- 1Department of Ultrasound, Taizhou Central Hospital (Taizhou University, Hospital), Taizhou, Zhejiang, China
- 2Department of Hepatobiliary Surgery, Hubei Cancer Hospital, Tongji Medical College, Huazhong University of Science and Technology, Wuhan, China
- 3Department of General Surgery, Taizhou Central Hospital (Taizhou University, Hospital), Taizhou, Zhejiang, China
- 4Precision Medicine Center, Taizhou Central Hospital (Taizhou University Hospital), Taizhou, Zhejiang, China
- 5Liyuan Hospital, Tongji Medical College, Huazhong University of Science and Technology, Wuhan, China
Hepatocellular carcinoma (HCC) is one of the most common digestive malignancies. HCC It ranges as the fifth most common cause of cancer mortality worldwide. While The prognosis of metastatic or advanced HCC is still quite poor. Recently, locoregional treatment, especially local ablation therapies, plays an important role in the treatment of HCC. Radiofrequency ablation (RFA) and high-intensity focused ultrasound (HIFU) ablation are the most common-used methods effective and feasible for treating HCC. However, the molecular mechanisms underlying the actions of ablation in the treatments for HCC and the HCC recurrence after ablation still are poorly understood. Hypoxia-inducible factor (HIF), the key gene switch for adaptive responses to hypoxia, has been found to play an essential role in the rapid aggressive recurrence of HCC after ablation treatment. In this review, we summarized the current evidence of the roles of HIF in the treatment of HCC with ablation. Fifteen relevant studies were included and further analyzed. Among them, three clinical studies suggested that HIF-1α might serve as a crucial role in the RAF treatment of HCC or the local recurrence of HCC after RFA. The remainder included experimental studies demonstrated that HIF-1, 2α might target the different molecules (e.g., BNIP3, CA-IX, and arginase-1) and signaling cascades (e.g., VEGFA/EphA2 pathway), constituting a complex network that promoted HCC invasion and metastasis after ablation. Currently, the inhibitors of HIF have been developed, providing important proof of targeting HIF for the prevention of HCC recurrence after IRFA and HIFU ablation. Further confirmation by prospective clinical and in-depth experimental studies is still warranted to illustrate the effects of HIF in HCC recurrence followed ablation treatment in the future.
Introduction
Hepatocellular carcinoma (HCC) is the third most common cancer and the fifth most common cause of cancer mortality worldwide (Calderaro et al., 2022; Sperandio et al., 2022). The high mortality of HCC is attributed to the lack of early detection and few effective therapies, especially for intermediate- or advanced HCC patients (Parikh and Pillai, 2021). With the development of medical technology, various new treatment techniques have brought new opportunities for HCC treatment. At present, hepatectomy, liver transplantation, and ablation are the standard treatment for HCC (Zhuang et al., 2021). However, the advanced neoplastic stage and the shortage of donors limit the application of hepatectomy and liver transplantation. Recently, locoregional treatment, especially local ablation therapies, plays an important role in the treatment of HCC.
Local thermal ablation techniques mainly include radiofrequency ablation (RFA), high-intensity focused ultrasound (HIFU) ablation, laser ablation, and microwave ablation (Jing et al., 2020). Among these, RFA and HIFU have been most frequently used worldwide in the treatment of HCC. RFA heats targeted tissue by ionic friction from current, reducing the local increase in temperature above 60 °C and then causing coagulative necrosis (Yousaf et al., 2020). HIFU employs the ultrasonic wave to heat tumor entities, resulting in coagulative necrosis of tumor tissue (Sofuni et al., 2022). Local ablation therapy is more secure and has fewer complications and shorter hospital stays than hepatectomy (Shin et al., 2021). In addition, RFA can be combined with other therapies to treat HCC, thereby providing a better therapeutic effect and overcoming its limitations. For example, a randomized, controlled pilot study reported that RFA combined with TACE showed better effectiveness than RFA alone for HCC (Morimoto et al., 2010). Nevertheless, due to residual viable tumors after local ablation, the rate of recurrence and metastasis is higher than that for surgical resection in HCC. It is reported that the 5-year overall recurrence rates were 63.5% with RFA, while surgical resection was 41.7% in patients with HCC(Huang et al., 2010). As a result, further studies of the molecular mechanism of HCC relapses after local ablation are thus needed so that novel medication targets can be developed.
Local thermal ablation damage has been found to be divided into three regions (central high-temperature zone, sublethal temperature transition zone, and surrounding normal tissue) (Sauvaget et al., 2022). In the transition zone, the damage of tumors can be reversed and eventually survive, thus resulting in the rapid development of tumors. There are increasing studies suggesting that epithelial-mesenchymal transitions (EMT), autophagy, and the hypoxic microenvironment play crucial roles in subsequent progression and metastasis after local ablation. Li et al. (2022) found that insufficient RFA (IRFA) promoted proliferation, invasion, migration, and EMT in HCC cells. Zhao et al. (2018) reported that autophagy has been shown to be activated in mice exposed to IRFA. Importantly, hydroxychloroquine (HCQ), a well-established inhibitor of autophagy, significantly suppressed HCC proliferation and recurrence induced by IRFA (Zhao et al., 2018). Recently, Frenzel et al. (2018) suggested that hypoxia-inducible factor (HIF), the key gene switch for adaptive responses to hypoxia, played an important role in the rapid aggressive recurrence of HCC after RFA. Currently, there is still a lack of narrative reviews on the role of HIF in the local ablation of HCC. In this review, we present the first attempt to comprehensively summarize the recent advances of HIF in local ablation of HCC. The aim of this article is to facilitate the clinical application of HIF in inhibiting the rapid aggressive recurrence of HCC after RFA.
Overview OF HIF
HIF, a heterodimeric transcription factor, plays a pivotal role in the ability to adapt to changes in oxygen levels (Kling et al., 2021). HIF was first described by Semenza and others in 1995 (Wang et al., 1995; Wang and Semenza, 1995). In 1991, he found a hypoxic inducible nuclear factor, bounding to the promoter of the EPO gene, and then increasing its expression (Semenza et al., 1991). This nuclear factor has been named HIF by Semenza and others in 1995 (Wang et al., 1995; Wang and Semenza, 1995). The huge importance of this discovery was reflected by the 2019 award of the Nobel Prize in Physiology or Medicine (Fitzpatrick, 2019). HIF is composed of α and β subunits. The α subunit is modulated in an oxygen-dependent manner but the β subunit is constitutively expressed (Macedo-Silva et al., 2020). There are three variants of the α subunit, i.e., HIF-1α, HIF-2α, and HIF-3α, and three paralogues of the β subunit, i.e., HIF-1β, HIF-2β, and HIF-3β (Tsai et al., 2020). HIF-1α is the most ubiquitously expressed of the three isoforms and has 48% amino acid sequence identity to HIF-2α (Fitzpatrick, 2019). It has been reported that HIF-1α and HIF-2α played a key role in the acute and chronic response to hypoxia, respectively (Galan-Cobo et al., 2015; Guo et al., 2021). Due to the existence of multiple HIF-3α variants, it is less well characterized. HIF-α is regulated by oxygen-dependent pathways. The HIF-α subunits are synthesized at a very high rate but undergo rapid degradation via oxygen-dependent prolyl hydroxylase (PHD) enzymes in the presence of oxygen (Strowitzki et al., 2019). The PHD enzymes could hydroxylate Pro402 and Pro564, two conserved proline residues (Snell et al., 2014). The post-translational hydroxylation enables the von Hipple-Lindau (VHL) to bind the HIF-α subunit for degradation (Liu et al., 2018). The human genome encodes three types of PHD enzymes, i.e., PHD1, 2, and 3 (Fujita et al., 2012). It has been reported that PHD2 regulated HIF-1α, while PHD1 regulated HIF-2α(Ren et al., 2011; Vara-Perez et al., 2021). As oxygen availability decrease, the PHDs activity is diminished, leading to the translocation of HIF-α into the nucleus (Nguyen et al., 2021). HIF-α binds to a hypoxia response element in the nucleus and results in the activation of target genes, which facilitates adaptation and survival of cells and contributes to angiogenesis, proliferation, and metastasis of the tumor (Kaelin and Ratcliffe, 2008).
Mounting evidence demonstrates that HIF may correlate with numerous human diseases (e.g., breast cancer, cervix cancer, and HCC) (Feng et al., 2020; Lal et al., 2021). As known, hypoxia is a feature of most solid tumors, where the oxygen level is usually below 1% (D'Ignazio et al., 2017). A series of reactions is caused by hypoxia, which affects tumor survival and progression and confers resistance to chemoradiotherapy by influencing angiogenesis and metabolism (Muz et al., 2015). HIF has been shown to be the main regulator of these responses (Rocha, 2007). Previous studies have shown that the expression levels of HIF-1α and/or HIF-2α were increased in various tumors such as breast cancer, prostate cancer, and pancreatic cancer, and were correlated with poor survival (Shaida et al., 2008; Zhang et al., 2017; De Francesco et al., 2018; Zhang H. S et al., 2019). Also (Hu et al., 2021), demonstrated that hypoxia significantly induced high expression of HIF-1α and HIF-2α, which promoted the proliferation, migration, and invasion of HCC cells. It was reported that the enhancement of HIF activity promoted tumor metastasis through the regulation of hundreds of genes related to immune escape, cancer stem cell maintenance, angiogenesis, and EMT (Rankin and Giaccia, 2016; Schito and Semenza, 2016). In addition to regulation by PHD and VHL mentioned above, the expression of HIF is also regulated by inflammation and epigenetic regulator in HCC. In the presence of persistent hypoxia, IL-1β is released by the necrotic debris of HCC cells, which promotes HIF-1α synthesis via cyclooxygenase 2 (COX-2) (Zhang et al., 2018). The histone deacetylase 5 (HDAC5), an epigenetic regulator, is essential for activating gene transcription and is also involved in the development and progression of tumors (Zhou et al., 2021a). The expression of HDAC5 has been suggested to be elevated in HCC tissues and cells compared with non-cancerous HCC tissues and control cells (Feng et al., 2014). The elevated expression of HDAC5 can induce the transcription of HIF-1α and lead to high expression of HIF-1α by silencing homeodomain-interacting protein kinase-2 (HIPK2) (Ye et al., 2017).
Studies have shown a close association between HIF and recurrence after ablation (Ikemoto et al., 2017). Previous studies indicated that ablation caused hypoxia adjacent to the ablated area, which is related to tumor proliferation, angiogenesis, and aggressiveness (Wan et al., 2016a; Gong et al., 2019). Moreover, the hypoxic status induced by ablation elicits HIF accumulation (Zhang J et al., 2021). (Wan et al., 2016b) discovered that the HSP70/HIF-1α signaling pathway was significantly enhanced in hyperthermia-induced lung cancer cells. Meanwhile, hyperthermia-induced lung cancer cells possess higher proliferation and angiogenesis potential (Wan et al., 2016a). The analogous result was observed in HCC. Kong et al. (2012) found that the expression level of HIF-1α was upregulated in heat-treated HCC cells. Also, a stronger pro-angiogenic effect was observed in heat-treated HCC cells (Kong et al., 2012). Importantly, this effect could be inhibited by HIF-1a inhibitor YC-1 (Kong et al., 2012). These results suggested that HIF may play a crucial role in the rapid progression of residual cancer after ablation.
