- Key Laboratory of Tropical Biological Resources of Ministry of Education, School of Pharmaceutical Sciences, Hainan University, Haikou, China
Zein, as one of the natural and GRAS proteins in plant, is renewable, nontoxic, biocompatible and biodegradable. Over the past decade, many research efforts have been devoted to zein-based biomaterials for several industrial applications. Combining with research experiences in our research group, the preparation methods, characterizations and pharmaceutical applications of zein-based nanoparticles were summarized in this review. Zein NPs with different particle nanostructures have been prepared by chemical crosslinking, desolvating, dispersing and micromixing strategies. The pharmaceutical applications of zein NPs are mainly focus on the drug delivery. Zein NPs can improve the drug stability, increase the oral bioavailability, control the drug release and enhance the drug targeting, thereby improving the pharmaceutical effect effectively. More efforts are required to analyze the relationship among preparation methods, particle nanostructures and pharmaceutical properties in virtue of quality by design approach, and further promote the scale-up production and clinical application of zein NPs.
1 Introduction
Nanoparticles based on nanotechnology and biotechnology have attracted significant interest in drug delivery in the past decades (Mitchell et al., 2021). Various nanoparticles have been developed to improve the pharmacological and therapeutic properties of conventional (“free”) drugs, including poor aqueous solubility, instability, low bioavailability, serious adverse effects, and lack of targeted delivery. Among the available materials for preparing nanoparticles, protein provides numerous advantages over lipid, carbohydrate, synthetic polymer and inorganic colloidal carriers namely biodegradability, wide availability, environmental tolerance, and high drug binding capacity (Hong et al., 2020). A number of proteins including animal-derived proteins (e.g., albumin, gelatin, casein, lactoferrin, β-lactoglobulin, elastin, and lysozyme) and plant-derived proteins (zein, gliadin, glutenin, walnut protein isolate, and soy protein isolate) have been widely used as main natural backbones for the fabrication of nanoparticles. Compared to animal-derived proteins, plant-derived proteins are less expensive and reduce the risk of spreading diseases.
Zein, the major storage protein of corn, has been of scientific interest since its isolation in 1821 (Gorham, 1821). Zein is actually a heterogeneous mixture of different peptides of various molecular weights (MW), which can be classified into four classes: α-zein (two bands of average MW 22 and 24 kDa), β-zein (an average MW of 17 kDa), γ-zein (two bands of average MW 18 and 27 kDa) and δ-zein (an average MW of 10 kDa) (Shukla and Cheryan, 2001; Paliwal and Palakurthi, 2014). Structurally, zein is composed mainly of nonpolar and uncharged amino acids, such as glutamine (21%–26%), leucine (20%), proline (10%), and alanine (10%) (Pomes, 1971). The unbalance of amino acid composition leads to the poor water solubility and low nutritional value of zein. However, the peculiar amino acid composition also gives zein many favorable properties compared to other proteins. Zein behaves as amphiphilic but more specifically as a hydrophobic protein, which is insolubility in water except in the presence of alcohol (60%–95%), high concentration of urea, alkaline pH (≥11) or anionic surfactants (Evans and Manley, 1941; Manley and Evans, 1943). In contrast to drug delivery using hydrophilic proteins, zein has the capability of physically entrapping of hydrophobic compounds and yielding sustained drug release. Moreover, zein has a GRAS (Generally Regarded as Safe) status and readily self-assembles into nanoparticles with various structures depending on the solvents and the processing conditions (Wang and Padua, 2012b). Thus, zein has been extensively studied in terms of encapsulating and delivering bioactive ingredients.
Over the past decades, many research efforts have been devoted to the development and application of zein-based biomaterials. Some recent review articles have covered their applications in food and nutrition (Kasaai, 2018), phytochemicals delivery (Hassan et al., 2022), oral drug delivery (Irache and Gonzalez-Navarro, 2017), tissue engineering (Paliwal and Palakurthi, 2014) and other biomedical applications (Demir et al., 2017). In pharmaceutical field, zein has been investigated as excellent natural building blocks for preparing nanoparticles to delivery various active compounds. Combining with research experiences in our research group, the preparation, characterization and pharmaceutical application of zein-based nanoparticles (zain NPs) was summarized in this review.
2 Preparation of zein-based nanoparticles
The construction of zein particles can be traced back to the study of Suzuki et al., in 1989, that is, zein microspheres conjugated with antitumor drugs (mitomycinc, daunomycin hydrochloride, peplomycin sulfate, PS-K) have been prepared by glutaraldehyde mediated crosslinking reaction (Matsuda et al., 1989; Suzuki et al., 1989). There are abundant hydroxyl, amino and carboxyl groups on the side chains of zein molecule, which can be chemically coupled with hydrophobic or hydrophilic drugs by adding crosslinking agents, resulting in high encapsulation efficiency (EE). However, zein particles formed by chemical crosslinking are heavily aggregated and usually micro-scale. Also, crosslinking reaction may produce toxic or inactive drug derivatives, and the residues of toxic crosslinking agents and organic solvents may cause safety risks of final products. Therefore, it is imperative to develop alternative methods for preparation of zein NPs. Up to now various methods have been applied for preparing zein-based nanoparticles, and can be broadly classified as desolvating, dispersing and micromixing strategies (Figure 1). Each strategy has its own set of advantages and disadvantages, which have been summarized in Table 1.
2.1 Desolvating strategy
Desolvating is the main preparation strategy of zein NPs. It refers to adding a reagent that can reduce zein solubility in the solution, so that zein molecules can self-assemble into nanoparticles. Many variations of the desolvating technique exist, includes solvent/nonsolvent method, coacervation method, pH-driven method and dialysis method.
2.1.1 Solvent/nonsolvent method
The most commonly used solvent/nonsolvent for the formation of zein NPs is the ethanol-water system. Under constant temperature, the solubility of zein varies between 2% and 60% (w/w) depending on the ethanol concentration (Mossé, 1961). The common procedure of solvent/nonsolvent method is as follows: 1) zein and drug are completely dissolved in 60%–90% alcohol; 2) the obtained solution is poured into deionized water (as nonsolvent), resulting in the supersaturation and self-assembly of zein molecules; 3) The solvent is removed by rotary evaporation, and zein NPs are collected after centrifugation, washing and drying. The solvent/nonsolvent process, also known as antisolvent precipitation or phase separation method, has been used to encapsulate ivermycin (Liu et al., 2005), ciprofloxacin (Fu et al., 2009), prednisolone (Lau et al., 2012), curcumin (Patel et al., 2010a), and many other drugs into zein NPs. In most cases, the obtained particles possess smooth surface, small and uniform particle size, and high drug loading capacity. For example, 5-fluorouracil/zein NPs were prepared using the phase separation method (Lai and Guo, 2011): under the optimal operating conditions, the surface of the nanoparticles was spherical and smooth, the particle size was 114.9 nm, and the EE and drug loading (DL) were 60.7% and 9.17%, respectively.
2.1.2 Coacervation method
The preparation process of coacervation method is similar to that of solvent/non-solvent method. The difference is that zein and drug are first dispersed into water (or anhydrous ethanol), and then ethanol (or water) is added dropwise. According to the ternary phase diagram of zein solubility in ethanol and water, zein molecules can self-assemble to a coacervation state when ethanol concentration is lower than 40% or higher than 90% (Mossé, 1961). The simple coacervates of zein to encapsulate and release gitoxin in a controlled phase was prepared (Muthuselvi and Dhathathreyan, 2006). However, the obtained particles were micron-sized, and the EE was low (1.77%–20.98%). Crosslinking agents are often added to obtain crosslinked particles, which can moderately reduce the size of particles and increase the EE (Karthikeyan et al., 2012). Besides, simple coacervates of zein tend to aggregate during the storage due to their high surface hydrophobicity and neutral isoelectric point. Complex coacervation of zein with proteins [e.g., casein (Bao et al., 2021) and whey protein (Wei et al., 2021)], phospholipids [e.g., sophorolipid (Chen et al., 2020) and lecithin (Fu et al., 2021)], and polysaccharides [e.g., alginate (Khan et al., 2019) and chitosan (Pauluk et al., 2019)] is regarded as an effective approach to prevent hydrophobic aggregation and enhance stability.
2.1.3 pH-driven method
pH-driven method is developed based on the solubility of zein in alkaline environment (pH ≥ 11.0) and insolubility in neutral or acidic aqueous solution. Briefly, zein is dispersed in deionized water, then the pH is adjusted to 11.0 or above with NaOH for zein dissolution, and then adjusted back to neutral or acidic by HCl. During the pH changing from alkalinity to neutrality, zein is protonated and gradually aggregated to nanoparticles (Yan et al., 2022). The pH-driven method has been widely used to prepare zein composite nanoparticles. For example, carvacrol-loaded zein/sodium caseinate composite nanoparticles were fabricated (Zheng et al., 2022): the obtained particles were spherical, the particle size was around 50–200 nm, and the EE was 77.96%–82.19%. Similarly, pH-driven zein/tea saponin composite nanoparticles were developed for encapsulation and oral delivery of curcumin (Yuan et al., 2021): the obtained particles were spherical, the particle size was around 100–250 nm, EE and DL were 83.73% and 22.33% respectively. Also, zein/propylene glycol alginate (Li et al., 2022), zein/pea protein (Zhao et al., 2022), zein/whey protein isolate (Zhan et al., 2020), zein/rhamnolipid (Dai et al., 2019), zein/whey protein isolate/carboxymethyl cellulose (Liu et al., 2022a) and other composite nanoparticles were prepared by pH-driven method. Coating and/or incorporating zein NPs with other hydrophilic or charged biopolymers can adjust their destabilization and aggregation behavior in aqueous suspension, thereby broadening their application in pharmaceutical and related industries. The progress in different methods and materials of surface-coated zein NPs and their encapsulated bioactive compound has been summarized recently (Yuan et al., 2022).
