mar. Drugs 20 (6), 383. doi:10.3390/md20060383
Fu, Y., Mei, S., Liu, D. Y., and Wu, J. S. (2013). Procyanidin regulates lipid metabolism through gut microbiota. Chin. J. Biol. 26 (02), 225–229. doi:10.13200/j.cjb.2013.02.86.fuy.024
Fushimi, T., Suruga, K., Oshima, Y., Fukiharu, M., Tsukamoto, Y., and Goda, T. (2006). Dietary acetic acid reduces serum cholesterol and triacylglycerols in rats fed a cholesterol-rich diet. Br. J. Nutr. 95 (5), 916–924. doi:10.1079/bjn20061740
Gao, L. L., Ma, J. M., Fan, Y. N., Zhang, Y. N., Ge, R., Tao, X. J., et al. (2021). Lycium barbarum polysaccharide combined with aerobic exercise ameliorated nonalcoholic fatty liver disease through restoring gut microbiota, intestinal barrier and inhibiting hepatic inflammation. Int. J. Biol. Macromol. 183, 1379–1392. doi:10.1016/j.ijbiomac.2021.05.066
Geng, J., Yang, C., Wang, B., Zhang, X., Hu, T., Gu, Y., et al. (2018). Trimethylamine N-oxide promotes atherosclerosis via CD36-dependent MAPK/JNK pathway. Biomed. Pharmacother. 97, 941–947. doi:10.1016/j.biopha.2017.11.016
Gillard, J., Clerbaux, L. A., Nachit, M., Sempoux, C., Staels, B., Bindels, L. B., et al. (2022). Bile acids contribute to the development of non-alcoholic steatohepatitis in mice. JHEP Rep. 4 (1), 100387. doi:10.1016/j.jhepr.2021.100387
Guo, H. H., Shen, H. R., Zhang, H. J., Wang, L. L., Guo, Y. X., and Jiang, J. D. (2022). Dengzhan Shengmai inhibits nonalcoholic fatty liver disease via regulating intestinal microenvironment. Acta Pharm. Sin., 1–21. doi:10.16438/j.0513-4870.2022-0908
Haeusler, R. A., Camastra, S., Nannipieri, M., Astiarraga, B., Castro-Perez, J., Xie, D., et al. (2016). Increased bile acid synthesis and impaired bile acid transport in human obesity. J. Clin. Endocrinol. Metab. 101 (5), 1935–1944. doi:10.1210/jc.2015-2583
Han, R., Qiu, H., Zhong, J., Zheng, N., Li, B., Hong, Y., et al. (2021). Si Miao Formula attenuates non-alcoholic fatty liver disease by modulating hepatic lipid metabolism and gut microbiota. Phytomedicine 85, 153544. doi:10.1016/j.phymed.2021.153544
He, H. (2021). Paeonol regulates bile acid metabolism and exerts anti-atherosclerotic effect based on intestinal flora. [dissertation/master’s thesis]. Hefei: Anhui University of Chinese Medicine.
He, K., Hu, Y., Ma, H., Zou, Z., Xiao, Y., Yang, Y., et al. (2016). Rhizoma Coptidis alkaloids alleviate hyperlipidemia in B6 mice by modulating gut microbiota and bile acid pathways. Biochim. Biophys. Acta 1862 (9), 1696–1709. doi:10.1016/j.bbadis.2016.06.006
Henning, S. M., Yang, J., Hsu, M., Lee, R. P., Grojean, E. M., Ly, A., et al. (2018). Decaffeinated green and black tea polyphenols decrease weight gain and alter microbiome populations and function in diet-induced obese mice. Eur. J. Nutr. 57 (8), 2759–2769. doi:10.1007/s00394-017-1542-8
Hong, L. (2021). Study on the mechanism of Shenlingbaizhu powder in preventing and treating nonalcoholic fatty liver based on intestinal microflora and short-chain fatty acids. [dissertation/master’s thesis]. Kunming: Yunnan University of Chinese Medicine.
Hu, M., Zhang, L., Ruan, Z., Han, P., and Yu, Y. (2021). The regulatory effects of Citrus peel powder on liver metabolites and gut flora in mice with non-alcoholic fatty liver disease (NAFLD). Foods 10 (12), 3022. doi:10.3390/foods10123022
Huang, F., Zheng, X., Ma, X., Jiang, R., Zhou, W., Zhou, S., et al. (2019). Theabrownin from Pu-erh tea attenuates hypercholesterolemia via modulation of gut microbiota and bile acid metabolism. Nat. Commun. 10 (1), 4971. doi:10.1038/s41467-019-12896-x
Huang, J. L. (2021a). Effect of fucoidan on prevention of diet-induced obesity-related hyperlipidemia and modulation gut microbiota in ICR mice. [dissertation/master’s thesis]. Dalian: Dalian Medical University.
Huang, J. Q., Lin, B., Xu, Q. X., Guang, X. F., Qian, L., and Wang, L. P. (2018). Effect of Gynostemma pentaphyllum tea on lipid metabolism and gut microbiota in hyperlipidemic rats. J. Chin. Inst. Food Sci. Technol. 18 (06), 27–32. doi:10.16429/j.1009-7848.2018.06.004
Huang, Q. (2021b). Jianpi Huazhuo Tiaozhi granule alleviates gut microbiota dysbiosis-induced atherosclerosis by regulating the enterohepatic FXR/FGF15 Axis. [dissertation/master’s thesis]. Nanchang: Jiangxi University of Chinese Medicine.
Human Microbiome Project, C. (2012). Structure, function and diversity of the healthy human microbiome. Nature 486 (7402), 207–214. doi:10.1038/nature11234
Janeiro, M. H., Ramirez, M. J., Milagro, F. I., Martinez, J. A., and Solas, M. (2018). Implication of trimethylamine N-oxide (TMAO) in disease: Potential biomarker or new therapeutic target. Nutrients 10 (10), 1398. doi:10.3390/nu10101398
Ji, W., Jiang, T., Sun, Z., Teng, F., Ma, C., Huang, S., et al. (2020). The enhanced pharmacological effects of modified traditional Chinese medicine in attenuation of atherosclerosis is driven by modulation of gut microbiota. Front. Pharmacol. 11, 546589. doi:10.3389/fphar.2020.546589
Jia, A. L., Zhang, Y. H., Diao, Y. Y., Shi, Y. W., Jiang, M. C., Li, J. G., et al. (2022). Effect of Ethyl Acetate Extract of Acanthopanacis Senticosi Radix et Rhizoma seu Caulis on Intestinal Flora in ApoE-/- Mice with Atherosclerosis. Chin. J. Exp. Traditional Med. Formulae 28 (05), 108–115. doi:10.13422/j.cnki.syfjx.20220152
Jia, W., Xie, G., and Jia, W. (2018). Bile acid-microbiota crosstalk in gastrointestinal inflammation and carcinogenesis. Nat. Rev. Gastroenterol. Hepatol. 15 (2), 111–128. doi:10.1038/nrgastro.2017.119
Jia, X., Xu, W., Zhang, L., Li, X., Wang, R., and Wu, S. (2021). Impact of gut microbiota and microbiota-related metabolites on hyperlipidemia. Front. Cell. Infect. Microbiol. 11, 634780. doi:10.3389/fcimb.2021.634780
Jiang, N., Xie, X., Zhang, Y. Y., Chen, C., Zeng, H., and Ma, Y. L. (2021). Study on huanglian Jiedu decoction regulating intestinal flora against non-alcoholic fatty liver disease and atherosclerosis in apoE-/- mice. J. Basic Chin. Med. 27 (06), 927–931+994. doi:10.19945/j.cnki.issn.1006-3250.2021.06.013
Jiang, Y. (2019). Effects of paeonol on intestinal flora and inflammatory factors in rats with non-alcoholic fatty liver. [dissertation/master’s thesis]. Hefei: Anhui University of Chinese Medicine.
