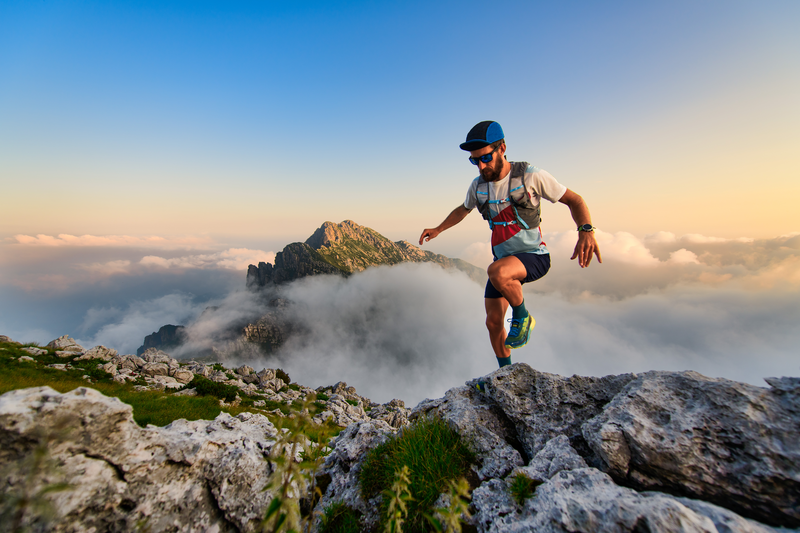
94% of researchers rate our articles as excellent or good
Learn more about the work of our research integrity team to safeguard the quality of each article we publish.
Find out more
REVIEW article
Front. Pharmacol. , 27 March 2023
Sec. Ethnopharmacology
Volume 14 - 2023 | https://doi.org/10.3389/fphar.2023.1122632
This article is part of the Research Topic The Role of Medicinal Plants and Natural Products in Modulating Oxidative Stress and Inflammatory Related Disorders, Volume II View all 8 articles
Acetaminophen (APAP) is a widely used analgesic and antipyretic over-the-counter medicine worldwide. Hepatotoxicity caused by APAP overdose is one of the leading causes of acute liver failure (ALF) in the US and in some parts of Europe, limiting its clinical application. Excessive APAP metabolism depletes glutathione and increases N-acetyl-p-benzoquinoneimide (NAPQI) levels, leading to oxidative stress, DNA damage, and cell necrosis in the liver, which in turn leads to liver damage. Studies have shown that natural products such as polyphenols, terpenes, anthraquinones, and sulforaphane can activate the hepatocyte antioxidant defense system with Nrf2 as the core player, reduce oxidative stress damage, and protect the liver. As the key enzyme metabolizing APAP into NAPQI, cytochrome P450 enzymes are also considered to be intriguing target for the treatment of APAP-induced liver injury. Here, we systematically review the hepatoprotective activity and molecular mechanisms of the natural products that are found to counteract the hepatotoxicity caused by APAP, providing reference information for future preclinical and clinical trials of such natural products.
Acetaminophen (APAP), also called paracetamol, is one of the most widely used analgesic and antipyretic over-the-counter drugs in the world (Lee, 2008; McCracken, 2015). According to the statistics, more than 60 million Americans take APAP every week. It is also worth noting that APAP is used in combination with other drugs, particularly opioids and diphenhydramine, without public awareness (Athersuch et al., 2018; Jasani et al., 2018; Rajaram and Subramanian, 2018). Although APAP is considered safe under therapeutic doses, its overdose can induce severe liver toxicity and even death (Agrawal and Khazaeni, 2022). The hepatotoxicity resulting from an overdose of APAP is the leading cause of acute liver failure (ALF) in the United States and in some parts of Europe, accounting for more than 50% of ALF cases in these regions (Yoon et al., 2016). In severe circumstances, liver transplantation is the only option that might possibly save the patient’s life. APAP-induced liver injury is emerging as a public health issue (Yang et al., 2022a).
The precise molecular mechanism of APAP-induced liver injury has not been fully elucidated yet. Under the therapeutic concentrations, approximately 60%–90% of APAP is metabolized in the liver by glucuronidation and sulfation, with a small part (approximately 5%–15%) being metabolized by the cytochrome P450 pathway (CYP450) (Kalsi et al., 2011; Marto et al., 2021). Probably due to the binding preference of APAP to the active site of each P450 isomer, it is oxidized via two pathways to form the toxic intermediate N-acetyl-p-benzoquinone imine (NAPQI) and the non-toxic catechol metabolite 3-hydroxy-APAP (3-OH-APAP) (Chen et al., 1998). Simultaneous quantification of these two oxidized metabolites by electrochemical HPLC assay demonstrated that human P450 2E1 selectively oxidizes APAP to NAPQI (determined as glutathione conjugate, GS-APAP), whereas human P450 2A6 selectively oxidizes APAP to 3-OH-APAP (Chen et al., 1998). Under the overdose condition, more APAP is converted to NAPQI by cytochrome P450 enzymes (Figure 1). Chen et al. (1998) NAPQI oxidizes the thiol groups of proteins and generates reactive oxygen species (ROS) (Iorga et al., 2017). Both NAPQI and ROS cause mitochondrial DNA damage, activation of the JNK signaling pathway, which further amplifies the mitochondrial ROS production, and causes the mitochondrial permeability transition (MPT) pore to open (Iorga et al., 2017). Glutathione conjugates with the generated NAPQI to harmless thiolate and cysteine compounds, which will be eliminated by the kidney. Overdose of APAP depletes glutathione reservoir, leading to the rise of NAPQI level. NAPQI will then bind to the cellular macromolecules, including proteins, lipids, and nucleic acids, resulting in centrilobular liver injury and hepatocyte death (McGill et al., 2012; More et al., 2017; Ramachandran et al., 2018; Guengerich, 2020). At earlier time, the cell death resulted from APAP toxicity has been suggested to be apoptosis (Ray et al., 1996; El-Hassan et al., 2003), necrosis (Iorga and Dara, 2019; Jaeschke et al., 2019). However, no definitive conclusion could be reached with the current evidence. Ferroptosis has also been indicated to be involved in APAP hepatotoxicity (Yamada et al., 2020), which is not supported by the data obtained under pathophysiologically relevant condition (Jaeschke et al., 2021; Adelusi et al., 2022). More recently, several studies suggested that pyroptosis is the type of cell death after APAP overdose (Wang et al., 2021; Liu et al., 2022; Rousta et al., 2022).
Currently, N-acetylcysteine (NAC) is the only U.S. Food and Drug Administration approved treatment for APAP induced liver injury (Andrade et al., 2019). However, because of the narrow therapeutic window for acute liver injury and the side effects of NAC, its clinical application has great limitations (Du et al., 2016). Inhibitors targeting the enzymatic activity of P450, such as 4-methylpyrazole (Fomepizole), are evaluated in vitro and in vivo as possible alternative treatments for APAP induced liver injury (Akakpo et al., 2018; Akakpo et al., 2019; Kang et al., 2020; Akakpo et al., 2022; Kaiser and Dart, 2022). Natural products have long been considered important sources of novel medicines and therapeutics. A number of natural products, such as triterpenoid saponins (Xu et al., 2018), schisandra lignans (Zhu et al., 2019), polysaccharides (Wang et al., 2018), iridoids (Shao et al., 2017), flavonoids and quinones (Darvin et al., 2018), have been shown to have protective activity against APAP-induced hepatotoxicity. In this review, we summarized the most recent research progress on natural product-derived ingredients, which have beneficial effects on the liver injury caused by APAP. The information from this review could serve as a reference for the further development of natural product-based treatments for APAP-induced liver injury.
A number of phytochemicals have been identified to have hepatoprotective activity, such as silymarin for the treatment of liver poisoning, chronic hepatitis and cirrhosis (Abenavoli et al., 2018), resveratrol and curcumin for the relief of liver damage, etc., (McGill et al., 2015; Khan et al., 2019) (Table 1). Liver-protecting natural products often have diverse activities, including antioxidant, anti-inflammatory, immunomodulatory, and antiviral effects (Kim et al., 2015; Ilyas et al., 2016; Abdullah et al., 2017; Sharifi-Rigi et al., 2019). These compounds have the effect of alleviating APAP-induced liver injury and can be further developed as antioxidants or hepatoprotective agents (Figure 2).
FIGURE 2. Chemical structures of potential metabolites for the treatment of acetaminophen-induced liver injury.