2.1.4 Dialysis method
Dialysis method is a variant of solvent/nonsolvent method. Briefly, the dialysis bag containing an alcohol solution of zein and drug is placed in the dialysate (aqueous phase). When the solution in dialysis bag is contacted with the dialysate, diffusion of the solvent through the semipermeable membrane takes place, resulting in the change of the polar environment of zein, and the formation of zein NPs (Rodríguez-Félix et al., 2020). Solvent/nonsolvent method involves a large number of organic solvent, and requires a tedious separation process to remove the particles from the solvent. For dialysis method, solvent attrition and the addition of nonsolvent occur simultaneously, which avoids the additional operations to remove organic solvents after particle formation. In our previous work (Liu et al., 2016b), a novel built-in ultrasonic dialysis process (BUDP) was developed by combining the ultrasonic dispersing with dialysis technologies. Our results indicated that zein particles obtained by BUDP present much smaller particle size and narrower size distribution than those obtained from the conventional dialysis method. As shown in Figure 2, zein particles prepared by the BUDP possessed a spherical morphology with mean particle size from 400 nm to 2500 nm under different process parameters. Based on the BUDP, polydopamine coated zein-indomethacin particles with high EE of 89.1% were prepared for colon-targeted drug delivery (Wang et al., 2018).
2.2 Dispersing strategy
For conventional desolvating methods, zein NPs are formed from the bulk solution. The formed zein NPs are easy to aggregate to form the flocculates or precipitates during the drying process, which limit to some extent their usages in pharmaceutical industries. A general strategy to break this limitation is dispersing the stock solution to small droplets before the desolvating or drying process. The dispersing methods include but not limited to liquid-liquid dispersion, spray drying, electrospraying, supercritical antisolvent (SAS) process and atomizing/antisolvent precipitation (AAP) process. There are mainly two procedures regarding the particle formation: the breakup of stock solution into droplets, and the self-assembly and solidification of these droplets into nanoparticles during solvent attrition.
2.2.1 Liquid–liquid dispersion
For the liquid–liquid dispersion, the stock solution of zein in 55%–90% aqueous ethanol is sheared to small droplets by a high-speed homogenizer before getting to the bulk deionized water. Due to the excellent miscibility of ethanol and water, ethanol in the dispersed droplets diffuses into the bulk water instantaneously, resulting in the solvent attrition. When the ethanol concentration in the dispersed droplets decreases below the solubilization limit of zein, these small droplets start to solidify and form nanoparticles. The size of zein NPs was typically 100–200 nm, and smaller particles were formed at a higher shear rate, and a higher ethanol concentration or a lower zein concentration in stock solutions (Zhong and Jin, 2009b).
2.2.2 Spray drying
Spray drying is a frequently-used liquid atomization technology for producing a drying powder in pharmaceutical industry. It mainly involves four steps: feed atomization, air flow contact, drying and particle formation, and separation of product from the drying air (Mohammed et al., 2020). Atomization that involves dispersing the stock solution into small droplets is crucial to obtain a spray-dried product with desired properties. There are different types of atomizers, including rotary atomizers, pressure nozzles, pneumatic nozzles and sonic nozzles (Sosnik and Seremeta, 2015). Spray drying was applied as a commercially practical process to microencapsulate lysozyme in zein to achieve sustained release of the antimicrobial (Zhong and Jin, 2009a). The solution of zein, thymol, and lysozyme in 90% (v/v) aqueous ethanol was spray dried with a B-290 mini spray-dryer. The obtained particles were microcapsules with an encapsulation efficiency of 35.4%–49.1%. A similar method was adopted to obtain nisin/zein microcapsules with particle size less than 3 μm (Xiao et al., 2011). Spray drying has the advantages of convenient operation, short drying time, good fluidity and dispersion of products, and is suitable for large-scale production. However, it is not suitable for encapsulating temperature-sensitive actives, and the produced zein particles are usually microscale.
2.2.3 Supercritical antisolvent process
SAS process, by exploiting the antisolvent effect of supercritical CO2 (scCO2), is a potential alternative to conventional antisolvent precipitation. The preparation process is as follows: 1) the drug and zein are dissolved in an liquid solvent that is miscible with scCO2; 2) the stock solution is sprayed through a micro-nozzle into the precipitation vessel containing scCO2; 3) when in contact with the scCO2, the solvent in the dispersed droplets diffuses into the scCO2 instantaneously, resulting in the solute supersaturation and formation of small particles. Lysozyme/zein particles were prepared via SAS process by using 90% ethanol in water as the liquid solvent (Zhong et al., 2009). However, the obtained particles were irregular, and the particle size larger than 50 μm. This may be due to the poor miscibility of 10% water and scCO2, resulting in a slow solvent attrition rate. In another study, coprecipitation of zein with diclofenac sodium at different polymer/drug ratio and concentration in the liquid solution were prepared by using DMSO as the liquid solvent (Franco et al., 2018). This time, microparticles with mean diameters ranging from 0.416 to 1.308 μm were obtained. Besides, lutein/zein NPs with mean particle size around 200–300 nm were successfully prepared via solution enhanced dispersion by supercritical fluids (SEDS, a modified SAS process) using acetone/DMSO (7:3, v/v) as the liquid solvent (Hu et al., 2012). SEDS process consists of a two (or three) coaxial passages nozzle to provide a simultaneous injection of the solution and scCO2. In this way, scCO2 acts both as an antisolvent and as a “spray enhancer” (Li and Zhao, 2017). ScCO2 as anti-solvent offers the advantages of mild operating temperature, being environmentally benign, less or solvent-free product. But the influence mechanism of operating parameters on the nanostructures and properties of obtained particles is complicated, involving the mutual interaction of fluid dynamics, phase equilibria and mass transport, and their influence on nucleation and growth mechanisms (Liu et al., 2013; Liu et al., 2015; Liu et al., 2021b). Thus, the preparation of zein NPs by SAS method needs further research.
2.2.4 Electrospraying
Electrospraying (or electrohydrodynamic atomization) is an emerging and rather popular technique for obtaining the nanoparticles from biopolymers. In this process, the stock solution of zein is dispersed into tiny charged droplets under an electric field. Electrospraying is performed in a gas atmosphere, with the exception of the effective evaporation of solvent from the interface between the working fluids and their surrounding air environment (Malik et al., 2022). Being highly charged, the therapeutic molecules can easily be incorporated into zein NPs with high loading efficacy. On the basis of electrospraying, curcumin/zein NPs with a particle size of 175–900 nm and an EE of 85%–90% (Gomez-Estaca et al., 2012), and tamoxifen citrate/zein NPs with a particle size of 0.68–1.54 μm (Liu et al., 2018) were prepared. Besides, β-Carotene were encapsulated in zein matrix at micro- and nano-level through spray drying and electrospraying techniques, respectively, and the results proved that electrospraying had higher encapsulation efficacy than spray drying (Mahalakshmi et al., 2020). Electrospraying presents the advantages of simple equipment, high EE and small particle size, and does not require a tedious separation process to remove the particles from the solvent. However, the particle size is gradually enlarged with the increase of zein concentration (2.5%–15%), in which undesired collapsed and shrunken particles are yielded at high zein concentration (Gomez-Estaca et al., 2012).
2.2.5 Atomizing/antisolvent precipitation process
In our recent work, a novel and simple AAP process was established for the preparation of zein NPs by taking the advantages of atomizing and antisolvent self-assembly (Wu et al., 2022). On the basis of AAP process, folate-conjugated zein (Fa-zein)/soy lecithin (SL)/carboxymethyl chitosan (CMC) core-shell nanoparticles were successfully produced for the delivery of docetaxel (DTX), as shown in Figure 3. In brief, the solution of zein, SL and DTX in ethanol-water (70:30, v/v) was charged at a given flow rate, and atomized into fine droplets by compressed air through a nanosprayer, and then introduced into the antisolvent phase (CMC aqueous solution), and then self-assembled spontaneously into nanoparticles as the change of solution polarity from hydrophobic to hydrophilic. At a suitable condition, core-shell DTX/FZLC NPs with high EE (79.22% ± 0.37%), small particle size (206.9 ± 48.73 nm) and high zeta potential (−41.8 ± 3.97 mV) were obtained, which could increase the DTX dissolution, sustain the DTX release, and enhance the DTX cytotoxicity significantly. These results suggested that AAP process was suitable for the fabrication of complex zein NPs with multilayer core-shell structures. Further investigations and applications of AAP process are worth to be conducted.
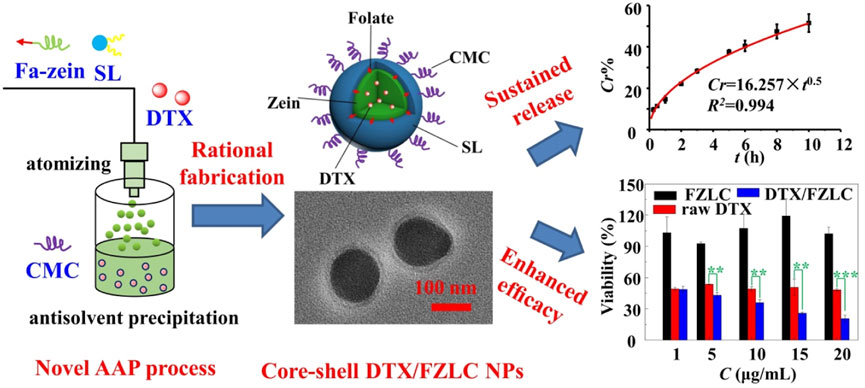
FIGURE 3. Rational fabrication and characteristic of docetaxel loaded folate-conjugated zein/soy lecithin/carboxymethyl chitosan core-shell nanoparticles (DTX/FZLC NPs) using a novel atomizing/antisolvent precipitation (AAP) process.
