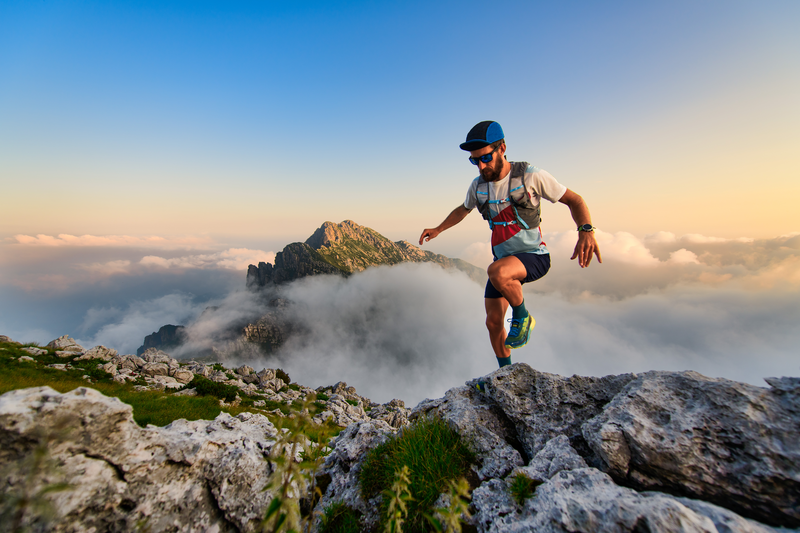
94% of researchers rate our articles as excellent or good
Learn more about the work of our research integrity team to safeguard the quality of each article we publish.
Find out more
REVIEW article
Front. Pharmacol. , 20 March 2023
Sec. Inflammation Pharmacology
Volume 14 - 2023 | https://doi.org/10.3389/fphar.2023.1057144
Ischemia-reperfusion injury (IRI) is an inevitable pathological process after organic transplantations. Although traditional treatments restore the blood supply of ischemic organs, the damage caused by IRI is always ignored. Therefore, the ideal and effective therapeutic strategy to mitigate IRI is warrented. Curcumin is a type of polyphenols, processing such properties as anti-oxidative stress, anti-inflammation and anti-apoptosis. However, although many researches have been confirmed that curcumin can exert great effects on the mitigation of IRI, there are still some controversies about its underlying mechanisms among these researches. Thus, this review is to summarize the protective role of curcumin against IRI as well as the controversies of current researches, so as to clarify its underlying mechanisms clearly and provide clinicians a novel idea of the therapy for IRI.
IRI is one of the most important clincial issues that limits the development of organic transplantations and tissue repair. Although it is critical to restore the blood supply of ischemic tissuses promptly, therapies targeting on the exact mechanisms of IRI-induced damage could make further improvements on the clinical efficacy.
During ischemia phase, intracellular mitochondrial oxidative phosphorylation induces the anaerobic metabolism, acidification and ion channels dysfunctions (Sarighieh et al., 2020). Subsequently, intracellular Ca2+ overload and abnormal hyperosmosis both occur and thus lead to the cell injury (Wu et al., 2018). During reperfusion phase, the excessive activation of oxidative stress and inflammation as well as Ca2+ overload seriously impair the structural integrity of outer membranes of mitochondia, which leads to the opening of the mitochondrial permeability transition pore (mPTP), resulting in the release of cytochrome C into cytoplasm and thus triggers apoptosis (Bonora et al., 2022; Fedotcheva et al., 2022). Simultaneously, the excessive free radicals leads to the lipid peroxidation of membranous structure, impairment of the structures and functions of proteins and nucleic acid, causing cell injury (Daverey et al., 2020).
Curcumin is isolated from turmeric and its molecular formula is C21H20O6, of which the main chain is unsaturated fat and aromatic family groups (Daverey et al., 2020). Compared with other types of Traditional Chinese Medicine, curcumin has a huge advantage because it processes several biological properties such as scavenging free radicals, anti-inflammation, anti-apoptosis, etc., (Pang et al., 2022). Recently, it has been accepted that curcumin can alleviate cell injury during ischemic phase and suppress the excessive oxidative stress and inflammation during reperfusion phase (Figure 1) (Table 1). However, there is still some controversies over the mechanisms of the suppression of curcumin on cell death.
Current therapeutic approaches are various and its foundations are alleviating ischemia and restoring reperfusion (Mohamadian et al., 2022). Therapeutic approaches can be divided into surgery, physiotherapy and pharmacology.
With the development of microsurgical techniques, surgery has promoted the prognosis of IRI. Removing thrombus, establishing collateral circulation and anastomosing broken blood vessels are important in alleviating ischemia and restoring reperfusion (Ferreira et al., 2022). For example, percutaneous coronary intervention (PCI) is applied to treat myocardial IRI. And percutaneous transluminal angioplasty (PTA), endarterectomy and bypass operation are applied for the treatments of organic IRI caused by vascular diseases.
Mild hypothermia and hyperbaric oxygen are important physiotherapeutic methods for IRI. The main functions of mild hypothermia include decreasing the metabolism and oxygen consumption of organs, alleviating vasopermeability and microcirculation. In clinics, mild hypothermia has been used for the treatments of myocardial IRI and cerebral IRI (Micó-Carnero et al., 2022).
Hyperbaric oxygen therapy refers to the inhalation of pure oxygen under more than one atmosphere, which can increase the blood oxygen concentration and oxygen reserve of tissues, promote the self-update and viability of cells (Neheman et al., 2020). For instance, hyperbaric oxygen therapy is a necessary method for cerebral IRI and chronic wounds.
Types of pharmacological therapies for IRI are various and can be divided into antioxidant and anti-inflammatory agents (Gao et al., 2022). Antioxidant agents can fight against oxidative stress by eliminating free radicals or consuming materials which can produce free radicals, which can be divided into composite and endogenous types. For example, composite antioxidative agents include edaravone, metformin, quercetin, probucol and α-thioctic acid et al. Endogenous antioxidative agents include melatonin, vitamin B, vitamin C, vitamin E and superoxide dismutases et al. Anti-inflammatory agents mainly include leucocyte inhibitors, interleukin-1 (IL-1) inhibitors (prednison, prednisolone and dexamethasone), TNF-α inhibitors, ICAM-1 inhibitors and cyclooxygenase 2 (COX2) inhibitors (Non-steroidal Anti-inflammatory Drugs, NSAIDs).
Surgery has two main disadvantages: expensive and traumatic, so that it is not suitable for elderly patients. Physiotherapy needs professional medical equipments and it usually acts affiliated role in treating IRI. Although pharmacological therapies are various, combinating different mechanisms drugs can exert the best therapeutic effectiveness and some of these durgs have serious side effects (Mohamadian et al., 2022). However, as a classic and popular type of TCM, curcumin has gained a great attention for its multiple mechanisms such as antioxidant, anti-inflammatory, suppressing Ca2+ overload and mediating autophagy et al. Therefore, due to its distinctive and various pharmacological properties, curcumin might be the promosing candidate for the treatment of IRI.
Ischemia phase, the first stage of IRI and ischemia, not only poses direct damage on normal cells, but also lays the foundation for the pathological changes induced by reperfusion injury. During ischemia phase, both the excessive upregulation of hypoxia-inducible factor-1α (HIF-1α) and anaerobic metabolism play significant roles in direct cell injury posed by ischemia. However, multitudes of researches have demonstrated that curcumin can attenuate ischemia-induced cell injury through mediating the mechanisms mentioned above.
