- 1School of Pharmacy, Chengdu University of Traditional Chinese Medicine, Chengdu, China
- 2School of Ethnic Medicine, Chengdu University of Traditional Chinese Medicine, Chengdu, China
- 3Innovative Institute of Chinese Medicine and Pharmacy, Chengdu University of Traditional Chinese Medicine, Chengdu, China
- 4Meishan Hosptial of Chengdu University of Traditional Chinese Medicine, Meishan, China
Radix Aconiti, also known as Tie-bang-chui (TBC), Pang-a-na-bao, and Bang-na, is a typical aconitum Tibetan medicine and a perennial herb of the genus Aconitum pendulum Busch. and A. flavum Hand. -Mazz. dry roots. It has high toxicity and remarkable efficacy; as such, it is a typical “highly toxic and effective” drug that needs be processed and used. Processing methods of this Tibetan medicine include non-heating of highland barley wine (HBW) and fructus chebulae soup (FCS). This work aimed to understand differences in chemical composition between non-heat processed products and raw TBC. In this study, high-performance thin-layer chromatography (HPTLC) and desorption electrospray ionization mass spectrometry imaging (DESI-MSI) were used to analyze the chemical composition of TBC processed by FCS (F-TBC) and HBW (H-TBC). The MRM mode of HPLC-QqQ-MS/MS was selected to determine the changes of several representative alkaloids to comparison with the former results. A total of 52 chemical constituents were identified in raw and processed products, and the chemical composition of F-TBC and H-TBC changed slightly compared with that of raw TBC. The processing mechanism of H-TBC was also different from that of F-TBC, which might be related to the large amount of acidic tannins in FCS. It was found that the content of all six alkaloids decreased after processing by FCS, and all five alkaloids decreased except aconitine increased after processing by HBW. The combination of HPTLC and DESI-MSI could be an effective method for rapid identification of chemical components and changing rules in ethnic medicine. The wide application of this technology provides not only an alternative method for the traditional separation and identification of secondary metabolism but also a reference for research on the processing mechanism and quality control of ethnic medicine.
1 Introduction
Radix Aconiti, also known as Tie-bang-chui (TBC), Pang-a-na-bao, Bang-na in Tibetan medicine, is a perennial herb of the genus Aconitum pendulum Busch. and A. flavum Hand. -Mazz. dry root and is mainly distributed in Qinghai, Gansu, Sichuan, Tibet and other regions of China. (Wang et al., 2016). TBC is a traditional medicinal material used in Tibetan medicine; which is sweet, slightly bitter, heat, and highly toxic and has remarkable efficacy. It is a typical “highly toxic and effective” drug with both strong toxicity and excellent efficacy. TBC is used to expel cold and relieve pain, dispel wind, and calm shock mainly for Long disease, cold disease, Huangshui disease, leprosy, madness, and so on (Dimaer, 1986).
TBC is commonly used as a highly poisonous aconite Tibetan medicine. Many processing methods are used for TBC in ancient and modern times to ensure its safety; these methods include heat processing (baking, stir-frying, simmering, steaming, boiling, etc.) and non-heat processing (liquid auxiliary material soaking, bleaching, etc.) (Li et al., 2022). The main materials used for processing this Tibetan medicine are highland barley wine (HBW) and fructus chebulae soup (FCS) (Figure 1). Previous literature review and on-the-spot investigation showed that non-heat processing methods are often used in Tibetan hospitals and medicine factories. However, research on the related mechanism of non-heat processing is still in the primary stage and needs further work.
Various analytical methods, such as thin layer chromatography (TLC), high-performance liquid chromatography (HPLC), liquid chromatography mass spectrometry (LC-MS), and so on, have been used to determine aconitum alkaloid components. HPLC separation is the most commonly used method, but it has some disadvantages, such as long analysis time, complex preparation of mobile phase, and high solvent consumption (Csupor et al., 2007; Zhi et al., 2020). Therefore, a time-saving method with low reagent requirement should be developed to visualize changes in alkaloid composition of non-heat processed TBC.
Desorption electrospray ionization (DESI) was first proposed by Cooks team. DESI has rapid, highly sensitive in situ detection and has made great progress, especially in situ tumor analysis, in situ brain imaging, real-time dynamic change detection of chemical composition in the processing of traditional Chinese medicine, etc. This method has been widely used in food, drugs, forensic medicine, environment, and other fields (Milojković-Opsenica et al., 2022).
TLC detection is the simplest and most effective method for central control of almost all chemical drugs and quality control of traditional Chinese medicines and their excipients, which was used for quality control analysis in nearly 94% of the varieties in the first part of the Chinese Pharmacopoeia in the 2020 edition and nearly 40 varieties in the fourth part of the Chinese Pharmacopoeia in the fourth part of the excipients. Low cost, flexible and convenient operation, simple sample pre-treatment, high throughput and easy instrument coupling are the outstanding advantages of TLC. High-performance thin-layer chromatography (HPTLC) is a thin-layer chromatographic method using a higher separation efficiency thin-layer plate, which has improved separation, sensitivity and reproducibility compared to conventional TLC. HPTLC is commonly used in drug analysis but is often insufficient to obtain information on chemical composition. HPTLC involves chromatography for separation and can be combined with DESI-MSI to obtain better identification ability than DESI-MSI or HPTLC alone. In recent years, HPTLC-DESI-MSI has been used to analyze plant metabolites and peptides (Bagatela et al., 2015; Kaddi et al., 2015; Liu et al., 2022). Therefore, HPTLC has been proved to be a simple, rapid, efficient, and solvent-saving technique for the separation and characterization of chemical components in complex samples. However, no research has investigated the use of HPTLC-DESI-MSI to separate and detect dynamic changes in chemical composition in the processing of ethnic medicines.
In this study, TBC samples from unheated FCS and HBW were prepared. TBC samples and mixed reference substances were separated on HPTLC and detected by DESI-MSI. According to the constructed database and reference materials, the chemical components of different processed products were qualitatively detected. Changes in alkaloid content in non-heat processed TBC were characterized quickly and intuitively. In order to verify the feasibility of using HPTLC-DESI-MSI to rapidly monitor the changes of the constituents before and after processing, the MRM mode of HPLC-QqQ-MS/MS was selected to determine the changes of several representative alkaloids to comparison with the former results, and it was found that the trends of the changes were consistent, which proved the feasibility of the method.
2 Materials and methods
2.1 Materials and reagents
The reference standards (HPLC>98%) of aconitine (39), acetylaconitine (42), mesaconitine (37), deoxyaconitine (36), hypaconitine (33), and benzoylaconine (31) were purchased from PUSH Biotechnology Co., Ltd. (Chengdu, China). Reserpine (DST Biological Co., Ltd., HPLC≥98%, Lot No. DST210628-056). LC-MS-grade methanol, acetonitrile and formic acid were obtained from Sigma–Aldrich (United States). HPTLC silica 60 F254 was acquired from Merck KGaA (Darmstadt, Germany). Analytical-grade anhydrous ether, chloroform, 25% ammonia, and methanol were provided by Chengdu Kelong Chemical Co., Ltd. (Chengdu, China). HBW was produced by Qinghai Huzhu Highland Barley Wine Co., Ltd. (Huzhu, China). UPLC-grade ultrapure water was produced by Elga Labwater Purelab system (Elga-Veolia, High Wycombe, United Kingdom). Leucine encephalin (LE) as internal standard (IS) was supplied by Waters (Waters Corporation, United States). TBC samples were collected from Qinghai. FCS was purchased from Chengdu New Lotus Market and identified by Professor Zhang Yi of Chengdu University of Traditional Chinese Medicine. The related specimens were stored in the School of Ethnic Medicine, Chengdu University of Traditional Chinese Medicine.
