- Division of Spine Surgery, Department of Orthopedic Surgery, Nanjing Drum Tower Hospital, The Affiliated Hospital of Nanjing University Medical School, Nanjing, China
Intervertebral disc degeneration (IDD) is the cardinal pathological mechanism that underlies low back pain. Mechanical stress of the intervertebral disc may result in a change in nucleus pulposus cells state, matrix degradation, and degeneration of the disc. Microtubules, which are components of the cytoskeleton, are involved in driving or regulating signal pathways, which sense and transmit mechano-transduction. Microtubule and the related proteins play an important role in the development of many diseases, while little is known about the role of microtubules in nucleus pulposus cells. Researchers have found that type II collagen (COL2) expression is promoted by microtubule stabilization in synovial mesenchymal stem cells. In this study, we demonstrated that microtubule stabilization promotes the expression of COL2 in nucleus pulposus cells. Stabilized microtubules stimulating Hippo signaling pathway, inhibiting YAP protein expression and activity. In addition, microtubules stabilization promotes the expression of COL2 and alleviates disc degeneration in rats. In summary, our study for the first time, identifies microtubule as a promising therapeutic target for IDD, up-regulating the synthesis of COL2 via Hippo-Yap pathway. Our findings may provide new insights into the etiologies and pathology for IDD, further, targeting of microtubule acetylation may be an effective strategy for the treatment of IDD.
Introduction
Intervertebral disc (IVD) locates at between the vertebrae and is responsible for the load-bearing as well as stress-sharing in the spine. Intervertebral disc degeneration (IDD) is considered to be the principal cause of low back pain, which brings a heavy economic and social burden to the global healthcare system (Priyadarshani et al., 2016). The biomechanical loading involved in the pathogenesis of IDD have been elucidated through previous researches (Walter et al., 2011; Paul et al., 2012; Paul et al., 2013). Researchers have shown that a certain compressive force on the spinal motion segment can induce catabolism in the IVD, both in vivo and in vivo (Wang et al., 2007; Wuertz et al., 2009; Paul et al., 2013). In addition, overload-induced IVD degeneration could be attenuated by inhibiting NP cell apoptosis (Yamada et al., 2014). Therefore, exploring the internal relationship between mechanical force and IDD is beneficial to the biological targeted therapy of IDD.
Microtubules (MT), filamentous actin (F-actin) and intermediate filaments (IFs) together form a three-dimensional cytoskeleton, which mediates force transmission. Most microtubules are not static, they exhibit cycles of growth, shortening and regrowth. This dynamic instability allows MT responding to changes in internal or external conditions, and thus allows a cell to alter its cytoskeleton accordingly. Dynamic MT can generate pushing forces, which could maintain the cell shape under compressive forces (Brangwynne et al., 2006). However, how the MT involves in the NP cells and how the MT affect the ECM in disc still remains unknown. Microtubules, besides their role in cell shape maintenance, are involved in secretory transport mechanisms in collagen and proteoglycan synthesis (Benjamin et al., 1994). Previous study has shown that MT stability in synovial mesenchymal stem cells can promote cartilage formation and increase type Ⅱ collagen (COL2) expression (Li et al., 2021). Since IDD progression is triggered and accelerated by the degradation of ECM and the reduced level of COL2, we hypothesized that MT stabilization could also promote the synthesis of COL2 in the NP cells, decelerating IDD progression.
Yes-associated protein (YAP) and transcriptional coactivator with PDZ-binding motif (TAZ) serve an important role in biomechanical and mechanical signaling that affects cell proliferation and differentiation. YAP/TAZ read diverse biomechanical signals and transduce them into biological effects in a manner that is specific for each type of cell and mechanical-stress, which highlights the central role of YAP as universal mechano-transducers and mechano-effectors. Studies reveal that F-actin plays such an important role in the mechano-transduction between extracellular matrix and intervertebral disc cells (Hayes et al., 1999; Chen et al., 2004). Moreover, primary cilium, the key mechano-signaling sensor which mainly consists of MT, was critical for YAP activity. However, the mechanism that regulate YAP activity in the context of MT stabilization and destabilization in NP cells remains unanswered.
Hence, we investigated the role of MT stabilization in the synthesis of COL2 in NP cells, using docetaxel (MT stabilizer) and nocodazole (MT destabilizer). Our results suggest that MT stabilization of NP cells would be a potential therapeutic target for enhancing the synthesis of COL2 and alleviating IDD.
Materials and methods
NP cells isolation and culture
All the experimental protocols were approved by the Ethics Committee of Nanjing Drum Tower Hospital, the Affiliated Hospital of Nanjing University Medical School. With the informed consent of the patients, normal NP tissues were obtained from eight patients (Three males and five females, aged 12–23 years, mean age 17.8 years) who underwent surgery for congenital scoliosis. Degenerative NP tissues were collected from eight patients (Three males and five females, aged 40–65 years, mean age 53.7 years) who underwent posterior spinal fusion surgery. The human NP tissue samples were classified according to their degenerative grades (Song et al., 2018). After cutting into pieces, human NP tissues were enzymatically digested in 0.25% trypsin (Gibco; 15050065) and 0.2% type II collagenase (Gibco; 17101015) for 3 h. Following filtration and washing in PBS, the suspension was centrifuged. Isolated cells were grown in Dulbecco’s modified Eagle medium (DMEM) supplemented with 10% fetal bovine serum (FBS) (Gibco; 10099141) and 1% penicillin-streptomycin (Invitrogen; 10378016). The medium was replaced twice a week, and cells at 3–5 passage were used for the subsequent experiments.
Western blot analysis
NP tissue and NP cells were lysed in the radioimmunoprecipitation assay (RIPA) buffer. The protein concentration was determined using a BCA kit (Thermo Scientific). 5% skim milk (Solarbio, D8340) was added to TBST (Solarbio, T1081) for 60 min to block membranes. After blocking, the membranes were incubated overnight at 4°C with the corresponding primary antibodies of Ace-tubulin (1:800; Cell Signaling Technology, 5335s), Collagen 2 (1:500; Proteintech, 28459-1-AP), SOX9 (1:1,000; Cell Signaling Technology, 82,630), Smad3 (1:1,000; Cell Signaling Technology, 9,523), YAP (1:1,000; Cell Signaling Technology, 14,074), p-YAP (1:1,000; Cell Signaling Technology, 13,008), TAZ (1:1,000; Cell Signaling Technology, 72,804), LATS1 (1:1,000; Cell Signaling Technology, 3,477), LATS2 (1:1,000; Cell Signaling Technology, 5,888), and GAPDH (1:1,000; Cell Signaling Technology, 5,174). Then, HP-linked secondary antibodies (1:5,000; Cell Signaling Technology, 7,074) were applied. The immunoblotting was detected by UVP ChemiDoc-It Imaging System (UVP, CA, USA) with the enhanced chemiluminescence detection kit (Thermo Fisher; 34,580) added to the membranes. Each blot was measured using ImageJ software for its integrated density.
Quantitative polymerase chain reaction
A total RNA extract was prepared with Trizol (Invitrogen, 15,596,018). Following reverse transcription, the RNA was converted into cDNA using PrimeScript RT Master Mix Kit (TaKaRa, RR036A). Real-time polymerase chain reactions (RT-PCRs) were carried out using the TaKaRa SYBR Premix Ex Taq (RR420A) and the ABI StepOnePlus Real-Time PCR System (Applied Biosystems, CA, USA). By normalizing by GAPDH, fold changes of interested mRNAs were calculated. The primers were designed as follows:
Col2: 5′-AAGGGACACCGAGGTTTCACTGG-3′, 5′-GGGCCTGTTTCTCCTGAGCGT-3′; SOX9: 5′-AGCGACAACTTTACCAG-3′; 5′-GGAAAACAGAGAACGAAAC-3; GAPDH: ACAGCAACAGGGTGGTGGAC-3′, 5′- TTTGAGGGTACAGCGAACTT-3′; YAP: 5′-GCTAGATAAAGAAAGCTTT-3′, 5′-CCAATAGTTCCGATCCCTT-3′; TAZ: 5′- GAATCAGCCTCTGAATCAT-3′, 5′-GTCTGAAGATCTGATCCCT-3′; LATS1: 5′-CCACCCTAC CCAAAACATCTG-3′, 5′- CGCTGCTGATGAGATTTGAGTAC-3′; LATS2: 5′-GCCAAAGACTTTTCCTGCCA-3′, 5′- TCTTTGCTCCCCAGGACTTT-3′.
