- 1Seoul National University College of Medicine, Seoul, Republic of Korea
- 2Department of Anesthesiology and Pain Medicine, Seoul National University Hospital, Seoul, Republic of Korea
- 3Department of Clinical Pharmacology and Therapeutics, Seoul National University Hospital, Seoul, Republic of Korea
Background: Sugammadex is known to reverse neuromuscular blockade induced by non-depolarizing agents. In children, the recommended dose for reversal of moderate neuromuscular blockade is 2 mg/kg. We investigated the pharmacokinetics and pharmacodynamics of sugammadex in Korean children.
Methods: Children (2–17 years of age) undergoing brain or spine surgery were enrolled and randomly assigned to control (neostigmine) and 2, 4, or 8 mg/kg sugammadex groups. Following induction of anesthesia and monitoring of the response to train-of-four stimulation, 1 mg/kg rocuronium was intravenously administered. Upon reappearance of the second twitch to train-of-four stimulation, the study drug was administered according to group allocation. The plasma concentrations of rocuronium and sugammadex were serially measured at nine predefined time points following study drug administration. To determine efficacy, we measured the time elapsed from drug administration to recovery of T4/T1 ≥ 0.9. For pharmacokinetics, non-compartmental analysis was performed and we monitored adverse event occurrence from the time of study drug administration until 24 h post-surgery.
Results: Among the 29 enrolled participants, the sugammadex (2 mg/kg) and control groups showed recovery times [median (interquartile range)] of 1.3 (1.0–1.9) and 7.7 (5.3–21.0) min, respectively (p = 0.002). There were no significant differences in recovery time among the participants in sugammadex groups. The pharmacokinetics of sugammadex were comparable to those of literature findings. Although two hypotensive events related to sugammadex were observed, no intervention was necessary.
Conclusion: The findings of this pharmacokinetic analysis and efficacy study of sugammadex in Korean children indicated that sugammadex (2 mg/kg) may be safely administered for reversing moderate neuromuscular blockade. Some differences in pharmacokinetics of sugammadex were observed according to age.
Clinical Trial Registration: http://clinicaltrials.gov (NCT04347486)
1 Introduction
In the field of anesthesia, it is well established that sugammadex can effectively reverse neuromuscular blockade induced by steroidal non-depolarizing neuromuscular blocking agents. Sugammadex binds to rocuronium by forming a one-to-one complex (Gijsenbergh et al., 2005), without causing muscarinic adverse effects or residual blockade, which can be a potential risk for patients when administering neostigmine (Gijsenbergh et al., 2005; Sorgenfrei et al., 2006).
During administration of general anesthesia for surgery, the degree of neuromuscular blockade is peripherally monitored, generally via train-of-four stimulation, in which 50 mA of electrical stimulation is applied to the peripheral nerves (Ali et al., 1970), primarily the ulnar nerve. Neuromuscular blockade is typically considered a “moderate” blockage, which is suitable for most types of surgeries, when the train-of-four count is 2. To reverse this degree of blockage, 2 mg/kg sugammadex is recommended for both adults and children (Sorgenfrei et al., 2006).
The pharmacokinetics of sugammadex in adult patients have been well reported, in which it is characterized by a linear relationship up to 16 mg/kg and a volume of distribution of 11–14 L (Keating, 2016). Sugammadex is mostly eliminated via renal excretion with an elimination half-life of approximately 2 h (Keating, 2016). Accordingly, the clearance of sugammadex is significantly reduced in patients with renal failure (Staals et al., 2010). In addition, ethnicity is associated with the pharmacokinetics of sugammadex (Keating, 2016).
However, there have been comparatively few pharmacokinetic studies of sugammadex in the pediatric population. Among those that have been conducted, the findings of an early study involving eight infants, 22 children, 28 adolescents, and 26 adults indicated that the efficacy of sugammadex was comparable among the different age groups (Plaud et al., 2009). However, due to the sparsity of suitable samples, the associated pharmacokinetics could not be sufficiently assessed (Plaud et al., 2009). Although several randomized clinical trials suggested comparable efficacy and safety profiles in pediatric and adult patients (Plaud et al., 2009; Ozgun et al., 2014; Won et al., 2016; Ammar et al., 2017; Liu et al., 2017; Matsui et al., 2019; Voss et al., 2022), precise evaluation would be necessary for specific dose adjustment in the pediatric population.
Therefore, we conducted a randomized clinical trial to evaluate the pharmacokinetics, efficacy, and safety of sugammadex in the context of reversing moderate neuromuscular blockade in Korean children. The study was designed to complement a previous study by our study group, in which we evaluate the pharmacokinetics, efficacy, and safety of sugammadex in the context of intense neuromuscular blockade (Ji et al., 2023). In the present study, we sought to evaluate whether a 2 mg/kg dose of sugammadex could effectively reverse neuromuscular blockade on reappearance of T2 in response to train-of-four stimulation.