2.3 Micromixing strategy
Different from the above methods, micromixing strategy prepares zein NPs in a confined volume, which allows a continuous operation and a better control on the fabrication of zein NPs. Thus, micromixing strategy is a continuous alternative to fabricate zein NPs. The representative methods are flash nanoprecipitation (FNP) and microfluidic technique.
2.3.1 Flash nanoprecipitation
In the FNP process, the solution stream collides with a nonsolvent stream in a confined mixing chamber, which enables fast mixing on the order of millisecond and creates kinetically trapped NPs typically (Li et al., 2014; Saad and Prud’homme, 2016). Based on FNP process, resveratrol-loaded zein NPs with controllable particle size (50–108 nm) and narrow size distribution (Xing et al., 2022) were fabricated. Similarly, polysaccharide-stabilized zein NPs were fabricated for doxorubicin sustained release (Ye et al., 2022a), and PEG-b-PLA/zein NPs were fabricated for paclitaxel delivery (Ye et al., 2022b).
2.3.2 Microfluidic technique
Microfluidic technique is also getting interest in the fabrication of zein NPs. Compared to FNP process, the flow in the microfluidic process is less turbulent due to the smaller channel diameter. The laminar flow regime of the solvents and predictable flow patterns produce consistent mixing conditions that result in particles of uniform diameter. A Y-junction microfluidics chip with staggered herringbone micromixers was successfully applied for the synthesis of zein NPs (van Ballegooie et al., 2019). And a T-junction configuration of the microfluidic chip was used to fabricate the zein-modified starch nanoparticle complexes for the encapsulation of nisin (Liu X. et al., 2022c). Both studies demonstrated that rapid and tunable microfluidic mixing could be used to continuously and reproducibly synthesize small and homogeneous zein NPs.
3 Characterization of zein-based nanoparticles
For a drug delivery system, there are desired pharmaceutical properties, such as stability, drug release behavior, targeting, pharmacokinetic (PK) and pharmacodynamics (PD) characteristics. As shown in Figure 4, these pharmaceutical properties are directly influenced by the particle nanostructures, such as morphology, particle size, polydispersity index (PDI), surface charge and drug loading. Thus, it is necessary to characterize the particle nanostructures.
3.1 Particle morphology
Particle morphology can be observed using techniques such as scanning electron microscopy (SEM), atomic force microscopy (AFM) and transmission electron microscope (TEM). As an amphiphilic molecule, zein can self-assemble into spheres, micro-capsule, films, rods and particles with other morphologies (Wang et al., 2008; Wang and Padua, 2010; Wang and Padua, 2012a). In most cases, the obtained zein NPs are nanospheres with smooth surface. A possible mechanism for zein self-assembly from single molecules to nanospheres was proposed (Wang and Padua, 2012b). It mainly refers to four steps: α-helix to β-sheet transformation, packing of β-sheet into stripes, curling of stripes into rings, growing and rounding of rings into nanospheres. In some cases, hollow zein NPs are fabricated for their high loading capability, sustained release and low density. For example, hollow zein NPs were fabricated in a two-step process (Xu et al., 2011): preparation of sacrificial cores from sodium carbonate and precipitation of zein onto cores. The obtained hollow zein NPs had average diameters as small as 65 nm, and had 30% higher loading of metformin compared to the solid zein NPs. In another study, crocin loaded hollow dextran sulfate/chitosan-coated zein NPs were fabricated using sodium carbonate as a sacrificing template by a layer-by-layer self-assembly technique (Song et al., 2022).
3.2 Particle size
Particle size is the most important characteristic of nanoparticles in pharmaceutical industry. In general, nanoparticles are applicable to a wide range of biological targets due to their high intracellular absorption. But particles that are ‘too small’ exhibit poor encapsulation efficiency, may migrate from the site of injection, and may exhibit undesirably rapid release of their payload (Gaumet et al., 2008). Particle size is often determined using dynamic light scattering (DLS). Samples analyzed by DLS devices consist of particles that are well-dispersed in liquid media. DLS theory is based on the Brownian motion of particles in suspension, i.e., larger particles move slower than smaller particles at the same temperature. DLS can be applied over a wide size range, from nanometers to micrometers. In the case of polydisperse systems, one can simply determine the mean size and PDI from a cumulant analysis or, by a more complicated method (Glatter, 2018). However, when the particles are irregular and have a strong polydispersity, direct observation and measurement from their SEM or TEM images is suggested.
The particle size of obtained zein particles can vary from nanometers to micrometers under different conditions or preparation methods. The control of particle size is of prime importance in encapsulation and controlled delivery. The size increase of zein NPs during evaporation-induced self-assembly was measured by TEM, and modeled from the hydrophobic and hydrophilic contributions to the interfacial free energy from zein and solvent (Wang and Padua, 2012a). R2 of 0.92 showed their model might allow the prediction of evaporation time and thus control over microsphere size. For self-assembly of zein NPs using BUDP, a semi-empirical model was developed for predicting particle size (Dp50) according to the analysis of influence mechanisms of operating parameters, i.e.,
3.3 Surface charge
Surface charge is a critical element in evaluating the dispersity and stability of nanoparticles in vitro and in vivo. It is often measured by the zeta potential measurement. In general, the absolute zeta potential values >30 mV can prevent the particles from clotting effectively, resulting in the good dispersity and stability of nanoparticles. At the isoelectric point, the zeta potential of particles is zero. Individual zein NPs are prone to destabilization and aggregation in aqueous and physiological fluids, because their neutral isoelectric point (pH 6.2) (Pascoli et al., 2018). The surface charge of zein NPs can be modified by adding surfactants, coating and/or incorporating with other biopolymers. For example, sodium caseinate was used to stabilize zein colloidal particles, which showed a shift of isoelectric point from 6.0 to around pH 5.0 (Patel et al., 2010b). In another study, the complex nanoparticles based on zein and fucoidan were developed to encapsulate resveratrol (Liu et al., 2022b). The obtained particles showed stable colloidal state and no visual aggregation at pH 2.0–8.0. These results are attributed to that fucoidan contains many sulfated groups and can be ionized at pH > 2.0, which provide electrostatic repulsion among particles.
3.4 Intermolecular interactions
The intermolecular interactions among different components of zein NPs are often characterized by circular dichroism spectroscopy, Fourier transform infrared (FT-IR) spectrophotometer and fluorescence spectrum. Circular dichroism spectroscopy is applied to characterize the conformational transitions of zein. When zein is combined with other components, the changes in the secondary structure of zein NPs can be observed (Liu et al., 2022b; Liu et al., 2022c). FTIR spectrum of zein shows peaks at around 3,310 cm−1 (O-H stretching), 1,660 cm−1 (amide I band) and 1,535 cm−1 (amide II band) (Sessa et al., 2013; Yin et al., 2015). Shift of these peaks provide evidence for the formation of hydrogen bonds, hydrophobic interactions, or crosslinking among different components of zein NPs. Fluorescence spectrum also can be applied to investigate intermolecular interactions. Zein contains a high proportion of tyrosine residues, which has a typical emission maximum around 304 nm after being excited at 280 nm. The interaction between zein and other compounds may lead to the micro-environmental alteration, which can be reflected through the fluorescence spectrum (Wei et al., 2019; Zhu et al., 2021).
In some cases, molecular dynamics (MD) simulation and molecular docking are used to analyze the intermolecular interactions. For example, the binding mechanism of zein with epigallocatechin-3-gallate (EGCG) was investigated via multi-spectroscopy and MD simulation (Liu et al., 2021a). MD simulation clarified that the van der Waals and electrostatic interactions were involved in binding of EGCG to zein, and the EGCG preferred to bind to the pocket of zein generated by residues Y171, Q174, L176 and L205. In another study, molecular docking was used to predict the precise binding sites and binding modes of ferulic acid to zein (Wang et al., 2022c), and the results revealed the binding stability under alkaline condition was stronger than that under acidic and neutral conditions.
3.5 Loading of drug
Zein molecule has a very special bricklike structure that provides sufficient space for drug entrapment (Geraghty et al., 1981; Zhang et al., 2016). The loading capacity of zein NPs is often evaluated by DL and EE, calculated using Eqs 1, 2, respectively:
The drug content was measured by a high-performance liquid chromatography system or UV-spectrophotometer. Due to the high hydrophobicity of zein molecule, zein NPs possess high loading capacity for hydrophobic drugs than hydrophilic and amphiphilic drugs (Karthikeyan et al., 2014).
The solid form of drug in zein NPs can be further analyzed by X-ray diffractometer (XRD) and differential scanning calorimeter (DSC). The XRD pattern of zein shows peaks at the diffraction angles of 2θ = 9.2o and 20o, where 2θ = 9.2o arises mainly from the α-helix structure of zein, whereas 2θ = 20o is thought to come from lateral α-helix packing (Chen and Subirade, 2009). Drug can exist in diverse solid forms, such as polymorphs, pseudo-polymorphs, co-crystals and amorphous solids (Liu et al., 2021b). In most cases, the drug is dispersed in zein NPs in an amorphous state. As an interesting study, drug nanocrystals (NC) were successfully incorporated into zein particles by combining the SAS process with BUDP in our previous work (Liu et al., 2016a; Wang et al., 2017), where the co-precipitation of 10-hydroxycamptothecin (HCPT) and zein prepared using the SAS process was dispersed into ethanol-water as the dialysis solution for BUDP. As shown in Figure 5, the obtained HCPT NC-zein particles were microspheres with a mean particle size around 1.0 µm, DL of 5.98% and EE of 95.68%.
