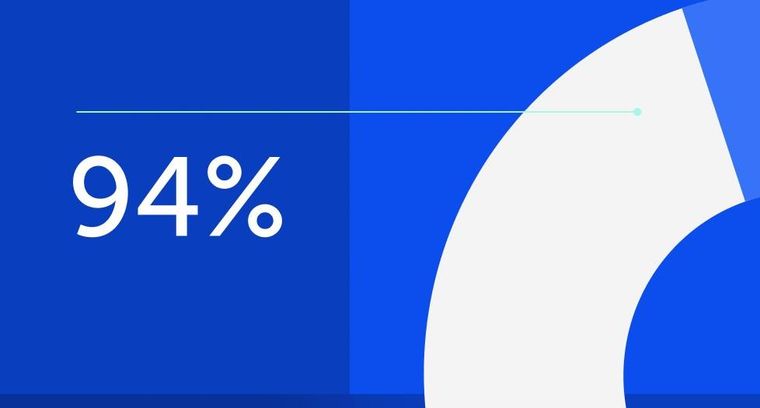
94% of researchers rate our articles as excellent or good
Learn more about the work of our research integrity team to safeguard the quality of each article we publish.
Find out more
ORIGINAL RESEARCH article
Front. Pharmacol., 14 March 2023
Sec. Experimental Pharmacology and Drug Discovery
Volume 14 - 2023 | https://doi.org/10.3389/fphar.2023.1068153
This article is part of the Research TopicModel-informed drug development and precision dosing in clinical pharmacology practiceView all 8 articles
Sildenafil is a potent vasodilator and phosphodiesterase type five inhibitor, commercially known as Revatio® and approved for the treatment of pulmonary arterial hypertension. Maternal administration of sildenafil during pregnancy is being evaluated for antenatal treatment of several conditions, including the prevention of pulmonary hypertension in fetuses with congenital diaphragmatic hernia. However, determination of a safe and effective maternal dose to achieve adequate fetal exposure to sildenafil remains challenging, as pregnancy almost always is an exclusion criterion in clinical studies. Physiologically-based pharmacokinetic (PBPK) modelling offers an attractive approach for dose finding in this specific population. The aim of this study is to exploit physiologically-based pharmacokinetic modelling to predict the required maternal dose to achieve therapeutic fetal exposure for the treatment congenital diaphragmatic hernia. A full-PBPK model was developed for sildenafil and N-desmethyl-sildenafil using the Simcyp simulator V21 platform, and verified in adult reference individuals, as well as in pregnant women, taking into account maternal and fetal physiology, along with factors known to determine hepatic disposition of sildenafil. Clinical pharmacokinetic data in mother and fetus were previously obtained in the RIDSTRESS study and were used for model verification purposes. Subsequent simulations were performed relying either on measured values for fetal fraction unbound (fu = 0.108) or on values predicted by the simulator (fu = 0.044). Adequate doses were predicted according to the efficacy target of 15 ng/mL (or 38 ng/mL) and safety target of 166 ng/mL (or 409 ng/mL), assuming measured (or predicted) fu values, respectively. Considering simulated median profiles for average steady state sildenafil concentrations, dosing regimens of 130 mg/day or 150 mg/day (administered as t.i.d.), were within the therapeutic window, assuming either measured or predicted fu values, respectively. For safety reasons, dosing should be initiated at 130 mg/day, under therapeutic drug monitoring. Additional experimental measurements should be performed to confirm accurate fetal (and maternal) values for fu. Additional characterization of pharmacodynamics in this specific population is required and may lead to further optimization of the dosing regimen.
Sildenafil (SIL) is a phosphodiesterase type 5 (PDE5) inhibitor, and used as a potent vasodilator approved by regulatory agencies for the treatment of erectile dysfunction (Viagra®) and pulmonary hypertension (PAH) (Revatio®) (Ghofrani et al., 2006; FDA, 2018; EMA, 2022). According to the biopharmaceutics classification system (BCS) SIL is a class 2 drug, rapidly absorbed, reaching Tmax in approximately 1 h and is highly bound to plasma protein (∼96%). It is mainly metabolized by cytochrome P450 (CYP) enzymes, primarily by CYP3A4 into the active metabolite N-desmethyl-sildenafil (DMS). SIL half-life is between 3 and 4 h for doses of 25–200 mg (Nichols et al., 2002; Zane et al., 2015).
There is evidence that, when administered in pregnancy, SIL inhibits the vasoconstriction in spiral arteries in uterus and increases the fetal and uteroplacental blood flow (Dastjerdi et al., 2012). For these reasons, SIL has been clinically investigated for the treatment of several pregnancy complications, including intrauterine growth restriction, preeclampsia, and fetal distress during labor (de Bie et al., 2022). The maternal administration of SIL during pregnancy is also being evaluated for antenatal treatment of congenital diaphragmatic hernia (CDH) (Luong et al., 2011; Russo et al., 2019a). CDH occurs when the diaphragm is not fully closed during fetal development and leads to pulmonary hypoplasia, pulmonary hypertension and cardiac dysfunction (Deprest et al., 2014). In animal studies, transplacental treatment with SIL has been shown to prevent the lung vascular changes that lead to pulmonary hypertension after birth (Russo et al., 2016; Kashyap et al., 2019). However, the optimal maternal dose required to achieve safe and effective fetal exposure remains elusive. It is well known that pregnancy leads to pronounced changes in physiology as well as pharmacokinetics (PK) compared with non-pregnant women (Abduljalil et al., 2012; Szeto et al., 2021). Unfortunately, clinical (PK) data in this vulnerable population are virtually non-existent, also because pregnancy is a very common exclusion criterion during clinical trials.
In recent years, non-clinical computational tools have already shown their utility for simulating PK in special populations, thereby for instance taking in account the ontogeny of proteins relevant to drug disposition. Such in silico tools ultimately allow the assessment of drug exposure for a given dosing regimen. More recently, Model Informed Drug Development (MIDD) has emerged as a powerful alliance for regulatory decisions on drug development. MIDD avoids unnecessary exposure in clinical studies with special populations by predicting drug exposure and enables understanding the PK and pharmacodynamics (PD) for example, in pregnant women (Dallmann et al., 2018; Wang et al., 2019; Kuemmel et al., 2020; Coppola et al., 2021).