Jin, J., Wang, J., Cheng, R., Ren, Y., Miao, Z., Luo, Y., et al. (2022). Orlistat and ezetimibe could differently alleviate the high-fat diet-induced obesity phenotype by modulating the gut microbiota. Front. Microbiol. 13, 908327. doi:10.3389/fmicb.2022.908327
Jonsson, A. L., and Backhed, F. (2017). Role of gut microbiota in atherosclerosis. Nat. Rev. Cardiol. 14 (2), 79–87. doi:10.1038/nrcardio.2016.183
Ju, M., Liu, Y., Li, M., Cheng, M., Zhang, Y., Deng, G., et al. (2019). Baicalin improves intestinal microecology and abnormal metabolism induced by high-fat diet. Eur. J. Pharmacol. 857, 172457. doi:10.1016/j.ejphar.2019.172457
Karr, S. (2017). Epidemiology and management of hyperlipidemia. Am. J. Manag. Care 23 (9), S139–S148.
Kasai, C., Sugimoto, K., Moritani, I., Tanaka, J., Oya, Y., Inoue, H., et al. (2015). Comparison of the gut microbiota composition between obese and non-obese individuals in a Japanese population, as analyzed by terminal restriction fragment length polymorphism and next-generation sequencing. BMC Gastroenterol. 15, 100. doi:10.1186/s12876-015-0330-2
Katsiki, N., Mikhailidis, D. P., and Mantzoros, C. S. (2016). Non-alcoholic fatty liver disease and dyslipidemia: An update. Metabolism 65 (8), 1109–1123. doi:10.1016/j.metabol.2016.05.003
Koeth, R. A., Lam-Galvez, B. R., Kirsop, J., Wang, Z., Levison, B. S., Gu, X., et al. (2019). l-Carnitine in omnivorous diets induces an atherogenic gut microbial pathway in humans. J. Clin. Investig. 129 (1), 373–387. doi:10.1172/JCI94601
Koeth, R. A., Wang, Z., Levison, B. S., Buffa, J. A., Org, E., Sheehy, B. T., et al. (2013). Intestinal microbiota metabolism of L-carnitine, a nutrient in red meat, promotes atherosclerosis. Nat. Med. 19 (5), 576–585. doi:10.1038/nm.3145
Koren, M. J., Sabatine, M. S., Giugliano, R. P., Langslet, G., Wiviott, S. D., Ruzza, A., et al. (2019). Long-term efficacy and safety of evolocumab in patients with hypercholesterolemia. J. Am. Coll. Cardiol. 74 (17), 2132–2146. doi:10.1016/j.jacc.2019.08.1024
Kou, S. J., Xiue, J. D., Yang, S. Z., and Zhang, G. J. (2022). Clinical effect of Yunpi Huazhuo granule in the treatment of nonalcoholic fatty liver disease and its influence on the intestinal flora of patients. Clin. Res. Pract. 7 (10), 115–117. doi:10.19347/j.cnki.2096-1413.202210032
Larkin, T. A., Astheimer, L. B., and Price, W. E. (2009). Dietary combination of soy with a probiotic or prebiotic food significantly reduces total and LDL cholesterol in mildly hypercholesterolaemic subjects. Eur. J. Clin. Nutr. 63 (2), 238–245. doi:10.1038/sj.ejcn.1602910
Lei, Y. L. (2020). Study on the syndrome characteristics of atherosclerosis in apo E-/-Mice induced by high-fat diet and the pharmacological mechanism of Xiexin decoction. [dissertation/master’s thesis]. Wuhan: Hubei University Of Traditional Chinese Medicine.
Li, C., Yu, S., Li, X., Cao, Y., Li, M., Ji, G., et al. (2022a). Medicinal formula huazhi-rougan attenuates non-alcoholic steatohepatitis through enhancing fecal bile acid excretion in mice. Front. Pharmacol. 13, 833414. doi:10.3389/fphar.2022.833414
Li, D. H. (2015). Protective study of berberine hydrochloride on intestinal mucosal barrier in NAFLD rats. [dissertation/master’s thesis]. Shijiazhuang: Hebei Medical University.
Li, L. S., Xu, X. M., Lu, X. H., Zhang, Y. M., Lin, W. J., and Xu, R. Q. (2019a). Effects of Alisma orientale on the diversity of gut microbiota in rats fed on high-fat and high-sugar diet. Chin. J. Microecol. 31 (04), 396–401. doi:10.13381/j.cnki.cjm.201904005
Li, N., Wu, Y. Y., Duan, J. L., Zheng, X. T., and Yao, K. W. (2021a). Explore effect of Jieyu qutan Huazhuo prescription on gut-liver Axis of rats with high-fat diet based on 16S rDNA sequencing. Chin. J. Exp. Traditional Med. Formulae 27 (09), 77–85. doi:10.13422/j.cnki.syfjx.20210327
Li, N., Wu, Y. Y., Duan, J. L., Zheng, X. T., and Yao, K. W. (2022b). Study on the intervention of Jieyu Qutan Huazhuo Prescription on diversity of intestinal flora in rats with high fat diet. Tianjin J. Traditional Chin. Med. 39 (03), 375–379.
Li, P., Hu, J., Zhao, H., Feng, J., and Chai, B. (2022c). Multi-omics reveals inhibitory effect of Baicalein on non-alcoholic fatty liver disease in mice. Front. Pharmacol. 13, 925349. doi:10.3389/fphar.2022.925349
Li, Q., Li, M., Li, F., Zhou, W., Dang, Y., Zhang, L., et al. (2020). Qiang-Gan formula extract improves non-alcoholic steatohepatitis via regulating bile acid metabolism and gut microbiota in mice. J. Ethnopharmacol. 258, 112896. doi:10.1016/j.jep.2020.112896
Li, T., Teng, H., An, F., Huang, Q., Chen, L., and Song, H. (2019b). The beneficial effects of purple yam (Dioscorea alata L.) resistant starch on hyperlipidemia in high-fat-fed hamsters. Food Funct. 10 (5), 2642–2650. doi:10.1039/c8fo02502a
Li, W. J. (2020). Effects of dietary EGCG on ameliorating intestinal immune function and relieving non-alcoholic fatty liver. [dissertation/master’s thesis]. Hefei: Anhui Agricultural University.
Li, W., Yang, H., Zhao, Q., Wang, X., Zhang, J., and Zhao, X. (2019c). Polyphenol-rich loquat fruit extract prevents fructose-induced nonalcoholic fatty liver disease by modulating glycometabolism, lipometabolism, oxidative stress, inflammation, intestinal barrier, and gut microbiota in mice. J. Agric. Food Chem. 67 (27), 7726–7737. doi:10.1021/acs.jafc.9b02523
Li, X., Su, C., Jiang, Z., Yang, Y., Zhang, Y., Yang, M., et al. (2021b). Berberine attenuates choline-induced atherosclerosis by inhibiting trimethylamine and trimethylamine-N-oxide production via manipulating the gut microbiome. NPJ Biofilms Microbiomes 7 (1), 36. doi:10.1038/s41522-021-00205-8
Li, X., Zhao, W., Xiao, M., Yu, L., Chen, Q., Hu, X., et al. (2022d). Penthorum chinense Pursh. extract attenuates non-alcholic fatty liver disease by regulating gut microbiota and bile acid metabolism in mice. J. Ethnopharmacol. 294, 115333. doi:10.1016/j.jep.2022.115333
Li, Y., Ji, X., Wu, H., Li, X., Zhang, H., and Tang, D. (2021c). Mechanisms of traditional Chinese medicine in modulating gut microbiota metabolites-mediated lipid metabolism. J. Ethnopharmacol. 278, 114207. doi:10.1016/j.jep.2021.114207
Li, Y., Liu, T., Yan, C., Xie, R., Guo, Z., Wang, S., et al. (2018a). Diammonium glycyrrhizinate protects against nonalcoholic fatty liver disease in mice through modulation of gut microbiota and restoration of intestinal barrier. Mol. Pharm. 15 (9), 3860–3870. doi:10.1021/acs.molpharmaceut.8b00347
Li, Z. H., Zhang, N., Wang, Y., Pang, M., and Liu, S. M. (2019d). Mechanism of Yinchenhao Decoction in treating non-alcoholic fatty liver based on 16S rRNA technique and metabonomics. CJTCMP 34 (05), 1908–1913.
