- 1Departamento de Nutrición y Bioquímica, Pontificia Universidad Javeriana, Bogotá, Colombia
- 2Semillero de Investigación, Biofísica y Fisiología de Canales Iónicos, Pontificia Universidad Javeriana, Bogotá, Colombia
Cancer is a multifactorial process associated with changes in signaling pathways leading to cell cycle variations and gene expression. The transient receptor potential melastatin 8 (TRPM8) channel is a non-selective cation channel expressed in neuronal and non-neuronal tissues, where it is involved in several processes, including thermosensation, differentiation, and migration. Cancer is a multifactorial process associated with changes in signaling pathways leading to variations in cell cycle and gene expression. Interestingly, it has been shown that TRPM8 channels also participate in physiological processes related to cancer, such as proliferation, survival, and invasion. For instance, TRPM8 channels have an important role in the diagnosis, prognosis, and treatment of prostate cancer. In addition, it has been reported that TRPM8 channels are involved in the progress of pancreatic, breast, bladder, colon, gastric, and skin cancers, glioblastoma, and neuroblastoma. In this review, we summarize the current knowledge on the role of TRPM8 channels in cancer progression. We also discuss the therapeutic potential of TRPM8 in carcinogenesis, which has been proposed as a molecular target for cancer therapy.
Introduction
Cancer is a non-communicable disease described as one of the most important global health problems and a leading cause of death before the age of 70 in 91 of 172 countries (Bray et al., 2018; Siegel et al., 2021). Chemotherapy and radiotherapy are the most effective options for treating diverse cancers (Dinakar et al., 2022). However, therapy resistance observed in some cancers or stages is responsible for therapeutic failure, metastasis, recurrence, and bad prognosis in patients (Klumpp et al., 2017; Nomura et al., 2021; Hashemi et al., 2022). Research in new and more effective therapies is being developed to improve cancer treatment’s efficacy. There are reports about new delivery technologies (Grolez et al., 2019b; Dinakar et al., 2022), combination therapy (Mokhtari et al., 2017; Liu T. et al., 2018), and the use of diverse proteins as therapeutic targets. Ion channel activity and associated signaling pathways are highly regulated in cancer, and TRPM8 has been proposed as a molecular target in cancer development and progression (Yee, 2015; Liu et al., 2016; Hantute-Ghesquier et al., 2018; Liu et al., 2020). TRPM8 is a non-selective cation channel with a preference for Ca2+ (McKemy et al., 2002; Bautista et al., 2007). Channel activity is related to diverse physiological processes, including proliferation and migration, and various reports have proposed TRPM8 channels as therapeutic targets for different cancer treatments, including bone, breast, skin, bladder, pancreas, prostate lung, and colon, among others (Borrelli et al., 2014; Ceylan et al., 2016; Liu J. F. et al., 2018; Du et al., 2018; Lan et al., 2019; Liu J. J. et al., 2022; Koivisto et al., 2022). This review aims to describe the role of the TRPM8 channel in carcinogenesis and the therapeutic potential of this channel in cancer treatment. Furthermore, it is given a current perspective of TRPM8 regulating molecules that could be used in cancer therapy alone or combined with other known drugs or therapies.
Structure and modulation of TRPM8 channels
Transient receptor potential (TRP) channels are a family of non-selective cation channels formed by different members with diverse biophysical and pharmacological properties. The melastatin-subfamily member 8 (TRPM8) channel is permeable to monovalent and divalent cations, including Na+, K+, and Ca2+, with higher permeability for Ca2+ (PCa/PNa = 3.2; McKemy et al., 2002). The channel can be activated by low temperature (<25 °C) and cooling agents such as menthol and icilin, in addition to membrane depolarization (McKemy et al., 2002; Bautista et al., 2007; Yee, 2016). TRPM8 channels are tetrameric proteins with six transmembrane domains (S1-S6) and intracellular N-terminal and C-terminal. The S2-S4 region has a high-affinity menthol binding region (Janssens and Voets, 2011), and the S3 domain has specific amino acids for icilin sensitivity (Chuang et al., 2004). The S4 segment, together with the S4-S5 linker, contains the voltage sensor (Voets et al., 2007a), and transmembrane domains S5 and S6 form the channel pore (Voets et al., 2007b). The intracellular C-terminal has a coiled-coil domain necessary for channel tetramerization (Tsuruda et al., 2006). In addition, it also has a region called the TRP domain which is essential for the channel regulation by temperature and phosphatidylinositol 4,5-biphosphate (PIP2; Liu and Qin, 2005; Rohács et al., 2005; Bandell et al., 2006; Phelps and Gaudet, 2007). TRPM8 phosphorylation in the N-terminus domain alters gating properties that negatively modulate the channel activity and modify the channel response to agonists (Rivera et al., 2021).
Diverse endogenous molecules modulate TRPM8 channel activity and expression. For example, PIP2 is necessary for TRPM8 activation induced by cold and menthol (Rohács et al., 2005; Zakharian et al., 2010). TRPM8 channel activity can also be controlled by TRPM8 channel-associated factors 1 and 2 (TCAF1 and TCAF2), which are regulatory proteins that bind to the channel and modulate their activity. The effects of both proteins are opposite as TCAF1 promotes the channel opening while TCAF2 restrains the channel activity (Gkika et al., 2015). Testosterone and estradiol have been reported to bind TRPM8 channels and modulate channel activity or expression. Treatment of human prostate cells with testosterone promotes a Ca2+ current that was inhibited by a TRPM8 channel antagonist, suggesting that the channel is a testosterone receptor (Asuthkar et al., 2015a). Estradiol has been demonstrated to regulate TRPM8 channel expression in breast cancer cell lines (Chodon et al., 2010). (Wondergem et al., 2008). Similarly, nerve growth factor (NGF) induced functional upregulation of the channel through increased channel expression (Kayama et al., 2017). The endocannabinoid anandamide has been shown to have an endogenous functional antagonist effect on TRPM8 channels as anandamide treatment promotes a decreased Ca2+ influx induced by icilin and menthol (De Petrocellis et al., 2007). Furthermore, extracellular and intracellular pH changes can modify TRPM8 channel activation properties, reducing the current amplitude and shifting the threshold for channel activation (Andersson et al., 2004). That is interesting, considering the reported changes in pH in the tumoral microenvironmental (Kato et al., 2013), which could regulate the TRPM8 channel activity.
TRPM8 channels are expressed in neuronal and non-neuronal tissues, including the breast, pancreas, bone, and colon, where they are involved in several physiological processes, including cell proliferation and differentiation. In some of these tissues, channel overexpression has been associated with events characteristic of tumors, like cell migration and invasion. However, in some cancers, it has been reported that TRPM8 channel activation is enough to promote proliferation and migration in some tissues but decrease these processes in others (Liu J. F. et al., 2018; Lan et al., 2019; Liu et al., 2020; Henao et al., 2021; Liu J. J. et al., 2022). In the next section, we will describe the reported functions of TRPM8 in diverse cancers.
Role of TRPM8 channels in cancer
Different reports demonstrate the role of TRPM8 channels in cancer through the modulation of channel expression or activity. TRPM8 is expressed in diverse cancer cells lines and tissues from patients with cancer, including colon cancer (Tsavaler et al., 2001; Borrelli et al., 2014; Liu J. J. et al., 2022); gastric cancer (Xu et al., 2021), oral squamous carcinoma (Okamoto et al., 2012), esophageal carcinoma (Lan et al., 2019), lung cancer (Du et al., 2014; Lai et al., 2020), osteosarcoma (Wang et al., 2013; Zhao and Xu, 2016; Liu Y. et al., 2022), breast cancer (Liu J. et al., 2014), skin cancer (Lan et al., 2019), glioblastoma (Alptekin et al., 2015; Zeng et al., 2019), pancreatic cancer (Liu J. F. et al., 2018) and prostate cancer (Bidaux et al., 2007), among others (Table 1). In addition, overexpression of TRPM8 channels has been demonstrated in gastric, colon, and bone cancers, with a higher expression in patients with metastases. In breast cancer, TRPM8 expression is hormone dependent as steroid deficiency induced a decrease in TRPM8 mRNA. This effect can be counteracted by the treatment with 17β-Estradiol, which restores TRPM8 mRNA levels decreased by steroid deprivation. In addition, silencing estrogen receptors (ERα) promotes a reduction in mRNA TRPM8 expression, suggesting that the channel expression is regulated by ERa (Chodon et al., 2010). Some reports demonstrated that TRPM8 is significantly overexpressed in the cell membrane and cytoplasm of human bladder cancer cells (Li et al., 2010; Wang et al., 2020). However, a considerable reduction in the expression level of TRPM8 mRNA was also reported in bladder cancer tissues. The authors suggest that observed differences could be related to variations in tumor stage (Ceylan et al., 2016; Table 1).
TRPM8 channel activity is also involved in cancer development and progression, and channel overexpression is regularly associated with poor prognosis. For example, in gastric, pancreatic, and bone cancers, adenocarcinoma, and glioblastoma, TRPM8 promotes invasion and metastasis. Moreover, the high expression has been correlated with a stronger aggressiveness, larger tumor size and clinical stage, shorter overall survival rate, and poor prognosis (Dhennin-Duthille et al., 2011; Yee et al., 2012b; Liu J. et al., 2014; Yee et al., 2014; Alptekin et al., 2015; Zhao and Xu, 2016; Liu J. F. et al., 2018; Du et al., 2018; Patel et al., 2019; Zeng et al., 2019; Xu et al., 2021; Liu Y. et al., 2022). Similarly, tissues from patients with urothelial carcinoma of the bladder showed an association of the channel expression with histologic grade and tumor stage, as patients with high TRPM8 expression showed a shorter overall survival and a poor outcome compared with patients with low channel expression (Xiao et al., 2014). In addition, in squamous cell carcinoma (SCC) non-melanoma skin cancer (NMSC), a higher expression of the channel was correlated with tumor size and poor tumor differentiation (Hemida et al., 2021).
The effects of TRPM8 channels in cancer prognosis are associated with modulation of cell viability, proliferation, migration, and apoptosis; phenomena demonstrated employing TRPM8 overexpression, knockdown, and channel regulation activity by agonist or antagonist (Figure 1). For instance, the upregulation of TRPM8 in colon cancer cells has been related to higher proliferation, migration, invasion, and metastasis of tumor cells. In contrast, channel inhibition reduces cell viability of colorectal carcinoma cell lines Caco-2 and HCT116 and promotes apoptosis (Tsavaler et al., 2001; Borrelli et al., 2014; Liu J. J. et al., 2022; Politi et al., 2022). A similar effect on cell proliferation was observed in gastric cancer (Xu et al., 2021). In oral squamous cell carcinoma (SCC), proliferation was not affected by channel activity; however, an important effect of TRPM8 in cell migration was observed, which was abolished by channel inhibition with antagonists. Interestingly, the cell migration level was lower than observed in control cells, suggesting that basally activated channels are involved in SCC migration (Okamoto et al., 2012). Esophageal cancer is another malignant tumor of the digestive system with a high incidence and mortality (Bray et al., 2018); in this kind of tumor, TRPM8 channels have an important effect in promoting cell viability and avoiding apoptosis (Lan et al., 2019). In bone cancer, pancreatic adenocarcinoma, glioblastoma, and bladder cancer, it was also demonstrated that TRPM8 promotes cell proliferation and migration. At the same time, its inhibition induces cell apoptosis (Yee et al., 2010; Wang et al., 2013; Alptekin et al., 2015; Liu J. F. et al., 2018; Zeng et al., 2019; Wang et al., 2020).
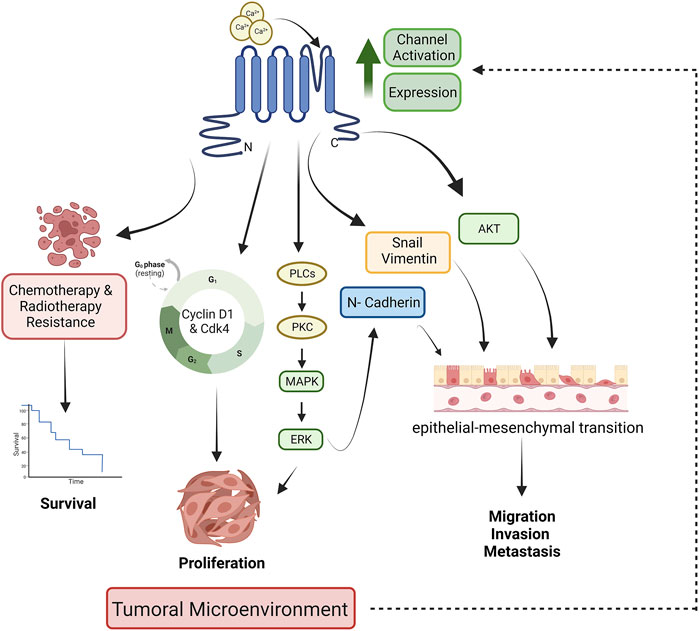
FIGURE 1. TRPM8-dependent mechanisms involved in tumor development. The tumoral microenvironment releases factors that increase the expression and activation of TRPM8 channels. Channel activation leads to the activation of signaling pathways whose activation decreases patient survival and increases proliferation, migration, invasiveness, and metastasis. Designed with biorender.