5-Ethynyl-20-deoxyuridine assay
Using Ribobio’s EdU Reagent Kit (Guangzhou, China), the manufacturer’s instructions were followed to investigate cell proliferation. Following treatment with nocodazole and docetaxel for 3 days, the degenerative NPCs were exposed to 10 μM EdU for 2 h. Fluorescence images were captured using a fluorescence microscope (Zeiss, Heidelberg, Germany).
Cell counting kit-8 assay
In accordance with the manufacturer’s instructions, the viability of NPCs was evaluated by using CCK8 assay ((Solarbio). We subsequently incubated each well for 2 h at 37°C with DMEM/F12 containing 10% (v/v) CCK-8 solution after washing with PBS. Microplate readers (Thermo Scientific, Logan, UT, United States) were used to measure absorbance at 450 nm.
Immunofluorescence staining
Analyses of immunofluorescence were performed as previously described (Wu et al., 2017). We fixed cells and tissues attached to slides with 4% paraformaldehyde and permeabilized them with 0.5% Triton X-100 in PBS. After washing the slides in PBS and blocking with 2% bovine serum albumin (BSA) in PBS for 2 h at 37°C, the slides were incubated with primary antibodies against: Ace-tubulin (1:800), Col2 (1:500), or YAP (1:200). A second goat anti-rabbit antibody (Boster) was then applied at 37°C for 2 h after the slides. A DAPI staining solution (Beyotime, Nantong, China) was applied to the nuclei for 5 min before images were taken under an Olympus BX53 microscope (Zeiss, Heidelberg, Germany). Using ImageJ software (version 1.8.0), five randomly selected microscopic fields per slide were analyzed to determine the average gray value.
Immunohistochemical staining
According to the manufacturer’s instructions, immunohistochemical staining and immunofluorescent analysis were performed. The serial sections were incubated overnight at 4°C with primary antibodies against Col2 (1:500; ab34712-Abcam) and Ace-tubulin (1:800; 5335s-Cell Signaling Technology). The slides were stained immunohistochemically with secondary antibodies conjugated with HRP, incubated at 37°C for 1 h. Photographs of sections were taken using a Zeiss fluorescence microscope (Zeiss, Heidelberg, Germany).
Short interfering RNA transfection
The siRNA sequence was designed and synthesized to target the human YAP1 gene: 5-GGUGAUACUAUCAACCAAATT-3 (Hippobio). We seeded NPCs in 6-well plates and grew them to a confluence of about 70%. Transfecting the cells with either 50 nM siRNA-YAP or a negative control, as directed by the manufacturer, took place for 12 h in Lipofectamine 3,000 (Thermo Fisher Scientific).
Animal experiments
In accordance with the guidelines of the Institutional Animal Care and Use Committee at Nanjing University Medical School, all experiments were reviewed and approved by the committee. In the study, SD-Rats (10 weeks) were anesthetized with 10% chloral hydrate (0.3 mL/100 g) injected intraperitoneally. After placing the animals in a prone position, ethanol was applied to the tail skin for disinfection. A 21 G needle with a stopper was used to percutaneously puncture the discs Co6-7, Co7-8, and Co8-9 to the depth of 5 mm, after which the needle was rotated 360 degrees and held for 30 s. Four weeks after acupuncture, the caudal intervertebral discs of rats were examined by microCT and MRI. This study used MicroCT instead of X-ray to calculate the intervertebral disc height index (DHI), which is conducive to more intuitive indication of the changes in intervertebral disc height. The calculation method of DHI is as previously reported (Han et al., 2008). Degeneration grade of rat intervertebral disc was calculated and counted by MRI. We performed three IVDs on rats (Co6/7, Co7/8, and Co8/9) for the different treatments in order to evaluate the therapeutic effectiveness of microtubule stabilization for IDD. PBS, Nocodazole (3 μg/mL), and Docataxel (30 μg/mL) were administered intradiscally at the same total injection volume (2 μL) using 33-gauge needles (Hamilton, Benade, Switzerland) for all three IVDs (Co6/7, Co7/8, and Co8/9). During the 4 weeks treatment period, injections were given every week. After 4 weeks of treatment, microCT and MRI were performed again, and the intervertebral disc tissue of rats was stored in formalin for subsequent tissue staining.
Histologic analysis
Using CO2 inhalation, all animals were sacrificed and the vertebral segments were dissected from the coccygeal segments 6–7, 7–8, and 8–9. Specimens were fixed in 10% formalin for 20 h at room temperature, then decalcified in an ethylenediaminetetraacetic acid (EDTA)-glycerol solution for 4 weeks. An embedding of decalcified tissue in paraffin, sectioning sagittally to a thickness of 5 μm. Hematoxylin-eosin was used to stain the slides of each disc, and a histological grading system was used to grade them (Han et al., 2008). Meanwhile, Masson staining and COL2 immunohistochemical staining was performed on the sectioned tissues.
Statistical analysis
The means and standard deviations were calculated for all data. In GraphPad Prism 8, one-way ANOVA was used for the statistical analysis. In this study, statistical significance was defined as a p < 0.05.
Results
High expression of ace-tubulin and low expression of COL2 in human degenerated NP cells
In order to harvest the degenerated NP cells, we collected human NP tissue samples from patients who underwent posterior transforaminal lumbar interbody fusion surgery due to lumbar spinal stenosis. The degenerated intervertebral discs all demonstrated low signal intensity (Pfirrmann grade Ⅳ or Ⅴ) in T2 Weight images of Magnetic Resonance (Figure 1A). The normal NP tissues in this study were derived from patients with congenital scoliosis who underwent posterior hemivertebra resection surgery (Figure 1A). The histological characteristics of degenerated NP tissues and normal NP tissues were evaluated using HE staining, Safranin O-fast green staining and Masson staining, to specify the different degeneration grade and collagen expression (Figure 1B). According to the HE staining, Safranin O-fast green staining and Masson staining, decreased number of NP cells and decreased expression of collagen were shown in the degenerated NP tissue. Since loss of COL2 presented a very important phenotype in the progression of IDD, we then compared the expression of COL2 in degenerated NP cells and normal NP cells. The lower expression of COL2 in degenerated NP cells was confirmed by Western blot, Cell immunofluorescence and Immunohistochemistry (Figures 1C, D, F).
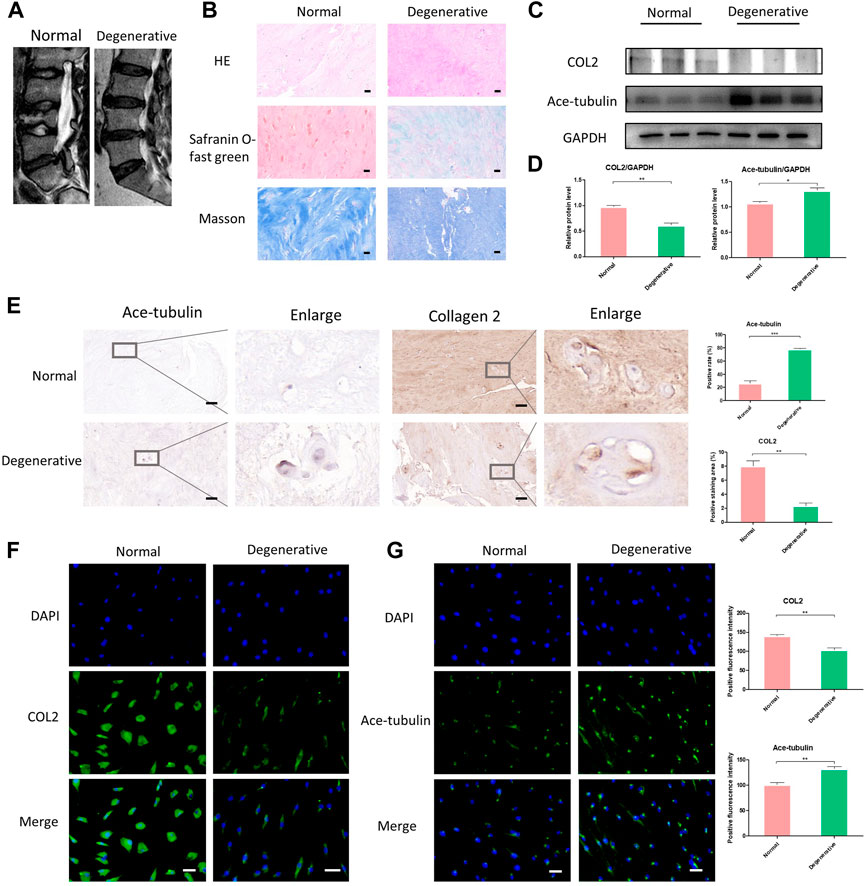
FIGURE 1. The differential expression of Ace-tubulin and type Ⅱ collagen (COL2) in human degenerated nucleus pulposus (NP) cells and normal NP cells. (A) T2 weighted imaging of human degenerative and normal intervertebral discs. (B) The HE staining, safranin O-fast green staining and Masson staining of human degenerated and normal nucleus pulposus tissues. (C, E) Western blot analysis and immunohistochemical staining of human degenerative and normal nucleus pulposus tissues. (D) Quantification of the data of (C). n = 4. (F, G) Cell immunofluorescence staining of human degenerated and normal NP cells, Scale bar, 100 μm. Data are represented as the mean ± SEM. *p < 0.05, **p < 0.01, ***p < 0.001.
