- 1College of Pharmacy, Shandong University of Traditional Chinese Medicine, Jinan, Shandong, China
- 2Jinan Hospital of Traditional Chinese Medicine, Jinan, Shandong, China
- 3Jinan Third People’s Hospital, Jinan, Shandong, China
- 4The Second Affiliated Hospital of Shandong University of Traditional Chinese Medicine, Jinan, Shandong, China
- 5Taian City Central Hospital, Taian, Shandong, China
Dendrophthoe falcata (L.f.) Ettingsh. (DF) and Dendrophthoe pentandra (L.) Miq. (DP) have been traditionally used for the treatment of various ailments, such as cancer, ulcers, asthma, paralysis, skin diseases, tuberculosis, and menstrual troubles, in the ethnomedicinal systems of India and Indonesia. Currently, the chemical structures of 46 compounds have been elucidated from DF and DP, including flavonoids, triterpenes, tannins, steroids, open-chain aliphatics, benzyl derivates, and cyclic chain derivatives. In vitro assays have revealed their anti-tumor and anti-microbial activities. In vivo studies have unraveled their pharmacological properties against tumors, depression, fertility disorders, inflammatory responses, and so on. Additionally, their weak toxicity to rats and brine shrimp, as well as their promising applications for pharmaceutical preparations and combined medication, were also revealed. Herein, we not only recapitulated traditional medical uses, phytochemistry, pharmacology, toxicity, and applications of DF and DP but also discussed current research limitations and future perspectives, which are instructive for those interested in them and are committed to advancing parasitic plants to the Frontier of phytomedicine. We highlighted that DF and DP will become promising medical plants rather than being discarded as notorious pests, provided that more and deeper research is undertaken.
1 Introduction
Medical plants are receiving increasingly attention from current researchers as they are available as prescription medications or dietary supplements in most regions (Jalali and Rahbardar, 2022; Jiang et al., 2022). Representative bioactive molecules yielded from natural plants, such as paclitaxel (Klein et al., 2022), progesterone (Prior, 2018), artemisinin (Hanboonkunupakarn et al., 2022), and ginkgolides (Omidkhoda et al., 2019) have been prioritized for clinical treatment at present. As the COVID-19 epidemic spreads around the world, cepharanthine, a botanical drug, has shown more prominent therapeutic effects than the traditional anti-virus drugs we used previously (Chen et al., 2022; Fan et al., 2022). The bioactive compounds are derived from secondary metabolites present in plants, including flavonoids, alkaloids, steroids, terpenes, phenylpropanoids, tannins, and others (Seca and Pinto, 2018; Yan et al., 2022). In general, natural bioactive molecules for clinical treatment are subjected to a series of tests, allowing for structural elucidation, pharmacological assays, and clinical trials. Sometimes, natural ingredients can be deemed as leading compounds for the synthesis of potential candidates for targeted therapies (Gyrdymova and Rubtsova, 2022; Song et al., 2022). Consequently, natural plants provide abundant phytochemicals that can open therapeutic windows for various diseases.
Interestingly, parasitic plants exist as a special class of plants that can share elements, RNA, proteins, and hormone pathways with their hosts (Tennakoon et al., 2011; Westwood and Kim, 2017; Chengala and Menon, 2021; Fàbregas and Fernie, 2022). Sine parasitic plants are present in several plants, they are endowed with a plethora of metabolites and diverse bioactivities (Lim et al., 2017). Mistletoes, in particular, emerge as a taxonomic group for parasitic plants, encompassing several families such as Loranthaceae, Viscaceae, Misodendraceae, Eremolepidaceae, and Santalaceae (Bar-Sela, 2011; Milner et al., 2020). Mistletoes have been traditionally regarded as medical plants with magical powers in view of their omnipotent medicinal properties (Adesina et al., 2013). Birds, as major dispersers, often carry mistletoe seeds to different plants, with the diameter, height, and canopy shape of the hosts being important external factors influencing their choice (Rawsthorne et al., 2011; Rahmad et al., 2014). The seeds can pierce host epidermal tissue, forming an organ called a “haustorium” that allows parasites and hosts to transfer nutrition, carbon, minerals, and water, resulting in a close relationship between them (Jadhav et al., 2005; Adesina et al., 2013; Muche et al., 2022). In taxonomy, parasites can be classified as holoparasites and hemiparasites, depending on whether they have access to photosynthetic resources (Pare and Raynal-Roques, 1999; Zervas et al., 2019). Based on the diverse host parts, parasites can also be called root hemiparasites, root holoparasites, stem parasites, endophytic parasites, and others (Tesitel, 2016).
Here, Dendrophthoe falcata (L.f.) Ettingsh. (DF) and Dendrophthoe pentandra (L.) Miq. (DP) (Loranthaceae) grow throughout the tropics and subtropics as ethnomedicinal plants (Figure 1), captivating our interest as they can be traditionally used for the treatment of common human ailments, such as cancer, ulcers, asthma, paralysis, skin diseases, menstrual troubles, pulmonary tuberculosis, wounds, and so on (Dashora et al., 2011b; Sinoriya et al., 2011; Haque, 2015; Lim et al., 2017; Atun et al., 2018; Subhashini et al., 2019). In folklore, DF is called “Banda” (Ghirtlahre et al., 2015) and “Vanda” (Khatoon et al., 2011), while DP is called “Benalu” (Elsyana et al., 2016). Additionally, DF and DP are also commonly known as Indian mistletoe and Indonesian mistletoe, respectively in Englishbecause their distribution is concentrated in India and Indonesia, respectively (Sinoriya et al., 2011; Lim et al., 2017; Alharits et al., 2020; Singh et al., 2020; Bhagat and Kondawara, 2021; Yapa et al., 2021). Moreover, they are rarely present in other countries like Thailand (Kwanda et al., 2013), China (Guo and Ren, 2019), and Bangladesh (Hasan et al., 2018). However, they can undermine economic crops such as Mangifera indica L., Myristica fragrans Houtt., and Artocarpus heterophyllus Lam. under infestation, often causing serious damage to local people (Kuramana et al., 2020; Sivaramakrishna et al., 2021; Yapa et al., 2021). In general, hemiparasites are generally considered as pests, and the locals often remove them from their hosts through chemical treatment and pruning (Wang et al., 2022).
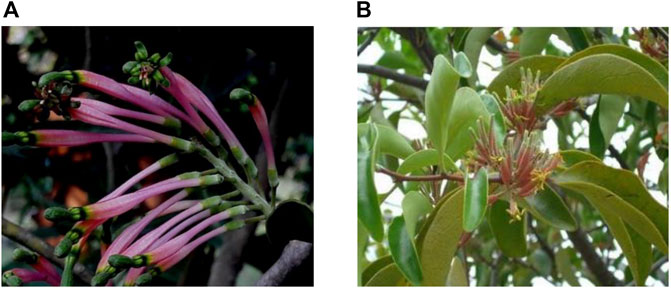
FIGURE 1. (A) Dendrophthoe falcata (L.f.) Ettingsh. (Kuramana et al., 2020), (B) Dendrophthoe pentandra (L.) Miq. (Zainuddin & Sulâ, 2015).
Herein, relevant references covering from 1961 to 2022 were retrieved from Google Scholar, Sci-Finder, PubMed, Web of Science, Wiley, Elsevier, and SpringerLink. The search terms were “Dendrophthoe” and the subject was limited to medicinal value. Although 137 species names for the genus Dendrophthoe have been recorded in “The Plant List” (www.theplantlist.org), including 59 accepted names, 29 synonyms, and 49 unassessed species, species with pharmacological properties were focused on DF and DP. Accordingly, we reviewed their research results with respect to traditional medical uses, phytochemistry, pharmacology, toxicity, and applications, which paved the way for relevant researchers.
2 Traditional medical uses
DF and DP are traditional medicinal plants that are handed down in and around India and Indonesia. The traditional medical uses of DF and DP are used to treat cancer, wounds, ulcers, asthma, paralysis, fertility, skin diseases, menstrual disorders, and so on (Pattanayak et al., 2012; Sembiring et al., 2016; Rafe et al., 2017; Hardiyanti et al., 2018; Bhagat and Kondawar, 2019; Yismairai et al., 2019). Their extensive therapeutic effects on human diseases have allowed the locals to record them in the Ayurvedic system of medicine and other ethnomedicinal systems (Bhagat and Kondawar, 2019; Pramestya et al., 2019). In general, they are often crushed and mixed with other ingredients to make edible tea, decoction or plaster to treat human diseases, while raw materials are fed to livestock to improve growth performance (Pattanayak and Mazumder, 2009a; Reddy et al., 2019; Barkah and Lokesh, 2020; Nigam, 2022). In folklore, DF and DP are given various trivial names due to cultural differences. Furthermore, the different therapeutic effects show certain geographical differences in ethnomedical systems. The brief traditional medical uses of DF and DP were compiled in Table 1.
The ethnomedical uses of DF are mainly documented in the Indian medical system. For example, DF is known as “Vanda”, “Vrksadani”, and “Vrksaruha” in the Indian Ayurvedic system of medicine, which is recorded to possess therapeutic effects on rheumatic complaints, leucorrhoea, contraception, bone fracture, asthma, menstrual disorders, wound healing, and schizophrenia (Khatoon et al., 2011; Madhuri et al., 2013; Jain and Sharma, 2016). The whole DF is used to treat cooling, bitter, astringent, aphrodisiac, narcotic, fertility, cancer, and diuretic in indigenous medicine (Bhagat and Kondawar, 2019; Reddy et al., 2019). Also, the whole DF is used as a cooling, astringent, aphrodisiac, narcotic, and diuretic agent in the traditional Indian system of medicine (Shalini et al., 2019a). Additionally, the ethnomedical properties of DF are documented in some countries. DF is called “Banda” by the tribals of India, and its leaves, stems, and flowers can be used to treat wounds, mania, menstrual troubles, breathing problems, psychic disorders, and pulmonary tuberculosis (Pattanayak et al., 2012; Bhagat and Kondawara, 2021). DF is also called “Farolla” in India and Bangladesh, which can be used for the treatment of asthma, wounds, ulcers, and pulmonary tuberculosis, as well as in formulations that are used as aphrodisiac, diuretic, astringent, and narcotic traditional medicines (Rafe et al., 2017). In other areas, DF is called “Pilla” in Sri Lankan, which can be used to treat cancerous tumors in the Sri Lankan ethnomedicinal system (Karunaratne and Uduwela, 2020). In Ayurveda, DF is known as “Vanda” or “Bandaka” and can be used to treat diarrhea, swelling, renal calculi, and epilepsy (Shahare and Bodele, 2019).
Ethnomedicinal surveys of DP are relatively few and are mainly reported in Indonesia. DP is commonly known as “Benalu” in Indonesiaand is used to treat skin diseases, small sores, coughs, after childbirth, and hypertension (Elsyana et al., 2016; Pramestya et al., 2019). In Sulawesi, DP can be used to treat mumps and cancer (Hasan et al., 2018; Yismairai et al., 2019). In Central Kalimantan and dyspnea in Southeast Sulawesi, DP can be used for the treatment of diabetes and tonsillitis (Alharits et al., 2019). In some areas of Thailand, DP can be used for the treatment of high blood pressure, chronic diseases, and immunological disorders (Kwanda et al., 2013).
3 Phytochemistry
Up to now, 46 compounds have been separated from DF and DP, including flavonoids (1–11), triterpenes (12–23), tannins (24–33), steroids (34–39), open-chain aliphatics (40–41), benzyl derivates (42–44), and cyclic chain derivatives (45–46). The names, chemical formulas, and masses of the 46 compounds were summarized in Table 2. Their chemical structures have been elucidated by NMR, MS, IR, HPLC, and UV methods, along with the comparison with the published data (Figure 2). Previous phytochemistry screening of DF and DP have reported the presence of alkaloids, anthraquinones, and coumarins (Asadujjaman et al., 2013; Fitrilia et al., 2015; Kristiningrum et al., 2018; Alharits et al., 2019; Susilowati and Sari, 2020; Tioline et al., 2021; Dhobale and Rothe, 2022), however, their structures have not been confirmed by modern spectroscopic techniques. Moreover, previous pharmacological studies on DF and DP have focused on the effects of crude extracts, organic fractions, and groups of phytochemicals, leading to limited bioactive compounds.