MIDD encompasses a spectrum of approaches, one of which is Physiologically-based Pharmacokinetic (PBPK) modeling (Darwich et al., 2006). PBPK modelling is the bottom-up computational approach where the association of the physiologic description of the organism with the physicochemical characteristics of the drug (or chemical) results in plasma or tissue concentration time profiles (Kuepfer et al., 2016). PBPK models allow to simulate different (patho)physiologic conditions, while for instance considering ontogeny of protein expression/function with progressing age of the virtual patients. PBPK modelling can thus help understand the PK drug behavior in the human body without the need to conduct (extensive) clinical studies in special populations (Kuepfer et al., 2016). Therefore, the aim of this work is to develop a PBPK model to predict the required maternal dose to achieve therapeutic fetal exposure for the treatment of congenital diaphragmatic hernia.
The Simcyp® simulator version 21 (Simcyp Ltd, Sheffield, UK) was used to develop the PBPK model in reference volunteer individuals and subsequently in pregnant women.
SIL and DMS physicochemical and in vitro PK properties were obtained from literature and are summarized in Table 1. The oral absorption was described using the Advanced Dissolution, Absorption and Metabolism (ADAM) model, the effective permeability (Peff) was estimated using the Mechanistic Permeability (MechPeff) model. The volume of distribution at steady state (Vss) was calculated using the Rodgers and Rowland method. The Kp Scalar was adjusted to match with the volume of distribution reported in literature (Nichols et al., 2002). Based on experiments with human liver microsomes (HLM) (Zane et al., 2015), SIL is metabolized into the main active metabolic DMS majorly by CYP3A4, and to a lesser extent by CYP3A5 > CYP3A7. Further proportional scaling was applied to intrinsic clearance reported by Zane et al. (2015) with a factor of 1.46 to the initial values for their sum to match total intrinsic clearance (314 μL/min/mg protein) as retro-gradually calculated based on in vivo clearance values (Milligan et al., 2002; Muirhead et al., 2002). For the active metabolite DMS the first order absorption model was used. The fraction absorbed (fa) and the first-order absorption rate constant (ka) were predicted based on the polar surface area (PSA) and number of hydrogen bond donors (HBD). In addition, the volume of distribution at steady state (Vss) was calculated using the Rodgers and Rowland method. The in vivo hepatic clearance of DMS was set at 40 L/h as derived from in vivo PK data (Muirhead et al., 2002; Nichols et al., 2002). Renal clearance of DMS was assumed to be negligible (Walker et al., 1999; Hyland et al., 2001).
TABLE 1. Summary of the input data for the PBPK model development of sildenafil and N-desmethyl-sildenafil.
For model evaluation, predicted plasma concentration time profiles were qualified against clinical observed data collected in literature for intravenous and oral administration of different doses. Population simulations were performed for 100 (10 trials × 10 individuals) virtual adult individuals, while matching age and weight to the demographics reported for the clinical study in literature (see Supplementary Table S1). The geometric mean fold error (GMFE) was calculated for the ratio of the predicted over observed concentration profile for Area Under Curve (AUC) and maximum concentration (Cmax) (Eq. 1). The model was accepted when the Cmax and AUC GMFE was within a two-fold error margin (0.5–2.00) (Xu et al., 2021).
In addition, to verify the predictive performance of the model, visual predictive checks (VPC) were used to compare the predicted concentration time profiles with observed plasma profiles reported for adult reference individuals. As an acceptance criterion, a maximum of 10% of observations outside the 90% prediction interval (5th-95th percentile) was used (European Medicine Agency, 2018).
The PBPK model developed in reference individuals was then used to establish a PBPK model in pregnant women. The Sim-Pregnancy population module in the Simcyp simulator was selected to consider all the physiologic changes (e.g., blood flow, body weight, enzyme expression, and albumin) in the population for the gestational age of 38 weeks. The maternal model was coupled with the fetal model using the permeability-limited placental model. Data from ex vivo human placenta experiments were used to inform the transplacental kinetics (Russo et al., 2018). These data were based on a previously developed model describing the ex vivo transfer through the placenta and to estimate the cotyledon clearance, CLcot 0.152 L/h (Hoeben et al., 2018).
The transplacental permeability clearance was calculated using the Simcyp simulator toolbox with input of the CLcot = 0.152 L/h/cotyledon, and assuming 17 cotyledons/placenta. Hence, the calculated passive diffusion clearance between mother and fetus (CLPDM/PDF), and used as input value in the Simulator, was calculated to be 0.0038168 L/h/mL placenta (Table 1).
The predicted PK profile for pregnant women was compared with the data provided in the clinical trial study RIDSTRESS (“Reducing the Incidence of Fetal Distress with Sildenafil Citrate”) conducted at the Mater Mother’s Hospital in Brisbane Australia. In the study, women of 18–50 years of age at gestational week equal or greater than 37 weeks, in early labor received via oral administration 50 mg of sildenafil citrate in fasted state. The maternal blood samples were collected 1–3 h after the dose administration. In addition, cord blood samples were collected right after the delivery (Turner et al., 2020). The samples were collected in Mater Mother’s Hospital in Brisbane Australia and shipped to BioNotus (Niel, Belgium) for analysis, as described below.
The established pregnancy PBPK model was subsequently used for simulating concentration-time profiles in pregnant women, at the following dosing regimens: doses of 50, 75, 100, 130, 150, 175, 200, 250, 300, 320, and 360 mg/day of SIL (immediate release tablets), administered as t.i.d. (three times a day). The simulations included predicting fetal concentration time profiles, allowing determination of the dosing regimen necessary to achieve fetal exposure within the (reference) therapeutic window of 47–500 ng/mL (Barst et al., 2012; Russo et al., 2016). However, it should be noted that the boundaries of this therapeutic window assume a fu of 0.036 applicable in reference individuals. As fu values are altered during pregnancy and in the fetal circulation, the final simulations were performed assuming either: 1) The fu in fetal plasma predicted by the Simcyp software, or the fu in fetal plasma determined experimentally in vitro as described below. Prediction of fetal fu was achieved by first calculating the Kd value for sildenafil binding to albumin with the following equation:
Where [P] ref is the reference protein concentration of 45 g/L, and fu = 0.036 in the adult reference population.