Microtubules are involved in secretory transport mechanisms in collagen and proteoglycan synthesis. Thus we explored the stability of microtubules in degenerated NP cells. As previously reported, acetylated tubulin (Ace-tubulin) was used as a marker and quantitative index for microtubule stabilization since tubulin acetylation was found mainly on stable microtubules resistant to depolymerization (Westermann and Weber, 2003). Tubulin acetylation is a consequence of microtubule stability. Here, in the results of Western blot, cell immunofluorescence and immunohistochemistry, the expression of Ace-Tubulin was enhanced in degenerated NP cells (Figures 1C, E, F). For the first time, we observed increased Ace-tubulin in the NP cells, representing enhanced microtubule stability.
MT stabilization promotes the expression of COL2 in NP cells
Since the microtubule stability may regulate the cellular function of NP cells, we then investigated the effect of upregulation and the downregulation of microtubule stability on NP cells. Docetaxel, the microtubule stabilizer, and nocodazole, the microtubule depolymerizer, were used for the stabilization and destabilization of the microtubules, respectively. Regarding the cytotoxicity induced by high concentrations of docetaxel and nocodazole, we evaluated the proliferation and cell viability of NPCs treated with different concentrations of docetaxel and nocodazole using the CCK-8 and EdU staining. The EdU staining showed that the proliferation of NP cells was not significantly affected by docetaxel (0.5, 1, and 2.5 nM) or nocodazole (5, 10, and 25 nM), but was significantly inhibited by docetaxel at higher concentrations (5 and 10 nM) and enhanced by nocodazole at higher concentrations (50 and 100 nM) (Figures 2A, B). NP cells were assessed with the CCK-8 assay for cell viability (Figure 2C). Based on the results, 25 nM nocodazole slightly enhanced NP cells proliferation, while docetaxel within 2.5 nM had no significant effect on cell viability. The above results indicated that 2.5 nM docetaxel and 25 nM nocodazole were safe and efficacious for further investigation.
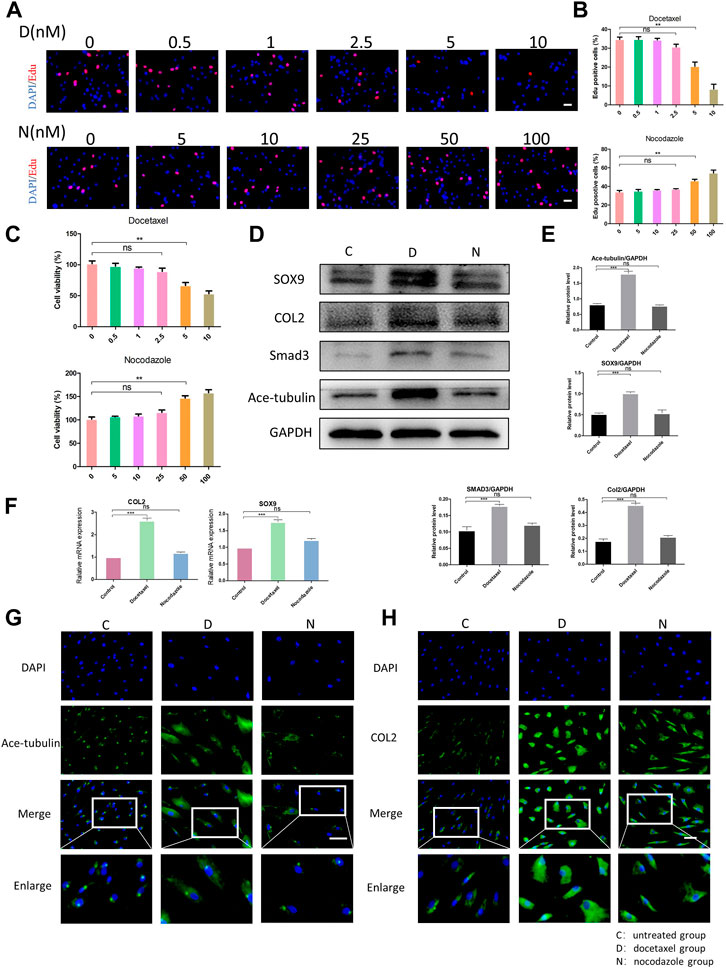
FIGURE 2. The effect of microtubule (MT) stabilization in the synthesis of COL2 in NP cells. (A) The EdU staining of degenerated NP cells after the treatment of different concentrations of docetaxel (D) (0.5, 1, 2.5, 5, 10 nM) and nocodazole (N) (5, 10, 25, 50, 100 nM) for 1 week. Scale bar, 100 µm. (B) Quantification of the data of (A). n = 4. (C) Cell Counting Kit-8 Assay is used to detect cell viability. The cell viability of NP cells after the treatment of different concentrations of docetaxel (0.5, 1, 2.5, 5, 10 nM) and nocodazole (5, 10, 25, 50, 100 nM) for 1 week. n = 4. (D) Western blot analysis of Ace-Tubulin, COL2, SOX9, and SMAD3 expression in NP cells treated with docetaxel and nocodazole for 1 week. (E) Quantification of the data of (D). n = 4. (F) Quantitative real time polymerase chain reaction (Q-PCR) of COL2 and SOX9 expression in NPCs treated with docetaxel and nocodazole for 1 week. (G, H) Cell immunofluorescence staining of Ace-Tubulin and COL2 expression in NP cells treated with docetaxel and nocodazole for 1 week. Scale bar, 100 µm. Data are represented as the mean ± SEM. *p < 0.05, **p < 0.01, ***p < 0.001.
After treating NP cells with docetaxel and nocodazole for 1 week, the expression of Ace-tubulin was enhanced in NP cells treated with docetaxel compared to the untreated group, but no difference was found in NP cells treated with nocodazole, according to the results of Western blot and cell immunofluorescence (Figures 2D, E, G). As the MT stability increased using docetaxel, the results of Western blot, Q-PCR and cell immunofluorescence showed upregulated expression of COL2 (Figures 2D, F, H). Moreover, as the upstream regulators of COL2, the expression of SOX9 and Smad3 were also upregulated as microtubules stabilization increased (Figures 2D, E). As shown above, MT stabilization could promote the expression of COL2 in NP cells.
MT stabilization inhibits the expression and activity of YAP/TAZ via hippo pathway
YAP/TAZ plays a critical role in response to mechanical stress, perturbation of the cytoskeleton and matrix remodeling (Calvo et al., 2013). YAP/TAZ nuclear accumulation is a key determinant of their function by inducing gene expression through interactions with partner proteins; while YAP/TAZ could also be phosphorylated and inactivated, leading to their cytoplasmic retention and degradation. We investigated the expression and phosphorylation level of YAP/TAZ in NP cells to determine whether MT stabilization was involved in the expression and activity of YAP. The Western blot analysis showed that both total YAP and phosphorylated YAP were significantly decreased in NP cell after treatment of docetaxel, which was confirmed by Q-PCR (Figures 3A–C). Moreover, the proportion of phosphorylated YAP in total YAP ascended after treatment of docetaxel compared to the untreated group (Figures 3A, B), indicating that MT stabilization not only inhibits the expression of YAP, but promoting phosphorylation of YAP. MT destabilization by nocodazole treatment had no significant effect on total YAP and phosphorylated YAP in NP cells. The expression of TAZ decreased in docetaxel group and unchanged in nocodazole group, which was similar to that of YAP. The immunofluorescence results also confirmed that the docetaxel treatment significantly decreased total YAP, while no noteworthy change in YAP was observed after nocodazole treatment (Figure 3D). The above results showed that MT stabilization inhibited the expression and activity of YAP/TAZ in nucleus pulposus cells.