2.2 Instruments
Lac part analytical weighing scales (Shanghai Liangping Instrumentation Co.), Ultrasonic Machine (Ningbo New Yicai Ultrasonic Equipment Co., Ltd.), Rotary evaporator (BUCHI Rotavapor R-300, Switzerland), Nitrogen blowing instrument (KL-512), Waters Snapt Q-Tof mass spectrometer equipped with a DESI source (United States), High performance liquid chromatograph (Model 1260) Tandem triple quadrupole mass spectrometer (Model 6420) (Agilent, United States), ShimNex CS HPLC C18 column (5μm, 4.6 mm × 250 mm).
2.3 Sample preparation
FCS were obtained by boiling FC 50 g with 1500 mL of water for 30 min, filtered to obtain filtrate, repeat boiling three times, consolidated filtrate, concentrated to 500 mL by rotary evaporator and cooled.
For traditional Tibetan medicine processing of TBC (Li et al., 2022), an appropriate amount of TBC powder was placed in a mortar, added three times the amount of HBW, soaked for 1 h, ground for 5 h, fermented at room temperature for 1 day, and dried to obtain TBC processed by HBW (H-TBC). TBC and three times amounts of FCS were obtained for 2 days, turned every 4 h, soaked, and dried to obtain TBC processed by FCS (F-TBC).
In brief, 2.5 g of powders of raw TBC (R-TBC), H-TBC, and F-TBC were accurately weighed and placed in 100 mL stopper conical flask. The mixture was added by 35 mL of the mixed solution of ether trichloromethane (3/1, v/v) and 3 mL of ammonia test solution. The mixture was shaken, extracted by ultrasound for 1 h, stood for 10 min, and filtered. The residue was discarded and concentrated by nitrogen blowing. The residue was transferred to a 25 mL volumetric flask with acetonitrile for constant volume (Lin et al., 2011). The sample solution was diluted to the appropriate concentration before use, and then filtered through a 0.22 µm filter membrane before testing.
Appropriate amounts of aconitine, acetyl aconitine, hypoaconitine, mesaconitine, deoxyaconitine, and benzoyl aconitine were accurately weighed. The mixed reference solution (D-TBC) containing 1.7 mg of aconitine (39), 1.50 mg of acetyl aconitine (42), 1.9 mg of hypoaconitine (33), 1.1 mg of mesaconitine (37), 1.6 mg of deoxyaconitine (36), and 1.4 mg of benzoyl aconitine (31) was prepared by adding acetonitrile and shaking well. The internal standard solution was prepared by weighing 1 mg of reserpine, dissolving it in acetonitrile, transferring it to a 50 mL volumetric flask, fixing the volume with acetonitrile, and filtering it through a 0.22 μm microporous organic membrane, waiting to be used.
2.4 HPTLC separation
Silica gel-precoated HPTLC plate (200 mm × 100 mm × 0.2 mm) was used for HPTLC separation. The extract solution or D-TBC with a volume of 5 µL was continuously sampled onto the HPTLC plate with a quantitative capillary. Cyclohexane/ethyl acetate/diethylamine (8/2/1, v/v/v) was used as the mobile phase. The sample was presaturated at room temperature (25°C) for 20 min and unfolded in the mobile phase until the front of the solvent reached the defined finish line. Finally, the sample was taken out, dried naturally, and detected by mass spectrometry.
2.5 HPTLC-DESI-MSI detection
DESI-MSI experiment was carried out on a Waters Synapt G2-SI Q-TOF mass spectrometer equipped with a DESI source (Figure 2). The DESI parameters were optimized to obtain good ion signal intensity: nebulizing gas (nitrogen) pressure of 0.45 MPa; spray solvent of 70% methanol water, 30% H2O, 0.2% formic acid, and 0.1 mM LE at a flow rate of 2 μL min-1; capillary voltage of 4.5 kV; and positive ionization mode. The pixel size (150 μm X and Y pixel size) was determined based on the total scanning time of the mass spectrogram and the speed of the X–Y pixel size scanner. The mass range was m/z 100–1000, and the scanning speed was 300 μm s-1. HDI software was used to process raw MS files and create and view MSI.
2.6 HPTLC-DESI-MSI data processing
The MS raw data file was imported into HDI for imaging, and the regions of interests (ROIs) expanded by four points were exported. The self-built database, retention factor (Rf), and reference materials were used for chemical composition identification.
2.7 HPLC -QqQ-MS detection
2.7.1 HPLC-QqQ-MS data acquisition
The mobile phase consisted of 0.1% formic acid water (A) and pure acetonitrile (B), the flow rate was 0.4 mL min−1, column temperature was maintained at 25°C, sample injection volume was 3 μL, and the gradient elution were as follows: 0–10 min, 23%–25% B; 10–25 min, 25%–45% B; 25–35 min, 45%–60% B; 35–45 min, 60%–60% B; 45–50 min, 60%–100%. The negative and positive ion modes were compared for the MS analysis. The positive mode resulted in a higher sensitivity and cleaner mass spectral background than the negative mode. ESI ion source, positive ion mode detection, atomization temperature: 300°C, nitrogen flow rate 5 L min-1, nebulizing gas pressure: 45 psi, capillary voltage: 3500 V. The collision energy and fragment ions voltage parameters were optimized as follows Table 1.
2.7.2 Method validation
The specificity of the method was inspected by ion flow diagrams of the blank solvent and different channels for each control alkaloid (Figure 3 and Supplementary Table S1). From the ion flowdiagram, it can be seen that the method has good specificity.
