- 1Department of Endocrinology, The First People’s Hospital of Changzhou, Changzhou, China
- 2Health Management Center, The First Affiliated Hospital of Soochow University, Suzhou, China
- 3Department of Neurosurgery, The First People’s Hospital of Changzhou, Changzhou, China
- 4Clinical Medical Research Center, The First People’s Hospital of Changzhou, Changzhou, China
- 5Department of Oncology, The First People’s Hospital of Changzhou, Changzhou, China
- 6LipidALL Technologies Company Limited, Changzhou, China
Introduction: We aimed to evaluate the influence of 1,25-dihydroxyvitamin D (1,25(OH)2D) on metabolic dysfunction and elucidate its underlying mechanism using a rat model of polycystic ovary syndrome (PCOS).
Methods: Twenty-four Sprague-Dawley rats were randomly divided into four groups: control group (CON, 2 ml/kg of oral 0.5% CMC), 1,25VD group (oral 0.5% CMC and 2.5 ug/kg intraperitoneal 1,25(OH)2D), PCOS group (1 mg/kg oral letrozole), PCOS+1,25VD group (1 mg/kg oral letrozole orally 2.5 ug/kg intraperitoneal 1,25(OH)2D). The treatments were administered for 8 weeks. Body weight, estrus cycle, insulin tolerance, and oral glucose tolerance of the rats in the different groups were assessed. The rats were euthanized at the 8th weeks, and plasma, ovarian, and liver samples were collected and analyzed. The hepatic lipid profile was characterized using HPLC/MRM.
Results: Letrozole-induced PCOS rats exhibited increased weight, insulin resistance, postprandial glucose abnormalities, and dyslipidemia. Compared with the PCOS group rats, the PCOS+1,25VD group rats showed reduced body weight, increased sensitivity to insulin, decreased postprandial glucose, and elevated levels of high-density lipoprotein cholesterol. Moreover, abnormally increased liver concentrations of total diacylglycerol (DG) and DG species in the PCOS rats were reversed by treatment with 1,25(OH)2D. Additionally, hepatic DG and insulin sensitivity were correlated.
Conclusion: 1,25(OH)2D inhibited hepatic DG accumulation and ameliorated metabolic dysfunction in PCOS rat models.
1 Introduction
Polycystic ovary syndrome (PCOS) is a commonly diagnosed endocrine disease, with a prevalence of PCOS approximately 4%–20% worldwide (Bozdag et al., 2016; Skiba et al., 2018). Hyperandrogenism, oligomenorrhea, and polycystic ovarian morphology are the most common clinical characteristics of PCOS (Bozdag et al., 2016; Skiba et al., 2018). PCOS may also be accompanied by other metabolic dysfunctions, including obesity, insulin resistance, and dyslipidemia (EA-SPcwg, 2004). The risk of developing cardiovascular disorders, type 2 diabetes, non-alcoholic fatty liver disease and endometrial carcinoma is more in women with PCOS than in healthy subjects (Barry et al., 2014; Rocha et al., 2017; Osibogun et al., 2020). The pathogenesis of PCOS is yet to be fully elucidated, and therefore, there is no effective cure. Current therapies have been designed to ameliorate heterogeneous clinical symptoms (Goodarzi et al., 2011; Walters et al., 2018). Hence, the development of novel and effective anti-PCOS therapies is in high demand.
Vitamin D deficiency is a global health concern (van Schoor and Lips, 2017). Vitamin D functions as a key regulator in maintaining calcium homeostasis and bone development, and accumulating evidence suggests that it also has wide extra-skeletal functions. Decreased vitamin D levels are reportedly associated with multiple disorders such as autoimmune diseases, cancers, cardiovascular diseases, and type 2 diabetes (Bouillon et al., 2008). Vitamin D deficiency is also common in PCOS and may be associated with the development of several of its clinical features (Trummer et al., 2018; Eftekhar et al., 2020). However, the role of vitamin D deficiency in the progression of PCOS needs to be further explored. Existing studies have produced contradictory results on the application of vitamin D as a supplement in treating PCOS (Plum and DeLuca, 2010; Pergialiotis et al., 2017). Therefore, it remains unclear whether vitamin D supplementation could play a positive role in improving the therapeutic efficacy of current anti-PCOS therapies.
Exposure to letrozole, an aromatase inhibitor, reportedly prevents the conversion of testosterone to estradiol, which further elevates the serum androgen levels and leads to polycystic ovarian morphology and other metabolic disorders in a rat model (Stener-Victorin et al., 2020). 1,25-dihydroxyvitamin D [1,25(OH) 2D], an active form of vitamin D, exerts its biological function by interacting with vitamin D receptors (Plum and DeLuca, 2010). Herein, we aimed to assess whether 1,25(OH)2D exerted beneficial effects on metabolic dysfunction in a letrozole-induced PCOS rat model. We also evaluated the hepatic lipid content of diacylglycerol (DG) and triacylglycerol (TG) using a lipidomics strategy. The results of the current study may provide novel evidence for the application of vitamin D supplements as a cost-effective therapeutic strategy in PCOS management.
2 Material and methods
2.1 Animals and treatments
Twenty-four (4-5-week-old) rats, weighing 78–97 g, were purchased from the Shanghai Family Planning Research Institute, Shanghai, China. The rats (six per cage) were housed under the following conditions: 12 h light/dark cycle, 21°C–22°C, 50%–60% humidity, and free access to food and distilled water. The Soochow University Animal Care and Use Committee (Suzhou, China) approved this study (No: Date of approval). The rats were divided into the following groups: 1) control group (CON, N = 6) where rats were intragastrically administered 0.5% carboxymethyl cellulose (CMC) at a daily dose of 2 ml/kg; 2) 1,25(OH)2D supplemental group (1,25VD, N = 6) where rats received 0.5% CMC gavage daily and an intraperitoneal (i.p.) injection of 2.5ug/kg of 1,25(OH)2D (Sigma Aldrich, St. Louis, MO, United States) dissolved in a 0.5% ethanol and 95.5% culture medium solution every 2 days (Yin et al., 2012); 3) Letrozole group (PCOS, N = 6) where rats were intragastrically administered 1 mg/kg of letrozole (Hengrui Medicine Co., Jiangsu, China) dissolved in 0.5% CMC solution daily and i. p. injection of the vehicle every 2 days; and 4) Letrozole + 1,25(OH)2D supplemental group (PCOS+1,25VD, N = 6) where rats received 1 mg/kg of letrozole gavage and 2.5 ug/kg of i.p. 1,25(OH) 2D injection every 2 days.