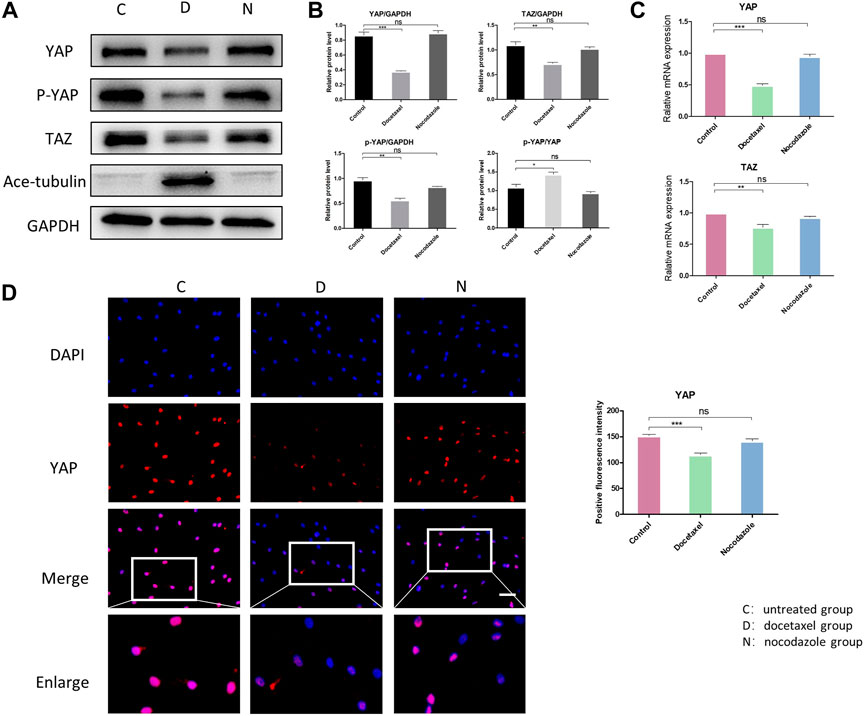
FIGURE 3. The effect of MT stabilization on YAP expression in NP cells. (A) Western blot analysis of YAP expression in NPCs treated with docetaxel and nocodazole for 1 week. (B) Quantification of the data of (A). n = 4. (C) Q-PCR of YAP and TAZ expression in NP cells treated with docetaxel and nocodazole for 1 week. (D) Cell immunofluorescence staining of YAP expression in NP cells treated with docetaxel and nocodazole for 1 week. Scale bar, 100 µm. Data are represented as the mean ± SEM. *p < 0.05, **p < 0.01, ***p < 0.001.
Hippo pathway regulates YAP/TAZ primarily through LATS kinases (LATS1 and LATS2)-mediated phosphorylation. Moreover, Hippo pathway is regulated by actin cytoskeletal factors, including capping proteins and cofilin (Gaspar and Tapon, 2014; Meng et al., 2016). A recent study also suggested that Patronin, a microtubule stabilizer, interacted with Hippo signaling in Drosophila (Yang and Choi, 2021). Therefore, we further investigated that whether the inhibition of YAP/TAZ by MT stabilization was mediated by Hippo pathway in NP cells. Our results in NP cells revealed that the docetaxel treatment significantly increased the expression of LATS1 and LATS2 (Figures 4A–C), which was consistent with previous findings that the components of the Hippo pathway promoted ciliogenesis and MT stabilization (Kim et al., 2014). In addition, the expression of LATS1/2 was not affected when NP cells were treated with nocodazole. Meanwhile, the enhanced expression of Ace-tubulin and inhibited expression of YAP was also found after docetaxel treatment by Western blot analysis. The above results illustrated that MT stabilization inhibited the expression and activity of YAP/TAZ via regulating Hippo pathway.
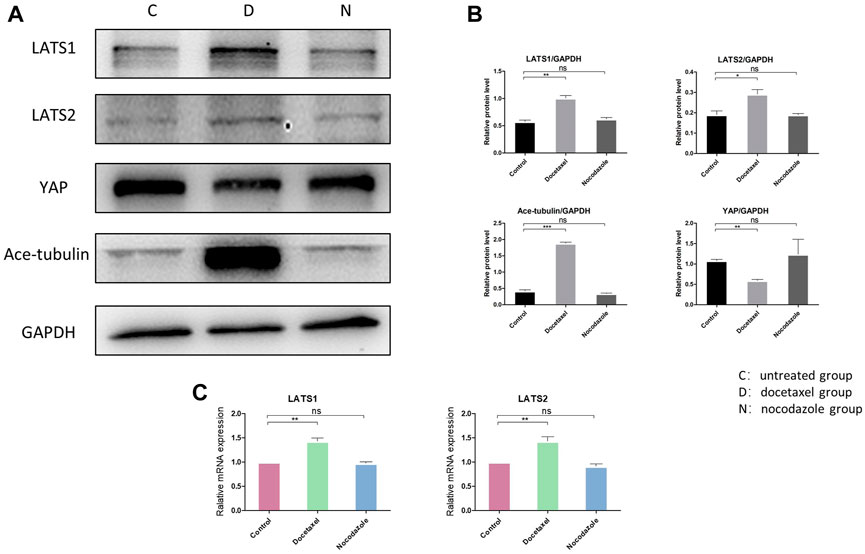
FIGURE 4. The effect of MT stabilization on Hippo pathway of NP cells. (A) Western blot analysis of LATS1, LATS2 and Ace-tubulin expression in NP cells treated with docetaxel and nocodazole for 1 week. (B) Quantification of the data of (A). n = 4. (C) Q-PCR of LATS1 and LATS2 expression in NP cells treated with docetaxel and nocodazole for 1 week. Data are represented as the mean ± SEM. *p < 0.05, **p < 0.01, ***p < 0.001.
YAP mediates MT stabilization to promote the synthesis of COL2
To clarify whether YAP plays a role in the up-regulated COL2 expression promoted by MT stabilization, YAP was knocked down by small interfering RNA (siRNA). As the total and phosphorylated YAP both decreased following YAP siRNA transfection, the nucleus pulposus related proteins, including COL2, SOX9 and Smad3, were all upregulated with and without docetaxel treatment; while the expression of LATS1, LATS2 and Ace-tubulin were not significantly altered (Figures 5A, B). Moreover, Lysophosphatidic acid (LPA) was utilized to promote the nucleus re-localization of YAP in the NP cells, which would up-regulate the activity of YAP (Yu et al., 2012). The results revealed that the increased expression of COL2, SOX9 and Smad3 by docetaxel were diminished with concurrent treatment of LPA, while the LPA treatment alone to the NP cells also significantly decreased the expression of COL2, SOX9 and Smad3 (Figures 6A–C). Therefore, the synthesis effect of COL2 induced by MT stabilization was via the regulation on YAP.
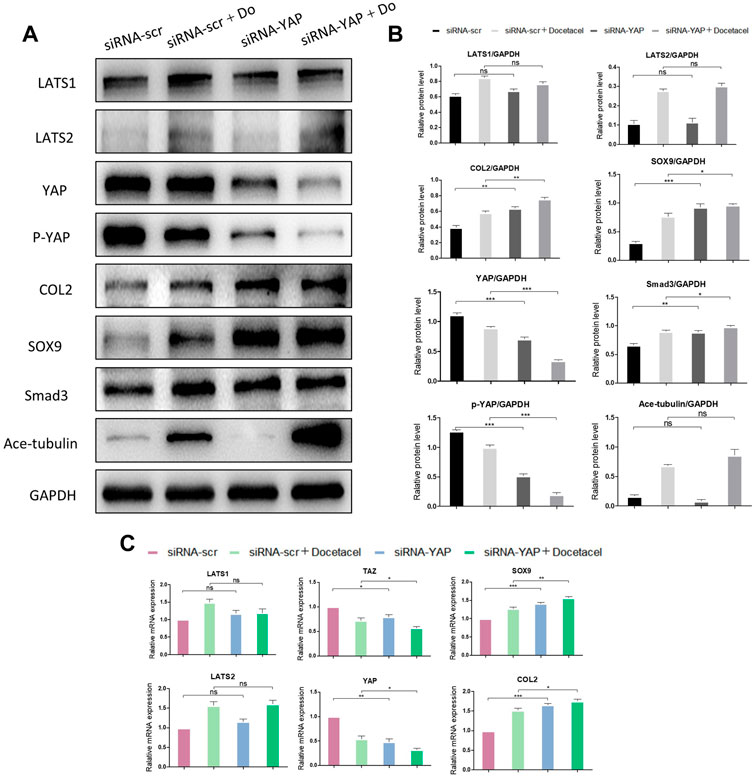
FIGURE 5. Knockdown of YAP promotes the synthesis of COL2 in NP cells. (A) Western blot analysis of Ace-Tubulin, phosphorylated YAP, YAP, LATS1, LATS2, COL2, Smad3 and SOX9 in NP cells transfected with siRNA-YAP and treated with docetaxel for 1 week. (B) Quantification of the data of (A). n = 4. (C) Q-PCR of YAP, TAZ, LATS1, LATS2, COL2, and SOX9 in NP cells transfected with siRNA-YAP and treated with docetaxel for 1 week. Data are represented as the mean ± SEM. *p < 0.05, **p < 0.01, ***p < 0.001.
