- 1Department of Biological Sciences and Biotechnology, Faculty of Sciences, Botswana International University of Science and Technology, Palapye, Botswana
- 2Virology Laboratory, Department of Cell and Systems Biology, University of Toronto, Toronto, ON, Canada
Cancer is the third leading cause of premature death in sub-Saharan Africa. Cervical cancer has the highest number of incidences in sub-Saharan Africa due to high HIV prevalence (70% of global cases) in African countries which is linked to increasing the risk of developing cervical cancer, and the continuous high risk of being infected with Human papillomavirus In 2020, the risk of dying from cancer amongst women was higher in Eastern Africa (11%) than it was in Northern America (7.4%). Plants continue to provide unlimited pharmacological bioactive compounds that are used to manage various illnesses, including cancer. By reviewing the literature, we provide an inventory of African plants with reported anticancer activity and evidence supporting their use in cancer management. In this review, we report 23 plants that have been used for cancer management in Africa, where the anticancer extracts are usually prepared from barks, fruits, leaves, roots, and stems of these plants. Extensive information is reported about the bioactive compounds present in these plants as well as their potential activities against various forms of cancer. However, information on the anticancer properties of other African medicinal plants is insufficient. Therefore, there is a need to isolate and evaluate the anticancer potential of bioactive compounds from other African medicinal plants. Further studies on these plants will allow the elucidation of their anticancer mechanisms of action and allow the identification of phytochemicals that are responsible for their anticancer properties. Overall, this review provides consolidated and extensive information not only on diverse medicinal plants of Africa but on the different types of cancer that these plants are used to manage and the diverse mechanisms and pathways that are involved during cancer alleviation.
1 Introduction
Over the past years, plants have gained momentum in research for the alleviation of major human diseases such as cancer and HIV/AIDS (Nkwe et al., 2021; Makhzoum and Hefferon, 2022). Plants have been used in the production of anti-HIV recombinant proteins to circumvent issues associated with the use of antiretroviral drugs (Tremouillaux-Guiller et al., 2020; Gaobotse et al., 2022a). Cancer, like HIV/AIDS, is a major cause of global morbidity and mortality. The World Health Organization (WHO) reported that cancer is the second leading cause of death globally and was responsible for an estimated 9.6 million deaths in 2018 (WHO, 2020). Approximately 70% of deaths from cancer occur in low and middle-income countries which are mostly in Africa. It is estimated that 13% of global annual deaths are due to lung, colorectal, stomach, liver, and breast cancers (WHO, 2020). It is also estimated that by the year 2030, cancer related deaths will increase to 17 million (Adeloye et al., 2016). According to (WHO, 2020), in Africa, the prevalence of cancer is higher in men than women. More than 19.3 million new cases of cancer were registered worldwide in the year 2020 and these numbers are expected to rise to 28.4 million by the year 2040 (Sung et al., 2021). In the same year of 2020, there were 10 million reported deaths due to different types of cancers across the world. In addition, female breast cancer has now surpassed lung cancer as the most diagnosed cancer in the world (Sung et al., 2021). However, lung cancer remains the leading cause of mortality amongst all the different types of cancer, causing an estimated 1.8 million deaths in the year 2020. This is equivalent to 18% of all cancer related deaths globally. On the other hand, female breast cancer contributed to only 6.9% of all global cancer related deaths in 2022 (Sung et al., 2021). Throughout history, human beings have acquired knowledge on the medicinal uses of plants and have applied this knowledge in folk medicine to treat different diseases (El-Seedi et al., 2013; Ouelbani et al., 2016). It is estimated that 80% of the world population still rely on plant-based material for primary healthcare while traditional medicine usage accounts for 60% of the world population (El-Seedi et al., 2013). Due to low income or long distances from urban treatment centers, many people in Africa commonly use medicinal plants for cancer treatment (Kabbaj et al., 2012; El-Seedi et al., 2013). Moreover, some Africans believe that medicinal plants are more effective than synthetic drugs in managing diseases such as cancer. However, comprehensive compilation of information on these medicinal plants of Africa is insufficient. The documented use of medicinal plants in Africa dates back a couple of centuries, although it might have been there earlier than that (Sawadogo et al., 2012; Ntie-Kang et al., 2013). Cancer remains one of the leading causes of death globally despite advancements in cancer management strategies. Although there have been recent efforts in the production of cancer vaccines in different host plants for different types of cancer (Gaobotse et al., 2022b), there is a need to explore medicinal plants further for cancer management strategies. This review is a compilation of information on diverse medicinal plants of Africa, found in different countries of the continent, that have been used in the management of different types of cancer.
Cancer is the third leading cause of premature death in sub-Saharan Africa, responsible for one in 7 deaths. In 2020, there were 801, 392 new cases of cancer with an estimated 520, 158 deaths in sub-Saharan Africa. In women, the most common types of cancer are breast (129, 400) and cervical cancer (110, 300) which together are responsible for three out of 10 cancer diagnoses. In men, the most common types of cancer are prostate (77, 300), liver (24, 700) and colorectal cancer (23, 000) (Bray et al., 2021; Bray et al., 2022).
The rich flora of Africa results from a vast difference in environmental and climatic conditions such as deserts, savannah, and tropical rain forests. Some of these plants have been used in traditional medicine to treat symptoms of cancer with reports that of all pharmaceutical drugs used, a quarter of them have been derived from plants originally used in traditional medicine (Sawadogo et al., 2012). Treatment of patients across Africa varies from one region to another and this is mostly influenced by the plants, protocols and recipes used. In West Africa, more than half of plants with anticancer metabolites are shrubs and the explants commonly used are the roots and stem barks, with methanol extracts contributing approximately 60% of the used extracts (Sawadogo et al., 2012). (Sawadogo et al., 2012) have shown that phytochemicals with high cytotoxicity towards many cancer cell lines are diterpenes, triterpenes and steroids. Leukaemia, breast, colon, and lung cancer cell lines have shown great sensitivity toward phytochemicals isolated from plants with anticancer activity in West Africa (Usman et al., 2022). Brassica rapa, which is found across almost all regions of Africa, has phytochemicals such as phenanthrene, diarylheptanoids and others with cytotoxic activity towards various human cancer cell lines (Wu et al., 2013). The African cabbage has been used by traditional healers to treat tumors and its extracts have been shown to be cytotoxic to carcinoma cell lines in mice (Bala et al., 2010). Colocasia esculenta, a tropical plant found in most regions of Africa, is said to produce fibers which antagonize the growth of colon cancer cells in rats (Brown et al., 2005) and its aqueous extracts inhibit breast and lung tumors (Kundu et al., 2012).
Twenty-three (23) anticancer plants that are native to Africa will be discussed in this review. These plants are Dicoma anomala, a perennial herb; Fagaropsis which are shrubs or deciduous trees with buttress roots; Tribulus terrestris, which is a small, silky, and hairy herb; Portulaca oleracea, which is an annual succulent plant and Withania somnifera, a small, bushy, evergreen shrub. Other plants that will be discussed are the semi-deciduous tree called Azanza garckeana; Cajanus cajan, which a perennial legume; Combretum caffrum, which is an African bush willow tree; and the flowering cherry plants called Prunus avium and Prunus africana. The review will also look at the tree species Securidaca longipedunculata; the shrub Annona senegalensis; the tropical fruit tree Annona muricata; the shrub plant Aerva javanica and the flowering plant Abelmoschus esculentus. The review will also discuss the dioecies Flueggea virosa; the climbing vine called Lagenaria siceraria; the aromatic evergreen called Xylopia aethiopica; the flowering perennial aquatic Nymphaea lotus as well as the deciduous shrub called Zanthoxylum chalybeum. The Mediterranean evergreen Ceratonia siliqua; the perennial softwood vegetable Moringa oleifera and the perennial herbaceous Peganum harmala will also be discussed in the review.
Amongst these 23 plants, T. terrestris can be consumed in diet. The leaves, fruits, and shoots of this plant can be cooked and consumed. Portulaca oleracea is an edible weed with high nutrition. It also has nutraceutical value that helps in the prevention, treatment, and management of some human diseases (Gunarathne et al., 2022). In India, Withania coagulans is used to ferment milk during cheese production (Ahmad et al., 2017). Azanza garckeana is a valuable source of fruits which are consumed while green or a bit ripe (Ochokwu et al., 2015). Cajanus cajan is a good source of protein and its seeds are cooked and consumed as peas (Talari and Shakappa, 2018). Prunus avium fruits comprise of a fleshy edible mesocarp as well as an edible protective exocarp (Usenik et al., 2015). However, the endocarp of the fruit is inedible. The leaves of S. longipedunculata are cooked and consumed while they are young while the roots of this plant are poisonous. The ripe fruits of A. senegalensis are edible while the flowers of the plant are used for food seasoning (Donhouedé et al., 2022). The fruits of A. muricata are also consumed raw or cooked. Abelmoschus esculentus is an important vegetable crop whose immature fruits can be consumed as vegetables (Gemede et al., 2015). The fruits of F. virosa are only edible when mature. The fruits, seeds, leaves, and oil of L. siceraria are edible (Zahoor et al., 2021). The tubers of N. lotus are consumed raw or roasted (Lim, 2014). The leaves of Z. chalybeum are dried and used as powdered vegetable (Balama et al., 2015).
2 Traditional African medicinal plants used in the management of different cancers
2.1 Dicoma anomala
Dicoma anomala is a perennial herb that is typically called stomach or fever bush. It is a member of the family Asteraceae. It has an erect stem covered with thin hairs as well as an underground tuber (Balogun and Ashafa, 2017). It is native to sub-Saharan Africa, and, in South Africa, it is widely distributed in the Free State, Gauteng, KwaZulu-Natal, Limpopo, Mpumalanga, Northern Cape, and North West provinces. This plant has been used traditionally in Africa to treat diseases such as colds, coughs, fever, ulcers, and diabetes (Balogun and Ashafa, 2017). It has wide ethnomedical uses and its roots are universally used to treat diseases that affect animals and humans. Among the different species of Dicoma, D. anomala, D. zeyheri, D. capensis and D. schinzii have been utilized for their medicinal properties and were duly classified based on their phytochemical composition. Some of these phytochemicals are acetylenic compounds, flavonoids, phenolic acids, phytosterols, sesquiterpenes and triterpenes. They are non-toxic to normal non-cancerous cells. These compounds occur primarily in the leaves and roots and have been used to treat various cancers such as prostate, kidney, ovarian and breast cancers (Greenwell and Rahman, 2015; Maroyi, 2018). D. anomala Sond roots have shown antiproliferative effects against MCF-7 breast cancer cells wherein sesquiterpene conjugated to silver nanoparticles demonstrated anticancer properties by causing oxidative damage in the cancerous cells (Shafiq et al., 2020). Dicoma capensis aqueous extracts have also shown anticancer properties against breast cancer cell lines such as MCF-12A, MDA-MB-231 and MCF-7 (Asita et al., 2015).
2.2 Fagaropsis
The genus Fagaropsis belongs to the family Rutaceae and is widely distributed in Africa. Fagaropsis are mostly shrubs or deciduous trees with buttress roots. The plant species Fagaropsis angolensis is found in Angola, Ethiopia, Kenya, Namibia, Tanzania, Uganda, and Zimbabwe; F. hildebrandtii is primarily found in Ethiopia, Kenya, Somalia, and Tanzania while F. velutina and F. glabra occur endemically in Madagascar (Sun et al., 2021). F. angolensis whole root methanol extracts have been shown to exhibit antitumor activity against throat cancer cells (Hep2) at an IC50 value of 10.05 ± 2.15 μg/mL. Whole root and root-stem methanol extracts showed high degrees of anticancer effects against CT26 colon cancer cells with IC50 values of 8.33 ± 1.42 μg/mL and 5.25 ± 0.35 ug/mL respectively (Yiaile, 2017). F. angolensis stem bark methanolic extracts demonstrated significant activity against DU-145 prostate cancer cells and HCC 1395 breast cancer cells at IC50 values of 12.8 ± 1.1 μg/mL and 53.9 ± 5.6 μg/mL respectively (Misonge et al., 2019). Anticancer effects of methanol extracts of the bark of F. angolensis against HCC 1395 cells were also reported by (Onyancha et al., 2018). Despite its anticancer activities, F. angolensis has shown high levels of toxicity on Vero cells. Therefore, more research is needed to evaluate the precise dosages of the active anticancer ingredients in this plant to mitigate toxicity issues and validate them for their use in folklore medicine.
2.3 Tribulus terrestris
Tribulus terrestris is a small, silky and hairy herb which is indigenous to tropical regions, including Africa, and is a member of the Zygophyllaceae family (Hashim et al., 2014). Methanol extracts of T. terrestris showed strong inhibition against SK-OV-3 ovarian carcinoma cells and MCF-7 breast cancer cells with IC50 values of 89.4 μg/mL and 74.1 μg/mL respectively (Abbas et al., 2022). Tribulus terrestris occurs as a perennial herb and is known for its antineoplastic effects against a wide range of human cancers. The high anticancer potential of T. terrestris has been attributed to its high content of steroidal saponins which have been shown to induce programmed cell death in MCF-7s by eliciting both extrinsic and intrinsic apoptotic pathways (Osbourn, 2003; Sun et al., 2003; Sparg et al., 2004; Kim et al., 2011; Angelova et al., 2013; Faizal and Geelen, 2013). (Patel et al., 2021) reported in silico studies implicating the anticancer properties to active saponin compounds such as nuatigenin saponin that could be essential in the treatment of breast cancer. T. terrestris fruit extracts are capable of inhibiting autophagy in TW2.6 and SAS oral cancer cells and can impact cell proliferation, growth, cell migration and invasion of neoplastic/metastatic cancer cells (Shu et al., 2021).
2.4 Portulaca oleracea
Portulaca oleracea is a common succulent plant that is often referred to as Purslane. It belongs to the genus Portulaca in the family Portulacaceae and is abundant in most regions of Africa such as Botswana (Uddin et al., 2014). The polysaccharides of P. oleracea L., POL-P3b, have shown inhibition of tumors by means of cell cycle arrest, elicitation of DNA damage and induction of apoptosis (Zhao et al., 2013). (Jia et al., 2021) demonstrated that there was a high-level induction of TNF-α, IFN-γ and IL-12 when POL-P3b was co-administered with a dendritic cell vaccine in mouse models, implicating Th1 immune response modulation by POL-P3b. Therefore, POL-P3b serves as an adjuvant that stimulates maturation and augment the antigen presentation capability of the Dendritic Cell (DC) vaccine. Additionally, POL-P3b upregulated the expression levels of MyD88, NF-κB and TLR4 in the DC vaccine and promoted Th1 cytokine secretion. The Ki 67 index is closely associated with the degree of tumor malignancy (Bleckmann et al., 2016; Christensen et al., 2016) and a combination of the DC vaccine and POL-P3b was found to be capable of reducing Ki 67 expression. Also, this combination triggered more significant apoptotic characteristics such as nuclear fragmentation, massive shrinkage of cells and high numbers of TUNEL-positive cells when compared to either the DC vaccine alone or POL-P3b alone. Additionally, higher immunomodulatory properties were observed in immune mice when administered with POL-P3b (Jia et al., 2021). Lymphocyte proliferation elicited by LPS and Con A was augmented, the CD4+/CD8+ ratio increased and the expression of cytokines, including that of TNF-α, IFN-γ, IL-4 and IL-12p70, was also significantly enhanced, suggesting that the POL-P3b adjuvant administered along with the vaccine can upregulate immune response reactions in mice. CD31, CD34 and VEGF expressions were more pronouncedly diminished when a combination of POL-P3b and the DC vaccine was administered and there was a synergistic suppression of angiogenesis. POL-P3b as an adjuvant for the DC vaccine also demonstrated diminished mortality and prolonged survival of tumor-bearing mice, showing the anti-tumor effect of POL-P3b. Therefore, POL-P3b functions as a highly propitious dietary adjuvant for the DC vaccine, inducing DC maturation. POL-P3b, in addition to inhibiting tumor growth by increasing tumor apoptosis, also inhibited lung metastasis, and induced non-toxic side effects in mouse models (Jia et al., 2021). Therefore, POL-P3b holds great promise as an efficient and safe immunomodulatory agent capable of regulating DC maturation and enhancing immune responses of the DC vaccine against breast cancer (Liu et al., 2021). isolated two novel amide glycosides, oleraciamide E and oleraciamide F, containing similar molecular structures from P. oleracea L. wherein oleraciamide E demonstrated anticholinesterase activity with an IC50 value of 52.43 ± 0.33 M and exhibited scavenging activity in 1,1-diphenyl-2-picrylhydrazyl (DPPH) radical quenching assays with an IC50 value of 24.64 ± 0.33 M which indicate antioxidant effects.
2.5 Withania somnifera
Withania somnifera is a small, bushy, evergreen shrub widely distributed across the world and abundant in South Africa and Botswana. It is a member of the Solanaceae family (Gaurav et al., 2015). It has been shown that W. somnifera root extracts can inhibit vimentin, a protein normally found in regions of metastasis, thus suggesting its counteracting effects on tumour formation in breast cancer (Yang et al., 2013). The anticancer potential of W. somnifera has been mainly attributed to Withanolide and Withaferin A, which are two principal phytochemicals from this plant. In vivo, in mice, it was reported that W. somnifera, through the activities of Withanolide and Withaferin-A, modulate different signalling pathways such as apoptosis, autophagy and reactive oxygen species (ROS) pathways (Shikder et al., 2020). In male Swiss albino mice, extracts from W. somnifera were shown to inhibit lung adenoma (Senthilnathan et al., 2006a) while the root extracts of this plant were shown to prevent ROS-induced injury in model mice (Senthilnathan et al., 2006b). Ethanol extracts of the roots of W. somnifera were shown to inhibit the proliferation of A549 lung cancer cells through the downregulation of PI3K, which reduced metastasis (Vamsi et al., 2020). In vitro, Withaferin-A has been shown to reduce the proliferation of the human breast cancer cell line MDA-MB-231 by inhibiting the two-pore domain potassium (K2P9) channel TASK-3 (Zúñiga et al., 2020). Withaferin-A has also been shown to inhibit the proliferation of other cancer cell lines such as the cervical cancer cell lines (HeLa, SKGII, ME180) and ovarian cancer cell lines (OKV-18 and SKOV3) (Sari et al., 2020). The activity of Withaferin-A against these cells is said to be brought about by the upregulation of the tumour suppressor p53 coupled with cell growth arrest and DNA damage signalling (Sari et al., 2020). Withanolides have been shown to inhibit the proliferation of MCF-7s by inducing apoptosis, inducing the overexpression of Hsp70 and reducing the expression of ER in MCF-7s (Chen et al., 2008). Withanolides were also found to suppress TGF-b1 and TNF-a induced Epithelial-Mesenchymal Transition (EMT) in the lung cancer cell lines H129 and A549 (Yang et al., 2013). Table 1 shows bioactive compounds of Withania, the plant parts they are derived from, the different types of cancer that they target, and their mechanisms of action during cancer alleviation.