The treatments were administered for 8 weeks. Rats were weighed twice per week. Vaginal smears were obtained daily during the 8th weeks to validate the induction of PCOS models. Insulin tolerance and oral glucose tolerance tests were completed on separate days of the 8th weeks. After the 8th weeks, the animals kept fasting overnight. Subsequently, they were anesthetized with chloral hydrate (3 ml/kg intraperitoneal). The blood samples were obtained from the abdominal aorta and centrifuged at 4500 rpm for 10 min at 4°C to collect the serum. Bilateral ovary and liver samples were dissected and weighed. One ovary was treated with formalin (10% solution) to determine morphological changes; the other ovary was stored at −80°C for the lipidomics analysis (Sun et al., 2017) (Figure 1). The present study has been approved by Soochow University Animal Care and Use Committee (NO.20210907).
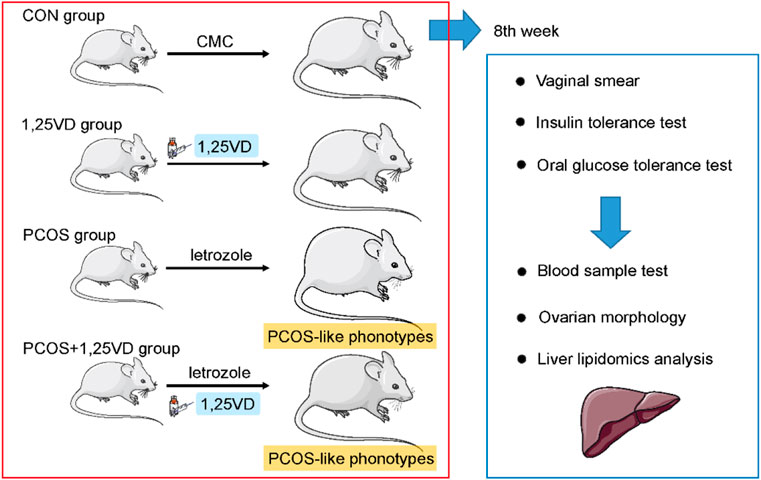
FIGURE 1. Schematic diagram of the animal study design. Rats were randomly assigned to the 1,25-dihydroxyvitamin D3 supplementation or vehicle group. Vaginal smear, insulin tolerance test and oral glucose tolerance test were conducted during the 8th weeks. Serum samples were collected for the evaluation of the hormone and lipid levels. Ovarian morphology was examined to verify the establishment of PCOS in the models. Hepatic samples were subjected to lipidomic profiling.
2.2 Insulin tolerance test
The rats were kept fasting overnight. Thereafter, the rats were intraperitoneally administered insulin (1 IU/kg). Blood samples were collected from the tail vein. Blood glucose levels at 0, 15, 30, 60, and 90 min after insulin administration were measured using a blood glucometer (ASCENSIA Diabetes Care).
2.3 Oral glucose tolerance test
The rats were intragastrically administered glucose (2 g/kg) after overnight fasting. Blood samples were collected from the tail vein. Blood glucose levels at 0, 15, 30, 60, 90, and 120 min after glucose administration were measured using a blood glucometer (ASCENSIA diabetes care).
2.4 Blood samples tests
Serum samples were collected and enzyme-linked immunosorbent assay (ELISA) kits (Sangon Biotech) were used to analyze testosterone, luteinizing hormone, and follicle-stimulating hormone levels. Serum 25-hydroxyvitamin D [25(OH)D] levels were measured using radioimmunoassay (Roche Diagnostics). Serum triglyceride, total cholesterol, high-density lipoprotein cholesterol (HDL-c), and low-density lipoprotein cholesterol (LDL-c) levels were analyzed using an automatic biochemical analyzer (Beckman Coulter AU5800).
2.5 Histology study
Histological studies were performed as previously described (Choubey et al., 2019). Ovarian samples were fixed using formalin, embedded in paraffin, and stained with hematoxylin-eosin (H&E) solution. Vaginal smears were stained with Giemsa solution (Upadhyay et al., 2021). A sterile, saline-soaked cotton swab was inserted daily into the rat’s vagina at 8:00 a.m., gently rotated clockwise, and then smeared onto a clean slide. The smear was allowed to dry and stained with Giemsa solution for 15 min. The type and proportion of cells observed under the light lens were used to determine the stage of the estrous cycle in the rats as follows: 1) Diestrus: Leukocyte predominance with occasional nucleated epithelial cells; 2) Pre-estrus: predominance of oval-shaped nucleated dermal cells with occasional leukocytes and non-nucleated keratinized epithelial cells; 3) Estrous: Predominance of lamellar, non-nucleated, keratinized epithelial cells and a few nucleated epithelial cells and leukocytes; and 4) Metestrus: Nucleated keratinized epithelial cells and leukocytes with a few nucleated epithelial cells.
2.6 Isolation of the liver lipids
The lipids were isolated from 30 mg of rat liver using the modified Bligh and Dyer’s method (Lam et al., 2016). Liver samples were homogenized using 750 µl of chloroform, methanol, and MilliQ H2O solution (3:6:1). The homogenates were centrifuged for 60 min (1500 rpm at 4°C). Thereafter, thee samples were treated with deionized H2O (350 µl) and chloroform (250 µl). Subsequently, the samples were centrifuged for 5 min (12000 rpm at 4°C). After centrifugation, the lower phase was transferred to a new tube. Chloroform (500 µl) was added to the aqueous phase to extract the remaining lipids. Finally, the lipids were collected, dried using SpeedVac, and stored at −80°C until needed.