Literature search
To maximally identify the eligible articles related to the role of HIF in HCC after ablation, we carried out a systematic search of MEDLINE, Google Scholar, EMBASE, and Cochrane Library databases. The search keyword in MEDLINE was: ((((((((((((((((((((“Carcinoma, Hepatocellular" [Mesh]) OR (Carcinomas, Hepatocellular)) OR (Hepatocellular Carcinomas)) OR (Liver Cell Carcinoma, Adult)) OR (Liver Cancer, Adult)) OR (Adult Liver Cancer)) OR (Adult Liver Cancers)) OR (Cancer, Adult Liver)) OR (Cancers, Adult Liver)) OR (Liver Cancers, Adult)) OR (Liver Cell Carcinoma)) OR (Carcinoma, Liver Cell)) OR (Carcinomas, Liver Cell)) OR (Cell Carcinoma, Liver)) OR (Cell Carcinomas, Liver)) OR (Liver Cell Carcinomas)) OR (Hepatocellular Carcinoma)) OR (Hepatoma)) OR (Hepatomas)) AND (((Hypoxia Inducible Factor) OR (Hypoxia-Inducible Factor)) OR (HIF))) AND (Ablation). The inclusion criteria are as follows: 1) clinical study reporting on the roles of HIF in HCC after ablation; 2) experimental research reporting on the effects of HIF in HCC after ablation and its possible molecular mechanisms. Finally, fifteen clinical or experimental studies were included for further analysis. The researchers then used a specific data collection table to extract relevant data from each study, including the general information of the article (e.g., the first author’s name, the year of publication), research subject (e.g., cell/animal model or patient), ablation surgical type, associated genes/pathways and agents, measure method of HIF, and the major findings of the study. Table 1 summarizes the relevant studies reporting the roles of HIF on HCC after ablation.
Clinical implications of HIF-1α in HCC after ablation
Among the 15 included studies, three clinical studies reported the clinical roles of HIF-1α on HCC after ablation. Yamada et al. (2014) conducted a clinical study of Eighty-eight (88) patients with HCC. These patients were categorized into two groups (i.e., RFA group and non-RFA group). There was no significant difference in TMN staging, number of tumors, maximum tumor diameter, and tumor markers between the two groups. The overall 5-year survival rate of the RFA groups was lower than those of non-RFA groups (39% vs. 68%; p = 003). As identified through qRT-PCR, the RFA group showed increased expression not only of HIF-1α, but also of epithelial cell adhesion molecule (EpCAM), a marker for tumor cell growth and metastasis, compared to the non-RFA group. Of note, the expression level of angiogenic factors VEGF was not different between the two groups. Yamada et al.‘s study revealed that the local recurrence of HCC after RFA was significantly associated with the increased expressions of HIF-1 and EpCAM, but not the expressions of VEGF (Yamada et al., 2014). As is well known, sorafenib, an oral multi-kinase inhibitor, can block the proliferation and induce cell apoptosis, which has been widely to treat advanced HCC. Gong et al. (2017) assigned 50 patients with HCC to control group and 40 patients with HCC to observation group. Two groups of patients were treated with conventional RFA alone and with a combination of RFA and oral sorafenib, respectively (Gong et al., 2017). The patients in the observation group showed more prolonged tumor-free survival (12.3 vs. 8.4 months), lower relapse (15.0% vs. 34.0%), and longer survival rates (87.5% vs. 70.0%) than those in the control group (Gong et al., 2017). Furthermore, the overall treatment efficacy in the observation group was superior to that in the control group (82.5% vs. 62.0%); however, the incidence of complications showed no differences between the two groups (17.5% vs. 20.0%) (Gong et al., 2017). The author further found that in comparison to the average levels before treatment, the average levels of serum VEGF and HIF-1α were decreased after the treatment (Gong et al., 2017). This study suggested that sorafenib in combination with RFA was a superior treatment for HCC and HIF-1α and VEGF played key roles during the treatment process. Similarly, another study performed by Yuan et al. (2017) showed that the combination of transcatheter arterial chemoembolization (TACE) and RAF significantly decreased liver cancer angiogenesis and reduced HCC cell proliferation speed than TACE alone. Moreover, the TACE combined with RAF reduced the expression levels of HIF-1α and early growth response protein 2 (EGR-2) to a greater extent than TACE alone (Yuan et al., 2017). In summary, the above three clinical studies suggested that HIF-1α might serve a crucial role in the RAF treatment of HCC or the local recurrence of HCC after RFA.
The roles of HIF and the related signaling pathway in HCC after ablation
HIF-1α contributes to the progression of HCC by promoting the expression of VEGFA and arginase-1
Arginase, a pivotal metabolic enzyme, is a part of the urea cycle and catalyzes the hydrolysis of L-arginine, resulting in ammonia detoxification in mammals (Cziraki et al., 2020). It has two isoforms: arginase-1 and arginase-2, localized in the cytosol and mitochondria, respectively (Labib et al., 2020). Arginase-1 is primarily detected in hepatocytes and can be served as an important marker of hepatocellular differentiation (Moudi et al., 2020). Recently, arginase-1 has been reported to be induced in activated macrophages and participates in the initiation and progression of various diseases, including HCC (You et al., 2018). In addition, HIF-1α is reported to be involved in the expression of the arginase-1 (Alexander et al., 2020). The HIF well-studied target is the vascular endothelial growth factor A (VEGFA) (Palazon et al., 2017). It is the main stimulator of tumor angiogenesis and is reported to be overexpressed in multiple solid cancers, including HCC (Zou et al., 2020). Lin et al. (2018) demonstrated that long non-coding RNA UBE2CP3, a cancer-promoting gene of HCC, enhanced the progression of liver cancer by promoting angiogenesis through the activation of ERK1/2/HIF-1α/VEGFA signaling pathway. A recent study conducted by Halpern et al. (2021) showed that a metastatic tumor model was established using a splenic injection of colon adenocarcinoma cells, and all mice undergoing RFA developed tumors at the ablation site on necropsy performed at 7 days. In contrast, the site of probe insertion had no tumors in the mice undergoing a sham procedure (Halpern et al., 2021). Furthermore, the expression of HIF-1α and VEGFA was significantly increased at the ablation site relative to unaffected adjacent liver tissues from the same mouse (Halpern et al., 2021). The expression of mRNA of HIF-1α, VEGFA, and arginase-1 were also significantly elevated in RFA/tumor-associated macrophages compared with that in unaffected liver (Halpern et al., 2021). Further study found that the tumor volume and the median number of total metastases were significantly decreased in mice treated with YC-1, a well-established inhibitor of HIF-1α, compared to vehicle control (Halpern et al., 2021). In agreement with the above study, Xu et al. (2013) found that the expression of HIF-1α and VEGFA were significantly increased in hepatic tumor model mice after RFA and this response could be inhibited by the combination of sorafenib and RFA. Therefore, HIF-1α might promote the development of hepatic metastases after RFA by increasing the expression of VEGFA and arginase-1.
HIF-1 and 2α promote angiogenesis and residual recurrence of HCC after HIFU ablation via VEGFA/EphA2 pathway.
High-intensity focused ultrasound (HIFU) is another local ablative therapy and is also difficult to achieve complete ablation of HCC, which contributes to the recurrence of HCC. Erythropoietin-producing hepatocellular A2 (EphA2), a member of the Eph family of receptor tyrosine kinases, is a crucial regulator of tumorigenesis and is highly expressed in multiple cancers, including HCC (Wilson et al., 2021). EphA2 has been shown to be activated through the phosphorylation on serine 897 mediated by AKT, RSK, and PKA kinases and alters downstream signaling, and then facilitating tumor progression (Zhou et al., 2015; Barquilla et al., 2016). Niu et al. (2021) reported that EphA2 was highly expressed in HCC. Additionally, EphA2 silencing significantly reduced cell proliferation and accelerated apoptosis (Niu et al., 2021). A study conducted by Cheng et al. (2002) demonstrated that EphA2 antisense oligonucleotides significantly suppressed endothelial expression of the EphA2 receptor and inhibited VEGF-induced cell migration. A recent study showed that the knockdown of HIF-1α by siRNA downregulated the expression of EphA2 and led to apoptosis and the disruption of vasculogenic mimicry (VM) associated phenotypes (Saha et al., 2022). Nevertheless, it is unknown whether EphA2 functions in HCC after HIFU ablation. Wu et al. (2014) investigated the roles of EphA2, VEGFA, and HIF-1α in a xenograft model of HCC in nude mice. The authors found that the protein and mRNA levels of HIF-1α and HIF-2α were significantly increased in the residual tumor tissues of the HIFU group compared to that in the control group (Wu et al., 2014). Furthermore, similar results were obtained for VEGF-A and EphA2 expression (Wu et al., 2014). The expression of CD31 is considered an indicator of calculated the microvascular density (MVD) and is significantly increased compared with the control grou p (Wu et al., 2014). Importantly, the alteration of CD31, VEGF-A, and EphA2 expression were basically consistent with the trends in HIF-1α and HIF-2α expression (Wu et al., 2014). Therefore, the authors suggested HIFU ablation might result in the hypoxia condition of residual tumor and induce tumor angiogenesis via HIF-1, 2α/VEGFA/EphA2 (Wu et al., 2014). Two other studies conducted by Wu et al. (2017); Wu et al. (2021) also demonstrated that overexpression of EphA2, VEGFA, and HIF-2α were closely associated with angiogenesis in residual HCC after HIFU ablation. Moreover, the expression of EphA2, VEGFA, and HIF-2α could be significantly inhibited by sorafenib (Wu et al., 2021). Thus, inhibiting HIF-1, 2α alone or in combination with angiogenesis inhibitors might prevent residual recurrence of HCC after HIFU ablation.
HIF-1α and HIF-2α promote liver metastases of colon carcinoma following RFA via the activation of downstream markers CA-IX and VEGF
The induction of angiogenesis is an important biological process in responseto hypoxia (Ben et al., 2022). Also, active pH regulation is an essential biological process in response to hypoxia, which allows tumor cells to maintain viability and proliferation in the acidic tumor microenvironment (Porter and Porter, 2018). Carbonic anhydrase IX (CA IX) has been proven to be a key component of this pH regulatory machinery (Benej et al., 2020). CA IX, a transmembrane protein, catalyzes the reversible dehydration of bicarbonate. In recent studies, CA IX is served as a potent biomarker of poor patient prognosis for many types of solid tumors, including HCC (Parks and Pouyssegur, 2017; Chen et al., 2018). Cho et al. (Cho et al., 2019) demonstrated that CA IX has overexpressed in HCC and CA IX inhibitor (acetazolamide) significantly suppressed the growth of HCC xenograft tumors in nude mice. In addition, HCC patients with high CA IX expression displayed a worse prognosis in the TCGA database (Cho et al., 2019). CA IX has been reported to be a downstream marker of HIF (Nijkamp et al., 2009). Nijkamp et al. (2009) established preclinical models with colorectal micrometastases to investigate the effect of RFA on the outgrowth of tumor cells at the lesion periphery. The authors found that tumor load in the transition zone (TZ) significantly increased after RFA compared with tumor load in the livers of sham-operated mice (48.5 ± 3.9% vs. 17.9 ± 2.0%, p = 0.00021) (Nijkamp et al., 2009). Furthermore, tumor load in the TZ following RFA had increased approximately 4-fold compared with tumor load in the reference zone (RZ) (Nijkamp et al., 2009). HIF-1α, HIF-2α, CA-IX, and VEGF were significantly up-regulated in the TZ (Nijkamp et al., 2009). As is noted, 17DMAG, an inhibitor of HIF, suppressed the expression of HIF-1α and HIF-2α, and significantly reduced tumor growth in the TZ (Nijkamp et al., 2009). However, 17DMAG had no obvious effect on tumor growth in the RZ of RFA-treated mice (Nijkamp et al., 2009). The above studies suggested that HIF-1, 2α might promote liver metastases of colon carcinoma following RFA by enhancing the expression of CA-IX and VEGF, the downstream markers of HIF.