Flavonoids are a common class of secondary metabolites present in plants, and they are generally in the form of flavonoid glycosides (Nijveldt et al., 2001; Yang et al., 2018). According to the flavonoids yielded from DF and DP, they can be classified as flavones and flavonols, which presented as basic skeletal nuclei of C6-C3-C6. Common flavonoids such as quercetin (1), quercitrin (2), rutin (3), and kaempferol 3-O-α-L-rhamnopyranoside (4) have been yielded from DF and DP. Among them, compound 1 was previously yielded from DF (Nair and Krishnakumary, 1990; Kachhawa J. et al., 2011) and DP (Artanti et al., 2006; Rahmawati et al., 2014). Compounds 2, 3, and 4 were all previously isolated from DF (Nair and Krishnakumary, 1990; Mallavadhani et al., 2006; Kachhawa J. et al., 2011) and DP (Artanti et al., 2006; Nguyen et al., 2010; Rahmawati et al., 2014; Sembiring et al., 2022). Furthermore, quercetagetin (5), meratin (6), hyperoside (7), kaempferol (8), and apigenin-8-C-β-D-(2″-O-β-D-glucopyranosyl)-glucopyranoside (9), were previously isolated only from DF (Nair and Krishnakumary, 1990; Gangwar and Saxena, 2010; Kachhawa J. et al., 2011; Khatoon et al., 2011). Quercetin 4′-O-β-glucopyranoside (10) and hesperidin (11) were previously separated only from DP (Nguyen et al., 2010; Rahmawati et al., 2014).
Triterpenes consisting of six isoprene units are present in plants both individually and as glycosides, with tetracyclic triterpenes and pentacyclic triterpenes being the most common (Hill and Connolly, 2011). Notably, the triterpenes currently yielded from DF and DP are all pentacyclic triterpenes. Among them, except for 3-friedelanol (23), which was isolated from DP (Nguyen et al., 2010), the remaining 11 compounds were yielded from DF (Mallavadhani et al., 2006; Rafe et al., 2017), including 3β-acetoxy-1β-(2-hydroxy-2-propoxy)-11α-hydroxyolean-12-ene (12), 3β-acetoxy-11α-ethoxy-1β-hydroxy-olean-12-ene (13), 3β-acetoxy-1β-hydroxy-11α-methoxy-olean-12-ene (14), 3β-acetoxy-1β,11α-dihydroxy-olean-12-ene (15), 3β-acetoxy-12-ene-11-one (16), 3β-acetoxy-1β,11α-dihydroxy-urs-12-ene (17), 3β-acetoxy-urs-12-ene-11-one (18), 3β-acetoxy-lup-20 (29)-ene (19), lupeol (20), 30-nor-lup-3β-acetoxy-20-one (21), (20S)-3β-acetoxy-lupan-29-oic acid (22).
Tannins, a distinct taxonomic group of phenolic compounds with molecular weights ranging from 500 Da to 3,000 Da, are abundant in almost all plant foods and beverages (Serrano et al., 2009). They can be classified as hydrolyzable tannins, condensed tannins, and complex tannins, based on the different phenolic metabolites (Khanbabaee and Van Ree, 2001; Arbenz and Averous, 2015). Gallic acid (24), a common phenol, was previously isolated from DF (Mallavadhani et al., 2006; Khatoon et al., 2011) and DP (Rahmawati et al., 2014), while epicatechin (26) was previously reported from DF (Khatoon et al., 2011) and DP (Rahmawati et al., 2014) Moreover, scleropentaside F (27), butane-2,3-diol-2-(6′-O-galloyl)-O-β-glucopyranoside (28), methyl gallate 3-O-β-D-glucopyranoside (29), methyl gallate 3-O-(6′-O-galloyl)-β-D-glucopyranoside (30), procyanidin B1 (32), procyanidin B3 (33) were previously yielded from DP (Sahakitpichan et al., 2017). In addition, caffeic acid (25) and ellagic acid (31) were previously separated from DF (Khatoon et al., 2011).
Steroids are a class of natural compounds with a parent nucleus of cyclopentane-poly hydro phenanthrene, a trans-B/C ring, and a hydroxyl at the C-14 position (Riad et al., 2002; Cole et al., 2019; Ermolenko et al., 2020). According to the different substitutes at the C-17 position, cardiac glycosides, phytosterols, and C21-steroids have been confirmed from the yielded compounds of DF and DP. Previous phytochemical investigations have reported odoroside H (34), strospeside (35), and neritaloside (36) from DF (Boonsong and Wright, 1961), along with daucosterol (38) and progesterone (39) from DP (Mochamad et al., 2019). Additionally, β-sitosterol (37) was previously yielded from DF (Rafe et al., 2017) and DP (Soemardji et al., 2005).
For others, compounds 40–46 were all isolated from DP (Nguyen et al., 2011; Sahakitpichan et al., 2017). Among them, glycerol-1,2-dihexadecanoate-3-O-β-D-galactopyranose (40) and heptacosanoic acid (41) are open-chain aliphatics; benzyl-O-α-L-rhamnopyranosyl-(1 → 6)-β-D-glucopyranoside (42), benzyl-O-β-D-glucopyranoside (43) and benzyl-O-β-D-apiofuranosyl-(1 → 6)-β-D-glucopyranoside (44) are benzyl derivates; and bridelionoside A (45) and kiwiionoside (46) are cyclic chain derivatives.
4 Pharmacology
Previous pharmacological studies of DF and DP have revealed potential therapeutic possibilities for numerous ailments. First of all, anti-tumor and anti-microbial activities have been unveiled in vitro assays. On the basis of in vitro assays, several in vivo assays have found pharmacological properties of DF and DP against tumors, depression, fertility disorders, inflammatory responses, and others. Non-etheless, the pharmacological activities of DF and DP were superficially studied because findings regarding active molecules, relevant action mechanisms, along with clinical trials in humans were absent, which means that further investigations are indispensable to address these issues.
4.1 In vitro assays
4.1.1 Anti-tumor activity
In vitro assays indicated that several extracts of DF or DP possess certain cytotoxic effects on breast cancer cells, Ehrlich ascitic carcinoma cells, skin cancer cells, chronic myeloid leukemia cells, and myeloma culture cells. To start with, MCF-7 cells were considered as a primary breast cancer model, and representative studies were listed below. The methanol extract of DP leaves was able to exert antiproliferative properties (0.04 μg/mL–100 μg/mL) with an IC50 value of 10.65 μg/mL, which might be attributed to the increased p53 and BAX, along with the decreased BCL-2 (Zainuddin et al., 2018). The ethyl acetate fraction of DP leaves growing on Lansium parasiticum (Osbeck) K.C.Sahni & Bennet (7.8 ppm–1,000 ppm) exhibited certain inhibitory activity with an IC50 value of 4.72 μg/mL (Yee et al., 2017). The aqueous extract of DF stems (62.5 μg/mL–500 μg/mL) exhibited higher inhibitory effects than ethanolic extract through MTT and SRB (sulforhodamine B) assays with IC50 values of 90 and 98 μg/mL, respectively (Dashora et al., 2011c). Furthermore, T47D cells were tested by the n-hexane fraction of DP, resulting in an IC50 value of 39.57 μg/mL (Subandrate et al., 2019). MCM-B2 cells were subjected to an n-hexane fraction of DP leaves growing on Annona squamosa L. (125 μg/mL), leading to an inhibitory activity of 41.5% (Elsyana et al., 2016). Additionally, the methanolic extract of DP enabled the K562 cells and the MCF-7 cells to arrest in G2/M and G1/S, respectively (Zamani et al., 2016; Zainuddin et al., 2018).
In addition, Ehrlich ascitic carcinoma cells, HaCat cells, and K562 cells were tested in previous studies of DF and DP. A study revealed that aqueous and ethanolic extracts (400 mg/kg) of DF stems growing on Mangifera indica L. were able to inhibit the growth of Ehrlich ascitic carcinoma cells with weights of 2.72 g and 1.96 g, respectively (Dashora et al., 2011a). The methanol extracts of DF leaves growing on Mangifera indica L. (10 μg/mL–80 μg/mL) could inhibit the growth of HaCat cells by MTT and SRB assays with cytostatic rates of 90.00% and 86.96%, respectively (Bhagat and Kondawara, 2021). The n-hexane fraction of DP leaves was unveiled to have suppressive effects on K562 cells with an IC50 value of 38.69% (Elsyana et al., 2016). Using methylene blue solution, a diethyl ether extract of DP stem barks was tested on myeloma culture cells, resulting in antiproliferation activity at doses ranging from 11.0 g/mL to 44 g/mL (Lazuardi, 2008).
At present, significant advances have been made in the molecular mechanisms of cell cycle arrest by natural products that can inhibit cell cycle protein-dependent kinases and regulate proteinsused to control cell proliferation (Bailon-Moscoso et al., 2017). Bioactive molecules that target cell cycle protein-dependent kinases, in particular, have been used as new anti-cancer medicines. In general, p53 can regulate p21 which inhibits cell cycle protein-dependent kinase2 (CDK2) and cyclin E, as evidenced by the monitoring of G1/S and G2/M phase correction sites (Engeland 2018). Unfortunately, the number of protein-dependent stimulating enzymes is small in previous studies, therefore, more potential proteins need to be identified. The underlying mechanism of cell cycle arrest of DP was presented in Figure 3.
4.1.2 Anti-microbial activity
In previous studies, the agar diffusion method was used to evaluate the anti-microbial activity by determining the diameter of the inhibition zone. For example, quercitrin isolated from DP leaves (1,000 μg/mL) was studied against Escherichia coli, Salmonella typhi, Staphylococcus aeureus, and Pseudomonas with inhibitory zones of 7.74 mm, 7.23 mm, 9.54 mm, and 8.82 mm, respectively (Hardiyanti et al., 2019c). Total flavonoids of DP leaves growing on Polyalthia longifolia (Sonn.) Thwaites (3%–9%) were deemed as potential antimicrobial agents against Streptococcus mutans, Escherichia coli, and Candida albicans with inhibitory zone diameters of 6 mm–17.25 mm, 3.55 mm–9.15 mm, and 4 mm–8.30 mm, respectively (Fahmi et al., 2018). Furthermore, the aqueous extract of DF was able to inhibit the proliferation of several strains. For instance, the aqueous extract of DF leaves parasitic on Azadirachta indica A.Juss. was able to attenuate Staphylococcus aureus with an inhibition zone of 10 mm (Kathayi and Nagarajan, 2018). The aqueous extract of DF twigs was demonstrated to exert antimicrobial activity against Shigella flexneri, Staphylococcus epidermidis, Bacillus subtilis, and Escherichia coli with inhibitory zones of 17 mm, 25 mm, 20 mm, and 22 mm, respectively (Shantipriya et al., 2015). For organic solvent extracts, the methanol and acetone extracts of the whole plant of DF (50 mg/kg) were found to inhibit Shigella flexneri by disc diffusion and agar well diffusion methods, with inhibition zones of 1.8 cm and 2.0 cm, respectively (Nayak et al., 2020).
A study found that the ethanol extract of aerial parts of DF against Aspergillus fumigatus, Staphylococcus epidermidis, Salmonella typhi with MIC values of 10 µg/disc (Pattanayak and Sunita, 2008). Unfortunately, few studies have reported the MICs and MLCs of the extracts of DF and DP and future studies should be carried out.
4.2 In vivo assays
4.2.1 Oncotherapy
Active ingredients from DF or DP have been tested in vivo for their therapeutic effects on targeted tumors in mouse models induced by various chemicals. To start with, the therapeutic effects of DF extracts on breast tumors were evaluated using rats induced by dimethylbenzene [a]anthracene (DMBA). For example, a study found that the aqueous extract of DF leaves growing on Shorea robusta Gaertn. (400 mg/kg) was orally administrated to DMBA -induced rats, which reduced the tumor volume by 24.934%, showing more a significant therapeutic effect than chemical tamoxifen (Lobo et al., 2010). Another study revealed that the ethanol extract of the aerial part of DF was orally administrated to DMBA-induced rats (250 mg/kg–950 mg/kg), presenting normal control levels of liver indexes accompanied by an obvious decrease in breast tumors compared to the olive oil control group (Pattanayak S. P. and Mazumder P., 2011).
