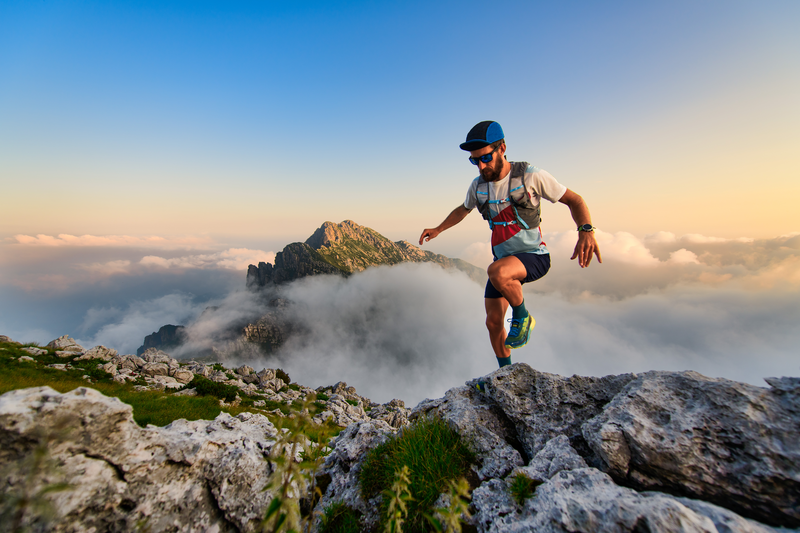
94% of researchers rate our articles as excellent or good
Learn more about the work of our research integrity team to safeguard the quality of each article we publish.
Find out more
REVIEW article
Front. Pharmacol. , 06 March 2023
Sec. Pharmacology of Anti-Cancer Drugs
Volume 14 - 2023 | https://doi.org/10.3389/fphar.2023.1078338
This article is part of the Research Topic Immune Checkpoint Inhibitor and Metabolites in the Tumor Microenvironment View all 9 articles
Unprecedented advances have been made in immune checkpoint inhibitors (ICIs) in the treatment of cancer. However, the overall benefits from ICIs are impaired by the increasing incidence of immune-related adverse events (irAEs). Although several factors and mechanisms have been proposed in the development of irAEs, there is still incomprehensive understanding of irAEs. Therefore, it is urgent to identify certain risk factors and biomarkers that predict the development of irAEs, as well as to understand the underlying mechanisms of these adverse events. Herein, we comprehensively summarize the state-of-the-art knowledge about clinical features and the related risk factors of irAEs. Particularly, we also discuss relevant mechanisms of irAEs and address the mechanism-based strategies, aiming to develop a tailored management approach for irAEs.
Immune checkpoint inhibitors (ICIs) therapy has revolutionized the management of multiple cancers and is now a mainstay approach for treating them. ICIs, including cytotoxic T lymphocyte protein 4 (CTLA-4), programmed cell death 1 (PD-1), and programmed cell death ligand 1 (PD-L1), mainly target proteins that negatively regulate T cell-meditated host immune response to cancer, thus enabling immune activation and antitumor response (Haslam and Prasad, 2019). Although patients in increasing number with cancer are eligible for ICIs therapy (Haslam et al., 2020), ICIs are also associated with a broad spectrum of side effects, termed as immune-related adverse events (irAEs) (Robert, 2020). These irAEs mirror aspects of primary autoimmune diseases and affect almost all organ systems (e.g., gastrointestinal tract, endocrine glands, skin and liver) with a range of severity (Dougan, 2017; Wang D. Y. et al., 2018; Postow et al., 2018; Liu et al., 2021a). Notably, irAEs are often accompanied with inflammatory side effects (Dougan, 2017), which are the important limitations to developing novel treatments.
It is worth noting that there are several aspects of irAEs that we have not yet understand, which has aroused several controversies in the field. For instance, several studies have indicated that there is a positive association of irAEs development with anti-tumor responses to ICIs or survival outcomes (Maher et al., 2019; Eggermont et al., 2020; Shankar et al., 2020). While other studies have supported poorer outcomes among patients with early or specific irAEs (Suresh and Naidoo, 2020). The possible reason is the difference of adjudication and attribution of irAEs, as well as immortal-time bias (Dall'Olio et al., 2020). Perhaps the management of irAEs is the realm that generates the greatest difficulties and controversies. Given that the anti-tumor responses and irAEs are representative of a robust immune reaction, corticosteroids or other immunosuppressive agents to control irAEs may lead to worse outcomes (Arbour et al., 2018).
As the administration of ICIs continues to increase in routine clinical practice, tailored management of irAEs has gained extensive attention. Current therapeutic recommendations against irAEs related to ICIs include discontinuation of immunotherapy and use of high-dose steroids. Meanwhile, American Society of Clinical Oncology (ASCO) (Brahmer et al., 2018), National Comprehensive Cancer Network (NCCN) (NCCN, 2021), Society for Immunotherapy of Cancer (SITC) (Brahmer et al., 2021) and European Society for Medical Oncology (ESMO) (Haanen et al., 2017) have also discussed the recommendations for specific irAE management. However, there is a need of further structured research to better identify the increased risk for irAEs and develop more individualized therapeutic strategies. In the present review, we highlight the current knowledge about clinical features and risk factors of irAEs, as well as address the mechanism-based strategies to develop a tailored management approach for these adverse events.
Due to the emerging use of ICIs for the treatment of cancer, the cumulated number of irAEs is increasing exponentially. irAEs have been observed in almost all organ system, including the gastrointestinal, cutaneous, pulmonary, neurologic, genitourinary, cardiovascular, and integumentary systems, with the median onset of 2–16 weeks from the commencement of therapy (Weber et al., 2015). Moreover, irAEs are graded using the Common Terminology Criteria for Adverse Events (CTCAE) and range from grade 1 to grade 5, which refers to mild, moderate, severe, life-threatening or fatal events (National Cancer Institute, 2019) (Table 1). Onset of irAEs ranged from a few days of ICI initiation to over 1 year after completion of therapy (Yoest, 2017; Parakh et al., 2018), and the risk of first-onset irAEs was threefold lower in the time period between 4 weeks and the end of treatment than during the first 4 weeks of treatment (Kanjanapan et al., 2019). For instance, the dermatological toxicities were observed at approximately 2–3 weeks, gastrointestinal and hepatic toxicities at 6–7 weeks, and endocrinologic events at 9 weeks after treatment initiation of ipilimumab (Weber et al., 2012). Noteworthily, irAEs presumably persisted long after the cessation of treatment, and it was possible that irAEs might last for many years after treatment. A recent case indicated that autoimmune hepatitis occurred 8 months after discontinuation of nivolumab in a patient with metastatic melanoma (Parakh et al., 2018). More importantly, the development of characteristic irAEs was positively associated with improved patient outcome (Maher et al., 2019; Eggermont et al., 2020; Shankar et al., 2020). A multicenter study reported an increase in overall survival in patients with related irAEs compared with those with no related irAEs (Maher et al., 2019). Likewise, another multicenter study demonstrated that there was a positive association between multisystem irAEs and improved survival in patients with advanced non-small-cell lung cancer (NSCLC) treated with ICIs (Shankar et al., 2020). Eggermont et al. revealed that patients with stage III melanoma who developed irAEs had a longer recurrence-free survival (RFS) (Eggermont et al., 2020). Given the immune mechanisms of anti-PD-1/PD-L1 antibodies, it was reasonable to associate the occurrence of autoimmune events with improved prognosis, as activation of the immune system can lead to tumor responses and autoimmunity. However, current evidence suggested that the early or specific irAEs were correlated with worse survival in ICI-treated patients with NSCLC. The reasonable explanation might be the difference of adjudication and attribution of irAEs, as well as immortal-time bias (Dall'Olio et al., 2020).
A recent study reported that the incidence of all grade irAEs from ICI monotherapy was as high as 90% (Pauken et al., 2019). irAEs were first identified after the introduction of anti-CTLA therapy in clinical practice (Wolchok et al., 2010; June et al., 2017). Previous studies indicated an overall incidence over 70% with anti-CTLA-4 and 27%–78% with anti-PD-1/anti-PD-L1 agents (Topalian et al., 2012; Kumar et al., 2017; Maughan et al., 2017; Elkrief et al., 2019; Wang et al., 2019). Among the diverse irAEs, cutaneous toxicities including rash, pruritus, and vitiligo were the most frequent (Weber et al., 2012). The overall incidence of cutaneous toxicities stemmed from CTLA-4 blockade ranged from 37% to 70% for all-grade and 1%–3% for grade 3 or higher cutaneous toxicities (Hodi et al., 2010; Eggermont et al., 2015). Whereas, cutaneous toxicities were less frequently reported with anti–PD-1 agents (17%–37%). Of note, ICIs could result in dose-dependent toxicities. Motzer et al. found that the incidence was 75%, 67% and 78% for all grade irAEs in patients with metastatic renal cell carcinoma receiving nivolumab 0.3, 2, and 10 mg/kg, respectively, and 5%, 17% and 13% for grade 3 or 4 irAEs (Motzer et al., 2015). Furthermore, a phase IIIb/IV study involving 360 advanced melanoma patients determined that the incidence of grade 3 or 4 irAEs was 33.9% with nivolumab 3 mg/kg plus ipilimumab 1 mg/kg versus 48.3% with nivolumab 1 mg/kg plus ipilimumab 3 mg/kg, while the incidence of all grade irAEs was 85.6% versus 93.8% (Lebbé et al., 2019). Anti-PD-1 therapy was associated with a lower rate of irAEs compared with anti-CTLA-4 therapy; however, other studies discovered that there were several treated patients (ranging from 7% to 24%) with severe or even fatal (grade 2 or higher) irAEs (Baumeister et al., 2016; Marrone et al., 2016). Moreover, a recent clinical study with metastatic melanoma patients revealed that 14% of patients experienced severe (grade 3 or 4) toxicities with PD-1/PD-L1 inhibitors alone (Vesely and Chen, 2020). The major fatal irAEs included cardiotoxicity, neurotoxicity and interstitial pneumonia, with the incidence of as high as 45% (Wang D. Y. et al., 2018). A recent review demonstrated that myocarditis, a type of fatal irAEs, was reported in up to 3% of patients treated with anti-CTLA-4 but less than 1% of patients receiving PD-L1 inhibitors (Dougan and Pietropaolo, 2020). Another study found high neurotoxicity in advanced melanoma patients after nivolumab, ipilimumab, or combination treatment with an overall rate of 2.8% and in combination treatment with a rate of 14% (Spain et al., 2017). Furthermore, a recent study highlighted ICIs-related stevens-Johnson syndrome/toxic epidermal necrolysis (SJS/TEN) events as fatal irAEs (Ma et al., 2021). For these fatal irAEs, the mortality rate of ICI-related SJS was 19.9%, and the mortality rate of ICI-related TEN was 61.6% (Zhu et al., 2021). Besides, patients with advanced melanoma (stage III or IV) could experience long-term toxicities after termination of anti-PD-1, and up to 43.2% of patients developed chronic irAEs (defined as irAEs persisting for at least 12 weeks after anti–PD-1 therapy cessation) (Patrinely et al., 2021).