Curcumin is able to suppress the excessive upregulation of HIF-1α, mitigating cell injury caused by ischemia. The foremost change of ischemia is hypoxia which can stimulate the stable expression of HIF-1α, leading to the upregulation and nuclear translocation of HIF-1α (Ding et al., 2022). Subsequently, in nucleoli, HIF-1α combines with hypoxia response elements (HRE) located in the promoter region of hypoxia response genes (HRG), which promotes vascular endothelial growth factor (VEGF) and erythropoietin (EPO) expressions, enhancing the ischemia tolerance (Zhao et al., 2020). However, excessive upregulation of HIF-1α contributes to the expressions of several pro-apoptosis genes such as cysteinyl aspartate specific proteinase 3 (Caspase-3) and BCL2-Associated X Protein (Bax), resulting in apoptosis induced by hypoxia and ischemia (Wang X. et al., 2020). Furthermore, several researches had confirmed that curcumin could mitigate IRI through suppressing the excessive upregulation of HIF-1α. Moulin et al. (2020) found that curcumin attenuated myocardial IRI through suppressing IRI-induced HIF-1 activation in mice. Wang et al. (2019) found that curcumin pretreatment (10 μM) could increase the survival rate of bone derived stromal cells (BMSCs) under IRI condition partly through the induction of HIF-1α destabilization.
In addition, a train of researches has manisfested that during ischemia phase, the suppression of prolyl hydroxylase (PHD) can contribute to the reduction of cellular oxygen consumption and the promotion of cellular ischemia tolerance. Huan et al. (2020) confirmed that the knockdown of PHD1 gene could promote the tolerance of skeletal muscle in mice against ischemia and hypoxia. However, researches investigating curcumin and PHD suppression during ischemia phase are still blank. Therefore, fulfilling this gap is helpful for the comprehensive elucidation of the machanisms of curcumin alleviating IRI.
Oxygen deficiency induces the anaerobic metabolism which causes the perturbation of intracellular homeostasis, leading to cell destruction and death (Nguyen et al., 2022). When anaerobic metabolism occurs, there are some corresponding changes in cells including decreasing levels of ATP and PH, increasing lactate concentration and ion channels dysfunctions, resulting in intracellular abnormal acidification and hyperosmosis (Versluis et al., 2022). A series of researches had confirmed that curcumin could suppress anaerobic metabolism, maintaining intracellular homeostasis. Wang K. et al. (2015) showed that curcumin could block anaerobic metabolism and induce mitochondrial-mediated apoptosis in two different lines of human colorectal cancer cells (HCT116 and HT29 cells) in a concentration-dependent manner through the downregulation of the activity of hexokinaseⅡ(HK Ⅱ), a key enzyme of anaerobic metabolism. In vivo, (Singla et al., 2010) also showed that curcumin co-administered with N-Methyl N-Nitrosourea (MNU, one of the potent neuro-carcinogens) could significantly reduce the activity of HK both in the cerebrum and cerebellum of mice.
Curcumin can suppress the excessive oxidative stress induced by IRI effectively. High free radicals levels and low antioxidase activities are characteristic of oxidative stress (Jelic et al., 2021). And its key mechanisms include mitochondrial injury, the aggregation and activation of a huge amount of neutrophils, increase in xanthine oxidase production as well as catecholamin oxidation (Lai et al., 2022). Curcumin can suppress IRI-induced oxidative stress by reducing free radicals levels and enhancing antioxidase activities during reperfusion phase.
Curcumin is a type of effective anti-inflammation agents. The unstable chemical property of free radicals can lead to the lipid peroxidation and destruction of the structures and functions of proteins as well nucleic acid, aggravating organic IRI (Peker et al., 2019). Reactive Oxygen Species (ROS) is the main component of free radicals, including superoxide anion (O−2), nitric oxide (NO), hydrogen peroxide (H2O2), etc. In vitro, (Zhu et al., 2019) demonstrated that curcumin could protected H9C2 cardiomyocytes against IRI, reversing the IRI-induced increases in ROS and malondialdehyde (MDA) levels. Bayrak et al. (2008) demonstrated that the oral adminstration of curcumin greatly alleviated renal IRI of rats via its inhibition of MDA and NO levels. In vivo, (Wicha et al., 2017) demonstrated that treatment with hexahydrocurcumin offered its neuroprotection against IRI in rats through significantly decreasing the levels of MDA and NO of damaged brain tissues. Otherwise, in vivo, a series of researches had confirmed that curcumin and its demethoxy derivatives such as demethoxycurcumin (Dmc), bisdemethoxycurcumin (Bdmc), tetrahydrocurcumin (THC), hexahydrocurcumin (HHC) and octahydrocurcumin (OHC) could eliminate 1,1-diphenyl-2-trinitrophenylhydrazine (DPPH), NO, hydroxy radical (OH·) and O−2 directly by providing electrons to reduce free radicals (Shaikh et al., 2021; Zhu et al., 2022; Shahbazizadeh et al., 2021). Taken together, curcumin can reduce free radical levels so as to mitigate IRI.
Curcumin can change the structures of many types of antioxidase such assuperoxide dismutases (SOD), catalase, glutathione reductase (GR) and glutathione peroxidase (GPx) and enhance its expressions and activities. On one hand, (Li et al., 2022). found that chronic curcumin treatment on cerebral IRI in rats could reduce neurological scores and inhibit IRI-induced apoptosis by enhancing enzyme activities of SOD, catalase and GR. Ilyas et al. (2016) also found that curcumin exerted its protective role against myocardial IRI in pigs by increasing enzyme activities of GR and GPx. Shen et al. (2007). demonstrated that curcumin could ameliorate warm hepatic IRI and inhibit hepatocyte apoptosis in rats through inducing the over expression of SOD and catalase. On the other hand, curcumin can increase antioxidase activities via altering its structures. Mofidi Najjar et al. (2017) showed that various concentrations of curcumin could increase the amount of α-helix content of catalase, which played an important role in the enhancement of the enzyme activity of catalase. Afterward, they also found that curcumin supplement resulted in the decreases in accessible surface area (ASA) and pKa of catalase, leading to the enhancement of the enzyme activity of bovine liver catalase (BLC) (Khataee et al., 2019). Therefore, curcumin can enhance the enzyme activities of various types of antioxidase, mitigating the excessive oxidative stress during reperfusion phase.
Curcumin can suppress over-activated inflammation during reperfusion phase. IRI increases the percentage of inflammatory cells infiltration. Take myocardial IRI of dogs for an example, the percentage of neutrophils in endocardium increases for about 25% only after reperfusion for 5 min. Inflammatory cells such as neutrophils, monocyte-macrophages and mast cells can harm normal cells through the direct damage or secreting inflammatory mediators (Hassanzadeh et al., 2022). Taken together, curcumin can suppress inflammatory cells infiltration and decrease inflammatory mediators levels, so as to suppress the over-activated inflammation induced by IRI during reperfusion injury.
Cell adhesion molecules mainly includes integrin, selectin, ICAM-1 and vascular adhesion molecule-1 (VCAM-1), which can mediate inflammatory cells infiltration (Sawada et al., 2020). Evidences showing that curcumin can decrease the level of TNF-α-induced VCAM-1 in vascular endothelial cells and the level of ICAM-1 in aneurysmal walls (Huyut et al., 2022; Fan et al., 2019). Otherwise, (Montiel-Dávalos et al., 2017) showed that air pollutants such as inhalable particles with an aerodynamic diameter of ≤10 μm (PM10) and titanium dioxide nanoparticles (TiO2-NPs) could induce the dysfunctions and abnormal activation of endothelial cells, which led to the over expressions of ICAM-1, VCAM-1, E-selectin and P-selectin, causing the excessive inflammatory cells infiltration and damage in the endothelial cells. And their results manifested that curcumin had an anti-inflammatory role by attenuating the dysfunctions and abnormal activation of endothelial cells by the exposures to PM10 and TiO2-NPs.