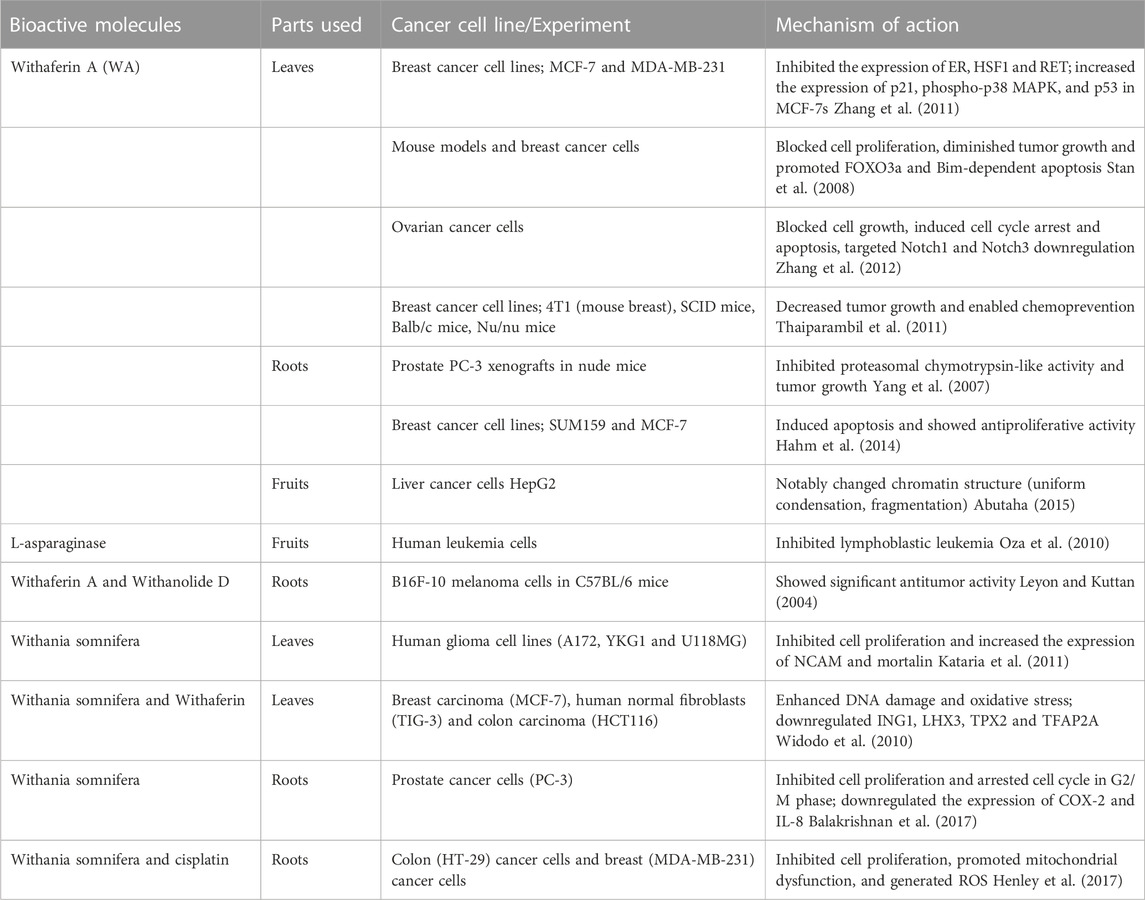
TABLE 1. Withania bioactive compounds, plant parts they are derived from, their target cancers, and their mechanisms of action.
2.6 Azanza garckeana
Azanza garckeana is a semi-deciduous tree and a member of the Malvaceae family that is commonly found in East and Southern Africa. According to (Michael et al., 2015), on evaluating phytochemicals of the seeds of this plant, it was discovered that they have tannins, saponins, flavonoids, alkaloids, phenols, glycosides and carotenoids. The benzopyrone ring structure, known for its antioxidant properties, is integral to flavonoids, making flavonoids to have antioxidant behaviour. This antioxidant activity is able to fight and remove free radicals in biological systems and inhibit the development of tumors (Michael et al., 2015). The seeds of A. garckeana are said to be able to reduce cancer development due to their possession flavonoids, which are able to interfere with oestrogen synthase, an enzyme that produces oestrogen (Okwu, 2005). A complex formed between Mansone G and β-Cyclodextrin, which are some of the phytochemicals extracted from A. garckeana, was found to exhibit high levels of cytotoxicity towards A549 lung cancer cells (Bioltif et al., 2020). Figure 1 shows the leaves of Portulaca oleracea, Tribulus terrestris, A. garckeana and Withania somnifera.
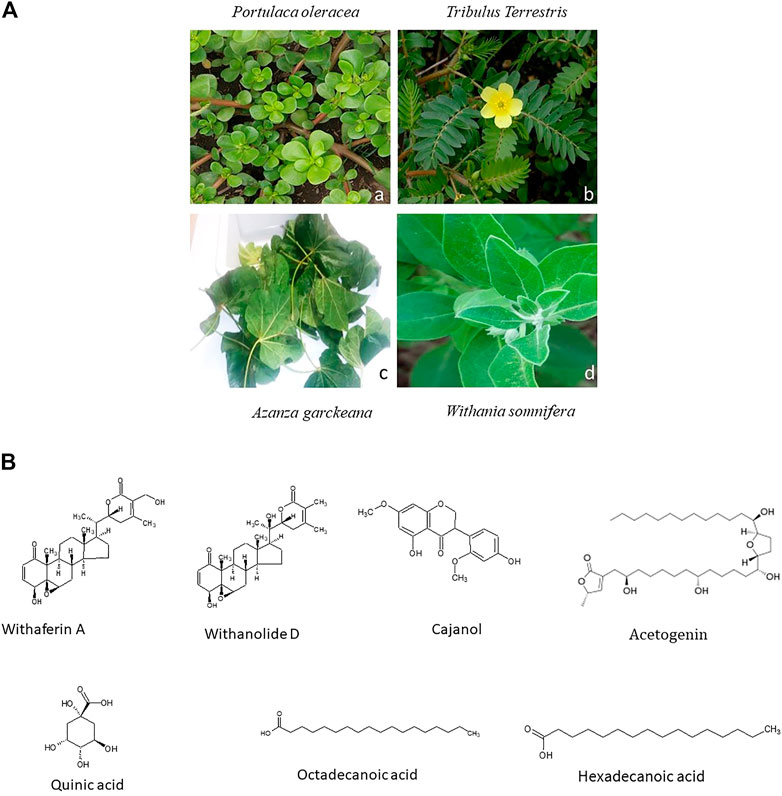
FIGURE 1. (A) Images of a selected African Medicinal Plants species used for Cancer management. Leaves of Portulaca oleracea are fleshy and oval (a) (Source: https://www.terrepromise.ca/en/products/lettuce/greens/[accessed 12 Oct 2022]), Tribulus Terrestris leaves are opposite each other and unequal in size (b) (Source: https://www.zimbabweflora.co.zw/speciesdata/image-display.php?species_id=132940&image_id=4 [accessed 3 Nov 2022]), Harvested leaves of Azanza garckeana showing a linear midrib fissure (c), Withania somnifera leaves are broadly ovate with curvy margins (d) (Source: https://www.magicgardenseeds.co.uk/The-Good-To-Know/Winter-Cherry-(Withania-somnifera)-A.WIT01- [accessed 27 October 2022]). (B) Structures of some Phytochemicals derived from African Medicinal Plants.
2.7 Cajanus cajan
Ovarian cancer is the second most prevalent gynecological malignancy and one of the most lethal cancers. The major challenge in the treatment of ovarian cancer is the emergence of multi-drug resistance during chemotherapy. Cajanol is derived from Cajanol cajan roots and has a multitude of pharmacological activities such as anti-tumor properties. Cajanol inhibits NF-κB phosphorylation and nuclear ectopia by interfering with PI3K expression and the phosphorylation of Akt. This diminishes the transcription and translation of the permeability glycoprotein and eventually decreases cancer related multi-drug resistance that is induced by the efflux of paclitaxel (Sui et al., 2021). Cajanus cajan is a medicinal plant that is native to the Southwest of Nigeria. It is grown for food in Nigeria (Ashidi et al., 2010). C. cajan synthesizes cajanin stilbene acid (CSA) which shares structural analogies with estrogen. CSA exerts antiestrogenic and anticancer activities towards estrogen receptor (ERα)-positive breast cancer cells. Particularly, it shows cytotoxicity towards MCF-7 cells resistant to tamoxifen while exhibiting low cytotoxicity towards ERα-negative breast tumor cells (Fu et al., 2015). This cytotoxicity is independent of the cellular p53 status. CSA binds to the same site as tamoxifen and 17β-estradiol on ERα. Thus, CSA displays its anticancer properties against ERα-positive breast cancer cells by interacting with and inhibiting ERα. The cytotoxicity of CSA towards MCF-7s resistant to tamoxifen indicates its promise as a tamoxifen alternative for treatment of breast cancer. When combined with tamoxifen, CSA enables synergistic cytotoxicity and promotes p53 protein expression. Cajanol, scientifically known as 5-hydroxy-3-(4-hydroxy-2-methoxyphenyl)-7-methoxychroman-4-one, is an isoflavone isolated from the roots of C. cajan. In MCF-7s, cajanol causes cell cycle arrest at the G2/M phase while inducing apoptosis through a mitochondrial pathway mediated by ROS (Luo et al., 2010). This leads to the disintegration of the outer membrane of the mitochondria and the release of cytochrome C followed by the elicitation of the caspase-3 and caspase-9 cascade which causes apoptosis. In COR-L23 lung cancer cells, C. cajan was shown to have anticancer activity with an IC50 value of 5–10 μg/mL. It was reported that stilbenes longistylins A and C present in the leaf extracts of the plant were responsible for this anticancer property (Ashidi et al., 2010).
2.8 Combretum caffrum
The bark of Combretum caffrum, the African bush willow tree, is a source of natural phenolic stilbene compounds called combrestatins (Pettit et al., 1987). Among these combrestatins, combrestatin A-4 (CA4) is the most efficient as an antitumor agent. CA4 inhibits tubulin polymerization, augments vascular permeability, and abrogates blood flow into tumors (Simoni et al., 2006). A drug candidate, Ecust004, has been developed as an optimized agent derived from the structure-activity investigations of the sulfamate derivatives of CA4 and Erianin. This is a strong inhibitor of steroid sulfatase and tubulin in addition to exhibiting antiproliferative activity against tumor cells at low concentrations. This sulfate modification augments the pharmacokinetic profiles and bioavailability of the parental CA4 and Erianin natural compounds (Raobaikady et al., 2005; Foster et al., 2008; Visagie et al., 2015). Ecust004 inhibits tumor proliferation in vivo and in vitro in addition to diminishing cell viability, migration, and invasion of MCF-7 and MDA-MB -231 cells at low dosages. Combrestatins comprise a category of closely analogous stilbenes including combretastatins A, dihydrostilbenes or combretastatins B, phenanthrenes or combretastatins C and macrocyclic lactones or combretastatins D which are all derived from the bark of C. caffrum. Some of these compounds are the strongest antitubulin agents ever known. Owing to the structural simplicity of these substances, several analogs have been synthesized. Amongst these, Combrestatin A4 phosphate is the most tested substance in preclinical and clinical trials (Karatoprak et al., 2020). This water-soluble prodrug is rapidly metabolized in the body to combrestatin A4 that displays antitumor, antiproliferative, anti-inflammatory and antioxidant activities. Nano-formulations of CA4 phosphate have important advantages such as increased water solubility, drug targeting capabilities, improved efficiency, protracted half-lives in circulation and lesser side effects. Therefore, combrestatins are favorable candidates for novel tumor therapeutics.
Combrestatins have been shown to be active against many human cancer cell lines and combrestatin A-4 is the strongest in terms of potency. Their principal action is their interaction with the colchicine binding site of the tubulin b subunit and the disruption of tubulin polymerization into microtubules (Izuegbuna, 2022). CA4 has been shown to be cytotoxic towards some cancer cell lines such as the leukemia cell line, P-388, which is resistant to daunorubicin (McGown and Fox, 1990). It acts by disrupting cell signaling pathways involved in the maintenance and regulation of the cytoskeleton of the endothelial cells occurring in the tumor vasculature, thus causing the selective disruption of blood flow through the tumors. Thereupon, these tumor cells undergo necrosis. Intriguingly, while enabling disruption of blood supply into the tumor cells, CA4 maintains normal blood flow into adjacent normal tissues (Nihei et al., 1999). CA4 activity is not dependent on temperature. This contrasts with colchicine, which interacts with a similar binding site on the tubulin molecule. The complexation of CA4 tubulin occurs easily even on ice (Nam, 2003). Another CA4 sodium phosphate (CA4P) pro-drug has also been synthesized. The action of CA4P is like that of CA4. It leads to microtubule depolymerization and represses tumor cell angiogenesis (Tozer et al., 2002). CA4P eventually induces apoptosis in tumor cells (Iyer et al., 1998), although it has been reported that it results in cell death through molecular pathways, rather than apoptosis, such as the mitotic catastrophe pathways (Nabha et al., 2001). These pathways, which lead to cell death, make combrestatin a powerful anticancer compound of high efficacy (Jaroch et al., 2016).
2.9 Prunus avium
Prunus avium (Sweet cherry) is a plant that is widely distributed in the north of Africa (Osafo et al., 2017). Dark sweet cherry is a rich source of phenolics and is characterized by its anti-invasive and anticancer activities. Prunus avium phenolics inhibit MDA-MB-453 breast cancer cells by triggering cell signaling pathways that elicit apoptosis and disrupt cell invasion. Amongst these phenolics, anthocyanins have shown augmented chemopreventative properties (Layosa et al., 2021). Polyphenols, especially anthocyanins, are well known for their anti-inflammatory, cytoprotective and antioxidant properties (Tsuda et al., 2000; Li et al., 2014; Huang et al., 2018; Silva et al., 2020). Table 2 shows examples of anticancer bioactive compounds found in P. avium and outcomes of their experimental uses.
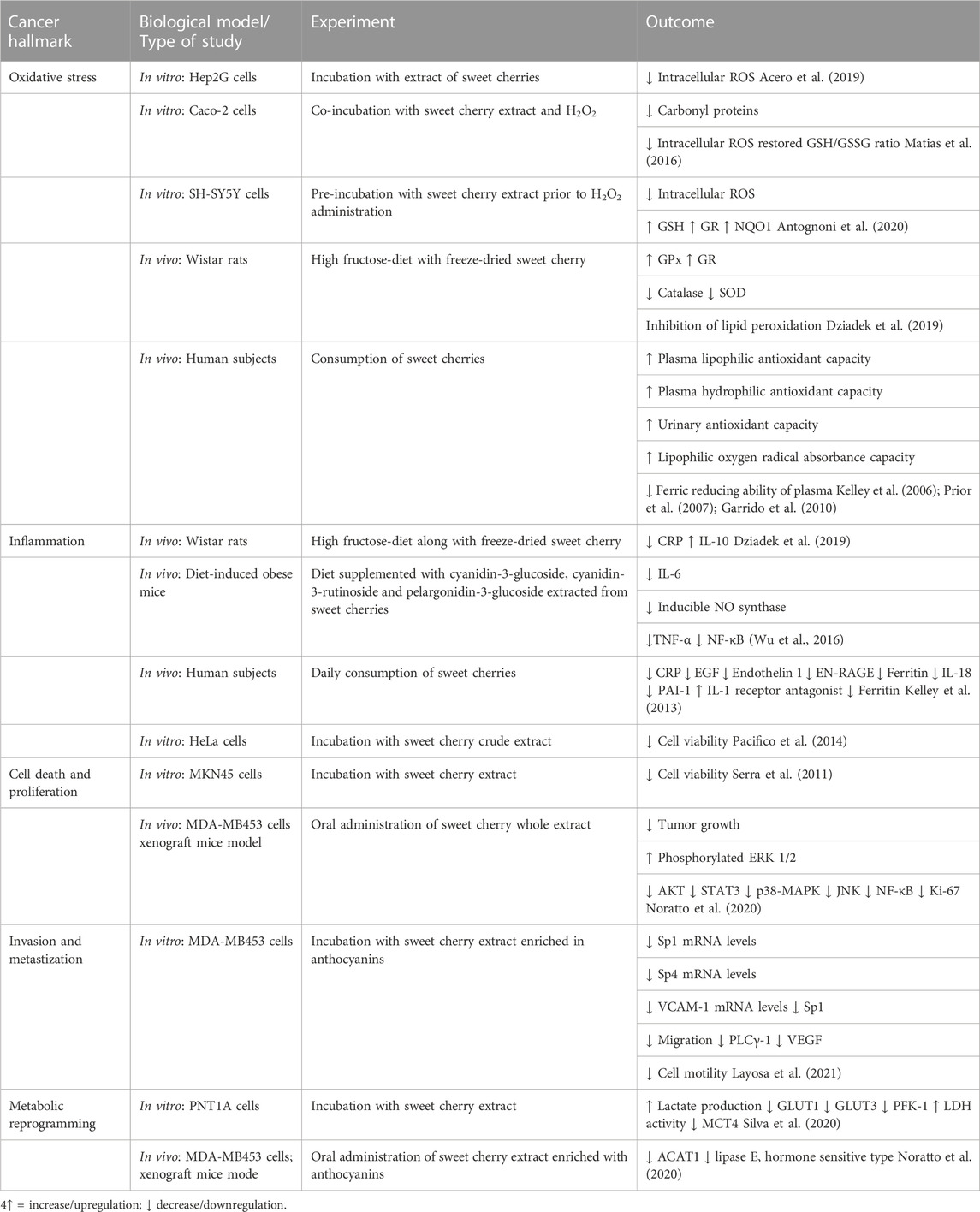
TABLE 2. Biological properties of Prunus avium extracts and their bioactive compounds that target hallmarks of cancer.
2.10 Prunus africana
Prunus africana is a widely distributed tree of African origin which is primarily found in Southern and Central Africa (Komakech et al., 2022). In previous ethnomedical studies, the bark decoction of P. Africana was used to treat prostate cancers (Grace et al., 2003; Ochwang’i et al., 2014; Tugume et al., 2016). In vivo studies, mice with transgenic adenocarcinoma in their prostate were fed with P. africana and demonstrated a significant decrease in the incidence of prostate cancer when compared to control mice fed with casein (Shenouda et al., 2007). Likewise, in human in vivo studies, P. africana bark extracts induced 50% inhibition of the growth of human prostate cancer (PC-3). It also elicited notable apoptosis in vitro in the PC-3 cell line (Shenouda et al., 2007). The anticancer property of the P. africana stem bark is attributed to several novel bioactive compounds such as β-amyrin, N-butylbenzene-sulfonamide, β-sitosterol, β-sitosterol-3-O-glucoside, ferulic acid, tartaric acid, oleanolic acid, lauric acid and ursolic acid (Komakech and Kang, 2019). However, P. africana is an endangered species and the supply of the P. africana stem bark does not meet global demand (Cunningham et al., 2016). A micropropagation protocol has been developed for P. africana towards enabling drug development in the future for the treatment of prostate cancer (Komakech et al., 2022). The presence of β-Sitosterol in P. Africana has been attributed to its anti-prostate cancer properties wherein it induces apoptosis in human prostate cancer cells during the development of carcinoma in prostate lymph-nodes (von Holtz et al., 1998; Awad et al., 2000). Also, the 2,3-dihydro-3,5-dihydroxy-6-methyl-4H-pyran-one in extracts of P. africana is capable of inactivating NF-kB which could account for its proapoptotic and antiproliferative activities on PC-3 cancer cells. Additionally, the occurrence of benzoic acid in these extracts could be responsible for its activity against cancer as earlier studies showed that derivatives of benzoic acid hinder growth of prostate cancer cells thus precluding oncogene expression by the inhibition of histone deacetylases (Anantharaju et al., 2017).
2.11 Securidaca longipedunculata
Securidaca longipedunculata Fresen (violet tree) belongs to the family Polygalaceae, which is called the mother of all medicines in Northern Nigeria. Traditional medical practitioners use extracts of Securidaca longipedunculata to manage cancer in Africa (Saidu et al., 2015). The antiproliferative effects of S. longipedunculata on Ehrlich ascites carcinoma in vivo and in vitro are due to the downregulation of angiogenesis and the elicitation DNA fragmentation (Lawal et al., 2012). Root bark extracts of S. longipedunculata have been reported to repress proliferation and stimulate apoptosis in U87 brain tumor cells through the cleavage of Poly-ADP-Ribose Polymerase (Ngulde et al., 2019). Ethanolic extracts of the plant hindered the proliferation of the U87 cells. The most polar fraction of these extracts accounted for this activity with an IC50 value of 20.535 μg/mL. When administered at 10 mg/kg, the extracts augmented the lifespan of tumor-harboring mice by decreasing tumor cell viability (Ngulde et al., 2019). Several active compounds have been identified in Securidaca longipendunculata including xanthones (muchimangins), methyl-salicylate, benzyl benzoates, bisdesmosidic saponins and triterpene saponins, of which xanthones have been implicated in antitumor and cytotoxic activities (Mitaine-Offer et al., 2010; Dibwe et al., 2014; Zuo et al., 2016; Obasi et al., 2018; Klein-Júnior et al., 2020). Saponins in Securidaca longepedunculata induced apoptosis and inhibited the migration and invasion of cervical cancer cells (Obasi et al., 2018). Xanthones of in S. longipedunculata impeded the proliferation of lung cancer cells and functioned as an elicitor of apoptosis (Zuo et al., 2016).