The effect of the channel on the growth, death, and apoptosis of bladder cancer cells was regulated by mitochondrial activity (Li et al., 2010; Xiao et al., 2014). It has been described that in glioblastoma, TRPM8 channels modulate the expression of apoptosis-related factors through regulation of the p38/MAPK (mitogen-activated protein kinases) pathway (Alptekin et al., 2015; Zeng et al., 2019). By using Lewis lung cancer (LLC), with different expression ratios of TRPM8 and TRPA1, it was observed that cells with a similar expression of TRPM8 and TRPA1 channels had less autophagy, greater migration, and greater invasiveness than cells with higher or lower TRPM8 expression. The previous results suggest that expression levels of TRPM8 are relevant in lung cancer (Du et al., 2014). Moreover, autophagy mediates breast cancer proliferation and migration by regulating TRPM8 expression and activity. TRPM8 overexpression and activation promote autophagy, while downregulation of the channel decreases it (Huang et al., 2020). In addition, wound healing and transwell assays demonstrated that high expression of TRPM8 promotes a fast movement and invasive ability in breast cancer cells (Liu J. et al., 2014).
In prostate cancer (PCa) cells, the expression of the TRPM8 channel is controlled by androgens via androgen receptor (AR) activation. This regulation occurs in lipid rafts microdomains where TRPM8 channels interact with the AR in a testosterone-dependent way, leading to translocation from the endoplasmic reticulum to the plasma membrane (Henshall et al., 2003; Bidaux et al., 2007). At initial PCa stages, the channel has a strong expression, which is lost in late and more aggressive advanced stages (Schmidt et al., 2006; Lunardi et al., 2021). The role of TRPM8 channels in prostate cancer is still controversial due to reports showing both pro-proliferative/antiproliferative and pro-apoptotic/anti-apoptotic effects of the channel. It was proposed that this discrepancy is related to differences in the androgen-sensitivity of cells as TRPM8 promotes proliferation in PCa androgen-sensitive cells LNCaP, but decreases proliferation in androgen-insensitive cells PC3 and DU145 (Yang et al., 2009; Zhu et al., 2011; Wang et al., 2012; Asuthkar et al., 2015b; Grolez and Gkika, 2016). However, channel inhibition or knockdown decreases proliferation in LNCaP, DU145, and PC3 cells, demonstrating a pro-proliferation role of TRPM8 channels independently of androgen-sensitivity (Valero et al., 2012; Liu T. et al., 2018). Anti-apoptotic role of the channel was observed in androgen-dependent LNCaP cells where channel activation promotes the expression of p53 and Caspase-9. On the other hand, a pro-apoptotic effect was demonstrated in both androgen-dependent and androgen-independent cells (Zhang and Barritt, 2004; Wang et al., 2012; Peng et al., 2015). Dissimilar results suggest that additional factors modulate the cell response to TRPM8 channel activity. The tumoral microenvironment has been proposed to modulate diverse cell processes, including ion channel activity (Pethő et al., 2019). It is feasible to consider that it could affect the channel response in prostate cancer.
TRPM8 channels modulate molecules involved in diverse mechanisms and pathways signaling related to the regulation of cell cycle progression, motility, and invasiveness, among others (Adiga et al., 2022). In addition to Ca2+, TRPM8 opening trigger the influx of Na+ and K+ ions which are essential for the control of diverse process involved in cancer progression as proliferation, cell volume regulation and apoptosis (Zhang and Barritt, 2006; Huang and January 2014). However, considering the higher permeability of Ca2+ ions, most of the literature about the role of the TRPM8 channel in cancer is focused on Ca2+ signaling (McKemy et al., 2002). In osteosarcoma and glioblastoma, TRPM8 promotes cell proliferation through the modulation of cyclin D1 and cyclin-dependent kinase 4 (Cdk4) expression (Wang et al., 2013; Alptekin et al., 2015; Zeng et al., 2019). In pancreatic tissue and diverse cell lines, it was demonstrated that TRPM8 overexpression has a role in cell proliferation by upregulating cyclin-dependent kinase inhibitors expression and promoting cell cycle progression (Yee et al., 2010). Knockdown of TRPM8 induced senescence and a significant decrease in cell proliferation. It was also observed that an increased proportion of cells in the G0/G1 phases and a decrease in the S and G2/M phases (Yee et al., 2010; Yee et al., 2012a; Liu J. F. et al., 2018). In addition, Yee et al. (2012a), Yee et al. (2012b) suggested that TRPM8 activation promotes an increase in intracellular Ca2+ concentration, leading to the activation of phospholipase C (PLC) and protein kinase C (PKC), which in turn activate the RAS/ERK pathway and regulate the transcription of diverse genes involved in cellular proliferation and survival (Yee et al., 2012a). The effect of the TRPM8 channel in PCa cells proliferation is related to the modulation of Cdk4 and Cdk6 expression (Yang et al., 2009; Yang et al., 2009; Wang et al., 2012; Asuthkar et al., 2015b; Gkika et al., 2015; Grolez et al., 2019a; Chinigò et al., 2022; Grolez et al., 2022).
In the colon, oral, esophageal, bladder, and breast cancers, have been described that TRPM8 is directly involved in invasiveness and metastasis by regulation of epithelial-mesenchymal transition (EMT; Liu J. J. et al., 2022; Chen et al., 2017; Wang et al., 2020; Liu J. et al., 2014). EMT is a process where epithelial cells lose their apical polarity and adopt a mesenchymal phenotype, thereby increasing properties such as migration and invasiveness as well as resistance to apoptosis, which are directly involved in cancer metastasis. This process involves changes in the expression of mesenchymal markers, including the calcium-dependent membrane proteins N-cadherin and E-cadherin, as well as vimentin and snail, among others (Kaufhold and Bonavida, 2014; Liu et al., 2015). E-cadherin participates in preserving the epithelial phenotype. In contrast, N-cadherin is prevalent in non-epithelial tissues, where it facilitates the activation of MAPK/extracellular signal-regulated kinases (ERK) and phosphoinositide-3-kinase (PI3K) pathways to enhance cell survival and migration in non-epithelial cells (Mrozik et al., 2018; Loh et al., 2019). In addition, proteins involved in cytoskeleton remodeling and cell adhesion, such as vimentin and snail, are also upregulated in EMT. Upregulation of N-cadherin, vimentin, and snail, along with the downregulation of E-cadherin, promotes cancer development by increasing cell migration and metastasis (Kaufhold and Bonavida, 2014; Loh et al., 2019). By transfecting siRNA-TRPM8 in SW480 (human colon cancer cell line) and CT26 (mouse colorectal adenocarcinoma cell line) and tissues samples from patients with colon cancer and liver metastasis, it was observed that TRPM8 upregulates vimentin, snail an N-cadherin while decreasing the expression of E-cadherin. Moreover, silencing of TRPM8 leads to the inhibition of EMT and decreases the proliferation, migration, invasion, and metastasis of colon cancer cells, proving the importance of the channel in these processes (Liu J. J. et al., 2022). Changes in vimentin and snail expression were also reported in bladder cancer in addition to the increase in the expression of b-catenin and paxillin, which are involved in the control of EMT markers and cell adhesion, respectively (Tumbarello et al., 2005; Kim et al., 2019; Wen et al., 2020).
AKT/GSK3-β pathway has an important role in the regulation of the mesenchymal markers (vimentin, snail, and E-cadherin) and the EMT process (Chen et al., 2013; Liu L. et al., 2014). GSK3-β participates in the degradation of some proteins, including snail1 and smad, which inhibit activation of EMT, being critical for tumorigenicity (Gonzalez and Medici, 2014; Nishimura et al., 2016). Activation of AKT signaling inhibits GSK-3β by phosphorylation and promotes EMT (Zhou et al., 2004; Bachelder et al., 2005; Kitagishi et al., 2012; Gonzalez and Medici, 2014; Loh et al., 2019). In bladder, breast, and bone cancer, it was reported that TRPM8 modulates AKT/GSK-3β signaling (Wang et al., 2013; Liu J. et al., 2014; Wang et al., 2020). The proposed mechanism suggests that TRPM8 promotes AKT phosphorylation which decreases GSK-3β and increases snail and vimentin expression and E-cadherin degradation (Liu J. et al., 2014). Knockdown of TRPM8 in bladder cancer cells decreases p-AKT and increases p-GSK-3β level, which alters the process regulated by the AKT/GSK-3β signaling (Wang et al., 2020).
Expression of membrane matrix metalloproteinases (MMPs) is increased in EMT to mediate the remodeling and degradation of extracellular matrix and promote cell invasion and metastasis (Kessenbrock et al., 2010; Pang et al., 2016). MMPs are overexpressed in the late stage of some cancer like head and neck SCC (Antra et al., 2022; Chan et al., 2022). In oral, bone, and prostate cancer, TRPM8 upregulates the activity of MMP2 and MMP9, stimulating invasiveness (Okamoto et al., 2012; Wang et al., 2013). Furthermore, MMPs can degrade E-cadherin and promote EMT (Gonzalez and Medici, 2014). The extracellular-signal-regulated kinase (ERK) one and two are proteins that belong to the family of MAPK and contribute to EMT (Olea-Flores et al., 2019). In diverse cancers, levels of p-ERK1/2 were correlated with upregulation of vimentin, snail, N-cadherin, and MMP-9, with a decrease in E-cadherin expression (Hong et al., 2016; Liu B. et al., 2018). In bone, bladder, prostate cancer, and glioblastoma, TRPM8 regulates the expression of ERK1/2. Knockdown of the channel decreased ERK phosphorylation together with migration and invasion (Wang et al., 2013; Alptekin et al., 2015; Liu T. et al., 2018; Zeng et al., 2019; Wang et al., 2020).
The programmed cell death one ligand (PD-L1) signaling regulates T cell activation to induce immune evasion and promote EMT in diverse cancers (Datar and Schalper, 2016; Kim et al., 2016; Chen et al., 2017; Raimondi et al., 2017; Aghajani et al., 2020). Upregulation of snail and vimentin and downregulation of E-cadherin is positively correlated with PD-L1 overexpression, suggesting a direct relation between PD-L1 and EMT (Datar and Schalper, 2016). In esophagus cancer, TRPM8 mediates the activation of the calcineurin-NFATc3 signaling pathway, which stimulate the increase in PD-L1 expression (Lan et al., 2019). PD-L1 is significantly associated with esophageal cancer progression as it promotes cell viability, proliferation, migration, and metastasis through the decreasing of anti-tumor response to CD8+T that promotes immune system evasion (Brahmer et al., 2012; Chen et al., 2017; Han et al., 2020). It was also demonstrated that PD-L1 overexpression enhanced the expression of mesenchymal markers related to the EMT phenotype. Furthermore, PD-L1 blockade inhibited tumor progression (Mcdermott and Atkins, 2013; Chen et al., 2017; Lan et al., 2019). PD-L1 has been proposed as a prognostic predictor for human esophageal cancer (Chen et al., 2014; Yagi et al., 2019), and modulating TRPM8 channel activity with antagonist could be a pharmacological strategy to regulate PD-L1 effects in esophageal cells. The described effects of TRPM8 are related to the role of the channel in Ca2+ signaling from calcium influx and release from intracellular stores. Changes in the intracellular Ca2+ concentration regulate diverse cell processes involved in cancer progression, through the activation or inhibition of cytosolic enzymes that regulate transcription factors, and proteins involved in cell cycle regulation and EMT (Shibasaki et al., 1997; Wang et al., 1999; Kim et al., 2011; Garg et al., 2014; Iamshanova et al., 2017; Adiga et al., 2022).
In pancreatic adenocarcinoma, TRPM8 channels are associated with TRPM8 channel-associated factors 1 and 2 (TCAF1 and TCAF2). TCAF1 and two promote TRPM8 trafficking to the cell surface and modulate the channel activity. The analysis of protein interactions demonstrated that TRPM8 channels are physically connected with TCAF2, which has an inhibitory role on channel activity, promoting prostate cancer cell migration. However, it was not observed that physical interaction with TCAF1 was reported to reduce prostate cancer cell migration (Gkika et al., 2015; Chelaru et al., 2022). TRPM8 and TCAF2 expression levels in pancreatic cancer cells have been associated with cancer invasiveness, metastasis, and tumoral stage (Chelaru et al., 2022).