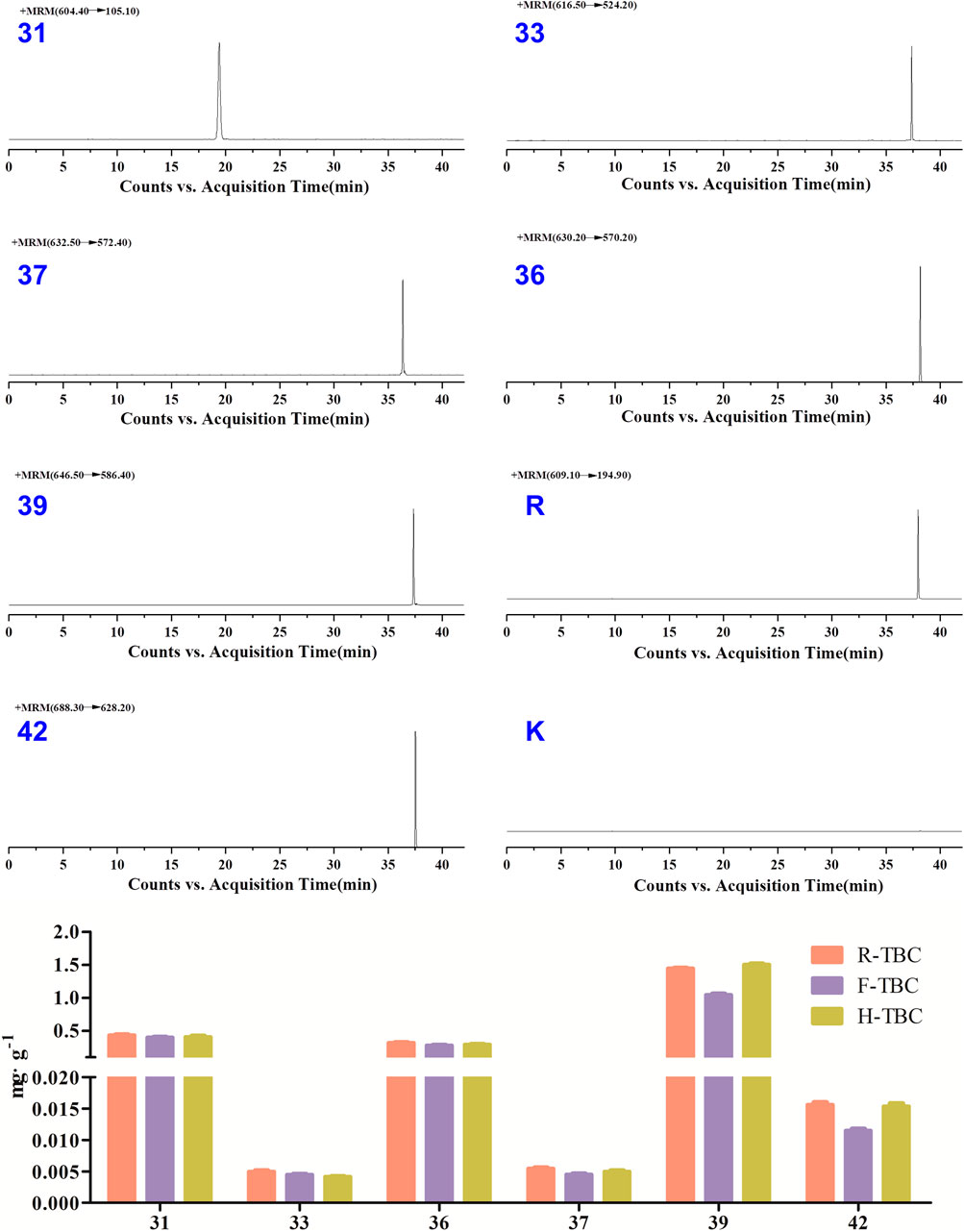
FIGURE 3. HPLC–QqQ–MS EIC of the 6 reference standard compounds, internal standard (reserpine, R) and blank solvent (B).
To measure the sensitivity and precision of the method based on MRM mode of HPLC-QqQ-MS/MS, the standard curve, linear range, recovery, limit of detection (LOD), limit of quantification (LOQ) and precision were examined. The precision was measured by calculating the relative standard deviation (RSD) of the intra- and inter-day variations in the signal intensity (peak area). Recoveries were determined using R-TBC sample spiked with standard alkaloids. The recovery was calculated with the following equation: recovery (%) = (actual test content - found content)/spiked content *100%. LOD and LOQ were evaluated at signal-to-noise ratios (S/N) of 3:1 and 10:1, respectively. Each standard of alkaloids was weighed and dissolved in acetonitrile to get a concentration about of 300 μg mL-1. A standard mixture was obtained by mixing the individual alkaloids standard solution, and then diluted to yield concentration ranges of benzoylaconine, mesaconitine, aconitine, acetylaconitine, hypaconitine, deoxyaconitine are 1.11–355.84, 0.09–143.36, 1.34–855.04, 0.15–240.64, 0.12–38.4 and 0.13–165.89 ng mL-1 separately for the construction of standard curves. For the intra-day variability test, the samples were analyzed six times within 1 day; while for the inter-day variability test, the samples were examined on three consecutive days. Three repetitions were analyzed.
Standard curves for six different concentrations of alkaloids were constructed by plotting the signal intensity (ratio of compound to internal standard peak area) versus concentration in HPLC-QqQ-MS/MS analysis. The standard curves, linearity, correlation, linear range, LOD, LOQ, intra-day and inter-day precision and stability are detailed in Table 2. The recovery rates of the six alkaloids are shown in Table 3. The standard curves of the six alkaloids showed good linear correlation (R2 > 0.999) in a certain concentration range. The values of LOD and LOQ obtained in HPLC-QqQ-MS/MS assay are less than 0.01 and 0.02 ng mL-1. The intra-day and the inter-day RSD of signal intensity (peak area) were less than 2.73% and 2.82%, respectively. Besides, the values of recovery varied from 85.73% to 98.01% for the six alkaloids. Thus, it suggested that the MRM mode of UPLC-QqQ-MS/MS have good reproducibility and precision.
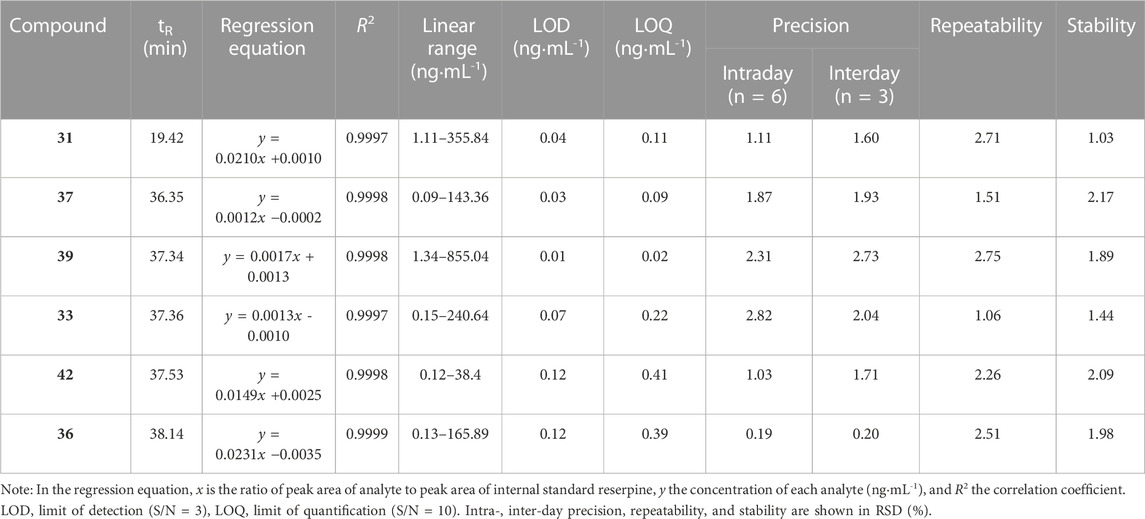
TABLE 2. HPLC-QqQ-MS detection parameters, regression equations, linear range, precision, repeatability, and stability of six alkaloids.