2.12 Annona senegalensis
Annona senegalensis, which is often called wild soursop, is a 2 to 5-m-tall shrub with alternate, oblong, simple, blue to greenish leaves. It is an important plant in Northern Nigeria and all its parts have been found to be useful in medicine. It contains a plethora of phytochemicals including alkaloids, glycosides, flavonoids, saponins, anthocyanins, tannins, and steroids (Potchoo et al., 2008; Johnson et al., 2017; Musa et al., 2017). Extracts from this plant have been shown to have antitumor activity in hepatocellular carcinoma induced by N-diethylnitrosamine in male Wistar rats. A. senegelensis n-hexane extracts have shown anticancer effects by augmenting liver architecture, enhancing antioxidant defense systems, downregulating anti-apoptotic, pro-inflammatory, angiogenic, farnesyl transferase and alpha-fetoprotein mRNA expression and upregulating P21 and P53 tumor suppressor mRNAs (Yakubu et al., 2020).
2.13 Annona muricata
Annona muricata is a tropical fruit tree that belongs to the family Annonaceae. This plant is widely cultivated in African countries such as Angola and some West African countries where its products such as fruits are consumed as food. Annona muricata is a plant of great applicability in traditional medicine. Ethanol extracts from the leaves of this plant contain several phytochemicals such as alkaloids, tannins, flavonoids, cardiac glycosides, reducing sugars, triterpenoids and saponins (Gavamukulya et al., 2014). Aqueous extracts of the leaves contain terpenoids, alkaloids, coumarin, flavonoids, fatty acids, steroids, phenols, saponins and tannins while ethyl acetate fractions contain saponins, phenols, flavonoids, polyphenols, and tannins (Agu and Okolie, 2017). Annona muricata expresses over 45 acetogenins in its leaves and seeds (Gleye et al., 1998). The ethyl acetate fraction of A. muricata leaves induced cytotoxic and antiproliferative activity against breast cancer cells by significantly decreasing mitochondrial membrane integrity, leading to the elicitation of apoptosis in the cells (Hadisaputri et al., 2021). This apoptotic mechanism was identified by alterations in cell morphology and the expression levels of caspase-3, caspase-9 and Bcl-2 mRNAs that were involved in the cytotoxic activity induced by the ethyl acetate fractions on MCF-7 cells (Jabir et al., 2021). prepared silver nanoparticles (AgNPs) by using A. muricata as a reducing agent and studying its potential as a novel therapeutic strategy against cancer. Specifically, the ability of these nanoparticles to decrease NLRP3 inflammasome activity by inducing autophagy was examined. The AgNPs showed antiproliferative effects against AMJ-13 and THP-1 cells through the stimulation of apoptosis by damaging the mitochondria and inducing the p53 protein pathway (Jabir et al., 2021). This AgNP-elicited autophagy decreased the levels of IL-1β and NLRP3 inflammasome activation. This study showed that A. muricata AgNPs could serve as a robust anticancer agent by inducing apoptosis and autophagy and causing inhibition of tumorigenesis (Eritja et al., 2017; Galluzzi et al., 2017). Annona muricata contains two important phytochemicals; acetogenins and flavonoids which have both been implicated in several pharmacological activities (Fang et al., 1993; Yang et al., 2015; Al-Dabbagh et al., 2018). Annonacin, the main acetogenin in A. muricata, has anticancer activities against skin, breast and endometrial cancers mediated by cell cycle arrest and the inhibition of other cellular signaling pathways (Yap et al., 2017; Yiallouris et al., 2018; Roduan et al., 2019). There is growing evidence that this antitumor activity is enabled by the induction of apoptosis in several breast and colon cancer cell lines (Rady et al., 2018). Additionally, the G1 phase cell cycle arrest has been reported to be involved in the antitumor activity of A. muricata leaf extracts. Both annonacin and ethyl acetate A. muricata bark extracts showed selective and robust cytotoxicity against DU-145 prostate carcinoma cells with respective IC50 values of 0.1 ± 0.07 μM and 55.501 ± 0.55 μg/mL. Normal RWPE-1 prostate cells were not affected. The chemotherapeutic agent, docetaxel, was, however, devoid of such selectivity. Moreover, when docetaxel was administered in combination with A. muricata ethyl acetate bark extracts, its impact against DU-145 cells was enhanced by 50% (Foster et al., 2020). This was attributed to a non-apoptotic mechanism mediating cell death. Annonacin and bark extracts of A. muricata acted as selective cytotoxic compounds with antiangiogenic and antimetastatic potential. Leaf methanolic extracts of A. muricata (LMAM) showed inhibitory effects on the growth of MCF-7s (Naik and Sellappan, 2020) in a dose-dependent fashion and without causing cytotoxic effects against normal breast cancer cells. This was achieved through an apoptotic pathway (Pieme et al., 2014). Caspase-3 was found to be upregulated, serving as a determinant of apoptosis. LMAM arrested cell cycle at the G1 phase and blocked the G1/S transition attributed to the activation of apoptosis and sub-G0/G1 cell cycle arrest. LMAM displayed major inhibitory activity against MCF-7 cancer cells with an IC50 value of 85.55 mg/mL.
2.14 Aerva javanica
Aerva javanica is a shrub that belongs to the Amaranthaceae family of plants. This plant is native to tropical African countries and has been reported to have anticancer properties in Ethiopia (Ayele, 2018). During its use in the treatment of breast cancer, the roots of A. javanica are ground into powder and mixed with blood from bats. This mixture is then consumed by the cancer patient early in the morning before breakfast (Teklehaymanot, 2009). The callus and leaf methanol extracts of A. javanica were tested against MCF-7s (Teklehaymanot, 2009; Abate et al., 2022) and were found to induce DNA fragmentation and cytotoxicity, which are indicators of apoptosis.
2.15 Abelmoschus esculentus
Abelmoschus esculentus, also known as Okra, is a native African plant of the family Malvaceae. It has been historically been used to treat different ailments such as constipation, hypoglycaemia, inflammation and microbial infections (de Sousa Ferreira Soares et al., 2012). The seeds of A. esculentus contain flavonoids such as isoquercitrin which have shown cytotoxicity towards carcinoma cell lines; MCF-7 (breast cancer), HepG2 (liver cancer) and HeLa (cervical cancer) (Agregán et al., 2022). Okra flower extracts, which are rich in flavonoids, are capable of inhibiting cell proliferation in colorectal tumors and this inhibitory activity has been found to be due to the dysfunction of the mitochondria which is caused by the activation of p53 and the elicitation of apoptosis and senescence. In mice models, flavonoids in A. esculentus have shown cancer preventative activities which precluded tumor appearance. Okra flower phytochemicals positively impacted liver cancer prognosis while Okra ethyl acetate extracts showed inhibitory activity against HepG2 cells at concentration ranges between 62.5 and 1,000 μg/mL (Solomon et al., 2016). Therefore, Okra flowers were shown to be a valuable source of anticancer molecules which could augment the health of cancer patients. A novel lectin has been found in Okra seeds and it promotes antitumor effects in MCF-7s wherein it inhibits cell growth. It also enhances p21, caspase-3 and caspase-9 expression in the carcinogenic cells (Monte et al., 2014). Cerium oxide (CeO2) nanoparticles were generated using A. esculentus as a stabilizing and reducing agent. Exposure of HeLa cells to these CeO2 nanoparticles at 10–125 μg/mL resulted in the loss of cell viability in the cervical cancer cells in a dose-dependent fashion (Ahmed et al., 2021). Abelmoschus esculentus has been shown to exhibit anticancer effects due to its high antioxidant activity against free radicals (Arapitsas, 2008; Ahmed and Kumar, 2016). It is a source of polysaccharides, flavonoids, terpenoids, tannins, alkaloids, enzymes, proteins, and vitamins (Musthafa et al., 2021) demonstrated the apoptotic potential of A. esculentus lectins against human glioblastoma cells by the modulation of the caspase-3 and caspase-7 gene expression and the downregulation of CLOCK and Bma1 circadian genes, implicating a correlation between these circadian genes and apoptotic cell death. Increased cytotoxicity, morphological changes, increased intracellular ROS generation and anti-migratory activity were also observed. Due to these effects, A. esculentus extracts can be considered as adjunct therapy in alleviating human glioblastoma. Furthermore, pulp extracts of A. esculentus were used to synthesize gold nanoparticles which showed enhanced anticancer effects (Devanesan and AlSalhi, 2021). Silver nanoparticles made using A. esculentus flower extracts showed antiproliferative, apoptotic and cytotoxic effects against A-549 and TERT-4 cancer cell lines. Quercetin diglucoside and isoquercitrin identified in A. esculentus were shown to be potently anti-ROS and thus could be used in cancer treatment (Taiwo et al., 2021).
2.16 Flueggea virosa
Flueggea virosa is widely distributed is the Southern Africa. Flueggea virosa leaves and twigs were used to isolate flueggines A 1) and B 2) indolizidine alkaloids, the latter of which showed notable inhibitory activities against the growth of breast cancer cells MDA-MB-231s (estrogen-independent) and MCF-7s (estrogen-dependent) with IC50 values of 147 ± 3 and 135 ± 5 nM respectively. This suggested that cell proliferation was inhibited irrespective of estrogen receptor status (Zhao et al., 2011).
2.17 Lagenaria siceraria
Lagenaria siceraria is a native African climbing plant that belongs to the Cucurbitaceae family (Abebe, 2016). (Mondal and Swamy, 2020) identified BGL24, a novel PP2-type lectin in the phloem exudate of this plant, which is also called Bottle gourd. This lectin displayed high specificity for chito-oligosaccharides. BGL24 showed moderate cytotoxicity towards MDA-Mb-231 breast cancer cells but did not impact normal splenocytes. The latex sap of L. siceraria (LSL) markedly elicited lymphocyte proliferation and demonstrated potent cytotoxicity against cancer both in vivo and in vitro (Vigneshwaran et al., 2016). LSL caused tumor regression and drastically impacted tumoral neovasculature. Additionally, LSL stimulated the apoptotic signaling cascade in tumor cells through the activation of caspase-3 and the induction of apoptotic cellular events. Therefore, LSL possesses immunopotentiating properties which negatively impact tumor progression by attacking angiogenesis and inducing programmed cell death, which are major hallmarks of cancer. Bottle gourd juice (BGJ) was examined for its chemopreventive properties against croton oil and 7,12-dimethylbenz(a)anthracene (DMBA) and was found to elicit skin papillomagenesis in murine models (Kumar et al., 2013). BGJ caused drastic reductions in the incidence, latency, number, multiplicity, size, and volume of the papillomas. This chemopreventive effect was mediated by reducing loss of stratification, decreasing the number of epithelial layers, diminishing dermal infiltration and protection from several cytoplasmic changes. Therefore, BGJ consumption could help in the inhibition of skin cancer.
2.18 Xylopia aethiopica
Cytotoxic metabolites have been identified in methanol extracts of X. aethiopica and were found to inhibit the growth of multidrug resistant and drug-sensitive cancer cell lines (Kuete et al., 2015). Among these metabolites, flavone elicited apoptosis in CCRF-CEM leukemia cells through the disruption of the mitochondrial membrane potential and isoquinoline triggered apoptosis via the production of ROS, implicating these compounds as antiproliferative agents against drug-resistant cancers. Essential oils obtained from Xylopia aethiopica sourced from Cameroon and Chad were found to be abundant in monoterpene hydrocarbons such as β-phellandrene, β-pinene, γterpinene and sabinene. Oxygenated monoterpenes were highly prevalent, amongst which terpinen-4-ol was most significant (Bakarnga-Via et al., 2014). Hydroethanolic extracts of X. aethiopica showed antiproliferative activity against HCT116 colon cancer cells as well as KG1a and U937 leukemia cell lines. Xylopia ethiopica extracts also demonstrated antiproliferative effects on human cervical cancer cells. Furthermore, α-cadinol and terpinen-4-ol in essential oils isolated from X. ethiopica were found to be active against laryngeal, lung, ovarian, breast, gastric and colon cancer cell lines (Bakarnga-Via et al., 2014). Additionally, βpinene, a monoterpene found in this oil, showed notable cytotoxic activity against epidermal skin and breast cancer cell lines. Fruit extracts of X. aethiopica caused the activation of caspase-3 and led to the cleavage of cytoskeletal proteins and the elicitation of DNA fragmentation factors, condensation of chromatin, formation of apoptotic bodies and eventually apoptosis as observed through morphological analysis (Fulda and Debatin, 2006; Ribeiro et al., 2021). (Adaramoye et al., 2011) showed that fruit extracts of X. aethiopica caused antiproliferative activity against human cervical carcinoma cells causing cell cycle arrest and elevated levels of p53 and p21 gene transcripts. Caspase-3 activation and apoptotic cell death have been demonstrated in MBA-MD-231 breast cancer cells upon exposure to hydroethanol extracts sourced from the Cameroonian varieties of X. aethiopica fruits (Choumessi et al., 2012).
2.19 Nymphaea lotus
Nymphaea lotus belongs to the family Nymphaeaceae and is a perennial aquatic flowering plant that is native to Egypt and grown in several regions in Madagascar, West Africa, and Central Africa (Slocum, 2005). Hydroethanolic leaf extracts of N. lotus contain saponins, tannins, flavonoids, phenolics and triterpenoids but are lacking in alkaloids. These extracts have been shown to have anti-inflammatory and cytotoxic activities along with high antioxidant potential against Jurkat and MCF-7s, properties that are attributed to the occurrence of abundant flavonoids and phenolics as well as micro/macro-elements such as sulfur, phosphorus, manganese, magnesium, zinc and copper (Saleem et al., 2001; Elegami et al., 2003; Laszczyk, 2009; Afolayan et al., 2013; Oyeyemi et al., 2015; N’guessan et al., 2021). These properties may account for the use of the leaves of Nymphaea lotus in traditional anticancer treatments.
2.20 Zanthoxylum chalybeum
Zanthoxylum chalybeum is a deciduous shrub with a rounded but open crown. It can grow between 1.5 and 40 cm in diameter, with large woody spines that can grow up to 2 cm long. It is a widely used traditional medicine in East Africa and can be harvested from the wild for local use as tea, medicine, toothbrush, and timber. Zanthoxylum chalybeum is reported to exhibit anti-cervical cancer properties, where part of the plant used is pound before adding water to drink (Tugume et al., 2016; Omara et al., 2020). Significant antiproliferative effects of crude extracts of alkaloids from Zanthoxylum species were observed against human cervical cancer cells (HeLa), human gastric cancer cells (SGC-7901), human hepatocyte carcinoma cells (Hep G2) and human colorectal adenocarcinoma cells (HT29), ranging from 60.71% to 93.63% at 200 μg/mL concentration (Tian et al., 2017). Zanthoxylum contains quaternary alkaloids which have been shown to be potential anti-cancer candidates that can penetrate through the cell membranes of carcinomas (Yung et al., 2016) and can attract the negative charges on DNA (Bai et al., 2006). Based on their structural skeleton, these quaternary alkaloids have been shown to belong to the berberine type, tetrahydroproberberine type, benzophenanthridine type, benzyltetrahydroisoquinoline type and aporphine type (Tian et al., 2017). In Z. chalybeum, the amount of quaternary alkaloids account for 83.4%. Bioactivity tests on Zanthoxylum showed both high inhibitory rates against cancer cells and high quaternary alkaloid content, therefore justifying its use in traditional anticancer medications. The active ingredients responsible for the anticancer activity of this plant include skimmianine, furoquinoline, benzophenanthridine, alkaloids, chelerythrine and nitidine, aporphine alkaloids, tembetarine, N-methylisocorydine, N- methylisocorydine (menisperine) and bernerine, phenylethylamine, candicine, alkamide, fagaramide, dihydrochelerythrine, lupeol and sesamin (Omara et al., 2020).
2.21 Ceratonia siliqua
Ceratonia siliqua is a Mediterranean evergreen plant that is abundantly distributed in North African countries such as Algeria, Morocco, Tunisia, and Egypt (Kaderi et al., 2015). Common ethnomedicinal uses of Ceratonia siliqua include treatment of gastrointestinal diseases, diarrhea, constipation and colon cancer (Benarba and Pandiella, 2018). These medicinal properties may chiefly be due to the presence of fibers and phenolic compounds (Rtibi et al., 2017). (Ghanemi et al., 2017) demonstrated that C. siliqua leaf phenolic extracts inhibited the growth of HTC-116 and CT-26 cell lines, in a dose dependent manner, confirming earlier similar findings by (Custódio et al., 2013).
2.22 Moringa oleifera
Moringa oleifera, a member of the Moringaceae family, is a drumstick tree which was first used medically by the ancient Egyptians before its cultivation around the globe which spread its medicinal benefits (Abd-Rabou et al., 2017). Moringa oleifera was found to be effective in treating colon cancer (Al-Asmari et al., 2015). The plant owes its anticancer properties to the presence of quercetin, kaempferol, β–D-glucopyranoside, tetracanoate, β–sitosterol glucoside, isothiocyanate (Kaur, 2015), hexadecenoic acid and eugenol (Al-Asmari et al., 2015). (Suphachai, 2014) reported the growth inhibition of hepatocarcinoma (HepG2), colorectal adenocarcinoma (Caco-2), and breast adenocarcinoma (MCF-7) cell lines by dichloromethane leaf extracts of Moringa oleifera with IC50 values between 112 and 113 μg/mL. Recently, in vitro in vivo anticancer activities of M. oleifera have been reported. In their study (Barhoi et al., 2021), identified quinic acid, octadecanoic acid and hexadecanoicacid (palmitic acid) as the active compounds during the activity of aqueous extracts of M. oleifera on Ehrlich ascites carcinoma (EAC) and Hep2 (Human laryngeal carcinoma) cells. They reported that, in vivo, aqueous extracts of M. oleifera led to a reduction in tumor weight and tumor volume in tumor bearing mice, consequently elongating the life span of the mice. Additionally, in vitro, the extracts of M. oleifera were toxic to both Hep2 and EAC cancer cell lines. Apoptosis was also induced through the alteration of the mitochondrial membrane potential in the EAC cells (Barhoi et al., 2021).
It has also been recently shown that methanolic extracts of M. oleifera leaves led to a reduction in cell growth in cervical cancer cells (Pandey and Khan, 2021). The cervical cancer cells were reported to have undergone apoptosis. The anticancer potential of the M. oleifera leaf extracts was due to the inhibitory activity of the extracts on Jab-1, which is an important biomarker associated with the development of different cancers (Pandey and Khan, 2021). The inhibition of M. oleifera on Jab-1 led to its downregulation. There was also an increase in the expression of the tumor suppressor p27, which led to cell growth arrest at the G0/G1 phase.
Methanol extracts of M. oleifera leaves have also been reported to have apoptotic effects on PC-3 prostate cancer cells (Khan et al., 2020). The anticancer activity of M. oleifera leaf methanolic extracts was due to the induction of ROS-mediated apoptosis and the activation of caspase-3 activity in the prostate cancer cells. Cell cycle arrest at the G0/G1 phase and changes in the expression of genes of the Hedgehog signalling pathway were also observed (Khan et al., 2020).
Moringa oleifera leaf extracts have been shown to have anticancer activity on the human squamous cell carcinoma 15 cell line (SCC15). In their study (Luetragoon et al., 2020), reported that the proliferation of SCC15 cells treated with M. oleifera leaf extracts was inhibited. The extracts were shown to induce cell cycle arrest at the G2/M phase and apoptosis in the cells. Cell migration and colony formation were also inhibited in the cells. Furthermore, there was a downregulation of the anti-apoptotic marker Bcl-2 and an upregulation of both Bax and caspase-3 (Luetragoon et al., 2020).