Silymarin, the flavonoid extract from the Silybum marianum (L.) Gaertn. [Asteraceae] plant, has been used to prevent various liver diseases (Polyak et al., 2010). Silymarin has been reported to act as an antioxidant by reducing free radical production and lipid peroxidation, function as a toxin blocker by inhibiting the binding of toxins to the hepatocyte cell membrane receptors (Abenavoli et al., 2010), and reduce the superoxide and peroxynitrite content by its scavenger activity (Papackova et al., 2018). Recent research has shown that silymarin reduces acute toxic liver injury caused by APAP by increasing hepatocyte proliferation, decreasing CYP2E1 activity and expression, and decreasing the production of toxic metabolites (Elsayed Elgarawany et al., 2020; Ihedioha et al., 2020; Yang et al., 2022b). Silibinin is the major active component of silymarin. The nanoparticles of silibinin exhibited antioxidant effects against intracellular oxidative stress by upregulating the Nrf2/ARE pathway, reducing ROS, modulating antioxidant enzyme responsiveness, and inhibiting downstream pathways of mitochondrial damage (Ding et al., 2022). The above findings suggest that silymarin has the potential to be further developed as an antioxidant against APAP.
Resveratrol, a non-flavonoid phenolic substance, has been shown to have a hepatoprotective effect by attenuating oxidative stress in the liver (Das, 2011; Dalaklioglu et al., 2013; Ahmad and Ahmad, 2014). It was found to be an irreversible inhibitor of CYP3A4 and a non-competitive reversible inhibitor of CYP2E1 (Piver et al., 2001; Wang et al., 2015). It also inhibits the activity of CYP3A11 and CYP1A2, preventing the bioactivation of APAP to the toxic metabolite NAPQI (Piver et al., 2001; Wang et al., 2015). Resveratrol treatment has been shown to reduce oxidative stress in mouse tissues by increasing the expression of antioxidants and phase II enzymes in response to the stress (Rubiolo et al., 2008; Cerný et al., 2009; Wong et al., 2009). Interestingly, studies have shown that the antioxidant activity of this compound has a circadian rhythm, having antioxidant properties when it is in the dark but pro-oxidant properties when it is in the light (Gadacha et al., 2009). SIRT6 downregulation inhibits Nrf2 activation, whereas sirtuin 6 (SIRT6) upregulation reduces oxidative stress-associated DNA damage and promotes hepatocyte proliferation, thereby preventing APAP hepatotoxicity (Zhou et al., 2021). Resveratrol, a potent SIRT1 activator, promotes cell survival by regulating SIRT1-dependent p53 deacetylation (Howitz et al., 2003; Kim et al., 2011). Another study showed that resveratrol induced SIRT1 expression and the expression of cyclin D1, cyclin-dependent kinase 4 (CDK4) and proliferating cell nuclear antigen (PCNA) to promote liver regeneration, thereby preventing acetaminophen-induced hepatotoxicity (Wang et al., 2015). In addition, resveratrol has been shown to protect against APAP-induced liver injury when administered therapeutically. It exerts a protective effect by scavenging peroxynitrite and preventing the release of AIF and EndoG from mitochondria and subsequent nuclear DNA breakage (Du et al., 2015). The above studies suggest that resveratrol may be an effective option for the treatment of APAP overdose.
Tannins is well-known for its anti-oxidative stress activity. The ability of taninins to protect the liver from damage caused by APAP has also been looked into. Tannins significantly reduced the phase I and phase II enzyme activities in mouse liver tissues (Krajka-Kuźniak and Baer-Dubowska, 2003). Tannic acid exerts anti-apoptotic activity by down-regulating the caspase-3, Bax, and up-regulating Bcl-2, and activates the antioxidant defense system by up-regulating Nrf2 and HO-1 (Zhang et al., 2017).
Salvianolic acid B, a rosmarinic acid dimer, is an active component of Salvia miltiorrhiza Bunge [Lamiaceae], and its reduction of drug-induced liver injury is associated with its antioxidant activity (Gao et al., 2012; Lin et al., 2015). In HepG2 cells, salvianolic acid B inhibited the expression of CYP3A4 and CYP1A2, induced the expression of GST (Wang et al., 2011). Also, salvianolic acid B pretreatment induces Nrf2 and phase II enzymes by activating phosphoinositide 3-kinase (PI3K) and protein kinase C (PKC) pathways, thereby preventing acetaminophen-induced hepatotoxicity in mice (Lin et al., 2015). The above studies suggest that the hepatoprotective activity of salvianolic acid B against APAP is achieved by inhibiting cytochrome P450 enzymes and/or synergizing phase II metabolic enzymes.
Chlorogenic acid, a phenolic compound with various biological activities, ameliorates liver injury in an experimentally induced model of oxidative stress (Pang et al., 2015). Chlorogenic acid is a P450 enzyme inhibitor, which can decrease the expression of CYP2E1 and CYP1A2 (Pang et al., 2015). Chlorogenic acid inhibited APAP-induced activation of caspase-3 and caspase-7, JNK, ERK1/2, and upstream molecular signaling of p38 MAPKs in animals, including apoptosis signal-regulated kinase 1 (ASK1), c-Raf, and Mitogen-activated protein kinases MEK1/2, MKK3/6 and MKK4 (Ji et al., 2013), thereby inhibiting apoptosis. Chlorogenic acid inhibits the binding of Nrf2 to its repressor protein Keap1 to activate the Nrf2 antioxidant signaling pathway, thereby preventing APAP-induced hepatotoxicity (Wei et al., 2018). Apart from that, the activation of Nrf2 by chlorogenic acid restores mitochondrial ion protein homologue (Lon) protein expression and reduces mitochondrial HSP60 release, attenuating APAP-induced inflammatory liver injury (Hu et al., 2020). Studies have shown that the hepatotoxicity of APAP can lead to mitochondrial dysfunction and affect PINK1-mediated mitosis. Chlorogenic acid stabilizes cell function by eliminating mitochondrial damage, increases PINK1-dependent mitosis, inhibits apoptosis of liver cells, and prevents APAP hepatotoxicity (Hu et al., 2022). Currently, chlorogenic acid is considered a promising hepatic detoxifier for APAP.
Caffeic acid is a common phenolic chemical found in a wide variety of plants. It may protect L02 cells from acetaminophen-induced damage by activating the Keap1-Nrf2 antioxidant defense mechanism. Studies have shown that caffeic acid can inhibit the expression of Keap1, reduce the stabilization of the Keap1 and Nrf2 complex, thereby activate Nrf2 and upregulate the expression of downstream antioxidant enzymes NQO1 and HO-1 (Pang et al., 2016).
Morin, a type of flavonol, is obtained from the wood of the Morus alba plant and has a variety of biological activities, including antioxidant, hypoglycemic, and liver protection. Studies have shown that it can resist the toxicity of APAP to hepatocytes by activating Nrf2. Specifically, the inhibition of Nrf2 ubiquitination increases nuclear Nrf2 retention and ARE-Nrf2 binding affinity (Rizvi et al., 2015a).
Procyanidins from almonds (a subclass of procyanidins), demonstrated protective efficacy against APAP-induced hepatotoxicity in HepG2 cells and mice (Truong et al., 2014). The fundamental mechanism is the activation of phase II detoxification enzymes or antioxidase controlled by Nrf2/ARE, including the expression of NAD(P)H quinone dehydrogenase 1 (NQO1), GPX, and superoxide dismutase (SOD) (Truong et al., 2014). At present, procyanidins are mainly used to alleviate AILI through antioxidants, and whether there are other effects needs further research.
Curcumin, a yellow phenolic pigment extracted from the rhizome of Curcuma longa L. [Zingiberaceae], belongs to the diarylheptane class of metabolites, and is known for its ability to treat a variety of human diseases (Shishodia et al., 2005). According to reports, curcumin is a P450 inhibitor that inhibits CYP2C9, CYP1A2, CYP2D6, CYP2B6 and CYP3A4, with particularly low IC50 values for CYP2C9 (Appiah-Opong et al., 2007). In the system of Ad-P450 cells, it inhibits five P450 enzymes in a concentration-dependent manner (Sasaki et al., 2017). Curcumin inhibits APAP-induced hepatocyte apoptosis by reducing the expression of pro-apoptotic genes Bax and caspase-3, inducing anti-apoptotic genes like Bcl-x1, and increasing the ratio of Bcl2/Bax (Bulku et al., 2012; Li et al., 2013). Curcumin prevents APAP-induced hepatitis by reducing oxidative stress, decreasing liver inflammation, restoring GSH and improving liver histopathology (Somanawat et al., 2013). Furthermore, its protective effect in APAP-induced hepatotoxicity was linked to reduced mitochondrial dysfunction, oxygen consumption, and membrane potential (Granados-Castro et al., 2016). At the same time, a series of studies reported that curcumin also exerts hepatoprotective activity by activating the Nrf2 signaling pathway and regulating ARE-driven antioxidant genes (Lu et al., 2015; Liu et al., 2017; Krupa et al., 2019).