2.7 Lipidomics
All lipidomic analyses were performed at LipidALL Technologies (Changzhou, China) using an Agilent 1290 II UPLC coupled with a Sciex QTRAP 6500 PLUS (Lam et al., 2021). Individual lipid species were quantified by referencing spiked internal standards. d9-PC32:0 (16:0/16:0), d9-PC36:1p (18:0p/18:1), d7-LPC18:1, d7-PE33:1 (15:0/18:1), d9-PE36:1p (18:0p/18:1), d7-LPE18:1, d31-PS, C17-LPS, d7-PA33:1 (15:0/18:1), C17 LPA, d7-PG33:1 (15:0/18:1), C17:1-LPG, d7-PI33:1 (15:0/18:1), C17-LPI, d5-CL72:8 (18:2)4, C17-SL and C14-BMP were purchased from Avanti Polar Lipids (AL, United States). GM3-d18:1/18:0-d3 cells were obtained from Matreya LLC (PA, United States). A modified version of the reverse-phase HPLC/MRM was used for the quantification of glycerol lipids (DG and TG). A 2.6 µm Phenomenex Kinetex-C18 column (i.d. 4.6 × 100 mm) was applied for 17 min (flow rate: 170 µl) to separate the neutral lipids using an isocratic mobile phase (including chloroform:methanol:0.1 M ammonium acetate in the ratio of 100:100:4 [v/v/v]). Short, medium, and long-chain TGs were quantified using TG (14:0)3-d5, TG (16:0)3-d5, and TG (18:0)3-d5 (CDN isotopes). DGs were determined using the internal standards d5-DG17:0/17:0 and d5-DG18:1/18:1 (Avanti Polar Lipids). Free fatty acids were determined using the internal standards d31-16:0 (Sigma-Aldrich) and d8-20:4 (Cayman Chemicals).
2.8 Statistical analysis
All data are expressed as mean ± standard deviation. Statistical analyses were performed using SPSS (version 26.0; SPSS Inc.). Comparisons between two groups were performed using Student’s t-test. Comparisons among multiple groups were performed using analysis of variance (ANOVA) with Tukey’s post hoc tests. Correlation analysis was performed using Pearson’s correlation coefficient. Statistical significance was set as p < 0.05.
3 Results
3.1 Establishment of letrozole-induced PCOS rat models
First, a histological analysis was performed to characterize the letrozole-induced PCOS rat model. Polycystic ovarian morphology was observed in letrozole-treated rats, with few normal follicles at different developmental stages and corpus luteum due to abnormal follicle development and anovulation (Figure 2A). Furthermore, as shown in Table 1, the numbers of primordial/primary follicles, secondary follicles, tertiary follicle, cysts were all decreased and the number of cystic follicles was markedly increased in PCOS group, suggesting that the PCOS model has been successfully established. The vaginal smear analysis proved that letrozole-treated rats tended to stay in the diestrus phase and had no estrus cycle (Figure 2B). Moreover, compared with the control group, letrozole treatment led to a nearly two-fold increase in the serum testosterone levels and an elevated LH/FSH ratio. Meanwhile, 1,25(OH)2D treatment had no significant effect on testosterone and the LH/FSH ratio in the PCOS rats (Figures 2C, D). Furthermore, the PCOS group showed reduced serum 25(OH)D levels compared with the CON group (Figure 2E). Furthermore, the levels of estradiol and progesterone were all decreased in PCOS group and increased by 1,25(OH)2D treatment (Figures 2F, G).
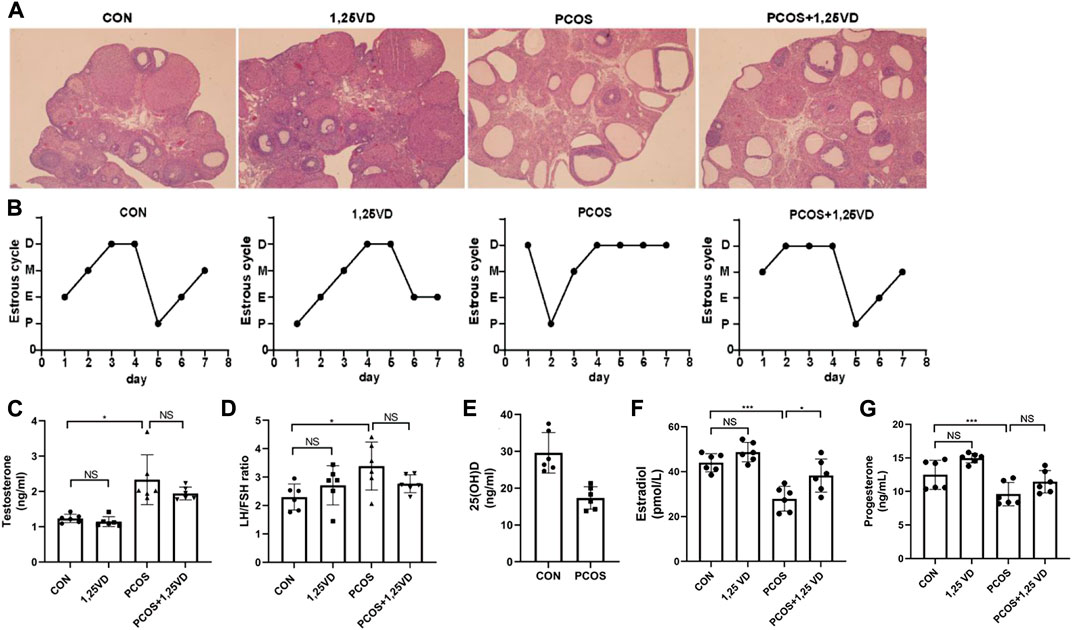
FIGURE 2. Ovarian morphology (A), estrus cycle (B), serum testosterone levels (C), LH/FSH ratio (D), 25(OH)D (E), estradiol (F) and progesterone (G) of the different groups. D: Diestrus, M: Metaoestrus, E: Estrus, P: Proestrus, LH: Luteinizing hormone, FSH: Follicle stimulating hormone. *p < 0.05, **p < 0.01, ***p < 0.001.
3.2 Supplementation of exogenous 1,25(OH)2D3 prevents weight gain, insulin resistance and HDL-c decrease in PCOS rats
At the beginning of the study, there were no significant differences in the body weight among the four groups. After 8 weeks of treatment, the weight of the rats in the PCOS group was significantly higher than that of the control rats (Figure 3A); However, treatment with 1,25(OH)2D significantly decreased the body weight of PCOS rats (Figure 3A). During the insulin tolerance test (ITT), there was a significant increase in glucose levels in PCOS rats compared with the control rats after 15, 30, and 60 min, whereas, the PCOS+1,25VD group showed reduced glucose levels after 30 and 60 min. The area under the curve (AUC) exhibited the same trend. Insulin resistance was observed in the PCOS group, whereas 1,25(OH)2D increased insulin sensitivity (Figures 3B, C). During the oral glucose tolerance test (OGTT), glucose levels decreased in PCOS rats at 15 min and were elevated at 60 min compared to the control rats. The glucose level in the PCOS + 1,25VD group was higher at 15 min than that in the PCOS group; Thereafter, the glucose levels decreased significantly after 60 min and 90 min (Figure 3D). The AUC, circulating TGs, and total cholesterol showed no significant differences among the four groups (Figures 3E–G). The circulating HDL-c levels decreased, while the circulating LDL-c levels increased in the PCOS group. Conversely, the circulating HDL-c levels increased in the PCOS+1,25VD group (Figures 3H, I).