The clinical features of irAEs are relatively obscure with subtle imaging changes and thus are difficult to determine, especially in the early stage. Therefore, it is imperative to understand and explore predictive risk factors or potential biomarkers for the occurrence of irAEs (Table 2).
Since irAEs are characterized by the abnormal activation of the immune system, patients with autoimmune disease may potentially have greater risks of developing irAEs. Retrospective studies indicated that patients with autoimmune diseases treated with ICIs had high rate (ranging from 28% to 60%) of having autoimmune disease flare (Danlos et al., 2018; Alexander et al., 2021; Fountzilas et al., 2022). A multicenter, retrospective study including 102 patients with underlying inflammatory bowel disease (IBD) treated with ICIs showed that gastrointestinal adverse events occurred in 41% of these patients compared with 11% of patients without histories of IBD (p < 0.001) (Abu-Sbeih et al., 2020). On the other hand, irAEs could also affect other new organ sites that were unaffected by autoimmunity prior to ICI therapy. A systemic review enrolling 123 patients with autoimmune diseases treated with ICIs indicated deterioration of existing autoimmune diseases in 50% of patients, de novo irAEs in 34% of patients, and both in 9% of patients (Abdel-Wahab et al., 2018). Another retrospective study analyzed 470 patients treated with ICIs and identified an association of the development of irAEs with both pre-existing history of autoimmune disease (adjusted OR = 2.57, 95% CI 1.46–4.52, p = 0.001) and family history of autoimmune disease (adjusted OR = 5.98, 95% CI 2.20–16.23, p < 0.001) (Michailidou et al., 2021). Inconsistently, a retrospective analysis of 417 patients treated with ICIs showed that there was no association between underlying autoimmune disease and irAE incidence or severity (Yeung et al., 2021). The possible explanation was the interindividual difference of patients’ status and risk for autoimmune disease at initiation of cancer immunotherapy.
In addition to the pre-existing autoimmune diseases, sex and body mass index was also identified as risk factors for the development of irAEs (Conforti et al., 2018; Valpione et al., 2018; Guzman-Prado et al., 2021). A recent meta-analysis revealed that higher BMI was associated with an increased risk of irAEs in patients on ICI therapies (OR = 2.62, 95% CI 1.70–4.03, p ≤ 0.00001) (Guzman-Prado et al., 2021). Moreover, a systematic review and meta-analysis reported that ICIs could improve overall survival for patients of both sexes, but males had a larger treatment effect from these drugs than females (Conforti et al., 2018). Another research supported that females were likely to be higher rates of irAEs (OR = 1.50, 95% CI 1.06–2.16 p = 0.022) (Valpione et al., 2018). On the contrary, ICIs-hypophysitis showed a more frequent occurrence in men than women, and the gender distribution of neurologic and vascular irAEs also appeared to be male-dominant (Triggianese et al., 2020). One of the possible explanations was that females were at higher risk of several autoimmune diseases. On the other hand, these differences might also be influenced by other variations such as genetic and ethnic factors. Further studies are needed to elucidate this disparity and the important roles of sex-specific factors in the development of irAEs.
Immune cells and cytokines play essential roles in tumor microenvironment and immune homeostasis (Harlin et al., 2009; Peng et al., 2012), which can be utilized by immunotherapy and contribute to the development of irAEs. In a multicenter study, Fujisawa et al. enrolled 101 patients with melanoma treated with nivolumab and revealed that increases in total white blood cell (WBC) count and decreases in relative lymphocyte count (RLC) were associated with severe irAEs (Fujisawa et al., 2017). Our previous study also concluded that levels of neutrophil-to-lymphocyte ratio (NLR), platelet-to-lymphocyte ratio (PLR) and neutrophil were associated with the increased risk of severe irAEs (Liu et al., 2021b). The possible explanation was that lymphopenia might suggest impaired cell-mediated immunity, whereas neutrophilia might reflect an intensive response to systemic inflammation (Ocana et al., 2017). In recent years, cytokine levels were also linked with the development of irAEs. Lower baseline levels and greater post-treatment increases in multiple chemokines were reportedly associated with irAEs (Khan S. et al., 2019). Emerging data showed that increased levels of proinflammatory cytokines, including IL1, IL2, and IFNα2, were strongly associated with severe irAEs, which could be integrated into the CYTOX score to predict severe irAE development (Lim et al., 2019). Tarhini et al. found that higher baseline IL17 levels were correlated with the development of grade 3 colitis in patients with melanoma treated with neoadjuvant ipilimumab (Tarhini et al., 2015). Intriguingly, Tanaka and others observed that increase in circulating IL-6 was significantly associated with irAEs in patients treated with nivolumab with psoriasiform dermatitis (Tanaka et al., 2017). Moreover, a retrospective analysis of 285 patients demonstrated that increased eosinophils, IL-6, IL-10, and immunoglobulin E were associated with immune-related cutaneous adverse events (Phillips et al., 2019). Mechanistically, elevated IL-6, as an autocrine regulator, promotes the development of Th17 cells and thus may enhance inflammation of the skin progressing to epidermal hyperplasia (Watanabe et al., 2009). Another prospective study observed that low baseline IL6 serum levels were associated with higher rates of irAEs in patients with metastatic melanoma treated with ipilimumab (Valpione et al., 2018). Similarly, a decrease in levels of IL-10 was reportedly associated with irAEs in patients treated with ipilimumab and anti-CTLA-4 ticilimumab (Reuben et al., 2006; Sun et al., 2008). These results are consistent with inference that IL6 increases tumor invasiveness and compromises the immune-inflammatory regulation (Patel and Gooderham, 2015; Roth et al., 2016), which may impair the immune response elicited by CTLA4 blockade and lower the risk of irAEs. Therefore, these findings collectively indicate the association of immune cells and cytokines with irAEs, which may be helpful in discovering potential biomarkers and practical therapeutic targets for irAEs.
Due to genetic factor as a contributing factor for autoimmunity, the role of genetic variability has also been implicated in the development of irAEs. A pilot study indicated that patients with ICI-induced inflammatory arthritis of European descent were more likely to be positive for HLA-DRB1 shared epitope alleles than healthy controls (Cappelli et al., 2019). Moreover, a striking predominance of HLA-DR4 occurred in patients treated with ICIs who developed autoimmune, insulin-dependent diabetes (Stamatouli et al., 2018). A cohort study including 173 patients with melanoma treated with ipilimumab identified that CTLA-4 SNP -1661A>G (rs4553808) was correlated with an increased risk of endocrine irAEs (Queirolo et al., 2018). Another analysis of 96 patients with NSCLC treated with nivolumab found an association of PDCD1 804 C>T (rs2227981) with decreased incidence of irAEs (Bins et al., 2018). These initial findings are required to be validated in the future studies with larger patient cohorts.
Increasing evidence provided that gut microbiota, such as Bacteroides, Clostridium and Faecalibacterium, was implicated in the maintenance of immune homeostasis stimulating the production of anti-inflammatory cytokines or inducing expansion of T-regulatory cells, which might be also involved in the development of irAEs (Mazmanian et al., 2005; Sokol et al., 2008; Atarashi et al., 2011; Clavel et al., 2017; Gut Microbiota May Mediate AEs, 2021; Pham et al., 2021).
A prospective study claimed that increased abundance of bacteria belonging to the Bacteroidetes phylum was associated with a reduced incidence of colitis in metastatic melanoma patients treated with ipilimumab (Dubin et al., 2016). Notably, another clinical study showed that a significant reduction of Firmicutes (2 times lower than baseline) was correlated with ipilimumab-induced colitis in metastatic melanoma patients (Chaput et al., 2017). Recently, Andrews et al. profiled the gut microbiota signatures using 16s RNA sequencing in patients treated with PD-1 and CTLA-4 inhibitors, and demonstrated a significantly higher abundance of Bacteroides compared with those without irAEs (Andrews et al., 2021). Also, our real-word study provided that patients with severe irAEs showed a visibly higher abundance of Streptococcus, Paecalibacterium, and Stenotrophomonas, and patients with mild irAEs had a higher abundance of Faecalibacterium and unidentified_Lachnospiraceae, which jointly suggested that gut microbiota could serve as an informative source for developing predictive biomarkers and predicting the occurrence of irAEs (Liu et al., 2021c). A previous study highlighted that the microbial-derived products could trigger an innate immune response, eventually leading to the activation of self-reactive immune cells (Khan Z. et al., 2019), which might interpretate that patients with bacterial disorder were more likely to experience irAEs. Future prospective clinical trials should focus on the biomarkers based on pretreatment risk of irAEs for better prediction of ICI-related irAEs.
Although there are certain commonalities between the irAEs and autoimmune diseases or autoinflammatory reactions, the mechanisms behind irAEs are not well understood. There are several proposed main mechanisms that contribute to irAEs in response to ICIs (Figure 1).
