- Faculty of Pharmacy, The University of Lahore, Lahore, Pakistan
Fenchone is a monoterpene present in the essential oils of various plants, including Foeniculum vulgare and Peumus boldus. Previous studies confirmed the anti-inflammatory, antioxidant, wound-healing, antidiarrheal, antifungal, antinociceptive, and bronchodilator activities of fenchone. Owing to various pharmacological activities of Fenchone, the current research was designed to evaluate its diuretic activity along with toxicity profiling. For evaluating acute toxicity, OECD guideline 425 was followed in which a single dose of 2000 mg/kg was orally administered to rats. For evaluating the diuretic potential in rats, three doses of Fenchone (100, 200, and 400 mg/kg) were assayed in comparison to furosemide (15 mg/kg) as the standard drug, followed by measurements of urinary volume, urinary electrolytes, uric acid, and urinary creatinine in saline-loaded rats for 8 h. The acute toxicity study showed a significant increase in hemoglobin (Hb), red blood cells (RBCs), alkaline phosphatase (ALP), and alkaline transaminase (ALT) along with a significant decrease in serum triglycerides, cholesterol, and uric acid levels when compared with the control group. The oxidative stress parameter, superoxide dismutase (SOD), was increased in the heart and spleen. Nitrite (NO) and glutathione were significantly increased in the kidney. The acute diuretic effect of Fenchone (400 mg/kg) significantly increased the urinary output, electrolytes (Na+, K+, and Ca++), urinary creatinine, and urinary uric acid in a dose-dependent manner. The Na+/K+ ratio was remarkably higher in the treatment group than that of the control group. The diuretic index, saluretic index, and Lipschitz value were also calculated from electrolyte concentration and urinary volume measurements, and the values were significantly increased in rats administered with fenchone at 400 mg/kg dose. The current study concluded that fenchone is safe and has remarkable diuretic action.
Introduction
In order to develop and test newer molecules before clinical trials, toxicity studies of compounds are fundamental (Anwar et al., 2021). A very vital consideration for the toxicity studies is ensuring the safety of the animals exposed to the study compounds (Morton, 1998). International governing organizations for toxicity determines dose-related perils on human subjects by evaluating the effect of study compounds on animals (Lewis et al., 2002). Toxicity profiling allows easy assessment of tissues along with the approximation of several biochemical and pathophysiological parameters (Dandekar et al., 2010; Arome and Chinedu, 2013). An association can be established between toxic and therapeutic doses by conducting toxicity studies of newer agents, and these extensive studies allow the researchers to determine whether to further proceed with clinical trials or not (Aneela et al., 2011). These experiments not only help in determining the safety of the newer agents in animal models but also foster the drug’s suitability for its therapeutic use (Jothy et al., 2011).
Natural therapies from medicinal plants are vital for developing new treatment protocols. Most of the current therapies have been isolated from natural plant extracts and semi-synthesized or chemically synthesized using natural constituents (Wright, 2019). Due to recent technological advances, natural therapies serve as a preliminary source of developing newer and innovative therapeutic medications, encompassing a vast range of conditions (Shen, 2015). Modern research on drug discovery from medicinal plants includes complex methods linking phytochemical, biological, botanical, and molecular activities (Balunas and Kinghorn, 2005). Phytochemicals, as a part of plant constituents, express distinct bio-activities with better acceptability, lesser side effects, better safety profile, and more cost-effective approaches. Also, they are broadly inspected for their capacity to offer better health outcomes (Krum and Pellizzer, 1998).
Diuretics are the mainstay for treating hypertension, ascites, pulmonary edema, and congestive heart failure and are either used in combination with other agents or alone (Lahlou, Tahraoui, Israili, and Lyoussi, 2007). The irrational use of diuretics poses certain restrictions regarding their side effects, including metabolic imbalances, electrolyte disturbances, activation of the renin–angiotensin aldosterone system (RAAS), and sexual dysfunction, and these side effects lead to the quest for novel diuretic agents from plant sources (Schlickmann et al., 2018).
Fenchone exists as a bicyclic monoterpene and is present in the essential oil of various fragrant plants, including Foeniculum vulgare and Peumus boldus (Kim, Song, Cho, and Lee, 2020). Previous pharmacological research has confirmed the anti-inflammatory, antioxidant, wound-healing, antidiarrheal, antifungal, antinociceptive, and bronchodilator activities of fenchone (Him, Ozbek, Turel, and Oner, 2008; Slavchev et al., 2014; Keskin et al., 2017; de Souza Pessoa et al., 2020; Rehman, Ansari, Samad, and Ahmad, 2022). The therapeutic efficacy of the fruits of Foeniculum vulgare is generally accredited to its essential oils. Various research studies uncovered that the essential oils as well as the individual elements demonstrate unique therapeutic potentials (Rather, Dar, Sofi, Bhat, and Qurishi, 2016). Therefore, the modern medicine era necessitates designing and developing newer diuretic agents that not only enhance the cardiovascular potentials by reducing mortality but also overcome the harmful effects of the drugs (Younis et al., 2021). Fenchone being present in many aromatic plants has shown to be a promising diuretic for treating hypertension (Agarwal, Gupta, Agrawal, Srivastava, and Saxena, 2008). Therefore, based on the reported results of the plant, fenchone was selected as the study compound and its diuretic potential and acute toxicity in rat models were determined.
Materials and methods
Chemicals and drugs
The chemicals employed for the research included pyrogallol solution from Oxford Labs (India); trichloroacetic acid, Griess reagent, and Ellman’s reagent (DNTB) from Omicron Sciences Limited (United Kingdom); and potassium phosphate buffer, carboxymethyl cellulose (CMC), thiobarbituric acid, furosemide, and fenchone (CAS No: 7,787-20-4) purchased from Sigma-Aldrich (United States).
Animals
Adult Wistar albino rats (weighing 150–250 gm) of age 4–8 weeks were housed in the animal house of The University of Lahore, Pakistan. All the animals were placed under normal conditions, provided with chow and water, and kept in a well-maintained atmosphere in a 12 h light/dark cycle at proper temperature (25°C ± 1°C). All animals were provided with housing environments in accordance with accepted principles for laboratory animal use and care (NIH publication number # 85-23, revised in 1985). All experimental procedures were approved (IREC-2022-17) by the Institutional Ethical Committee of the Faculty of Pharmacy, The University of Lahore.