Through the activity of its bioactive compound 4-[(α-L-Rhamnosyloxy) benzyl] isothiocyanate (MIC-1), which is found in the seeds, M. oleifera has been reported to inhibit the migration and proliferation of renal cancer cells (Xie et al., 2022). This regulatory activity is said to be brought about by the regulation of the PTP1B-dependent Src/Ras/Raf/ERK signalling pathway in 786-O hypertriploid renal cell carcinoma (RCC) cancer cells. MCI-1 from M. oleifera seeds also induced cell cycle arrest and caused the downregulation of the expression of cell cycle-related proteins in the 786-O cells (Xie et al., 2022).
2.23 Peganum harmala
Peganum harmala is a perennial herbaceous plant with a woody underground root stalk. It is a member of the Nitriaceae family and mostly grows in temperate deserts and Mediterranean regions (Alves-Silva et al., 2017). reported the ethnomedicinal uses of P. harmala as a remedy for the treatment of breast, liver, and bone cancer. It is also considered as a treatment for other different types of cancer (Kabbaj et al., 2012). Peganum harmala seeds from Morocco are ground with honey during cancer treatment. Despite the ethnomedicinal uses of P. harmala, the plant is also toxic and may cause hallucinogenic effects because of the presence of β-carbolines such as harmaline, harmine, harmalol, harmol, tetrahydroharmine, and the quinazoline derivatives vasicinone and deoxyvasicinone (Passos and Mironidou-Tzouveleki, 2016). Table 3 gives a summary of some of the in vivo and in vitro studies that has been carried out on plants that have been discussed in the manuscript as well as the identified bioactive compounds in these plants and the effects of these compounds on cancer progression.
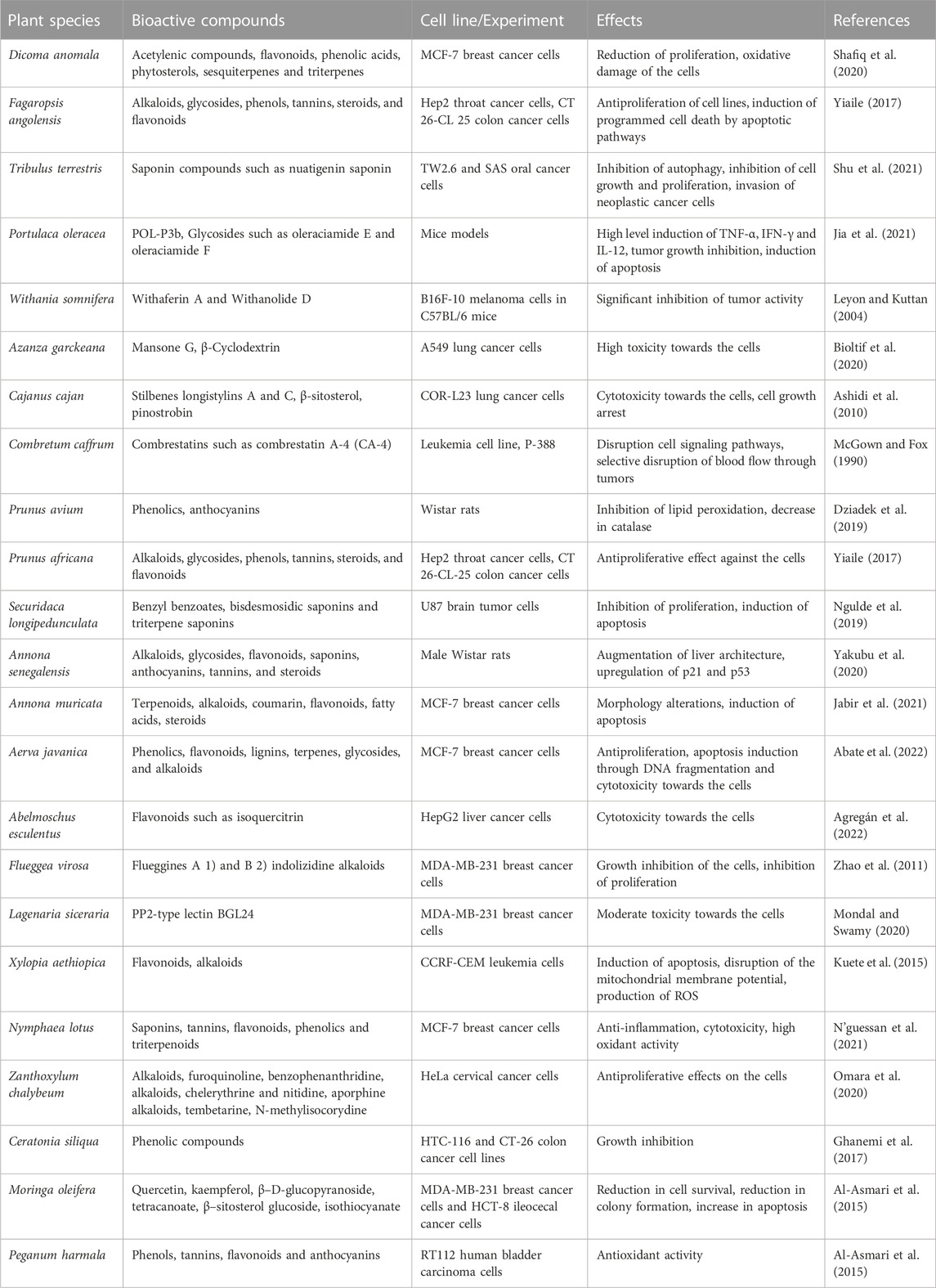
TABLE 3. A summary of pharmacological information of all the medicinal plants that have been discussed in the manuscript.
3 Semi-synthetic studies for new drugs derived from natural compounds for optimizing anticancer activity
Over 25% of drugs used for combating human diseases are directly sourced from plants and another 25% are chemically modified natural products (Amin et al., 2009). Polyphenols constitute a plethora of naturally occurring compounds found in vegetables and fruits. Their health-enhancing characteristics and their application in the prophylaxis and therapy of many human cancers are well known. Several anti-cancer drugs are altered forms of these natural compounds. Etoposide (VP-16, epipodophyllotoxin) is an anticancer drug derived semi-synthetically from a non-alkaloid lignan, podophyllotoxin sourced from the rhizomes and dried roots of Podophyllum emodi or Podophyllum peltatum (Kluska and Woźniak, 2021). Etoposide has been widely employed in cancer chemotherapy to combat various types of cancer such as adrenocortical carcinoma (Paragliola et al., 2020), brain tumors (Ruggiero et al., 2020), breast cancer (Atienza et al., 1995), leukemia (Economides et al., 2019), testicular carcinoma (Alsdorf et al., 2019) and small cell lung carcinoma (Noronha et al., 2020).
Polyphenols enhance the therapeutic action of etoposide by augmenting its cytotoxicity in various cancer cell lines like the retinoblastoma (Sreenivasan and Krishnakumar, 2015), glioblastoma (Ramachandran et al., 2012), breast cancer (Ermakova et al., 2006), cervical cancer (Ruíz et al., 2018), liver cancer (Jia et al., 2016), gastric cancer (Yu et al., 2011; Jia et al., 2016), osteosarcoma (Ferreira de Oliveira et al., 2018), lymphoma (Noda et al., 2007; Li et al., 2008), colon cancer (HWANG et al., 2007; Amiri et al., 2013), head and neck cancer (Heiduschka et al., 2014) and leukemia (Mahbub et al., 2015; Papież et al., 2016). This effect of polyphenols is caused by an increase in DNA damage and apoptosis, production of ROS and arrest of the cell cycle. Investigations on the human breast cancer cell line, MDAMB-231 revealed that flavonoids such as cyanidin, fisetin, (−)-catechin, kaempferol, naringenin, genistein and quercetin, blocked the DNA damage checkpoints and the respective repair pathways. These polyphenols inhibited Chk1 Ser345 phosphorylation induced by etoposide, and this led to the abrogation of the ATR-Chk1 pathway (Kuo et al., 2016). Hence, polyphenols can enhance the therapeutic action of etoposide chemotherapy by rendering the cancer cells more drug sensitive (Fiorito et al., 2014). synthesized a select group of natural and semi-synthetic 1,4-Naphthoquinones whose activity in inhibiting cell growth was studied in vitro on six human cancer cell lines. Among these compounds, only lapachol and its acetate as well as 3-geranyllawsone showed the highest activity with 15–22 μM IC50 values. Several novel anticancer drugs currently under commercial production are derived from natural plant sources and these include etoposide, irinotecan, topotecan, taxotere, taxol, teniposide, vinorelbine, vincristine and vinblastine (Wang, 1998). Thus, natural products serve as the most important source of novel anticancer agents.
Therefore, 1,4-Naphthoquinones constitute a major category of natural products found in plants. Plumbagin (obtained from Plumbago, Nepenthes Drosera spp.), juglone (sourced from the black walnut, Juglans nigra L. (Juglandaceae), and the K vitamins are significant examples of these 1,4-Naphthoquinones. The action mechanisms underlying these observed effects are primarily due to their ability to react with topoisomerases and to produce semiquinone radicals as well as ROS inside the cell (da Silva et al., 2002). (Kode et al., 2020) synthesized Compound 9a containing 1-methyl, 2- and 3-methoxy substituents within the aromatic ring of phenstatin based indole linked chalcone compounds. This was found to be effective against the SCC-29B human oral cancer cell line, spheroids as well as AW13516, an oral cancer mouse xenograft model. Compound 9a anticancer activity was found to be effected by the disruption of glucose metabolism and cellular integrity wherein the latter was caused by repression of tubulin polymerization. Compound 9a was found to directly interact with tubulin at the colchicine binding site in addition to interacting with the active sites of key enzymes involved in the pathway of glucose metabolism (Kode et al., 2020). Thus, compound 9a has found great applicability as a favorable tubulin polymerization inhibitor candidate in anti-cancer therapeutics. Compound 9a greatly reduced the tumor volume while not causing any toxicity in murine models. Additionally, it decreased angiogenesis in xenografts of mice, diminished collagen levels and significantly reduced cellular processes including cellular integrity and organization of the cytoskeleton, while disrupting the uptake of glucose in tumor xenografts. The tubulin polymerization was inhibited which led to profilament bending, destabilization and formation of tubulin ring intermediates.
Lupeol (LU) and Oleanolic acid (OA) are members of the class of natural triterpenes and possess wide-ranging biological activities and cytotoxicity against many cancer cell lines. From these two compounds, 6 novel semi-synthetic triterpenes were synthesized and studied for their pharmacological action (Fontana et al., 2022). Compared to its precursor, Lupeol, the lupane-like compounds showed enhanced activity whereas the oleane-like compounds exhibited more complex properties. Both LU and OA derivatives displayed a pattern of interaction with the NF-κB p65 subunit that justified the similarity in their capability to hinder the p65 binding to DNA. Also, some of the tested derivatives could augment IκB-α levels precluding the NF-κB translocation to the nucleus. This study revealed the pharmacological activity of triterpenes towards leukemia cells, making them valuable anticancer drug candidates.
The different OA and LU derivatives were investigated for their cytotoxic effects against acute myeloid leukemia cancer cell line HL60 as well as its multidrug-resistant (MDR) variant HL60R wherein the latter displayed multidrug resistance, P-gp overexpression, constitutive expression of the NF-κB transcription factor, inhibition of proteins associated with apoptosis and poor prognosis (Fontana et al., 2022). These derivatives showed more activity than the original compounds and displayed equivalent cytotoxic effects against both HL60 and HL60R cell lines. Also, these compounds remarkably disrupted the NF-κB pathway both downstream and upstream to NF-κB activation within the MDR cell line.
All these compounds were able to hinder transcription factor transactivation in the HL60R cell line. Both LU and OA derivatives displayed similar patterns of interaction with the NF-κB p65 unit, that justified the similarity in their potential to inhibit the interaction of p65 to DNA as well as some of its molecular targets. The capability of many of the derivatives to augment IκB-α levels precludes the transcription factor from movement to the nucleus, thus accounting for the indirect effect of NF-κB inhibition. This investigation showed that pre-treatment with OA and LU derivatives could potentiate increased sensitivity of the cancer cells to standard drugs used in chemotherapy, thereby representing novel therapeutic approaches to combat acute myeloid leukemia.
Terpenoids are the largest class of natural compounds and are known for their anticancer activity. (Hussain et al., 2018), explored the anticancer effects of a novel semi-synthetic terpenoid, 16-oxo-cleroda-3,13 (14)E-diene-15-oic acid 1), derived from cleroda diterpene isolated from Polyalthia longifolia var. pendula against neuroblastoma (Faizi et al., 2008). The γ-amino γ-lactone (PGEA-AN, 2) of one was chosen for further study as it displayed the highest cytotoxic activity upon initial screening.
PGEA-AN was shown to modulate the p53 system resulting in death of the neuroblastoma cells while not affecting the renal system making it a promising candidate for anticancer activity against neuroblastoma. Further, PGEA-AN augments mitochondrial membrane permeability (MMP). Induction of P53 in neuroblastomas is common observation associated with chemotherapeutic agents (Nikolaev et al., 2003; Liontas and Yeger, 2004). Notwithstanding the mode of cell death, p53 has been implicated in the mitochondrial pathway leading to cell death resulting from apoptosis or necrosis (Cui et al., 2002; Vaseva et al., 2012). The p53 gene directly upregulates BAX, a proapoptotic gene (Toshiyuki and Reed, 1995). BAX overexpression leads to augmentation of apoptosis mediated by multiple factors (Kobayashi et al., 2000). Activation of BAX results in its translocation into mitochondria which in turn increases MMP (Youle and Strasser, 2008), causing reduction or loss of mitochondrial transmembrane potential, pores in the mitochondrial membrane and promotes necrotic or apoptotic cell death (Tsujimoto and Shimizu, 2007).
Peganum harmala L is a medicinal plant of great importance due to its plenitude of alkaloid content rich in ß-carbolines (Daoud et al., 2014). report the anticancer activity of a semi-synthetic derivative, B-nine to three formed from two harmane molecules that are bound by a butyl group. This compound showed strong potency and anti-proliferative effects against a human colorectal carcinoma cell line, a human breast cancer cell line and a human lung cancer cell line. A dose-dependent elicitation of apoptosis or necroptosis was observed against all these cell lines in addition to repression of cancer cell migration. Also, B-nine to three exhibited anti-angiogenic effects in vitro as observed by the drug-mediated inhibition of tube formation within the human umbilical vascular endothelial cell line (HUVEC).
As per epidemiological findings, chronic inflammation has been implicated in 25% of cancer cases. Hence, blockage of carcinogenesis induced by inflammation could be a viable therapeutic approach for the chemoprevention of cancer. Moreover, anti-inflammatory drugs have been shown to decrease the incidence of cancer. Saponins are natural compounds having tumor inhibitory properties. The triterpenoid derivative of oleanolic acid, 2-cyano-3, 12-dioxooleana-1, 9 (11)-dien-28-oate (CDDO) inhibited NFκB signaling and displayed antitumoral and anti-inflammatory activities, authenticating its therapeutic potential (Place et al., 2003; Shishodia et al., 2006). This effect was also substantiated in murine models of prostate cancer (Gao et al., 2011).
Semi-synthetic analogs of cycloartane-type sapogenols (9,19-cyclolanostanes) were prepared (Debeleç-Bütüner et al., 2018) and five of these analogs were investigated for their anticancer activity. Of these, astragenol derivatives 1 and 2, and the cycloastragenol derivatives 3, 4, and 5 showed strong inhibition of NFκB signaling resulting in the blockage of NFκB transcriptional activation and suppression of cell proliferation. Therefore, these semi-synthetic compounds displayed strong potential for chemoprevention of prostate cancer driven by the inflammatory NFκB signaling pathway.
4 Conclusion
Several synthetic drugs have been used to treat cancer but cause long term effects. This review provides an update on advances in the use of secondary metabolites from different African medicinal plants with anticancer potential on different biological models including human cell lines, animals, and human models. Most of the studies that we assessed employed in vitro assays with some in vivo assays. We report some plants that have contributed to cancer management in Africa, where the anticancer extracts are usually prepared from bark, fruits, leaves, roots, and stems. In vitro and in vivo studies mentioned in this review have shown that bioactive compounds and metabolites from African medicinal plants use a variety of mechanisms during cancer management. Carrying out in vitro studies on anticancer plants is a very important initial step in pharmacological testing. Testing plant phytochemicals on cancer cell lines allow scientists to gauge the anticancer potential of these phytochemicals in a safe and controlled environment of the lab. In vitro studies also allow testing of different combinations of phytochemicals on cancer cells to determine any synergistic advantages. Once the effects of the phytochemicals on cancer cells are determined, the phytochemicals are then tested in vivo using model organisms such as mice and rats. After the efficacy of the phytochemicals is confirmed in vivo, pre-clinical studies are then undertaken to test the phytochemicals on a small population of clinical candidates. If successful, and after approval by appropriate regulatory bodies, the phytochemicals, in drug form, can then be clinically applied in cancer management. Therefore, in vitro, and in vivo studies of potential anticancer phytochemicals from plants play a crucial role in the ultimate clinical application and commercialization of phytochemicals of these plants, providing alternative cancer management strategies to mankind. However, there is insufficient research on other African medicinal plants for potential use in cancer management. Therefore, there is a need to isolate and evaluate the anticancer potential of the bioactive compounds in the understudied medicinal plants of Africa and elucidate their mechanisms of anticancer activity. Furthermore, more research on animal models is required as it can lead to more clinical studies. Despite the anticancer potential of the reviewed African medicinal plants, there is insufficient data on in vivo studies and targets of cancer. In vivo studies are crucial for pre-clinical studies and ultimate drug development. Furthermore, toxicological evidence is insufficient in some of the mentioned studies. In some populations, some medicinal plants may be allergenic or harmful, while in other cases, specific plant parts may either be edible or poisonous. Therefore, toxicology investigations are necessary for the determination of adverse effects of some of the plant extracts. They are also important in the establishment of limits of exposure levels.
Many genes, proteins and signaling pathways play important roles in cancer development, progression, and alleviation. Based on the findings of this manuscript, the following genes and pathways should be investigated for preclinical studies as they are significant to cancer biology; the tumour suppressors p21 and p53 as well as the following pathways: NF-κB, AKT, PI3K, p38-MAPK pathways. In summation, the take home message of this review is that African plants have been shown to have potential anticancer activity. It is important that in vitro studies mentioned in this review are advanced to in vivo investigations and the in vivo studies are elevated to pre-clinical studies. This review has collated information that may be critical in guiding researchers interested in exploring native African plants for use in cancer management. Figure 2 summarize the different pathways on which different plants their compounds and extracts.
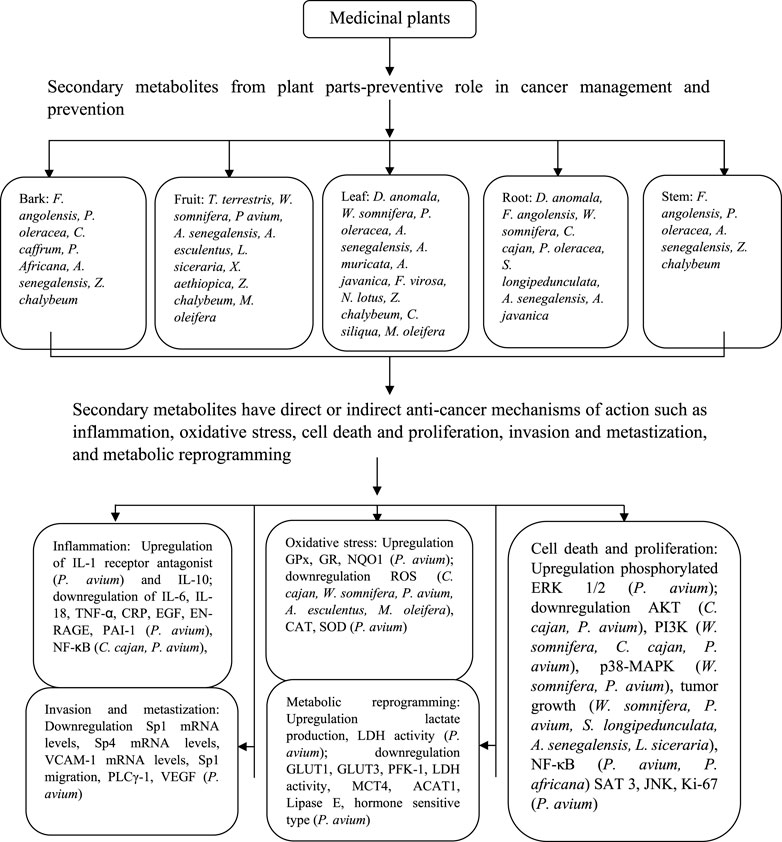
FIGURE 2. Diagrammatic depiction of medicinal plant parts involved in therapeutic effects against various types of cancers and their target pathways.