2 Methods
2.1 Study design and population
This study was designed as a randomized, controlled, single-blinded exploratory study comparing groups administered different doses of sugammadex, and was conducted in a single tertiary hospital located in Seoul, Republic of Korea. The study adhered to the tenets of the Declaration of Helsinki (revised in 2013), and the protocol was approved by the Institutional Review Board of Seoul National University Hospital (no.: 2002-148-1105, approval date: 03/25/2020) and the Ministry of Food and Drug Safety of the Republic of Korea (no.: 32,828, approval date: 04/13/2020). The study was registered at http://clinicaltrials.gov (NCT04347486, principal investigator: Hee-Soo Kim, published date: 04/14/2020) and was conducted in accordance with the Good Clinical Practice guidelines of the International Council for Harmonization and the Declaration of Helsinki. Participants were recruited between April 2020 and December 2021.
Children aged between 2 and 17 years who were scheduled to undergo elective brain or spinal surgery under general anesthesia were enrolled. Written informed consent was obtained from the parent(s) of each participant, as well as from the participants older than 6 years of age. The exclusion criteria were a history of hypersensitivity to any anesthetic agent, presence of cardiovascular or urological disease, presence of renal or hepatic impairment, use of a neuromuscular blocking agent or drugs that can affect the action of neuromuscular blocking agents prior to anesthetic induction, a history of malignant hyperthermia, anticipation of massive hemorrhage during surgery, and the refusal of one or both parents or legal guardians to permit enrollment.
2.2 Study protocol
When the participants arrived at the operating room, non-invasive blood pressure, oxygen levels (determined using pulse oximetry), and heart activity (determined using electrocardiography) were monitored. Anesthesia was induced by intravenous (IV) injection of sodium thiopental or propofol. After loss of consciousness, anesthesia was maintained with the continuous IV infusion of an opioid and propofol. To monitor neuromuscular blockade, a ToFscan® device (IDMED, Marseille, France) was attached to each participant’s unilateral ulnar nerve. Train-of-four stimulation, which applies four twitch stimulations with an intensity of 50 mA and frequency of 2 Hz, was commenced and repeated at 15-s intervals until the end of surgery. The responses were measured via acceleromyography and automatically recorded on a computer using a vital recorder (Lee and Jung, 2018). Thereafter, 1 mg/kg of rocuronium was administered IV, followed by arterial catheterization at an extremity for continuous monitoring of invasive blood pressure. On the reappearance of the second twitch (T2) in response to the train-of-four stimulation, the study drug was administered IV.
2.3 Randomization and blinding
Allocation of patients to treatment groups was determined based on a randomization table obtained from https://sealedenvelope.com/. Having enrolled participants, they were allocated to one of the following groups: a control group (0.03 mg/kg neostigmine) or groups treated with 2, 4, or 8 mg/kg sugammadex. The study groups were assembled by a single anesthesiologist. The participants and their parents were blinded to group allocation.
2.4 Pharmacokinetic measurements
Nine arterial blood samples were collected for pharmacokinetic measurements: 2 min after rocuronium injection, and immediately prior to and at 2, 5, 15, 60, 120, 240, and 480 min post-administration of the study drug. For each sampling time point, the plasma concentrations of rocuronium and sugammadex were measured. In all groups, the concentration of sugammadex was not measured at the first time point and at all points in the control group. When sampling was not performed at the exact scheduled time, the actual sampling time was recorded (Choi et al., 2013).
2.5 Measurement of plasma concentrations
At each pharmacokinetic sampling point, 1 mL of arterial blood was withdrawn and immediately stored in a sodium heparin tube (BD Vacutainer® sodium heparin [N] 75 USP Units; Becton Dickinson Korea, Seoul, Korea). After centrifuging at 3,000 rpm (1,167 × g) for 10 min, the supernatant was stored in a sterile internal cryogenic vial (Cryotain™; SCILAB Korea, Seoul, Korea). The collected specimens were stored in a freezer below −70°C until analysis. The plasma concentrations of sugammadex and rocuronium were determined using liquid chromatography–tandem mass spectrometry. The assays were conducted in compliance with Good Laboratory Practice regulations. As this assay cannot be used to discriminate the sugammadex–rocuronium complex from the free forms of these drugs, all plasma concentrations were considered total plasma concentrations. The internal standards used for sugammadex and rocuronium were phenformin-d5 and rivastigmine-d4 (Toronto Research Chemicals, North York, Canada), respectively.
The assay methods for rocuronium and sugammadex were validated previously and partial validation was performed based on a risk-based approach (Farenc et al., 2001; de Zwart et al., 2011; Briggs et al., 2014; de Moraes et al., 2014). Calibration curves for rocuronium were established in the ranges of 100–10000 ng/mL (r = 0.9965) and 2–200 ng/mL (r = 0.9980). Accuracy was between −3.0% and −1.0%, −8.8 and −7.5 in the range of 100–10000 and 2–200 ng/mL, respectively. The corresponding precisions were 2.0%–10.0% and 2.8%–5.0%, respectively. Accuracy and precision of the diluted samples were −1.0% and 7.0%. Carryover effect was not detected.
The assay method for sugammadex was also validated. Calibration curve for sugammadex was established in the range of 0.1–100 μg/mL (r = 0.9981). Accuracy was between −2.7% and −1.0% and precision was between 1.7% and 6.2%. Accuracy and precision of the diluted samples were 6.0% and 4.7%, respectively. Carryover effect was not detected.