Modulation of TRPM8 activity by agonists and antagonists has also been associated with the enhanced antitumoral activity of some chemotherapeutics. It was demonstrated that the combination of the channel antagonist AMTB with cisplatin (one of the most used chemotherapeutics in osteosarcoma treatment) has a higher antitumoral effect than cisplatin alone. Treatment with both molecules promotes a significant reduction of tumor size by arresting tumor cell growth and metastasis together with the induction of apoptosis (Liu Y. et al., 2022). In addition, the knockdown of the TRPM8 channel did not affect apoptosis but facilitated the apoptosis induced by the anti-tumor molecule epirubicin (EPI), suggesting a role of the channel in epirubicin resistance (Wang et al., 2013). Furthermore, in lung cancer, the enhanced efficiency of the chemotherapeutic doxorubicin (DOX) was reported in A549 cells treated with the TRPM8 agonist borneol. The proposed mechanism involves the activation of intracellular TRPM8 channels, which trigger intracellular Ca2+ mobilization. In addition, the role of TRPM8 channels in apoptosis induced by borneol and DOX was reported, mediated by the overproduction of reactive oxygen species (ROS; Lai et al., 2020). On the contrary, TRPM8 expression decreases pancreatic cancer cell sensitivity to the chemotherapeutic gemcitabine, which is associated with changes in the expression of proteins responsible for multidrug resistance, apoptosis, and gemcitabine metabolism (Liu J. F. et al., 2018).
Radioresistance has been observed after radiation therapy, and TRPM8 contributes to the radioresistance of B16 melanoma cells. TRPM8 expression increases in B16 melanoma cells after g-irradiation, which improves DNA damage response (DDR) for DNA repair and cell recovery. Channel inhibition with the antagonist AMTB or TRPM8 knockdown decreased the tumor growth induced by g-irradiation by suppressing DDR and promoting radio sensitizing. In addition, channel activation with the agonist W-12 increased the survival rate of cancer cells (Nomura et al., 2021). Similar effects were observed in glioblastoma, where TRPM8 participates in cancer cell resistance. Klumpp et al., 2017 reported that ionizing radiation upregulates TRPM8 in glioblastoma and promotes DNA repair and clonogenic survival by cell cycle control and prevention of apoptosis (Klumpp et al., 2017).
Changes in the cell microenvironment observed in cancer progression include modifications in mechanical signals, which can be regulated by ion channels (Butcher et al., 2009; Pethő et al., 2019). In addition, Wei et al. (2015) proved that increased matrix stiffness in the tumor microenvironment directly activates EMT, tumor invasion, and metastasis through the EMT-inducing transcription factor TWIST1 (Wei et al., 2015). TRPM8 has a role in the mechanosensitive response of breast epithelial cells which could be dysregulated by the activation of KRas oncogene. In normal breast epithelial cells, mechanical stimulation induces rapid activation of NADPH-oxidase 2 (NOX2) and the production of ROS. These events promote TRPM8 channel activation and a persistent extracellular calcium influx. Overexpression of the constitutively active KRAs oncogene modifies the Ca2+ signaling derived from mechanical stimulation. It reduces the ability of cells to respond to ROS, which could modify cancer cells’ responses to the mechanical microenvironment and affect patient survival (Pratt et al., 2020). In bladder cancer, TRMP8 also regulates ROS production and the expression levels of the enzymes Catalase, HO-1, and SOD2, which regulate tumor growth in vivo, demonstrating that TRPM8 has an essential role in bladder cancer (Wang et al., 2020).
Hypoxia is closely associated with cell proliferation, angiogenesis, metabolism, and the tumor immune response (Li et al., 2021). TRPM8 channel overexpression or activation promotes tumor growth under hypoxic conditions in prostate cancer, an effect facilitated by the upregulation of HIF-1α protein through Ca2+-dependent inhibition of RACK1(Yu et al., 2014). TRPM8 is preferentially expressed in the plasma membrane in non-cancer cells. However, in tumoral tissues, the channel is removed from the plasma membrane and targeted for degradation. Inhibition of the ubiquitination cascade increases the activity of the TRPM8 channel in the plasma membrane which has been proposed as a therapeutic strategy for regulating cell growth and proliferation in prostate cancer cells (Asuthkar et al., 2015b; Asuthkar et al., 2017).
It was demonstrated that pancreatic cancer cells express a non-glycosylated form of TRPM8 channel with biophysical properties different from other cells. Interestingly, that variant inhibits pancreatic adenocarcinoma cell migration and proliferation, contrary to the reported TRPM8 channel effect, suggesting an important role of post-translational modifications in cancer development (Cucu et al., 2014; Ulăreanu et al., 2017). In addition, Huang et al., 2022 demonstrated the importance of Y1022 phosphorylation in cancer progression. Y1022, ubicated in the C-terminal of TRPM8, is phosphorylated by lymphocyte-specific protein tyrosine kinase (LCK), which modulates channel multimerization and increases the TRPM8 current density. In AsPC-1 and PANC-1 pancreatic cell lines, mutant TRPM8 Y1022F fails to increase cell proliferation and migration, demonstrating the importance of that residue in pancreatic cancer cell proliferation, migration, and tumorigenesis in vitro and in vivo. In that sense, the authors suggest that channel regulation by LKC may be a potential therapeutic target for pancreatic cancer (Huang et al., 2022).
Therapeutic potential of TRPM8 in cancer
TRPM8 has been proposed as a therapeutic target for cancer treatment because of its demonstrated role in cancer development and progression. In the last years, diverse molecules have been proven to have in vitro and in vivo effects on the TRPM8 channel activity. Moreover, some of them have been tested in preclinical and clinical trials (Knowlton and Mckemy, 2011; Liu et al., 2016; Pérez de Vega et al., 2016). This section reviews TRPM8 channel agonists and antagonists, which have been demonstrated to regulate cellular characteristics associated with diverse cancers. We also review information from assays focused on the potential effect of channel modulation in cancer treatment.
TRPM8 agonists modulate various properties in cancer cells, and menthol is one of the most extensively used pharmacological studies (Izquierdo et al., 2021). Menthol is an FDA GRAS (generally regarded as safe) molecule without known considerable adverse effects. In human malignant melanoma cell lines G-361 and A-375, menthol induces a dose-dependent increase in intracellular Ca2+ concentration and promotes a cytotoxic effect (Yamamura et al., 2008; Kijpornyongpan et al., 2014). The menthol-induced effect on A-375 cells was comparable to that induced by 5-fluorouracil and was abolished by the antagonist BCTC (Kijpornyongpan et al., 2014). In T24 human bladder cancer cells, menthol increases the intracellular Ca2+ concentration, mitochondrial membrane depolarization, and cell death in a dose-dependent way, suggesting the potential use of TRPM8 agonists in the cancer cell (Li et al., 2010). Contrary to these reports, in glioblastoma cells (DBTRG) have been demonstrated that menthol promotes cell migration and stimulates both Ca2+ influx and release from intracellular stores in a dose-dependent effect (Wondergem et al., 2008; Wondergem and Bartley, 2009). Similarly, in glioblastoma cell lines U-87MG and T98G were observed that the TRPM8 agonist icilin increased chemotaxis (Klumpp et al., 2017). In both cases, the effect of channel agonists was inhibited by the TRPM8 antagonist 2-Aminoethoxydiphenyl borate (2-APB) and BCTC, respectively (Wondergem et al., 2008; Wondergem and Bartley, 2009; Klumpp et al., 2017). The differential observed effect could be related to the divergent roles of the channel in cancer treatment. A proliferative effect of the channel was observed in some cancers, including breast, pancreas, glioblastoma, and bone cancer; a pro-apoptotic effect was demonstrated in prostate cancer.
Even though the cytotoxic effects induced by menthol in cancer cells, there are no reports from clinical trials using menthol as a therapeutic target in cancer treatment. Considering the role of TRPM8 channels in pain signaling, the clinical trials with menthol have been focused on pain treatment (Fernández-carvajal et al., 2020). For instance, the clinical trial NCT05429814 proposed to evaluate topical menthol in chemotherapy-related peripheral neuropathy in patients with breast cancer. In addition, by applying the TRPM8 agonist in patients with neuropathic pain, Fallon et al., 2015 suggested that topical menthol could potentially treat neuropathic pain derived from chemotherapy (Fallon et al., 2015). Both reports suggest the use of a topical formulation of menthol, which reduces the occurrence of adverse effects. However, it could limit the effectiveness of using these molecules for cancer treatment. Another critical thing restricting the use of menthol as a cancer target is its unselective effect because it has been reported that it can modulate other ion channels (Hansen et al., 2017).
D3263 has been described as a selective TRPM8 agonist. Preclinical studies analyzed the effect of oral administration of D3263 alone or in combination with Finasteride, an FDA-approved pharmacologic agent for treating benign prostate hyperplasia (BPH; Duncan et al., 2009; Tacklind et al., 2010). Results demonstrate a dose-response reduction in mean rat prostate hyperplasia, and an additive effect with Finasteride, suggesting D3263 for BPH treatment (Duncan et al., 2009; Moran et al., 2011). Significantly, in 2009, a Phase I clinical trial (NCT00839631) was carried out to test the effect of D3262 on solid tumors, including prostate, colon, breast, lung, pancreas, leiomyosarcoma, and Kaposi’s sarcoma. After oral administration of D3262 to 23 participants, all patients were reported to feel a cold sensation (like a menthol sensation) on the skin and mucous membranes. Preliminary results of the trial revealed that three men with advanced prostate cancer showed evidence of disease stabilization (Tolcher et al., 2010). Although the study was finished, no clinical results were reported or recent information about the effect of these molecules on cancer progression.
TRPM8 channel antagonist is also proposed as a potential alternative in cancer treatment from results derived from studies with osteosarcoma, bladder, and colorectal cancer among others. Wang et al., 2020 demonstrate that TRPM8 inhibition with BCTC promotes a reduction in cell viability, proliferation, and migration in bladder cancer cells (Wang et al., 2020). Similarly, the TRPM8 antagonist AMTB decreases cell proliferation and migration and promotes apoptosis of osteosarcoma cell lines. The described mechanism suggests that AMTB affects Smad2 and Smad3 phosphorylation and represses the TGFb pathway (Liu Y. et al., 2022). Cannabigerol (CBG), cannabidiol (CBD), and cannabidivarin (CBDV) are cannabinoids with antagonist effects on TRPM8 channels. In vitro and in vivo assays demonstrated that all of them decrease colorectal cancer cell viability and reduce cell growth (De Petrocellis et al., 2013; Borrelli et al., 2014; Mangal et al., 2021). Borrelli et al. (2014) demonstrated a mechanism associated with apoptosis induction and ROS production in a concentration-dependent way. The TRPM8 antagonist AMTB reproduced a lesser potent effect on cell viability. Furthermore, mRNA TRPM8 silencing decreased the effect of CBG on cell viability (Borrelli et al., 2014). Similarly, a CBD pro-apoptotic effect, p53 activation, and ROS elevation were observed in prostate carcinoma (De Petrocellis et al., 2013). These results lead the authors to suggest the use of non-THC cannabinoids as therapeutic strategies for colorectal cancer and prostate carcinoma (De Petrocellis et al., 2013; Borrelli et al., 2014; Mangal et al., 2021). In esophageal cancer cells, the addition of the TRPM8 antagonist RQ-00203078 decreases cancer proliferation and cell viability by apoptosis induction (Lan et al., 2019). Similarly, oral squamous carcinoma cells treated with the antagonist RQ-00203078 showed a decrease in cell migration. In addition, the antagonist reduced invading ability by suppressing the MMP activity. Considering the importance of invasiveness in oral SCC, channel inhibition could be a potential treatment for that cancer (Okamoto et al., 2012).
There is contradictory information about the effect of TRPM8 modulation in prostate cancer, as both agonists and antagonists have been shown to decrease proliferation and migration and promote apoptosis in PCa cells in vitro (Grolez and Gkika, 2016). That effect has been associated with the differences in channel expression during cancer progression. High expression is found in the initial cancer stages, and loss of expression is observed in the late and more aggressive stages (Henshall et al., 2003). In addition, it was demonstrated that the TRPM8 expression level depends on the cell line. Androgen-dependent LNCaP and androgen-independent cells PC-3 and DU145 showed different expression levels of the TRPM8 channel (Henshall et al., 2003).
