- Faculty of Medicine, University Center of Espirito Santo, Colatina, Brazil
Alzheimer’s disease (AD) is the most common type of dementia in the elderly. Several hypotheses emerged from AD pathophysiological mechanisms. However, no neuronal protective or regenerative drug is available nowadays. Researchers still work in drug development and are finding new molecular targets to treat AD. Therefore, this study aimed to summarize main advances in AD pharmacological therapy. Clinical trials registered in the National Library of Medicine database were selected and analyzed accordingly to molecular targets, therapeutic effects, and safety profile. The most common outcome was the lack of efficacy. Only seven trials concluded that tested drugs were safe and induced any kind of therapeutic improvement. Three works showed therapeutic effects followed by toxicity. In addition to aducanumab recent FDA approval, antibodies against amyloid-β (Aβ) showed no noteworthy results. 5-HT6 antagonists, tau inhibitors and nicotinic agonists’ data were discouraging. However, anti-Aβ vaccine, BACE inhibitor and anti-neuroinflammation drugs showed promising results.
Introduction
It is estimated that 55 million people have dementia worldwide and, by 2050, this number may increase to 139 million due to population aging. In 2019, dementia global cost was estimated to be 1.3 trillion dollars and led to 1.6 million deaths (WHO, 2017; WHO, 2021; WHO, 2022). Alzheimer’s disease (AD) is the most common type of dementia in the elderly and affects mainly females. It was estimated that among AD diagnosis, 44% are 75- to 84-year-old patients and 38% are 85 years or older. Thus, AD is a social and economic global burden (Hebert et al., 2013). This neurodegenerative disease is related to loss of cognitive functions caused by several pathological pathways: amyloid-β (Aβ) deposition, hyperphosphorylated tau protein, cholinergic disorder, excessive glutamatergic stimulation, oxidative stress, and neuroinflammation (Hardy and Allsop, 1991; Gomez-Isla et al., 1997; Fiala et al., 2007; Holmes et al., 2009; Tolar et al., 2020). The first case was reported by Alois Alzheimer in 1906, and, despite all improvements in understanding AD pathogenesis, nowadays, therapies only help to manage some symptoms. AD lingers without a cure or strategy to mitigate its progression (Alzheimer, 1906; Alzheimer et al., 1995; Tolar et al., 2020).
AD diagnosis continues to be mainly based on clinical evaluation of cognitive and physical examination. However, pathological changes occur years before symptoms arise, and the earlier diagnosis may be accomplished by detecting molecular biomarkers (Aβ and tau) or cortical atrophy using magnetic resonance imaging. Despite all available technology, the greatest sensitivity and efficacy is found only in postmortem cerebral autopsy (Ranasinghe et al., 2021; Vaillant-Beuchot et al., 2021; Troutwine et al., 2022).
Current treatments available include use of cholinesterase inhibitors for patients with any stage of AD and memantine for people with moderate to severe dementia. Main drugs approved are rivastigmine, galantamine, memantine and donepezil. However, they only improve quality of life when prescribed at the appropriate time (Botchway et al., 2018; Scheltens et al., 2021). Two decades after memantine approval, the United States (US) Food and Drug Administration (FDA) approved aducanumab in 2021, the first monoclonal antibody anti-Aβ and the latest AD drug approved. In addition to the entire thrill, this new drug is expensive and there is some doubt related to its benefits (Mafi et al., 2022). Therefore, this study aimed to describe main advances in AD pharmacological therapy through an analysis of latest clinical trial results registered in the US National Institutes of Health (NIH), contributing to theoretical information for drug development pipelines and future clinical practice.
Potential targets for drug design
This work analyzed 43 AD new drug clinical trials registered in the National Library of Medicine database ClinicalTrials.gov funded by the NIH with data published in PubMed between 2015 and October 2022, using the following keywords: “Alzheimer’s disease” AND “drug.” Molecular target, therapeutic effect, safety profile and side effects were evaluated in each study (Table 1). Only new drugs’ clinical trials registered in the NIH were included. Exclusion criteria were as follows: drugs already approved by the FDA (even if it is in a new formulation or delivery system) and pharmacological strategies that aim to solve only non-cognitive or degenerative symptoms. Those works tested 27 new drugs and 23 different molecular targets. In summary, we evaluated three anti-Aβ therapeutic vaccines, five anti-Aβ antibodies, a tau aggregation inhibitor, three BACE-1 inhibitors, 2 5-HT6 receptor antagonists, 2 nicotinic receptors agonists, a muscarinic agonist, a glutaminyl cyclase inhibitor and 10 anti-neuroinflammation drugs (Figure 1). Pathways related to those drugs are better detailed in Supplementary Data S1.
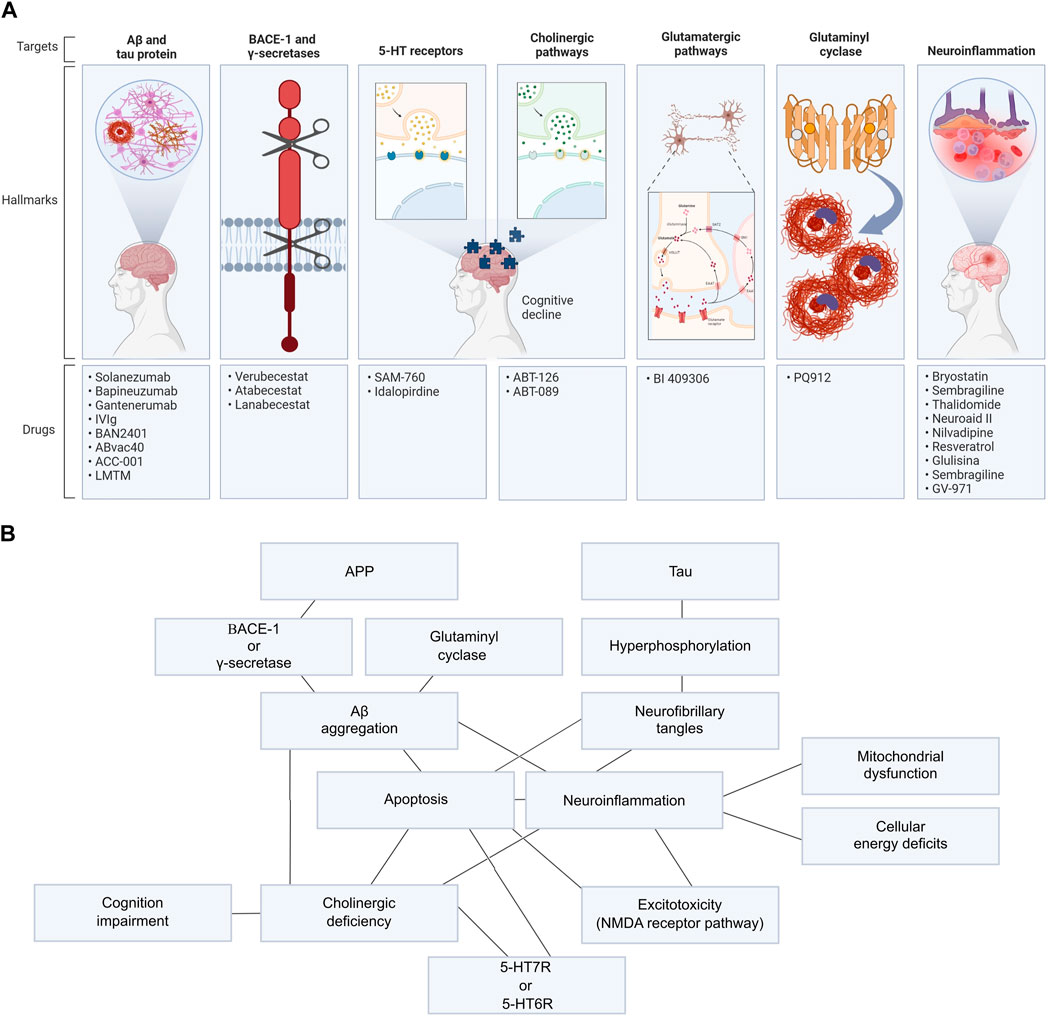
FIGURE 1. Main potential targets for drug design in AD and relation of its pathways. (A) AD’s targets used to develop new drugs in clinical trials evaluated in this work. (B) All mechanisms related to AD pathogenesis and progression are connected and were explored in these clinical trials, such as Aβ and tau aggregation; BACE-1, γ-secretase, or glutaminyl cyclase activity; neuroinflammation; excitotoxicity; 5-HT7R or 5-HT6R hyperstimulation and cholinergic impairment.