Curcumin can decrease the abnormally high contents of multiple types of chemokines induced by IRI. In IRI, the excessive inflammatory reactions are over activated, which leads to the release of a huge amount of chemokines, contributing to inflammatory cells infiltration. Jia et al., (2014) showed that curcumin treatment could lower IL-8 level in rabbit ear IRI model. (Wang et al., 2011; Kim et al., 2012) showed that curcumin decreased monocyte chemotatic protein-1 (MCP-1) level in retinal IRI and myocardial IRI respectively. In addition, (Nguyen-Ngo et al., 2020) also found that curcumin significantly suppressed TNF-induced such chemokines as C–C motif ligand 2 (CCL2), CCL3, CCL4, C-X-C motif ligand 1 (CXCL1), CXCL5 and CXCL8 expressions in human placenta, visceral adipose tissue and subcutaneous adipose tissue. Therefore, researches had confirmed both in vitro and vivo that curcumin could inhibit the release of a huge amount of chemokines, mitigating inflammatory cells infiltration.
During ischemia phase, ligands such as high mobility group box 1 (HMGB1) and RNA are significantly released by necrotic cells, which are combined by Toll-like receptors (TLRs) (Upadhyay et al., 2022). Subsequently, the excessive activation of TLRs translocates the nuclear transcription factors into nucleoli through the Myeloid Differentiation Factor 88 (MyD88) dependent signaling pathway, which produces pro-inflammatory cytokines and chemokines, inducing cascade inflammatory reactions (Zhang et al., 2021). Zou et al. (2020) showed that curcumin post-treatment could suppress TLR4 expression in the injuried lung tissues induced by limb ischaemia-reperfusion in rats. Zhang et al. (2018) also confirmed that curcumin supplementation suppressed TLR4 expression and macrophage infiltration in atherosclerosis plaque as well as protected against atherosclerosis in ApoE−/− mice. Additionally, (Wang Y. et al., 2015) demonstrated that in mice sepsis models, curcumin analog L48H37 could inhibit lipopolysaccharide-induced TLR4 signaling pathway activation so that led to the decrease of downstream inflammatory mediators expression.
Vasoactive amines such as histamine and 5-hydroxytryptamine (5-HT) are released earliest during the inflammatory reactions, which can be suppressed by curcumin (Chong et al., 2022). Histamine mainly exists in the granules of mast cells and basophilic granulocytes, increasing vascular permeability through the combination with histamine 1 receptors in vascular endothelial cells (Matsumoto et al., 2021; Midzyanovskaya et al., 2021). In vitro, several researches had all confirmed that curcumin could inhibit the activation and degranulation of mast cells during reperfusion phase, which led to the suppression of histamine release, resulting in the decrease of vascular permeability (Nabil et al., 2018; Lee et al., 2018; Kong D. et al., 2020). 5-HT mainly exists in platelets, of which the release leads to the vasoconstriction (Krege et al., 2022). Arbabi Jahan et al. (2018) demonstrated that curcumin could reduce 5-HT 7 gene expression. Also, (Mohammed et al., 2020) showed that curcumin nanoparticles exerted its protective effect on the cardiotoxicity induced by doxorubicin via the suppression of abnormal vasoconstriction through inhibiting platelet aggregation and 5-HT release. Taken together, the protective role of curcumin in IRI partly contributes to the reductions of vasoactive amines contents and the mitigation of abnormal vascular changes.
The metabolite of arachidonic acid (AA) includes prostaglandin, leukotriene (LT) and lipoxin, which are important inflammatory mediators participating in inflammation and coagulation responses (Yamaguchi et al., 2022). Dileep et al. (2011) found that curcumin and its analogs suppressed AA release via inhibiting the enzyme activity of phospholipase A2 (PLA2) in that PLA2 contributed to AA release by hydrolyzing membrane phospholipids. In addition, curcumin can block the synthesis of AA and its metabolite by suppressing the cyclooxygenase 2 (COX2) and lipoxygenase (LOX) (Kocaadam et al., 2017). Therefore, curcumin can alleviate IRI-induced inflammatory reactions through the reductions of AA and its metabolite.
TNF-α, IL-1β and IL-6 play significant roles in the initiation and promotion of inflammation, produced by activated macrophages, mast cells and endothelial cells (Oettgen, 2022; Xiao et al., 2022). When IRI occurs, as strong extracellular stimuli, ischemia, oxygen deficiency and oxidative stress can initiate the transcriptions and expressions of pro-inflammatory factors (TNF-α, IL-1β and IL-6) through the upregulation of intracellular nuclear factor κB (NF-κB) and Notch signaling pathways (Li et al., 2017; Fan et al., 2014). In turn, these types of pro-inflammatory factors also can upregulate intracellular NF-κB and Notch signaling pathways, and thus forms vicious “positive feedback” (Karatepe et al., 2009; Christopoulos et al., 2021). On one hand, (Xu et al., 2019). found that curcumin inhibited oxygen glucose deprivation/reperfusion (OGD/R)-induced cell damage by downregulating RelA p65, an important subunit of NF-κB. Also, (Liu et al., 2018) showed that curcumin exerted positive effects on hepatic IRI in mice through activating peroxisome proliferator-activated receptor γ (PPAR γ) by the downregulation of NF-κB signaling pathway. On the other hand, (Bo et al., 2020) demonstrated that curcumin post-treatment alleviated lung IRI in rats via the inhibition of Notch2/Hes-1 signaling pathway and the releases of pro-inflammatory factors (TNF-α and IL-1β). Taken together, curcumin treatment is related to the inhibition of pro-inflammatory releases through the regulation of inflammation-associated signaling pathways.
Ca2+ overload plays an important role in the pathogenesis of IRI and it mainly occurs during reperfusion phase, of which the principal causes are the increased calcium influx, abnormal activation of protein kinase C (PKC) as well as cell membranes damage (Kashio et al., 2022; Wang et al., 2022). However, curcumin can inhibit IRI-induced Ca2+ overload through targeting the mechanisms mentioned above.
Increased Ca2+ influx greatly contributes to IRI-induced Ca2+ overload. During ischemia phase, intracellular anaerobic metabolism and energy deficiency both lead to the reduction of Na+ pump activity, resulting in the increase of intracellular Na+ content. During reperfusion phase, ischemic cells recover the supply of oxygen and nutritious materials and high intracellular Na+ content immediately activates Na+/Ca2+ exchangers, which causes the excessive Ca2+ influx, leading to the Ca2+ overload and cell injury (Junho et al., 2022). In vitro, (Wang Z. et al., 2020) found that curcumin protected against cerebral IRI in rats through the blockage of excessive Ca2+ influx by inhibiting P2X7 receptor activation, a one of the conduits for Ca2+ influx in dendric cells. Nazıroğlu et al. (2019) confirmed that curcumin treatment could block the increased Ca2+ influx in renal collecting duct cells through the downregulation of transient receptor potential M2 (TRPM2) channel which mediated oxidative stress-induced Ca2+ influx. Moreover, in vivo, (Daverey et al., 2020) also showed that curcumin mediated its protective role in spinal cord white matter hypoxia of rats through extracellular inhibition of calcium channels as well as intracellular inhibition of Ca2+. In turn, curcumin treatment can attenuate IRI-induced Ca2+ overload by blocking increased Ca2+ influx.
Increased endogenous catecholamin release induced by IRI contributes to PKC activation and activated PKC promotes Na+/Ca2+ exchange and Ca2+ influx. Furthermore, (Chen et al., 1998) established cerebral IRI models in rats finding that the activated PKC aggravated cerebral IRI because it could lead to vasoconstriction and degenerate cytoskeleton components. Fan et al. (2019) showed that PKC β inhibitor could mitigate inflammatory cells infiltration in renal IRI tissues and promote the expression of alternatively activated macrophage (M2), a type of macrophages processing anti-inflammatory effects. To the best of our knowledge, several researches had confirmed that curcumin could suppress PKC activation. Badr et al. (2018) confirmed that curcumin analogue J1 blunted the phosphorylation of PKC-theta in the breast cancer cells. Liu et al. (1993) showed that treatment with 15 or 20 μm curcumin for 15 min could inhibit 12-0-tetradecanoyl-phorbol-13-acetate (TPA)-induced PKC activity in mice fibroblast cells. Haryuna et al. (2019) also found that intraperitoneal administration with curcumin for 3 or 8 days could reduce PKC expression in the cochlear fibroblasts of diabetic rats.