The mice induced by azoxymethane (AOM) were used to test the therapeutic effects of DP extracts. For instance, the ethanolic extract of DP was able to prevent colonic tumor damage. The oral administration of the ethanol extract of DP leaves growing on Mangifera indica L. (0.25 mg/g and 0.5 mg/g) was used for the treatment of mice induced by AOM, resulting in the colonic tissue with abnormal characteristics (Wicaksono and Permana, 2013). Moreover, the ethanolic extract of DF was found to release relevant cytokines and to arrest the cell cycle of colonic tumors. The mice induced by AOM were orally administrated with the ethanol extract of DP leaves growing on Mangifera indica L. (125 mg/kg–500 mg/kg), leading to a decrease in IL-22 and myeloperoxidase levels and an increase in the percentage of cells in the S phase compared with the model group (5% dextran sodium sulfate), which was correlated with p53 expression (Endharti et al., 2016).
4.2.2 Therapeutics for depression
The oral administration of various extracts of DF or DP to rats or mice has been evaluated via several psychotropic tests, resulting in more active behavior than the diet without DF or DP. In the open field and hole cross tests, the ethanolic extract of DF stems grown on Swietenia fabrilis Salisb (200 mg/kg) inhibited locomotion in acetic acid-induced writhing models by 97.33% and 85.91%, respectively (Haque, 2016). In addition, the ethanol extract of DF leaves (250 mg/kg and 500 mg/kg) was orally administrated to mice, revealing a significant increase in the percentages of arms open, EPM arm open time, and motor coordination in the rotating bar test, and an increase in the percentage of time spent in the light box and the number of transitions in the light-dark box test, which was associated with a GABA(A) receptor-mediated mechanism of action (Aryal and Khan, 2015). The oral administration of ethanolic extract of DF leaves enabled the rats to exhibit potent anti-depression activity with average response times of immobility of 86.7 and 76.08, respectively, compared to the control group treated with the vehicle (Dubey et al., 2015). The gavage of the ethanolic extract of DF leaves (200 mg/kg and 400 mg/kg) significantly increased motor activity and resting time and decreased depression in the forced swimming experiment and the tail suspension experiment in mice compared with the vehicle-treated group (Logesh Kumar et al., 2019).
4.2.3 Effects on fertility
Kaempferol, isolated from the stems of DF, was orally administrated to rats at 50 mg/rat/day for 60 days, manifesting low sperm motility and density as well as a marked reduction in serum testosterone levels than the normal control group (Kachhawa J. B. et al., 2011). Several extracts, in particular, have been demonstrated to possess potential therapeutic effects on fertility disorders. In terms of methanolic extract of DF or DP, follicle-stimulating hormone (FSH) and progesterone levels in female rats were tested by the oral administration of the methanolic extract of DP leaves (100 mg/kg) using the random evidence investigator analyzer, showing values of 9.28 miu/mL ± 6.72 miu/mL and 33.55 nmol/L ± 13.96 nmol/L, respectively (Mochamad and Hermanto, 2014). The rats were orally administrated with the ethanol extract of the aerial parts of DF (250 mg/kg–950 mg/kg), revealing anti-implantation activity, based on a decrease in the number of litters delivered after postcoital testing (Pattanayak and Mazumder, 2009b). Additionally, the oral administration of the methanolic extract of DF stems at 200 mg/rat/day could reduce the weights of reproductive organs, sperm count, and sperm motility in male rats compared to the vehicle-treated control group, leading to sterile state (Gupta and Kachhawa, 2008). The methanol extract of DF stems was orally administrated to male rats at 200 mg/rat/day, resulting in a reduction of sperm count and sperm motility, along with a decrease in the content of total protein, sialic acid, and glycogen in the testicles than the standard drug group [lonidamine(1-(2,4-dichlorobenzyl)-1H-indazole-3carboxylic acid] (Gupta and Kachhawa, 2007). The oral administration of methanol extract of DF stems (200 mg/kg) resulted in decreased sperm motility, density, and serum testosterone levels, and the degenerative changes in the varicocele in male albino rats compared with the untreated control group (Gupta and Kachhawa, 2007). Furthermore, the oral administration of chloroform fraction of DF stems at 50 mg/day revealed the suppression of sperm density and motility in the epididymal tail, accompanied by decreased protein and sialic acid in the testis and cauda epididymis, as well as increased testicular cholesterol compared to the untreated group (Kachhawa J. B. et al., 2011).
4.2.4 Therapeutics for inflammatory responses
The paw edema in rats or mice induced by chemicals was considered as a representative inflammatory model. For instance, the instillation of ethanolic extract of DP leaves grown on Mangifera indica L. (600 mg/kg) to 2,4,6-trinitrobenzenesulfonic acid-induced mice contributed to attenuating colon shortening and myeloperoxidase, along with significant improvement in colitis compared to the control group (50% ethanol-phosphate-buffered saline) (Endharti and Permana, 2017). For paw edema in rats induced by carrageenan, the oral administration of aqueous and methanolic extracts of DF leaves growing on Mangifera indica L. (300 mg/kg) resulted in inhibitory indices of 30.95% and 23.41%, respectively, and reduced the formation of granuloma tissue by 51% and 48%, respectively (Patil et al., 2011).
Similar results were also presented by the treatment of fractions with diverse polarities. The gavage of chloroform fraction of DF stems growing on Swietenia fabrilis Salisb. (200 mg/kg) enabled the inhibition rate of the carrageenan-induced paw edema mice to reach 90.24% (Haque, 2016). The ethyl acetate fraction of the whole plant of DP (2000 mg/kg) was orally administrated to rats induced by carrageenan, resulting in an inhibition of 41.46%, which might be ascribed to the presence of quercetin (Mustarichie et al., 2015). The gavage of petroleum ether fraction of DF leaves growing on Swietenia fabrilis Salisb. (200 mg/kg) was found to exert pharmacological activity against paw edema in mice induced by carrageenan with an inhibitory volume of 90.24% (Haque et al., 2014c).
4.2.5 Immunoregulation
Polysaccharides are considered to be the primary active ingredient of plants (Huang et al., 2022; Li et al., 2022). Currently, polysaccharides of DF have been used as dietary supplements. Polysaccharides extracted from DF leaves growing on Azadirachta indica A.Juss., for example, were fed to fish as a supplemented diet (1%), and the results showed an increase in lysozyme and TNF-α expression, as well as an increase in relative survival percentage compared to the positive control group (0.1% MacroGard™) (Shalini et al., 2019a). The tilapias were fed polysaccharides isolated from DF leaves growing on Azadirachta indica A.Juss. (200 mg/kg), resulting in a reduction in the percentage of mortality resulting from Aeromonas hydrophila infections and upregulation of IL-1β and lysozyme genes compared with the control group (MacroGard™, 20 mg/kg) (Shalini et al., 2019b).
Additionally, the oral administration of ethanolic extract of DF or DP enabled the mice to improve their immune systems. For example, a study found that the ethanol extract of DP leaves growing on Mangifera indica L. (150 mg/kg–600 mg/kg) was able to increase IL-2 levels and the percentage of CD4+CD28+ and CD8+CD28+ in aged female mice compared to the control group (phosphate buffer saline) (Handono et al., 2021). The ethanol extract of the aerial parts of DF parasitic on Azadirachta indica A.Juss (250 mg/kg–950 mg/kg) was employed in female rats, revealing a significant increase in NO production by peritoneal macrophages and phagocytic activity of polymorphonuclear cells and the reticuloendothelial system compared with the untreated group (Pattanayak S. P. and Mazumder P. M., 2011). The pro-inflammatory indices mentioned above, such as IL-1β, TNF-α, and IL-2, are involved in innate immunity, which can enhance the expression of non-specific immune mechanisms and immune-related genes, revealing a potential link between inflammatory response and immunity.
4.2.6 Therapeutics for hyperglycemia and hypertension
Alloxan-induced rats were commonly considered diabetic models and have been used to evaluate the therapeutic effects of several extracts. A study found that alloxan-induced rats gavaged with the methanol extract of DF stems growing on Mangifera indica L. (200 mg/kg) could result in decreased levels of blood cholesterol and triglyceride, which might indicate that DF was possible to possess a therapeutic effect on type-II diabetes mellitus (Anarthe et al., 2008). In addition, the oral administration of the methanol extract of DP leaves (50 mg/kg and 400 mg/kg) enabled alloxan-induced rats to have significant antidiabetic activity with a notable oral glucose tolerance equivalent to the antihyperglycemic metformin (Hasan et al., 2018).
Active ingredients of DP were found to have protective and regulatory effects on arterial vasculature. A study pointed out that the hypertensive rats gavaged with the methanol extract of DP leaves growing on the Mangifera indica L. (200 mg/kg) reduced the amount of cellular necrosis and altered the width of the white matter areas of the brain in hypertensive rats treated with deoxycorticosterone acetate salt (Saputri et al., 2021).
4.2.7 Therapeutics for other ailments
DF and DP were also used for attenuating hypercholesteremia, promoting urination, treating diarrhea, protecting the liver, and retarding oxidation. Despite the number of studies involving in the above therapeutic properties was limited, these findings also indicated certain pharmacotherapeutic possibilities that need further investigation. The gavage of water extract of DP leaves (800 mg/kg) was able to reduce the total cholesterol levels and improve liver histopathology in mice treated with a hypercholesterolemic diet (Mu’nisa et al., 2019). The oral administration of the petroleum ether fraction of DF leaves (250 mg/kg and 500 mg/kg) resulted in higher urine output compared with furosemide in the control group (Reddy et al., 2019). The castor oil-induced mice were gavaged with the ethanol extract of DF leaves growing on Swietenia fabrilis Salisb. (200 mg/kg), showing a reduction in the severity and frequency of diarrheal pairs with an inhibitory rate of 83.47% (Haque et al., 2014b). The CCl4-induced mice were orally administrated with water and ethanol extracts of DF leaves growing on Swietenia fabrilis Salisb. (200 mg/kg), leading to increased activities of liver markers, including transaminase (AST), alanine transaminase (ALT), alkaline phosphatase (ALP), total protein, and bilirubin (Haque et al., 2014a). An assay revealed that the gavage of ethanolic extract of the aerial part of DF (250 mg/kg and 500 mg/kg) could exert anti-oxidant effects in excisional and incisional wound models in rats through the inhibition of lipid peroxidation, the decrease of glutathione and superoxide dismutase, along with the increase of catalase activity (Pattanayak and Sunita, 2008). The above results of in vivo studies of DF and DP were summarized in Table 3.
5 Toxicity
Preliminary toxic tests for DF and DP were focused on oral toxicity in mice and brine shrimp lethality assays, showing relatively high LC50 or LD50 values with a wide range of safe doses in general. Additionally, the toxic effects on the physiological organ functions of rats were small, suggesting low toxicity to a certain extent.
5.1 Mouse oral toxicity test
Several toxic studies have reported with the oral administration of the ethanolic extract of DF to rats. An oral acute toxic test indicated that aqueous and ethanolic extracts of DF stems growing on Mangifera indica L. resulted in LD50 values of 4 g/kg (Dashora et al., 2011a). The rats subjected to the ethanolic extract of aerial parts of DF growing on Azadirachta indica A.Juss. were found to have an LD50 value of 1.75 g/kg (Pattanayak and Mazumder, 2010). The ethanol extract of aerial parts of DF parasitic on Azadirachta indica A.Juss. enabled the neurobehavioral rats to reach an LD50 value of 4.55 g/kg (Pattanayak and Mazumder, 2009a). Similar reports were also seen in DP. The ethanol extract of DP growing on Mangifera indica L. was employed to male and female rats, showing LD50 values of 34.28 g/kg and 22.41 g/kg, respectively (Nurfaat, 2016). The oral administration of aqueous and ethanolic extracts of DP to rats revealed LD50 values of 17.78 g/kg and 12.59 g/kg, respectively (Mustarichie et al., 2016).
In addition to the above results, several studies have found neither lethality nor significant toxic effects following the administration of high doses of extracts to rats. For instance, the oral administration of the ethanol extract of DF leaves to rats showed neither abnormal effects nor moribund stages (Aryal and Khan, 2015). Aqueous and ethanolic extracts of DF leaves growing on Swietenia fabrilis Salisb. were orally administrated to rats at doses of 200 mg/kg–3,200 mg/kg, resulting in no obvious changes in internal organs compared to the normal group (Haque et al., 2014a). The ethanolic extract of DF barks was employed on rats, resulting in no lethality occurrence even if the doses were upgraded to 2,000 mg/kg (Pattanayak et al., 2008). Similarly, β-sitosterol and quercitrin isolated from DP leaves were demonstrated to be non-toxic, with lethality up to 2,000 mg/kg (Soemardji et al., 2005).
