- Department of Pharmacology and Toxicology, College of Pharmacy, Umm Al-Qura University, Makkah, Saudi Arabia
Background: Vancomycin is a glycopeptide antibiotic with a high risk of acute liver injury. Resveratrol is believed to protect the liver against toxicity.
Aim: To investigate the ability of resveratrol to attenuate vancomycin-induced liver toxicity in rats injected with vancomycin.
Method: Twenty-four adult male Wistar rats were distributed into three groups. The control group received only a vehicle, while the treated group received either vancomycin 200 (mg/kg, i. p.) only or vancomycin (200 mg/kg, i. p.) with resveratrol (20 mg/kg, oral gavage). All groups received their dose once daily for 7 days. Hepatic damage was assessed by measuring biochemical parameter levels in serum, aspartate transaminase (AST), alanine transaminase (ALT), alkaline phosphatase (ALP), and lactate dehydrogenase (LDH). Also, antioxidants and inflammation biomarkers such as Interleukin-6 (IL-6), malondialdehyde (MDA), nitric oxide (NO), and glutathione (GSH) were measured. Furthermore, the vancomycin-induced pathological changes in the liver were evaluated by histopathological studies.
Results: In the vancomycin-treated group, hepatic serum biomarkers such as AST, ALT, ALP, IL-6, and MDA were elevated, while NO and GSH were depleted. However, resveratrol co-treatment with vancomycin prevented the elevation of AST, ALT, ALP, IL-6, and MDA and it protected the liver from NO and GSH depletion. Also, regarding vancomycin-induced degeneration of hepatocytes, resveratrol co-treatment with vancomycin prevented such degeneration and improved mononuclear cells in the liver.
Conclusion: The results showed that oral administration of resveratrol has a significant hepatoprotective effect against vancomycin-induced hepatotoxicity.
Introduction
Many drugs are known to produce liver injury, and these adverse hepatic events usually result in severe liver injury if not treated properly (Bissell et al., 2001). It has been estimated that drug-induced liver failure represents half of the cases of all forms of acute and chronic liver disease (Kaplowitz, 2001). Approximately 10% of chronic hepatitis cases occur due to drug use, and 5% from hospital admissions, while 50% of acute liver failure cases occur due to drug use (Pandit et al., 2012). Hepatotoxicity associated with antibiotics is asymptomatic and usually presents mild hepatic injury (Thiim and Friedman, 2003). Vancomycin is a glycopeptide antibiotic with known bactericidal activity, and it is considered the drug of choice for treating methicillin-resistant Staphylococcus aureus infections (David and Daum, 2010; Steinmetz et al., 2015). However, several side effects have been reported with vancomycin, such as hypotension, phlebitis, nephrotoxicity, and hepatotoxicity (Badran et al., 2011; Bamgbola, 2016).
Moreover, a few reports have shown that chronic use of glycopeptide antibiotics has the potential to elevate liver enzymes and induce hepatotoxicity (Cadle et al., 2006; Chen et al., 2011; Brunetti et al., 2020). However, data to support the influence of vancomycin on liver dysfunction are limited, and the mechanism of vancomycin-induced hepatotoxicity has not been studied effectively. Many risk factors contribute to vancomycin-induced hepatotoxicities, such as long-term treatments, high doses, obesity, patient age, and overall health (Larrey, 2002; Breedt et al., 2005; Kohno et al., 2007; Florescu et al., 2008). Moreover, the hepatic injury associated with vancomycin could also be due to sepsis, bacterial endotoxins, fever, or hemolysis (Sibai, 2004; Shah et al., 2010; Kouijzer et al., 2021). While different strategies have been suggested to reduce any potential risk of hepatotoxicity associated with vancomycin treatment (Aldaz et al., 2000; Hwang et al., 2015; Regal et al., 2019; Tsutsuura et al., 2021), the exact mechanism for this injury is not fully understood. Several studies have suggested that vancomycin-induced toxicity could be due to several factors, including the generation of free radicals, oxidative stress, and inflammation, which cause liver injury in animal studies (Sahin et al., 2006; El Bohi et al., 2021). In addition, reactive oxygen species (ROS) are usually generated within cells, leading to the initiation of oxidative stress-related intermediates, which contribute to chronic inflammation and liver fibrogenesis (Bataller and Brenner, 2005; Friedman, 2008; Novo and Parola, 2008). Therefore, herbal compounds with antioxidant and anti-inflammatory properties have been considered.
Indeed, cumulative reports have suggested that herbal compounds have a great potential to attenuate drug-induced liver toxicity due to their antioxidant and anti-inflammatory properties (Abou Seif, 2016; Parthasarathy and Evan Prince, 2021). Hence, many herbal compounds have been used as traditional medicines for liver disorders (Ali et al., 2008; Zhang et al., 2018; Philips et al., 2020; Das et al., 2022). In addition, these are potential sources of new therapeutic agents that could be used to prevent hepatic injuries. For example, resveratrol has long been known to have antioxidant and anti-inflammatory effects. Moreover, researchers have recently become more interested in resveratrol, from its ability to extend human lifespans to its effect on chemoprevention, cardiovascular diseases, and neurodegenerative disorders, as reported in several studies (Gescher and Steward, 2003; Srivastava et al., 2013; Pourhanifeh et al., 2019; Banez et al., 2020; Labban et al., 2021a; Labban et al., 2021b). The antioxidant properties of resveratrol have been demonstrated in several in vitro studies. The antioxidant property of resveratrol has been demonstrated by inhibiting nicotinamide adenine dinucleotide phosphate oxidases, which inhibit the production of reactive oxygen species (ROS) (Halliwell, 2007; Yousefian et al., 2019). As well as protecting cells from oxidative stress, resveratrol also promotes the expression of antioxidative enzymes and their substrates (Miguel et al., 2021; Santos et al., 2021). Recently, it has been suggested that resveratrol has hepatoprotection properties through its anti-inflammatory and antioxidant effects (Chupradit et al., 2022; Ma et al., 2022; Tong et al., 2022). It has also been reported that resveratrol attenuates acetaminophen toxic metabolite N-acetyl-p-benzoquinone-imine and facilitates liver regeneration by modulating the silent mating type information regulation two homolog (SIRT1), tumor protein P53, and Tumor Necrosis Factor-alpha (TNF-α) (Sener et al., 2006; Wang et al., 2015). Moreover, resveratrol has been shown to improve glutathione (GSH) levels and antioxidant enzyme activities, and to decrease ROS production in liver tissues (Bujanda et al., 2008; Rivera et al., 2008; Rubiolo and Vega, 2008; Sebai et al., 2010). Also, one study reported that the thioacetamide-induced hepatotoxic effect associated with TNF-α and iNOS elevation was inhibited by resveratrol (Ebrahim et al., 2022).
This study investigates the effect of high doses of vancomycin administered to induce liver toxicity. A few studies have investigated similar regimens and found that high doses of vancomycin were associated with elevated levels of liver enzymes, the tissue activities of catalase, superoxide dismutase activities, lipid peroxidation, and malondialdehyde (MDA) (Ahmida, 2012; Çağlayan et al., 2019; El Bohi et al., 2021). Moreover, this research investigates the ability of resveratrol to attenuate vancomycin-induced liver toxicity through several biomarkers, such as liver tissues, inflammatory mediators, liver enzymes, and antioxidant property markers.
Materials and methods
Drugs
Resveratrol (ProHealth, United States) and vancomycin (Medis, Tunisia) were used in the study. All other chemicals and reagents used were of analytical grade. Resveratrol and vancomycin were dissolved in a saline solution (0.9% NaCl) as a vehicle for both drugs.
Dose selection
The vancomycin dose was based on several recent studies using 200 mg/kg, i. p. to induce hepatotoxicity (Kucukler et al., 2020) and nephrotoxicity (Ahmida, 2012) once daily for seven consecutive days. The resveratrol dose was based on several studies using the same dose against several compounds, such as dimethylnitrosamine (Lee et al., 2010) and concanavalin (Zhou et al., 2015).