In summary, the main issue among failed trials was lack of efficiency (Butchart et al., 2015; Lenz et al., 2015; Brody et al., 2016; Florian et al., 2016; Gault et al., 2016; Gauthier et al., 2016; Ivanoiu et al., 2016; Pasquier et al., 2016; Van Dyck et al., 2016; Vandenberghe et al., 2016; Decourt et al., 2017; Ostrowitzki et al., 2017; Relkin et al., 2017; Atri et al., 2018; Egan et al., 2018; Fullerton et al., 2018; Honig et al., 2018; Lawlor et al., 2018; Liu-Seifert et al., 2018; Salloway et al., 2018; Wilcock et al., 2018; Frölich et al., 2019; Rosenbloom et al., 2021). Good outcomes were obtained in seven trials that induced any type of therapeutic improvement without toxicity (Turner et al., 2015; Logovinsky et al., 2016; Moussa et al., 2017; Nave et al., 2017; Sakamoto et al., 2017; Lacosta et al., 2018; Timmers et al., 2018; Xiao et al., 2021; Chen et al., 2022). Three works showed therapeutic effect followed by toxicity (Scheltens et al., 2018; Egan et al., 2019; Farlow et al., 2019).
Aβ and tau protein
Main therapeutic targets studied for AD were directly or indirectly related to neurofibrillary tangles (tau protein) and senile plaques (Aβ protein). Nevertheless, only aducanumab, an antibody anti-Aβ, was approved (Tolar et al., 2020; Mafi et al., 2022). Aβ and tau proteins highlight as drug targets and are related to AD pathogenesis. Amyloid precursor protein (APP) cleavage by β-secretases (BACE-1) or γ-secretases results in insoluble Aβ protein, a hallmark of AD. Therefore, the therapeutic rationale is to disassemble and degrade amyloid plaques chemically or by recruiting microglia and activating phagocytosis to stop or undo neuronal damage triggered by those protein accumulation (Da Mesquita et al., 2021; Vaillant-Beuchot et al., 2021; Troutwine et al., 2022). Active or passive immunotherapies are the most studied strategies due to their specific response, although they may induce autoantibodies, edema or hemorrhage (Geylis and Steinitz, 2006; Sengupta et al., 2016). Indeed, those following trials might have failed due to the timing of intervention. Earlier treatments could lead to better results (Botchway et al., 2018; Scheltens et al., 2021).
Solanezumab (Honig et al., 2018; Liu-Seifert, et al., 2018), bapineuzumab (Arai et al., 2016; Brody et al., 2016; Ivanoiu et al., 2016; Vandenberghe et al., 2016; Salloway et al., 2018a; Salloway et al., 2018b), BAN2401 (Logovinsky et al., 2016), and gantenerumab (Ostrowitzki et al., 2017) are monoclonal anti-Aβ antibodies. In addition to bapineuzumab phase 1 trial results of increasing plasma concentrations of β-amyloid1-40 in patients (Arai et al., 2016), all anti-Aβ antibodies failed in therapeutic efficiency, discouraging further studies. Another strategy was developed from natural anti-Aβ antibodies arising in the absence of vaccination or passive immunization in adults’ blood or AD patients. Relkin et al. (2017) evaluated intravenous immunoglobulins IgG-type (IVIg) derived from plasma of healthy donors to treat patients with mild to moderate AD. In addition to a good safety profile and decreasing plasma Aβ42, no clinical effect was observed.
On the other hand, clinical studies of vaccines were less encouraged due to the full-length Aβ1–42 peptide (AN1792) trial safety issues. This work demonstrated decrease in Aβ plaques, benefits on some cognitive and memory measures, and reductions in cerebrospinal fluid (CSF) tau. However, meningoencephalitis was presented in approximately 6% of AN1792-treated patients due to cytotoxic T-cell response (Nicoll et al., 2003; Ferrer et al., 2004; Masliah et al., 2005). Safety is no longer an issue in recent studies, which included vanutide cridificar (ACC-001), a vaccine designed to elicit antibodies against N-terminal peptide Aβ1-7. In this work, safety and tolerability profile was acceptable, but it lacks therapeutic effect (Pasquier et al., 2016; Van Dyck et al., 2016). ABvac40, a vaccine against the C-terminal end of Aβ40, as well showed safety and tolerability, but also, most of the individuals receiving ABvac40 developed specific anti-Aβ40 antibodies (Lacosta et al., 2018).
In addition to tau central role in AD pathogenesis, clinical trials focusing on this target are rare because of toxicity and/or lack of efficacy. Tau provides microtubule stability and contributes to the regulation of intracellular trafficking by its phosphorylation. Hyperphosphorylation of tau destabilizes tau–microtubule interactions, leading to instability, transport defects along microtubules and neuronal death (Dixit et al., 2008; Iqbal et al., 2010; Vershinin et al., 2017). Methylthioninium chloride (MTC; commonly known as “methylene blue”) was the first reported tau aggregation inhibitor (TAI) without disrupting normal tau–tubulin interactions (Melis et al., 2015). However, MTC had no therapeutic effect in phase 2 trial probably due to its poor tolerability and pharmacokinetic issues. MTC exists in equilibrium with its oxygen-sensitive redox couple, leucomethylthionium (LMTM) (Harrington et al., 2015). Although LMTM combines superior pharmacological properties with TAI activity than MTC, phase 3 trial showed no benefit to AD patients and led to treatment discontinuation (Gauthier et al., 2016; Wilcock et al., 2018).
BACE-1 and γ-secretases
Other important targets related to AD pathogenesis are BACE-1 and γ-secretases; once those enzyme activities were responsible for Aβ development, as described earlier in this work. However, disappointingly several inhibitors of those enzyme studies were discontinued due to futility and toxicity, including cognitive impairment. Severe toxicity was especially high in γ-secretase inhibitors, indicating that its inhibition cannot be achieved in a safe way due to its physiologic effect in the Notch pathway (Cebers et al., 2017; McDade et al., 2021; Yang et al., 2021).
Verubecestat, an oral BACE-1 inhibitor, had terrible clinical trial outcomes. Moreover, in phase 2, cognition and daily function decreased in patients using verubecestat and showed no therapeutic effect in phase 3 trial (Egan et al., 2018; Egan et al., 2019). On its turn, atabecestat is an oral BACE inhibitor capable of decreasing cerebrospinal fluid (CSF) Aβ1-40 with low toxicity (Timmers et al., 2018). Another oral BACE inhibitor is lanabecestat (AZD3293; LY3314814), and it decreased plasma and CSF Aβ (Sakamoto et al., 2017).