Cell membranes are significant structures for maintaining the ionic equilibrium between the intracellular and extracellular. The destruction of cell membranes increases its permeability which causes Ca2+ influx following the concentrationgradient, greatly contributing to Ca2+ overload. Chakraborti et al. (2018) found that curcumin could alleviate ROS-induced damage on cell membranes by suppressing the phospholipase D (PLD) activity in pulmonary artery smooth muscle cells under oxidative stress condition. Other study also showed that curcumin could attenuate mitochondrial dysfunction and stabilize the cell membranes, reducing injury severity in adult models of spinal cord injury, cancer as well as cardiovascular disease (Rocha-Ferreira et al., 2019).
Autophagy-induced cell death, also termed as autosis, has been confirmed to exist in IRI and the regulation of autophagy plays an important role in the fate of cells that suffer with IRI (Shi et al., 2019; Cao et al., 2021) demonstrated that curcumin analogues, 7-(4-Hydroxy-3-methoxyphenyl)-1-phenyl-4E-hepten-3-one (AO-2) could alleviate OGD/R-induced damage on cortical neurons isolated from rats by inhibiting autophagy and cell apoptosis through an mTOR-dependent mechanism. On the contrary, (Yang et al., 2013) established the murine myocardial I/R model demonstrating that curcumin exerted its protective role against myocardial IRI through the upregulation of autophagy in murine cardiomyocytes. Additionally, in vitro and vivo, a series of researches had confirmed that several therapeutic agents or measure for cerebral IRI such as astragaloside IV, resveratrol and ischemic postconditioning (IPC) inhibited cerebral damage following IRI mainly through the upregulation of autophagy (Zhang et al., 2019; Sun et al., 2018; He et al., 2017). However, whether autophagy is beneficial or harmful to the IRI still remains controversial, of which the possible reasons include complicated autophagy regulation networks, differences in the durations of ischemia and reperfusion as well as different interventions, etc., (Wang X. et al., 2020; Kong D. et al., 2020). Collectively, the exact autophagy mechanisms mediating curcumin alleviating IRI still need further investigations.
Pytoptosis, also termed as inflammation-related cell death, is an important natural immune response of the body (Hu et al., 2022; Naryzhnaya et al., 2022). Although there are no researches reporting the possible relationships between pyroptosis and curcumin alleviating IRI yet, the inhibition of pyroptosis has been confirmed to mitigate IRI. Nucleotide-binding oligomerization domain-like receptor protein 3 (NLRP3) is the key protein of IRI-induced pyroptosis (Luan et al., 2022). In the development and progression of IRI, increased NLRP3 level induces violent pytoptosis, which is led by the upregulation of NF-κB signaling pathway, increased mitochondrial fragments, impaired autophagy functions and high ROS levels, etc., (Song et al., 2018; Yu et al., 2022). Therefore, a series of researches had confirmed that several types of TCM such as total glucosides of paeonia (TGP), emodin, β-asarone and gastrodin could alleviate myocardial IRI and intestinal IRI via inhibiting pyroptosis in cardiocytes and intestinal mucosal epithelial cells (Zheng et al., 2019; Ye et al., 2019; Xiao et al., 2020; Sun et al., 2019). As for curcumin treatment, (Yin et al. 2020) found that curcumin could attenuate necrotising microscopic colitis by inhibiting pyroptosis in newborn rats. Yu et al. (2020) found that curcumin could protect against doxorubicin-induced cardiac injury via suppressing pyroptosis in mice. Taken together, the inhibition of pyroptosis by curcumin might be one of the most significant mechanisms of allevating IRI and more high-qualified researches should be performed.
Ferroptosis, a new type of regulated cell death that has been discovered recently, which is induced by iron-dependent lipid peroxidation (Li H. et al., 2020; Ma et al., 2023). Currently, it has been widely accepted that it plays an importantly detrimental role in many IRI models (Yan, 2020). As mentioned above, IRI is accompanied by oxidative damage and the accumulation of ROS. Additionally, Zhao et al., (2018) and Scindia et al., (2019) both had found that IRI could cause elevated intracellular iron levels, which was induced by excessive oxidative stress, and iron overload was also a main source of oxidative stress in turn, aggravating IRI. These pathomechanisms related to IRI are main causes of ferroptosis. Elevated intracellular iron promoted lipid oxidation by Fenton reaction, contributing to the induction of ferroptosis (Hirschhorn et al., 2019). Therefore, given the relationships between ferroptosis and IRI, a series of researches had demonstrated the protective role of iron chelators against IRI models. Tuo et al. (2017) showed that tau alleviated cerebral IRI in rats by ferroptotic inhibition, of which the main mechanism was mediating stroke-induced iron accumulation and outcome. In vivo, (Fang et al., 2019) also showed that ferrostatin-1, a classical type of iron chelators, could ameliorated heart failure induced by both acute and chronic myocardial IRI in mice. In addition, (Linkermann, 2016) concluded that such iron chelators as desferoxamine, ferrostatin-1, liproxstatin and the compound 16–86 could greatly attenuate tubular cell necrosis and synchronized death of renal tubules caused by renal IRI. In vitro, (Li et al., 2018). demonstrated that supplement with liproxstatin-1 and deferoxamine could block testicular IRI-induced cell death of germ cells and Sertoli cells. To our best understanding, curcumin treatment has been reported to inhibit ferroptosis. Guerrero et al. (2019) had showed that curcumin treatment could mitigate renal damage relating to rhabdomyolysis through inhibting ferroptosis-induced cell death. Li R et al. also showed that curcumin could suppress the growth of breast cancer cells and decrease the survival rate of osteosarcoma cells by inducing ferroptosis-mediated cell death. Collectively, curcumin can be both ferroptosis inhibitor and ferroptosis trigger (Tang et al., 2021). However, whether curcumin can exert its protective role against IRI by ferroptosis-associated mechanisms should be explored further.
There are also some side effects of curcumin. For example, external application of curcumin might cause contact dermatitis and urticaria (Chen L. et al., 2022). And its possible explantation is that external application of curcumin can induce the allergic reaction of skin. Oral administration of curcumin might cause gastrointestinal pain, nausea, vomit and liver poisoning (Xie et al., 2022). Otherwise, the overdose of curcumin can aggravate the cholecystolithiasis in that curcumin is mainly metabolized by the liver, so that the excessive administration of curcumin can induce liver dysfunction. In addition, curcumin can inhibit the coagulation process so that people suffered from hemorrhagic diseases, postoperative patients and pregnant women should take curcumin with great caution (Chen et al., 2022). Futhermore, curcumin can reduce the blood pressure and blood glucose so that people who take hypotensor and hypoglycemic drugs are forbidden to take curcumin. In clinics, take as mycardial infarction patients an example. In my opinion, administration of curcumin should act as an auxiliary role in treating mycardial infarction and should be given right after the ischemic event (Li J. et al., 2020). Taken together, there is a long way from scientific to clinical.
Our review also had a few limitations. For instance, the study did not review the molecular structure of curcumin. Besides, this review did not include our own exprimental data. In addition, the characteristics of the molecular structure of curcumin and relevant experiments should be investigated in our next aticle in the future.
In conclusion, curcumin has been confirmed to alleviate IRI. During ischemia phase, curcumin can suppress the excessive upregulation of HIF-1α and anaerobic metabolism, reducing ischemia-induced injury on cells. During reperfusion phase, curcumin can inhibit the excessive oxidative stress through decreasing free radicals levels and increasing antioxidase activities. Simultaneously, curcumin can mitigate the excessive inflammation through suppressing inflammatory cells infiltration and decreasing inflammatory mediators contents. Additionally, curcumin also can inhibit intracellular Ca2+ overload by blocking the increased Ca2+ influx, suppressing PKC activation and maintaining the integerity of cell membranes.