FIGURE 1. Relevant mechanisms of irAEs in response to ICIs. ICIs including PD-1, PD-L1 and CTLA-4 inhibitors, can inhibit immune checkpoints (PD-L1 and B7-1,2) and induce a series of cellular alterations, such as activated autoimmune CD8+ T cells and impaired Treg cell survival, and subsequently lead to T cell activation. On the one hand, activated T cell interacts with B cell, which can result in autoantibody production and ultimately lead to irAEs. On the other hand, T cell activation can induce the production of proinflammatory cytokines, such as IL-17A, IL-21 and IL-22, which consequently impaires the Treg cell function, induces T cell proliferation or reactivation and directly or indirectly contributes to irAEs.
Previous studies utilized a CTLA-4 knockout mice model and found that the mice developed T cell lymphoproliferation and T cell-mediated autoimmunity (Wing et al., 2008; Klocke et al., 2016). In accordance with these data in mice, individualsx with CTLA-4-related genetic disorders also had regulatory T (Treg) cell defects and autoimmune infiltration (Lo et al., 2016). Consistent with these results, several recent researches suggested that activation or reactivation of T cells was thought to be a dominant factor in the development of ICI-related irAEs (June et al., 2017; Dougan et al., 2021a). Recently, Takahashi et al. found that CD8+ lymphocyte infiltration was significantly greater in irAE colitis than that in ulcerative colitis (Takahashi et al., 2023). Further research confirmed that activated cytotoxic CD8+ T cells accelerated anti-PD-1 antibody-induced psoriasis-like dermatitis via IL-6 (Tanaka et al., 2020). Typically, Treg cells downregulate immune responses by inhibiting effector T cell proliferation and cytokine release to regulate self-tolerance. The functional homeostasis between Treg cells and type 17 T helper (Th17) cells served as a prominent factor in irAEs associated with ICIs (Knochelmann et al., 2018). Enhanced Th17 cell responses could contribute to the production of proinflammatory cytokines such as IL-17A, IL-21 and IL-22, consequently participating in the pathogenesis of autoimmune diseases and irAEs (Noack and Miossec, 2014; Tarhini et al., 2015).
Interestingly, a comprehensive review highlighted that cross-reactivity between anti-tumor T cells and similar antigens on healthy cells might also underlie the pathogenesis of some irAEs (Michot et al., 2016). For instance, vitiligo appeared to be more frequent in patients with melanoma, which suggested cross-reactivity between T cells and tumor antigens and melanocytes (Sandigursky and Mor, 2018). Due to the low selectivity among the tumor-reactive T cell subjects, cross-reactivity had also been suggested for ICI-related myocarditis \(Cheng and Loscalzo, 2017). Johnson et al. reported that two patients developed fatal myocarditis after treatment with ipilimumab and nivolumab (Johnson et al., 2016). Possible mechanism for this toxicity was that the tumor, skeletal muscle, and heart shared the common high-frequency T-cell receptors, which led to T-cell-driven drug reaction. In recent years, Berner et al. identified nine shared antigens between tumor tissue and skin, which was able to stimulate CD8+ and CD4+ T cells in vitro, highlighting a potential mechanism of ICI-mediated autoimmune toxic effects (Berner et al., 2019).
In addition to the regulation of T cells, both anti-CTLA-4 and anti-PD-1/PD-L1 antibodies could increase cytokine production. Anti-CTLA-4 antibody could enhance CD4+ and CD8+ T cell activation, subsequently releasing cytokines including tumor necrosis factor (TNF), interferon-γ (IFNγ) and IL-2, which contributed to further T cell proliferation and reactivation (Laurent et al., 2013; Seidel et al., 2018). Besides, Luoma et al. observed the upregulated expression of cytokines and their receptors in both T cell and myeloid cell of ICI-related colitis patients, which suggested that other innate and T cell-derived cytokines were associated with ICI-related colitis and could serve as additional treatment targets (Luoma et al., 2020). In recent years, TNF inhibitors have been successfully adopted to treat different irAEs in patients receiving ICIs, further highlighting the importance of cytokines in the pathogenesis of irAEs (Kim et al., 2017). Fundamentally, further studies are required to address the precise roles of these cytokines in irAEs and the development of T cell activation or reactivation.
Interactions between T cells and B cells can result in autoantibody production and play an important role in humoral immunity, while aberrant interactions are associated with autoimmunity (Petersone et al., 2018). Das et al. found B cell changes in patients with irAEs after the first cycle of therapy with either anti-CTLA4 or anti-PD1, or in combination, and they further identified B cell activation in cells with genomic profiles of CD21lo B cells by single-cell RNA sequencing, which implied that targeting B cells might reduce irAEs in these patients (Das et al., 2018). On the other hand, the development of autoantibodies was common with ipilimumab treatment, and autoantibody formation was associated with irAEs and antitumor efficacy (de Moel et al., 2019). Toi et al. indicated that the presence of the preexisting antibodies was independently associated with the development of irAEs in patients with NSCLC treated with anti-PD-1 (Toi et al., 2019). A recent study indicated that ICIs might exacerbate bullous pemphigoid (BP) as an irAE through the generation of anti-BP180-NC16A IgG autoantibodies (Sadik et al., 2019). Whether these autoantibodies reflect an underlying mechanism needs further elaborated experimental researches to validate.
Understanding the related mechanisms driving irAEs will contribute to targeted therapeutic strategies. Alongside the recommendations and therapeutic algorithms of ASCO [11], NCCN [12], SITC [13] and ESMO [14] for irAE management, several mechanism-based therapeutic strategies are also discussed (Table 3).
Given that activation or reactivation of T cells is considered to be the dominant factor in the development of ICI-related irAEs, inhibition of T cell response and migration may be a tailored strategy for irAEs. Glucocorticoids are the preferred treatment for most irAEs except for endocrine irAEs and effective in mitigating symptoms. Animal models demonstrated that glucocorticoids might inhibit antitumor T cell responses and were well known to induce apoptosis of activated T cell (Pauken et al., 2019). Ambiguously, there are some disparities in findings from retrospective studies of patients’ treatment with ICIs who received glucocorticoids, though it is suggested that they overall had deleterious effects on antitumor responses, particularly when glucocorticoids were used at initiation of immunotherapy (Arbour et al., 2018; Faje et al., 2018). Moreover, there is a need to introduce steroid-sparing strategies with the occurrence of steroid-refractory irAEs, mainly including immune-related hepatitis, nephritis and pancreatitis. For instance, patients with steroid-refractory pneumonitis can be treated with mycophenolate-containing immunosuppressants (Thompson et al., 2019). Due to the significant side effects in the treatment of ICI-associated colitis with corticosteroids or immunosuppressive agents, a recent study provided novel evidence that modulation of the gut microbiome via fecal microbiota transplantation could abrogate ICI-associated colitis with a substantial reduction in CD8+ T-cell density (Wang Y. et al., 2018). Recent studies reported that vedolizumab, an inhibitor of α4β7 integrin that inhibited the migration of T cells into gastrointestinal mucosa, could be used instead of infliximab against immune-related colitis (Bergqvist et al., 2017; Abu-Sbeih et al., 2018), proposing α4β7 integrin as an attractive target for immunotherapy toxicities.
Owing to the important roles of acute inflammatory cytokines, such as TNFα, IL-6 and IL-1β in irAEs, inhibiting these cytokines may be a promising strategy for the treatment of irAEs. Of these, inhibition of TNFα appeared to be effective for severe, refractory, immune-related colitis and inflammatory arthritis (Baddley et al., 2018; Mooradian et al., 2020; Dougan et al., 2021b). Johnson et al. suggested that for grades 3 and 4 colitis patients, the addition of infliximab (targeted TNFα) to glucocorticoids was significantly associated with a shorter time to symptom resolution than utilization of glucocorticoids alone (Johnson et al., 2018). In a mice model, TNF inhibitors concomitantly with combined CTLA-4 and PD-1 immunotherapy ameliorated colitis and improved antitumor efficacy, which provided clinically feasible strategies to dissociate efficacy and toxicity for cancer immunotherapy (Perez-Ruiz et al., 2019).
Monoclonal antibodies have also been recommended for the management of some steroid-refractory irAEs (Kim et al., 2017; Siakavellas and Bamias, 2018). Clinical improvement was noted in 80% of patients with nivolumab-associated grades 3 and 4 irAEs (predominantly pneumonitis) who received tocilizumab (targeted IL-6) (Stroud et al., 2019). Infliximab, a chimeric human-murine monoclonal antibody, was used to the treatment of steroid-refractory irAEs (Araujo et al., 2021). In recent years, a series of cases of successful response to monoclonal antibodies, such as abatacept (targeted CTLA-4) and alemtuzumab (targeted CD52), were reported in patients with immune-related myocarditis (Esfahani et al., 2019b; Salem et al., 2019). A recent case also demonstrated that secukinumab (targeted IL-17A) could effectively manage ICI-induced inflammatory arthropathy (Ma et al., 2022). Additionally, intravenous immunoglobulin comprised of pooled IgG antibodies from the serum of thousands of donors was considered as a second-line therapy for neurological and hematological irAEs (Schwab and Nimmerjahn, 2013). Since several irAEs were caused directly by autoantibodies, such as some hematological or neuromuscular irAEs, plasma exchange could be a feasible strategy against irAEs by removing the pathogenetic autoantibody from the circulation, especially in severe cases of myasthenia gravis or Guillain–Barré syndrome (Touat et al., 2017). For instance, Verma et al. showed that rituximab (targeted CD20) could be used to treat patients with immunotherapy-induced myasthenia gravis (Verma et al., 2021). Clinical trials evaluating strategies to prevent irAEs are needed to institute in the future.