Assuming the Kd is similar between adult and fetal albumin (JORI et al., 2009), the fu in fetal plasma was then calculated based on input of this Kd value (1.68 µM) along with the reported albumin concentration in the fetus (36 g/L), as follows:
The maternal and fetal concentrations of SIL and DMS from RIDSTRESS study (46 samples) were analyzed using Liquid Chromatography-Mass Spectrometry (LC-MS/MS). The LC-MS/MS system used for the analysis was a UPLC Shimadzu Nexera X2 coupled to a LC-MS8050. The column for the analysis was a Kinetex® XB-C18 2.6 µm, 50 × 2.1 mm, 100 Å. The column temperature was set to 30°C to have a constant and stable environment for the flow, the flow rate was set to 0.3 mL/min. The mobile phase was 0.1% formic acid in water (mobile phase A) and acetonitrile: methanol (50:50 v/v) (mobile phase B), isocratic mode. One µL sample was injected and the run time was 6 min. The MS parameters were optimized to reflect the best sensitivity and signal stability. SIL and DMS transitions were 475.00/58.15 and 461.10/85.20, respectively. The measurement range was 7.8–1,000 ng/mL. A 1/x2-weighted linear regression model was used to adequately describe the concentration–peak area relationship. The calibration curve standards and quality control standards were in compliance with bioanalytical guidelines (ICH guideline M10) for this run.
To determine the SIL free fraction, fetal plasma samples were equilibrium dialyzed for 4 h at 37°C against blank phosphate buffered saline with the HTDialysis device (Gales Ferry, United States of America) using 12 kDa (molecular weight cut off) membrane dialysis strips. Prior to bioanalysis, the collected PBS and fetal plasma samples after dialysis were diluted in 1:1 with blank plasma or blank buffer, respectively, to create a common matrix (50% plasma in PBS). Next, the samples were precipitated using four volumes of methanol, vortexed, centrifugated and the clear supernatant was transferred into vials for LC-MS/MS analysis.
Assay conditions were the same as described above, with the following modifications. The LC-MS/MS column temperature was set to 40°C and the flow rate was set to 0.45 mL/min. 10 µL sample was injected and the run time was 3 min. The measurement range was 1–200 ng/mL, a 1/x-weighted linear regression model was used. The calibration curve standards and quality control standards were in compliance with bioanalytical guidelines (ICH guideline M10) for this run.
The fu was calculated by dividing the SIL concentration in buffer (Cfree) by the SIL concentration in the plasma after equilibrium dialysis.
To perform the PTA analyses, the percentages of simulated individuals with average (Cavg), trough (Ctr) or peak (Cmax) plasma concentrations equal to or above a prespecified target plasma concentration were calculated for different dosing regimens. Because of the sensitivity of the model simulations to the fu values, PTA analyses were performed both for predicted and experimentally determined fetal fu values.
A full-PBPK model was developed for SIL and DMS in adult reference individuals. The GMFE of the predicted over observed ratio for SIL was 0.79 for AUC and 0.99 for Cmax. For DMS GMFE was 0.96 for AUC and 1.5 for Cmax after intravenous and single oral administration at different doses (25, 50, 100, and 200 mg). The AUC and Cmax predictions and observed results are summarized in Supplementary Table S1. The predicted GMFE values of SIL were comparable to the observed data within the two-fold range of the observed values. In Figure 1 the predictive performance graph shows that the calculated ratios are mostly between 1.5-fold when compared to the AUC and Cmax predictions. The VPC showed that the PK profile was well predicted when compared with the observed data collected from literature and are presented in Supplementary Figure S1. The results suggests that the PBPK model developed for adult reference individuals describes well the PK profiles of SIL and DMS and qualifies as a base model to establish a PBPK model in pregnant women.
FIGURE 1. Predictive performance graphs for Cmax and AUC of sildenafil (represented by red points) and N-Desmethyl-Sildenafil (represented by blue points). A comparison was made between observed and simulated Cmax and AUC values for adult reference individuals at different dose levels. Solid lines represent the median line of unity, dashed lines and solid lines represent the two-fold and 1.5-fold difference, respectively.
Equilibrium dialysis was used for the determination of fraction unbound of SIL in selected plasma samples prepared from the cord blood obtained in the RIDTRESS study (Turner et al., 2020). The measured fu values are given in Table 2, ranging between 0.067 and 0.167, with a mean (± SD) value 0.108 (±0.041). Out of a total of six samples, one value (0.698) was found to be an outlier and censored.
The PBPK model as successfully developed and verified for the reference population, was used to establish a PBPK model for pregnant women at 38 weeks in gestation including the fetus. Population simulations for virtual pregnant women were performed and the demographic information was set according to the available clinical data (RIDSTRESS). The predicted plasma concentration-time profile is given in Figures 2, 3 for maternal and umbilical vein plasma concentrations, respectively. The predicted PK profile was compared with the clinical observed data after oral administration of 50 mg in fasted state. The model was evaluated considering the VPCs for both mother and fetus. Given that the RIDSTRESS study was not designed as a PK study (which may have caused additional variability in observed data), the plasma concentration time profiles had a reasonable agreement with the clinical observations. While it was assumed that subjects in the RIDSTRESS trial received a single oral dose of 50 mg sildenafil, the fact that one fetal concentration at t = 0 h was about 13 ng/mL (see Figure 3) strongly suggests that some subjects may have received prior doses. Consistently, seven maternal concentrations were found far above the 90% prediction interval, again indicating that these subjects may have received multiple doses. With only two DMS measurements below the 90% prediction interval, the model provided a good prediction of the concentration time profile of the metabolite.
FIGURE 2. Overlay of the predicted concentration-time profile of sildenafil (A) and N-Desmethyl-Sildenafil (B) after oral dose of 50 mg under fasted conditions to virtual pregnant women (n = 100; gestational age 38 weeks). The red line represents the median predicted profile while the black dashed lines represent the 5th and 95th percentile profiles. The points represent individual measurements from the RIDSTRESS study.
FIGURE 3. Comparison between the predicted concentration-time profile of SIL in umbilical cord vein plasma and observations after an oral dose of 50 mg sildenafil under fasted conditions in pregnant women (n = 100; gestational age 38 weeks). The red line represents the median predicted profile while the black dashed lines represent the 5th and 95th percentile profiles. The circle shape points represent cord blood measurement from the RIDSTRESS study, and the triangle shape points represents the concentrations below the lower limit of quantitation (LLOQ), represented here at the LLOQ.