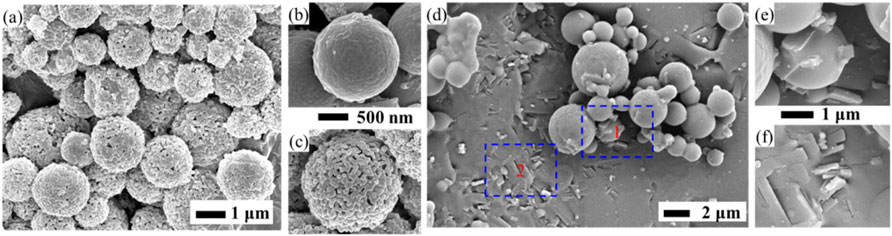
FIGURE 5. SEM images of 10-hydroxycamptothecin nanocrystals (HCPT NC)-zein particles after Na2CO3 treatment (A), magnified images of HCPT NC-Zein particles before (B) and after (C) Na2CO3 treating; HCPT NC-Zein particles partially dissolved with ethanol–water solution (D), magnified images of zone 1 (E) and 2 (F).
4 Pharmaceutical application of zein-based nanoparticles
As shown in Figure 6, a search on the database Web of Science revealed that the number of published articles on zein has been constantly rising in the last decade. About half of the publications on zein are related to research for “nanoparticles” over the past 5 years. And the pharmaceutical application of zein NPs is mainly focus on the drug delivery. Zein NPs can not only protect the drug from the influence of the external environment, improve the drug stability and bioavailability, reduce the drug toxicity and side effects, but more importantly, have a good performance of controlling the drug release and enhancing the drug targeting, thereby improving the drug PK-PD effectively.
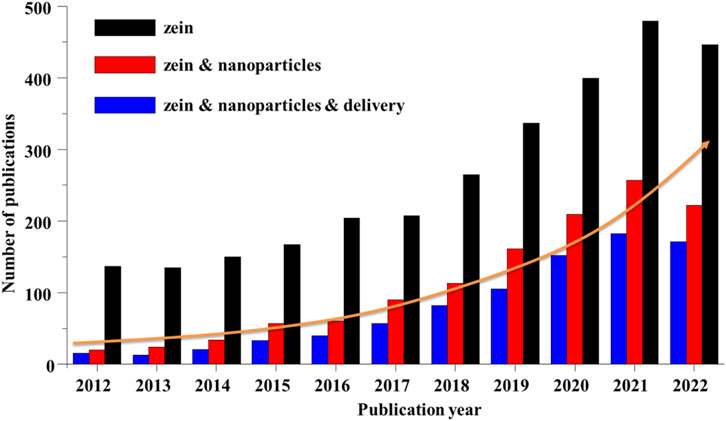
FIGURE 6. Number of publications on zein, zein & nanoparticles, zein & nanoparticles & delivery during the years 2012–2022. All the data were obtained through the Web of Science system as of 12 January 2023.
4.1 Improving the storage stability
Storage stability is vital to the shelf-lives of pharmaceutical products. Zein NPs usually have a smooth surface without pores, strong hydrophobicity and low permeability to oxygen, which can effectively prevent the contact of drug with external environment (e.g., light, oxygen, heat and humidity), thereby improving the storage stability. For example, the storage stability of 7,8-dihydroxyflavone (7,8-DHF) was strengthened greatly after encapsulation in zein NPs, especially with the addition of glycosylated lactoferrin (Chen et al., 2022). Similarly, zein NPs has been used to improving the storage stability of cannabidiol (Wang et al., 2022a), resveratrol (Khan et al., 2021), curcumin (Yuan et al., 2020) and others.
4.2 Increasing the oral bioavailability
The oral route is the preferred way for drug administration due to its advantages on patient compliance, safety, cost-effectiveness and feasibility. However, the complex nature of the gastrointestinal tract offers numerous obstacles, e.g., low pH of the gastric environment, presence of enzymes, intestinal flora and first-pass effect (Alqahtani et al., 2021). Due to their low oral bioavailability, many drugs have to formulate and administer as injections.
Zein NPs have already demonstrated an important potential to increase the oral bioavailability of both small and large molecules. Firstly, zein matrix is an excellent barrier for the protection of drugs (e.g., insulin (Reboredo et al., 2022), antigen (Hurtado-López and Murdan, 2006) and peptide (Khalid Danish et al., 2022)) in the gastrointestinal tract due to its water insoluble in a low pH environment (pH < 11.0). Secondly, the ability of zein in solid dispersion system can enhance drug dissolution rate of poorly water-soluble drug (Nguyen et al., 2017). In most cases, the drug is dispersed in zein NPs in an amorphous state. For the amorphous state, the drug molecules are not organized in a definite lattice pattern, and there is no crystal lattice energy to disrupt during dissolution, resulting in the highest level of dissolution (Liu et al., 2020). Thirdly, zein NPs possess a good bioadhesive nature with biological surfaces through hydrophobic interactions and hydrogen bonding. The mucoadhesive properties of zein NPs could lead to a slow drug release close to the cell membrane and a long retention time in the upper gastrointestinal tract for high drug absorption (Patel et al., 2010a). Moreover, their trans-mucus permeability and transmembrane transport can be further enhanced through the surface coating and modification (Bao et al., 2021).
4.3 Controlling the drug release
Drug release is a significant factor of nanoparticles for successful drug delivery. The drug release behavior is mainly dependent on drug solubility, diffusion, and biodegradation. It can be evaluated by a number of kinetic models, including statistical methods (e.g., exploratory data analysis method, repeated measures design, multivariate approach and multivariate analysis of variance), model dependent methods (e.g., zero order, first order, Higuchi, Korsmeyer-Peppas model, Hixson Crowell, Baker-Lonsdale model and Weibull model) and other model independent methods (Costa and Lobo, 2001; Dash et al., 2010).
Zein has been demonstrated to be an effective excipient in extensive studies for controlled drug delivery due to its hydrophobicity, biodegradability and biocompatibility. Zein is more suitable for sustained release of hydrophobic drugs than hydrophilic and amphiphilic drugs, due to the better binding affinity and greater interactions (hydrophobic, electrostatic, and hydrogen bonding) of hydrophobic drug with zein (Karthikeyan et al., 2014). Recently, the ability of zein in the controlled release of poorly water-soluble drugs has been reviewed (Tran et al., 2019). Moreover, for the controlled release of drug in specific environment, pH-responsive zein NPs have be prepared by coating with tannic acid (Liang et al., 2018), PDA (Zha et al., 2020), sodium caseinate and poly ethylene imine (Pourhossein et al., 2020). Also, there are few studies focus on the controlled release of zein NPs by other specific stimuli (Marín et al., 2018; Zhong et al., 2022).
4.4 Enhancing the drug targeting
Targeted drug delivery that refers to predominant drug accumulation within a target zone, is an effective approach to decreasing drug toxicity and enhancing therapeutic effects. The targeting of drugs can be viewed on two levels: organ targeting and cellular targeting (Koo et al., 2005; Manzari et al., 2021). The organ targeting is mainly dependent on the particle size, morphology and material properties of the carrier employed. Zein NPs have capabilities of size-dependent organ targeting through leaky tumor capillary fenestrations into a tumor vasculature according to the enhanced permeability and retention (EPR) effect (Maeda, 2015). For example, the organ targeting of 5-fluorouracil loaded zein NPs was studied (Lai and Guo, 2011): the targeting efficiency of drug in liver increased from 22.67% to 54.00% after the encapsulation, and the relative uptake rate (zein NPs/plain drug solution) was in the order of liver (2.79) > spleen (1.94) > plasma (1.09) > lung (0.70) > heart (0.34) > kidney (0.24), indicating that zein NPs was specific to the liver tissue. The development of magnetic NPs can further enhance the delivery and controlled release of a drug to the targeting organ, especially the fabrication of temperature and pH-responsive magnetic NPs (Marín et al., 2018; Pang et al., 2018).
In spite of the preferred accumulation of zein NPs through organ targeting mechanisms, there is some degree of non-specific action in this method. It is of critical importance to develop the cellular targeting zein NPs through a more specific interaction at a molecular level between the carrier and the targeted cell (Byrne et al., 2008). There are abundant hydroxyl, amino and carboxyl groups on the side chains of zein molecular, which offer the possibility of conjugation and modification of zein molecules with targeting ligands or molecules (Allen, 2002; Xu et al., 2013). For example, hyaluronic acid (HA) cross-linked zein NPs were prepared (Seok et al., 2018; Zhang et al., 2020). Given the high targetability of HA to CD44 expressing cancer cells, the obtained HA-zein NPs could enter tumor cells effectively via a CD44 mediated endocytosis thereby enhancing the PK/PD. In our previous work (Liu et al., 2017), zein was decorated with folic acid (FA) for targeted delivery of HCPT. As shown in Figure 7, FA conjugation could not only facilitate the formation of small nanoparticles with good dispersity and stability, sustain the drug release, but more importantly improve the cellular up-take in folate receptor positive cells thereby enhancing the antitumor activity.