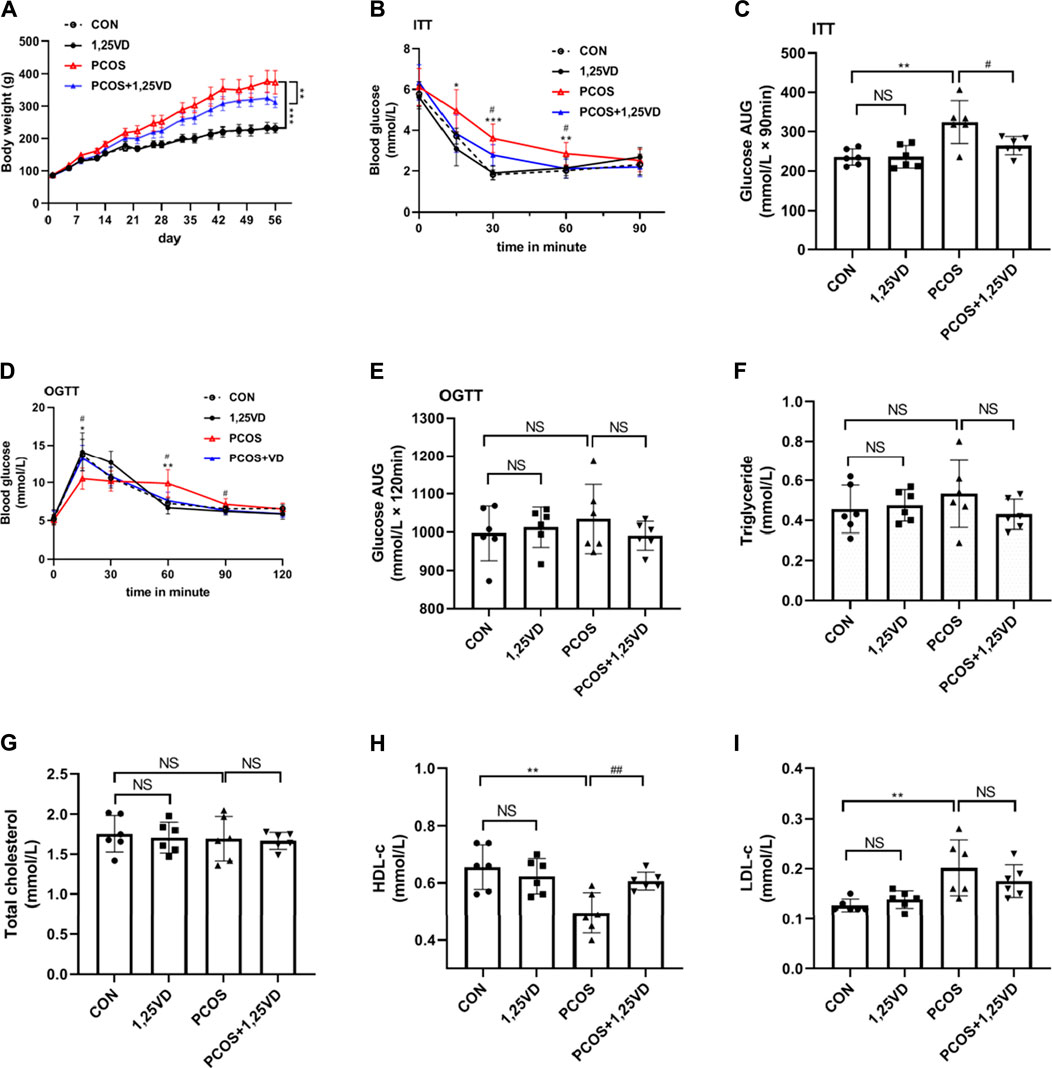
FIGURE 3. Comparison of the body weight (A), ITT (B), area under the curve (AUC) for glucose in ITT (C), OGTT (D), AUC for glucose in OGTT (E), serum triglyceride levels (F), total cholesterol levels (G), HDL-c levels (H) and LDL-c levels (I) among the study groups. *: PCOS vs. CON p < 0.05, **: PCOS vs. CON p < 0.01, ***: PCOS vs. CON p < 0.001, #: PCOS+1,25VD vs. PCOS p < 0.05, ##: PCOS+1,25VD vs. PCOS p < 0.01. HDL-c: High density lipoprotein cholesterol, LDL-c: Low density lipoprotein cholesterol, ITT, OGTT.
3.3 1,25(OH)2D3 reduces hepatic DG content in PCOS rats
After 8 weeks of treatment, the liver weight of the rats in the PCOS group was significantly higher than that of the control rats (Figure 4A), whereas the liver/body weight ratio in the PCOS group was lower than that in the control group (Figure 4B). Liver weight and liver/body weight ratio showed no significant differences between the PCOS and PCOS+1,25VD groups (Figures 4A, B). Given the importance of the liver in insulin metabolism, we further analyzed the hepatic lipid profiles of different groups to investigate whether lipid molecules are responsible for the effects of 1,25(OH)2D3 supplementation. At the 8th weeks, the total content of cardiolipins (CL), phosphatidylethanolamines (PE), and DGs was remarkably higher in the PCOS group than in the CON group. In contrast, the contents of phosphatidylglycerols (PG) and lysophosphatidylserines (LPS) decreased in the PCOS group. Supplementation with 1,25(OH)2D in PCOS rats decreased the DG content (Figures 4C, D).