HIF-1α/BNIP3 pathway promotes residual HCC cell progression by enhancing autophagy after IRFA
Autophagy is a highly conserved, intracellular self-protective process, critically required for the degradation of damaged organelles and cytoplasmic material (Verma et al., 2021). There are accumulating data showing that autophagy plays a key role in a variety of tumors, including HCC. In pancreatic cancer, autophagy promoted tumor growth in syngeneic host mice, but the inhibition of autophagy reduced tumor growth by restoring surface levels of major histocompatibility complex class I (Yamamoto et al., 2020). In HCC, the expression of LC3-II, a key autophagic marker, was significantly increased and associated with poor prognosis of HCC. Moreover, inhibition of autophagy reduced the ability of tumor cells to survive (Han et al., 2021; Zhang K et al., 2021). Wang et al. (2018) found that the activity of autophagy was markedly enhanced in the residual HCC cells after RFA and the autophagy inhibitor 3-methyladenine (3-MA) significantly inhibited the cell viability and invasion induced by IRFA. Recently, mounting studies have shown that Bcl-2 19-kDa interacting protein 3 (BNIP3) is served as a mitochondrial protein and plays a critical role in autophagy. For example, Xu et al. (2021) reported that latent membrane protein1 (LMP1) promoted radioresistance by inducing autophagy through BNIP3 in nasopharyngeal carcinoma. Additionally, BNIP3 can be induced by hypoxia and is confirmed to be the target molecule of HIF-1α (Chen W et al., 2016). Zhang Y. et al. (2019) demonstrated that HIF-1α played a protective role against myocardial ischemia-reperfusion injury by inducing BNIP3-mediated autophagy. However, the role of HIF-1α/BNIP3-mediated autophagy in RFA-induced HCC promotion remains unclear. A recent study conducted by Xu et al. (2019) showed that the proliferation, migration, and invasion abilities of residual HCC cells were significantly elevated after the IRFA was simulated in vitro. Compared with the 37°C-si-NC group, the expression of LC3B-II, HIF-1α and BNIP3 were significantly increased in the 47°C-si-NC group, which indicated that IRFA could induce the activation of autophagy and the upregulation of HIF-1α and BNIP3 in HCC cells (Xu et al., 2019). Notably, BNIP3 silencing significantly decreased the expression levels of HIF-1α and LC3B-II as well as slowed the proliferation, migration, and invasion of HCC cells mediated by IRFA (Xu et al., 2019). These studies revealed that IRFA promoted residual HCC cell progression by inducing autophagy via the HIF-1α/BNIP3 pathway.
ATO inhibits tumor growth and angiogenesis of HCC by blocking the paracrine signaling of Ang-1 and Ang-2 through the inhibition of the p-Akt/HIF-1α pathway after IRFA
Angiogenesis is an essential process in the growth and metastasis of HCC (Zhu et al., 2022). Angiogenesis is not only regulated by VEGF but also by angiopoietin-1 (Ang-1) and angiopoietin-2 (Ang-2), and their receptor Tie-2 (Engin et al., 2012). Ang-1 and Ang-2 are the members of the angiopoietin (Ang) family and regulate angiogenesis via the TEK tyrosine kinase endothelial receptor (Lin et al., 2020; Xie et al., 2020). Also, Ang-1 and Ang-2 have been shown to be the prognostic biomarkers of HCC(Lin et al., 2020; Xie et al., 2020). Pestana et al. (2018) revealed that a high level of Ang-2 was closely associated with the poor prognosis of HCC patients. In contrast, HCC patients with a high level of Ang-1 showed a longer overall survival (Pestana et al., 2018). Contrary to the studies discussed above, another study found that activated hepatic stellate cells (aHSCs) promoted angiogenesis through secreting Ang-1 (Lin et al., 2020). Wang Z et al. (2021) reported that morphine significantly promoted angiogenesis by activating Akt/HIF-1α pathway in HCC. However, whether Akt/HIF-1α axis is involved in the progression of residual HCC after RFA by modulating Ang-1 or Ang-2 was still unclear. A more recent study indicated that IRFA was simulated using a water bath and promoted tumor growth and angiogenesis of HCC in vitro and in vivo (Dong et al., 2022). The above phenomenon could be suppressed by arsenic trioxide (ATO) (Dong et al., 2022). Furthermore, higher levels of Ang-1, Ang-2, and p-Tie2 were detected in a conditioned medium from RFA-treated than from untreated HCC cells (Dong et al., 2022). The expression of HIF-1α and p-Akt were also upregulated following RFA in HCC cells (Dong et al., 2022). The levels of Ang-1, Ang-2, p-Tie2, HIF-1α, and p-Akt were suppressed by ATO (Dong et al., 2022). Further studies found that Ang-1 or Ang-2 knockdown impaired the ability of the conditioned medium to promote angiogenesis (Dong et al., 2022). Similar results can be obtained when Tie2 expression was silenced using siRNA (Dong et al., 2022). In addition, YC-1, an inhibitor of HIF-1α, markedly inhibited the increased expression of Ang-1 and Ang-2 in HCC cells (Dong et al., 2022). The levels of Ang-1, Ang-2, p-Tie2, and p-Akt were upregulated following HIF-1α overexpression, and the effect of ATO was attenuated (Dong et al., 2022). These studies indicated that ATO suppressed tumor growth and angiogenesis of HCC by regulating paracrine Ang-1 and Ang-2 secretion through the p-Akt/HIF-1α pathway after IRFA.
HIF-1α facilitates the progression of HCC by promoting the warburg effect and EMT after IRFA
Continuous aerobic glycolysis has been demonstrated to trigger oncogene development in the cancer cells. Aerobic glycolysis is known as the Warburg effect and is one of the tumor hallmarks (Liu et al., 2022a). The enhanced Warburg effect is closely linked to EMT and poor patient prognosis in multiple cancer, including HCC. Zhou et al. (2021b) reported that zinc finger E-box-binding homeobox 1 (ZEB1), predicting worse overall survival in cancer patients, facilitated tumorigenesis and metastasis of HCC by enhancing the Warburg effect. It was reported that HIF-1α regulated the Warburg effect by influencing glycolysis, accumulation of lactic acid, and infiltration of the extracellular matrix (Zhou et al., 2022). Lyu et al. (2022) reported that HIF-1α accelerated the Warburg effect and promoted ovarian cancer tumorigenesis by upregulating WT1-associated protein. Liu et al. (2021) demonstrated that by regulating the miR-30c/HIF-1α pathway, FBI-1, an important regulator of HCC, promoted the Warburg effect or EMT of HCC cells and contributed to the drug resistance of HCC cells. A recent study demonstrated that the Warburg effect and level of HIF-1α are enhanced in HCC cells after sublethal heat stress (Chen et al., 2021). 2-NBDG uptake experiment showed that a dramatically increased uptake of glucose was observed in HCC cells after sublethal heat stress (Chen et al., 2021). In addition, HCC cells under normal glucose conditions had a higher survival rates following sublethal heat stress than that under complete glucose-deprivation conditions (Chen et al., 2021). These data indicated that the increased uptake of glucose induced by Warburg effect promoted HCC cell proliferation and invasion (Chen et al., 2021). Further study found that dimethyloxallyl glycine, a specific HIF-1α agonist, improved glucose uptake, and promoted glycolysis-related markers (GLUT-1/3 and LDHA) expression in HCC cells (Chen et al., 2021). Taken together, HIF-1α might facilitate the progression of HCC by regulating the Warburg effect after IRFA.
As known, E-cadherin, N-cadherin, vimentin, and Snail are the markers of EMT (Cessna et al., 2022). Tong et al. (2017) mimicked the IRFA through thermal treatment under hypoxic conditions and found that the protein levels of HIF-1α, N-cadherin, vimentin, Snail, and TGF-β1 following IRFA were significantly elevated, while the expression of E-cadherin was reduced in HCC cells. Moreover, the formation of a hypoxic microenvironment following IRFA significantly promotes HCC cell migration and invasion. Furthermore, N-cadherin, vimentin, Snail, and TGF-β1 expression in shHIF-1α cells were decreased in conjunction with an increased in E-cadherin expression. Previous studies have already demonstrated that TGF-β1 promoted EMT by increasing the expression of vimentin and Snail in HCC (Chen and Yan, 2021; Zhang Z et al., 2021). Of note, upregulation of vimentin and Snail induced by IRFA could be completely inhibited by pretreatment with SB431542, an inhibitor of the TGF-β receptor. All of these data indicated that HIF-1α promoted EMT by TGF-β1 after IRFA, contributing to HCC invasion and metastasis. Figure 1 shows the potential molecular mechanisms of HIF-1, 2α promote angiogenesis and residual recurrence of HCC after IRFA and HIFU ablation.
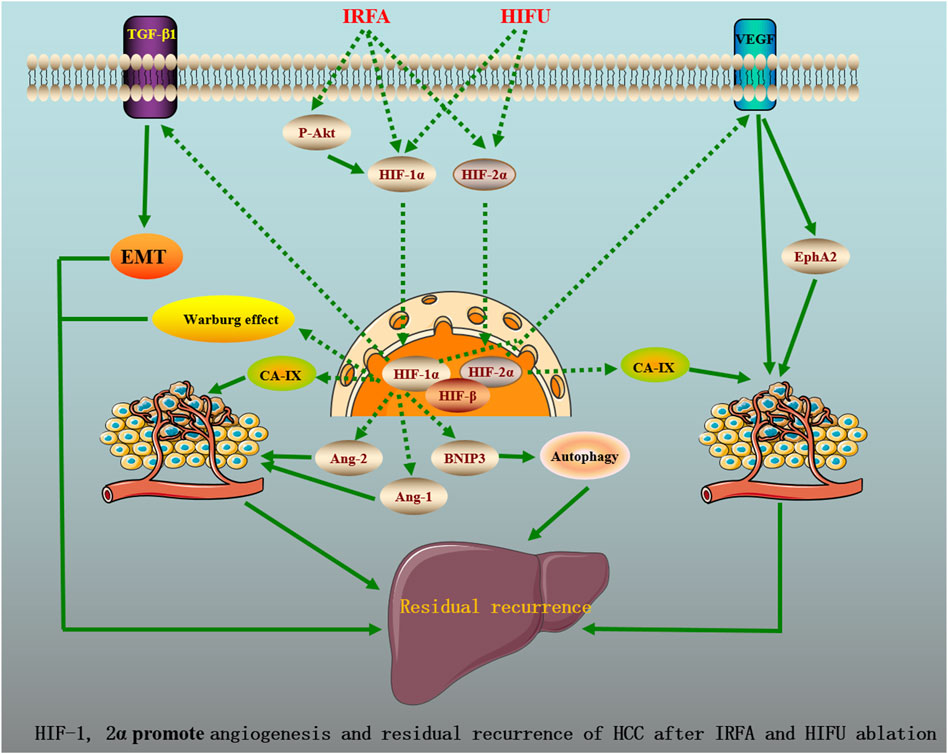
FIGURE 1. HIF-1, 2a promote angiogenesis and residual recurrence of HCC after IRFA and HIFU ablation.