Recently, using the androgen-independent cell line PC3 with stable heterologous expression of TRPM8 channels (PC3-M8), it was reported that TRPM8 activation limited tumor growth by decreasing clonogenicity and cell proliferation through cell cycle arrest at the G0/G1 phase. Furthermore, by using the agonist WS12 inside lipid nanocapsules, the authors demonstrate that TRPM8 activation decreases cell migration, and metastasis, suggesting a protective role of the channel on prostate cancer progression (Grolez et al., 2019b; Grolez et al., 2022). Invasiveness was also inhibited through the decrease in the EMT by Rac1 and Cdc42 inhibition (Grolez et al., 2022). The effects of TRPM8 inhibition on cell migration demonstrated the nanocarriers’ efficiency in delivering the channel agonist in vivo. In addition to the protective effect of TRPM8 in prostate cancer, it was demonstrated the advantage of using nanocarriers on the improved activity of the channel agonist, as encapsulation decreased the concentration required to activate the channel. It is suggested that channel activation with TRPM8 agonist in nanocarriers could be used in the early stages of prostate cancer to decrease tumor cell dissemination (Grolez et al., 2019b; Grolez et al., 2022). In DU145 cells, menthol induced a drop in cell migration and inhibited cell growth without apoptosis. After treatment with menthol, a decrease in cell proliferation and a substantial increase in G0/G1 phase were observed. Furthermore, menthol induces the downregulation of the CDK2, CDK4, and CDK6, involved in cell progression, and phosphor-FAK, which participates in cell migration. These effects were partially abolished by the addition of the TRPM8 channel antagonist BCTC (Wang et al., 2012).
In another way, the positive effect of TRPM8 antagonists for prostate cancer treatment was demonstrated using the androgen-dependent prostate cancer cells LNCaP. Donato et al., 2021 analyzed several molecules and reported that TRPM8 channel antagonists inhibit proliferation, migration, and invasiveness of the androgen-dependent prostate cancer cells LNCaP cells. TRPM8 antagonists reverse the androgen-induced increase in calcium influx and the rapid signaling activation that led to the androgen-elicited invasion and proliferation in various PC-derived cells. Furthermore, LNCaP migration and invasion stimulated by androgens were decreased by channel antagonists (Donato et al., 2021). Sesamin, a molecule from Traditional Chinese medicine, has a selective dose-dependent antagonist effect on the TRPM8 channel. In vitro assays demonstrated that Sesamin inhibited cell growth in prostate adenocarcinoma cells LNCaP and DU145 through an antiproliferative effect. However, in vivo effects have not been reported (Sui et al., 2020). These results suggest that the effect of channel antagonists or agonists depends on the type of prostate cells, androgen-dependent or androgen-independent. However, it was reported that antagonist AMTB decreased the proliferation and migration of both androgen-dependent and androgen-independent prostate cancer cells, demonstrating that there is an additional mechanism controlling the response of pancreatic cancer cells to TRPM8 channel modulation (Valero et al., 2012).
Combination therapy uses two or more therapeutic agents for cancer treatment (Mokhtari et al., 2017), and there are exciting reports about the potential use of TRPM8 modulators combined with currently used chemotherapeutics. In osteosarcoma, an increased sensitivity to cisplatin was observed when combined with AMTB, suggesting that channel inhibition increase the antitumoral effect of the chemotherapeutic. Similar results were found in a preclinical, experimental model of osteosarcoma (Liu Y. et al., 2022). Following that, knocking down TRPM8 channels in osteosarcoma and prostate cancer cell lines increased apoptosis induced by epirubicin, suggesting an enhancement in chemosensitivity (Wang et al., 2013; Liu T. et al., 2018). In aggressive prostate cancer cells was demonstrated that the agonist D-3263 significantly improves the pro-apoptotic effect of enzalutamide and docetaxel, antiandrogen and antineoplastic, respectively, used in the treatment of prostate cancer (Genovesi et al., 2022).
Furthermore, Nagai et al. (2019) reported that menthol enhances the effect of anti-cancer drugs Paclitaxel and Vincristine on human hepatocellular carcinoma HepG2 cells where cell viability was significantly decreased through downregulation of CYP3A4 (Nagai et al., 2019). Although the reported mechanism is not related to TRPM8 channel activity, it is possible that the increase in intracellular Ca2+ concentration, derived from channel activation, led to the modification of the CYP3A4 expression, as has been reported for other cytochromes (Clyne et al., 1996). Radiotherapy is currently used in treating several cancers, and it has been proposed that combining this therapy with TRPM8 modulation could be helpful in cancer therapy. When prostate cancer cells were stimulated with ionizing radiation combined with the TRPM8 agonist WS-12, a significant induction of apoptosis was observed, suggesting that channel activation improves the effect of radiation therapy (Alaimo et al., 2020); Alaimo et al., 2021).
From analyzing the TRPM8 expression, drug activities, and known drug targets, Pan et al., 2022 reported a correlation between the expression of the TRPM8 channel and the response to Paclitaxel (Pan et al., 2022). Paclitaxel is a chemotherapeutic used in diverse cancers which acts as a mitotic inhibitor by interfering with the normal function of microtubule growth. Paclitaxel induces an increase in ROS production and stimulates signaling pathways as p38/MAPK, related to apoptosis induction. In addition, it mediates the activation of regulatory proteins Bad and Bax, which promote apoptosis, and Bcl2, which suppresses apoptosis (Kampan et al., 2015; Zhu and Chen, 2019). In human prostate carcinoma, it was demonstrated that TRPM8 channel activation with TRPM8 agonists promotes apoptosis, an effect mediated by an increase in intracellular Ca2+ concentration (Zhang and Barritt, 2004; Alaimo et al., 2020).
Furthermore, human prostate carcinoma cells treated with the agonist WS-12 plus docetaxel, an analog of paclitaxel (Pienta, 2001), showed significant activation of apoptotic cell death (Alaimo et al., 2020). Interestingly, paclitaxel promotes a substantial increase in TRPM8 protein expression in IB4(−) neurons, which contributes to heat and cold hypersensitivity observed in chemotherapy-induced peripheral neuropathy (CIPN) induced by paclitaxel. When the channel was inhibited with the antagonist AMTB, the cold-hyperalgesia was reduced (Villalba-Riquelme et al., 2022). Considering these results, it could be interesting to carry on specific assays to evaluate the precise role of TRPM8 in response to paclitaxel in cancer prostate and other cancer cells.
TRPM8 was linked to the effect of other chemotherapeutics, including Lapatinib, Erlotinib, Afatinib, and Dabrafenib, which are approved for treating diverse cancers (Pan et al., 2022; Zhang et al., 2022). FDA approved Afatinib, Dabrafenib, and Erlotinib to treat non-small cell lung cancer (NSCLC; Rosell et al., 2012; Hirsh, 2017; Hu et al., 2017; Yuan et al., 2022). In addition, Afatinib has been proposed for breast and gastric cancer (Lin et al., 2012; Hirsh, 2017; Hu et al., 2017; Zhu et al., 2021); Dabrafenib is reported for the treatment of patients with advanced melanoma and colorectal cancer (Yuan et al., 2022), and Lapatinib is used in the treatment of breast cancer (Segovia-Mendoza et al., 2015; Eustace et al., 2018). Those molecules are tyrosine kinase inhibitors of the epidermal growth factor receptor (EGFR) and ErbB-family that regulate growth inhibition, apoptosis, and autophagy through the modulation of diverse pathways, including PI3K-Akt, the Ras-Raf-MEK-ERK1/2 and the phospholipase C (PLC) pathway (Segovia-Mendoza et al., 2015; Suzawa et al., 2016; Hirsh, 2017; Hu et al., 2017; Sekhon et al., 2017; Khunger et al., 2018; Yuan et al., 2022). ERK1/ERK2 and associated pathways have been reported to be modulated by TRPM8 expression in bone cancer (Wang et al., 2013), bladder cancer (Wang et al., 2020), pancreatic cancer (Yee et al., 2012a) and glioblastoma (Alptekin et al., 2015). In addition, in pancreatic cancer (Yee et al., 2012a) and breast cancer (Pratt et al., 2020), there were shown changes in Ras expression induced by TRPM8 activity. More profound studies are necessary, focusing on the relationship between the TRPM8 channel and the activity of chemotherapeutics currently used in cancer treatment to get an improved therapy using FDA-approved drugs.
There is increasing information about the role of TRPM8 modulation in cancer. However, to our knowledge, there are no TRPM8 modulators FDA-approved for cancer treatment. One important factor that restricts promissory molecules’ selection is channel selectivity. Molecules such as menthol or icilin, two of the most common agonists of TRPM8 channels, and the antagonist BCTC have been demonstrated to activate other members of the TRP family (Hansen et al., 2017; Heber et al., 2020). Another significant consideration is the development of undesired side effects observed in the early phases of clinical trials (Izquierdo et al., 2021). For example, some studies have demonstrated changes in core body temperature regulation and shifts in temperature sensation after using TRPM8 channel modulators (Andrews et al., 2015). In this way, developing drug delivery technology as nanocarriers used by Grolez et al. (2019a), Grolez et al. (2019b) for biodistribution of TRPM8 modulators could improve treatment efficacy and minimize side effects (Din et al., 2017; Grolez et al., 2019b). Finally, an important focus is the possibility of using both TRPM8 agonists and antagonists for cancer treatment (Pérez de Vega et al., 2016). More research is needed to decipher the complex modulatory effect of TRPM8 in the development and progress of diverse cancers, focusing on characterizing molecules highly selective for the TRPM8 channel that could be used in cancer treatment with minimal adverse effects.
Author contributions
SO, ZC, SA, JS and YT wrote the manuscript and approved the submitted version.
Funding
Pontificia Universidad Javeriana, al Ministerio de Ciencia, tecnología e Innovación, al Ministerio de Educación Nacional, al Ministerio de Industria, Comercio y Turismo e ICETEX, 2ª Convocatoria Ecosistema científico – Colombia Científica 792-2017, Programa “Generación de alternativas terapéuticas en cáncer a partir de plantas a través de procesos de investigación y desarrollo traslacional articulados en sistemas de valor sostenibles ambiental y económicamente” (Contrato no. FP44842-221-2018). Vicerrectoría de Investigación, Pontificia Universidad Javeriana, Grant ID 7928 (Caracterización electrofisiológica de células pancreáticas obtenidas a partir de la diferenciación de células mesenquimales de pulpa dental).
Conflict of interest
The authors declare that the research was conducted in the absence of any commercial or financial relationships that could be construed as a potential conflict of interest.
Publisher’s note
All claims expressed in this article are solely those of the authors and do not necessarily represent those of their affiliated organizations, or those of the publisher, the editors and the reviewers. Any product that may be evaluated in this article, or claim that may be made by its manufacturer, is not guaranteed or endorsed by the publisher.
