- Institute of Medicinal Biotechnology, Chinese Academy of Medical Sciences and Peking Union Medical College, Beijing, China
Neurodegenerative diseases (NDs) are common chronic disorders associated with progressive nervous system damage, including Alzheimer’s disease, Parkinson’s disease, and Huntington’s disease, among others. Mitochondria are abundant in various nervous system cells and provide a bulk supply of the adenosine triphosphate necessary for brain function, considered the center of the free-radical theory of aging. One common feature of NDs is mitochondrial dysfunction, which is involved in many physiopathological processes, including apoptosis, inflammation, oxidative stress, and calcium homeostasis. Recently, genetic studies revealed extensive links between mitochondrion impairment and dysregulation of non-coding RNAs (ncRNAs) in the pathology of NDs. Traditional Chinese medicines (TCMs) have been used for thousands of years in treating NDs. Numerous modern pharmacological studies have demonstrated the therapeutic effects of prescription, herbal medicine, bioactive ingredients, and monomer compounds of TCMs, which are important for managing the symptoms of NDs. Some highly effective TCMs exert protective effects on various key pathological features regulated by mitochondria and play a pivotal role in recovering disrupted signaling pathways. These disrupted signaling pathways are induced by abnormally-expressed ncRNAs associated with mitochondrial dysfunction, including microRNAs, long ncRNAs, and circular RNAs. In this review, we first explored the underlying ncRNA mechanisms linking mitochondrial dysfunction and neurodegeneration, demonstrating the implication of ncRNA-induced mitochondrial dysfunction in the pathogenesis of NDs. The ncRNA-induced mitochondrial dysfunctions affect mitochondrial biogenesis, dynamics, autophagy, Ca2+ homeostasis, oxidative stress, and downstream apoptosis. The review also discussed the targeting of the disease-related mitochondrial proteins in NDs and the protective effects of TCM formulas with definite composition, standardized extracts from individual TCMs, and monomeric compounds isolated from TCM. Additionally, we explored the ncRNA regulation of mitochondrial dysfunction in NDs and the effects and potential mechanisms of representative TCMs in alleviating mitochondrial pathogenesis and conferring anti-inflammatory, antioxidant, and anti-apoptotic pathways against NDs. Therefore, this review presents an overview of the role of mitochondrion-related ncRNAs and the target genes for TCM-based therapeutic interventions in NDs, providing insight into understanding the “multi-level compound-target-pathway regulatory” treatment mechanism of TCMs.
1 Introduction
Neurodegenerative diseases (NDs) are a set of disorders with intricate etiologies manifesting as heterogeneous symptoms which impact different areas of the brain and spinal cord. Mitochondrial dysfunction is a major pathogenic factor in the occurrence and development of NDs associated with aging, such as Alzheimer’s disease (AD), Parkinson’s disease (PD), Amyotrophic lateral sclerosis (ALS), and Huntington’s disease (HD). When the brain ages, its metabolic rate decreases, resulting in pathological features such as mitochondrial malfunction, abnormal energy metabolism, calcium imbalance, cell cycle deregulation, apoptosis, and reactive oxygen species (ROS) production. Mitochondria are the synthesis site of 90% of adenosine triphosphate (ATP) and the power source which maintains various vital activities in the body. Several studies reported that many patients with NDs exhibit abnormal glucose metabolism in the cerebral cortex and hippocampus, which damages the oxidative phosphorylation system, before developing obvious clinicopathological symptoms, such as neuron loss and cognitive decline (Jadiya et al., 2021). This suggested that mitochondrial dysfunction may be an early sign of NDs. Therefore, mitochondrial impairments are regarded as common neurodegeneration signatures during aging.
Mitochondria are involved in various cellular activities, including ROS production, ionic homeostasis maintenance, fatty acid decomposition, and apoptosis regulation. Alterations in mitochondrial motility, biogenesis, morphology, dynamics, or mutations of the mitochondrial DNA cause variation in nuclear-encoded mitochondrial genes, affecting normal mitochondrial functions via anterograde signalings. Mitochondrial dysfunction can cause neurodegeneration through various mechanisms, including interference with cell signaling pathways, oxidative stress, apoptosis, and microglia activation (Johnson et al., 2021). Neurodegeneration can also exacerbate mitochondrial dysfunction and further aggravate NDs, creating a vicious cycle. However, the detailed molecular mechanisms by which mitochondrial dysfunction affects neurodegeneration remain largely unknown. Recently, there have been increasing reports that non-coding RNAs (ncRNAs) and various endogenous regulators, including microRNAs (miRNAs), long ncRNAs (lncRNAs), and circular RNAs (circRNAs), are implicated in mitochondrial dysfunction and mitochondrion associated signaling transduction (du Mee et al., 2020; Zeng et al., 2021; Sun et al., 2022a; Jiang et al., 2022). A small proportion of ncRNAs found in mitochondria are reportedly transcribed from the mitochondrial genome, while the majority are nuclear-encoded ncRNAs that enter the mitochondria from the nucleus (Sharma et al., 2019). These ncRNAs directly regulate mitochondrial gene expression or indirectly act on different signaling pathways resulting in mitochondrial dysfunction. Therefore, ncRNAs have been demonstrated to play an important role in the etiology of NDs by mediating neurodegeneration via mitochondrial dysfunction.
Traditional Chinese medicine (TCM) has become one of the most important sources for exploring and developing modern medicines due to their application in treating various diseases for more than 5,000 years (Wang et al., 2018). Additionally, TCM can be further optimized to treat complex diseases due to its multi-component, multi-target, and multi-pathway synergistic effects (Liu et al., 2021a; Cai et al., 2022a). Over the past few years, TCM has received increasing attention in treating NDs, and many NDs treatments of TCM origin are in different clinical trial stages (Shan et al., 2018). In this review, we discuss the mitochondrial dysfunction regulatory mechanisms of ncRNAs implicated in NDs and summarize various TCMs that treat NDs by regulating mitochondrial dysfunction from modern pharmacological studies. We also highlight the possibility that some TCMs can treat NDs by interfering with ncRNAs to regulate mitochondrial dysfunction (Figure 1). Thus, this review emphasizes the broad application prospects of TCM, aiming to provide new insights and strategies for treating NDs.
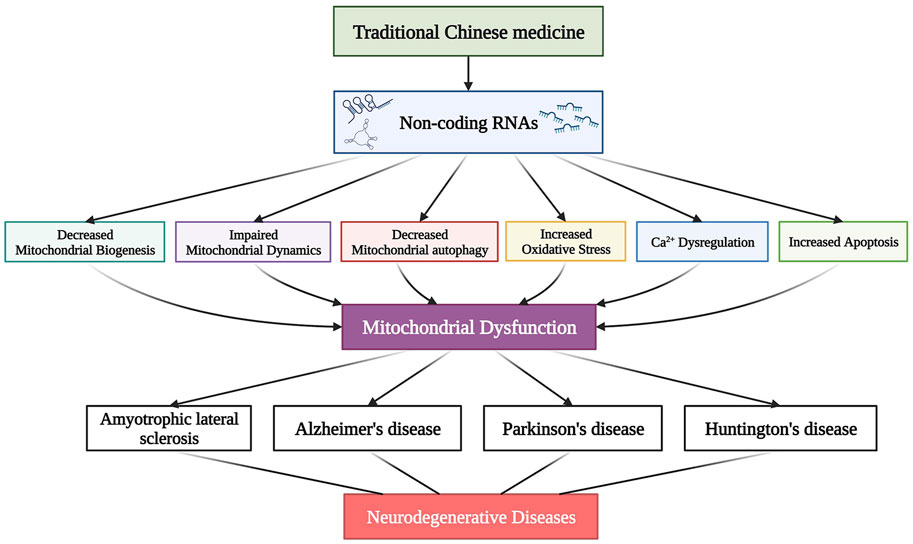
FIGURE 1. Traditional Chinese medicine (TCM) modulates mitochondrial dysfunction by directly acting on ncRNAs to alleviate various neurodegenerative diseases (NDs).
2 ncRNAs target mitochondria to regulate NDs
Mitochondrial dysfunction, such as reduced mitochondrial biogenesis, impaired mitochondrial dynamics, abnormal mitochondrial autophagy (mitophagy), Ca2+ overload, activation of ROS-mediated oxidative stress, and downstream apoptosis, are the main research areas in NDs. Mitochondria are susceptible to various genetic and environmental factors. ncRNAs regulate the intersection of mitochondrial signaling pathways by modulating the levels of transcriptional and post-transcriptional mitochondrion-related genes. Therefore, identifying ncRNA-mediated mitochondrion-targeting drugs may provide new therapeutic targets for NDs. Figure 2 highlights the mitochondrial dysfunctions during NDs and summarizes the corresponding dysfunction of ncRNAs.
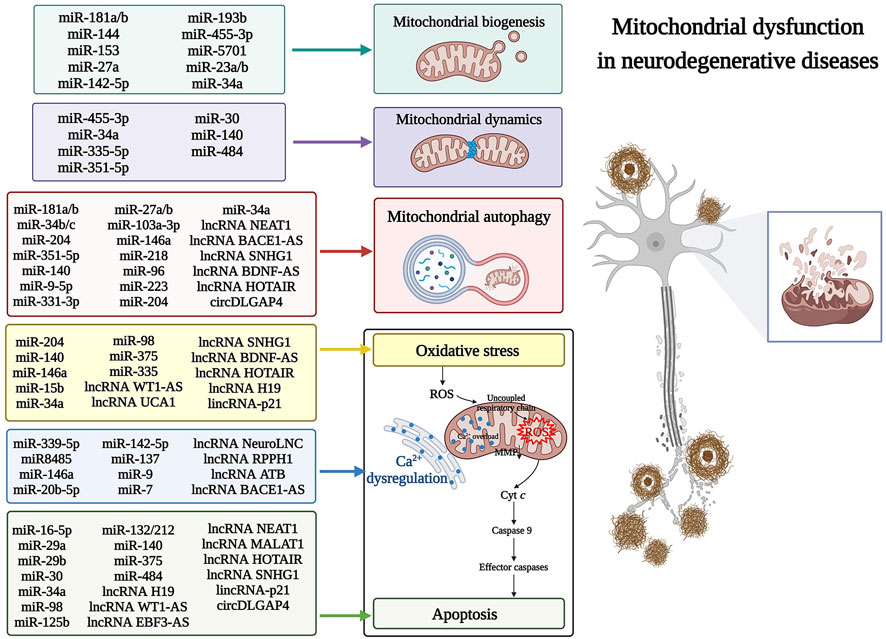
FIGURE 2. The non-coding RNAs (ncRNAs) that regulate mitochondrial dysfunction in neurodegenerative diseases (NDs). Various mitochondrial dysfunctions are associated with the dysregulation of ncRNAs in NDs. These dysfunctions include the pathogenesis of mitochondrial biogenesis, dynamics, autophagy, calcium dyshomeostasis, reactive oxygen species (ROS)-mediated mitochondrial oxidative stress, and downstream apoptosis. Cyt c, cytochrome c; ROS, reactive oxygen species; MMP, mitochondrial membrane potential.
2.1 ncRNAs regulate mitochondrial gene expression
Regulation of mitochondrial biogenesis and dynamics, accompanied by mitochondrial clearance and quality control, has been used as a possible treatment strategy for mitochondrial diseases. microRNA-181a and 181b (miR-181a/b), belonging to the miR-181 family, have been highly expressed in various brain regions of AD patients. Additionally, miR-181a/b has been recently shown to target key genes regulating mitochondrial biogenesis and mitophagy. These genes included PPARG coactivator 1-alpha (PPARGC1A), nuclear respiratory factor 1 (NRF1) (major regulators of mitochondrial biogenesis), cytochrome c oxidase copper chaperone COX11 (COX11), coenzyme Q10B (COQ10B) (involved in mitochondrial respiratory chain assembly), and autophagy-related 5 (ATG5) and parkin RBR E3 ubiquitin-protein ligase (PARK2) (key players in mitophagy). Knockdown of miR-181a/b enhanced the expression of mitochondrial biogenesis-related genes and mitophagy protein markers, maintaining mitochondrial homeostasis and alleviating neural deformation (Indrieri et al., 2019; Figure 2).