Li, Z., Yi, C. X., Katiraei, S., Kooijman, S., Zhou, E., Chung, C. K., et al. (2018b). Butyrate reduces appetite and activates Brown adipose tissue via the gut-brain neural circuit. Gut 67 (7), 1269–1279. doi:10.1136/gutjnl-2017-314050
Liang, X., Zhang, Z., Lv, Y., Tong, L., Liu, T., Yi, H., et al. (2020). Reduction of intestinal trimethylamine by probiotics ameliorated lipid metabolic disorders associated with atherosclerosis. Nutrition 79-80, 110941. doi:10.1016/j.nut.2020.110941
Lin, L., Liu, Z. J., Lei, Z. Y., Zeng, W. Y., Cheng, F. J., Lin, Q. N., et al. (2020). Study on the effect of tea seed saponins on intestinal microflora in rats with hyperlipidemia. Nat. Prod. es Dev. 32 (02), 173–181. doi:10.16333/j.1001-6880.2020.2.001
Ling, Q. H., Wu, D., Le, M., and Chen, J. J. (2022). Study on mechanism of Cangju Qinggan Formula in treatment of non⁃alcoholic fatty liver based on“liver⁃bile acid⁃intestinal microecology”. Acad. J. Shanghai Univ. Traditional Chin. Med. 36 (S1), 143–148. doi:10.16306/j.1008-861x.2022.S1.034
Liu, F. Y., Wen, J., Hou, J., Zhang, S. Q., Sun, C. B., Zhou, L. C., et al. (2021a). Gastrodia remodels intestinal microflora to suppress inflammation in mice with early atherosclerosis. Int. Immunopharmacol. 96, 107758. doi:10.1016/j.intimp.2021.107758
Liu, H., Xu, J., Li, H., Zhang, L., and Xu, P. (2021b). Network pharmacology-based investigation to explore the effect and mechanism of Erchen decoction against the nonalcoholic fatty liver disease. Anat. Rec. Hob. 304 (11), 2605–2619. doi:10.1002/ar.24770
Liu, M. L. (2019). Research of the effect and mechanism of Gegen Qinlian Decoction onnon-alcoholic steatohepatitis via intestinal liver axis. [dissertation/master’s thesis]. Shanghai: Shanghai University of Traditional Chinese Medicine.
Liu, S. Y. (2016). Study of mechanism of baicalin on metabolic inf lammation in the respect of intestinal flora and permeability. [dissertation/master’s thesis]. Guangzhou: Guangzhou University of Chinese Medicine.
Liu, X. (2022). The intervention of luteolin in non-alcoholic fatty liver disease and the preparation of colon-targeted nanoparticles. [dissertation/master’s thesis]. Qingdao: Qingdao University of Science and Technology.
Liu, Y. R. (2021). Inhibition mechanisms of paeonol on the inflammation of vascular endothelial cells in atherosclerosis by regulating gut microbiota and its metabolife α-hydroxyisobytyric acid. [dissertation/master's thesis]. Hefei: Anhui University of Chinese Medicine.
Lu, Y., Fan, C., Li, P., Lu, Y., Chang, X., and Qi, K. (2016). Short chain fatty acids prevent high-fat-diet-induced obesity in mice by regulating G protein-coupled receptors and gut microbiota. Sci. Rep. 6, 37589. doi:10.1038/srep37589
Lu, Y., Wan, H., Wu, Y., Yang, J., Yu, L., He, Y., et al. (2022). Naoxintong Capsule alternates gut microbiota and prevents hyperlipidemia in high-fat-diet fed rats. Front. Pharmacol. 13, 843409. doi:10.3389/fphar.2022.843409
Luo, H., Wu, H., Wang, L., Xiao, S., Lu, Y., Liu, C., et al. (2021). Hepatoprotective effects of Cassiae Semen on mice with non-alcoholic fatty liver disease based on gut microbiota. Commun. Biol. 4 (1), 1357. doi:10.1038/s42003-021-02883-8
Lv, Z., Shan, X., Tu, Q., Wang, J., Chen, J., and Yang, Y. (2021). Ginkgolide B treatment regulated intestinal flora to improve high-fat diet induced atherosclerosis in ApoE(-/-) mice. Biomed. Pharmacother. 134, 111100. doi:10.1016/j.biopha.2020.111100
Ma, G., Pan, B., Chen, Y., Guo, C., Zhao, M., Zheng, L., et al. (2017). Trimethylamine N-oxide in atherogenesis: Impairing endothelial self-repair capacity and enhancing monocyte adhesion. Biosci. Rep. 37 (2). doi:10.1042/BSR20160244
Ma, S. R., Tong, Q., Lin, Y., Pan, L. B., Fu, J., Peng, R., et al. (2022). Berberine treats atherosclerosis via a vitamine-like effect down-regulating Choline-TMA-TMAO production pathway in gut microbiota. Signal Transduct. Target Ther. 7 (1), 207. doi:10.1038/s41392-022-01027-6
Magne, F., Gotteland, M., Gauthier, L., Zazueta, A., Pesoa, S., Navarrete, P., et al. (2020). The firmicutes/bacteroidetes ratio: A relevant marker of gut dysbiosis in obese patients? Nutrients 12 (5), 1474. doi:10.3390/nu12051474
Manco, M., Putignani, L., and Bottazzo, G. F. (2010). Gut microbiota, lipopolysaccharides, and innate immunity in the pathogenesis of obesity and cardiovascular risk. Endocr. Rev. 31 (6), 817–844. doi:10.1210/er.2009-0030
Maruvada, P., Leone, V., Kaplan, L. M., and Chang, E. B. (2017). The human microbiome and obesity: Moving beyond associations. Cell. Host Microbe 22 (5), 589–599. doi:10.1016/j.chom.2017.10.005
Matey-Hernandez, M. L., Williams, F. M. K., Potter, T., Valdes, A. M., Spector, T. D., and Menni, C. (2018). Genetic and microbiome influence on lipid metabolism and dyslipidemia. Physiol. Genomics 50 (2), 117–126. doi:10.1152/physiolgenomics.00053.2017
Miao, J., Ling, A. V., Manthena, P. V., Gearing, M. E., Graham, M. J., Crooke, R. M., et al. (2015). Flavin-containing monooxygenase 3 as a potential player in diabetes-associated atherosclerosis. Nat. Commun. 6, 6498. doi:10.1038/ncomms7498
Miao, L., Peng, Q., Sun, M. Q., Zhang, Y. H., Zhang, Y., Ren, C. Y., et al. (2022). Regulatory effect of Quyu huatan Tongmai prescription on intestinal microflora in golden hamster with hyperlipidemia. Chin. J. Exp. Traditional Med. Formulae 28 (01), 109–120. doi:10.13422/j.cnki.syfjx.20212403
Morelli, M. B., Wang, X., and Santulli, G. (2019). Functional role of gut microbiota and PCSK9 in the pathogenesis of diabetes mellitus and cardiovascular disease. Atherosclerosis 289, 176–178. doi:10.1016/j.atherosclerosis.2019.07.023
Mu, F., Zhao, J. J., Ji, Z. M., Chen, S. Q., and Zhao, H. H. (2020). 16S rDNA-based high-throughput sequencing techniques for mining of core flora affecting hyperlipidemia. Chin. Remedies Clin. 20 (05), 701–704.