Animals
Twenty-four male adult Wistar rats (weighing 170–207 g) were used. The animals were housed in plastic cages (4 rats per cage) under a 12 h light/12 h dark schedule in a humidity-controlled room and were fed a normal diet. They had access to food and water ad libitum and were monitored daily to ensure proper animal welfare. The rats were acclimatized for 1 week before starting the experiment. Then, the rats were distributed into three groups (n = 8 in each group). The rats received only a vehicle in the first group (control). In the second group, vancomycin, the rats received vancomycin (200 mg/kg, i. p.) once daily for seven consecutive days. In the last group, vancomycin + resveratrol, the rats received vancomycin (200 mg/kg, i. p.) and resveratrol (20 mg/kg, oral gavage) once daily for seven consecutive days. All the treatments were carried out within 7 days, and the animals were euthanized using CO2 and sacrificed on the eighth day. The tissue and serum samples were collected, homogenized, centrifuged, for analysis.
Measurement of biochemical parameters
The serum samples were used for the measurement of all biochemical parameters. The usage of serum samples was based on several reportes that have useed similar methods to assess hepatic function. Aspartate transaminase (AST), Alanine transferase (ALT) as reported in (Yin et al., 2019), Alkaline phosphatase (ALP) (Ibrahim et al., 2020), Interleukin-6 (IL-6) (Xia et al., 2019), nitric oxide (NO) (Fathy et al., 2019), GSH (Ibrahim et al., 2020) and MDA (Omara et al., 2021).
Markers of liver tissue damage
The serum samples were analyzed using assay kits and ELISA for liver functions. Aspartate transaminase (AST), Alanine transferase (ALT) and Alkaline phosphatase (ALP) were assessed using ELISA kits (MyBioSource kits catalog: MBS269614, MBS264975, MBS011598, MBS726781; MyBioSource, Inc.) A centrifuge was then performed at approximately 1000× g for 15 min. Serum was collected, and the assay was immediately performed according to the manufacturer’s recommendations (MyBioSource, Inc.). A standard curve was established using a series diluent. A Microplate reader (450 nm detection wavelength filter, 570 nm or 630 nm correction wavelength filters) was used to perform all the tests.
Markers of inflammation
Interleukin-6 (IL-6) and nitric oxide (NO) levels in the serum were measured with the fully automatic ELISA DSX best 2000® microtiter plate and the ELISA kits. A centrifuge was then performed at approximately 1000× g for 15 min. Serum was collected, and the assay was immediately performed according to the manufacturer’s recommendations (MyBioSource, Inc.).
Markers of antioxidant and prooxidant
GSH and MDA levels in the serum were measured using an ELISA DSX best 2000® microtiter plate and the ELISA kits. In a serum separator tube, the serum was clotted for 2 hours at room temperature and overnight at 2°C–8°C. A centrifuge was then performed at approximately 1000× g for 15 min. Serum was collected, and the assay was immediately performed according to the manufacturer’s recommendations (MyBioSource, Inc.). A Microplate reader (450 nm detection wavelength filter) was used to perform all the tests.
Histopathology
The liver tissues were used for the histopathological assessment and prepared in 10% formalin solution for 2 days. In addition, the tissue was embedded in paraffin blocks following routine tissue tracking procedures. Finally, Hematoxylin and eosin stains were used to stain the slides. Masson’s Trichrome method was employed, which involves deparaffinizing and rehydrating the liver in descending series of alcohols before staining them with Biebrich scarlet-acid fuchsin solution. A solution of phosphomolybdic-phosphotungstic acid was then used to differentiate the sections.
Statistical analysis
Statistical analyses were performed using GraphPad Prism™ (v9.3.1). Data were expressed as mean ± standard error of the mean. A one-way ANOVA, followed by Tukey’s post hoc test, was used for comparisons. A p-value of <0.05 was considered statistically significant.
Results
Measured levels of AST, ALT, ALP, and LDH
The effect of vancomycin on AST, ALT, ALP and LDH was observed in the rats’ serum. The one-way ANOVA test revealed a significant main effect on the AST serum levels [F (2, 21) = 216.0, p < 0.0001, Figure 1A]. Further analysis using Tukey’s multiple comparisons test revealed that rats injected with only vancomycin had significantly increased levels of AST compared to the control group (p < 0.0001). Interestingly, the rats injected with resveratrol and vancomycin were protected against vancomycin-induced toxicity. In addition, the vancomycin + resveratrol group of rats showed a significant increase in AST levels, p = 0.0083, compared to the control group.
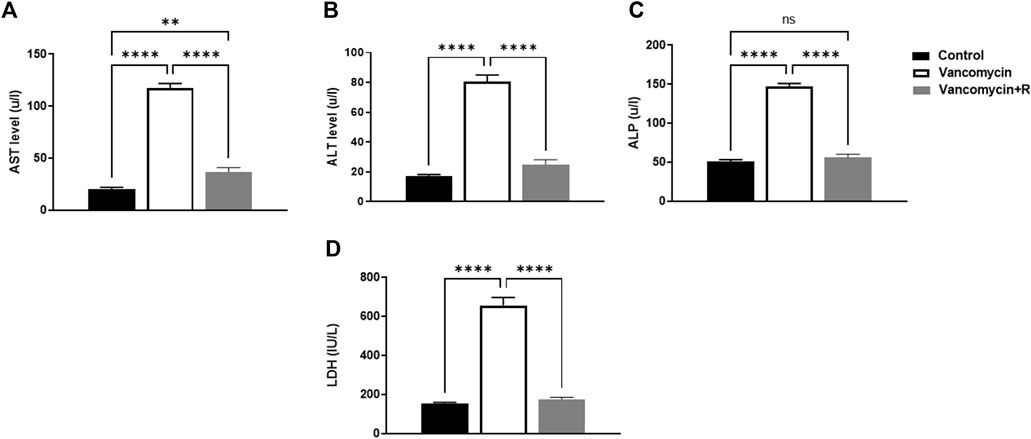
FIGURE 1. (A) Aspartate transaminase (AST) levels in u/l, (B) Alanine aminotransferase (ALT) in u/l, (C) Alkaline phosphatase (ALP) in unit/liter (u/l), and (D) lactate dehydrogenase (LDH) (IU/L), were measured in the control rats, rats injected with vancomycin only, and rats injected with vancomycin and resveratrol. Significant difference: ns = non-significant, **p < 0.001 ****p < 0.0001.
Moreover, vancomycin had a significant main effect on the ALT serum levels in the groups [F (2, 21) = 124.0, p < 0.0001, Figure 1B]. Further analysis using Tukey’s multiple comparisons test revealed that the control group rats displayed no change in ALT, p = 0.1949. However, the rats injected with only vancomycin displayed a significant increase in ALT compared to the control group (p < 0.0001). Interestingly, the rats injected with resveratrol and vancomycin were protected against vancomycin-induced toxicity.
Another significant main effect on ALP serum levels in the groups [F (2, 21) = 209.3, p < 0.0001, Figure 1C]. Further analysis using Tukey’s multiple comparisons test revealed that the control rats displayed no change in ALP, p = 0.5888. The rats injected with vancomycin showed a significant increase in ALP compared to the control group (p < 0.0001), and the rats injected with resveratrol and vancomycin were protected against vancomycin-induced toxicity.
Moreover, significant main effect on LDH serum levels in the groups [F (2, 21) = 130.9, p < 0.0001, Figure 1D]. Additional analysis using Tukey’s multiple comparisons tests indicated that the control group rats had no change in LDH, p = 0.8200. However, the rats injected with only vancomycin displayed a significant increase in LDH compared to the control group (p < 0.0001). Remarkably, resveratrol demonstrated a protective role against vancomycin-induced toxicity.
Measured levels of IL-6 and NO
The effect of vancomycin on IL-6 and NO levels was observed in the rats’ serum. The one-way ANOVA test revealed that vancomycin had a significant main effect on the IL-6 serum levels in the groups F (2, 21) = 141.8, p < 0.0001, Figure 2A. Further analysis using Tukey’s multiple comparisons test revealed that the control rats displayed no change in IL-6, p = 0.9185. However, the rats injected with only vancomycin had a significant increase in IL-6 compared to the control group (p < 0.0001). Interestingly, the rats injected with resveratrol and vancomycin were protected against vancomycin-induced toxicity.
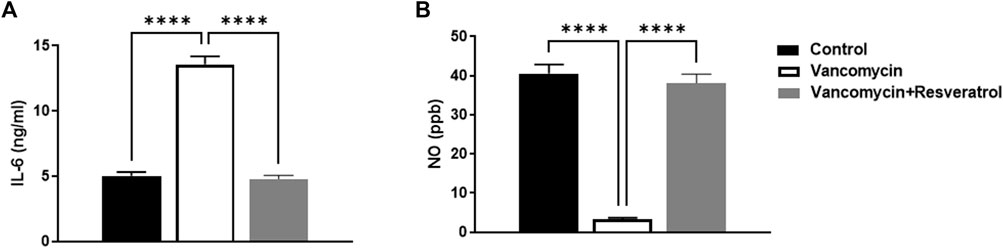
FIGURE 2. Interleukin-6 (IL-6) levels in ng/ml and (B) nitric oxide (NO) levels in parts per million (ppb) were measured in the control rats, rats injected with vancomycin only, and rats injected with vancomycin and resveratrol. Significant difference: ****p < 0.0001.