The pregnancy PBPK model was subsequently used to perform steady-state simulations in populations of 1,000 pregnant women receiving doses of 50, 75, 100, 130, 150, 175, 200, 250, 300, 320, and 360 mg/day, administered as t.i.d. The corresponding predicted maternal and fetal concentrations are shown in Supplementary Figures S2, S3.
The suitability of maternal and fetal exposures was evaluated using Cavg (see Table 3). This was justified given the (very) limited fluctuation of concentrations between troughs and peaks within each steady state dosing interval (irrespective of the percentile). The therapeutic “target” concentration range of 47 ng/mL (efficacy) to 500 ng/mL (toxicity) was used as a reference, but required modification in this special population (because this target range is based on fu values of 0.036 reported for reference individuals). Therefore, in a first scenario, this target range was corrected, assuming a predicted fu value of 0.044. This resulted in a corrected therapeutic window of 38–409 ng/mL. In a second scenario, the significantly higher (experimentally determined) fu value (0.108) was used, yielding binding-corrected values of 15 ng/mL and 166 ng/mL for efficacy and toxicity, respectively (see Figure 4). Furthermore, taking into consideration the interindividual variability in the population, Ctrough (Ctr) values at the 5th percentile were also considered for efficacy, while the Cmax values at the 95th percentile were used for toxicity evaluation (see Table 3).
TABLE 3. Simulated steady state average concentrations (Cavg) at the median (50th percentile), steady state maximum concentrations at the 95th percentile (Cmax) and steady state trough concentrations at the fifth percentile (Ctr) after administration of 50 mg/day to 360 mg/day, given in three times (t.i.d.), concentrations are given in ng/mL.
FIGURE 4. Target concentrations for efficacy and safety when relying on either reference (fu: 0.036), experimentally determined (fu: 0.108) or predicted (fu: 0.044) fraction unbound.
When simulations were performed based on the in vitro measured fu value (0.108), and considering only the median Cavg as PK metric, doses starting at 130 mg/day (Cavg of 15.06 ng/mL), are considered above the proposed efficacy target and not exceeding (Cmax of 30.61 ng/mL) the safety target. However, at this dose the Ctr at the 5th percentile (5.81 ng/mL), is well below the efficacy target (15 ng/mL). Given the limited fluctuation (Ctr/Cmax) in SIL concentrations throughout dosing intervals, also the average concentration at the 5th percentile is well below the efficacy target. Supplementary Figure S3 indeed illustrates that about 50% of the population is outside of the therapeutic range when dosing at 130 mg/day. Requiring Ctr > efficacy target in at least 95% of the population, doses starting at 360 mg/day (Cavg 41.59 ng/mL; Ctr 16.11 ng/mL; Cmax 84.86) are above the efficacy target, and not exceeding the safety target.
The results obtained with simulations using the predicted fu, and first considering only Cavg, doses starting at 150 mg/day (Cavg = 41.70 ng/mL), are above the efficacy target (38 ng/mL) and not exceeding the safety target (Cmax = 85.89 ng/mL). However, at this dose level the 5th percentile of Ctr (or Cavg) does not reach the efficacy target (Ctr = 15.69 ng/mL). With the maximum dose simulated of 360 ng/mL, the 5th percentile Ctr is (slightly) under the efficacy target (Ctr = 37.67 ng/mL < 38 ng/mL), but the 95th percentile Cmax of 206.13 ng/mL is still under the safety threshold (409 ng/mL). Doses above 360 mg/day were not considered in simulations, since at this dose level the maternal exposure considering Cmax at the 95th percentile was above the safety threshold (500 ng/mL), Supplementary Figure S2H; Table 3.
PTA analyses were performed to identify dosing regimens achieving the targeted plasma concentrations in at least 95% of the population. The PTA analyses were carried out by comparing simulated steady state Cavg, Ctr and Cmax values obtained at different dosing regimens with thresholds for efficacy and toxicity, considering both scenarios for obtaining fetal fu values (predicted versus measured). The results showed a probability of reaching the target concentration in at least 95% of the population at 360 mg/day when fu in vitro is considered. However, when fu predicted is assumed, the range is only achieved in at least 95% of the population for Cmax and Cavg, while 93.5% were above the target for Ctr (see Supplementary Figure S4).
SIL is a potent vasodilator used in treatment of erectile dysfunction and pulmonary hypertension in adults (Ghofrani et al., 2006). In recent years, there is a growing interest in the use of SIL during pregnancy to prevent pulmonary hypertension in fetuses with CDH (de Bie et al., 2022). Animal studies have shown that SIL has a potential therapeutic effect in fetuses with congenital diaphragmatic hernia, rescuing peripheral pulmonary vasculature and airway complexity (Luong et al., 2011; Russo et al., 2016; Kashyap et al., 2019; de Bie et al., 2021a). Ex vivo human placenta perfusion experiments with SIL confirmed a high rate of transfer across the human placenta (Russo et al., 2018). Taken together, these findings suggest that maternally administered SIL during pregnancy can reach the fetus to exert its putative therapeutic effect. Importantly, these quantitative transplacental clearance data can be exploited to predict fetal exposure to maternally administered SIL with modelling and simulation. Hence the aim of this study was to exploit a PBPK modelling approach for predicting an adequate maternal dosing regimen of SIL resulting in therapeutic fetal exposure. It is important to note that it was the specific intention of the present endeavor to determine a therapeutic dosing strategy exclusively for fetuses with CDH, and explicitly not for other fetal conditions. Of note, results from the STRIDER trial, which was designed to evaluate the use of sildenafil in fetal growth restriction, revealed increased perinatal mortality in one but not all trial centers, causing early trial termination (Groom et al., 2018; Hawkes, 2018; Pels et al., 2020). Nevertheless, as repeatedly commented by (Russo et al., 2019b; 2022) the negative findings in the STIDER trial should not be simply extrapolated to other fetal conditions, including CDH.
Successful verification of the PBPK model for sildenafil and its metabolite in adult reference populations provided an adequate starting point for establishing the pregnancy PBPK model, allowing the subsequent prediction of maternal and fetal SIL exposure during pregnancy. With a PBPK modelling approach, pronounced changes in physiology as well PK of the pregnant population can be accounted for (Abduljalil et al., 2022; Silva et al., 2022). For instance, a remarkable change during pregnancy is the 60% increase in CYP3A4 activity (Hebert et al., 2008). This was reflected in the significantly higher SIL apparent clearance (CL/F) during pregnancy as compared to reference individuals. Importantly, to adequately inform the transplacental passage of SIL in the PBPK model, the previously generated ex vivo human placenta data were leveraged.