Roles of HIF inhibitors for the treatment of HCC and its prospects
von Hippel-Lindau (VHL) serves as a tumor suppressor gene. Hypoxia enhances phagocytosis in neutrophils, and in neutrophils containing a mutated VHL, increased HIF-1α levels lead to decreased apoptosis and increased bacterial phagocytosis under normoxia conditions. HIF-1α can be induced by hypoxia or mutations of VHL. VHL is required to mediate the degradation of HIF-1α in the ubiquitin-proteasome pathway. It was reported that inactivation of the VHL tumor suppressor gene, which results in pseudohypoxia stabilization of both HIF-1α and HIF-2α, is an initiating genetic event for both hereditary (VHL disease) and sporadic renal cell carcinoma (RCC) (Kaelin, 2007). Genetic and functional studies have supported a protumorigenic role of HIF-2α and a tumor suppressor role for HIF-1α in ccRCC, which has prompted the development of HIF-2α–specific inhibitors (Choueiri and Kaelin, 2020). HIF-1α is believed to play an important role during RCC initiation and is elevated in the earliest preneoplastic lesions in VHL patients (Mandriota et al., 2002).
Now, selective HIF-2α antagonists (PT2385 and PT2399) that inhibit HIF-2 transcriptional activity were identified. PT2399 suppressed tumor growth in most human patient-derived xenografts (PDXs) with greater antitumor activity than that of the tyrosine kinase inhibitor (TKI) sunitinib (Chen J et al., 2016). PT2977 (also known as MK-6482, belzutifan), a PT2385 derivative with an improved pharmacokinetic profile and potency, demonstrated promising single-agent activity in heavily pretreated patients with advanced RCC and VHL-associated non-metastatic ccRCC and was recently approved by the FDA for the treatment of cancers associated with VHL disease (Courtney et al., 2020). Inconsistent results were found in the common isoforms of HIF, HIF-1α, and HIF-2α. A recent study using tissue from 380 patients revealed a significant association of high HIF-2α with increased OS, whereas high HIF-1α was significantly associated with higher-grade tumors and reduced OS in both the univariable and multivariable settings (Cowman et al., 2020). As aforementioned, HIF-1α was considered to be a tumor suppressor. This is inconsistent with the finding that high HIF-1α expression was associated with poor survival in RCC (Minardi et al., 2015). Therefore, the inhibition of HIF-1α should continue to be explored as a therapeutic strategy for RCC.
In the HCC setting, HIF-α inhibitors may also play roles in the treatment of HCC. Clinical data have demonstrated that overexpressed HIF-1α and HIF-2α in HCC patients are reliable markers of a poor prognosis (Dai et al., 2018; Mendez-Blanco et al., 2018). At present, only one clinical study was available on ClinicalTrials.gov and Pubmed. This phase I study evaluated the intravenous infusion effect of the HIF- 1α mRNA antagonist RO7070179 in HCC patients failing to respond to systemic therapy, but this study did not show the results [ClinicalTrials.gov Identifier: NCT02564614].
Of note, the majority of studies are preclinical findings. For example, Salman et al. (2022) showed that HIF-1 and HIF-2 inhibitor 32-134D eradicate HCC in combination with anti-PD1 therapy . LW6 is a drug that inhibits hypoxia by reducing HIF-1α accumulation and gene transcriptional activity. Xu et al. (2022) found that LW6 can promote apoptosis of HCC cells by inhibiting HIF-1α, inhibiting tumor angiogenesis, and downregulating the expression of PD-L1, which is an effective choice for the treatment of HCC. Mu et al. (2021) found that HIF-2α knockdown decreased the expression of downstream c-MYC, suppressed hypoxic cell proliferation, and induced HCC cell apoptosis, whereas HIF-1α knockdown did not. Wu et al. the expression of HIF-2α can be inhibited by sorafenib, which is likely to provide an effective adjunct treatment for patients with HCC following HIFU ablation (Wu et al., 2021).
Based on the above evidence, preclinical studies demonstrated that HIF inhibitors have been proposed as one of the effective treatments for HCC. Further clinical investigations would allow for a better understanding and help to propose more effective strategies to increase the efficacy of HIF inhibitors treatment for HCC.
Combination therapies in the management of early and unresectable HCC
There have been many studies indicative of the therapeutic benefit of the combination of anticancer therapies for early and unresectable HCC, such as RFA combined with a chemotherapeutic drug, and thermal ablation combined with transarterial chemoembolization. The combination of local ablation therapies with immunotherapies represents a promising therapeutic strategy for treating HCC. The primary immunotherapeutic strategies include immune checkpoint inhibitors therapy (e.g., PD-1 and PD-L1), cell-based therapies, and tumor vaccine therapy. Though the role of PD-1 inhibitors in the adjuvant setting is still under investigation, the administration of PD-1 blockade in addition to RFA was found to be promising. A previous study reported that PD-1 inhibitor combined with RFA for recurrent HCC resulted in a significantly improved 1-year RFS rate compared to RFA alone (Wang X et al., 2021). A multicenter RCT (Lee et al., 2015) showed that adjuvant immunotherapy with activated cytokine-induced killer cells increased recurrence-free and OS in patients with HCC who underwent curative treatment with RFA. In addition, Ji et al. (2021) showed that RFA and TACE combined with postoperative autologous cytokine-induced killer (CIK) cell immunotherapy reinfusion have significant efficacy in the treatment of primary HCC. A preclinical study demonstrated that PI3Kγ inhibitors could enhance anti-PD-1 therapy for the treatment of residual tumors after IRFA (Liu et al., 2022b). However, though these studies provide a strong rationale for combining RFA and the immunotherapies in the clinical setting, the roles of HIF in this action are extremely scarce.
Advances are currently being made in this area of HCC with ablation treatment. A single-arm phase 2 trial (Qiao et al., 2022) demonstrated that addition of anti-PD-1 adjuvant therapy after TACE combined with ablation significantly prolong the relapse-free survival with controllable safety for HCC patients with high recurrence risk. Repeat hepatectomy was considered to serve as the first choice for solitary small recurrent HCC patients with late recurrence, while MWA should be selected for those with early recurrence (Wang et al., 2022). A randomized controlled phase 2 trial conducted by Radosevic et al. (2022) showed that MWA created larger ablation zones than RFA (p = 0.036). The authors concluded that both MWA and RFA were effectiveness and safety in liver tumors between 1.5 and 4 cm. Suh et al. (2021) revealed that no-touch RFA using twin internally cooled wet electrodes demonstrated significantly lower cumulative local tumor progression rates than conventional RFA for small HCCs. A phase 2 study (Iwata et al., 2021) reported that image-guided proton therapy (IGPT) was a safe and effective treatment for solitary operable or ablation-treatable HCC. Zaitoun et al. (2021) revealed that combined therapy with conventional TACE + MWA is safe, well-tolerated, and more effective than TACE or MWA alone for treatment of HCC with 3–5 cm. Previous study showed that sorafenib combined with TACE and RFA resulted in longer recurrence-free survival and better OS than did TACE-RFA in patients with medium or large HCC (Zhu et al., 2018). However, a subsequent study developed by Bockorny et al. (2022) suggested that priming of sorafenib did not enhance the effect of RFA in intermediate sized HCC. The above studies showed that recent studies continue to explore more effective therapeutic strategies by combining hepatectomy or ablation for treating different staging of HCC (Table 2).
With the advance of screening technology and increased awareness of cancer surveillance, more and more HCC could be detected at early stage, rendering curative therapeutics applicable. The therapeutic strategies of HCC are evolving rapidly. In the 2022 update of BCLC strategy for HCC management, local ablation still plays leading part among the recommended curative treatments for early-stage HCC (Chen S et al., 2022). As compare to the less effective alcohol injection, an increased use of radiofrequency ablation has been found to improve management of intermediate stage patients with HCC (Garuti et al., 2021). Adjuvant therapies (e.g. immunotherapy) have been found to prevent against HCC recurrence after curative treatment, which could significantly improve the prognosis. For the combination with imunotherapy, ablative techniques are gaining more and more attention for their capability of boosting local and systemic immune effects, which makes combination strategy a promising weapon for HCC treatment (Chen M et al., 2022). Besides, tyrosine kinase inhibitors, oncolytic virotherapy, and cancer vaccines, may also boost anti-tumor immunity for HCC. These novel therapeutic strategies show great potential to synergize with ablation in the treatment of primary and metastatic HCC.
It was reported that sorafenib in combination with RFA could improve the treatment of HCC due to sorafenib suppresses cell proliferation and induces apoptosis in hepatoma cells by the HIF-1/VEGFA pathway (Gong et al., 2017). The combination of current sorafenib treatment with gene therapy or inhibitors against HIFs has been documented as promising approach to overcome sorafenib resistance both in vitro and in vivo. Wu et al. (2021) reported that the synergistic effect of the combination of HIFU with sorafenib therapy inhibited HCC tumor growth when compared with HIFU treatment alone or with no treatment, which was closely related to the decreased HIF-2α, VEGFA. Gong et al. (2017) also found that sorafenib in combination with RFA significantly improved the outcomes of early small HCC, which might be associated with decreased serum levels of active tumor growth factors HIF-1α. In addition, a combination of sorafenib and HIFs-targeted therapy or HIFs inhibitors can overcome HCC sorafenib resistance (Zeng et al., 2021). Besides, chloroquine significantly increased the apoptosis of HCC cells after IRFA and inhibited the enrichment of CSCs, and this effect was significantly enhanced by the combination of C-MET inhibitors (Zhao et al., 2018). Except for ablation, HIF inhibitors also play a promising role in treating early or unresectable HCC. For example, HIF inhibitor 32-134D was found to eradicate murine hepatocellular carcinoma in combination with anti-PD1 therapy (Salman et al., 2022). HIF-2α-targeted interventional chemoembolization was detected for the effective elimination of HCC (Chen S et al., 2022).
Taken together, RFA combined with other anti-cancer approaches (i.e. sorafenib, transarterial chemoembolization, and chloroquine) exerts a better curative effect in terms of tumor suppression than RFA alone, probably through inhibiting HIF-1α.
Conclusion
HIF-1, 2α, the potent factors of tumor angiogenesis, play essential roles in the mechanisms of recurrence of HCC after ablation and are of great significance for the efficacy evaluation of ablation of patients with HCC and the development of individualized treatment options. HIF-1, 2α targets the different molecules (e.g., BNIP3, CA-IX, and arginase-1) and signaling cascades (e.g., VEGFA/EphA2 pathway) constituting a complex network that promotes HCC invasion and metastasis after ablation. Currently, the inhibitors of HIF have been developed, providing important proofof targeting HIF for the prevention of HCC recurrence after ablation. Though the effects of HIF-1, 2α on HCC after ablation have been preliminarily elucidated, the dynamic changes in HIF expression after ablation of HCC patients remain to be further studied.
Author contributions
All authors listed have made a substantial, direct, and intellectual contribution to the work and approved it for publication.