3 Results
3.1 HPTLC-DESI-MSI analysis of main chemical components in different TBC processed products
According to the investigation of the previous conditions and in alkaloid composition analysis, the response signal of the alkaloid components obtained using 70% methanol water +0.2% formic acid spray solvent was strong (Liu et al., 2022). Therefore, this condition was adopted in the present work. In literature, the contents of six components such as benzoylaconine (31), hypaconitine (33), deoxyaconitine (36), mesaconitine (37), aconitine (39), and acetylaconitine (42) in TBC were relatively high. The development conditions of TBC were investigated with the six components as the target (Supplementary Table S2). A relatively ideal result was obtained through optimization (Figure 4A). The HPTLC aluminum plate easily blackens under the action of alkaloid chromogenic agent, so the unfolding results were observed under UV 254 nm. Five obvious spots were found in different processed products of TBC, but these spots could not be completely compared with the mixed reference substance. In this regard, ambient mass spectrometry (MS) imaging was used for HPTLC direct in situ imaging and analysis. As shown in Figure 4B, the six components in the mixed control substance were well separated in HPTLC. The contents of hypaconitine (33) and mesaconitine (37) in the samples were lower than those of benzoylaconine (31), deoxyaconitine (36), aconitine (39), and acetylaconitine (42). Under limited conditions, the two components were not found in the imaging results. Based on the results of HPTLC and DESI-MSI, the main chemical constituents of TBC changed after processing HBW and FCS. The content of aconitine (39) in H-TBC was more than those of the raw product and F-TBC, while the contents of benzoylaconine (31), deoxyaconitine (36) and acetylaconitine (42) in H-TBC were less than R-TBC, but more than F-TBC. The results indicated that some chemical reactions possibly occurred during the processing of TBC.
3.2 HPLC-QqQ-MS for the determination of changes in the content of six alkaloids before and after TBC processing
The content of six alkaloids before and after TBC processing have been relative quantified using the signal intensity (peak area) of corresponding product ion by the MRM mode of HPLC-QqQ-MS/MS. The results showed that the content of aconitine (39) was up to 1 mg g-1 or higher and in H-TBC>R-TBC>F-TBC, benzoylaconitine (31), deoxyaconitine (36) and acetylaconitine (42) were all much lower than aconitine (39), but in R-TBC > H-TBC > F-TBC. The content of hypaconitine (33) and mesaconitine (37) was extremely low, only 0.005 mg g-1 in R-TBC and even lower after processing. It was found that the content of all six alkaloids decreased after processing by FCS, and all five alkaloids decreased except aconitine (39) increased after processing by HBW, which was consistent with the result Figure 4 of HPTLC-DESI-MSI assayed in 3.1. Due to the difference in the sensitivity of the detection methods, hypaconitine (33) and mesaconitine (37) were detected in very small amounts in HPLC-QqQ-MS/MS but not observed in HPTLC-DESI-MSI. In conclusion, the results of this content determination fully validated the feasibility of HPTLC-DESI-MSI to rapidly and accurately identify and visually present most of the variation differences of the components except for the very trace components.
3.3 Visual characterization of the chemical composition of different processed products of TBC by HPTLC-DESI-MSI
TLC can only analyze main components and needs a reference substance for comparison. The results have a high possibility of being false positive. DESI-MSI can characterize not only known components but also unknown components, and the m/z obtained can be used for identification (Mohana Kumara et al., 2019). Therefore, more accurate information can be obtained by HPTLC combined with DESI-MSI and can be directly visualized.
TBC, as a plant of the genus Aconitum, contains a large number of alkaloids, such as diterpenoid and aliphatic alkaloids. Most of these alkaloids have m/z between 300 and 900. After the comparison with reference substances, accurate molecular weight, and related references and combined with the m/z of DESI-MSI, 52 chemical constituents were inferred and identified from TBC and its different processed products. The specific information is shown in Figures 5, 6 and Table 4. In Figure 5, most alkaloids are concentrated in the m/z 300–700 and m/z 800–900 region ranges. The aliphatic alkaloids m/z are mainly between 900–800 (Csupor et al., 2009), the contents of 8-palmitoleic acid −14-benzoylmesaconine (46), 14-benzoylaconine-8-palmitate (48), and 8-linolenic acid benzoyldeoxyaconine (49) are high in F-TBC. Combined with HPTLC and DESI-MSI, it is found 8-O-Linoleoyl-14-benzoylaconine (52) is less in F-TBC, while the content of lipodeoxyaconitine (50) is the largest in H-TBC. The m/z of diterpenoid alkaloids was mainly concentrated in the range of 500–700 (Wang et al., 2003), among which the alkaloids with the biggest difference were aconine (23), 16-epi-pyrodeoxyaconitine (27), deoxyaconitine (36), and aconitine (39). Compared with TBC raw products, the contents of aconitine (39) in F-TBC decreased significantly, while the contents of aconine (23) and 16-epi-pyrodeoxyaconitine (27) increased. In addition, the content of aconitine (39) in H-TBC was significantly increased. However, no significant change in 16-epi-pyrodeoxyaconitine (27) was found in H-TBC compared with that in R-TBC. Hence, the processing and detoxification mechanism of F-TBC may be related to the conversion mode of alkaloids shown in Figure 7, which is consistent with previous literature reports (Wang et al., 2010).
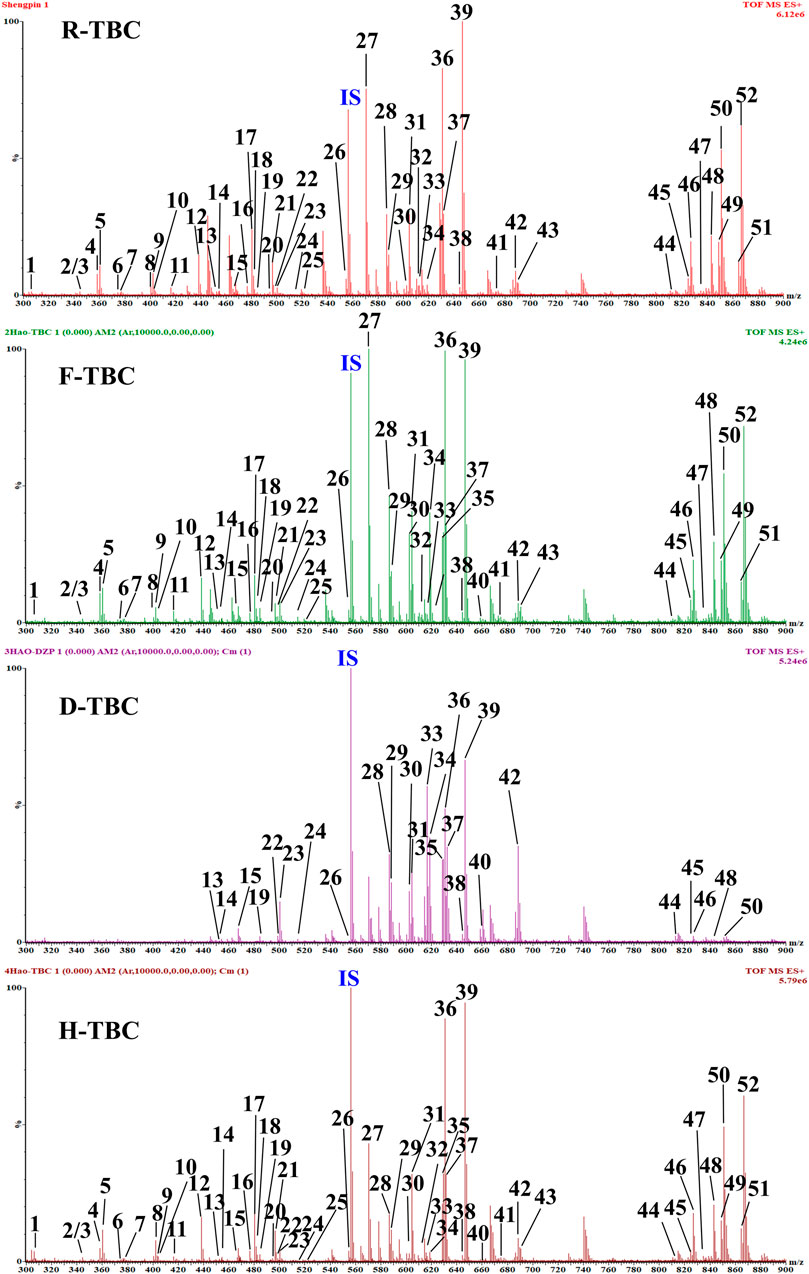
FIGURE 5. Signal of DESI-MSI acquired for mass range m/z 300–900 from HPTLC plate regions of the D-TBC, R-TBC, F-TBC and H-TBC in the positive ion mode. Putatively identfied alkaloids are labeled with m/z and compound number. See Table 4 for more details on the compound identification.