The stability of the internal mitochondrial metabolism is regulated by the dynamic processes of mitochondrial fusion and fission. A recent study found that miR-351-5p directly targeted mitochondrial Rho GTPase 2 (MIRO2), inhibiting its expression and causing large mitochondrial fission and fragmentation, thereby leading to neural progenitor cell death in the hippocampus (Woo et al., 2020; Figure 2). Furthermore, such miRNA regulation is reportedly associated with a functional correlation between mitochondrial dynamics and mitophagy. Excessive mitochondrial fission promotes mitophagy through the PTEN-induced kinase 1 (PINK1)/Parkin-mediated mitophagy pathway, with a convergence of the miR-351-5p/MIRO2 axis in the pathology of AD (Chen et al., 2016). With the ongoing development of high-throughput sequencing and microarray technologies, many novel ncRNAs with regulatory roles in NDs are being identified (Cai et al., 2022b; Sun et al., 2022b; Zhao et al., 2022). For example, miR-455-3p is a newly discovered miRNA that showed significant up-regulation in AD (Kumar et al., 2017), and its overexpression positively regulated the expression of mitochondrial biogenesis genes. This increased the mRNA and protein levels of key genes [PPARGC1A, NRF1, NRF2, transcription factor A, and mitochondrial (TFAM)] in mitochondrial biogenesis. Moreover, miR-455-3p decreased the mitochondrial fission proteins [Dynamin-related protein 1 (DRP1) and mitochondrial fission 1 (FIS1)] but significantly increased the fusion proteins [Optic nerve atrophy 1 (OPA1), Mitogen 1 (MFN1), and 2 (MFN2)], protecting the mitochondria from the toxic effects of β-amyloid (Aβ) (Kumar et al., 2019; Figure 2).
2.2 ncRNAs regulate Ca2+ homeostasis, ROS-mediated oxidative stress, and downstream apoptotic machinery in mitochondria
In addition to their role in bioenergy production, mitochondria display other important cellular functions, including regulating Ca2+ homeostasis, ROS signaling, and apoptosis. Intracytoplasmic Ca2+ homeostasis is essential for neurons to conduct and sustain their physiological activities. Mitochondria are calcium buffering sites that protect cells against high cytoplasmic Ca2+. However, impaired synaptic function and synaptic loss cause a continuous increase of Ca2+ concentration in the neuron cytoplasm, leading to mitochondrial oxidative stress, membrane potential changes, reduced ATP production, and ultimately affecting mitochondrial function. The highly neuro-specific lncRNA, NeuroLNC, affected calcium dynamics by interacting with the RNA-binding protein TAR DNA binding protein (TARDBP), which promotes selective stabilization of presynaptic protein-encoded mRNA (Keihani et al., 2019; Figure 2). Moreover, mutations in miR8485, another ncRNA that binds TARDBP, led to overexpression of neurexin 1 (NRXN1), resulting in presynaptic Ca2+ overload, mitochondrial Ca2+ uptake, and neurodegeneration (Fan et al., 2014; Kattimani and Veerappa, 2018). Under pathological conditions of NDs, intramitochondrial calcium overload, caused by intracellular calcium imbalance, promotes ROS production from different sources and induces downstream apoptosis by complementary action of ROS overexposure (Baev et al., 2022; Figure 2).
In addition to directly regulating mitochondrial genes, some ncRNAs, such as miR-34a and miR-7, can also regulate mitochondrial oxidative stress and downstream apoptosis to influence the onset and progression of NDs (Figure 2). miR-34a, a miRNA specifically up-regulated in AD and used in distinguishing AD patients from those with PD or HD, disrupts mitochondrial oxidative phosphorylation by inhibiting the expression of electron transport chain components (Sarkar et al., 2016). Moreover, overexpressing miR-34a reduced the PPARGC1A, NRF1, and TFAM levels significantly, inhibiting mitochondrial biogenesis (Thounaojam et al., 2019). Knockdown of miR-34a increased the levels of autophagy-related proteins, such as microtubule-associated protein one light chain three alpha (LC3) II/I, beclin 1 (BECN1), and ATG7, accelerated degradation of P62, and induced abnormal expression of DRP1 and MFN2. This improved autophagy and mitochondrial dynamics (Kou et al., 2017). In addition, miR-34a was reportedly up-regulated in the frontal cortex and hippocampus of early AD patients in the BraaK phase III/IV and was shown to be embedded in an upstream regulator of oxidative stress (Nunomura and Perry, 2020). Furthermore, targeting the anti-apoptotic protein BCL2 apoptosis regulator (BCL2) with miR-34a inhibited endogenous apoptosis and promoted neuroprotection (Cosín-Tomás et al., 2017). The multi-target effects of miR-34a reported on oxidative stress, mitochondrial health, and apoptosis in AD are due to its multi-functional activities in mitochondria. miR-7 significantly reduced the brain regions associated with dopaminergic neurodegeneration among PD patients. Currently, miR-7 replacement therapy has been proposed to slow PD progression from the early stages by modulating mitochondrial function, apoptosis, oxidative stress, and directly targeting PD-related genes. This reduces α-synuclein (α-Syn) accumulation in Lewy bodies and enhances the survival of the remaining neurons, indicating the potential for key mechanisms involved in neuropathology (Titze-de-Almeida and Titze-de-Almeida, 2018). Recent studies have shown that lncRNA small molecule RNA host gene 1 (SNHG1) up-regulated in PD can regulate the expression of BCL2 associated X, an apoptosis regulator (BAX), by interacting with miR-216a-3p (Wang et al., 2021a). SNHG1 can also regulate apoptosis in PD by binding miR-153-3p to modulate PTEN/Akt/mTOR signaling (Zhao et al., 2020). Moreover, silencing SNHG1 reportedly protected SK-N-SH and MN9D cells from 1-methyl-4-phenylpyridinium (MPP+)-induced oxidative stress (Xiao et al., 2021) and promoted mitophagy (Qian et al., 2019). These multi-linked activities make SNHG1 a potential therapeutic target for treating PD (Figure 2). So far, compared to miRNAs and lncRNAs, there are fewer studies on the involvement of circRNAs in NDs. CircDLGAP4 is reportedly down-regulated in the in vivo and in vitro PD models. However, up-regulating circDLGAP4 promoted cell viability and autophagy and inhibited apoptosis and mitochondrial damage, thereby alleviating the pathological changes of PD (Feng et al., 2020; Figure 2). These findings indicate that ncRNAs contribute to mitochondrial dysfunction by altering multiple mitochondrion-encoding genes, prompting the question of whether ncRNA-mediated mitochondrion-targeting therapeutics may be a viable treatment strategy for heterogeneous NDs.
3 TCM improves NDs by regulating mitochondrial dysfunction
Several clinal trials have been conducted to demonstrate the potential of NDs treatment using TCM. Approximately one-quarter of modern drugs are derived from natural products (Musthaba et al., 2010). Many TCMs exert neuroprotective effects to modulate mitochondrial dysfunction (Jiang et al., 2019). These effects include antioxidation, apoptosis inhibition, restoration of mitochondrial structure, biogenesis and dynamics, and mitophagy activation. The following section summarizes the TCM formulas with definite composition (Table 1), standardized extracts from individual TCMs (Table 2), and monomeric compounds isolated from TCM (Table 3) that can modulate mitochondrial dysfunction in ND-related models.
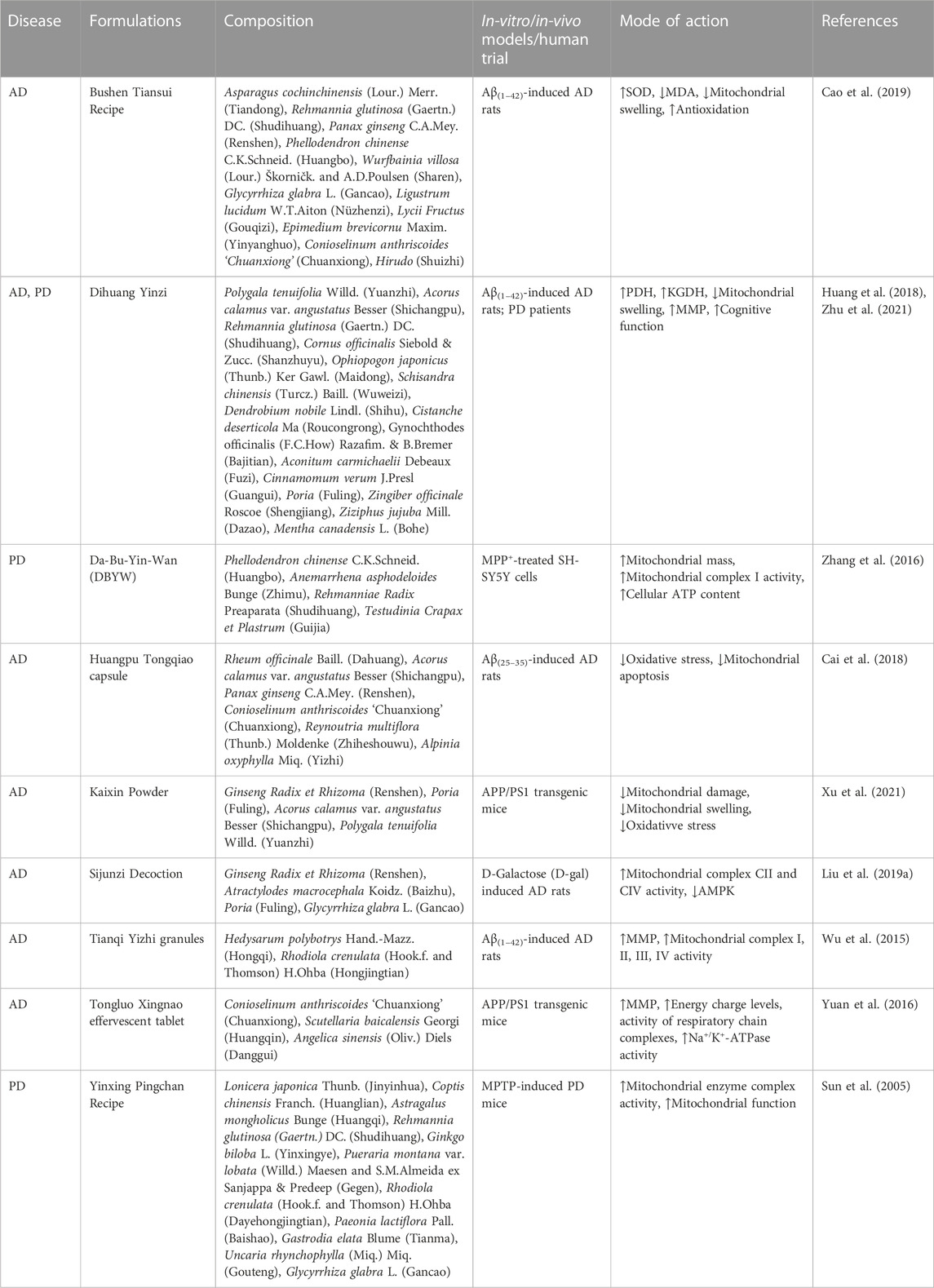
TABLE 1. List of traditional Chinese medicine (TCM) formulas treating neurodegenerative diseases (NDs) by alleviating mitochondrial dysfunction.
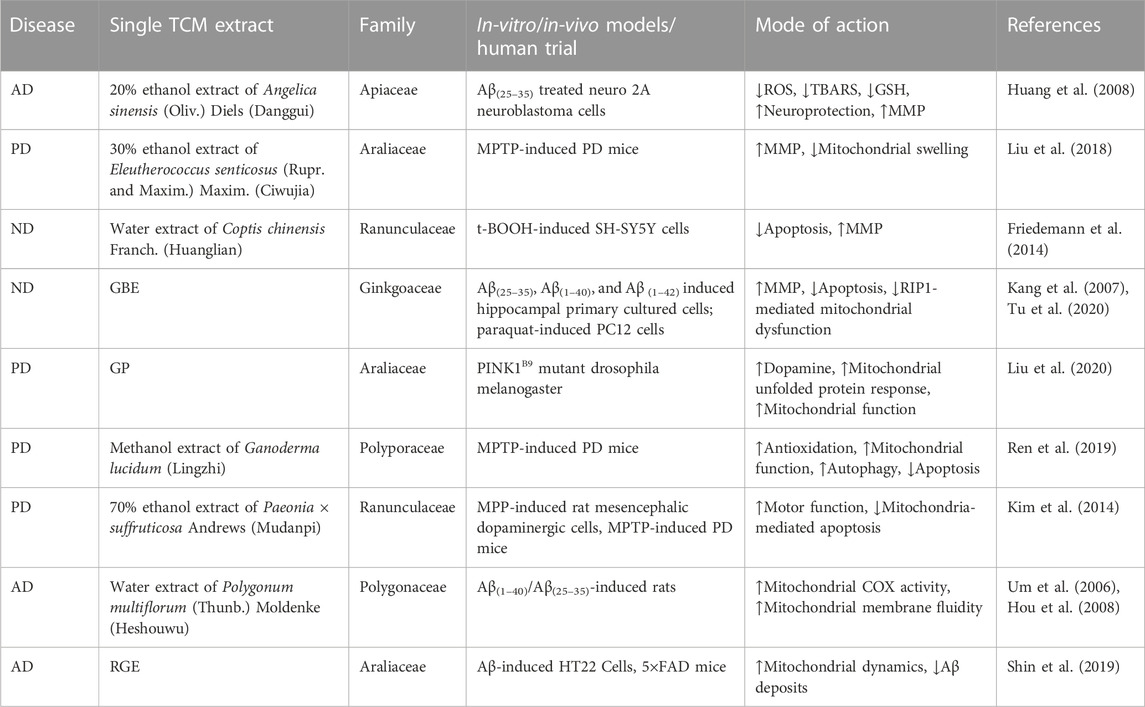
TABLE 2. List of standardized extracts from individual traditional Chinese medicines (TCMs) exerting anti-neurodegenerative disease (anti-ND) effects by modulating mitochondrial dysfunction.
