- 1The Affiliated Anning First Hospital, Faculty of Life Science and Technology, Kunming University of Science and Technology, Kunming, China
- 2The Second Affiliated Hospital of Guangzhou University of Chinese Medicine, Guangdong Provincial Hospital of Chinese Medicine, Guangzhou, China
Influenza pneumonia has challenged public health and social development. One of the hallmarks of severe influenza pneumonia is overproduction of pro-inflammatory cytokines and chemokines, which result from the continuous activation of intracellular signaling pathways, such as the NF-κB pathway, mediated by the interplay between viruses and host pattern recognition receptors (PRRs). It has been reported that traditional Chinese medicines (TCMs) can not only inhibit viral replication and inflammatory responses but also affect the expression of key components of PRRs and NF-κB signaling pathways. However, whether the antiviral and anti-inflammatory roles of TCM are related with its effects on NF-κB signaling pathway activated by PRRs remains unclear. Here, we reviewed the mechanism of PRRs-mediated activation of NF-κB signaling pathway following influenza virus infection and summarized the influence of anti-influenza TCMs on inflammatory responses and the PRRs/NF-κB signaling pathway, so as to provide better understanding of the mode of action of TCMs in the treatment of influenza pneumonia.
1 Introduction
Influenza virus is one of the most common respiratory pathogens. According to the estimates of World Health Organization, about 1 billion people worldwide were infected with influenza virus each year, including 3 to 5 million serious cases and 290,000 to 650,000 deaths. The serious cases are often accompanied by viral lung disease, 10% of which even developed viral pneumonia (Cavallazzi and Ramirez, 2018; Riquelme et al., 2022). Previous studies showed that severe pneumonia induced by Influenza virus infection was closely associated with high morbidity and mortality, which posed a significant burden on public health and social development (Gu et al., 2019; Gu et al., 2021; Javanian et al., 2021).
One of the prominent characteristics of severe influenza pneumonia is the incidence of excessive inflammatory responses (Fukuyama and Kawaoka, 2011; Yang et al., 2017). This virus could be recognized by host pattern recognition receptors (PRRs) (Chen et al., 2018; Keilman, 2019), which promotes the activation of transcription factors such as the nuclear factor κB (NF-κB) and downstream production of pro-inflammatory cytokines and chemokines to resist viral infection (Oslund and Baumgarth, 2011; Pinto et al., 2011; Yu et al., 2020). When influenza virus multiplies in large quantities, it may lead to the continuous activation of these signaling pathways, resulting in the overexpression of the inflammatory factors, which would subsequently cause cytokine storm and severe viral pneumonia. More and more studies indicate the essential role of the NF-κB signaling pathway in the virus-induced overproduction of pro-inflammatory factors (Ludwig, 2009; Pandey et al., 2022). How to manipulate the PRR-mediated NF-κB signaling pathway is of great significance for anti-influenza research (Gao et al., 2021).
Traditional Chinese medicine (TCM) has been used for influenza treatment for thousands of years. In TCM theory, influenza was recognized as “plague” (infectious disease) or “current cold,” which was caused by the invasion of external pathogens or environmental changes. Several TCMs, such as Maxing Shigan Decoction, Lianhua Qingwen Capsule, and Jinhua Qinggan Granules, have been recommended by influenza diagnosis and treatment plan (2020 edition) issued by National Health Commission of the People’s Republic of China for clinical influenza treatment to relieve symptoms including chills, fever, cough, headache, and dyspnea (Wu et al., 2020). Compared to chemical drugs, TCMs have advantages including complex ingredients and are tolerant to drug resistance (Wang et al., 2006; Li J. H. et al., 2016; Ding et al., 2017; Zhao et al., 2021a). Further, TCMs have been reported to be involved in regulation of some PRR signaling pathway or inhibiting the nuclear translocation of NF-κB p65 and the expression of pro-inflammatory cytokines and chemokines induced by influenza virus infection. However, the mechanisms of TCMs in treating influenza viral pneumonia via regulating the PRR/NF-κB signaling pathway are unclear (Yang et al., 2022). Here, we reviewed the mechanism of influenza-induced TLRs or RIG-I-mediated activation of NF-κB pathway and summarized the effects of TCMs on PRR/NF-κB signaling pathway to provide support for TCM research of anti-influenza and immune regulation.
2 The occurrence of influenza pneumonia maybe related to the activation of PRRs-mediated NF-κB signaling pathway
2.1 Influenza virus recognition by toll-like receptors (TLRs)
The influenza viral infection could be recognized by different classes of PRRs. The first class of PRRs is toll-like receptors (TLRs). In humans, the family of TLRs consists of 10 members (TLR1-10) and all of them have a N-terminal leucine-rich repeats (LRR) domain which interacts with specific pathogen-associated molecular patterns (PAMPs), a transmembrane domain, and a toll/IL-1 receptor (TIR) domain which is responsible for recruitment of downstream signal adapter proteins (Gao et al., 2013; Kawasaki and Kawai, 2014). In response to ligand binding, TLRs form dimers which recruit particular adaptor proteins for the activation of downstream signaling. All TLRs except TLR3 initiate downstream signaling through the adapter protein myeloid differentiation primary response 88 gene (MyD88)-dependent pathway. For TLR4, it uses TIR domain containing adaptor protein (TIRAP) as additional adapter in this pathway. The activation of MyD88 leads to the phosphorylation of IL-1R-associated kinases 1 and 4 (IRAK1/4) which binds with TNF receptor-associated factor 6 (TRAF6) to mediate the activation of the transforming growth factor β-activated kinase 1 (TAK1)/TAK1-binding protein (TAB) complex, and then induces the translocation of transcription factors such as NF-κB to regulate the expression of inflammatory cytokine genes (Kawai and Akira, 2007; Jiang et al., 2020). Besides MyD88-dependent pathway, TLR3 recruits TIR-domain-containing adapter-inducing interferon-β (TRIF) and the endocytosed TLR4 recruits TRIF and TRIF-related adaptor molecule (TRAM), which activates TRIF-dependent pathway. The interaction between TRIF and TRAF6 recruits the receptor-interacting protein 1 (RIP1) and activates the TAK1/TAB complex that leads to the activation of NF-κB and downstream inflammatory responses. Alternatively, TRIF interacts with TRAF3 and recruits TANK (TRAF family member associated NF-κB activator)-binding kinase 1 (TBK1) and the inhibitor of NF-κB (IκB) kinase ε (IKKε), which drives the phosphorylation of the interferon regulatory factor 3 (IRF3) or IRF7 and downstream production of type I interferons (IFNs) (Kawai and Akira, 2007; Owen et al., 2020).
TLR3, TLR4, TLR7, and TLR8 have been reported to play a role in influenza virus infection. Continuous activation of TLR3 has been found both in patients that died in the 2009 pandemic and in the lungs of C57BL/6 mice infected with influenza virus, while the deletion of TLR3 in mice was associated with reduced chemokine expression and longer survival period (Le Goffic et al., 2006; Mauad et al., 2010). TLR4/MyD88 signaling was suggested to affect influenza virus infection because reduction of viral titers was detected in cells treated with an anti-TLR4 antibody or in MyD88 knockout cells (Marchant et al., 2010). Mice with TLR4 deficiency had increased survival from lethal infection of influenza virus and the administration of TLR4 antagonist eritoran reduced viral titers and cytokine expression and improved pulmonary pathological changes and pulmonary function (Shirey et al., 2013). The use of a TLR7/8 agonist inhibited influenza virus replication and induced IFN production both in vitro and in vivo (Hammerbeck et al., 2007). TLR7 recognizes ssRNA derived from influenza virus, whereas the relevance of TLR8 in this viral infection still needs more investigation (Iwasaki and Pillai, 2014).
2.2 Influenza virus recognition by RIG-I
Retinoic acid-inducible gene I (RIG-I) is another class of PRR detecting viruses within the cytosol of infected cells. It contains a pair of N-terminal caspase activation and recruitment domains (CARDs), the Helicase domain in which there are two RecA domains and an alpha-helical insertion domain (Hel2i), and a C-terminal domain. In resting cells, RIG-I locks in an autorepressed conformation by clamping the CARDs to the surface of Hel-2 (Thoresen et al., 2021). On detection of viral RNA, the Helicase domain of RIG-I binds to ATP, which promotes conformational changes allowing the CARDs to connect to mitochondrial antiviral signaling proteins (MAVS). MAVS then assembles with TRAF2/3/6, TBK1, and IKK complex to function as a signaling hub which drives the activation of IRF3, IRF7, and NF-κB and downstream production of IFNs and proinflammatory cytokines and chemokines (Iwasaki and Pillai, 2014; Thoresen et al., 2021).
In cells infected with influenza virus, RIG-I mainly recognizes viral nucleocapsids and viral genomic RNA bearing 5′-ppp (Rehwinkel et al., 2010). RIG-I initiates several anti-influenza defense mechanisms which include the production of type I IFNs, direct inhibition of virus replication, and promoting the assembly of an inflammasome complex to aid in viral clearance (Weber-Gerlach and Weber, 2016). It has been reported that the suppression of RIG-I pathway is common in children with severe influenza virus infection and is associated with increased risk of clinical complications (Novak et al., 2020). In addition, influenza virus has been suggested to disrupt RIG-I dependent signaling through MAVS that several subunits of RNA polymerase of this virus have an ability to translocate to the mitochondria and impair innate immunity (Weis and Te Velthuis, 2021).
2.3 The activation of NF-κB pathway and overproduction of pro-inflammatory factors following influenza virus infection
The NF-κB pathway has long been recognized as a prototypical proinflammatory signaling pathway. NF-κB is an evolutionarily conserved transcription factor. In mammals, there are five members in the NF-κB family, namely p50, p52, p65 (RelA), RelB, and c-Rel, of which p65 and p50 form a heterodimer and largely involve in the classical pathway of NF-κB activation. In the resting state, NF-κB p65:p50 dimers are sequestered in the cytoplasm in an inactive state by members of the IκB proteins. Once engaged with an activating signal, the IκB proteins are phosphorylated by IKK complex and this leads to ubiquitination and subsequent proteasomal degradation of the IκB, and then the NF-κB dimer is released and translocates into the nucleus so that to promote the transcription of pro-inflammatory genes such as TNF-α, IL-6, IL-8, IFN-β, and IL-1β (Mitchell et al., 2016; Mitchell and Carmody, 2018).