Comparison of the model-predicted concentration time profiles in pregnant women with observations obtained in the RIDSTRESS study (Turner et al., 2020) revealed an acceptable predictive performance for SIL and DMS in pregnant women. As mentioned in the results section, seven maternal plasma concentrations positioned relatively far above the prediction interval, whereas only two DMS concentrations were outside (below) the prediction interval. At the fetal side, only a limited number of cord blood samples were above the quantification limit, but predictions were in agreement with some observations above the LLOQ. Fetal DMS concentrations were found to be very low (<LLLQ), which supports the assumptions that DMS does not cross the placenta (based on a more hydrophilic character compared to SIL), and is not generated by the fetus in clinically relevant concentrations. The latter appears consistent with the much lower conversion by (fetal) CYP3A7 compared to CYP3A4 (Zane et al., 2015). Based on these comparisons, the pregnancy PBPK model for sildenafil was considered adequate to be used for simulation-based dose predictions. Unfortunately, no other PK data from maternal or cord blood samples were available to further support model evaluation. This includes the absence of observations before term gestational age.
Prior to engaging in model-based simulations, one specific aspect of the pregnancy PBPK model that deserves particular attention is the dual impact of altered fetal (and maternal) protein binding as compared to the reference population. First, such changes in fu are likely to affect the maternal/fetal distribution of SIL. Indeed, a significantly higher free fraction of SIL in the fetal circulation will eventually result in lower total concentrations for the same unbound concentration at equilibrium. Secondly, a significant increase in fu in the fetus requires a reevaluation of SIL efficacy and safety levels, expressed as total concentrations.
To obtain accurate values for the altered SIL fu values, two approaches were applied. In one approach, the fraction unbound was calculated based on the change in albumin concentration, thereby assuming no changes in affinity for SIL (i.e., Kd remains unchanged). This is likely a valid approach to calculate the maternal fu during pregnancy (because of decreasing albumin levels during pregnancy). However, the reliability of this approach for predicting the fetal SIL fu value may be questioned. For this reason, and in the absence of a fetus-specific Kd value, it was decided to also experimentally determine the fetal fu value.
In a first scenario, the PBPK model was built using fu values determined by in vitro experiments. The simulated fetal concentration time profiles were evaluated according to the (fu-adapted) efficacy target of 15 ng/mL (considering the fifth percentile Ctr) as well as the toxicity threshold of 166 ng/mL (considering the 95th percentile Cmax). In the second scenario, the PBPK model was built using predicted fu values. In this case, simulated fetal concentration time profiles were evaluated according to the efficacy target of 38 ng/mL as well as the toxicity threshold of 409 ng/mL. To achieve these targets, required maternal dosing regimens are 300 mg/day when considering fifth percentile Cavg values, while even up to 360 mg/day would be needed when considering Ctr at the fifth percentile. However, albeit borderline, the 360 mg/day yields maternal peak plasma concentrations above the toxicity threshold. Simulated fetal plasma concentrations never exceeded the toxicity thresholds. Hence a more pragmatic and especially safer approach is to select a dosing regimen based on the median Cavg concentration reaching the efficacy target, suggesting 130–150 mg/day administered as t.i.d. as a safe initial dosing regimen. Interestingly, both simulation strategies (based on two different fu values) led to similar dose predictions. This similarity was not unexpected, as lower fetal binding leads to less partitioning of sildenafil across the placenta, but also leads to reduced sildenafil target levels when expressed as total concentrations. Therefore, these opposing effects of protein binding were expected to cancel out at least partially.
Remarkably, the fu value obtained from in vitro experiments was found to be 2.5-fold higher compared to the predicted value. It is worth mentioning here the limitations associated with fu values measured in vitro in plasma obtained from cord blood samples. The sample size was low (n = 6; one outlier), and intersubject variability was (very) high, suggesting that protein degradation in some samples may not be excluded (potentially explaining the very high fu value in the outlier measurement). Although the impact of reduced protein binding turned out to be relatively limited, additional measurements of maternal and fetal fu are warranted to inform more accurately future PBPK models.
In guidance to support the clinicians regarding the initial recommended dosing regimen of SIL, it seems justifiable to take the lowest dosing regimen from the two (fu-related) scenario’s presented above. Considering the Cavg metric, our findings support t.i.d. Dosing regimens of 130 mg/day and 150 mg/day to be within the therapeutic range. In both cases, the Ctr at the fifth percentile is still under the proposed target. However, for safety reasons, an initial dose regimen of 130 mg/day administered as t.i.d., combined with subsequent dose titration by therapeutic drug monitoring (TDM) is recommended (Kang and Lee, 2009). Importantly, actual treatments for CDH will occur in earlier stages of pregnancy as opposed to the setting of the data obtained in the RIDSTRESS clinical study, which was used to verify the pregnancy PBPK model developed here. Thus, considering a progressive increase in sildenafil clearance during the trimesters of pregnancy, the proposed ‘lower’ dosing regimen of 130 mg/day becomes more relevant and justifiable.
In support of this dosing regimen proposed based on PBPK simulations in the present study, it is noteworthy that Samangaya et al. (2009) already reported administration of 80 mg SIL three times a day in pregnant women with preeclampsia. They had followed a titration scheme with initial doses of 20 mg escalating to 80 mg t.i.d. and achieving peak drug concentrations of 271 ng/mL, without any adverse effects reported. (Samangaya et al., 2009), providing further confidence in the proposed regimen of 130 mg/day administered as t.i.d. at least from a maternal safety perspective.