Author contributions
Conceptualization, GG, KM, and AM; methodology, GG, KM, and AM; writing—original draft preparation, GG, SV, PB, KM, TK, and AM; writing—review and editing, GG, SV, PB, KM, TK, DN, GR, and AM. All authors have read and agreed to the published version of the manuscript.
Acknowledgments
We would like to acknowledge the Botswana International University of Science and Technology (BIUST) and the University of Toronto for providing the resources to complete this manuscript.
Conflict of interest
The authors declare that the research was conducted in the absence of any commercial or financial relationships that could be construed as a potential conflict of interest.
Publisher’s note
All claims expressed in this article are solely those of the authors and do not necessarily represent those of their affiliated organizations, or those of the publisher, the editors and the reviewers. Any product that may be evaluated in this article, or claim that may be made by its manufacturer, is not guaranteed or endorsed by the publisher.
References
Abate, L., Tadesse, M. G., Bachheti, A., and Bachheti, R. K. (2022). Traditional and phytochemical bases of herbs, shrubs, climbers, and trees from Ethiopia for their anticancer response. BioMed Res. Int. 2022, 1589877. doi:10.1155/2022/1589877
Abbas, M. W., Hussain, M., Akhtar, S., Ismail, T., Qamar, M., Shafiq, Z., et al. (2022). Bioactive compounds, antioxidant, anti-inflammatory, anti-cancer, and toxicity assessment of Tribulus terrestris—in vitro and in vivo studies. Antioxidants 11, 1160. doi:10.3390/antiox11061160
Abd-Rabou, A. A., Abdalla, A. M., Ali, N. A., and Zoheir, K. M. (2017). Moringa oleifera root induces cancer apoptosis more effectively than leave nanocomposites and its free counterpart. Asian Pac. J. cancer Prev. APJCP 18, 2141–2149. doi:10.22034/APJCP.2017.18.8.2141
Abebe, W. (2016). An overview of Ethiopian traditional medicinal plants used for cancer treatment. Eur. J. Med. Plants 14, 1–16. doi:10.9734/ejmp/2016/25670
Abutaha, N. (2015). In vitro antiproliferative activity of partially purified Withania somnifera fruit extract on different cancer cell lines. J. Balk. Union Oncol. 20, 625–630.
Acero, N., Gradillas, A., Beltran, M., García, A., and Mingarro, D. M. (2019). Comparison of phenolic compounds profile and antioxidant properties of different sweet cherry (Prunus avium L.) varieties. Food Chem. 279, 260–271. doi:10.1016/j.foodchem.2018.12.008
Adaramoye, O. A., Sarkar, J., Singh, N., Meena, S., Changkija, B., Yadav, P. P., et al. (2011). Antiproliferative action of Xylopia aethiopica fruit extract on human cervical cancer cells. Phytotherapy Res. 25, 1558–1563. doi:10.1002/ptr.3551
Adeloye, D., David, R. A., Aderemi, A. V., Iseolorunkanmi, A., Oyedokun, A., Iweala, E. E., et al. (2016). An estimate of the incidence of prostate cancer in Africa: A systematic review and meta-analysis. PloS one 11, e0153496. doi:10.1371/journal.pone.0153496
Afolayan, A. J., Sharaibi, O. J., and Kazeem, M. I. (2013). Phytochemical analysis and in vitro antioxidant activity of Nymphaea lotus L. Int. J. Pharmacol. 9, 297–304. doi:10.3923/ijp.2013.297.304
Agregán, R., Pateiro, M., Bohrer, B. M., Shariati, M. A., Nawaz, A., Gohari, G., et al. (2022). Biological activity and development of functional foods fortified with okra (Abelmoschus esculentus). Crit. Rev. Food Sci. Nutr., 1–16. doi:10.1080/10408398.2022.2026874
Agu, K. C., and Okolie, P. N. (2017). Proximate composition, phytochemical analysis, and in vitro antioxidant potentials of extracts of Annona muricata (Soursop). Food Sci. Nutr. 5, 1029–1036. doi:10.1002/fsn3.498
Ahmad, R., Fatima, A., Srivastava, A., and Khan, M. A. (2017). Evaluation of apoptotic activity of Withania coagulans methanolic extract against human breast cancer and Vero cell lines. J. Ayurveda Integr. Med. 8, 177–183. doi:10.1016/j.jaim.2017.01.001
Ahmed, B. T., and Kumar, S. A. (2016). Antioxidant and Antidiabetic properties of Abelmoschus esculentus extract–an in vitro assay. Res. J. Biotechnol. 11, 34–41.
Ahmed, H. E., Iqbal, Y., Aziz, M. H., Atif, M., Batool, Z., Hanif, A., et al. (2021). Green synthesis of CeO2 nanoparticles from the Abelmoschus esculentus extract: Evaluation of antioxidant, anticancer, antibacterial, and wound-healing activities. Molecules 26, 4659. doi:10.3390/molecules26154659
Al-Asmari, A. K., Albalawi, S. M., Athar, M. T., Khan, A. Q., Al-Shahrani, H., and Islam, M. (2015). Moringa oleifera as an anti-cancer agent against breast and colorectal cancer cell lines. PloS one 10, e0135814. doi:10.1371/journal.pone.0135814
Al-Dabbagh, B., Elhaty, I. A., Al Hrout, A., Al Sakkaf, R., El-Awady, R., Ashraf, S. S., et al. (2018). Antioxidant and anticancer activities of Trigonella foenum-graecum, Cassia acutifolia and Rhazya stricta. BMC complementary Altern. Med. 18, 240–312. doi:10.1186/s12906-018-2285-7
Alsdorf, W., Seidel, C., Bokemeyer, C., and Oing, C. (2019). Current pharmacotherapy for testicular germ cell cancer. Expert Opin. Pharmacother. 20, 837–850. doi:10.1080/14656566.2019.1583745
Alves-Silva, J. M., Romane, A., Efferth, T., and Salgueiro, L. (2017). North African medicinal plants traditionally used in cancer therapy. Front. Pharmacol. 8, 383. doi:10.3389/fphar.2017.00383
Amin, A., Gali-Muhtasib, H., Ocker, M., and Schneider-Stock, R. (2009). Overview of major classes of plant-derived anticancer drugs. Int. J. Biomed. Sci. IJBS 5, 1–11.
Amiri, F., Zarnani, A.-H., Zand, H., Koohdani, F., Jeddi-Tehrani, M., and Vafa, M. (2013). Synergistic anti-proliferative effect of resveratrol and etoposide on human hepatocellular and colon cancer cell lines. Eur. J. Pharmacol. 718, 34–40. doi:10.1016/j.ejphar.2013.09.020
Anantharaju, P. G., Reddy, B. D., Padukudru, M. A., Kumari Chitturi, C. M., Vimalambike, M. G., and Madhunapantula, S. V. (2017). Naturally occurring benzoic acid derivatives retard cancer cell growth by inhibiting histone deacetylases (HDAC). Cancer Biol. Ther. 18, 492–504. doi:10.1080/15384047.2017.1324374
Angelova, S., Gospodinova, Z., Krasteva, M., Antov, G., Lozanov, V., Markov, T., et al. (2013). Antitumor activity of Bulgarian herb Tribulus terrestris L. on human breast cancer cells. J. Biosci. Biotechnol. 2.
Antognoni, F., Potente, G., Mandrioli, R., Angeloni, C., Freschi, M., Malaguti, M., et al. (2020). Fruit quality characterization of new sweet cherry cultivars as a good source of bioactive phenolic compounds with antioxidant and neuroprotective potential. Antioxidants 9, 677. doi:10.3390/antiox9080677
Arapitsas, P. (2008). Identification and quantification of polyphenolic compounds from okra seeds and skins. Food Chem. 110, 1041–1045. doi:10.1016/j.foodchem.2008.03.014
Ashidi, J., Houghton, P., Hylands, P., and Efferth, T. (2010). Ethnobotanical survey and cytotoxicity testing of plants of South-western Nigeria used to treat cancer, with isolation of cytotoxic constituents from Cajanus cajan Millsp. leaves. J. Ethnopharmacol. 128, 501–512. doi:10.1016/j.jep.2010.01.009
Asita, A. O., Heisi, D. H., and Tjale, T. (2015). Modulation of mutagen-induced genotoxicity by two Lesotho medicinal plants in Allium cepa L. Environ. Nat. Resour. Res. 5, 37. doi:10.5539/enrr.v5n3p37
Atienza, D. M., Vogel, C. L., Trock, B., and Swain, S. M. (1995). Phase II study of oral etoposide for patients with advanced breast cancer. Cancer 76, 2485–2490. doi:10.1002/1097-0142(19951215)76:12<2485::aid-cncr2820761212>3.0.co;2-j
Awad, A. B., Gan, Y., and Fink, C. S. (2000). Effect of β-sitosterol, a plant sterol, on growth, protein phosphatase 2A, and phospholipase D in LNCaP cells. Nutr. cancer 36, 74–78. doi:10.1207/S15327914NC3601_11
Ayele, T. (2018). A review on traditionally used medicinal plants/herbs for cancer therapy in Ethiopia: Current status, challenge and future perspectives. Org. Chem. Curr. Res. 7, 8. doi:10.4172/2161-0401.1000192
Bai, L.-P., Zhao, Z.-Z., Cai, Z., and Jiang, Z.-H. (2006). DNA-binding affinities and sequence selectivity of quaternary benzophenanthridine alkaloids sanguinarine, chelerythrine, and nitidine. Bioorg. Med. Chem. 14, 5439–5445. doi:10.1016/j.bmc.2006.05.012
Bakarnga-Via, I., Hzounda, J. B., Fokou, P. V. T., Tchokouaha, L. R. Y., Gary-Bobo, M., Gallud, A., et al. (2014). Composition and cytotoxic activity of essential oils from Xylopia aethiopica (Dunal) A. Rich, Xylopia parviflora (A. Rich) Benth.) and Monodora myristica (Gaertn) growing in Chad and Cameroon. BMC complementary Altern. Med. 14, 125–128. doi:10.1186/1472-6882-14-125
Bala, A., Kar, B., Haldar, P. K., Mazumder, U. K., and Bera, S. (2010). Evaluation of anticancer activity of Cleome gynandra on Ehrlich's Ascites Carcinoma treated mice. J. Ethnopharmacol. 129, 131–134. doi:10.1016/j.jep.2010.03.010
Balakrishnan, A. S., Nathan, A. A., Kumar, M., Ramamoorthy, S., and Mothilal, S. K. R. (2017). Withania somnifera targets interleukin-8 and cyclooxygenase-2 in human prostate cancer progression. Prostate Int. 5, 75–83. doi:10.1016/j.prnil.2017.03.002
Balama, C., Makatta, A., Maduka, S., and Tewele, C. (2015). Nutrient content of dried leaves of Zanthoxylum chalybeum Engl. growing in semi-arid areas of Iringa region, Tanzania. Tanzan. J. For. Nat. Conservation 84.
Balogun, F., and Ashafa, A. (2017). Aqueous root extracts of Dicoma anomala (Sond.) extenuates postprandial hyperglycaemia in vitro and its modulation on the activities of carbohydrate-metabolizing enzymes in streptozotocin-induced diabetic Wistar rats. South Afr. J. Bot. 112, 102–111. doi:10.1016/j.sajb.2017.05.014
Barhoi, D., Upadhaya, P., Barbhuiya, S. N., Giri, A., and Giri, S. (2021). Aqueous extract of moringa oleifera exhibit potential anticancer activity and can be used as a possible cancer therapeutic agent: A study involving in vitro and in vivo approach. J. Am. Coll. Nutr. 40, 70–85. doi:10.1080/07315724.2020.1735572
Benarba, B., and Pandiella, A. (2018). Colorectal cancer and medicinal plants: Principle findings from recent studies. Biomed. Pharmacother. 107, 408–423. doi:10.1016/j.biopha.2018.08.006
Bioltif, Y. E., Edward, N. B., and Tyeng, T. D. (2020). A chemical overview of Azanza garckeana. Biol. Med. Nat. Prod. Chem. 9, 91–95. doi:10.14421/biomedich.2020.92.91-95
Bleckmann, A., Conradi, L.-C., Menck, K., Schmick, N. A., Schubert, A., Rietkötter, E., et al. (2016). β-catenin-independent WNT signaling and Ki67 in contrast to the estrogen receptor status are prognostic and associated with poor prognosis in breast cancer liver metastases. Clin. Exp. metastasis 33, 309–323. doi:10.1007/s10585-016-9780-3
Bray, F., Laversanne, M., Weiderpass, E., and Soerjomataram, I. (2021). The ever-increasing importance of cancer as a leading cause of premature death worldwide. Cancer 127, 3029–3030. doi:10.1002/cncr.33587
Bray, F., Parkin, D. M., Gnangnon, F., Tshisimogo, G., Peko, J.-F., Adoubi, I., et al. (2022). Cancer in sub-saharan Africa in 2020: A review of current estimates of the national burden, data gaps, and future needs. Lancet Oncol. 23, 719–728. doi:10.1016/S1470-2045(22)00270-4
Brown, A. C., Reitzenstein, J. E., Liu, J., and Jadus, M. R. (2005). The anti-cancer effects of poi (Colocasia esculenta) on colonic adenocarcinoma cells in vitro. Phytotherapy Res. An Int. J. Devoted Pharmacol. Toxicol. Eval. Nat. Prod. Deriv. 19, 767–771. doi:10.1002/ptr.1712
Chen, W.-Y., Chang, F.-R., Huang, Z.-Y., Chen, J.-H., Wu, Y.-C., and Wu, C.-C. (2008). Tubocapsenolide A, a novel withanolide, inhibits proliferation and induces apoptosis in MDA-MB-231 cells by thiol oxidation of heat shock proteins. J. Biol. Chem. 283, 17184–17193. doi:10.1074/jbc.M709447200
Choumessi, A. T., Loureiro, R., Silva, A. M., Moreira, A. C., Pieme, A. C., Tazoacha, A., et al. (2012). Toxicity evaluation of some traditional African spices on breast cancer cells and isolated rat hepatic mitochondria. Food Chem. Toxicol. 50, 4199–4208. doi:10.1016/j.fct.2012.08.008
Christensen, D. K., Armaiz-Pena, G. N., Ramirez, E., Matsuo, K., Zimmerman, B., Zand, B., et al. (2016). SSRI use and clinical outcomes in epithelial ovarian cancer. Oncotarget 7, 33179–33191. doi:10.18632/oncotarget.8891
Cui, H., Schroering, A., and Ding, H.-F. (2002). p53 mediates DNA damaging drug-induced apoptosis through a caspase-9-dependent pathway in SH-SY5Y neuroblastoma cells. Mol. cancer Ther. 1, 679–686.
Cunningham, A., Anoncho, V., and Sunderland, T. (2016). Power, policy and the Prunus africana bark trade, 1972–2015. J. Ethnopharmacol. 178, 323–333. doi:10.1016/j.jep.2015.11.042
Custódio, L., Escapa, A. L., Patarra, J., Aligué, R., Alberício, F., Neng, N. R., et al. (2013). Sapwood of carob tree (Ceratonia siliqua L.) as a potential source of bioactive compounds. Rec. Nat. Prod. 7, 225–229.
Da Silva, A. J., Buarque, C. D., Brito, F. V., Aurelian, L., Macedo, L. F., Malkas, L. H., et al. (2002). Synthesis and preliminary pharmacological evaluation of new (+/-) 1,4-naphthoquinones structurally related to lapachol. Bioorg. Med. Chem. 10, 2731–2738. doi:10.1016/s0968-0896(02)00100-1
Daoud, A., Song, J., Xiao, F., and Shang, J. (2014). B-9-3, a novel β-carboline derivative exhibits anti-cancer activity via induction of apoptosis and inhibition of cell migration in vitro. Eur. J. Pharmacol. 724, 219–230. doi:10.1016/j.ejphar.2013.12.038
De Sousa Ferreira Soares, G., Assreuy, A. M. S., De Almeida Gadelha, C. A., De Morais Gomes, V., Delatorre, P., Da Conceição Simões, R., et al. (2012). Purification and biological activities of Abelmoschus esculentus seed lectin. protein J. 31, 674–680. doi:10.1007/s10930-012-9447-0
Debeleç-Bütüner, B., Öztürk, M. B., Tağ, Ö., Akgün, İ. H., Yetik-Anacak, G., Bedir, E., et al. (2018). Cycloartane-type sapogenol derivatives inhibit NFκB activation as chemopreventive strategy for inflammation-induced prostate carcinogenesis. Steroids 135, 9–20. doi:10.1016/j.steroids.2018.04.005
Devanesan, S., and Alsalhi, M. S. (2021). Green synthesis of silver nanoparticles using the flower extract of Abelmoschus esculentus for cytotoxicity and antimicrobial studies. Int. J. Nanomedicine 16, 3343–3356. doi:10.2147/IJN.S307676
Dibwe, D. F., Awale, S., Kadota, S., Morita, H., and Tezuka, Y. (2014). Muchimangins G–J, fully substituted xanthones with a diphenylmethyl substituent, from Securidaca longepedunculata. J. Nat. Prod. 77, 1241–1244. doi:10.1021/np5000445
Donhouedé, J. C., Salako, K. V., Gandji, K., Idohou, R., Tohoun, R., Hounkpèvi, A., et al. (2022). Food and medicinal uses of Annona senegalensis pers.: A country-wide assessment of traditional theoretical knowledge and actual uses in Benin, west Africa. J. Ethnobiol. ethnomedicine 18, 10–15. doi:10.1186/s13002-022-00510-2
Dziadek, K., Kopeć, A., Piątkowska, E., and Leszczyńska, T. (2019). High-fructose diet-induced metabolic disorders were counteracted by the intake of fruit and leaves of sweet cherry in wistar rats. Nutrients 11, 2638. doi:10.3390/nu11112638
Economides, M. P., Mccue, D., Borthakur, G., and Pemmaraju, N. (2019). Topoisomerase II inhibitors in AML: Past, present, and future. Expert Opin. Pharmacother. 20, 1637–1644. doi:10.1080/14656566.2019.1621292
El-Seedi, H. R., Burman, R., Mansour, A., Turki, Z., Boulos, L., Gullbo, J., et al. (2013). The traditional medical uses and cytotoxic activities of sixty-one Egyptian plants: Discovery of an active cardiac glycoside from urginea maritima. J. Ethnopharmacol. 145, 746–757. doi:10.1016/j.jep.2012.12.007
Elegami, A. A., Bates, C., Gray, A. I., Mackay, S. P., Skellern, G. G., and Waigh, R. D. (2003). Two very unusual macrocyclic flavonoids from the water lily Nymphaea lotus. Phytochemistry 63, 727–731. doi:10.1016/s0031-9422(03)00238-3
Eritja, N., Chen, B.-J., Rodríguez-Barrueco, R., Santacana, M., Gatius, S., Vidal, A., et al. (2017). Autophagy orchestrates adaptive responses to targeted therapy in endometrial cancer. Autophagy 13, 608–624. doi:10.1080/15548627.2016.1271512
Ermakova, S. P., Kang, B. S., Choi, B. Y., Choi, H. S., Schuster, T. F., and Ma, W. Y. (2006). (-)-Epigallocatechin gallate overcomes resistance to etoposide-induced cell death by targeting the molecular chaperone glucose-regulated protein 78. Cancer Res. 66, 9260–9269.