Acute oral toxicity
The OECD 425 guidelines were followed for assessing acute oral toxicity. Healthy female albino rats were fasted overnight but had free access to water (OECD, 2001). Initially, fenchone was administered at 2000 mg/kg dose orally for the limit test to a single rat according to body weight, and the animal was closely observed for 30 min and then for next 4 h and 24 h. If there were no mortality rates, the same dose was administered to four other rats, and the rats were monitored for any alteration in general behavior, allergic reactions, body weight, and mortality for 14 days. The same protocol was followed for the normal control, which received only .5% carboxymethylcellulose (CMC) as a vehicle according to their body weight (Anwar et al., 2021).
Estimation of hematological and biochemical parameters
The rats were anesthetized by administering 3%–4% isoflurane by diluting it with oxygen, and blood was withdrawn by cardiac puncture after 14 days. The hematological parameters encompassed hemoglobin (Hb), red blood cells (RBCs), packed cell volume (PCV), mean corpuscular volume (MCV), mean corpuscular hemoglobin (MCH), mean corpuscular hemoglobin concentration (MCHC), and white blood cells (WBCs), while blood was isolated in tubes having EDTA sodium, followed by centrifugation at 1,400 g for 10–15 min for the isolation of serum for measuring various biochemical parameters like AST, ALT, ALP, bilirubin, creatinine, uric acid, triglycerides, and cholesterol. All these parameters were assessed by utilizing their specific kits (Konan, Bacchi, Lincopan, Varela, and Varanda, 2007).
Estimation of oxidative stress parameters
Lastly, rats were killed by cervical dislocation, and vital organs like the heart, liver, kidney, and spleen were excised and weighed separately. The tissue homogenate was prepared by mixing 1 gm of tissue with 10 mL of phosphate buffer (.1 M, pH 7.4) followed by centrifugation. The supernatant was isolated for the measurement of oxidative stress parameters like GSH, SOD, NO, and MDA (Saleem et al., 2017; Anwar et al., 2021). All the tests were executed in triplicate.
Superoxide dismutase (SOD) estimation
A volume of 2.8 mL of potassium phosphate buffer (.1 M, pH 7.4) was mixed with .1 mL of tissue homogenate, followed by .1 mL pyrogallol solution. Mixture absorbance was measured at 325 nm, and the following SOD standard cure regression line was drawn for estimating SOD values (Nazir et al., 2021):
Nitrite (NO) level estimation
The supernatant of tissue homogenate and Griess reagent were taken in equal proportion and mixed thoroughly, and this mixture was allowed to incubate for 10 min, followed by the measurement of absorbance at 546 nm. A sodium nitrite regression line was used for estimating nitrite levels (Nazir et al., 2021) as follows:
Glutathione (GSH) estimation
Tissue homogenate (1 mL) was precipitated with 10% trichloroacetic acid (1 mL) along with phosphate buffer (4 mL). The aliquot supernatant was separated after precipitation followed by the addition of .5 mL of 5, 5 dithiobis 2 nitrobenzoic acid (DNTB). Mixture absorbance was measured at 412 nm, and the following formula was utilized for estimating the amount of GSH in the tissue homogenate (Saeed, Saleem, Anwar, Ahmad, and Anwar, 2020):
where Y is the absorbance at 412 nm, DF is the dilution factor, BT is the tissue homogenate, and VU is the volume of aliquot.
Malondialdehyde (MDA) estimation
Tissue homogenate (1 mL) was added to 3 mL of thiobarbituric acid (TBA) reagent. The resulting mixture was stirred and allowed to incubate for 15 min at room temperature, followed by cooling on an ice bath and centrifugation. The layer of supernatant was separated for measuring the absorbance at 532 nm. The following formula was utilized for estimating the concentration of MDA in tissue homogenate (Nazir et al., 2021):
where Vt is the total volume mixture, 1.56×105 is the extinction coefficient, Wt is the weight of the dissected tissue, and Vu is the volume of aliquot.
Histopathological evaluation
The excised organs including the heart, liver, kidney, and spleen were placed in paraformaldehyde (4%), fixed in paraffin, and cut into 5-µm sections, followed by staining with eosin and hematoxylin and observed using the microscope for any pathological changes (Anwar et al., 2021).
Assessment of acute diuretic activity of fenchone
Acute diuretic activity
The rats were placed in metabolic cages 7 days prior to the procedure to acclimatize them to the experimental conditions. The rats, weighing 150–250 gm, were divided into five groups, with five rats in each group. Initially, all the rats received 5 mL/100 gm (p.o) of .9% normal saline to ensure a uniform water and salt load (Younis et al., 2021). After 45 min, Group I, which was the normal control group, received only .5% CMC (1 mL/kg), while Group II was the reference standard and received 15 mg/kg furosemide by the oral route, and the test groups (III, IV, and V) were given 100, 200, and 400 mg/kg of fenchone, respectively, through oral gavage.
Animals were kept in metabolic cages, and urine sampling was carried out after 2, 4, and 6 h of treatment for analysis of Na+, K+, Ca++, urinary creatinine, pH, and uric acid.
Electrolyte estimation
pH was measured using a pH meter, and Na+, K+, Ca++, uric acid, and urinary creatinine were measured (Prathibhakumari and Prasad, 2014) using Bio-active Diagnostic System kits.
Diuretic index, saluretic index, and Lipschitz value
Based on the urine volume and electrolyte concentrations, the diuretic index, saluretic index, and Lipschitz value were also calculated.
Diuretic index = urinary volume of the treatment group/urinary volume of the normal control group,
Saluretic index Na+(SINa+) = urinary Na+ concentration of the treatment group/urinary Na+ concentration of the normal control group,
Saluretic index K+(SIK+) = urinary K+ concentration of the treatment group/urinary K+ concentration of the normal control group,
Saluretic index (SI) = SINa+ + SIK+ of the treatment group/SINa+ + SIK+ of the normal control group,
Lipschitz value = urinary volume of the treatment group/urinary volume of the standard group.
Statistical analysis
The results of the experiments were evaluated using GraphPad Prism version 5, and the data were presented as mean ± SEM. Both one-way ANOVA and two-way ANOVA were used for analyzing the data statistically, followed by the Tukey comparison test and Bonferroni and Dunnett’s post hoc tests. p< .05 was considered significant. p < .01 and p < .001 indicated moderate and highly significant levels, respectively.
Results
Effect of treatment on the behavioral pattern in the acute toxicity study
Table 1 shows that no mortality or morbidity occurred during the acute toxicity study with fenchone (F) treatment (2000 mg/kg). No significant difference was noted in behavioral parameters when compared to the normal control group.