5-HT receptors
Lately, serotonin receptors emerged as targets in cognitive impairment and AD (Hung and Fu, 2017; Andrews et al., 2018; Kucwaj-Brysz et al., 2021; Solas et al., 2021; Higa et al., 2022). 5-HT6R and 5-HT7R are the most studied serotonin receptors in this scenario due to their brain distribution and noteworthy cognitive properties in vivo (Kucwaj-Brysz et al., 2021). Furthermore, 5-HT6R induces signaling that changes cholinergic, monoaminergic, and glutamatergic brain signaling with little periphery adverse effects (Andrews et al., 2018; Liu et al., 2019; Kucwaj-Brysz et al., 2021). However, no selective 5-HT6R or 5-HT7R drug successfully confirmed its therapeutic activity in clinical trials (Hung and Fu, 2017; Andrews et al., 2018; Kucwaj-Brysz et al., 2021). Main adverse effects related to those targets are decreased food intake and body weight (Andrews et al., 2018).
PF-05212377 (SAM-760) and idalopirdine are selective antagonists of 5-HT6R with a good safety profile; however, they failed to demonstrate efficacy. Altogether, those findings suggest that 5-HT6 antagonists should not be a main target to AD therapy (Atril et al., 2018; Fullerton et al., 2018).
Cholinergic pathways
According to the cholinergic hypothesis, AD is related to the reduction of acetylcholine. Therefore, most frequent pharmacologic therapy for AD is to increase cholinergic pathways through acetylcholinesterase inhibition (IAch) (Bartus et al., 1982; Recio-Barbero et al., 2021). IAch drugs, such as rivastigmine, donepezil, tacrine and galantamine, only provide a modest and not lingering symptomatic benefit to cognitive decline (Mohammad et al., 2017; Kumar et al., 2020; Sabandal et al., 2022). Most common side effects in cholinergic drugs are gastrointestinal issues, fatigue, cramps and sinus node dysfunction (Briggs et al., 2016; Mohammad et al., 2017).
A novel cholinergic approach for AD is the modulation of α7 nicotinic receptors (nAChRs), important receptors in the hippocampus and prefrontal cortex for learning, memory, and executive function. Targeting only α7 nAChR, instead of all cholinergic receptors, as IAch drugs do, should reduce toxicity (Colovic et al., 2013; Yakel, 2013).
Both ABT-126, a selective α7 nicotinic receptor agonist, and ABT-089, an α4β2 neuronal nicotinic receptor partial agonist, showed no therapeutic effect (Lenz et al., 2015; Florian et al., 2016; Gault et al., 2016). ABT-126 was generally well tolerated but also induced agitation, constipation, diarrhea, fall and headache (Florian et al., 2016; Gault et al., 2016).
Glutamatergic pathways
Glutamatergic neurotransmission related to N-methyl-d-aspartate (NMDA) function in cortical and hippocampal brain regions also plays a relevant role in AD pathogenesis. Memantine is an approved medication for this target. Activation of NMDA receptor signaling pathway produces secondary messengers, such as cyclic guanosine monophosphate (cGMP). Therefore, inhibition of phosphodiesterase type 9 (PDE9), which hydrolyzes cGMP, could increase cGMP levels and enhance cognition through long-term potentiation (LTP) (Reneerkens et al., 2009; Dubois et al., 2010). BI 409306 is a PDE9 inhibitor that was promising in rodents’ test, but no clinically meaningful changes were detected (Frölich et al., 2019).
Glutaminyl cyclase
Glutaminyl cyclase (QC) plays a central role in synaptotoxic Aβ oligomer formation with pro-inflammatory potential. QC is an enzyme (glutaminyl peptide cyclotransferase, EC 2.3.2.5) that converts glutamate residue at position 3 of the N-terminus of truncated Aβ to AβpE3 peptide (Coimbra et al., 2019) and is involved in several pathological disorders, especially in AD (Vijayan and Zhang, 2019). Evidence have shown that AβpE3, a modified form of Aβ, may contribute to tau hyperphosphorylation (Mandler et al., 2014; Hennekens et al., 2015; Bayer, 2022). Indeed, AβpE3 is upstream in the neurotoxic amyloid cascade triggering neurodegeneration and influencing the severity of AD pathology (Pivtoraiko et al., 2015; Moro, et al., 2018). Since QC may catalyze the generation of cerebral AβpE3, its activity is overexpressed in AD brains, showing that QC inhibitors may be a good option for the development of AD-modifying strategies. The inhibitory activity of current inhibitors is mainly triggered by zinc-binding groups that coordinate zinc ion in the active site and other common features (Coimbra et al., 2019). Moreover, the inhibition of this enzyme also reduces the formation of mature CCL2, and thus suppresses neuroinflammation (Vijayan and Zhang, 2019). Although Scheltens et al. (2018) showed cognitive improvement with PQ912, some participants discontinued the trial due to high-dose toxicity. PQ912 targeted glutaminyl cyclase enzymes in patients with mild AD. The study was promising due to the functional improvement, inhibition of deterioration of synaptic activity, and reduction of neuroinflammation in patients.
Neuroinflammation
Chronic brain inflammation is another pathological hallmark of AD. Neuroinflammation is initiated when glial cells are activated by neural environment or neuronal injury. Particularly, tumor necrosis factor-α (TNF-α) signaling plays a master role in this scenario and has been associated with neuronal excitotoxicity, synapse loss, and propagation of the inflammatory state. TNF-α signaling also exacerbates amyloidogenesis, including upregulation of BACE-1 expression (Song et al., 2021; Chen et al., 2022). Etanercept and thalidomide are TNF-α antagonists but none had therapeutic effects in clinical trials (Butchart et al., 2015; Decourt et al., 2017). Etanercept limitation is related to its pharmacokinetics; it is unable to cross the brain–blood barrier (Butchart et al., 2015). Thalidomide had poor tolerability (Decourt et al., 2017).
Some already approved drugs were also in evaluation for AD as an off-label strategy. A calcium channel blocker antihypertensive drug, nilvadipine, reduced amyloid production, increased cerebral blood flow, and has demonstrated anti-inflammatory and anti-tau activity in preclinical studies. These properties could be related to a blood pressure control or anti-amyloid mechanisms (Paris et al., 2004; Paris et al., 2014). However, clinical trial results do not suggest benefit of nilvadipine as a treatment to AD (Lawlor et al., 2018; Abdullah et al., 2020). Insulin also was evaluated due to its metabolic, mitochondrial, and protease activity, influencing clearance of Aβ peptide and phosphorylation of tau (Valla et al., 2010; Kellar and Craft, 2020). Intranasal glulisine, an insulin analog lacking potential olfactory toxicity due to zinc ingredient commonly found in insulin formulations, also failed in therapeutic effects (Rosenbloom et al., 2021). Oxidative stress is one of multiple factors contributing to AD pathogenesis. Monoamine oxidase B (MAO-B) enzymes are related to this mechanism in astrocytes due to oxidative deamination of neurotransmitters. A number of MAO-B inhibitors (MAO-Bi) have been studied for AD and Parkinson’s disease, such as sembragiline a selective MAO-Bi. Sembragiline demonstrated a good safety profile and potential effect on neuropsychiatric symptoms and behaviorally impaired (Nave et al., 2017).