5.2 Brine shrimp lethality test
The brine shrimp lethality test (BSLT) with convenient and sensitive characteristics was designed to preliminarily evaluate the toxic effects of DF and DP and the results are presented as LC50 values in general. Compared with the oral toxic effects in mice, brine shrimp tend to have lower LC50 values than mice due to the sensitivity of the extracts. Moreover, DP tends to show a higher LC50 value than DF, based on current results.
Most of the DP studies have been undertaken to investigate the toxicity of organic fractions and extracts. For instance, the n-hexane fraction of DP leaves was found to possess toxic effects on shrimp larvae with an LC50 value of 41.59 ppm (Hardiyanti and Marpaung, 2018). The n-hexane fraction of DP leaves growing on clove was revealed to have an LC50 value of 55.31 μg/mL by BSLT (Elsyana et al., 2016). For organic extracts, the methanolic extract yielded from DP leaves was conducted by BSLT, resulting in an LC50 value of 13.95 μg/mL (Hardiyanti et al., 2019a). Additionally, similar results were also found in phytochemicals. An in silico approach revealed that quercitrin isolated from DP possessed a harmless LD50, but long-term use was not suggested (Sjakoer et al., 2021). The total flavonoids of DP leaves growing on Polyalthia longifolia (Sonn.) Thwaites were found to have an LC50 value of 30.06 mg/L by BLST (Fahmi et al., 2018).
Some studies have also reported the toxicity of DF. The methanol, ethyl acetate, and n-hexane extracts of DF leaves were investigated by BSLT, resulting in LC50 values of 38.815 μg/mL, 54.827 μg/mL, and 38.815 μg/mL, respectively (Runtuwene and Kamu, 2020). The aqueous and ethanolic extracts of stems of DF were investigated by BLST, displaying LC50 values of 90 and 120 μg/mL, respectively (Dashora et al., 2011a). The ethanolic extract of DF leaves was found to possess an LC50 value of 100 μg/mL by BLST (Hasan et al., 2006).
6 Applications
6.1 Pharmaceutical preparations
DF can be used as raw materials to manufacture tablets with higher absorption and stability than traditional preparation methods. For instance, the mucilage of DF as a supplementary material at a concentration of 6% could be prepared as paracetamol granules using the wet granulation technique, which possessed a friability of 0.98%–0.53%, a disintegration time of 10 min–17 min, and a dissolution of more than 9% in 70 min (Kothawade et al., 2010; Lazuardi et al., 2021). The twigs of DF could be processed into matrix tablets of astemizole with better homogeneity in terms of weight and content than tablets without DF, which might indicate that they are potential natural materials to make sustained-release matrix tablets (Bhoyar et al., 2012).
The active ingredients of DF could be processed into nanoparticles that were conducive to facilitating their metabolism, absorption, distribution, and excretion, which might provide a novel insight into the pharmacokinetics. Quercetin isolated from DF was formulated by spontaneous emulsification with oil, Tween 80, and phosphate bufferto yield quercetin nanoemulsions with an average particle size ranging from 10.2 nm to 36.5 nm and a polydispersity index of less than 0.5 (Atun et al., 2020). The aqueous extract of DF as a reducing and stabilizing agent could be processed into silver nanoparticles having cytotoxic effects on MCF-7 cells by optimizing various reaction conditions (Sathishkumar et al., 2014).
6.2 Combined medication
DP combined with common clinical drugs was found to produce a synergistic effect on certain conditionsand exhibited more significant therapeutic effects compared to individual use. For example, the ethanolic extract of DP combined with doxorubicin could decrease intracellular calcium concentration and surviving levels and increase the number of apoptotic MCF-7 cells, which exhibited more significant cytotoxic effects than doxorubicin alone (Endharti et al., 2018b). Compared with the 5-fluorouracil only group, the combination of ethanolic extract of DP and 5-fluorouracil more significantly decreased the survival of HeLa cells by increasing the percentage of p21 and apoptosis, showing a synergistic effect to restrain the proliferation of tumor cells (Endharti et al., 2018a). The ethanolic extract nanoparticles of DP together with cisplatin could produce a synergistic effect on HeLa cells, which possessed more significant cytotoxic effects than individual medicines (Mutiah et al., 2018). Furthermore, some studies have reported that combined medication might further extend the therapeutic effects of DF and DP. An assay indicated that methanolic extracts of DP and Scurrula. atropurpurea (Bl.) Dans were able to exert renal reinforcement by reducing the levels of creatinine, urea nitrogen, urea, and renal cell necrosis in rats, which further enlarged the pharmacotherapeutic range of DP (Anjani et al., 2021). The ethanolic extract of DP paired with paclitaxel was able to attenuate the growth of MCF-7 cells by reducing the expression of tubulin βIII and microtubule-associated protein 4, which might provide a promising method for the treatment of breast cancer (Permana et al., 2021).
With the development of medical plants combined with modern technology, potential active molecules have been revealed in medicine made from two or more plants. For example, bioactive molecules including flavonol, kaempferol, casticin, quercetin, quercitrin, and isoquercitrin were yielded from DP and Scurrula atropurpurea (Blume) Danser via molecular-specific docking analysis, and found to possess suppressive effects on the angiotensin-converting enzyme responsible for hypertension (Sjakoer et al., 2021). Quercetin, kaempferol, and aloe-emodin produced from DP and Cassia alata L. were able to inhibit the SARS coronavirus major peptidase and the 3-chymotrypsin-like cysteine protease 3 enzyme using a computational approach, suggesting their potential development as candidates against COVID-19 (Ernawati et al., 2021).
7 Discussion
In terms of phytochemistry, the issue of unclear active ingredients and the insufficient number of 46 compounds in DF and DP has led to limited elucidation for pharmacological activities. In recent years, phytochemistry screening has undergone great changes with the emergence of methods characterized by rapid, trace, and large-scale application of a variety of advanced technological tools, including high-throughput screening, chemical and biological characterization (Alves-Silva et al., 2020; Blay et al., 2020; Le Basle et al., 2020). The application of high-throughput screening, GC-MS, LC-MS, NMR, chemometrics, and pattern recognition theory contributed to the simultaneous online separation and the acquisition of qualitative/quantitative data for multiple groups of compounds. In the future, the above techniques and theory should be applied in DF and DP research, which make it possible to elucidate the pharmacological basis of botanical drugs.
The traditional medical uses of DF and DP have shown extensive therapeutic possibilities for human ailments with regional differences. However, these uses were simply recorded in the relevant medical systems with the lack of preparation methods and instruction for specific clinical applications, which require refinement and clarification by conducting civilian surveys. It is worth noting that the treatment of ulcers, asthma, tuberculosis, rheumatic complaints, wounds, and paralysis for traditional uses has not yet been validated compared to pharmacological results in current research. Given traditional medical uses as a valuable reference for current research, which require us to ascertain unproven uses by performing relevant pharmacological experiments.
Although remarkable results have been achieved in verifying the efficacy of DF and DP through cellular and animal models, studies on their mechanisms have been rarely reported. In general, phytopharmaceuticals are characterized by their multi-component and multi-target properties, which tend to cause difficulties for researchers in elucidating their molecular mechanisms. With the development of chemistry, biology, and informatics, the integrated application of these disciplines is playing an increasingly important role in pharmacology. Notably, multidimensional association models based on “chemical fingerprint-metabolic”, “fingerprint-network”, “target-disease effect”, and “pharmacokinetic-pharmacodynamic” assays have become a hot topic of current research (Gallo, 2010; Nielsen et al., 2011; Katz and Baltz, 2016; Xiao et al., 2022). Through theories and methods such as metabolomics, proteomics, transcriptomics, phenomics, genomics database mining, and bioinformatics analysis, a solid foundation has been laid to elucidate the scientific content of phytopharmaceuticals, which should be promoted and applied in the future research of DF and DP.
In order to evaluate the safety of DF and DP, several toxic tests in mice and brine shrimp have been established. As a result, their various extracts appear to be safe, considering their high LC50 or LD50 values and the absence of significant adverse effects. However, these findings are emphasized in the preliminary assessment of individual lethality and physiological manifestations. Therefore, future toxicological studies on the mechanism of action and metabolic transformation should be conducted using isolated organs, cultured cells, or organelles. Recent findings have revealed new prospects in pharmaceutical preparations and combined medication for DF and DP. As we have seen, DF was deemed as an important raw material that could be processed into tablets and nanoparticles characterized by better absorption, metabolism, and distribution, providing new perspectives for pharmacokinetics. In addition, DP combined with classic drugs was found to exhibit stronger therapeutic effects compared to individual usage. However, there was a significant imbalance in the applications of DF and DP. As we have seen, the pharmaceutical preparation was concentrated on DF, while the combined medication was focused on DP. Perhaps, the pharmaceutical preparation for DP and the combined medication for DF need further investigation, which not only extends the scope of application but also contributes to explaining the relationship between DF and DP.
Modern molecular docking and network pharmacology techniques have emerged as convenient and efficient assays for identifying potential bioactive molecules and action targets, as a result of the integration of computer technology and medical plants. Notably, the applicability of these techniques is based on the presence of active molecules in plants, which also demonstrates the close link between phytochemistry and pharmacology. From a foresight perspective, what illuminates the research direction is the combination of pharmacology and phytochemistry with reference to traditional medical uses. Particularly, other Dendrophthoe species like Dendrophthoe trigona (Wt. & Arn.) Danser and Dendrophthoe praelonga (Blume) Miq. were also found to have certain biological activities (Joshi et al., 2012; Patel et al., 2012; Irfan et at., 2020), which need further studies to develop their medical value.
8 Conclusion
Despite the fact that DF and DP were considered as pests that can harm multiple hosts, their traditional uses for treating a variety of ailments such as cancer, ulcers, asthma, paralysis, and skin diseases, have allowed them to capture our attention. Current research has achieved remarkable advances in chemical constituents, pharmacological properties, safety, and applications for DF and DP. As we can see, phytochemical investigations have revealed the presence of 46 compounds from DF and DP, including flavonoids, triterpenes, tannins, steroids, open-chain aliphatics, benzyl derivates, and cyclic chain derivatives. Pharmacological studies have demonstrated therapeutic possibilities of DF and DP against tumors, depression, fertility disorders, inflammatory responses, and others. Toxicity tests suggested that active extracts of DF and DP possessed a wide range of safety without significant adverse effects. In addition, pharmaceutical preparation for DF and combined medication for DP indicated the great potential for medical applications. However, there are still many limitations that need to be addressed, and the following are some suggestions to address these issues.First, traditional medical uses of DF and DP were simply recorded in ethnomedical systems without specific introduction, which needs us to carry out ethnomedical surveys for further refinement and clarification. Second, the issue of unclear active molecules and insufficient compounds from DF and DP limits the elucidation of pharmacological activities, and the application of high-throughput screening, LC-MS, and GC-MS may contribute to discovering compounds. Third, pharmacological research of DF and DP has not been illustrated in molecular biology, leaving underlying mechanisms for corresponding diseases unclear. Therefore, common assays such as western blot, polymerase chain reactions, and metabolomics should be undertaken in future research. Forth, toxic tests of DF and DP were not rigorous, and the addition of cytotoxicity, genotoxicity, and clinical trial tests will make their safety assessment more comprehensive. Fifth, DF and DP focused on diverse applications, and their biochemical differences and relationship need further investigation.
Author contributions
DK, LW, and YN: Data curation, writing—original draft, and review & editing, methodology. LC, BS, and DW: Data curation, investigation, formal analysis, writing—review & editing. JT, WZ, XL, YC, and FW: Investigation, methodology, and data curation. HZ and RJ: Resources, supervision, and writing—original draft.
Funding
This work was funded by the Project of the Shandong Province Key Research and Development Program (2021CXGC010511), the project of Shandong postgraduate education quality curriculum (SDYKC21051), and the innovation training program for college students of Shandong University of Traditional Chinese Medicine (202255).
Conflict of interest
The authors declare that the research was conducted in the absence of any commercial or financial relationships that could be construed as a potential conflict of interest.
Publisher’s note
All claims expressed in this article are solely those of the authors and do not necessarily represent those of their affiliated organizations, or those of the publisher, the editors and the reviewers. Any product that may be evaluated in this article, or claim that may be made by its manufacturer, is not guaranteed or endorsed by the publisher.