References
Adiga, D., Radhakrishnan, R., Chakrabarty, S., Kumar, P., and Kabekkodu, S. P. (2022). The role of calcium signaling in regulation of epithelial-mesenchymal transition. Cells Tissues Organs 211, 134–156. doi:10.1159/000512277
Aghajani, M. J., Yang, T., Schmitz, U., James, A., McCafferty, C. E., de Souza, P., et al. (2020). Epithelial-to-mesenchymal transition and its association with PD-L1 and CD8 in thyroid cancer. Endocr. Connect. 9, 1028–1041. doi:10.1530/EC-20-0268
Alaimo, A., Felice, D. D., Genovesi, S., Lorenzoni, M., and Lunardi, A. (2021). Tune the channel: TRPM8 targeting in prostate cancer. Oncoscience 8, 97–100. doi:10.18632/oncoscience.543
Alaimo, A., Lorenzoni, M., Ambrosino, P., Bertossi, A., Bisio, A., Macchia, A., et al. (2020). Calcium cytotoxicity sensitizes prostate cancer cells to standard-of-care treatments for locally advanced tumors. Cell. Death Dis. 11, 1039. doi:10.1038/s41419-020-03256-5
Alptekin, M., Eroglu, S., Tutar, E., Sencan, S., Geyik, M. A., Ulasli, M., et al. (2015). Gene expressions of TRP channels in glioblastoma multiforme and relation with survival. Tumor Biol. 36, 9209–9213. doi:10.1007/s13277-015-3577-x
Andersson, D. A., Chase, H. W. N., and Bevan, S. (2004). TRPM8 activation by menthol, icilin, and cold is differentially modulated by intracellular pH. J. Neurosci. 24, 5364–5369. doi:10.1523/JNEUROSCI.0890-04.2004
Andrews, M. D., Af Forselles, K., Beaumont, K., Galan, S. R. G., Glossop, P. A., Grenie, M., et al. (2015). Discovery of a selective TRPM8 antagonist with clinical efficacy in cold-related pain. ACS Med. Chem. Lett. 6, 419–424. doi:10.1021/ml500479v
Antra, Parashar P., Hungyo, H., Jain, A., Ahmad, S., and Tandon, V. (2022). Unraveling molecular mechanisms of head and neck cancer. Crit. Rev. Oncol. Hematol. 178, 103778. doi:10.1016/j.critrevonc.2022.103778
Asuthkar, S., Demirkhanyan, L., Mueting, S. R., Cohen, A., and Zakharian, E. (2017). High-throughput proteome analysis reveals targeted TRPM8 degradation in prostate cancer. Oncotarget 8, 12877–12890. doi:10.18632/oncotarget.14178
Asuthkar, S., Elustondo, P. A., Demirkhanyan, L., Sun, X., Baskaran, P., Velpula, K. K., et al. (2015a). The TRPM8 protein is a testosterone receptor: I. Biochemical evidence for direct TRPM8-testosterone interactions. J. Biol. Chem. 290, 2659–2669. doi:10.1074/jbc.M114.610824
Asuthkar, S., Velpula, K. K., Elustondo, P. A., Demirkhanyan, L., and Zakharian, E. (2015b). TRPM8 channel as a novel molecular target in androgen-regulated prostate cancer cells. Oncotarget 6, 17221–17236. doi:10.18632/oncotarget.3948
Bachelder, R. E., Yoon, S. O., Franci, C., García De Herreros, A., and Mercurio, A. M. (2005). Glycogen synthase kinase-3 is an endogenous inhibitor of Snail transcription: Implications for the epithelial - mesenchymal transition. J. Cell. Biol. 168, 29–33. doi:10.1083/jcb.200409067
Bandell, M., Dubin, A. E., Petrus, M. J., Orth, A., Mathur, J., Sun, W. H., et al. (2006). High-throughput random mutagenesis screen reveals TRPM8 residues specifically required for activation by menthol. Nat. Neurosci. 9, 493–500. doi:10.1038/nn1665
Bautista, D. M., Siemens, J., Glazer, J. M., Tsuruda, P. R., Basbaum, A. I., Stucky, C. L., et al. (2007). The menthol receptor TRPM8 is the principal detector of environmental cold. Nature 448, 204–208. doi:10.1038/nature05910
Bidaux, G., Flourakis, M., Thebault, S., Zholos, A., Beck, B., Gkika, D., et al. (2007). Prostate cell differentiation status determines transient receptor potential melastatin member 8 channel subcellular localization and function. J. Clin. Invest. 117, 1647–1657. doi:10.1172/JCI30168
Borrelli, F., Pagano, E., Romano, B., Panzera, S., Maiello, F., Coppola, D., et al. (2014). Colon carcinogenesis is inhibited by the TRPM8 antagonist cannabigerol, a Cannabis-derived non-psychotropic cannabinoid. Carcinogenesis 35, 2787–2797. doi:10.1093/carcin/bgu205
Brahmer, J. R., Tykodi, S. S., Chow, L. Q. M., Hwu, W.-J., Topalian, S. L., Hwu, P., et al. (2012). Safety and activity of anti–PD-L1 antibody in patients with advanced cancer. N. Engl. J. Med. 366, 2455–2465. doi:10.1056/nejmoa1200694
Bray, F., Ferlay, J., Soerjomataram, I., Siegel, R. L., Torre, L. A., and Jemal, A. (2018). Global cancer statistics 2018: GLOBOCAN estimates of incidence and mortality worldwide for 36 cancers in 185 countries. Ca. Cancer J. Clin. 68, 394–424. doi:10.3322/caac.21492
Butcher, D. T., Alliston, T., and Weaver, V. M. (2009). A tense situation: Forcing tumour progression. Nat. Rev. Cancer 9, 108–122. doi:10.1038/nrc2544
Ceylan, G. G., Önalan, E. E., Kuloğlu, T., Aydoğ, G., Keleş, İ., Tonyali, Ş., et al. (2016). Potential role of melastatin-related transient receptor potential cation channel subfamily M gene expression in the pathogenesis of urinary bladder cancer. Oncol. Lett. 12, 5235–5239. doi:10.3892/ol.2016.5359
Chan, L. P., Tseng, Y. P., Wang, H. C., Chien, C. Y., Wu, C. W., Wang, L. F., et al. (2022). Growth regulated oncogene-α contribute to EMT/MMPs pathway by binding its receptors in head and neck squamous cell carcinoma. Life Sci. 306, 120791. doi:10.1016/j.lfs.2022.120791
Chelaru, N. R., Chiosa, A., Sorop, A., Spiridon, A., Cojocaru, F., Domocos, D., et al. (2022). The association between TRP channels expression and clinicopathological characteristics of patients with pancreatic adenocarcinoma. Int. J. Mol. Sci. 23, 9045. doi:10.3390/ijms23169045
Chen, L., Deng, H., Lu, M., Xu, B., Wang, Q., Jiang, J., et al. (2014). B7-H1 expression associates with tumor invasion and predicts patient’s survival in human esophageal cancer. Int. J. Clin. Exp. Pathol. 7, 6015–6023.
Chen, L., Xiong, Y., Li, J., Zheng, X., Zhou, Q., Turner, A., et al. (2017). PD-L1 expression promotes epithelial to mesenchymal transition in human esophageal cancer. Cell. Physiol. biochem. 42, 2267–2280. doi:10.1159/000480000
Chen, W. F., Gao, W. D., Li, Q. L., Zhou, P. H., Xu, M. D., and Yao, L. Q. (2013). SLIT2 inhibits cell migration in colorectal cancer through the AKT-GSK3β signaling pathway. Int. J. Colorectal Dis. 28, 933–940. doi:10.1007/s00384-013-1641-9
Chinigò, G., Grolez, G. P., Audero, M., Bokhobza, A., Bernardini, M., Cicero, J., et al. (2022). TRPM8-Rap1A interaction sites as critical determinants for adhesion and migration of prostate and other epithelial cancer cells. Cancers (Basel) 14, 2261. doi:10.3390/cancers14092261
Chodon, D., Guilbert, A., Dhennin-Duthille, I., Gautier, M., Telliez, M.-S., Sevestre, H., et al. (2010). Estrogen regulation of TRPM8 expression in breast cancer cells. BMC Cancer 10, 212. doi:10.1186/1471-2407-10-212
Chuang, H. H., Neuhausser, W. M., and Julius, D. (2004). The super-cooling agent icilin reveals a mechanism of coincidence detection by a temperature-sensitive TRP channel. Neuron 43, 859–869. doi:10.1016/j.neuron.2004.08.038
Clyne, C. D., White, P. C., and Rainey, W. E. (1996). Calcium regulates human CYP11B2 transcription. Endocr. Res. 22, 485–492. doi:10.1080/07435809609043735
Cucu, D., Chiritoiu, G., Petrescu, S., Babes, A., Stanica, L., Duda, D. G., et al. (2014). Characterization of functional transient receptor potential melastatin 8 channels in human pancreatic ductal adenocarcinoma cells. Pancreas 43, 795–800. doi:10.1097/MPA.0000000000000106
Datar, I., and Schalper, K. A. (2016). Epithelial-mesenchymal transition and immune evasion during lung cancer progression: The chicken or the egg? Clin. Cancer Res. 22, 3422–3424. doi:10.1158/1078-0432.CCR-16-0336
De Petrocellis, L., Ligresti, A., Schiano Moriello, A., Iappelli, M., Verde, R., Stott, C. G., et al. (2013). Non-THC cannabinoids inhibit prostate carcinoma growth in vitro and in vivo: Pro-apoptotic effects and underlying mechanisms. Br. J. Pharmacol. 168, 79–102. doi:10.1111/j.1476-5381.2012.02027.x
De Petrocellis, L., Starowicz, K., Moriello, A. S., Vivese, M., Orlando, P., and Di Marzo, V. (2007). Regulation of transient receptor potential channels of melastatin type 8 (TRPM8): Effect of cAMP, cannabinoid CB1 receptors and endovanilloids. Exp. Cell. Res. 313, 1911–1920. doi:10.1016/j.yexcr.2007.01.008
Dhennin-Duthille, I., Gautier, M., Faouzi, M., Guilbert, A., Brevet, M., Vaudry, D., et al. (2011). High expression of transient receptor potential channels in human breast cancer epithelial cells and tissues: Correlation with pathological parameters. Cell. Physiol. biochem. 28, 813–822. doi:10.1159/000335795
Din, F. U., Aman, W., Ullah, I., Qureshi, O. S., Mustapha, O., Shafique, S., et al. (2017). Effective use of nanocarriers as drug delivery systems for the treatment of selected tumors. Int. J. Nanomedicine 12, 7291–7309. doi:10.2147/IJN.S146315
Dinakar, Y. H., Karole, A., Parvez, S., Jain, V., and Mudavath, S. L. (2022). Organ-restricted delivery through stimuli-responsive nanocarriers for lung cancer therapy. Life Sci. 310, 121133. doi:10.1016/j.lfs.2022.121133
Donato, M. D., Ostacolo, C., Giovannelli, P., Sarno, V. D., Monterrey, I. M. G., Campiglia, P., et al. (2021). Therapeutic potential of TRPM8 antagonists in prostate cancer. Sci. Rep. 11, 23232. doi:10.1038/s41598-021-02675-4
Du, G. J., Li, J. H., Liu, W. J., Liu, Y. H., Zhao, B., Li, H. R., et al. (2014). The combination of TRPM8 and TRPA1 expression causes an invasive phenotype in lung cancer. Tumor Biol. 35, 1251–1261. doi:10.1007/s13277-013-1167-3
Du, J. D., Zheng, X., Chen, Y. L., Huang, Z. Q., Cai, S. W., Jiao, H. B., et al. (2018). Elevated transient receptor potential melastatin 8 (Trpm8) expression is correlated with poor prognosis in pancreatic cancer. Med. Sci. Monit. 24, 3720–3725. doi:10.12659/MSM.909968
Duncan, D., Stewart, F., Frohlich, M., and Urdal, D. (2009). Preclinical evaluation of the Trpm8 ion channel agonist D-3263 for benign prostatic hyperplasia. J. Urol. 181, 503. doi:10.1016/s0022-5347(09)61422-1
Eustace, A. J., Conlon, N. T., McDermott, M. S. J., Browne, B. C., O’Leary, P., Holmes, F. A., et al. (2018). Development of acquired resistance to lapatinib may sensitise HER2-positive breast cancer cells to apoptosis induction by obatoclax and TRAIL. BMC Cancer 18, 965. doi:10.1186/s12885-018-4852-1
Fallon, M. T., Storey, D. J., Krishan, A., Weir, C. J., Mitchell, R., Fleetwood-Walker, S. M., et al. (2015). Cancer treatment-related neuropathic pain: Proof of concept study with menthol—a TRPM8 agonist. Support. Care Cancer 23, 2769–2777. doi:10.1007/s00520-015-2642-8
Fernández-carvajal, A., González-muñiz, R., Fernández-, G., and Ferrer-montiel, A. (2020). Investigational drugs in early phase clinical trials targeting thermotransient receptor potential (thermoTRP) channels. Expert Opin. Investig. Drugs 29, 1209–1222. doi:10.1080/13543784.2020.1825680
Garg, R., Benedetti, L. G., Abera, M. B., Wang, H., Abba, M., and Kazanietz, M. G. (2014). Protein kinase C and cancer: What we know and what we do not. Oncogene 33, 5225–5237. doi:10.1038/onc.2013.524
Genovesi, S., Moro, R., Vignoli, B., De Felice, D., Canossa, M., Montironi, R., et al. (2022). Trpm8 expression in human and mouse castration resistant prostate adenocarcinoma paves the way for the preclinical development of TRPM8-based targeted therapies. Biomolecules 12, 193. doi:10.3390/biom12020193
Gkika, D., Lemonnier, L., Shapovalov, G., Gordienko, D., Poux, C., Bernardini, M., et al. (2015). TRP channel-associated factors are a novel protein family that regulates TRPM8 trafficking and activity. J. Cell. Biol. 208, 89–107. doi:10.1083/jcb.201402076
Gonzalez, D. M., and Medici, D. (2014). Signaling mechanisms of the epithelial-mesenchymal transition. Sci. Signal. 7, re8. doi:10.1126/scisignal.2005189
Grolez, G. P., Hammadi, M., Barras, A., Gordienko, D., Slomianny, C., Völkel, P., et al. (2019b). Encapsulation of a TRPM8 agonist, WS12, in lipid nanocapsules potentiates PC3 prostate cancer cell migration inhibition through channel activation. Sci. Rep. 9, 7926. doi:10.1038/s41598-019-44452-4
Grolez, G. P., Chinigò, G., Barras, A., Hammadi, M., Noyer, L., Kondratska, K., et al. (2022). TRPM8 as an anti–tumoral target in prostate cancer growth and metastasis dissemination. Int. J. Mol. Sci. 23, 6672. doi:10.3390/ijms23126672
Grolez, G. P., and Gkika, D. (2016). TRPM8 puts the chill on prostate cancer. Pharmaceuticals 9, 44. doi:10.3390/ph9030044
Grolez, G. P., Gordiendko, D. V., Clarisse, M., Hammadi, M., Desruelles, E., Fromont, G., et al. (2019a). TRPM8-androgen receptor association within lipid rafts promotes prostate cancer cell migration. Cell. Death Dis. 10, 652. doi:10.1038/s41419-019-1891-8
Han, Y., Liu, D., and Li, L. (2020). PD-1/PD-L1 pathway: Current researches in cancer. Am. J. Cancer Res. 10, 727–742.