The NF-κB signaling pathway is continuously activated when influenza viruses multiply in large quantities, which leads to excess expression of pro-inflammatory genes (Wurzer et al., 2004; Vitiello et al., 2012; Ramos and Fernandez-Sesma, 2015; Pandey et al., 2022) (Figure 1). In patients with influenza pneumonia including 2009 H1N1, H1N1, H3N1, and H7N1, higher levels of proinflammatory cytokines and chemokines were detected, such as IL-6, IL-8, and TNFs (To et al., 2010; Xie et al., 2021). Overproduction of proinflammatory cytokines and chemokines induced by influenza virus infection has been suggested to relate to widespread tissue damage (Liu et al., 2016; Karki and Kanneganti, 2021). Based on the fact that the majority of immunoreceptors including TLRs and RIG-I control the expression of diverse inflammatory genes via the activation of NF-κB, it is highly possible that PRRs-mediated NF-κB activation plays a pathogenic role in influenza pneumonia.
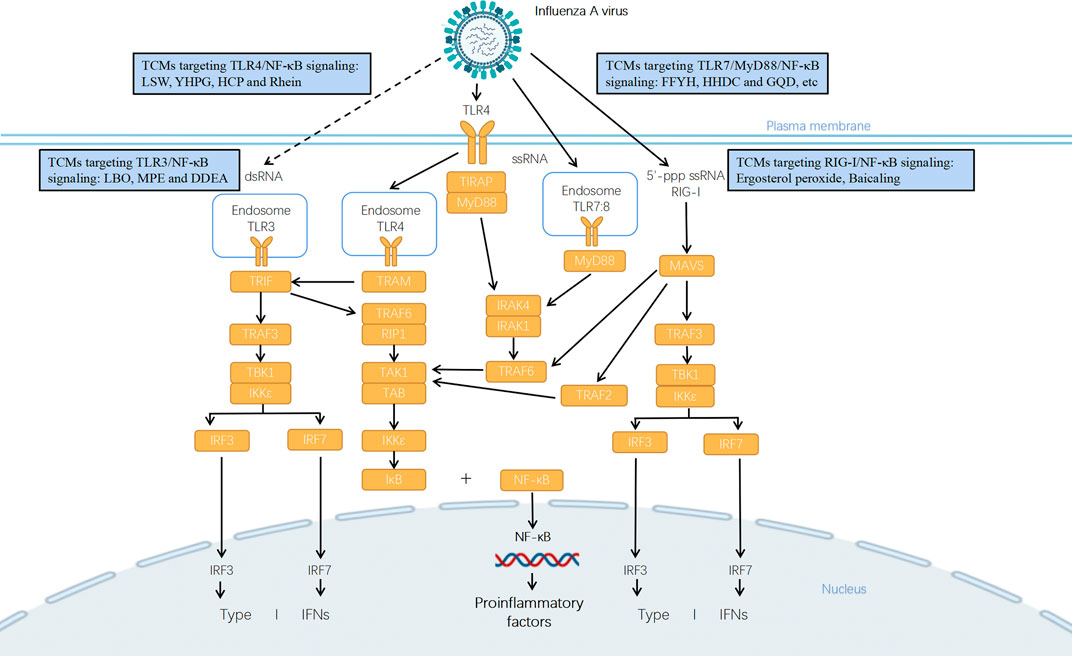
FIGURE 1. Potential mechanisms of anti-influenza TCMs in treatment of influenza pneumonia via TLRs/RIG-I signaling pathway. Influenza virus infection is detected by multiple PRRs such as TLRs and RIG-I. TLR3 recruits TIR-domain-containing adapter-inducing interferon-β (TRIF) and the endocytosed TLR4 recruits TRIF and TRIF-related adaptor molecule (TRAM), which activates TRIF-dependent pathway. The interaction between TRIF and TNF receptor-associated factor 6 (TRAF6) recruits the receptor-interacting protein 1 (RIP1) and activates the transforming growth factor β-activated kinase 1 (TAK1)/TAK1-binding protein (TAB) complex that leads to activation of NF-κB and downstream transcription of pro-inflammatory genes. Alternatively, TRIF interacts with TRAF3 and recruits TANK (TRAF family member associated NF-κB activator)-binding kinase 1 (TBK1) and the inhibitor of NF-κB (IκB) kinase ε (IKKε), which drives the phosphorylation of the interferon regulatory factor 3 (IRF3) or IRF7 and downstream production of type I interferons (IFNs). TLR3 recognizes dsRNA in endosomes, but it recognizes currently unknown RNA structures presenting in influenza virus-infected cells (presented in dotted line). TLR4, TLR7, and TLR8 initiate downstream signaling through the adapter protein myeloid differentiation primary response differentiation gene 88 (MyD88)-dependent pathway. For TLR4, it uses TIR domain containing adaptor protein (TIRAP) as additional adapter in this pathway. TLR7 recognizes the ssRNA genomes of influenza virus taken up into the endosome. The activation of MyD88 leads to the phosphorylation of IL-1R-associated kinases 1 and 4 (IRAK1/4) which binds with TRAF6 to mediate the activation of TAK1/TAB complex, and then induces the translocation of NF-κB to regulate the expression of cytokines and chemokines. RIG-I mainly recognizes viral nucleocapsids and viral genomic RNA bearing 5′-ppp. It connects to MAVS which then assembles with TRAF2/3/6, TBK1, and IKK complex to function as a signaling hub that drives the activation of IRF3, IRF7, and NF-κB and downstream production of IFNs and proinflammatory cytokines and chemokines. A variety of TCMs could regulate the expression of key components in TLRs/RIG-I signaling pathways so as to inhibit viral replication and virus-induced pro-inflammatory response.
3 The effects of TCMs on PRRs-mediated activation of NF-κB induced by influenza virus infection
The composition, effective dose, cell and animal models, virus strain, drug used as a positive control, antiviral and anti-inflammatory effects, and possible mechanism of TCMs and their ingredients against influenza virus infection and influenza pneumonia have been summarized in Figure 1 and Tables 1–3.
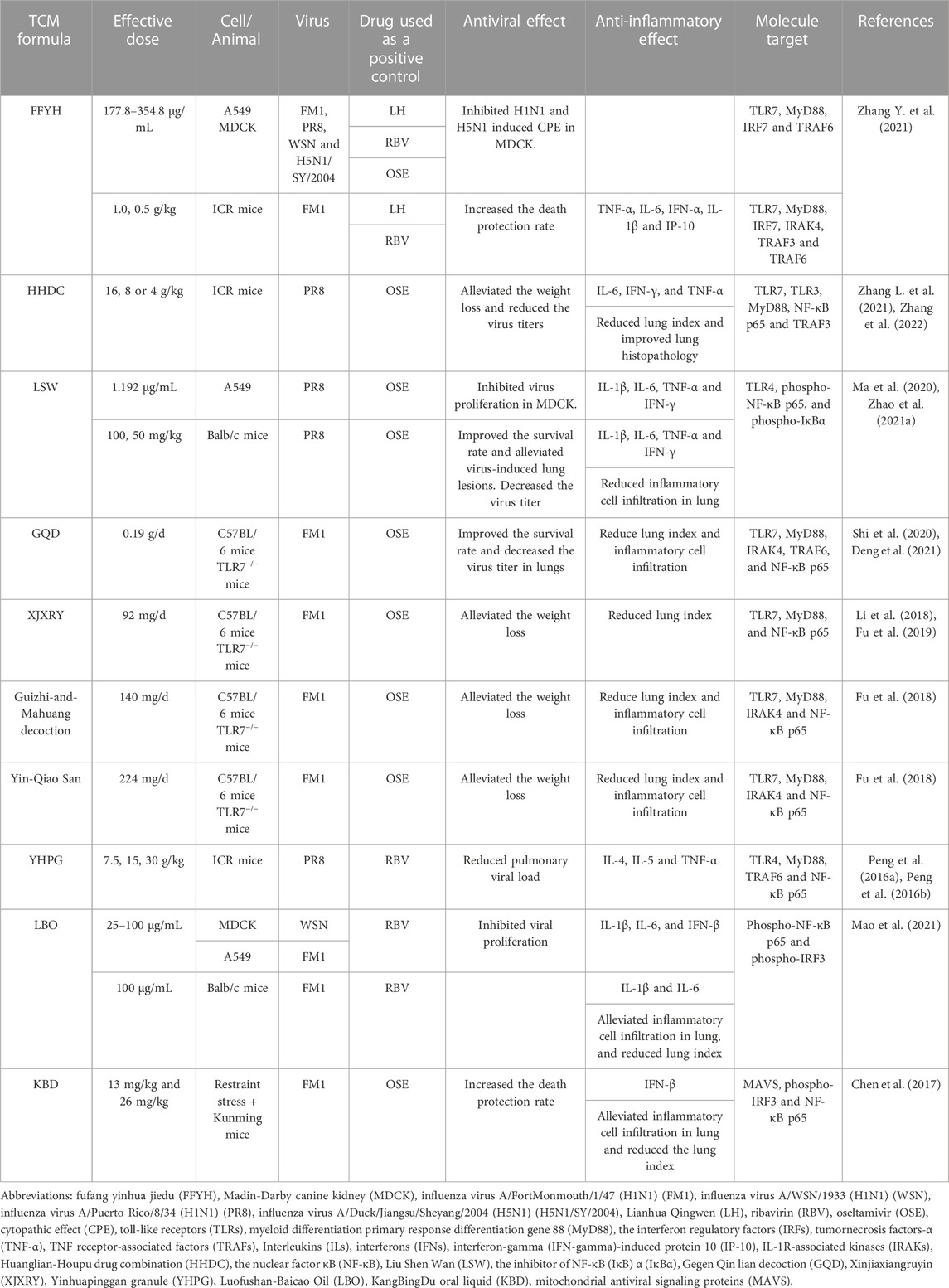
TABLE 1. The effects of TCM formulae on PRRs-mediated activation of NF-κB induced by influenza virus infection.