Faizal, A., and Geelen, D. (2013). Saponins and their role in biological processes in plants. Phytochem. Rev. 12, 877–893. doi:10.1007/s11101-013-9322-4
Faizi, S., Khan, R. A., Mughal, N. R., Malik, M. S., Sajjadi, K. E. S., and Ahmad, A. (2008). Antimicrobial activity of various parts of Polyalthia longifolia var. pendula: Isolation of active principles from the leaves and the berries. Phytotherapy Res. 22, 907–912. doi:10.1002/ptr.2414
Fang, X. P., Rieser, M. J., Gu, Z. M., Zhao, G. X., and Mclaughlin, J. L. (1993). Annonaceous acetogenins: An updated review. Phytochem. Anal. 4, 27–48. doi:10.1002/pca.2800040108
Ferreira De Oliveira, J. M. P., Pacheco, A. R., Coutinho, L., Oliveira, H., Pinho, S., Almeida, L., et al. (2018). Combination of etoposide and fisetin results in anti-cancer efficiency against osteosarcoma cell models. Archives Toxicol. 92, 1205–1214. doi:10.1007/s00204-017-2146-z
Fiorito, S., Epifano, F., Bruyère, C., Mathieu, V., Kiss, R., and Genovese, S. (2014). Growth inhibitory activity for cancer cell lines of lapachol and its natural and semi-synthetic derivatives. Bioorg. Med. Chem. Lett. 24, 454–457. doi:10.1016/j.bmcl.2013.12.049
Fontana, G., Badalamenti, N., Bruno, M., Castiglione, D., Notarbartolo, M., Poma, P., et al. (2022). Synthesis, in vitro and in silico analysis of new oleanolic acid and lupeol derivatives against leukemia cell lines: Involvement of the NF-κB pathway. Int. J. Mol. Sci. 23, 6594. doi:10.3390/ijms23126594
Foster, K., Oyenihi, O., Rademan, S., Erhabor, J., Matsabisa, M., Barker, J., et al. (2020). Selective cytotoxic and anti-metastatic activity in DU-145 prostate cancer cells induced by Annona muricata L. bark extract and phytochemical, annonacin. BMC Complementary Med. Ther. 20, 375–415. doi:10.1186/s12906-020-03130-z
Foster, P. A., Newman, S. P., Leese, M. P., Bernetiere, S., Diolez, C., Camara, J., et al. (2008). A new micronized formulation of 2-methoxyestradiol-bis-sulfamate (STX140) is therapeutically potent against breast cancer. Anticancer Res. 28, 577–581.
Fu, Y., Kadioglu, O., Wiench, B., Wei, Z., Wang, W., Luo, M., et al. (2015). Activity of the antiestrogenic cajanin stilbene acid towards breast cancer. J. Nutr. Biochem. 26, 1273–1282. doi:10.1016/j.jnutbio.2015.06.004
Fulda, S., and Debatin, K.-M. (2006). Extrinsic versus intrinsic apoptosis pathways in anticancer chemotherapy. Oncogene 25, 4798–4811. doi:10.1038/sj.onc.1209608
Galluzzi, L., Pedro, B.-S., Manuel, J., Demaria, S., Formenti, S. C., and Kroemer, G. (2017). Activating autophagy to potentiate immunogenic chemotherapy and radiation therapy. Nat. Rev. Clin. Oncol. 14, 247–258. doi:10.1038/nrclinonc.2016.183
Gao, X., Deeb, D., Liu, Y., Arbab, A. S., Divine, G. W., Dulchavsky, S. A., et al. (2011). Prevention of prostate cancer with oleanane synthetic triterpenoid CDDO-Me in the TRAMP mouse model of prostate cancer. Cancers 3, 3353–3369. doi:10.3390/cancers3033353
Gaobotse, G., Trémouillaux-Guiller, J., Venkataraman, S., Sherif, M. K., Makhzoum, A., and Hefferon, K. (2022a). “Plant molecular pharming of biologics to combat HIV,” in Applications in plant biotechnology (CRC Press), 296–348.
Gaobotse, G., Venkataraman, S., Mmereke, K. M., Moustafa, K., Hefferon, K., and Makhzoum, A. (2022b). Recent progress on vaccines produced in transgenic plants. Vaccines 10, 1861. doi:10.3390/vaccines10111861
Garrido, M., Paredes, S. D., Cubero, J., Lozano, M., Toribio-Delgado, A. F., Muñoz, J. L., et al. (2010). Jerte Valley cherry-enriched diets improve nocturnal rest and increase 6-sulfatoxymelatonin and total antioxidant capacity in the urine of middle-aged and elderly humans. Journals Gerontology Ser. A Biomed. Sci. Med. Sci. 65, 909–914. doi:10.1093/gerona/glq099
Gaurav, N., Kumar, A., Tyagi, M., Kumar, D., Chauhan, U., and Singh, A. (2015). Morphology of Withania somnifera (distribution, morphology, phytosociology of Withania somnifera L. Dunal). Int. J. Curr. Sci. Res. 1, 164–173.
Gavamukulya, Y., Abou-Elella, F., Wamunyokoli, F., and Ael-Shemy, H. (2014). Phytochemical screening, anti-oxidant activity and in vitro anticancer potential of ethanolic and water leaves extracts of Annona muricata (Graviola). Asian Pac. J. Trop. Med. 7, S355–S363. doi:10.1016/S1995-7645(14)60258-3
Gemede, H. F., Ratta, N., Haki, G. D., Woldegiorgis, A. Z., and Beyene, F. (2015). Nutritional quality and health benefits of okra (Abelmoschus esculentus): A review. J. Food Process Technol. 6, 2. doi:10.4172/2157-7110.1000458
Ghanemi, F. Z., Belarbi, M., Fluckiger, A., Nani, A., Dumont, A., De Rosny, C., et al. (2017). Carob leaf polyphenols trigger intrinsic apoptotic pathway and induce cell cycle arrest in colon cancer cells. J. Funct. Foods 33, 112–121. doi:10.1016/j.jff.2017.03.032
Gleye, C., Duret, P., Laurens, A., Hocquemiller, R., and Cavé, A. (1998). cis-Monotetrahydrofuran acetogenins from the roots of Annona muricata. J. Nat. Prod. 61, 576–579. doi:10.1021/np970494m
Grace, O., Prendergast, H., Jäger, A., Van Staden, J., and Van Wyk, A. (2003). Bark medicines used in traditional healthcare in KwaZulu-Natal, South Africa: An inventory. South Afr. J. Bot. 69, 301–363. doi:10.1016/s0254-6299(15)30318-5
Greenwell, M., and Rahman, P. (2015). Medicinal plants: Their use in anticancer treatment. Int. J. Pharm. Sci. Res. 6, 4103–4112. doi:10.13040/IJPSR.0975-8232.6(10).4103-12
Gunarathne, R., Nadeeshani, H., Lu, A., Li, J., Zhang, B., Ying, T., et al. (2022). Potential nutraceutical use of Tribulus terrestris L. In human health. Food Rev. Int., 1–30. doi:10.1080/87559129.2022.2067172
Hadisaputri, Y. E., Habibah, U., Abdullah, F. F., Halimah, E., Mutakin, M., Megantara, S., et al. (2021). Antiproliferation activity and apoptotic mechanism of soursop (Annona muricata l.) leaves extract and fractions on mcf7 breast cancer cells. Breast Cancer Targets Ther. 13, 447–457. doi:10.2147/BCTT.S317682
Hahm, E. R., Lee, J., and Singh, S. V. (2014). Role of mitogen-activated protein kinases and Mcl-1 in apoptosis induction by withaferin A in human breast cancer cells. Mol. Carcinog. 53, 907–916. doi:10.1002/mc.22050
Hashim, S., Bakht, T., Marwat, K. B., and Jan, A. (2014). Medicinal properties, phytochemistry and pharmacology of Tribulus terrestris L.(Zygophyllaceae). Pak J. Bot. 46, 399–404.
Heiduschka, G., Bigenzahn, J., Brunner, M., and Thurnher, D. (2014). Resveratrol synergistically enhances the effect of etoposide in HNSCC cell lines. Acta oto-laryngologica 134, 1071–1078. doi:10.3109/00016489.2014.888592
Henley, A. B., Yang, L., Chuang, K.-L., Sahuri-Arisoylu, M., Wu, L.-H., Bligh, S. A., et al. (2017). Withania somnifera root extract enhances chemotherapy through ‘Priming. PLoS One 12, e0170917. doi:10.1371/journal.pone.0170917
Huang, W., Yan, Z., Li, D., Ma, Y., Zhou, J., and Sui, Z. (2018). Antioxidant and anti-inflammatory effects of blueberry anthocyanins on high glucose-induced human retinal capillary endothelial cells. Oxidative Med. Cell. Longev. 2018, 1862462. doi:10.1155/2018/1862462
Hussain, S. S., Rafi, K., Faizi, S., Razzak, Z. A., and Simjee, S. U. (2018). A novel, semi-synthetic diterpenoid 16 (R and S)-phenylamino-cleroda-3, 13 (14), Z-dien-15, 16 olide (PGEA-AN) inhibits the growth and cell survival of human neuroblastoma cell line SH-SY5Y by modulating P53 pathway. Mol. Cell. Biochem. 449, 105–115. doi:10.1007/s11010-018-3347-3
Hwang, J. T., Kwak, D. W., Lin, S. K., Kim, H. M., Kim, Y. M., and Park, O. J. (2007). Resveratrol induces apoptosis in chemoresistant cancer cells via modulation of AMPK signaling pathway. Ann. N. Y. Acad. Sci. 1095, 441–448. doi:10.1196/annals.1397.047
Iyer, S., Chaplin, D. J., Rosenthal, D. S., Boulares, A. H., Li, L.-Y., and Smulson, M. E. (1998). Induction of apoptosis in proliferating human endothelial cells by the tumor-specific antiangiogenesis agent combretastatin A-4. Cancer Res. 58, 4510–4514.
Izuegbuna, O. (2022). Leukemia chemoprevention and therapeutic potentials: Selected medicinal plants with anti-leukemic activities. Nutr. Cancer 74, 437–449. doi:10.1080/01635581.2021.1924209
Jabir, M. S., Saleh, Y. M., Sulaiman, G. M., Yaseen, N. Y., Sahib, U. I., Dewir, Y. H., et al. (2021). Green synthesis of silver nanoparticles using Annona muricata extract as an inducer of apoptosis in cancer cells and inhibitor for NLRP3 inflammasome via enhanced autophagy. Nanomaterials 11, 384. doi:10.3390/nano11020384
Jaroch, K., Karolak, M., Górski, P., Jaroch, A., Krajewski, A., Ilnicka, A., et al. (2016). Combretastatins: In vitro structure-activity relationship, mode of action and current clinical status. Pharmacol. Rep. 68, 1266–1275. doi:10.1016/j.pharep.2016.08.007
Jia, G., Shao, X., Zhao, R., Zhang, T., Zhou, X., Yang, Y., et al. (2021). Portulaca oleracea L. polysaccharides enhance the immune efficacy of dendritic cell vaccine for breast cancer. Food & Funct. 12, 4046–4059. doi:10.1039/d0fo02522d
Jia, H., Yang, Q., Wang, T., Cao, Y., Jiang, Q.-Y., Sun, H.-W., et al. (2016). Rhamnetin induces sensitization of hepatocellular carcinoma cells to a small molecular kinase inhibitor or chemotherapeutic agents. Biochimica Biophysica Acta (BBA)-General Subj. 1860, 1417–1430. doi:10.1016/j.bbagen.2016.04.007
Johnson, T. O., Olatunde, A., and Nwachukwu, L. (2017). Phytochemical composition of Annona senegalensis leaf and its antioxidant activity during Trypanosoma brucei brucei induced oxidative stress in mice. J. Pharm. Bioresour. 14, 219–227.
Kabbaj, F., Meddah, B., Cherrah, Y., and Faouzi, E. (2012). Ethnopharmacological profile of traditional plants used in Morocco by cancer patients as herbal therapeutics. Phytopharmacology 2, 243–256.
Kaderi, M., Ben Hamouda, G., Zaeir, H., Hanana, M., and Hamrouni, L. (2015). Notes ethnobotanique et phytopharmacologique sur Ceratonia siliqua (L.). Phytothérapie 13, 144–147. doi:10.1007/s10298-014-0904-4
Karatoprak, G. Ş., Küpeli Akkol, E., Genç, Y., Bardakcı, H., Yücel, Ç., and Sobarzo-Sánchez, E. (2020). Combretastatins: An overview of structure, probable mechanisms of action and potential applications. Molecules 25, 2560. doi:10.3390/molecules25112560
Kataria, H., Shah, N., Kaul, S. C., Wadhwa, R., and Kaur, G. (2011). Water extract of ashwagandha leaves limits proliferation and migration, and induces differentiation in glioma cells. Evidence-Based Complementary Altern. Med. 2011, 267614. doi:10.1093/ecam/nep188
Kaur, H. (2015). Shantanu,“Anticancer activity of a constituent from Moringa oleifera leaves. J. Chem. Pharm. Res. 7, 701–705.
Kelley, D. S., Adkins, Y., Reddy, A., Woodhouse, L. R., Mackey, B. E., and Erickson, K. L. (2013). Sweet bing cherries lower circulating concentrations of markers for chronic inflammatory diseases in healthy humans. J. Nutr. 143, 340–344. doi:10.3945/jn.112.171371
Kelley, D. S., Rasooly, R., Jacob, R. A., Kader, A. A., and Mackey, B. E. (2006). Consumption of Bing sweet cherries lowers circulating concentrations of inflammation markers in healthy men and women. J. Nutr. 136, 981–986. doi:10.1093/jn/136.4.981
Khan, F., Pandey, P., Ahmad, V., and Upadhyay, T. K. (2020). Moringa oleifera methanolic leaves extract induces apoptosis and G0/G1 cell cycle arrest via downregulation of Hedgehog Signaling Pathway in human prostate PC-3 cancer cells. J. Food Biochem. 44, e13338. doi:10.1111/jfbc.13338
Kim, H. J., Kim, J. C., Min, J. S., Kim, M.-J., Kim, J. A., Kor, M. H., et al. (2011). Aqueous extract of Tribulus terrestris Linn induces cell growth arrest and apoptosis by down-regulating NF-κB signaling in liver cancer cells. J. Ethnopharmacol. 136, 197–203. doi:10.1016/j.jep.2011.04.060
Klein-Júnior, L. C., Campos, A., Niero, R., Corrêa, R., Vander Heyden, Y., and Filho, V. C. (2020). Xanthones and cancer: From natural sources to mechanisms of action. Chem. Biodivers. 17, e1900499. doi:10.1002/cbdv.201900499
Kluska, M., and Woźniak, K. (2021). Natural polyphenols as modulators of etoposide anti-cancer activity. Int. J. Mol. Sci. 22, 6602. doi:10.3390/ijms22126602
Kobayashi, T., Sawa, H., Morikawa, J., Zhang, W., and Shiku, H. (2000). Bax induction activates apoptotic cascade via mitochondrial cytochrome c release and Bax overexpression enhances apoptosis induced by chemotherapeutic agents in DLD-1 colon cancer cells. Jpn. J. cancer Res. 91, 1264–1268. doi:10.1111/j.1349-7006.2000.tb00913.x
Kode, J., Kovvuri, J., Nagaraju, B., Jadhav, S., Barkume, M., Sen, S., et al. (2020). Synthesis, biological evaluation, and molecular docking analysis of phenstatin based indole linked chalcones as anticancer agents and tubulin polymerization inhibitors. Bioorg. Chem. 105, 104447. doi:10.1016/j.bioorg.2020.104447
Komakech, R., and Kang, Y. (2019). Ethnopharmacological potential of African cherry [Prunus africana]. J. Herb. Med. 17, 100283. doi:10.1016/j.hermed.2019.100283
Komakech, R., Yim, N.-H., Shim, K.-S., Jung, H., Byun, J.-E., Lee, J., et al. (2022). Root extract of a micropropagated Prunus africana medicinal plant induced apoptosis in human prostate cancer cells (PC-3) via caspase-3 activation. Evidence-Based Complementary Altern. Med. 2022, 8232851. doi:10.1155/2022/8232851
Kuete, V., Sandjo, L. P., Mbaveng, A. T., Zeino, M., and Efferth, T. (2015). Cytotoxicity of compounds from Xylopia aethiopica towards multi-factorial drug-resistant cancer cells. Phytomedicine 22, 1247–1254. doi:10.1016/j.phymed.2015.10.008
Kumar, N., Kale, R. K., and Tiku, A. B. (2013). Chemopreventive effect of Lagenaria siceraria in two stages DMBA plus croton oil induced skin papillomagenesis. Nutr. cancer 65, 991–1001. doi:10.1080/01635581.2013.814800
Kundu, N., Campbell, P., Hampton, B., Lin, C.-Y., Ma, X., Ambulos, N., et al. (2012). Antimetastatic activity isolated from Colocasia esculenta (taro). Anti-cancer drugs 23, 200–211. doi:10.1097/CAD.0b013e32834b85e8
Kuo, C.-Y., Zupkó, I., Chang, F.-R., Hunyadi, A., Wu, C.-C., Weng, T.-S., et al. (2016). Dietary flavonoid derivatives enhance chemotherapeutic effect by inhibiting the DNA damage response pathway. Toxicol. Appl. Pharmacol. 311, 99–105. doi:10.1016/j.taap.2016.09.019
Laszczyk, M. N. (2009). Pentacyclic triterpenes of the lupane, oleanane and ursane group as tools in cancer therapy. Planta medica. 75, 1549–1560. doi:10.1055/s-0029-1186102
Lawal, R., Ozaslan, M., Odesanmi, O., Karagoz, I., Kilic, I., and Ebuehi, O. (2012). Cytotoxic and antiproliferative activity of Securidaca longepedunculata aqueous extract on Ehrlich ascites carcinoma cells in Swiss albino mice.
Layosa, M. a. A., Lage, N. N., Chew, B. P., Atienza, L., Mertens-Talcott, S., Talcott, S., et al. (2021). Dark sweet cherry (Prunus avium) phenolics enriched in anthocyanins induced apoptosis in MDA-MB-453 breast cancer cells through MAPK-dependent signaling and reduced invasion via akt and plcγ-1 downregulation. Nutr. Cancer 73, 1985–1997. doi:10.1080/01635581.2020.1817514
Leyon, P., and Kuttan, G. (2004). Effect of Withania somnifera on B16F-10 melanoma induced metastasis in mice. Phytotherapy Res. An Int. J. Devoted Pharmacol. Toxicol. Eval. Nat. Prod. Deriv. 18, 118–122. doi:10.1002/ptr.1378
Li, A.-N., Li, S., Zhang, Y.-J., Xu, X.-R., Chen, Y.-M., and Li, H.-B. (2014). Resources and biological activities of natural polyphenols. Nutrients 6, 6020–6047. doi:10.3390/nu6126020
Li, Z.-M., Jiang, W.-Q., Zhu, Z.-Y., Zhu, X.-F., Zhou, J.-M., Liu, Z.-C., et al. (2008). Synergistic cytotoxicity of Bcl-xL inhibitor, gossypol and chemotherapeutic agents in non-Hodgkin’s lymphoma cells. Cancer Biol. Ther. 7, 51–60. doi:10.4161/cbt.7.1.5128
Liontas, A., and Yeger, H. (2004). Curcumin and resveratrol induce apoptosis and nuclear translocation and activation of p53 in human neuroblastoma. Anticancer Res. 24, 987–998.