Natural products were also studied. A marine derivative PKC epsilon activator, namely, bryostatin, increased synaptic numbers via synaptic growth factors but showed no efficacy in trial (Farlow et al., 2019). MLC901 (NeuroAiD II) contains extracts from nine herbal components, and triggered neurogenesis and neuroproliferation in rodents and human stem cell cultures due to activating ATP-dependent potassium channels (KATP) and modulating neuroinflammation. Clinically, MLC601 as monotherapy showed better tolerability and comparable efficacy to AChEIs in patients with mild to moderate AD, vascular dementia, and mild cognitive impairment (Chen et al., 2022). Sodium oligomannate (GV-971) is a marine-derived oligosaccharide that can modulate gut microbiota, reducing neuroinflammation in the brain as observed in animal models. GV-971 demonstrated significant efficacy in improving cognition and was safe and well-tolerated (Xiao et al., 2021).
SIRT-1 showed good results in phase two clinical trials (Turner, et al., 2015; Moussa et al., 2017). SIRT-1 is a sirtuin, a deacetylase protein regulated by NAD+/NADH activated by caloric restriction, which itself decreases age-dependent cognitive decline in animal models (Turner et al., 2015; Moussa et al., 2017). Resveratrol is a potent activator of SIRT-1 and helped to maintain blood–brain barrier (BBB) integrity by reducing oxidative stress, and inhibiting of NF-κB and matrix metalloproteinase-9 (MMP9) release (Lin et al., 2010; Wang et al., 2016; Espinoza et al., 2017).
In addition, resveratrol induced adaptive immune responses (Moussa et al., 2017). However, in phase 2 study, a reduction in brain volume was also found, and Turner et al. (2015) suggested lack of benefits. Moussa et al. (2017) showed that resveratrol significantly attenuated declined Aβ levels in CSF, and decreased cognitive and functional decline. The drug also reduced plasma levels of pro-inflammatory producers.
Conclusion
In addition to all technology and information in AD pathogenesis, the most common outcome of AD new drug clinical trials was the lack of efficacy. However, those results may be limited by the disease stage of patients because earlier therapy has better performance in AD. Due to aducanumab recent FDA approval, many of the studies were using antibodies against Aβ, but they showed no noteworthy results. 5-HT6 antagonists, tau inhibitors, and nicotinic agonists’ data were also discouraging. However, anti-Aβ vaccine, BACE inhibitor, and anti-neuroinflammation drugs led to promising results with some drugs showing clinical improvements and no toxicity.
Author contributions
CCF and LL collected and analyzed the references. CCF, LL, ST, and NM designated the table and figure. CCF, LL, CM-P, JRJ, RB, OC-N, BS, RPA, FC, ST, and NM wrote and reviewed the manuscript. All authors contributed to the article and approved the submitted version.
Funding
This work was supported by Fundação de Amparo à Pesquisa e Inovação do Espírito Santo (FAPES) grant number 931/2022 P: 2022-2N1DV.
Conflict of interest
The authors declare that the research was conducted in the absence of any commercial or financial relationships that could be construed as a potential conflict of interest.
Publisher’s note
All claims expressed in this article are solely those of the authors and do not necessarily represent those of their affiliated organizations, or those of the publisher, the editors, and the reviewers. Any product that may be evaluated in this article, or claim that may be made by its manufacturer, is not guaranteed or endorsed by the publisher.
Supplementary material
The Supplementary Material for this article can be found online at: https://www.frontiersin.org/articles/10.3389/fphar.2023.1101452/full#supplementary-material
References
Abdullah, L., Crawford, F., Tsolaki, M., Börjesson-Hanson, A., Olde Rikkert, M., Pasquier, F., et al. (2020). The influence of baseline Alzheimer's disease severity on cognitive decline and CSF biomarkers in the NILVAD trial. Front. Neurol. 11, 149. doi:10.3389/fneur.2020.00149
Alzheimer, A., Stelzmann, R. A., Schnitzlein, H. N., and Murtagh, F. R. (1995). An English translation of Alzheimer's 1907 paper Uber eine eigenartige Erkankung der Hirnrinde. Clin. Anat. 6, 429–431. doi:10.1002/ca.980080612
Alzheimer, A. (1906). Über einen eigenartigen schweren Erkrankungsprozeß der Hirnrinde. Neurol. Cent. 23, 1129–1136.
Andrews, M., Tousi, B., and Sabbagh, M. N. (2018). 5HT6 antagonists in the treatment of Alzheimer’s dementia: Current progress. Neurol. Ther. 7, 51–58. doi:10.1007/s40120-018-0095-y
Arai, H., Umemura, K., Ichimiya, Y., Iseki, E., Eto, K., Miyakawa, K., et al. (2016). Safety and pharmacokinetics of bapineuzumab in a single ascending-dose study in Japanese patients with mild to moderate Alzheimer's disease. Geriatr. Gerontol. Int. 5, 644–650. doi:10.1111/ggi.12516
Atri, A., Frölich, L., Ballard, C., Tariot, P. N., Molinuevo, J. L., Boneva, N., et al. (2018). Effect of idalopirdine as adjunct to cholinesterase inhibitors on change in cognition in patients with alzheimer disease: Three randomized clinical trials. JAMA 2, 130–142. doi:10.1001/jama.2017.20373
Bakker, C., Tasker, T., Liptrot, J., Hart, E. P., Klaassen, E. S., Prins, S., et al. (2021). First-in-man study to investigate safety, pharmacokinetics and exploratory pharmacodynamics of HTL0018318, a novel M1 -receptor partial agonist for the treatment of dementias. Br. J. Clin. Pharmacol. 7, 2945–2955. doi:10.1111/bcp.14710
Bartus, R. T., Dean, R. L., Beer, B., and Lippa, A. S. (1982). The cholinergic hypothesis of geriatric memory dysfunction. Science 217, 408–414. doi:10.1126/science.7046051
Bayer, T. A. (2022). Pyroglutamate Aβ cascade as drug target in Alzheimer’s disease. Mol. Psychiatry. 27, 1880–1885. doi:10.1038/s41380-021-01409-2
Botchway, B. O. A., Moore, M. K., Akinleye, F. O., Iyer, I. C., and Fang, M. (2018). Nutrition: Review on the possible treatment for Alzheimer's disease. J. Alzheimers Dis. 3, 867–883. doi:10.3233/JAD-170874
Briggs, R., Kennelly, S. P., and O'Neill, D. (2016). Drug treatments in Alzheimer's disease. Clin. Med. (Lond). 3, 247–253. doi:10.7861/clinmedicine.16-3-247