From a (fetal) efficacy perspective, it should be recognized that the reference target concentration (47 ng/mL) for efficacy employed in the present study is based on data regarding efficacy of sildenafil in various pediatric (and adult) populations. This implies that the optimal dose level may change as more relevant data on efficacious concentrations in this specific fetal population become available. Interestingly, the two sheep studies in this field have both demonstrated effects of sildenafil at fetal concentrations well below the currently used therapeutic range. Both an acute pulmonary vasodilatory effect (de Bie et al., 2021b) and an effect on neonatal cardiopulmonary hemodynamics (suggesting a longer-term effect on pulmonary vascular development) (Kashyap et al., 2019) were observed at concentrations that are currently assumed to be ‘subtherapeutic’. It is therefore possible that the required fetal sildenafil concentration to achieve the desired developmental effect is lower than the concentration required to achieve acute vasodilation in neonates. Consistently, based on the data published (Ballard et al., 1998; Kashyap et al., 2019; de Bie et al., 2021a; de Bie et al., 2021b) it is not unlikely that concentrations below those required for achieving 53% PDE5 inhibition are still sufficient to obtain long-term therapeutic effect (due to vascular remodeling) in the fetal population for this specific indication.
In conclusion, this work illustrates the utility of PBPK modelling and simulation as powerful tool for dose finding in special populations, while avoiding unnecessary exposure of vulnerable patients. In the present study, the PBPK modelling approach supported dose finding in pregnant women to achieve therapeutic exposure in the fetus. At the same time, pregnancy-associated physiologic changes were accounted for in the model. Based on our findings, and with specific attention to safety, a dosing regimen of 130 mg/day (administered as t.i.d.) appears recommended as initial prescription of SIL, specifically (and exclusively) in antenatal treatment of congenital diaphragmatic hernia associated with pulmonary hypoplasia and hypertension.
Therapeutic drug monitoring will be required to adapt the initial dosing regimen in specific patients. In addition, these concentration measurements will inform and optimize future PBPK models for this indication and population.
Maternal administration of sildenafil during pregnancy is being evaluated for antenatal treatment of congenital diaphragmatic hernia (CDH) associated with pulmonary hypoplasia and hypertension. However, determination of a safe and effective maternal dosing regimen to achieve adequate fetal exposure to sildenafil remains challenging. In the present study, PBPK modelling and simulation approaches are exploited to determine for the first time a dosing regimen recommendation for treatment initiation with sildenafil, specifically in the CDH indication. Upon treatment initiation, maternal sildenafil dosing should be adapted based on therapeutic drug monitoring.
The original contributions presented in the study are included in the article/Supplementary Material, further inquiries can be directed to the corresponding author.
The studies involving human participants were reviewed and approved by Australia New Zealand Clinical Trials Registry (ANZCTRN12615000319572). All versions of the protocol were submitted for approval to the local Human Research Ethics Committee (HREC; HREC/15/MHS/33) and research governance office (RG-15-088). The patients/participants provided their written informed consent to participate in this study.
JM, PA, NN, FM, and AD have contributed to the development of PBPK model. FR and JD have contributed with the ex-vivo and in vivo reference data. JT and SK have contributed with in vivo data. BL and PA developed the methods for measurement of SIL and DMS plasma and cord blood concentration and for determination of fu. BL and PA analyzed the assay data. RH developed the PTA analyses and generated the graphs. JM drafted the manuscript. All authors reviewed the manuscript. All authors approved the final manuscript as submitted and agree to be accountable for all aspects of work.
NN received a PhD scolarship by Research-Foundation-Flanders (1S50721N).
The authors declare that the research was conducted in the absence of any commercial or financial relationships that could be construed as a potential conflict of interest.
All claims expressed in this article are solely those of the authors and do not necessarily represent those of their affiliated organizations, or those of the publisher, the editors and the reviewers. Any product that may be evaluated in this article, or claim that may be made by its manufacturer, is not guaranteed or endorsed by the publisher.
The Supplementary Material for this article can be found online at: https://www.frontiersin.org/articles/10.3389/fphar.2023.1068153/full#supplementary-material
Abduljalil, K., Furness, P., Johnson, T. N., Rostami-Hodjegan, A., and Soltani, H. (2012). Anatomical, physiological and metabolic changes with gestational age during normal pregnancy: A database for parameters required in physiologically based pharmacokinetic modelling. Clin. Pharmacokinet. 51, 365–396. doi:10.2165/11597440-000000000-00000
Abduljalil, K., Ning, J., Pansari, A., Pan, X., and Jamei, M. (2022). Prediction of maternal and fetoplacental concentrations of cefazolin, cefuroxime, and amoxicillin during pregnancy using bottom-up physiologically based pharmacokinetic models. Drug Metabolism Dispos. 50, 386–400. doi:10.1124/dmd.121.000711
Ballard, S. A., Gingell, C. J., Tang, K., Turner, L. A., Price, M. E., and Naylor, A. M. (1998). Effects of sildenafil on the relaxation of human corpus cavernosum tissue in vitro and on the activities of cyclic nucleotide phosphodiesterase isozymes. J. Urol. 159, 2164–2171. doi:10.1097/00005392-199806000-00142
Barst, R. J., Ivy, D. D., Gaitan, G., Szatmari, A., Rudzinski, A., Garcia, A. E., et al. (2012). A randomized, double-blind, placebo-controlled, dose-ranging study of oral sildenafil citrate in treatment-naive children with pulmonary arterial hypertension. Circulation 125, 324–334. doi:10.1161/CIRCULATIONAHA.110.016667
Coppola, P., Kerwash, E., and Cole, S. (2021). Physiologically based pharmacokinetics model in pregnancy: A regulatory perspective on model evaluation. Front. Pediatr. 9, 687978. doi:10.3389/fped.2021.687978
Dallmann, A., Ince, I., Coboeken, K., Eissing, T., and Hempel, G. (2018). A physiologically based pharmacokinetic model for pregnant women to predict the pharmacokinetics of drugs metabolized via several enzymatic pathways. Clin. Pharmacokinet. 57, 749–768. doi:10.1007/s40262-017-0594-5
Darwich, A. S., Polasek, T. M., Aronson, J. K., Ogungbenro, K., Wright, D. F. B., Achour, B., et al. (2006). Model-informed precision dosing: Background, requirements, validation, implementation, and forward trajectory of individualizing drug therapy. Annu. Rev. Pharmacol. Toxicol. Wales 61, 225–245. doi:10.1146/annurev-pharmtox-033020-113257
Dastjerdi, M. V., Hosseini, S., and Bayani, L. (2012). Sildenafil citrate and uteroplacental perfusion in fetal growth restriction. J. Res. Med. Sci. 17, 632–636.