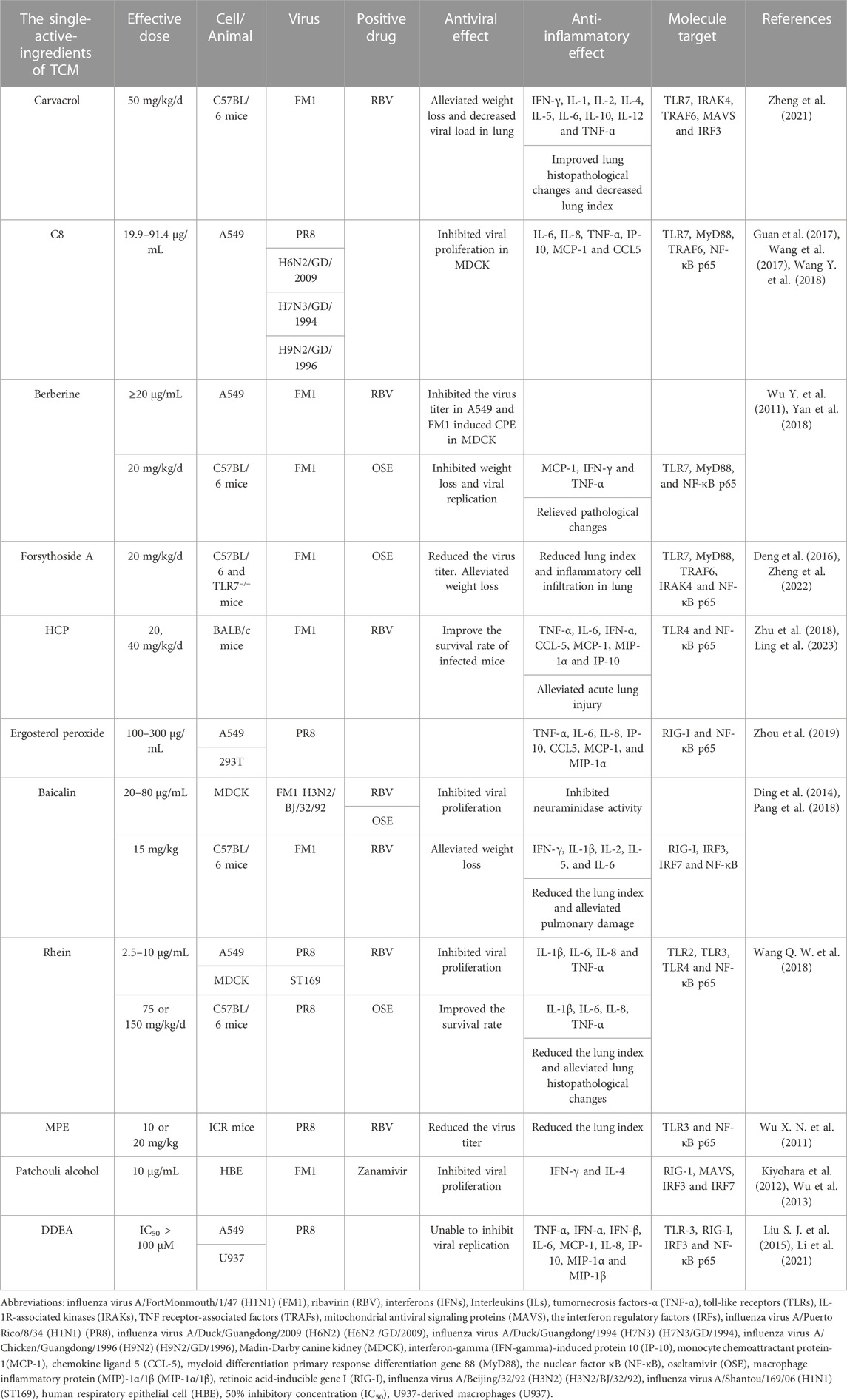
TABLE 2. The effects of single-active-ingredients of TCM on PRRs-mediated activation of NF-κB induced by influenza virus infection.
3.1 Traditional Chinese medicine formulas
3.1.1 Fufang yinhua jiedu (FFYH) granules
FFYH is a Chinese patent medicine optimized based on Yin-Qiao-San. It consists of Artemisia annua L., Lonicerae japonica Thunb., Nepeta tenuifolia Benth., Mentha canadensis L., Chrysanthemum indicum L., Isatis tinctoria L., Forsythia suspensa (Thunb.) Vahl., Commelina communis L., Glycine max (L.) Merr., and Peucedanum praeruptorum Dunn. FFYH exhibits antiviral activity against several influenza A viruses, such as H1N1, H3N2, H5N1, H7N9, and H9N2, through cytopathic effect inhibition assay and hemagglutination tests in vitro. ICR mice infected with influenza virus A/FortMonmouth/1/47 (H1N1) (FM1) showed wrinkled hair, reduced feed intake and weight loss, respiratory distress, and the serum levels of TNF-α, IL-6, IP-10, IFN-γ, and IL-1β were significantly elevated. FFYH treatment alleviated the above disease symptoms and acute lung injury, and significantly reduced the expression levels of serum TNF-α and other inflammatory factors in infected mice. Moreover, the upregulation of expression of TLR7, MyD88, IRF7, IRAK4, TRAF6, and TRAF3 induced by viral infection were significantly suppressed under FFYH treatment both in vitro and in vivo. The inhibition of ‘cytokine storm’ via the regulation of TLR7/MyD88 signaling pathway was suggested as a potential mechanism of FFYH against influenza pneumonia (Zhang Y. et al., 2021).
3.1.2 Huanglian-Houpu drug combination (HHDC)
HHDC is a botanical drug pair containing huanglian (Coptis chinensis Franch.) and houpu (the bark of Magnolia officinalis), which has a good effect on dysentery and gastrointestinal diseases (Luo et al., 2019; Wang et al., 2019; Zhang et al., 2022). The active ingredients in HHDC are berberine hydrochloride and magnolol via high-performance liquid chromatography (HPLC) analysis and these two components were suggested as the main anti-influenza components in HHDC. HHDC significantly reduced the viral load, pulmonary virus titer, and the level of inflammatory cell infiltration in the lung tissues of mice infected with influenza virus A/Puerto Rico/8/34 (H1N1) (PR8), as well as significantly reduced the serum levels of IL-6, IFN-γ, and TNF-α in a dose-dependent manner. The mRNA and protein expression levels of TLR7, TLR3, MyD88, NF-κB p65, and TRAF3 in the lungs of mice received high-dose HHDC treatment were significantly lower than those of the virus-infected group (Zhang L. et al., 2021). Therefore, HHDC may act against influenza pneumonia by regulating the TLR/MyD88/NF-κB signaling pathway.
3.1.3 Liu Shen Wan (LSW)
LSW is a classic Chinese patent medicine having anti-inflammatory and analgesic activities (Ma et al., 2007). The main ingredients of LSW are the gall-stone of Bos taurus domesticus Gmelin, the excretion of Moschus, the excretion of Venenum Bufonis, the shell of Pernulo, realgar, and borneol. It has been widely used for over a century to treat influenza, tonsillitis, and pharyngitis (Zhao et al., 2021b). In MDCK and A549 cells infected with PR8, LSW inhibited viral proliferation as well as significantly downregulated the expression levels of IL-1β, TNF-α, IL-6, and IFN-γ. In mice infected with PR8, LSW significantly improved the survival rate and reduced viral titers and the secretion of TNF-α, IL-1β, IL-6, and IFN-γ in pulmonary tissue. Moreover, LSW significantly reduced the expression level of TLR4, phospho-NF-κB p65, and phospho-IκBα but upregulated IκBα expression both in vivo and in vitro (Ma et al., 2020). It is suggested that LSW exhibited the antiviral and anti-inflammatory effects by regulating the TLR4/NF-κB signaling pathway.
3.1.4 Gegen Qinlian decoction (GQD)
GQD is composed of the root of Pueraria lobata (Willd.) Ohwi, Scutellaria baicalensis Georgi, C. chinensis Franch., and Glycyrrhiza glabra L. (Zhang et al., 2016; Deng et al., 2021). It has anti-bacterial and anti-inflammatory effects and is widely used in the treatment of fever and cough (Yu et al., 2005; Kwon et al., 2008; Nayak et al., 2014; Zhang et al., 2016; Deng et al., 2021). GQD can inhibit the proliferation of the FM1 strain, improve the survival rate, and significantly reduce the virus-induced mRNA expression levels of TLR7, MyD88, IRAK4, TRAF6, and NF-κB p65 in wild-type C57BL/6 mice. However, in TLR7-deficient mice, the treatment of GQD has no effect on the upregulated expression levels of MyD88 and NF-κB p65 induced by the virus infection. GQD has been proposed playing antiviral and immunomodulatory roles against influenza pneumonia by affecting the TLR7 signaling pathway (Shi et al., 2020).
3.1.5 Xinjiaxiangruyin (XJXRY)
XJXRY is often used in the clinical treatment of influenza in summer (Li et al., 2018). It consists of Mosla chinensis Maxim, L. japonica Thunb, Lablab purpureus, the bark of M. officinalis, and F. suspensa (Thunb.) Vahl. It effectively reduced lung inflammation and downregulated the mRNA and protein expression levels of TLR7, MyD88, and NF-κB p65 in C57BL/6 mice infected with FM1 in a hot and humid environment (Fu et al., 2019). It was suggested that XJXRY may reduce virus-induced lung injury by downregulating the expression levels of key factors in TLR7 signaling pathway.
3.1.6 Guizhi-and-Mahuang decoction
Guizhi-and-Mahuang decoction has anti-influenza, antipyretic, analgesic, anti-inflammatory, and anti-asthma activities (Du et al., 2013). It consists of Ephedra sinica Stapf, Neolitsea cassia, Prunus armeniaca, G. glabra L., Paeonia lactiflora Pall., Zingiber officinale Roscoe, and Ziziphus jujuba Mill. It was found that this decoction had a protective effect on FM1-infected mice by reducing the lung index and viral loads, as well as inhibiting the expression levels of TLR7, MyD88, IRAK4, and NF-κB p65. In TLR7-deficient mice, viral infection still induced the mRNA expression level of MyD88 and NF-κB but they were lower than those in wild type mice. Moreover, the mRNA expression levels of these genes were similar between viral infection group and Guizhi-and-Mahuang decoction treatment group (Fu et al., 2018). The regulation of TLR7/NF-κB signaling pathway by Guizhi-and-Mahuang decoction is likely to play an important role in the treatment of influenza-induced viral pneumonia.