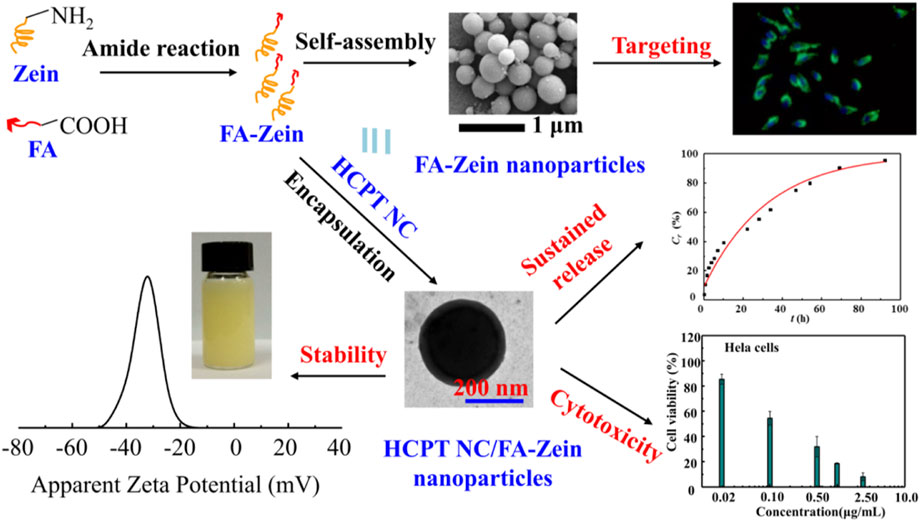
FIGURE 7. Synthesis and characterization of folic acid (FA) cross-linked zein nanoparticles for the targeted delivery of 10-hydroxycamptothecin (HCPT).
5 Future perspectives
Although zein NPs have made a great progress in their preparation, characterization and pharmaceutical application, they have yet to gain a foothold in the market up to now. Some issues remain to be considered before their scale-up production and clinical applications: 1) the effective strategy for the chemical modification and surface coating of zein NPs; 2) the precise preparation of zein NPs with desired nanostructures; 3) correlation of in vitro and in vivo characteristics; 4) evaluation of the PK-PD relationship and clinical feasibility.
In Figure 8, a simple roadmap for the research and development of zein NPs is put forward on the basis of quality-by-design (QbD) approach. QbD approach is useful to the development of standardized procedures for getting the optimized product (Bastogne, 2017; Cunha et al., 2020; Soni et al., 2020). The quality target product profile (QTPP) of zein NPs can be specified firstly according to their pharmaceutical application. Then, critical quality attributes (CQAs) are selected from parameters that interfere with QTPP. In general, pharmaceutical properties are directly influenced by the particle nanostructures. CQAs of zein NPs can be further related to their nanostructures. After that, zein NPs with desired CQAs can be obtained by controlling the critical process parameters (CPPs) of preparation method and the critical material attributes (CMAs) of materials. And the design space of CPPs and CMAs is defined and optimized by risk assessment, design of experiment and process analytical technology.
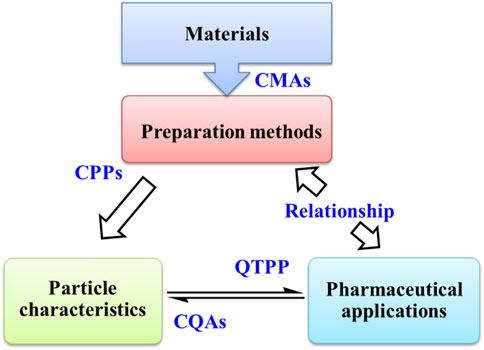
FIGURE 8. The relationship among preparation methods, particle characteristics and pharmaceutical applications of zein-based nanoparticles in virtue of quality by design approach. CMAs: critical material attributes, CPPs: critical process parameters, CQAs: critical quality attributes, QTPP: quality target product profile.
6 Conclusion
Zein is a hydrophobic plant protein extracted from corn gluten meal. It possesses many favorable features as a natural building block for the development of drug delivery systems, e.g., a GRAS state, unique solubility and self-assembly properties. Zein NPs can be prepared by chemical crosslinking strategy, desolvating strategy (solvent/nonsolvent method, coacervation method, pH-driven method and dialysis method), dispersing strategy (liquid-liquid dispersion, spray drying, electrospraying, SAS process and AAP process) and micromixing strategy (FNP process and microfluidic technique). The obtained zein NPs exhibit different particle nanostructures, such as morphology, particle size, surface charge, intermolecular interactions and drug loading, which can well characterized by modern analysis methods. Zein NPs can be applied in pharmaceutical preparations to improve the drug stability, increase the oral bioavailability, control the drug release and enhance the drug targeting, thereby improving the drug PK-PD effectively. More studies in the future should deeply analyze the relationship among preparation methods, particle nanostructures and pharmaceutical properties in virtue of QbD approach.
Author contributions
GL and JL contributed to conception and investigation. GL wrote the first draft of the manuscript. DA wrote sections of the manuscript. SD provided advice and supervision. All authors contributed to manuscript revision, read, and approved the submitted version.
Funding
Financial supports from the National Natural Science Foundation of China (No. 22008046), the Natural Science Foundation of Hainan Province (No. 820QN249), and the Scientific Research Foundation of Hainan University [KYQD(ZR)1925] are greatly appreciated.
Conflict of interest
The authors declare that the research was conducted in the absence of any commercial or financial relationships that could be construed as a potential conflict of interest.
Publisher’s note
All claims expressed in this article are solely those of the authors and do not necessarily represent those of their affiliated organizations, or those of the publisher, the editors and the reviewers. Any product that may be evaluated in this article, or claim that may be made by its manufacturer, is not guaranteed or endorsed by the publisher.
References
Allen, T. M. (2002). Ligand-targeted therapeutics in anticancer therapy. Nat. Rev. Cancer 2 (10), 750–763. doi:10.1038/nrc903
Alqahtani, M. S., Kazi, M., Alsenaidy, M. A., and Ahmad, M. Z. (2021). Advances in oral drug delivery. Front. Pharmacol. 12, 618411. doi:10.3389/fphar.2021.618411
Bao, X., Qian, K., and Yao, P. (2021). Insulin- and cholic acid-loaded zein/casein–dextran nanoparticles enhance the oral absorption and hypoglycemic effect of insulin. J. Mater. Chem. B 9 (31), 6234–6245. doi:10.1039/D1TB00806D
Bastogne, T. (2017). Quality-by-design of nanopharmaceuticals – A state of the art. Nanomedicine Nanotechnol. Biol. Med. 13 (7), 2151–2157. doi:10.1016/j.nano.2017.05.014
Byrne, J. D., Betancourt, T., and Brannon-Peppas, L. (2008). Active targeting schemes for nanoparticle systems in cancer therapeutics. Adv. Drug Deliv. Rev. 60 (15), 1615–1626. doi:10.1016/j.addr.2008.08.005
Chen, L., and Subirade, M. (2009). Elaboration and characterization of soy/zein protein microspheres for controlled nutraceutical delivery. Biomacromolecules 10 (12), 3327–3334. doi:10.1021/bm900989y
Chen, Y., Gao, X., Liu, S., Cai, Q., Wu, L., Sun, Y., et al. (2022). Establishment and characterization of stable zein/glycosylated lactoferrin nanoparticles to enhance the storage stability and in vitro bioaccessibility of 7,8-dihydroxyflavone. Front. Nutr. 8, 806623. doi:10.3389/fnut.2021.806623
Chen, Y., Xia, G., Zhao, Z., Xue, F., Chen, C., and Zhang, Y. (2020). Formation, structural characterization, stability and in vitro bioaccessibility of 7,8-dihydroxyflavone loaded zein-/sophorolipid composite nanoparticles: Effect of sophorolipid under two blending sequences. Food and Funct. 11 (2), 1810–1825. doi:10.1039/C9FO02704A
Costa, P., and Lobo, J. M. S. (2001). Modeling and comparison of dissolution profiles. Eur. J. Pharm. Sci. 13 (2), 123–133. doi:10.1016/s0928-0987(01)00095-1
Cunha, S., Costa, C. P., Moreira, J. N., Sousa Lobo, J. M., and Silva, A. C. (2020). Using the quality by design (QbD) approach to optimize formulations of lipid nanoparticles and nanoemulsions: A review. Nanomedicine Nanotechnol. Biol. Med. 28, 102206. doi:10.1016/j.nano.2020.102206
Dai, L., Zhou, H., Wei, Y., Gao, Y., and McClements, D. J. (2019). Curcumin encapsulation in zein-rhamnolipid composite nanoparticles using a pH-driven method. Food Hydrocoll. 93, 342–350. doi:10.1016/j.foodhyd.2019.02.041
Dash, S., Murthy, P. N., Nath, L., and Chowdhury, P. (2010). Kinetic modeling on drug release from controlled drug delivery systems. Acta Pol. Pharm. 67 (3), 217–223.
Demir, M., Ramos-Rivera, L., Silva, R., Nazhat, S. N., and Boccaccini, A. R. (2017). Zein-based composites in biomedical applications. J. Biomed. Mater. Res. Part A 105 (6), 1656–1665. doi:10.1002/jbm.a.36040
Evans, C. D., and Manley, R. H. (1941). Solvents for zein. Primary solvents. Ind. Eng. Chem. 33, 1416–1417. doi:10.1021/ie50383a019
Franco, P., Reverchon, E., and De Marco, I. (2018). Zein/diclofenac sodium coprecipitation at micrometric and nanometric range by supercritical antisolvent processing. J. CO2 Util. 27, 366–373. doi:10.1016/j.jcou.2018.08.015
Fu, J.-X., Wang, H.-J., Zhou, Y.-Q., and Wang, J.-Y. (2009). Antibacterial activity of ciprofloxacin-loaded zein microsphere films. Mater. Sci. Eng. C 29 (4), 1161–1166. doi:10.1016/j.msec.2008.09.031
Fu, W., Liang, Y., Xie, Z., Wu, H., Zhang, Z., and Lv, H. (2021). Preparation and evaluation of lecithin/zein hybrid nanoparticles for the oral delivery of Panax notoginseng saponins. Eur. J. Pharm. Sci. 164, 105882. doi:10.1016/j.ejps.2021.105882
Gaumet, M., Vargas, A., Gurny, R., and Delie, F. (2008). Nanoparticles for drug delivery: The need for precision in reporting particle size parameters. Eur. J. Pharm. Biopharm. 69 (1), 1–9. doi:10.1016/j.ejpb.2007.08.001
Geraghty, D., Peifer, M. A., Rubenstein, I., and Messing, J. (1981). The primary structure of a plant storage protein: Zein. Nucleic Acids Res. 9, 5163–5174. doi:10.1093/nar/9.19.5163
Glatter, O. (2018). “Chapter 11 - dynamic light scattering (DLS),” in Scattering methods and their application in colloid and interface science. Editor O. Glatter (Elsevier), 223–263.