Funding
This project was supported by the grants from Zhejiang Province Public Welfare Technology Application Research Project (No. LGF21H160022), the Natural Science Foundation of Zhejiang Province (No. LQ22H160055), Science and Technology Plan Project of Taizhou (No. 21ywb26, 21ywb29, and 22ywa14), Medical Science and Technology Project of Zhejiang Province (No. 2017KY711, 2023KY403, 2023KY404, and 2023KY1340).
Conflict of interest
The authors declare that the research was conducted in the absence of any commercial or financial relationships that could be construed as a potential conflict of interest.
Publisher’s note
All claims expressed in this article are solely those of the authors and do not necessarily represent those of their affiliated organizations, or those of the publisher, the editors and the reviewers. Any product that may be evaluated in this article, or claim that may be made by its manufacturer, is not guaranteed or endorsed by the publisher.
References
Alexander, R. K., Liou, Y. H., Knudsen, N. H., Starost, K. A., Xu, C., Hyde, A. L., et al. (2020). Bmal1 integrates mitochondrial metabolism and macrophage activation. eLife 9, e54090. doi:10.7554/eLife.54090
Barquilla, A., Lamberto, I., Noberini, R., Heynen-Genel, S., Brill, L. M., and Pasquale, E. B. (2016). Protein kinase A can block EphA2 receptor-mediated cell repulsion by increasing EphA2 S897 phosphorylation. Mol. Biol. Cell. 27 (17), 2757–2770. doi:10.1091/mbc.E16-01-0048
Ben, D. C., Mandi, K., Frye, M., Acheampong, A., Radi, A., De Becker, B., et al. (2022). Chemerin regulates normal angiogenesis and hypoxia-driven neovascularization. Angiogenesis 25 (2), 159–179. doi:10.1007/s10456-021-09818-1
Benej, M., Svastova, E., Banova, R., Kopacek, J., Gibadulinova, A., Kery, M., et al. (2020). CA IX stabilizes intracellular pH to maintain metabolic reprogramming and proliferation in hypoxia. Front. Oncol. 10, 1462. doi:10.3389/fonc.2020.01462
Bockorny, B., Bullock, A. J., Abrams, T. A., Faintuch, S., Alsop, D. C., Goldberg, S. N., et al. (2022). Priming of sorafenib prior to radiofrequency ablation does not increase treatment effect in hepatocellular carcinoma. Dig. Dis. Sci. 67 (7), 3455–3463. doi:10.1007/s10620-021-07156-2
Calderaro, J., Seraphin, T. P., Luedde, T., and Simon, T. G. (2022). Artificial intelligence for the prevention and clinical management of hepatocellular carcinoma. J. Hepatol. 76 (6), 1348–1361. doi:10.1016/j.jhep.2022.01.014
Cessna, H., Baritaki, S., Zaravinos, A., and Bonavida, B. (2022). The role of RKIP in the regulation of EMT in the tumor microenvironment. Cancers (Basel) 14 (19), 4596. doi:10.3390/cancers14194596
Chen, J., Bai, M., Ning, C., Xie, B., Zhang, J., Liao, H., et al. (2016). Gankyrin facilitates follicle-stimulating hormone-driven ovarian cancer cell proliferation through the PI3K/AKT/HIF-1α/cyclin D1 pathway. Oncogene 35 (19), 2506–2517. doi:10.1038/onc.2015.316
Chen, M., Shu, G., Lv, X., Xu, X., Lu, C., Qiao, E., et al. (2022). HIF-2α-targeted interventional chemoembolization multifunctional microspheres for effective elimination of hepatocellular carcinoma. Biomaterials 284, 121512. doi:10.1016/j.biomaterials.2022.121512
Chen, S., Zeng, X., Su, T., Xiao, H., Lin, M., Peng, Z., et al. (2022). Combinatory local ablation and immunotherapies for hepatocellular carcinoma: Rationale, efficacy, and perspective. Front. Immunol. 13, 1033000. doi:10.3389/fimmu.2022.1033000
Chen, W., Hill, H., Christie, A., Kim, M. S., Holloman, E., Pavia-Jimenez, A., et al. (2016). Targeting renal cell carcinoma with a HIF-2 antagonist. Nature 539 (7627), 112–117. doi:10.1038/nature19796
Chen, X., and Yan, N. (2021). Stachydrine inhibits TGF-β1-induced epithelial-mesenchymal transition in hepatocellular carcinoma cells through the TGF-β/Smad and PI3K/Akt/mTOR signaling pathways. Anticancer Drugs 32 (8), 786–792. doi:10.1097/CAD.0000000000001066
Chen, Y., Bei, J., Liu, M., Huang, J., Xie, L., Huang, W., et al. (2021). Sublethal heat stress-induced O-GlcNAcylation coordinates the Warburg effect to promote hepatocellular carcinoma recurrence and metastasis after thermal ablation. Cancer Lett. 518, 23–34. doi:10.1016/j.canlet.2021.06.001
Chen, Z., Ai, L., Mboge, M. Y., Tu, C., Mckenna, R., Brown, K. D., et al. (2018). Differential expression and function of caix and caxii in breast cancer: A comparison between tumorgraft models and cells. PLoS One 13 (7), e0199476. doi:10.1371/journal.pone.0199476
Cheng, N., Brantley, D. M., Liu, H., Lin, Q., Enriquez, M., Gale, N., et al. (2002). Blockade of EphA receptor tyrosine kinase activation inhibits vascular endothelial cell growth factor-induced angiogenesis. Mol. Cancer Res. 1 (1), 2–11.
Cho, E. J., Yu, S. J., Kim, K., Cho, H., Cho, Y. Y., Lee, Y. B., et al. (2019). Carbonic anhydrase-IX inhibition enhances the efficacy of hexokinase II inhibitor for hepatocellular carcinoma in a murine model. J. Bioenerg. Biomembr. 51 (2), 121–129. doi:10.1007/s10863-019-09788-6
Choueiri, T. K., and Kaelin, W. J. (2020). Targeting the HIF2-VEGF axis in renal cell carcinoma. Nat. Med. 26 (10), 1519–1530. doi:10.1038/s41591-020-1093-z
Courtney, K. D., Ma, Y., Diaz, D. L. A., Christie, A., Xie, Z., Woolford, L., et al. (2020). HIF-2 complex dissociation, target inhibition, and acquired resistance with PT2385, a first-in-class HIF-2 inhibitor, in patients with clear cell renal cell carcinoma. Clin. Cancer Res. 26 (4), 793–803. doi:10.1158/1078-0432.CCR-19-1459
Cowman, S. J., Fuja, D. G., Liu, X. D., Tidwell, R., Kandula, N., Sirohi, D., et al. (2020). Macrophage HIF-1α is an independent prognostic indicator in kidney cancer. Clin. Cancer Res. 26 (18), 4970–4982. doi:10.1158/1078-0432.CCR-19-3890
Cziraki, A., Lenkey, Z., Sulyok, E., Szokodi, I., and Koller, A. (2020). L-Arginine-Nitric oxide-asymmetric dimethylarginine pathway and the coronary circulation: Translation of basic science results to clinical practice. Front. Pharmacol. 11, 569914. doi:10.3389/fphar.2020.569914
D'Ignazio, L., Batie, M., and Rocha, S. (2017). Hypoxia and inflammation in cancer, focus on HIF and NF-κB. Biomedicines 5 (2), 21. doi:10.3390/biomedicines5020021
Dai, X., Pi, G., Yang, S. L., Chen, G. G., Liu, L. P., and Dong, H. H. (2018). Association of PD-L1 and HIF-1α coexpression with poor prognosis in hepatocellular carcinoma. Transl. Oncol. 11 (2), 559–566. doi:10.1016/j.tranon.2018.02.014
De Francesco, E. M., Maggiolini, M., and Musti, A. M. (2018). Crosstalk between notch, HIF-1α and GPER in breast cancer EMT. Int. J. Mol. Sci. 19 (7), 2011. doi:10.3390/ijms19072011
Dong, S., Li, Z., Kong, J., Wu, S., Gao, J., and Sun, W. (2022). Arsenic trioxide inhibits angiogenesis of hepatocellular carcinoma after insufficient radiofrequency ablation via blocking paracrine angiopoietin-1 and angiopoietin-2. Int. J. Hyperth. 39 (1), 888–896. doi:10.1080/02656736.2022.2093995
Engin, H., Ustundag, Y., Ozel, T. I., and Gokmen, A. (2012). Plasma concentrations of ang-1, ang-2 and tie-2 in gastric cancer. Eur. Cytokine Netw. 23 (1), 21–24. doi:10.1684/ecn.2012.0301
Feng, G. W., Dong, L. D., Shang, W. J., Pang, X. L., Li, J. F., Liu, L., et al. (2014). HDAC5 promotes cell proliferation in human hepatocellular carcinoma by up-regulating Six1 expression. Eur. Rev. Med. Pharmacol. Sci. 18 (6), 811–816.
Feng, J., Dai, W., Mao, Y., Wu, L., Li, J., Chen, K., et al. (2020). Simvastatin re-sensitizes hepatocellular carcinoma cells to sorafenib by inhibiting HIF-1α/PPAR-γ/PKM2-mediated glycolysis. J. Exp. Clin. Cancer Res. 39 (1), 24. doi:10.1186/s13046-020-1528-x
Fitzpatrick, S. F. (2019). Immunometabolism and sepsis: A role for HIF? Front. Mol. Biosci. 6, 85. doi:10.3389/fmolb.2019.00085
Frenzel, T., Siekmann, J., Grohmann, C., Valentiner, U., Schmitz, R., Riecken, K., et al. (2018). Locally ablative radiation therapy of a primary human small cell lung cancer tumor decreases the number of spontaneous metastases in two xenograft models. Int. J. Radiat. Oncol. Biol. Phys. 100 (4), 1044–1056. doi:10.1016/j.ijrobp.2017.11.044
Fujita, N., Markova, D., Anderson, D. G., Chiba, K., Toyama, Y., Shapiro, I. M., et al. (2012). Expression of prolyl hydroxylases (PHDs) is selectively controlled by HIF-1 and HIF-2 proteins in nucleus pulposus cells of the intervertebral disc: Distinct roles of PHD2 and PHD3 proteins in controlling HIF-1α activity in hypoxia. J. Biol. Chem. 287 (20), 16975–16986. doi:10.1074/jbc.M111.334466
Galan-Cobo, A., Ramirez-Lorca, R., Serna, A., and Echevarria, M. (2015). Overexpression of AQP3 modifies the cell cycle and the proliferation rate of mammalian cells in culture. PLoS One 10 (9), e0137692. doi:10.1371/journal.pone.0137692
Garuti, F., Neri, A., Avanzato, F., Gramenzi, A., Rampoldi, D., Rucci, P., et al. (2021). The changing scenario of hepatocellular carcinoma in Italy: An update. Liver Int. 41 (3), 585–597. doi:10.1111/liv.14735
Gong, J., Zhou, S., and Yang, S. (2019). Vanillic acid suppresses HIF-1α expression via inhibition of mTOR/p70S6K/4E-BP1 and raf/MEK/ERK pathways in human colon cancer HCT116 cells. Int. J. Mol. Sci. 20 (3), 465. doi:10.3390/ijms20030465
Gong, Q., Qin, Z., and Hou, F. (2017). Improved treatment of early small hepatocellular carcinoma using sorafenib in combination with radiofrequency ablation. Oncol. Lett. 14 (6), 7045–7048. doi:10.3892/ol.2017.7174
Guo, S., Zhang, D., Lu, X., Zhang, Q., Gu, R., Sun, B., et al. (2021). Hypoxia and its possible relationship with endometrial receptivity in adenomyosis: A preliminary study. Reprod. Biol. Endocrinol. 19 (1), 7. doi:10.1186/s12958-020-00692-y
Halpern, A. L., Fitz, J. G., Fujiwara, Y., Yi, J., Anderson, A. L., Zhu, Y., et al. (2021). Hepatic thermal injury promotes colorectal cancer engraftment in C57/black 6 mice. Am. J. Physiol. Cell. Physiol. 320 (1), C142–C151. doi:10.1152/ajpcell.00071.2020
Han, Z., Liu, D., Chen, L., He, Y., Tian, X., Qi, L., et al. (2021). PNO1 regulates autophagy and apoptosis of hepatocellular carcinoma via the MAPK signaling pathway. Cell. Death Dis. 12 (6), 552. doi:10.1038/s41419-021-03837-y
Hu, W., Zheng, S., Guo, H., Dai, B., Ni, J., Shi, Y., et al. (2021). PLAGL2-EGFR-HIF-1/2α signaling loop promotes HCC progression and Erlotinib insensitivity. Hepatology 73 (2), 674–691. doi:10.1002/hep.31293
Huang, J., Yan, L., Cheng, Z., Wu, H., Du, L., Wang, J., et al. (2010). A randomized trial comparing radiofrequency ablation and surgical resection for HCC conforming to the Milan criteria. Ann. Surg. 252 (6), 903–912. doi:10.1097/SLA.0b013e3181efc656
Ikemoto, T., Shimada, M., and Yamada, S. (2017). Pathophysiology of recurrent hepatocellular carcinoma after radiofrequency ablation. Hepatol. Res. 47 (1), 23–30. doi:10.1111/hepr.12705
Iwata, H., Ogino, H., Hattori, Y., Nakajima, K., Nomura, K., Hashimoto, S., et al. (2021). A phase 2 study of image-guided proton therapy for operable or ablation-treatable primary hepatocellular carcinoma. Int. J. Radiat. Oncol. Biol. Phys. 111 (1), 117–126. doi:10.1016/j.ijrobp.2021.03.049
Ji, Q., Fu, Y., Zhu, X., Wang, L., and Ling, C. (2021). Effect of RFA and TACE combined with postoperative cytokine-induced killer cell immunotherapy in primary hepatocellular carcinoma. J. BUON. 26 (1), 235–242.