2.6 Pharmacokinetic assessments
The pharmacokinetic parameters of sugammadex and rocuronium were calculated based on non-compartmental analysis via Phoenix WinNonlin (Version 8.1; Certara United States, Princeton, NJ, United States). The maximum plasma concentration (Cmax) and the time to reach Cmax (Tmax) were obtained directly based on the observed values. The area under the plasma concentration-time curve (AUC) to the last measurable concentration (AUClast) was calculated using the linear up-log down trapezoidal method. AUC extrapolated to infinity (AUCinf) was calculated as the sum of AUClast and the last measurable concentration divided by the elimination phase constant (λz), which was regressed on the log-transformed concentration. The terminal phase half-life (t1/2) was calculated as the natural logarithm of 2 divided by λz. The volume of distribution and clearance were calculated as dose/(λz × AUCinf) and dose/AUCinf, respectively. Dose-normalized Cmax, AUClast, and AUCinf values were calculated as each parameter divided by the administered dose. We compared the obtained pharmacokinetic parameters with those of our previous study for reversal of intense neuromuscular blockade (Ji et al., 2023).
2.7 Pharmacodynamic measurements
Responses to train-of-four stimulation were continuously monitored at 15-s intervals. When the number of twitches was fewer than four, only the number of twitches was recorded. After the appearance of the fourth twitch, the ratio of the fourth twitch to the first twitch (T4/T1) was recorded, as this is considered to represent receptor occupancy by rocuronium. As a T4/T1 ratio ≥90% is considered sufficient for extubation, the time from administration of the study drug to recovery of a T4/T1 ratio ≥90% was taken to be the primary pharmacodynamic outcome.
2.8 Monitoring of safety
Electrocardiogram, mean blood pressure, pulse oximetry, and body temperature were monitored until 24 h after the end of surgery. We monitored the occurrence of any changes >30% of the baseline in heart rate or mean blood pressure, pulse oximetry of <92%, changes in body temperature to above 38.3°C or below 35.5°C, and other complications, including nausea, vomiting, urticaria, and any anaphylactic reactions.
2.9 Statistical analysis
Given the exploratory nature of the study, sample size was calculated empirically. We allotted eight children to each group on the basis of previously conducted pediatric pharmacokinetic studies for sugammadex that had assessed group sizes of 4–10 children (Plaud et al., 2009; Voss et al., 2022). Normality was assessed using the Shapiro–Wilk test. Baseline characteristics and recovery times of patients in the different study groups were compared using analysis of variance for normally distributed data, and the Kruskal–Wallis test with post hoc analysis using the Mann–Whitney U test with Bonferroni correction for non-normally distributed data. Analyses were performed using R ver. 4.0.3 (The R Foundation, http://r-project.org), with the acceptable alpha error rate set at 0.05.
For non-compartmental analysis, subgroup analysis was done for Cmax, AUClast, and AUCinf.: parameters of rocuronium were compared between each dose group and parameters of sugammadex were compared between age group classified according to a recent pharmacodynamics study (Voss et al., 2022) using the Kruskal–Wallis test. Non-parametric post hoc evaluation was performed with the Dwass, Steel, and Critchlow–Fligner procedures. Parameters from non-compartmental analyses of this study and our previous study (Ji et al., 2023) were compared for both drugs using Student’s t-test or Wilcoxon rank sum test. These analyses were done via SAS software (version 9.3; SAS Institute, Inc., Cary, NC, United States).
3 Results
Among the 32 enrolled participants, we analyzed the pharmacodynamic data of 29 children. The remaining three participants were excluded owing to technical errors in data recording. For pharmacokinetic analysis, we obtained measurements of 257 plasma concentrations of rocuronium from 29 participants and 148 concentrations of sugammadex from 21 participants. Two concentration data points of rocuronium from each participant were removed from the analysis owing to the inevitable additional administration of rocuronium during the study. Figure 1 shows a flow diagram of the Consolidated Standards of Reporting Trials. We detected no significant differences among the groups with respect to baseline characteristics, which are shown in detail in Table 1. Distributions of the ages and body weights of the participants are shown as histograms in Figure 2.
3.1 Efficacy of sugammadex
Average (±Standard deviation) time interval between rocuronium administration and sugammadex administration was 48.7 (±11.4) minutes. We found that the time [median (interquartile range)] from administration of sugammadex to achieving a T4/T1 ratio of 0.9 in response to train-of-four stimulation differed significantly among the groups (p < 0.001). For the control group, we recorded a recovery time of 7.7 (5.3–21.0) min, whereas that for participants in the 2, 4, and 8 mg/kg sugammadex groups was 1.3 (1.0–1.9), 0.9 (0.8–0.9), and 0.6 (0.4–1.0) min, respectively. In the post hoc analysis, we detected a significant difference between the control group and each of the groups treated with sugammadex (p = 0.002, 0.001, and 0.001 for the groups treated with 2, 4, and 8 mg/kg sugammadex, respectively). However, there were no significant differences among the groups treated with sugammadex. Figure 3 shows box plots of the recovery time for each group.