Edited the final text: JZ; wrote the manuscript: MB and DL.
The authors declare that the research was conducted in the absence of any commercial or financial relationships that could be construed as a potential conflict of interest.
All claims expressed in this article are solely those of the authors and do not necessarily represent those of their affiliated organizations, or those of the publisher, the editors and the reviewers. Any product that may be evaluated in this article, or claim that may be made by its manufacturer, is not guaranteed or endorsed by the publisher.
Arbabi Jahan, A., Rad, A., Ghanbarabadi, M., Amin, B., and Mohammad-Zadeh, M. (2018). The role of serotonin and its receptors on the anticonvulsant effect of curcumin in pentylenetetrazol-induced seizures. Life Sci. 211, 252–260. doi:10.1016/j.lfs.2018.09.007
Badr, G., Gul, H. I., Yamali, C., Mohamed, A., Badr, B. M., Gul, M., et al. (2018). Curcumin analogue 1,5-bis(4-hydroxy-3-((4-methylpiperazin-1-yl)methyl)phenyl)penta-1,4-dien-3-one mediates growth arrest and apoptosis by targeting the PI3K/AKT/mTOR and PKC-theta signaling pathways in human breast carcinoma cells. Bioorg. Chem. 78, 46–57. doi:10.1016/j.bioorg.2018.03.006
Bayrak, O., Uz, E., Bayrak, R., Turgut, F., Atmaca, A. F., Sahin, S., et al. (2008). Curcumin protects against ischemia/reperfusion injury in rat kidneys. World J. urology 26 (3), 285–291. doi:10.1007/s00345-008-0253-4
Bo, H., and Feng, X. (2020). Post-treatment curcumin reduced ischemia-reperfusion-induced pulmonary injury via the Notch2/Hes-1 pathway. J. Int. Med. Res. 48 (4), 300060519892432. doi:10.1177/0300060519892432
Bonora, M., Giorgi, C., and Pinton, P. (2022). Molecular mechanisms and consequences of mitochondrial permeability transition. Nat. Rev. Mol. Cell. Biol. 23 (4), 266–285. doi:10.1038/s41580-021-00433-y
Cao, Y., Li, Q., Yang, Y., Ke, Z., Chen, S., Li, M., et al. (2021). Cardioprotective effect of stem-leaf saponins from Panax notoginseng on mice with sleep derivation by inhibiting abnormal autophagy through PI3K/Akt/mTOR pathway. Front. Cardiovasc Med. 8, 694219. doi:10.3389/fcvm.2021.694219
Chakraborti, S., Sarkar, J., Bhuyan, R., and Chakraborti, T. (2018). Role of curcumin in PLD activation by Arf6-cytohesin1 signaling axis in U46619-stimulated pulmonary artery smooth muscle cells. Mol. Cell. Biochem. 438 (1-2), 97–109. doi:10.1007/s11010-017-3117-7
Chen, K. N., and Dong, W. W. (1998). Changes of activity of protein kinase C during cerebral ischemia/reperfusion in rats. Acta Acad. Med. Ae Mil. Tertiae 05, 28–30.
Chen, L., Yue, B., Liu, Z., Luo, Y., Ni, L., Shen, W., et al. (2022). Preparation of curcumin-loaded cochleates: Characterisation, stability and antioxidant properties. Food & Funct. 13 (21), 11273–11282. doi:10.1039/d2fo01419j
Chong, S., Xie, Q., Ma, T., Xiang, Q., Zhou, Y., and Cui, Y. (2022). Risk of infection in roxadustat treatment for anemia in patients with chronic kidney disease: A systematic review with meta-analysis and trial sequential analysis. Front. Pharmacol. 13, 967532. doi:10.3389/fphar.2022.967532
Christopoulos, P. F., Gjølberg, T. T., Krüger, S., Haraldsen, G., Andersen, J. T., and Sundlisæter, E. (2021). Targeting the Notch signaling pathway in chronic inflammatory diseases. Front. Immunol. 12, 668207. doi:10.3389/fimmu.2021.668207
Daverey, A., and Agrawal, S. K. (2020). Neuroprotective effects of Riluzole and Curcumin in human astrocytes and spinal cord white matter hypoxia. Neurosci. Lett. 738, 135351. doi:10.1016/j.neulet.2020.135351
Dileep, K. V., Tintu, I., and Sadasivan, C. (2011). Molecular docking studies of curcumin analogs with phospholipase A2. Interdiscip. Sci. Comput. life Sci. 3 (3), 189–197. doi:10.1007/s12539-011-0090-9
Ding, J., Mei, S., Cheng, W., Ni, Z., and Yu, C. (2022). Curcumin treats endometriosis in mice by the HIF signaling pathway. Am. J. Transl. Res. 14 (4), 2184–2198.
Fan, X. M., and Rong, S. (2019). Effect of protein kinase C β inhibitor on renal ischemia-reperfusion injury and expression level of macrophage subtypes in rat models. Organ Transplant. 10 (04), 423–428.
Fan, Z., Jing, H., Yao, J., Li, Y., Hu, X., Shao, H., et al. (2014). The protective effects of curcumin on experimental acute liver lesion induced by intestinal ischemia-reperfusion through inhibiting the pathway of NF-κB in a rat model. Oxidative Med. Cell. Longev. 2014, 191624. doi:10.1155/2014/191624
Fang, X., Wang, H., Han, D., Xie, E., Yang, X., Wei, J., et al. (2019). Ferroptosis as a target for protection against cardiomyopathy. Proc. Natl. Acad. Sci. U. S. A. 116 (7), 2672–2680. doi:10.1073/pnas.1821022116
Fedotcheva, T., Shimanovsky, N., and Fedotcheva, N. (2022). Involvement of multidrug resistance modulators in the regulation of the mitochondrial permeability transition pore. Membranes 12 (9), 890. doi:10.3390/membranes12090890
Ferreira-Silva, M., Faria-Silva, C., Carvalheiro, M. C., Simões, S., Marinho, H. S., Marcelino, P., et al. (2022). Quercetin liposomal nanoformulation for ischemia and reperfusion injury treatment. Pharmaceutics 14 (1), 104. doi:10.3390/pharmaceutics14010104
Gao, Q., Deng, H., Yang, Z., Yang, Q., Zhang, Y., Yuan, X., et al. (2022). Sodium danshensu attenuates cerebral ischemia-reperfusion injury by targeting AKT1. Front. Pharmacol. 13, 946668. doi:10.3389/fphar.2022.946668
Guerrero-Hue, M., García-Caballero, C., Palomino-Antolín, A., Rubio-Navarro, A., Vázquez-Carballo, C., Herencia, C., et al. (2019). Curcumin reduces renal damage associated with rhabdomyolysis by decreasing ferroptosis-mediated cell death. FASEB J. official Publ. Fed. Am. Soc. Exp. Biol. 33 (8), 8961–8975. doi:10.1096/fj.201900077R
Haryuna, T., Farhat, F., and Indriany, S. (2019). Decreased protein kinase C expression in the cochlear fibroblasts of diabetic rat models induced by curcumin. Turkish J. Pharm. Sci. 16 (2), 169–174. doi:10.4274/tjps.galenos.2018.20981
Hassanzadeh, K., Vahabzadeh, Z., Bucarello, L., Dragotto, J., Corbo, M., Maccarone, R., et al. (2022). Protective effect of curcuma extract in an ex vivo model of retinal degeneration via antioxidant activity and targeting the SUMOylation. Oxidative Med. Cell. Longev. 2022, 8923615. doi:10.1155/2022/8923615
He, Q., Li, Z., Wang, Y., Hou, Y., Li, L., and Zhao, J. (2017). Resveratrol alleviates cerebral ischemia/reperfusion injury in rats by inhibiting NLRP3 inflammasome activation through Sirt1-dependent autophagy induction. Int. Immunopharmacol. 50, 208–215. doi:10.1016/j.intimp.2017.06.029