3.1.7 Yin-Qiao San
Yin-Qiao San is a classic TCM with antipyretic and anti-inflammatory functions and used for prevention and control of viral infectious diseases (Zhang et al., 2019). It consists of F. suspensa (Thunb.) Vahl, L. japonica Thunb., Platycodon grandiflorus, M. canadensis L., Lophatherum gracile Brongn., G. glabra L., N. tenuifolia Benth., G. max (L.) Merr., Arctium lappa L., and Phragmites australis. In wild type mice, FM1 infection significantly upregulated the levels of TLR7, MyD88, IRAK4 and NF-κB while the treatment of Yin-Qiao San statistically reduced virus-induced expression of these genes. In TLR7-deficient mice, Yin-Qiao San failed to inhibit upregulation of NF-κB under viral infection. Yin-Qiao San may target some elements in the TLR7/MyD88/NF-κB pathway to achieve its therapeutic effects against influenza pneumonia (Fu et al., 2018).
3.1.8 Yinhuapinggan granule (YHPG)
YHPG is optimized from the classic ephedra decoction and can alleviate cough. It consists of the root of P. lobata (Willd.) Ohwi, L. japonica Thunb., Reynoutria japonica Houtt., E. sinica Stapf, P. armeniaca, and G. glabra L. (Jin et al., 2022). YHPG inhibited the proliferation of PR8 in MDCK cells. In addition, in PR8-infected ICR mice, YHPG alleviated the lung damage, decreased the expression levels of IL-4, IL-5, and TNF-α but upregulated the levels of IL-2 and IFN-γ in serum, and significantly downregulated the mRNA expression levels of TLR4, MyD88, TRAF6, and NF-κB p65 in the lungs. It is proposed that the potential mechanism of YHPG in treatment of influenza pneumonia is related to the inhibition of cytokine storm mediated by the TLR4/NF-κB signaling pathway (Peng et al., 2016a; Peng et al., 2016b).
3.1.9 Luofushan-baicao oil (LBO)
LBO is an essential oil-rich TCM formula and usually used to alleviate cold, headache, insect bites, and swelling (Hayashi et al., 2007; Wu et al., 2010; Li Y. et al., 2016; Mao et al., 2021). It consists of seventy-nine kinds of botanical drugs and related extractions, and the detailed composition of this formula was reported in the study by Mao et al. (Mao et al., 2021). LBO significantly inhibited the proliferation of influenza virus A/WSN/1933 (H1N1) (WSN) and virus titer in MDCK cells and reduced the expression of viral nuclear protein, IL-1β, IL-6. In a mouse model infected with FM1, inhalation of LBO aerosol reduced the lung damage and the lung index, and decreased the serum IL-1β and IL-6 levels. Further, in Poly I: C-stimulated A549 cells, the mRNA expression of IL-1β, IL-6, and IFN-β as well as the protein expression of NF-κB p65 and IRF3 were significantly lower in LBO treatment group. LBO has been suggested as an inhibitor of the TLR3/NF-κB signaling pathway to prevent influenza virus infection and balance immune function that it could be developed as a substitute medicinal agent for the prevention of influenza (Mao et al., 2021).
3.1.10 KangBingDu (KBD) oral liquid
KBD is a Chinese patent drug developed from the ‘Baihu Decoction’ and ‘Qingwen Baidu Yin’ and is widely used for treatment of viral infections (Li et al., 2022). It consists of I. tinctoria L., P. australis, Rehmannia glutinosa (Gaertn.) DC., Curcuma aromatica Salisb., Anemarrhena asphodeloides Bunge, Acorus calamus, Pogostemon cablin (Blanco) Benth., F. suspensa (Thunb.) Vahl, and gypsum fibrosum. It has been demonstrated that KBD inhibited viral loads, increased survival rate, and attenuated lung damage in FM1-infected Kunming mice. KBD treatment upregulated the protein levels of MAVS, phospho-IRF3, and downstream IFN-β. Meanwhile, the suppression of protein levels of NF-κB p65, TNF-α, and IL-1β were observed, whereas viral infection induced the expression of these proteins. It was proposed that KBD can alleviate lung injury and exert anti-influenza effect through the impact on the activation of the MAVS/NF-κB signaling pathway (Chen et al., 2017).
3.2 The single-active-ingredients of TCMs
3.2.1 Carvacrol
Carvacrol is one of the main components of M. chinensis Maxim, which has analgesic, anti-inflammatory, antiviral, insecticidal, and antioxidant effects (Suntres et al., 2015). It was demonstrated that carvacrol significantly reduced the lung index and the expression levels of IFN-γ, IL-2, IL-4, IL-5, and IL-10 in the lung tissue of FM1-infected C57BL/6 mice. Furthermore, following the treatment with carvacrol, the mRNA expression levels of TLR7, MyD88, IRAK4, NF-κB p65, MAVS, and IRF3 were decreased, and the protein expression levels of RIG-I, MyD88, and NF-κB were significantly downregulated. It has been suggested that carvacrol may regulate excessive secretion of inflammatory factors via inhibiting both TLR and RIG-I signaling pathways which were activated under influenza virus infection so as to reduce lung damage resulting from viral infection (Zheng et al., 2021).
3.2.2 C8
The compound C8 was isolated from the botanical drug choulingdan (Laggera crispata), which is often used to treat diseases such as pharyngitis and stomatitis (Wu et al., 2006; Shi et al., 2007; Wang et al., 2017). The main content of C8 includes 107.98 mg/g odontodanic acid and 111.3 mg/g odontodandiol. C8 showed broad-spectrum anti-influenza activity that can inhibit replication of H1N1, H6N2, H7N3, and H7N9 influenza viruses in MDCK cells (Guan et al., 2017; Wang et al., 2017). It significantly inhibited the mRNA expression levels of IL-1β, IL-6, IL-8, and MCP-1, as well as the protein levels of TLR7, MyD88, and TRAF6 and the nuclear translocation of NF-κB p65 in PR8-infected A549 cells. C8 is expected to target the TLR7/NF-κB signaling pathway to interfere with the infection of influenza virus (Wang Y. et al., 2018).
3.2.3 Berberine
Berberine is an isoquinoline alkaloid isolated from a Chinese botanical drug huanglian (C. chinensis Franch.) and has anti-hypertensive (Guo et al., 2015), anti-inflammatory (Liu S. J. et al., 2015) and antiviral effects (Kuo et al., 2004; Shin et al., 2015). The anti-influenza activity of berberine was studied using A549 cells infected with FM1 virus, and the results showed that this drug inhibited viral proliferation in a time- and dose-dependent manner. In C57BL/6 mice, viral infection induced tissue damage and the expression of IL-4, TNF-α, and IFN-γ in the lungs, which were alleviated following the treatment of berberine. Moreover, the mRNA and protein expression levels of TLR7, MyD88, and NF-κB p65 in the lungs of mice were significantly increased after viral infection but significantly downregulated in berberine treatment group. However, further investigations are needed to see whether berberine has a direct effect on the above signaling pathways (Wu Y. et al., 2011; Yan et al., 2018).
3.2.4 Forsythiaside A
Lianqiao (F. suspensa (Thunb.) Vahl) is a well-known Chinese botanical drug and plays an important role in treating fever, inflammation, ulcers, and gonorrhea (Qu et al., 2008). So far, over 300 ingredients have been extracted from lianqiao (F. suspensa (Thunb.) Vahl). Among them, the main bioactive index component is forsythiaside A, which has anti-bacterial, antioxidant, and antiviral activities (Deng et al., 2016; Zeng et al., 2017; Zhou et al., 2022). In FM1-infected C57BL/6 mice, forsythiaside A reversed weight loss and alleviated lung damage and significantly reduced the mRNA and protein expression levels of TLR7, MyD88, and NF-κB p65 in the lung tissues. However, the mRNA and protein expression levels of MyD88 and NF-κB p65 in the lung tissues of TLR7-deficient mice infected with FM1 were similar between the forsythiaside A treatment group and the viral infection group. Forsythiaside A was indicated to interfere with the activation of the TLR7 signaling pathway to influence the inflammatory response and the subsequent lung injury caused by viral infection (Zheng et al., 2022).
3.2.5 Polysaccharides isolated from Houttuynia cordata thunb (HCP)
Yuxingcao (H. cordata Thunb.) is an important botanical drug with antiviral, antibacterial, anti-inflammatory, and antioxidant activities (Li et al., 2013; Cheng et al., 2014; Ling et al., 2023). HCP, one of the active components of this botanical drug, alleviated pulmonary and intestinal damages in BALB/c mice infected with FM1, and improved the survival rate of the infected mice. Moreover, HCP not only inhibited excessive release of TNF-α, IL-6, and IFN-α but also suppressed the upregulation of TLR4 and NF-κB protein expression in the lung tissues of infected mice. It is possible that HCP acts against influenza virus infection by inhibiting the activation of the TLR4/NF-κB signaling pathway (Zhu et al., 2018).
3.2.6 Ergosterol peroxide
Ergosterol peroxide isolated from the root of nanbanlangen (Strobilanthes cusia (Nees) Kuntze) has been proved to have anti-tumor, antioxidant, and antibacterial effects (Ramos-Ligonio et al., 2012; Wu et al., 2012; Kang et al., 2015). It was demonstrated that in A549 cells infected with PR8 strain, ergosterol peroxide inhibited the overexpression of pro-inflammatory cytokines, including TNF-α, IL-6, IL-8, IP-10, CCL5, MCP-1, and MIP-1α. Meanwhile, this drug significantly downregulated the mRNA and protein expression levels of RIG-I in PR8-infected cells. The transcriptional activity of NF-κB stimulated by PR8 virus or by TNF-α treatment was significantly lower following ergosterol peroxide treatment. It has been speculated that the anti-inflammation activity of ergosterol peroxide from banlangen under influenza virus infection is mediated via inhibition of the obstructing RIG-I signaling pathway (Zhou et al., 2019).
3.2.7 Baicalin
Baicalin is a flavonoid isolated from the root of a botanical drug huangqin (S. baicalensis Georgi), and it has been used for treating cough (Ding et al., 2014; Zhu et al., 2016). It was demonstrated that baicalin reduced the lung index, and pulmonary damage, and the secretion of IFN-γ, IL-1β, IL-2, IL-5, and IL-6 in the lung tissues of FM1-infected C57BL/6 mice. Meanwhile, the transcriptional and translational levels of RIG-I, IRF3, IRF7, and NF-κB in the lung tissues were significantly increased in response to viral infection, while the treatment of baicalin significantly decreased the expression and production of these genes. The effects of baicalin on several factors in RIG-I signaling pathway highlighted the advantages of TCM in regulating host immunity (Pang et al., 2018).