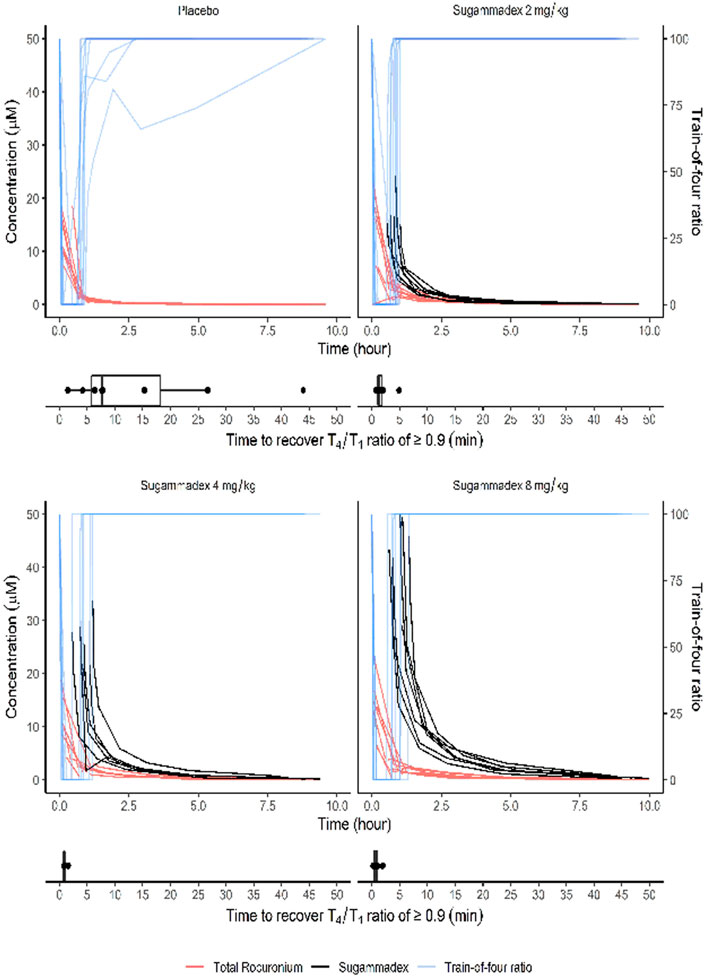
FIGURE 3. The T4/T1 ratio in response to train-of-four stimulation shown together with plasma molar concentrations of sugammadex and rocuronium. The box plots show the time from study drug administration to recovery of a T4/T1 ratio ≥0.9 in response to train-of-four stimulation. Molar concentrations were calculated based on the molecular weights of rocuronium (529.8 g/mol) and sugammadex (2,002 g/mol).
3.2 Pharmacokinetics
Plasma sugammadex concentrations were found to be characterized by a biexponential decline, with a terminal half-life of 1.7–1.8 h. The systemic exposure to sugammadex was established to be proportional to the administered dose. Clearance (0.07 L/min) was consistent across dose levels and the volume of distribution ranged between 8.8 and 11.9 L (Figure 4; Table 2).
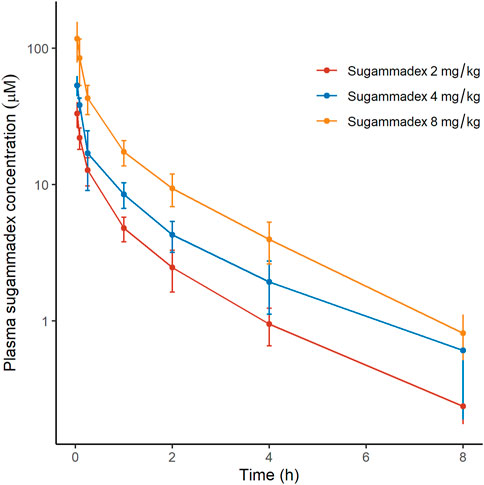
FIGURE 4. Plasma concentrations of sugammadex as a function of elapsed time after rocuronium administration, discriminated by allocated group. The y-axis is logarithmically scaled, and the error bars represent standard deviations.
Age group was found to be significantly associated with pharmacokinetics parameters, with reductions in dose-normalized Cmax, AUClast, and AUCinf being observed with increasing age. In contrast, both the clearance and volume of distribution were observed to be higher in older children, whereas the elimination half-life was consistent among all age groups. Subgroup analysis of data from our previous study (Ji et al., 2023) based on age group showed similar results (Table 3).
Plasma rocuronium concentration was also characterized by a biexponential decline, with a half-life of 1.6–1.7 h. Systemic exposure to rocuronium was comparable between the control and sugammadex groups, with clearance and volume values ranging between 0.11 and 0.16 L/min and 15.9 and 21.1 L, respectively (Figure 5; Table 2). Figure 3 shows the molar concentrations of sugammadex and rocuronium, along with the T4/T1 ratio of the individual participants.