Mu, H. N., Zhou, Q., Yang, R. Y., Tang, W. Q., Li, H. X., Wang, S. M., et al. (2021). Caffeic acid prevents non-alcoholic fatty liver disease induced by a high-fat diet through gut microbiota modulation in mice. Food Res. Int. 143, 110240. doi:10.1016/j.foodres.2021.110240
Nakano, H., Wu, S., Sakao, K., Hara, T., He, J., Garcia, S., et al. (2020). Bilberry anthocyanins ameliorate NAFLD by improving dyslipidemia and gut microbiome dysbiosis. Nutrients 12 (11), 3252. doi:10.3390/nu12113252
Naraoka, Y., Yamaguchi, T., Hu, A., Akimoto, K., and Kobayashi, H. (2018). Short chain fatty acids upregulate adipokine production in type 2 diabetes-derived human adipocytes. Acta Endocrinol. (Buchar) 14 (3), 287–293. doi:10.4183/aeb.2018.287
Nie, C., He, T., Zhang, W., Zhang, G., and Ma, X. (2018). Branched chain amino acids: Beyond nutrition metabolism. Int. J. Mol. Sci. 19 (4), 954. doi:10.3390/ijms19040954
Nie, J., Zhang, L., Zhao, G., and Du, X. (2019). Quercetin reduces atherosclerotic lesions by altering the gut microbiota and reducing atherogenic lipid metabolites. J. Appl. Microbiol. 127 (6), 1824–1834. doi:10.1111/jam.14441
Pan, Z., Zhang, W. F., Lv, J. L., Wei, G., Yu, L. L., Huang, L. P., et al. (2020). Clinical observation of Zhibitai Capsule on nonalcoholic fatty liver disease. Chin. Archives Traditional Chin. Med. 38 (11), 78–80. doi:10.13193/j.issn.1673-7717.2020.11.021
Pang, H. T. (2021). Effect and mechanism of Tianhuang Formula on improving NAFLD by gut microbiota. [dissertation/master’s thesis]. Guangzhou: Guangdong Pharmaceutical University.
Park, S., Cho, S. M., Jin, B. R., Yang, H. J., and Yi, Q. J. (2019). Mixture of blackberry leaf and fruit extracts alleviates non-alcoholic steatosis, enhances intestinal integrity, and increases Lactobacillus and Akkermansia in rats. Exp. Biol. Med. (Maywood) 244 (18), 1629–1641. doi:10.1177/1535370219889319
Perez-Monter, C., Alvarez-Arce, A., Nuno-Lambarri, N., Escalona-Nandez, I., Juarez-Hernandez, E., Chavez-Tapia, N. C., et al. (2022). Inulin improves diet-induced hepatic steatosis and increases intestinal Akkermansia genus level. Int. J. Mol. Sci. 23 (2), 991. doi:10.3390/ijms23020991
Perry, R. J., Peng, L., Barry, N. A., Cline, G. W., Zhang, D., Cardone, R. L., et al. (2016). Acetate mediates a microbiome-brain-beta-cell axis to promote metabolic syndrome. Nature 534 (7606), 213–217. doi:10.1038/nature18309
Petersen, C., Bell, R., Klag, K. A., Lee, S. H., Soto, R., Ghazaryan, A., et al. (2019). T cell-mediated regulation of the microbiota protects against obesity. Science 365 (6451), eaat9351. doi:10.1126/science.aat9351
Plociennikowska, A., Hromada-Judycka, A., Borzecka, K., and Kwiatkowska, K. (2015). Co-operation of TLR4 and raft proteins in LPS-induced pro-inflammatory signaling. Cell. Mol. Life Sci. 72 (3), 557–581. doi:10.1007/s00018-014-1762-5
Porras, D., Nistal, E., Martinez-Florez, S., Pisonero-Vaquero, S., Olcoz, J. L., Jover, R., et al. (2017). Protective effect of quercetin on high-fat diet-induced non-alcoholic fatty liver disease in mice is mediated by modulating intestinal microbiota imbalance and related gut-liver axis activation. Free Radic. Biol. Med. 102, 188–202. doi:10.1016/j.freeradbiomed.2016.11.037
Qi, Y., Liu, W., Yan, X., Zhang, C., Zhang, C., Liu, L., et al. (2022). Tongxinluo may alleviate inflammation and improve the stability of atherosclerotic plaques by changing the intestinal flora. Front. Pharmacol. 13, 805266. doi:10.3389/fphar.2022.805266
Qiu, B. D., Zang, Y., Wang, S., Liu, N., Liu, L., and Mei, Q. B. (2017). Biejia Jian Wan improves intestinal flora imbalance and attenuates nonalcoholic fatty liver disease in ats. Chin. J. Exp. Traditional Med. Formulae 23 (04), 145–151. doi:10.13422/j.cnki.syfjx.2017040145
Randrianarisoa, E., Lehn-Stefan, A., Wang, X., Hoene, M., Peter, A., Heinzmann, S. S., et al. (2016). Relationship of serum trimethylamine N-oxide (TMAO) levels with early atherosclerosis in humans. Sci. Rep. 6, 26745. doi:10.1038/srep26745
Rastelli, M., Knauf, C., and Cani, P. D. (2018). Gut microbes and health: A focus on the mechanisms linking microbes, obesity, and related disorders. Obes. (Silver Spring) 26 (5), 792–800. doi:10.1002/oby.22175
Ridaura, V. K., Faith, J. J., Rey, F. E., Cheng, J., Duncan, A. E., Kau, A. L., et al. (2013). Gut microbiota from twins discordant for obesity modulate metabolism in mice. Science 341 (6150), 1241214. doi:10.1126/science.1241214
Robbins, G. R., Wen, H., and Ting, J. P. (2014). Inflammasomes and metabolic disorders: Old genes in modern diseases. Mol. Cell. 54 (2), 297–308. doi:10.1016/j.molcel.2014.03.029
Ross, R. (1999). Atherosclerosis-an inflammatory disease. N. Engl. J. Med. 340 (2), 115–126. doi:10.1056/NEJM199901143400207
Salazar, N., Neyrinck, A. M., Bindels, L. B., Druart, C., Ruas-Madiedo, P., Cani, P. D., et al. (2019). Functional effects of EPS-producing Bifidobacterium administration on energy metabolic alterations of diet-induced obese mice. Front. Microbiol. 10, 1809. doi:10.3389/fmicb.2019.01809
Sanchez, H. N., Moroney, J. B., Gan, H., Shen, T., Im, J. L., Li, T., et al. (2020). B cell-intrinsic epigenetic modulation of antibody responses by dietary fiber-derived short-chain fatty acids. Nat. Commun. 11 (1), 60. doi:10.1038/s41467-019-13603-6
Sang, T., Guo, C., Guo, D., Wu, J., Wang, Y., Wang, Y., et al. (2021). Suppression of obesity and inflammation by polysaccharide from sporoderm-broken spore of Ganoderma lucidum via gut microbiota regulation. Carbohydr. Polym. 256, 117594. doi:10.1016/j.carbpol.2020.117594
Schoeler, M., and Caesar, R. (2019). Dietary lipids, gut microbiota and lipid metabolism. Rev. Endocr. Metab. Disord. 20 (4), 461–472. doi:10.1007/s11154-019-09512-0
Schulz, M. D., Atay, C., Heringer, J., Romrig, F. K., Schwitalla, S., Aydin, B., et al. (2014). High-fat-diet-mediated dysbiosis promotes intestinal carcinogenesis independently of obesity. Nature 514 (7523), 508–512. doi:10.1038/nature13398
Shen, S. H., Zhong, T. Y., Peng, C., Fang, J., and Lv, B. (2020). Structural modulation of gut microbiota during alleviation of non-alcoholic fatty liver disease with Gynostemma pentaphyllum in rats. BMC Complement. Med. Ther. 20 (1), 34. doi:10.1186/s12906-020-2835-7
Shin, N. R., Lee, J. C., Lee, H. Y., Kim, M. S., Whon, T. W., Lee, M. S., et al. (2014). An increase in the Akkermansia spp. population induced by metformin treatment improves glucose homeostasis in diet-induced obese mice. Gut 63 (5), 727–735. doi:10.1136/gutjnl-2012-303839
Shou, D. W. (2021). Study on the mechanism of resistant starch interveningnonalcoholic fatty liver disease via gut microbiota. [dissertation/master's thesis]. Guangzhou: South China University of Technology.