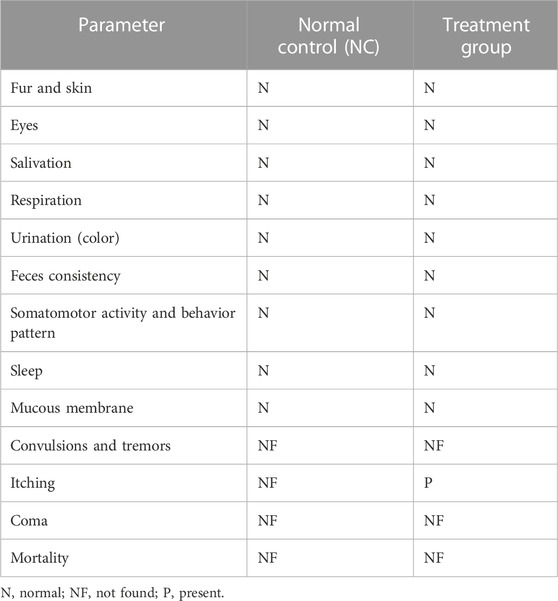
TABLE 1. Behavioral changes after treatment with a single dose of fenchone (F) (2000 mg/kg) in the acute oral toxicity study when compared to those of the normal control.
Effects of treatment on body weight and organ weight
The body weight of the treated animals was noted from the first day to the 14th day. The treatment group showed a parallel increase in body weight similar to the normal control group in the early days, but a significant decrease was observed on the 14th day in comparison with the first day as shown in Tables 2, 3. A non-significant difference was observed in all organ weights when compared with the control group.
Treatment effect on complete blood count in the acute toxicity study
On the 14th day, blood was withdrawn by cardiac puncture from the treated animals. A significant rise in the RBC count and Hb level was observed in animals treated with fenchone (2000 mg/kg) when compared with those of the control group as shown in Table 4. However, other parameters are parallel to the normal control group.
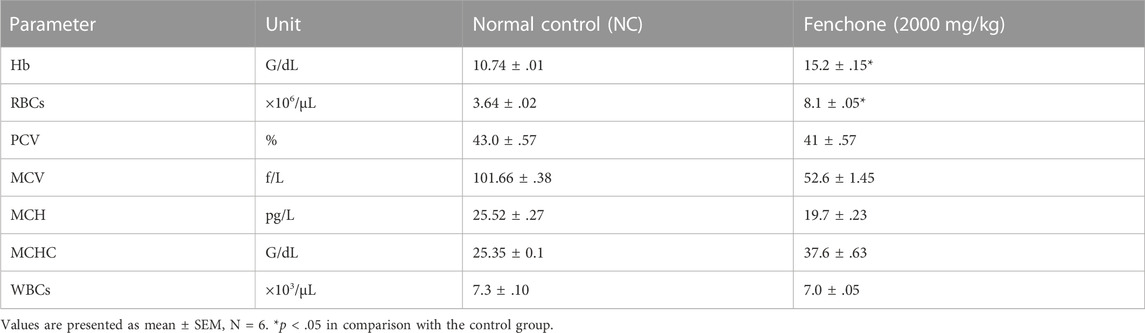
TABLE 4. Analysis of complete blood count in animals treated with fenchone (2000 mg/kg) in the acute toxicity study.
Analysis of biochemical markers in treatment groups in the acute toxicity study
Table 5 illustrates that animals treated with fenchone (2,000 mg/kg) showed a significant decrease in the triglyceride and cholesterol levels in comparison with those of the control group. However, hepatic functioning markers were significantly higher than those of the control group. Surprisingly, fenchone significantly decreased the uric acid levels in the treated animals when compared with the control.
Oxidative stress marker analysis in the acute toxicity study
Figure 1 shows the results of oxidative stress biomarker analysis in the chosen organs of the treated animals. The results showed that in the kidneys of the Fenchone-treated animals, there was a significant decrease in SOD, NO, and GSH when compared to those of the normal control group. A highly significant increase in SOD levels was observed in the heart. Lipid peroxidation did not show any significant difference in all chosen organs when compared to that of the normal control group.
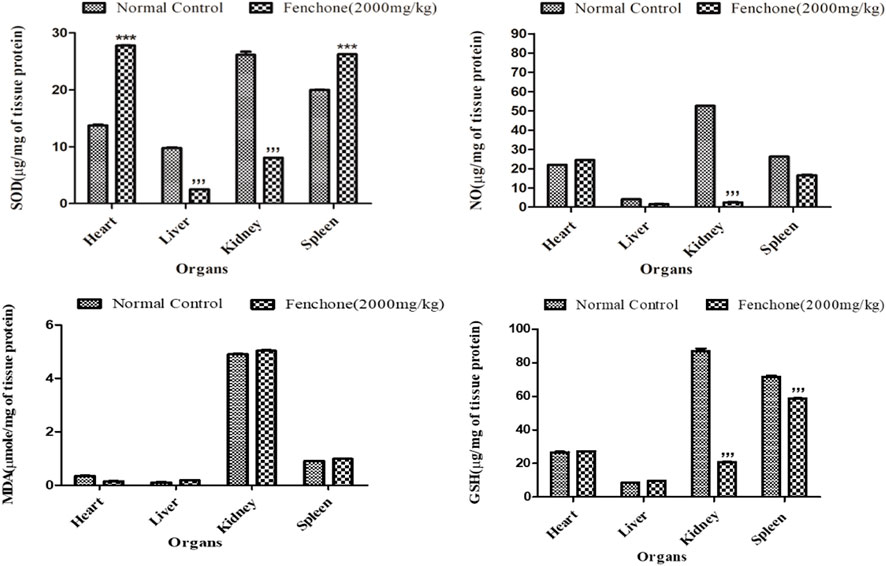
FIGURE 1. Oxidative stress marker analysis in treated animals in the acute toxicity study. Data are stated as mean ± SEM, N = 3. ***p < .001 represents a significant increase, while ’’’p < .001 represents a significant decrease vs. normal control.
Histopathological analysis
Histopathological analysis showed no remarkable change in the architecture of cells (Figure 2).