de Bie, F. R., Basurto, D., Kumar, S., Deprest, J., and Russo, F. M. (2022). Sildenafil during the 2nd and 3rd trimester of pregnancy: Trials and tribulations. Int. J. Environ. Res. Public Health 19, 11207. doi:10.3390/ijerph191811207
de Bie, F. R., Russo, F. M., van Brantegem, P., Coons, B. E., Moon, J. K., Yang, Z., et al. (2021a). Pharmacokinetics and pharmacodynamics of sildenafil in fetal lambs on extracorporeal support. Biomed. Pharmacother. 143, 112161. doi:10.1016/j.biopha.2021.112161
de Bie, F. R., Sharma, D., Lannoy, D., Allegaert, K., Storme, L., Deprest, J., et al. (2021b). Transplacental transfer and fetal pharmacodynamics of sildenafil in the pregnant sheep model. Fetal Diagn Ther. 48, 411–420. doi:10.1159/000515435
Deprest, J., Brady, P., Nicolaides, K., Benachi, A., Berg, C., Vermeesch, J., et al. (2014). Prenatal management of the fetus with isolated congenital diaphragmatic hernia in the era of the TOTAL trial. Semin. Fetal Neonatal Med. 19, 338–348. doi:10.1016/j.siny.2014.09.006
European Medicines Agency (2018). EMA guideline on the reporting of physiologically based pharmacokinetic (pbpk) modelling and simulation. EMA/CHMP/458101/2016. London: European Medicines Agency.
European Medicines Agency (2005). Revatio (Sildenafil): Scientifc Discussion [online]. Available at: https://www.ema.europa.eu/en/documents/scientific-discussion/revatio-epar-scientific-discussion_en.pdf (Cited October 7, 2002)
Ghofrani, H. A., Osterloh, I. H., and Grimminger, F. (2006). Sildenafil: From angina to erectile dysfunction to pulmonary hypertension and beyond. Nat. Rev. Drug Discov. 5, 689–702. doi:10.1038/nrd2030
Groom, K. M., Ganzevoort, W., Alfirevic, Z., Lim, K., and Papageorghiou, A. T.STRIDER Consortium (2018). Clinicians should stop prescribing sildenafil for fetal growth restriction (FGR): Comment from the STRIDER consortium. Ultrasound Obstetrics Gynecol. 52, 295–296. doi:10.1002/uog.19186
Hawkes, N. (2018). Trial of Viagra for fetal growth restriction is halted after baby deaths. BMJ 362, k3247. doi:10.1136/bmj.k3247
Hebert, M. F., Easterling, T. R., Kirby, B., Carr, D. B., Buchanan, M. L., Rutherford, T., et al. (2008). Effects of pregnancy on CYP3A and P-glycoprotein activities as measured by disposition of midazolam and digoxin: A university of Washington specialized center of research study. Clin. Pharmacol. Ther. 84, 248–253. doi:10.1038/clpt.2008.1
Hoeben, E., Annaert, P., van Calsteren, K., Conings, S., Allegaert, K., Deprest, J., et al. 2018, Modeling the transfer of sildenafil across the placenta with data of an ex-vivo human cotyledon perfusion experiment.
Hyland, R., Roe, E. G. H., Jones, B. C., and Smith, D. A. (2001). Identification of the cytochrome P450 enzymes involved in the N -demethylation of sildenafil. Br. J. Clin. Pharmacol. 51, 239–248. doi:10.1046/j.1365-2125.2001.00318.x
Jori, G., Reddi, E., and Rubaltelli, F. F. (2009). Structural and functional differences between fetal and adult serum albumin. Int. J. Pept. Protein Res. 31, 17–21. doi:10.1111/j.1399-3011.1988.tb00002.x
Kang, J.-S., and Lee, M.-H. (2009). Overview of therapeutic drug monitoring. Korean J. Intern Med. 24, 1–10. doi:10.3904/kjim.2009.24.1.1
Kashyap, A. J., Dekoninck, P. L. J., Rodgers, K. A., Thio, M., Mcgillick, E. V., Amberg, B. J., et al. (2019). Antenatal sildenafil treatment improves neonatal pulmonary hemodynamics and gas exchange in lambs with diaphragmatic hernia. Ultrasound Obstetrics Gynecol. 54, 506–516. doi:10.1002/uog.20415
Kuemmel, C., Yang, Y., Zhang, X., Florian, J., Zhu, H., Tegenge, M., et al. (2020). Consideration of a credibility assessment framework in model-informed drug development: Potential application to physiologically-based pharmacokinetic modeling and simulation. CPT Pharmacometrics Syst. Pharmacol. 9, 21–28. doi:10.1002/psp4.12479
Kuepfer, L., Niederalt, C., Wendl, T., Schlender, J., Willmann, S., Lippert, J., et al. (2016). Applied concepts in PBPK modeling: How to build a PBPK/PD model. CPT Pharmacometrics Syst. Pharmacol. 5, 516–531. doi:10.1002/psp4.12134
Luong, C., Rey-Perra, J., Vadivel, A., Gilmour, G., Sauve, Y., Koonen, D., et al. (2011). Antenatal sildenafil treatment attenuates pulmonary hypertension in experimental congenital diaphragmatic hernia. Circulation 123, 2120–2131. doi:10.1161/CIRCULATIONAHA.108.845909
Milligan, P. A., Marshall, S. F., and Karlsson, M. O. (2002). A population pharmacokinetic analysis of sildena®l citrate in patients with erectile dysfunction. Br. J. Clin. Pharmacol. 53, 45S–52S. doi:10.1046/j.0306-5251.2001.00032.x
Muirhead, G. J., Rance, D. J., Walker, D. K., and Wastall, P. (2002). Comparative human pharmacokinetics and metabolism of single-dose oral and intravenous sildenafil. Br. J. Clin. Pharmacol. 53, 13S–20S. doi:10.1046/j.06-5251.2001.00028.x
Nichols, D. J., Muirhead, G. J., and Harness, J. A. (2002). Pharmacokinetics of sildenafil after single oral doses in healthy male subjects: Absolute bioavailability, food effects and dose proportionality. Br. J. Clin. Pharmacol. 53, 5S–12S. doi:10.1046/j.0306-5251.2001.00027.x
Pels, A., Derks, J., Elvan-Taspinar, A., van Drongelen, J., de Boer, M., Duvekot, H., et al. (2020). Maternal sildenafil vs placebo in pregnant women with severe early-onset fetal growth restriction: A randomized clinical trial. JAMA Netw. Open 3, e205323. doi:10.1001/jamanetworkopen.2020.5323