Soares, E., Soares, A. C., Trindade, P. L., Monteiro, E. B., Martins, F. F., Forgie, A. J., et al. (2021). Jaboticaba (Myrciaria jaboticaba) powder consumption improves the metabolic profile and regulates gut microbiome composition in high-fat diet-fed mice. Biomed. Pharmacother. 144, 112314. doi:10.1016/j.biopha.2021.112314
Sonnenburg, J. L., and Backhed, F. (2016). Diet-microbiota interactions as moderators of human metabolism. Nature 535 (7610), 56–64. doi:10.1038/nature18846
Sui, G. Y., Zhang, N., Song, N., Jia, L. Q., and Yang, G. L. (2021a). Effect of huayu qutan formula on gut microbiota-driven TMA/FMO3/TMAO pathway in ApoE-/- atherosclerosis model mice. J. Traditional Chin. Med. 62 (08), 700–706. doi:10.13288/j.11-2166/r.2021.08.013
Sui, G. Y., Zhang, N., Song, N., Lv, M. J., Wang, Y., Jia, L. Q., et al. (2021b). Study on the anti-atherosclerotic effect mechanism of Huayu Qutan Formula based on the gut microbiota-bile acid-FXR signal pathway. CJTCMP 36 (10), 5815–5820.
Sultana, A., Cochran, B. J., Tabet, F., Patel, M., Torres, L. C., Barter, P. J., et al. (2016). Inhibition of inflammatory signaling pathways in 3T3-L1 adipocytes by apolipoprotein A-I. FASEB J. 30 (6), 2324–2335. doi:10.1096/fj.201500026R
Sun, C., Wang, Z., Hu, L., Zhang, X., Chen, J., Yu, Z., et al. (2022). Targets of statins intervention in LDL-C metabolism: Gut microbiota. Front. Cardiovasc Med. 9, 972603. doi:10.3389/fcvm.2022.972603
Sun, N. N. (2020). Characteristics of oral flora in atheroscleroticpopulation and mechanism study of Eucommiaulmoides on improving atherosclerosis basedon intestinal flora. [dissertation/master's thesis]. Jinan: Shandong University of Traditional Chinese Medicine.
Sun, S. S., Wang, K., Ma, K., Bao, L., and Liu, H. W. (2019). An insoluble polysaccharide from the sclerotium of Poria cocos improves hyperglycemia, hyperlipidemia and hepatic steatosis in ob/ob mice via modulation of gut microbiota. Chin. J. Nat. Med. 17 (1), 3–14. doi:10.1016/S1875-5364(19)30003-2
Sun, W. L., Li, X. Y., Dou, H. Y., Wang, X. D., Li, J. D., Shen, L., et al. (2021). Myricetin supplementation decreases hepatic lipid synthesis and inflammation by modulating gut microbiota. Cell. Rep. 36 (9), 109641. doi:10.1016/j.celrep.2021.109641
Sun, Y. X., Huang, Y., Zeng, M., Gan, J. L., Huang, P. F., Jiang, X. J., et al. (2020). Based on “intestinal flora-inflammation” pathway to investigate effect and mechanism of Danlou Tablet on atherosclerosis of ApoE-/- mice. Chin. Traditional Herb. Drugs 51 (09), 2492–2500.
Tang, B., Xiao, X. Y., Liu, Y. J., Yao, T. Z., and Tan, Z. J. (2016). Effects of Jiangzhiligan Decoction on intestinal microbiota and enzyme Effects of Jiangzhiligan Decoction on intestinal microbiota and enzyme activities of rats with non-alcoholic fatty liver disease induced by high-fat diet. Chin. J. Appl. Environ. Biol. 22 (03), 442–445.
Tang, W. J. (2019). Modulation of the gut microbiota in rats by HuganQingzhiTablets during the treatment of high-fat diet-induced nonalcoholic fatty liver disease. [dissertation/master's thesis]. Guangzhou: Southern Medical University.
Tang, W., Yao, X., Xia, F., Yang, M., Chen, Z., Zhou, B., et al. (2018). Modulation of the gut microbiota in rats by hugan Qingzhi tablets during the treatment of high-fat-diet-induced nonalcoholic fatty liver disease. Oxid. Med. Cell. Longev. 2018, 7261619. doi:10.1155/2018/7261619
Tian, Y., Cai, J., Gui, W., Nichols, R. G., Koo, I., Zhang, J., et al. (2019). Berberine directly affects the gut microbiota to promote intestinal farnesoid X receptor activation. Drug Metab. Dispos. 47 (2), 86–93. doi:10.1124/dmd.118.083691
Tomas, J., Mulet, C., Saffarian, A., Cavin, J. B., Ducroc, R., Regnault, B., et al. (2016). High-fat diet modifies the PPAR-gamma pathway leading to disruption of microbial and physiological ecosystem in murine small intestine. Proc. Natl. Acad. Sci. U. S. A. 113 (40), E5934–E5943. doi:10.1073/pnas.1612559113
Tong, A. J., Hu, R. K., Wu, L. X., Lv, X. C., Li, X., Zhao, L. N., et al. (2020). Ganoderma polysaccharide and chitosan synergistically ameliorate lipid metabolic disorders and modulate gut microbiota composition in high fat diet-fed golden hamsters. J. Food Biochem. 44 (1), e13109. doi:10.1111/jfbc.13109
Vourakis, M., Mayer, G., and Rousseau, G. (2021). The role of gut microbiota on cholesterol metabolism in atherosclerosis. Int. J. Mol. Sci. 22 (15), 8074. doi:10.3390/ijms22158074
Wahlström, A., Sayin, S. I., Marschall, H. U., and Bäckhed, F. (2016). Intestinal crosstalk between bile acids and microbiota and its impact on host metabolism. Cell. Metab. 24 (1), 41–50. doi:10.1016/j.cmet.2016.05.005
Walker, A. W., Duncan, S. H., McWilliam Leitch, E. C., Child, M. W., and Flint, H. J. (2005). pH and peptide supply can radically alter bacterial populations and short-chain fatty acid ratios within microbial communities from the human colon. Appl. Environ. Microbiol. 71 (7), 3692–3700. doi:10.1128/AEM.71.7.3692-3700.2005
Wang, A. L. (2019). Study on the role and mechanism of intestinal flora modulation of atherosclerotic lipid metabolism by Qing Xin Jie Yu formula. [dissertation/master's thesis]. Beijing: Beijing University of Chinese Medicine.