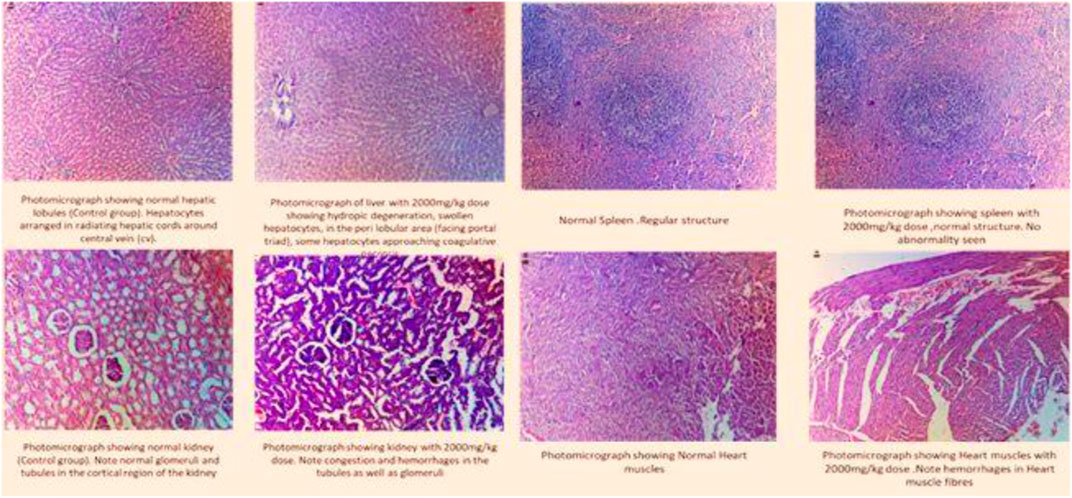
FIGURE 2. Histogram of the selected organs treated with fenchone (2000 mg/kg) in the acute toxicity study.
Effect of different doses of fenchone on urinary electrolytes (Na+ and K+) and Na+/K+ ratio in acute diuretic activity
The effects of different doses of fenchone (100, 200, and 400 mg/kg) and furosemide (15 mg/kg) on sodium and potassium are shown in Figure 3. Fenchone (200 mg/kg and 400 mg/kg) resulted in a significant increase in sodium and potassium excretion. Na+ and K+ excretion rates induced by fenchone (400 mg/kg) were 184.06 ± 1.21 and 46.21 ± .51, whereas in the normal control group the rates were 136.93 ± .95 and 25.53 ± 1.31 mmol/L, respectively. Moreover, fenchone (400 mg/kg) resulted in more electrolyte excretion of sodium and potassium than furosemide. Moreover, there was a significant increase in the Na+/K+ ratio at a dose of 400 mg/kg fenchone when compared with that of the control group.
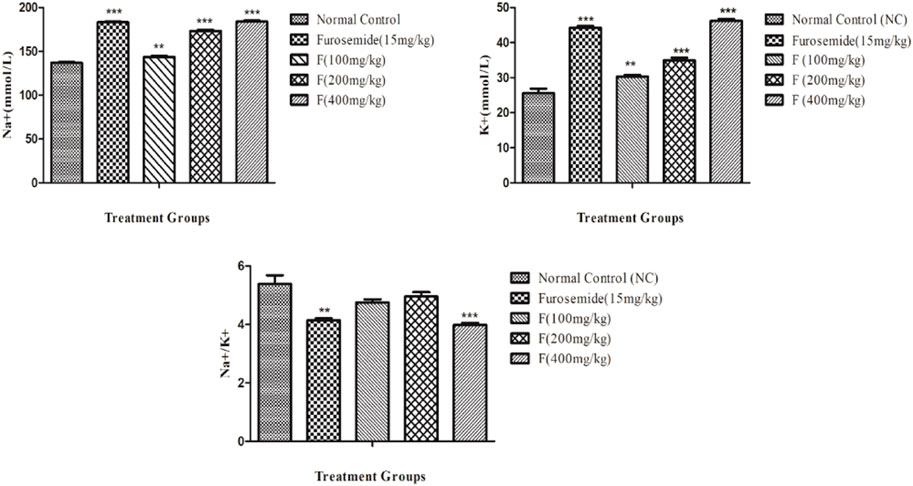
FIGURE 3. Effect of different doses of fenchone on urinary electrolytes (Na+ and K+) where furosemide was taken as the standard drug. Data are stated as mean ± SEM, N = 6. ***p < .001 represents a significant increase, while ’’’p < .001 represents a significant decrease vs. normal control.
Effect of different doses of fenchone on urinary creatinine and uric acid
The effects of different doses of fenchone (100, 200, and 400 mg/kg) and furosemide (15 mg/kg) on urinary creatinine and uric acid are shown in Figure 4. There was a significant increase in the excretion of creatinine in the urine, and it was the highest with fenchone (400 mg/kg) when compared to the control. Moreover, the concentration of uric acid in the urine also increased significantly with a higher dose of fenchone (400 mg/kg), which was 9.14 ± .10 when compared to that of the normal control group, which was 4.49 ± .02. Both urinary creatinine and uric acid excretion results were comparable to furosemide.
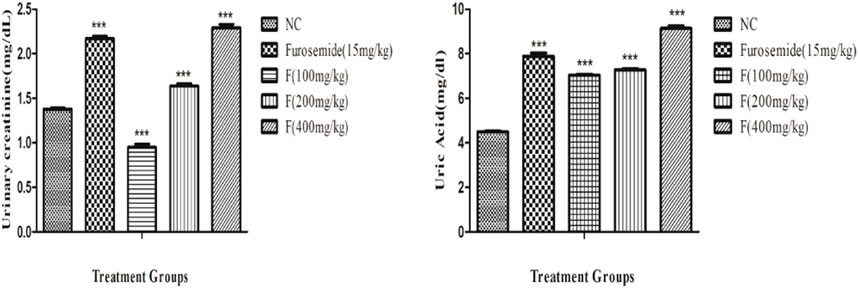
FIGURE 4. Effect of different doses of fenchone on urinary creatinine and uric acid excretion where furosemide was taken as the standard drug. Data are stated as mean ± SEM, N = 6. ***p < .001 represents a significant increase, while ’’’p < .001 represents a significant decrease vs. normal control.
Effect of different doses of fenchone on urinary calcium
There was a significant increase in the urinary calcium excretion, and it was the highest with fenchone (400 mg/kg) when compared to the control. The urinary excretion of Ca++ with fenchone at 400 mg/kg was 22.08 ± .28 when compared to that of the normal control group which was 10.11 ± .33 as shown in Figure 5.
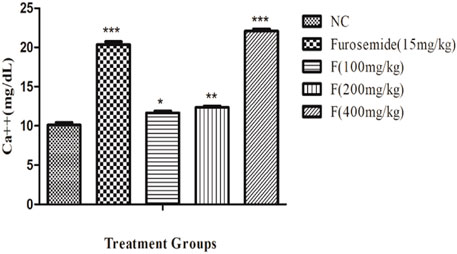
FIGURE 5. Effect of different doses of fenchone on urinary calcium where furosemide was taken as the standard drug. Data are stated as mean ± SEM, N = 6. ***p < .001 represents a significant increase, while ’’’p < .001 represents a significant decrease vs. normal control.