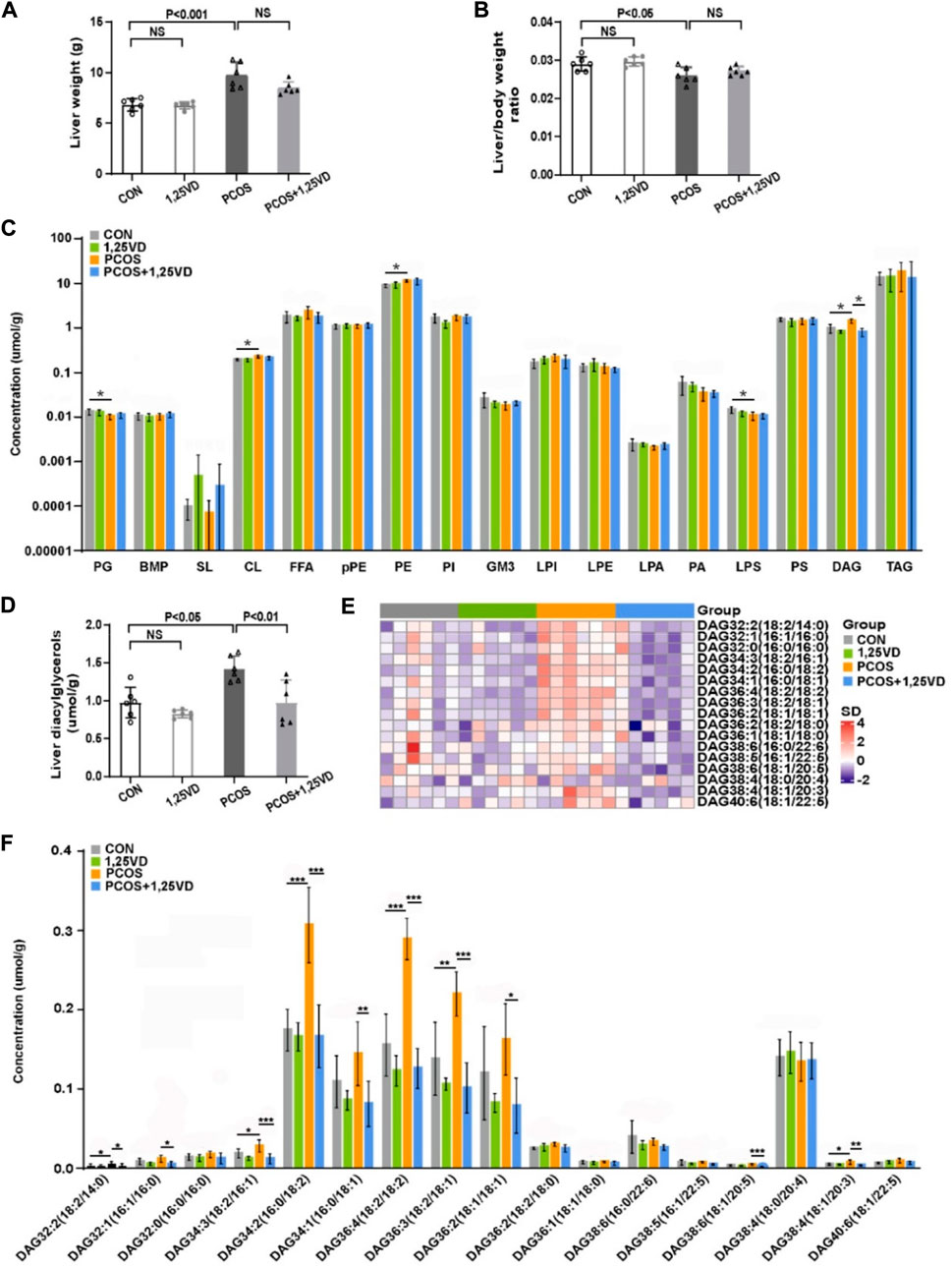
FIGURE 4. Comparison of the liver weight (A), liver/body weight ratio (B) and hepatic lipid profile (C–F) among the study groups. PG: Phosphatidylglycerols, BMP: Bis (monoacylglycerol) phosphate, SL: Sulfatides, CL: Cardiolipins, FFA: Free fatty acids, pPE: PlasmalogenPE, PE: Phosphatidylethanolamines, PI: Phosphatidylinositols, GM3: Monosialogangliosides, LPI: Lyso-PI, LPE: Lyso-PE, LPA: Lyso-PA, PA: Phosphatidic acids, LPS: Lyso-PS, PS: Phosphatidylserines, DAG: Diacylglycerols, TAG: Triaclglycerols. *p < 0.05, **p < 0.01, ***p < 0.001.
4 The contents of six DGs [DG 32:2 (18:2/14:0), DG 34:3 (18:2/16:1), DG 34:2 (16:0/18:2), DG 36:4 (18:2/18:2), DG 36:3 (18:2/18:1) and DG 38:4 (18:1/20:3)] were markedly increased in the PCOS group compared with the control group. 1,25(OH)2D3 supplementation significantly decreased the DG content [DG 32:2 (18:2/14:0), DG 32:1 (16:1/16:0), DG 34:3 (18:2/16:1), DG 34:2 (16:0/18:2), DG 34:1 (18:0/18:1), DG 36:4 (18:2/18:2), DG 36:3 (18:2/18:1), DG 36:2 (18:1/18:1), DG 38:6 (18:1/20:5) and DG 38:4 (18:1/20:3)] (Figure 4F).
4 Discussion
In this study, we found a significant decrease in vitamin D concentration in letrozole-induced PCOS rats compared to that in control rats. The key findings of the present study were the metabolic improvement of 1,25(OH)2D3 in PCOS rats and the potential regulatory effects of 1,25(OH)2D3 on hepatic DG content.
PCOS is a heterogeneous disease accompanied by metabolic abnormalities, such as obesity, insulin resistance, dyslipidemia, and endothelial dysfunction. Improvement of metabolic dysfunction is of paramount importance to reduce the risk of metabolic and cardiovascular disorders in patients with PCOS later in life. Additionally, improvement of metabolic dysfunction is beneficial for reproductive function and pregnancy outcomes (Teede et al., 2010). In the current study, we confirmed that letrozole gavage administration could induce PCOS-like phenotypes in rats, including cystic ovarian morphology with a lack of corpora lutea, disturbance of the estrus cycle, and elevated testosterone levels and LH/FSH ratio. Vitamin D deficiency is reportedly associated with metabolic disturbances in PCOS, and the prevalence of vitamin D deficiency among PCOS women (67%–85%) is much higher than among the healthy population (20%–48%) (Thomson et al., 2012; He et al., 2015). However, whether vitamin D supplementation could exert therapeutic effects on PCOS remains controversial. The discordant results of existing studies may be due to the differences in treatment duration, type, vitamin D dosage, and the characteristics of the study participants (Trummer et al., 2018; Guo et al., 2020). In current work, we also found a significant decrease in vitamin D concentration in letrozole-induced PCOS rats compared to in control rats. These results suggest that vitamin D levels are abnormally changed in PCOS. However, the underlying mechanism requires further investigation.