Brody, M., Liu, E., Di, J., Lu, M., Margolin, R. A., Werth, J. L., et al. (2016). A phase II, randomized, double-blind, placebo-controlled study of safety, pharmacokinetics, and biomarker results of subcutaneous bapineuzumab in patients with mild to moderate Alzheimer's disease. J. Alzheimers Dis. 4, 1509–1519. doi:10.3233/JAD-160369
Butchart, J., Brook, L., Hopkins, V., Teeling, J., Püntener, U., Culliford, D., et al. (2015). Etanercept in alzheimer disease: A randomized, placebo-controlled, double-blind, phase 2 trial. Neurology 21, 2161–2168. doi:10.1212/WNL.0000000000001617
Cebers, G., Alexander, R. C., Haeberlein, S. B., Han, D., Goldwater, R., Ereshefsky, L., et al. (2017). AZD3293: Pharmacokinetic and pharmacodynamic effects in healthy subjects and patients with Alzheimer’s disease. J. Alzheim. Dis. 55, 1039–1053. doi:10.3233/JAD-160701
Chen, C. L. H., Lu, Q., Moorakonda, R. B., Kandiah, N., Tan, B. Y., Villaraza, S. G., et al. (2022). Alzheimer's disease THErapy with NEuroaid (ATHENE): A randomized double-blind delayed-start trial. J. Am. Med. Dir. Assoc. 3, 379–386.e3. e3. doi:10.1016/j.jamda.2021.10.018
Chen, C., Liao, J., Xia, Y., Liu, X., Jones, R., Haran, J., et al. (2022). Gut microbiota regulate Alzheimer's disease pathologies and cognitive disorders via PUFA-associated neuroinflammation. Gut 11, 2233–2252. doi:10.1136/gutjnl-2021-326269
Coimbra, J. R. M., Sobral, P. J. M., Santos, A. E., Moreira, P. I., and Salvador, J. A. R. (2019). An overview of glutaminyl cyclase inhibitors for Alzheimer’s disease. Futur. Chem. 24, 3179–3194. doi:10.4155/fmc-2019-0163
Colovic, M. B., Krstic, D. Z., Lazarevic-Pasti, T. D., Bondzic, A. M., and Vasic, V. M. (2013). Acetylcholinesterase inhibitors: Pharmacology and toxicology. Curr. Neuropharmacol. 3, 315–335. doi:10.2174/1570159X11311030006
Da Mesquita, S., Papadopoulos, Z., Dykstra, T., Brase, L., Farias, F. G., Wall, M., et al. (2021). Meningeal lymphatics affect microglia responses and anti-Aβ immunotherapy. Nature 7858, 255–260. doi:10.1038/s41586-021-03489-0
Decourt, B., Drumm-Gurnee, D., Wilson, J., Jacobson, S., Belden, C., Sirrel, S., et al. (2017). Poor safety and tolerability hamper reaching a potentially therapeutic dose in the use of thalidomide for Alzheimer's disease: Results from a double-blind, placebo-controlled trial. Curr. Alzheimer Res. 4, 403–411. doi:10.2174/1567205014666170117141330
Dixit, R., Ross, J. L., Goldman, Y. E., and Holzbaur, E. L. (2008). Differential regulation of dynein and kinesin motor proteins by tau. Science 5866, 1086–1089. doi:10.1126/science.1152993
Dubois, B., Feldman, H. H., Jacova, C., Cummings, J. L., Dekosky, S. T., Barberger-Gateau, P., et al. (2010). Revising the definition of Alzheimer's disease: A new lexicon. Lancet Neurol. 11, 1118–1127. doi:10.1016/S1474-4422(10)70223-4
Egan, M. F., Kost, J., Tariot, P. N., Aisen, P. S., Cummings, J. L., Vellas, B., et al. (2018). Randomized trial of verubecestat for mild-to-moderate Alzheimer's disease. N. Engl. J. Med. 18, 1691–1703. doi:10.1056/NEJMoa1706441
Egan, M. F., Kost, J., Voss, T., Mukai, Y., Aisen, P. S., Cummings, J. L., et al. (2019). Randomized trial of verubecestat for prodromal Alzheimer's disease. N. Engl. J. Med. 15, 1408–1420. doi:10.1056/NEJMoa1812840
Espinoza, J. L., Trung, L. Q., Inaoka, P. T., Yamada, K., An, D. T., Mizuno, S., et al. (2017). The repeated administration of resveratrol has measurable effects on circulating T-cell subsets in humans. Oxid. Med. Cell Longev. 2017, 6781872. doi:10.1155/2017/6781872
Farlow, M. R., Thompson, R. E., Wei, L. J., Tuchman, A. J., Grenier, E., Crockford, D., et al. (2019). A randomized, double-blind, placebo-controlled, phase II study assessing safety, tolerability, and efficacy of bryostatin in the treatment of moderately severe to severe Alzheimer's disease. J. Alzheimers Dis. 2, 555–570. doi:10.3233/JAD-180759
Ferrer, I., Boada Rovira, M., Sanchez Guerra, M. L., Rey, M. J., and Costa-Jussa, F. (2004). Neuropathology and pathogenesis of encephalitis following amyloid-immunization in Alzheimer’s disease. Brain Pathol. 14, 11–20. doi:10.1111/j.1750-3639.2004.tb00493.x
Fiala, J. C., Feinberg, M., Peters, A., and Barbas, H. (2007). Mitochondrial degeneration in dystrophic neurites of senile plaques may lead to extracellular deposition of fine filaments. Brain Struct. Funct. 2, 195–207. doi:10.1007/s00429-007-0153-1
Florian, H., Meier, A., Gauthier, S., Lipschitz, S., Lin, Y., Tang, Q., et al. (2016). Efficacy and safety of ABT-126 in subjects with mild-to-moderate Alzheimer's disease on stable doses of acetylcholinesterase inhibitors: A randomized, double-blind, placebo-controlled study. J. Alzheimers Dis. 4, 1237–1247. doi:10.3233/JAD-150978
Frölich, L., Wunderlich, G., Thamer, C., Roehrle, M., Garcia, M., and Dubois, B. (2019). Evaluation of the efficacy, safety and tolerability of orally administered BI 409306, a novel phosphodiesterase type 9 inhibitor, in two randomised controlled phase II studies in patients with prodromal and mild Alzheimer's disease. Alzheimers Res. Ther. 1, 18. doi:10.1186/s13195-019-0467-2
Fullerton, T., Binneman, B., David, W., Delnomdedieu, M., Kupiec, J., Lockwood, P., et al. (2018). A Phase 2 clinical trial of PF-05212377 (SAM-760) in subjects with mild to moderate Alzheimer's disease with existing neuropsychiatric symptoms on a stable daily dose of donepezil. Alzheimers Res. Ther. 1, 38. doi:10.1186/s13195-018-0368-9
Gault, L. M., Lenz, R. A., Ritchie, C. W., Meier, A., Othman, A. A., Tang, Q., et al. (2016). ABT-126 monotherapy in mild-to-moderate Alzheimer's dementia: Randomized double-blind, placebo and active controlled adaptive trial and open-label extension. Alzheimers Res. Ther. 1, 44. doi:10.1186/s13195-016-0210-1
Gauthier, S., Feldman, H. H., Schneider, L. S., Wilcock, G. K., Frisoni, G. B., Hardlund, J. H., et al. (2016). Efficacy and safety of tau-aggregation inhibitor therapy in patients with mild or moderate Alzheimer's disease: A randomised, controlled, double-blind, parallel-arm, phase 3 trial. Lancet 10062, 2873–2884. doi:10.1016/S0140-6736(16)31275-2
Geylis, V., and Steinitz, N. (2006). Immunotherapy of Alzheimer's disease (AD): From murine models to anti-amyloid beta (abeta) human monoclonal antibodies. Autoimmun. Rev. 5, 33–39. doi:10.1016/j.autrev.2005.06.007