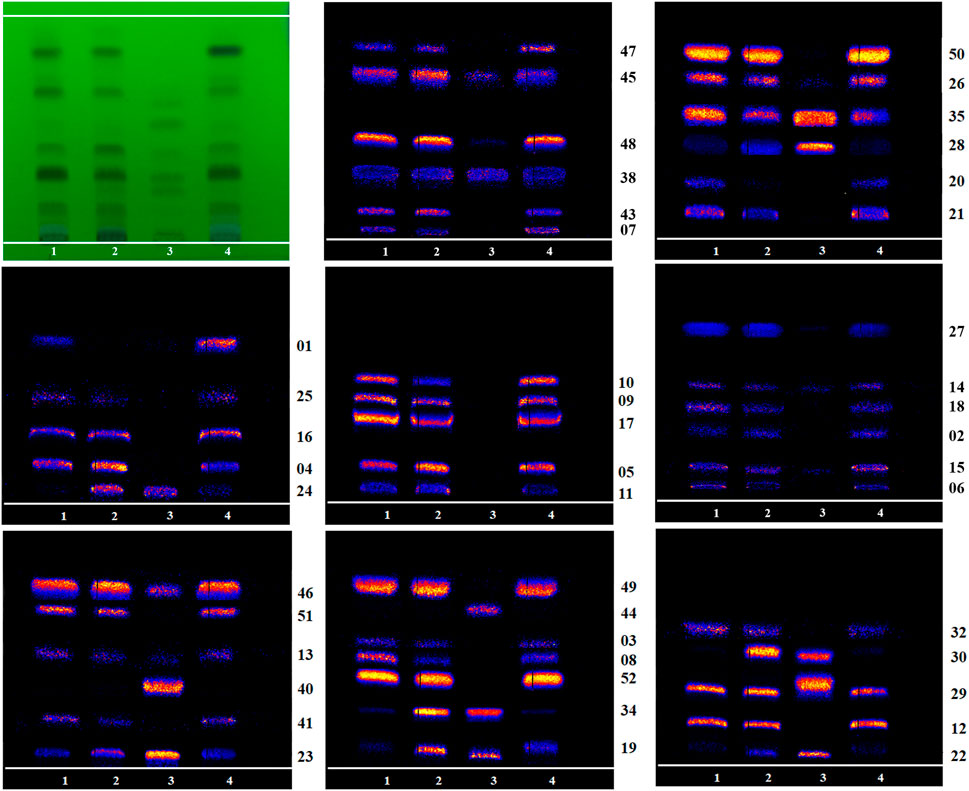
FIGURE 6. Mass Spectrometry Imaging comparison of 46 other identifiable alkaloid components on HPTLC (1: R-TBC, 2: F-TBC, 3: D-TBC, 4: H-TBC).
As shown in Figure 5, compared with R-TBC, the relative contents of napelline (5), pseudaconine (19), 8-O-methylaconine (24), 8-linolenicacid-lenzoylhypaconine (47) in T-TBC and H-TBC increased significantly, while the contents of dehydrolucidusculine (8), 14-acetylneoline (17), 8-acetyl-15-hydroxyneoline (21), kongboendine (26), and 1-demethoxyyunaconitinone/anhydroaconitine (35) decreased significantly. Interestingly, songorine (4), nagaconitine A (43) and 8-heptadecenoic acid-benzoylhypaconine (45) increased in F-TBC but decreased in H-TBC, samandarin (1) and karakanine (7) decreased in F-TBC and increased in H-TBC. In addition, it was worth noting that some components increased irregularly. For example, compared with R-TBC, the content of 10-hydroxyllycoctonine (22), 16-epi-pyroaconitine (28), austroconitine B/geniculatine B (30) and 8-O-methyl-14-benzoylaconine (34) in F-TBC increased, while the content of acoridine (9), 12-acetyl-12-epi-napelline (10), olividine (20), and 1, 14-diacetylneoline (25) decreased, unchanged in H-TBC. Finally, compared with R-TBC, the contents of guan fu base Z (11) and 16-epi-pyrodeoxyaconitine (27) in H-TBC decreased, but the two components did not change in F-TBC. Based on the changes in these chemical components, the processing mechanism of F-TBC and H-TBC is very different and worthy of further study.
4 Discussion
In this study, the combined technique of HPTLC and DESI-MSI was adopted, instead of the commonly used HPLC and LC-MS detection, because this combined technique requires simple equipment operation, no complicated sample preparation process, fast analysis time and less solvent consumption, which is a more time-saving and environment-friendly analysis method (Tang et al., 2021; Milojković-Opsenica et al., 2022). In addition, DESI-MSI can be used for in situ detection and direct identification of compounds according to ion mass-charge ratio, which makes up for the limitation of HPTLC in identifying compounds dependent on reference substances (Parrot et al., 2018; Ristivojevi´c et al., 2020). The results showed that HPTLC was used for chromatographic separation of extracts, and only 5 main compound spots could be seen under fluorescence, and 52 compounds could be identified by in situ detection combined with DESI-MSI, and the relative content difference between the extracts before and after processing with TBC was visually displayed. The content of the six target alkaloids before and after different processing methods were also detected by HPLC-QqQ-MS, and the differences in content changes were highly consistent with the results of HPTLC-DESI-MSI analysis. This study further confirms that HPTLC-DESI-MSI is a simple, rapid, efficient, and solvent-saving technique with significant advantages in the separation and characterization of chemical components in complex samples.
The results showed certain changes in alkaloids after the processing of F-TBC and H-TBC, such as pseudaconine (19), 8-O-methylaconine (24), and deoxyaconitine (36) were decreased. However, the changes of compounds caused by the two processing methods were not identical. Compared with aconitine (39) increased in H-TBC, but in F-TBC was decreased more, and aconine (23), 16-epi-pyrodeoxyaconitine (27), 16-epi-pyroaconitine (28), benzoylaconine (31), 8-O-methyl-14-benzoylaconine (34), 8-heptadecenoic acid-benzoylhypaconine (45), 14-benzoylaconine-8-palmitate (48), 8-O-linoleoyl-14-benzoylaconine (52), and other components increased. Almost all of these added components were the products of degradation or hydrolysis of ester aconitine and aconitine. Studies have been confirmed that the main toxic components of TBC are diester-diterpenoid alkaloids such as aconitine, deoxyaconitine and 3-acetylaconitine (Singhuber et al., 2009; Zhang et al., 2014). FCS mainly contains tannin and phenolic acids. In the processing process, FCS can accelerate the leaching of alkaloids and reduce the concentration of toxic alkaloids (Li et al., 2021; Zha et al., 2000). At the same time, some of the diester-diterpenoid alkaloids were converted into less toxic monoester-diterpenoid or non-esterified diterpene alkaloids (Chen R et al., 2014) (Figure 6). However, compared with F-TBC, aconitine (39) increased, aconine (23), 16-epi-pyrodeoxyaconitine (27), and benzoylaconine (31) almost unchanged, while samandarin (1), 14-O-acetylsenbusine A (15), and 8-linolenicacid-benzoylhypaconine (47) increased slightly. Songorine (4), Guan Fu base Z (11), 8-acetyl-15-hydroxyneoline (21), 13-deoxyanhydroaconitine (32), nagaconitine A (43), and 8-Heptadecenoic acid-benzoylhypaconine (45) decreased slightly, but the changes of these compounds did not show obvious transformation trend, and their chemical mechanism was unclear. These results also indicated that the processing mechanisms of F-TBC and H-TBC were completely different.