Ginsenosides are the main steroid chemicals found in ginseng roots, extracted from Panax ginseng C.A.Mey. [Araliaceae]. Ginsenoside Rg3 promotes the expression of multidrug resistance proteins (MRP) 1 and 3, activates Nrf2-mediated antioxidant gene expression, participates in detoxification, and reduces liver cytotoxicity (Gum and Cho, 2013a). Meanwhile, ginsenoside Rg3 significantly increased glutathione S-transferase α2 (GSTA2) protein expressionand activated the transcription of GSTA2 downstream of multiple cellular signaling pathways, including protein kinase A (PKA), PI3K and JNK (Gum and Cho, 2013b). Other studies have also shown that it exerts hepatoprotective effects through its anti-oxidant activity. For example, ginsenosides has been shown to increase GPX, SOD and catalase (CAT) activity and restore GSH levels, while inhibiting ERK and JNK MAPK pathways (Park et al., 2012; Li et al., 2014). Therefore, ginsenosides mainly reduce the hepatotoxicity of APAP by inhibiting oxidative stress.
Tanshinone IIA is isolated from Salvia miltiorrhiza Bunge [Lamiaceae] as a diterpene quinone (Fu et al., 2007). It can inhibit various CYP substrates and CYP isomers (Wang et al., 2010). According to the results from in vitro and in vivo studies, tanshinone IIA pretreatment protects the liver from APAP-induced liver injury by activating Nrf2 and increasing the mRNA and protein levels of the Nrf2 target genes glutamate-cysteine ligase catalytic subunit (GCLC), NQO1, and HO-1 (Wang et al., 2016).
Andrographolide, a ladanditerpene extracted from Andrographis paniculata, is a MAPK/Nrf2 pathway activator (Yang et al., 2017). Studies have shown that it inhibits the mRNA and protein expression of CYP1A2, CYP2D6 and CYP3A4 (Ooi et al., 2011). Andrographolide inhibits CYP3A4 activity by binding and antagonizing PXR function, and is a potential CYP3A4 inhibitor that may have clinical significance (Ooi et al., 2011). In addition, andrographolide has a protective effect on APAP-induced hepatotoxicity both in vivo and in vitro (Yan et al., 2018). Mechanistically, it activates Nrf2 and its nuclear translocation, thereby enhancing the expression of downstream antioxidant genes to relieve oxidative stress.
Rhein, an anthraquinone derivative of Rheum palmatum L. [Polygonaceae], induces apoptosis through a caspase-dependent pathway (Shi et al., 2008). Rhein has been shown to reduce APAP-induced oxidative damage to the liver cells (Zhao et al., 2011). Compared with the rats treated with APAP alone, rhein treated animals demonstrated significant reduction of the biochemical indicators of liver injury, including aspartate aminotransferase (AST), alanine aminotransferase (ALT), urea nitrogen (UREA), creatinine (CREA), nitric oxide (NO), and malondialdehyde (MDA). The glutathione (GSH) content was significantly restored, and the histopathological damage in the liver was also significantly improved after rhein treatment. However, its mechanism of action needs to be further studied (Zhao et al., 2011). It has been shown that rhein inhibits CYP2C9, CYP1A2, CYP2E1, CYP2D6 and CYP3A enzymes in rat liver (Tang et al., 2009). It is possible that rhein relieves APAP-induced liver injury through inhibiting the activity of P450 enzymes.
Emodin is an emodin compound isolated from Rheum palmatum L. [Polygonaceae] plants. Emodin and aloe-emodin are isomers with inhibitory effects on CYP1B1 activity (Meng et al., 2022). The conformational relationship indicates that aloe-emodin is more effective due to the different positions of the hydroxyl groups (Meng et al., 2022). Studies have found that emodin has protective effects on the APAP-induced acute liver injury in rats (Bhadauria, 2010). Emodin pretreatment significantly decreased ALT, AST and alkaline phosphatase (ALP) levels; increased albumin (ALB) levels; attenuated SOD and GSH depletion and MDA accumulation. The upregulation of the antioxidant enzymes, including Nrf2, HO-1 and NQO1, eventually leads to the relief of oxidative stress. To protect the liver from acetaminophen-induced inflammation and apoptosis, emodin suppresses interferon (IFN)-α, cyclic GMP-AMP synthase (cGAS), and downstream stimulators of interferon gene (STING) expression (Shen et al., 2022). Emodin also inhibits NLRP3 expression and reduces pro-inflammatory factors like interleukin-1β (IL-1β), IL-6 and TNF-α. These findings imply that emodin protects hepatocytes from APAP-induced liver damage by activating Nrf2-mediated antioxidant stress pathways, inhibiting the NLRP3 inflammasome, and downregulating the cGAS-STING signaling pathway (Shen et al., 2022). Our current understanding is that emodin can exert therapeutic effects through multiple pathways, including antioxidant, anti-inflammatory, and inhibition of CYP450.
Sulforaphane is a metabolite that protects the liver from the toxicity caused by conventional drugs (Noh et al., 2015; Nazmy et al., 2017). Previous research has shown that the hepatoprotective effect of sulforaphane is associated with the suppression of cytochrome P450 enzymes (McCarty, 2001). Inhibition of CYP450 enzymatic activity protects hepatocytes from the toxic metabolites of APAP. The metabolites of sulforaphane inhibit CYP2D6 activity (Vanduchova et al., 2016). Further studies showed that sulforaphane exerts hepatoprotective effects through activating the Nrf2 pathway (Hu et al., 2004). Since oxidative stress is the main cause of APAP-induced hepatotoxicity, sulforaphane protects the liver from APAP overload toxicity by activating the Nrf2 pathway and increasing the endogenous antioxidant response (Schmidt, 1984). One potential mechanism is the Nrf2-ARE pathway, which induces a phase 2 detoxification response that promotes disruption of Nrf2-Keap protein interactions, translocation of Nrf2 to the nucleus, and regulation of target gene expression through the ARE, enhancing cellular defense against oxidative damage (Hu et al., 2004; Lau et al., 2008). Antimycin-like interaction of sulforaphane with the mitochondrial respiratory chain at the complex III level generates ROS, leading to membrane lipid peroxidation and 4-hydroxynonenal production (Sharma et al., 2010). 4-Hydroxynonenal is involved in the signaling of cell proliferation and apoptosis, as well as regulating gene expression in different cell types (Cheng et al., 2001; Yang et al., 2001; Yang et al., 2002; Sharma et al., 2004; Awasthi et al., 2005; Vatsyayan et al., 2011). Interestingly, it activates defense mechanisms against oxidative stress at low concentrations, such as Nrf2 and heat shock factor 1 (Sharma et al., 2008a; Sharma et al., 2008b; Chaudhary et al., 2010), but induces apoptosis at higher supraphysiological concentrations (Awasthi et al., 2008). The study results showed that sulforaphane pretreatment significantly induced the expression of Nrf2, HO-1 and Nqo1 mRNAs and suppressed APAP-induced glutathione (GSH) depletion and lipid peroxidation (Noh et al., 2015). Therefore, sulforaphane should be cautiously developed as a treatment for APAP-induced liver injury. The combination of sulforaphane and APAP at low doses decreased intracellular ROS formation and increased the protein levels of CAT, GPx, Nrf2, NQO1, and HO-1. It indicates that sulforaphane protects against oxidative damage by APAP by enhancing cellular antioxidant activity (Vuong et al., 2019).
Understanding the rationale for the hepatoprotective activity of natural products could guide future drug development. Inhibition of CYP450 enzyme activity and activation of the Nrf2 signaling pathway and GSH synthesis are two intriguing targets for the treatment of acute liver injury caused by APAP. However, improving the therapeutic window of natural hepatoprotective agents, developing drug carriers with excellent properties, and reducing their toxicity remain current and future problems to be overcome. Well-designed randomized clinical studies are needed to systematically evaluate the evidence for the use of these hepatoprotective agents as treatment options for APAP-induced liver injury, to determine the timing of initiation of the therapy, and to further define the optimal treatment regimen.