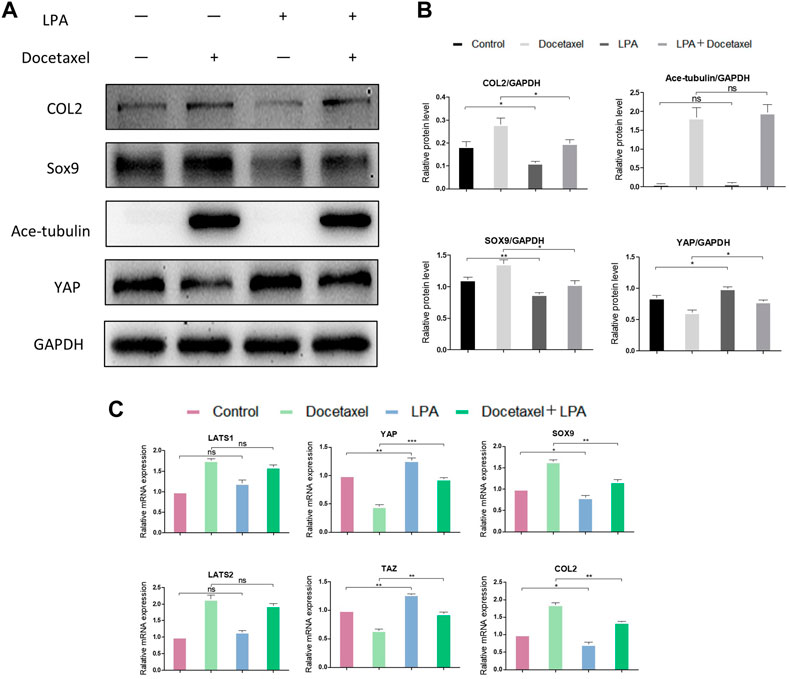
FIGURE 6. The effect of YAP activation on the synthesis of COL2 in NP cells. (A) Western blot analysis of Ace-Tubulin, YAP, COL2 and SOX9 in NP cells treated with lysophosphatidic acid (LPA) and treated with docetaxel for 1 week. (B) Quantification of the data of (A). n = 4. (C) Q-PCR of LATS1, LATS2, TAZ, YAP, COL2 and SOX9 in NP cells treated with LPA and treated with docetaxel for 1 week. Data are represented as the mean ± SEM. *p < 0.05, **p < 0.01, ***p < 0.001.
MT stabilization alleviate IDD in vivo
To further investigate the therapeutic effects of MT stabilization on IDD, we used a classic rat caudal intervertebral disc acupuncture animal model for this study. PBS, nocodazole and docetaxel were injected into three consecutive independent intervertebral discs of each rat 4 weeks after acupuncture. MRI and microCT examinations of rat IVD were performed at 4 weeks after the modeling and 4 weeks after the treatment to determine the degree of degeneration. A percent disc height index (%DHI) was calculated based on the microCT results. With a low percent DHI in all rats 4 weeks after needle puncture, the intervertebral disc showed intervertebral space stenosis or collapse, indicating degenerative changes of IVD (Figures 7A, B). After 4 weeks of treatment with docetaxel and nocodazole, DHI in docetaxel group recovered significantly, while DHI in control group and nocodazole group did not increase significantly (Figure 7A). In addition, the Pfirrmann score was derived from MRI results and used to assess the degree of IDD. Four weeks after needle puncture, the Pfirrmann scores of IVD in all rats showed no significant difference. After 4 weeks of treatment with docetaxel and nocazole, Pfirrmann scores were significantly lower in the docetaxel group compared to the PBS group and the nocodazole group. Intervertebral discs were collected for HE staining, Masson staining, and COL2 immunohistochemical staining after treating rats for 4 weeks. The results showed that the Docetaxel group had a significantly better degenerative score than the PBS group and the Nocodazole group, and the expression of COL2 was also significantly increased compared with PBS group and Nocodazole group (Figure 7C).
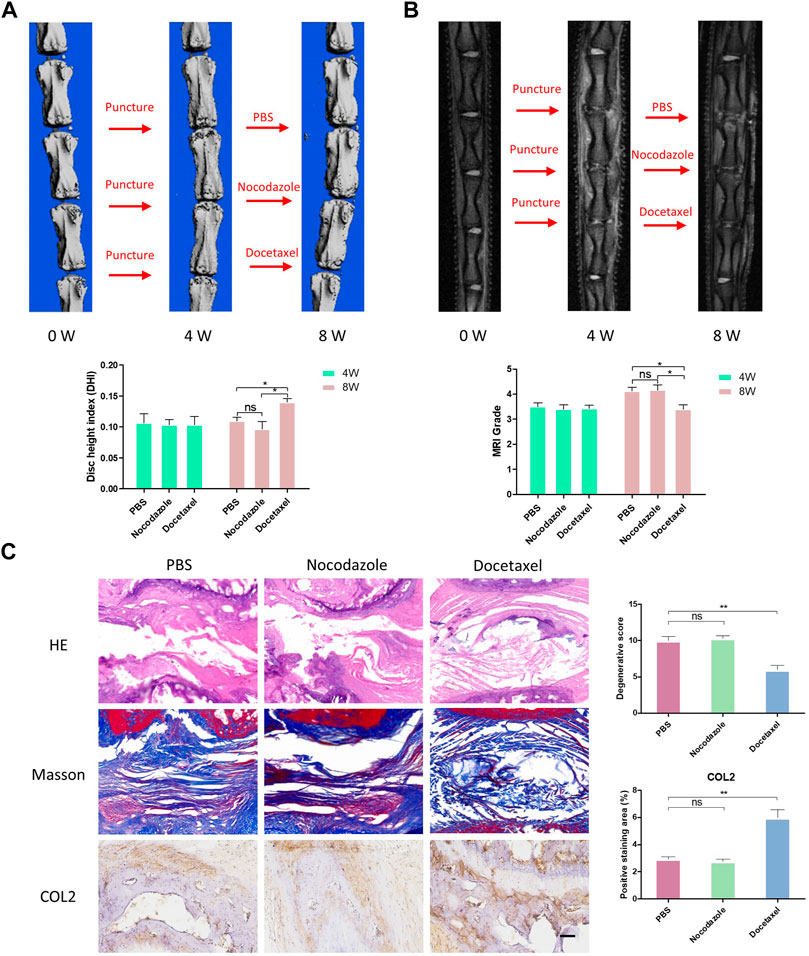
FIGURE 7. MT stabilization alleviates intervertebral disc degeneration (IDD) by promoting the synthesis of COL2 in vivo. (A) After 4 weeks of acupuncture and 4 weeks of treatment with PBS, nocodazole, and docetaxel, 3D micro-CT reconstruction and disc height of rat caudal IVD were collected and measured. (B) After 4 weeks of acupuncture and 4 weeks of treatment with PBS, Nokotazol and docetaxel, MRI and disc degenerative degree (low signal ratio in disc) of rat caudal IVD were collected and measured. (C) HE staining, Masson staining and COL2 immunohistochemistry staining of rat caudal IVD after treatment with PBS, Nocodazole and Docetaxel for 4 weeks. Data are represented as the mean ± SEM. *p < 0.05, **p < 0.01, ***p < 0.001.
Discussion
Microtubules outline the overall shape of cells by serving as the supports for morphologies such as axons and cilia (Goodson and Jonasson, 2018). Microtubules could also feel tension at the cell membrane, where they are sometimes coupled to motor proteins (Ligon et al., 2001), but the role of microtubule on mechanical force related degenerative diseases still remains unclear. In this study, we demonstrated that MT stabilization promoted the expression of Col2 and Sox9 in nucleus pulposus cells by stimulating Hippo pathway and inhibiting YAP expression and activity. MT stabilization promoted the expression of Col2 and alleviates disc degeneration in vivo in rats (Figure 8).