According to the processing experiment, H-TBC and F-TBC belong to the non-heating processing methods of traditional Tibetan medicine, which are obviously different from other heating processing methods such as boiling, steaming and frying. It has been reported that heating processing method could hydrolyze or pyrolyze diester-diterpenoid toxic alkaloids, such as deoxyaconitine (36), aconitine (39), 3-acetylaconitine (42) into monoester-diterpenoid or non-esterified diterpene alkaloids (Wang et al., 2010). For example, the content of aconitine (39) was less than one-tenth the amount of raw aconitine after heating and was undetectable (Zhi et al., 2020). Although the content of deoxyaconitine (36) and 3-acetylaconitine (42) was decreased by non-heating processing of H-TBC and F-TBC not as significant as that of heating processing, especially, the content of aconitine (39) was instead increased during the HBW processing. Previous studies proved the toxicity after heating type processing of TBC < F-TBC<H-TBC, and the efficacy was the drug effect was consistent with the toxicity, which indicated that the diester-diterpenoid alkaloids were not only the toxic substances of TBC, but also the key pharmacodynamic substances (Zhou et al., 2015).
In conclusion, the chemical transformation mechanism of TBC non-heating processing method was significantly different from that of heating processing method. Compared with the heating processing method, the chemical transformation mechanism of the non-heating processing was more complex and diverse, and the main chemical transformation mechanism of the processing of F-TBC was basically clear through this study. While the chemical composition change of H-TBC was not found out obvious rules. Therefore, the mechanism of the two non-heating processing methods needs to be further studied, and the principle of detoxification needs to be further clarified through body experiments, which will provide guidance for the correct clinical use of the traditional non-heating processing F-TBC and H-TBC, and expanding the application range of TBC.
5 Conclusion
In this study, a combination of HPTLC and DESI-MSI was developed for rapid and high-resolution characterization of alkaloid changes in raw and processed products of TBC. A total of 52 chemical constituents were identified in TBC, and 27 chemical constituents were changed during processing, including 9 common constituents in F-TBC and H-TBC, 12 chemical constituents unique in F-TBC, and 6 constituents unique in H-TBC. According to the changes in diester and monoester alkaloids, these components of F-TBC and H-TBC did not change much, and a large number of diester alkaloids were retained. Monoester and ethanolamine alkaloids increased or decreased. From the change of the whole chemical composition, F-TBC has a certain change rule. FCS contains a large amount of tannins and is acidic, which can accelerate the hydrolysis of toxic diester alkaloids. Tannins in FCS can complex with alkaloids to form insoluble substances. H-TBC does not have this rule. In conclusion, HPTLC-DESI-MSI is a feasible method to quickly and accurately identify and visualize most of the variation differences in components except for very trace components, which can be used to separate and identify alkaloids of different processed varieties of TBC. This study not only provides an alternative method for the traditional separation and identification of secondary metabolism but also develops a method reference for the study of processing mechanism and quality control of ethnic medicine.
Data availability statement
The original contributions presented in the study are included in the article/Supplementary Material, further inquiries can be directed to the corresponding authors.
Author contributions
All the authors have accepted responsibility for the entire content of this submitted manuscript and approved submission. XT, Investigation, Methodology, Data curation, Writing-original draft. QH, Investigation, Methodology, Data curation. ZP, Detect, Formal analysis. YL, Project administration, Supervision. ZF, Investigation, Collection materials. CL, Preparation of experimental solution. CT, Validation, Writing -review and editing. YZ, Resources, Project administration, Supervision, Validation, Writing -review and editing.
Funding
The National Natural Science Foundation of China (No. 82130113), the National Key Research and Development Program of China (No. 2017YFC1703904), the China Postdoctoral Science Foundation (No. 2021MD703800), and the Science Foundation for Youths of Science & Technology Department of Sichuan Province (No. 2022NSFSC1449 and 2023NSFSC1775).
Conflict of interest
The authors declare that the research was conducted in the absence of any commercial or financial relationships that could be construed as a potential conflict of interest.
Publisher’s note
All claims expressed in this article are solely those of the authors and do not necessarily represent those of their affiliated organizations, or those of the publisher, the editors and the reviewers. Any product that may be evaluated in this article, or claim that may be made by its manufacturer, is not guaranteed or endorsed by the publisher.