The significant main effect on NO serum levels in the groups [F (2, 21) = 118.3, p < 0.0001, Figure 2B]. Tukey’s multiple comparisons test revealed that the control group rats displayed no change in NO serum levels, p = 0.6613. However, the rats injected with only vancomycin showed a significant NO increase compared to the control group (p < 0.0001). Remarkably, the rats injected with resveratrol and vancomycin were protected against vancomycin-induced toxicity.
Measured levels of MDA and GSH
The effect of vancomycin on MDA was observed in the rats’ serum. A further one-way ANOVA test showed that vancomycin had a significant main effect on the MDA serum levels in the groups [F (2, 21) = 190.2, p < 0.0001, Figure 3A]. Also, Tukey’s multiple comparisons test revealed that the control group rats displayed a significant change in MDA serum levels, p < 0.0057. Again, the rats injected with only vancomycin displayed a significant increase in LDH compared to the control group (p < 0.0001), while the rats injected with resveratrol and vancomycin were protected against vancomycin-induced toxicity.
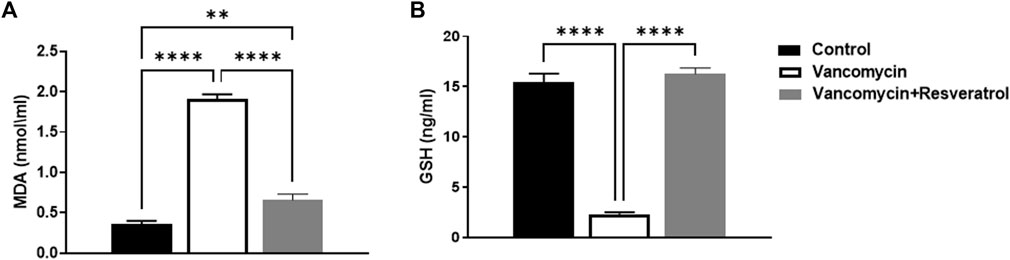
FIGURE 3. (A) Malondialdehyde (MDA) (nmol/ml) and (B) Glutathione (GSH) levels in ng/ml were measured in the control rats, rats injected with vancomycin only, and rats injected with vancomycin and resveratrol. Significant difference: **p < 0.001; ****p < 0.0001.
The effect of vancomycin on GSH levels was observed in rats’ serum. The test revealed a significant main effect on GSH serum levels in the groups [F (2, 21) = 167.3 < 0.0001, Figure 3B]. Further analysis using Tukey’s multiple comparisons test revealed that the control group rats displayed no change in GSH, p = 0.5894. However, rats injected with only vancomycin displayed a significant decrease in GSH compared to the control group (p < 0.0001). Interestingly, the rats injected with resveratrol and vancomycin were protected against vancomycin-induced toxicity.
Histopathological results
For the control group, a microscopic examination of the liver revealed a normal appearance, as shown in Figure 4A. For the control + vancomycin group, liver-intralobular mononuclear inflammatory infiltrations and Mallory bodies were evident due to the degeneration of hepatocytes, as shown in Figure 4B. For the vancomycin + resveratrol group, the liver showed a marked improvement in the mononuclear cells, as shown in Figure 4C.
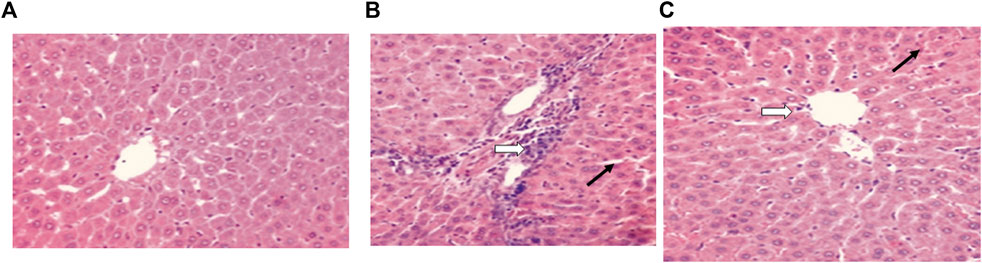
FIGURE 4. Histopathological liver evaluation in (A) control rats, (B) rats injected with vancomycin only, liver-Intralobular mononuclear inflammatory infiltrations (white arrow), and Mallory bodies (black arrow) due to degeneration of hepatocytes and increased vacuolation in the cytoplasm of hepatocytes appeared as indistinct clear vacuoles (black arrow) indicate glycogen infiltration and (C) rats injected with vancomycin and resveratrol, liver-showed marked improved on a mononuclear cell, and decrease number of mononuclear inflammatory infiltrates (white arrow) and decrease of Mallory bodies (black arrow).
Discussion
Biological systems depend on the liver to detoxify xenobiotics (Apte and Krishnamurthy, 2011). Several studies have shown that hepatic damage disrupts the body’s regular metabolism (Fabbrini and Magkos, 2015; Kurland et al., 2015). There are several causes of acute liver failure, including viral hepatitis, toxic liver damage caused by poisons and drugs, and ischemia (Jalan et al., 2012; Bernal and Wendon, 2013). The liver metabolises xenobiotics as the body’s first line of defense against ingested toxins and drugs, which often cause necrosis and apoptosis (Tsochatzis et al., 2014). A growing body of research focuses on the potential toxicity of antibiotics in the liver (Acevedo, 2015; Fernández et al., 2016; Zoratti et al., 2022). Vancomycin tends to cause adverse events after prolonged use, and large doses may be toxic to the liver (Kucukler et al., 2020). This study aimed to determine whether resveratrol plays a beneficial protective role against vancomycin-induced toxicity in the livers of male Wistar rats.
This study’s findings align with several other research studies that present vancomycin’s potential toxicity (Kucukler et al., 2020). However, to our knowledge, no previous study has investigated the protective role of resveratrol. This study revealed an elevation in serum biomarkers such as AST, ALT, and ALP levels in the groups given only vancomycin or vancomycin with resveratrol, compared to the control group. The serum level of ALT is the most widely used clinical biomarker of hepatic function (Senior, 2012). Furthermore, GSH, an antioxidant, was restored to the normal level in the rats injected with vancomycin and resveratrol, indicating the antioxidant activity of the latter.
Moreover, the rats injected with vancomycin only had significantly reduced GSH levels, confirming the previous findings. Several parameters were affected by the administration of vancomycin. The levels of IL-6, LDH, MDA, and NO were highly increased in the rats injected with vancomycin. Vancomycin administration caused hepatocyte damage, leading to liver enzyme elevation. Hepatotoxic studies commonly measure liver enzyme levels such as ALT, AST, and ALP as serum hepatic biomarkers for determining liver lesions (Deshpande et al., 1998; Sadeghi et al., 2008; Mehrzadi et al., 2018). In this study, vancomycin caused a significant elevation in the serum hepatic biomarkers ALT, AST, and ALP. The concentration of ALT and AST enzymes in serum reflects the severity of liver damage as these enzymes are present in high concentrations in the liver (Adeyemi and Akanji, 2011; Adeyemi and Adewumi, 2014; Yilmaz et al., 2017). In addition, many tissues in the body contain ALP; therefore, it can be considered a non-specific enzyme (López-Posadas et al., 2011). Furthermore, hepatobiliary duct dysfunction or the destruction of hepatic cell membranes can cause a rise in serum ALP, which could indicate a problem with the excretory function (Lowe et al., 2017; Kashima et al., 2018). On the other hand, co-treatment of vancomycin with resveratrol protected against vancomycin-induced hepatic damage, evidenced by the significantly decreased levels of the hepatic serums AST, ALT, and ALP.