Gomez-Estaca, J., Balaguer, M. P., Gavara, R., and Hernandez-Munoz, P. (2012). Formation of zein nanoparticles by electrohydrodynamic atomization: Effect of the main processing variables and suitability for encapsulating the food coloring and active ingredient curcumin. Food Hydrocoll. 28 (1), 82–91. doi:10.1016/j.foodhyd.2011.11.013
Hassan, E. A., Hathout, R. M., Gad, H. A., and Sammour, O. A. (2022). A holistic review on zein nanoparticles and their use in phytochemicals delivery. J. Drug Deliv. Sci. Technol. 73, 103460. doi:10.1016/j.jddst.2022.103460
Hong, S., Choi, D. W., Kim, H. N., Park, C. G., Lee, W., and Park, H. H. (2020). Protein-based nanoparticles as drug delivery systems. Pharmaceutics 12 (7), 604. doi:10.3390/pharmaceutics12070604
Hu, D., Lin, C., Liu, L., Li, S., and Zhao, Y. (2012). Preparation, characterization, and in vitro release investigation of lutein/zein nanoparticles via solution enhanced dispersion by supercritical fluids. J. Food Eng. 109 (3), 545–552. doi:10.1016/j.jfoodeng.2011.10.025
Hurtado-López, P., and Murdan, S. (2006). Zein microspheres as drug/antigen carriers: A study of their degradation and erosion, in the presence and absence of enzymes. J. Microencapsul. 23 (3), 303–314. doi:10.1080/02652040500444149
Irache, J. M., and Gonzalez-Navarro, C. J. (2017). Zein nanoparticles as vehicles for oral delivery purposes. NANOMEDICINE 12 (11), 1209–1211. doi:10.2217/nnm-2017-0075
Karthikeyan, K., Lakra, R., Rajaram, R., and Korrapati, P. S. (2012). Development and characterization of zein-based micro carrier system for sustained delivery of aceclofenac sodium. AAPS PharmSciTech 13 (1), 143–149. doi:10.1208/s12249-011-9731-x
Karthikeyan, K., Vijayalakshmi, E., and Korrapati, P. S. (2014). Selective interactions of zein microspheres with different class of drugs: An in vitro and in silico analysis. AAPS PharmSciTech 15 (5), 1172–1180. doi:10.1208/s12249-014-0151-6
Kasaai, M. R. (2018). Zein and zein -based nano-materials for food and nutrition applications: A review. Trends Food Sci. Technol. 79, 184–197. doi:10.1016/j.tifs.2018.07.015
Khalid Danish, M., Gleeson, J. P., Brayden, D. J., Byrne, H. J., Frías, J. M., and Ryan, S. M. (2022). Formulation, characterisation and evaluation of the antihypertensive peptides, isoleucine-proline-proline and leucine-lysine-proline in chitosan nanoparticles coated with zein for oral drug delivery. Int. J. Mol. Sci. 23 (19), 11160. doi:10.3390/ijms231911160
Khan, M. A., Chen, L., and Liang, L. (2021). Improvement in storage stability and resveratrol retention by fabrication of hollow zein-chitosan composite particles. Food Hydrocoll. 113, 106477. doi:10.1016/j.foodhyd.2020.106477
Khan, M. A., Yue, C., Fang, Z., Hu, S., Cheng, H., Bakry, A. M., et al. (2019). Alginate/chitosan-coated zein nanoparticles for the delivery of resveratrol. J. Food Eng. 258, 45–53. doi:10.1016/j.jfoodeng.2019.04.010
Koo, O. M., Rubinstein, I., and Onyuksel, H. (2005). Role of nanotechnology in targeted drug delivery and imaging: A concise review. Nanomedicine 1 (3), 193–212. doi:10.1016/j.nano.2005.06.004
Lai, L. F., and Guo, H. X. (2011). Preparation of new 5-fluorouracil-loaded zein nanoparticles for liver targeting. Int. J. Pharm. 404 (1–2), 317–323. doi:10.1016/j.ijpharm.2010.11.025
Lau, E. T., Johnson, S. K., Mikkelsen, D., Halley, P. J., and Steadman, K. J. (2012). Preparation and in vitro release of zein microparticles loaded with prednisolone for oral delivery. J. Microencapsul. 29 (7), 706–712. doi:10.3109/02652048.2012.686527
Li, K.-K., Zhang, X., Huang, Q., Yin, S.-W., Yang, X.-Q., Wen, Q.-B., et al. (2014). Continuous preparation of zein colloidal particles by Flash NanoPrecipitation (FNP). J. Food Eng. 127, 103–110. doi:10.1016/j.jfoodeng.2013.12.001
Li, M., Liu, Y., Liu, Y., Zhang, X., Han, D., and Gong, J. (2022). pH-driven self-assembly of alcohol-free curcumin-loaded zein-propylene glycol alginate complex nanoparticles. Int. J. Biol. Macromol. 213, 1057–1067. doi:10.1016/j.ijbiomac.2022.06.046
Li, S., and Zhao, Y. (2017). Preparation of zein nanoparticles by using solution-enhanced dispersion with supercritical CO(2) and elucidation with computational fluid dynamics. Int. J. Nanomedicine 12, 3485–3494. doi:10.2147/ijn.s135239
Liang, H., Zhou, B., Li, J., Liu, X., Deng, Z., and Li, B. (2018). Engineering multifunctional coatings on nanoparticles based on oxidative coupling assembly of polyphenols for stimuli-responsive drug delivery. J. Agric. Food Chem. 66 (26), 6897–6905. doi:10.1021/acs.jafc.8b01208
Liu, C., Lv, N., Ren, G., Wu, R., Wang, B., Cao, Z., et al. (2021a). Explore the interaction mechanism between zein and EGCG using multi-spectroscopy and molecular dynamics simulation methods. Food Hydrocoll. 120, 106906. doi:10.1016/j.foodhyd.2021.106906
Liu, G., Gong, L., Zhang, J., Wu, Z., Deng, H., and Deng, S. (2020). Development of nimesulide amorphous solid dispersions via supercritical anti-solvent process for dissolution enhancement. Eur. J. Pharm. Sci. 152, 105457. doi:10.1016/j.ejps.2020.105457
Liu, G., Jiang, Y., and Wang, X. (2015). Tailoring particle microstructures via supercritical CO₂ processes for particular drug delivery. Curr. Pharm. Des. 21 (19), 2543–2562. doi:10.2174/1381612821666150416101116
Liu, G., Li, J., and Deng, S. (2021b). Applications of supercritical anti-solvent process in preparation of solid multicomponent systems. Pharmaceutics 13 (4), 475. doi:10.3390/pharmaceutics13040475
Liu, G., Li, S., Huang, Y., Wang, H., and Jiang, Y. (2016a). Incorporation of 10-hydroxycamptothecin nanocrystals into zein microspheres. Chem. Eng. Sci. 155, 405–414. doi:10.1016/j.ces.2016.08.029
Liu, G., Pang, J., Huang, Y., Xie, Q., Guan, G., and Jiang, Y. (2017). Self-assembled nanospheres of folate-decorated zein for the targeted delivery of 10-hydroxycamptothecin. Industrial Eng. Chem. Res. 56 (30), 8517–8527. doi:10.1021/acs.iecr.7b01632
Liu, G., Wang, H., and Jiang, Y. (2013). Recrystallization and micronization of camptothecin by the supercritical antisolvent process: Influence of solvents. Industrial Eng. Chem. Res. 52 (43), 15049–15056. doi:10.1021/ie401173g
Liu, G., Wei, D., Wang, H., Hu, Y., and Jiang, Y. (2016b). Self-assembly of zein microspheres with controllable particle size and narrow distribution using a novel built-in ultrasonic dialysis process. Chem. Eng. J. 284, 1094–1105. doi:10.1016/j.cej.2015.09.067
Liu, Q., Li, Y., Zhou, Y., Jiang, L., Lyu, Q., Liu, G., et al. (2022a). Zein-whey protein isolate-carboxymethyl cellulose complex as carrier of apigenin via pH-driven method: Fabrication, characterization, stability, and in vitro release property. Food Chem. 387, 132926. doi:10.1016/j.foodchem.2022.132926
Liu, Q., Qin, Y., Jiang, B., Chen, J., and Zhang, T. (2022b). Development of self-assembled zein-fucoidan complex nanoparticles as a delivery system for resveratrol. Colloids Surfaces B Biointerfaces 216, 112529. doi:10.1016/j.colsurfb.2022.112529
Liu, X., Ibarra-Sánchez, L. A., Miller, M. J., and Lee, Y. (2022c). Fabrication of zein-modified starch nanoparticle complexes via microfluidic chip and encapsulation of nisin. Curr. Res. Food Sci. 5, 1110–1117. doi:10.1016/j.crfs.2022.07.005
Liu, X., Sun, Q., Wang, H., Zhang, L., and Wang, J.-Y. (2005). Microspheres of corn protein, zein, for an ivermectin drug delivery system. Biomaterials 26 (1), 109–115. doi:10.1016/j.biomaterials.2004.02.013
Liu, Z.-P., Zhang, Y.-Y., Yu, D.-G., Wu, D., and Li, H.-L. (2018). Fabrication of sustained-release zein nanoparticles via modified coaxial electrospraying. Chem. Eng. J. 334, 807–816. doi:10.1016/j.cej.2017.10.098
Maeda, H. (2015). Toward a full understanding of the EPR effect in primary and metastatic tumors as well as issues related to its heterogeneity. Adv. Drug Deliv. Rev. 91, 3–6. doi:10.1016/j.addr.2015.01.002