Gomez-Isla, T., Hollister, R., West, H., Mui, S., Growdon, J. H., Petersen, R. C., et al. (1997). Neuronal loss correlates with but exceeds neurofibrillary tangles in Alzheimer’s disease. Ann. Neurol. 1, 17–24. doi:10.1002/ana.410410106
Hardy, J., and Allsop, D. (1991). Amyloid deposition as the central event in the aetiology of Alzheimer’s disease. Trends Pharmacol. Sci. 12, 383–388. doi:10.1016/0165-6147(91)90609-v
Harrington, C. R., Storey, J. M., Clunas, S., Harrington, K. A., Horsley, D., Ishaq, A., et al. (2015). Cellular models of aggregation-dependent template-directed proteolysis to characterize tau aggregation inhibitors for treatment of alzheimer disease. J. Biol. Chem. 17, 10862–10875. doi:10.1074/jbc.M114.616029
Hebert, L. E., Weuve, J., Scherr, P. A., and Evans, D. A. (2013). Alzheimer disease in the United States (2010-2050) estimated using the 2010 census. Neurology 80, 1778–1783. doi:10.1212/WNL.0b013e31828726f5
Hennekens, C. H., Bensadon, B. A., Zivin, R., and Gaziano, J. M. (2015). Hypothesis: Glutaminyl cyclase inhibitors decrease risks of Alzheimer’s disease and related dementias. Expert. Rev. Neurother. 11, 1245–1248. doi:10.1586/14737175.2015.1088784
Higa, G. S. V., Francis-Oliveira, J., Carlos-Lima, E., Tamais, A. M., Borges, F. D. S., Kihara, A. H., et al. (2022). 5-HT-dependent synaptic plasticity of the prefrontal cortex in postnatal development. Sci. Rep. 1, 21015. doi:10.1038/s41598-022-23767-9
Holmes, C., Cunningham, C., Zotova, E., Woolford, J., Dean, C., Kerr, S., et al. (2009). Systemic inflammation and disease progression in Alzheimer disease. Neurology 10, 768–774. doi:10.1212/WNL.0b013e3181b6bb95
Honig, L. S., Vellas, B., Woodward, M., Boada, M., Bullock, R., Borrie, M., et al. (2018). Trial of solanezumab for mild dementia due to Alzheimer's disease. N. Engl. J. Med. 4, 321–330. doi:10.1056/NEJMoa1705971
Hung, S. Y., and Fu, W. M. (2017). Drug candidates in clinical trials for Alzheimer’s disease. J. Biomed. Sci. 24, 47. doi:10.1186/s12929-017-0355-7
Iqbal, K., Liu, F., Gong, C. X., and Grundke-Iqbal, I. (2010). Tau in Alzheimer disease and related tauopathies. Curr. Alzheimer Res. 8, 656–664. doi:10.2174/156720510793611592
Ivanoiu, A., Pariente, J., Booth, K., Lobello, K., Luscan, G., Hua, L., et al. (2016). Long-term safety and tolerability of bapineuzumab in patients with Alzheimer's disease in two phase 3 extension studies. Alzheimers Res. Ther. 1, 24. doi:10.1186/s13195-016-0193-y
Kellar, D., and Craft, S. (2020). Brain insulin resistance in Alzheimer's disease and related disorders: Mechanisms and therapeutic approaches. Lancet Neurol. 9, 758–766. doi:10.1016/S1474-4422(20)30231-3
Kucwaj-Brysz, K., Baltrukevich, H., Czarnota, K., and Handzlik, J. (2021). Chemical update on the potential for serotonin 5-HT6 and 5-HT7 receptor agents in the treatment of Alzheimer’s disease. Bioorg. Med. Chem. Lett. 49, 128275. doi:10.1016/j.bmcl.2021.128275
Kumar, N., Gahlawat, A., Kumar, R. N., Singh, Y. P., Modi, G., and Garg, P. (2020). Drug repurposing for Alzheimer’s disease: In silico and in vitro investigation of FDA-approved drugs as acetylcholinesterase inhibitors. J. Biomol. Struct. Dyn. 10, 2878–2892. doi:10.1080/07391102.2020.1844054
Lacosta, A. M., Pascual-Lucas, M., Pesini, P., Casabona, D., Pérez-Grijalba, V., Marcos-Campos, I., et al. (2018). Safety, tolerability and immunogenicity of an active anti-aβ40 vaccine (ABvac40) in patients with Alzheimer's disease: A randomised, double-blind, placebo-controlled, phase I trial. Alzheimers Res. Ther. 1, 12. doi:10.1186/s13195-018-0340-8
Lawlor, B., Segurado, R., Kennelly, S., Olde Rikkert, M. G. M., Howard, R., Pasquier, F., et al. (2018). Nilvadipine in mild to moderate alzheimer disease: A randomised controlled trial. PLoS Med. 9, e1002660. doi:10.1371/journal.pmed.1002660
Lenz, R. A., Pritchett, Y. L., Berry, S. M., Llano, D. A., Han, S., Berry, D. A., et al. (2015). Adaptive, dose-finding phase 2 trial evaluating the safety and efficacy of ABT-089 in mild to moderate Alzheimer disease. Alzheimer Dis. Assoc. Disord. 3, 192–199. doi:10.1097/WAD.0000000000000093
Lin, Y. L., Chang, H. C., Chen, T. L., Chang, J. H., Chiu, W. T., Lin, J. W., et al. (2010). Resveratrol protects against oxidized LDL-induced breakage of the blood-brain barrier by lessening disruption of tight junctions and apoptotic insults to mouse cerebrovascular endothelial cells. J. Nutr. 12, 2187–2192. doi:10.3945/jn.110.123505
Liu, P., Yin, Y. L., Wang, T., Hou, L., Wang, X. X., Wang, M., et al. (2019). Ligand-induced activation of ERK1/2 signaling by constitutively active Gs-coupled 5-HT receptors. Acta. Pharmacol. Sin. 9, 1157–1167. doi:10.1038/s41401-018-0204-6
Liu-Seifert, H., Case, M. G., Andersen, S. W., Holdridge, K. C., Aisen, P. S., Kollack-Walker, S., et al. (2018). Delayed-start analyses in the phase 3 solanezumab EXPEDITION3 study in mild Alzheimer's disease. J. Prev. Alzheimers Dis. 1, 8–14. doi:10.14283/jpad.2018.1
Logovinsky, V., Satlin, A., Lai, R., Swanson, C., Kaplow, J., Osswald, G., et al. (2016). Safety and tolerability of BAN2401--a clinical study in Alzheimer's disease with a protofibril selective Aβ antibody. Alzheimers Res. Ther. 1, 14. doi:10.1186/s13195-016-0181-2
Mafi, J. N., Leng, M., Arbanas, J. C., Tseng, C. H., Damberg, C. L., Sarkisian, C., et al. (2022). Estimated annual spending on aducanumab in the US medicare program. JAMA Health Forum 1, e214495. doi:10.1001/jamahealthforum.2021.4495
Mandler, M., Walker, L., Santic, R., Hanson, P., Upadhaya, A. R., Colby, S. J., et al. (2014). Pyroglutamylated amyloid-beta is associated with hyperphosphorylated tau and severity of Alzheimer’s disease. Acta. Neuropathol. 1, 67–79. doi:10.1007/s00401-014-1296-9
Masliah, E., Hansen, L., Adame, A., Crews, L., Bard, F., Lee, C., et al. (2005). Abeta vaccination effects on plaque pathology in the absence of encephalitis in Alzheimer disease. Neurology 64, 129–131. doi:10.1212/01.WNL.0000148590.39911.DF
McDade, E., Voytyuk, I., Aisen, P., Bateman, R. J., Carrillo, M. C., De Strooper, B., et al. (2021). The case for low-level BACE1 inhibition for the prevention of Alzheimer disease. Nat. Rev. Neurol. 11, 703–714. doi:10.1038/s41582-021-00545-1