It is well known that vancomycin is almost completely eliminated from the body by the kidneys; however, the mechanism by which nephrotoxicity occurs is still unclear. It has been demonstrated in experimental animals that the drug may cause tubular ischemia and acute tubulointerstitial injury by inducing oxidative stress in the proximal renal tubule cells (King and Smith, 2004; Gupta et al., 2011a). Here, vancomycin increased the serum levels of IL-6 (a pro-inflammatory cytokine). The cell surface receptors of the IL-6 family of cytokines regulate cell function (Taga and Kishimoto, 1997). IL-6 consists of two structural subunits: a ligand-binding subunit called the IL-6 receptor and a signal-transducing glycoprotein called Gp130 (Yamauchi-Takihara and Kishimoto, 2000). The liver synthesizes several acute phase proteins in response to IL-6 as it is involved in the pathogenesis of many fibrogenic diseases (Choi et al., 1994). In recent studies, IL-6 has been linked to acute and chronic liver damage (Cao et al., 1998; Gewiese-Rabsch et al., 2010; Bergmann et al., 2017; Shao et al., 2020). In addition, many xenobiotics drugs can injure the liver and trigger the release of pro-inflammatory cytokines like TNF-α and IL6 into the bloodstream (Takai et al., 2016; Olaniyan et al., 2018; Wu et al., 2018). By demonstrating the changes in cytokines that occur in hepatic cells, rodent models can illustrate the molecular changes associated with human hepatic cell death. In this study, we also tested the serum level of MDA, an oxidative stress biomarker that serves as an index of oxidative damage in the liver (Bakan et al., 2002). MDA has been reported to induce collagen production by hepatic stellate cells, resulting in fibrosis (Hadizadeh et al., 2017). Also, it has been reported that vancomycin could initiate an intracellular production of peroxides that triggers the production of MDA (Oktem et al., 2005). Thus, in this study, the vancomycin-induced high serum levels of MDA could be due to vancomycin’s free radical trapping activity and oxidative stress.
GSH has several functions, including serving as an antioxidant, and playing a role in redox and cell signaling (Franco and Cidlowski, 2009; Mari et al., 2009). It acts by reducing hydrogen peroxide, scavenging ROS, and reactive nitrogen species (RNS); therefore, it protects cells against oxidative damage (Day and Suzuki, 2005; Winter et al., 2017). The build-up of an oxidized form of GSH, glutathione disulfide (GSSG), and the depletion of GSH are closely related to ROS and RNS effects on the liver and cells (Yuan and Kaplowitz, 2009; Eskandari et al., 2012). Hepatic NO and its derivatives are essential in liver physiology and pathophysiology (Laskin et al., 2001; Chen et al., 2003; Diesen and Kuo, 2010). It is also a second messenger that acts in several pathways and plays a crucial role in regulating blood pressure by relaxing the endothelium, attacking tumor cells, and stimulating the brain (Gupta et al., 2011b; Korde Choudhari et al., 2013; Picon-Pages et al., 2019). Although NO has multiple and complex roles, it has been suggested that it affects the pathogenesis and progression of liver diseases (Iwakiri and Kim, 2015; Ekhlasi et al., 2017; Wang et al., 2018). On the other hand, LDH (a non-specific tissue damage biomarker) was elevated in vancomycin-treated animals. Numerous tissues and organs in the body produce LDH, including the muscles, liver, heart, pancreas, kidneys, brain, and blood. Body tissue damage can be detected by using the LDH test, determining its location and severity (Farhana and Lappin, 2022). In this study, vancomycin administration causes liver damage, contributing to LDH elevation in the serum. This elevation could arise from vancomycin, causing damage to the kidneys. It has been reported that vancomycin can cause kidney damage (Naghibi et al., 2007), making this test a non-specific marker for liver damage.
Resveratrol is a natural compound extensively studied in preclinical studies as a nutraceutical and therapeutic agent. In addition, the antioxidant properties of resveratrol have been demonstrated in a wide range of hepatic disorders (Pan et al., 2017; Bircan et al., 2018). The antioxidant effect functions mainly by reducing ROS and eliminating direct free radicals, while improving the activity of endogenous antioxidant enzymes superoxide dismutase, catalase, and GSH (Carrizzo et al., 2013; de Oliveira et al., 2018). Furthermore, it has been reported that resveratrol is involved in several vital pathways regulating de novo fibrogenesis deposition in the liver (Hessin et al., 2017). For example, resveratrol (10 and 20 mg/kg/day) was administered to cirrhotic rats, where it reduced portal pressure, improved vasodilatory acetylcholine responsiveness, and reduced the production of thromboxane A2, resulting in liver tissue regeneration (Di Pascoli et al., 2013; Zhang et al., 2016).
Globally, liver illnesses continue to be a severe health burden. For the treatment of this category of disorders, new and secure therapeutic options are required. This study shows that resveratrol is a good alternative in this area. This approach could significantly improve potential resveratrol therapeutic applications. Understanding how resveratrol improves many liver disease conditions may lead to novel treatment possibilities. For example, resveratrol produces beneficial effects and reduces possible toxic effects when combined with other medications and substances. As a result, there is still future work to be done because there are still significant gaps in our knowledge about this chemical.
Data availability statement
The raw data supporting the conclusions of this article will be made available by the authors, without undue reservation.
Ethics statement
The study was conducted according to the guidelines for ethical scientific research at Umm Alqura University. The Biomedical Committee of Research Ethics at the faculty of medicine at Umm Al-Qura University approved the study (Approval No. HAPO-02-K-012-2022-06-1127).
Author contributions
FA, NA participated protocol design, data collection and in conducting the study. FA wrote the manuscript. NA analyzed the data. All authors reviewed the approved the final manuscript.
Funding
This project was funded by the Deanship for Research and Innovation, Ministry of Education in Saudi Arabia through project number: IFP22UQU4310453DSR244.
Acknowledgments
The authors extend their appreciation to the Deanship for Research and Innovation, Ministry of Education in Saudi Arabia for funding this research work through project number: IFP22UQU4310453DSR244.
Conflict of interest
The authors declare that the research was conducted in the absence of any commercial or financial relationships that could be construed as a potential conflict of interest.
Publisher’s note
All claims expressed in this article are solely those of the authors and do not necessarily represent those of their affiliated organizations, or those of the publisher, the editors and the reviewers. Any product that may be evaluated in this article, or claim that may be made by its manufacturer, is not guaranteed or endorsed by the publisher.
References
Abou Seif, H. S. (2016). Physiological changes due to hepatotoxicity and the protective role of some medicinal plants. Beni-suef Univ. J. basic Appl. Sci. 5 (2), 134–146. doi:10.1016/j.bjbas.2016.03.004
Acevedo, J. (2015). Multiresistant bacterial infections in liver cirrhosis: Clinical impact and new empirical antibiotic treatment policies. World J. hepatology 7 (7), 916–921. doi:10.4254/wjh.v7.i7.916
Adeyemi, O. S., and Adewumi, I. (2014). Biochemical evaluation of silver nanoparticles in wistar rats. Int. Sch. Res. Not. 2014, 196091. doi:10.1155/2014/196091
Adeyemi, O. S., and Akanji, M. A. (2011). Biochemical changes in the kidney and liver of rats following administration of ethanolic extract of Psidium guajava leaves. Hum. Exp. Toxicol. 30 (9), 1266–1274. doi:10.1177/0960327110388534
Ahmida, M. H. (2012). Protective role of curcumin in nephrotoxic oxidative damage induced by vancomycin in rats. Exp. Toxicol. Pathol. 64 (3), 149–153. doi:10.1016/j.etp.2010.07.010
Aldaz, A., Ortega, A., Idoate, A., Giraldez, J., Brugarolas, A., and BrugArolAs, A. (2000). Effects of hepatic function on vancomycin pharmacokinetics in patients with cancer. Ther. Drug Monit. 22 (3), 250–257. doi:10.1097/00007691-200006000-00004
Ali, S. S., Kasoju, N., Luthra, A., Singh, A., Sharanabasava, H., Sahu, A., et al. (2008). Indian medicinal herbs as sources of antioxidants. Food Res. Int. 41 (1), 1–15. doi:10.1016/j.foodres.2007.10.001
Apte, U., and Krishnamurthy, P. (2011). Detoxification Functions of the Liver. Molecular pathology of liver diseases. Springer, 147–163.