Jing, X., Zhou, Y., Ding, J., Wang, Y., Qin, Z., Wang, Y., et al. (2020). The learning curve for thermal ablation of liver cancers: 4,363-Session experience for a single central in 18 years. Front. Oncol. 10, 540239. doi:10.3389/fonc.2020.540239
Kaelin, W. J., and Ratcliffe, P. J. (2008). Oxygen sensing by metazoans: The central role of the HIF hydroxylase pathway. Mol. Cell. 30 (4), 393–402. doi:10.1016/j.molcel.2008.04.009
Kaelin, W. J. (2007). The von Hippel-Lindau tumor suppressor protein and clear cell renal carcinoma. Clin. Cancer Res. 13, 680s–684s. doi:10.1158/1078-0432.CCR-06-1865
Kling, L., Schreiber, A., Eckardt, K. U., and Kettritz, R. (2021). Hypoxia-inducible factors not only regulate but also are myeloid-cell treatment targets. J. Leukoc. Biol. 110 (1), 61–75. doi:10.1002/JLB.4RI0820-535R
Kong, J., Kong, J., Pan, B., Ke, S., Dong, S., Li, X., et al. (2012). Insufficient radiofrequency ablation promotes angiogenesis of residual hepatocellular carcinoma via HIF-1α/VEGFA. PLoS One 7 (5), e37266. doi:10.1371/journal.pone.0037266
Labib, O. H., Harb, O. A., Khalil, O. H., Baiomy, T. A., Gertallah, L. M., and Ahmed, R. Z. (2020). The diagnostic value of arginase-1, FTCD, and MOC-31 expression in early detection of hepatocellular carcinoma (HCC) and in differentiation between HCC and metastatic adenocarcinoma to the liver. J. Gastrointest. Cancer 51 (1), 88–101. doi:10.1007/s12029-019-00211-2
Lal, S., Shekher, A., PuneetNarula, A. S., Abrahamse, H., and Gupta, S. C. (2021). Cannabis and its constituents for cancer: History, biogenesis, chemistry and pharmacological activities. Pharmacol. Res. 163, 105302. doi:10.1016/j.phrs.2020.105302
Lee, J. H., Lee, J. H., Lim, Y. S., Yeon, J. E., Song, T. J., Yu, S. J., et al. (2015). Adjuvant immunotherapy with autologous cytokine-induced killer cells for hepatocellular carcinoma. Gastroenterology 148 (7), 1383–1391. doi:10.1053/j.gastro.2015.02.055
Li, G., Kong, J., Dong, S., Niu, H., Wu, S., and Sun, W. (2022). Circular BANP knockdown inhibits the malignant progression of residual hepatocellular carcinoma after insufficient radiofrequency ablation. Chin. Med. J. Engl. Publish Ahead of Print. doi:10.1097/CM9.00000000000001822
Lin, J., Cao, S., Wang, Y., Hu, Y., Liu, H., Li, J., et al. (2018). Long non-coding RNA UBE2CP3 enhances HCC cell secretion of VEGFA and promotes angiogenesis by activating ERK1/2/HIF-1α/VEGFA signalling in hepatocellular carcinoma. J. Exp. Clin. Cancer Res. 37 (1), 113. doi:10.1186/s13046-018-0727-1
Lin, N., Meng, L., Lin, J., Chen, S., Zhang, P., Chen, Q., et al. (2020). Activated hepatic stellate cells promote angiogenesis in hepatocellular carcinoma by secreting angiopoietin-1. J. Cell. Biochem. 121 (2), 1441–1451. doi:10.1002/jcb.29380
Liu, J., Yang, C., Huang, X. M., Lv, P. P., Yang, Y. K., Zhao, J. N., et al. (2021). Knockdown of FBI-1 inhibits the Warburg effect and enhances the sensitivity of hepatocellular carcinoma cells to molecular targeted agents via miR-3692/HIF-1α. Front. Oncol. 11, 796839. doi:10.3389/fonc.2021.796839
Liu, S. J., Wang, J. Y., Peng, S. H., Li, T., Ning, X. H., Hong, B. A., et al. (2018). Genotype and phenotype correlation in von Hippel-Lindau disease based on alteration of the HIF-alpha binding site in VHL protein. Genet. Med. 20 (10), 1266–1273. doi:10.1038/gim.2017.261
Liu, X., Liu, H., Zeng, L., and Lv, Y. (2022a). BRCA1 overexpression attenuates breast cancer cell growth and migration by regulating the pyruvate kinase M2-mediated Warburg effect via the PI3K/AKT signaling pathway. PeerJ 10, e14052. doi:10.7717/peerj.14052
Liu, X., Zhang, W., Xu, Y., Xu, X., Jiang, Q., Ruan, J., et al. (2022b). Targeting PI3Kγ/AKT pathway remodels LC3-associated phagocytosis induced immunosuppression after radiofrequency ablation. . 9 (7), e2102182. doi:10.1002/advs.202102182
Lyu, Y., Zhang, Y., Wang, Y., Luo, Y., Ding, H., Li, P., et al. (2022). HIF-1α regulated WTAP overexpression promoting the Warburg effect of ovarian cancer by m6A-dependent manner. J. Immunol. Res. 2022, 6130806. doi:10.1155/2022/6130806
Macedo-Silva, C., Miranda-Goncalves, V., Lameirinhas, A., Lencart, J., Pereira, A., Lobo, J., et al. (2020). JmjC-KDMs KDM3A and KDM6B modulate radioresistance under hypoxic conditions in esophageal squamous cell carcinoma. Cell. Death Dis. 11 (12), 1068. doi:10.1038/s41419-020-03279-y
Mandriota, S. J., Turner, K. J., Davies, D. R., Murray, P. G., Morgan, N. V., Sowter, H. M., et al. (2002). HIF activation identifies early lesions in VHL kidneys: Evidence for site-specific tumor suppressor function in the nephron. Cancer Cell. 1 (5), 459–468. doi:10.1016/s1535-6108(02)00071-5
Mendez-Blanco, C., Fondevila, F., Garcia-Palomo, A., Gonzalez-Gallego, J., and Mauriz, J. L. (2018). Sorafenib resistance in hepatocarcinoma: Role of hypoxia-inducible factors. Exp. Mol. Med. 50 (10), 1–9. doi:10.1038/s12276-018-0159-1
Minardi, D., Lucarini, G., Santoni, M., Mazzucchelli, R., Burattini, L., Conti, A., et al. (2015). Survival in patients with clear cell renal cell carcinoma is predicted by HIF-1α expression. Anticancer Res. 35 (1), 433–438.