3.2.8 Rhein
Rhein is extensively found in several TCMs, including Rheum palmatum L., Aloe barbadensis Miller, Cassia angustifolia Vahl., and Polygonum multiflorum Thunb. It has antioxidant, antiviral, anti-inflammatory, anti-tumor, and immunomodulatory activities (Sun et al., 2016). A recent study has shown that rhein significantly inhibited the proliferation of influenza virus A/ShanTou/169/06 (H1N1) (ST169) in MDCK cells and reduced the expression levels of IL-1β, IL-6, IL-8, and TNF-α in PR8-infected A549 cells. In mice infected with PR8, rhein significantly improved the survival rate and reduced the lung index and pulmonary expression levels of IL-1β, IL-6, IL-8, TNF-α. Further, rhein significantly reduced the protein levels of TLR2, TLR3, and TLR4, as well as the phosphorylation of NF-κB p65 in PR8-infected A549 cells. The addition of activators for TLR4 and NF-κB could antagonize the inhibitory effect of rhein on virus replication (Wang Q. W. et al., 2018). Thus, the anti-influenza effect of rhein is likely related to inhibiting the activation of TLRs/NF-κB signaling pathway which may also alleviate the production of pro-inflammatory cytokines.
3.2.9 Polyphenol extract from the bark of houpu (MPE)
MPE is a polyphenol compound extracted from the bark of houpu (the bark of M. officinalis) (Sarrica et al., 2018). It was reported that MPE reduced the mortality rate and the lung index, improved inflammatory changes of lung tissues, and decreased the serum levels of TNF-α and IL-6 in a dose-dependent manner in PR8-infected ICR mice. Further, MPE significantly reduced the protein level of TLR3 and phospho-NF-κB p65 in the lung tissues. It is proposed that MPE may play a role in regulating the immune response and alleviating viral pneumonia by inhibition of the activation of TLR3/NF-κB signaling pathway (Wu X. N. et al., 2011).
3.2.10 Patchouli alcohol
Patchouli alcohol is the main component of guanghuoxiang (P. cablin (Blanco) Benth.) which is a botanical drug (Kiyohara et al., 2012). Following FM1 virus infection, patchouli alcohol inhibited the expression of pro-inflammatory cytokines such as IFN-γ and IL-4 in HBE cells, and significantly downregulated the mRNA expression levels of RIG-I, MAVS, IRF3, and IRF7 in dendritic cells and macrophages (Wu et al., 2013). It has been suggested that patchouli alcohol plays antiviral and anti-inflammatory roles via regulating the innate immune response. However, the detailed effects on the virus and these immune factors are needed to verify this speculation.
3.2.11 (2Z,4E)-deca-2,4-dienoic acid (DDEA)
Wild dongchongxiacao (cordyceps) mainly consists of the stroma of the fungus Cordyceps sinensis (Berk.) Sacc. and the dead caterpillar of Hepialus armoricanus, which is a rare TCM with immunomodulatory activity (Ng and Wang, 2005; Paterson, 2008; Liu Y. et al., 2015). DDEA was a novel fatty acid identified in Ophiocordyceps sinensis which has been cultured as an alternative of the wild dongchongxiacao. In A549 cells infected with PR8, the chemically synthesized DDEA could not inhibit viral proliferation but inhibited the mRNA and protein expression of TNF-α, IFN-α, IFN-β, IL-6, IL-8, MCP-1, IP-10, and MIP-1β. Moreover, DDEA significantly reduced the protein expression levels of TLR3, RIG-I, phospho-IRF3, and phospho-NF-κB p65 in infected cells. It is suggested that DDEA may regulate the immune function by regulating TLR3 and RIG-I-dependent signaling pathways (Li et al., 2021).
4 Conclusion
TCMs are derived from natural plants and have advantages such as wide clinical applications and negligible side effects. Here we reviewed the research progress of the mechanism of different sorts of TCMs against influenza, including prescriptions, the Chinese patent drugs, and active ingredient. A variety of TCMs can inhibit the replication of influenza viruses and improve the symptoms of infected mice. However, for many TCMs, it is still unclear on which stage in the viral replication cycle they act. The investigation of the mode of action of TCMs would provide hints for their clinical use. Besides the antiviral effects, TCMs exhibit immune modulatory effects including regulating the expression of PRRs such as TLR3, TLR4, TLR7, RIG-I, and MAVS, and the key proteins of the NF-κB signaling pathway and the inhibition of the pro-inflammatory response. Interestingly, TLR7/MyD88/NF-κB signaling pathway was more frequently reported to be interfered by several formulae and active ingredients of TCMs. Although several studies demonstrated that the virus replication triggers the activation of inflammatory responses, whether the decrease in PRR expression and NF-κB activation is secondary to a decrease in viral burden was less analyzed. In addition, it has not yet been conclusively demonstrated whether the drugs inhibit the pro-inflammatory response by affecting key proteins of these signaling pathways and thus inhibit the occurrence of viral pneumonia. Some of the proinflammatory factors directly induced by virus infection could further induce a second wave of cytokines, which complexes the immune responses induced by viral infection. Recent studies have begun to recognize the multifaceted roles of cytokines during influenza infection, but the function and place of immune effectors in the immune network are still needed to be defined (Guo and Thomas, 2017). To provide support for research of anti-influenza drugs, the relationship between virus and signaling elements should be further investigated. The current studies mainly focus on the effects of TCMs against influenza A virus, while the activity of these drugs against influenza B virus is less reported. It should be noted that the immunomodulatory role of drugs has a wide array of potential uses and TCMs have the advantage of providing complementary treatment for new emerging diseases. Therefore, TCM targeting PRRs might also yield some benefit to other illnesses related to host immunopathology and acute lung injury beyond Influenza. In a word, the detailed roles of PRR-mediated NF-κB signaling pathways in regulating the inflammatory responses and the efficient pharmacodynamic substances should be further investigated while retaining the characteristics of TCMs, which will help promote global recognition of TCMs.
Author contributions
SD and XX contributed to revising the manuscript. SD, YL, and ZZ provided financial support. LZ and XY wrote the manuscript.
Funding
This study is supported by Guangdong Provincial Key Laboratory of Research on Emergency in TCM Open Subject (9344); National Key Research and Development Program (2022YFC0867400); Major special projects of Yunnan Provincial Science and Technology Department (202202AG050013).
Conflict of interest
The authors declare that the research was conducted in the absence of any commercial or financial relationships that could be construed as a potential conflict of interest.
Publisher’s note
All claims expressed in this article are solely those of the authors and do not necessarily represent those of their affiliated organizations, or those of the publisher, the editors and the reviewers. Any product that may be evaluated in this article, or claim that may be made by its manufacturer, is not guaranteed or endorsed by the publisher.
References
Cavallazzi, R., and Ramirez, J. A. (2018). Influenza and viral pneumonia. Clin. Chest Med. 39, 703–721. doi:10.1016/j.ccm.2018.07.005
Chen, H., Jie, C., Tang, L. P., Meng, H., Li, X. B., Li, Y. B., et al. (2017). New insights into the effects and mechanism of a classic traditional Chinese medicinal formula on influenza prevention. Phytomedicine 27, 52–62. doi:10.1016/j.phymed.2017.02.004
Chen, X., Liu, S., Goraya, M. U., Maarouf, M., Huang, S., and Chen, J. L. (2018). Host immune response to influenza A virus infection. Front. Immunol. 9, 320. doi:10.3389/fimmu.2018.00320
Cheng, B. H., Chan, J. Y., Chan, B. C., Lin, H. Q., Han, X. Q., Zhou, X., et al. (2014). Structural characterization and immunomodulatory effect of a polysaccharide HCP-2 from Houttuynia cordata. Carbohydr. Polym. 103, 244–249. doi:10.1016/j.carbpol.2013.12.048
Deng, L., Pang, P., Zheng, K., Nie, J., Xu, H., Wu, S., et al. (2016). Forsythoside A controls influenza A virus infection and improves the prognosis by inhibiting virus replication in mice. Molecules 21, 524. doi:10.3390/molecules21050524
Deng, L., Shi, Y., Liu, P., Wu, S., Lv, Y., Xu, H., et al. (2021). GeGen QinLian decoction alleviate influenza virus infectious pneumonia through intestinal flora. Biomed. Pharmacother. 141, 111896. doi:10.1016/j.biopha.2021.111896
Ding, Y., Dou, J., Teng, Z., Yu, J., Wang, T., Lu, N., et al. (2014). Antiviral activity of baicalin against influenza A (H1N1/H3N2) virus in cell culture and in mice and its inhibition of neuraminidase. Arch. Virol. 159, 3269–3278. doi:10.1007/s00705-014-2192-2