Melis, V., Magbagbeolu, M., Rickard, J. E., Horsley, D., Davidson, K., Harrington, K. A., et al. (2015). Effects of oxidized and reduced forms of methylthioninium in two transgenic mouse tauopathy models. Behav. Pharmacol. 4, 353–368. doi:10.1097/FBP.0000000000000133
Mohammad, D., Chan, P., Bradley, J., Lanctot, K., and Herrmann, N. (2017). Acetylcholinesterase inhibitors for treating dementia symptoms-a safety evaluation. Expert Opin. Drug. Saf. 16, 1009–1019. doi:10.1080/14740338.2017.1351540
Moro, M. L., Phillips, A. S., Gaimster, K., Paul, C., Mudher, A., Nicoll, J. A. R., et al. (2018). Pyroglutamate and isoaspartate modified amyloid-beta in ageing and Alzheimer’s disease. Acta. Neuropathol. Commun. 1, 3. doi:10.1186/s40478-017-0505-x
Moussa, C., Hebron, M., Huang, X., Ahn, J., Rissman, R. A., Aisen, P. S., et al. (2017). Resveratrol regulates neuro-inflammation and induces adaptive immunity in Alzheimer's disease. J. Neuroinflammation. 1, 1. doi:10.1186/s12974-016-0779-0
Nave, S., Doody, R. S., Boada, M., Grimmer, T., Savola, J. M., Delmar, P., et al. (2017). Sembragiline in moderate Alzheimer's disease: Results of a randomized, double-blind, placebo-controlled phase II trial (MAyflOwer RoAD). J. Alzheimers Dis. 4, 1217–1228. doi:10.3233/JAD-161309
Nicoll, J. A., Wilkinson, D., Holmes, C., Steart, P., Markham, H., and Weller, R. O. (2003). Neuropathology of human alzheimer disease after immunization with amyloid-beta peptide: A case report. Nat. Med. 9, 448–452. doi:10.1038/nm840
Ostrowitzki, S., Lasser, R. A., Dorflinger, E., Scheltens, P., Barkhof, F., Nikolcheva, T., et al. (2017). A phase III randomized trial of gantenerumab in prodromal Alzheimer's disease. Alzheimers Res. Ther. 1, 95. doi:10.1186/s13195-017-0318-y
Paris, D., Ait-Ghezala, G., Bachmeier, C., Laco, G., Beaulieu-Abdelahad, D., Lin, Y., et al. (2014). The spleen tyrosine kinase (Syk) regulates Alzheimer amyloid-β production and Tau hyperphosphorylation. J. Biol. Chem. 49, 33927–33944. doi:10.1074/jbc.M114.608091
Paris, D., Quadros, A., Humphrey, J., Patel, N., Crescentini, R., Crawford, F., et al. (2004). Nilvadipine antagonizes both Abeta vasoactivity in isolated arteries, and the reduced cerebral blood flow in APPsw transgenic mice. Brain Res. 1, 53–61. doi:10.1016/j.brainres.2003.11.061
Pasquier, F., Sadowsky, C., Holstein, A., Leterme Gle, P., Peng, Y., Jackson, N., et al. (2016). Two phase 2 multiple ascending-dose studies of vanutide cridificar (ACC-001) and QS-21 adjuvant in mild-to-moderate Alzheimer's disease. J. Alzheimers Dis. 4, 1131–1143. doi:10.3233/JAD-150376
Pivtoraiko, V. N., Abrahamson, E. E., Leurgans, S. E., Dekosky, S. T., Mufson, E. J., and Ikonomovic, M. D. (2015). Cortical pyroglutamate amyloid-beta levels and cognitive decline in Alzheimer’s disease. Neurobiol. Aging. 1, 12–19. doi:10.1016/j.neurobiolaging.2014.06.021
Ranasinghe, K. G., Petersen, C., Kudo, K., Mizuiri, D., Rankin, K. P., Rabinovici, G. D., et al. (2021). Reduced synchrony in alpha oscillations during life predicts post mortem neurofibrillary tangle density in early-onset and atypical Alzheimer's disease. Alzheimers Dement. 12, 2009–2019. doi:10.1002/alz.12349
Recio-Barbero, M., Segarra, R., Zabala, A., González-Fraile, E., González-Pinto, A., and Ballesteros, J. (2021). Cognitive enhancers in schizophrenia: A systematic review and meta-analysis of alpha-7 nicotinic acetylcholine receptor agonists for cognitive deficits and negative symptoms. Front. Psychiatry. 12, 631589. doi:10.3389/fpsyt.2021.631589
Relkin, N. R., Thomas, R. G., Rissman, R. A., Brewer, J. B., Rafii, M. S., van Dyck, C. H., et al. (2017). A phase 3 trial of IV immunoglobulin for Alzheimer disease. Neurology 18, 1768–1775. doi:10.1212/WNL.0000000000003904
Reneerkens, O. A., Rutten, K., Steinbusch, H. W., Blokland, A., and Prickaerts, J. (2009). Selective phosphodiesterase inhibitors: A promising target for cognition enhancement. Psychopharmacol. Berl. 1-3, 419–443. doi:10.1007/s00213-008-1273-x
Rosenbloom, M., Barclay, T. R., Kashyap, B., Hage, L., O'Keefe, L. R., Svitak, A., et al. (2021). A phase II, single-center, randomized, double-blind, placebo-controlled study of the safety and therapeutic efficacy of intranasal glulisine in amnestic mild cognitive impairment and probable mild Alzheimer's disease. Drugs Aging 5, 407–415. doi:10.1007/s40266-021-00845-7
Sabandal, P. R., Saldes, E. B., and Han, K. A. (2022). Acetylcholine deficit causes dysfunctional inhibitory control in an aging-dependent manner. Sci. Rep. 1, 20903. doi:10.1038/s41598-022-25402-z
Sakamoto, K., Matsuki, S., Matsuguma, K., Yoshihara, T., Uchida, N., Azuma, F., et al. (2017). BACE1 inhibitor lanabecestat (AZD3293) in a phase 1 study of healthy Japanese subjects: Pharmacokinetics and effects on plasma and cerebrospinal fluid Aβ peptides. J. Clin. Pharmacol. 11, 1460–1471. doi:10.1002/jcph.950
Salloway, S., Marshall, G. A., Lu, M., and Brashear, H. R. (2018). Long-term safety and efficacy of bapineuzumab in patients with mild-to-moderate Alzheimer's disease: A phase 2, open-label extension study. Curr. Alzheimer Res. 13, 1231–1243. doi:10.2174/1567205015666180821114813
Salloway, S. P., Sperling, R., Fox, N. C., Sabbagh, M. N., Honig, L. S., Porsteinsson, A. P., et al. (2018). Long-term follow up of patients with mild-to-moderate Alzheimer's disease treated with bapineuzumab in a phase III, open-label, extension study. J. Alzheimers Dis. 3, 689–707. doi:10.3233/JAD-171157
Scheltens, P., De Strooper, B., Kivipelto, M., Holstege, H., Chételat, G., Teunissen, C. E., et al. (2021). Alzheimer's disease. Lancet 10284, 1577–1590. doi:10.1016/S0140-6736(20)32205-4
Scheltens, P., Hallikainen, M., Grimmer, T., Duning, T., Gouw, A. A., Teunissen, C. E., et al. (2018). Safety, tolerability and efficacy of the glutaminyl cyclase inhibitor PQ912 in Alzheimer's disease: Results of a randomized, double-blind, placebo-controlled phase 2a study. Alzheimers Res. Ther. 1, 107. doi:10.1186/s13195-018-0431-6
Sengupta, U., Nilson, A. N., and Kayed, U. (2016). The role of amyloid-β oligomers in toxicity, propagation, and immunotherapy. EBioMedicine 6, 42–49. doi:10.1016/j.ebiom.2016.03.035
Solas, M., van Dam, D., Janssens, J., Ocariz, U., Vermeiren, Y., de Deyn, P. P., et al. (2021). 5-HT7 receptors in Alzheimer’s disease. Neurochem. Int. 150, 105185. doi:10.1016/j.neuint.2021.105185