Despite the benefits of tailored management for irAEs, early intervention and prophylaxis has grown in importance. A retrospective analysis of 179 patients with immune-related colitis of all grades found that compared with patients who received immunosuppressive therapy (infliximab or vedolizumab) > 10 days after onset of colitis, patients who received immunosuppression therapy early (≤10 days) were less likely to be hospitalized, experienced steroid taper failure less frequently, had a shorter course of steroid treatment and had a shorter duration of symptoms (Abu-Sbeih et al., 2019). The JAK-STAT and mTOR pathway played important roles in cellular processes and mediated downstream signaling of numerous cytokines. A previous studies indicated that excessive JAK1 signaling contributed to cancer evasion and development of autoimmunity (Chan et al., 2019), and inhibition of JAK-STAT displayed synergistic effects with ICIs to overcome treatment resistance (Lu et al., 2017). Furthermore, the addition of the mTOR inhibitor in a melanoma patient treated with PD-1 blockade could promote ongoing anti-PD-1 efficacy and reduce levels of irAE-related cytokines/chemokines (e.g., IL-5, IL-17, and CXCL10) (Esfahani et al., 2019a). There are also several prophylactic strategies through disease-specific therapy (such as vedolizumab for IBD) before commencing ICI to prevent toxicity in patients with underlying active autoimmune disease (Haanen et al., 2020b). Due to the fact that not all patients with autoimmune diseases experienced irAEs, prophylactic strategies might be considered and studied in prospective trials. Nevertheless, proactive commencement of disease-specific therapy was a reasonable strategy for patients with a high risk of new toxicity and few alternative treatment options (Haanen et al., 2020a).
Although ICIs have revolutionized the current treatment and outlook for multiple cancer types, their therapeutic efficacy is restricted by irAEs. We have attempted to summarize the clinical and mechanistic features of irAEs and the related risk factors for their occurrence. We also generalized the mechanism-based therapeutic strategies to mitigate irAEs while maintaining therapeutic benefit of ICIs (Figure 2).
FIGURE 2. Mechanism-based therapeutic strategies for managing irAEs. Mechanism-based therapeutic strategies including inhibition of T cell activation, inhibition of T cell proliferation, reactivation or migration, cytokines blockade and monoclonal antibodies are listed. Glucocorticoids inhibit T cell activation for most irAEs except for endocrine irAEs, and fecal microbiota transplantation abrogates ICI-associated colitis with a reduction in CD8+ T-cell density. Vedolizumab and mycophenolate-containing immunosuppressants were used for steroid-refractory irAEs by inhibting T cell migration, proliferation or reactivation. Cytokines blockade including infliximab and TNF inhibtors were considered in severe, refractory and immune-related colitis. Monoclonal antibodies including tocilizumab, abatacept, alemtuzumab and immunoglobulin were recommended for the management of several steroid-refractory irAEs.
At present, the mechanism of irAEs is not fully elucidated, though tissue-specific irAEs are supposed to be meditated by T cells, B cells and a mixed aetiology. Recent development of murine irAEs models, including patient-derived xenograft and humanized mouse models, had a critical role for designing specific therapies and guiding precision medicine approaches in clinic (Shultz et al., 2019). Furthermore, novel insights from clinical and high-throughput data to develop accurate irAEs models contributed to understanding the etiology and pathogenesis of irAEs following immunotherapy, as well as prioritizing the clinical therapeutic regimens for clinicians (Bayless et al., 2021).
Currently, development of novel immunotherapeutic approaches may provide alternative treatments for irAEs. As the gut is a known modulator of specific T cell subsets (Gopalakrishnan et al., 2018), and the gut microbiome plays a role in driving autoimmunity and irAEs (McCulloch et al., 2022), altering the microbiome may be a promising strategy for irAEs. Wang et al. reported a successful treatment of ICI-related colitis through fecal microbiota transplant (Wang Y. et al., 2018). Another intriguing targeted immunotherapeutic approach is the use of bi-specific antibodies (binding to two or three different entities simultaneously). T cell-based bispecific antibodies could bridge T cells to tumor cells to facilitate more focal T cell activation (Labrijn et al., 2019), which might be a novel potential approach to abrogate the toxicity of ICIs while maintaining their therapeutic benefit.
Taken together, comprehensively understanding the clinical and mechanistic features of irAEs will be conducive to taking mechanism-based measures in clinical practice and thus providing the crucial help for clinicians to deal with them.
WL, ZL and YL wrote and edited the manuscript. BS reviewed the final draft and all authors approved it for publication.
This work was supported by the National Natural Science Foundation of China (grant number 82104307 to BS, grant number 82204534 to WL), Natural Science Foundation of Hunan Province (grant number 2021JJ40865 to BS, grant number 2021JJ40847 to WL), Scientific Research Fund Project of Hunan Provincial Health Commission (grant number 202313016776 to BS).
The authors declare that the research was conducted in the absence of any commercial or financial relationships that could be construed as a potential conflict of interest.
All claims expressed in this article are solely those of the authors and do not necessarily represent those of their affiliated organizations, or those of the publisher, the editors and the reviewers. Any product that may be evaluated in this article, or claim that may be made by its manufacturer, is not guaranteed or endorsed by the publisher.
ICIs, Immune checkpoint inhibitors; irAEs, immune-related adverse events; CTLA-4, cytotoxic T lymphocyte protein 4; PD-1, programmed cell death 1; PD-L1, programmed cell death ligand 1; ASCO, American Society of Clinical Oncology; NCCN, National Comprehensive Cancer Network; SITC, Society for Immunotherapy of Cancer; ESMO, European Society for Medical Oncology; CTCAE, Common Terminology Criteria for Adverse Events; NSCLC, non-small-cell lung cancer; IBD, inflammatory bowel disease; WBC, white blood cell; RLC, relative lymphocyte count; NLR, neutrophil-to-lymphocyte ratio; PLR, platelet-to-lymphocyte ratio; Treg, regulatory T; Th17, type 17 T helper; BP, bullous pemphigoid; TNF, tumor necrosis factor; FNγ, interferon-γ.
Abdel-Wahab, N., Shah, M., Lopez-Olivo, M. A., and Suarez-Almazor, M. E. (2018). Use of immune checkpoint inhibitors in the treatment of patients with cancer and preexisting autoimmune disease: A systematic review. Ann. Intern Med. 168 (2), 121–130. doi:10.7326/m17-2073
Abu-Sbeih, H., Ali, F. S., Alsaadi, D., Jennings, J., Luo, W., Gong, Z., et al. (2018). Outcomes of vedolizumab therapy in patients with immune checkpoint inhibitor-induced colitis: A multi-center study. J. Immunother. Cancer 6 (1), 142. doi:10.1186/s40425-018-0461-4
Abu-Sbeih, H., Ali, F. S., Wang, X., Mallepally, N., Chen, E., Altan, M., et al. (2019). Early introduction of selective immunosuppressive therapy associated with favorable clinical outcomes in patients with immune checkpoint inhibitor-induced colitis. J. Immunother. Cancer 7 (1), 93. doi:10.1186/s40425-019-0577-1
Abu-Sbeih, H., Faleck, D. M., Ricciuti, B., Mendelsohn, R. B., Naqash, A. R., Cohen, J. V., et al. (2020). Immune checkpoint inhibitor therapy in patients with preexisting inflammatory bowel disease. J. Clin. Oncol. 38 (6), 576–583. doi:10.1200/jco.19.01674
Alexander, S., Swami, U., Kaur, A., Gao, Y., Fatima, M., Ginn, M. M., et al. (2021). Safety of immune checkpoint inhibitors in patients with cancer and pre-existing autoimmune disease. Ann. Transl. Med. 9 (12), 1033. doi:10.21037/atm-20-8124
Andrews, M. C., Duong, C. P. M., Gopalakrishnan, V., Iebba, V., Chen, W. S., Derosa, L., et al. (2021). Gut microbiota signatures are associated with toxicity to combined CTLA-4 and PD-1 blockade. Nat. Med. 27 (8), 1432–1441. doi:10.1038/s41591-021-01406-6
Araujo, D. V., Muniz, T. P., Yang, A., Keshavarzi, S., Sorotsky, H., Butler, M. O., et al. (2021). Real world outcomes and hepatotoxicity of infliximab in the treatment of steroid-refractory immune-related adverse events. Curr. Oncol. 28 (3), 2173–2179. doi:10.3390/curroncol28030201
Arbour, K. C., Mezquita, L., Long, N., Rizvi, H., Auclin, E., Ni, A., et al. (2018). Impact of baseline steroids on efficacy of programmed cell death-1 and programmed death-ligand 1 blockade in patients with non-small-cell lung cancer. J. Clin. Oncol. 36 (28), 2872–2878. doi:10.1200/jco.2018.79.0006
Atarashi, K., Tanoue, T., Shima, T., Imaoka, A., Kuwahara, T., Momose, Y., et al. (2011). Induction of colonic regulatory T cells by indigenous Clostridium species. Science 331 (6015), 337–341. doi:10.1126/science.1198469
Baddley, J. W., Cantini, F., Goletti, D., Gómez-Reino, J. J., Mylonakis, E., San-Juan, R., et al. (2018). ESCMID study group for infections in compromised hosts (ESGICH) consensus document on the safety of targeted and biological therapies: An infectious diseases perspective (soluble immune effector molecules [I]: Anti-tumor necrosis factor-α agents). Clin. Microbiol. Infect. 24 (2), S10–S20. doi:10.1016/j.cmi.2017.12.025