Wang, B., Kong, Q., Li, X., Zhao, J., Zhang, H., Chen, W., et al. (2020a). A high-fat diet increases gut microbiota biodiversity and energy expenditure due to nutrient difference. Nutrients 12 (10), 3197. doi:10.3390/nu12103197
Wang, F., Zhao, C., Tian, G., Wei, X., Ma, Z., Cui, J., et al. (2020b). Naringin alleviates atherosclerosis in ApoE(-/-) mice by regulating cholesterol metabolism involved in gut microbiota remodeling. J. Agric. Food Chem. 68 (45), 12651–12660. doi:10.1021/acs.jafc.0c05800
Wang, G., Zhu, G., Chen, C., Zheng, Y., Ma, F., Zhao, J., et al. (2021a). Lactobacillus strains derived from human gut ameliorate metabolic disorders via modulation of gut microbiota composition and short-chain fatty acids metabolism. Benef. Microbes 12 (3), 267–281. doi:10.3920/BM2020.0148
Wang, H. B. (2016). Role of Trimethylamine N-oxide in Atherosclerotic progression and the modulative effects of eliminating sputum and removing stasis formulation. [dissertation/master's thesis]. Shanghai: Naval Medical University.
Wang, H., Zhang, H., Gao, Z., Zhang, Q., and Gu, C. (2022a). The mechanism of berberine alleviating metabolic disorder based on gut microbiome. Front. Cell. Infect. Microbiol. 12, 854885. doi:10.3389/fcimb.2022.854885
Wang, J., Song, N., Chen, S., Zhai, Y. R., Wang, Y., Yu, Y., et al. (2022b). Exploring the effect and mechanism of XiangSha LiuJunZi Tang on hepatic triglyceride synthesis in rats with spleen deficiency hyperlipidemia based on intestinal flora regulation of TMAO/PERK/FOXO1 pathway. Lishizhen Med. Materia Medica Res. 33 (02), 286–291.
Wang, J. Y. (2020). Study on the prevention and treatment of burdock inulin on nonalcoholic fatty liver disease. [dissertation/master's thesis]. Jinan: Shandong University.
Wang, L. J., Li, Y. W., Yang, L. B., Yan, N., Wang, H., and Jia, S. B. (2021b). Protective effects of inulin on atherosclerosis in ApoE-/- mice. Mod. Food Sci. Technol. 37 (10), 7–18. doi:10.13982/j.mfst.1673-9078.2021.10.0180
Wang, L., Zeng, B., Liu, Z., Liao, Z., Zhong, Q., Gu, L., et al. (2018). Green tea polyphenols modulate colonic microbiota diversity and lipid metabolism in high-fat diet treated HFA mice. J. Food Sci. 83 (3), 864–873. doi:10.1111/1750-3841.14058
Wang, R. R., Zhang, L. F., Chen, L. P., Wang, J. Y., Zhang, L., Xu, Y. S., et al. (2021c). Structural and functional modulation of gut microbiota by Jiangzhi granules during the amelioration of nonalcoholic fatty liver disease. Oxid. Med. Cell. Longev. 2021, 2234695. doi:10.1155/2021/2234695
Wang, T., Wu, Q., and Zhao, T. (2020c). Preventive effects of Kaempferol on high-fat diet-induced obesity complications in C57bl/6 mice. Biomed. Res. Int. 2020, 4532482. doi:10.1155/2020/4532482
Wang, X., Dong, J., Liang, W., Fang, Y., Liang, M., Xu, L., et al. (2022c). Porphyran from porphyra haitanensis alleviates obesity by reducing lipid accumulation and modulating gut microbiota homeostasis. Front. Pharmacol. 13, 942143. doi:10.3389/fphar.2022.942143
Wang, X., Liu, D., Wang, Z., Cai, C., Jiang, H., and Yu, G. (2021d). Porphyran-derived oligosaccharides alleviate NAFLD and related cecal microbiota dysbiosis in mice. FASEB J. 35 (6), e21458. doi:10.1096/fj.202000763RRR
Wang, X. X. (2022). Study of the effect and mechanisms of probiotics alleviating atherosclerosis based on choline/TMA/TMAO metabolic pathway. [dissertation/master's thesis]. Wuxi: Jiangnan University.
Wang, Y., Lv, S., Shen, T., Ye, M., Wang, D., Zhou, P., et al. (2021e). Qinghua Fang inhibits high-fat diet-induced non-alcoholic fatty liver disease by modulating gut microbiota. Ann. Palliat. Med. 10 (3), 3219–3234. doi:10.21037/apm-21-448
Wang, Y., Xu, Y., Xu, X., Wang, H., Wang, D., Yan, W., et al. (2022d). Ginkgo biloba extract ameliorates atherosclerosis via rebalancing gut flora and microbial metabolism. Phytother. Res. 36 (6), 2463–2480. doi:10.1002/ptr.7439
Wang, Z., Klipfell, E., Bennett, B. J., Koeth, R., Levison, B. S., Dugar, B., et al. (2011). Gut flora metabolism of phosphatidylcholine promotes cardiovascular disease. Nature 472 (7341), 57–63. doi:10.1038/nature09922
Witjes, J. J., Smits, L. P., Pekmez, C. T., Prodan, A., Meijnikman, A. S., Troelstra, M. A., et al. (2020). Donor fecal microbiota transplantation alters gut microbiota and metabolites in obese individuals with steatohepatitis. Hepatol. Commun. 4 (11), 1578–1590. doi:10.1002/hep4.1601
Woting, A., and Blaut, M. (2016). The intestinal microbiota in metabolic disease. Nutrients 8 (4), 202. doi:10.3390/nu8040202
Wu, C., Zhao, Y., Zhang, Y., Yang, Y., Su, W., Yang, Y., et al. (2022). Gut microbiota specifically mediates the anti-hypercholesterolemic effect of berberine (BBR) and facilitates to predict BBR's cholesterol-decreasing efficacy in patients. J. Adv. Res. 37, 197–208. doi:10.1016/j.jare.2021.07.011
Wu, D. N., Guan, L., Jiang, Y. X., Ma, S. H., Sun, Y. N., Lei, H. T., et al. (2019). Microbiome and metabonomics study of quercetin for the treatment of atherosclerosis. Cardiovasc Diagn Ther. 9 (6), 545–560. doi:10.21037/cdt.2019.12.04
Wu, J., Wu, L., Lou, Y., Yang, M. F., Liu, Y., and Hong, Z. F. (2021). Study on the effect of compound gentian Yin Chen granules on the colon and intestinal microorganism of rats with non-alcoholic fatty liver. Lishizhen Med. Materia Medica Res. 32 (10), 2380–2383.
Wu, M., Yang, S., Wang, S., Cao, Y., Zhao, R., Li, X., et al. (2020). Effect of berberine on atherosclerosis and gut microbiota modulation and their correlation in high-fat diet-fed ApoE-/- mice. Front. Pharmacol. 11, 223. doi:10.3389/fphar.2020.00223
Wu, S., Hu, R., Nakano, H., Chen, K., Liu, M., He, X., et al. (2018). Modulation of gut microbiota by Lonicera caerulea L. Berry polyphenols in a mouse model of fatty liver induced by high fat diet. Molecules 23 (12), 3213. doi:10.3390/molecules23123213
Xie, W. L., Peng, H. B., Li, Y., Huang, L. P., Chen, S. X., Wu, Y. F., et al. (2021). Liver with liver stagnation and spleen deficiency syndrome and intestinal microflora. Chin. J. Exp. Traditional Med. Formulae 27 (03), 129–137. doi:10.13422/j.cnki.syfjx.20210212
Xing, Y. W., Lei, G. T., Wu, Q. H., Jiang, Y., and Huang, M. X. (2019). Procyanidin B2 protects against diet-induced obesity and non-alcoholic fatty liver disease via the modulation of the gut microbiota in rabbits. World J. Gastroenterol. 25 (8), 955–966. doi:10.3748/wjg.v25.i8.955
Xu, J. H. (2013). Inhibition and mechanisms of berberine on the metabolic endotoxemia in rats induced by high fat diets. [dissertation/master's thesis]. Shanghai: Naval Medical University.