Badran, E., Shamayleh, A., and Irshaid, Y. (2011). Pharmacokinetics of vancomycin in neonates admitted to the neonatology unit at the Jordan University Hospital. Int. J. Clin. Pharmacol. Ther. 49 (4), 252–257. doi:10.5414/CP201456
Bakan, E., Taysi, S., Polat, M. F., Dalga, S., Umudum, Z., Bakan, N., et al. (2002). Nitric oxide levels and lipid peroxidation in plasma of patients with gastric cancer. Jpn. J. Clin. Oncol. 32 (5), 162–166. doi:10.1093/jjco/hyf035
Bamgbola, O. (2016). Review of vancomycin-induced renal toxicity: An update. Ther. Adv. Endocrinol. Metab. 7 (3), 136–147. doi:10.1177/2042018816638223
Banez, M. J., Geluz, M. I., Chandra, A., Hamdan, T., Biswas, O. S., Bryan, N. S., et al. (2020). A systemic review on the antioxidant and anti-inflammatory effects of resveratrol, curcumin, and dietary nitric oxide supplementation on human cardiovascular health. Nutr. Res. 78, 11–26. doi:10.1016/j.nutres.2020.03.002
Bataller, R., and Brenner, D. A. (2005). Liver fibrosis. J. Clin. investigation 115 (2), 209–218. doi:10.1172/JCI24282
Bergmann, J., Müller, M., Baumann, N., Reichert, M., Heneweer, C., Bolik, J., et al. (2017). IL-6 trans-signaling is essential for the development of hepatocellular carcinoma in mice. Hepatology 65 (1), 89–103. doi:10.1002/hep.28874
Bernal, W., and Wendon, J. (2013). Acute liver failure. N. Engl. J. Med. 369 (26), 2525–2534. doi:10.1056/NEJMra1208937
Bircan, F., Pasaoglu, O., and Turkozkan, N. (2018). The effects of resveratrol on hepatic oxidative stress in metabolic syndrome model induced by high fructose diet. Bratisl. Lek. listy 119 (1), 36–40. doi:10.4149/BLL_2018_008
Bissell, D. M., Gores, G. J., Laskin, D. L., and Hoofnagle, J. H. (2001). Drug-induced liver injury: Mechanisms and test systems. Hepatology 33 (4), 1009–1013. doi:10.1053/jhep.2001.23505
Breedt, J., Teras, J., Gardovskis, J., Maritz, F. J., Vaasna, T., Ross, D. P., et al. (2005). Safety and efficacy of tigecycline in treatment of skin and skin structure infections: Results of a double-blind phase 3 comparison study with vancomycin-aztreonam. Antimicrob. Agents Chemother. 49 (11), 4658–4666. doi:10.1128/AAC.49.11.4658-4666.2005
Brunetti, L., Song, J. H., Suh, D., Kim, H. J., Seong, Y. H., Lee, D. S., et al. (2020). The risk of vancomycin toxicity in patients with liver impairment. Ann. Clin. Microbiol. Antimicrob. 19 (1), 13. doi:10.1186/s12941-020-00354-2
Bujanda, L., Hijona, E., Larzabal, M., Beraza, M., Aldazabal, P., Garcia-Urkia, N., et al. (2008). Resveratrol inhibits nonalcoholic fatty liver disease in rats. BMC Gastroenterol. 8 (1), 40. doi:10.1186/1471-230X-8-40
Cadle, R. M., Mansouri, M. D., and Darouiche, R. O. (2006). Vancomycin-induced elevation of liver enzyme levels. Ann. Pharmacother. 40 (6), 1186–1189. doi:10.1345/aph.1G668
Çağlayan, C., Taslimi, P., Demir, Y., Küçükler, S., Kandemir, F. M., and Gulcin, I. (2019). The effects of zingerone against vancomycin-induced lung, liver, kidney and testis toxicity in rats: The behavior of some metabolic enzymes. J. Biochem. Mol. Toxicol. 33 (10), e22381. doi:10.1002/jbt.22381
Cao, Q., Batey, R., Pang, G., Russell, A., and Clancy, R. (1998). IL-6, IFN-gamma and TNF-alpha production by liver-associated T cells and acute liver injury in rats administered concanavalin A. Immunol. Cell Biol. 76 (6), 542–549. doi:10.1046/j.1440-1711.1998.00779.x
Carrizzo, A., Forte, M., Damato, A., Trimarco, V., Salzano, F., Bartolo, M., et al. (2013). Antioxidant effects of resveratrol in cardiovascular, cerebral and metabolic diseases. Food Chem. Toxicol. 61, 215–226. doi:10.1016/j.fct.2013.07.021
Chen, T., Zamora, R., Zuckerbraun, B., and Billiar, T. R. (2003). Role of nitric oxide in liver injury. Curr. Mol. Med. 3 (6), 519–526. doi:10.2174/1566524033479582
Chen, Y., Yang, X. Y., Zeckel, M., Killian, C., Hornbuckle, K., Regev, A., et al. (2011). Risk of hepatic events in patients treated with vancomycin in clinical studies: A systematic review and meta-analysis. Drug Saf. 34 (1), 73–82. doi:10.2165/11539560-000000000-00000
Choi, I., Kang, H. S., Yang, Y., and Pyun, K. H. (1994). IL-6 induces hepatic inflammation and collagen synthesis in vivo. Clin. Exp. Immunol. 95 (3), 530–535. doi:10.1111/j.1365-2249.1994.tb07031.x
Chupradit, S., Bokov, D., Zamanian, M. Y., Heidari, M., and Hakimizadeh, E. (2022). Hepatoprotective and therapeutic effects of resveratrol: A focus on anti-inflammatory and antioxidative activities. Fundam. Clin. Pharmacol. 36 (3), 468–485. doi:10.1111/fcp.12746
Das, R., Mitra, S., Tareq, A. M., Emran, T. B., Hossain, M. J., Alqahtani, A. M., et al. (2022). Medicinal plants used against hepatic disorders in Bangladesh: A comprehensive review. J. Ethnopharmacol. 282, 114588. doi:10.1016/j.jep.2021.114588
David, M. Z., and Daum, R. S. (2010). Community-associated methicillin-resistant Staphylococcus aureus: Epidemiology and clinical consequences of an emerging epidemic. Clin. Microbiol. Rev. 23 (3), 616–687. doi:10.1128/CMR.00081-09
Day, R. M., and Suzuki, Y. J. (2005). Cell proliferation, reactive oxygen and cellular glutathione. Dose-response 3 (3), 425–442. dose-response. doi:10.2203/dose-response.003.03.010
de Oliveira, M. R., Chenet, A. L., Duarte, A. R., Scaini, G., and Quevedo, J. (2018). Molecular mechanisms underlying the anti-depressant effects of resveratrol: A review. Mol. Neurobiol. 55 (6), 4543–4559. doi:10.1007/s12035-017-0680-6
Deshpande, U. R., Gadre, S. G., Raste, A. S., Pillai, D., Bhide, S. V., and Samuel, A. M. (1998). Protective effect of turmeric (Curcuma longa L.) extract on carbon tetrachloride-induced liver damage in rats. Indian J. Exp. Biol. 36 (6), 573–577.
Di Pascoli, M., Divi, M., Rodriguez-Vilarrupla, A., Rosado, E., Gracia-Sancho, J., Vilaseca, M., et al. (2013). Resveratrol improves intrahepatic endothelial dysfunction and reduces hepatic fibrosis and portal pressure in cirrhotic rats. J. Hepatol. 58 (5), 904–910. doi:10.1016/j.jhep.2012.12.012
Diesen, D. L., and Kuo, P. C. (2010). Nitric oxide and redox regulation in the liver: Part I. General considerations and redox biology in hepatitis. J. Surg. Res. 162 (1), 95–109. doi:10.1016/j.jss.2009.09.019
Ebrahim, H. A., Kamar, S. S., Haidara, M. A., Latif, N. S. A., Ellatif, M. A., ShamsEldeen, A. M., et al. (2022). Association of resveratrol with the suppression of TNF-α/NF-kB/iNOS/HIF-1α axis-mediated fibrosis and systemic hypertension in thioacetamide-induced liver injury. Naunyn Schmiedeb. Arch. Pharmacol. 395 (9), 1087–1095. doi:10.1007/s00210-022-02264-w
Ekhlasi, G., Zarrati, M., Agah, S., Hosseini, A. F., Hosseini, S., Shidfar, S., et al. (2017). Effects of symbiotic and vitamin E supplementation on blood pressure, nitric oxide and inflammatory factors in non-alcoholic fatty liver disease. EXCLI J. 16, 278–290. doi:10.17179/excli2016-846
El Bohi, K. M., Abdel-Motal, S. M., Khalil, S. R., Abd-Elaal, M. M., Metwally, M. M. M., and Wm, E. L. (2021). The efficiency of pomegranate (Punica granatum) peel ethanolic extract in attenuating the vancomycin-triggered liver and kidney tissues injury in rats. Environ. Sci. Pollut. Res. Int. 28 (6), 7134–7150. doi:10.1007/s11356-020-10999-3
Eskandari, M. R., Fard, J. K., Hosseini, M. J., and Pourahmad, J. (2012). Glutathione mediated reductive activation and mitochondrial dysfunction play key roles in lithium induced oxidative stress and cytotoxicity in liver. Biometals 25 (5), 863–873. doi:10.1007/s10534-012-9552-8
Fabbrini, E., and Magkos, F. (2015). Hepatic steatosis as a marker of metabolic dysfunction. Nutrients 7 (6), 4995–5019. doi:10.3390/nu7064995
Farhana, A., and Lappin, S. L. (2022). Biochemistry, lactate dehydrogenase. StatPearls. Treasure island (FL). StatPearls Publishing.