Baumeister, S. H., Freeman, G. J., Dranoff, G., and Sharpe, A. H. (2016). Coinhibitory pathways in immunotherapy for cancer. Annu. Rev. Immunol. 34, 539–573. doi:10.1146/annurev-immunol-032414-112049
Bayless, N. L., Bluestone, J. A., Bucktrout, S., Butterfield, L. H., Jaffee, E. M., Koch, C. A., et al. (2021). Development of preclinical and clinical models for immune-related adverse events following checkpoint immunotherapy: A perspective from SITC and AACR. J. Immunother. Cancer 9 (9), e002627. doi:10.1136/jitc-2021-002627
Bergqvist, V., Hertervig, E., Gedeon, P., Kopljar, M., Griph, H., Kinhult, S., et al. (2017). Vedolizumab treatment for immune checkpoint inhibitor-induced enterocolitis. Cancer Immunol. Immunother. 66 (5), 581–592. doi:10.1007/s00262-017-1962-6
Berner, F., Bomze, D., Diem, S., Ali, O. H., Fässler, M., Ring, S., et al. (2019). Association of checkpoint inhibitor-induced toxic effects with shared cancer and tissue antigens in non-small cell lung cancer. JAMA Oncol. 5 (7), 1043–1047. doi:10.1001/jamaoncol.2019.0402
Bins, S., Basak, E. A., El Bouazzaoui, S., Koolen, S. L. W., Oomen-de Hoop, E., van der Leest, C. H., et al. (2018). Association between single-nucleotide polymorphisms and adverse events in nivolumab-treated non-small cell lung cancer patients. Br. J. Cancer 118 (10), 1296–1301. doi:10.1038/s41416-018-0074-1
Brahmer, J. R., Abu-Sbeih, H., Ascierto, P. A., Brufsky, J., Cappelli, L. C., Cortazar, F. B., et al. (2021). Society for Immunotherapy of Cancer (SITC) clinical practice guideline on immune checkpoint inhibitor-related adverse events. J. Immunother. Cancer 9 (6), e002435. doi:10.1136/jitc-2021-002435
Brahmer, J. R., Lacchetti, C., Schneider, B. J., Atkins, M. B., Brassil, K. J., Caterino, J. M., et al. (2018). Management of immune-related adverse events in patients treated with immune checkpoint inhibitor therapy: American society of clinical Oncology clinical practice guideline. J. Clin. Oncol. 36 (17), 1714–1768. doi:10.1200/jco.2017.77.6385
Cappelli, L. C., Dorak, M. T., Bettinotti, M. P., Bingham, C. O., and Shah, A. A. (2019). Association of HLA-DRB1 shared epitope alleles and immune checkpoint inhibitor-induced inflammatory arthritis. Rheumatol. Oxf. 58 (3), 476–480. doi:10.1093/rheumatology/key358
Chan, L. C., Li, C. W., Xia, W., Hsu, J. M., Lee, H. H., Cha, J. H., et al. (2019). IL-6/JAK1 pathway drives PD-L1 Y112 phosphorylation to promote cancer immune evasion. J. Clin. Invest. 129 (8), 3324–3338. doi:10.1172/JCI126022
Chaput, N., Lepage, P., Coutzac, C., Soularue, E., Le Roux, K., Monot, C., et al. (2017). Baseline gut microbiota predicts clinical response and colitis in metastatic melanoma patients treated with ipilimumab. Ann. Oncol. 28 (6), 1368–1379. doi:10.1093/annonc/mdx108
Cheng, F. X., and Loscalzo, J. (2017). Autoimmune cardiotoxicity of cancer immunotherapy. Trends Immunol. 38 (2), 77–78. doi:10.1016/j.it.2016.11.007
Clavel, T., Gomes-Neto, J. C., Lagkouvardos, I., and Ramer-Tait, A. E. (2017). Deciphering interactions between the gut microbiota and the immune system via microbial cultivation and minimal microbiomes. Immunol. Rev. 279 (1), 8–22. doi:10.1111/imr.12578
Conforti, F., Pala, L., Bagnardi, V., De Pas, T., Martinetti, M., Viale, G., et al. (2018). Cancer immunotherapy efficacy and patients' sex: A systematic review and meta-analysis. Lancet Oncol. 19 (6), 737–746. doi:10.1016/s1470-2045(18)30261-4
Dall'Olio, F. G., Di Nunno, V., and Massari, F. (2020). Immortal time bias question in the association between toxicity and outcome of immune checkpoint inhibitors. J. Clin. Oncol. 38 (1), 105–106. doi:10.1200/jco.19.01728
Danlos, F. X., Voisin, A. L., Dyevre, V., Michot, J. M., Routier, E., Taillade, L., et al. (2018). Safety and efficacy of anti-programmed death 1 antibodies in patients with cancer and pre-existing autoimmune or inflammatory disease. Eur. J. Cancer 91, 21–29. doi:10.1016/j.ejca.2017.12.008
Das, R., Bar, N., Ferreira, M., Newman, A. M., Zhang, L., Bailur, J. K., et al. (2018). Early B cell changes predict autoimmunity following combination immune checkpoint blockade. J. Clin. Invest. 128 (2), 715–720. doi:10.1172/jci96798
de Moel, E. C., Rozeman, E. A., Kapiteijn, E. H., Verdegaal, E. M. E., Grummels, A., Bakker, J. A., et al. (2019). Autoantibody development under treatment with immune-checkpoint inhibitors. Cancer Immunol. Res. 7 (1), 6–11. doi:10.1158/2326-6066.cir-18-0245
Dougan, M. (2017). Checkpoint blockade toxicity and immune homeostasis in the gastrointestinal tract. Front. Immunol. 8, 1547. doi:10.3389/fimmu.2017.01547
Dougan, M., Luoma, A. M., Dougan, S. K., and Wucherpfennig, K. W. (2021a). Understanding and treating the inflammatory adverse events of cancer immunotherapy. Cell. 184 (6), 1575–1588. doi:10.1016/j.cell.2021.02.011
Dougan, M., and Pietropaolo, M. (2020). Time to dissect the autoimmune etiology of cancer antibody immunotherapy. J. Clin. Invest. 130 (1), 51–61. doi:10.1172/jci131194
Dougan, M., Wang, Y., Rubio-Tapia, A., and Lim, J. K. (2021b). AGA clinical practice update on diagnosis and management of immune checkpoint inhibitor colitis and hepatitis: Expert review. Gastroenterology 160 (4), 1384–1393. doi:10.1053/j.gastro.2020.08.063
Dubin, K., Callahan, M. K., Ren, B., Khanin, R., Viale, A., Ling, L., et al. (2016). Intestinal microbiome analyses identify melanoma patients at risk for checkpoint-blockade-induced colitis. Nat. Commun. 7, 10391. doi:10.1038/ncomms10391
Eggermont, A. M. M., Chiarion-Sileni, V., and Grob, J. J. (2015). Correction to lancet oncol 2015; 16: 522-30. Adjuvant ipilimumab versus placebo after complete resection of high-risk stage III melanoma (EORTC 18071): A randomised, double-blind, phase 3 trial. Lancet Oncol. 16 (6), e262. doi:10.1016/S1470-2045(15)70271-8
Eggermont, A. M. M., Kicinski, M., Blank, C. U., Mandala, M., Long, G. V., Atkinson, V., et al. (2020). Association between immune-related adverse events and recurrence-free survival among patients with stage III melanoma randomized to receive pembrolizumab or placebo: A secondary analysis of a randomized clinical trial. JAMA Oncol. 6 (4), 519–527. doi:10.1001/jamaoncol.2019.5570
Elkrief, A., El Raichani, L., Richard, C., Messaoudene, M., Belkaid, W., Malo, J., et al. (2019). Antibiotics are associated with decreased progression-free survival of advanced melanoma patients treated with immune checkpoint inhibitors. Oncoimmunology 8 (4), e1568812. doi:10.1080/2162402x.2019.1568812
Esfahani, K., Al-Aubodah, T. A., Thebault, P., Lapointe, R., Hudson, M., Johnson, N. A., et al. (2019a). Targeting the mTOR pathway uncouples the efficacy and toxicity of PD-1 blockade in renal transplantation. Nat. Commun. 10 (1), 4712. doi:10.1038/s41467-019-12628-1
Esfahani, K., Buhlaiga, N., Thébault, P., Lapointe, R., Johnson, N. A., and Miller, W. H. (2019b). Alemtuzumab for immune-related myocarditis due to PD-1 therapy. N. Engl. J. Med. 380 (24), 2375–2376. doi:10.1056/NEJMc1903064
Faje, A. T., Lawrence, D., Flaherty, K., Freedman, C., Fadden, R., Rubin, K., et al. (2018). High-dose glucocorticoids for the treatment of ipilimumab-induced hypophysitis is associated with reduced survival in patients with melanoma. Cancer 124 (18), 3706–3714. doi:10.1002/cncr.31629
Fountzilas, E., Lampaki, S., Koliou, G. A., Koumarianou, A., Levva, S., Vagionas, A., et al. (2022). Real-world safety and efficacy data of immunotherapy in patients with cancer and autoimmune disease: The experience of the hellenic cooperative Oncology group. Cancer Immunol. Immunother. 71 (2), 327–337. doi:10.1007/s00262-021-02985-6
Fujisawa, Y., Yoshino, K., Otsuka, A., Funakoshi, T., Fujimura, T., Yamamoto, Y., et al. (2017). Fluctuations in routine blood count might signal severe immune-related adverse events in melanoma patients treated with nivolumab. J. Dermatol Sci. 88 (2), 225–231. doi:10.1016/j.jdermsci.2017.07.007
Gopalakrishnan, V., Helmink, B. A., Spencer, C. N., Reuben, A., and Wargo, J. A. (2018). The influence of the gut microbiome on cancer, immunity, and cancer immunotherapy. Cancer Cell. 33 (4), 570–580. doi:10.1016/j.ccell.2018.03.015
Gut Microbiota May Mediate AEs (2021). Gut microbiota may mediate AEs. Cancer Discov. 11 (9), 2118. doi:10.1158/2159-8290.cd-nb2021-0371
Guzman-Prado, Y., Ben Shimol, J., and Samson, O. (2021). Body mass index and immune-related adverse events in patients on immune checkpoint inhibitor therapies: A systematic review and meta-analysis. Cancer Immunol. Immunother. 70 (1), 89–100. doi:10.1007/s00262-020-02663-z
Haanen, J., Carbonnel, F., Robert, C., Kerr, K. M., Peters, S., Larkin, J., et al. (2017). Management of toxicities from immunotherapy: ESMO clinical practice guidelines for diagnosis, treatment and follow-up. Ann. Oncol. 28 (4), iv119–iv142. doi:10.1093/annonc/mdx225
Haanen, J., Ernstoff, M. S., Wang, Y., Menzies, A. M., Puzanov, I., Grivas, P., et al. (2020b). Autoimmune diseases and immune-checkpoint inhibitors for cancer therapy: Review of the literature and personalized risk-based prevention strategy. Ann. Oncol. 31 (6), 724–744. doi:10.1016/j.annonc.2020.03.285