Effect of different doses of fenchone on urinary pH and urinary volume
The acute diuretic effect of different doses of fenchone (100, 200, and 400 mg/kg) is shown in Table 6. The fenchone (400 mg/kg) dose resulted in increased urinary output at a 6-h interval following treatment. There was a dose-dependent increase in urinary volume measured at 6 h in 400 mg/kg fenchone-treated rats which was 5.22 ± .20 mL/100 gm, while the urinary volume was 2.23 ± .14 in the control. Moreover, the urinary output at 6 h in rats treated with fenchone (400 mg/kg) was comparable to the output in rats treated with furosemide, a standard diuretic drug. Urinary output data also suggested that the increase in urine load was due to the increased polarity resulting from this activity. Finally, the urine pH values showed a significant increase with fenchone (200 and 400 mg/kg) treatment when compared with those of the normal control group.
Effect of fenchone on the diuretic index, Lipschitz value, saluretic index of Na+ and K+, and saluretic index
By utilizing the excreted concentration of electrolytes (Na+ and K+) and urinary output in the control, standard, and treatment groups, the diuretic index, Lipschitz value, and saluretic index were calculated as shown in Table 7, and the results were quite significant at a dose of 400 mg/kg F.
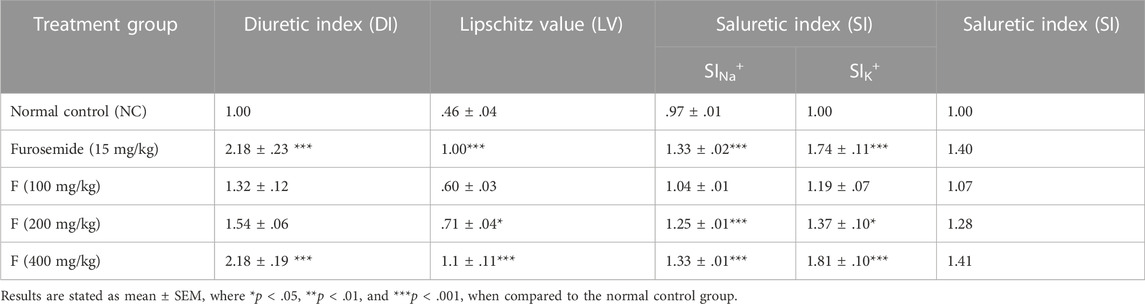
TABLE 7. Effect of fenchone on the diuretic index, Lipschitz value, saluretic index of Na+ and K+, and saluretic index.
Discussion
Toxicity profiling of newer molecules has gained attention and interest since it helps provide future directions to researchers, manufacturers, and distributors. The rules and regulations for toxicity studies of newer compounds help in regulating and ensuring their safety for further use in human beings. The procedure involves administration of the test compound followed by examination and investigation of biochemical parameters in animal models (Rand and Petrocelli, 1985), since the main purpose of the toxicity study is to administer the single highest dose that could result in inducing toxic effects (Klaassen, 2013).
The acute toxicity study involved the oral administration of a single dose of fenchone (2000 mg/kg) to female rats since female rats are more responsive to any biochemical change than male rats (OECD, 2001). Until the 14th day, no mortality or fatal toxicity was noted, indicating that the LD50 of F is significantly greater than 2000 mg/kg. Generally, it has been observed that variations in the behavior pattern and body weight are the primary indicators of toxicity (Variya, Bakrania, Madan, and Patel, 2019), and the results indicated no change in the behavioral pattern of the animals. However, there was a significant decrease in the body weight on day 14 when compared to that of the normal control group, whereas a non-significant difference was observed in the organ weights. Fenchone, an important constituent of fennel seeds, causes weight loss by boosting metabolism (Yamini, Sefidkon, and Pourmortazavi, 2002), so the decrease in weight loss due to fenchone treatment might be attributed to this.
Both biochemical evaluation and hematological evaluation provide a genuine overview regarding toxicity along with organ dysfunction. Pluripotent stem cells give rise to white blood cells (WBCs), red blood cells (RBCs), and platelets after maturation and differentiation (Ugwah-Oguejiofor et al., 2019). The Hb level and RBC count were observed to be increased in the acute toxicity study in contrast to the control group owing to the antioxidant properties that helped in stabilizing the RBC membrane and raising the level of RBCs by influencing the kidneys and liver, thereby increasing the level of erythropoietin (Mansouri et al., 2015). All the remaining parameters were normal when compared to those of the control group.
Dyslipidemia is linked with obesity since it may cause insulin resistance in the periphery by increasing the hepatic output of fats from the diet and raising triglyceride hydrolysis (Klop, Elte, and Castro Cabezas, 2013). Triglyceride and cholesterol levels were significantly reduced in the acute toxicity study that might be attributed to altered expression of the leptin receptor (Zakernezhad et al., 2021).
Estimating biochemical parameters in the liver and kidney is of primary importance since both these organs play a vital role in drug metabolism and excretion, respectively (Bariweni, Yibala, and Ozolua, 2018), and any variation in either liver or kidney function tests shows toxicity (Abdelkader et al., 2020). Liver enzymes including ALT and AST were increased at a dose of 2000 mg/kg F that might have resulted from cell damage to the liver since ALT and AST are the enzymes present in the cytosol and mitochondria of hepatocytes (Samadi-Noshahr, Hadjzadeh, Moradi-Marjaneh, and Khajavi-Rad, 2021), whereas there was a significant decrease in the uric acid levels.
Uninterrupted exposure or formation of free oxidative radicals in living organisms can lead to disturbances in redox homeostasis, resulting in damage to cellular nucleic acids, proteins, and lipids (Jones, 2006; Zhang et al., 2020). If the interrupting stimulus is not removed, irreversible cell damage will ensue, resulting in responses of numerous defensive cascades including gene regulatory cycle, repair, and various antioxidant routes to protect against oxidative stress (Nair et al., 2015). Endogenous oxidants and antioxidants were measured in the specific organs of rats to assess any change or cellular stress induced by F dosing. The results showed that there was a significant increase in SOD levels in the heart and spleen that might be attributed to the immunomodulatory effect of the drug (Anwar et al., 2021), whereas a significant decrease was observed in the liver and kidney. Similarly, there was a significant decrease in NO and GSH levels in the kidney showing that it might have been excreted from the kidney, indicating a decline in oxidative stress markers, and these results can be correlated to the histopathological slides.