Ding, Y., Zeng, L., Li, R., Chen, Q., Zhou, B., Chen, Q., et al. (2017). The Chinese prescription lianhuaqingwen capsule exerts anti-influenza activity through the inhibition of viral propagation and impacts immune function. BMC Complement. Altern. Med. 17, 130. doi:10.1186/s12906-017-1585-7
Du, X. L., Sui, F., Huo, H. R., Zhang, H. W., Luo, K., Li, L. F., et al. (2013). Reciprocal effects of Guizhi decoction to the Guizhi decoction syndrome by toll-like receptor mRNA expression and cytokines secretion. Chin. J. Integr. Med. 19, 826–835. doi:10.1007/s11655-013-1325-2
Fu, Y. J., Yan, Y. Q., Qin, H. Q., Wu, S., Shi, S. S., Zheng, X., et al. (2018). Effects of different principles of Traditional Chinese Medicine treatment on TLR7/NF-κB signaling pathway in influenza virus infected mice. Chin. Med. 13, 42. doi:10.1186/s13020-018-0199-4
Fu, Y. J., Yan, Y. Q., Zheng, X., Shi, S. S., Wu, S., and Jiang, Z. Y. (2019). Effects of Xinjiaxiangruyin on the TLR7 pathway in influenza virus-infected lungs of mice housed in a hygrothermal environment. Chin. Med. 14, 39. doi:10.1186/s13020-019-0256-7
Fukuyama, S., and Kawaoka, Y. (2011). The pathogenesis of influenza virus infections: The contributions of virus and host factors. Curr. Opin. Immunol. 23, 481–486. doi:10.1016/j.coi.2011.07.016
Gao, R., Bhatnagar, J., Blau, D. M., Greer, P., Rollin, D. C., Denison, A. M., et al. (2013). Cytokine and chemokine profiles in lung tissues from fatal cases of 2009 pandemic influenza A (H1N1): Role of the host immune response in pathogenesis. Am. J. Pathol. 183, 1258–1268. doi:10.1016/j.ajpath.2013.06.023
Gao, X., Chan, P. K. S., Lui, G. C. Y., Hui, D. S. C., Chu, I. M., Sun, X., et al. (2021). Interleukin-38 ameliorates poly(I:C) induced lung inflammation: Therapeutic implications in respiratory viral infections. Cell Death Dis. 12, 53. doi:10.1038/s41419-020-03283-2
Gu, Y., Hsu, A. C., Pang, Z., Pan, H., Zuo, X., Wang, G., et al. (2019). Role of the innate cytokine storm induced by the influenza A virus. Viral Immunol. 32, 244–251. doi:10.1089/vim.2019.0032
Gu, Y., Zuo, X., Zhang, S., Ouyang, Z., Jiang, S., Wang, F., et al. (2021). The mechanism behind influenza virus cytokine storm. Viruses 13, 1362. doi:10.3390/v13071362
Guan, W., Li, J., Chen, Q., Jiang, Z., Zhang, R., Wang, X., et al. (2017). Pterodontic acid isolated from laggera pterodonta inhibits viral replication and inflammation induced by influenza A virus. Molecules 22, 1738. doi:10.3390/molecules22101738
Guo, X. J., and Thomas, P. G. (2017). New fronts emerge in the influenza cytokine storm. Semin. Immunopathol. 39, 541–550. doi:10.1007/s00281-017-0636-y
Guo, Z., Sun, H., Zhang, H., and Zhang, Y. (2015). Anti-hypertensive and renoprotective effects of berberine in spontaneously hypertensive rats. Clin. Exp. Hypertens. 37, 332–339. doi:10.3109/10641963.2014.972560
Hammerbeck, D. M., Burleson, G. R., Schuller, C. J., Vasilakos, J. P., Tomai, M., Egging, E., et al. (2007). Administration of a dual toll-like receptor 7 and toll-like receptor 8 agonist protects against influenza in rats. Antivir. Res. 73, 1–11. doi:10.1016/j.antiviral.2006.07.011
Hayashi, K., Imanishi, N., Kashiwayama, Y., Kawano, A., Terasawa, K., Shimada, Y., et al. (2007). Inhibitory effect of cinnamaldehyde, derived from Cinnamomi cortex, on the growth of influenza A/PR/8 virus in vitro and in vivo. Antivir. Res. 74, 1–8. doi:10.1016/j.antiviral.2007.01.003
Iwasaki, A., and Pillai, P. S. (2014). Innate immunity to influenza virus infection. Nat. Rev. Immunol. 14, 315–328. doi:10.1038/nri3665
Javanian, M., Barary, M., Ghebrehewet, S., Koppolu, V., Vasigala, V., and Ebrahimpour, S. (2021). A brief review of influenza virus infection. J. Med. Virol. 93, 4638–4646. doi:10.1002/jmv.26990
Jiang, G., Gong, M., Song, H., Sun, W., Zhao, W., and Wang, L. (2020). Tob2 inhibits TLR-induced inflammatory responses by association with TRAF6 and MyD88. J. Immunol. 205, 981–986. doi:10.4049/jimmunol.2000057
Jin, L., Zhang, Y., Yang, J., Zhou, H., Jia, G., He, Y., et al. (2022). Investigation of pharmacological mechanisms of yinhua pinggan granule on the treatment of pneumonia through network pharmacology and in vitro. Biomed. Res. Int. 2022, 1602447. doi:10.1155/2022/1602447
Kang, J. H., Jang, J. E., Mishra, S. K., Lee, H. J., Nho, C. W., Shin, D., et al. (2015). Ergosterol peroxide from Chaga mushroom (Inonotus obliquus) exhibits anti-cancer activity by down-regulation of the β-catenin pathway in colorectal cancer. J. Ethnopharmacol. 173, 303–312. doi:10.1016/j.jep.2015.07.030
Karki, R., and Kanneganti, T. D. (2021). The 'cytokine storm': Molecular mechanisms and therapeutic prospects. Trends Immunol. 42, 681–705. doi:10.1016/j.it.2021.06.001
Kawai, T., and Akira, S. (2007). TLR signaling. Semin. Immunol. 19, 24–32. doi:10.1016/j.smim.2006.12.004
Kawasaki, T., and Kawai, T. (2014). Toll-like receptor signaling pathways. Front. Immunol. 5, 461. doi:10.3389/fimmu.2014.00461
Keilman, L. J. (2019). Seasonal influenza (flu). Nurs. Clin. North Am. 54, 227–243. doi:10.1016/j.cnur.2019.02.009
Kiyohara, H., Ichino, C., Kawamura, Y., Nagai, T., Sato, N., and Yamada, H. (2012). Patchouli alcohol: In vitro direct anti-influenza virus sesquiterpene in Pogostemon cablin Benth. J. Nat. Med. 66, 55–61. doi:10.1007/s11418-011-0550-x
Kuo, C. L., Chi, C. W., and Liu, T. Y. (2004). The anti-inflammatory potential of berberine in vitro and in vivo. Cancer Lett. 203, 127–137. doi:10.1016/j.canlet.2003.09.002
Kwon, H. A., Kwon, Y. J., Kwon, D. Y., and Lee, J. H. (2008). Evaluation of antibacterial effects of a combination of coptidis rhizoma, mume fructus, and schizandrae fructus against Salmonella. Int. J. Food Microbiol. 127, 180–183. doi:10.1016/j.ijfoodmicro.2008.06.020
Le Goffic, R., Balloy, V., Lagranderie, M., Alexopoulou, L., Escriou, N., Flavell, R., et al. (2006). Detrimental contribution of the Toll-like receptor (TLR)3 to influenza A virus-induced acute pneumonia. PLoS Pathog. 2, e53. doi:10.1371/journal.ppat.0020053
Li, H., Ai, Y., Zhao, T., Zhang, D., Lv, X., Jia, S., et al. (2022). Postmarketing reevaluation of Chinese traditional therapy kangbingdu oral liquid in the treatment of the common cold. Evid. Based Complement. Altern. Med. 2022, 9968171. doi:10.1155/2022/9968171
Li, J. H., Wang, R. Q., Guo, W. J., and Li, J. S. (2016). Efficacy and safety of traditional Chinese medicine for the treatment of influenza A (H1N1): A meta-analysis. J. Chin. Med. Assoc. 79, 281–291. doi:10.1016/j.jcma.2015.10.009
Li, Q., Pang, P., Zheng, K., Sun, L., Wang, J., and Chen, X. (2018). Xin-Jia-Xiang-Ru-Yin alleviated H1N1-induced acute lung injury and inhibited the IFN-γ-related regulatory pathway in summer flu. Biomed. Pharmacother. 108, 201–207. doi:10.1016/j.biopha.2018.09.022
Li, R. F., Zhou, X. B., Zhou, H. X., Yang, Z. F., Jiang, H. M., Wu, X., et al. (2021). Novel fatty acid in cordyceps suppresses influenza A (H1N1) virus-induced proinflammatory response through regulating innate signaling pathways. ACS Omega 6, 1505–1515. doi:10.1021/acsomega.0c05264
Li, W., Fan, T., Zhang, Y., Fan, T., Zhou, P., Niu, X., et al. (2013). Houttuynia cordata Thunb. volatile oil exhibited anti-inflammatory effects in vivo and inhibited nitric oxide and tumor necrosis factor-α production in LPS-stimulated mouse peritoneal macrophages in vitro. Phytother. Res. 27, 1629–1639. doi:10.1002/ptr.4905
Li, Y., Lai, Y., Wang, Y., Liu, N., Zhang, F., and Xu, P. (2016). 1, 8-cineol protect against influenza-virus-induced pneumonia in mice. Inflammation 39, 1582–1593. doi:10.1007/s10753-016-0394-3
Ling, L., Ren, A., Lu, Y., Zhang, Y., Zhu, H., Tu, P., et al. (2023). The synergistic effect and mechanisms of flavonoids and polysaccharides from Houttuynia cordata on H1N1-induced pneumonia in mice. J. Ethnopharmacol. 302, 115761. doi:10.1016/j.jep.2022.115761
Liu, Q., Zhou, Y. H., and Yang, Z. Q. (2016). The cytokine storm of severe influenza and development of immunomodulatory therapy. Cell Mol. Immunol. 13, 3–10. doi:10.1038/cmi.2015.74
Liu, S. J., Yin, C. X., Ding, M. C., Wang, Y. Z., and Wang, H. (2015). Berberine inhibits tumor necrosis factor-α-induced expression of inflammatory molecules and activation of nuclear factor-κB via the activation of AMPK in vascular endothelial cells. Mol. Med. Rep. 12, 5580–5586. doi:10.3892/mmr.2015.4061
Liu, Y., Wang, J., Wang, W., Zhang, H., Zhang, X., and Han, C. (2015). The chemical constituents and pharmacological actions of cordyceps sinensis. Evid. Based Complement. Altern. Med. 2015, 575063. doi:10.1155/2015/575063
Ludwig, S. (2009). Targeting cell signalling pathways to fight the flu: Towards a paradigm change in anti-influenza therapy. J. Antimicrob. Chemother. 64, 1–4. doi:10.1093/jac/dkp161