Haanen, J., Ernstoff, M., Wang, Y., Menzies, A., Puzanov, I., Grivas, P., et al. (2020a). Rechallenge patients with immune checkpoint inhibitors following severe immune-related adverse events: Review of the literature and suggested prophylactic strategy. J. Immunother. Cancer 8 (1), e000604. doi:10.1136/jitc-2020-000604
Harlin, H., Meng, Y., Peterson, A. C., Zha, Y., Tretiakova, M., Slingluff, C., et al. (2009). Chemokine expression in melanoma metastases associated with CD8+ T-cell recruitment. Cancer Res. 69 (7), 3077–3085. doi:10.1158/0008-5472.can-08-2281
Haslam, A., Gill, J., and Prasad, V. (2020). Estimation of the percentage of US patients with cancer who are eligible for immune checkpoint inhibitor drugs. JAMA Netw. Open 3 (3), e200423. doi:10.1001/jamanetworkopen.2020.0423
Haslam, A., and Prasad, V. (2019). Estimation of the percentage of US patients with cancer who are eligible for and respond to checkpoint inhibitor immunotherapy drugs. JAMA Netw. Open 2 (5), e192535. doi:10.1001/jamanetworkopen.2019.2535
Hodi, F. S., O'Day, S. J., McDermott, D. F., Weber, R. W., Sosman, J. A., Haanen, J. B., et al. (2010). Improved survival with ipilimumab in patients with metastatic melanoma. N. Engl. J. Med. 363 (8), 711–723. doi:10.1056/NEJMoa1003466
Johnson, D. B., Balko, J. M., Compton, M. L., Chalkias, S., Gorham, J., Xu, Y., et al. (2016). Fulminant myocarditis with combination immune checkpoint blockade. N. Engl. J. Med. 375 (18), 1749–1755. doi:10.1056/NEJMoa1609214
Johnson, D. H., Zobniw, C. M., Trinh, V. A., Ma, J., Bassett, R. L., Abdel-Wahab, N., et al. (2018). Infliximab associated with faster symptom resolution compared with corticosteroids alone for the management of immune-related enterocolitis. J. Immunother. Cancer 6 (1), 103. doi:10.1186/s40425-018-0412-0
June, C. H., Warshauer, J. T., and Bluestone, J. A. (2017). Is autoimmunity the Achilles' heel of cancer immunotherapy? Nat. Med. 23 (5), 540–547. doi:10.1038/nm.4321
Kanjanapan, Y., Day, D., Butler, M. O., Wang, L., Joshua, A. M., Hogg, D., et al. (2019). Delayed immune-related adverse events in assessment for dose-limiting toxicity in early phase immunotherapy trials. Eur. J. Cancer 107, 1–7. doi:10.1016/j.ejca.2018.10.017
Khan, S., Khan, S. A., Luo, X., Fattah, F. J., Saltarski, J., Gloria-McCutchen, Y., et al. (2019a). Immune dysregulation in cancer patients developing immune-related adverse events. Br. J. Cancer 120 (1), 63–68. doi:10.1038/s41416-018-0155-1
Khan, Z., Hammer, C., Guardino, E., Chandler, G. S., and Albert, M. L. (2019b). Mechanisms of immune-related adverse events associated with immune checkpoint blockade: Using germline genetics to develop a personalized approach. Genome Med. 11 (1), 39. doi:10.1186/s13073-019-0652-8
Kim, S. T., Tayar, J., Trinh, V. A., Suarez-Almazor, M., Garcia, S., Hwu, P., et al. (2017). Successful treatment of arthritis induced by checkpoint inhibitors with tocilizumab: A case series. Ann. Rheum. Dis. 76 (12), 2061–2064. doi:10.1136/annrheumdis-2017-211560
Klocke, K., Sakaguchi, S., Holmdahl, R., and Wing, K. (2016). Induction of autoimmune disease by deletion of CTLA-4 in mice in adulthood. Proc. Natl. Acad. Sci. U. S. A. 113 (17), E2383–E2392. doi:10.1073/pnas.1603892113
Knochelmann, H. M., Dwyer, C. J., Bailey, S. R., Amaya, S. M., Elston, D. M., Mazza-McCrann, J. M., et al. (2018). When worlds collide: Th17 and Treg cells in cancer and autoimmunity. Cell. Mol. Immunol. 15 (5), 458–469. doi:10.1038/s41423-018-0004-4
Kumar, V., Chaudhary, N., Garg, M., Floudas, C. S., Soni, P., and Chandra, A. B. (2017). Current diagnosis and management of immune related adverse events (irAEs) induced by immune checkpoint inhibitor therapy. Front. Pharmacol. 8, 49. doi:10.3389/fphar.2017.00049
Labrijn, A. F., Janmaat, M. L., Reichert, J. M., and Parren, P. (2019). Bispecific antibodies: A mechanistic review of the pipeline. Nat. Rev. Drug Discov. 18 (8), 585–608. doi:10.1038/s41573-019-0028-1
Laurent, S., Queirolo, P., Boero, S., Salvi, S., Piccioli, P., Boccardo, S., et al. (2013). The engagement of CTLA-4 on primary melanoma cell lines induces antibody-dependent cellular cytotoxicity and TNF-α production. J. Transl. Med. 11, 108. doi:10.1186/1479-5876-11-108
Lebbé, C., Meyer, N., Mortier, L., Marquez-Rodas, I., Robert, C., Rutkowski, P., et al. (2019). Evaluation of two dosing regimens for nivolumab in combination with ipilimumab in patients with advanced melanoma: Results from the phase IIIb/IV CheckMate 511 trial. J. Clin. Oncol. 37 (11), 867–875. doi:10.1200/jco.18.01998
Lim, S. Y., Lee, J. H., Gide, T. N., Menzies, A. M., Guminski, A., Carlino, M. S., et al. (2019). Circulating cytokines predict immune-related toxicity in melanoma patients receiving anti-PD-1-based immunotherapy. Clin. Cancer Res. 25 (5), 1557–1563. doi:10.1158/1078-0432.ccr-18-2795
Liu, W., Chen, B., Liu, Y., Luo, Z., Sun, B., and Ma, F. (2021a). Durvalumab-induced demyelinating lesions in a patient with extensive-stage small-cell lung cancer: A case report. Front. Pharmacol. 12, 799728. doi:10.3389/fphar.2021.799728
Liu, W., Liu, Y., Ma, F., Sun, B., Wang, Y., Luo, J., et al. (2021b). Peripheral blood markers associated with immune-related adverse effects in patients who had advanced non-small cell lung cancer treated with PD-1 inhibitors. Cancer Manag. Res. 13, 765–771. doi:10.2147/cmar.s293200
Liu, W., Ma, F., Sun, B., Liu, Y., Tang, H., Luo, J., et al. (2021c). Intestinal microbiome associated with immune-related adverse events for patients treated with anti-PD-1 inhibitors, a real-world study. Front. Immunol. 12, 756872. doi:10.3389/fimmu.2021.756872
Lo, B., Fritz, J. M., Su, H. C., Uzel, G., Jordan, M. B., and Lenardo, M. J. (2016). CHAI and LATAIE: New genetic diseases of CTLA-4 checkpoint insufficiency. Blood 128 (8), 1037–1042. doi:10.1182/blood-2016-04-712612
Lu, C. W., Talukder, A., Savage, N. M., Singh, N., and Liu, K. (2017). JAK-STAT-mediated chronic inflammation impairs cytotoxic T lymphocyte activation to decrease anti-PD-1 immunotherapy efficacy in pancreatic cancer. Oncoimmunology 6 (3), e1291106. doi:10.1080/2162402X.2017.1291106
Luoma, A. M., Suo, S., Williams, H. L., Sharova, T., Sullivan, K., Manos, M., et al. (2020). Molecular pathways of colon inflammation induced by cancer immunotherapy. Cell. 182 (3), 655–671. doi:10.1016/j.cell.2020.06.001
Ma, K. S., Saeed, H. N., Chodosh, J., Wang, C. W., Chung, Y. C., Wei, L. C., et al. (2021). Ocular manifestations of anti-neoplastic immune checkpoint inhibitor-associated Stevens-Johnson syndrome/toxic epidermal necrolysis in cancer patients. Ocul. Surf. 22, 47–50. doi:10.1016/j.jtos.2021.06.010
Ma, V. T., Lao, C. D., Fecher, L. A., and Schiopu, E. (2022). Successful use of secukinumab in two melanoma patients with immune checkpoint inhibitor-induced inflammatory arthropathy. Immunotherapy 14 (8), 593–598. doi:10.2217/imt-2021-0274
Maher, V. E., Fernandes, L. L., Weinstock, C., Tang, S., Agarwal, S., Brave, M., et al. (2019). Analysis of the association between adverse events and outcome in patients receiving a programmed death protein 1 or programmed death ligand 1 antibody. J. Clin. Oncol. 37 (30), 2730–2737. doi:10.1200/jco.19.00318
Marrone, K. A., Ying, W., and Naidoo, J. (2016). Immune-related adverse events from immune checkpoint inhibitors. Clin. Pharmacol. Ther. 100 (3), 242–251. doi:10.1002/cpt.394
Maughan, B. L., Bailey, E., Gill, D. M., and Agarwal, N. (2017). Incidence of immune-related adverse events with program death receptor-1- and program death receptor-1 ligand-directed therapies in genitourinary cancers. Front. Oncol. 7, 56. doi:10.3389/fonc.2017.00056
Mazmanian, S. K., Liu, C. H., Tzianabos, A. O., and Kasper, D. L. (2005). An immunomodulatory molecule of symbiotic bacteria directs maturation of the host immune system. Cell. 122 (1), 107–118. doi:10.1016/j.cell.2005.05.007
McCulloch, J. A., Davar, D., Rodrigues, R. R., Badger, J. H., Fang, J. R., Cole, A. M., et al. (2022). Intestinal microbiota signatures of clinical response and immune-related adverse events in melanoma patients treated with anti-PD-1. Nat. Med. 28 (3), 545–556. doi:10.1038/s41591-022-01698-2
Michailidou, D., Khaki, A. R., Morelli, M. P., Diamantopoulos, L., Singh, N., and Grivas, P. (2021). Association of blood biomarkers and autoimmunity with immune related adverse events in patients with cancer treated with immune checkpoint inhibitors. Sci. Rep. 11 (1), 9029. doi:10.1038/s41598-021-88307-3
Michot, J. M., Bigenwald, C., Champiat, S., Collins, M., Carbonnel, F., Postel-Vinay, S., et al. (2016). Immune-related adverse events with immune checkpoint blockade: A comprehensive review. Eur. J. Cancer 54, 139–148. doi:10.1016/j.ejca.2015.11.016
Mooradian, M. J., Wang, D. Y., Coromilas, A., Lumish, M., Chen, T., Giobbie-Hurder, A., et al. (2020). Mucosal inflammation predicts response to systemic steroids in immune checkpoint inhibitor colitis. J. Immunother. Cancer 8 (1), e000451. doi:10.1136/jitc-2019-000451
Motzer, R. J., Rini, B. I., McDermott, D. F., Redman, B. G., Kuzel, T. M., Harrison, M. R., et al. (2015). Nivolumab for metastatic renal cell carcinoma: Results of a randomized phase II trial. J. Clin. Oncol. 33 (13), 1430–1437. doi:10.1200/jco.2014.59.0703
National Cancer Institute (2019). Common terminology criteria for adverse events (CTCAE). NCI. Available at: https://ctep.cancer.gov/protocolDevelopment/electronic_applications/ctc.htm.