It has been established that diuretics are the mainstay for treating hypertension and edematous conditions, which are expressed as excessive extracellular fluid. In general, diuretics are known to increase the excessive salt and water excretion from the body, thus reducing blood flow resistance by decreasing blood pressure and volume (Younis et al., 2021). Even though diuretics are available abundantly for human use, the side effects pose a need for newer agents from plants or their constituents (Wright, Van-Buren, Kroner, and Koning, 2007), displaying numerous pharmacological effects on renal physiology and acting on distinct and well-established targets like nitric oxide-cGMP, carbonic anhydrase, renin–angiotensin system, and renal carriers (Aparecida Livero, Vergutz Menetrier, Luiz Botelho Lourenco, and Gasparotto Junior, 2017). In the present research, fenchone resulted in a significant dose-dependent increase in diuretic activity, with a maximum response at 400 mg/kg dose. A single dose of fenchone significantly increased the urinary output from the second hour and increased with time, and the effect was the highest with F 400 mg/kg (5.22 ± .20) after 8 h. This effect was significantly higher than that of the standard drug furosemide (15 mg/kg) which was 4.83 ± .29, but the onset time was less with fenchone. The time difference in the onset of diuretic action can be related to the absorption profiles of fenchone. The increase in the urinary output of F can be attributed to the increase in compound polarity that might have increased the renal circulation along with glomerular filtration and thus increased the formation of urine (Chen et al., 2014).
The current study also revealed that F 400 mg/kg dose also resulted in inducing a significant increase in urinary electrolytes Na+ (184.06 ± 1.21) and K+ (46.21 ± .51) when compared to those of furosemide which were 183.06 ± 1.24 for Na+ and 44.23 ± .49 for K+, showing both saluretic and kaliuretic activities that might be due to their interaction with polar groups (Martín-Herrera, Abdala, Benjumea, and Pérez-Paz, 2007). Similarly, there was an increase in the Na+/K+ ratio at F 400 mg/kg dose that might be attributed to an increase in sodium loss along with potassium retention (Feng et al., 2013), which is an essential feature of a good diuretic that produces reduced hyperkalemic effects (Ghelani, Chapala, and Jadav, 2016).
Creatinine is a reliable marker for assessing kidney function (Prathibhakumari and Prasad, 2014). Urinary creatinine was significantly increased at both 200 mg/kg and 400 mg/kg doses, but a higher effect was observed with fenchone (400 mg/kg) in a dose-dependent manner, so its use can be considered safe in patients with renal insufficiencies.
Uric acid is the end-product of nucleic acid metabolism in the human body, and this homeostasis is attained by either modulating the formation, breakdown, or excretion of uric acid. Hyperuricemia is generally characterized by an elevation in the levels of uric acid and is considered a risk factor for causing cardiovascular disease, diabetes, hypertension, chronic kidney disease, and gout (Zhang et al., 2018). The current research revealed that the F (200 and 400 mg/kg) dose resulted in a significant increase in the excretion of uric acid in the urine with much higher results at 400 mg/kg dose, and thus it can be used in treating gout.
Conclusion
The current study showed that the LD50 of fenchone is greater than 2,000 mg/kg, no mortality or signs of toxicity were reported, and it has a remarkable diuretic potential comparable to furosemide. Moreover, oral treatment with F resulted in increased excretion of sodium and potassium in the urine, along with increases in the Na+/K+ ratio, diuretic index, Lipschitz value, and saluretic index. The results suggest that fenchone (F) may be considered a safe therapeutic agent in patients with cardiovascular and renal insufficiencies, but further mechanistic studies would be essential before introducing it into clinical trials.
Data availability statement
The raw data supporting the conclusion of this article will be made available by the authors, without undue reservation.
Ethics statement
The animal study was reviewed and approved by the Institutional Ethical Committee of the Faculty of Pharmacy, The University of Lahore, with Approval No. IREC-2022-17.
Author contributions
AB carried out the research and drafted the manuscript, MM and WY designed the project, and IA carried out the statistical analysis.
Acknowledgments
The authors thank The University of Lahore for providing research facilities to carry out the research.
Conflict of Interest
The authors declare that the research was conducted in the absence of any commercial or financial relationships that could be construed as a potential conflict of interest.
Publisher’s note
All claims expressed in this article are solely those of the authors and do not necessarily represent those of their affiliated organizations, or those of the publisher, the editors, and the reviewers. Any product that may be evaluated in this article, or claim that may be made by its manufacturer, is not guaranteed or endorsed by the publisher.
References
Arome, D., and Chinedu, E. (2013). The importance of toxicity testing. J. Pharm. Biosci. 4, 146–148.
Abdelkader, N. F., Elyamany, M., Gad, A. M., Assaf, N., Fawzy, H. M., and Elesawy, W. H. (2020). Ellagic acid attenuates liver toxicity induced by valproic acid in rats. J. Pharmacol. Sci. 143 (1), 23–29. doi:10.1016/j.jphs.2020.01.007
Agarwal, R., Gupta, S. K., Agrawal, S. S., Srivastava, S., and Saxena, R. (2008). Oculohypotensive effects of Foeniculum vulgare in experimental models of glaucoma. Indian J. Physiol. Pharmacol. 52 (1), 77–83.
Aneela, S., De, S., Kanthal, L., Choudhury, N., Das, B., and Sagar, K. (2011). Acute oral toxicity studies of Pongamia Pinnata and Annona squamosa on albino wister rats. Int. J. Res. Pharm. Chem. 1 (4), 820–824.
Anwar, F., Saleem, U., Rehman, A.-U., Ahmad, B., Froeyen, M., Mirza, M. U., et al. (2021). Toxicity evaluation of the naphthalen-2-yl 3, 5-dinitrobenzoate: A drug candidate for alzheimer disease. Front. Pharmacol. 12, 607026. doi:10.3389/fphar.2021.607026
Aparecida Livero, F., Vergutz Menetrier, J., Luiz Botelho Lourenco, E., and Gasparotto Junior, A. (2017). Cellular and molecular mechanisms of diuretic plants: An overview. Curr. Pharm. Des. 23 (8), 1247–1252. doi:10.2174/1381612822666161014114437
Balunas, M. J., and Kinghorn, A. D. (2005). Drug discovery from medicinal plants. Life Sci. 78 (5), 431–441. doi:10.1016/j.lfs.2005.09.012
Bariweni, M. W., Yibala, O. I., and Ozolua, R. I. (2018). Toxicological studies on the aqueous leaf extract of Pavetta crassipes (K. Schum) in rodents. J. Pharm. Pharmacogn. Res. 6 (1), 1–16.