JnL and QZ conceived the study. JqL, QL, ZL, JtL collected and analyzed the data. JqL, QL, QZ, and JnL wrote the manuscript. All the authors have read the manuscript and proved it for publication.
This study was supported by the National Natural Science Foundation of China to QZ (No.32270407) and the open fund of State Key Laboratory of Southwestern Chinese Medicine Resources (No.SCMR202103) and Natural Science Foundation of Sichuan (No.2022NSFSC0583) to JL.
The authors declare that the research was conducted in the absence of any commercial or financial relationships that could be construed as a potential conflict of interest.
All claims expressed in this article are solely those of the authors and do not necessarily represent those of their affiliated organizations, or those of the publisher, the editors and the reviewers. Any product that may be evaluated in this article, or claim that may be made by its manufacturer, is not guaranteed or endorsed by the publisher.
Abdullah, A., Phern, T. C., Rahim, N. F. H. M., Zaharuddin, N. I., Salihin, N. M., Yusof, A. H., et al. (2017). The effects of cosmos caudatus (ulam Raja) on the levels of expression of Nrf2 target genes in mice liver. J. Pharm. Nutr. Sci. 7, 147–157. doi:10.6000/1927-5951.2017.07.04.1
Abenavoli, L., Capasso, R., Milic, N., and Capasso, F. (2010). Milk thistle in liver diseases: Past, present, future. Phytother. Res. 24, 1423–1432. doi:10.1002/ptr.3207
Abenavoli, L., Izzo, A. A., Milić, N., Cicala, C., Santini, A., and Capasso, R. (2018). Milk thistle (Silybum marianum): A concise overview on its chemistry, pharmacological, and nutraceutical uses in liver diseases. Phytother. Res. 32, 2202–2213. doi:10.1002/ptr.6171
Adelusi, O. B., Ramachandran, A., Lemasters, J. J., and Jaeschke, H. (2022). The role of Iron in lipid peroxidation and protein nitration during acetaminophen-induced liver injury in mice. Toxicol. Appl. Pharmacol. 445, 116043. doi:10.1016/j.taap.2022.116043
Agrawal, S., and Khazaeni, B. (2022). Acetaminophen toxicity. St. Petersburg, Florida: StatPearls Publishing. Statpearls [internet].
Ahmad, A., and Ahmad, R. (2014). Resveratrol mitigate structural changes and hepatic stellate cell activation in N'-nitrosodimethylamine-induced liver fibrosis via restraining oxidative damage. Chem. Biol. Interact. 221, 1–12. doi:10.1016/j.cbi.2014.07.007
Akakpo, J. Y., Ramachandran, A., Curry, S. C., Rumack, B. H., and Jaeschke, H. (2022). Comparing N-acetylcysteine and 4-methylpyrazole as antidotes for acetaminophen overdose. Arch. Toxicol. 96, 453–465. doi:10.1007/s00204-021-03211-z
Akakpo, J. Y., Ramachandran, A., Duan, L., Schaich, M. A., Jaeschke, M. W., Freudenthal, B. D., et al. (2019). Delayed treatment with 4-methylpyrazole protects against acetaminophen hepatotoxicity in mice by inhibition of c-jun n-terminal kinase. Toxicol. Sci. 170, 57–68. doi:10.1093/toxsci/kfz077
Akakpo, J. Y., Ramachandran, A., Kandel, S. E., Ni, H. M., Kumer, S. C., Rumack, B. H., et al. (2018). 4-Methylpyrazole protects against acetaminophen hepatotoxicity in mice and in primary human hepatocytes. Hum. Exp. Toxicol. 37, 1310–1322. doi:10.1177/0960327118774902
Andrade, R. J., Aithal, G. P., Björnsson, E. S., Kaplowitz, N., Kullak-Ublick, G. A., Larrey, D., et al. (2019). EASL clinical practice guidelines: Drug-induced liver injury. J. hepatology 70, 1222–1261. doi:10.1016/j.jhep.2019.02.014
Appiah-Opong, R., Commandeur, J. N., Van Vugt-Lussenburg, B., and Vermeulen, N. P. (2007). Inhibition of human recombinant cytochrome P450s by curcumin and curcumin decomposition products. Toxicology 235, 83–91. doi:10.1016/j.tox.2007.03.007
Athersuch, T. J., Antoine, D. J., Boobis, A. R., Coen, M., Daly, A. K., Possamai, L., et al. (2018). Paracetamol metabolism, hepatotoxicity, biomarkers and therapeutic interventions: A perspective. Toxicol. Res. (Camb) 7, 347–357. doi:10.1039/c7tx00340d
Awasthi, Y. C., Ansari, G. A., and Awasthi, S. (2005). Regulation of 4-hydroxynonenal mediated signaling by glutathione S-transferases. Methods Enzymol. 401, 379–407. doi:10.1016/S0076-6879(05)01024-4
Awasthi, Y. C., Sharma, R., Sharma, A., Yadav, S., Singhal, S. S., Chaudhary, P., et al. (2008). Self-regulatory role of 4-hydroxynonenal in signaling for stress-induced programmed cell death. Free Radic. Biol. Med. 45, 111–118. doi:10.1016/j.freeradbiomed.2008.04.007
Bhadauria, M. (2010). Dose-dependent hepatoprotective effect of emodin against acetaminophen-induced acute damage in rats. Exp. Toxicol. Pathol. 62, 627–635. doi:10.1016/j.etp.2009.08.006
Bulku, E., Stohs, S. J., Cicero, L., Brooks, T., Halley, H., and Ray, S. D. (2012). Curcumin exposure modulates multiple pro-apoptotic and anti-apoptotic signaling pathways to antagonize acetaminophen-induced toxicity. Curr. Neurovasc Res. 9, 58–71. doi:10.2174/156720212799297083
Cerný, D., Canová, N. K., Martínek, J., Horínek, A., Kmonícková, E., Zídek, Z., et al. (2009). Effects of resveratrol pretreatment on tert-butylhydroperoxide induced hepatocyte toxicity in immobilized perifused hepatocytes: Involvement of inducible nitric oxide synthase and hemoxygenase-1. Nitric Oxide 20, 1–8. doi:10.1016/j.niox.2008.08.006
Chaudhary, P., Sharma, R., Sharma, A., Vatsyayan, R., Yadav, S., Singhal, S. S., et al. (2010). Mechanisms of 4-hydroxy-2-nonenal induced pro- and anti-apoptotic signaling. Biochemistry 49, 6263–6275. doi:10.1021/bi100517x
Chen, W., Koenigs, L. L., Thompson, S. J., Peter, R. M., Rettie, A. E., Trager, W. F., et al. (1998). Oxidation of acetaminophen to its toxic quinone imine and nontoxic catechol metabolites by baculovirus-expressed and purified human cytochromes P450 2E1 and 2A6. Chem. Res. Toxicol. 11, 295–301. doi:10.1021/tx9701687
Cheng, J. Z., Sharma, R., Yang, Y., Singhal, S. S., Sharma, A., Saini, M. K., et al. (2001). Accelerated metabolism and exclusion of 4-hydroxynonenal through induction of RLIP76 and hGST5.8 is an early adaptive response of cells to heat and oxidative stress. J. Biol. Chem. 276, 41213–41223. doi:10.1074/jbc.M106838200
Dalaklioglu, S., Genc, G. E., Aksoy, N. H., Akcit, F., and Gumuslu, S. (2013). Resveratrol ameliorates methotrexate-induced hepatotoxicity in rats via inhibition of lipid peroxidation. Hum. Exp. Toxicol. 32, 662–671. doi:10.1177/0960327112468178
Darvin, S. S., Esakkimuthu, S., Toppo, E., Balakrishna, K., Paulraj, M. G., Pandikumar, P., et al. (2018). Hepatoprotective effect of lawsone on rifampicin-isoniazid induced hepatotoxicity in in vitro and in vivo models. Environ. Toxicol. Pharmacol. 61, 87–94. doi:10.1016/j.etap.2018.05.006
Das, A. (2011). Heat stress-induced hepatotoxicity and its prevention by resveratrol in rats. Toxicol. Mech. Methods 21, 393–399. doi:10.3109/15376516.2010.550016
Dokumacioglu, E., İskender, H., Aktas, M., Hanedan, B., Dokumacioglu, A., Sen, T., et al. (2017). The effect of sulforaphane on oxidative stress and inflammation in rats with toxic hepatitis induced by acetaminophene. Bratisl. Lek. Listy 118, 453–459. doi:10.4149/BLL_2017_088