Liu, X., Wu, H., Tao, X., Ying, X., and Stien, D. (2021). Two amide glycosides from Portulaca oleracea L. and its bioactivities. Nat. Prod. Res. 35, 2655–2659. doi:10.1080/14786419.2019.1660333
Luetragoon, T., Pankla Sranujit, R., Noysang, C., Thongsri, Y., Potup, P., Suphrom, N., et al. (2020). Anti-Cancer effect of 3-Hydroxy-β-Ionone identified from moringa oleifera Lam. Leaf on human squamous cell carcinoma 15 cell line. Molecules 25, 3563. doi:10.3390/molecules25163563
Luo, M., Liu, X., Zu, Y., Fu, Y., Zhang, S., Yao, L., et al. (2010). Cajanol, a novel anticancer agent from Pigeonpea [Cajanus cajan (L.) Millsp.] roots, induces apoptosis in human breast cancer cells through a ROS-mediated mitochondrial pathway. Chemico-Biological Interact. 188, 151–160. doi:10.1016/j.cbi.2010.07.009
Mahbub, A., Le Maitre, C., Haywood-Small, S., Cross, N., and Jordan-Mahy, N. (2015). Polyphenols act synergistically with doxorubicin and etoposide in leukaemia cell lines. Cell death Discov. 1, 15043–15112. doi:10.1038/cddiscovery.2015.43
Makhzoum, A., and Hefferon, K. (2022). Applications in plant biotechnology: Focus on plant secondary metabolism and plant molecular pharming. CRC Press.
Maroyi, A. (2018). Dicoma anomala sond.: A review of its botany, ethnomedicine, phytochemistry and pharmacology. Asian J. Pharm. Clin. Res. 11, 70–77. doi:10.22159/ajpcr.2018.v11i6.25538
Matias, A. A., Rosado-Ramos, R., Nunes, S. L., Figueira, I., Serra, A. T., Bronze, M. R., et al. (2016). Protective effect of a (poly) phenol-rich extract derived from sweet cherries culls against oxidative cell damage. Molecules 21, 406. doi:10.3390/molecules21040406
Mcgown, A. T., and Fox, B. W. (1990). Differential cytotoxicity of combretastatins A1 and A4 in two daunorubicin-resistant P388 cell lines. Cancer Chemother. Pharmacol. 26, 79–81. doi:10.1007/BF02940301
Michael, K., Onyia, L., and Jidauna, S. (2015). Evaluation of phytochemicals in Azanza garckeana (Gorontula) seed. J. Agric. Veterinary Sci. 8, 71–74.
Misonge, O. J., Kamindu, G. N., Sabina, W., and Muita, G. (2019). An ethnobotanical survey of plants used for the treatment and management of cancer in Embu County, Kenya. J. Med. Plants 7, 39–46.
Mitaine-Offer, A.-C., Pénez, N., Miyamoto, T., Delaude, C., Mirjolet, J.-F., Duchamp, O., et al. (2010). Acylated triterpene saponins from the roots of Securidaca longepedunculata. Phytochemistry 71, 90–94. doi:10.1016/j.phytochem.2009.09.022
Mondal, S., and Swamy, M. J. (2020). Purification, biochemical/biophysical characterization and chitooligosaccharide binding to BGL24, a new PP2-type phloem exudate lectin from bottle gourd (Lagenaria siceraria). Int. J. Biol. Macromol. 164, 3656–3666. doi:10.1016/j.ijbiomac.2020.08.246
Monte, L. G., Santi-Gadelha, T., Reis, L. B., Braganhol, E., Prietsch, R. F., Dellagostin, O. A., et al. (2014). Lectin of Abelmoschus esculentus (okra) promotes selective antitumor effects in human breast cancer cells. Biotechnol. Lett. 36, 461–469. doi:10.1007/s10529-013-1382-4
Musa, D., Anakaa, T., and Bashir, K. (2017). Phytochemical analysis of crude extracts from Annona senegalensis and its antisnake venom potential on albino rats. FUDMA J. Sci. (FJS) Maiden Ed. 1, 139–142.
Musthafa, S. A., Muthu, K., Vijayakumar, S., George, S. J., Murali, S., Govindaraj, J., et al. (2021). Lectin isolated from Abelmoschus esculentus induces caspase mediated apoptosis in human U87 glioblastoma cell lines and modulates the expression of circadian clock genes. Toxicon 202, 98–109. doi:10.1016/j.toxicon.2021.08.025
Nabha, S. M., Mohammad, R. M., Wall, N. R., Dutcher, J. A., Salkini, B. M., Pettit, G. R., et al. (2001). Evaluation of combretastatin A-4 prodrug in a non-hodgkin's lymphoma xenograft model: Preclinical efficacy. Anti-cancer drugs 12, 57–63. doi:10.1097/00001813-200101000-00008
Naik, A. V., and Sellappan, K. (2020). In vitro evaluation of Annona muricata L.(Soursop) leaf methanol extracts on inhibition of tumorigenicity and metastasis of breast cancer cells. Biomarkers 25, 701–710. doi:10.1080/1354750X.2020.1836025
Nam, N.-H. (2003). Combretastatin A-4 analogues as antimitotic antitumor agents. Curr. Med. Chem. 10, 1697–1722. doi:10.2174/0929867033457151
N’guessan, B. B., Asiamah, A. D., Arthur, N. K., Frimpong-Manso, S., Amoateng, P., Amponsah, S. K., et al. (2021). Ethanolic extract of Nymphaea lotus L.(Nymphaeaceae) leaves exhibits in vitro antioxidant, in vivo anti-inflammatory and cytotoxic activities on Jurkat and MCF-7 cancer cell lines. BMC Complementary Med. Ther. 21, 22–13. doi:10.1186/s12906-020-03195-w
Ngulde, S. I., Sandabe, U. K., Abounader, R., Dawson, T. K., Zhang, Y., Iliya, I., et al. (2019). Ethanol extract of Securidaca longipedunculata induces apoptosis in brain tumor (U87) cells. BioMed Res. Int. 2019, 9826590. doi:10.1155/2019/9826590
Nihei, Y., Suga, Y., Morinaga, Y., Ohishi, K., Okano, A., Ohsumi, K., et al. (1999). A novel combretastatin A-4 derivative, AC-7700, shows marked antitumor activity against advanced solid tumors and orthotopically transplanted tumors. Jpn. J. cancer Res. 90, 1016–1025. doi:10.1111/j.1349-7006.1999.tb00850.x
Nikolaev, A. Y., Li, M., Puskas, N., Qin, J., and Gu, W. (2003). Parc: A cytoplasmic anchor for p53. Cell 112, 29–40. doi:10.1016/s0092-8674(02)01255-2
Nkwe, D. O., Lotshwao, B., Rantong, G., Matshwele, J., Kwape, T. E., Masisi, K., et al. (2021). Anticancer mechanisms of bioactive compounds from Solanaceae: An update. Cancers (Basel) 13, 4989. doi:10.3390/cancers13194989
Noda, C., He, J., Takano, T., Tanaka, C., Kondo, T., Tohyama, K., et al. (2007). Induction of apoptosis by epigallocatechin-3-gallate in human lymphoblastoid B cells. Biochem. biophysical Res. Commun. 362, 951–957. doi:10.1016/j.bbrc.2007.08.079
Noratto, G., Layosa, M. A., Lage, N. N., Atienza, L., Ivanov, I., Mertens-Talcott, S. U., et al. (2020). Antitumor potential of dark sweet cherry sweet (Prunus avium) phenolics in suppressing xenograft tumor growth of MDA-MB-453 breast cancer cells. J. Nutr. Biochem. 84, 108437. doi:10.1016/j.jnutbio.2020.108437
Noronha, V., Sekhar, A., Patil, V. M., Menon, N., Joshi, A., Kapoor, A., et al. (2020). Systemic therapy for limited stage small cell lung carcinoma. J. Thorac. Dis. 12, 6275–6290. doi:10.21037/jtd-2019-sclc-11
Ntie-Kang, F., Zofou, D., Babiaka, S. B., Meudom, R., Scharfe, M., Lifongo, L. L., et al. (2013). AfroDb: A select highly potent and diverse natural product library from african medicinal plants. PloS one 8, e78085. doi:10.1371/journal.pone.0078085
Obasi, T. C., Braicu, C., Iacob, B. C., Bodoki, E., Jurj, A., Raduly, L., et al. (2018). Securidaca–saponins are natural inhibitors of AKT, MCL-1, and BCL2L1 in cervical cancer cells. Cancer Manag. Res. 10, 5709–5724. doi:10.2147/CMAR.S163328
Ochokwu, I., Dasuki, A., and Oshoke, J. (2015). Azanza garckeana (goron tula) as an edible indigenous fruit in North eastern part of Nigeria. J. Biol. Agericulture Healthc. 5, 26–31.
Ochwang’i, D. O., Kimwele, C. N., Oduma, J. A., Gathumbi, P. K., Mbaria, J. M., and Kiama, S. G. (2014). Medicinal plants used in treatment and management of cancer in Kakamega County, Kenya. J. Ethnopharmacol. 151, 1040–1055. doi:10.1016/j.jep.2013.11.051
Okwu, D. (2005). Phytochemicals, vitamins and mineral contents of two Nigerian medicinal plants. Int. J. Mol. Med. Adv. Sci. 1, 375–381.
Omara, T., Kiprop, A. K., Ramkat, R. C., Cherutoi, J., Kagoya, S., Moraa Nyangena, D., et al. (2020). Medicinal plants used in traditional management of cancer in Uganda: A review of ethnobotanical surveys, phytochemistry, and anticancer studies. Evidence-Based Complementary Altern. Med. 2020, 3529081. doi:10.1155/2020/3529081
Onyancha, J. M., Gikonyo, N. K., Wachira, S. W., Mwitari, P. G., and Gicheru, M. M. (2018). Anticancer activities and safety evaluation of selected Kenyan plant extracts against breast cancer cell lines. J. Pharmacogn. Phytotherapy 10, 21–26. doi:10.5897/jpp2017.0465
Osafo, N., Boakye, Y. D., Agyare, C., Obeng, S., Foli, J. E., and Minkah, P. a. B. (2017). African plants with antiproliferative properties. Nat. Prod. Cancer Drug Discov. doi:10.5772/intechopen.68568
Osbourn, A. E. (2003). Saponins in cereals. Phytochemistry 62, 1–4. doi:10.1016/s0031-9422(02)00393-x
Ouelbani, R., Bensari, S., Mouas, T. N., and Khelifi, D. (2016). Ethnobotanical investigations on plants used in folk medicine in the regions of Constantine and Mila (North-East of Algeria). J. Ethnopharmacol. 194, 196–218. doi:10.1016/j.jep.2016.08.016
Oyeyemi, I. T., Yekeen, O. M., Odusina, P. O., Ologun, T. M., Ogbaide, O. M., Olaleye, O. I., et al. (2015). Genotoxicity and antigenotoxicity study of aqueous and hydro-methanol extracts of Spondias mombin L., Nymphaea lotus L. and Luffa cylindrical L. using animal bioassays. Interdiscip. Toxicol. 8, 184–192. doi:10.1515/intox-2015-0028
Oza, V. P., Parmar, P. P., Kumar, S., and Subramanian, R. (2010). Anticancer properties of highly purified L-asparaginase from Withania somnifera L. against acute lymphoblastic leukemia. Appl. Biochem. Biotechnol. 160, 1833–1840. doi:10.1007/s12010-009-8667-z
Pacifico, S., Di Maro, A., Petriccione, M., Galasso, S., Piccolella, S., Di Giuseppe, A. M., et al. (2014). Chemical composition, nutritional value and antioxidant properties of autochthonous Prunus avium cultivars from Campania Region. Food Res. Int. 64, 188–199. doi:10.1016/j.foodres.2014.06.020
Pandey, P., and Khan, F. (2021). Jab1 inhibition by methanolic extract of moringa oleifera leaves in cervical cancer cells: A potent targeted therapeutic approach. Nutr. cancer 73, 2411–2419. doi:10.1080/01635581.2020.1826989
Papież, M. A., Krzyściak, W., Szade, K., Bukowska-Straková, K., Kozakowska, M., Hajduk, K., et al. (2016). Curcumin enhances the cytogenotoxic effect of etoposide in leukemia cells through induction of reactive oxygen species. Drug Des. Dev. Ther. 10, 557–570. doi:10.2147/DDDT.S92687
Paragliola, R. M., Corsello, A., Locantore, P., Papi, G., Pontecorvi, A., and Corsello, S. M. (2020). Medical approaches in adrenocortical carcinoma. Biomedicines 8, 551. doi:10.3390/biomedicines8120551
Passos, I. D., and Mironidou-Tzouveleki, M. (2016). “Hallucinogenic plants in the Mediterranean countries,” in Neuropathology of drug addictions and substance misuse (Elsevier), 761–772.
Patel, A., Bhatt, M., Soni, A., and Sharma, P. (2021). Identification of steroidal saponins from Tribulus terrestris and their in silico docking studies. J. Cell. Biochem. 122, 1665–1685. doi:10.1002/jcb.30113
Pettit, G. R., Singh, S. B., Niven, M. L., Hamel, E., and Schmidt, J. M. (1987). Isolation, structure, and synthesis of combretastatins A-1 and B-1, potent new inhibitors of microtubule assembly, derived from Combretum caffrum. J. Nat. Prod. 50, 119–131. doi:10.1021/np50049a016
Pieme, C. A., Kumar, S. G., Dongmo, M. S., Moukette, B. M., Boyoum, F. F., Ngogang, J. Y., et al. (2014). Antiproliferative activity and induction of apoptosis by Annona muricata (Annonaceae) extract on human cancer cells. BMC complementary Altern. Med. 14, 516–610. doi:10.1186/1472-6882-14-516
Place, A. E., Suh, N., Williams, C. R., Risingsong, R., Honda, T., Honda, Y., et al. (2003). The novel synthetic triterpenoid, CDDO-imidazolide, inhibits inflammatory response and tumor growth in vivo. Clin. Cancer Res. 9, 2798–2806.
Potchoo, Y., Richard, D., Sakie, E., Guissou, I., Kini, F., and Yaro, B. (2008). Comparative phytochemical content of leaves extracts of two Annona senegalensis Pers: The one from Togo and the other originates from Burkina Faso. J. Biol. Sci. 8, 577–583. doi:10.3923/jbs.2008.577.583
Prior, R. L., Gu, L., Wu, X., Jacob, R. A., Sotoudeh, G., Kader, A. A., et al. (2007). Plasma antioxidant capacity changes following a meal as a measure of the ability of a food to alter in vivo antioxidant status. J. Am. Coll. Nutr. 26, 170–181. doi:10.1080/07315724.2007.10719599
Rady, I., Bloch, M. B., Chamcheu, R.-C. N., Banang Mbeumi, S., Anwar, M. R., Mohamed, H., et al. (2018). Anticancer properties of graviola (Annona muricata): A comprehensive mechanistic review. Oxidative Med. Cell. Longev. 2018, 1826170. doi:10.1155/2018/1826170
Ramachandran, C., Nair, S. M., Escalon, E., and Melnick, S. J. (2012). Potentiation of etoposide and temozolomide cytotoxicity by curcumin and turmeric force in brain tumor cell lines. J. Complementary Integr. Med. 9, Article 20. doi:10.1515/1553-3840.1614
Raobaikady, B., Reed, M. J., Leese, M. P., Potter, B. V., and Purohit, A. (2005). Inhibition of MDA-MB-231 cell cycle progression and cell proliferation by C-2-substituted oestradiol mono-and bis-3-O-sulphamates. Int. J. cancer 117, 150–159. doi:10.1002/ijc.21066
Ribeiro, V., Ferreres, F., Macedo, T., Gil-Izquierdo, Á., Oliveira, A. P., Gomes, N. G., et al. (2021). Activation of caspase-3 in gastric adenocarcinoma AGS cells by Xylopia aethiopica (Dunal) A. Rich. fruit and characterization of its phenolic fingerprint by HPLC-DAD-ESI (Ion Trap)-MSn and UPLC-ESI-QTOF-MS2. Food Res. Int. 141, 110121. doi:10.1016/j.foodres.2021.110121
Roduan, M. R., Abd Hamid, R., and Mohtarrudin, N. (2019). Modulation of cancer signalling pathway (s) in two-stage mouse skin tumorigenesis by annonacin. BMC complementary Altern. Med. 19, 1–16.
Rtibi, K., Selmi, S., Grami, D., Saidani, K., Sebai, H., Amri, M., et al. (2017). Ceratonia siliqua L.(immature carob bean) inhibits intestinal glucose absorption, improves glucose tolerance and protects against alloxan-induced diabetes in rat. J. Sci. Food Agric. 97, 2664–2670. doi:10.1002/jsfa.8091
Ruggiero, A., Ariano, A., Triarico, S., Capozza, M. A., Romano, A., Maurizi, P., et al. (2020). Temozolomide and oral etoposide in children with recurrent malignant brain tumors. Drugs Context 9, 1–9. doi:10.7573/dic.2020-3-1
Ruíz, G., Valencia-González, H. A., León-Galicia, I., García-Villa, E., García-Carrancá, A., and Gariglio, P. (2018). Inhibition of RAD51 by siRNA and resveratrol sensitizes cancer stem cells derived from HeLa cell cultures to apoptosis. Stem cells Int. 2018, 2493869. doi:10.1155/2018/2493869
Saidu, I. N., Umar, K. S., and Isa, M. H. (2015). Ethnobotanical survey of anticancer plants in Askira/Uba local government area of Borno State, Nigeria. Afr. J. Pharm. Pharmacol. 9, 123–130. doi:10.5897/ajpp2014.4083
Saleem, A., Ahotupa, M., and Pihlaja, K. (2001). Total phenolics concentration and antioxidant potential of extracts of medicinal plants of Pakistan. Z. für Naturforsch. C 56, 973–978. doi:10.1515/znc-2001-11-1211
Sari, A. N., Bhargava, P., Dhanjal, J. K., Putri, J. F., Radhakrishnan, N., Shefrin, S., et al. (2020). Combination of withaferin-A and CAPE provides superior anticancer potency: Bioinformatics and experimental evidence to their molecular targets and mechanism of action. Cancers 12, 1160. doi:10.3390/cancers12051160
Sawadogo, W. R., Schumacher, M., Teiten, M.-H., Dicato, M., and Diederich, M. (2012). Traditional West African pharmacopeia, plants and derived compounds for cancer therapy. Biochem. Pharmacol. 84, 1225–1240. doi:10.1016/j.bcp.2012.07.021
Senthilnathan, P., Padmavathi, R., Banu, S. M., and Sakthisekaran, D. (2006a). Enhancement of antitumor effect of paclitaxel in combination with immunomodulatory Withania somnifera on benzo (a) pyrene induced experimental lung cancer. Chemico-biological Interact. 159, 180–185. doi:10.1016/j.cbi.2005.11.003
Senthilnathan, P., Padmavathi, R., Magesh, V., and Sakthisekaran, D. (2006b). Chemotherapeutic efficacy of paclitaxel in combination with Withania somnifera on benzo (a) pyrene-induced experimental lung cancer. Cancer Sci. 97, 658–664. doi:10.1111/j.1349-7006.2006.00224.x
Serra, A. T., Duarte, R. O., Bronze, M. R., and Duarte, C. M. (2011). Identification of bioactive response in traditional cherries from Portugal. Food Chem. 125, 318–325. doi:10.1016/j.foodchem.2010.07.088
Shafiq, A., Moore, J., Suleman, A., Faiz, S., Farooq, O., Arshad, A., et al. (2020). Elevated soluble galectin-3 as a marker of chemotherapy efficacy in breast cancer patients: A prospective study. Int. J. breast cancer 2020, 4824813. doi:10.1155/2020/4824813
Shenouda, N. S., Sakla, M. S., Newton, L. G., Besch-Williford, C., Greenberg, N. M., Macdonald, R. S., et al. (2007). Phytosterol Pygeum africanum regulates prostate cancer in vitro and in vivo. Endocrine 31, 72–81. doi:10.1007/s12020-007-0014-y
Shikder, M., Al Hasib, T., and Kabir, M. L. (2020). Anticancer mechanism of Withania somnifera and its bioactive compounds: A short review along with computational molecular docking study.