Luo, H., Wu, H., Yu, X., Zhang, X., Lu, Y., Fan, J., et al. (2019). A review of the phytochemistry and pharmacological activities of Magnoliae officinalis cortex. J. Ethnopharmacol. 236, 412–442. doi:10.1016/j.jep.2019.02.041
Ma, H. Y., Kou, J. P., Wang, J. R., and Yu, B. Y. (2007). Evaluation of the anti-inflammatory and analgesic activities of Liu-Shen-Wan and its individual fractions. J. Ethnopharmacol. 112, 108–114. doi:10.1016/j.jep.2007.02.008
Ma, Q., Huang, W., Zhao, J., and Yang, Z. (2020). Liu Shen Wan inhibits influenza a virus and excessive virus-induced inflammatory response via suppression of TLR4/NF-κB signaling pathway in vitro and in vivo. J. Ethnopharmacol. 252, 112584. doi:10.1016/j.jep.2020.112584
Mao, X., Gu, S., Sang, H., Ye, Y., Li, J., Teng, Y., et al. (2021). Essential oil-rich Chinese formula luofushan-baicao oil inhibits the infection of influenza A virus through the regulation of NF-κB P65 and IRF3 activation. Evid. Based Complement. Altern. Med. 2021, 5547424. doi:10.1155/2021/5547424
Marchant, D., Singhera, G. K., Utokaparch, S., Hackett, T. L., Boyd, J. H., Luo, Z., et al. (2010). Toll-like receptor 4-mediated activation of p38 mitogen-activated protein kinase is a determinant of respiratory virus entry and tropism. J. Virol. 84, 11359–11373. doi:10.1128/JVI.00804-10
Mauad, T., Hajjar, L. A., Callegari, G. D., Da Silva, L. F., Schout, D., Galas, F. R., et al. (2010). Lung pathology in fatal novel human influenza A (H1N1) infection. Am. J. Respir. Crit. Care Med. 181, 72–79. doi:10.1164/rccm.200909-1420OC
Mitchell, J. P., and Carmody, R. J. (2018). NF-κB and the transcriptional control of inflammation. Int. Rev. Cell Mol. Biol. 335, 41–84. doi:10.1016/bs.ircmb.2017.07.007
Mitchell, S., Vargas, J., and Hoffmann, A. (2016). Signaling via the NFκB system. Wiley Interdiscip. Rev. Syst. Biol. Med. 8, 227–241. doi:10.1002/wsbm.1331
Nayak, M. K., Agrawal, A. S., Bose, S., Naskar, S., Bhowmick, R., Chakrabarti, S., et al. (2014). Antiviral activity of baicalin against influenza virus H1N1-pdm09 is due to modulation of NS1-mediated cellular innate immune responses. J. Antimicrob. Chemother. 69, 1298–1310. doi:10.1093/jac/dkt534
Ng, T. B., and Wang, H. X. (2005). Pharmacological actions of Cordyceps, a prized folk medicine. J. Pharm. Pharmacol. 57, 1509–1519. doi:10.1211/jpp.57.12.0001
Novak, T., Hall, M. W., Mcdonald, D. R., Newhams, M. M., Mistry, A. J., Panoskaltsis-Mortari, A., et al. (2020). RIG-I and TLR4 responses and adverse outcomes in pediatric influenza-related critical illness. J. Allergy Clin. Immunol. 145, 1673–1680.e11. doi:10.1016/j.jaci.2020.01.040
Oslund, K. L., and Baumgarth, N. (2011). Influenza-induced innate immunity: Regulators of viral replication, respiratory tract pathology & adaptive immunity. Future Virol. 6, 951–962. doi:10.2217/fvl.11.63
Owen, A. M., Fults, J. B., Patil, N. K., Hernandez, A., and Bohannon, J. K. (2020). TLR agonists as mediators of trained immunity: Mechanistic insight and immunotherapeutic potential to combat infection. Front. Immunol. 11, 622614. doi:10.3389/fimmu.2020.622614
Pandey, P., Al Rumaih, Z., Kels, M. J. T., Ng, E., Kc, R., Chaudhri, G., et al. (2022). Targeting ectromelia virus and TNF/NF-κB or STAT3 signaling for effective treatment of viral pneumonia. Proc. Natl. Acad. Sci. U. S. A. 119, e2112725119. doi:10.1073/pnas.2112725119
Pang, P., Zheng, K., Wu, S., Xu, H., Deng, L., Shi, Y., et al. (2018). Baicalin downregulates RLRs signaling pathway to control influenza A virus infection and improve the prognosis. Evid. Based Complement. Altern. Med. 2018, 4923062. doi:10.1155/2018/4923062
Paterson, R. R. (2008). Cordyceps: A traditional Chinese medicine and another fungal therapeutic biofactory? Phytochemistry 69, 1469–1495. doi:10.1016/j.phytochem.2008.01.027
Peng, X. Q., Zhou, H. F., Lu, Y. Y., Chen, J. K., Wan, H. T., and Zhang, Y. Y. (2016a). Protective effects of Yinhuapinggan granule on mice with influenza viral pneumonia. Int. Immunopharmacol. 30, 85–93. doi:10.1016/j.intimp.2015.11.029
Peng, X. Q., Zhou, H. F., Zhang, Y. Y., Yang, J. H., Wan, H. T., and He, Y. (2016b). Antiviral effects of Yinhuapinggan granule against influenza virus infection in the ICR mice model. J. Nat. Med. 70, 75–88. doi:10.1007/s11418-015-0939-z
Pinto, R., Herold, S., Cakarova, L., Hoegner, K., Lohmeyer, J., Planz, O., et al. (2011). Inhibition of influenza virus-induced NF-kappaB and Raf/MEK/ERK activation can reduce both virus titers and cytokine expression simultaneously in vitro and in vivo. Antivir. Res. 92, 45–56. doi:10.1016/j.antiviral.2011.05.009
Qu, H., Zhang, Y., Wang, Y., Li, B., and Sun, W. (2008). Antioxidant and antibacterial activity of two compounds (forsythiaside and forsythin) isolated from Forsythia suspensa. J. Pharm. Pharmacol. 60, 261–266. doi:10.1211/jpp.60.2.0016
Ramos, I., and Fernandez-Sesma, A. (2015). Modulating the innate immune response to influenza A virus: Potential therapeutic use of anti-inflammatory drugs. Front. Immunol. 6, 361. doi:10.3389/fimmu.2015.00361
Ramos-Ligonio, A., López-Monteon, A., and Trigos, A. (2012). Trypanocidal activity of ergosterol peroxide from Pleurotus ostreatus. Phytother. Res. 26, 938–943. doi:10.1002/ptr.3653
Rehwinkel, J., Tan, C. P., Goubau, D., Schulz, O., Pichlmair, A., Bier, K., et al. (2010). RIG-I detects viral genomic RNA during negative-strand RNA virus infection. Cell 140, 397–408. doi:10.1016/j.cell.2010.01.020
Riquelme, O. R., Rioseco, Z. M., Riquelme, O. M., Riquelme, D. J., Caro, M. J., Oyarzún, M. D., et al. (2022). Diferencias y similitudes clínicas y epidemiológicas de la neumonía viral en dos pandemias. Rev. Med. Chil. 150, 316–323. doi:10.4067/S0034-98872022000300316
Sarrica, A., Kirika, N., Romeo, M., Salmona, M., and Diomede, L. (2018). Safety and toxicology of magnolol and honokiol. Planta Med. 84, 1151–1164. doi:10.1055/a-0642-1966
Shi, S., Huang, K., Zhang, Y., Zhao, Y., and Du, Q. (2007). Purification and identification of antiviral components from Laggera pterodonta by high-speed counter-current chromatography. J. Chromatogr. B Anal. Technol. Biomed. Life Sci. 859, 119–124. doi:10.1016/j.jchromb.2007.09.016
Shi, Y., Xu, H., Xiao, Y., Liu, P., Pang, P., Wu, S., et al. (2020). Gegen qinlian decoction downregulates the TLR7 signalling pathway to control influenza A virus infection. Biomed. Pharmacother. 121, 109471. doi:10.1016/j.biopha.2019.109471
Shin, H. B., Choi, M. S., Yi, C. M., Lee, J., Kim, N. J., and Inn, K. S. (2015). Inhibition of respiratory syncytial virus replication and virus-induced p38 kinase activity by berberine. Int. Immunopharmacol. 27, 65–68. doi:10.1016/j.intimp.2015.04.045
Shirey, K. A., Lai, W., Scott, A. J., Lipsky, M., Mistry, P., Pletneva, L. M., et al. (2013). The TLR4 antagonist Eritoran protects mice from lethal influenza infection. Nature 497, 498–502. doi:10.1038/nature12118
Sun, H., Luo, G., Chen, D., and Xiang, Z. (2016). A comprehensive and system review for the pharmacological mechanism of action of rhein, an active anthraquinone ingredient. Front. Pharmacol. 7, 247. doi:10.3389/fphar.2016.00247
Suntres, Z. E., Coccimiglio, J., and Alipour, M. (2015). The bioactivity and toxicological actions of carvacrol. Crit. Rev. Food Sci. Nutr. 55, 304–318. doi:10.1080/10408398.2011.653458
Thoresen, D., Wang, W., Galls, D., Guo, R., Xu, L., and Pyle, A. M. (2021). The molecular mechanism of RIG-I activation and signaling. Immunol. Rev. 304, 154–168. doi:10.1111/imr.13022
To, K. K., Hung, I. F., Li, I. W., Lee, K. L., Koo, C. K., Yan, W. W., et al. (2010). Delayed clearance of viral load and marked cytokine activation in severe cases of pandemic H1N1 2009 influenza virus infection. Clin. Infect. Dis. 50, 850–859. doi:10.1086/650581
Vitiello, M., Galdiero, M., Finamore, E., Galdiero, S., and Galdiero, M. (2012). NF-κB as a potential therapeutic target in microbial diseases. Mol. Biosyst. 8, 1108–1120. doi:10.1039/c2mb05335g
Wang, J., Wang, L., Lou, G. H., Zeng, H. R., Hu, J., Huang, Q. W., et al. (2019). Coptidis rhizoma: A comprehensive review of its traditional uses, botany, phytochemistry, pharmacology and toxicology. Pharm. Biol. 57, 193–225. doi:10.1080/13880209.2019.1577466
Wang, Q. W., Su, Y., Sheng, J. T., Gu, L. M., Zhao, Y., Chen, X. X., et al. (2018). Anti-influenza A virus activity of rhein through regulating oxidative stress, TLR4, Akt, MAPK, and NF-κB signal pathways. PLoS One 13, e0191793. doi:10.1371/journal.pone.0191793
Wang, X., Jia, W., Zhao, A., and Wang, X. (2006). Anti-influenza agents from plants and traditional Chinese medicine. Phytother. Res. 20, 335–341. doi:10.1002/ptr.1892
Wang, Y., Li, J., Yan, W., Chen, Q., Jiang, Z., Zhang, R., et al. (2018). An active component containing pterodontic acid and pterodondiol isolated from Laggera pterodonta inhibits influenza A virus infection through the TLR7/MyD88/TRAF6/NF-κB signaling pathway. Mol. Med. Rep. 18, 523–531.