Du, K., Mcgill, M. R., Xie, Y., Bajt, M. L., and Jaeschke, H. (2015). Resveratrol prevents protein nitration and release of endonucleases from mitochondria during acetaminophen hepatotoxicity. Food Chem. Toxicol. 81, 62–70. doi:10.1016/j.fct.2015.04.014
Du, K., Ramachandran, A., and Jaeschke, H. (2016). Oxidative stress during acetaminophen hepatotoxicity: Sources, pathophysiological role and therapeutic potential. Redox Biol. 10, 148–156. doi:10.1016/j.redox.2016.10.001
El-Hassan, H., Anwar, K., Macanas-Pirard, P., Crabtree, M., Chow, S. C., Johnson, V. L., et al. (2003). Involvement of mitochondria in acetaminophen-induced apoptosis and hepatic injury: Roles of cytochrome c, Bax, bid, and caspases. Toxicol. Appl. Pharmacol. 191, 118–129. doi:10.1016/s0041-008x(03)00240-0
Elsayed Elgarawany, G., Abdou, A. G., Maher Taie, D., and Motawea, S. M. (2020). Hepatoprotective effect of artichoke leaf extracts in comparison with silymarin on acetaminophen-induced hepatotoxicity in mice. J. Immunoass. Immunochem. 41, 84–96. doi:10.1080/15321819.2019.1692029
Fu, J., Huang, H., Liu, J., Pi, R., Chen, J., and Liu, P. (2007). Tanshinone IIA protects cardiac myocytes against oxidative stress-triggered damage and apoptosis. Eur. J. Pharmacol. 568, 213–221. doi:10.1016/j.ejphar.2007.04.031
Gadacha, W., Ben-Attia, M., Bonnefont-Rousselot, D., Aouani, E., Ghanem-Boughanmi, N., and Touitou, Y. (2009). Resveratrol opposite effects on rat tissue lipoperoxidation: Pro-oxidant during day-time and antioxidant at night. Redox Rep. 14, 154–158. doi:10.1179/135100009X466131
Gao, H. Y., Li, G. Y., Lou, M. M., Li, X. Y., Wei, X. Y., and Wang, J. H. (2012). Hepatoprotective effect of matrine salvianolic acid B salt on carbon tetrachloride-induced hepatic fibrosis. J. Inflamm. (Lond) 9, 16. doi:10.1186/1476-9255-9-16
Granados-Castro, L. F., Rodríguez-Rangel, D. S., Fernández-Rojas, B., León-Contreras, J. C., Hernández-Pando, R., Medina-Campos, O. N., et al. (2016). Curcumin prevents paracetamol-induced liver mitochondrial alterations. J. Pharm. Pharmacol. 68, 245–256. doi:10.1111/jphp.12501
Guengerich, F. P. (2020). Cytochrome P450 2E1 and its roles in disease. Chem. Biol. Interact. 322, 109056. doi:10.1016/j.cbi.2020.109056
Gum, S. I., and Cho, M. K. (2013b). Korean red ginseng extract prevents APAP-induced hepatotoxicity through metabolic enzyme regulation: The role of ginsenoside Rg3, a protopanaxadiol. Liver Int. 33, 1071–1084. doi:10.1111/liv.12046
Gum, S. I., and Cho, M. K. (2013a). The amelioration of N-acetyl-p-benzoquinone imine toxicity by ginsenoside Rg3: The role of nrf2-mediated detoxification and mrp1/mrp3 transports. Oxid. Med. Cell Longev. 2013, 957947. doi:10.1155/2013/957947
Howitz, K. T., Bitterman, K. J., Cohen, H. Y., Lamming, D. W., Lavu, S., Wood, J. G., et al. (2003). Small molecule activators of sirtuins extend Saccharomyces cerevisiae lifespan. Nature 425, 191–196. doi:10.1038/nature01960
Hu, B., Li, J., Gong, D., Dai, Y., Wang, P., Wan, L., et al. (2022). Long-term consumption of food-derived chlorogenic acid protects mice against acetaminophen-induced hepatotoxicity via promoting PINK1-dependent mitophagy and inhibiting apoptosis. Toxics 10, 665. doi:10.3390/toxics10110665
Hu, F., Guo, Q., Wei, M., Huang, Z., Shi, L., Sheng, Y., et al. (2020). Chlorogenic acid alleviates acetaminophen-induced liver injury in mice via regulating Nrf2-mediated HSP60-initiated liver inflammation. Eur. J. Pharmacol. 883, 173286. doi:10.1016/j.ejphar.2020.173286
Hu, R., Hebbar, V., Kim, B.-R., Chen, C., Winnik, B., Buckley, B., et al. (2004). In vivo pharmacokinetics and regulation of gene expression profiles by isothiocyanate sulforaphane in the rat. J. Pharmacol. Exp. Ther. 310, 263–271. doi:10.1124/jpet.103.064261
Ihedioha, J. I., Anyogu, D. C., and Ogbonna, M. E. (2020). The effects of silymarin on acetaminophen-induced acute hepatic and renal toxicities in domestic pigeons (Columba livia). J. Avian Med. Surg. 34, 348–357. doi:10.1647/1082-6742-34.4.348
Ilyas, U., Katare, D. P., Aeri, V., and Naseef, P. P. (2016). A review on hepatoprotective and immunomodulatory herbal plants. Pharmacogn. Rev. 10, 66–70. doi:10.4103/0973-7847.176544
Iorga, A., and Dara, L. (2019). Cell death in drug-induced liver injury. Adv. Pharmacol. 85, 31–74. doi:10.1016/bs.apha.2019.01.006
Iorga, A., Dara, L., and Kaplowitz, N. (2017). Drug-induced liver injury: Cascade of events leading to cell death, apoptosis or necrosis. Int. J. Mol. Sci. 18, 1018. doi:10.3390/ijms18051018
Jaeschke, H., Adelusi, O. B., and Ramachandran, A. (2021). Ferroptosis and acetaminophen hepatotoxicity: Are we going down another rabbit hole? Gene Expr. 20, 169–178. doi:10.3727/105221621X16104581979144
Jaeschke, H., Ramachandran, A., Chao, X., and Ding, W. X. (2019). Emerging and established modes of cell death during acetaminophen-induced liver injury. Arch. Toxicol. 93, 3491–3502. doi:10.1007/s00204-019-02597-1
Jasani, B., Weisz, D. E., and Mcnamara, P. J. (2018). Evidence-based use of acetaminophen for hemodynamically significant ductus arteriosus in preterm infants. Semin. Perinatol. 42, 243–252. doi:10.1053/j.semperi.2018.05.007
Ji, L., Jiang, P., Lu, B., Sheng, Y., Wang, X., and Wang, Z. (2013). Chlorogenic acid, a dietary polyphenol, protects acetaminophen-induced liver injury and its mechanism. J. Nutr. Biochem. 24, 1911–1919. doi:10.1016/j.jnutbio.2013.05.007
Kaiser, S. K., and Dart, R. C. (2022). The roles of antidotes in emergency situations. Emerg. Med. Clin. North Am. 40, 381–394. doi:10.1016/j.emc.2022.01.008
Kalsi, S. S., Wood, D. M., Waring, W. S., and Dargan, P. I. (2011). Does cytochrome P450 liver isoenzyme induction increase the risk of liver toxicity after paracetamol overdose? Open Access Emerg. Med. 3, 69–76. doi:10.2147/OAEM.S24962
Kang, A. M., Padilla-Jones, A., Fisher, E. S., Akakpo, J. Y., Jaeschke, H., Rumack, B. H., et al. (2020). The effect of 4-methylpyrazole on oxidative metabolism of acetaminophen in human volunteers. J. Med. Toxicol. 16, 169–176. doi:10.1007/s13181-019-00740-z
Khan, H., Ullah, H., and Nabavi, S. M. (2019). Mechanistic insights of hepatoprotective effects of curcumin: Therapeutic updates and future prospects. Food Chem. Toxicol. 124, 182–191. doi:10.1016/j.fct.2018.12.002
Kim, D. H., Jung, Y. J., Lee, J. E., Lee, A. S., Kang, K. P., Lee, S., et al. (2011). SIRT1 activation by resveratrol ameliorates cisplatin-induced renal injury through deacetylation of p53. Am. J. Physiol. Ren. Physiol. 301, F427–F435. doi:10.1152/ajprenal.00258.2010
Kim, Y.-S., Hwang, J.-W., Sung, S.-H., Jeon, Y.-J., Jeong, J.-H., Jeon, B.-T., et al. (2015). Antioxidant activity and protective effect of extract of Celosia cristata L. flower on tert-butyl hydroperoxide-induced oxidative hepatotoxicity. Food Chem. 168, 572–579. doi:10.1016/j.foodchem.2014.07.106