References
Adesina, S. K., Illoh, H. C., Johnny, I. I., and Jacobs, I. E. (2013). African mistletoes (Loranthaceae); ethnopharmacology, chemistry and medicinal values: An update. Afr. J. Tradit. Complement. Altern. Med. 10 (4), 161–170. doi:10.4314/ajtcam.v10i4.26
Alharits, L., Handayani, W., Yasmanand, H. N. M., and Hemelda, N. M. (2019). Phytochemical analysis and antioxidant activity of leaves and flowers extracts of mistletoe (Dendrophthoe pentandra (L.) Miq.), collected from UI Campus, Depok. AIP Conf. Proc. 2168 (1), 020101. doi:10.1063/1.5132528
Alharits, L., Hemelda, N. M., and Handayani, W. (2020). Allelopathic activity of Dendrophthoe pentandra as a potential bioplanticide to inhibit seed germination and seedling growth of Eleusine indica. Nusant. Biosci. 12 (1), 33–39. doi:10.13057/nusbiosci/n120106
Alves-Silva, J. M., Guerra, I., Gonçalves, M. J., Cavaleiro, C., Cruz, M. T., Figueirinha, A., et al. (2020). Chemical composition of Crithmum maritimum L. essential oil and hydrodistillation residual water by GC-MS and HPLC-DAD-MS/MS, and their biological activities. Ind. Crops Prod. 149, 112329. doi:10.1016/j.indcrop.2020.112329
Anarthe, S., Bhalke, R., Jadhav, R., and Surana, S. (2008). Antidiabetic activity of stems of hemipararsite Dendrophthoe falcata Linn. parasitic growing on Mangifera indica. Dhaka Univ. J. Pharm. Sci. 7 (2), 177–180. doi:10.3329/dujps.v7i2.2175
Anjani, M., As, N. A., and Mubarakati, N. J. (2021). Study of subchronic during 28 days: Toxicity test of methanolic extract combination of Scurulla atropurpurea and Dendrophthoe pentandra against malfunctioning kidney function of female wistar rats. Biosci. Trop. 6 (2), 58–63. doi:10.33474/e-jbst.v6i2.322
Arbenz, A., and Averous, L. (2015). Chemical modification of tannins to elaborate aromatic biobased macromolecular architectures. Green Chem. 17 (5), 2626–2646. doi:10.1039/c5gc00282f
Artanti, N., Ma'arifa, Y., and Hanafi, M. (2006). Isolation and identification of active antioxidant compound from star fruit (Averrhoa carambola) mistletoe (Dendrophthoe pentandra (L.) Miq.) ethanol extract. J. Appl. Sci. 6 (8), 1659–1663. doi:10.3923/jas.2006.1659.1663
Aryal, D., and Khan, N. (2015). Anxiolytic and motor coordination activity of ethanolic and aqueous extracts of Dendrophthoe falcata leaves in mice. Int. J. Pharm. Sci. Res. 2353, 134. doi:10.13040/IJPSR.0975-8232.6(4).1000-08
Asadujjaman, M., Hossain, A. M., and Karmakar, U. K. (2013). Assessment of DPPH free radical scavenging activity of some medicinal plants. Pharmacologyonline 1, 161–165.
Atun, S., Arianingrum, R., Cahyaningsih, L., Pratiwi, F. A., Kusumaningrum, R., and Khairuddean, M. (2020). Formulation and characterization of quercitrin nanoemulsion isolated from Dendropthoe falcata and its antioxidant activity test. Rasayan J. Chem. 13 (3), 1347–1356. doi:10.31788/rjc.2020.1335868
Atun, S., Handayani, S., Rakhmawati, A., Purnamaningsih, N. A., Naila, B. I. A., and Lestari, A. (2018). Study of potential phenolic compounds from stems of Dendrophthoe falcata (Loranthaceae) plant as antioxidant and antimicrobial agents. Orient. J. Chem. 34 (5), 2342–2349. doi:10.13005/ojc/340515
Bailon-Moscoso, N., Cevallos-Solorzano, G., Romero-Benavides, J. C., and Orellana, M. I. R. (2017). Natural compounds as modulators of cell cycle arrest: Application for anticancer chemotherapies. Curr. Genomics 18 (2), 106–131. doi:10.2174/1389202917666160808125645
Bar-Sela, G. (2011). White-Berry Mistletoe (Viscum album L.) as complementary treatment in cancer: Does it help? Eur. J. Integr. Med. 3 (2), 55–62. doi:10.1016/j.eujim.2011.03.002
Barkah, H. J. E., and Lokesh, S. (2020). Effect of epiphytes extract on the peroxidase activity in the crop seedlings challenged with fungal pathogens. Asian J. Pharm. Clin. Res. 13 (1), 58–62. doi:10.22159/ajpcr.2020.v13i1.35884
Bhagat, V. C. A., and Kondawara, M. S. (2021). GC-MS analysis and in-vitro cytotoxicity study of Dendrophthoe falcata and Tridax procumbens extracts against HaCat cells line. Int. J. Pharm. Sci. Res. 12 (4), 2175–2184. doi:10.13040/ijpsr.0975-8232.12(4).2175-84
Bhagat, V. C., and Kondawar, M. S. (2019). Antitubercular potential of Dendrophthoe falcate (L.) and Tridax procumbens (L.) plants extracts against H37Rv Stain of Mycobacteria tuberculosis. Int. J. Pharm. Sci. Res. 10 (1), 251–259. doi:10.13040/ijpsr.0975-8232.10(1).251-59
Bhoyar, P. K., Baheti, J. R., Lukkad, H. R., Mishra, S. H., and Jain, S. S. (2012). Formulation and evaluation of once-daily sustained release aceclofenac Dendropthoe falcata gum matrix tablets. World J. Pharm. Res. 1 (2), 216–223.
Blay, V., Tolani, B., Ho, S. P., and Arkin, M. R. (2020). High-throughput screening: today's biochemical and cell-based approaches. Drug Discov. Today 25 (10), 1807–1821. doi:10.1016/j.drudis.2020.07.024
Boonsong, C., and Wright, S. E. (1961). Cardiac glycosides present in mistletoes growing on Nerium oleander. Aust. J. Chem. 14, 449–457. doi:10.1071/ch9610449
Chen, C., Wang, N., Wang, B., Zhang, Q., Hu, Y., Cheng, G., et al. (2022). Network analysisbased strategy to investigate the protective effect of cepharanthine on rat acute respiratory distress syndrome. Front. Pharmacol. 13, 1054339. doi:10.3389/fphar.2022.1054339
Chengala, L. S., and Menon, G. S. (2021). Host parasite interaction and protein profile of Dendrophthoe falcata (L.f) ettingsh var. Pubescens (Hook.f) Associated with different hosts. BIOINFOLET- J. Life Sci. 18 (1), 105–110.
Cole, T. J., Short, K. L., and Hooper, S. B. (2019). The science of steroids. Semin. Fetal Neonatal Med. 24 (3), 170–175. doi:10.1016/j.siny.2019.05.005
Dashora, N., Sodde, V., Bhagat, J., S Prabhu, K., and Lobo, R. (2011a). Antitumor activity of Dendrophthoe falcata against ehrlich ascites carcinoma in Swiss albino mice. Pharm. Crops 2 (1), 1–7. doi:10.2174/2210290601102010001
Dashora, N., Sodde, V., Prabhu, K. S., and Lobo, R. (2011b). Antioxidant activities of Dendrophthoe falcata (L.f.) Etting. Pharm. Crops 2, 24–27. doi:10.2174/2210290601102010024
Dashora, N., Sodde, V., Prabhu, K. S., and Lobo, R. (2011c). In vitro cytotoxic activity of Dendrophthoe falcata on human breast adenocarcinoma Cells-MCF-7. Int. J. Cancer Res. 7 (1), 47–54. doi:10.3923/ijcr.2011.47.54
Dhobale, G. N., and Rothe, S. P. (2022). Phytochemical analysis and antioxidant activity of Dendrophthoe falcata (L.f.) Ettingsh fruits growing on Cassine glauca (Rottb) O. Ktze from Melghat (M.S). World. J. Pharm. Pharm. Sci. 11 (2), 1218–1228. doi:10.20959/wjpps20222-21156
Dubey, G., Tiwari, D. K., and Tripathi, K. (2015). Comparative evaluation of anti-depressant activity of leaves extract of Dendrophthoe falcata collected from two different host trees. Int. J. Pharm. Phytopharm. Res. 4 (5), 288–290.
Elsyana, V., Bintang, M., and Priosoeryanto, B. P. (2016). Cytotoxicity and antiproliferative activity assay of Clove Mistletoe (Dendrophthoe pentandra (L.) Miq.) leaves extracts. Adv. Pharmacol. Sci. 2016, 3242698. doi:10.1155/2016/3242698
Endharti, A. T., and Permana, S. (2017). Extract from mango mistletoes Dendrophthoe pentandra ameliorates TNBS-induced colitis by regulating CD4+ T cells in mesenteric lymph nodes. BMC Complement. Altern. Med. 17, 468–475. doi:10.1186/s12906-017-1973-z
Endharti, A. T., Sulastri, E., Umanailo, R., YunialceNurseta, T., and Handono, K. (2018a). Mango mistletoe Dendrophthoe pentandra leaf extract acts synergistically with 5-fluorouracil to induce apoptosis and increase p21 expression in human cervical adenocarcinoma HeLa cells by reducing survivin expression. J. App. Pharm. Sci. 8 (7), 10–15. doi:10.7324/japs.2018.8702
Endharti, A. T., Wahyuningtyas, T. E., HardiniHandono, K., Widjajanto, E., and Permana, S. (2018b). Dendrophthoe pentandra leaves extract promotes apoptotic effects of doxorubicin in human breast cancer cell via modulation of intracellular calcium and survivin. J. App. Pharm. Sci. 8 (8), 39–43. doi:10.7324/japs.2018.8806
Endharti, A. T., Wulandari, A., Listyana, A., Norahmawati, E., and Permana, S. (2016). Dendrophthoe pentandra (L.) Miq extract effectively inhibits inflammation, proliferation and induces p53 expression on colitis-associated colon cancer. BMC Complement. Altern. Med. 16, 374–381. doi:10.1186/s12906-016-1345-0
Engeland, K. (2018). Cell cycle arrest through indirect transcriptional repression by p53: I have a dream. Cell Death Differ. 25 (1), 114–132. doi:10.1038/cdd.2017.172
Ermolenko, E. V., Imbs, A. B., Gloriozova, T. A., Poroikov, V. V., Sikorskaya, T. V., and Dembitsky, V. M. (2020). Chemical diversity of soft coral steroids and their pharmacological activities. Mar. Drugs 18 (12), 613. doi:10.3390/md18120613
Ernawati, T., Angelina, M., Dewi, R. T., and Fajriah, S. (2021). Investigation of the potential COVID-19 inhibitor from Cassia alata and Dendrophthoe petandra using computational approach. IOP Conf. Ser. Mat. Sci. Eng. 1011, 012017. doi:10.1088/1757-899x/1011/1/012017
Fàbregas, N., and Fernie, A. R. (2022). The reliance of phytohormone biosynthesis on primary metabolite precursors. J. Plant Physiol. 268, 153589. doi:10.1016/j.jplph.2021.153589
Fahmi, A., Nasution, R. B., and Nainggolan, H. (2018). Antimicrobial and toxicity tests of flavonoid total Dendrophthoe pentandra (L) miq from false ashoke tree (Polyalthia longifolia). J. Pendidik. Kim. 10 (1), 366–370. doi:10.24114/jpkim.v10i1.9677
Fan, H. H., He, S. T., Han, P. J., Hong, B. X., Liu, K., Li, M. C., et al. (2022). Cepharanthine: A promising old drug against SARS-CoV-2. Adv. Biol. 6, e2200148. doi:10.1002/adbi.202200148
Fitrilia, T., Bintang, M., and Safithri, M. (2015). Phytochemical screening and antioxidant activity of clove mistletoe leaf extracts (Dendrophthoe pentandra (L.) Miq). IOSR J. Pharm. 5 (8), 13–18.
Gallo, J. M. (2010). Pharmacokinetic/pharmacodynamic-driven drug development. Mt. Sinai J. Med. 77 (4), 381–388. doi:10.1002/msj.20193
Gangwar, J. P., and Saxena, P. N. (2010). Chemical constituents from Dendrophthoe falcate. An. Univ. Bucuresti Chim. 19 (1), 31–34.
Ghirtlahre, S. K., Awasthi, A. K., Nirala, Y. P. S., and Sahu, C. M. (2015). New record of mistletoe as a potential exotic weed: Serious threat to sapota cultivation in Chhattisgarh. J. Plant Dev. Sci. 7 (3), 217–219.