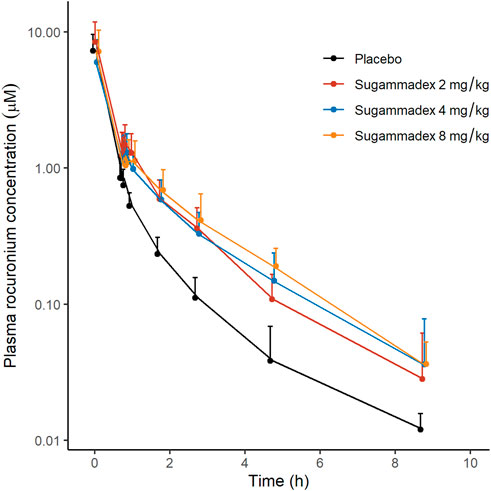
FIGURE 5. Plasma concentrations of rocuronium as a function of elapsed time after rocuronium administration, discriminated by allocated group. The y-axis is logarithmically scaled, and the error bars represent standard deviations.
There were no statistically significant difference between this study and our previous study in any of the parameters for sugammadex. For rocuronium, Cmax and AUCs were similar, while t1/2 and Vz tended to show larger values in this study (Table 2).
3.3 Safety profiles of sugammadex
During the period between administration of the study drug and 24 h after the end of surgery, 20 of the 29 participants experienced a range of adverse side effects, including fever, hypothermia, hypotension, hypertension, tachycardia, bradycardia, headache, nausea, and vomiting. Among these, two hypotensive events were considered to be associated with the use of sugammadex, although these were self-limiting and no subsequent intervention was necessary. Other adverse events were considered to be surgery-related rather than attributable to the use of a neuromuscular reversal agent. Detailed profiles of the adverse events are presented in Table 4.
4 Discussion
In this study, we evaluated the efficacy of sugammadex in reversing rocuronium-induced neuromuscular blockade in Korean children, in the context of a conventional reversal at reappearance of T2 in response to train-of-four stimulation. In addition, we performed a non-compartmental analysis of sugammadex and rocuronium according to each sugammadex dose group. One type of adverse event was suspected to be related to sugammadex use.
Previous studies have investigated the pharmacodynamics of sugammadex in the context of the reversal of moderate neuromuscular blockade in children. The median recovery time of 1.3 min from 2 mg/kg sugammadex observed in the present study is comparable to that previously reported (Plaud et al., 2009; Kleijn et al., 2011; Voss et al., 2022). In response to higher doses, we established that the reversal times were similar to or less than those recorded following the administration of 2 mg/kg sugammadex. As the median recovery time of 1.3 min is considered sufficiently short, we can conclude that the recovery profile of sugammadex in Korean children is similar to that reported in the literature. In this regard, Herring et al. (Herring et al., 2017) reported a pooled analysis of adult data, which revealed a geometric mean of recovery time of 1.9 min in response to the administration of 2 mg/kg sugammadex, compared with a value of 10.6 min recorded in those treated with neostigmine. The median recovery time of 1.3 min recorded in the present study is accordingly comparable to that obtained by these authors, thereby indicating that a dose of 2 mg/kg sugammadex would be suitable for use in reversing moderate neuromuscular blockade in children.
In contrast to pharmacodynamics, pharmacokinetic analyses of sugammadex in children have rarely been reported. Among those studies that have been conducted is a recent phase IV trial, in which the pharmacokinetics of 2 and 4 mg/kg sugammadex were evaluated in 40 pediatric patients (Voss et al., 2022), the findings of which revealed that pediatric patients aged between 6 and 17 years were characterized by similar systemic exposure to their adult counterparts (Voss et al., 2022). Contrastingly, pediatric patients between the ages 2 and 6 years were observed to have between 25% and 40% lower systemic exposure (Voss et al., 2022). Overall, the pharmacokinetic profiles of these patients were similar to those characterized in our Korean pediatric study.
We established that the age-based pharmacokinetic parameters of sugammadex were closely correlated with pediatric ontogeny, with the values of both the volume of distribution and clearance being high in older children. Indeed, the clearance of sugammadex in adolescent patients (i.e., those aged between 12 and 17) was similar to that reported for adult patients (Keating, 2016). Given that sugammadex is primarily eliminated via renal excretion, the observed changes in clearance are assumed to reflect the increasing size and maturation of kidney function with increasing age (Anderson, 2012). The volume of distribution was found to increase to a similar extent, as indicated by an unchanged elimination half-life. However, in this regard, the ontogeny of hepatic function also deserves consideration, given that sugammadex recovery time has been demonstrated to be slower after liver transplantation, even though the metabolism of sugammadex is minimal (Deana et al., 2020). This result is also consistent with our previous study on reversal of deep neuromuscular blockade. Considering these, there is possibility that less dose may be needed for younger children to obtain similar effect. Further pharmacodynamics studies for effect of age on pharmacodynamics of sugammadex may help.