Krajka-Kuźniak, V., and Baer-Dubowska, W. (2003). The effects of tannic acid on cytochrome P450 and phase II enzymes in mouse liver and kidney. Toxicol. Lett. 143, 209–216. doi:10.1016/s0378-4274(03)00177-2
Krupa, P., Svobodova, B., Dubisova, J., Kubinova, S., Jendelova, P., and Machova Urdzikova, L. (2019). Nano-formulated curcumin (Lipodisq™) modulates the local inflammatory response, reduces glial scar and preserves the white matter after spinal cord injury in rats. Neuropharmacology 155, 54–64. doi:10.1016/j.neuropharm.2019.05.018
Lau, A., Villeneuve, N. F., Sun, Z., Wong, P. K., and Zhang, D. D. J. P. R. (2008). Dual roles of Nrf2 in cancer. Pharmacol. Res. 58, 262–270. doi:10.1016/j.phrs.2008.09.003
Lee, W. M. (2008). Acetaminophen-related acute liver failure in the United States. Hepatology Res. official J. Jpn. Soc. Hep atology 38, S3–S8. doi:10.1111/j.1872-034X.2008.00419.x
Li, G., Chen, J. B., Wang, C., Xu, Z., Nie, H., Qin, X. Y., et al. (2013). Curcumin protects against acetaminophen-induced apoptosis in hepatic injury. World J. Gastroenterol. 19, 7440–7446. doi:10.3748/wjg.v19.i42.7440
Li, J. P., Gao, Y., Chu, S. F., Zhang, Z., Xia, C. Y., Mou, Z., et al. (2014). Nrf2 pathway activation contributes to anti-fibrosis effects of ginsenoside Rg1 in a rat model of alcohol- and CCl4-induced hepatic fibrosis. Acta Pharmacol. Sin. 35, 1031–1044. doi:10.1038/aps.2014.41
Lin, M., Zhai, X., Wang, G., Tian, X., Gao, D., Shi, L., et al. (2015). Salvianolic acid B protects against acetaminophen hepatotoxicity by inducing Nrf2 and phase II detoxification gene expression via activation of the PI3K and PKC signaling pathways. J. Pharmacol. Sci. 127, 203–210. doi:10.1016/j.jphs.2014.12.010
Liu, T., Yang, L., Gao, H., Zhuo, Y., Tu, Z., Wang, Y., et al. (2022). 3,4-dihydroxyphenylethyl alcohol glycoside reduces acetaminophen-induced acute liver failure in mice by inhibiting hepatocyte ferroptosis and pyroptosis. PeerJ 10, e13082. doi:10.7717/peerj.13082
Liu, W., Xu, Z., Li, H., Guo, M., Yang, T., Feng, S., et al. (2017). Protective effects of curcumin against mercury-induced hepatic injuries in rats, involvement of oxidative stress antagonism, and Nrf2-ARE pathway activation. Hum. Exp. Toxicol. 36, 949–966. doi:10.1177/0960327116677355
Lu, C., Zhang, F., Xu, W., Wu, X., Lian, N., Jin, H., et al. (2015). Curcumin attenuates ethanol-induced hepatic steatosis through modulating Nrf2/FXR signaling in hepatocytes. IUBMB Life 67, 645–658. doi:10.1002/iub.1409
Marto, N., Morello, J., Antunes, A. M. M., Azeredo, S., Monteiro, E. C., and Pereira, S. A. (2021). A simple method to measure sulfonation in man using paracetamol as probe drug. Sci. Rep. 11, 9036. doi:10.1038/s41598-021-88393-3
Mccarty, M. F. (2001). Inhibition of CYP2E1 with natural agents may be a feasible strategy for minimizing the hepatotoxicity of ethanol. Med. Hypotheses 56, 8–11. doi:10.1054/mehy.1999.1015
Mccracken, K. (2015). James Logan Prize Essay. The challenges of cancer pain assessment. Ulst. Med. J. 84, 55–57.
Mcgill, M. R., Du, K., Weemhoff, J. L., and Jaeschke, H. (2015). Critical review of resveratrol in xenobiotic-induced hepatotoxicity. Food Chem. Toxicol. 86, 309–318. doi:10.1016/j.fct.2015.11.003
Mcgill, M. R., Sharpe, M. R., Williams, C. D., Taha, M., Curry, S. C., and Jaeschke, H. (2012). The mechanism underlying acetaminophen-induced hepatotoxicity in humans and mice involves mitochondrial damage and nuclear DNA fragmentation. J. Clin. Invest. 122, 1574–1583. doi:10.1172/JCI59755
Meng, X., An, X., Zhou, L., Fu, B., and Jia, L. (2022). The isomers, aloe-emodin and emodin, possess differential inhibitory activities against CYP1B1 enzyme. Steroids 185, 109055. doi:10.1016/j.steroids.2022.109055
More, S. S., Nugent, J., Vartak, A. P., Nye, S. M., and Vince, R. (2017). Hepatoprotective effect of ψ-glutathione in a murine model of acetaminophen-induced liver toxicity. Chem. Res. Toxicol. 30, 777–784. doi:10.1021/acs.chemrestox.6b00291
Nazmy, E. A., El-Khouly, O. A., Atef, H., and Said, E. (2017). Sulforaphane protects against sodium valproate-induced acute liver injury. Can. J. Physiol. Pharmacol. 95, 420–426. doi:10.1139/cjpp-2016-0447
Noh, J.-R., Kim, Y.-H., Hwang, J. H., Choi, D.-H., Kim, K.-S., Oh, W.-K., et al. (2015). Sulforaphane protects against acetaminophen-induced hepatotoxicity. Food Chem. 80, 193–200. doi:10.1016/j.fct.2015.03.020
Ooi, J. P., Kuroyanagi, M., Sulaiman, S. F., Muhammad, T. S., and Tan, M. L. (2011). Andrographolide and 14-deoxy-11, 12-didehydroandrographolide inhibit cytochrome P450s in HepG2 hepatoma cells. Life Sci. 88, 447–454. doi:10.1016/j.lfs.2010.12.019
Pang, C., Sheng, Y. C., Jiang, P., Wei, H., and Ji, L. L. (2015). Chlorogenic acid prevents acetaminophen-induced liver injury: The involvement of CYP450 metabolic enzymes and some antioxidant signals. J. Zhejiang Univ. Sci. B 16, 602–610. doi:10.1631/jzus.B1400346
Pang, C., Zheng, Z., Shi, L., Sheng, Y., Wei, H., Wang, Z., et al. (2016). Caffeic acid prevents acetaminophen-induced liver injury by activating the Keap1-Nrf2 antioxidative defense system. Free Radic. Biol. Med. 91, 236–246. doi:10.1016/j.freeradbiomed.2015.12.024
Papackova, Z., Heczkova, M., Dankova, H., Sticova, E., Lodererova, A., Bartonova, L., et al. (2018). Silymarin prevents acetaminophen-induced hepatotoxicity in mice. PLoS One 13, e0191353. doi:10.1371/journal.pone.0191353
Park, H. M., Kim, S. J., Mun, A. R., Go, H. K., Kim, G. B., Kim, S. Z., et al. (2012). Korean red ginseng and its primary ginsenosides inhibit ethanol-induced oxidative injury by suppression of the MAPK pathway in TIB-73 cells. J. Ethnopharmacol. 141, 1071–1076. doi:10.1016/j.jep.2012.03.038
Piver, B., Berthou, F., Dreano, Y., and Lucas, D. (2001). Inhibition of CYP3A, CYP1A and CYP2E1 activities by resveratrol and other non volatile red wine components. Toxicol. Lett. 125, 83–91. doi:10.1016/s0378-4274(01)00418-0
Polyak, S. J., Morishima, C., Lohmann, V., Pal, S., Lee, D. Y., Liu, Y., et al. (2010). Identification of hepatoprotective flavonolignans from silymarin. Proc. Natl. Acad. Sci. U. S. A. 107, 5995–5999. doi:10.1073/pnas.0914009107
Rajaram, P., and Subramanian, R. (2018). Management of acute liver failure in the intensive care unit setting. Clin. Liver Dis. 22, 403–408. doi:10.1016/j.cld.2018.01.013
Ramachandran, A., Visschers, R. G. J., Duan, L., Akakpo, J. Y., and Jaeschke, H. (2018). Mitochondrial dysfunction as a mechanism of drug-induced hepatotoxicity: Current understanding and future perspectives. J. Clin. Transl. Res. 4, 75–100. doi:10.18053/jctres.04.201801.005
Ray, S. D., Mumaw, V. R., Raje, R. R., and Fariss, M. W. (1996). Protection of acetaminophen-induced hepatocellular apoptosis and necrosis by cholesteryl hemisuccinate pretreatment. J. Pharmacol. Exp. Ther. 279, 1470–1483.