Consequentially, to further explore the roles of vitamin D in PCOS, active 1,25(OH)2D3 was injected into PCOS rat models in the present study, which reduced the weight, increased insulin sensitivity, and ameliorated lipid disturbances in PCOS rats. Previous data suggested that approximately 50% of women with PCOS are overweight or obese, and excess weight aggravates metabolic and reproductive disorders in PCOS (Glueck and Goldenberg, 2019), and our results were consistent with the previous observation. It has been reported that, excessive accumulation of adipose tissue can immobilize fat-soluble vitamin D and induce oxidative stress and inflammation in PCOS, which interferes with vitamin D metabolism (Helal et al., 2021; Mancini et al., 2021). In current work, we observed that letrozole-treated rats exhibited a significant increase in body weight and a decrease in serum 25(OH)D concentration; these results are consistent with previous observations (Grzesiak et al., 2021). Moreover, a previous study confirmed amelioration of weight gain after a subcutaneous injection of 1,25(OH)2D3 (Azhar et al., 2021). Furthermore, the results of randomized, placebo-controlled clinical trials conducted in women with PCOS found that vitamin D supplementation led to metabolic benefits (Foroozanfard et al., 2017; Javed et al., 2019). Javed et al. (2019) Found a modest reduction in homeostatic model assessment-insulin resistance (HOMA-IR) in women with PCOS who were orally administered vitamin D (3200 IU/day) for 3 months. Foroozanfard et al. (2017) Conducted a trial in 90 insulin-resistant patients with PCOS where the participants were allocated to take either 4000 or 1000 IU of vitamin D per day or placebo for 3 months. They observed reduced fasting plasma glucose levels, HOMA-IR, serum triglycerides levels, and total-, LDL-, and total/HDL-cholesterol levels in the high-dose vitamin D group. In PCOS rats, we also observed a decrease in blood glucose levels at 15 min, but a more significant increase in blood glucose levels at 60 min during the OGTT, which indicates altered patterns of glucose metabolism. Furthermore, we found reduced HDL-c levels and elevated LDL-c levels in PCOS rats. These suggest that treatment with 1,25(OH)2D3 in a letrozole-induced PCOS rat model could prevent weight gain, increase HDL-c levels, improve insulin sensitivity, and decrease postprandial blood glucose levels. Our results are consistent with those of previous studies.
Insulin resistance plays a key role in the onset and progression of PCOS and DG is reportedly associated with insulin-related signaling pathways and is a predictor of insulin resistance (Kumashiro et al., 2011; Finck and Hall, 2015; Jayasinghe et al., 2019). Previous studies on other metabolic diseases, such as obesity and non-alcoholic fatty liver disease, have indicated that the ectopic accumulation of lipids is one of the main causes of insulin resistance in non-adipose tissues. In a letrozole-induced PCOS model, we found that ITT reduced the hypoglycemic effect of insulin, which could be ameliorated by treatment with 1,25(OH)2D3. Furthermore, the liver is an important target organ of insulin; However, how lipid content is affected in such a model and whether 1,25(OH)2D3 has regulatory effects remain poorly understood. A previous study conducted using obese rat models showed that insulin resistance is associated with increased DG concentrations (Turinsky et al., 1990). Assessment of liver biopsy samples from obese individuals also revealed that DG content was responsible for the development of insulin resistance (Kumashiro et al., 2011). Multiple studies have suggested that elevated DG levels may impair insulin action in fatty liver disease (Finck and Hall, 2015). In the liver, DGs have been proposed to activate protein kinase Cε (PKCε) (Kumashiro et al., 2011); The activated PKCε phosphorylates the insulin receptor Thr1160 in the kinase activation loop and inhibits insulin receptor kinase activity (Petersen et al., 2016). Furthermore, a subcellular fractionation study revealed that sn-1,2-DG located in the liver plasma membrane is responsible for impeding insulin signaling. Measurement of in vivo hepatic insulin signaling indicated that the acute knockdown of hepatic DG acyltransferase-2 increases the accumulation of DG, which in turn activates PKCε and induces insulin resistance in the liver (Lyu et al., 2020). Overall, DG plays a key role in regulating hepatic insulin sensitivity, especially during the pathophysiological processes of obesity, diabetes, and non-alcoholic fatty liver disease. Our data established an in vivo letrozole-induced PCOS rodent model with insulin resistance and abnormal liver DG levels. In addition, the current results suggest that 1,25(OH)2D3 could decrease the level of DG in PCOS and also confirmed the positive correlation between DG and ITT-AUC. In summary, changes in hepatic DG might be associated with the anti-obesity and insulin-sensitizing effects of 1,25(OH)2D3. Further investigation is required to assess possible underlying mechanisms.
The current study had several limitations. First, the serum 1,25(OH)2D levels in rats were not measured. Serum 25(OH)D commonly reflects the vitamin D nutrient status (Bouillon et al., 2008); however, it cannot be used to assess the active vitamin D levels after 1,25(OH)2D supplementation. Another limitation was the relatively short study duration. Finally, the correlation between 1,25(OH)2D and insulin sensitivity did not prove causality, and the mechanism of DG variation that could affect insulin signaling in PCOS was not investigated in the current study.
In conclusion, vitamin D supplementation could prevent obesity, insulin resistance, and dyslipidemia in a letrozole-induced PCOS rat model. Moreover, this study sheds light on the regulatory effects of 1,25(OH)2D3 on hepatic DG levels, as indicated by the lipidomic analysis. This phenomenon may explain the protective effects of vitamin D on PCOS. Further studies are required to assess the possible mechanisms underlying the DG-modulating effects of vitamin D. The results of the current study may provide novel evidence for the clinical application of vitamin D as a dietary supplement for long-term management of PCOS.
Data availability statement
The original contributions presented in the study are included in the article/supplementary material, further inquiries can be directed to the corresponding author.
Ethics statement
The animal study was reviewed and approved by the ethics committee of the Third Affiliated Hospital of Soochow University.
Author contributions
XY, JY, and DS performed most of the experiments and prepared the manuscript; KL, XJ, LW, SX, YJ, KG, ZZ, and BL performed some of the experiments; FH designed the study and revised the manuscript.
Funding
The research was supported by Changzhou Sci&Tech Program (Grant No. CJ20220056and Changzhou Sci&Tech Program (Grant No. CJ20220063).
Conflict of interest
Authors ZZ and BL were employed by the LipidALL Technologies Company Limited.
The remaining authors declare that the research was conducted in the absence of any commercial or financial relationships that could be construed as a potential conflict of interest.