Wang, Y., Zhou, B., Lu, J., Chen, Q., Ti, H., Huang, W., et al. (2017). Inhibition of influenza virus via a sesquiterpene fraction isolated from Laggera pterodonta by targeting the NF-κB and p38 pathways. BMC Complement. Altern. Med. 17, 25. doi:10.1186/s12906-016-1528-8
Weber-Gerlach, M., and Weber, F. (2016). Standing on three legs: Antiviral activities of RIG-I against influenza viruses. Curr. Opin. Immunol. 42, 71–75. doi:10.1016/j.coi.2016.05.016
Weis, S., and Te Velthuis, A. J. W. (2021). Influenza virus RNA synthesis and the innate immune response. Viruses 13, 780. doi:10.3390/v13050780
Wu, L., Chen, Y., Ma, Y., Yang, Z., Yang, N., Deng, W., et al. (2020). Clinical practice guideline on treating influenza in adult patients with Chinese patent medicines. Pharmacol. Res. 160, 105101. doi:10.1016/j.phrs.2020.105101
Wu, Q. P., Xie, Y. Z., Deng, Z., Li, X. M., Yang, W., Jiao, C. W., et al. (2012). Ergosterol peroxide isolated from Ganoderma lucidum abolishes microRNA miR-378-mediated tumor cells on chemoresistance. PLoS One 7, e44579. doi:10.1371/journal.pone.0044579
Wu, S., Patel, K. B., Booth, L. J., Metcalf, J. P., Lin, H. K., and Wu, W. (2010). Protective essential oil attenuates influenza virus infection: An in vitro study in MDCK cells. BMC Complement. Altern. Med. 10, 69. doi:10.1186/1472-6882-10-69
Wu, X. L., Ju, D. H., Chen, J., Yu, B., Liu, K. L., He, J. X., et al. (2013). Immunologic mechanism of Patchouli alcohol anti-H1N1 influenza virus may through regulation of the RLH signal pathway in vitro. Curr. Microbiol. 67, 431–436. doi:10.1007/s00284-013-0381-y
Wu, X. N., Yu, C. H., Cai, W., Hua, J., Li, S. Q., and Wang, W. (2011). Protective effect of a polyphenolic rich extract from Magnolia officinalis bark on influenza virus-induced pneumonia in mice. J. Ethnopharmacol. 134, 191–194. doi:10.1016/j.jep.2010.11.074
Wu, Y., Li, J. Q., Kim, Y. J., Wu, J., Wang, Q., and Hao, Y. (2011). In vivo and in vitro antiviral effects of berberine on influenza virus. Chin. J. Integr. Med. 17, 444–452. doi:10.1007/s11655-011-0640-3
Wu, Y., Zhou, C., Li, X., Song, L., Wu, X., Lin, W., et al. (2006). Evaluation of antiinflammatory activity of the total flavonoids of Laggera pterodonta on acute and chronic inflammation models. Phytother. Res. 20, 585–590. doi:10.1002/ptr.1918
Wurzer, W. J., Ehrhardt, C., Pleschka, S., Berberich-Siebelt, F., Wolff, T., Walczak, H., et al. (2004). NF-kappaB-dependent induction of tumor necrosis factor-related apoptosis-inducing ligand (TRAIL) and Fas/FasL is crucial for efficient influenza virus propagation. J. Biol. Chem. 279, 30931–30937. doi:10.1074/jbc.M403258200
Xie, Y., Yu, Y., Zhao, L., Ning, P., Luo, Q., Zhang, Y., et al. (2021). Specific cytokine profiles predict the severity of influenza A pneumonia: A prospectively multicenter pilot study. Biomed. Res. Int. 2021, 9533044. doi:10.1155/2021/9533044
Yan, Y. Q., Fu, Y. J., Wu, S., Qin, H. Q., Zhen, X., Song, B. M., et al. (2018). Anti-influenza activity of berberine improves prognosis by reducing viral replication in mice. Phytother. Res. 32, 2560–2567. doi:10.1002/ptr.6196
Yang, M., Wang, Y., Yue, Y., Liang, L., Peng, M., Zhao, M., et al. (2022). Traditional Chinese medicines as effective agents against influenza virus-induced pneumonia. Biomed. Pharmacother. 153, 113523. doi:10.1016/j.biopha.2022.113523
Yang, Y., Zhong, H., Song, T., He, J., Guo, L., Tan, X., et al. (2017). Epidemiological and clinical characteristics of humans with avian influenza A (H7N9) infection in Guangdong, China, 2013-2017. Int. J. Infect. Dis. 65, 148–155. doi:10.1016/j.ijid.2017.07.021
Yu, H., Lin, L., Zhang, Z., Zhang, H., and Hu, H. (2020). Targeting NF-κB pathway for the therapy of diseases: Mechanism and clinical study. Signal Transduct. Target Ther. 5, 209. doi:10.1038/s41392-020-00312-6
Yu, L. Z., Wu, J. Y., Luo, J. B., Chen, Y. R., and Lin, H. (2005). Effects of different compositions of Gegenqinlian decoction on experimental shigellosis in rabbits. Di Yi Jun Yi Da Xue Xue Bao 25, 1132–1134.
Zeng, X. Y., Yuan, W., Zhou, L., Wang, S. X., Xie, Y., and Fu, Y. J. (2017). Forsythoside A exerts an anti-endotoxin effect by blocking the LPS/TLR4 signaling pathway and inhibiting Tregs in vitro. Int. J. Mol. Med. 40, 243–250. doi:10.3892/ijmm.2017.2990
Zhang, F. L., Yin, X. J., Yan, Y. L., and Wu, Q. F. (2022). Pharmacokinetics and pharmacodynamics of huanglian-houpo decoction based on berberine hydrochloride and magnolol against H1N1 influenza virus. Eur. J. Drug Metab. Pharmacokinet. 47, 57–67. doi:10.1007/s13318-021-00724-x
Zhang, L., Zhang, B., Wang, L., Lou, M., and Shen, Y. (2021). Huanglian-Houpo drug combination ameliorates H1N1-induced mouse pneumonia via cytokines, antioxidant factors and TLR/MyD88/NF-κB signaling pathways. Exp. Ther. Med. 21, 428. doi:10.3892/etm.2021.9845
Zhang, Q., Xu, G., Li, J., Guo, X., Wang, H., Li, B., et al. (2016). Metabonomic study on the plasma of streptozotocin-induced diabetic rats treated with Ge Gen Qin Lian Decoction by ultra high performance liquid chromatography-mass spectrometry. J. Pharm. Biomed. Anal. 120, 175–180. doi:10.1016/j.jpba.2015.12.031
Zhang, Y., Lyu, C., Fong, S. Y. K., Wang, Q., Li, C., Ho, N. J., et al. (2019). Evaluation of potential herb-drug interactions between oseltamivir and commonly used anti-influenza Chinese medicinal herbs. J. Ethnopharmacol. 243, 112097. doi:10.1016/j.jep.2019.112097
Zhang Y., Y., Wang, R., Shi, W., Zheng, Z., Wang, X., Li, C., et al. (2021). Antiviral effect of fufang yinhua jiedu (FFYH) granules against influenza A virus through regulating the inflammatory responses by TLR7/MyD88 signaling pathway. J. Ethnopharmacol. 275, 114063. doi:10.1016/j.jep.2021.114063
Zhao, J., Hao, Y., Xia, X., Li, R., Huang, X., Yang, Z., et al. (2021a). Chaiqin qingning capsule inhibits influenza virus infection and inflammation in vitro and in vivo. Evid. Based Complement. Altern. Med. 2021, 6640731. doi:10.1155/2021/6640731
Zhao, J., Wang, Y., Huang, X., Ma, Q., Song, J., Wu, X., et al. (2021b). Liu Shen Wan inhibits influenza virus-induced secondary Staphylococcus aureus infection in vivo and in vitro. J. Ethnopharmacol. 277, 114066. doi:10.1016/j.jep.2021.114066
Zheng, K., Wu, S. Z., Lv, Y. W., Pang, P., Deng, L., Xu, H. C., et al. (2021). Carvacrol inhibits the excessive immune response induced by influenza virus A via suppressing viral replication and TLR/RLR pattern recognition. J. Ethnopharmacol. 268, 113555. doi:10.1016/j.jep.2020.113555
Zheng, X., Chen, Z., Shi, S., Yan, H., Zhou, J., Jiang, L., et al. (2022). Forsythiaside A improves Influenza A virus infection through TLR7 signaling pathway in the lungs of mice. BMC Complement. Med. Ther. 22, 164. doi:10.1186/s12906-022-03644-8
Zhou, B., Liang, X., Feng, Q., Li, J., Pan, X., Xie, P., et al. (2019). Ergosterol peroxide suppresses influenza A virus-induced pro-inflammatory response and apoptosis by blocking RIG-I signaling. Eur. J. Pharmacol. 860, 172543. doi:10.1016/j.ejphar.2019.172543
Zhou, M., Zhao, X., Liao, L., Deng, Y., Liu, M., Wang, J., et al. (2022). Forsythiaside A regulates activation of hepatic stellate cells by inhibiting NOX4-dependent ros. Oxid. Med. Cell Longev. 2022, 9938392. doi:10.1155/2022/9938392
Zhu, H., Lu, X., Ling, L., Li, H., Ou, Y., Shi, X., et al. (2018). Houttuynia cordata polysaccharides ameliorate pneumonia severity and intestinal injury in mice with influenza virus infection. J. Ethnopharmacol. 218, 90–99. doi:10.1016/j.jep.2018.02.016
Keywords: TCM, influenza viruses, viral pneumonia, pattern recognition receptor, NF-κB signaling pathway
Citation: Zhang L, Ye X, Liu Y, Zhang Z, Xia X and Dong S (2023) Research progress on the effect of traditional Chinese medicine on the activation of PRRs-mediated NF-κB signaling pathway to inhibit influenza pneumonia. Front. Pharmacol. 14:1132388. doi: 10.3389/fphar.2023.1132388
Received: 27 December 2022; Accepted: 28 March 2023;
Published: 07 April 2023.
Edited by:
Ruiwen Zhang, University of Houston, United StatesReviewed by:
Ioanna Galani, Biomedical Research Foundation of the Academy of Athens (BRFAA), GreeceDaniel Prantner, University of Maryland, United States
Tram N. Q. Pham, Montreal Clinical Research Institute (IRCM), Canada
Copyright © 2023 Zhang, Ye, Liu, Zhang, Xia and Dong. This is an open-access article distributed under the terms of the Creative Commons Attribution License (CC BY). The use, distribution or reproduction in other forums is permitted, provided the original author(s) and the copyright owner(s) are credited and that the original publication in this journal is cited, in accordance with accepted academic practice. No use, distribution or reproduction is permitted which does not comply with these terms.
*Correspondence: Zhongde Zhang, RG9jdG9yenpkOTlAMTYzLmNvbQ==; Xueshan Xia, b2xpdmVyeGlhMjAwMEBhbGl5dW4uY29t; Shuwei Dong, ZG9uZ3N3QGt1c3QuZWR1LmNu