Supplementary material
The Supplementary Material for this article can be found online at: https://www.frontiersin.org/articles/10.3389/fphar.2023.1104473/full#supplementary-material
References
A, P., Chen, D. L., Chen, Q. H., Jian, X. X., and Wang, F. P. (2002). Structure of kongboendine. Nat. Prod. Res. Dev. 14, 6–8. doi:10.3969/j.issn.1001-6880.2002.05.002
Bagatela, B. S., Lopes, A. P., Cabral, E. C., Perazzo, F. F., and Ifa, D. R. (2015). High-performance thin-layer chromatography/desorption electrospray ionization mass spectrometry imaging of the crude extract from the peels of citrus aurantium L (rutaceae). Rapid Commun. Mass Sp. 29, 1530–1534. doi:10.1002/rcm.7246
Chen, R., Sun, G., Zhang, Q., and Sun, X. (2014). Advances in research on processing and toxicology reduction of Aconiti lateralis Radix praeparata. Chin. J. Exp. Tradit. Med. Formulae 20, 237–241. doi:10.13422/j.cnki.syfjx.2014150237
Chen, S., Li, W. H., Chen, X. F., Liu, M., Zhu, Z. Y., and Chai, Y. F. (2014). Research progress of components and quality control analysis of alkaloids in fuzi. Chin. J. Pharm. Anal. 34, 1709–1717. doi:10.16155/j.0254-1793.2014.10.038
Csupor, D., Strömberg, M., Bohlin, L., Woelkart, K., Wenzig, E., Hohmann, J., et al. (2007). The effect of the extraction and processing methods on the alkaloid composition of roots of aconitum carmichaeli. Planta Med. 73, 391. doi:10.1055/s-2007-987171
Csupor, D., Wenzig, E. M., Zupkó, I., Wölkart, K., Hohmann, J., and Bauer, R. (2009). Qualitative and quantitative analysis of aconitine-type and lipo-alkaloids of aconitum carmichaelii roots. J. Chromatogr. A 1216, 2079–2086. doi:10.1016/j.chroma.2008.10.082
Díaz, J. G., Ruiz, J. G., and De, L. (2000). Alkaloids from delphinium staphisagria. J. Nat. Prod. 63, 1136–1139. doi:10.1021/np990453l
Frejat, F. O. A., Xu, W. L., Shan, L. H., and Zhou, X. L. (2017). Three New lactone-type diterpenoid alkaloids from aconitum rotundifolium kar. and kir. Heterocycles 94, 1903–1908. doi:10.3987/COM-17-13768
FuenteReinaValencia, G. D. L. M. E., and Rodríguez-Ojeda, A. (1988). The diterpenoid alkaloids from aconitum napellus. Heterocycles 27, 1109–1113. doi:10.3987/COM-87-4445
Grandez, M., Madinaveitia, A., Gavín, J. A., Alva, A., and De La Fuente, G. (2002). Alkaloids from consolida oliveriana. J. Nat. Prod. 65, 513–516. doi:10.1021/np010065v
Habermehl, G., and Ott, H. H. (1976). ChemInform abstract: Samandarin and related alkaloids, xxiii. 20-METHYL-5-PREGNENE-3β,16β-DIOL. Chem. Inf. 7, 135–138. doi:10.1002/chin.197609340
Kaddi, C. D., Bennett, R. V., Paine, M. R., Banks, M. D., Weber, A. L., Fernández, F. M., et al. (2015). DetectTLC: Automated reaction mixture screening utilizing quantitative mass spectrometry image features. J. Am. Soc. Mass Spectr. 27, 359–365. doi:10.1007/s13361-015-1293-9
Kitagawa, I., Chen, Z. L., Yoshihara, M., and Yoshikawa, M. (1984). Chemical studies on crude drug processing. II. Aconiti tuber (1). On the constituents of" chuan-Wu", the dried tuber of aconitum carmichaeli debx. Yakugaku Zasshi 104, 848–857. doi:10.1248/yakushi1947.104.8_848
Li, C. Y., Zhou, Z., Xu, T., Wang, N. Y., Tang, C., Tan, X. Y., et al. (2022). Aconitum pendulum and aconitum flavum: A narrative review on traditional uses, phytochemistry, bioactivities and processing methods. J. Ethnopharmacol. 292, 115216. doi:10.1016/j.jep.2022.115216
Li, H., Zhang, G., Chen, T., Gao, Y., Song, L., Hou, H., et al. (2021). Research progress on mechanism of reducing toxicity and increasing efficiency of compatibility of Mongolian medicinal materials Terminalia chebula and aconitum kusnezoffii. World Chin. Med. 24, 3701–3706. doi:10.3969/j.issn.1673-7202.2021.24.025
Li, L. Y., Chen, Q. H., Zhou, X. L., Chen, D. L., Wang, F. P., and Che, C. T. (2003). New norditerpenoid alkaloids from aconitum hemsleyanum var. Leueanthus. J. Nat. Prod. 66, 269–271. doi:10.1021/np020350u
Li, Q., Sun, S., Wang, M., Li, C., Yuan, D., and Fu, H. (2018). Chemical constituents and analgesic activity of aconitum kusnezoffii reichb. J. Chin. Pharm. Sci. 27, 855–863. doi:10.5246/jcps.2018.12.087
Lin, W., Fan, Z., Upur, H., and TingTing, Z. (2011). Influences on contents of alkaloid in aconitum pendulum Busch samples processed by different methods. Chin. J. Tradit. Chin. Med. Pharm. 26, 1307–1309. doi:10.1007/s10008-010-1224-4
Liu, Y., Yang, X., Zhou, C., Wang, Z., Kuang, T., Sun, J., et al. (2022). Unveiling dynamic changes of chemical constituents in raw and processed fuzi with different steaming time points using desorption electrospray ionization mass spectrometry imaging combined with metabolomics. Front. Pharmacol. 13, 842890. doi:10.3389/fphar.2022.842890
Lu, L., Yue, H., Song, F.-R., Tsao, R., Liu, Z. Q., and Liu, S. Y. (2010). Rapid profiling of alkaloids in several medicinal herbs by matrix-assisted laser desorption/ionization mass spectrometry. Chem. Res. Chin. U. 26, 11–16. doi:10.1002/mas.1280100503
Milojković-Opsenica, D. M., Trifković, J. Ð., Ristivojević, P. M., and Andrić, F. L. (2022). Thin-layer chromatography in the authenticity testing of bee-products. J. Chromatogr. B 1188, 123068. doi:10.1016/j.jchromb.2021.123068
Mohana Kumara, P., Uma Shaanker, R., and Pradeep, T. (2019). UPLC and ESI-MS analysis of metabolites of rauvolfia tetraphylla L. And their spatial localization using desorption electrospray ionization (DESI) mass spectrometric imaging. Phytochemistry 159, 20–29. doi:10.1016/j.phytochem.2018.11.009
Parrot, D., Papazian, S., Foil, D., and Tasdemir, D. (2018). Imaging the unimaginable: Desorption electrospray ionization-imaging mass spectrometry (DESI-IMS) in natural product research. Planta Med. 84, 584–593. doi:10.1055/s-0044-100188
Peiqin, C., Lan, H., Lisheng, D., Guolin, Z., and Bogang, L. (1997). Lactone-Type Norditerpenoid Alkaloids from Aconitum Pulchellum. Nat. Prod. Res. Dev. 9, 1–3. doi:10.16333/j.1001-6880.1997.01.001
Reinecke, M., Watson, W. H., de Chang, C., and Yan, W. M. (1986). A 2-D NMR structure determination of guan-fu base Z, a New diterpene alkaloid from the Chinese herb guan-Bai-Fu-Tzu (aconitum koreanum). Heterocycles (Sendai) 24, 49–61. doi:10.3987/R-1986-01-0049
Ristivojevi´c, P., Trifkovi´c, J., Andri´c, F., and Milojkovi´c-Opsenica, D. (2020). Recent trends in image evaluation of HPTLC chromatograms. J. Liq. Chromatogr. Relat. Technol. 43, 291–299. doi:10.1080/10826076.2020.1725555
Singhuber, J., Zhu, M., Prinz, S., and Kopp, B. (2009). Aconitum in traditional Chinese medicine: A valuable drug or an unpredictable risk? J. Ethnopharmacol. 126, 18–30. doi:10.1016/j.jep.2009.07.031