Morimoto, M., Numata, K., Kondou, M., Nozaki, A., Morita, S., and Tanaka, K. (2010). Midterm outcomes in patients with intermediate-sized hepatocellular carcinoma: A randomized controlled trial for determining the efficacy of radiofrequency ablation combined with transcatheter arterial chemoembolization. Cancer-Am. Cancer Soc. 116 (23), 5452–5460. doi:10.1002/cncr.25314
Moudi, B., Mahmoudzadeh-Sagheb, H., and Heidari, Z. (2020). Hepatocyte paraffin 1 and arginase-1 are effective panel of markers in HBV-related HCC diagnosis in fine-needle aspiration specimens. BMC Res. Notes 13 (1), 388. doi:10.1186/s13104-020-05230-y
Mu, H., Yu, G., Li, H., Wang, M., Cui, Y., Zhang, T., et al. (2021). Mild chronic hypoxia-induced HIF-2α interacts with c-MYC through competition with HIF-1α to induce hepatocellular carcinoma cell proliferation. . 44 (5), 1151–1166. doi:10.1007/s13402-021-00625-w
Muz, B., de la Puente, P., Azab, F., and Azab, A. K. (2015). The role of hypoxia in cancer progression, angiogenesis, metastasis, and resistance to therapy. 3, 83–92. doi:10.2147/HP.S93413
Nguyen, T. H., Conotte, S., Belayew, A., Decleves, A. E., Legrand, A., and Tassin, A. (2021). Hypoxia and hypoxia-inducible factor signaling in muscular dystrophies: Cause and consequences. Int. J. Mol. Sci. 22 (13), 7220. doi:10.3390/ijms22137220
Nijkamp, M. W., van der Bilt, J. D., de Bruijn, M. T., Molenaar, I. Q., Voest, E. E., van Diest, P. J., et al. (2009). Accelerated perinecrotic outgrowth of colorectal liver metastases following radiofrequency ablation is a hypoxia-driven phenomenon. Ann. Surg. 249 (5), 814–823. doi:10.1097/SLA.0b013e3181a38ef5
Niu, X., Sun, H., Qiu, F., Liu, J., Yang, T., and Han, W. (2021). miR-10b-5p suppresses the proliferation and invasion of primary hepatic carcinoma cells by downregulating EphA2. Biomed. Res. Int. 2021, 1382061. doi:10.1155/2021/1382061
Palazon, A., Tyrakis, P. A., Macias, D., Velica, P., Rundqvist, H., Fitzpatrick, S., et al. (2017). An HIF-1α/VEGF-A Axis in cytotoxic T cells regulates tumor progression. Cancer Cell. 32 (5), 669–683. doi:10.1016/j.ccell.2017.10.003
Parikh, N. D., and Pillai, A. (2021). Recent advances in hepatocellular carcinoma treatment. Clin. Gastroenterol. Hepatol. 19 (10), 2020–2024. doi:10.1016/j.cgh.2021.05.045
Parks, S. K., and Pouyssegur, J. (2017). Targeting pH regulating proteins for cancer therapy-Progress and limitations. Semin. Cancer Biol. 43, 66–73. doi:10.1016/j.semcancer.2017.01.007
Pestana, R. C., Hassan, M. M., Abdel-Wahab, R., Abugabal, Y. I., Girard, L. M., Li, D., et al. (2018). Clinical and prognostic significance of circulating levels of angiopoietin-1 and angiopoietin-2 in hepatocellular carcinoma. Oncotarget 9 (102), 37721–37732. doi:10.18632/oncotarget.26507
Porter, E. T., and Porter, F. S. (2018). A strain gauge monitor (SGM) for continuous valve gape measurements in bivalve molluscs in response to laboratory induced diel-cycling hypoxia and pH. J. Vis. Exp. 138, 57404. doi:10.3791/57404
Qiao, W., Wang, Q., Hu, C., Zhang, Y., Li, J., Sun, Y., et al. (2022). Interim efficacy and safety of PD-1 inhibitors in preventing recurrence of hepatocellular carcinoma after interventional therapy. Front. Immunol. 13, 1019772. doi:10.3389/fimmu.2022.1019772
Radosevic, A., Quesada, R., Serlavos, C., Sanchez, J., Zugazaga, A., Sierra, A., et al. (2022). Microwave versus radiofrequency ablation for the treatment of liver malignancies: A randomized controlled phase 2 trial. Sci. Rep. 12 (1), 316. doi:10.1038/s41598-021-03802-x
Rankin, E. B., and Giaccia, A. J. (2016). Hypoxic control of metastasis. Science 352 (6282), 175–180. doi:10.1126/science.aaf4405
Ren, H., Liu, N. Y., Song, X. F., Ma, Y. S., and Zhai, X. Y. (2011). A novel specific application of pyruvate protects the mouse retina against white light damage: Differential stabilization of HIF-1α and HIF-2α. Invest. Ophthalmol. Vis. Sci. 52 (6), 3112–3118. doi:10.1167/iovs.10-5605
Rocha, S. (2007). Gene regulation under low oxygen: Holding your breath for transcription. Trends biochem. Sci. 32 (8), 389–397. doi:10.1016/j.tibs.2007.06.005
Saha, D., Mitra, D., Alam, N., Sen, S., Mustafi, S. M., Majumder, P. K., et al. (2022). Lupeol and Paclitaxel cooperate in hindering hypoxia induced vasculogenic mimicry via suppression of HIF-1α-EphA2-Laminin-5γ2 network in human oral cancer. J. Cell. Commun. Signal. doi:10.1007/s12079-022-00693-z
Salman, S., Meyers, D. J., Wicks, E. E., Lee, S. N., Datan, E., Thomas, A. M., et al. (2022). HIF inhibitor 32-134D eradicates murine hepatocellular carcinoma in combination with anti-PD1 therapy. J. Clin. Invest. 132 (9), e156774. doi:10.1172/JCI156774
Sauvaget, C., Bazikamwe, S., Lucas, E., Ndayikengurukiye, A., Harerimana, S., and Barango, P. (2022). Evaluation of effectiveness, acceptability and safety of thermal ablation in the treatment of cervical neoplasia in Burundi. Int. J. Cancer. 151 (7), 1120–1126. doi:10.1002/ijc.34117
Schito, L., and Semenza, G. L. (2016). Hypoxia-inducible factors: Master regulators of cancer progression. Trends Cancer 2 (12), 758–770. doi:10.1016/j.trecan.2016.10.016
Semenza, G. L., Nejfelt, M. K., Chi, S. M., and Antonarakis, S. E. (1991). Hypoxia-inducible nuclear factors bind to an enhancer element located 3' to the human erythropoietin gene. Proc. Natl. Acad. Sci. U. S. A. 88 (13), 5680–5684. doi:10.1073/pnas.88.13.5680
Shaida, N., Launchbury, R., Boddy, J. L., Jones, C., Campo, L., Turley, H., et al. (2008). Expression of BNIP3 correlates with hypoxia-inducible factor (HIF)-1alpha, HIF-2alpha and the androgen receptor in prostate cancer and is regulated directly by hypoxia but not androgens in cell lines. Prostate 68 (3), 336–343. doi:10.1002/pros.20707
Shin, S. W., Ahn, K. S., Kim, S. W., Kim, T. S., Kim, Y. H., and Kang, K. J. (2021). Liver resection versus local ablation therapies for hepatocellular carcinoma within the milan criteria: A systematic review and meta-analysis. Ann. Surg. 273 (4), 656–666. doi:10.1097/SLA.0000000000004350
Snell, C. E., Turley, H., Mcintyre, A., Li, D., Masiero, M., Schofield, C. J., et al. (2014). Proline-hydroxylated hypoxia-inducible factor 1α (HIF-1α) upregulation in human tumours. PLoS One 9 (2), e88955. doi:10.1371/journal.pone.0088955
Sofuni, A., Asai, Y., Mukai, S., Yamamoto, K., and Itoi, T. (2022). High-intensity focused ultrasound therapy for pancreatic cancer. J. Med. Ultrason. doi:10.1007/s10396-022-01208-4
Sperandio, R. C., Pestana, R. C., Miyamura, B. V., and Kaseb, A. O. (2022). Hepatocellular carcinoma immunotherapy. Annu. Rev. Med. 73, 267–278. doi:10.1146/annurev-med-042220-021121
Strowitzki, M. J., Cummins, E. P., and Taylor, C. T. (2019). Protein hydroxylation by hypoxia-inducible factor (HIF) hydroxylases: Unique or ubiquitous? Cells 8 (5), 384. doi:10.3390/cells8050384
Suh, Y. S., Choi, J. W., Yoon, J. H., Lee, D. H., Kim, Y. J., Lee, J. H., et al. (2021). No-touch vs. Conventional radiofrequency ablation using twin internally cooled wet electrodes for small hepatocellular carcinomas: A randomized prospective comparative study. Korean J. Radiol. 22 (12), 1974–1984. doi:10.3348/kjr.2021.0319
Tong, Y., Yang, H., Xu, X., Ruan, J., Liang, M., Wu, J., et al. (2017). Effect of a hypoxic microenvironment after radiofrequency ablation on residual hepatocellular cell migration and invasion. Cancer Sci. 108 (4), 753–762. doi:10.1111/cas.13191
Tsai, Y. M., Wu, K. L., Chang, Y. Y., Chang, W. A., Huang, Y. C., Jian, S. F., et al. (2020). Loss of miR-145-5p causes ceruloplasmin interference with PHD-iron Axis and HIF-2α stabilization in lung adenocarcinoma-mediated angiogenesis. Int. J. Mol. Sci. 21 (14), 5081. doi:10.3390/ijms21145081
Vara-Perez, M., Rossi, M., Van den Haute, C., Maes, H., Sassano, M. L., Venkataramani, V., et al. (2021). BNIP3 promotes HIF-1α-driven melanoma growth by curbing intracellular iron homeostasis. EMBO J. 40 (10), e106214. doi:10.15252/embj.2020106214
Verma, A. K., Bharti, P. S., Rafat, S., Bhatt, D., Goyal, Y., Pandey, K. K., et al. (2021). Autophagy paradox of cancer: Role, regulation, and duality. Oxid. Med. Cell. Longev. 2021, 8832541. doi:10.1155/2021/8832541
Wan, J., Wu, W., Huang, Y., Ge, W., and Liu, S. (2016a). Incomplete radiofrequency ablation accelerates proliferation and angiogenesis of residual lung carcinomas via HSP70/HIF-1α. Oncol. Rep. 36 (2), 659–668. doi:10.3892/or.2016.4858
Wan, J., Wu, W., and Zhang, R. (2016b). Local recurrence of small cell lung cancer following radiofrequency ablation is induced by HIF-1α expression in the transition zone. Oncol. Rep. 35 (3), 1297–1308. doi:10.3892/or.2015.4541
Wang, G. L., Jiang, B. H., Rue, E. A., and Semenza, G. L. (1995). Hypoxia-inducible factor 1 is a basic-helix-loop-helix-PAS heterodimer regulated by cellular O2 tension. Proc. Natl. Acad. Sci. U. S. A. 92 (12), 5510–5514. doi:10.1073/pnas.92.12.5510
Wang, G. L., and Semenza, G. L. (1995). Purification and characterization of hypoxia-inducible factor 1. J. Biol. Chem. 270 (3), 1230–1237. doi:10.1074/jbc.270.3.1230
Wang, W. Q., Lv, X., Li, J., Li, J., Wang, J. L., Yuan, T., et al. (2022). Repeat hepatectomy versus microwave ablation for solitary and small (≤3 cm) recurrent hepatocellular carcinoma with early or late recurrence: A propensity score matched study. Eur. J. Surg. Oncol. doi:10.1016/j.ejso.2022.12.016
Wang, X., Deng, Q., Feng, K., Chen, S., Jiang, J., Xia, F., et al. (2018). Insufficient radiofrequency ablation promotes hepatocellular carcinoma cell progression via autophagy and the CD133 feedback loop. Oncol. Rep. 40 (1), 241–251. doi:10.3892/or.2018.6403
Wang, X, X., Liu, G., Chen, S., Bi, H., Xia, F., Feng, K., et al. (2021). Combination therapy with PD-1 blockade and radiofrequency ablation for recurrent hepatocellular carcinoma: A propensity score matching analysis. Int. J. Hyperth. 38 (1), 1519–1528. doi:10.1080/02656736.2021.1991011