Xu, L., Fu, J., Fang, F., Chen, L. Z., and Zhuang, G. F. (2019). Effect of modfied yinchen wuling san in treating non-alcoholic fatty liver disease with moisture and heat implication and on intestinal microflora. Chin. J. Exp. Traditional Med. Formulae 25 (12), 127–132. doi:10.13422/j.cnki.syfjx.201901232
Xu, X. M., Lin, W. J., Zhang, Y. M., and Xu, R. Q. (2017). Hypolipidemic effect of Zexie Tang and correlation with intestinal microflora. Chin. J. Exp. Traditional Med. Formulae 23 (03), 116–121. doi:10.13422/j.cnki.syfjx.2017030116
Yang, L., Chen, K. C., Luo, D. S., and Guo, J. (2022a). Efficacy and mechanism of tianhuang formula in regulating lipid metabolism disorders in senile mice based on gut microbiota-tßmca-FXR Axis. Pharmacology and Clinics of Chinese materia medica, 1–18. doi:10.13412/j.cnki.zyyl.20220615.001
Yang, Q., Xu, Y., Shen, L., Pan, Y., Huang, J., Ma, Q., et al. (2022b). Guanxinning tablet attenuates coronary atherosclerosis via regulating the gut microbiota and their metabolites in Tibetan minipigs induced by a high-fat diet. J. Immunol. Res. 2022, 7128230. doi:10.1155/2022/7128230
Yang, S., Cao, S., Li, C., Zhang, J., Liu, C., Qiu, F., et al. (2022c). Berberrubine, a main metabolite of berberine, alleviates non-alcoholic fatty liver disease via modulating glucose and lipid metabolism and restoring gut microbiota. Front. Pharmacol. 13, 913378. doi:10.3389/fphar.2022.913378
Yang, X., Mo, W., Zheng, C., Li, W., Tang, J., and Wu, X. (2020). Alleviating effects of noni fruit polysaccharide on hepatic oxidative stress and inflammation in rats under a high-fat diet and its possible mechanisms. Food Funct. 11 (4), 2953–2968. doi:10.1039/d0fo00178c
Yang, Y., Li, M., Wang, Q., Huang, H., Zhao, Y., Du, F., et al. (2022d). Pueraria lobata starch regulates gut microbiota and alleviates high-fat high-cholesterol diet induced non-alcoholic fatty liver disease in mice. Food Res. Int. 157, 111401. doi:10.1016/j.foodres.2022.111401
Yao, Y. W. (2017). The role of gut-liver axis in the pathogenesis of NAFLD mice and possible mechanism of resveratrol. [dissertation/master's thesis], [Changsha]. Shijiazhuang: Hebei Medical University.
Yoshida, N., Yamashita, T., Osone, T., Hosooka, T., Shinohara, M., Kitahama, S., et al. (2021). Bacteroides spp. promotes branched-chain amino acid catabolism in Brown fat and inhibits obesity. iScience 24 (11), 103342. doi:10.1016/j.isci.2021.103342
You, Y. M. (2016). The effects and mechanisms of Zanthoxylum alkylamides on sugar and lipid metabolism in rats. [dissertation/master's thesis]. Chongqing: Southwest University.
Yu, Z. H., Biao, Y. N., Zhang, M. Q., Liu, C. X., Li, Z. X., Han, Y. L., et al. (2021). Correlation between effect of Danggui Shaoyao san on prevention and treatment of nonalcoholic fatty liver disease and intestinal microecology. J. Hebei Med. Univ. 36 (04), 1–5. doi:10.16370/j.cnki.13-1214/r.2021.04.001
Yuan, X. W., Jiang, N., Bai, D., Chen, B., Li, Y. M., Zeng, H., et al. (2021). Effect of Guizhi Tang on atherosclerosis by regulating immune and intestinal flora. Chin. J. Exp. Traditional Med. Formulae 27 (04), 24–29. doi:10.13422/j.cnki.syfjx.20202402
Yuan, Y., Liu, Q., Zhao, F., Cao, J., Shen, X., and Li, C. (2019). Holothuria leucospilota polysaccharides ameliorate hyperlipidemia in high-fat diet-induced rats via short-chain fatty acids production and lipid metabolism regulation. Int. J. Mol. Sci. 20 (19), 4738. doi:10.3390/ijms20194738
Zhang, H. Y., Tian, J. X., Lian, F. M., Li, M., Liu, W. K., Zhen, Z., et al. (2021a). Therapeutic mechanisms of traditional Chinese medicine to improve metabolic diseases via the gut microbiota. Biomed. Pharmacother. 133, 110857. doi:10.1016/j.biopha.2020.110857
Zhang, L. D., Xiong, W., Xu, Q., Liu, X. H., Huang, J. Q., Zhang, Q. Q., et al. (2021b). Effect of Usnea diffracta against atherosclerosis in rats and on microbial flora of ileum: An investigation based on interior-exterior relationship between heart and small intestine. Chin. J. Exp. Traditional Med. Formulae 27 (14), 36–46. doi:10.13422/j.cnki.syfjx.20211302
Zhang, L., Shi, M., Ji, J., Hu, X., and Chen, F. (2019). Gut microbiota determines the prevention effects of Luffa cylindrica (L.) Roem supplementation against obesity and associated metabolic disorders induced by high-fat diet. FASEB J. 33 (9), 10339–10352. doi:10.1096/fj.201900488R
Zhang, p., Wang, L., Chen, J., and Xu, F. (2022a). Effects of Rosa roxburghii Tratt polysaccharide on ileal mucosal barrier function in mice with non-alcoholic fatty liver. Food Sci., 1–20.
Zhang, Q., Fan, X. Y., Guo, W. L., Cao, Y. J., Lin, Y. C., Cheng, W. J., et al. (2020a). The protective mechanisms of macroalgae Laminaria japonica consumption against lipid metabolism disorders in high-fat diet-induced hyperlipidemic rats. Food Funct. 11 (4), 3256–3270. doi:10.1039/d0fo00065e
Zhang, S., Hong, F., Ma, C., and Yang, S. (2022b). Hepatic lipid metabolism disorder and atherosclerosis. Endocr. Metab. Immune Disord. Drug Targets 22 (6), 590–600. doi:10.2174/1871530322666211220110810
Zhang, S., Li, H., Yuan, L., Zhang, J., Han, L., Liu, R., et al. (2020b). Molecular characterization of gut microbiota in highlipid dietinduced hyperlipidemic rats treated with simvastatin. Int. J. Mol. Med. 45 (5), 1601–1615. doi:10.3892/ijmm.2020.4516
Zhang, S., Wang, Y., Lu, F., Mohammed, S. A. D., Liu, H., Ding, S., et al. (2022c). Mechanism of action of Shenerjiangzhi formulation on hyperlipidemia induced by consumption of a high-fat diet in rats using network pharmacology and analyses of the gut microbiota. Front. Pharmacol. 13, 745074. doi:10.3389/fphar.2022.745074
Zhang, X. J., Deng, Y. X., Shi, Q. Z., He, M. Y., Chen, B., and Qiu, X. M. (2014). Hypolipidemic effect of the Chinese polyherbal Huanglian Jiedu decoction in type 2 diabetic rats and its possible mechanism. Phytomedicine 21 (5), 615–623. doi:10.1016/j.phymed.2013.11.004
Zhang, X. J. (2020a). Structural identification and effect of LowMolecular weight polysaccharides isolatedfrom Rosa Laevigata michx. Fruits on LipidMetabolism and gut microbiota in high-fat diet-fed rats. [dissertation/master's thesis]. Changsha: Hunan Agricultural University.
Zhang, X. Y., Wang, Y. Q., Jiang, D. M., Shi, X. W., and Du, F. Q. (2022d). Effects of qingan Qushi Huoxie prescription on intestinal flora in nonalcoholic fatty liver disease rats. West. J. Traditional Chin. Med. 35 (03), 19–22.