Publisher’s note
All claims expressed in this article are solely those of the authors and do not necessarily represent those of their affiliated organizations, or those of the publisher, the editors and the reviewers. Any product that may be evaluated in this article, or claim that may be made by its manufacturer, is not guaranteed or endorsed by the publisher.
References
Azhar, A., Haider, G., Naseem, Z., Farooqui, N., Farooqui, M. U., and Rehman, R. (2021). Morphological changes in the experimental model of polycystic ovary syndrome and effects of vitamin D treatment. J. obstetrics Gynaecol. Res. 47 (3), 1164–1171. doi:10.1111/jog.14671
Barry, J. A., Azizia, M. M., and Hardiman, P. J. (2014). Risk of endometrial, ovarian and breast cancer in women with polycystic ovary syndrome: A systematic review and meta-analysis. Hum. Reprod. Update 20 (5), 748–758. doi:10.1093/humupd/dmu012
Bouillon, R., Carmeliet, G., Verlinden, L., van Etten, E., Verstuyf, A., Luderer, H. F., et al. (2008). Vitamin D and human health: Lessons from vitamin D receptor null mice. Endocr. Rev. 29 (6), 726–776. doi:10.1210/er.2008-0004
Bozdag, G., Mumusoglu, S., Zengin, D., Karabulut, E., and Yildiz, B. O. (2016). The prevalence and phenotypic features of polycystic ovary syndrome: A systematic review and meta-analysis. Hum. Reprod. 31 (12), 2841–2855. doi:10.1093/humrep/dew218
Choubey, M., Ranjan, A., Bora, P. S., Baltazar, F., Martin, L. J., and Krishna, A. (2019). Role of adiponectin as a modulator of testicular function during aging in mice. Biochimica biophysica acta Mol. basis Dis. 1865 (2), 413–427. doi:10.1016/j.bbadis.2018.11.019
Ea-Spcwg, Rotterdam (2004). Revised 2003 consensus on diagnostic criteria and long-term health risks related to polycystic ovary syndrome (PCOS). Hum. Reprod. 19 (1), 41–47. doi:10.1093/humrep/deh098
Eftekhar, M., Mirhashemi, E. S., Molaei, B., and Pourmasumi, S. (2020). Is there any association between vitamin D levels and polycystic ovary syndrome (PCOS) phenotypes? Arch. Endocrinol. Metab. 64 (1), 11–16. doi:10.20945/2359-3997000000177
Finck, B. N., and Hall, A. M. (2015). Does diacylglycerol accumulation in fatty liver disease cause hepatic insulin resistance? BioMed Res. Int. 2015, 104132. doi:10.1155/2015/104132
Foroozanfard, F., Talebi, M., Samimi, M., Mehrabi, S., Badehnoosh, B., Jamilian, M., et al. (2017). Effect of two different doses of vitamin D supplementation on metabolic profiles of insulin-resistant patients with polycystic ovary syndrome: A randomized, double-blind, placebo-controlled trial. Horm. Metab. Res. 49 (8), 612–617. doi:10.1055/s-0043-112346
Glueck, C. J., and Goldenberg, N. (2019). Characteristics of obesity in polycystic ovary syndrome: Etiology, treatment, and genetics. Metabolism: Clin. Exp. 92, 108–120. doi:10.1016/j.metabol.2018.11.002
Goodarzi, M. O., Dumesic, D. A., Chazenbalk, G., and Azziz, R. (2011). Polycystic ovary syndrome: Etiology, pathogenesis and diagnosis. Nat. Rev. Endocrinol. 7 (4), 219–231. doi:10.1038/nrendo.2010.217
Grzesiak, M., Burzawa, G., Kurowska, P., Blaszczyk, K., Szlaga, A., Blasiak, A., et al. (2021). Altered vitamin D3 metabolism in the ovary and periovarian adipose tissue of rats with letrozole-induced PCOS. Histochem Cell Biol. 155 (1), 101–116. doi:10.1007/s00418-020-01928-z
Guo, S., Tal, R., Jiang, H., Yuan, T., and Liu, Y. (2020). Vitamin D supplementation ameliorates metabolic dysfunction in patients with PCOS: A SystematicReview of RCTs and insight into the underlying mechanism. Int. J. Endocrinol. 2020, 7850816. doi:10.1155/2020/7850816
He, C., Lin, Z., Robb, S. W., and Ezeamama, A. E. (2015). Serum vitamin D levels and polycystic ovary syndrome: A systematic review and meta-analysis. Nutrients 7 (6), 4555–4577. doi:10.3390/nu7064555
Helal, B. A. F., Ismail, G. M., Nassar, S. E., and Zeid, A. A. A. (2021). Effect of vitamin D on experimental model of polycystic ovary syndrome in female rats. Life Sci. 283, 119558. doi:10.1016/j.lfs.2021.119558
Javed, Z., Papageorgiou, M., Deshmukh, H., Kilpatrick, E. S., Mann, V., Corless, L., et al. (2019). A randomized, controlled trial of vitamin D supplementation on cardiovascular risk factors, hormones, and liver markers in women with polycystic ovary syndrome. Nutrients 11 (1), 188. doi:10.3390/nu11010188
Jayasinghe, S. U., Tankeu, A. T., and Amati, F. (2019). Reassessing the role of diacylglycerols in insulin resistance. Trends Endocrinol. metabolism: TEM 30 (9), 618–635. doi:10.1016/j.tem.2019.06.005
Kumashiro, N., Erion, D. M., Zhang, D., Kahn, M., Beddow, S. A., Chu, X., et al. (2011). Cellular mechanism of insulin resistance in nonalcoholic fatty liver disease. Proc. Natl. Acad. Sci. U S A 108 (39), 16381–16385. doi:10.1073/pnas.1113359108
Lam, S. M., Chua, G. H., Li, X-J., Su, B., and Shui, G. (2016). Biological relevance of fatty acyl heterogeneity to the neural membrane dynamics of rhesus macaques during normative aging. Oncotarget Actions Search PubMed Search NLM Catalog Add Search 7 (35), 55970–55989. doi:10.18632/oncotarget.11190
Lam, S. M., Zhang, C., Wang, Z., Ni, Z., Zhang, S., Yang, S., et al. (2021). A multi-omics investigation of the composition and function of extracellular vesicles along the temporal trajectory of COVID-19. Nat. Metab. 3 (7), 909–922. doi:10.1038/s42255-021-00425-4