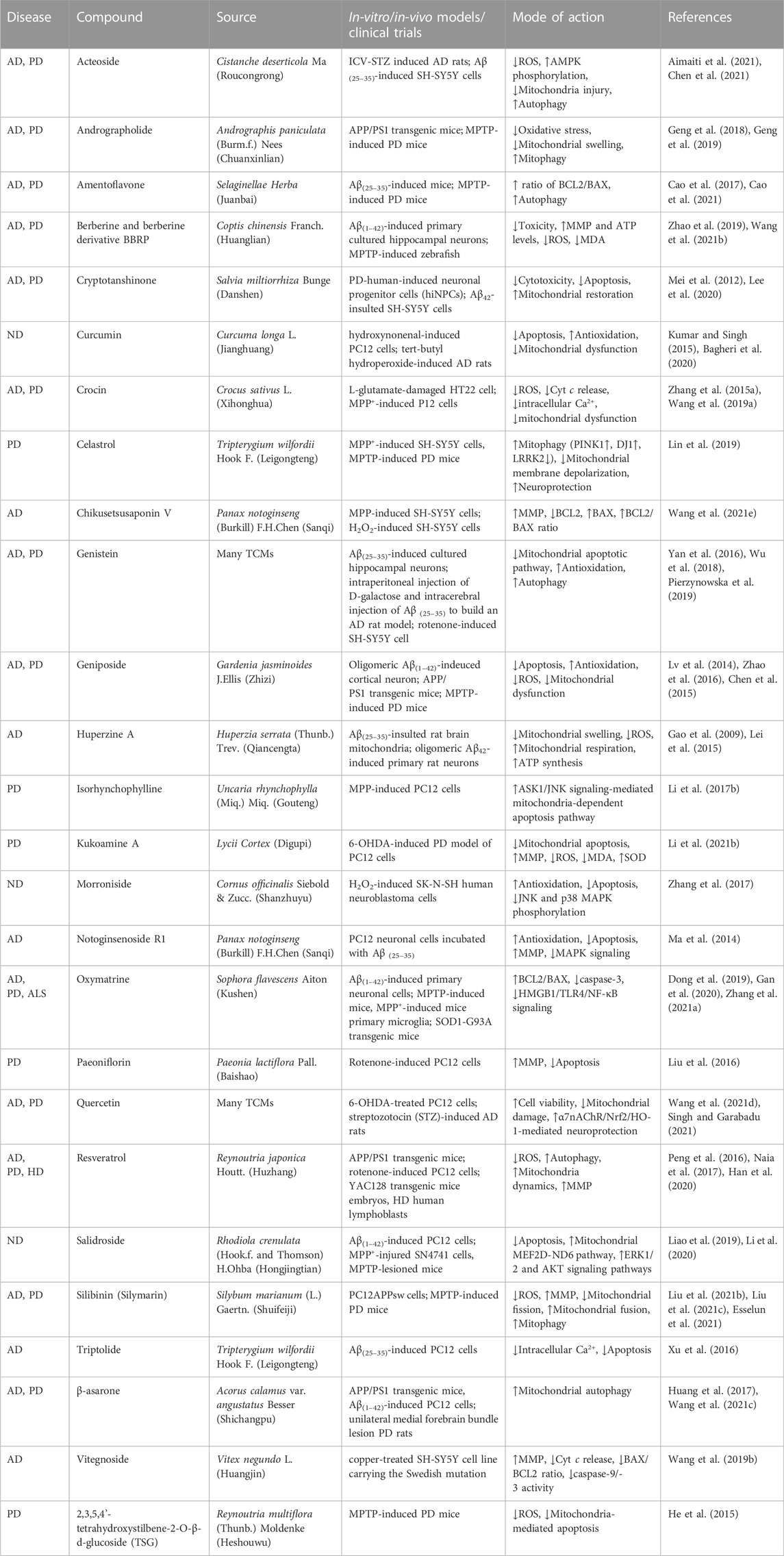
TABLE 3. List of monomeric compounds isolated from traditional Chinese medicines (TCMs) exerting anti-neurodegenerative disease (anti-ND) effects by regulating mitochondrial dysfunction.
3.1 TCM formulas with a definite composition
Many classical formulas documented in ancient Chinese medical books can exert neuroprotective effects, and the efficacy of these formulas in treating NDs has been demonstrated by several studies using modern testing techniques. The compatibility of TCM formulas ensures a synergistic effect by increasing the dissolution rate of the major active compounds while reducing the content of toxic components in TCM. This provides a good safety profile of the compounds while exerting therapeutic effects. One study summarized the drug use pattern of TCM formulas for AD treatment and found that 150 TCMs were used with 132 formulas, among which Acorus calamus var. angustatus Besser (Shichangpu) was the most frequently used TCM (Hu et al., 2012; Ma et al., 2015).
Dihuang Yinzi, consisting of twelve Chinese herbs, improved the daily living and cognitive activities in patients with mild to moderate AD in clinical trials, and its efficacy might be superior to that of donepezil (Zhang et al., 2018). The medicinal chemistry of the cerebrospinal fluid was evaluated after administering Dihuang Yinzi, and five migrating components were found in the cerebrospinal fluid. Among the five components, one was the prototype component contained in Dihuang Yinzi [schizandrin derived from Schisandra chinensis (Turcz.) Baill. (Wuweizi)] and four were novel metabolites produced by Schisandra chinensis (Turcz.) Baill. (Wuweizi), Polygala tenuifolia Willd. (Yuanzhi), Ophiopogon japonicus (Thunb.) Ker Gawl. (Maidong), and Gynochthodes officinalis (F.C.How) Razafim. and B. Bremer (Bajitian). The four novel components might be the main pharmacologically active compounds that prevent cognitive dysfunction (Guo, 2016). Recent studies showed that Dihuang Yinzi significantly increased the PDH and KGDH levels, the key enzymes of the mitochondrial tricarboxylic acid cycle, in the brain of AD rats. Thus, Dihuang Yinzi promoted the tricarboxylic acid cycle and improved glucose utilization. It also significantly increased the mitochondrial membrane potential (MMP), protected the integrity of the inner mitochondrial membrane, and reduced mitochondrial swelling by depolarizing the inner mitochondrial membrane (Huang et al., 2018).
Relevant research has found that the water extract of Sijunzi decoction facilitates Aβ transportation across the blood-brain barrier (BBB) and reduces the aggregation of Aβ plaques in the brain (Guo et al., 2020). Further analysis of the pharmacological ingredients of Sijunzi decoction revealed that ginsenoside Rh2, panaxadiol, Poria cocos polysaccharide, and isoliquiritigenin were the principal active ingredients of the decoction (Zhang et al., 2021b). Furthermore, recent studies showed that Sijunzi decoction promotes central energy generation and minimizes behavioral abnormalities in AD by modulating the activities of respiratory complex II and III of the mitochondrial electron transport chain and AMP-dependent protein kinase (AMPK) signaling pathway (Liu et al., 2019a). AMPK first activates factors related to the mitochondrial quantity and quality regulation to provide mitochondrial protection. Recent studies reported that AMPK inhibits the expression of ATP synthase inhibitor protein, suggesting that AMPK inhibition may stimulate the oxidative respiratory chain and promote energy production. Moreover, the AMPK content was reduced in the groups treated with Sijunzi decoction, indicating that Sijunzi decoction might effectively treat AD by stimulating the mitochondrion-dominated central energy production.
Yinxing Pingchan recipe, composed of eleven herbal medicines, is a TCM product with years of clinical application in PD therapy. This recipe can significantly modulate the antioxidant system of cells, increasing their ability to resist free radical damage and effectively reduce MDA production (Zhang et al., 2004). The dismantled formulas of Yinxing Pingchan recipe (such as Ginkgo biloba L. (Yinxingye), Pueraria montana var. lobata (Willd.) Maesen and S.M.Almeida ex Sanjappa & Predeep (Gegen), and Rhodiola crenulata (Hook.f. and Thomson) H.Ohba (Dayehongjingtian)) also have significant antioxidant effects, which reduce free radicals and lipid peroxide levels. These effects have been demonstrated to be associated with the ability to enhance the survival of DA neurons. Additionally, these dismantled formulas inhibited MPTP-induced DA neuron loss and apoptosis to varying degrees in the substantia nigra of the PD mouse model. Thus, it is speculated that the Yinxing Pingchan recipe may protect the mitochondria and prevent apoptosis of DA neurons by enhancing mitochondrial enzyme complex activity, eventually slowing down the progression of PD (Sun et al., 2005).
3.2 Standardized extracts from individual TCMs
Several preclinical and human trials suggested that standardized extracts from individual TCMs may have anti-AD activities. Ginseng Radix et Rhizoma Rubra (Hongshen) and Ginseng Radix et Rhizoma (Renshen) are from the same family and have similar pharmacological effects due to their partially identical compounds. The water extract of Talinum paniculatum (Jacq.) Gaertn. (RGE) significantly alleviated Aβ-induced mitochondrial pathology by reducing the mitochondrial fusion/fission imbalances and restoring damaged mitochondrial respiratory chains in the in vivo and in vitro AD models. This suggested that RGE may be a mitochondria-targeted medicine for treating AD (Shin et al., 2019).
Eleutherococcus senticosus (Rupr. and Maxim.) Maxim. (Ciwujia) extract (EAS) demonstrated extensive therapeutic effects via EAS-enhanced motor coordination in a PD mouse model. Analysis at the superstructural level revealed that EAS prevented diencephalon mitochondrial swelling and attenuated the decrease of MMP. EAS also inhibited oxidative stress and restored the normal expression of PD-related proteins [Parkin, PINK1, DJ1, α-syn, and leucine-rich repeat kinase 2 (LRRK2)], demonstrating that EAS exerts its neuroprotective effects in PD by ameliorating mitochondrial dysfunction and structural damage (Liu et al., 2018). Modern pharmacological studies showed that the active compounds of Ginseng Radix et Rhizoma (Renshen) have a protective effect against neurotoxicity in different PD models. Ginseng protein (GP) is an important pharmacologically active compound that has been shown to exhibit neuroprotective effects in AD (Li et al., 2017a). It was found that GP treatment delayed the onset of PD-like phenotypes, promoted mitochondrial function, and protected mitochondria from oxidative stress-induced damage (Liu et al., 2020). This further confirmed the potential and therapeutic mechanisms of Ginseng Radix et Rhizoma (Renshen) as a valuable PD treatment.
Ginkgo biloba extract (GBE), a mixture obtained from Ginkgo biloba L. (Yinxingye), has a unique pharmacological activity making it one of the most commonly used drugs in the clinical prevention and treatment of AD. GBE contains two classes of pharmacologically active components; terpenes (including bilobalide and ginkgolide A, B, and C) and flavonoids (including meletin, isorhamnetin, and kaempferol). GBE has been demonstrated to directly regulate mitochondria through multiple mechanisms in various in vivo and in vitro AD models. For example, GBE improves mitochondrial function by increasing MMP and ATP levels or stimulating mitochondrial biogenesis by improving the damaged mitochondrial respiratory chain (Stockburger et al., 2018). EGb761 contains 24% of flavonoid glycosides and 6% of terpenes (ginkgolide A, B, and C, 2.8%–3.4%; bilobalide, 2.6%–3.2%), and the ginkgolide and bilobalide showed significant mitochondrial protective characteristics (Lejri et al., 2019). Furthermore, in vivo and in vitro experiments showed that treating AD with EGb761 significantly increased the viability of mitochondrial complex I, IV, and V and reversed MMP and ATP production in a dose-dependent manner. A clinical trial indicated that EGb761 protected patients from neurological dysfunction and improved their cognitive ability by inhibiting the damaging effects of oxidative free radicals on neurons (Zhu et al., 2017). GBE also has efficacy in treating other NDs; for example, EGb761 protected against MPTP-induced neuronal or neuron-like cell apoptosis in PD by increasing the activation of BCL2, maintaining the stability of MMP, and reducing the activation of caspase-3 through a mitochondrion-dependent pathway. This indicated the potential of EGb761 therapy in treating AD (Kang et al., 2007). Additionally, in vivo and in vitro preclinical studies and relevant clinical trials suggest that EGb761 may have promising therapeutic effects for preventing and treating AD and other age-related NDs (Singh et al., 2019).