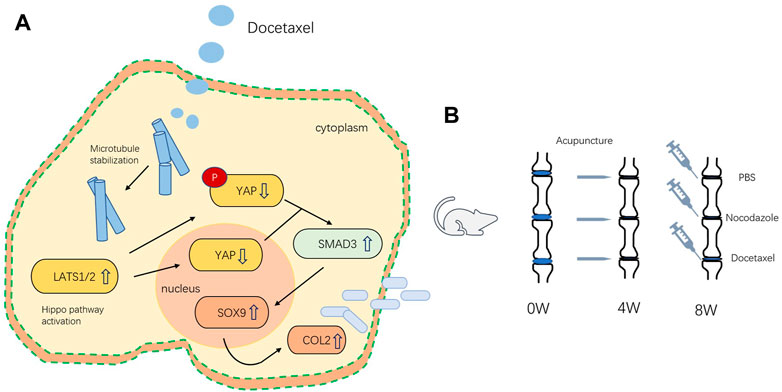
FIGURE 8. (A) Schematic of the mechanism by which microtubule stabilization promotes the synthesis of COL2 in NP Cell. (B) Schematic illustration of rat intervertebral disc modeling and treatment.
MT stabilization has been reported to have a significant effect on promoting the chondrogenic differentiation of Synovial mesenchymal stem cells derived from human osteoarthritis synovium (Li et al., 2021). However, few study investigated the relationship between microtubule and IDD. For the first time, we found that microtubule stabilization could promote the expression of chondrogenic makers, Col2 and Sox9, suggesting that MT stabilization would be a promising strategy for alleviating IDD. The length of primary cilia were found to decrease significantly both in annulus fibrosus cells and NP cells during IDD (Li et al., 2020), suggesting the potential impact of microtubule on IDD. Primary cilia are highly conserved microtubule-based organelles that project from the cell surface into the extracellular environment. Studies from bone and cartilage have demonstrated that primary cilia play critical roles during cell mechano-sensation and mechano-transduction (Goetz and Anderson, 2010; Ruhlen and Marberry, 2014). Li et al. (2020) further proved that cilia loss not only caused cell apoptosis in NP cells, but also lead to decreased Col1α1 and Col2α1 levels and increased MMP3 levels, suggesting that primary cilia are critical for the regulation of disc degeneration. Since primary cilia are microtubule-based organelles, the previous studies supported our results that MT stabilization would being positive benefit to the intervertebral disc.
Similar to the degeneration of intervertebral disc, MT destabilization has been reported to be one of the possible pathology of neurodegeneration, leading to Parkinson’s Disease (Cartelli and Cappelletti, 2017); on the other hand, MT stabilization can block degeneration of axon and even enable axon regrowth, making the MT-based therapy a promising option for Parkinson’s Disease (Baas and Ahmad, 2013). The use of MT stabilizer, Paclitaxel, has showed a beneficial effect by rescuing the PARK2 mutation-derived alterations of MT assembly in human neurons (Ren et al., 2009). In this study, we chose docetaxel as the MT stabilizer, which shared a similar mechanism with paclitaxel: the promotion of microtubule assembly and inhibition of microtubule disassembly. Our rat IDD model also proved the ability of MT stabilizer in blocking the disc degeneration and in promoting the expression of Col2. However, the benefits do not come without potential risks; since MTs need to be remodeled during the entire lifespan, MT stabilizers would block the dynamic behavior of MT and therefore affect MT-dependent functions in many aspects. Moreover, considering the difficulty of docetaxel delivery to intervertebral disc, the use of MT-interacting peptides (Fleming et al., 2011), which would modulate MT assembly while having better chances not to cause side effects, could be a helpful tool for physicians and researchers.
Acetylated microtubules have been considered to be stable, long-lived microtubules (Szyk et al., 2014). The traditionally prevailing model is that tubulin acetylation is a consequence of microtubule stabilization, however, in the living cells, the mechanical force could make acetylated microtubules more long-lived. At the same time, longer-lived microtubules are still more likely to experience mechanical stress, thus further accumulating acetylation marks (Janke and Montagnac, 2017). It’s very interesting that the MT depolymerizer, nocodazole, showed no significant effect on the expression of Ace-tubulin, YAP/TAZ and Col2 according to our data. Nocodazole is a small molecule that binds soluble tubulin and prevents its polymerization, working as a microtubule-destabilizing agent (Gudimchuk and McIntosh, 2021). Recently, Xu et al. (2017) found that after treating cells with nocodazole, dynamic microtubules were depolymerized, and most remaining microtubules displayed the typical characteristics of long-lived microtubules with high levels of acetylation, they named those long-lived microtubules as nocodazole-resistant microtubules. The data from Xu et al. (2017)’s study proved that nocodazole could depolymerize dynamic microtubules but the remining long-lived microtubules were still highly bent, acetylated, and detyrosinated and microtubule number was similar in control cells compared to the nocodazole treated cells. Xu’s study supported our finding that nocodazole does not necessarily decrease the expression of Ace-tubulin in degenerated NP cells. It could possibly attribute to the long-lived nature of microtubules in degenerated NP cells since the degenerated NP cells suffers more mechanical force. Similarly, since the long-lived microtubules was difficult to be depolymerized by nocodazole, the nocodazole treatment may not change the YAP/TAZ expression. Our data is supported by previous report that disruption of microtubules with nocodazole did not alter YAP/TAZ localization (Dupont et al., 2011).
YAP activity and subcellular localization are influenced by changes in the cytoskeleton and in cell shape (Gao et al., 2017). In NP cells, specifically, Zhang et al. (2021) demonstrated Hippo pathway was suppressed in the early stages of disc injury, and that YAP and F-actin activity decreased gradually with age in natural IDD. Our results revealed that the stabilization of MT, another component of cytoskeleton, would lead to the decreased expression of YAP and the increased proportion of YAP phosphorylation, which represented the decreased activity of YAP. YAP levels in NP tissue has been reported decreased gradually with time, suggesting that they are closely related to the development of IDD (Ding et al., 2022). In addition, consistent with our findings, Li et al. reported that MT stabilization inhibited the expression of YAP in Synovial mesenchymal stem cells to drive chondrogenesis. The precise mechanisms that how MT stabilization could suppress the activity of YAP has not been illustrated. Actin stress fibres are reported to be required for YAP/TAZ activity (Dupont et al., 2011), while Enomoto (1996) confirmed that taxol, a MT stabilization drug, could suppress the formation of actin stress fibres. Moreover, YAP activity is inhibited upon mechanical stress and microtubules are stabilized under tension (Dupont et al., 2011; Hamant et al., 2019). Taken together, MT stabilization may inhibit YAP/TAZ activity by interactions between cytoskeleton components.
The Hippo pathway controls the nuclear localization and stability of YAP/TAZ, playing important roles in maintaining homeostasis and tissue regeneration. Studies have shown that the activity of cytoskeleton are involved in the regulation of Hippo signaling pathways (Zhang et al., 2021). In our study, YAP de-activation was accompanied by upregulation of LATS1/2, indicating that Hippo signaling pathway acted as the upstream negative regulator of YAP/TAZ in NP cells when microtubule stabilization was promoted. Researches have provided a variety of mechanisms that regulating YAP/TAZ, including classic Hippo signaling pathway, ROCK signaling, TGF-β signaling, Snail/Slug binding interactions and Hedgehog signaling pathway. In the classic Hippo pathway, LATS1/2 phosphorylate the YAP/TAZ, which results in their cytoplasmic retention and degradation in the proteasome. In contrast, Zhang et al. reported that YAP activation was accompanied by upregulation of LATS1 in young rats intervertebral discs, they explained this finding as the YAP-LAST negative feedback loop which could limit the hyperactivity of YAP, but their conclusion may need further validation (Zhang et al., 2018).