Mahalakshmi, L., Leena, M. M., Moses, J. A., and Anandharamakrishnan, C. (2020). Micro- and nano-encapsulation of β-carotene in zein protein: Size-dependent release and absorption behavior. Food and Funct. 11 (2), 1647–1660. doi:10.1039/C9FO02088H
Malik, S., Subramanian, S., Hussain, T., Nazir, A., and Ramakrishna, S. (2022). Electrosprayed nanoparticles as drug delivery systems for biomedical applications. Curr. Pharm. Des. 28 (5), 368–379. doi:10.2174/1381612827666210929114621
Manley, R. H., and Evans, C. D. (1943). Binary solvents for zein. Ind. Eng. Chem. 35, 661–665. doi:10.1021/ie50402a008
Manzari, M. T., Shamay, Y., Kiguchi, H., Rosen, N., Scaltriti, M., and Heller, D. A. (2021). Targeted drug delivery strategies for precision medicines. Nat. Rev. Mater. 6 (4), 351–370. doi:10.1038/s41578-020-00269-6
Marín, T., Montoya, P., Arnache, O., Pinal, R., and Calderón, J. (2018). Bioactive films of zein/magnetite magnetically stimuli-responsive for controlled drug release. J. Magnetism Magnetic Mater. 458, 355–364. doi:10.1016/j.jmmm.2018.03.046
Matsuda, Y., Suzuki, T., Sato, E., Sato, M., Koizumi, S., Unno, K., et al. (1989). Novel preparation of zein microspheres conjugated with PS-K available for cancer immunotherapy. Chem. Pharm. Bull. 37 (3), 757–759. doi:10.1248/cpb.37.757
Mitchell, M. J., Billingsley, M. M., Haley, R. M., Wechsler, M. E., Peppas, N. A., and Langer, R. (2021). Engineering precision nanoparticles for drug delivery. Nat. Rev. Drug Discov. 20 (2), 101–124. doi:10.1038/s41573-020-0090-8
Mohammed, N. K., Tan, C. P., Manap, Y. A., Muhialdin, B. J., and Hussin, A. S. M. (2020). Spray drying for the encapsulation of oils—a review. Molecules 25 (17), 3873. doi:10.3390/molecules25173873
Muthuselvi, L., and Dhathathreyan, A. (2006). Simple coacervates of zein to encapsulate Gitoxin. Colloids Surfaces B Biointerfaces 51 (1), 39–43. doi:10.1016/j.colsurfb.2006.05.012
Nguyen, M. N.-U., Van Vo, T., Tran, P. H.-L., and Tran, T. T.-D. (2017). Zein-based solid dispersion for potential application in targeted delivery. J. Pharm. Investigation 47 (4), 357–364. doi:10.1007/s40005-017-0314-z
Paliwal, R., and Palakurthi, S. (2014). Zein in controlled drug delivery and tissue engineering. J. Control. Release 189, 108–122. doi:10.1016/j.jconrel.2014.06.036
Pang, J., Li, Z., Li, S., Lin, S., Wang, H., Xie, Q., et al. (2018). Folate-conjugated zein/Fe3O4 nanocomplexes for the enhancement of cellular uptake and cytotoxicity of gefitinib. J. Mater. Sci. 53 (21), 14907–14921. doi:10.1007/s10853-018-2684-7
Pascoli, M., de Lima, R., and Fraceto, L. F. (2018). Zein nanoparticles and strategies to improve colloidal stability: A mini-review. Front. Chem. 6, 6. doi:10.3389/fchem.2018.00006
Patel, A., Hu, Y., Tiwari, J. K., and Velikov, K. P. (2010a). Synthesis and characterisation of zein–curcumin colloidal particles. Soft Matter 6 (24), 6192–6199. doi:10.1039/c0sm00800a
Patel, A. R., Bouwens, E. C. M., and Velikov, K. P. (2010b). Sodium caseinate stabilized zein colloidal particles. J. Agric. Food Chem. 58 (23), 12497–12503. doi:10.1021/jf102959b
Pauluk, D., Padilha, A. K., Khalil, N. M., and Mainardes, R. M. (2019). Chitosan-coated zein nanoparticles for oral delivery of resveratrol: Formation, characterization, stability, mucoadhesive properties and antioxidant activity. Food Hydrocoll. 94, 411–417. doi:10.1016/j.foodhyd.2019.03.042
Pourhossein, A., Rafizadeh, M., and Chen, P. (2020). Stimuli-responsive zein-based nanoparticles as a potential carrier for ellipticine: Synthesis, release, and in vitro delivery. Polym. Adv. Technol. 31 (9), 2007–2019. doi:10.1002/pat.4924
Reboredo, C., González-Navarro, C. J., Martínez-López, A. L., Martínez-Ohárriz, C., Sarmento, B., and Irache, J. M. (2022). Zein-based nanoparticles as oral carriers for insulin delivery. Pharmaceutics 14 (1), 39. doi:10.3390/pharmaceutics14010039
Rodríguez-Félix, F., Del-Toro-Sánchez, C. L., and Tapia-Hernández, J. A. (2020). A new design for obtaining of white zein micro- and nanoparticles powder: Antisolvent-dialysis method. Food Sci. Biotechnol. 29 (5), 619–629. doi:10.1007/s10068-019-00702-9
Saad, W. S., and Prud’homme, R. K. (2016). Principles of nanoparticle formation by flash nanoprecipitation. Nano Today 11 (2), 212–227. doi:10.1016/j.nantod.2016.04.006
Seok, H.-Y., Sanoj Rejinold, N., Lekshmi, K. M., Cherukula, K., Park, I.-K., and Kim, Y.-C. (2018). CD44 targeting biocompatible and biodegradable hyaluronic acid cross-linked zein nanogels for curcumin delivery to cancer cells: In vitro and in vivo evaluation. J. Control. Release 280, 20–30. doi:10.1016/j.jconrel.2018.04.050
Sessa, D. J., Cheng, H., Kim, S., Selling, G. W., and Biswas, A. (2013). Zein-based polymers formed by modifications with isocyanates. Industrial Crops Prod. 43, 106–113. doi:10.1016/j.indcrop.2012.06.034
Shukla, R., and Cheryan, M. (2001). Zein: The industrial protein from corn. Industrial crops Prod. 13 (3), 171–192. doi:10.1016/s0926-6690(00)00064-9
Song, G., Liu, J., Wang, Q., Wang, D., Chu, B., Li, L., et al. (2022). Layer-by-layer self-assembly of hollow dextran sulfate/chitosan-coated zein nanoparticles loaded with crocin: Fabrication, structural characterization and potential biological fate. Food Hydrocoll. 125, 107420. doi:10.1016/j.foodhyd.2021.107420
Soni, G., Kale, K., Shetty, S., Gupta, M. K., and Yadav, K. S. (2020). Quality by design (QbD) approach in processing polymeric nanoparticles loading anticancer drugs by high pressure homogenizer. Heliyon 6 (4), e03846. doi:10.1016/j.heliyon.2020.e03846
Sosnik, A., and Seremeta, K. P. (2015). Advantages and challenges of the spray-drying technology for the production of pure drug particles and drug-loaded polymeric carriers. Adv. Colloid Interface Sci. 223, 40–54. doi:10.1016/j.cis.2015.05.003
Suzuki, T., Sato, E., Matsuda, T., Tada, H., Unno, K., and Kato, T. (1989). Preparation of zein microspheres conjugated with antitumor drugs available for selective cancer chemotherapy and development of a simple colorimetric determination of drugs in microspheres. Chem. Pharm. Bull. 37, 1051–1054. doi:10.1248/cpb.37.1051
Tran, P. H. L., Duan, W., Lee, B.-J., and Tran, T. T. D. (2019). The use of zein in the controlled release of poorly water-soluble drugs. Int. J. Pharm. 566, 557–564. doi:10.1016/j.ijpharm.2019.06.018
van Ballegooie, C., Man, A., Andreu, I., Gates, B. D., and Yapp, D. (2019). Using a microfluidics system to reproducibly synthesize protein nanoparticles: Factors contributing to size, homogeneity, and stability. Processes 7 (5), 290. doi:10.3390/pr7050290
Wang, C., Wang, J., Sun, Y., Freeman, K., Mchenry, M. A., Wang, C., et al. (2022a). Enhanced stability and oral bioavailability of cannabidiol in zein and whey protein composite nanoparticles by a modified anti-solvent approach. Foods 11 (3), 376. doi:10.3390/foods11030376
Wang, F., Elbadawi, M., Tsilova, S. L., Gaisford, S., Basit, A. W., and Parhizkar, M. (2022b). Machine learning predicts electrospray particle size. Mater. Des. 219, 110735. doi:10.1016/j.matdes.2022.110735
Wang, H., Zhang, X., Zhu, W., Jiang, Y., and Zhang, Z. (2018). Self-assembly of zein-based microcarrier system for colon-targeted oral drug delivery. Industrial Eng. Chem. Res. 57 (38), 12689–12699. doi:10.1021/acs.iecr.8b02092
Wang, H., Zhu, W., Huang, Y., Li, Z., Jiang, Y., and Xie, Q. (2017). Facile encapsulation of hydroxycamptothecin nanocrystals into zein-based nanocomplexes for active targeting in drug delivery and cell imaging. Acta Biomater. 61, 88–100. doi:10.1016/j.actbio.2017.04.017