3.3 Monomeric compounds isolated from TCMs
TCMs exert their curative effects through the isolated active compounds. Many TCMs have been pharmacodynamically characterized, and some, such as Andrographis paniculata (Burm.f.) Nees (Chuanxinlian), Crocus sativus L. (Xihuanghua), Gardenia jasminoides J.Ellis (Zhizi), Tripterygium wilfordii Hook F. (Leigongteng), have been shown to effectively improve damaged neurological function.
Andrographolide (AG), a diterpene lactone compound, is one of the main active ingredients of Andrographis paniculata (Burm.f.) Nees (Chuanxinlian). With the recent continuous research on the pharmacological functions of AG, several studies have shown that AG has good neuroprotective effects and promising clinical applications (Lu et al., 2019). A seven-month-long prophylactic administration of AG sulfonate reduced the oxidative stress and mitochondrial swelling in the Aβ precursor protein (APP)/Presenilin-1 (PS1) transgenic mice (Geng et al., 2018). AG also prevented excessive mitochondrial fission and neuronal damage in the striatum of PD mice by binding to DRP1, a target AG protein (Geng et al., 2019).
Crocin, an ester compound with antioxidant and anti-inflammatory effects from Crocus sativus L. (Xihonghua), reportedly delays the development of neurological diseases (Farkhondeh et al., 2018). Crocin pretreatment in an in vitro AD model significantly increased cell viability, reduced apoptosis, alleviated mitochondrial dysfunction, and inhibited intracellular oxidative stress and Ca2+ overload (Wang et al., 2019a). Furthermore, crocin also inhibited mitochondrial dysfunction in PD by restoring MMP and ATP synthesis and inhibiting the cytochrome c (Cyt c) release into the cytoplasm (Zhang et al., 2015a). In addition to Crocus sativus L. (Xihuanghua), crocin is also one of the active compounds of Gardenia jasminoides J.Ellis (Zhizi). However, the main pharmacologically active ingredient of G. jasminoides J.Ellis (Zhizi) is geniposide, which exhibits similar pharmacological effects as crocin. Research has proven that geniposide is protective against mitochondrial dysfunction by preventing AD progression (Lv et al., 2014; Zhao et al., 2016). Moreover, geniposide also exerted neuroprotective effects in PD through the mitochondrion-mediated apoptotic pathway (Chen et al., 2015). Among many anti-free radical natural products, silibinin is one of the few drugs widely used in clinical practice. The exact efficacy and low toxicity of silibinin were demonstrated through clinical applications over the last 30 years. Recent studies reported that silibinin alleviated motor dysfunction in a PD mouse model by inhibiting oxidative stress, neuroinflammation, and imbalance of mitochondrial dynamics and promoting mitophagy and mitochondrial biogenesis (Liu et al., 2021b; Liu et al., 2021c). In an in vivo AD model, the silibinin intervention significantly reduced the Ca2+ overload-induced mitochondrial swelling and improved the fluidity of the mitochondrial membrane (Esselun et al., 2021).
Huperzine A (HupA) is a sesquiterpenoid alkaloid isolated from Huperzia serrata (Thunb.) Trev. (Qiancengta), whose potential in treating AD has been demonstrated through numerous preclinical studies and clinical trials (Yang et al., 2013). HupA exhibited a good safety profile in clinical trials without serious adverse events. It has been proposed that the neuroprotective mechanism of HupA involves improving energy metabolism and preserving the mitochondrial structure. This was evidenced by the inhibition of Aβ-induced decrease in mitochondrial respiration, ATP synthesis, and transmembrane potential, and the effective prevention of Cyt c release, ROS increase, and mitochondrial swelling in the in vivo and in vitro experiments (Gao et al., 2009; Lei et al., 2015). Early studies suggested that salidroside, the main active compound of Rhodiola crenulata (Hook.f. and Thomson) H.Ohba (Hongjingtian), is a potential neuroprotective agent (Zhong et al., 2018). Salidroside improved cell viability by inhibiting Aβ1-42-induced cytotoxicity and the mitochondrion-mediated endogenous apoptotic pathway (Liao et al., 2019). Damaged mitochondrial complex I and oxidative stress play a crucial role in degenerating dopaminergic (DA) neurons during PD progression. Studies showed that treating PD with salidroside improved cell viability, inhibited apoptosis, restored MMP and Mitochondrial Complex I activity, and protected DA neurons by mediating the mitochondrial MEF2D-ND6 pathway (Li et al., 2020). Triptolide (a diterpenoid) and celastrol (a triterpenoid) are the most active components of Tripterygium wilfordii Hook F. (Leigongteng), which have been used to effectively treat several rheumatic diseases and immune system disorders (Tong et al., 2021). Several studies demonstrated that triptolide and celastrol have protective effects on neurons and glial cells but through different mechanisms. The apoptosis and oxidative stress inhibition is the key protective mechanism of triptolide against AD (Xu et al., 2016). Unlike triptolide, celastrol ensures mitochondrial quality by isolating damaged mitochondria for autophagosome degradation. This suggests that celastrol reduces DA neuronal death, mitochondrial membrane depolarization, and ATP reduction by activating mitochondrial autophagy to degrade damaged mitochondria, and inhibiting apoptosis of DA neurons, providing a new prevention and treatment mechanism for PD (Lin et al., 2019).
4 TCM prevents mitochondrial dysfunction in NDs by regulating ncRNAs
Currently, the active compounds of TCM reportedly regulate the expression of genes through ncRNAs, which participate in various signaling pathways associated with different therapeutic activities, such as anti-tumor, anti-inflammation, and anti-atherosclerosis activities. However, there are fewer reports on anti-ND research, especially mitochondrial dysfunction modulation for treating NDs, which is still in its early stages. The TCMs regulating various mitochondrial dysfunctions by targeting ncRNAs associated with NDs are listed in Table 4. The subsequent section summarizes several active compounds of TCM that have been well-studied.
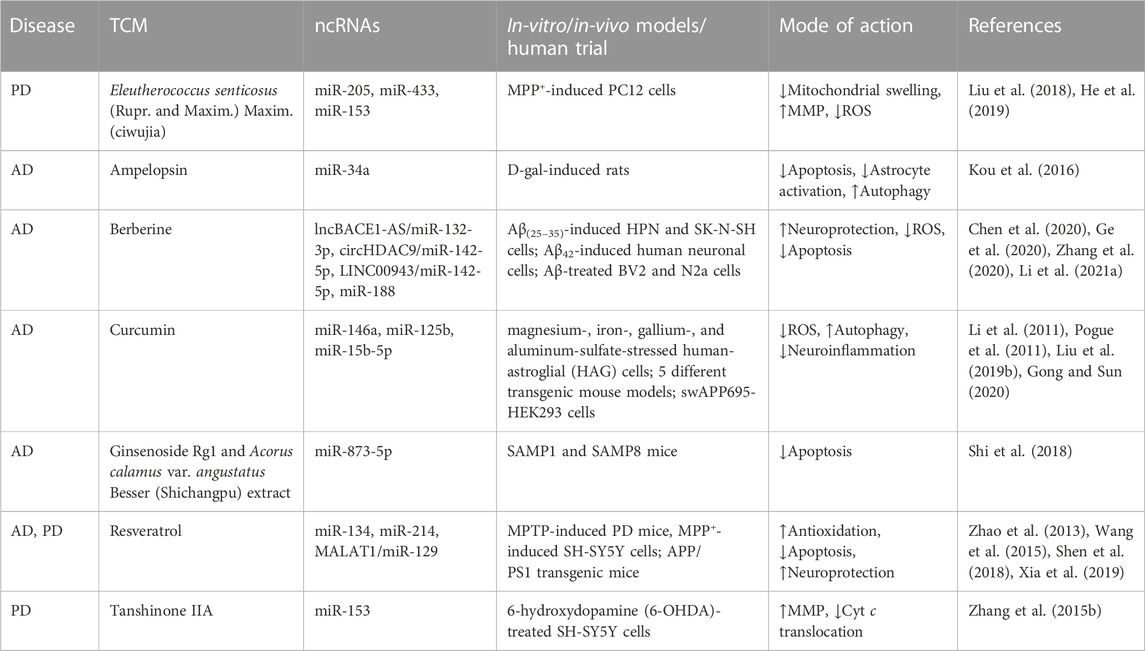
TABLE 4. Summary of traditional Chinese medicines (TCMs) alleviating mitochondrial dysfunction in neurodegenerative diseases (NDs) by modulating non-coding RNAs (ncRNAs).
Curcumin is a polyphenol and a principal bioactive component of Curcuma longa L. (Jianghuang), whose neuroprotective effects have been well-studied because of its pleiotropy and broad-spectrum targets in the brain. Several studies reported that curcumin maintains mitochondrial dynamics, biogenesis, synaptic transmission, and integrity by improving mitochondrial fusion/fission balance (Reddy et al., 2016). Moreover, curcumin exhibits better effects when used with other compounds, such as quercetin (Waseem and Parvez, 2016) and berberine (Lin et al., 2020). Various curcumin derivatives improve its bioavailability, stability, and BBB permeability and enhance its neuroprotective and mitochondrial-protective effects (Bagheri et al., 2020). Further research investigated whether curcumin treatment of NDs is associated with ncRNAs. The results showed that during AD development, miR-125b, miR-146a, and miR-15b-5p were significantly up-regulated (Li et al., 2011). A recent study found that curcumin, an NF-κB inhibitor, significantly reduced miR-146a and miR-125b levels (Pogue et al., 2011) by up-regulating the expression of the complement factor H protein, inhibiting M1 microglia phenotype, reducing the inflammatory response, and promoting phagocytosis and clearance of Aβ plaques (Gong and Sun, 2020). Another study showed that curcumin up-regulated miR-15b-5p expression to reduce APP and Aβ levels in swAPP695-HEK293 cells (Liu et al., 2019b). Furthermore, curcumin modulated oxidative stress, apoptosis, and neuroinflammation-mediated mitochondrial dysfunction by targeting miRNAs, indicating its potential development as a drug for treating NDs (Xu et al., 2019).
The role of berberine (isoquinoline alkaloid), the main active ingredient extracted from Coptis chinensis Franch. (Huanglian), in neurological disorders has been increasingly explored. In recent years, berberine has been developed to protect motor neurons and exhibits potential therapeutic effects in neurodegenerative lesions. Additionally, some studies have confirmed the role of berberine in maintaining synaptic structure and function. Berberine improved mitochondrial dynamics and biogenesis and prevented synaptic loss by maintaining the MMP and preventing ATP reduction (Zhao et al., 2019). Although the underlying protective mechanisms of berberine remain elusive, results demonstrated the berberine derivative, BBRP, rapidly and specifically accumulated in mitochondria and inhibited the accumulation of PINK1 protein when it exerted anti-PD effects. This suggested that the potential berberine target in the brain for PD therapy is mitochondria (Wang et al., 2021b). It has been reported that berberine could inhibit the NF-κB pathway by down-regulating LINC00943 and up-regulating miR-142-5p levels, thereby protecting the cells from MPP-induced neuronal injury (Li et al., 2021a). Furthermore, berberine elevated circRNA HDAC9 expression and reduced miR-142-5p level, attenuating the toxicity in human nerve cells in AD (Zhang et al., 2020). Another study showed that berberine inhibited apoptosis and oxidative stress but activated synaptic activity and plasticity by acting on lncRNA BACE1-AS to up-regulate miR-132-3p level in neurons, inducing a significant restoration of Aβ-induced neuronal cell viability (Ge et al., 2020). Moreover, it was shown that berberine accelerated cell viability and inhibited caspase-3 activity and apoptosis through the miR-188/NOS1 axis, thereby reducing neuronal injury (Chen et al., 2020). Thus, based on these studies, berberine exerts its neuroprotective effects by targeting multiple miRNAs, lncRNAs, circRNAs, and various signaling pathways, providing new targets for the treatment action of NDs.
Resveratrol, a natural polyphenol, is one of the active ingredients of Reynoutria japonica Houtt. (Huzhang) and is found in various natural plants. Numerous in vivo and ex vivo experiments showed that resveratrol exerts neuroprotective effects in various NDs, including AD, PD, HD, and ALS. Moreover, resveratrol regulates mitochondrial dysfunction in NDs through different mechanisms. In AD, resveratrol prevented Aβ-induced mitochondrial fusion/fission imbalance by maintaining the levels of dynamics-related genes (MFN2 and DRP1). Resveratrol also inhibited the activity of Cyt c oxidase, alleviating mitochondrial swelling and fragmentation (Yang et al., 2020). In addition to the aforementioned molecular mechanisms, resveratrol might also act by down-regulating miR-134 and miR-124 expression, stimulating the CREB/BDNF signaling pathway and restoring synaptic plasticity to improve learning and memory (Zhao et al., 2013; Shen et al., 2018). In PD, resveratrol administration modulated mitochondrial biogenesis by enhancing mitochondrial mass and fusion/fission balancing, increasing the ATP levels (Peng et al., 2016). Moreover, the level of miR-214 was significantly reduced in the mesencephalon of PD mice and MPP+-induced SH-SY5Y cells; however, treatment with resveratrol increased the miR-214 expression level in the PD-associated models and reduced mRNA and protein expression of α-syn (Wang et al., 2015). Recently, miR-214 has been suggested to target genes regulating neuronal growth and differentiation in various ND models. Furthermore, resveratrol administration ameliorated PD symptoms by modulating the MALAT1/miR-129/SNCA pathway involved in mitochondrion-mediated apoptosis (Xia et al., 2019), suggesting a potential association of resveratrol action with miRNA function and mitochondrial signal.