Rizvi, F., Mathur, A., and Kakkar, P. (2015a). Morin mitigates acetaminophen-induced liver injury by potentiating Nrf2 regulated survival mechanism through molecular intervention in PHLPP2-Akt-Gsk3β axis. Apoptosis 20, 1296–1306. doi:10.1007/s10495-015-1160-y
Rizvi, F., Mathur, A., Krishna, S., Siddiqi, M. I., and Kakkar, P. (2015b). Suppression in PHLPP2 induction by morin promotes Nrf2-regulated cellular defenses against oxidative injury to primary rat hepatocytes. Redox Biol. 6, 587–598. doi:10.1016/j.redox.2015.10.002
Rousta, A. M., Mirahmadi, S. M., Shahmohammadi, A., Mehrabi, Z., Fallah, S., Baluchnejadmojarad, T., et al. (2022). Therapeutic potential of isorhamnetin following acetaminophen-induced hepatotoxicity through targeting NLRP3/NF-κB/Nrf2. Drug Res. (Stuttg) 72, 245–254. doi:10.1055/a-1792-2678
Rubiolo, J. A., Mithieux, G., and Vega, F. V. (2008). Resveratrol protects primary rat hepatocytes against oxidative stress damage: Activation of the Nrf2 transcription factor and augmented activities of antioxidant enzymes. Eur. J. Pharmacol. 591, 66–72. doi:10.1016/j.ejphar.2008.06.067
Sasaki, T., Sato, Y., Kumagai, T., Yoshinari, K., and Nagata, K. (2017). Effect of health foods on cytochrome P450-mediated drug metabolism. J. Pharm. Health Care Sci. 3, 14. doi:10.1186/s40780-017-0083-x
Schmidt, R. H. (1984). Sulforaphane prevents acetaminophen-induced hepatic injury in mice. Electronic Theses and Dissertations. .
Shao, S. Y., Yang, Y. N., Feng, Z. M., Jiang, J. S., and Zhang, P. C. (2017). New iridoid glycosides from the fruits of Forsythia suspensa and their hepatoprotective activities. Bioorg Chem. 75, 303–309. doi:10.1016/j.bioorg.2017.10.006
Sharifi-Rigi, A., Heidarian, E., and Amini, S. A. (2019). Protective and anti-inflammatory effects of hydroalcoholic leaf extract of Origanum vulgare on oxidative stress, TNF-α gene expression and liver histological changes in paraquat-induced hepatotoxicity in rats. Archives Physiology Biochem. 125, 56–63. doi:10.1080/13813455.2018.1437186
Sharma, R., Brown, D., Awasthi, S., Yang, Y., Sharma, A., Patrick, B., et al. (2004). Transfection with 4-hydroxynonenal-metabolizing glutathione S-transferase isozymes leads to phenotypic transformation and immortalization of adherent cells. Eur. J. Biochem. 271, 1690–1701. doi:10.1111/j.1432-1033.2004.04067.x
Sharma, R., Sharma, A., Chaudhary, P., Pearce, V., Vatsyayan, R., Singh, S. V., et al. (2010). Role of lipid peroxidation in cellular responses to D,L-sulforaphane, a promising cancer chemopreventive agent. Biochemistry 49, 3191–3202. doi:10.1021/bi100104e
Sharma, R., Sharma, A., Chaudhary, P., Vatsyayan, R., Pearce, V., Jeyabal, P. V., et al. (2008a). 4-Hydroxynonenal induces p53-mediated apoptosis in retinal pigment epithelial cells. Arch. Biochem. Biophys. 480, 85–94. doi:10.1016/j.abb.2008.09.016
Sharma, R., Sharma, A., Dwivedi, S., Zimniak, P., Awasthi, S., and Awasthi, Y. C. (2008b). 4-Hydroxynonenal self-limits fas-mediated DISC-independent apoptosis by promoting export of Daxx from the nucleus to the cytosol and its binding to Fas. Biochemistry 47, 143–156. doi:10.1021/bi701559f
Shen, P., Han, L., Chen, G., Cheng, Z., and Liu, Q. (2022). Emodin attenuates acetaminophen-induced hepatotoxicity via the cGAS-STING pathway. Inflammation 45, 74–87. doi:10.1007/s10753-021-01529-5
Shi, P., Huang, Z., and Chen, G. (2008). Rhein induces apoptosis and cell cycle arrest in human hepatocellular carcinoma BEL-7402 cells. Am. J. Chin. Med. 36, 805–813. doi:10.1142/S0192415X08006259
Shishodia, S., Sethi, G., and Aggarwal, B. B. (2005). Curcumin: Getting back to the roots. Ann. N. Y. Acad. Sci. 1056, 206–217. doi:10.1196/annals.1352.010
Somanawat, K., Thong-Ngam, D., and Klaikeaw, N. (2013). Curcumin attenuated paracetamol overdose induced hepatitis. World J. Gastroenterol. 19, 1962–1967. doi:10.3748/wjg.v19.i12.1962
Tang, J. C., Yang, H., Song, X. Y., Song, X. H., Yan, S. L., Shao, J. Q., et al. (2009). Inhibition of cytochrome P450 enzymes by rhein in rat liver microsomes. Phytother. Res. 23, 159–164. doi:10.1002/ptr.2572
Truong, V. L., Bak, M. J., Jun, M., Kong, A. N., Ho, C. T., and Jeong, W. S. (2014). Antioxidant defense and hepatoprotection by procyanidins from almond (Prunus amygdalus) skins. J. Agric. Food Chem. 62, 8668–8678. doi:10.1021/jf5027247
Vanduchova, A., Tomankova, V., Anzenbacher, P., and Anzenbacherova, E. (2016). Influence of sulforaphane metabolites on activities of human drug-metabolizing cytochrome P450 and determination of sulforaphane in human liver cells. J. Med. Food 19, 1141–1146. doi:10.1089/jmf.2016.0063
Vatsyayan, R., Chaudhary, P., Sharma, A., Sharma, R., Rao Lelsani, P. C., Awasthi, S., et al. (2011). Role of 4-hydroxynonenal in epidermal growth factor receptor-mediated signaling in retinal pigment epithelial cells. Exp. Eye Res. 92, 147–154. doi:10.1016/j.exer.2010.11.010
Vuong, L. D., Nguyen, Q. N., and Truong, V. L. (2019). Anti-inflammatory and anti-oxidant effects of combination between sulforaphane and acetaminophen in LPS-stimulated RAW 264.7 macrophage cells. Immunopharmacol. Immunotoxicol. 41, 413–419. doi:10.1080/08923973.2019.1569049
Wang, J., Luo, W., Li, B., Lv, J., Ke, X., Ge, D., et al. (2018). Sagittaria sagittifolia polysaccharide protects against isoniazid- and rifampicin-induced hepatic injury via activation of nuclear factor E2-related factor 2 signaling in mice. J. Ethnopharmacol. 227, 237–245. doi:10.1016/j.jep.2018.09.002
Wang, Q. L., Wu, Q., Tao, Y. Y., Liu, C. H., and El-Nezami, H. (2011). Salvianolic acid B modulates the expression of drug-metabolizing enzymes in HepG2 cells. Hepatobiliary Pancreat. Dis. Int. 10, 502–508. doi:10.1016/s1499-3872(11)60085-4
Wang, W., Guan, C., Sun, X., Zhao, Z., Li, J., Fu, X., et al. (2016). Tanshinone IIA protects against acetaminophen-induced hepatotoxicity via activating the Nrf2 pathway. Phytomedicine 23, 589–596. doi:10.1016/j.phymed.2016.02.022
Wang, X., Cheung, C. M., Lee, W. Y., Or, P. M., and Yeung, J. H. (2010). Major tanshinones of Danshen (Salvia miltiorrhiza) exhibit different modes of inhibition on human CYP1A2, CYP2C9, CYP2E1 and CYP3A4 activities in vitro. Phytomedicine 17, 868–875. doi:10.1016/j.phymed.2010.05.003
Wang, Y., Jiang, Y., Fan, X., Tan, H., Zeng, H., Wang, Y., et al. (2015). Hepato-protective effect of resveratrol against acetaminophen-induced liver injury is associated with inhibition of CYP-mediated bioactivation and regulation of SIRT1-p53 signaling pathways. Toxicol. Lett. 236, 82–89. doi:10.1016/j.toxlet.2015.05.001