In this study, the activity of YAP could regulate the synthesis of Col2, which serves as a marker of disc regeneration. For the first time, we observed that the YAP agonist (LPA) could suppress the synthesis of Col2 while YAP knockdown promoted synthesis in NP cells. Increased YAP expression levels has been reported to increase osteogenesis and decrease adipogenesis of mesenchymal stem cells and initiate dedifferentiation of chondrocytes (Zhong et al., 2013), and thus the decreased YAP activity may indicate chondrogenesis. Moreover, Zhong et al. treated mesenchymal stem cells and chondrocytes with Cytochalasin D, they found that Cytochalasin D could repressed nuclear YAP accumulation and thus result in MSC adipogenesis and the maintenance of the chondrocyte phenotype. The regulation of YAP on Col2 may rely on Smad3. Wei et al. inhibited Smad in degenerated NP cells and found the Smad inhibitor could abolish the effect of YAP. Our data also demonstrated increased Smad3 expression in conditions of both MT stabilization and YAP knockdown, and Smad3 has been reported as a key regulator of Col2 (Chen et al., 2012). In addition, in our in vivo study, the recovery of disc height, the improvement of MRI signal and the up-regulated Col2 expression after the injection of docetaxel into the caudal disc of rats demonstrated the therapeutic potential that targeting microtubule.
It is very interesting that we observed increased ace-tubulin in degenerated NP tissues derived from patients with IDD, which has not been reported in previous studies. The acetylation of tubulin is a consequence of microtubule stabilization since artificially chemical stabilization of microtubules leads to acetylation of tubulin (Palazzo et al., 2003). The mechanical force exposed to the cells could lead to depolymerization of microtubule, while acetylation-induced increase in flexibility would allow microtubules to better resist mechanical force, serving as a self-repair mechanism and making the acetylated microtubules more long-lived (Xu et al., 2017). Moreover, longer-lived microtubules are more likely to experience mechanical stress, further accumulating Ace-tubulin (Schaedel et al., 2015). Thus, the increased Ace-tubulin in degenerated NP tissues is probably due to the mechanical stress on intervertebral discs, and the MT stabilization may participate in a “self-regeneration” mechanisms during IDD since MT stabilization could promote synthesis of Col2.
In summary, our study for the first time, identifies microtubule stabilization as a promising therapeutic target for IDD, up-regulating the synthesis of Col2. Moreover, Hippo-Yap pathway participates in the regulation of Col2 in the context of stabilized microtubule. Our findings may provide new insights into the etiologies and pathology for IDD, further, targeting of microtubule acetylation may be an effective strategy for the treatment of IDD.
Data availability statement
The raw data supporting the conclusion of this article will be made available by the authors, without undue reservation.
Ethics statement
The studies involving human participants were reviewed and approved by The Ethics Committee of Nanjing Drum Tower Hospital, the Affiliated Hospital of Nanjing University Medical School. The patients/participants provided their written informed consent to participate in this study. The animal study was reviewed and approved by The Ethics Committee of Nanjing Drum Tower Hospital, the Affiliated Hospital of Nanjing University Medical School. Written informed consent was obtained from the individual(s) for the publication of any potentially identifiable images or data included in this article.
Author contributions
All authors contributed to the material preparation, data collection and analysis. The study conception and design were performed by XZ and HB. The experimental part of the study was mainly completed by XZ and cooperated by SS. The first draft of the manuscript was written by XZ and HB. All authors commented on previous versions of the manuscript. All authors read and approved the final manuscript.
Funding
This work was financially Supported by Jiangsu Provincial Key Medical Center (Project’s number: YXZXA2016009)
Conflict of interest
The authors declare that the research was conducted in the absence of any commercial or financial relationships that could be construed as a potential conflict of interest.
Publisher’s note
All claims expressed in this article are solely those of the authors and do not necessarily represent those of their affiliated organizations, or those of the publisher, the editors and the reviewers. Any product that may be evaluated in this article, or claim that may be made by its manufacturer, is not guaranteed or endorsed by the publisher.
Supplementary material
The Supplementary Material for this article can be found online at: https://www.frontiersin.org/articles/10.3389/fphar.2023.1102318/full#supplementary-material
References
Baas, P. W., and Ahmad, F. J. (2013). Beyond taxol: Microtubule-based treatment of disease and injury of the nervous system. Brain 136, 2937–2951. doi:10.1093/brain/awt153
Benjamin, M., Archer, C. W., and Ralphs, J. R. (1994). Cytoskeleton of cartilage cells. Microsc. Res. Tech. 28, 372–377. doi:10.1002/jemt.1070280503
Brangwynne, C. P., MacKintosh, F. C., Kumar, S., Geisse, N. A., Talbot, J., Mahadevan, L., et al. (2006). Microtubules can bear enhanced compressive loads in living cells because of lateral reinforcement. J. Cell Biol. 173, 733–741. doi:10.1083/jcb.200601060
Calvo, F., Ege, N., Grande-Garcia, A., Hooper, S., Jenkins, R. P., Chaudhry, S. I., et al. (2013). Mechanotransduction and YAP-dependent matrix remodelling is required for the generation and maintenance of cancer-associated fibroblasts. Nat. Cell Biol. 15, 637–646. doi:10.1038/ncb2756
Cartelli, D., and Cappelletti, G. (2017). Microtubule destabilization paves the way to Parkinson's disease. Mol. Neurobiol. 54, 6762–6774. doi:10.1007/s12035-016-0188-5
Chen, C. G., Thuillier, D., Chin, E. N., and Alliston, T. (2012). Chondrocyte-intrinsic Smad3 represses Runx2-inducible matrix metalloproteinase 13 expression to maintain articular cartilage and prevent osteoarthritis. Arthritis Rheum. 64, 3278–3289. doi:10.1002/art.34566
Chen, J., Yan, W., and Setton, L. A. (2004). Static compression induces zonal-specific changes in gene expression for extracellular matrix and cytoskeletal proteins in intervertebral disc cells in vitro. Matrix Biol. 22, 573–583. doi:10.1016/j.matbio.2003.11.008
Ding, B., Xiao, L., and Xu, H. (2022). YAP1 controls degeneration of human cartilage chondrocytes in response to mechanical tension. Cell Biol. Int. 46, 1637–1648. doi:10.1002/cbin.11851
Dupont, S., Morsut, L., Aragona, M., Enzo, E., Giulitti, S., Cordenonsi, M., et al. (2011). Role of YAP/TAZ in mechanotransduction. Nature 474, 179–183. doi:10.1038/nature10137
Enomoto, T. (1996). Microtubule disruption induces the formation of actin stress fibers and focal adhesions in cultured cells: Possible involvement of the rho signal cascade. Cell Struct. Funct. 21, 317–326. doi:10.1247/csf.21.317
Fleming, S. M., Mulligan, C. K., Richter, F., Mortazavi, F., Lemesre, V., Frias, C., et al. (2011). A pilot trial of the microtubule-interacting peptide (NAP) in mice overexpressing alpha-synuclein shows improvement in motor function and reduction of alpha-synuclein inclusions. Mol. Cell Neurosci. 46, 597–606. doi:10.1016/j.mcn.2010.12.011
Gao, J., He, L., Shi, Y., Cai, M., Xu, H., Jiang, J., et al. (2017). Cell contact and pressure control of YAP localization and clustering revealed by super-resolution imaging. Nanoscale 9, 16993–17003. doi:10.1039/c7nr05818g
Gaspar, P., and Tapon, N. (2014). Sensing the local environment: Actin architecture and hippo signalling. Curr. Opin. Cell Biol. 31, 74–83. doi:10.1016/j.ceb.2014.09.003
Goetz, S. C., and Anderson, K. V. (2010). The primary cilium: A signalling centre during vertebrate development. Nat. Rev. Genet. 11, 331–344. doi:10.1038/nrg2774