Wang, Q., Tang, Y., Yang, Y., Lei, L., Lei, X., Zhao, J., et al. (2022c). The interaction mechanisms, and structural changes of the interaction between zein and ferulic acid under different pH conditions. Food Hydrocoll. 124, 107251. doi:10.1016/j.foodhyd.2021.107251
Wang, Q., Yin, L., and Padua, G. W. (2008). Effect of hydrophilic and lipophilic compounds on zein microstructures. Food Biophys. 3 (2), 174–181. doi:10.1007/s11483-008-9080-9
Wang, Y., and Padua, G. W. (2010). formation of zein microphases in Ethanol− water. Langmuir 26 (15), 12897–12901. doi:10.1021/la101688v
Wang, Y., and Padua, G. W. (2012a). Formation of zein spheres by evaporation-induced self-assembly. Colloid Polym. Sci. 290 (15), 1593–1598. doi:10.1007/s00396-012-2749-0
Wang, Y., and Padua, G. W. (2012b). Nanoscale characterization of zein self-assembly. Langmuir 28 (5), 2429–2435. doi:10.1021/la204204j
Wei, Y., Guo, A., Liu, Z., Zhang, L., Liao, W., Liu, J., et al. (2021). Development of curcumin loaded core–shell zein microparticles stabilized by cellulose nanocrystals and whey protein microgels through interparticle interactions. Food and Funct. 12 (15), 6936–6949. doi:10.1039/D1FO00959A
Wei, Y., Yu, Z., Lin, K., Sun, C., Dai, L., Yang, S., et al. (2019). Fabrication and characterization of resveratrol loaded zein-propylene glycol alginate-rhamnolipid composite nanoparticles: Physicochemical stability, formation mechanism and in vitro digestion. Food Hydrocoll. 95, 336–348. doi:10.1016/j.foodhyd.2019.04.048
Wu, Z., Li, J., Zhang, X., Li, Y., Wei, D., Tang, L., et al. (2022). Rational fabrication of folate-conjugated zein/soy lecithin/carboxymethyl chitosan core–shell nanoparticles for delivery of docetaxel. ACS Omega 7 (15), 13371–13381. doi:10.1021/acsomega.2c01270
Xiao, D., Davidson, P. M., and Zhong, Q. (2011). Release and antilisterial properties of nisin from zein capsules spray-dried at different temperatures. LWT-Food Sci. Technol. 44 (10), 1977–1985. doi:10.1016/j.lwt.2011.07.017
Xing, M., Zhao, H., Ahmed, R., Wang, X., Liu, J., Wang, J., et al. (2022). Fabrication of resveratrol-loaded zein nanoparticles based on flash nanoprecipitation. Colloids Surfaces A Physicochem. Eng. Aspects 654, 129829. doi:10.1016/j.colsurfa.2022.129829
Xu, H., Jiang, Q., Reddy, N., and Yang, Y. (2011). Hollow nanoparticles from zein for potential medical applications. J. Mater. Chem. 21 (45), 18227–18235. doi:10.1039/c1jm11163a
Xu, S., Olenyuk, B. Z., Okamoto, C. T., and Hamm-Alvarez, S. F. (2013). Targeting receptor-mediated endocytotic pathways with nanoparticles: Rationale and advances. Adv. Drug Deliv. Rev. 65 (1), 121–138. doi:10.1016/j.addr.2012.09.041
Yan, X., Li, M., Xu, X., Liu, X., and Liu, F. (2022). Zein-based nano-delivery systems for encapsulation and protection of hydrophobic bioactives: A review. Front. Nutr. 9, 999373. doi:10.3389/fnut.2022.999373
Ye, W., Zhang, G., Liu, X., Ren, Q., Huang, F., and Yan, Y. (2022a). Fabrication of polysaccharide-stabilized zein nanoparticles by flash nanoprecipitation for doxorubicin sustained release. J. Drug Deliv. Sci. Technol. 70, 103183. doi:10.1016/j.jddst.2022.103183
Ye, W., Zhu, F., Cai, Y., Wang, L., Zhang, G., Zhao, G., et al. (2022b). Improved paclitaxel delivery with PEG-b-PLA/zein nanoparticles prepared via flash nanoprecipitation. Int. J. Biol. Macromol. 221, 486–495. doi:10.1016/j.ijbiomac.2022.09.021
Yin, H., Lu, T., Liu, L., and Lu, C. (2015). Preparation, characterization and application of a novel biodegradable macromolecule: Carboxymethyl zein. Int. J. Biol. Macromol. 72, 480–486. doi:10.1016/j.ijbiomac.2014.08.025
Yuan, Y., Li, H., Zhu, J., Liu, C., Sun, X., Wang, D., et al. (2020). Fabrication and characterization of zein nanoparticles by dextran sulfate coating as vehicles for delivery of curcumin. Int. J. Biol. Macromol. 151, 1074–1083. doi:10.1016/j.ijbiomac.2019.10.149
Yuan, Y., Ma, M., Xu, Y., and Wang, D. (2022). Surface coating of zein nanoparticles to improve the application of bioactive compounds: A review. Trends Food Sci. Technol. 120, 1–15. doi:10.1016/j.tifs.2021.12.025
Yuan, Y., Xiao, J., Zhang, P., Ma, M., Wang, D., and Xu, Y. (2021). Development of pH-driven zein/tea saponin composite nanoparticles for encapsulation and oral delivery of curcumin. Food Chem. 364, 130401. doi:10.1016/j.foodchem.2021.130401
Zha, L., Qian, J., Wang, B., Liu, H., Zhang, C., Dong, Q., et al. (2020). In vitro/in vivo evaluation of pH-sensitive Gambogenic acid loaded Zein nanoparticles with polydopamine coating. Int. J. Pharm. 587, 119665. doi:10.1016/j.ijpharm.2020.119665
Zhan, X., Dai, L., Zhang, L., and Gao, Y. (2020). Entrapment of curcumin in whey protein isolate and zein composite nanoparticles using pH-driven method. Food Hydrocoll. 106, 105839. doi:10.1016/j.foodhyd.2020.105839
Zhang, Q., Wang, J., Liu, D., Zhu, W., Guan, S., Fan, L., et al. (2020). Targeted delivery of honokiol by zein/hyaluronic acid core-shell nanoparticles to suppress breast cancer growth and metastasis. Carbohydr. Polym. 240, 116325. doi:10.1016/j.carbpol.2020.116325
Zhang, Y., Cui, L., Li, F., Shi, N., Li, C., Yu, X., et al. (2016). Design, fabrication and biomedical applications of zein-based nano/micro-carrier systems. Int. J. Pharm. 513 (1-2), 191–210. doi:10.1016/j.ijpharm.2016.09.023
Zhao, Y., Han, X., Yin, H., Li, Q., Zhou, J., Zhang, H., et al. (2022). Preparation and characterisation of curcumin-loaded pea protein-zein nanocomplexes using pH-driven method. Int. J. Food Sci. Technol. 57 (6), 3589–3603. doi:10.1111/ijfs.15683
Zheng, H., Wang, J., You, F., Zhou, M., and Shi, S. (2022). Fabrication, characterization, and antimicrobial activity of carvacrol-loaded zein nanoparticles using the pH-driven method. Int. J. Mol. Sci. 23 (16), 9227. doi:10.3390/ijms23169227
Zhong, Q., Jin, M., Davidson, P. M., and Zivanovic, S. (2009). Sustained release of lysozyme from zein microcapsules produced by a supercritical anti-solvent process. Food Chem. 115 (2), 697–700. doi:10.1016/j.foodchem.2008.12.063
Zhong, Q., and Jin, M. (2009a). Nanoscalar structures of spray-dried zein microcapsules and in vitro release kinetics of the encapsulated lysozyme as affected by formulations. J. Agric. food Chem. 57 (9), 3886–3894. doi:10.1021/jf803951a
Zhong, Q., and Jin, M. (2009b). Zein nanoparticles produced by liquid–liquid dispersion. Food Hydrocoll. 23 (8), 2380–2387. doi:10.1016/j.foodhyd.2009.06.015
Zhong, X., Wen, H., Zeng, R., Deng, H., Su, G., Zhou, H., et al. (2022). Zein-functionalized mesoporous silica as nanocarriers for nanopesticides with pH/enzyme dual responsive properties. Industrial Crops Prod. 188, 115716. doi:10.1016/j.indcrop.2022.115716
Keywords: zein, nanoparticle, drug delivery system, protein, preparation, characterization
Citation: Liu G, An D, Li J and Deng S (2023) Zein-based nanoparticles: Preparation, characterization, and pharmaceutical application. Front. Pharmacol. 14:1120251. doi: 10.3389/fphar.2023.1120251
Received: 09 December 2022; Accepted: 19 January 2023;
Published: 01 February 2023.
Edited by:
Donato Cosco, University of Catanzaro ‘‘Magna Graecia’’, ItalyReviewed by:
Alejandro Sanchez Chardi, Autonomous University of Barcelona, SpainChunhong Dong, Georgia State University, United States
Copyright © 2023 Liu, An, Li and Deng. This is an open-access article distributed under the terms of the Creative Commons Attribution License (CC BY). The use, distribution or reproduction in other forums is permitted, provided the original author(s) and the copyright owner(s) are credited and that the original publication in this journal is cited, in accordance with accepted academic practice. No use, distribution or reproduction is permitted which does not comply with these terms.
*Correspondence: Guijin Liu, bGl1Z2pAaGFpbmFudS5lZHUuY24=; Junjian Li, OTkzOTA5QGhhaW5hbnUuZWR1LmNu; Shiming Deng, ZHNtNzAxQGhhaW5hbnUuZWR1LmNu