Wang, Y., Zhao, Y., Wang, Z., Sun, R., Zou, B., Li, R., et al. (2021). Peroxiredoxin 3 inhibits acetaminophen-induced liver pyroptosis through the regulation of mitochondrial ROS. Front. Immunol. 12, 652782. doi:10.3389/fimmu.2021.652782
Wei, M., Zheng, Z., Shi, L., Jin, Y., and Ji, L. (2018). Natural polyphenol chlorogenic acid protects against acetaminophen-induced hepatotoxicity by activating ERK/Nrf2 antioxidative pathway. Toxicol. Sci. 162, 99–112. doi:10.1093/toxsci/kfx230
Wong, Y. T., Gruber, J., Jenner, A. M., Ng, M. P., Ruan, R., and Tay, F. E. (2009). Elevation of oxidative-damage biomarkers during aging in F2 hybrid mice: Protection by chronic oral intake of resveratrol. Free Radic. Biol. Med. 46, 799–809. doi:10.1016/j.freeradbiomed.2008.12.016
Xu, G. B., Xiao, Y. H., Zhang, Q. Y., Zhou, M., and Liao, S. G. (2018). Hepatoprotective natural triterpenoids. Eur. J. Med. Chem. 145, 691–716. doi:10.1016/j.ejmech.2018.01.011
Yamada, N., Karasawa, T., Kimura, H., Watanabe, S., Komada, T., Kamata, R., et al. (2020). Ferroptosis driven by radical oxidation of n-6 polyunsaturated fatty acids mediates acetaminophen-induced acute liver failure. Cell Death Dis. 11, 144. doi:10.1038/s41419-020-2334-2
Yan, H., Huang, Z., Bai, Q., Sheng, Y., Hao, Z., Wang, Z., et al. (2018). Natural product andrographolide alleviated APAP-induced liver fibrosis by activating Nrf2 antioxidant pathway. Toxicology 396-397, 1–12. doi:10.1016/j.tox.2018.01.007
Yang, C. H., Yen, T. L., Hsu, C. Y., Thomas, P. A., Sheu, J. R., and Jayakumar, T. (2017). Multi-targeting andrographolide, a novel NF-κB inhibitor, as a potential therapeutic agent for stroke. Int. J. Mol. Sci. 18, 1638. doi:10.3390/ijms18081638
Yang, T., Liang, Z., Wen, C., Jiang, X., and Wang, L. (2022b). Silymarin protects against acute liver injury induced by acetaminophen by downregulating the expression and activity of the CYP2E1 enzyme. Molecules 27, 8855. doi:10.3390/molecules27248855
Yang, T., Wang, H., Wang, X., Li, J., and Jiang, L. (2022a). The dual role of innate immune response in acetaminophen-induced liver injury. Biol. (Basel) 11, 1057. doi:10.3390/biology11071057
Yang, Y., Cheng, J. Z., Singhal, S. S., Saini, M., Pandya, U., Awasthi, S., et al. (2001). Role of glutathione S-transferases in protection against lipid peroxidation. Overexpression of hGSTA2-2 in K562 cells protects against hydrogen peroxide-induced apoptosis and inhibits JNK and caspase 3 activation. J. Biol. Chem. 276, 19220–19230. doi:10.1074/jbc.M100551200
Yang, Y., Sharma, R., Zimniak, P., and Awasthi, Y. C. (2002). Role of alpha class glutathione S-transferases as antioxidant enzymes in rodent tissues. Toxicol. Appl. Pharmacol. 182, 105–115. doi:10.1006/taap.2002.9450
Yoon, E., Babar, A., Choudhary, M., Kutner, M., and Pyrsopoulos, N. (2016). Acetaminophen-induced hepatotoxicity: A comprehensive update. J. Clin. Transl. Hepatol. 4, 131–142. doi:10.14218/JCTH.2015.00052
Zhang, J., Song, Q., Han, X., Zhang, Y., Zhang, Y., Zhang, X., et al. (2017). Multi-targeted protection of acetaminophen-induced hepatotoxicity in mice by tannic acid. Int. Immunopharmacol. 47, 95–105. doi:10.1016/j.intimp.2017.03.027
Zhao, Y. L., Zhou, G. D., Yang, H. B., Wang, J. B., Shan, L. M., Li, R. S., et al. (2011). Rhein protects against acetaminophen-induced hepatic and renal toxicity. Food Chem. Toxicol. 49, 1705–1710. doi:10.1016/j.fct.2011.04.011
Zhou, Y., Fan, X., Jiao, T., Li, W., Chen, P., Jiang, Y., et al. (2021). SIRT6 as a key event linking P53 and NRF2 counteracts APAP-induced hepatotoxicity through inhibiting oxidative stress and promoting hepatocyte proliferation. Acta Pharm. Sin. B 11, 89–99. doi:10.1016/j.apsb.2020.06.016
Zhu, P., Li, J., Fu, X., and Yu, Z. (2019). Schisandra fruits for the management of drug-induced liver injury in China: A review. Phytomedicine 59, 152760. doi:10.1016/j.phymed.2018.11.020
APAP Acetaminophen
ALF acute liver failure
NAPQI N-acetyl-p-benzoquinoneimide
NAC N-acetylcysteine
AILI APAP-induced liver injury
COX cyclooxygenase
GPX glutathione peroxidase
CYP450 cytochrome P450 system
ROS reactive oxygen species
HO-1 heme oxygenase 1
iNOS inducible nitric oxide synthase
CDK4 cyclin-dependent kinase 4
SIRT1 Sirtuin 1
TNF-α tumor necrosis factor-α
AIF apoptosis-inducing factor
PI3K phosphoinositide 3-kinase
PKC protein kinase C
ASK1 apoptosis signal-regulated kinase 1
NQO1 NAD(P)H quinone dehydrogenase 1
SOD superoxide dismutase
CAT catalase
GSTA2 glutathione S-transferase α2
PKA protein kinase A
GCLC glutamate-cysteine ligase catalytic subunit
ALT alanine aminotransferase
AST aspartate aminotransferase
CREA creatinine
UREA urea nitrogen
NO nitric oxide
MDA malondialdehyde
GSH glutathione
ALP lkaline phosphatase
ALB albumin
IL-1β interleukin-1β
cGAS cyclic GMP-AMP synthase
STING stimulators of interferon gene
Keywords: acetaminophen, liver injury, natural products, oxidative stress, Nrf2
Citation: Liao J, Lu Q, Li Z, Li J, Zhao Q and Li J (2023) Acetaminophen-induced liver injury: Molecular mechanism and treatments from natural products. Front. Pharmacol. 14:1122632. doi: 10.3389/fphar.2023.1122632
Received: 13 December 2022; Accepted: 13 March 2023;
Published: 27 March 2023.
Edited by:
Enrico Sangiovanni, University of Milan, ItalyReviewed by:
Ayaz Shahid, Western University of Health Sciences, United StatesCopyright © 2023 Liao, Lu, Li, Li, Zhao and Li. This is an open-access article distributed under the terms of the Creative Commons Attribution License (CC BY). The use, distribution or reproduction in other forums is permitted, provided the original author(s) and the copyright owner(s) are credited and that the original publication in this journal is cited, in accordance with accepted academic practice. No use, distribution or reproduction is permitted which does not comply with these terms.
*Correspondence: Qi Zhao, emhhb3FpQGNkdS5lZHUuY24=; Jian Li, bGlqaWFuMDFAY2R1LmVkdS5jbg==
†These authors have contributed equally to this work and share first authorship
Disclaimer: All claims expressed in this article are solely those of the authors and do not necessarily represent those of their affiliated organizations, or those of the publisher, the editors and the reviewers. Any product that may be evaluated in this article or claim that may be made by its manufacturer is not guaranteed or endorsed by the publisher.
Research integrity at Frontiers
Learn more about the work of our research integrity team to safeguard the quality of each article we publish.