Wang, Z, Z., Jiang, L., Wang, J., Chai, Z., and Xiong, W. (2021). Morphine promotes angiogenesis by activating PI3K/Akt/HIF-1α pathway and upregulating VEGF in hepatocellular carcinoma. J. Gastrointest. Oncol. 12 (4), 1761–1772. doi:10.21037/jgo-20-394
Wilson, K., Shiuan, E., and Brantley-Sieders, D. M. (2021). Oncogenic functions and therapeutic targeting of EphA2 in cancer. Oncogene 40 (14), 2483–2495. doi:10.1038/s41388-021-01714-8
Wu, L., Fu, Z., Zhou, S., Gong, J., Liu, C. A., Qiao, Z., et al. (2014). HIF-1α and HIF-2α: Siblings in promoting angiogenesis of residual hepatocellular carcinoma after high-intensity focused ultrasound ablation. PLoS One 9 (2), e88913. doi:10.1371/journal.pone.0088913
Wu, L., Zhang, Y. S., Ye, M. L., Shen, F., Liu, W., Hu, H. S., et al. (2017). Overexpression and correlation of HIF-2α, VEGFA and EphA2 in residual hepatocellular carcinoma following high-intensity focused ultrasound treatment: Implications for tumor recurrence and progression. Exp. Ther. Med. 13 (6), 3529–3534. doi:10.3892/etm.2017.4428
Wu, L., Zhou, J., Zhou, W., Huang, X. F., Chen, Q., Wang, W., et al. (2021). Sorafenib blocks the activation of the HIF-2α/VEGFA/EphA2 pathway, and inhibits the rapid growth of residual liver cancer following high-intensity focused ultrasound therapy in vivo. Pathol. Res. Pract. 220, 153270. doi:10.1016/j.prp.2020.153270
Xie, J. Y., Wei, J. X., Lv, L. H., Han, Q. F., Yang, W. B., Li, G. L., et al. (2020). Angiopoietin-2 induces angiogenesis via exosomes in human hepatocellular carcinoma. Cell. Commun. Signal 18 (1), 46. doi:10.1186/s12964-020-00535-8
Xu, H., Chen, Y., Li, Z., Zhang, H., Liu, J., and Han, J. (2022). The hypoxia-inducible factor 1 inhibitor LW6 mediates the HIF-1α/PD-L1 axis and suppresses tumor growth of hepatocellular carcinoma in vitro and in vivo. Eur. J. Pharmacol. 930, 175154. doi:10.1016/j.ejphar.2022.175154
Xu, M., Xie, X. H., Xie, X. Y., Xu, Z. F., Liu, G. J., Zheng, Y. L., et al. (2013). Sorafenib suppresses the rapid progress of hepatocellular carcinoma after insufficient radiofrequency ablation therapy: An experiment in vivo. Acta Radiol. 54 (2), 199–204. doi:10.1258/ar.2012.120249
Xu, S., Zhou, Z., Peng, X., Tao, X., Zhou, P., Zhang, K., et al. (2021). EBV-LMP1 promotes radioresistance by inducing protective autophagy through BNIP3 in nasopharyngeal carcinoma. Cell. Death Dis. 12 (4), 344. doi:10.1038/s41419-021-03639-2
Xu, W. L., Wang, S. H., Sun, W. B., Gao, J., Ding, X. M., Kong, J., et al. (2019). Insufficient radiofrequency ablation-induced autophagy contributes to the rapid progression of residual hepatocellular carcinoma through the HIF-1α/BNIP3 signaling pathway. BMB Rep. 52 (4), 277–282. doi:10.5483/BMBRep.2019.52.4.263
Yamada, S., Utsunomiya, T., Morine, Y., Imura, S., Ikemoto, T., Arakawa, Y., et al. (2014). Expressions of hypoxia-inducible factor-1 and epithelial cell adhesion molecule are linked with aggressive local recurrence of hepatocellular carcinoma after radiofrequency ablation therapy. Ann. Surg. Oncol. 21, S436–S442. doi:10.1245/s10434-014-3575-z
Yamamoto, K., Venida, A., Yano, J., Biancur, D. E., Kakiuchi, M., Gupta, S., et al. (2020). Autophagy promotes immune evasion of pancreatic cancer by degrading MHC-I. Nature 581 (7806), 100–105. doi:10.1038/s41586-020-2229-5
Ye, M., Fang, Z., Gu, H., Song, R., Ye, J., Li, H., et al. (2017). Histone deacetylase 5 promotes the migration and invasion of hepatocellular carcinoma via increasing the transcription of hypoxia-inducible factor-1α under hypoxia condition. Tumour Biol. 39 (6), 1010428317705034. doi:10.1177/1010428317705034
You, J., Chen, W., Chen, J., Zheng, Q., Dong, J., and Zhu, Y. (2018). The oncogenic role of ARG1 in progression and metastasis of hepatocellular carcinoma. Biomed. Res. Int. 2018, 2109865. doi:10.1155/2018/2109865
Yousaf, M. N., Ehsan, H., Muneeb, A., Wahab, A., Sana, M. K., Neupane, K., et al. (2020). Role of radiofrequency ablation in the management of unresectable pancreatic cancer. Front. Med. (Lausanne) 7, 624997. doi:10.3389/fmed.2020.624997
Yuan, G., Zeng, C. L., Zhu, D. D., and Shi, X. J. (2017). Influences of RFA combined with TACE on the HIF-1alpha and EGR level of patients with primary hepatic carcinoma. Eur. Rev. Med. Pharmacol. Sci. 21 (8), 1738–1745.
Zaitoun, M., Elsayed, S. B., Zaitoun, N. A., Soliman, R. K., Elmokadem, A. H., Farag, A. A., et al. (2021). Combined therapy with conventional trans-arterial chemoembolization (cTACE) and microwave ablation (MWA) for hepatocellular carcinoma >3-<5 cm. Int. J. Hyperth. 38 (1), 248–256. doi:10.1080/02656736.2021.1887941
Zeng, Z., Lu, Q., Liu, Y., Zhao, J., Zhang, Q., Hu, L., et al. (2021). Effect of the hypoxia inducible factor on sorafenib resistance of hepatocellular carcinoma. Front. Oncol. 11, 641522. doi:10.3389/fonc.2021.641522
Zhang, H. S., Zhang, Z. G., Du, G. Y., Sun, H. L., Liu, H. Y., Zhou, Z., et al. (2019). Nrf2 promotes breast cancer cell migration via up-regulation of G6PD/HIF-1α/Notch1 axis. J. Cell. Mol. Med. 23 (5), 3451–3463. doi:10.1111/jcmm.14241
Zhang, J., Zhang, M., Huang, J., Zhang, G., Li, C., Wang, X., et al. (2021). Development and validation of an autophagy-related gene signature for predicting the prognosis of hepatocellular carcinoma. Biomed. Res. Int. 2021, 7771037. doi:10.1155/2021/7771037
Zhang, J., Zhang, Q., Lou, Y., Fu, Q., Chen, Q., Wei, T., et al. (2018). Hypoxia-inducible factor-1α/interleukin-1β signaling enhances hepatoma epithelial-mesenchymal transition through macrophages in a hypoxic-inflammatory microenvironment. Hepatology 67 (5), 1872–1889. doi:10.1002/hep.29681
Zhang, K., Fang, T., Shao, Y., and Wu, Y. (2021). TGF-beta-MTA1-SMAD7-SMAD3-SOX4-EZH2 signaling Axis promotes viability, migration, invasion and EMT of hepatocellular carcinoma cells. Cancer Manag. Res. 13, 7087–7099. doi:10.2147/CMAR.S297765
Zhang, Q., Lou, Y., Zhang, J., Fu, Q., Wei, T., Sun, X., et al. (2017). Hypoxia-inducible factor-2α promotes tumor progression and has crosstalk with Wnt/β-catenin signaling in pancreatic cancer. Mol. Cancer 16 (1), 119. doi:10.1186/s12943-017-0689-5
Zhang, Y., Liu, D., Hu, H., Zhang, P., Xie, R., and Cui, W. (2019). HIF-1α/BNIP3 signaling pathway-induced-autophagy plays protective role during myocardial ischemia-reperfusion injury. Biomed. Pharmacother. 120, 109464. doi:10.1016/j.biopha.2019.109464
Zhang, Z., Li, Q., Wang, F., Ma, B., Meng, Y., and Zhang, Q. (2021). Identifying hypoxia characteristics to stratify prognosis and assess the tumor immune microenvironment in renal cell carcinoma. Front. Genet. 12, 606816. doi:10.3389/fgene.2021.606816
Zhao, Z., Wu, J., Liu, X., Liang, M., Zhou, X., Ouyang, S., et al. (2018). Insufficient radiofrequency ablation promotes proliferation of residual hepatocellular carcinoma via autophagy. Cancer Lett. 421, 73–81. doi:10.1016/j.canlet.2018.02.024
Zhou, L., Zhao, Y., Pan, L. C., Wang, J., Shi, X. J., Du, G. S., et al. (2022). Sirolimus increases the anti-cancer effect of Huai Er by regulating hypoxia inducible factor-1α-mediated glycolysis in hepatocellular carcinoma. World J. Gastroenterol. 28 (32), 4600–4619. doi:10.3748/wjg.v28.i32.4600
Zhou, Y., Jin, X., Ma, J., Ding, D., Huang, Z., Sheng, H., et al. (2021a). HDAC5 loss impairs RB repression of pro-oncogenic genes and confers CDK4/6 inhibitor resistance in cancer. Cancer Res. 81 (6), 1486–1499. doi:10.1158/0008-5472.CAN-20-2828
Zhou, Y., Lin, F., Wan, T., Chen, A., Wang, H., Jiang, B., et al. (2021b). ZEB1 enhances Warburg effect to facilitate tumorigenesis and metastasis of HCC by transcriptionally activating PFKM. Theranostics 11 (12), 5926–5938. doi:10.7150/thno.56490
Zhou, Y., Yamada, N., Tanaka, T., Hori, T., Yokoyama, S., Hayakawa, Y., et al. (2015). Crucial roles of RSK in cell motility by catalysing serine phosphorylation of EphA2. Nat. Commun. 6, 7679. doi:10.1038/ncomms8679
Zhu, J., Wu, Y., Yu, Y., Li, Y., Shen, J., and Zhang, R. (2022). MYBL1 induces transcriptional activation of ANGPT2 to promote tumor angiogenesis and confer sorafenib resistance in human hepatocellular carcinoma. Cell. Death Dis. 13 (8), 727. doi:10.1038/s41419-022-05180-2
Zhu, K., Huang, J., Lai, L., Huang, W., Cai, M., Zhou, J., et al. (2018). Medium or large hepatocellular carcinoma: Sorafenib combined with transarterial chemoembolization and radiofrequency ablation. Radiology 288 (1), 300–307. doi:10.1148/radiol.2018172028
Zhuang, B. W., Li, W., Wang, W., Li, B., Lu, M. D., Kuang, M., et al. (2021). Treatment effect of radiofrequency ablation versus liver transplantation and surgical resection for hepatocellular carcinoma within milan criteria: A population-based study. Eur. Radiol. 31 (7), 5379–5389. doi:10.1007/s00330-020-07551-9
Keywords: hepatocellular carcinoma, hypoxia-inducible factor, ablation, therapy, mechanism
Citation: Xiao C, Liu S, Ge G, Jiang H, Wang L, Chen Q, Jin C, Mo J, Li J, Wang K, Zhang Q and Zhou J (2023) Roles of hypoxia-inducible factor in hepatocellular carcinoma under local ablation therapies. Front. Pharmacol. 14:1086813. doi: 10.3389/fphar.2023.1086813
Received: 01 November 2022; Accepted: 18 January 2023;
Published: 06 February 2023.
Edited by:
Sarah El-Nakeep, Ain Shams University, EgyptReviewed by:
Anuhya Kommalapati, Mayo Clinic, United StatesAntonio Facciorusso, University of Foggia, Italy
Copyright © 2023 Xiao, Liu, Ge, Jiang, Wang, Chen, Jin, Mo, Li, Wang, Zhang and Zhou. This is an open-access article distributed under the terms of the Creative Commons Attribution License (CC BY). The use, distribution or reproduction in other forums is permitted, provided the original author(s) and the copyright owner(s) are credited and that the original publication in this journal is cited, in accordance with accepted academic practice. No use, distribution or reproduction is permitted which does not comply with these terms.
*Correspondence: Qianqian Zhang, WmhhbmdxcTIwMjIxMTMwQDEyNi5jb20=; Jianyu Zhou, emhvdWp5OTk4MEAxMjYuY29t
†These authors have contributed equally to this work and share first authorship