Hansen, E. O., Arendt-Nielsen, L., and Boudreau, S. A. (2017). A comparison of oral sensory effects of three TRPA1 agonists in young adult smokers and non-smokers. Front. Physiol. 8, 663. doi:10.3389/fphys.2017.00663
Hantute-Ghesquier, A., Haustrate, A., Prevarskaya, N., and Lehen’kyi, V. (2018). TRPM family channels in cancer. Pharmaceuticals 11, 58–14. doi:10.3390/ph11020058
Hashemi, M., Hasani, S., Hajimazdarany, S., Mirmazloomi, S. R., Makvandy, S., Zabihi, A., et al. (2022). Non-coding RNAs targeting notch signaling pathway in cancer: From proliferation to cancer therapy resistance. Int. J. Biol. Macromol. 222, 1151–1167. doi:10.1016/j.ijbiomac.2022.09.203
Heber, S., Ciotu, C. I., Hartner, G., Gold-Binder, M., Ninidze, N., Gleiss, A., et al. (2020). TRPV1 antagonist BCTC inhibits pH 6.0-induced pain in human skin. Pain 161, 1532–1541. doi:10.1097/j.pain.0000000000001848
Hemida, A. S., Hammam, M. A., Heriz, N. A. E. M., and Shehata, W. A. (2021). Expression of transient receptor potential channel of melastatin number 8 (TRPM8) in non- melanoma skin cancer: A clinical and immunohistochemical study. J. Immunoass. Immunochem. 42, 620–632. doi:10.1080/15321819.2021.1918709
Henao, J. C., Grismaldo, A., Barreto, A., Rodríguez-Pardo, V. M., Mejía-Cruz, C. C., Leal-Garcia, E., et al. (2021). TRPM8 channel promotes the osteogenic differentiation in human bone marrow mesenchymal stem cells. Front. Cell. Dev. Biol. 9, 592946. doi:10.3389/fcell.2021.592946
Henshall, S. M., Afar, D. E. H., Hiller, J., Horvath, L. G., Quinn, D. I., Rasiah, K. K., et al. (2003). Survival analysis of genome-wide gene expression profiles of prostate cancers identifies new prognostic targets of disease relapse. Cancer Res. 63, 4196–4203.
Hirsh, V. (2017). New developments in the treatment of advanced squamous cell lung cancer: Focus on afatinib. Onco. Targets. Ther. 10, 2513–2526. doi:10.2147/OTT.S104177
Hong, S. K., Park, J. R., Kwon, O. S., Kim, K. T., Bae, G. Y., and Cha, H. J. (2016). Induction of integrin β3 by sustained ERK activity promotes the invasiveness of TGFβ-induced mesenchymal tumor cells. Cancer Lett. 376, 339–346. doi:10.1016/j.canlet.2016.04.012
Hu, X., Shi, S., Wang, H., Yu, X., Wang, Q., Jiang, S., et al. (2017). Blocking autophagy improves the anti-Tumor activity of afatinib in lung adenocarcinoma with activating EGFR mutations in vitro and in vivo. Sci. Rep. 7, 4559. doi:10.1038/s41598-017-04258-8
Huang, X., and Jan, L. Y. (2014). Targeting potassium channels in cancer. J. Cell. Biol. 206 (2), 151–162. doi:10.1083/jcb.201404136
Huang, Y., Li, S., Jia, Z., Zhao, W., Zhou, C., Zhang, R., et al. (2020). Transient receptor potential melastatin 8 (TRPM8) channel regulates proliferation and migration of breast cancer cells by activating the AMPK-ULK1 pathway to enhance basal autophagy. Front. Oncol. 10, 573127. doi:10.3389/fonc.2020.573127
Huang, Y., Li, S., Liu, Q., Wang, Z., Li, S., Liu, L., et al. (2022). The LCK-14-3-3ζ-TRPM8 axis regulates TRPM8 function/assembly and promotes pancreatic cancer malignancy. Cell. Death Dis. 13, 524. doi:10.1038/s41419-022-04977-5
Iamshanova, O., Fiorio Pla, A., and Prevarskaya, N. (2017). Molecular mechanisms of tumour invasion: Regulation by calcium signals. J. Physiol. 595, 3063–3075. doi:10.1113/JP272844
Izquierdo, C., Mart, M., and Isabel, G. (2021). TRPM8 channels: Advances in structural studies and pharmacological modulation. Int. J. Mol. Sci. 22 (16), 8502. doi:10.3390/ijms22168502
Janssens, A., and Voets, T. (2011). Ligand stoichiometry of the cold- and menthol-activated channel TRPM8. J. Physiol. 589, 4827–4835. doi:10.1113/jphysiol.2011.216523
Kampan, N. C., Madondo, M. T., McNally, O. M., Quinn, M., and Plebanski, M. (2015). Paclitaxel and its evolving role in the management of ovarian cancer. Biomed. Res. Int. 2015, 413076. doi:10.1155/2015/413076
Kato, Y., Ozawa, S., Miyamoto, C., Maehata, Y., Suzuki, A., Maeda, T., et al. (2013). Acidic extracellular microenvironment and cancer. Cancer Cell. Int. 13, 89. doi:10.1186/1475-2867-13-89
Kaufhold, S., and Bonavida, B. (2014). Central role of Snail1 in the regulation of EMT and resistance in cancer: A target for therapeutic intervention. J. Exp. Clin. Cancer Res. 33, 62–19. doi:10.1186/s13046-014-0062-0
Kayama, Y., Shibata, M., Takizawa, T., Ibata, K., Nakahara, J., Shimizu, T., et al. (2017). Signaling pathways relevant to nerve growth factor-induced upregulation of transient receptor potential M8 expression. Neuroscience 367, 178–188. doi:10.1016/j.neuroscience.2017.10.037
Kessenbrock, K., Plaks, V., and Werb, Z. (2010). Matrix metalloproteinases: Regulators of the tumor microenvironment. Cell. 141, 52–67. doi:10.1016/j.cell.2010.03.015
Khunger, A., Khunger, M., and Velcheti, V. (2018). Dabrafenib in combination with trametinib in the treatment of patients with BRAF V600-positive advanced or metastatic nonsmall cell lung cancer: Clinical evidence and experience. Ther. Adv. Respir. Dis. 12, 1753466618767611–1753466618767619. doi:10.1177/1753466618767611
Kijpornyongpan, T., Sereemaspun, A., and Chanchao, C. (2014). Dose-dependent cytotoxic effects of menthol on human malignant melanoma A-375 cells: Correlation with TRPM8 transcript expression. Asian Pac. J. Cancer Prev. 15, 1551–1556. doi:10.7314/APJCP.2014.15.4.1551
Kim, J., Thorne, S. H., Sun, L., Huang, B., and Mochly-Rosen, D. (2011). Sustained inhibition of PKCα reduces intravasation and lung seeding during mammary tumor metastasis in an in vivo mouse model. Oncogene 30, 323–333. doi:10.1038/onc.2010.415
Kim, S., Koh, J., Kim, M. Y., Kwon, D., Go, H., Kim, Y. A., et al. (2016). PD-L1 expression is associated with epithelial-to-mesenchymal transition in adenocarcinoma of the lung. Hum. Pathol. 58, 7–14. doi:10.1016/j.humpath.2016.07.007
Kim, W. K., Kwon, Y., Jang, M., Park, M., Kim, J., Cho, S., et al. (2019). Β-catenin activation down-regulates cell-cell junction-related genes and induces epithelial-to-mesenchymal transition in colorectal cancers. Sci. Rep. 9, 18440. doi:10.1038/s41598-019-54890-9
Kitagishi, Y., Kobayashi, M., Kikuta, K., and Matsuda, S. (2012). Roles of PI3K/AKT/GSK3/mTOR pathway in cell signaling of mental illnesses. Depress. Res. Treat. 2012, 752563. doi:10.1155/2012/752563
Klumpp, D., Frank, S. C., Klumpp, L., Sezgin, E. C., Eckert, M., Edalat, L., et al. (2017). TRPM8 is required for survival and radioresistance of glioblastoma cells. Oncotarget 8, 95896–95913. doi:10.18632/oncotarget.21436
Knowlton, W. M., and Mckemy, D. D. (2011). TRPM8: From cold to cancer, peppermint to pain. Peppermint Pain 12, 68–77. doi:10.2174/138920111793937961
Koivisto, A. P., Belvisi, M. G., Gaudet, R., and Szallasi, A. (2022). Advances in TRP channel drug discovery: From target validation to clinical studies. Nat. Rev. Drug Discov. 21, 41–59. doi:10.1038/s41573-021-00268-4
Lai, H., Liu, C., Hou, L., Lin, W., Chen, T., Hong, A., et al. (2020). TRPM8-regulated calcium mobilization plays a critical role in synergistic chemosensitization of Borneol on Doxorubicin. Theranostics 10, 10154–10170. doi:10.7150/thno.45861
Lan, X., Zhao, J., Song, C., Yuan, Q., and Liu, X. (2019). TRPM8 facilitates proliferation and immune evasion of esophageal cancer cells. Biosci. Rep. 39, BSR20191878–12. doi:10.1042/BSR20191878
Li, Q., Wang, X., Yang, Z., Wang, B., and Li, S. (2010). Menthol induces cell death via the TRPM8 channel in the human bladder cancer cell line T24. Oncology 77, 335–341. doi:10.1159/000264627
Li, Y., Zhao, L., and Li, X. F. (2021). Hypoxia and the tumor microenvironment. Technol. Cancer Res. Treat. 20, 15330338211036304–15330338211036309. doi:10.1177/15330338211036304
Lin, N. U., Winer, E. P., Wheatley, D., Carey, L. A., Houston, S., Mendelson, D., et al. (2012). A phase II study of afatinib (BIBW 2992), an irreversible ErbB family blocker, in patients with HER2-positive metastatic breast cancer progressing after trastuzumab. Breast Cancer Res. Treat. 133, 1057–1065. doi:10.1007/s10549-012-2003-y
Liu, B., and Qin, F. (2005). Functional control of cold- and menthol-sensitive TRPM8 ion channels by phosphatidylinositol 4,5-bisphosphate. J. Neurosci. 25, 1674–1681. doi:10.1523/JNEUROSCI.3632-04.2005
Liu, B., Xing, X., Li, X., Guo, Q., Xu, T., and Xu, K. (2018). ZNF259 promotes breast cancer cells invasion and migration via ERK/GSK3β/snail signaling. Cancer Manag. Res. 10, 3159–3168. doi:10.2147/CMAR.S174745
Liu, C. Y., Lin, H. H., Tang, M. J., and Wang, Y. K. (2015). Vimentin contributes to epithelial-mesenchymal transition cancer cell mechanics by mediating cytoskeletal organization and focal adhesion maturation. Oncotarget 6, 15966–15983. doi:10.18632/oncotarget.3862
Liu, J., Chen, Y., Shuai, S., Ding, D., Li, R., and Luo, R. (2014). TRPM8 promotes aggressiveness of breast cancer cells by regulating EMT via activating AKT/GSK-3β pathway. Tumor Biol. 35, 8969–8977. doi:10.1007/s13277-014-2077-8
Liu, J. F., Hu, G. H., Gong, Y. J., Yu, Q. L., He, B., Li, W. H., et al. (2018). Silencing of TRPM8 inhibits aggressive tumor phenotypes and enhances gemcitabine sensitivity in pancreatic cancer. Pancreatology 18, 935–944. doi:10.1016/j.pan.2018.08.011
Liu, J. J., Li, L. Z., and Xu, P. (2022). Upregulation of TRPM8 can promote the colon cancer liver metastasis through mediating Akt/GSK-3 signal pathway. Biotechnol. Appl. Biochem. 69, 230–239. doi:10.1002/bab.2102
Liu, L., Dai, Y., Chen, J., Zeng, T., Li, Y., Chen, L., et al. (2014). Maelstrom promotes hepatocellular carcinoma metastasis by inducing epithelial-mesenchymal transition by way of Akt/GSK-3β/Snail signaling. Hepatology 59, 531–543. doi:10.1002/hep.26677
Liu, T., Liao, Y., Tao, H., Zeng, J., Wang, G., Yang, Z., et al. (2018). RNA interference-mediated depletion of TRPM8 enhances the efficacy of epirubicin chemotherapy in prostate cancer LNCaP and PC3 cells. Oncol. Lett. 15, 4129–4136. doi:10.3892/ol.2018.7847