Ronique Gobry, V. Â., Raldine Bouchard, Â., Carrupt, P.-A., Testa, B., and Girault, H. H. (2000). Physicochemical characterization of sildenafil: Ionization, lipophilicity behavior, and ionic-partition diagram studied by two-phase titration and electrochemistry. Helvetica, 83, 1465–1474. doi:10.1002/1522-2675(20000705)83:7<1465:AID-HLCA1465>3.0.CO;2-%23
Russo, F. M., Conings, S., Allegaert, K., van Mieghem, T., Toelen, J., van Calsteren, K., et al. (2018). Sildenafil crosses the placenta at therapeutic levels in a dually perfused human cotyledon model. Am. J. Obstet. Gynecol. 219, 619.e1–619. doi:10.1016/j.ajog.2018.08.041
Russo, F. M., de Bie, F., Hodges, R., Flake, A., and Deprest, J. (2019a). Sildenafil for antenatal treatment of congenital diaphragmatic hernia: From bench to bedside. Curr. Pharm. Des. 25, 601–608. doi:10.2174/1381612825666190320151856
Russo, F. M., de Bie, F. R., Partridge, E. A., Allegaert, K., and Deprest, J. (2022). The antenatal sildenafil STRIDER trial for severe fetal growth restriction, are post hoc reflections ad rem? Eur. J. Pediatr. 181, 3775–3776. doi:10.1007/s00431-022-04569-7
Russo, F. M., Hooper, S., Tibboel, D., DeKoninck, P., Benachi, A., Tréluyer, J. M., et al. (2019b). Antenatal therapy with sildenafil: don’t throw the baby out with the bathwater. Ultrasound Obstetrics Gynecol. 53, 274–275. doi:10.1002/uog.20201
Russo, F. M., Toelen, J., Eastwood, M. P., Jimenez, J., Miyague, A. H., Velde, G. vande, et al. (2016). Transplacental sildenafil rescues lung abnormalities in the rabbit model of diaphragmatic hernia. Thorax 71, 517–525. doi:10.1136/thoraxjnl-2015-207949
Samangaya, R. A., Mires, G., Shennan, A., Skillern, L., Howe, D., McLeod, A., et al. (2009). A randomised, double-blinded, placebo-controlled study of the phosphodiesterase type 5 inhibitor sildenafil for the treatment of preeclampsia. Hypertens. Pregnancy 28, 369–382. doi:10.3109/10641950802601278
Silva, L. L., Silvola, R. M., Haas, D. M., and Quinney, S. K. (2022). Physiologically based pharmacokinetic modelling in pregnancy: Model reproducibility and external validation. Br. J. Clin. Pharmacol. 88, 1441–1451. doi:10.1111/bcp.15018
Szeto, K. X., le Merdy, M., Dupont, B., Bolger, M. B., and Lukacova, V. (2021). PBPK modeling approach to predict the behavior of drugs cleared by kidney in pregnant subjects and fetus. AAPS J. 23, 89. doi:10.1208/s12248-021-00603-y
Turner, J., Dunn, L., Tarnow-Mordi, W., Flatley, C., Flenady, V., and Kumar, S. (2020). Safety and efficacy of sildenafil citrate to reduce operative birth for intrapartum fetal compromise at term: A phase 2 randomized controlled trial. Am. J. Obstetrics Gynecol. 222, 401–414. doi:10.1016/j.ajog.2020.01.025
Walker, D. K., Ackland, M. J., James, G. C., Muirhead, G. J., Rance, D. J., Wastall, P., et al. (1999). Pharmacokinetics and metabolism of sildenafil in mouse, rat, rabbit, dog and man. Xenobiotica 29, 297–310. doi:10.1080/004982599238687
Wang, Y., Zhu, H., Madabushi, R., Liu, Q., Huang, S. M., and Zineh, I. (2019). Model-informed drug development: Current US regulatory practice and future considerations. Clin. Pharmacol. Ther. 105, 899–911. doi:10.1002/cpt.1363
Xu, M., Zheng, L., Zeng, J., Xu, W., Jiang, X., and Wang, L. (2021). Physiologically based pharmacokinetic modeling of tramadol to inform dose adjustment and drug-drug interactions according to CYP2D6 phenotypes. Pharmacother. J. Hum. Pharmacol. Drug Ther. 41, 277–290. doi:10.1002/phar.2494
Keywords: physiologically-based pharmacokinetic modelling, PBPK, model informed drug development, MIDD, sildenafil, revatio, congenital diaphragmatic hernia, pregnant women
Citation: Macente J, Nauwelaerts N, Russo FM, Deprest J, Allegaert K, Lammens B, Hernandes Bonan R, Turner JM, Kumar S, Diniz A, Martins FS and Annaert P (2023) PBPK-based dose finding for sildenafil in pregnant women for antenatal treatment of congenital diaphragmatic hernia. Front. Pharmacol. 14:1068153. doi: 10.3389/fphar.2023.1068153
Received: 12 October 2022; Accepted: 20 February 2023;
Published: 14 March 2023.
Edited by:
Jiao Zheng, Shanghai Jiao Tong University, ChinaReviewed by:
Xuben Yu, First Affiliated Hospital of Wenzhou Medical University, ChinaCopyright © 2023 Macente, Nauwelaerts, Russo, Deprest, Allegaert, Lammens, Hernandes Bonan, Turner, Kumar, Diniz, Martins and Annaert. This is an open-access article distributed under the terms of the Creative Commons Attribution License (CC BY). The use, distribution or reproduction in other forums is permitted, provided the original author(s) and the copyright owner(s) are credited and that the original publication in this journal is cited, in accordance with accepted academic practice. No use, distribution or reproduction is permitted which does not comply with these terms.
*Correspondence: Pieter Annaert, cGlldGVyLmFubmFlcnRAa3VsZXV2ZW4uYmU=
Disclaimer: All claims expressed in this article are solely those of the authors and do not necessarily represent those of their affiliated organizations, or those of the publisher, the editors and the reviewers. Any product that may be evaluated in this article or claim that may be made by its manufacturer is not guaranteed or endorsed by the publisher.
Research integrity at Frontiers
Learn more about the work of our research integrity team to safeguard the quality of each article we publish.