Fathy, M., Khalifa, E. M., and Fawzy, M. A. (2019). Modulation of inducible nitric oxide synthase pathway by eugenol and telmisartan in carbon tetrachloride-induced liver injury in rats. Life Sci. 216, 207–214. doi:10.1016/j.lfs.2018.11.031
Fernández, J., Tandon, P., Mensa, J., and Garcia Tsao, G. (2016). Antibiotic prophylaxis in cirrhosis: Good and bad. Hepatology 63 (6), 2019–2031. doi:10.1002/hep.28330
Florescu, I., Beuran, M., Dimov, R., Razbadauskas, A., Bochan, M., Fichev, G., et al. (2008). Efficacy and safety of tigecycline compared with vancomycin or linezolid for treatment of serious infections with methicillin-resistant Staphylococcus aureus or vancomycin-resistant enterococci: A phase 3, multicentre, double-blind, randomized study. J. Antimicrob. Chemother. 62 (1), i17–i28. doi:10.1093/jac/dkn250
Franco, R., and Cidlowski, J. A. (2009). Apoptosis and glutathione: Beyond an antioxidant. Cell Death Differ. 16 (10), 1303–1314. doi:10.1038/cdd.2009.107
Friedman, S. L. (2008). Mechanisms of hepatic fibrogenesis. Gastroenterology 134 (6), 1655–1669. doi:10.1053/j.gastro.2008.03.003
Gescher, A. J., and Steward, W. P. (2003). Relationship between mechanisms, bioavailibility, and preclinical chemopreventive efficacy of resveratrol: A conundrum. Cancer Epidemiol. Prev. Biomarkers 12 (10), 953–957.
Gewiese-Rabsch, J., Drucker, C., Malchow, S., Scheller, J., and Rose-John, S. (2010). Role of IL-6 trans-signaling in CCl₄induced liver damage. Biochimica Biophysica Acta (BBA)-Molecular Basis Dis. 1802 (11), 1054–1061. doi:10.1016/j.bbadis.2010.07.023
Gupta, A., Biyani, M., Khaira, A., and KhAirA, A. (2011). Vancomycin nephrotoxicity: Myths and facts. Neth J. Med. 69 (9), 379–383.
Gupta, K. J., Fernie, A. R., Kaiser, W. M., and van Dongen, J. T. (2011). On the origins of nitric oxide. Trends Plant Sci. 16 (3), 160–168. doi:10.1016/j.tplants.2010.11.007
Hadizadeh, F., Faghihimani, E., and Adibi, P. (2017). Nonalcoholic fatty liver disease: Diagnostic biomarkers. World J. Gastrointest. Pathophysiol. 8 (2), 11–26. doi:10.4291/wjgp.v8.i2.11
Halliwell, B. (2007). Dietary polyphenols: Good, bad, or indifferent for your health? Cardiovasc. Res. 73 (2), 341–347. doi:10.1016/j.cardiores.2006.10.004
Hessin, A. F., Hegazy, R. R., Hassan, A. A., Yassin, N. Z., and Kenawy, S. A. (2017). Resveratrol prevents liver fibrosis via two possible pathways: Modulation of alpha fetoprotein transcriptional levels and normalization of protein kinase C responses. Indian J. Pharmacol. 49 (4), 282–289. doi:10.4103/ijp.IJP_299_16
Hwang, J. H., Lee, J. H., Hwang, J. H., Chung, K. M., Lee, E. J., Yoon, Y. J., et al. (2015). Comparison of arbekacin and vancomycin in treatment of chronic suppurative otitis media by methicillin resistant Staphylococcus aureus. J. Korean Med. Sci. 30 (6), 688–693. doi:10.3346/jkms.2015.30.6.688
Ibrahim, J., Kabiru, A. Y., Abdulrasheed-Adeleke, T., Lawal, B., and Adewuyi, A. H. (2020). Antioxidant and hepatoprotective potentials of curcuminoid isolates from turmeric (Curcuma longa) rhizome on CCl4-induced hepatic damage in Wistar rats. J. Taibah Univ. Sci. 14 (1), 908–915. doi:10.1080/16583655.2020.1790928
Iwakiri, Y., and Kim, M. Y. (2015). Nitric oxide in liver diseases. Trends Pharmacol. Sci. 36 (8), 524–536. doi:10.1016/j.tips.2015.05.001
Jalan, R., Gines, P., Olson, J. C., Mookerjee, R. P., Moreau, R., Garcia-Tsao, G., et al. (2012). Acute-on chronic liver failure. J. hepatology 57 (6), 1336–1348. doi:10.1016/j.jhep.2012.06.026
Kaplowitz, N. (2001). Drug-induced liver disorders: Implications for drug development and regulation. Drug Saf. 24 (7), 483–490. doi:10.2165/00002018-200124070-00001
Kashima, J., Okuma, Y., Shimizuguchi, R., and Chiba, K. (2018). Bile duct obstruction in a patient treated with nivolumab as second-line chemotherapy for advanced non-small-cell lung cancer: A case report. Cancer Immunol. Immunother. 67 (1), 61–65. doi:10.1007/s00262-017-2062-3
King, D. W., and Smith, M. A. (2004). Proliferative responses observed following vancomycin treatment in renal proximal tubule epithelial cells. Toxicol Vitro 18 (6), 797–803. doi:10.1016/j.tiv.2004.03.013
Kohno, S., Yamaguchi, K., Aikawa, N., Sumiyama, Y., Odagiri, S., Aoki, N., et al. (2007). Linezolid versus vancomycin for the treatment of infections caused by methicillin-resistant Staphylococcus aureus in Japan. J. Antimicrob. Chemother. 60 (6), 1361–1369. doi:10.1093/jac/dkm369
Korde Choudhari, S., Chaudhary, M., Bagde, S., Gadbail, A. R., and Joshi, V. (2013). Nitric oxide and cancer: A review. World J. Surg. Oncol. 11 (1), 118–211. doi:10.1186/1477-7819-11-118
Kouijzer, I. J., van Leerdam, E. J., Gompelman, M., Tuinte, R. A., Aarntzen, E. H., Berrevoets, M. A., et al. (2021). Intravenous to oral switch in complicated Staphylococcus aureus bacteremia without endovascular infection: A retrospective single-center cohort study. Clin. Infect. Dis. 73 (5), 895–898. doi:10.1093/cid/ciab156
Kucukler, S., Darendelioğlu, E., Caglayan, C., Ayna, A., Yıldırım, S., and Kandemir, F. M. (2020). Zingerone attenuates vancomycin-induced hepatotoxicity in rats through regulation of oxidative stress, inflammation and apoptosis. Life Sci. 259, 118382. doi:10.1016/j.lfs.2020.118382
Kurland, I. J., Broin, P. O., Golden, A., Su, G., Meng, F., Liu, L., et al. (2015). Integrative metabolic signatures for hepatic radiation injury. PLoS One 10 (6), e0124795. doi:10.1371/journal.pone.0124795
Labban, S., Alghamdi, B. S., Alshehri, F. S., and Kurdi, M. (2021). Effects of melatonin and resveratrol on recognition memory and passive avoidance performance in a mouse model of Alzheimer's disease. Behav. Brain Res. 402, 113100. doi:10.1016/j.bbr.2020.113100
Labban, S., Alshehri, F. S., Kurdi, M., Alatawi, Y., and Alghamdi, B. S. (2021). Melatonin improves short-term spatial memory in a mouse model of alzheimer's disease. Degener. Neurol. Neuromuscul. Dis. 11, 15–27. doi:10.2147/DNND.S291172
D. Larrey (Editor) (2002). “Epidemiology and individual susceptibility to adverse drug reactions affecting the liver,” Seminars in liver disease (Medical Publishers, Inc.). Copyright© 2002 by Thieme333 Seventh Avenue, New.