5 Summary and prospect
Because of their diverse phenotypes and complex pathogenesis, there have been no effective drugs to cure NDs. However, due to their therapeutic effects, TCMs may act as potential ncRNA regulators, providing a new perspective for elucidating the “multi-compound-multi-target-multi-pathway” treatment mechanism of NDs. Mitochondrial dysfunction is an early pathological phenomenon in NDs, important for drug discovery in preclinical studies. This review explored the mechanisms by which the most studied ncRNAs contributed to the mitochondrial dysfunction in NDs. The review also focused on the TCM formulas (including Sijunzi Decoction and Dihuang Yinzi), standardized extracts from individual TCMs [e. g. Eleutherococcus senticosus (Rupr. and Maxim.) Maxim. (Ciwujia) extract and GBE] and the monomeric compounds isolated from various TCMs (such as andrographolide, crocin, and silibinin) with promising clinical applications in treating NDs. These active components of TCM formulas exerted neuroprotective effects in various ND models by targeting mitochondria. The neuroprotective effects included inhibiting oxidative stress, suppressing apoptosis, activating mitophagy, and maintaining mitochondrial homeostasis. Furthermore, we reviewed the ongoing research efforts on representative components which regulate ncRNA-evoked mitochondrial function and pathways for NDs therapy. Given the complexity and instability of the TCM ingredients, further analysis and exploration of the multi-targets and multi-pathways involved in the ncRNA-mitochondrial regulation network are needed. Therefore, understanding the role and mechanisms of ncRNAs in mitochondrial function will help discover new targets and potential drug candidates for NDs.
Author contributions
ZC and ML wrote the manuscript; LZ, KZ, CW, and TS participated in writing; RL and ZL supervised and revised the draft. All authors read and approved the final manuscript.
Funding
This work was supported by the National Natural Science Foundation of China (No. U1803281 and 82173806), Non-profit Central Research Institute Fund of Chinese Academy of Medical Sciences (2022-JKCS-08), and Chinese Academy of Medical Sciences (CAMS) Innovation Fund for Medical Science (2021-1-I2M-030 and 2022-I2M-2-002).
Conflict of interest
The authors declare that the research was conducted in the absence of any commercial or financial relationships that could be construed as a potential conflict of interest.
Publisher’s note
All claims expressed in this article are solely those of the authors and do not necessarily represent those of their affiliated organizations, or those of the publisher, the editors and the reviewers. Any product that may be evaluated in this article, or claim that may be made by its manufacturer, is not guaranteed or endorsed by the publisher.
References
Aimaiti, M., Wumaier, A., Aisa, Y., Zhang, Y., Xirepu, X., Aibaidula, Y., et al. (2021). Acteoside exerts neuroprotection effects in the model of Parkinson's disease via inducing autophagy: Network pharmacology and experimental study. Eur. J. Pharmacol. 903, 174136. doi:10.1016/j.ejphar.2021.174136
Baev, A. Y., Vinokurov, A. Y., Novikova, I. N., Dremin, V. V., Potapova, E. V., and Abramov, A. Y. (2022). Interaction of mitochondrial calcium and ROS in neurodegeneration. Cells 11 (4), 706. doi:10.3390/cells11040706
Bagheri, H., Ghasemi, F., Barreto, G. E., Rafiee, R., Sathyapalan, T., and Sahebkar, A. (2020). Effects of curcumin on mitochondria in neurodegenerative diseases. Biofactors 46 (1), 5–20. doi:10.1002/biof.1566
Cai, B., Xie, D., Wang, Y., Li, J., Wang, T., Ye, S., et al. (2018). Effect of huangpu tongqiao capsule on oxidative stress of hippocampal neurons and mitochondrial apoptotic pathway in rats with Alzheimer's disease. Chin. J. Integr. Tradit. West Med. Chin. Ed. 38, 707–711. doi:10.7661/j.cjim.20171018.269
Cai, Z., Liu, M., Yuan, F., Zeng, L., Zhao, K., Sun, T., et al. (2022a). Exploration of the molecular mechanisms of Hyssopus cuspidatus boriss treatment of asthma in an mRNA-miRNA network via bioinformatics analysis. Biomed. Res. Int. 2022, 7111901. doi:10.1155/2022/7111901
Cai, Z., Zhao, K., Zeng, L., Liu, M., Sun, T., Li, Z., et al. (2022b). The relationship between the aberrant long non-coding RNA-mediated competitive endogenous RNA network and Alzheimer's disease pathogenesis. Int. J. Mol. Sci. 23 (15), 8497. doi:10.3390/ijms23158497
Cao, B., Zeng, M., Zhang, Q., Zhang, B., Cao, Y., Wu, Y., et al. (2021). Amentoflavone ameliorates memory deficits and abnormal autophagy in aβ25-35-induced mice by mTOR signaling. Neurochem. Res. 46 (4), 921–934. doi:10.1007/s11064-020-03223-8
Cao, Q., Qin, L., Huang, F., Wang, X., Yang, L., Shi, H., et al. (2017). Amentoflavone protects dopaminergic neurons in MPTP-induced Parkinson's disease model mice through PI3K/Akt and ERK signaling pathways. Toxicol. Appl. Pharmacol. 319, 80–90. doi:10.1016/j.taap.2017.01.019
Cao, Y., Wang, Z., and Song, W. (2019). Effects of bushen tiansui fang on learning and memory abilities and oxidative stress of mitochondria of Alzheimer's disease model rat. J. Tradit. Chin. Med. Univ. 39, 459–464.
Chen, J., Gao, L., Zhang, Y., Su, Y., Kong, Z., Wang, D., et al. (2021). Acteoside-improved streptozotocin-induced learning and memory impairment by upregulating hippocampal insulin, glucose transport, and energy metabolism. Phytother. Res. 35 (1), 392–403. doi:10.1002/ptr.6811
Chen, M., Chen, Z., Wang, Y., Tan, Z., Zhu, C., Li, Y., et al. (2016). Mitophagy receptor FUNDC1 regulates mitochondrial dynamics and mitophagy. Autophagy 12 (4), 689–702. doi:10.1080/15548627.2016.1151580
Chen, M., Li, L., Liu, C., and Song, L. (2020). Berberine attenuates Aβ-induced neuronal damage through regulating miR-188/NOS1 in Alzheimer's disease. Mol. Cell Biochem. 474 (1-2), 285–294. doi:10.1007/s11010-020-03852-1
Chen, Y., Zhang, Y., Li, L., and Holscher, C. (2015). Neuroprotective effects of geniposide in the MPTP mouse model of Parkinson's disease. Eur. J. Pharmacol. 768, 21–27. doi:10.1016/j.ejphar.2015.09.029
Cosín-Tomás, M., Antonell, A., Lladó, A., Alcolea, D., Fortea, J., Ezquerra, M., et al. (2017). Plasma miR-34a-5p and miR-545-3p as early biomarkers of Alzheimer’s disease: Potential and limitations. Mol. Neurobiol. 54 (7), 5550–5562. doi:10.1007/s12035-016-0088-8
du Mee, D., Bak, M., Østergaard, E., and Rasmussen, L. J. (2020). Mitochondrial dysfunction induced by variation in the non-coding genome - A proposed workflow to improve diagnostics. Mitochondrion 53, 255–259. doi:10.1016/j.mito.2020.05.013
Dong, P., Ji, X., Han, W., Han, H., Bak, M., Østergaard, E., et al. (2019). Oxymatrine attenuates amyloid beta 42 (Abeta1-42)-induced neurotoxicity in primary neuronal cells and memory impairment in ratsMitochondrial dysfunction induced by variation in the non-coding genome - a proposed workflow to improve diagnostics. Can. J. Physiol. PharmacolMitochondrion 9753 (2), 99255–106259. doi:10.1139/cjpp-2018-0299
Esselun, C., Bruns, B., Hagl, S., Grewal, R., and Eckert, G. P. (2021). Impact of silibinin A on bioenergetics in PC12APPsw cells and mitochondrial membrane properties in murine brain mitochondria. Antioxidants (Basel) 10 (10), 1520. doi:10.3390/antiox10101520
Fan, Z., Chen, X., and Chen, R. (2014). Transcriptome-wide analysis of TDP-43 binding small RNAs identifies miR-NID1 (miR-8485), a novel miRNA that represses NRXN1 expression. Genomics 103 (1), 76–82. doi:10.1016/j.ygeno.2013.06.006
Farkhondeh, T., Samarghandian, S., Shaterzadeh, Y. H., and Samini, F. (2018). The protective effects of crocin in the management of neurodegenerative diseases: A review. Am. J. Neurodegener. Dis. 7 (1), 1–10.
Feng, Z., Zhang, L., Wang, S., and Hong, Q. (2020). Circular RNA circDLGAP4 exerts neuroprotective effects via modulating miR-134-5p/CREB pathway in Parkinson's disease. Biochem. Biophys. Res. Commun. 522 (2), 388–394. doi:10.1016/j.bbrc.2019.11.102
Friedemann, T., Otto, B., Klatschke, K., Schumacher, U., Tao, Y., Leung, A. K., et al. (2014). Coptis chinensis Franch. exhibits neuroprotective properties against oxidative stress in human neuroblastoma cells. J. Ethnopharmacol. 155 (1), 607–615. doi:10.1016/j.jep.2014.06.004
Gan, P., Ding, L., Hang, G., Xia, Q., Huang, Z., Ye, X., et al. (2020). Oxymatrine attenuates dopaminergic neuronal damage and microglia-mediated neuroinflammation through cathepsin D-dependent HMGB1/TLR4/NF-κB pathway in Parkinson's disease. Front. Pharmacol. 11, 776. doi:10.3389/fphar.2020.00776
Gao, X., Zheng, C. Y., Yang, L., Tang, X. C., and Zhang, H. Y. (2009). Huperzine A protects isolated rat brain mitochondria against beta-amyloid peptide. Free Radic. Biol. Med. 46 (11), 1454–1462. doi:10.1016/j.freeradbiomed.2009.02.028
Ge, Y., Song, X., Liu, J., Liu, C., and Xu, C. (2020). The combined therapy of berberine treatment with lncRNA BACE1-AS depletion attenuates aβ25-35 induced neuronal injury through regulating the expression of miR-132-3p in neuronal cells. Neurochem. Res. 45 (4), 741–751. doi:10.1007/s11064-019-02947-6
Geng, J., Liu, W., Gao, J., Jiang, C., Fan, T., Sun, Y., et al. (2019). Andrographolide alleviates Parkinsonism in MPTP-PD mice via targeting mitochondrial fission mediated by dynamin-related protein 1. Br. J. Pharmacol. 176 (23), 4574–4591. doi:10.1111/bph.14823
Geng, J., Liu, W., Xiong, Y., Ding, H., Jiang, C., Yang, X., et al. (2018). Andrographolide sulfonate improves Alzheimer-associated phenotypes and mitochondrial dysfunction in APP/PS1 transgenic mice. Biomed. Pharmacother. 97, 1032–1039. doi:10.1016/j.biopha.2017.11.039
Gong, J., and Sun, D. (2020). Study on the mechanism of curcumin to reduce the inflammatory response of temporal lobe in Alzheimer's disease by regulating miR-146a. Minerva Med. 113 (1), 109–118. doi:10.23736/S0026-4806.20.06463-0
Guo, S. Q. (2016). Identification and analysis of constituents absorbed into cerebrospinal fluid after oral administration of Dihuang Yinzi. J. North Pharm. 13 (2), 132–134.