Hirschhorn, T., and Stockwell, B. R. (2019). The development of the concept of ferroptosis. Free Radic. Biol. Med. 133, 130–143. doi:10.1016/j.freeradbiomed.2018.09.043
Hu, Y., Wen, Q., Cai, Y., Liu, Y., Ma, W., Li, Q., et al. (2022). Alantolactone induces concurrent apoptosis and GSDME-dependent pyroptosis of anaplastic thyroid cancer through ROS mitochondria-dependent caspase pathway. Phytomedicine 108, 154528. doi:10.1016/j.phymed.2022.154528
Huan, X., Oumei, C., Hongmei, Q., Junxia, Y., Xiaojiao, M., and Qingsong, J. (2020). PDE9 inhibition promotes proliferation of neural stem cells via cGMP-PKG pathway following oxygen-glucose deprivation/reoxygenation injury in vitro. Neurochem. Int. 133, 104630. doi:10.1016/j.neuint.2019.104630
Huyut, Z., Uçar, B., Altındağ, F., Yıldızhan, K., and Huyut, M. T. (2022). Effect of curcumin on lipid profile, fibrosis, and apoptosis in liver tissue in abemaciclib-administered rats. Drug Chem. Toxicol. 2022, 1–9. doi:10.1080/01480545.2022.2135007
Ilyas, E. I., Nur, B. M., Laksono, S. P., Bahtiar, A., Estuningtyas, A., Vitasyana, C., et al. (2016). Effects of curcumin on parameters of myocardial oxidative stress and of mitochondrial glutathione turnover in reoxygenation after 60 minutes of hypoxia in isolated perfused working Guinea pig hearts. Adv. Pharmacol. Sci. 2016, 6173648. doi:10.1155/2016/6173648
Jelic, M. D., Mandic, A. D., Maricic, S. M., and Srdjenovic, B. U. (2021). Oxidative stress and its role in cancer. J. cancer Res. Ther. 17 (1), 22–28. doi:10.4103/jcrt.JCRT_862_16
Jia, S., Xie, P., Hong, S. J., Galiano, R., Singer, A., Clark, R. A., et al. (2014). Intravenous curcumin efficacy on healing and scar formation in rabbit ear wounds under nonischemic, ischemic, and ischemia-reperfusion conditions. Wound repair Regen. official Publ. Wound Heal. Soc. Eur. Tissue Repair Soc. 22 (6), 730–739. doi:10.1111/wrr.12231
Junho, C., González-Lafuente, L., Neres-Santos, R. S., Navarro-García, J. A., Rodríguez-Sánchez, E., Ruiz-Hurtado, G., et al. (2022). Klotho relieves inflammation and exerts a cardioprotective effect during renal ischemia/reperfusion-induced cardiorenal syndrome. Biomed. Pharmacother. = Biomedecine Pharmacother. 153, 113515. doi:10.1016/j.biopha.2022.113515
Karatepe, O., Gulcicek, O. B., Ugurlucan, M., Adas, G., Battal, M., Kemik, A., et al. (2009). Curcumin nutrition for the prevention of mesenteric ischemia-reperfusion injury: An experimental rodent model. Transplant. Proc. 41 (9), 3611–3616. doi:10.1016/j.transproceed.2009.08.002
Kashio, M., Masubuchi, S., and Tominaga, M. (2022). Protein kinase C-mediated phosphorylation of transient receptor potential melastatin type 2 Thr738 counteracts the effect of cytosolic Ca2+ and elevates the temperature threshold. J. physiology 600 (19), 4287–4302. doi:10.1113/JP283350
Khataee, S., Dehghan, G., Rashtbari, S., Yekta, R., and Sheibani, N. (2019). Synergistic inhibition of catalase activity by food colorants sunset yellow and curcumin: An experimental and MLSD simulation approach. Chemico-biological Interact. 311, 108746. doi:10.1016/j.cbi.2019.108746
Kim, Y. S., Kwon, J. S., Cho, Y. K., Jeong, M. H., Cho, J. G., Park, J. C., et al. (2012). Curcumin reduces the cardiac ischemia-reperfusion injury: Involvement of the toll-like receptor 2 in cardiomyocytes. J. Nutr. Biochem. 23 (11), 1514–1523. doi:10.1016/j.jnutbio.2011.10.004
Kocaadam, B., and Şanlier, N. (2017). Curcumin, an active component of turmeric (Curcuma longa), and its effects on health. Crit. Rev. food Sci. Nutr. 57 (13), 2889–2895. doi:10.1080/10408398.2015.1077195
Kong D., D., Zhang, Z., Chen, L., Huang, W., Zhang, F., Wang, L., et al. (2020). Curcumin blunts epithelial-mesenchymal transition of hepatocytes to alleviate hepatic fibrosis through regulating oxidative stress and autophagy. Redox Biol. 36, 101600. doi:10.1016/j.redox.2020.101600
Kong, Z. L., Sudirman, S., Lin, H. J., and Chen, W. N. (2020). In vitro anti-inflammatory effects of curcumin on mast cell-mediated allergic responses via inhibiting FcεRI protein expression and protein kinase C delta translocation. Cytotechnology 72 (1), 81–95. doi:10.1007/s10616-019-00359-6
Krege, J. H., Lipton, R. B., Baygani, S. K., Komori, M., Ryan, S. M., and Vincent, M. (2022). Lasmiditan for patients with migraine and contraindications to triptans: A post hoc analysis. Pain Ther. 11 (2), 701–712. doi:10.1007/s40122-022-00388-8
Lai, K. H., Chen, Y. L., Lin, M. F., El-Shazly, M., Chang, Y. C., Chen, P. J., et al. (2022). Lonicerae japonicae flos attenuates neutrophilic inflammation by inhibiting oxidative stress. Antioxidants (Basel, Switz. 11 (9), 1781. doi:10.3390/antiox11091781
Lee, H. K., Park, S. B., Chang, S. Y., and Jung, S. J. (2018). Antipruritic effect of curcumin on histamine-induced itching in mice. Korean J. physiology Pharmacol. official J. Korean Physiological Soc. Korean Soc. Pharmacol. 22 (5), 547–554. doi:10.4196/kjpp.2018.22.5.547
Li, H., Sureda, A., Devkota, H. P., Pittalà, V., Barreca, D., Silva, A. S., et al. (2020). Curcumin, the golden spice in treating cardiovascular diseases. Biotechnol. Adv. 38, 107343. doi:10.1016/j.biotechadv.2019.01.010
Li, J., Cao, F., Yin, H. L., Huang, Z. J., Lin, Z. T., Mao, N., et al. (2020). Ferroptosis: Past, present and future. Cell. death Dis. 11 (2), 88. doi:10.1038/s41419-020-2298-2
Li, L., Cheng, J., Ji, Y., Liu, J., Zhai, R., and Wang, H. (2022). High-Throughput Sequencing and Bioinformatics analysis reveals the neurogenesis key targets of Curcumin action in mouse brain with MCAO. Comb. Chem. High. Throughput Screen. doi:10.2174/1386207325666220803090112
Li, L., Hao, Y., Zhao, Y., Wang, H., Zhao, X., Jiang, Y., et al. (2018). Ferroptosis is associated with oxygen-glucose deprivation/reoxygenation-induced Sertoli cell death. Int. J. Mol. Med. 41 (5), 3051–3062. doi:10.3892/ijmm.2018.3469
Li, W., Suwanwela, N. C., and Patumraj, S. (2017). Curcumin prevents reperfusion injury following ischemic stroke in rats via inhibition of NF-κB, ICAM-1, MMP-9 and caspase-3 expression. Mol. Med. Rep. 16 (4), 4710–4720. doi:10.3892/mmr.2017.7205
Linkermann, A. (2016). Nonapoptotic cell death in acute kidney injury and transplantation. Kidney Int. 89 (1), 46–57. doi:10.1016/j.kint.2015.10.008