Liu, Y., Leng, A., Li, L., Yang, B., Shen, S., Chen, H., et al. (2022). AMTB, a TRPM8 antagonist, suppresses growth and metastasis of osteosarcoma through repressing the TGFβ signaling pathway. Cell. Death Dis. 13, 288. doi:10.1038/s41419-022-04744-6
Liu, Y., Mikrani, R., He, Y., Faran Ashraf Baig, M. M., Abbas, M., Naveed, M., et al. (2020). TRPM8 channels: A review of distribution and clinical role. Eur. J. Pharmacol. 882, 173312. doi:10.1016/j.ejphar.2020.173312
Liu, Z., Wu, H., Wei, Z., Wang, X., Shen, P., Wang, S., et al. (2016). TRPM8: A potential target for cancer treatment. J. Cancer Res. Clin. Oncol. 142, 1871–1881. doi:10.1007/s00432-015-2112-1
Loh, C. Y., Chai, J. Y., Tang, T. F., Wong, W. F., Sethi, G., Shanmugam, M. K., et al. (2019). The e-cadherin and n-cadherin switch in epithelial-to-mesenchymal transition: Signaling, therapeutic implications, and challenges. Cells 8 (10), 1118. doi:10.3390/cells8101118
Lunardi, A., Barbareschi, M., Carbone, F. G., Morelli, L., Brunelli, M., Fortuna, N., et al. (2021). TRPM8 protein expression in hormone naïve local and lymph node metastatic prostate cancer. Pathologica 113, 95–101. doi:10.32074/1591-951X-262
Mangal, N., Erridge, S., Habib, N., Sadanandam, A., Reebye, V., and Hans, M. (2021). Cannabinoids in the landscape of cancer. J. Cancer Res. Clin. Oncol. 147, 2507–2534. doi:10.1007/s00432-021-03710-7
Mcdermott, D. F., and Atkins, M. B. (2013). PD-1 as a potential target in cancer therapy. Cancer Med. 2, 662–673. doi:10.1002/cam4.106
McKemy, D. D., Neuhausser, W. M., and Julius, D. (2002). Identification of a cold receptor reveals a general role for TRP channels in thermosensation. Nature 416, 52–58. doi:10.1038/nature719
Mergler, S., Derckx, R., Reinach, P. S., Garreis, F., Böhm, A., Schmelzer, L., et al. (2014). Calcium regulation by temperature-sensitive transient receptor potential channels in human uveal melanoma cells. Cell Signal 26 (1), 56–69. doi:10.1016/j.cellsig.2013.09.017
Mokhtari, R. B., Homayouni, T. S., Baluch, N., Morgatskaya, E., Kumar, S., Das, B., et al. (2017). Combination therapy in combating cancer systematic review: Combination therapy in combating cancer background. Oncotarget 8, 38022–38043. doi:10.18632/oncotarget.16723
Moran, M. M., Mcalexander, M. A., and Bíró, T. (2011). Transient receptor potential channels as therapeutic targets. Nat. Rev. Drug Discov. 10, 601–620. doi:10.1038/nrd3456
Mrozik, K. M., Blaschuk, O. W., Cheong, C. M., Zannettino, A. C. W., and Vandyke, K. (2018). N-cadherin in cancer metastasis, its emerging role in haematological malignancies and potential as a therapeutic target in cancer. BMC Cancer 18, 939. doi:10.1186/s12885-018-4845-0
Nagai, K., Fukuno, S., Omachi, A., Omotani, S., Hatsuda, Y., Myotoku, M., et al. (2019). Enhanced anti-cancer activity by menthol in HepG2 cells exposed to paclitaxel and vincristine: Possible involvement of CYP3A4 downregulation. Drug Metab. Pers. Ther. 34, 1–5. doi:10.1515/dmpt-2018-0029
Nishimura, H., Nakamura, O., Yamagami, Y., Mori, M., Horie, R., Fukuoka, N., et al. (2016). GSK-3 inhibitor inhibits cell proliferation and induces apoptosis in human osteosarcoma cells. Oncol. Rep. 35, 2348–2354. doi:10.3892/or.2016.4565
Nomura, D., Abe, R., and Tsukimoto, M. (2021). Involvement of TRPM8 channel in radiation-induced DNA damage repair mechanism contributing to radioresistance of B16 melanoma. Biol. Pharm. Bull. 44, 642–652. doi:10.1248/bpb.b20-00934
Okamoto, Y., Ohkubo, T., Ikebe, T., and Yamazaki, J. (2012). Blockade of TRPM8 activity reduces the invasion potential of oral squamous carcinoma cell lines. Int. J. Oncol. 40, 1431–1440. doi:10.3892/ijo.2012.1340
Olea-Flores, M., Zuñiga-Eulogio, M. D., Mendoza-Catalán, M. A., Rodríguez-Ruiz, H. A., Castañeda-Saucedo, E., Ortuño-Pineda, C., et al. (2019). Extracellular-signal regulated kinase: A central molecule driving epithelial–mesenchymal transition in cancer. Int. J. Mol. Sci. 20, 2885. doi:10.3390/ijms20122885
Pan, T., Gao, Y., Xu, G., Zhou, P., Li, S., Guo, J., et al. (2022). Pan-cancer analyses reveal the genetic and pharmacogenomic landscape of transient receptor potential channels. npj Genomic Med. 7, 32–11. doi:10.1038/s41525-022-00304-1
Pang, L., Li, Q., Li, S., He, J., Cao, W., Lan, J., et al. (2016). Membrane type 1-matrix metalloproteinase induces epithelial-to-mesenchymal transition in esophageal squamous cell carcinoma: Observations from clinical and in vitro analyses. Sci. Rep. 6, 22179. doi:10.1038/srep22179
Patel, S. H., Edwards, M. J., and Ahmad, S. A. (2019). Intracellular ion channels in pancreas cancer. Cell. Physiol. biochem. 53, 44–51. doi:10.33594/000000193
Peng, M., Wang, Z., Yang, Z., Tao, L., Liu, Q., Yi, L., et al. (2015). Overexpression of short TRPM8 variant α promotes cell migration and invasion, and decreases starvation-induced apoptosis in prostate cancer LNCaP cells. Oncol. Lett. 10, 1378–1384. doi:10.3892/ol.2015.3373
Pérez de Vega, M. J., Gómez-Monterrey, I., Ferrer-Montiel, A., and González-Muñiz, R. (2016). Transient receptor potential melastatin 8 channel (TRPM8) modulation: Cool entryway for treating pain and cancer. J. Med. Chem. 59, 10006–10029. doi:10.1021/acs.jmedchem.6b00305
Pethő, Z., Najder, K., Bulk, E., and Schwab, A. (2019). Mechanosensitive ion channels push cancer progression. Cell. Calcium 80, 79–90. doi:10.1016/j.ceca.2019.03.007
Phelps, C. B., and Gaudet, R. (2007). The role of the N terminus and transmembrane domain of TRPM8 in channel localization and tetramerization. J. Biol. Chem. 282, 36474–36480. doi:10.1074/jbc.M707205200
Pienta, K. J. (2001). Preclinical mechanisms of action of docetaxel and docetaxel combinations in prostate cancer. Semin. Oncol. 28, 3–7. doi:10.1016/s0093-7754(01)90148-4
Politi, M., Ferrante, C., Menghini, L., Angelini, P., Flores, G. A., Muscatello, B., et al. (2022). Hydrosols from rosmarinus officinalis, salvia officinalis, and cupressus sempervirens: Phytochemical analysis and bioactivity evaluation. Plants 11, 349. doi:10.3390/plants11030349
Pratt, S. J. P., Lee, R. M., Chang, K. T., Hernández-Ochoa, E. O., Annis, D. A., Ory, E. C., et al. (2020). Mechanoactivation of NOX2-generated ROS elicits persistent TRPM8 Ca2+signals that are inhibited by oncogenic KRas. Proc. Natl. Acad. Sci. U. S. A. 117, 26008–26019. doi:10.1073/pnas.2009495117
Raimondi, C., Carpino, G., Nicolazzo, C., Gradilone, A., Gianni, W., Gelibter, A., et al. (2017). PD-L1 and epithelial-mesenchymal transition in circulating tumor cells from non-small cell lung cancer patients: A molecular shield to evade immune system? Oncoimmunology 6, 1315488. doi:10.1080/2162402X.2017.1315488
Rivera, B., Moreno, C., Lavanderos, B., Hwang, J. Y., Fernández-Trillo, J., Park, K. S., et al. (2021). Constitutive phosphorylation as a key regulator of trpm8 channel function. J. Neurosci. 41, 8475–8493. doi:10.1523/JNEUROSCI.0345-21.2021
Rohács, T., Lopes, C. M. B., Michailidis, I., and Logothetis, D. E. (2005). PI(4,5)P2 regulates the activation and desensitization of TRPM8 channels through the TRP domain. Nat. Neurosci. 8, 626–634. doi:10.1038/nn1451
Rosell, R., Carcereny, E., Gervais, R., Vergnenegre, A., Massuti, B., Felip, E., et al. (2012). Erlotinib versus standard chemotherapy as first-line treatment for European patients with advanced EGFR mutation-positive non-small-cell lung cancer (eurtac): A multicentre, open-label, randomised phase 3 trial. Lancet Oncol. 13, 239–246. doi:10.1016/S1470-2045(11)70393-X
Schmidt, U., Fuessel, S., Koch, R., Baretton, G. B., Lohse, A., Toamsetti, S., et al. (2006). Quantitative multi-gene expression profiling of primary prostate cancer. Prostate 66, 1521–1534. doi:10.1002/pros.20490
Segovia-Mendoza, M., González-González, M. E., Barrera, D., Díaz, L., and García-Becerra, R. (2015). Efficacy and mechanism of action of the tyrosine kinase inhibitors gefitinib, lapatinib and neratinib in the treatment of her2-positive breast cancer: Preclinical and clinical evidence. Am. J. Cancer Res. 5, 2531–2561.
Sekhon, N., Kumbla, R. ., and Mita, M. (2017). “Current trends in cancer therapy,” in Cardio-Oncology: Principles, prevention and management (Amsterdam, Netherlands: Elsevier). doi:10.1016/B978-0-12-803547-4/00001-X
Shibasaki, F., Kondo, E., Akagi, T., and McKeon, F. (1997). Suppression of signalling through transcription factor NF-AT by interactions between calcineurin and Bcl-2. Nature 386, 728–731. doi:10.1038/386728a0
Siegel, R. L., Miller, K. D., Fuchs, H. E., and Jemal, A. (2021). Cancer statistics, 2021. Ca. Cancer J. Clin. 71, 7–33. doi:10.3322/caac.21654
Sui, Y., Li, S., Zhao, Y., Liu, Q., Qiao, Y., Feng, L., et al. (2020). Identi fi cation of a natural compound, sesamin, as a novel TRPM8 antagonist with inhibitory e ff ects on prostate adenocarcinoma. Fitoterapia 145, 104631. doi:10.1016/j.fitote.2020.104631
Suzawa, K., Toyooka, S., Sakaguchi, M., Morita, M., Yamamoto, H., Tomida, S., et al. (2016). Antitumor effect of afatinib, as a human epidermal growth factor receptor 2-targeted therapy, in lung cancers harboring HER2 oncogene alterations. Cancer Sci. 107, 45–52. doi:10.1111/cas.12845
Tacklind, J., Fink, H. A., Macdonald, R., Rutks, I., and Wilt, T. J. (2010). Finasteride for benign prostatic hyperplasia. Cochrane Database Syst. Rev. 2010, CD006015. doi:10.1002/14651858.CD006015.pub3
Tolcher, A., Patnaik, A., Papadopoulos, K., Mays, T., Stephan, T., Humble, D. J., et al. (2010). 376 Preliminary results from a Phase 1 study of D-3263 HCl, a TRPM8 calcium channel agonist, in patients with advanced cancer. Eur. J. Cancer Suppl. 8, 119. doi:10.1016/s1359-6349(10)72083-8
Tsavaler, L., Shapero, M. H., Morkowski, S., and Lauis, R. (2001). Trp-p8, a novel prostate-specific gene, is up-regulated in prostate cancer and other malignancies and shares high homology with transient receptor potential calcium channel proteins. Cancer Res. 61, 3760–3769.