Guo, Y., Zheng, Q., Wu, L., Zhang, M., Zhang, K., Xiao, S., et al. (2020). Effect of Sijunzi Decoction on regulation of Aβ-related proteins across blood-brain barrier. China J. Chin. Mat. Med. 45, 2924–2931. doi:10.19540/j.cnki.cjcmm.20200217.402
Han, Y., Chu, X., Cui, L., Fu, S., Gao, C., Li, Y., et al. (2020). Neuronal mitochondria-targeted therapy for Alzheimer's disease by systemic delivery of resveratrol using dual-modified novel biomimetic nanosystems. Drug Deliv. 27 (1), 502–518. doi:10.1080/10717544.2020.1745328
He, H., Wang, S., Tian, J., Chen, L., Zhang, W., Zhao, J., et al. (2015). Protective effects of 2,3,5,4'-tetrahydroxystilbene-2-O-β-D-glucoside in the MPTP-induced mouse model of Parkinson's disease: Involvement of reactive oxygen species-mediated JNK, P38 and mitochondrial pathways. Eur. J. Pharmacol. 767, 175–182. doi:10.1016/j.ejphar.2015.10.023
He, X., Pan, S., Wu, C., Sun, H., and Bai, Y. (2019). Effective component of acanthopanax senticosus on the expression of micro-RNA in cell of Parkinson's disease mode. Jilin J. Chin. Med. 39, 381–384. doi:10.13463/j.cnki.jlzyy.2019.03.030
Hou, D. R., Wang, Y., Xue, L., Tian, Y., Chen, K., Song, Z., et al. (2008). Effect of polygonum multiflorum on the fluidity of the mitochondria membrane and activity of COX in the hippocampus of rats with Abeta 1-40-induced Alzheimer's disease. Zhong Nan Da Xue Xue Bao Yi Xue Ban. 33 (11), 987–992.
Hu, Z., Huang, Y., Liu, G., Liu, F., Zhou, W., and Zhang, Y. (2012). Research progress of the impact of Chinese medicine on hippocampus based on the animal model of VD. Pharmacol. Clin. Chin. Mater Med. 28, 252–256. doi:10.13412/j.cnki.zyyl.2012.05.070
Huang, L., Deng, M., Zhang, S., Lu, S., Gui, X., and Fang, Y. (2017). β-asarone and levodopa coadministration increases striatal levels of dopamine and levodopa and improves behavioral competence in Parkinson's rat by enhancing dopa decarboxylase activity. Biomed. Pharmacother. 94, 666–678. doi:10.1016/j.biopha.2017.07.125
Huang, Q., Zhao, Y., Gao, J., Jing, Z., Zhang, N., and Ma, T. (2018). Effect of Dihuang Yinzi on mitochondrial structure and function in central nerve of Alzheimer's disease rats. Chin. J. Exp. Tradit. Med. Formulae 24, 99–104. doi:10.13422/j.cnki.syfjx.20182137
Huang, S. H., Lin, C. M., and Chiang, B. H. (2008). Protective effects of Angelica sinensis extract on amyloid beta-peptide-induced neurotoxicity. Phytomedicine 15 (9), 710–721. doi:10.1016/j.phymed.2008.02.022
Indrieri, A., Carrella, S., Romano, A., Spaziano, A., Marrocco, E., Fernandez-Vizarra, E., et al. (2019). miR-181a/b downregulation exerts a protective action on mitochondrial disease models. EMBO Mol. Med. 11 (5), e8734. doi:10.15252/emmm.201708734
Jadiya, P., Garbincius, J. F., and Elrod, J. W. (2021). Reappraisal of metabolic dysfunction in neurodegeneration: Focus on mitochondrial function and calcium signaling. Acta Neuropathol. Commun. 9 (1), 124. doi:10.1186/s40478-021-01224-4
Jiang, H., Fang, J., Xing, J., Wang, L., Wang, Q., Wang, Y., et al. (2019). Tilianin mediates neuroprotection against ischemic injury by attenuating CaMKII-dependent mitochondrion-mediated apoptosis and MAPK/NF-κB signaling. Life Sci. 216, 233–245. doi:10.1016/j.lfs.2018.11.035
Jiang, H., Liu, J., Guo, S., Zeng, L., Cai, Z., Zhang, J., et al. (2022). miR-23b-3p rescues cognition in Alzheimer's disease by reducing tau phosphorylation and apoptosis via GSK-3β signaling pathways. Mol. Ther. Nucleic Acids 28, 539–557. doi:10.1016/j.omtn.2022.04.008
Johnson, J., Mercado-Ayon, E., Mercado-Ayon, Y., Dong, Y. N., Halawani, S., Ngaba, L., et al. (2021). Mitochondrial dysfunction in the development and progression of neurodegenerative diseases. Arch. Biochem. Biophys. 702, 108698. doi:10.1016/j.abb.2020.108698
Kang, X., Chen, J., Xu, Z., Li, H., and Wang, B. (2007). Protective effects of Ginkgo biloba extract on paraquat-induced apoptosis of PC12 cells. Toxicol Vitro 21 (6), 1003–1009. doi:10.1016/j.tiv.2007.02.004
Kattimani, Y., and Veerappa, A. M. (2018). Dysregulation of NRXN1 by mutant MIR8485 leads to calcium overload in pre-synapses inducing neurodegeneration in Multiple sclerosis. Mult. Scler. Relat. Disord. 22, 153–156. doi:10.1016/j.msard.2018.04.005
Keihani, S., Kluever, V., Mandad, S., Bansal, V., Rahman, R., Fritsch, E., et al. (2019). The long noncoding RNA neuroLNC regulates presynaptic activity by interacting with the neurodegeneration-associated protein TDP-43. Sci. Adv. 5 (12), eaay2670. doi:10.1126/sciadv.aay2670
Kim, H. G., Park, G., Piao, Y., Kang, M. S., Pak, Y. K., Hong, S. P., et al. (2014). Effects of the root bark of Paeonia suffruticosa on mitochondria-mediated neuroprotection in an MPTP-induced model of Parkinson's disease. Food Chem. Toxicol. 65, 293–300. doi:10.1016/j.fct.2013.12.037
Kou, X., Li, J., Liu, X., Chang, J., Zhao, Q., Jia, S., et al. (2017). Swimming attenuates d-galactose-induced brain aging via suppressing miR-34a-mediated autophagy impairment and abnormal mitochondrial dynamics. J. Appl. Physiol. 122 (6), 1462–1469. doi:10.1152/japplphysiol.00018.2017
Kou, X., Liu, X., Chen, X., Li, J., Yang, X., Fan, J., et al. (2016). Ampelopsin attenuates brain aging of D-gal-induced rats through miR-34a-mediated SIRT1/mTOR signal pathway. Oncotarget 7 (46), 74484–74495. doi:10.18632/oncotarget.12811
Kumar, A., and Singh, A. (2015). A review on mitochondrial restorative mechanism of antioxidants in Alzheimer's disease and other neurological conditions. Front. Pharmacol. 6, 206. doi:10.3389/fphar.2015.00206
Kumar, S., Reddy, A. P., Yin, X., and Reddy, P. H. (2019). Novel MicroRNA-455-3p and its protective effects against abnormal APP processing and amyloid beta toxicity in Alzheimer's disease. Biochim. Biophys. Acta Mol. Basis Dis. 1865 (9), 2428–2440. doi:10.1016/j.bbadis.2019.06.006
Kumar, S., Vijayan, M., and Reddy, P. H. (2017). MicroRNA-455-3p as a potential biomarker for Alzheimer's disease: An update. Front. Aging Neurosci. 10, 41. doi:10.3389/fnagi.2018.00041
Lee, J. E., Sim, H., Yoo, H. M., Lee, M., Baek, A., Jeon, Y. J., et al. (2020). Neuroprotective effects of cryptotanshinone in a direct reprogramming model of Parkinson's disease. Molecules 25 (16), 3602. doi:10.3390/molecules25163602
Lei, Y., Yang, L., Ye, C. Y., Qin, M. Y., Yang, H. Y., Jiang, H. L., et al. (2015). Involvement of intracellular and mitochondrial Aβ in the ameliorative effects of huperzine A against oligomeric aβ42-induced injury in primary rat neurons. PLoS One 10 (5), e0128366. doi:10.1371/journal.pone.0128366
Lejri, I., Agapouda, A., Grimm, A., and Eckert, A. (2019). Mitochondria- and oxidative stress-targeting substances in cognitive decline-related disorders: From molecular mechanisms to clinical evidence. Oxid. Med. Cell Longev. 2019, 9695412. doi:10.1155/2019/9695412
Li, H., Song, J., Zhang, J., Wang, T., Yan, Y., Tao, Z., et al. (2017a). Ginseng protein reverses amyloid beta peptide and H2O2 cytotoxicity in neurons, and ameliorates cognitive impairment in AD rats induced by a combination of D-galactose and AlCl3. Phytother. Res. 31 (2), 284–295. doi:10.1002/ptr.5747
Li, H., Zhang, X. J., and Dong, M. X. (2017b). Isorhynchophylline attenuates MPP+-Induced apoptosis through endoplasmic reticulum stress- and mitochondria-dependent pathways in PC12 cells: Involvement of antioxidant activity. Neuromolecular Med. 19 (4), 480–492. doi:10.1007/s12017-017-8462-x
Li, T., Zhang, W., Kang, X., Yang, R., Li, R., Huang, L., et al. (2020). Salidroside protects dopaminergic neurons by regulating the mitochondrial MEF2D-ND6 pathway in the MPTP/MPP+-induced model of Parkinson's disease. J. Neurochem. 153 (2), 276–289. doi:10.1111/jnc.14868
Li, X., Jiang, X., Chu, H., Zhao, Q., Ding, H., and Cai, C. (2021b). Neuroprotective effects of kukoamine A on 6-OHDA-induced Parkinson's model through apoptosis and iron accumulation inhibition. Chin. Herb. Med. 13 (1), 105–115. doi:10.1016/j.chmed.2020.12.004
Li, X., Su, Y., Li, N., Zhang, F. R., and Zhang, N. (2021a). Berberine attenuates MPP+-Induced neuronal injury by regulating linc00943/miR-142-5p/KPNA4/NF-κB pathway in SK-N-sh cells. Neurochem. Res. 46 (12), 3286–3300. doi:10.1007/s11064-021-03431-w
Li, Y. Y., Cui, J. G., Hill, J. M., Bhattacharjee, S., Zhao, Y., and Lukiw, W. J. (2011). Increased expression of miRNA-146a in Alzheimer's disease transgenic mouse models. Neurosci. Lett. 487 (1), 94–98. doi:10.1016/j.neulet.2010.09.079
Liao, Z. L., Su, H., Tan, Y. F., Qiu, Y. J., Zhu, J. P., Chen, Y., et al. (2019). Salidroside protects PC-12 cells against amyloid β-induced apoptosis by activation of the ERK1/2 and AKT signaling pathways. Int. J. Mol. Med. 43 (4), 1769–1777. doi:10.3892/ijmm.2019.4088
Lin, L., Li, C., Zhang, D., Yuan, M., Chen, C. H., and Li, M. (2020). Synergic effects of berberine and curcumin on improving cognitive function in an Alzheimer's disease mouse model. Neurochem. Res. 45 (5), 1130–1141. doi:10.1007/s11064-020-02992-6
Lin, M. W., Lin, C. C., Chen, Y. H., Yang, H. B., and Hung, S. Y. (2019). Celastrol inhibits dopaminergic neuronal death of Parkinson's disease through activating mitophagy. Antioxidants (Basel) 9 (1), 37. doi:10.3390/antiox9010037
Liu, H. Y., Fu, X., Li, Y. F., Li, X. L., Ma, Z. Y., Zhang, Y., et al. (2019b). miR-15b-5p targeting amyloid precursor protein is involved in the anti-amyloid eflect of curcumin in swAPP695-HEK293 cells. Neural Regen. Res. 14 (9), 1603–1609. doi:10.4103/1673-5374.255979
Liu, H., Yu, C., Xu, T., Zhang, X., and Dong, M. (2016). Synergistic protective effect of paeoniflorin and β-ecdysterone against rotenone-induced neurotoxicity in PC12 cells. Apoptosis 21 (12), 1354–1365. doi:10.1007/s10495-016-1293-7
Liu, H. Y., Wang, L., Liu, H., Ma, D., Liu, W., Yu, H., et al. (2019a). Effect of Sijunzi tang on behavior and energy metabolism of hippocampal neurons in rats with Alzheimer’s disease. Chin. J. Exp. Tradit. Med. Formulae 25, 1–6. doi:10.13422/j.cnki.syfjx.20191538
Liu, M., Yu, S., Wang, J., Qiao, J., Liu, Y., Wang, S., et al. (2020). Ginseng protein protects against mitochondrial dysfunction and neurodegeneration by inducing mitochondrial unfolded protein response in Drosophila melanogaster PINK1 model of Parkinson's disease. J. Ethnopharmacol. 247, 112213. doi:10.1016/j.jep.2019.112213
Liu, S. M., Li, X. Z., Zhang, S. N., Yang, Z. M., Wang, K. X., Lu, F., et al. (2018). Acanthopanax senticosus protects structure and function of mesencephalic mitochondria in A mouse model of Parkinson's disease. Chin. J. Integr. Med. 24 (11), 835–843. doi:10.1007/s11655-018-2935-5
Liu, X., Liu, W., Wang, C., Chen, Y., Liu, P., Hayashi, T., et al. (2021b). Silibinin attenuates motor dysfunction in a mouse model of Parkinson's disease by suppression of oxidative stress and neuroinflammation along with promotion of mitophagy. Physiol. Behav. 239, 113510. doi:10.1016/j.physbeh.2021.113510
Liu, X., Shan, G., Jiang, H., Zeng, L., Zhao, K., Li, Y., et al. (2021a). Identification of miRNA and their regulatory effects induced by total flavonoids from dracocephalum moldavica in the treatment of vascular dementia. Front. Pharmacol. 12, 796628. doi:10.3389/fphar.2021.796628
Liu, X., Wang, C., Liu, W., Song, S., Fu, J., Hayashi, T., et al. (2021c). Oral administration of silibinin ameliorates cognitive deficits of Parkinson's disease mouse model by restoring mitochondrial disorders in Hippocampus. Neurochem. Res. 46 (9), 2317–2332. doi:10.1007/s11064-021-03363-5
Lu, J., Ma, Y., Wu, J., Huang, H., Wang, X., Chen, Z., et al. (2019). A review for the neuroprotective effects of andrographolide in the central nervous system. Biomed. Pharmacother. 117, 109078. doi:10.1016/j.biopha.2019.109078