Liu, J. Y., Lin, S. J., and Lin, J. K. (1993). Inhibitory effects of curcumin on protein kinase C activity induced by 12-O-tetradecanoyl-phorbol-13-acetate in NIH 3T3 cells. Carcinogenesis 14 (5), 857–861. doi:10.1093/carcin/14.5.857
Liu, Y., Zhang, W., Cheng, Y., Miao, C., Gong, J., and Wang, M. (2018). Activation of PPARγ by Curcumin protects mice from ischemia/reperfusion injury induced by orthotopic liver transplantation via modulating polarization of Kupffer cells. Int. Immunopharmacol. 62, 270–276. doi:10.1016/j.intimp.2018.07.013
Luan, F., Lei, Z., Peng, X., Chen, L., Peng, L., Liu, Y., et al. (2022). Cardioprotective effect of cinnamaldehyde pretreatment on ischemia/reperfusion injury via inhibiting NLRP3 inflammasome activation and gasdermin D mediated cardiomyocyte pyroptosis. Chemico-biological Interact. 368, 110245. doi:10.1016/j.cbi.2022.110245
Ma, Q., Hui, Y., Huang, B. R., Yang, B. F., Li, J. X., Fan, T. T., et al. (2023). Ferroptosis and cuproptosis prognostic signature for prediction of prognosis, immunotherapy and drug sensitivity in hepatocellular carcinoma: Development and validation based on TCGA and ICGC databases. Transl. Cancer Res. 12 (1), 46–64. doi:10.21037/tcr-22-2203
Matsumoto, N., Ebihara, M., Oishi, S., Fujimoto, Y., Okada, T., and Imamura, T. (2021). Histamine H1 receptor antagonists selectively kill cisplatin-resistant human cancer cells. Sci. Rep. 11 (1), 1492. doi:10.1038/s41598-021-81077-y
Micó-Carnero, M., Zaouali, M. A., Rojano-Alfonso, C., Maroto-Serrat, C., Ben Abdennebi, H., and Peralta, C. (2022). A potential route to reduce ischemia/reperfusion injury in organ preservation. Cells 11 (17), 2763. doi:10.3390/cells11172763
Midzyanovskaya, I. S., Petrenko, T. E., Birioukova, L. M., and Tuomisto, L. M. (2021). Reduced H3 histamine receptor binding densities in the upper layers of motor cortex in rats prone to audiogenic convulsive seizures. Epilepsy Res. 170, 106543. doi:10.1016/j.eplepsyres.2020.106543
Mofidi Najjar, F., Ghadari, R., Yousefi, R., Safari, N., Sheikhhasani, V., Sheibani, N., et al. (2017). Studies to reveal the nature of interactions between catalase and curcumin using computational methods and optical techniques. Int. J. Biol. Macromol. 95, 550–556. doi:10.1016/j.ijbiomac.2016.11.050
Mohamadian, M., Parsamanesh, N., Chiti, H., Sathyapalan, T., and Sahebkar, A. (2022). Protective effects of curcumin on ischemia/reperfusion injury. Phytother. Res. 36, 4299. doi:10.1002/ptr.7620
Mohammed, H. S., Hosny, E. N., Khadrawy, Y. A., Magdy, M., Attia, Y. S., Sayed, O. A., et al. (2020). Protective effect of curcumin nanoparticles against cardiotoxicity induced by doxorubicin in rat. Biochimica biophysica acta. Mol. basis Dis. 1866 (5), 165665. doi:10.1016/j.bbadis.2020.165665
Montiel-Dávalos, A., Silva Sánchez, G. J., Huerta-García, E., Rueda-Romero, C., Soca Chafre, G., Mitre-Aguilar, I. B., et al. (2017). Curcumin inhibits activation induced by urban particulate material or titanium dioxide nanoparticles in primary human endothelial cells. PloS one 12 (12), e0188169. doi:10.1371/journal.pone.0188169
Moulin, S., Arnaud, C., Bouyon, S., Pépin, J. L., Godin-Ribuot, D., and Belaidi, E. (2020). Curcumin prevents chronic intermittent hypoxia-induced myocardial injury. Ther. Adv. chronic Dis. 11, 2040622320922104. doi:10.1177/2040622320922104
Nabil, S., El-Rahman, S., Al-Jameel, S. S., and Elsharif, A. M. (2018). Conversion of curcumin into heterocyclic compounds as potent anti-diabetic and anti-histamine agents. Biol. Pharm. Bull. 41 (7), 1071–1077. doi:10.1248/bpb.b18-00170
Naryzhnaya, N. V., Maslov, L. N., Popov, S. V., Mukhomezyanov, A. V., Ryabov, V. V., Kurbatov, B. K., et al. (2022). Pyroptosis is a drug target for prevention of adverse cardiac remodeling: The crosstalk between pyroptosis, apoptosis, and autophagy. J. Biomed. Res. 36, 375–389. doi:10.7555/JBR.36.20220123
Nazıroğlu, M., Çiğ, B., Yazğan, Y., Schwaerzer, G. K., Theilig, F., and Pecze, L. (2019). Albumin evokes Ca2+-induced cell oxidative stress and apoptosis through TRPM2 channel in renal collecting duct cells reduced by curcumin. Sci. Rep. 9 (1), 12403. doi:10.1038/s41598-019-48716-x
Neheman, A., Rappaport, Y. H., Verhovsky, G., Bush, N., Snodgrass, W., Lang, E., et al. (2020). Hyperbaric oxygen therapy for pediatric "hypospadias cripple"-evaluating the advantages regarding graft take. J. Pediatr. urology 16 (2), e1–e163. doi:10.1016/j.jpurol.2020.01.002
Nguyen, T. P., Koyama, M., and Nakasaki, K. (2022). Effects of oxygen supply rate on organic matter decomposition and microbial communities during composting in a controlled lab-scale composting system. Waste Manag. (New York, N.Y.) 153, 275–282. doi:10.1016/j.wasman.2022.09.004
Nguyen-Ngo, C., Willcox, J. C., and Lappas, M. (2020). Anti-inflammatory effects of phenolic acids punicalagin and curcumin in human placenta and adipose tissue. Placenta 100, 1–12. doi:10.1016/j.placenta.2020.08.002
Oettgen, H. C. (2022). Mast cells in food allergy: Inducing immediate reactions and shaping long-term immunity. J. Allergy Clin. Immunol. 151 (1), 21–25. doi:10.1016/j.jaci.2022.10.003
Pang, B. Y., Wang, Y. H., Ji, X. W., Leng, Y., Deng, H. B., and Jiang, L. H. (2022). Systematic review and meta-analysis of the intervention effect of curcumin on rodent models of myocardial infarction. Front. Pharmacol. 13, 999386. doi:10.3389/fphar.2022.999386
Peker, K., Ökesli, S., Kıyıcı, A., and Deyişli, C. (2019). The effects of ketamine and lidocaine on free radical production after tourniquet-induced ischemia-reperfusion injury in adults. Ulus. Travma Acil Cerrahi Derg. 25 (2), 111–117. doi:10.5505/tjtes.2018.63439
Rocha-Ferreira, E., Sisa, C., Bright, S., Fautz, T., Harris, M., Contreras Riquelme, I., et al. (2019). Curcumin: Novel treatment in neonatal hypoxic-ischemic brain injury. Front. physiology 10, 1351. doi:10.3389/fphys.2019.01351
Sarighieh, M. A., Montazeri, V., Shadboorestan, A., Ghahremani, M. H., and Ostad, S. N. (2020). The inhibitory effect of curcumin on hypoxia inducer factors (hifs) as a regulatory factor in the growth of tumor cells in breast cancer stem-like cells. Drug Res. 70 (11), 512–518. doi:10.1055/a-1201-2602
Sawada, Y., Mashima, E., Saito-Sasaki, N., and Nakamura, M. (2020). The role of cell adhesion molecule 1 (CADM1) in cutaneous malignancies. Int. J. Mol. Sci. 21 (24), 9732. doi:10.3390/ijms21249732