NCCN (2021). Management of immunotherapy-related toxicities. (Version03.2021). Available at: https://www.nccn.org/professionals/physician_gls/pdf/immunotherapy.pdf.
Noack, M., and Miossec, P. (2014). Th17 and regulatory T cell balance in autoimmune and inflammatory diseases. Autoimmun. Rev. 13 (6), 668–677. doi:10.1016/j.autrev.2013.12.004
Ocana, A., Nieto-Jiménez, C., Pandiella, A., and Templeton, A. J. (2017). Neutrophils in cancer: Prognostic role and therapeutic strategies. Mol. Cancer 16 (1), 137. doi:10.1186/s12943-017-0707-7
Parakh, S., Cebon, J., and Klein, O. (2018). Delayed autoimmune toxicity occurring several months after cessation of anti-PD-1 therapy. Oncologist 23 (7), 849–851. doi:10.1634/theoncologist.2017-0531
Patel, S. A., and Gooderham, N. J. (2015). IL6 mediates immune and colorectal cancer cell cross-talk via miR-21 and miR-29b. Mol. Cancer Res. 13 (11), 1502–1508. doi:10.1158/1541-7786.mcr-15-0147
Patrinely, J. R., Johnson, R., Lawless, A. R., Bhave, P., Sawyers, A., Dimitrova, M., et al. (2021). Chronic immune-related adverse events following adjuvant anti-PD-1 therapy for high-risk resected melanoma. JAMA Oncol. 7 (5), 744–748. doi:10.1001/jamaoncol.2021.0051
Pauken, K. E., Dougan, M., Rose, N. R., Lichtman, A. H., and Sharpe, A. H. (2019). Adverse events following cancer immunotherapy: Obstacles and opportunities. Trends Immunol. 40 (6), 511–523. doi:10.1016/j.it.2019.04.002
Peng, W., Liu, C., Xu, C., Lou, Y., Chen, J., Yang, Y., et al. (2012). PD-1 blockade enhances T-cell migration to tumors by elevating IFN-γ inducible chemokines. Cancer Res. 72 (20), 5209–5218. doi:10.1158/0008-5472.can-12-1187
Perez-Ruiz, E., Minute, L., Otano, I., Alvarez, M., Ochoa, M. C., Belsue, V., et al. (2019). Prophylactic TNF blockade uncouples efficacy and toxicity in dual CTLA-4 and PD-1 immunotherapy. Nature 569 (7756), 428–432. doi:10.1038/s41586-019-1162-y
Petersone, L., Edner, N. M., Ovcinnikovs, V., Heuts, F., Ross, E. M., Ntavli, E., et al. (2018). T cell/B cell collaboration and autoimmunity: An intimate relationship. Front. Immunol. 9, 1941. doi:10.3389/fimmu.2018.01941
Pham, F., Moinard-Butot, F., Coutzac, C., and Chaput, N. (2021). Cancer and immunotherapy: A role for microbiota composition. Eur. J. Cancer 155, 145–154. doi:10.1016/j.ejca.2021.06.051
Phillips, G. S., Wu, J., Hellmann, M. D., Postow, M. A., Rizvi, N. A., Freites-Martinez, A., et al. (2019). Treatment outcomes of immune-related cutaneous adverse events. J. Clin. Oncol. 37 (30), 2746–2758. doi:10.1200/jco.18.02141
Postow, M. A., Sidlow, R., and Hellmann, M. D. (2018). Immune-related adverse events associated with immune checkpoint blockade. N. Engl. J. Med. 378 (2), 158–168. doi:10.1056/NEJMra1703481
Queirolo, P., Dozin, B., Morabito, A., Banelli, B., Carosio, R., Fontana, V., et al. (2018). CTLA-4 gene variant -1661A>G may predict the onset of endocrine adverse events in metastatic melanoma patients treated with ipilimumab. Eur. J. Cancer 97, 59–61. doi:10.1016/j.ejca.2018.04.005
Reuben, J. M., Lee, B. N., Li, C., Gomez-Navarro, J., Bozon, V. A., Parker, C. A., et al. (2006). Biologic and immunomodulatory events after CTLA-4 blockade with ticilimumab in patients with advanced malignant melanoma. Cancer 106 (11), 2437–2444. doi:10.1002/cncr.21854
Robert, C. (2020). A decade of immune-checkpoint inhibitors in cancer therapy. Nat. Commun. 11 (1), 3801. doi:10.1038/s41467-020-17670-y
Roth, I., Campbell, H., Rubio, C., Vennin, C., Wilson, M., Wiles, A., et al. (2016). The Δ133p53 isoform and its mouse analogue Δ122p53 promote invasion and metastasis involving pro-inflammatory molecules interleukin-6 and CCL2. Oncogene 35 (38), 4981–4989. doi:10.1038/onc.2016.45
Sadik, C. D., Langan, E. A., Grätz, V., Zillikens, D., and Terheyden, P. (2019). Checkpoint inhibition may trigger the rare variant of anti-LAD-1 IgG-positive, anti-bp180 NC16A IgG-negative bullous pemphigoid. Front. Immunol. 10, 1934. doi:10.3389/fimmu.2019.01934
Salem, J. E., Allenbach, Y., Vozy, A., Brechot, N., Johnson, D. B., Moslehi, J. J., et al. (2019). Abatacept for severe immune checkpoint inhibitor-associated myocarditis. N. Engl. J. Med. 380 (24), 2377–2379. doi:10.1056/NEJMc1901677
Sandigursky, S., and Mor, A. (2018). Immune-related adverse events in cancer patients treated with immune checkpoint inhibitors. Curr. Rheumatol. Rep. 20 (10), 65. doi:10.1007/s11926-018-0770-0
Schwab, I., and Nimmerjahn, F. (2013). Intravenous immunoglobulin therapy: How does IgG modulate the immune system? Nat. Rev. Immunol. 13 (3), 176–189. doi:10.1038/nri3401
Seidel, J. A., Otsuka, A., and Kabashima, K. (2018). Anti-PD-1 and anti-CTLA-4 therapies in cancer: Mechanisms of action, efficacy, and limitations. Front. Oncol. 8, 86. doi:10.3389/fonc.2018.00086
Shankar, B., Zhang, J., Naqash, A. R., Forde, P. M., Feliciano, J. L., Marrone, K. A., et al. (2020). Multisystem immune-related adverse events associated with immune checkpoint inhibitors for treatment of non-small cell lung cancer. JAMA Oncol. 6 (12), 1952–1956. doi:10.1001/jamaoncol.2020.5012
Shultz, L. D., Keck, J., Burzenski, L., Jangalwe, S., Vaidya, S., Greiner, D. L., et al. (2019). Humanized mouse models of immunological diseases and precision medicine. Mamm. Genome 30 (5-6), 123–142. doi:10.1007/s00335-019-09796-2
Siakavellas, S. I., and Bamias, G. (2018). Checkpoint inhibitor colitis: A new model of inflammatory bowel disease? Curr. Opin. Gastroenterol. 34 (6), 377–383. doi:10.1097/mog.0000000000000482
Sokol, H., Pigneur, B., Watterlot, L., Lakhdari, O., Bermúdez-Humarán, L. G., Gratadoux, J. J., et al. (2008). Faecalibacterium prausnitzii is an anti-inflammatory commensal bacterium identified by gut microbiota analysis of Crohn disease patients. Proc. Natl. Acad. Sci. U. S. A. 105 (43), 16731–16736. doi:10.1073/pnas.0804812105
Spain, L., Walls, G., Julve, M., O'Meara, K., Schmid, T., Kalaitzaki, E., et al. (2017). Neurotoxicity from immune-checkpoint inhibition in the treatment of melanoma: A single centre experience and review of the literature. Ann. Oncol. 28 (2), 377–385. doi:10.1093/annonc/mdw558
Stamatouli, A,M., Quandt, Z., Perdigoto, A. L., Clark, P. L., Kluger, H., Weiss, S. A., et al. (2018). Collateral damage: Insulin-dependent diabetes induced with checkpoint inhibitors. Diabetes 67 (8), 1471–1480. doi:10.2337/dbi18-0002
Stroud, C. R., Hegde, A., Cherry, C., Naqash, A. R., Sharma, N., Addepalli, S., et al. (2019). Tocilizumab for the management of immune mediated adverse events secondary to PD-1 blockade. J. Oncol. Pharm. Pract. 25 (3), 551–557. doi:10.1177/1078155217745144
Sun, J., Schiffman, J., Raghunath, A., Ng Tang, D., Chen, H., and Sharma, P. (2008). Concurrent decrease in IL-10 with development of immune-related adverse events in a patient treated with anti-CTLA-4 therapy. Cancer Immun. 8, 9.