Lv, C., Liu, X., Liu, H., Chen, T., and Zhang, W. (2014). Geniposide attenuates mitochondrial dysfunction and memory deficits in APP/PS1 transgenic mice. Curr. Alzheimer Res. 11 (6), 580–587. doi:10.2174/1567205011666140618095925
Ma, B., Meng, X., Wang, J., Sun, J., Ren, X., Qin, M., et al. (2014). Notoginsenoside R1 attenuates amyloid-beta-induced damage in neurons by inhibiting reactive oxygen species and modulating MAPK activation. Int. Immunopharmacol. 22 (1), 151–159. doi:10.1016/j.intimp.2014.06.018
Ma, Y., Tian, S., Sun, L., Yao, S., Liang, Z., Li, S., et al. (2015). The effect of acori graminei rhizoma and extract fractions on spatial memory and hippocampal neurogenesis in amyloid beta 1-42 injected mice. CNS Neurol. Disord. Drug Targets 14 (3), 411–420. doi:10.2174/1871527314666150225124348
Mei, Z., Yan, P., Situ, B., Mou, Y., and Liu, P. (2012). Cryptotanshinione inhibits beta-amyloid aggregation and protects damage from beta-amyloid in SH-SY5Y cells. Neurochem. Res. 37 (3), 622–628. doi:10.1007/s11064-011-0652-6
Musthaba, M., Baboota, S., Athar, T. M., Thajudeen, K. Y., Ahmed, S., and Ali, J. (2010). Patented herbal formulations and their therapeutic applications. Recent Pat. Drug Deliv. Formul. 4 (3), 231–244. doi:10.2174/187221110793237538
Naia, L., Rosenstock, T. R., Oliveira, A. M., Oliveira-Sousa, S. I., Caldeira, G. L., Carmo, C., et al. (2017). Comparative mitochondrial-based protective effects of resveratrol and nicotinamide in Huntington's disease models. Mol. Neurobiol. 54 (7), 5385–5399. doi:10.1007/s12035-016-0048-3
Nunomura, A., and Perry, G. (2020). RNA and oxidative stress in Alzheimer's disease: Focus on microRNAs. Oxid. Med. Cell Longev. 2020, 2638130. doi:10.1155/2020/2638130
Peng, K., Tao, Y., Zhang, J., Wang, J., Ye, F., Dan, G., et al. (2016). Resveratrol regulates mitochondrial biogenesis and fission/fusion to attenuate rotenone-induced neurotoxicity. Oxid. Med. Cell Longev. 2016, 6705621. doi:10.1155/2016/6705621
Pierzynowska, K., Podlacha, M., Gaffke, L., Majkutewicz, I., Mantej, J., Wegrzyn, A., et al. (2019). Autophagy-dependent mechanism of genistein-mediated elimination of behavioral and biochemical defects in the rat model of sporadic Alzheimer's disease. Neuropharmacology 148, 332–346. doi:10.1016/j.neuropharm.2019.01.030
Pogue, A. I., Percy, M. E., Cui, J. G., Li, Y. Y., Bhattacharjee, S., Hill, J. M., et al. (2011). Up-regulation of NF-kB-sensitive miRNA-125b and miRNA-146a in metal sulfate-stressed human astroglial (HAG) primary cell cultures. J. Inorg. Biochem. 105 (11), 1434–1437. doi:10.1016/j.jinorgbio.2011.05.012
Qian, C., Ye, Y., Mao, H., Yao, L., Sun, X., Wang, B., et al. (2019). Downregulated lncRNA-SNHG1 enhances autophagy and prevents cell death through the miR-221/222/p27/mTOR pathway in Parkinson’s disease. Exp. Cell Res. 384 (1), 111614. doi:10.1016/j.yexcr.2019.111614
Reddy, P. H., Manczak, M., Yin, X., Grady, M. C., Mitchell, A., Kandimalla, R., et al. (2016). Protective effects of a natural product, curcumin, against amyloid beta induced mitochondrial and synaptic toxicities in Alzheimer's disease. J. Investig. Med. 64 (8), 1220–1234. doi:10.1136/jim-2016-000240
Ren, Z. L., Wang, C. D., Wang, T., Ding, H., Zhou, M., Yang, N., et al. (2019). Ganoderma lucidum extract ameliorates MPTP-induced parkinsonism and protects dopaminergic neurons from oxidative stress via regulating mitochondrial function, autophagy, and apoptosis. Acta Pharmacol. Sin. 40 (4), 441–450. doi:10.1038/s41401-018-0077-8
Sarkar, S., Jun, S., Rellick, S., Quintana, D. D., Cavendish, J. Z., and Simpkins, J. W. (2016). Expression of microRNA-34a in Alzheimer's disease brain targets genes linked to synaptic plasticity, energy metabolism, and resting state network activity. Brain Res. 1646, 139–151. doi:10.1016/j.brainres.2016.05.026
Shan, C. S., Zhang, H. F., Xu, Q. Q., Shi, Y. H., Wang, Y., Li, Y., et al. (2018). Herbal medicine formulas for Parkinson's disease: A systematic review and meta-analysis of randomized double-blind placebo-controlled clinical trials. Front. Aging Neurosci. 10, 349. doi:10.3389/fnagi.2018.00349
Sharma, N., Pasala, M. S., and Prakash, A. (2019). Mitochondrial DNA: Epigenetics and environment. Environ. Mol. Mutagen 60 (8), 668–682. doi:10.1002/em.22319
Shen, J., Xu, L., Qu, C., Sun, H., and Zhang, J. (2018). Resveratrol prevents cognitive deficits induced by chronic unpredictable mild stress: Sirt1/miR-134 signalling pathway regulates CREB/BDNF expression in hippocampus in vivo and in vitro. Behav. Brain Res. 349, 1–7. doi:10.1016/j.bbr.2018.04.050
Shi, R., Zhang, S., Cheng, G., Yang, X., Zhao, N., and Chen, C. (2018). Ginsenoside Rg1 and acori graminei rhizoma attenuates neuron cell apoptosis by promoting the expression of miR-873-5p in Alzheimer's disease. Neurochem. Res. 43 (8), 1529–1538. doi:10.1007/s11064-018-2567-y
Shin, S. J., Jeon, S. G., Kim, J. I., Jeong, Y. O., Kim, S., Park, Y. H., et al. (2019). Red Ginseng attenuates aβ-induced mitochondrial dysfunction and aβ-mediated pathology in an animal model of Alzheimer's disease. Int. J. Mol. Sci. 20 (12), 3030. doi:10.3390/ijms20123030
Singh, N. K., and Garabadu, D. (2021). Quercetin exhibits α7nAChR/nrf2/HO-1-Mediated neuroprotection against STZ-induced mitochondrial toxicity and cognitive impairments in experimental rodents. Neurotox. Res. 39 (6), 1859–1879. doi:10.1007/s12640-021-00410-5
Singh, S. K., Srivastav, S., Castellani, R. J., Plascencia-Villa, G., and Perry, G. (2019). Neuroprotective and antioxidant effect of ginkgo biloba extract against AD and other neurological disorders. Neurotherapeutics 16 (3), 666–674. doi:10.1007/s13311-019-00767-8
Stockburger, C., Eckert, S., Eckert, G. P., Friedland, K., and Müller, W. E. (2018). Mitochondrial function, dynamics, and permeability transition: A complex love triangle as A possible target for the treatment of brain aging and Alzheimer's disease. J. Alzheimers Dis. 64 (1), S455–S467. doi:10.3233/JAD-179915
Sun, H., Bai, L., Zhang, J., Xu, H., and Cui, L. (2005). Effects of yinxing pingchan recipe and its components on activity of mitochondrial enzyme complex in brain of mice with Parkinson’s disease. Chin. J. Integr. Tradit. West Med. Chin. Ed. 25, 1008–1011. doi:10.7661/CJIM.2005.11.1008
Sun, T., Zeng, L., Cai, Z., Liu, Q., Li, Z., and Liu, R. (2022b). Comprehensive analysis of dysregulated circular RNAs and construction of a ceRNA network involved in the pathology of Alzheimer's disease in a 5 × FAD mouse model. Front. Aging Neurosci. 14, 1020699. doi:10.3389/fnagi.2022.1020699
Sun, T., Zhao, K., Liu, M., Cai, Z., Zeng, L., Zhang, J., et al. (2022a). miR-30a-5p induces Aβ production via inhibiting the nonamyloidogenic pathway in Alzheimer's disease. Pharmacol. Res. 178, 106153. doi:10.1016/j.phrs.2022.106153
Thounaojam, M. C., Jadeja, R. N., Warren, M., Powell, F. L., Raju, R., Gutsaeva, D., et al. (2019). MicroRNA-34a (miR-34a) mediates retinal endothelial cell premature senescence through mitochondrial dysfunction and loss of antioxidant activities. Antioxidants (Basel). 8 (9), 328. doi:10.3390/antiox8090328
Titze-de-Almeida, R., and Titze-de-Almeida, S. S. (2018). miR-7 replacement therapy in Parkinson's disease. Curr. Gene Ther. 18 (3), 143–153. doi:10.2174/1566523218666180430121323
Tong, L., Zhao, Q., Datan, E., Lin, G. Q., Minn, I., Pomper, M. G., et al. (2021). Triptolide: Reflections on two decades of research and prospects for the future. Nat. Prod. Rep. 38 (4), 843–860. doi:10.1039/d0np00054j
Tu, J. L., Chen, W. P., Cheng, Z. J., Zhang, G., Luo, Q. H., Li, M., et al. (2020). EGb761 ameliorates cell necroptosis by attenuating RIP1-mediated mitochondrial dysfunction and ROS production in both in vivo and in vitro models of Alzheimer's disease. Brain Res. 1736, 146730. doi:10.1016/j.brainres.2020.146730
Um, M. Y., Choi, W. H., Aan, J. Y., Kim, S. R., and Ha, T. Y. (2006). Protective effect of Polygonum multiflorum Thunb on amyloid beta-peptide 25-35 induced cognitive deficits in mice. J. Ethnopharmacol. 104 (1-2), 144–148. doi:10.1016/j.jep.2005.08.054
Wang, C., Cai, X., Hu, W., Li, Z., Kong, F., Chen, X., et al. (2019a). Investigation of the neuroprotective effects of crocin via antioxidant activities in HT22 cells and in mice with Alzheimer's disease. Int. J. Mol. Med. 43 (2), 956–966. doi:10.3892/ijmm.2018.4032
Wang, C., Jiang, H., Wang, L., Yi, H., Li, Z., and Liu, R. (2019b). Vitegnoside mitigates neuronal injury, mitochondrial apoptosis, and inflammation in an Alzheimer's disease cell model via the p38 MAPK/JNK pathway. J. Alzheimers Dis. 72 (1), 199–214. doi:10.3233/JAD-190640
Wang, H., Han, R., He, H. J., Li, J., Chen, S. Y., Gu, Y., et al. (2021d). Administration of quercetin improves mitochondria quality control and protects the neurons in 6-OHDA-lesioned Parkinson's disease models. Aging (Albany NY) 13 (8), 11738–11751. doi:10.18632/aging.202868
Wang, H., Sheng, W., Tan, Z., Ren, Q., Wang, R., Stoika, R., et al. (2021b). Treatment of Parkinson's disease in Zebrafish model with a berberine derivative capable of crossing blood brain barrier, targeting mitochondria, and convenient for bioimaging experiments. Comp. Biochem. Physiol. C Toxicol. Pharmacol. 249, 109151. doi:10.1016/j.cbpc.2021.109151
Wang, H., Wang, H., Pan, Q., Kang, J., Liang, Z., and Zhang, R. (2021c). The combination of beta-asarone and icariin inhibits amyloid-beta and reverses cognitive deficits by promoting mitophagy in models of Alzheimer's disease. Oxid. Med. Cell Longev. 2021, 7158444. doi:10.1155/2021/7158444
Wang, H., Xie, Q., Liu, Y., Jiang, S., Li, W., Li, B., et al. (2021e). Panax japonicus and chikusetsusaponins: A review of diverse biological activities and pharmacology mechanism. Chin. Herb. Med. 13 (1), 64–77. doi:10.1016/j.chmed.2020.12.003
Wang, H., Zhang, M., Wei, T., Zhou, J., Zhang, Y., and Guo, D. (2021a). Long non-coding RNA SNHG1 mediates neuronal damage in Parkinson's disease model cells by regulating miR-216a-3p/Bcl-2-associated X protein. Ann. Transl. Med. 9 (10), 851. doi:10.21037/atm-21-1613
Wang, J., Wong, Y. K., and Liao, F. (2018). What has traditional Chinese medicine delivered for modern medicine? Expert Rev. Mol. Med. 20, e4. doi:10.1017/erm.2018.3
Wang, Z. H., Zhang, J. L., Duan, Y. L., Zhang, Q. S., Li, G. F., and Zheng, D. L. (2015). MicroRNA-214 participates in the neuroprotective effect of Resveratrol via inhibiting α-synuclein expression in MPTP-induced Parkinson's disease mouse. Biomed. Pharmacother. 74, 252–256. doi:10.1016/j.biopha.2015.08.025
Waseem, M., and Parvez, S. (2016). Neuroprotective activities of curcumin and quercetin with potential relevance to mitochondrial dysfunction induced by oxaliplatin. Protoplasma 253 (2), 417–430. doi:10.1007/s00709-015-0821-6
Woo, H. N., Park, S., Kim, H. L., Jung, M. K., Pack, C. G., Park, J., et al. (2020). miR-351-5p/Miro2 axis contributes to hippocampal neural progenitor cell death via unbalanced mitochondrial fission. Mol. Ther. Nucleic Acids 23, 643–656. doi:10.1016/j.omtn.2020.12.014
Wu, H. C., Hu, Q. L., Zhang, S. J., Wang, Y. M., Jin, Z. K., Lv, L. F., et al. (2018). Neuroprotective effects of genistein on SH-SY5Y cells overexpressing A53T mutant α-synuclein. Neural Regen. Res. 13 (8), 1375–1383. doi:10.4103/1673-5374.235250
Wu, Y., Zhao, J., Ma, T., and Wei, D. (2015). Effects of tianqi yizhi granules on mitochondria of brain tissues and energy metabolism in alzheimer rats. Chin. J. Inf. Tradit. Chin. Med. 22, 60–64.