Scindia, Y., Leeds, J., and Swaminathan, S. (2019). Iron homeostasis in healthy kidney and its role in acute kidney injury. Seminars Nephrol. 39 (1), 76–84. doi:10.1016/j.semnephrol.2018.10.006
Shahbazizadeh, S., Naji-Tabasi, S., Shahidi-Noghabi, M., and Pourfarzad, A. (2021). Development of cress seed gum hydrogel and investigation of its potential application in the delivery of curcumin. J. Sci. food Agric. 101 (15), 6505–6513. doi:10.1002/jsfa.11322
Shahedi, A., Talebi, A. R., Mirjalili, A., and Pourentezari, M. (2021). Protective effects of curcumin on chromatin quality, sperm parameters, and apoptosis following testicular torsion-detorsion in mice. Clin. Exp. reproductive Med. 48 (1), 27–33. doi:10.5653/cerm.2020.03853
Shaikh, S. B., Prabhu, A., and Bhandary, Y. P. (2021). Curcumin targets p53-fibrinolytic system in TGF-β1 mediated alveolar epithelial mesenchymal transition in alveolar epithelial cells. Endocr. metabolic immune Disord. drug targets 21 (8), 1441–1452. doi:10.2174/1871530320666200929142503
Shen, S. Q., Zhang, Y., Xiang, J. J., and Xiong, C. L. (2007). Protective effect of curcumin against liver warm ischemia/reperfusion injury in rat model is associated with regulation of heat shock protein and antioxidant enzymes. World J. gastroenterology 13 (13), 1953–1961. doi:10.3748/wjg.v13.i13.1953
Shi, Q., Zhang, Q., Peng, Y., Zhang, X., Wang, Y., and Shi, L. (2019). A natural diarylheptanoid protects cortical neurons against oxygen-glucose deprivation-induced autophagy and apoptosis. J. Pharm. Pharmacol. 71 (7), 1110–1118. doi:10.1111/jphp.13096
Singla, N., and Dhawan, D. K. (2010). Modulation of carbohydrate metabolism during N-methyl N-nitrosourea induced neurotoxicity in mice: Role of curcumin. Neurochem. Res. 35 (4), 660–665. doi:10.1007/s11064-009-0117-3
Song, N., and Li, T. (2018). Regulation of NLRP3 inflammasome by phosphorylation. Front. Immunol. 9, 2305. doi:10.3389/fimmu.2018.02305
Sun, W., Lu, H., Lyu, L., Yang, P., Lin, Z., Li, L., et al. (2019). Gastrodin ameliorates microvascular reperfusion injury-induced pyroptosis by regulating the NLRP3/caspase-1 pathway. J. physiology Biochem. 75 (4), 531–547. doi:10.1007/s13105-019-00702-7
Sun, Y., Zhang, T., Zhang, Y., Li, J., Jin, L., Sun, Y., et al. (2018). Ischemic postconditioning alleviates cerebral ischemia-reperfusion injury through activating autophagy during early reperfusion in rats. Neurochem. Res. 43 (9), 1826–1840. doi:10.1007/s11064-018-2599-3
Tang, X., Ding, H., Liang, M., Chen, X., Yan, Y., Wan, N., et al. (2021). Curcumin induces ferroptosis in non-small-cell lung cancer via activating autophagy. Thorac. cancer 12 (8), 1219–1230. doi:10.1111/1759-7714.13904
Tuo, Q. Z., Lei, P., Jackman, K. A., Li, X. L., Xiong, H., Li, X. L., et al. (2017). Tau-mediated iron export prevents ferroptotic damage after ischemic stroke. Mol. psychiatry 22 (11), 1520–1530. doi:10.1038/mp.2017.171
Upadhyay, R., and Batuman, V. (2022). Aristolochic acid I induces proximal tubule injury through ROS/HMGB1/mt DNA mediated activation of TLRs. J. Cell. Mol. Med. 26 (15), 4277–4291. doi:10.1111/jcmm.17451
Versluis, D. M., Schoemaker, R., Looijesteijn, E., Muysken, D., Jeurink, P. V., Paques, M., et al. (2022). A multiscale spatiotemporal model including a switch from aerobic to anaerobic metabolism reproduces succession in the early infant gut microbiota. mSystems 7 (5), e0044622. doi:10.1128/msystems.00446-22
Wang, K., Fan, H., Chen, Q., Ma, G., Zhu, M., Zhang, X., et al. (2015). Curcumin inhibits aerobic glycolysis and induces mitochondrial-mediated apoptosis through hexokinase II in human colorectal cancer cells in vitro. Anti-cancer drugs 26 (1), 15–24. doi:10.1097/CAD.0000000000000132
Wang, L., Li, C., Guo, H., Kern, T. S., Huang, K., and Zheng, L. (2011). Curcumin inhibits neuronal and vascular degeneration in retina after ischemia and reperfusion injury. PloS one 6 (8), e23194. doi:10.1371/journal.pone.0023194
Wang, W., and Xu, J. (2020). Curcumin attenuates cerebral ischemia-reperfusion injury through regulating mitophagy and preserving mitochondrial function. Curr. neurovascular Res. 17 (2), 113–122. doi:10.2174/1567202617666200225122620
Wang, X., Shen, K., Wang, J., Liu, K., Wu, G., Li, Y., et al. (2020). Hypoxic preconditioning combined with curcumin promotes cell survival and mitochondrial quality of bone marrow mesenchymal stem cells, and accelerates cutaneous wound healing via PGC-1α/SIRT3/HIF-1α signaling. Free Radic. Biol. Med. 159, 164–176. doi:10.1016/j.freeradbiomed.2020.07.023
Wang, X., Zhang, Y., Yang, Y., Zhang, W., Luo, L., Han, F., et al. (2019). Curcumin pretreatment protects against hypoxia/reoxgenation injury via improvement of mitochondrial function, destabilization of HIF-1α and activation of Epac1-Akt pathway in rat bone marrow mesenchymal stem cells. Biomed. Pharmacother. 109, 1268–1275. doi:10.1016/j.biopha.2018.11.005
Wang, Y., Morishima, M., and Ono, K. (2022). Protein kinase C regulates expression and function of the Cav3.2 T-type Ca2+ channel during maturation of neonatal rat cardiomyocyte. Membranes 12 (7), 686. doi:10.3390/membranes12070686
Wang, Y., Shan, X., Dai, Y., Jiang, L., Chen, G., Zhang, Y., et al. (2015). Curcumin analog L48H37 prevents lipopolysaccharide-induced TLR4 signaling pathway activation and sepsis via targeting MD2. J. Pharmacol. Exp. Ther. 353 (3), 539–550. doi:10.1124/jpet.115.222570
Wang, Z., Ren, W., Zhao, F., Han, Y., Liu, C., and Jia, K. (2020). Curcumin amends Ca2+ dysregulation in microglia by suppressing the activation of P2X7 receptor. Mol. Cell. Biochem. 465 (1-2), 65–73. doi:10.1007/s11010-019-03668-8
Wicha, P., Tocharus, J., Janyou, A., Jittiwat, J., Changtam, C., Suksamrarn, A., et al. (2017). Hexahydrocurcumin protects against cerebral ischemia/reperfusion injury, attenuates inflammation, and improves antioxidant defenses in a rat stroke model. PloS one 12 (12), e0189211. doi:10.1371/journal.pone.0189211
Wu, M. Y., Yiang, G. T., Liao, W. T., Tsai, A. P., Cheng, Y. L., Cheng, P. W., et al. (2018). Current mechanistic concepts in ischemia and reperfusion injury. Cell. Physiol. Biochem. 46 (4), 1650–1667. doi:10.1159/000489241
Xiao, B., Huang, X., Wang, Q., and Wu, Y. (2020). Beta-asarone alleviates myocardial ischemia-reperfusion injury by inhibiting inflammatory response and NLRP3 inflammasome mediated pyroptosis. Biol. