- Department of Pharmacy, Tongji Hospital, Tongji Medical College, Huazhong University of Science and Technology, Wuhan, China
Chimeric antigen receptor T (CAR T)-cell therapy promises to revolutionize the management of hematologic malignancies and possibly other tumors. However, the main side effect of cytokine release syndrome (CRS) is a great challenge for its clinical application. Currently, treatment of CRS caused by CAR T-cell therapy is limited to tocilizumab (TCZ) and corticosteroids in clinical guidelines. However, the theoretical risks of these two agents may curb clinicians’ enthusiasm for their application, and the optimal treatment is still debated. CAR T-cell therapy induced-CRS treatment is a current research focus. Glycyrrhizin, which has diverse pharmacological effects, good tolerance, and affordability, is an ideal therapeutic alternative for CRS. It can also overcome the shortcoming of TCZ and corticosteroids. In this brief article, we discuss the therapeutic potential of glycyrrhizin for treating CRS caused by CAR T-cell therapy from the perspective of its pharmacological action.
1 Introduction
Chimeric antigen receptor T (CAR T) cells, in the simplest form, are T cells that are genetically engineered with a CAR structure that can recognize a specific antigen on the tumor cell surface and destroy malignant cells. Over the past decades, significant progress has been made in the development of CAR T-cell therapy. This therapy has initiated a new class of therapies and gained fresh prominence for antitumor treatment, especially in hematologic malignancies. Today, CAR T-cell therapy is a therapy that can cure patients with certain hematologic malignancies, although oncologists have not used the word “cure” lightly (Freyer and Porter, 2020).
One of the major hallmark challenges associated with almost all CAR T-cell therapies is the development of cytokine release syndrome (CRS). CRS is a potentially life-threatening systemic inflammatory response driven by elevations in inflammatory cytokines and chemokines. It is characterized by flu-like symptoms, hypotension, hypoxia, and even multi-organ failure in severe cases. The onset latency of CRS is determined by a multitude of factors, and CRS usually occurs within the first 2 weeks after CAR T-cell administration. Currently, only corticosteroids and tocilizumab (TCZ, an IL-6 receptor antagonist that blocks IL-6-mediated signal transduction by inhibiting IL-6 binding to IL-6 receptor and is the only FDA-approved therapy for treating CART-cell-associated CRS (Si and Teachey, 2020)) are recommended by many national and international guidelines for the management of CRS (Santomasso et al., 2021; Hayden et al., 2022). However, the theoretical risks of impairing the function of CAR T cells with the use of corticosteroids, worsening neurotoxicity with the use of TCZ, and predisposing patients to infections with the use of either agents may tend to stifle clinicians’ initiative to prescribe these two drugs though data supporting these risks are relatively limited (Banerjee et al., 2021). Contradictory evidence of the lethal effect of corticosteroids on CAR T cells has been found in different studies (Brentjens et al., 2013; Davila et al., 2014; Liu et al., 2020a). Nevertheless, it has been demonstrated in real-world analyses that higher cumulative doses of corticosteroids exposure, as well as both prolonged and early use of corticosteroids, were associated with worse overall survival, following CAR T-cell therapy (Strati et al., 2021), suggesting that the risk of corticosteroids affecting the amplification and persistence of CAR T cells is real. IL-6 is mainly eliminated via IL-6 receptor-mediated clearance, and TCZ treatment can induce a marked increase in serum IL-6 (Nishimoto et al., 2008). The transient rise of serum IL-6 can increase the passive diffusion of IL-6 into cerebrospinal fluid (CSF), but TCZ cannot cross the blood–brain barrier as easily as IL-6 (Nellan et al., 2018). Theoretically, TCZ could exacerbate cytokine-mediated neurotoxicity by causing an unopposed increase of IL-6 in CSF. This hypothesis is supported by the fact that patients who received TCZ treatment for CAR T-cell-related CRS were more likely to experience neurotoxicity (Frigault et al., 2020). IL-6 is a pivotal cytokine in the integrated immune response. One of its roles is to support the host in responding to infections (Rose-John et al., 2017). Hence, IL-6 receptor antagonist TCZ treatment might increase the risk of infections, as has been generally observed in corticosteroid therapies. Corticosteroid therapies can induce complicated infections by suppressing the immune response and altering host defense (Dale and Petersdorf, 1973). Although some data suggest that short-term use of TCZ for CRS will not significantly increase the susceptibility to infectious complications (Frigault et al., 2020), the use of corticosteroids is associated with higher rates of infections (Baird et al., 2021). More importantly, treatment of CRS with TCZ is clinically ineffective in more than 30% of patients, and corticosteroid-refractory CRS can also develop in some patients (Pan et al., 2021). Therefore, a novel treatment to minimize the lethal severity of CRS and maximize the benefits associated with CAR T-cell therapy is urgently needed in the clinical setting.
2 Glycyrrhizin and its potential advantages in the treatment of CRS induced by CAR T-cell therapy
Glycyrrhizin, also known as glycyrrhizinic acid, is a triterpene glycoside (saponin) with a molecular formula of C42H62O16 and a weight of 823 g/mol. It is the main water-soluble component of licorice root extract and consists of one molecule of glycyrrhetinic acid and two molecules of glucuronic acid. Glycyrrhizin has been used in China for more than 4,000 years. It is widely employed to treat a variety of diseases and conditions because it possesses multiple pharmacological properties, including anti-inflammation, antioxidative, immunomodulatory, antiviral, anticancer, and hepatoprotective effects (Chen et al., 2020; Rehman et al., 2020; Bakr et al., 2022).
The most important pharmacological effect of glycyrrhizin is anti-inflammation, and its anti-inflammatory actions are similar to those of glucocorticoids due to the structural similarity of glycyrrhizin with adrenocortical hormones (Chen et al., 2020). Glycyrrhizin elicits broad-spectrum anti-inflammatory actions via interacting with various inflammatory factors and pathways, as shown in Figure 1, which were summarized in detail by Richard (2021). However, unlike glucocorticoids that elicit immune-suppressing effects, glycyrrhizin is expected to enhance the immune response (Li et al., 2011; Soufy et al., 2012; Xu et al., 2018a). Moreover, unlike glucocorticoids that preferentially affect T lymphocytes for rapid apoptotic cell death (Tuosto et al., 1994), glycyrrhizin displays a mild action to slowly induce the apoptotic death of lymphocytes (Oh et al., 1999).
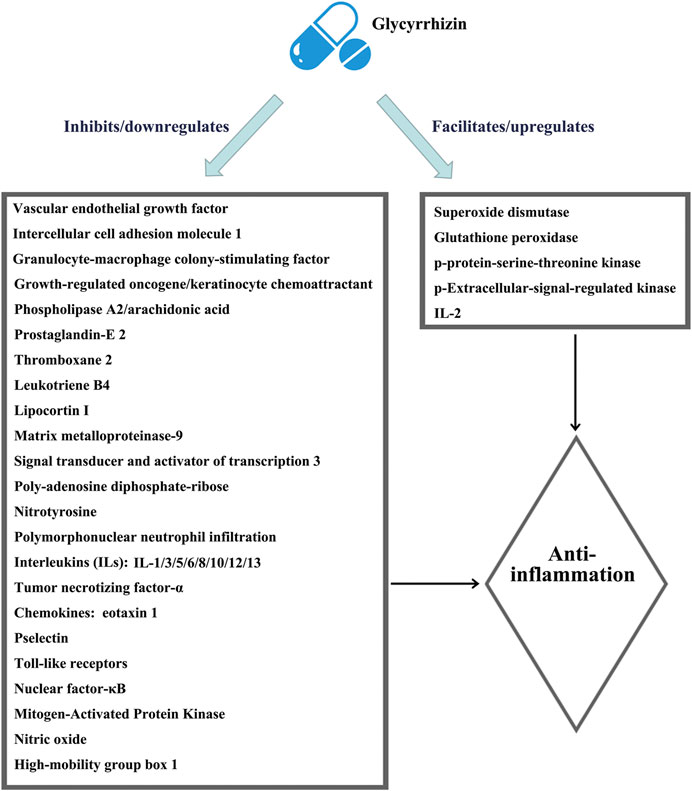
FIGURE 1. Schematic illustration of the comprehensive anti-inflammatory mechanisms of glycyrrhizin. The figure is drawn based on Figure 1 of Richard (2021).
Some studies suggested that glycyrrhizin can stimulate both T- and B-lymphocyte proliferation (Chavali et al., 1987; Jiang et al., 2020). Compared with glucocorticoids, it seems that the negative impact of glycyrrhizin on the pharmacokinetics/survival of CAR T cells is extremely low, and administration of glycyrrhizin will not put the patient into a condition with a risk of infections. In addition, with its broad-spectrum anti-inflammatory properties, glycyrrhizin can minimize the rate of clinical inefficacy when managing CRS, and it should be more effective than the therapies like TCZ that only target one single cytokine. No evidence indicates that glycyrrhizin can worsen neurotoxicity. Therefore, as expected from the established role of glycyrrhizin, glycyrrhizin could be a promising treatment for CRS with some distinct advantages over glucocorticoids and TCZ.
3 Broad-spectrum anti-inflammatory capability forms the cornerstone of glycyrrhizin in managing CRS induced by CAR T-cell therapy
In recent years, great progress has been made in exploring the potential pathophysiology of CRS trigged by CAR T-cell therapies. It is now generally accepted that CAR T cells are activated following target tumor cells and then induce the release of various inflammatory factors such as IFN-γ, TNF-α, which leads to the activation of bystander myeloid cell populations (e.g., monocytes, macrophages, and dendritic cells) and endothelial cells. These cells further promote the rapid production and secretion of proinflammatory cytokines such as IL-6 and IL-1β that trigger a cascade reaction and contribute to inflammatory toxicities. Large amounts of IL-6, in turn, activate the T cells and other immune cells and then lead to a positive CRS feedback loop (Cobb and Lee, 2021; Cosenza et al., 2021; Schubert et al., 2021). Therefore, strategies targeted at the cytokines mentioned previously or that can reduce myeloid and/or endothelial cell activation may prevent CRS. Numerous studies have shown that glycyrrhizin can downregulate the mRNA expression and the production of cytokines such as IL-1β, IL-6, TNF-α, and IFN-γ (Ni et al., 2011; Chen et al., 2017; Yu et al., 2017; Xu et al., 2018a; Sun et al., 2018; Tian et al., 2019) and mitigate the activation or dysfunction of monocytes, macrophages, dendritic cells, and endothelial cells in a variety of pathological models (Matsushima and Baba, 1992; Feng et al., 2013; Wakabayashi et al., 2018; Gowda et al., 2021). Some clinical trials have confirmed the anti-inflammatory properties of glycyrrhizin (Takeuchi-Hatanaka et al., 2016; Li et al., 2019; Cao et al., 2020). On a broader aspect, glycyrrhizin can represent an excellent therapeutic modality for CRS triggered by CAR T-cell therapies (Figure 2).
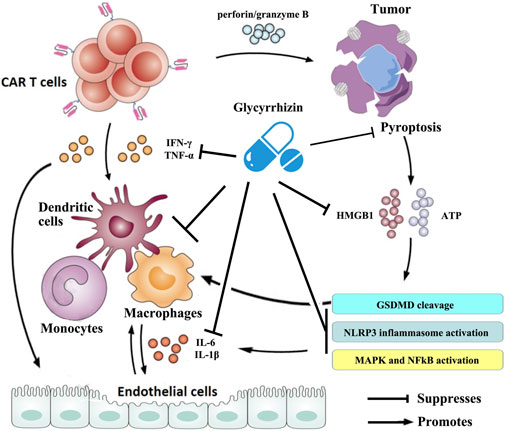
FIGURE 2. Schematic illustration of the therapeutic benefits of glycyrrhizin for CRS triggered by CAR T-cell therapy. The figure is drawn based on Figure 1 of Schubert et al. (2021) and Xiao et al. (2021).
4 Suppressing pyroptosis contributes to glycyrrhizin’s potential therapeutic effects in managing CRS induced by CAR T-cell therapy
Recent research conducted by Liu et al. showed that CAR T cells rapidly activate caspase-3 in human B leukemic cells and other targeted tumor cells through releasing of perforin/granzyme B and leading to pyroptosis of the targeted cells. Consequently, pyroptosis-released factors activate caspase-1 for gasdermin D (GSDMD) cleavage in macrophages to stimulate macrophages to produce proinflammatory cytokines, which may trigger CRS in CAR T-cell-treated patients (Liu et al., 2020b). However, it was reported that glycyrrhizin plays a role in inhibiting caspase 1/GSDMD and suppressing pyroptosis (Hua et al., 2019; Wang et al., 2020), indicating that it could block the occurrence of CRS in CAR T-cell treated patients. Moreover, the results from Liu et al. demonstrated that pyroptosis-released factors, particularly extensive extracellular adenosine 5′-triphosphate (ATP) and high-mobility group box 1 (HMGB1), contribute to the release of the CRS-related cytokine by macrophages (Liu et al., 2020a). ATP activates NACHT, LRR, and the PYD domain-containing protein 3 (NLRP3) inflammasome that cleaves caspase-1 in macrophages to promote the release of IL-1β. HMGB1 may induce IL-6 production in macrophages after tumor cell pyroptosis through the activation of mitogen-activated protein kinase (MAPK) and nuclear factor κB (NF-κB) (Liu et al., 2020b). It has been observed that glycyrrhizin has efficacy in suppressing the NLRP3 inflammasome and inhibiting the activation of NF-κB and MAPK signaling pathways (Yao and Sun, 2019). More importantly, glycyrrhizin itself was frequently used as an inhibitor of HMGB1. Research has also confirmed that glycyrrhizin can rescue macrophage activation induced by multiple etiological factors (Li et al., 2015; Gowda et al., 2021). These evidences suggest that glycyrrhizin has a potential effect in regulating CRS during CAR T-cell therapy by intervening in pyroptosis and/or its cascades (Figure 2).
5 Targeting multiple intracellular signaling pathways contributes to the potential therapeutic effects of glycyrrhizin in managing CRS induced by CAR T-cell therapy
Several studies have found that the use of small molecule inhibitors, including ruxolitinib (an inhibitor of Janus kinases 1 and 2) and itacitinib (a selective Janus kinase 1 inhibitor), may also help prevent CRS induced by CAR T-cell therapy (Huarte et al., 2020; Pan et al., 2021; Xu et al., 2022). Strikingly, it was identified that glycyrrhizin could inhibit the phosphorylation of Janus kinases 1 and 2 and reduce the activity of the JAK/STAT signaling pathway (Xu et al., 2018b; Wu et al., 2018). Moreover, recent studies have confirmed that dasatinib can switch off the cytokine release to reduce the risk of CRS by inhibiting the SRC family kinase lymphocyte-specific protein tyrosine kinase (LCK) (Mestermann et al., 2019; Leclercq et al., 2021). SRC family kinases share a common architecture that underlies a shared regulatory mechanism (Sicheri and Kuriyan, 1997). It should be noted that glycyrrhizin could decrease SRC kinase activity reported in a previous study (Wu et al., 2015), suggesting that it may have a similar capacity of effectively blocking CRS to dasatinib. These data indicate that glycyrrhizin holds a great developmental and application prospect in the treatment of CRS caused by CAR T-cell therapy.
6 Other potential therapeutic benefits of glycyrrhizin in CAR T-cell therapy
Glycyrrhizin, owing to its antipyretic action, hypertension activity, and inhibitory effect on airway mucus hyperproduction (Yanagawa et al., 2004; Nishimoto et al., 2010; Nazari et al., 2017), could be used as a supportive remedy for the fever, hypotension, and hypoxia that are the manifestations of CRS. In addition, adverse events, including immune effector cell-associated neurotoxicity syndrome (ICANS)/neurotoxicity and opportunistic infections, are common in CAR T-cell therapy, and they are associated with CRS (Hill et al., 2018; Ruff et al., 2020). Overwhelming evidence affirms that glycyrrhizin has beneficial antiviral, antibacterial, antifungal, and neuroprotective activities (Huan et al., 2021; Astaf’eva and Sukhenko, 2014; Utsunomiya et al., 1999; Paudel et al., 2020). These activities make glycyrrhizin a potential agent for adjuvant or preventive therapy in simultaneously managing other side effects of CAR T-cell therapy.
It is a general consensus that glycyrrhizin has broad activity against a wide variety of tumor cell types (Roohbakhsh et al., 2016). Furthermore, previous reports showed that glycyrrhizin was tolerated by normal human leukocytes/peripheral blood mononuclear cells, but it was effective in the treatment of both chronic myeloid leukemia and lymphoma in vitro or in vivo (Hibasami et al., 2006; Hostetler et al., 2017). These make glycyrrhizin an attractive agent for combinational therapy with CAR T cells in hematological malignancies, raising its value beyond CRS management. It has been identified that adding a programmed cell death protein-1 (PD-1) blockade to CAR T-cell therapy can escalate CAR T-cell function and, to some extent, improve prognosis and efficacy (Song and Zhang, 2020). However, it has been reported that PD-L1/PD-1 upregulation can be mediated by autocrine and paracrine activation of HMGB1 signaling (Wang et al., 2019; Xu et al., 2021). Glycyrrhizin, a direct inhibitor of HMGB1, may hold promise for a similar job as PD-1 blockade when combined with CAR T cells, reaffirming the superiority of using glycyrrhizin in CAR T-cell therapy.
7 Discussion
The optimal treatment regimen of CRS caused by CAR T-cell therapy is still a matter of debate and not well-defined. Glycyrrhizin is valued for its many pharmacological effects discussed previously, which make it a promising therapeutic alternative. It can overcome the shortcomings of the current mainstream therapeutic strategies (corticosteroids and TCZ) against CRS. Glycyrrhizin can inhibit almost all factors responsible for inflammatory reactions (Rehman et al., 2020). This is a decisive advantage over alternative strategies in CRS management, including those that can only block or neutralize IL-1, IL-6, and GM-CSF. That glycyrrhizin is safe, tolerable, convenient, and affordable adds interest to its clinical application. Therefore, glycyrrhizin can be preemptively or even prophylactically used in the early-grade CRS and can be administered for a longer course. However, the conjecture we proposed is based on the extensive pharmacological properties of glycyrrhizin. The clinical efficacy and safety and appropriate dosage regimens of glycyrrhizin in real-life clinical scenarios need to be further investigated with well-designed clinical trials.
Data availability statement
The original contributions presented in the study are included in the article/Supplementary Material; further inquiries can be directed to the corresponding authors.
Author contributions
PL and JL conceptualized and planned the review. XQ and PL wrote the manuscript. JL revised the manuscript. All authors read and approved the final manuscript.
Conflict of interest
The authors declare that the research was conducted in the absence of any commercial or financial relationships that could be construed as a potential conflict of interest.
Publisher’s note
All claims expressed in this article are solely those of the authors and do not necessarily represent those of their affiliated organizations, or those of the publisher, the editors, and the reviewers. Any product that may be evaluated in this article, or claim that may be made by its manufacturer, is not guaranteed or endorsed by the publisher.
References
Astaf'eva, O. V., and Sukhenko, L. T. (2014). Comparative analysis of antibacterial properties and chemical composition of Glycyrrhiza glabra L. from Astrakhan region (Russia) and Calabria region (Italy). Bull. Exp. Biol. Med. 156 (6), 829–832. doi:10.1007/s10517-014-2462-8
Baird, J. H., Epstein, D. J., Tamaresis, J. S., Ehlinger, Z., Spiegel, J. Y., Craig, J., et al. (2021). Immune reconstitution and infectious complications following axicabtagene ciloleucel therapy for large B-cell lymphoma. Blood Adv. 5 (1), 143–155. doi:10.1182/bloodadvances.2020002732
Bakr, A. F., Shao, P., and Farag, M. A. (2022). Recent advances in glycyrrhizin metabolism, health benefits, clinical effects and drug delivery systems for efficacy improvement; a comprehensive review. Phytomedicine 99, 153999. doi:10.1016/j.phymed.2022.153999
Banerjee, R., Fakhri, B., and Shah, N. (2021). Toci or not toci: Innovations in the diagnosis, prevention, and early management of cytokine release syndrome. Leuk. Lymphoma 62, 2600–2611. doi:10.1080/10428194.2021.1924370
Brentjens, R. J., Davila, M. L., Riviere, I., Park, J., Wang, X., Cowell, L. G., et al. (2013). CD19-targeted T cells rapidly induce molecular remissions in adults with chemotherapy-refractory acute lymphoblastic leukemia. Sci. Transl. Med. 5 (177), 177ra38. doi:10.1126/scitranslmed.3005930
Cao, Z. Y., Liu, Y. Z., Li, J. M., Ruan, Y. M., Yan, W. J., Zhong, S. Y., et al. (2020). Glycyrrhizic acid as an adjunctive treatment for depression through anti-inflammation: A randomized placebo-controlled clinical trial. J. Affect Disord. 265, 247–254. doi:10.1016/j.jad.2020.01.048
Chavali, S. R., Francis, T., and Campbell, J. B. (1987). An in vitro study of immunomodulatory effects of some saponins. Int. J. Immunopharmacol. 9 (6), 675–683. doi:10.1016/0192-0561(87)90038-5
Chen, K., Yang, R., Shen, F. Q., and Zhu, H. L. (2020). Advances in pharmacological activities and mechanisms of glycyrrhizic acid. Curr. Med. Chem. 27 (36), 6219–6243. doi:10.2174/0929867325666191011115407
Chen, X., Fang, D., Li, L., Chen, L., Li, Q., Gong, F., et al. (2017). Glycyrrhizin ameliorates experimental colitis through attenuating interleukin-17-producing T cell responses via regulating antigen-presenting cells. Immunol. Res. 65 (3), 666–680. doi:10.1007/s12026-017-8894-2
Cobb, D. A., and Lee, D. W. (2021). Cytokine release syndrome biology and management. Cancer J. 27 (2), 119–125. doi:10.1097/PPO.0000000000000515
Cosenza, M., Sacchi, S., and Pozzi, S. (2021). Cytokine release syndrome associated with T-cell-based therapies for hematological malignancies: Pathophysiology, clinical presentation, and treatment. Int. J. Mol. Sci. 22 (14), 7652. doi:10.3390/ijms22147652
Dale, D. C., and Petersdorf, R. G. (1973). Corticosteroids and infectious diseases. Med. Clin. North Am. 57 (5), 1277–1287. doi:10.1016/s0025-7125(16)32228-3
Davila, M. L., Riviere, I., Wang, X., Bartido, S., Park, J., Curran, K., et al. (2014). Efficacy and toxicity management of 19-28z CAR T cell therapy in B cell acute lymphoblastic leukemia. Sci. Transl. Med. 6 (224), 224ra25. doi:10.1126/scitranslmed.3008226
Feng, L., Zhu, M. M., Zhang, M. H., Wang, R. S., Tan, X. B., Song, J., et al. (2013). Protection of glycyrrhizic acid against AGEs-induced endothelial dysfunction through inhibiting RAGE/NF-κB pathway activation in human umbilical vein endothelial cells. J. Ethnopharmacol. 148 (1), 27–36. doi:10.1016/j.jep.2013.03.035
Freyer, C. W., and Porter, D. L. (2020). Advances in CAR T therapy for hematologic malignancies. Pharmacotherapy 40 (8), 741–755. doi:10.1002/phar.2414
Frigault, M. J., Nikiforow, S., Mansour, M. K., Hu, Z. H., Horowitz, M. M., Riches, M. L., et al. (2020). Tocilizumab not associated with increased infection risk after CAR T-cell therapy: Implications for COVID-19? Blood 136 (1), 137–139. doi:10.1182/blood.2020006216
Gowda, P., Patrick, S., Joshi, S. D., Kumawat, R. K., and Sen, E. (2021). Glycyrrhizin prevents SARS-CoV-2 S1 and Orf3a induced high mobility group box 1 (HMGB1) release and inhibits viral replication. Cytokine 142, 155496. doi:10.1016/j.cyto.2021.155496
Hayden, P. J., Roddie, C., Bader, P., Basak, G. W., Bonig, H., Bonini, C., et al. (2022). Management of adults and children receiving CAR T-cell therapy: 2021 best practice recommendations of the European society for blood and marrow transplantation (EBMT) and the joint accreditation committee of ISCT and EBMT (JACIE) and the European haematology association (EHA). Ann. Oncol. 33 (3), 259–275. doi:10.1016/j.annonc.2021.12.003
Hibasami, H., Iwase, H., Yoshioka, K., and Takahashi, H. (2006). Glycyrrhetic acid (a metabolic substance and aglycon of glycyrrhizin) induces apoptosis in human hepatoma, promyelotic leukemia and stomach cancer cells. Int. J. Mol. Med. 17 (2), 215–219. doi:10.3892/ijmm.17.2.215
Hill, J. A., Li, D., Hay, K. A., Green, M. L., Cherian, S., Chen, X., et al. (2018). Infectious complications of CD19-targeted chimeric antigen receptor-modified T-cell immunotherapy. Blood 131 (1), 121–130. doi:10.1182/blood-2017-07-793760
Hostetler, B. J., Uchakina, O. N., Ban, H., and McKallip, R. J. (2017). Treatment of hematological malignancies with glycyrrhizic acid. Anticancer Res. 37 (3), 997–1004. doi:10.21873/anticanres.11409
Hua, S., Ma, M., Fei, X., Zhang, Y., Gong, F., and Fang, M. (2019). Glycyrrhizin attenuates hepatic ischemia-reperfusion injury by suppressing HMGB1-dependent GSDMD-mediated kupffer cells pyroptosis. Int. Immunopharmacol. 68, 145–155. doi:10.1016/j.intimp.2019.01.002
Huan, C., Xu, Y., Zhang, W., Guo, T., Pan, H., and Gao, S. (2021). Research progress on the antiviral activity of glycyrrhizin and its derivatives in liquorice. Front. Pharmacol. 12, 680674. doi:10.3389/fphar.2021.680674
Huarte, E., O'Connor, R. S., Peel, M. T., Nunez-Cruz, S., Leferovich, J., Juvekar, A., et al. (2020). Itacitinib (INCB039110), a JAK1 inhibitor, reduces cytokines associated with cytokine release syndrome induced by CAR T-cell therapy. Clin. Cancer Res. 26 (23), 6299–6309. doi:10.1158/1078-0432.CCR-20-1739
Jiang, R., Gao, J., Shen, J., Zhu, X., Wang, H., Feng, S., et al. (2020). Glycyrrhizic acid improves cognitive levels of aging mice by regulating T/B cell proliferation. Front. Aging Neurosci. 12, 570116. doi:10.3389/fnagi.2020.570116
Leclercq, G., Haegel, H., Schneider, A., Giusti, A. M., Marrer-Berger, E., Boetsch, C., et al. (2021). Src/lck inhibitor dasatinib reversibly switches off cytokine release and T cell cytotoxicity following stimulation with T cell bispecific antibodies. J. Immunother. Cancer 9 (7), e002582. doi:10.1136/jitc-2021-002582
Li, L., Ma, Q., and Li, H. (2019). Effect of vitiligo treatment using compound glycyrrhizin combined with fractional carbon dioxide laser and topical triamcinolone acetonide on serum interleukin-17 and tissue growth factor-β levels. J. Int. Med. Res. 47 (11), 5623–5631. doi:10.1177/0300060519871382
Li, X. L., Zhou, A. G., Zhang, L., and Chen, W. J. (2011). Antioxidant status and immune activity of glycyrrhizin in allergic rhinitis mice. Int. J. Mol. Sci. 12 (2), 905–916. doi:10.3390/ijms12020905
Li, X., Yue, Y., Zhu, Y., and Xiong, S. (2015). Extracellular, but not intracellular HMGB1, facilitates self-DNA induced macrophage activation via promoting DNA accumulation in endosomes and contributes to the pathogenesis of lupus nephritis. Mol. Immunol. 65 (1), 177–188. doi:10.1016/j.molimm.2015.01.023
Liu, S., Deng, B., Yin, Z., Pan, J., Lin, Y., Ling, Z., et al. (2020a). Corticosteroids do not influence the efficacy and kinetics of CAR T cells for B-cell acute lymphoblastic leukemia. Blood Cancer J. 10 (2), 15. doi:10.1038/s41408-020-0280-y
Liu, S., Fang, Y., Chen, X., Wang, Z., Liang, X., Zhang, T., et al. (2020b). Gasdermin E-mediated target cell pyroptosis by CAR T cells triggers cytokine release syndrome. Sci. Immunol. 5 (43), eaax7969. doi:10.1126/sciimmunol.aax7969
Matsushima, Y., and Baba, T. (1992). An antigranulomatous effect of glycyrrhizin. J. Exp. Pathol. 6 (1-2), 25–30.
Mestermann, K., Giavridis, T., Weber, J., Rydzek, J., Frenz, S., Nerreter, T., et al. (2019). The tyrosine kinase inhibitor dasatinib acts as a pharmacologic on/off switch for CAR T cells. Sci. Transl. Med. 11 (499), eaau5907. doi:10.1126/scitranslmed.aau5907
Nazari, S., Rameshrad, M., and Hosseinzadeh, H. (2017). Toxicological effects of Glycyrrhiza glabra (licorice): A review. Phytother. Res. 31 (11), 1635–1650. doi:10.1002/ptr.5893
Nellan, A., McCully, C. M. L., Cruz Garcia, R., Jayaprakash, N., Widemann, B. C., Lee, D. W., et al. (2018). Improved CNS exposure to tocilizumab after cerebrospinal fluid compared to intravenous administration in rhesus macaques. Blood 132 (6), 662–666. doi:10.1182/blood-2018-05-846428
Ni, Y. F., Kuai, J. K., Lu, Z. F., Yang, G. D., Fu, H. Y., Wang, J., et al. (2011). Glycyrrhizin treatment is associated with attenuation of lipopolysaccharide-induced acute lung injury by inhibiting cyclooxygenase-2 and inducible nitric oxide synthase expression. J. Surg. Res. 165 (1), e29–e35. doi:10.1016/j.jss.2010.10.004
Nishimoto, N., Terao, K., Mima, T., Nakahara, H., Takagi, N., and Kakehi, T. (2008). Mechanisms and pathologic significances in increase in serum interleukin-6 (IL-6) and soluble IL-6 receptor after administration of an anti-IL-6 receptor antibody, tocilizumab, in patients with rheumatoid arthritis and Castleman disease. Blood 112 (10), 3959–3964. doi:10.1182/blood-2008-05-155846
Nishimoto, Y., Hisatsune, A., Katsuki, H., Miyata, T., Yokomizo, K., and Isohama, Y. (2010). Glycyrrhizin attenuates mucus production by inhibition of MUC5AC mRNA expression in vivo and in vitro. J. Pharmacol. Sci. 113 (1), 76–83. doi:10.1254/jphs.09344fp
Oh, C., Kim, Y., Eun, J., Yokoyama, T., Kato, M., and Nakashima, I. (1999). Induction of T lymphocyte apoptosis by treatment with glycyrrhizin. Am. J. Chin. Med. 27 (2), 217–226. doi:10.1142/S0192415X99000252
Pan, J., Deng, B., Ling, Z., Song, W., Xu, J., Duan, J., et al. (2021). Ruxolitinib mitigates steroid-refractory CRS during CAR T therapy. J. Cell Mol. Med. 25 (2), 1089–1099. doi:10.1111/jcmm.16176
Paudel, Y. N., Angelopoulou, E., Semple, B., Piperi, C., Othman, I., and Shaikh, M. F. (2020). Potential neuroprotective effect of the HMGB1 inhibitor glycyrrhizin in neurological disorders. ACS Chem. Neurosci. 11 (4), 485–500. doi:10.1021/acschemneuro.9b00640
Rehman, M. U., Farooq, A., Ali, R., Bashir, S., Bashir, N., Majeed, S., et al. (2020). Preclinical evidence for the pharmacological actions of glycyrrhizic acid: A comprehensive review. Curr. Drug Metab. 21 (6), 436–465. doi:10.2174/1389200221666200620204914
Richard, S. A. (2021). Exploring the pivotal immunomodulatory and anti-inflammatory potentials of glycyrrhizic and glycyrrhetinic acids. Mediat. Inflamm. 2021, 6699560. doi:10.1155/2021/6699560
Roohbakhsh, A., Iranshahy, M., and Iranshahi, M. (2016). Glycyrrhetinic acid and its derivatives: Anti-cancer and cancer chemopreventive properties, mechanisms of action and structure- cytotoxic activity relationship. Curr. Med. Chem. 23 (5), 498–517. doi:10.2174/0929867323666160112122256
Rose-John, S., Winthrop, K., and Calabrese, L. (2017). The role of IL-6 in host defence against infections: Immunobiology and clinical implications. Nat. Rev. Rheumatol. 13 (7), 399–409. doi:10.1038/nrrheum.2017.83
Ruff, M. W., Siegler, E. L., and Kenderian, S. S. (2020). A concise review of neurologic complications associated with chimeric antigen receptor T-cell immunotherapy. Neurol. Clin. 38 (4), 953–963. doi:10.1016/j.ncl.2020.08.001
Santomasso, B. D., Nastoupil, L. J., Adkins, S., Lacchetti, C., Schneider, B. J., Anadkat, M., et al. (2021). Management of immune-related adverse events in patients treated with chimeric antigen receptor T-cell therapy: ASCO guideline. J. Clin. Oncol. 39 (35), 3978–3992. doi:10.1200/JCO.21.01992
Schubert, M. L., Schmitt, M., Wang, L., Ramos, C. A., Jordan, K., Muller-Tidow, C., et al. (2021). Side-effect management of chimeric antigen receptor (CAR) T-cell therapy. Ann. Oncol. 32 (1), 34–48. doi:10.1016/j.annonc.2020.10.478
Si, S., and Teachey, D. T. (2020). Spotlight on tocilizumab in the treatment of CAR-T-cell-induced cytokine release syndrome: Clinical evidence to date. Ther. Clin. Risk Manag. 16, 705–714. doi:10.2147/TCRM.S223468
Sicheri, F., and Kuriyan, J. (1997). Structures of Src-family tyrosine kinases. Curr. Opin. Struct. Biol. 7 (6), 777–785. doi:10.1016/s0959-440x(97)80146-7
Song, W., and Zhang, M. (2020). Use of CAR T cell therapy, PD-1 blockade, and their combination for the treatment of hematological malignancies. Clin. Immunol. 214, 108382. doi:10.1016/j.clim.2020.108382
Soufy, H., Yassein, S., Ahmed, A. R., Khodier, M. H., Kutkat, M. A., Nasr, S. M., et al. (2012). Antiviral and immune stimulant activities of glycyrrhizin against duck hepatitis virus. Afr. J. Tradit. Complement. Altern. Med. 9 (3), 389–395. doi:10.4314/ajtcam.v9i3.14
Strati, P., Ahmed, S., Furqan, F., Fayad, L. E., Lee, H. J., Iyer, S. P., et al. (2021). Prognostic impact of corticosteroids on efficacy of chimeric antigen receptor T-cell therapy in large B-cell lymphoma. Blood 137 (23), 3272–3276. doi:10.1182/blood.2020008865
Sun, Y., Chen, H., Dai, J., Wan, Z., Xiong, P., Xu, Y., et al. (2018). Glycyrrhizin protects mice against experimental autoimmune encephalomyelitis by inhibiting high-mobility group box 1 (HMGB1) expression and neuronal HMGB1 release. Front. Immunol. 9, 1518. doi:10.3389/fimmu.2018.01518
Takeuchi-Hatanaka, K., Yasuda, T., Naruishi, K., Katsuragi-Fuke, K., Inubushi, J., Ootsuki, H., et al. (2016). Effects of new over-the-counter periodontal ointment-containing applicator with single-tuft brush on cytokine levels in gingival crevicular fluid during supportive periodontal therapy phase: A randomized double-blind clinical trial. J. Periodontal Res. 51 (3), 321–331. doi:10.1111/jre.12311
Tian, X., Liu, Y., Liu, X., Gao, S., and Sun, X. (2019). Glycyrrhizic acid ammonium salt alleviates Concanavalin A-induced immunological liver injury in mice through the regulation of the balance of immune cells and the inhibition of hepatocyte apoptosis. Biomed. Pharmacother. 120, 109481. doi:10.1016/j.biopha.2019.109481
Tuosto, L., Cundari, E., Gilardini Montani, M. S., and Piccolella, E. (1994). Analysis of susceptibility of mature human T lymphocytes to dexamethasone-induced apoptosis. Eur. J. Immunol. 24 (5), 1061–1065. doi:10.1002/eji.1830240508