We found that patients in the groups receiving sugammadex were characterized by slightly higher plasma concentrations of rocuronium than those recorded in control group (Figure 5). Given that the complex of rocuronium and sugammadex is indistinguishable from free rocuronium, it is assumed that plasma concentrations will not undergo a marked decline, even after the administration of sugammadex. In addition, Ploeger et al. have reported that the observed plasma concentrations of rocuronium can increase following the administration of sugammadex (Ploeger et al., 2009), which they attribute to the movement of free rocuronium from the tissue compartment to the plasma compartment to establish a steady state, as free rocuronium binds to sugammadex. In a study on the effects of sugammadex on the pharmacokinetics of rocuronium, Gijsenbergh et al. reported a dose-dependent increase in the AUCinf of rocuronium with increasing sugammadex concentration (Gijsenbergh et al., 2005). As shown in Figure 5, we detected notable differences among groups in this regard. Furthermore, in our non-compartmental analysis, we detected increases in AUClast and AUCinf of rocuronium in response to the administration of sugammadex, although the differences were not statistically significant (p = 0.765, 0.089, 0.064 for Cmax, AUClast, AUCinf, respectively). We speculate that this inconsistency could be attributable to the small number of participants assessed in this study. These changes were also observed in our previous study on reversal of intense blockade, and the differences were significant (Ji et al., 2023). The Cmax of rocuronium should not differ since the highest concentration of rocuronium is reached prior to the administration of sugammadex.
Differences were observed on t1/2 and Vz of rocuronium between this study and our previous study. This may be due to difference of the timing of sugammadex administration in both studies (48.7 min in this study vs. 14.9 min in previous study, p < 0.001).
As potential adverse effects of sugammadex, bradycardia, QT interval prolongation, atrioventricular block, hypotension, atrial fibrillation, hypersensitivity, and anaphylaxis have been reported (de Kam et al., 2018; Min et al., 2018; Kapoor, 2020). Among these, bradycardia has been reported to occur in approximately 8% of children administered sugammadex, although the incidence is lower than that observed following the administration of neostigmine (Alsuhebani et al., 2020). In the present study, one (4.8%) of the 21 participants who received sugammadex developed bradycardia, although in this case, the event was considered to be unrelated to the use of sugammadex, as it occurred approximately 9 h after administration. Moreover, we observed two occurrences of hypotension following the administration of sugammadex, although we were unable to establish whether these were associated with the administration of sugammadex or anesthetic drugs. Nevertheless, given that no intervention was necessary for either of these events, they were not considered to be serious.
Our study had some limitations. Firstly, we did not establish a pharmacokinetic–pharmacodynamic model of sugammadex for the reversal of neuromuscular blockade, as has been done in some previous studies (Ploeger et al., 2009; Kleijn et al., 2011). For the purpose of this study, we decided not to include a pharmacokinetic model, given that precise pharmacokinetic modeling is difficult, owing to the inability to discriminate the rocuronium–sugammadex complex from the free forms of these drugs. In addition, as recovery generally occurred within 2 min, it was difficult for multiple blood samplings during this interval to construct a viable pharmacokinetic–pharmacodynamic model. Secondly, given that we enrolled a relatively small number of participants, we did not include a subgroup analysis of pharmacodynamics according to the age spectrum. Accordingly, further efficacy studies involving a larger number of participants are required.
In conclusion, we report a pharmacokinetic and efficacy study of sugammadex administered for the reversal of moderate neuromuscular blockade in Korean children, along with an analysis based on age group. We accordingly established that a 2 mg/kg dose of sugammadex appears to be safe and effective in reversing rocuronium-induced neuromuscular blockade on reappearance of the T2 twitch in response to train-of-four stimulation in Korean children older than 2 years of age. Also, pharmacokinetic parameters of sugammadex differed according to age. Further studies, including post-marketing surveillance and study on effect of age, are needed to enable a more robust conclusion regarding the efficacy and safety of this drug.
Data availability statement
The raw data supporting the conclusion of this article will be made available by the authors, without undue reservation.
Ethics statement
The studies involving human participants were reviewed and approved by Institutional Review Board of Seoul National University Hospital. Written informed consent to participate in this study was provided by the participants’ legal guardian/next of kin.
Author contributions
S-HJ, KH, Y-EJ, and H-SK contributed to conceptualization. S-HJ, KH, JO, H-JJ, J-HL, and H-SK participated in data curation. S-HJ, KH, JO, H-JJ, E-HK, J-TK, and H-SK contributed to formal analysis. S-HJ, H-JJ, Y-EJ, and H-SK acquired funding. S-HJ, H-JJ, Y-EJ, E-HK, J-TK, and H-SK participated in investigation. S-HJ, KH, JO, H-JJ, and H-SK provided methodology. S-HJ, KH, JO, and H-SK managed software. S-HJ, KH, and JO performed validation. S-HJ, KH, and JO visualized the results. S-HJ, KH, JO, and H-SK wrote the original draft. All authors contributed to manuscript revision, and have read and approved the submitted version.
Funding
This research was supported by a grant from the Ministry of Food and Drug Safety, Republic of Korea (18183MFDS492) in years 2020–2022. The funder provided a budget for study drug and measurement of the plasma concentrations of drugs. The funder was not involved in the study design, the collection, analysis, or interpretation of data, the writing of this article, or the decision to submit it for publication.
Acknowledgments
English language editing was provided by Editage (http://editage.co.kr)
Conflict of interest
The authors declare that the research was conducted in the absence of any commercial or financial relationships that could be construed as a potential conflict of interest.