Chen, D.-Q., Feng, Y.-L., Tian, T., Chen, H., Yin, L., Zhao, Y.-Y., et al. (2014). Diuretic and anti-diuretic activities of fractions of Alismatis rhizoma. J. Ethnopharmacol. 157, 114–118. doi:10.1016/j.jep.2014.09.022
Dandekar, P., Dhumal, R., Jain, R., Tiwari, D., Vanage, G., and Patravale, V. (2010). Toxicological evaluation of pH-sensitive nanoparticles of curcumin: Acute, sub-acute and genotoxicity studies. Food Chem. Toxicol. 48 (8-9), 2073–2089. doi:10.1016/j.fct.2010.05.008
de Souza Pessoa, M. L., Silva, L. M. O., Araruna, M. E. C., de Lima Serafim, C. A., Júnior, E. B. A., Silva, A. O., et al. (2020). Antifungal activity and antidiarrheal activity via antimotility mechanisms of (-)-fenchone in experimental models. World J. Gastroenterology 26 (43), 6795–6809. doi:10.3748/wjg.v26.i43.6795
Feng, Y.-L., Lei, P., Tian, T., Yin, L., Chen, D.-Q., Chen, H., et al. (2013). Diuretic activity of some fractions of the epidermis of Poria cocos. J. Ethnopharmacol. 150 (3), 1114–1118. doi:10.1016/j.jep.2013.10.043
Ghelani, H., Chapala, M., and Jadav, P. (2016). Diuretic and antiurolithiatic activities of an ethanolic extract of Acorus calamus L. rhizome in experimental animal models. J. traditional complementary Med. 6 (4), 431–436. doi:10.1016/j.jtcme.2015.12.004
Him, A., Ozbek, H., Turel, I., and Oner, A. C. (2008). Antinociceptive activity of alpha-pinene and fenchone. Pharmacologyonline 3, 363–369.
Jones, D. P. (2006). Redefining oxidative stress. Antioxidants redox Signal. 8 (9-10), 1865–1879. doi:10.1089/ars.2006.8.1865
Jothy, S. L., Zakaria, Z., Chen, Y., Lau, Y. L., Latha, L. Y., and Sasidharan, S. (2011). Acute oral toxicity of methanolic seed extract of Cassia fistula in mice. Molecules 16 (6), 5268–5282. doi:10.3390/molecules16065268
Keskin, I., Gunal, Y., Ayla, S., Kolbasi, B., Sakul, A., Kilic, U., et al. (2017). Effects of Foeniculum vulgare essential oil compounds, fenchone and limonene, on experimental wound healing. Biotech. Histochem. 92 (4), 274–282. doi:10.1080/10520295.2017.1306882
Kim, T., Song, B., Cho, K. S., and Lee, I.-S. (2020). Therapeutic potential of volatile terpenes and terpenoids from forests for inflammatory diseases. Int. J. Mol. Sci. 21 (6), 2187. doi:10.3390/ijms21062187
Klaassen, C. D. (2013). Casarett and doull's toxicology: The basic science of poisons, 1236. New York, NY, USA: McGraw-Hill.
Klop, B., Elte, J. W. F., and Castro Cabezas, M. (2013). Dyslipidemia in obesity: Mechanisms and potential targets. Nutrients 5 (4), 1218–1240. doi:10.3390/nu5041218
Konan, N. A., Bacchi, E. M., Lincopan, N., Varela, S. D., and Varanda, E. A. (2007). Acute, subacute toxicity and genotoxic effect of a hydroethanolic extract of the cashew (Anacardium occidentale L.). J. Ethnopharmacol. 110 (1), 30–38. doi:10.1016/j.jep.2006.08.033
Krum, H., and Pellizzer, A.-M. (1998). New and emerging drug treatments for hypertension. Aust. Fam. physician 27 (4), 235–240.
Lahlou, S., Tahraoui, A., Israili, Z., and Lyoussi, B. (2007). Diuretic activity of the aqueous extracts of Carum carvi and Tanacetum vulgare in normal rats. J. Ethnopharmacol. 110 (3), 458–463. doi:10.1016/j.jep.2006.10.005
Lewis, R. W., Billington, R., Debryune, E., Gamer, A., Lang, B., and Carpanini, F. (2002). Recognition of adverse and nonadverse effects in toxicity studies. Toxicol. Pathol. 30 (1), 66–74. doi:10.1080/01926230252824725
Mansouri, E., Kooti, W., Bazvand, M., Boroon, M. G., Amirzargar, A., Afrisham, R., et al. (2015). The effect of hydro-alcoholic extract of Foeniculum vulgare Mill on leukocytes and hematological tests in male rats. Jundishapur J. Nat. Pharm. Prod. 10 (1), e18396. doi:10.17795/jjnpp-18396
Martín-Herrera, D., Abdala, S., Benjumea, D., and Pérez-Paz, P. (2007). Diuretic activity of Withania aristata: An endemic Canary Island species. J. Ethnopharmacol. 113 (3), 487–491. doi:10.1016/j.jep.2007.07.005
Morton, D. M. (1998). Importance of species selection in drug toxicity testing. Toxicol. Lett. 102, 545–550. doi:10.1016/s0378-4274(98)00263-x
Nair, A. R., Lee, W.-K., Smeets, K., Swennen, Q., Sanchez, A., Thévenod, F., et al. (2015). Glutathione and mitochondria determine acute defense responses and adaptive processes in cadmium-induced oxidative stress and toxicity of the kidney. Archives Toxicol. 89 (12), 2273–2289. doi:10.1007/s00204-014-1401-9
Nazir, S., Anwar, F., Saleem, U., Ahmad, B., Raza, Z., Sanawar, M., et al. (2021). Drotaverine inhibitor of PDE4: Reverses the streptozotocin induced alzheimer’s disease in mice. Neurochem. Res. 46 (7), 1814–1829. doi:10.1007/s11064-021-03327-9
Oecd, (2001). OECD guideline 425: Acute oral toxicity—up-and-down procedure. France: OECD Guidelines for the Testing of Chemicals: Organization for Economic Cooperation and Development Paris.
Prathibhakumari, P., and Prasad, G. (2014). Pharmacological investigation on the diuretic activity of the aqueous fruit extract of Neolamarckia cadamba (Roxb) Bosser. J. Pharm. Res. 8 (2), 130–135.