Suresh, K., and Naidoo, J. (2020). Lower survival in patients who develop pneumonitis following immunotherapy for lung cancer. Clin. Lung Cancer 21 (3), e169–e170. doi:10.1016/j.cllc.2019.10.009
Takahashi, Y., Nagaya, T., Iwaya, Y., Okamura, T., Hirayama, A., Iwaya, M., et al. (2023). CD8+ lymphocyte infiltration is a specific feature of colitis induced by immune checkpoint inhibitors. Dig. Dis. Sci. 68 (2), 451–459. doi:10.1007/s10620-022-07598-2
Tanaka, R., Ichimura, Y., Kubota, N., Saito, A., Nakamur, Y., Ishitsuka, Y., et al. (2020). Activation of CD8 T cells accelerates anti-PD-1 antibody-induced psoriasis-like dermatitis through IL-6. Commun. Biol. 3 (1), 571. doi:10.1038/s42003-020-01308-2
Tanaka, R., Okiyama, N., Okune, M., Ishitsuka, Y., Watanabe, R., Furuta, J., et al. (2017). Serum level of interleukin-6 is increased in nivolumab-associated psoriasiform dermatitis and tumor necrosis factor-α is a biomarker of nivolumab recativity. J. Dermatol Sci. 86 (1), 71–73. doi:10.1016/j.jdermsci.2016.12.019
Tarhini, A. A., Zahoor, H., Lin, Y., Malhotra, U., Sander, C., Butterfield, L. H., et al. (2015). Baseline circulating IL-17 predicts toxicity while TGF-β1 and IL-10 are prognostic of relapse in ipilimumab neoadjuvant therapy of melanoma. J. Immunother. Cancer 3, 39. doi:10.1186/s40425-015-0081-1
Thompson, J. A., Schneider, B. J., Brahmer, J., Andrews, S., Armand, P., Bhatia, S., et al. (2019). Management of immunotherapy-related toxicities, version 1.2019. J. Natl. Compr. Canc Netw. 17 (3), 255–289. doi:10.6004/jnccn.2019.0013
Toi, Y., Sugawara, S., Sugisaka, J., Ono, H., Kawashima, Y., Aiba, T., et al. (2019). Profiling preexisting antibodies in patients treated with anti-PD-1 therapy for advanced non-small cell lung cancer. JAMA Oncol. 5 (3), 376–383. doi:10.1001/jamaoncol.2018.5860
Topalian, S. L., Hodi, F. S., Brahmer, J. R., Gettinger, S. N., Smith, D. C., McDermott, D. F., et al. (2012). Safety, activity, and immune correlates of anti-PD-1 antibody in cancer. N. Engl. J. Med. 366 (26), 2443–2454. doi:10.1056/NEJMoa1200690
Touat, M., Talmasov, D., Ricard, D., and Psimaras, D. (2017). Neurological toxicities associated with immune-checkpoint inhibitors. Curr. Opin. Neurol. 30 (6), 659–668. doi:10.1097/wco.0000000000000503
Triggianese, P., Novelli, L., Galdiero, M. R., Chimenti, M. S., Conigliaro, P., Perricone, R., et al. (2020). Immune checkpoint inhibitors-induced autoimmunity: The impact of gender. Autoimmun. Rev. 19 (8), 102590. doi:10.1016/j.autrev.2020.102590
Valpione, S., Pasquali, S., Campana, L. G., Piccin, L., Mocellin, S., Pigozzo, J., et al. (2018). Sex and interleukin-6 are prognostic factors for autoimmune toxicity following treatment with anti-CTLA4 blockade. J. Transl. Med. 16 (1), 94. doi:10.1186/s12967-018-1467-x
Verma, N., Jaffer, M., Pina, Y., Peguero, E., and Mokhtari, P. (2021). Rituximab for immune checkpoint inhibitor myasthenia gravis. Cureus 13 (7), e16337. doi:10.7759/cureus.16337
Vesely, M. D., and Chen, L. (2020). Normalization cancer immunotherapy for melanoma. J. Invest. Dermatol 140 (6), 1134–1142. doi:10.1016/j.jid.2020.02.005
Wang, D. Y., Salem, J. E., Cohen, J. V., Chandra, S., Menzer, C., Ye, F., et al. (2018a). Fatal toxic effects associated with immune checkpoint inhibitors: A systematic review and meta-analysis. JAMA Oncol. 4 (12), 1721–1728. doi:10.1001/jamaoncol.2018.3923
Wang, Y., Wiesnoski, D. H., Helmink, B. A., Gopalakrishnan, V., Choi, K., DuPont, H. L., et al. (2018b). Fecal microbiota transplantation for refractory immune checkpoint inhibitor-associated colitis. Nat. Med. 24 (12), 1804–1808. doi:10.1038/s41591-018-0238-9
Wang, Y., Zhou, S., Yang, F., Qi, X., Wang, X., Guan, X., et al. (2019). Treatment-related adverse events of PD-1 and PD-L1 inhibitors in clinical trials: A systematic review and meta-analysis. JAMA Oncol. 5 (7), 1008–1019. doi:10.1001/jamaoncol.2019.0393
Watanabe, H., Kawaguchi, M., Fujishima, S., Ogura, M., Matsukura, S., Takeuchi, H., et al. (2009). Functional characterization of IL-17F as a selective neutrophil attractant in psoriasis. J. Invest. Dermatol 129 (3), 650–656. doi:10.1038/jid.2008.294
Weber, J. S., D'Angelo, S. P., Minor, D., Hodi, F. S., Gutzmer, R., Neyns, B., et al. (2015). Nivolumab versus chemotherapy in patients with advanced melanoma who progressed after anti-CTLA-4 treatment (CheckMate 037): A randomised, controlled, open-label, phase 3 trial. Lancet Oncol. 16 (4), 375–384. doi:10.1016/s1470-2045(15)70076-8
Weber, J. S., Kähler, K. C., and Hauschild, A. (2012). Management of immune-related adverse events and kinetics of response with ipilimumab. J. Clin. Oncol. 30 (21), 2691–2697. doi:10.1200/jco.2012.41.6750
Wing, K., Onishi, Y., Prieto-Martin, P., Yamaguchi, T., Miyara, M., Fehervari, Z., et al. (2008). CTLA-4 control over Foxp3+ regulatory T cell function. Science 322 (5899), 271–275. doi:10.1126/science.1160062
Wolchok, J. D., Neyns, B., Linette, G., Negrier, S., Lutzky, J., Thomas, L., et al. (2010). Ipilimumab monotherapy in patients with pretreated advanced melanoma: A randomised, double-blind, multicentre, phase 2, dose-ranging study. Lancet Oncol. 11 (2), 155–164. doi:10.1016/s1470-2045(09)70334-1
Yeung, C., Kartolo, A., Holstead, R., Moffat, G. T., Hanna, L., Hopman, W., et al. (2021). Safety and clinical outcomes of immune checkpoint inhibitors in patients with cancer and preexisting autoimmune diseases. J. Immunother. 44 (9), 362–370. doi:10.1097/cji.0000000000000377
Yoest, J. M. (2017). Clinical features, predictive correlates, and pathophysiology of immune-related adverse events in immune checkpoint inhibitor treatments in cancer: A short review. Immunotargets Ther. 6, 73–82. doi:10.2147/itt.s126227
Zhu, J., Chen, G., He, Z., Zheng, Y., Gao, S., Li, J., et al. (2021). Stevens-johnson syndrome/toxic epidermal necrolysis in patients treated with immune checkpoint inhibitors: A safety analysis of clinical trials and fda pharmacovigilance database. EClinicalMedicine 37, 100951. doi:10.1016/j.eclinm.2021.100951
Keywords: immune checkpoint inhibitors, immune-related adverse events, clinical features, risk factors, mechanism-based strategies
Citation: Liu W, Luo Z, Liu Y and Sun B (2023) Current landscape and tailored management of immune-related adverse events. Front. Pharmacol. 14:1078338. doi: 10.3389/fphar.2023.1078338
Received: 24 October 2022; Accepted: 24 February 2023;
Published: 06 March 2023.
Edited by:
Yuhong Liu, Guangzhou University of Chinese Medicine, ChinaReviewed by:
Utpreksha Vaish, University of Alabama at Birmingham, United StatesCopyright © 2023 Liu, Luo, Liu and Sun. This is an open-access article distributed under the terms of the Creative Commons Attribution License (CC BY). The use, distribution or reproduction in other forums is permitted, provided the original author(s) and the copyright owner(s) are credited and that the original publication in this journal is cited, in accordance with accepted academic practice. No use, distribution or reproduction is permitted which does not comply with these terms.
*Correspondence: Bao Sun, c2N5X2NzdTIwMTZAY3N1LmVkdS5jbg==
Disclaimer: All claims expressed in this article are solely those of the authors and do not necessarily represent those of their affiliated organizations, or those of the publisher, the editors and the reviewers. Any product that may be evaluated in this article or claim that may be made by its manufacturer is not guaranteed or endorsed by the publisher.
Research integrity at Frontiers
Learn more about the work of our research integrity team to safeguard the quality of each article we publish.