Publisher’s note
All claims expressed in this article are solely those of the authors and do not necessarily represent those of their affiliated organizations, or those of the publisher, the editors and the reviewers. Any product that may be evaluated in this article, or claim that may be made by its manufacturer, is not guaranteed or endorsed by the publisher.
References
Ali, H. H., Utting, J. E., and Gray, C. (1970). Stimulus frequency in the detection of neuromuscular block in humans. Br. J. Anaesth. 42 (11), 967–978. doi:10.1093/bja/42.11.967
Alsuhebani, M., Sims, T., Hansen, J. K., Hakim, M., Walia, H., Miller, R., et al. (2020). Heart rate changes following the administration of sugammadex in children: A prospective, observational study. J. Anesth. 34 (2), 238–242. doi:10.1007/s00540-019-02729-y
Ammar, A. S., Mahmoud, K. M., and Kasemy, Z. A. (2017). A comparison of sugammadex and neostigmine for reversal of rocuronium-induced neuromuscular blockade in children. Acta Anaesthesiol. Scand. 61 (4), 374–380. doi:10.1111/aas.12868
Anderson, B. J. (2012). My child is unique; the pharmacokinetics are universal. Paediatr. Anaesth. 22 (6), 530–538. doi:10.1111/j.1460-9592.2011.03788.x
Briggs, R. J., Nicholson, R., Vazvaei, F., Busch, J., Mabuchi, M., Mahesh, K. S., et al. (2014). Method transfer, partial validation, and cross validation: Recommendations for best practices and harmonization from the global bioanalysis consortium harmonization team. AAPS J. 16 (6), 1143–1148. doi:10.1208/s12248-014-9650-3
Choi, L., Crainiceanu, C. M., and Caffo, B. S. (2013). Practical recommendations for population PK studies with sampling time errors. Eur. J. Clin. Pharmacol. 69 (12), 2055–2064. doi:10.1007/s00228-013-1576-7
de Kam, P. J., Nolte, H., Good, S., Yunan, M., Williams-Herman, D. E., Burggraaf, J., et al. (2018). Sugammadex hypersensitivity and underlying mechanisms: A randomised study of healthy non-anaesthetised volunteers. Br. J. Anaesth. 121 (4), 758–767. doi:10.1016/j.bja.2018.05.057
de Moraes, N. V., Lauretti, G. R., Filgueira, G. C., Lopes, B. C., and Lanchote, V. L. (2014). Analysis of rocuronium in human plasma by liquid chromatography-tandem mass spectrometry with application in clinical pharmacokinetics. J. Pharm. Biomed. Anal. 90, 180–185. doi:10.1016/j.jpba.2013.11.032
de Zwart, M. A., ten Bruggencate-Broeders, J., van Hal, H. J., Megens, R. H., and Frasa, H. W. (2011). Determination of sugammadex in human plasma, urine, and dialysate using a high-performance liquid chromatography/tandem mass spectrometry assay. J. Chromatogr. B Anal. Technol. Biomed. Life Sci. 879 (19), 1573–1586. doi:10.1016/j.jchromb.2011.03.050
Deana, C., Barbariol, F., D'Inca, S., Pompei, L., and Rocca, G. D. (2020). SUGAMMADEX versus neostigmine after ROCURONIUM continuous infusion in patients undergoing liver transplantation. BMC Anesthesiol. 20 (1), 70. doi:10.1186/s12871-020-00986-z
Farenc, C., Enjalbal, C., Sanchez, P., Bressolle, F., Audran, M., Martinez, J., et al. (2001). Quantitative determination of rocuronium in human plasma by liquid chromatography-electrospray ionization mass spectrometry. J. Chromatogr. A 910 (1), 61–67. doi:10.1016/s0021-9673(00)01164-x
Gijsenbergh, F., Ramael, S., Houwing, N., and van Iersel, T. (2005). First human exposure of Org 25969, a novel agent to reverse the action of rocuronium bromide. Anesthesiology 103 (4), 695–703. doi:10.1097/00000542-200510000-00007
Herring, W. J., Woo, T., Assaid, C. A., Lupinacci, R. J., Lemmens, H. J., Blobner, M., et al. (2017). Sugammadex efficacy for reversal of rocuronium- and vecuronium-induced neuromuscular blockade: A pooled analysis of 26 studies. J. Clin. Anesth. 41, 84–91. doi:10.1016/j.jclinane.2017.06.006
Ji, S. H., Huh, K. Y., Oh, J., Jeong, H. J., Jang, Y. E., Kim, E. H., et al. (2023). Reversal of rocuronium-induced intense neuromuscular blockade by sugammadex in Korean children: A pharmacokinetic and pharmacodynamic analysis. Clin. Transl. Sci. 16 (1), 92–103. doi:10.1111/cts.13429
Kapoor, M. C. (2020). Cardiovascular adverse effects of sugammadex. J. Anaesthesiol. Clin. Pharmacol. 36 (4), 469–470. doi:10.4103/joacp.JOACP_132_20
Keating, G. M. (2016). Sugammadex: A review of neuromuscular blockade reversal. Drugs 76 (10), 1041–1052. doi:10.1007/s40265-016-0604-1