Guo, X. Y., and Ren, Z. J. (2019). Characterization of the complete plastome of Dendrophthoe pentandra (Loranthaceae), a stem hemiparasite. Mitochondrial DNA Part B 4 (2), 3099–3100. doi:10.1080/23802359.2019.1667280
Gupta, R. S., and Kachhawa, J. B. S. (2008). Contraceptive and toxicological evaluation of Dendrophthoe falcata stem extract in male albino rats. J. Herbs Spices Med. Plants 13 (3), 37–46. doi:10.1300/j044v13n03_04
Gupta, R. S., and Kachhawa, J. B. S. (2007). Evaluation of contraceptive activity of methanol extract of Dendrophthoe falcata stem in male albino rats. J. Ethnopharmacol. 112 (1), 215–218. doi:10.1016/j.jep.2007.02.022
Gyrdymova, Y. V., and Rubtsova, S. A. (2022). Caryophyllene and caryophyllene oxide: A variety of chemical transformations and biological activities. Chem. Pap. 76 (1), 1–39. doi:10.1007/s11696-021-01865-8
Hanboonkunupakarn, B., Tarning, J., Pukrittayakamee, S., and Chotivanich, K. (2022). Artemisinin resistance and malaria elimination: Where are we now? Front. Pharmacol. 13, 876282. doi:10.3389/fphar.2022.876282
Handono, K., Pratama, M. Z., Sermoati, I. A., Yuniati, M. G., Haryati, N. P. S., Norahmawati, E., et al. (2021). The effect of mango mistletoes (Dendrophthoe pentandra) leaves extract on percentage of CD4+ CD28+, CD8+ CD28+, and interleukin-2 levels of aged balb/c mice. Open Access Maced. J. Med. Sci. 9 (A), 414–421. doi:10.3889/oamjms.2021.6182
Haque, A. (2016). Antinociceptive, anti-inflammatory and central nervous system depressant effects of crude ethanol extract and its aqueous, chloroform and petroleum ether fractions of Dendrophthoe falcata (Linn.) stem. Pharmacologyonline 1, 12–21.
Haque, A. (2015). In vitro evaluation of phytochemical profile and antioxidant effect of chloroform and petroleum ether extracts of Dendrophthoe falcata (linn) leaves. Pharmacologyonline 2, 6–13.
Haque, A., Tahmina, A. S., Sarker, I., Hossain, M., Islam, S., and Islam, A. (2014a). Antioxidant and hepatoprotective effects of aqueous and ethanol extracts of Dendrophthoe falcate Linn leaves. Pharmacologyonline 1, 90–101.
Haque, A., Zaman, A., Hossain, M., Sarker, I., Islam, S., and Al Baki, A. (2014c). Evaluation of analgesic, anti-inflammatory and CNS depressant potential of Dendrophthoe falcata (Linn.) leaves extracts in experimental mice model. Am. J. Biomed. Sci. 6 (3), 139–156. doi:10.5099/aj140300139
Haque, A., Zaman, A., Hossain, M., Sarker, I., and Islam, S. (2014b). Evaluation of antidiarrhoeal and insecticidal activities of ethanol extract and its fractions of Dendrophthoe falcata (L.) leaves. Int. J. Pharm. Sci. Res. 5 (9), 3653. doi:10.13040/IJPSR.0975-8232.5(9).3653-63
Hardiyanti, R., Marpaung, L., Adnyana, I. K., and Simanjuntak, P. (2018). Antioxidant and antibacterial activities of various extracts of duku's mistletoe leaf (Dendrophthoe pentandra (L.) Miq) collected from Medan, Indonesia. Asian J. Pharm. Clin. Res. 11 (12), 526–529. doi:10.22159/ajpcr.2018.v11i12.29725
Hardiyanti, R., Marpaung, L., Adnyana, I. K., and Simanjuntak, P. (2019a). Biochemical evaluation of Duku's mistletoe leave (Dendrophthoe pentandra (L.) Miq) extract with antidiabetic potential. Rasayan J. Chem. 12 (3), 1569–1574. doi:10.31788/rjc.2019.1235272
Hardiyanti, R., Marpaung, L., Adnyana, I. K., and Simanjuntak, P. (2019c). Isolation of quercitrin from Dendrophthoe pentandra (L.) Miq leaves and it's antioxidant and antibacterial activities. Rasayan J. Chem. 12 (4), 1822–1827. doi:10.31788/rjc.2019.1235353
Hardiyanti, R., and Marpaung, L. (2018). Phytochemical and toxicity test of n-hexan extracts of duku′s mistletoe leaf (Dendrophtoe pentandra) and (Scurrula ferruginea) collected from Medan, Indonesia. AIP Conf. Proc. 2049, 030015. doi:10.1063/1.5082516
Hasan, M., Ali, M. T., Khan, R., Palit, P., Islam, A., Seel, V., et al. (2018). Hepatoprotective, antihyperglycemic and antidiabetic effects of Dendrophthoe pentandra leaf extract in rats. Clin. Phytosci. 4 (16), 16–17. doi:10.1186/s40816-018-0076-9
Hasan, M. S., Ahmed, M. I., Mondal, S., Masud, M. M., Sadhu, S. K., Ishibashi, M., et al. (2006). Antioxidant, antinociceptive activity and general toxicity study of Dendrophthoe falcata and isolation of quercitrin as the major component. Orient. Pharm. Exp. Med. 6 (4), 355–360. doi:10.3742/OPEM.2006.6.4.355
Hill, R. A., and Connolly, J. D. (2011). Triterpenoids. Nat. Prod. Rep. 28 (6), 1087–1117. doi:10.1039/c1np00012h
Huang, P., Duan, W., Zhang, J., Jiao, J., Leng, A., and Qu, J. (2022). Plant polysaccharides with anti-lung injury effects as a potential therapeutic strategy for COVID-19. Front. Pharmacol. 13, 982893. doi:10.3389/fphar.2022.982893
Irfan, M., Winarno, H., Hanafi, M., and Artanti, Nina. (2020). The isolation and identification of active compound of Dendrophthoe praelonga (Blume) Miq. extract against breast cancer cells (MCF-7). J. Jamu Indones. 5, 85–91. doi:10.29244/jji.v5i3.117
Jadhav, R. B., Anarthe, S. J., Surana, S. J., and Gokhale, S. B. (2005). Host-hemiparasite transfer of the C-glucosyl xanthone mangiferin between Mangifera indica and Dendrophthoe falcata. J. Plant Interact. 1 (2), 171–177. doi:10.1080/17429140600986161
Jain, A., and Sharma, M. (2016). In-vitro antimicrobial screening of Dendrophthoe falcate (L.F.) Ettingsh. Int. J. Curr. Microbiol. Appl. Sci. 5 (12), 594–602. doi:10.20546/ijcmas.2016.512.064
Jalali, J., and Rahbardar, M. G. (2022). Ameliorative effects of Portulaca oleracea L. (purslane) on the metabolic syndrome: A review. J. Ethnopharmacol. 299, 115672. doi:10.1016/j.jep.2022.115672
Jiang, M. Y., Zhao, S. J., Yang, S. S., Lin, X., He, X. G., Wei, X. Y., et al. (2022). An "essential herbal medicine"-licorice: A review of phytochemicals and its effects in combination preparations. J. Ethnopharmacol. 249, 112439. doi:10.1016/j.jep.2019.112439
Joshi, A., Patel, B., and Parmar, R. (2012). Anti-oxidant potential of Dendrophthoe trigona (wt. And Arn.) danser. . J. Curr. Pharma Res. 2 (4), 631–633. doi:10.33786/jcpr.2012.v02i04.004
Kachhawa, J. B., Gupta, R. S., and Sharma, K. K. (2011b). Ethnopharmacological evaluation of male contraceptive efficacy of Dendrophthoe falcata in albino rats. Planta Med. 77 (12), 1316. doi:10.1055/s-0031-1282427
Kachhawa, J., Gupta, R., and Sharma, K. (2011a). Evaluation of contraceptive potential of isolated fractions from Dendrophthoe falcata stem methanol extract in male albino rats. Planta Med. 77 (5), 132. doi:10.1055/s-0031-1273661
Karunaratne, R., and Uduwela, D. (2020). A comparison of the bioactivity of Dendrophthoe falcata on the hosts; Limonia acidissima and Mangifera indica. Ceylon J. Sci. 49 (2), 159–164. doi:10.4038/cjs.v49i2.7736
Kathayi, A., and Nagarajan, N. (2018). Pharmacognostical standardization, antimicrobial and phytochemical studies of Dendrophthoe falcata (L.f) Ettingsh (Loranthaceae) growing on the host plant Azadirachta indica (Mealiaceae). World J. Pharm. Pharm. Sci. 7 (6), 1–10. doi:10.20959/wjpr20184-11545
Katz, L., and Baltz, R. H. (2016). Natural product discovery: Past, present, and future. J. Ind. Microbiol. Biotechnol. 43, 155–176. doi:10.1007/s10295-015-1723-5
Khanbabaee, K., and Van Ree, T. (2001). Tannins: Classification and definition. Nat. Prod. Rep. 18 (6), 641–649. doi:10.1039/b101061l
Khatoon, S., Singh, H., and Goel, A. (2011). Use of HPTLC to establish the chemotype of a parasitic plant,Dendrophthoe falcata(Linn. f.) Etting. (Loranthaceae), growing on different substrates. J. Planar Chromatogr. - Mod. TLC 24 (1), 60–65. doi:10.1556/jpc.24.2011.1.12
Klein, I., Boenert, J., Lange, F., Christensen, B., Wassermann, M. K., Wiesen, M. H., et al. (2022). Glia from the central and peripheral nervous system are differentially affected by paclitaxel chemotherapy via modulating their neuroinflammatory and neuroregenerative properties. Front. Pharmacol. 13, 1038285. doi:10.3389/fphar.2022.1038285
Kothawade, S. N., Shinde, P. B., Agrawal, M. R., Aragade, P. D., and Kamble, H. V. (2010). Preliminary evaluation of Dendrophthoe falcata mucilage as tablet binder. Int. J. PharmTech Res. 2 (2), 1474–1476.
Kristiningrum, N., Wulandari, L., and Zuhriyah, A. (2018). Phytochemical screening, total phenolic content, and antioxidant activity of water, ethyl acetate, and n-hexane fractions from mistletoe Moringa oleifera Lam. (Dendrophthoe pentandra (L.) Miq.) Asian J. Pharm. Clin. Res. 11 (10), 104–106. doi:10.22159/ajpcr.2018.v11i10.25271
Kuramana, S., Gandipilli, G., and Kumar, P. K. R. (2020). Studies on biology, seed dispersal and host interaction of Dendrophthoe falcata (L.f.) etting. - a stem parasite of Mangifera indica L. (Mango). World J. Adv. Res. Rev. 8 (3), 097–103. doi:10.30574/wjarr.2020.8.3.0465
Kwanda, N., Noikotr, K., Sudmoon, R., Tanee, T., and Chaveerach, A. (2013). Medicinal parasitic plants on diverse hosts with their usages and barcodes. J. Nat. Med. 67 (3), 438–445. doi:10.1007/s11418-012-0695-2
Lazuardi, M., Suharjomo, S., Chien, C. H., He, J. L., Lee, C. W., Peng, C. K., et al. (2021). Progesteron-benzeri bileşiklerin %10 lipozom i?çerisinde kapsüllenmesi bu bileşiklerin enjektabl dozaj formunun uygulandığı sıçanlardaki konsantrasyonlarını artırır. Kafkas Univ. Veteriner Fak. Derg. 28, 27–34. doi:10.9775/kvfd.2021.26268
Lazuardi, M. (2008). The in vitro assessment of anti proliferation activity of crude diethyl ether extract of Dendrophthoe species against to myeloma culture cell. Dent. J. Maj. Kedokt. Gigi) 41 (1), 5–9. doi:10.20473/j.djmkg.v41.i1.p5-9
Le Basle, Y., Chennell, P., Tokhadze, N., Astier, A., and Sautou, V. (2020). Physicochemical stability of monoclonal antibodies: A review. J. Pharm. Sci. 109 (1), 169–190. doi:10.1016/j.xphs.2019.08.009
Li, X. Y., Rao, Z. L., Xie, Z. Q., Qi, H., and Zeng, N. (2022). Isolation, structure and bioactivity of polysaccharides from Atractylodes macrocephala: A review. J. Ethnopharmacol. 296, 115506. doi:10.1016/j.jep.2022.115506
Lim, Y. C., Rajabalaya, R., and David, S. R. (2017). A hidden treasure: The borneo mistletoes. Pharmacogn. Rev. 11 (22), 153–157. doi:10.4103/phrev.phrev_16_17
Lobo, R., Dashora, N., Sodde, V., and Prabhu, K. S. (2010). Anticancer activity of Dendrophthoe flacata on ehrlich′s ascites carcinoma treated mice. J. Pharm. Pharmacol. 62 (10), 1248–1249.