Song, T., Song, X., Zhu, C., Patrick, R., Skurla, M., Santangelo, I., et al. (2021). Mitochondrial dysfunction, oxidative stress, neuroinflammation, and metabolic alterations in the progression of Alzheimer's disease: A meta-analysis of in vivo magnetic resonance spectroscopy studies. Ageing Res. Rev. 72, 101503. doi:10.1016/j.arr.2021.101503
Timmers, M., Streffer, J. R., Russu, A., Tominaga, Y., Shimizu, H., Shiraishi, A., et al. (2018). Pharmacodynamics of atabecestat (JNJ-54861911), an oral BACE1 inhibitor in patients with early Alzheimer's disease: Randomized, double-blind, placebo-controlled study. Alzheimers Res. Ther. 1, 85. doi:10.1186/s13195-018-0415-6
Tolar, M., Abushakra, S., and Sabbagh, M. (2020). The path forward in Alzheimer's disease therapeutics: Reevaluating the amyloid cascade hypothesis. Alzheimers Dement. 11, 1553–1560. doi:10.1016/j.jalz.2019.09.075
Troutwine, B. R., Strope, T. A., Franczak, E., Lysaker, C. R., Hamid, L., Mansel, C., et al. (2022). Mitochondrial function and Aβ in Alzheimer's disease postmortem brain. Neurobiol. Dis. 171, 105781. doi:10.1016/j.nbd.2022.105781
Turner, R. S., Thomas, R. G., Craft, S., van Dyck, C. H., Mintzer, J., Reynolds, B. A., et al. (2015). A randomized, double-blind, placebo-controlled trial of resveratrol for Alzheimer disease. Neurology 16, 1383–1391. doi:10.1212/WNL.0000000000002035
Vaillant-Beuchot, L., Mary, A., Pardossi-Piquard, R., Bourgeois, A., Lauritzen, I., Eysert, F., et al. (2021). Accumulation of amyloid precursor protein C-terminal fragments triggers mitochondrial structure, function, and mitophagy defects in Alzheimer's disease models and human brains. Acta. Neuropathol. 1, 39–65. doi:10.1007/s00401-020-02234-7
Valla, J., Yaari, R., Wolf, A. B., Kusne, Y., Beach, T. G., Roher, A. E., et al. (2010). Reduced posterior cingulate mitochondrial activity in expired young adult carriers of the APOE ε4 allele, the major late-onset Alzheimer's susceptibility gene. J. Alzheimers Dis. 1, 307–313. doi:10.3233/JAD-2010-100129
van Dyck, C. H., Sadowsky, C., Le Prince Leterme, G., Booth, K., Peng, Y., Marek, K., et al. (2016). Vanutide cridificar (ACC-001) and QS-21 adjuvant in individuals with early Alzheimer's disease: Amyloid imaging positron emission tomography and safety results from a phase 2 study. J. Prev. Alzheimers Dis. 2, 75–84. doi:10.14283/jpad.2016.91
Vandenberghe, R., Rinne, J. O., Boada, M., Katayama, S., Scheltens, P., Vellas, B., et al. (2016). Bapineuzumab for mild to moderate Alzheimer's disease in two global, randomized, phase 3 trials. Alzheimers Res. Ther. 1, 18. doi:10.1186/s13195-016-0189-7
Vershinin, M., Carter, B. C., Razafsky, D. S., King, S. J., and Gross, S. P. (2017). Multiple-motor based transport and its regulation by Tau. Proc. Natl. Acad. Sci. U. S. A. 1, 87–92. doi:10.1073/pnas.0607919104
Vijayan, D. K., and Zhang, K. Y. J. (2019). Human glutaminyl cyclase: Structure, function, inhibitors and involvement in Alzheimer’s disease. Pharmacol. Res. 147, 104342. doi:10.1016/j.phrs.2019.104342
Wang, D., Li, S. P., Fu, J. S., Zhang, S., Bai, L., and Guo, L. (2016). Resveratrol defends blood-brain barrier integrity in experimental autoimmune encephalomyelitis mice. J. Neurophysiol. 5, 2173–2179. doi:10.1152/jn.00510.2016
Wilcock, G. K., Gauthier, S., Frisoni, G. B., Jia, J., Hardlund, J. H., Moebius, H. J., et al. (2018). Potential of low dose leuco-methylthioninium bis(hydromethanesulphonate) (LMTM) monotherapy for treatment of mild Alzheimer's disease: Cohort analysis as modified primary outcome in a phase III clinical trial. J. Alzheimers Dis. 1, 435–457. doi:10.3233/JAD-170560
World Health Organization (WHO) (2017). Global action plan on the public health response to dementia 2017–2025. Available at: https://apps.who.int/iris/bitstream/handle/10665/259615/9789241513487-eng.pdf [Accessed October 10, 2022].
World Health Organization (WHO) (2022). Global health estimates. Available at: https://www.who.int/data/global-health-estimates [Accessed October 10, 2022].
World Health Organization (WHO) (2021). Global status report on the public health response to dementia. Available at: https://apps.who.int/iris/handle/10665/344701 (Accessed October 10, 2022).
Xiao, S., Chan, P., Wang, T., Hong, Z., Wang, S., Kuang, W., et al. (2021). A 36-week multicenter, randomized, double-blind, placebo-controlled, parallel-group, phase 3 clinical trial of sodium oligomannate for mild-to-moderate Alzheimer's dementia. Alzheimers Res. Ther. 1, 62. doi:10.1186/s13195-021-00795-7
Yakel, J. L. (2013). Cholinergic receptors: Functional role of nicotinic ACh receptors in brain circuits and disease. Pflugers Arch. 4, 441–450. doi:10.1007/s00424-012-1200-1
Keywords: Alzheimer’s disease, molecular target, drug development, pharmacological treatment, new drugs
Citation: Conti Filho CE, Loss LB, Marcolongo-Pereira C, Rossoni Junior JV, Barcelos RM, Chiarelli-Neto O, Silva BSd, Passamani Ambrosio R, Castro FCdAQ, Teixeira SF and Mezzomo NJ (2023) Advances in Alzheimer’s disease’s pharmacological treatment. Front. Pharmacol. 14:1101452. doi: 10.3389/fphar.2023.1101452
Received: 17 November 2022; Accepted: 11 January 2023;
Published: 26 January 2023.
Edited by:
Carla Denise Bonan, Pontifical Catholic University of Rio Grande do Sul, BrazilReviewed by:
Md. Siddiqul Islam, Southeast University, BangladeshCopyright © 2023 Conti Filho, Loss, Marcolongo-Pereira, Rossoni Junior, Barcelos, Chiarelli-Neto, Silva, Passamani Ambrosio, Castro, Teixeira and Mezzomo. This is an open-access article distributed under the terms of the Creative Commons Attribution License (CC BY). The use, distribution or reproduction in other forums is permitted, provided the original author(s) and the copyright owner(s) are credited and that the original publication in this journal is cited, in accordance with accepted academic practice. No use, distribution or reproduction is permitted which does not comply with these terms.
*Correspondence: Sarah Fernandes Teixeira, sfteixeira@unesc.br; Nathana Jamille Mezzomo, nathanajamillemezzomo@gmail.com
†These authors have contributed equally to this work and share first authorship