Zhang, Y., Gu, Y., Chen, Y., Huang, Z., Li, M., Jiang, W., et al. (2021c). Dingxin Recipe IV attenuates atherosclerosis by regulating lipid metabolism through LXR-α/SREBP1 pathway and modulating the gut microbiota in ApoE-/- mice fed with HFD. J. Ethnopharmacol. 266, 113436. doi:10.1016/j.jep.2020.113436
Zhang, Y., Tang, K., Deng, Y., Chen, R., Liang, S., Xie, H., et al. (2018). Effects of shenling baizhu powder herbal formula on intestinal microbiota in high-fat diet-induced NAFLD rats. Biomed. Pharmacother. 102, 1025–1036. doi:10.1016/j.biopha.2018.03.158
Zhang, Y., Yang, L., Zhao, N., Hong, Z., Cai, B., Le, Q., et al. (2021d). Soluble polysaccharide derived from Laminaria japonica attenuates obesity-related nonalcoholic fatty liver disease associated with gut microbiota regulation. Mar. Drugs 19 (12), 699. doi:10.3390/md19120699
Zhang, Y. Y. (2020b). Effect of Compound danshen dripping Pills on liver steatosis and intestinal flora in NAFLD mice. [dissertation/master's thesis]. Zhengzhou: Zhengzhou University.
Zhang-Sun, W., Augusto, L. A., Zhao, L., and Caroff, M. (2015). Desulfovibrio desulfuricans isolates from the gut of a single individual: Structural and biological lipid A characterization. FEBS Lett. 589 (1), 165–171. doi:10.1016/j.febslet.2014.11.042
Zhao, J., Liang, F. Y., Meng, H., and Kuang, R. (2022a). Changes of intestinal microflora in rats with high-fat diet-induced hyperlipidemia by 16S rDNA sequencing. Chin. J. Microecology 34 (03), 257–261. doi:10.13381/j.cnki.cjm.202203002
Zhao, J., Liu, P., Wu, Y., Guo, P., Liu, L., Ma, N., et al. (2018). Dietary fiber increases butyrate-producing bacteria and improves the growth performance of weaned piglets. J. Agric. Food Chem. 66 (30), 7995–8004. doi:10.1021/acs.jafc.8b02545
Zhao, L., Ma, P., Peng, Y., Wang, M., Peng, C., Zhang, Y., et al. (2021). Amelioration of hyperglycaemia and hyperlipidaemia by adjusting the interplay between gut microbiota and bile acid metabolism: Radix Scutellariae as a case. Phytomedicine 83, 153477. doi:10.1016/j.phymed.2021.153477
Zhao, Z., Wang, J., Ren, W., Bian, Y., Wang, Y., Wang, L., et al. (2022b). Effect of jiangan-jiangzhi Pill on gut microbiota and chronic inflammatory response in rats with non-alcoholic fatty liver. Chem. Biodivers. 19 (5), e202100987. doi:10.1002/cbdv.202100987
Zheng, H. L., Zhai, Y. R., Wu, Y., Meng, J. W., Ma, Y. X., Lv, M. J., et al. (2022). The effect of Huayu Qutan prescription on intestinal flora and intestinal mucosal barrier injury in ApoE-/- AS mice. Chin. Archives Traditional Chin. Med., 1–16.
Zhong, F. W., Li, G. X., and Zeng, L. (2022a). Gynostemma pentaphyllum saponins alleviate non-alcoholic fatty liver disease in rats by regulating intestinal flora and short-chain fatty acid metabolism. China J. Chin. Materia Medica 47 (09), 2500–2508. doi:10.19540/j.cnki.cjcmm.20211208.401
Zhong, M., Yan, Y., Yuan, H., Xu, G., Cai, F., Yang, Y., et al. (2022b). Astragalus mongholicus polysaccharides ameliorate hepatic lipid accumulation and inflammation as well as modulate gut microbiota in NAFLD rats. Food Funct. 13 (13), 7287–7301. doi:10.1039/d2fo01009g
Zhou, T. T., Chen, G. T., Cao, N., He, J. G., He, G. W., Xiao, C. Y., et al. (2021a). Preventive effect of green Brick tea on non-alcoholic fatty liver disease via gut microbiota changes. J. Tea Sci. 41 (05), 669–680. doi:10.13305/j.cnki.jts.20210914.001
Zhou, X., Chen, J., Sun, B., Wang, Z., Zhu, J., Yue, Z., et al. (2021b). Leucine, but not isoleucine or valine, affects serum lipid profiles and browning of WAT in mice. Food Funct. 12 (15), 6712–6724. doi:10.1039/d1fo00341k
Zhou, X., Li, J., Guo, J., Geng, B., Ji, W., Zhao, Q., et al. (2018). Gut-dependent microbial translocation induces inflammation and cardiovascular events after ST-elevation myocardial infarction. Microbiome 6 (1), 66. doi:10.1186/s40168-018-0441-4
Zhou, Z. Q. (2020). Based on intestinal microecology to study the intervention mechanism of Xiaoyao San on liver depression and spleen deficiency of non-alcoholic fatty liver disease. [dissertation/master's thesis]. Chengdu: Chengdu University of Traditional Chinese Medicine.
Zhu, B. R. (2021). Based on the theory of traditional Chinesemedicine of heart and small intestine connection toexplore the research of Alisma Orientalis Beverage on gut microbiota for atherosclerosis. [dissertation/master's thesis]. Nanjing: Nanjing University Of Chinese Medicine.
Zhu, F., Li, Y. M., Feng, T. T., Wu, Y., Zhang, H. X., Jin, G. Y., et al. (2019). Freeze-dried Si-Ni-San powder can ameliorate high fat diet-induced non-alcoholic fatty liver disease. World J. Gastroenterol. 25 (24), 3056–3068. doi:10.3748/wjg.v25.i24.3056
Zhu, L., Zhang, D., Zhu, H., Zhu, J., Weng, S., Dong, L., et al. (2018). Berberine treatment increases Akkermansia in the gut and improves high-fat diet-induced atherosclerosis in Apoe(-/-) mice. Atherosclerosis 268, 117–126. doi:10.1016/j.atherosclerosis.2017.11.023
Zimmermann, F., Roessler, J., Schmidt, D., Jasina, A., Schumann, P., Gast, M., et al. (2020). Impact of the gut microbiota on atorvastatin mediated effects on blood lipids. J. Clin. Med. 9 (5), 1596. doi:10.3390/jcm9051596
Keywords: intestinal flora, lipid metabolism disorders, obesity, hyperlipidemia, non-alcoholic fatty liver disease, atherosclerosis, traditional Chinese medicine
Citation: Liu M, Shi W, Huang Y, Wu Y and Wu K (2023) Intestinal flora: A new target for traditional Chinese medicine to improve lipid metabolism disorders. Front. Pharmacol. 14:1134430. doi: 10.3389/fphar.2023.1134430
Received: 30 December 2022; Accepted: 13 February 2023;
Published: 01 March 2023.
Edited by:
Juei-Tang Cheng, Chang Jung Christian University, TaiwanReviewed by:
Weijian Bei, Guangdong Metabolic Disease Research Center of Integrated Chinese and Western Medicine, ChinaLiang Ning, The Chinese University of Hong Kong, China
Copyright © 2023 Liu, Shi, Huang, Wu and Wu. This is an open-access article distributed under the terms of the Creative Commons Attribution License (CC BY). The use, distribution or reproduction in other forums is permitted, provided the original author(s) and the copyright owner(s) are credited and that the original publication in this journal is cited, in accordance with accepted academic practice. No use, distribution or reproduction is permitted which does not comply with these terms.
*Correspondence: Yeke Wu, 337872366@qq.com; Keming Wu, wu_keming1@126.com
†These authors have contributed equally to this work