Logesh Kumar, P. R., Santhosh Kumar, E., Prasanna, S., Gopalsamy, P., Swetha, D., and Gnanapriya, S. (2019). Anti depressant activity of ethanolic extract of Dendropthoe falcata Linn in mice. World J. Pharm. Pharm. Sci. 8 (4), 1874–1886. doi:10.20959/wjpps20194-13631
Madhuri, M., Deepthi, B., Vinatha, V., and Chmm, P. R. (2013). Analgesic activity of aqueous and alcohol root extracts of shoots of Dendrophthoe falcata. Int. J. Res. Phytochem. Pharmacol. 3 (1), 54–56.
Mallavadhani, U. V., Narasimhan, K., Sudhakar, A. V. S., Mahapatra, A., Li, W., and Van Breemen, R. B. (2006). Three new pentacyclic triterpenes and some flavonoids from the fruits of an Indian ayurvedic plant Dendrophthoe falcata and their estrogen receptor binding activity. Chem. Pharm. Bull. 54 (5), 740–744. doi:10.1248/cpb.54.740
Milner, K. V., Leigh, A., Gladstone, W., and Watson, D. M. (2020). Subdividing the spectrum: Quantifying host specialization in mistletoes. Botany 98 (9), 533–543. doi:10.1139/cjb-2019-0207
Mochamad, L., and Hermanto, B. (2014). Dendrophthoe pentandra methanolic leaf extract increases progesterone levels in female rats. Universa Med. 33 (2), 100–108.
Mochamad, L., Hermanto, B., and Hestianah, E. P. (2019). Determination of progesterone compounds in the crude methanol extract of benalu duku leaves. Vet. World 12 (3), 358–366. doi:10.14202/vetworld.2019.358-366
Mu'nisa, A., Hala, Y., Taiyeb, A. M., and Sari, D. K. (2019). Effect of boiled water parasite kapok (Dendrophthoe pentandra) leaves on total cholesterol levels and histopathology of hepar of hypercholesterolemic mice. Mat. Sci. Forum 967, 51–55. doi:10.4028/www.scientific.net/msf.967.51
Muche, M., Muasya, A. M., and Tsegay, B. A. (2022). Biology and resource acquisition of mistletoes, and the defense responses of host plants. Ecol. Process. 11 (1), 24–13. doi:10.1186/s13717-021-00355-9
Mustarichie, R., Warya, S., Saptarini, N. M., and Musfiroh, I. (2016). Acute and subchronic toxicities of Indonesian mistletoes Dendrophthoe pentandra L. (Miq.) ethanol extract. J. App. Pharm. Sci. 6 (9), 109–114. doi:10.7324/japs.2016.60916
Mustarichie, R., Warya, S., Saptarini, N. M., and Ramdhani, D. (2015). Total flavonoid content and anti-inflammatory properties of Indonesian mistletoes (Dendrophthoe pentandra (L.) Miq.) ethanol extract. World J. Pharm. Res. 4 (4), 287–302.
Mutiah, R., Suryadinata, A., and Nurani, P. S. (2018). Cytotoxic evaluation of cisplatin in combination with ethanolic extract of Avocado parasite (Dendrophthoe pentandra (L) Miq.) against Hela cells. Maj. Kesehat. FKUB 5 (3), 133–143. doi:10.21776/ub.majalahkesehatan.005.03.2
Nair, A. G. R., and Krishnakumary, P. (1990). Flavonoids of Dendrophthoe falcata Ettingsh growing on different host plants. Indian J. Chem. Sect. B 29 (6), 584–585.
Nayak, R. P., Mishra, S., Mahalik, G., and Dimri, R. (2020). Antibacterial activity of Dendrophthoe falcata (lf) ettingsh against Shigella flexneri. Med. Biol. Health Odisha 92, 1–26. doi:10.13140/RG.2.2.25180.21124
Nguyen, H. H., Nguyen, C. H., Nguyen, C. K., Chu, D. K., and Mai, A. H. (2010). Chemical constituent of Dendrophthoe pentandra (L.) miq., (Loranthaceae). Tap. Chi Hoa Hoc 48 (2), 245–249.
Nguyen, H. H., Nguyen, C. H., and Nguyen, C. K. (2011). Study of chemical constituents of Dendrophthoe pentandra (L.) Miq., (Loranthaceae), parasitic plant on Artocarpus integrifolia and Annona squamosa. Tap. Chi Hoa Hoc 49 (2), 181–185.
Nielsen, T. T., Støttrup, N. B., Løfgren, B., and Bøtker, H. E. (2011). Metabolic fingerprint of ischaemic cardioprotection: Importance of the malate-aspartate shuttle. Cardiovasc. Res. 91 (3), 382–391. doi:10.1093/cvr/cvr051
Nigam, Y. P. (2022). The bornean mistletoes as versatile parasites: A systematic review. Syst. Rev. Pharm. 13 (1), 42–47. doi:10.31858/0975-8453.13.1.42-47
Nijveldt, R. J., Van Nood, E., Van Hoorn, D. E. C., Boelens, P. G., Van Norren, K., and Van Leeuwen, P. A. M. (2001). Flavonoids: A review of probable mechanisms of action and potential applications. Am. J. Clin. Nutr. 74 (4), 418–425. doi:10.1093/ajcn/74.4.418
Nurfaat, D. L. (2016). Acute toxicity test of ethanol extract of benalu mangga (Dendrophthoe petandra) on Swiss webster mice. Indones. J. Pharm. Sci. Technol. 3 (2), 53–65.
Omidkhoda, S. F., Razavi, B. M., and Hosseinzadeh, H. (2019). Protective effects of Ginkgo biloba L. against natural toxins, chemical toxicities, and radiation: A comprehensive review. Phytother. Res. 33 (11), 2821–2840. doi:10.1002/ptr.6469
Pare, J., and Raynal-Roques, A. (1999). Embryology, taxonomy and evolution in parasitic flowering plants. Acta Biol. cracov. Ser. Bot. 41, 85–94.
Patel, B., Joshi, A., and Parmar, R. (2012). Phytochemical investigation of Dendrophthoe trigona (wt. And Arn.) danser. . J. Curr. Pharma Res. 2 (3), 580–583. doi:10.33786/jcpr.2012.v02i03.013
Patil, S., Anarthe, S., Jadhav, R., and Surana, S. (2011). Evaluation of anti-inflammatory activity and in-vitro antioxidant activity of Indian mistletoe, the hemiparasite Dendrophthoe falcata L.f. (Loranthaceae). Iran. J. Pharm. Res. 10 (2), 253–259.
Pattanayak, S. P., and Mazumder, P. M. (2009a). Assessment of neurobehavioral toxicity of Dendrophthoe falcata (L.f) Ettingsh in rats by functional observational battery after a subacute exposure. Pharmacogn. Mag. 5 (18), 98–105.
Pattanayak, S. P., and Mazumder, P. M. (2009b). Effect of Dendrophthoe falcata (L.f.) ettingsh on female reproductive system in wistar rats: A focus on antifertility efficacy. Contraception 80 (3), 314–320. doi:10.1016/j.contraception.2009.03.001
Pattanayak, S. P., and Mazumder, P. M. (2011b). Immunomodulatory activities of Dendrophthoe falcata (L.f.) ettingsh in experimental animals: In vitro and in vivo investigations. J. Sci. Res. 3 (3), 619–630. doi:10.3329/jsr.v3i3.7655
Pattanayak, S. P., and Mazumder, P. M. (2010). Phytochemical screening and safety evaluation of hydroalcoholic extract of Dendrophthoe falcata Ettingsh: Summary of acute and subacute toxicological data. Pharm. Lett. 2 (5), 127–138.
Pattanayak, S. P., Mazumder, P. M., and Sunita, P. (2008). Preliminary studies on antinociceptive activity of Dendrophthoe falcata (L.F) Ettingsh (Loranthaceae). Pharmacologyonline 2008 (3), 370–378.
Pattanayak, S. P., Mazumder, P. M., and Sunita, P. (2012). Total phenolic content, flavonoid content and in vitro antioxidant activities of Dendrophthoe falcata (L.f.) Ettingsh. . Res. J. Med. Plant 6 (2), 136–148. doi:10.3923/rjmp.2012.136.148
Pattanayak, S. P., and Mazumder, P. (2011a). Therapeutic potential of Dendrophthoe falcata (L.f.) Ettingsh on 7, 12-dimethylbenz (a) anthracene-induced mammary tumorigenesis in female rats: Effect on antioxidant system, lipid peroxidation, and hepatic marker enzymes. Comp. Clin. Pathol. 20 (4), 381–392. doi:10.1007/s00580-010-1008-3
Pattanayak, S. R., and Sunita, P. (2008). Wound healing, anti-microbial and antioxidant potential of Dendrophthoe falcata (L.f) Ettingsh.f) Ettingsh. J. Ethnopharmacol. 120 (2), 241–247. doi:10.1016/j.jep.2008.08.019
Permana, S., Lukman, H., Norahmawati, E., Puspita, O. E., Faisal, D., Al Zein, M., et al. (2021). The combination therapy of targeting both paclitaxel and Dendrophthoe pentandra leaves extract nanoparticles for improvement breast cancer treatment efficacy by reducing TUBB3 and MAP4 expressions. Acta Biochim. Pol. 68 (4), 679–686. doi:10.18388/abp.2020_5563
Pramestya, C. D., Yasman, Y., Hemelda, N. M., and Handayani, W. (2019). Organo-specific distribution of untargeted metabolomics of mistletoe′s extract, Dendrophthoe pentandra (L.) Miq., from FKM UI, Kampus UI Depok. AIP Conf. Proc. 2168, 020089. doi:10.1063/1.5132516
Prior, J. C. (2018). Progesterone for treatment of symptomatic menopausal women. Climacteric 21 (4), 358–365. doi:10.1080/13697137.2018.1472567
Rafe, M. R., Ahsan, M., Hasan, C. M., and Masud, M. M. (2017). Chemical and biological studies of leaf extract of Dendrophthoe falcata linn. Dhaka Univ. J. Pharm. Sci. 16 (2), 215–219. doi:10.3329/dujps.v16i2.35259
Rahmad, Z. B., Addo-Fordjour, P., Asyraf, M., and Rosely, N. F. N. (2014). Mistletoe abundance, distribution and associations with trees along roadsides in Penang, Malaysia. Trop. Ecol. 55 (2), 255–262.