Tsuruda, P. R., Julius, D., and Minor, D. L. (2006). Coiled coils direct assembly of a cold-activated TRP channel. Neuron 51, 201–212. doi:10.1016/j.neuron.2006.06.023
Tumbarello, D. A., Brown, M. C., Hetey, S. E., and Turner, C. E. (2005). Regulation of paxillin family members during epithelial-mesenchymal transformation: A putative role for paxillin delta. J. Cell. Sci. 118, 4849–4863. doi:10.1242/jcs.02615
Ulăreanu, R., Chiriţoiu, G., Cojocaru, F., Deftu, A., Ristoiu, V., Stănică, L., et al. (2017). N-glycosylation of the transient receptor potential melastatin 8 channel is altered in pancreatic cancer cells. Tumor Biol. 39, 1010428317720940–10. doi:10.1177/1010428317720940
Valero, M. L., Mello de Queiroz, F., Stühmer, W., Viana, F., and Pardo, L. (2012). TRPM8 ion channels differentially modulate proliferation and cell cycle distribution of normal and cancer prostate cells. PLoS One 7, e51825. doi:10.1371/journal.pone.0051825
Villalba-Riquelme, E., de la Torre-Martínez, R., Fernández-Carvajal, A., and Ferrer-Montiel, A. (2022). Paclitaxel in vitro reversibly sensitizes the excitability of IB4(−) and IB4(+) sensory neurons from male and female rats. Br. J. Pharmacol. 179, 3693–3710. doi:10.1111/bph.15809
Voets, T., Owsianik, G., Janssens, A., Talavera, K., and Nilius, B. (2007a). TRPM8 voltage sensor mutants reveal a mechanism for integrating thermal and chemical stimuli. Nat. Chem. Biol. 3, 174–182. doi:10.1038/nchembio862
Voets, T., Owsianik, G., and Nilius, B. (2007b). TRPM8. Handb. Exp. Pharmacol. 179, 329–344. doi:10.1007/978-3-540-34891-7_20
Wang, G., Cao, R., Qian, K., Peng, T., Yuan, L., Chen, L., et al. (2020). Trpm8 inhibition regulates the proliferation, migration and ros metabolism of bladder cancer cells. Onco. Targets. Ther. 13, 8825–8835. doi:10.2147/OTT.S257056
Wang, H. G., Pathan, N., Ethell, I. M., Krajewski, S., Yamaguchi, Y., Shibasaki, F., et al. (1999). Ca2+-Induced apoptosis through calcineurin dephosphorylation of BAD. Science 284, 339–343. doi:10.1126/science.284.5412.339
Wang, Y., Wang, X., Yang, Z., Zhu, G., Chen, D., and Meng, Z. (2012). Menthol inhibits the proliferation and motility of prostate cancer DU145 cells. Pathol. Oncol. Res. 18, 903–910. doi:10.1007/s12253-012-9520-1
Wang, Y., Yang, Z., Meng, Z., Cao, H., Zhu, G., Liu, T., et al. (2013). Knockdown of TRPM8 suppresses cancer malignancy and enhances epirubicin-induced apoptosis in human osteosarcoma cells. Int. J. Biol. Sci. 10, 90–102. doi:10.7150/ijbs.7738
Wei, S. C., Fattet, L., Tsai, J. H., Guo, Y., Pai, V. H., Majeski, H. E., et al. (2015). Matrix stiffness drives epithelial-mesenchymal transition and tumour metastasis through a TWIST1-G3BP2 mechanotransduction pathway. Nat. Cell. Biol. 17, 678–688. doi:10.1038/ncb3157
Wen, L., Zhang, X., Zhang, J., Chen, S., Ma, Y., Hu, J., et al. (2020). Paxillin knockdown suppresses metastasis and epithelial-mesenchymal transition in colorectal cancer via the ERK signalling pathway. Oncol. Rep. 44, 1105–1115. doi:10.3892/or.2020.7687
Wondergem, R., and Bartley, J. W. (2009). Menthol increases human glioblastoma intracellular Ca2+, BK channel activity and cell migration. J. Biomed. Sci. 16, 90–7. doi:10.1186/1423-0127-16-90
Wondergem, R., Ecay, T. W., Mahieu, F., Owsianik, G., and Nilius, B. (2008). HGF/SF and menthol increase human glioblastoma cell calcium and migration. Biochem. Biophys. Res. Commun. 372, 210–215. doi:10.1016/j.bbrc.2008.05.032
Xiao, N., Jiang, L. M., Ge, B., Zhang, T. Y., Zhao, X. K., and Zhou, X. (2014). Over-expression of TRPM8 is associated with poor prognosis in urothelial carcinoma of bladder. Tumor Biol. 35, 11499–11504. doi:10.1007/s13277-014-2480-1
Xu, Q., Kong, N., Zhang, J., Bai, N., Bi, J., and Li, W. (2021). Expression of transient receptor potential cation channel subfamily M member 8 in gastric cancer and its clinical significance. Exp. Ther. Med. 21, 377. doi:10.3892/etm.2021.9808
Yagi, T., Baba, Y., Okadome, K., Kiyozumi, Y., Hiyoshi, Y., Ishimoto, T., et al. (2019). Tumour-associated macrophages are associated with poor prognosis and programmed death ligand 1 expression in oesophageal cancer. Eur. J. Cancer 111, 38–49. doi:10.1016/j.ejca.2019.01.018
Yamamura, H., Ugawa, S., Ueda, T., Morita, A., and Shimada, S. (2008). TRPM8 activation suppresses cellular viability in human melanoma. Am. J. Physiol. - Cell. Physiol. 295, 296–301. doi:10.1152/ajpcell.00499.2007
Yang, Z. H., Wang, X. H., Wang, H. P., and Hu, L. Q. (2009). Effects of TRPM8 on the proliferation and motility of prostate cancer PC-3 cells. Asian J. Androl. 11, 157–165. doi:10.1038/aja.2009.1
Yee, N. S. (2015). Roles of TRPM8 ion channels in cancer: Proliferation, survival, and invasion. Cancers (Basel) 7, 2134–2146. doi:10.3390/cancers7040882
Yee, N. S. (2016). TRPM8 ion channels as potential cancer biomarker and target in pancreatic cancer. 1st ed. Amsterdam, Netherlands: Elsevier. doi:10.1016/bs.apcsb.2016.01.001
Yee, N. S., Brown, R. D., Lee, M. S., Zhou, W., Jensen, C., Gerke, H., et al. (2012a). TRPM8 ion channel is aberrantly expressed and required for preventing replicative senescence in pancreatic adenocarcinoma: Potential role of TRPM8 as a biomarker and target. Cancer Biol. Ther. 13, 592–599. doi:10.4161/cbt.20079
Yee, N. S., Chan, A. S., Yee, J. D., and Yee, R. K. (2012b). TRPM7 and TRPM8 ion channels in pancreatic adenocarcinoma: Potential roles as cancer biomarkers and targets. Sci. (Cairo) 2012, 415158–8. doi:10.6064/2012/415158
Yee, N. S., Li, Q., Kazi, A. A., Yang, Z., Berg, A., and Yee, R. K. (2014). Aberrantly over-expressed TRPM8 channels in pancreatic adenocarcinoma: Correlation with tumor Size/Stage and requirement for cancer cells invasion. Cells 3, 500–516. doi:10.3390/cells3020500
Yee, N. S., Zhou, W., and Lee, M. L. (2010). Transient receptor potential channel TRPM8 is over-expressed and required for cellular proliferation in pancreatic adenocarcinoma. Cancer Lett. 297, 49–55. doi:10.1016/j.canlet.2010.04.023
Yu, S., Xu, Z., Zou, C., Wu, D., Wang, Y., Yao, X., et al. (2014). Ion channel TRPM8 promotes hypoxic growth of prostate cancer cells via an O2-independent and RACK1-mediated mechanism of HIF-1α stabilization. J. Pathol. 234, 514–525. doi:10.1002/path.4413
Yuan, J., Lv, T., Yang, J., Wu, Z., Yan, L., Yang, J., et al. (2022). The lipid transporter HDLBP promotes hepatocellular carcinoma metastasis through BRAF-dependent epithelial-mesenchymal transition. Cancer Lett. 549, 215921. doi:10.1016/j.canlet.2022.215921
Zakharian, E., Cao, C., and Rohacs, T. (2010). Gating of transient receptor potential melastatin 8 (TRPM8) channels activated by cold and chemical agonists in planar lipid bilayers. J. Neurosci. 30, 12526–12534. doi:10.1523/JNEUROSCI.3189-10.2010
Zeng, J., Wu, Y., Zhuang, S., Qin, L., Hua, S., Mungur, R., et al. (2019). Identification of the role of TRPM8 in glioblastoma and its effect on proliferation, apoptosis and invasion of the U251 human glioblastoma cell line. Oncol. Rep. 42, 1517–1526. doi:10.3892/or.2019.7260
Zhang, L., and Barritt, G. J. (2004). Evidence that TRPM8 is an androgen-dependent Ca2+ channel required for the survival of prostate cancer cells. Cancer Res. 64, 8365–8373. doi:10.1158/0008-5472.CAN-04-2146
Zhang, L., and Barritt, G. J. (2006). TRPM8 in prostate cancer cells: A potential diagnostic and prognostic marker with a secretory function? Endocrine-Related Cancer 13 (1), 27–38. doi:10.1677/erc.1.01093
Zhang, W., Qiao, X. Y., Li, Q., Cui, C., Qiao, C. M., Shen, Y. Q., et al. (2022). Comprehensive pan-cancer analysis of TRPM8 in tumor metabolism and immune escape. Front. Oncol. 12, 914060–16. doi:10.3389/fonc.2022.914060
Zhao, W., and Xu, H. (2016). High expression of TRPM8 predicts poor prognosis in patients with osteosarcoma. Oncol. Lett. 12, 1373–1379. doi:10.3892/ol.2016.4764
Zheng, J., Liu, F., Du, S., Li, M., Wu, T., Tan, X., et al. (2019). Mechanism for regulation of melanoma cell death via activation of thermo-TRPV4 and TRPV2. J. Oncol., 7362875. eCollection 2019. doi:10.1155/2019/7362875
Zhou, B. P., Deng, J., Xia, W., Xu, J., Li, Y. M., Gunduz, M., et al. (2004). Dual regulation of Snail by GSK-3beta-mediated phosphorylation in control of epithelial-mesenchymal transition. Nat. Cell. Biol. 6, 931–940. doi:10.1038/ncb1173
Zhu, G., Wang, X., Yang, Z., Cao, H., Meng, Z., Wang, Y., et al. (2011). Effects of TRPM8 on the proliferation and angiogenesis of prostate cancer PC-3 cells in vivo. Oncol. Lett. 2, 1213–1217. doi:10.3892/ol.2011.410
Zhu, L., and Chen, L. (2019). Progress in research on paclitaxel and tumor immunotherapy. Cell. Mol. Biol. Lett. 24, 40–11. doi:10.1186/s11658-019-0164-y
Keywords: TRPM8, cancer, molecular target, signaling pathway, TRPM8 pharmacology
Citation: Ochoa SV, Casas Z, Albarracín SL, Sutachan JJ and Torres YP (2023) Therapeutic potential of TRPM8 channels in cancer treatment. Front. Pharmacol. 14:1098448. doi: 10.3389/fphar.2023.1098448
Received: 14 November 2022; Accepted: 20 February 2023;
Published: 22 March 2023.
Edited by:
Daniel Yakubovich, Ariel University, IsraelReviewed by:
Ye Yu, China Pharmaceutical University, ChinaJuan Carlos Gomora, Universidad Nacional Autónoma de México, Mexico
Copyright © 2023 Ochoa, Casas, Albarracín, Sutachan and Torres. This is an open-access article distributed under the terms of the Creative Commons Attribution License (CC BY). The use, distribution or reproduction in other forums is permitted, provided the original author(s) and the copyright owner(s) are credited and that the original publication in this journal is cited, in accordance with accepted academic practice. No use, distribution or reproduction is permitted which does not comply with these terms.
*Correspondence: Sara V. Ochoa, sa.ochoa@javeriana.edu.co; Yolima P. Torres, yolima.torres@javeriana.edu.co