Xia, D., Sui, R., and Zhang, Z. (2019). Administration of resveratrol improved Parkinson's disease-like phenotype by suppressing apoptosis of neurons via modulating the MALAT1/miR-129/SNCA signaling pathway. J. Cell Biochem. 120 (4), 4942–4951. doi:10.1002/jcb.27769
Xiao, X., Tan, Z., Jia, M., Zhou, X., Wu, K., Ding, Y., et al. (2021). Long noncoding RNA SNHG1 knockdown ameliorates apoptosis, oxidative stress and inflammation in models of Parkinson's disease by inhibiting the miR-125b-5p/MAPK1 Axis. Neuropsychiatr. Dis. Treat. 17, 1153–1163. doi:10.2147/NDT.S286778
Xu, H., Nie, B., Liu, L., Zhang, C., Zhang, Z., Xu, M., et al. (2019). Curcumin prevents brain damage and cognitive dysfunction during ischemic-reperfusion through the regulation of miR-7-5p. Curr. Neurovasc Res. 16 (5), 441–454. doi:10.2174/1567202616666191029113633
Xu, P., Wang, H., Li, Z., and Yang, Z. (2016). Triptolide attenuated injury via inhibiting oxidative stress in Amyloid-Beta25-35-treated differentiated PC12 cells. Life Sci. 145, 19–26. doi:10.1016/j.lfs.2015.12.018
Xu, Y., Shen, X., Lan, R., Zhu, S., Zhang, J., and Lu, F. (2021). Mechanism of kaixinsan on learning and memory ability and synaptic function of APP/PS1 mice. Chin. J. Exp. Tradit. Med. Formulae 27, 15–22. doi:10.13422/j.cnki.syfjx.20212036
Yan, W., Biao, C., Jing, S., Ting-ting, W., Run-ze, C., Chang-ju, M., et al. (2016). Genistein suppresses the mitochondrial apoptotic pathway in hippocampal neurons in rats with Alzheimer's disease. Neural Regen. Res. 11 (7), 1153–1158. doi:10.4103/1673-5374.187056
Yang, G., Wang, Y., Tian, J., and Liu, J. P. (2013). Huperzine A for Alzheimer's disease: A systematic review and meta-analysis of randomized clinical trials. PLoS One 8 (9), e74916. doi:10.1371/journal.pone.0074916
Yang, P., Sheng, D., Guo, Q., Wang, P., Xu, S., Qian, K., et al. (2020). Neuronal mitochondria-targeted micelles relieving oxidative stress for delayed progression of Alzheimer's disease. Biomaterials 238, 119844. doi:10.1016/j.biomaterials.2020.119844
Yuan, D., Tao, M., Ren, X., Wei, J., Fu, W., Ma, Y., et al. (2016). Tongluo Xingnao Effervescent Tablet preserves mitochondrial energy metabolism and attenuates cognition deficits in APPswe/PS1De9 mice. Neurosci. Lett. 630, 101–108. doi:10.1016/j.neulet.2016.07.044
Zeng, L., Jiang, H., Ashraf, G. M., Liu, J., Wang, L., Zhao, K., et al. (2021). Implications of miR-148a-3p/p35/PTEN signaling in tau hyperphosphorylation and autoregulatory feedforward of Akt/CREB in Alzheimer's disease. Mol. Ther. Nucleic Acids 27, 256–275. doi:10.1016/j.omtn.2021.11.019
Zhang, G. F., Ha, S., Wang, X. L., Shi, Y. L., Duan, S. S., and Li, Z. A. (2015b). Tanshinone IIA protects dopaminergic neurons against 6-hydroxydopamine-induced neurotoxicity through miR-153/NF-E2-related factor 2/antioxidant response element signaling pathway. Neuroscience 303, 489–502. doi:10.1016/j.neuroscience.2015.06.030
Zhang, G. F., Zhang, Y., and Zhao, G. (2015a). Crocin protects PC12 cells against MPP+-induced injury through inhibition of mitochondrial dysfunction and ER stress. Neurochem. Int. 89, 101–110. doi:10.1016/j.neuint.2015.07.011
Zhang, J., Li, D., Yang, G., Zhang, X., Chen, L., Zhang, Y., et al. (2021a). Oxymatrine extends survival by attenuating neuroinflammation in a mouse model of amyotrophic lateral sclerosis. Neuroscience 465, 11–22. doi:10.1016/j.neuroscience.2021.04.019
Zhang, J., Sun, H., Bai, L., Xu, H., and Wu, H. (2004). The experimental study on treatment of Yinxingchuchan Recipe and its different combination on free radical in the model mouse with Parkinson disease. Chin. Arch. Tradit. Chin. Med. 911–913. doi:10.13193/j.archtcm.2004.05.140.zhangj.072
Zhang, J. X., Wang, R., Xi, J., Shen, L., Zhu, A. Y., Qi, Q., et al. (2017). Morroniside protects SK-N-SH human neuroblastoma cells against H2O2-induced damage. Int. J. Mol. Med. 39 (3), 603–612. doi:10.3892/ijmm.2017.2882
Zhang, J., Zhou, X., Wu, D., Yang, M., Zheng, C., and Tong, T. (2021b). Study on mechanism of Sijunzi Decoction in treating Alzheimer’s disease based on network pharmacology. Nat. Prod. Res. Dev. 33, 313–321. doi:10.16333/j.1001-6880.2021.2.016
Zhang, L., Wang, Y. Y., Zhou, J. B., Ke, B., Yang, Y. B., Qin, J., et al. (2018). Effects and mechanisms of Dihuang Yinzi Decoction on the treatment of Alzheimer’s disease patients. China J. Tradit. Chin. Med. Pharm. 33 (11), 4948–4952.
Zhang, N., Gao, Y., Yu, S., Sun, X., and Shen, K. (2020). Berberine attenuates Aβ42-induced neuronal damage through regulating circHDAC9/miR-142-5p axis in human neuronal cells. Life Sci. 252, 117637. doi:10.1016/j.lfs.2020.117637
Zhang, Y., Gong, X. G., Wang, Z. Z., Sun, H. M., Guo, Z. Y., Gai, C., et al. (2016). Protective effects of DJ-1 medicated Akt phosphorylation on mitochondrial function are promoted by Da-Bu-Yin-Wan in 1-methyl-4-phenylpyridinium-treated human neuroblastoma SH-SY5Y cells. J. Ethnopharmacol. 187, 83–93. doi:10.1016/j.jep.2016.04.029
Zhao, C., Lv, C., Li, H., Du, S., Liu, X., Li, Z., et al. (2016). Geniposide protects primary cortical neurons against oligomeric abeta1-42-induced neurotoxicity through a mitochondrial pathway. PLoS One 89, 101–110. doi:10.1016/j.neuint.2015.07.011
Zhao, C., Su, P., Lv, C., Guo, L., Cao, G., Qin, C., et al. (2019). Berberine alleviates amyloid β-induced mitochondrial dysfunction and synaptic loss. Oxid. Med. Cell Longev. 2019, 7593608. doi:10.1155/2019/7593608
Zhao, J., Geng, L., Chen, Y., and Wu, C. (2020). SNHG1 promotes MPP+-induced cytotoxicity by regulating PTEN/AKT/mTOR signaling pathway in SH-SY5Y cells via sponging miR-153-3p. Biol. Res. 53 (1), 1. doi:10.1186/s40659-019-0267-y
Zhao, K., Zeng, L., Cai, Z., Liu, M., Sun, T., Li, Z., et al. (2022). RNA sequencing-based identification of the regulatory mechanism of microRNAs, transcription factors, and corresponding target genes involved in vascular dementia. Front. Neurosci. 16, 917489. doi:10.3389/fnins.2022.917489
Zhao, Y. N., Li, W. F., Li, F., Zhang, Z., Dai, Y. D., Xu, A. L., et al. (2013). Resveratrol improves learning and memory in normally aged mice through microRNA-CREB pathway. Biochem. Biophys. Res. Commun. 435 (4), 597–602. doi:10.1016/j.bbrc.2013.05.025
Zhong, Z., Han, J., Zhang, J., Xiao, Q., Hu, J., and Chen, L. (2018). Pharmacological activities, mechanisms of action, and safety of salidroside in the central nervous system. Drug Des. Devel Ther. 12, 1479–1489. doi:10.2147/DDDT.S160776
Zhu, B., Wang, C., and Xu, Q. (2021). Clinical study on Dihuang Yinzi combined with cognitive rehabilitation for mild cognitive impairment in Parkinson disease. New J. Tradit. Chin. Med. 53, 38–41. doi:10.13457/j.cnki.jncm.2021.17.009
Keywords: mitochondrial dysfunction, multi-targeting, neurodegenerative diseases, non-coding RNAs, traditional Chinese medicine
Citation: Cai Z, Liu M, Zeng L, Zhao K, Wang C, Sun T, Li Z and Liu R (2023) Role of traditional Chinese medicine in ameliorating mitochondrial dysfunction via non-coding RNA signaling: Implication in the treatment of neurodegenerative diseases. Front. Pharmacol. 14:1123188. doi: 10.3389/fphar.2023.1123188
Received: 13 December 2022; Accepted: 17 February 2023;
Published: 01 March 2023.
Edited by:
Xia Wu, Capital Medical University, ChinaReviewed by:
Linlin Yin, Beijing Tiantan Hospital, Capital Medical University, ChinaJie Liu, Jinan Central Hospital, China
Junli Wang, Minzu University of China, China
Copyright © 2023 Cai, Liu, Zeng, Zhao, Wang, Sun, Li and Liu. This is an open-access article distributed under the terms of the Creative Commons Attribution License (CC BY). The use, distribution or reproduction in other forums is permitted, provided the original author(s) and the copyright owner(s) are credited and that the original publication in this journal is cited, in accordance with accepted academic practice. No use, distribution or reproduction is permitted which does not comply with these terms.
*Correspondence: Zhuorong Li, bGl6aHVvcm9uZ0BpbWIucHVtYy5lZHUuY24=; Rui Liu, bGl1cnVpQGltYi5wdW1jLmVkdS5jbg==
†These authors have contributed equally to this work