Utsunomiya, T., Kobayashi, M., Herndon, D. N., Pollard, R. B., and Suzuki, F. (1999). Effects of glycyrrhizin, an active component of licorice roots, on Candida albicans infection in thermally injured mice. Clin. Exp. Immunol. 116 (2), 291–298. doi:10.1046/j.1365-2249.1999.00890.x
Wakabayashi, A., Shimizu, M., Shinya, E., and Takahashi, H. (2018). HMGB1 released from intestinal epithelia damaged by cholera toxin adjuvant contributes to activation of mucosal dendritic cells and induction of intestinal cytotoxic T lymphocytes and IgA. Cell Death Dis. 9 (6), 631. doi:10.1038/s41419-018-0665-z
Wang, W., Chapman, N. M., Zhang, B., Li, M., Fan, M., Laribee, R. N., et al. (2019). Upregulation of PD-L1 via HMGB1-activated IRF3 and NF-κB contributes to UV radiation-induced immune suppression. Cancer Res. 79 (11), 2909–2922. doi:10.1158/0008-5472.CAN-18-3134
Wang, Y., Zhang, H., Chen, Q., Jiao, F., Shi, C., Pei, M., et al. (2020). TNF-α/HMGB1 inflammation signalling pathway regulates pyroptosis during liver failure and acute kidney injury. Cell Prolif. 53 (6), e12829. doi:10.1111/cpr.12829
Wu, H., Wu, T., Hua, W., Dong, X., Gao, Y., Zhao, X., et al. (2015). PGE2 receptor agonist misoprostol protects brain against intracerebral hemorrhage in mice. Neurobiol. Aging 36 (3), 1439–1450. doi:10.1016/j.neurobiolaging.2014.12.029
Wu, X., Wang, W., Chen, Y., Liu, X., Wang, J., Qin, X., et al. (2018). Glycyrrhizin suppresses the growth of human NSCLC cell line HCC827 by downregulating HMGB1 level. Biomed. Res. Int. 2018, 1–7. doi:10.1155/2018/6916797
Xiao, X., Huang, S., Chen, S., Wang, Y., Sun, Q., Xu, X., et al. (2021). Mechanisms of cytokine release syndrome and neurotoxicity of CAR T-cell therapy and associated prevention and management strategies. J. Exp. Clin. Cancer Res. 40 (1), 367. doi:10.1186/s13046-021-02148-6
Xu, H. Z., Li, T. F., Wang, C., Ma, Y., Liu, Y., Zheng, M. Y., et al. (2021). Synergy of nanodiamond-doxorubicin conjugates and PD-L1 blockade effectively turns tumor-associated macrophages against tumor cells. J. Nanobiotechnology 19 (1), 268. doi:10.1186/s12951-021-01017-w
Xu, M., Gong, L., Wang, B., Wu, Y., Wang, Y., Mei, X., et al. (2018b). Glycyrrhizin attenuates Salmonella enterica serovar typhimurium infection: New insights into its protective mechanism. Front. Immunol. 9, 2321. doi:10.3389/fimmu.2018.02321
Xu, M., Min, Z., and Wei, Y. (2018a). Glycyrrhizin inhibits IFN-gamma-induced CXCL10 by suppressing the JAK/STAT1 signal pathway in HaCaT cells. Xi Bao Yu Fen Zi Mian Yi Xue Za Zhi 34 (8), 708–713.
Xu, N., Yang, X. F., Xue, S. L., Tan, J. W., Li, M. H., Ye, J., et al. (2022). Ruxolitinib reduces severe CRS response by suspending CAR T cell function instead of damaging CAR T cells. Biochem. Biophys. Res. Commun. 595, 54–61. doi:10.1016/j.bbrc.2022.01.070
Yanagawa, Y., Ogura, M., Fujimoto, E., Shono, S., and Okuda, E. (2004). Effects and cost of glycyrrhizin in the treatment of upper respiratory tract infections in members of the Japanese maritime self-defense force: Preliminary report of a prospective, randomized, double-blind, controlled, parallel-group, alternate-day treatment assignment clinical trial. Curr. Ther. Res. Clin. Exp. 65 (1), 26–33. doi:10.1016/S0011-393X(04)90002-1
Yao, L., and Sun, T. (2019). Glycyrrhizin administration ameliorates Streptococcus aureus-induced acute lung injury. Int. Immunopharmacol. 70, 504–511. doi:10.1016/j.intimp.2019.02.046
Keywords: glycyrrhizin, chimeric antigen receptor T-cell therapy, cytokine release syndrome, tocilizumab, corticosteroids
Citation: Qi X, Li J and Luo P (2023) Glycyrrhizin for treatment of CRS caused by CAR T-cell therapy: A pharmacological perspective. Front. Pharmacol. 14:1134174. doi: 10.3389/fphar.2023.1134174
Received: 30 December 2022; Accepted: 13 February 2023;
Published: 27 February 2023.
Edited by:
Leming Sun, Northwestern Polytechnical University, ChinaReviewed by:
Reza Elahi, Zanjan University of Medical Sciences, IranCopyright © 2023 Qi, Li and Luo. This is an open-access article distributed under the terms of the Creative Commons Attribution License (CC BY). The use, distribution or reproduction in other forums is permitted, provided the original author(s) and the copyright owner(s) are credited and that the original publication in this journal is cited, in accordance with accepted academic practice. No use, distribution or reproduction is permitted which does not comply with these terms.
*Correspondence: Pan Luo, bHVvcGFuMTA2QDEyNi5jb20=; Juan Li, bGlqdWFuQHRqaC50am11LmVkdS5jbg==