Rand, G. M., and Petrocelli, S. R. (1985). Fundamentals of aquatic toxicology: Methods and applications. Washington, D.C, USA: U.S. Department of Energy.
Rather, M. A., Dar, B. A., Sofi, S. N., Bhat, B. A., and Qurishi, M. A. (2016). Foeniculum vulgare: A comprehensive review of its traditional use, phytochemistry, pharmacology, and safety. Arabian J. Chem. 9, S1574–S1583. doi:10.1016/j.arabjc.2012.04.011
Rehman, N. U., Ansari, M. N., Samad, A., and Ahmad, W. (2022). In silico, ex vivo and in vivo studies of roflumilast as a potential antidiarrheal and antispasmodic agent: Inhibition of the PDE-4 enzyme and voltage-gated Ca++ ion channels. Molecules 27 (4), 1008. doi:10.3390/molecules25041008
Saeed, M., Saleem, U., Anwar, F., Ahmad, B., and Anwar, A. (2020). Inhibition of valproic acid-induced prenatal developmental abnormalities with antioxidants in rats. ACS omega 5 (10), 4953–4961. doi:10.1021/acsomega.9b03792
Saleem, U., Amin, S., Ahmad, B., Azeem, H., Anwar, F., and Mary, S. (2017). Acute oral toxicity evaluation of aqueous ethanolic extract of Saccharum munja Roxb. roots in albino mice as per OECD 425 TG. Toxicol. Rep. 4, 580–585. doi:10.1016/j.toxrep.2017.10.005
Samadi Noshahr, Z., Hadjzadeh, M. A. R., Moradi-Marjaneh, R., and Khajavi Rad, A. (2021). The hepatoprotective effects of fennel seeds extract and trans-Anethole in streptozotocin induced liver injury in rats. Food Sci. Nutr. 9 (2), 1121–1131. doi:10.1002/fsn3.2090
Schlickmann, F., Boeing, T., Mariano, L. N. B., da Silva, R. d. C. M. V. d., Fonseca, A., da Silva, L. M., et al. (2018). Gallic acid, a phenolic compound isolated from Mimosa bimucronata (DC.) Kuntze leaves, induces diuresis and saluresis in rats. Naunyn-Schmiedeberg's Archives Pharmacol. 391 (6), 649–655. doi:10.1007/s00210-018-1502-8
Shen, B. (2015). A new golden age of natural products drug discovery. Cell 163 (6), 1297–1300. doi:10.1016/j.cell.2015.11.031
Slavchev, I., Dobrikov, G. M., Valcheva, V., Ugrinova, I., Pasheva, E., and Dimitrov, V. (2014). Antimycobacterial activity generated by the amide coupling of (−)-fenchone derived aminoalcohol with cinnamic acids and analogues. Bioorg. Med. Chem. Lett. 24 (21), 5030–5033. doi:10.1016/j.bmcl.2014.09.021
Ugwah-Oguejiofor, C. J., Okoli, C. O., Ugwah, M. O., Umaru, M. L., Ogbulie, C. S., Mshelia, H. E., et al. (2019). Acute and sub-acute toxicity of aqueous extract of aerial parts of Caralluma dalzielii NE Brown in mice and rats. Heliyon 5 (1), e01179. doi:10.1016/j.heliyon.2019.e01179
Variya, B. C., Bakrania, A. K., Madan, P., and Patel, S. S. (2019). Acute and 28-days repeated dose sub-acute toxicity study of gallic acid in albino mice. Regul. Toxicol. Pharmacol. 101, 71–78. doi:10.1016/j.yrtph.2018.11.010
Wright, C., Van-Buren, L., Kroner, C., and Koning, M. (2007). Herbal medicines as diuretics: A review of the scientific evidence. J. Ethnopharmacol. 114 (1), 1–31. doi:10.1016/j.jep.2007.07.023
Wright, G. D. (2019). Unlocking the potential of natural products in drug discovery. Microb. Biotechnol. 12 (1), 55–57. doi:10.1111/1751-7915.13351
Yamini, Y., Sefidkon, F., and Pourmortazavi, S. (2002). Comparison of essential oil composition of Iranian fennel (Foeniculum vulgare) obtained by supercritical carbon dioxide extraction and hydrodistillation methods. Flavour Fragr. J. 17 (5), 345–348. doi:10.1002/ffj.1117
Younis, W., Schini-Kerth, V., Nocchi, S. R., Silva, D. B., de Souza, P., Bukhari, I. A., et al. (2021). Involvement of muscarinic receptors in hypotensive and diuretic effects of aqueous soluble fraction from Asphodelus tenuifolius cav. Evidence-Based Complementary Altern. Med. 2021, 1–15. doi:10.1155/2021/6653270
Zakernezhad, F., Barati, M., Sanadgol, N., Movahhedi, M., Majd, A., and Golab, F. (2021). The association between fennel extract, serum lipid profile, and leptin receptor expression. Basic Clin. Neurosci. 12 (6), 711–720. doi:10.32598/bcn.2021.998.2
Zhang, C., Zhang, J., Zhu, L., Du, Z., Wang, J., Wang, J., et al. (2020). Fluoxastrobin-induced effects on acute toxicity, development toxicity, oxidative stress, and DNA damage in Danio rerio embryos. Sci. Total Environ. 715, 137069. doi:10.1016/j.scitotenv.2020.137069
Keywords: LD50, oxidative stress, fenchone, sodium, potassium
Citation: Bashir A, Mushtaq MN, Younis W and Anjum I (2023) Fenchone, a monoterpene: Toxicity and diuretic profiling in rats. Front. Pharmacol. 14:1119360. doi: 10.3389/fphar.2023.1119360
Received: 08 December 2022; Accepted: 02 January 2023;
Published: 26 January 2023.
Edited by:
Tariq Ismail, Abbottabad Campus, PakistanReviewed by:
Irum Shahzadi, COMSATS University Islamabad, PakistanIzhar Ullah, University of Poonch Rawalakot, Pakistan
Copyright © 2023 Bashir, Mushtaq, Younis and Anjum. This is an open-access article distributed under the terms of the Creative Commons Attribution License (CC BY). The use, distribution or reproduction in other forums is permitted, provided the original author(s) and the copyright owner(s) are credited and that the original publication in this journal is cited, in accordance with accepted academic practice. No use, distribution or reproduction is permitted which does not comply with these terms.
*Correspondence: Muhammad Naveed Mushtaq, muhammad.mushtaq2@pharm.uol.edu.pk; Asifa Bashir, aasifa.basheer@gmail.com