Lyu, K., Zhang, Y., Zhang, D., Kahn, M., Ter Horst, K. W., Rodrigues, M. R. S., et al. (2020). A membrane-bound diacylglycerol species induces PKC-mediated hepatic insulin resistance. Cell Metab. 32 (4), 654–664. doi:10.1016/j.cmet.2020.08.001
Mancini, A., Bruno, C., Vergani, E., d'Abate, C., Giacchi, E., and Silvestrini, A. (2021). Oxidative stress and low-grade inflammation in polycystic ovary syndrome: Controversies and new insights. Int. J. Mol. Sci. 22 (4), 1667. doi:10.3390/ijms22041667
Osibogun, O., Ogunmoroti, O., and Michos, E. D. (2020). Polycystic ovary syndrome and cardiometabolic risk: Opportunities for cardiovascular disease prevention. Trends Cardiovasc Med. 30 (7), 399–404. doi:10.1016/j.tcm.2019.08.010
Pergialiotis, V., Karampetsou, N., Panagopoulos, P., Trakakis, E., and Papantoniou, N. (2017). The effect of vitamin D supplementation on hormonal and glycaemic profile of patients with PCOS: A meta-analysis of randomised trials. Int. J. Clin. Pract. 71 (6), e12957. doi:10.1111/ijcp.12957
Petersen, M. C., Madiraju, A. K., Gassaway, B. M., Marcel, M., Nasiri, A. R., Butrico, G., et al. (2016). Insulin receptor Thr1160 phosphorylation mediates lipid-induced hepatic insulin resistance. J. Clin. Investigation 126 (11), 4361–4371. doi:10.1172/JCI86013
Plum, L. A., and DeLuca, H. F. (2010). Vitamin D, disease and therapeutic opportunities. Nat. Rev. Drug Discov. 9 (12), 941–955. doi:10.1038/nrd3318
Rocha, A. L. L., Faria, L. C., Guimaraes, T. C. M., Moreira, G. V., Candido, A. L., Couto, C. A., et al. (2017). Non-alcoholic fatty liver disease in women with polycystic ovary syndrome: Systematic review and meta-analysis. J. Endocrinol. Invest 40 (12), 1279–1288. doi:10.1007/s40618-017-0708-9
Skiba, M. A., Islam, R. M., Bell, R. J., and Davis, S. R. (2018). Understanding variation in prevalence estimates of polycystic ovary syndrome: A systematic review and meta-analysis. Hum. Reprod. Update 24 (6), 694–709. doi:10.1093/humupd/dmy022
Stener-Victorin, E., Padmanabhan, V., Walters, K. A., Campbell, R. E., Benrick, A., Giacobini, P., et al. (2020). Animal models to understand the etiology and pathophysiology of polycystic ovary syndrome. Endocr. Rev. 41 (4), bnaa010. doi:10.1210/endrev/bnaa010
Sun, J., Jin, C., Wu, H., Zhao, J., Cui, Y., Liu, H., et al. (2017). Effects of electro-acupuncture on ovarian P450arom, P450c17α and mRNA expression induced by letrozole in PCOS rats. J. PLoS ONE 8 (11), e79382. doi:10.1371/journal.pone.0079382
Teede, H., Deeks, A., and Moran, L. (2010). Polycystic ovary syndrome: A complex condition with psychological, reproductive and metabolic manifestations that impacts on health across the lifespan. BMC Med. 8, 41. doi:10.1186/1741-7015-8-41
Thomson, R. L., Spedding, S., and Buckley, J. D. (2012). Vitamin D in the aetiology and management of polycystic ovary syndrome. Clin. Endocrinol. 77 (3), 343–350. doi:10.1111/j.1365-2265.2012.04434.x
Trummer, C., Schwetz, V., Kollmann, M., Wölfler, M., Julia, M., Pieber, T. R., et al. (2018). Effects of vitamin D supplementation on metabolic and endocrine parameters in PCOS: A randomized-controlled trial. Eur. J. Nutr. 58 (5), 2019–2028. doi:10.1007/s00394-018-1760-8
Turinsky, J., O’Sullivan, D. M., and Bayly, B. P. (1990). 1,2-diacylglycerol and ceramide levels in insulin-resistant tissues of the rat in vivo. J. Biol. Chem. 265, 16880–16885. doi:10.1016/s0021-9258(17)44844-7
Upadhyay, S., Krishna, A., and Singh, A. (2021). Role of 14-3-3β protein on ovarian folliculogenesis, steroidogenesis and its correlation in the pathogenesis of PCOS in mice. General Comp. Endocrinol. 313, 113900. doi:10.1016/j.ygcen.2021.113900
van Schoor, N., and Lips, P. (2017). Global overview of vitamin D status. Endocrinol. Metab. Clin. North Am. 46 (4), 845–870. doi:10.1016/j.ecl.2017.07.002
Walters, K. A., Gilchrist, R. B., Ledger, W. L., Teede, H. J., Handelsman, D. J., and Campbell, R. E. (2018). New perspectives on the pathogenesis of PCOS: Neuroendocrine origins. Trends Endocrinol. Metab. 29 (12), 841–852. doi:10.1016/j.tem.2018.08.005
Keywords: vitamin D, polycystic ovary syndrome, metabolic dysfunction, lipidomics, diacyglycerol
Citation: Yuan X, Yang J, Sun D, Luo K, Jiang X, Wang L, Xiang S, Jiang Y, Ge K, Zhou Z, Li B and Hua F (2023) 1,25-Dihydroxyvitamin D inhibits hepatic diacyglycerol accumulation and ameliorates metabolic dysfunction in polycystic ovary syndrome rat models. Front. Pharmacol. 14:1077014. doi: 10.3389/fphar.2023.1077014
Received: 22 October 2022; Accepted: 06 March 2023;
Published: 05 April 2023.
Edited by:
Andrew Bahn, University of Otago, New ZealandReviewed by:
Rodrigo O. Maranon, CCT CONICET Tucuman, ArgentinaRenato Simões Gaspar, University of São Paulo, Brazil
Copyright © 2023 Yuan, Yang, Sun, Luo, Jiang, Wang, Xiang, Jiang, Ge, Zhou, Li and Hua. This is an open-access article distributed under the terms of the Creative Commons Attribution License (CC BY). The use, distribution or reproduction in other forums is permitted, provided the original author(s) and the copyright owner(s) are credited and that the original publication in this journal is cited, in accordance with accepted academic practice. No use, distribution or reproduction is permitted which does not comply with these terms.
*Correspondence: Fei Hua, aHVhZmVpMTk3MEBzdWRhLmVkdS5jbg==
†These authors have contributed equally to this work