Song, B., Wang, F., Yu-Ze, L. I., Zhang, H. W., Huang, W. L., Cui, L. I., et al. (2019). Chemical constituents from aconitum szechenyianum. Chin. Trad. Pat. Med. 41, 1871–1875. doi:10.3969/j.issn.1001-1528.2019.08.024
Sultankhodzhaev, M., Beshitaishvili, L., Yunusov, M., Yagudaev, M., and Yunusov, S. Y. (1980). Structure of aconifine. Struct. Aconifine. Chem. Nat. Compd. 16, 481–486. doi:10.1007/BF00571044
Sultankhodzhaev, M. (1993). Karakanine-A New alkaloid from the epigeal part of aconitum karakolicum. Chem. Nat. Compd. 29, 51–52. doi:10.1007/bf00631014
Takayama, H., Okazaki, T., Yamaguchi, K., Aimi, N., Haginiwa, J., and Sakai, S. I. (1988). Structure of two New diterpene alkaloids, 3-epi-Ignavinol and 2, 3-dehydrodelcosine. Chem. Pharm. Bull. 36, 3210–3212. doi:10.1248/cpb.36.3210
Tang, C., Guo, T., Zhang, Z., Yang, P., and Song, H. (2021). Rapid visualized characterization of phenolic taste compounds in tea extract by high-performance thin-layer chromatography coupled to desorption electrospray ionization mass spectrometry. Food Chem. 355, 129555. doi:10.1016/j.foodchem.2021.129555
Tang, T., Chen, D., and Wang, F. (2014). A New C-19-Diterpenoid alkaloid from aconitum vilmorinianum. Chin. J. Org. Chem. 34, 909–915. doi:10.6023/cjoc201401013
Wada, K., Bando, H., and Amiya, T. (1985). Two New C20-diterpenoids alkaloids from aconium yesoense var. Macroyesoense (nakai) tamura, structures of dehydrolucidusculine and N-deethyldehydrolucidusculine. Heterocycles (Sendai) 23, 2473–2477. doi:10.3987/R-1985-10-2473
Wang, B., Dong, J., Ji, J., Yuan, J., Wang, J., Wu, J., et al. (2016). Study on the alkaloids in Tibetan Medicine Aconitum pendulum Busch. by HPLC-MSn combined with column chromatography. J. Chromatogr. Sci. 54 (5), 752–758. doi:10.1093/chromsci/bmw002
Wang, H., Lao, A., Fujimoto, Y., and Tatsuno, T. (1985). The structure of polyschistine A, B and C: Three New diterpenoid alkaloids from aconitum polyschistum hand-mazz. Heterocycles (Sendai) 23, 803–807. doi:10.3987/R-1985-04-0803
Wang, L., Zhang, X. L., Wang, X., Zhang, Y. F., Chen, J., Tan, P., et al. (2017). Comparative study on unprocessed Aconiti Radix and unprocessed Aconiti lateralis Radix by UPLC-MS∼n. Chin. J. Pharm. Anal. 37, 1640–1647. doi:10.16155/j.0254-1793.2017.09.14
Wang, Y. J., Zhang, J., Zeng, C. J., Yao, Z., and Zhang, Y. (2011). Study on the diterpene alkaloids from processed roots of Aconitum pendulum. West China J. Pharm. Sci. 26, 11–13. doi:10.13375/j.cnki.wcjps.2011.01.020
Wang, Y., Liu, Z. Q., Song, F. R., and Liu, S. Y. (2003). Study on aconitum diterpenoid alkaloids from flowers of aconitum kusnezoffi and its decoction by ESI-MS. Acta Pharm. Sin. 38, 290–293. doi:10.16438/j.0513-4870.2003.04.013
Wang, Y., Zhang, J., Tian, H., Zeng, C., Yao, Z., and Zhang, Y. (2010). Study on processing principle of aconitum pendulum. China J. Chin. Mater. Med. 35, 588–592. doi:10.4268/cjcmm20100510
Wei, X. Y., Qiu, Z. D., Chen, J. L., Sun, R. Q., Huang, L. Q., and Lai, C. (2019). Research advancement in mechanisms of processing and compatibility for detoxication of aconitums. China J. Chin. Mater. Med. 44, 3695–3704. doi:10.19540/j.cnki.cjcmm.20190629.301
Xu, T. F., Liu, S., Meng, L. L., Pi, Z. F., Song, F. R., and Liu, Z. Q. (2016). Bioactive heterocyclic alkaloids with diterpene structure isolated from traditional Chinese medicines. J. Chromatogr. B 1026, 56–66. doi:10.1016/j.jchromb.2015.09.023
Xu, W. M., Zhang, M., Liu, H. W., Wei, K., He, M., Li, X. Y., et al. (2019). Antiviral activity of aconite alkaloids from Aconitum carmichaelii Debx. Nat. Prod. Res. 33, 1486–1490. doi:10.1080/14786419.2017.1416385
Yang, L. H., Lin, L. M., Wang, Z. M., Li, C., and Li, Z. (2016). Advance on chemical compounds of Tibetan medicinal plants of aconitum genus. China J. Chin. Mater. Med. 41, 362–376. doi:10.4268/cjcmm20160302
Yang, L., Zhang, Y., Mei, S., and Zhu, Z. (2019). Diterpenoid alkaloids from the roots of aconitum brachypodum diels. And their chemotaxonomic significance. Biochem. Syst. Ecol. 85, 43–45. doi:10.1016/j.bse.2019.05.004
Zha, W. Q., Wang, X. T., and Yuan, S. T. (2000). The Influence of Traditional Chinese Drug Processing Methods on the Toxic Constituent of Phytolacca Acinosa. J. Beijing Univ. Tradit. Chin. Med. 23, 50–55. doi:10.3321/j.issn:1006-2157.2000.05.016
Zhang, L., Liu, F. L., Liu, C. A., Zhai, X. Y., Liao, Q. H., and Yang, S. L. (2014). Simultaneous quantitative determination of five alkaloids in Catharanthus roseus by HPLC-ESI-MS/MS. Chin. Trad. Herb. Drug. 45, 786–793. doi:10.1016/S1875-5364(14)60120-5
Zhang, S., and Jia, S. (1999). Isolation and identification of New diterpenoid alkalotds from root of aconitum excelsum reichb. Acta Pharm. Sin. 34, 766–770. doi:10.16438/j.0513-4870.1999.10.010
Zhao, D. K., Shi, X. Q., Zhang, L. M., Yang, D. Q., Guo, H. C., Chen, Y. P., et al. (2017). Four New diterpenoid alkaloids with antitumor effect from aconitum nagarum var. Heterotrichum. Chin. Chem. Lett. 28, 358–361. doi:10.1016/j.cclet.2016.09.012
Zhi, M. R., Gu, X. R., Han, S., Liu, K. Y., Liu, Z. Q., Tang, Y. N., et al. (2020). Chemical variation in aconti kusnezoffii Radix before and after processing based on UPLC-orbitrap-MS. China J. Chin. Mater. Med. 45, 1082–1089. doi:10.19540/j.cnki.cjcmm.20191221.301
Keywords: DESI-MSI, HPTLC, radix aconiti, processing, alkaloids
Citation: Tan X, He Q, Pei Z, Liu Y, Feng Z, Li C, Tang C and Zhang Y (2023) Rapid visual characterization of alkaloid changes in traditional processing of Tibetan medicine Aconitum pendulum by high-performance thin-layer chromatography coupled with desorption electrospray ionization mass spectrometry imaging. Front. Pharmacol. 14:1104473. doi: 10.3389/fphar.2023.1104473
Received: 21 November 2022; Accepted: 13 April 2023;
Published: 21 April 2023.
Edited by:
Anthony Booker, University of Westminster, United KingdomReviewed by:
Yonggang Liu, Beijing University of Chinese Medicine, ChinaDevendra Kumar Padey, Lovely Professional University, India
Copyright © 2023 Tan, He, Pei, Liu, Feng, Li, Tang and Zhang. This is an open-access article distributed under the terms of the Creative Commons Attribution License (CC BY). The use, distribution or reproduction in other forums is permitted, provided the original author(s) and the copyright owner(s) are credited and that the original publication in this journal is cited, in accordance with accepted academic practice. No use, distribution or reproduction is permitted which does not comply with these terms.
*Correspondence: Ce Tang, dGFuZ2NlQGNkdXRjbS5lZHUuY24=; Yi Zhang, emhhbmd5aUBjZHV0Y20uZWR1LmNu
†These authors have contributed equally to this work