Rahmawati, S. I., Ishimaru, K., Hou, D.-X., and Hayashi, N. (2014). Antioxidant activity and phenolic content of mistletoe extracts following high-temperature batch extraction. Food Sci. Technol. Res. 20 (2), 201–206. doi:10.3136/fstr.20.201
Rawsthorne, J., Watson, D. M., and Roshier, D. A. (2011). Implications of movement patterns of a dietary generalist for mistletoe seed dispersal. Aust. Ecol. 36 (6), 650–655. doi:10.1111/j.1442-9993.2010.02200.x
Reddy, P. S., Sowmya, B., Sravani, N., Sandhya, P., and Mohan, C. K. (2019). Evaluation of diuretic potential of petroleum ether extract of Dendrophthoe falcata leaves in wistar rats. Asian J. Pharm. Pharmacol. 5 (6), 1086–1090. doi:10.31024/ajpp.2019.5.6.2
Riad, M., Mogos, M., Thangathurai, D., and Lumb, P. D. (2002). Steroids. Curr. Opin. Crit. Care 8 (4), 281–284. doi:10.1097/00075198-200208000-00002
Runtuwene, M., and Kamu, V. (2020). Total phenolic content and toxicity test of Muharang Bawine (Dendrophthoe falcate (Lf) Etinggsh) leaves by BLST method (Brine shrimp lethality test). Chem. Prog. 13 (1), 11–16. doi:10.35799/cp.13.1.2020.28830
Sahakitpichan, P., Disadee, W., Buntawong, R., Chimnoi, N., Ruchirawat, S., and Kanchanapoom, T. (2017). A furan-2-carbonyl C-glucoside and an alkyl glucoside from the parasitic plant, Dendrophthoe pentandra. Phytochem. Lett. 21, 90–93. doi:10.1016/j.phytol.2017.05.024
Saputri, S., Sjakoer, N., and Mubarakati, N. (2021). Effects of mango mistletoe (Dendrophthoe pentandra L. Miq) extracts on brain in hypertensive rats treated with deoxycorticosterone acetate (DOCA)-salt. J. Smart Bioprospect. Technol. 2, 055–060. doi:10.21776/ub.jsmartech.2021.002.02.55
Sathishkumar, G., Gobinath, C., Wilson, A., and Sivaramakrishnan, S. (2014). Dendrophthoe falcata (L.f) ettingsh (neem mistletoe): A potent bioresource to fabricate silver nanoparticles for anticancer effect against human breast cancer cells (MCF-7).f) ettingsh (neem mistletoe): A potent bioresource to fabricate silver nanoparticles for anticancer effect against human breast cancer cells (MCF-7). Spectrochim. Acta Part A 128, 285–290. doi:10.1016/j.saa.2014.02.096
Seca, A. M. L., and Pinto, D. (2018). Plant secondary metabolites as anticancer agents: Successes in clinical trials and therapeutic application. Int. J. Mol. Sci. 19 (1), 263. doi:10.3390/ijms19010263
Sembiring, H. B., Lenny, S., and Marpaung, L. (2016). Antioxidant activity of flavonoid compounds from cocoa pod leaves (Dendrophthoe pentandra (L.) Miq.) Chimica Natura Acta 4 (3), 117–122. doi:10.24198/cna.v4.n3.10920
Sembiring, H. B., Marpaung, L., Basyuni, M., and Simanjuntak, P. (2022). Glycosylated flavonoid compound from cocoa parasite leaves (Dendrophthoe pentandra (L.) Mig.) Rasayan J. Chem. 15 (1), 700–704. doi:10.31788/rjc.2022.1516382
Serrano, J., Puupponen-Pimia, R., Dauer, A., Aura, A. M., and Saura-Calixto, F. (2009). Tannins: Current knowledge of food sources, intake, bioavailability and biological effects. Mol. Nutr. Food Res. 53, 310–329. doi:10.1002/mnfr.200900039
Shahare, N., and Bodele, S. (2019). Phytochemical and GC-MS analysis of Dendrophthoe falcata (lf) ettingsh stem. Eur. J. Med. Plants 29, 1–8. doi:10.9734/ejmp/2019/v29i430159
Shalini, K. S., Yengkhom, O., Subramani, P. A., and Dinakaran, M. R. (2019a). Polysaccharide fraction of the hemi-parasitic mistletoe, Dendrophthoe falcata (L) Ettingsh leaves enhances innate immune responses and disease resistance in Oreochromis niloticus (Linnaeus). Int. J. Res. Pharm. Sci. 10 (1), 56–64. doi:10.26452/ijrps.v10i1.1780
Shalini, K. S., Yengkhom, O., Subramani, P. A., and Michael, R. D. (2019b). Polysaccharide fraction from the Indian mistletoe, Dendrophthoe falcata (L.f.) Ettingsh enhances innate immunity and disease resistance in Oreochromis niloticus (Linn.) Fish. Shellfish Immunol. 88, 407–414. doi:10.1016/j.fsi.2019.03.008
Shantipriya, A., Jatoth, K., Mangilal, T., and Bookya, K. (2015). Comparative pharmacological (antimicrobial) screening of aqueous and alcohol root extracts of shoots of Dendrophthoe falcata. World J. Pharm. Pharm. Sci. 4 (9), 773–778.
Singh, L. J., Ranjan, V., Rasingam, L., and Swamy, J. (2020). A new species of genus Dendrophthoe mart. (Lorantheae-Loranthaceae) from the peninsular India. J. Asia-Pac. Biodivers. 13 (3), 487–493. doi:10.1016/j.japb.2020.03.017
Sinoriya, P., Sharma, V., and Sinoriya, A. (2011). A review on Dendrophthoe falcata (Linn. f.) Asian J. Pharm. Clin. Res. 4 (2), 1–5.
Sivaramakrishna, P., Yugandhar, P., and Ekka, G. A. (2021). A new species Dendrophthoe laljii (Loranthaceae) infesting Artocarpus heterophyllus Lam. (Moraceae) in andaman and nicobar islands, India. J. Asia-Pac. Biodivers. 14 (3), 452–459. doi:10.1016/j.japb.2021.03.010
Sjakoer, N., Mubarakati, N., and Taufiq, A. (2021). Investigation of excellent ACE inhibitor agents from Scurrula atropurpurea and Dendrophthoe pentandra for anti-hypertension. CMUJ. Nat. Sci. 20 (3), e2021068.
Soemardji, A. A., Soeganda, A. G., and Soediro, I. (2005). The acute toxicity of isolates from n-hexane and ethanolic fraction of Dendrophthoe pentandra (L.) Miq. which have immunostimulatory activity. Indones. J. Pharm. 16 (4), 227–231. doi:10.14499/indonesianjpharm0iss0pp227-231
Song, H., Kim, K. I., Han, J. M., Park, W. Y., Seo, H. S., Lim, S., et al. (2022). Ionizing radiation technology to improve the physicochemical and biological properties of natural compounds by molecular modification: A review. Radiat. Phys. Chem. 194, 110013. doi:10.1016/j.radphyschem.2022.110013
Subandrate, S., Diba, M. F., Salni, T., and Nita, S. (2019). Cytotoxicity, antiproliferative and apoptotic effect of n-hexane fraction of lime parasite (Dendrophtoe pentandra). Molekul 14 (1), 1–5. doi:10.20884/1.jm.2019.14.1.442
Subhashini, K., Kumar, P. R., and Gaddeyya, G. (2019). A comprehensive review on Dendrophthoe falcata (lf) ettingsh. (Loranthaceae). Trop. Plant Res. 6 (3), 514–520. doi:10.22271/tpr.2019.v6.i3.064
Susilowati, S., and Sari, I. N. (2020). Comparison of total flavonoid contents of Dendrophthoe Petandra leaves infusion in fresh and dry materials. J. Farm. 9 (2), 33–40. doi:10.37013/jf.v9i2.108
Tennakoon, K. U., Chak, W. H., and Bolin, J. F. (2011). Nutritional and isotopic relationships of selected bornean tropical mistletoe-host associations in Brunei Darussalam. Funct. Plant Biol. 38 (6), 505–513. doi:10.1071/fp10211
Tesitel, J. (2016). Functional biology of parasitic plants: A review. Plant Ecol. Evol. 149 (1), 5–20. doi:10.5091/plecevo.2016.1097
Tioline, N. W., Sinulingga, S., Subandrate, S., Fatmawati, F., and Safyudin, S. (2021). Inhibition effect of Dendrophthoe pentandra (L.) Miq leaves infusion on alpha-glucosidase enzymes. J. Tek. Kim. 27 (3), 82–87. doi:10.36706/jtk.v27i3.767
Wang, L., Kong, D. G., Tian, J. L., Zhao, W., Chen, Y. R., An, Y., et al. (2022). Tapinanthus species: A review of botany and biology, secondary metabolites, ethnomedical uses, current pharmacology and toxicology. J. Ethnopharmacol. 296, 115462. doi:10.1016/j.jep.2022.115462
Westwood, J. H., and Kim, G. (2017). RNA mobility in parasitic plant - host interactions. RNA Biol. 14 (4), 450–455. doi:10.1080/15476286.2017.1291482
Wicaksono, M. H. B., and Permana, S. (2013). Potential of ethanol fraction of Benalu Mangga (Dendrophthoe pentandra) as anti-colon cancer agent in mice (Mus musculus Balb/c) after Dextran Sulvate (DSS) and Azoxymethane (AOM) induction. J. Trop. Biol. 1 (2), 75–79.
Xiao, D., Wang, J., Zhong, Y., Sun, H., Wang, M., Wang, X., et al. (2022). Study on HPLC fingerprint, network pharmacology, and antifungal activity of Rumex japonicus Houtt. J. AOAC Int. 105 (6), 1741–1754. doi:10.1093/jaoacint/qsac079
Yan, X., Li, Q., Jin, L., Wu, S., Duan, W., Chen, Y., et al. (2022). Current advances on the phytochemical composition, pharmacologic effects, toxicology, and product development of Phyllanthi Fructus. Front. Pharmacol. 13, 1017268. doi:10.3389/fphar.2022.1017268
Yang, B., Liu, H. L., Yang, J. L., Gupta, V. K., and Jiang, Y. M. (2018). New insights on bioactivities and biosynthesis of flavonoid glycosides. Trends Food Sci. Technol. 79, 116–124. doi:10.1016/j.tifs.2018.07.006
Yapa, S., Mohotti, A., and Samita, S. (2021). Mistletoe (Dendrophthoe neelgherrensis Wigh & Arn. Tiegh.) parasitism on yield of Nutmeg (Myristica fragrans Houtt.): Have we been underrating the destructivity? Trop. Agric. Res. 33 (1), 18–28. doi:10.4038/tar.v33i1.8533
Yee, L., Fauzi, N., Najihah, N., Daud, N., and Sulain, M. (2017). Study of Dendrophthoe pentandra ethyl acetate extract as potential anticancer candidate on safety and toxicity aspects. J. Anal. Pharm. Res. 6 (1), 1–11. doi:10.15406/japlr.2017.05.00167
Yismairai, E., Hemelda, N. M., and YasmanHandayani, W. (2019). Antioxidant activity of extract of mistletoe, Dendrophthoe pentandra (L.) Miq., lived in three different host plants, collected from Kampus UI, Depok. AIP Conf. Proc. 2168, 020100. doi:10.1063/1.5132527
Zainuddin, N. A. S. N., and Sulâ, M. D. (2015). Antiproliferative effect of Dendrophthoe pentandra extracts towards human breast adenocarcinoma cells (MCF-7). J. Teknol. 77 (2), 35–39. doi:10.11113/jt.v77.5983
Zainuddin, N. A. S. N., Zakaria, Y., and Sul'Ain, M. D. (2018). Dendrophthoe pentandra induced apoptosis and cell cycle arrest at G1/S in human breast adenocarcinoma cells, MCF-7 via up-regulation of p53. J. Appl. Pharm. Sci. 8 (9), 130–141. doi:10.7324/japs.2018.8919
Zamani, A., Mat Jusoh, S. A., Al-Jamal, H. A. N., Sul'ain, M. D., and Johan, M. F. (2016). Anti-proliferative effects of Dendrophthoe pentandra methanol extract on BCR/ABL-positive and Imatinib-resistant leukemia cell lines. Asian pac. J. Cancer Prev. 17 (11), 4857–4861. doi:10.22034/apjcp.2016.17.11.4857
Keywords: traditional uses, phytochemistry, pharmacology, toxicity, applications, Dendrophthoe falcata (L.f.) Ettingsh., Dendrophthoe pentandra (L.) Miq
Citation: Kong D, Wang L, Niu Y, Cheng L, Sang B, Wang D, Tian J, Zhao W, Liu X, Chen Y, Wang F, Zhou H and Jia R (2023) Dendrophthoe falcata (L.f.) Ettingsh. and Dendrophthoe pentandra (L.) Miq.: A review of traditional medical uses, phytochemistry, pharmacology, toxicity, and applications. Front. Pharmacol. 14:1096379. doi: 10.3389/fphar.2023.1096379
Received: 12 November 2022; Accepted: 23 January 2023;
Published: 02 February 2023.
Edited by:
Ligia Salgueiro, University of Coimbra, PortugalReviewed by:
Artur Figueirinha, University of Coimbra, PortugalMuhammad Taher, International Islamic University Malaysia, Malaysia
Copyright © 2023 Kong, Wang, Niu, Cheng, Sang, Wang, Tian, Zhao, Liu, Chen, Wang, Zhou and Jia. This is an open-access article distributed under the terms of the Creative Commons Attribution License (CC BY). The use, distribution or reproduction in other forums is permitted, provided the original author(s) and the copyright owner(s) are credited and that the original publication in this journal is cited, in accordance with accepted academic practice. No use, distribution or reproduction is permitted which does not comply with these terms.
*Correspondence: Honglei Zhou, zhouhongleitcm@126.com; Ruyi Jia, jrycardiology@163.com