Kleijn, H. J., Zollinger, D. P., van den Heuvel, M. W., and Kerbusch, T. (2011). Population pharmacokinetic-pharmacodynamic analysis for sugammadex-mediated reversal of rocuronium-induced neuromuscular blockade. Br. J. Clin. Pharmacol. 72 (3), 415–433. doi:10.1111/j.1365-2125.2011.04000.x
Lee, H. C., and Jung, C. W. (2018). Vital Recorder-a free research tool for automatic recording of high-resolution time-synchronised physiological data from multiple anaesthesia devices. Sci. Rep. 8 (1), 1527. doi:10.1038/s41598-018-20062-4
Liu, G., Wang, R., Yan, Y., Fan, L., Xue, J., and Wang, T. (2017). The efficacy and safety of sugammadex for reversing postoperative residual neuromuscular blockade in pediatric patients: A systematic review. Sci. Rep. 7 (1), 5724. doi:10.1038/s41598-017-06159-2
Matsui, M., Konishi, J., Suzuki, T., Sekijima, C., Miyazawa, N., and Yamamoto, S. (2019). Reversibility of rocuronium-induced deep neuromuscular block with sugammadex in infants and children-A randomized study. Biol. Pharm. Bull. 42 (10), 1637–1640. doi:10.1248/bpb.b19-00044
Min, K. C., Bondiskey, P., Schulz, V., Woo, T., Assaid, C., Yu, W., et al. (2018). Hypersensitivity incidence after sugammadex administration in healthy subjects: A randomised controlled trial. Br. J. Anaesth. 121 (4), 749–757. doi:10.1016/j.bja.2018.05.056
Ozgun, C., Cakan, T., Baltaci, B., and Basar, H. (2014). Comparison of reversal and adverse effects of sugammadex and combination of - anticholinergic-Anticholinesterase agents in pediatric patients. J. Res. Med. Sci. 19 (8), 762–768.
Plaud, B., Meretoja, O., Hofmockel, R., Raft, J., Stoddart, P. A., van Kuijk, J. H., et al. (2009). Reversal of rocuronium-induced neuromuscular blockade with sugammadex in pediatric and adult surgical patients. Anesthesiology 110 (2), 284–294. doi:10.1097/ALN.0b013e318194caaa
Ploeger, B. A., Smeets, J., Strougo, A., Drenth, H. J., Ruigt, G., Houwing, N., et al. (2009). Pharmacokinetic-pharmacodynamic model for the reversal of neuromuscular blockade by sugammadex. Anesthesiology 110 (1), 95–105. doi:10.1097/ALN.0b013e318190bc32
Sorgenfrei, I. F., Norrild, K., Larsen, P. B., Stensballe, J., Ostergaard, D., Prins, M. E., et al. (2006). Reversal of rocuronium-induced neuromuscular block by the selective relaxant binding agent sugammadex: A dose-finding and safety study. Anesthesiology 104 (4), 667–674. doi:10.1097/00000542-200604000-00009
Staals, L. M., Snoeck, M. M., Driessen, J. J., van Hamersvelt, H. W., Flockton, E. A., van den Heuvel, M. W., et al. (2010). Reduced clearance of rocuronium and sugammadex in patients with severe to end-stage renal failure: A pharmacokinetic study. Br. J. Anaesth. 104 (1), 31–39. doi:10.1093/bja/aep340
Voss, T., Wang, A., DeAngelis, M., Speek, M., Saldien, V., Hammer, G. B., et al. (2022). Sugammadex for reversal of neuromuscular blockade in pediatric patients: Results from a phase IV randomized study. Paediatr. Anaesth. 32 (3), 436–445. doi:10.1111/pan.14370
Keywords: anesthesia, pediatric, pharmacodynamics, pharmacokinetics, sugammadex, rocuronium
Citation: Ji S-H, Huh KY, Oh J, Jeong H-J, Jang Y-E, Kim E-H, Lee J-H, Kim J-T and Kim H-S (2023) Conventional reversal of rocuronium-induced neuromuscular blockade by sugammadex in Korean children: pharmacokinetics, efficacy, and safety analyses. Front. Pharmacol. 14:1127932. doi: 10.3389/fphar.2023.1127932
Received: 20 December 2022; Accepted: 05 April 2023;
Published: 13 April 2023.
Edited by:
Tony K. L. Kiang, University of Alberta, CanadaReviewed by:
Cristian Deana, Azienda Sanitaria Universitaria Integrata di Udine, ItalyLuigi Vetrugno, University of Studies G. d’ Annunzio Chieti and Pescara, Italy
Copyright © 2023 Ji, Huh, Oh, Jeong, Jang, Kim, Lee, Kim and Kim. This is an open-access article distributed under the terms of the Creative Commons Attribution License (CC BY). The use, distribution or reproduction in other forums is permitted, provided the original author(s) and the copyright owner(s) are credited and that the original publication in this journal is cited, in accordance with accepted academic practice. No use, distribution or reproduction is permitted which does not comply with these terms.
*Correspondence: Hee-Soo Kim, ZGFtaTA2MDVAc251LmFjLmty
†These authors share first authorship