Goodson, H. V., and Jonasson, E. M. (2018). Microtubules and microtubule-associated proteins. Cold Spring Harb. Perspect. Biol. 10, a022608. doi:10.1101/cshperspect.a022608
Gudimchuk, N. B., and McIntosh, J. R. (2021). Regulation of microtubule dynamics, mechanics and function through the growing tip. Nat. Rev. Mol. Cell Biol. 22, 777–795. doi:10.1038/s41580-021-00399-x
Hamant, O., Inoue, D., Bouchez, D., Dumais, J., and Mjolsness, E. (2019). Are microtubules tension sensors? Nat. Commun. 10, 2360. doi:10.1038/s41467-019-10207-y
Han, B., Zhu, K., Li, F. C., Xiao, Y. X., Feng, J., Shi, Z. L., et al. (2008). A simple disc degeneration model induced by percutaneous needle puncture in the rat tail. Spine (Phila Pa 1976) 33, 1925–1934. doi:10.1097/BRS.0b013e31817c64a9
Hayes, A. J., Benjamin, M., and Ralphs, J. R. (1999). Role of actin stress fibres in the development of the intervertebral disc: Cytoskeletal control of extracellular matrix assembly. Dev. Dyn. 215, 179179–179189. doi:10.1002/(SICI)1097-0177(199907)215:3<179::AID-AJA1>3.0.CO;2-Q
Janke, C., and Montagnac, G. (2017). Causes and consequences of microtubule acetylation. Curr. Biol. 27, R1287–r1292. doi:10.1016/j.cub.2017.10.044
Kim, M., Kim, M., Lee, M. S., Kim, C. H., and Lim, D. S. (2014). The MST1/2-SAV1 complex of the Hippo pathway promotes ciliogenesis. Nat. Commun. 5, 5370. doi:10.1038/ncomms6370
Li, J., Sun, Z., Lv, Z., Jiang, H., Liu, A., Wang, M., et al. (2021). Microtubule stabilization enhances the chondrogenesis of synovial mesenchymal stem cells. Front. Cell Dev. Biol. 9, 748804. doi:10.3389/fcell.2021.748804
Li, X., Yang, S., Han, L., Mao, K., and Yang, S. (2020). Ciliary IFT80 is essential for intervertebral disc development and maintenance. Faseb J. 34, 6741–6756. doi:10.1096/fj.201902838R
Ligon, L. A., Karki, S., Tokito, M., and Holzbaur, E. L. (2001). Dynein binds to beta-catenin and may tether microtubules at adherens junctions. Nat. Cell Biol. 3, 913–917. doi:10.1038/ncb1001-913
Meng, Z., Moroishi, T., and Guan, K. L. (2016). Mechanisms of Hippo pathway regulation. Genes Dev. 30, 1–17. doi:10.1101/gad.274027.115
Palazzo, A., Ackerman, B., and Gundersen, G. G. (2003). Cell biology: Tubulin acetylation and cell motility. Nature 421, 230. doi:10.1038/421230a
Paul, C. P., Schoorl, T., Zuiderbaan, H. A., Zandieh Doulabi, B., van der Veen, A. J., van de Ven, P. M., et al. (2013). Dynamic and static overloading induce early degenerative processes in caprine lumbar intervertebral discs. PLoS One 8, e62411. doi:10.1371/journal.pone.0062411
Paul, C. P., Zuiderbaan, H. A., Zandieh Doulabi, B., van der Veen, A. J., van de Ven, P. M., Smit, T. H., et al. (2012). Simulated-physiological loading conditions preserve biological and mechanical properties of caprine lumbar intervertebral discs in ex vivo culture. PLoS One 7, e33147. doi:10.1371/journal.pone.0033147
Priyadarshani, P., Li, Y., and Yao, L. (2016). Advances in biological therapy for nucleus pulposus regeneration. Osteoarthr. Cartil. 24, 206–212. doi:10.1016/j.joca.2015.08.014
Ren, Y., Jiang, H., Yang, F., Nakaso, K., and Feng, J. (2009). Parkin protects dopaminergic neurons against microtubule-depolymerizing toxins by attenuating microtubule-associated protein kinase activation. J. Biol. Chem. 284, 4009–4017. doi:10.1074/jbc.M806245200
Ruhlen, R., and Marberry, K. (2014). The chondrocyte primary cilium. Osteoarthr. Cartil. 22, 1071–1076. doi:10.1016/j.joca.2014.05.011
Schaedel, L., John, K., Gaillard, J., Nachury, M. V., Blanchoin, L., and Théry, M. (2015). Microtubules self-repair in response to mechanical stress. Nat. Mater 14, 1156–1163. doi:10.1038/nmat4396
Song, Y., Li, S., Geng, W., Luo, R., Liu, W., Tu, J., et al. (2018). Sirtuin 3-dependent mitochondrial redox homeostasis protects against AGEs-induced intervertebral disc degeneration. Redox Biol 19, 339–353. doi:10.1016/j.redox.2018.09.006
Szyk, A., Deaconescu, A. M., Spector, J., Goodman, B., Valenstein, M. L., Ziolkowska, N. E., et al. (2014). Molecular basis for age-dependent microtubule acetylation by tubulin acetyltransferase. Cell 157, 1405–1415. doi:10.1016/j.cell.2014.03.061
Walter, B. A., Korecki, C. L., Purmessur, D., Roughley, P. J., Michalek, A. J., and Iatridis, J. C. (2011). Complex loading affects intervertebral disc mechanics and biology. Osteoarthr. Cartil. 19, 1011–1018. doi:10.1016/j.joca.2011.04.005
Wang, D. L., Jiang, S. D., and Dai, L. Y. (2007). Biologic response of the intervertebral disc to static and dynamic compression in vitro. Spine (Phila Pa 1976) 32, 2521–2528. doi:10.1097/BRS.0b013e318158cb61
Westermann, S., and Weber, K. (2003). Post-translational modifications regulate microtubule function. Nat. Rev. Mol. Cell Biol. 4, 938–947. doi:10.1038/nrm1260
Wu, X., Song, Y., Liu, W., Wang, K., Gao, Y., Li, S., et al. (2017). IAPP modulates cellular autophagy, apoptosis, and extracellular matrix metabolism in human intervertebral disc cells. Cell Death Discov. 3, 16107. doi:10.1038/cddiscovery.2016.107
Wuertz, K., Godburn, K., MacLean, J. J., Barbir, A., Donnelly, J. S., Roughley, P. J., et al. (2009). In vivo remodeling of intervertebral discs in response to short- and long-term dynamic compression. J. Orthop. Res. 27, 1235–1242. doi:10.1002/jor.20867
Xu, Z., Schaedel, L., Portran, D., Aguilar, A., Gaillard, J., Marinkovich, M. P., et al. (2017). Microtubules acquire resistance from mechanical breakage through intralumenal acetylation. Science 356, 328–332. doi:10.1126/science.aai8764
Yamada, K., Sudo, H., Iwasaki, K., Sasaki, N., Higashi, H., Kameda, Y., et al. (2014). Caspase 3 silencing inhibits biomechanical overload-induced intervertebral disk degeneration. Am. J. Pathol. 184, 753–764. doi:10.1016/j.ajpath.2013.11.010
Yang, D. W., and Choi, K. W. (2021). Suppression of Patronin deficiency by altered Hippo signaling in Drosophila organ development. Cell Death Differ. 28, 233–250. doi:10.1038/s41418-020-0597-x
Yu, F. X., Zhao, B., Panupinthu, N., Jewell, J. L., Lian, I., Wang, L. H., et al. (2012). Regulation of the Hippo-YAP pathway by G-protein-coupled receptor signaling. Cell 150, 780–791. doi:10.1016/j.cell.2012.06.037
Zhang, C., Wang, F., Xie, Z., Chen, L., Sinkemani, A., Yu, H., et al. (2018). Dysregulation of YAP by the Hippo pathway is involved in intervertebral disc degeneration, cell contact inhibition, and cell senescence. Oncotarget 9, 2175–2192. doi:10.18632/oncotarget.23299
Zhang, C., Wang, F., Xie, Z., Chen, L., and Wu, X. (2021). The hippo pathway orchestrates cytoskeletal organisation during intervertebral disc degeneration. Acta histochem. 123, 151770. doi:10.1016/j.acthis.2021.151770
Keywords: intervertebral disc degeneration, microtubule stabilization, mechanical stress, nucleus pulposus, intervertebral disc
Citation: Zhang X, Shu S, Feng Z, Qiu Y, Bao H and Zhu Z (2023) Microtubule stabilization promotes the synthesis of type 2 collagen in nucleus pulposus cell by activating hippo-yap pathway. Front. Pharmacol. 14:1102318. doi: 10.3389/fphar.2023.1102318
Received: 18 November 2022; Accepted: 18 January 2023;
Published: 26 January 2023.
Edited by:
Chen-he Zhou, Zhejiang University, ChinaReviewed by:
Feng-Juan Lyu, South China University of Technology, ChinaXuewen Kang, Lanzhou University Second Hospital, China
Yu Song, Huazhong University of Science and Technology, China
Copyright © 2023 Zhang, Shu, Feng, Qiu, Bao and Zhu. This is an open-access article distributed under the terms of the Creative Commons Attribution License (CC BY). The use, distribution or reproduction in other forums is permitted, provided the original author(s) and the copyright owner(s) are credited and that the original publication in this journal is cited, in accordance with accepted academic practice. No use, distribution or reproduction is permitted which does not comply with these terms.
*Correspondence: Hongda Bao, baohongda123@gmail.com; Zezhang Zhu, zhuzezhang@126.com