Shishodia, S., Sethi, G., Konopleva, M., Andreeff, M., and Aggarwal, B. B. (2006). A synthetic triterpenoid, CDDO-Me, inhibits IkappaBalpha kinase and enhances apoptosis induced by TNF and chemotherapeutic agents through down-regulation of expression of nuclear factor kappaB-regulated gene products in human leukemic cells. Clin. cancer Res. 12, 1828–1838. doi:10.1158/1078-0432.CCR-05-2044
Shu, C. W., Weng, J. R., Chang, H. W., Liu, P. F., Chen, J. J., Peng, C. C., et al. (2021). Tribulus terrestris fruit extract inhibits autophagic flux to diminish cell proliferation and metastatic characteristics of oral cancer cells. Environ. Toxicol. 36, 1173–1180. doi:10.1002/tox.23116
Silva, G. R., Vaz, C. V., Catalão, B., Ferreira, S., Cardoso, H. J., Duarte, A. P., et al. (2020). Sweet cherry extract targets the hallmarks of cancer in prostate cells: Diminished viability, increased apoptosis and suppressed glycolytic metabolism. Nutr. cancer 72, 917–931. doi:10.1080/01635581.2019.1661502
Simoni, D., Romagnoli, R., Baruchello, R., Rondanin, R., Rizzi, M., Pavani, M. G., et al. (2006). Novel combretastatin analogues endowed with antitumor activity. J. Med. Chem. 49, 3143–3152. doi:10.1021/jm0510732
Solomon, S., Muruganantham, N., and Senthamilselvi, M. (2016). Anticancer activity of Abelmoschus esculentus (flower) against human liver cancer. Int. J. Pharmacol. Biol. Sci. 6, 154–157. doi:10.21276/ijpbs.2016.6.3.18
Sparg, S., Light, M., and Van Staden, J. (2004). Biological activities and distribution of plant saponins. J. Ethnopharmacol. 94, 219–243. doi:10.1016/j.jep.2004.05.016
Sreenivasan, S., and Krishnakumar, S. (2015). Synergistic effect of curcumin in combination with anticancer agents in human retinoblastoma cancer cell lines. Curr. eye Res. 40, 1153–1165. doi:10.3109/02713683.2014.987870
Stan, S. D., Hahm, E.-R., Warin, R., and Singh, S. V. (2008). Withaferin A causes FOXO3a-and Bim-dependent apoptosis and inhibits growth of human breast cancer cells in vivo. Cancer Res. 68, 7661–7669. doi:10.1158/0008-5472.CAN-08-1510
Sui, M., Yang, H., Guo, M., Li, W., Gong, Z., Jiang, J., et al. (2021). Cajanol sensitizes a2780/taxol cells to paclitaxel by inhibiting the PI3K/akt/NF-κB signaling pathway. Front. Pharmacol. 12, 783317. doi:10.3389/fphar.2021.783317
Sun, B., Qu, W., and Bai, Z. (2003). The inhibitory effect of saponins from Tribulus terrestris on Bcap-37 breast cancer cell line in vitro. Zhong yao cai= Zhongyaocai= J. Chin. Med. Mater. 26, 104–106.
Sun, K., Liu, Q.-Y., Wang, A., Gao, Y.-W., Zhao, L.-C., and Guan, W.-B. (2021). Comparative analysis and phylogenetic implications of plastomes of five genera in subfamily Amyridoideae (Rutaceae). Forests 12, 277. doi:10.3390/f12030277
Sung, H., Ferlay, J., Siegel, R. L., Laversanne, M., Soerjomataram, I., Jemal, A., et al. (2021). Global cancer statistics 2020: GLOBOCAN estimates of incidence and mortality worldwide for 36 cancers in 185 countries. CA a cancer J. Clin. 71, 209–249. doi:10.3322/caac.21660
Suphachai, C. (2014). Antioxidant and anticancer activities of Moringa oleifera leaves. J. Med. Plants Res. 8, 318–325. doi:10.5897/jmpr2013.5353
Taiwo, B. J., Popoola, T. D., Van Heerden, F. R., and Fatokun, A. A. (2021). Isolation and characterisation of two quercetin glucosides with potent anti-reactive oxygen species (ROS) activity and an olean-12-en triterpene glucoside from the fruit of Abelmoschus esculentus (L.) moench. Chem. Biodivers. 18, e2000670. doi:10.1002/cbdv.202000670
Talari, A., and Shakappa, D. (2018). Role of pigeon pea (Cajanus cajan L.) in human nutrition and health: A review. Asian J. Dairy Food Res. 37, 212–220. doi:10.18805/ajdfr.dr-1379
Teklehaymanot, T. (2009). Ethnobotanical study of knowledge and medicinal plants use by the people in Dek Island in Ethiopia. J. Ethnopharmacol. 124, 69–78. doi:10.1016/j.jep.2009.04.005
Thaiparambil, J. T., Bender, L., Ganesh, T., Kline, E., Patel, P., Liu, Y., et al. (2011). Withaferin A inhibits breast cancer invasion and metastasis at sub-cytotoxic doses by inducing vimentin disassembly and serine 56 phosphorylation. Int. J. cancer 129, 2744–2755. doi:10.1002/ijc.25938
Tian, Y., Zhang, C., and Guo, M. (2017). Comparative study on alkaloids and their anti-proliferative activities from three Zanthoxylum species. BMC complementary Altern. Med. 17, 460–516. doi:10.1186/s12906-017-1966-y
Toshiyuki, M., and Reed, J. C. (1995). Tumor suppressor p53 is a direct transcriptional activator of the human bax gene. Cell 80, 293–299. doi:10.1016/0092-8674(95)90412-3
Tozer, G. M., Kanthou, C., Parkins, C. S., and Hill, S. A. (2002). The biology of the combretastatins as tumour vascular targeting agents. Int. J. Exp. pathology 83, 21–38. doi:10.1046/j.1365-2613.2002.00211.x
Tremouillaux-Guiller, J., Moustafa, K., Hefferon, K., Gaobotse, G., and Makhzoum, A. (2020). Plant-made HIV vaccines and potential candidates. Curr. Opin. Biotechnol. 61, 209–216. doi:10.1016/j.copbio.2020.01.004
Tsuda, T., Horio, F., and Osawa, T. (2000). The role of anthocyanins as an antioxidant under oxidative stress in rats. Biofactors 13, 133–139. doi:10.1002/biof.5520130122
Tsujimoto, Y., and Shimizu, S. (2007). Role of the mitochondrial membrane permeability transition in cell death. Apoptosis 12, 835–840. doi:10.1007/s10495-006-0525-7
Tugume, P., Kakudidi, E. K., Buyinza, M., Namaalwa, J., Kamatenesi, M., Mucunguzi, P., et al. (2016). Ethnobotanical survey of medicinal plant species used by communities around Mabira Central Forest Reserve, Uganda. J. Ethnobiol. ethnomedicine 12, 5–28. doi:10.1186/s13002-015-0077-4
Uddin, M., Juraimi, A. S., Hossain, M. S., Nahar, M., Un, A., Ali, M., et al. (2014). Purslane weed (Portulaca oleracea): A prospective plant source of nutrition, omega-3 fatty acid, and antioxidant attributes. Sci. World J. 2014, 951019. doi:10.1155/2014/951019
Usenik, V., Stampar, F., Petkovsek, M. M., and Kastelec, D. (2015). The effect of fruit size and fruit colour on chemical composition in ‘Kordia’sweet cherry (Prunus avium L.). J. Food Compos. Analysis 38, 121–130. doi:10.1016/j.jfca.2014.10.007
Usman, M., Khan, W. R., Yousaf, N., Akram, S., Murtaza, G., Kudus, K. A., et al. (2022). Exploring the phytochemicals and anti-cancer potential of the members of fabaceae family: A comprehensive review. Molecules 27, 3863. doi:10.3390/molecules27123863
Vamsi, K. B., Glory, T. J., Florida, T., Aneesh, N., Rekha, L., and Thangaraj, P. (2020). Gene expression analysis of EGFR and PI3K genes in A549 lung cancer cell line treated with Withania somnifera root extract. Res. J. Biotechnol. 15, 2.
Vaseva, A. V., Marchenko, N. D., Ji, K., Tsirka, S. E., Holzmann, S., and Moll, U. M. (2012). p53 opens the mitochondrial permeability transition pore to trigger necrosis. Cell 149, 1536–1548. doi:10.1016/j.cell.2012.05.014
Vigneshwaran, V., Thirusangu, P., Madhusudana, S., Krishna, V., Pramod, S. N., and Prabhakar, B. (2016). The latex sap of the ‘Old World Plant’Lagenaria siceraria with potent lectin activity mitigates neoplastic malignancy targeting neovasculature and cell death. Int. Immunopharmacol. 39, 158–171. doi:10.1016/j.intimp.2016.07.024
Visagie, M. H., Birkholtz, L.-M., and Joubert, A. M. (2015). A 2-methoxyestradiol bis-sulphamoylated derivative induces apoptosis in breast cell lines. Cell & Biosci. 5, 19–15. doi:10.1186/s13578-015-0010-5
Von Holtz, R. L., Fink, C. S., and Awad, A. B. (1998). β-sitosterol activates the sphingomyelin cycle and induces apoptosis in LNCaP human prostate cancer cells.
Wang, H. (1998). Plant-derived anticancer agents currently in clinical use or in clinical trials. IDrugs investigational drugs J. 1, 92–102.
WHO (2020). Global Health Estimates: Life expectancy and leading causes of death and disability. World Health Organization.
Widodo, N., Priyandoko, D., Shah, N., Wadhwa, R., and Kaul, S. C. (2010). Selective killing of cancer cells by Ashwagandha leaf extract and its component Withanone involves ROS signaling. PloS one 5, e13536. doi:10.1371/journal.pone.0013536
Wu, Q., Cho, J.-G., Yoo, K.-H., Jeong, T.-S., Park, J.-H., Kim, S.-Y., et al. (2013). A new phenanthrene derivative and two diarylheptanoids from the roots of Brassica rapa ssp. campestris inhibit the growth of cancer cell lines and LDL-oxidation. Archives pharmacal Res. 36, 423–429. doi:10.1007/s12272-013-0068-8
Wu, T., Yin, J., Zhang, G., Long, H., and Zheng, X. (2016). Mulberry and cherry anthocyanin consumption prevents oxidative stress and inflammation in diet-induced obese mice. Mol. Nutr. Food Res. 60, 687–694. doi:10.1002/mnfr.201500734
Xie, J., Qian, Y.-Y., Yang, Y., Peng, L.-J., Mao, J.-Y., Yang, M.-R., et al. (2022). Isothiocyanate from moringa oleifera seeds inhibits the growth and migration of renal cancer cells by regulating the PTP1B-dependent src/ras/raf/ERK signaling pathway. Front. Cell Dev. Biol. 9, 790618. doi:10.3389/fcell.2021.790618
Yakubu, O. F., Metibemu, D. S., Adelani, I. B., Adesina, G. O., Edokwe, C. B., Oseha, O. E., et al. (2020). Annona senegalensis extract demonstrates anticancer properties in N-diethylnitrosamine-induced hepatocellular carcinoma in male Wistar rats. Biomed. Pharmacother. 131, 110786. doi:10.1016/j.biopha.2020.110786
Yang, C., Gundala, S. R., Mukkavilli, R., Vangala, S., Reid, M. D., and Aneja, R. (2015). Synergistic interactions among flavonoids and acetogenins in Graviola (Annona muricata) leaves confer protection against prostate cancer. Carcinogenesis 36, 656–665. doi:10.1093/carcin/bgv046
Yang, H., Shi, G., and Dou, Q. P. (2007). The tumor proteasome is a primary target for the natural anticancer compound Withaferin A isolated from “Indian winter cherry”. Mol. Pharmacol. 71, 426–437. doi:10.1124/mol.106.030015
Yang, Z., Garcia, A., Xu, S., Powell, D. R., Vertino, P. M., Singh, S., et al. (2013). Withania somnifera root extract inhibits mammary cancer metastasis and epithelial to mesenchymal transition. PLoS One 8, e75069. doi:10.1371/journal.pone.0075069
Yap, C. V., Subramaniam, K. S., Khor, S. W., and Chung, I. (2017). Annonacin exerts antitumor activity through induction of apoptosis and extracellular signal-regulated kinase inhibition. Pharmacogn. Res. 9, 378–383. doi:10.4103/pr.pr_19_17
Yiaile, A. L. (2017). “vitro antiploriferative activity,” in Phytochemical composition and toxicity studies of Fagaropsis angolensis and Prunus africana crude extracts (University of Nairobi).
Yiallouris, A., Patrikios, I., Johnson, E. O., Sereti, E., Dimas, K., De Ford, C., et al. (2018). Annonacin promotes selective cancer cell death via NKA-dependent and SERCA-dependent pathways. Cell Death Dis. 9, 764–813. doi:10.1038/s41419-018-0772-x
Youle, R. J., and Strasser, A. (2008). The BCL-2 protein family: Opposing activities that mediate cell death. Nat. Rev. Mol. Cell Biol. 9, 47–59. doi:10.1038/nrm2308
Yu, L.-L., Wu, J.-G., Dai, N., Yu, H.-G., and Si, J.-Μ. (2011). Curcumin reverses chemoresistance of human gastric cancer cells by downregulating the NF-κB transcription factor. Oncol. Rep. 26, 1197–1203. doi:10.3892/or.2011.1410
Yung, B. C., Li, J., Zhang, M., Cheng, X., Li, H., Yung, E. M., et al. (2016). Lipid nanoparticles composed of quaternary amine–tertiary amine cationic lipid combination (QTsome) for therapeutic delivery of AntimiR-21 for lung cancer. Mol. Pharm. 13, 653–662. doi:10.1021/acs.molpharmaceut.5b00878
Zahoor, M., Ikram, M., Nazir, N., Naz, S., Batiha, G. E.-S., Kamran, A. W., et al. (2021). A comprehensive review on the medicinal importance; biological and therapeutic efficacy of Lagenaria siceraria (mol.) (bottle gourd) standley fruit. Curr. Top. Med. Chem. 21, 1788–1803. doi:10.2174/1568026621666210701124628
Zhang, X., Mukerji, R., Samadi, A. K., and Cohen, M. S. (2011). Down-regulation of estrogen receptor-alpha and rearranged during transfection tyrosine kinase is associated with withaferin a-induced apoptosis in MCF-7 breast cancer cells. BMC complementary Altern. Med. 11, 84–10. doi:10.1186/1472-6882-11-84
Zhang, X., Samadi, A. K., Roby, K. F., Timmermann, B., and Cohen, M. S. (2012). Inhibition of cell growth and induction of apoptosis in ovarian carcinoma cell lines CaOV3 and SKOV3 by natural withanolide Withaferin A. Gynecol. Oncol. 124, 606–612. doi:10.1016/j.ygyno.2011.11.044
Zhao, B.-X., Wang, Y., Zhang, D.-M., Jiang, R.-W., Wang, G.-C., Shi, J.-M., et al. (2011). Flueggines A and B, two new dimeric indolizidine alkaloids from Flueggea virosa. Org. Lett. 13, 3888–3891. doi:10.1021/ol201410z
Zhao, R., Gao, X., Cai, Y., Shao, X., Jia, G., Huang, Y., et al. (2013). Antitumor activity of Portulaca oleracea L. polysaccharides against cervical carcinoma in vitro and in vivo. Carbohydr. Polym. 96, 376–383. doi:10.1016/j.carbpol.2013.04.023
Zúñiga, R., Concha, G., Cayo, A., Cikutović-Molina, R., Arevalo, B., González, W., et al. (2020). Withaferin A suppresses breast cancer cell proliferation by inhibition of the two-pore domain potassium (K2P9) channel TASK-3. Biomed. Pharmacother. 129, 110383. doi:10.1016/j.biopha.2020.110383
Zuo, J., Jiang, H., Zhu, Y.-H., Wang, Y.-Q., Zhang, W., and Luan, J.-J. (2016). Regulation of MAPKs signaling contributes to the growth inhibition of 1, 7-dihydroxy-3, 4-dimethoxyxanthone on multidrug resistance A549/taxol cells. Evidence-Based Complementary Altern. Med. 2016, 2018704. doi:10.1155/2016/2018704
Glossary
ACAT1 Acetyl-CoA acetyltransferase
Akt Ak strain transforming serine/threonine protein kinase
ASC adaptor protein Apoptosis-associated Speck-like protein containing a CARD BGL24 bottle gourd Lagenaria siceraria
Bcl-2 B-Cell Leukemia/lymphoma-2
Bim BH3-containing protein
Con A Concanavalin A
COX-2 CycloOXygenase-2
CRP C-Reactive Protein
DC Dendritic Cell
EGF Epidermal Growth Factor
ENRAGE Extracellular Newly identified ligand for the Receptor for Advanced Glycation End products
ER Estrogen Receptor
ERK Extracellular signal-Regulated Kinase
FOXO3a Forkhead box O3a
GLUT glucose transporter
GPx glutathione peroxidase
GSH reduced glutathione
GSSG Glutathione Disulfide GR Glutathione Reductase
H2O2 Hydrogen peroxide
HSF1 Heat Shock Factor
IC50 Inhibitory Concenration50
IFN-γ Interferon-γ
ING1 Inhibitor of Growth family, member1
IL-12 Interleukin-12
JNK c-Jun N-terminal kinase
LDH Lactate dehydrogenase
LHX3 LIM Homeobox 3
LPS Lipopolysaccharide
MAPK Mitogen-Activated Protein Kinase
MCT4 MonoCarboxylate Transporter 4
MyD88 Myeloid Differentiation primary response 88
NCAM Neural Cell Adhesion Molecule
NF-κB Nuclear Factor-κB
NLRP3 NLR family Pyrin domain containing three
NO synthase Nitric Oxide synthase
NQO1 NADPH quinone oxidoreductase
PI3K Phosphoinositide three Kinase
PAI-1 Plasminogen Activator Inhibitor-1
PFK-1 Phosphofructokinase-1
PLCγ-1 Phosphoinositide-specific Phospholipase γ1
PP2 Phloem Protein 2
RET REarranged during Transfection tyrosine kinase
ROS Reactive Oxygen Species
SCID Severe Combined Immunodeficiency
SOD SuperOxide Dismutase
Sp1/Sp4 Specificity protein transcription factors
STAT3 Signal Transducer and Activator of Transcription three
TFAP2A Transcription Factor AP-2 alpha
Th1 T helper cell
TLR4 Toll-Like Receptor4
TNF-α Tumor Necrosis Factor-α
TPX2 Microtubule-associated Protein
TUNEL Terminal deoxynucleotidyl transferase TdT-catalyzed dUTP-Nick End Labeling
VCAM-1 Vascular Cell Adhesion Molecule-1
VEGF Vascular Endothelial Growth Factor
Keywords: sub-Saharan Africa, medicinal plants, cancer, bioactive compounds, cancer management, anticancer mechanism
Citation: Gaobotse G, Venkataraman S, Brown PD, Masisi K, Kwape TE, Nkwe DO, Rantong G and Makhzoum A (2023) The use of African medicinal plants in cancer management. Front. Pharmacol. 14:1122388. doi: 10.3389/fphar.2023.1122388
Received: 12 December 2022; Accepted: 03 February 2023;
Published: 14 February 2023.
Edited by:
Hidayat Hussain, Leibniz Institute of Plant Biochemistry, GermanyReviewed by:
Mohamed S. Nafie, Suez Canal University, EgyptLaiba Arshad, Forman Christian College, Pakistan
Ibrahim Malami, Usmanu Danfodiyo University, Nigeria
Sreelatha Sarangapani, TLL, Singapore
Copyright © 2023 Gaobotse, Venkataraman, Brown, Masisi, Kwape, Nkwe, Rantong and Makhzoum. This is an open-access article distributed under the terms of the Creative Commons Attribution License (CC BY). The use, distribution or reproduction in other forums is permitted, provided the original author(s) and the copyright owner(s) are credited and that the original publication in this journal is cited, in accordance with accepted academic practice. No use, distribution or reproduction is permitted which does not comply with these terms.
*Correspondence: Goabaone Gaobotse, Z2FvYm90c2VnQGJpdXN0LmFjLmJ3; Kabo Masisi, bWFzaXNpa0BiaXVzdC5hYy5idw==; Abdullah Makhzoum, bWFraHpvdW1AYml1c3QuYWMuYnc=