Laskin, J. D., Heck, D. E., Gardner, C. R., and Laskin, D. L. (2001). Prooxidant and antioxidant functions of nitric oxide in liver toxicity. Antioxid. Redox Signal 3 (2), 261–271. doi:10.1089/152308601300185214
Lee, E. S., Shin, M. O., Yoon, S., and Moon, J. O. (2010). Resveratrol inhibits dimethylnitrosamine-induced hepatic fibrosis in rats. Arch. Pharm. Res. 33 (6), 925–932. doi:10.1007/s12272-010-0616-4
López-Posadas, R., Gonzalez, R., Ballester, I., Martínez-Moya, P., Romero-Calvo, I., Suárez, M. D., et al. (2011). Tissue-nonspecific alkaline phosphatase is activated in enterocytes by oxidative stress via changes in glycosylation. Inflamm. bowel Dis. 17 (2), 543–556. doi:10.1002/ibd.21381
Ma, Z., Sheng, L., Li, J., Qian, J., Wu, G., Wang, Z., et al. (2022). Resveratrol alleviates hepatic fibrosis in associated with decreased endoplasmic reticulum stress-mediated apoptosis and inflammation. Inflammation 45 (2), 812–823. doi:10.1007/s10753-021-01586-w
Mari, M., Morales, A., Colell, A., Garcia-Ruiz, C., and Fernandez-Checa, J. C. (2009). Mitochondrial glutathione, a key survival antioxidant. Antioxid. Redox Signal 11 (11), 2685–2700. doi:10.1089/ARS.2009.2695
Mehrzadi, S., Fatemi, I., Esmaeilizadeh, M., Ghaznavi, H., Kalantar, H., and Goudarzi, M. (2018). Hepatoprotective effect of berberine against methotrexate induced liver toxicity in rats. Biomed. Pharmacother. 97, 233–239. doi:10.1016/j.biopha.2017.10.113
Miguel, C., Noya-Riobó, M., Mazzone, G., Villar, M., and Coronel, M. (2021). Antioxidant, anti-inflammatory and neuroprotective actions of resveratrol after experimental nervous system insults. Special focus on the molecular mechanisms involved. Neurochem. Int. 150, 105188. doi:10.1016/j.neuint.2021.105188
Naghibi, B., Ghafghazi, T., Hajhashemi, V., and Talebi, A. (2007). Vancomycin-induced nephrotoxicity in rats: Is enzyme elevation a consistent finding in tubular injury? J. Nephrol. 20 (4), 482–488.
Novo, E., and Parola, M. (2008). Redox mechanisms in hepatic chronic wound healing and fibrogenesis. Fibrogenes. Tissue Repair 1, 5–58. doi:10.1186/1755-1536-1-5
Oktem, F., Arslan, M. K., Ozguner, F., Candir, O., Yilmaz, H. R., Ciris, M., et al. (2005). In vivo evidences suggesting the role of oxidative stress in pathogenesis of vancomycin-induced nephrotoxicity: Protection by erdosteine. Toxicology 215 (3), 227–233. doi:10.1016/j.tox.2005.07.009
Olaniyan, M. F., Atibor, R. A., and Afolabi, T. (2018). Evaluation of tumor necrosis factor alpha (TNFα), interleukin 4, interleukin 6, aspartate aminotransferase, and alanine aminotransferase in rabbits overdosed with ibuprofen and supplemented with guava leaf (Psidium guajava) extract. Biomed. Biotechnol. Res. J. (BBRJ) 2 (4), 254. doi:10.4103/bbrj.bbrj_121_18
Omara, F., Aziz, S., El-Sheikh, S., and Said, M. (2021). Ascorbic acid attenuated the hepatic parenchymal necrosis induced by azithromycin-etoricoxib interaction in rats. J. Anim. Health Prod. 9 (1), 42–48. doi:10.17582/journal.jahp/2021/9.s1.42.48
Pan, Y., Zhang, H., Zheng, Y., Zhou, J., Yuan, J., Yu, Y., et al. (2017). Resveratrol exerts antioxidant effects by activating SIRT2 to deacetylate Prx1. Biochemistry 56 (48), 6325–6328. doi:10.1021/acs.biochem.7b00859
Pandit, A., Sachdeva, T., and Bafna, P. (2012). Drug-induced hepatotoxicity: A review. J. Appl. Pharm. Sci. 2 (5), 233–243.
Parthasarathy, M., and Evan Prince, S. (2021). The potential effect of phytochemicals and herbal plant remedies for treating drug-induced hepatotoxicity: A review. Mol. Biol. Rep. 48 (5), 4767–4788. doi:10.1007/s11033-021-06444-4
Philips, C. A., Ahamed, R., Rajesh, S., George, T., Mohanan, M., and Augustine, P. (2020). Comprehensive review of hepatotoxicity associated with traditional Indian Ayurvedic herbs. World J. Hepatol. 12 (9), 574–595. doi:10.4254/wjh.v12.i9.574
Picon-Pages, P., Garcia-Buendia, J., and Munoz, F. J. (2019). Functions and dysfunctions of nitric oxide in brain. Biochim. Biophys. Acta Mol. Basis Dis. 1865 (8), 1949–1967. doi:10.1016/j.bbadis.2018.11.007
Pourhanifeh, M. H., Shafabakhsh, R., Reiter, R. J., and Asemi, Z. (2019). The effect of resveratrol on neurodegenerative disorders: Possible protective actions against autophagy, apoptosis, inflammation and oxidative stress. Curr. Pharm. Des. 25 (19), 2178–2191. doi:10.2174/1381612825666190717110932
Regal, R. E., Ren, S. P., Paige, G., and Alaniz, C. (2019). Evaluation of vancomycin dosing in patients with cirrhosis: Beginning de-liver-ations about a new nomogram. Hosp. Pharm. 54 (2), 125–129. doi:10.1177/0018578718772266
Rivera, H., Shibayama, M., Tsutsumi, V., Perez-Alvarez, V., and Muriel, P. (2008). Resveratrol and trimethylated resveratrol protect from acute liver damage induced by CCl4 in the rat. J. Appl. Toxicol. 28 (2), 147–155. doi:10.1002/jat.1260
Rubiolo, J. A., and Vega, F. V. (2008). Resveratrol protects primary rat hepatocytes against necrosis induced by reactive oxygen species. Biomed. Pharmacother. 62 (9), 606–612. doi:10.1016/j.biopha.2008.06.034
Sadeghi, H., Nikbakht, M. R., Izadpanah, G., and Sabzali, S. (2008). Hepatoprotective effect of Cichorium intybus on CCl4-induced liver damage in rats. Afr. J. Biochem. Res. 2 (6), 141–144.
Sahin, M., Cam, H., Olgar, S., Tunc, S. E., Arslan, C., Uz, E., et al. (2006). Protective role of erdosteine on vancomycin-induced oxidative stress in rat liver. Mol. Cell Biochem. 291 (1-2), 155–160. doi:10.1007/s11010-006-9209-4
Santos, M. A., Franco, F. N., Caldeira, C. A., de Araújo, G. R., Vieira, A., Chaves, M. M., et al. (2021). Antioxidant effect of Resveratrol: Change in MAPK cell signaling pathway during the aging process. Archives Gerontology Geriatrics 92, 104266. doi:10.1016/j.archger.2020.104266
Sebai, H., Sani, M., Yacoubi, M. T., Aouani, E., Ghanem-Boughanmi, N., and Ben-Attia, M. (2010). Resveratrol, a red wine polyphenol, attenuates lipopolysaccharide-induced oxidative stress in rat liver. Ecotoxicol. Environ. Saf. 73 (5), 1078–1083. doi:10.1016/j.ecoenv.2009.12.031
Sener, G., Toklu, H. Z., Sehirli, A. O., Velioglu-Ogunc, A., Cetinel, S., and Gedik, N. (2006). Protective effects of resveratrol against acetaminophen-induced toxicity in mice. Hepatol. Res. 35 (1), 62–68. doi:10.1016/j.hepres.2006.02.005
Senior, J. R. (2012). Alanine aminotransferase: A clinical and regulatory tool for detecting liver injury-past, present, and future. Clin. Pharmacol. Ther. 92 (3), 332–339. doi:10.1038/clpt.2012.108
Shah, A. A., Patton, M., Chishty, W. H., and Hussain, A. (2010). Analysis of elevated liver enzymes in an acute medical setting: Jaundice may indicate increased survival in elderly patients with bacterial sepsis. Saudi J. Gastroenterol. 16 (4), 260–263. doi:10.4103/1319-3767.70609
Shao, M., Xu, Q., Wu, Z., Chen, Y., Shu, Y., Cao, X., et al. (2020). Exosomes derived from human umbilical cord mesenchymal stem cells ameliorate IL-6-induced acute liver injury through miR-455-3p. Stem Cell Res. Ther. 11 (1), 37–13. doi:10.1186/s13287-020-1550-0
Sibai, B. M. (2004). Diagnosis, controversies, and management of the syndrome of hemolysis, elevated liver enzymes, and low platelet count. Obstet. Gynecol. 103 (5), 981–991. doi:10.1097/01.AOG.0000126245.35811.2a
Srivastava, P., Prabhu, V. V., Yadav, N., Gogada, R., and Chandra, D. (2013). Effect of dietary resveratrol in the treatment of cancer. Cancer chemoprevention and treatment by diet therapy. Springer, 1–22.
Steinmetz, T., Eliakim-Raz, N., Goldberg, E., Leibovici, L., and Yahav, D. (2015). Association of vancomycin serum concentrations with efficacy in patients with MRSA infections: A systematic review and meta-analysis. Clin. Microbiol. Infect. 21 (7), 665–673. doi:10.1016/j.cmi.2015.04.003
Taga, T., and Kishimoto, T. (1997). Gp130 and the interleukin-6 family of cytokines. Annu. Rev. Immunol. 15, 797–819. doi:10.1146/annurev.immunol.15.1.797
Takai, S., Oda, S., Tsuneyama, K., Fukami, T., Nakajima, M., and Yokoi, T. (2016). Establishment of a mouse model for amiodarone-induced liver injury and analyses of its hepatotoxic mechanism. J. Appl. Toxicol. 36 (1), 35–47. doi:10.1002/jat.3141
Thiim, M., and Friedman, L. S. (2003). Hepatotoxicity of antibiotics and antifungals. Clin. Liver Dis. 7 (2), 381–399. vi-vii. doi:10.1016/s1089-3261(03)00021-7
Tong, Y., Yu, X., Huang, Y., Zhang, Z., Mi, L., and Bao, Z. (2022). Hepatic-targeted nano-enzyme with resveratrol loading for precise relief of nonalcoholic steatohepatitis. ChemMedChem. doi:10.1002/cmdc.202200468
Tsochatzis, E. A., Bosch, J., and Burroughs, A. K. (2014). Liver cirrhosis. Lancet 383 (9930), 1749–1761. doi:10.1016/S0140-6736(14)60121-5
Tsutsuura, M., Moriyama, H., Kojima, N., Mizukami, Y., Tashiro, S., Osa, S., et al. (2021). The monitoring of vancomycin: A systematic review and meta-analyses of area under the concentration-time curve-guided dosing and trough-guided dosing. BMC Infect. Dis. 21 (1), 153. doi:10.1186/s12879-021-05858-6
Wang, Y., Jiang, Y., Fan, X., Tan, H., Zeng, H., Wang, Y., et al. (2015). Hepato-protective effect of resveratrol against acetaminophen-induced liver injury is associated with inhibition of CYP-mediated bioactivation and regulation of SIRT1–p53 signaling pathways. Toxicol. Lett. 236 (2), 82–89. doi:10.1016/j.toxlet.2015.05.001
Wang, Y. Y., Chen, M. T., Hong, H. M., Wang, Y., Li, Q., Liu, H., et al. (2018). Role of reduced nitric oxide in liver cell apoptosis inhibition during liver damage. Arch. Med. Res. 49 (4), 219–225. doi:10.1016/j.arcmed.2018.09.001
Winter, A. N., Ross, E. K., Daliparthi, V., Sumner, W. A., Kirchhof, D. M., Manning, E., et al. (2017). A cystine-rich whey supplement (Immunocal®) provides neuroprotection from diverse oxidative stress-inducing agents in vitro by preserving cellular glutathione. Oxid. Med. Cell Longev. 2017, 3103272. doi:10.1155/2017/3103272
Wu, K., Fan, J., Huang, X., Wu, X., and Guo, C. (2018). Hepatoprotective effects exerted by Poria Cocos polysaccharides against acetaminophen-induced liver injury in mice. Int. J. Biol. Macromol. 114, 137–142. doi:10.1016/j.ijbiomac.2018.03.107
Xia, H. M., Wang, J., Xie, X. J., Xu, L. J., and Tang, S. Q. (2019). Green tea polyphenols attenuate hepatic steatosis, and reduce insulin resistance and inflammation in high-fat diet-induced rats. Int. J. Mol. Med. 44 (4), 1523–1530. doi:10.3892/ijmm.2019.4285
Yamauchi-Takihara, K., and Kishimoto, T. (2000). Cytokines and their receptors in cardiovascular diseases—Role of gp130 signalling pathway in cardiac myocyte growth and maintenance. Int. J. Exp. pathology 81 (1), 1–16. doi:10.1046/j.1365-2613.2000.00139.x
Yilmaz, S., Kaya, E., and Comakli, S. (2017). Vitamin E (alpha tocopherol) attenuates toxicity and oxidative stress induced by aflatoxin in rats. Adv. Clin. Exp. Med. 26 (6), 907–917. doi:10.17219/acem/66347
Yin, Y., Liu, H., Zheng, Z., Lu, R., and Jiang, Z. (2019). Genistein can ameliorate hepatic inflammatory reaction in nonalcoholic steatohepatitis rats. Biomed. Pharmacother. 111, 1290–1296. doi:10.1016/j.biopha.2019.01.004
Yousefian, M., Shakour, N., Hosseinzadeh, H., Hayes, A. W., Hadizadeh, F., and Karimi, G. (2019). The natural phenolic compounds as modulators of NADPH oxidases in hypertension. Phytomedicine 55, 200–213. doi:10.1016/j.phymed.2018.08.002
Yuan, L., and Kaplowitz, N. (2009). Glutathione in liver diseases and hepatotoxicity. Mol. Asp. Med. 30 (1-2), 29–41. doi:10.1016/j.mam.2008.08.003
Zhang, C., Wang, N., Xu, Y., Tan, H. Y., Li, S., and Feng, Y. (2018). Molecular mechanisms involved in oxidative stress-associated liver injury induced by Chinese herbal medicine: An experimental evidence-based literature review and network Pharmacology study. Int. J. Mol. Sci. 19 (9), 2745. doi:10.3390/ijms19092745
Zhang, D. Q., Sun, P., Jin, Q., Li, X., Zhang, Y., Zhang, Y. J., et al. (2016). Resveratrol regulates activated hepatic stellate cells by modulating NF-κB and the PI3K/akt signaling pathway. J. Food Sci. 81 (1), H240–H245. doi:10.1111/1750-3841.13157
Zhou, Y., Chen, K., He, L., Xia, Y., Dai, W., Wang, F., et al. (2015). The protective effect of resveratrol on concanavalin-A-induced acute hepatic injury in mice. Gastroenterol. Res. Pract. 2015, 506390. doi:10.1155/2015/506390
Keywords: vancomycin, resveratrol, hepatotoxicity, hepatoprotection, glycopeptide
Citation: Alshehri FS and Alorfi NM (2023) Protective role of resveratrol against VCM-induced hepatotoxicity in male wistar rats. Front. Pharmacol. 14:1130670. doi: 10.3389/fphar.2023.1130670
Received: 23 December 2022; Accepted: 30 January 2023;
Published: 07 February 2023.
Edited by:
Ochuko Lucky Erukainure, University of the Free State, South AfricaReviewed by:
Rebecca Reddy, Durban University of Technology, South AfricaFils Armand Ella, University of the Free State, South Africa
Babangida Sanusi, Ahmadu Bello University, Nigeria
Copyright © 2023 Alshehri and Alorfi. This is an open-access article distributed under the terms of the Creative Commons Attribution License (CC BY). The use, distribution or reproduction in other forums is permitted, provided the original author(s) and the copyright owner(s) are credited and that the original publication in this journal is cited, in accordance with accepted academic practice. No use, distribution or reproduction is permitted which does not comply with these terms.
*Correspondence: Fahad S. Alshehri, ZnNzaGVocmlAdXF1LmVkdS5zYQ==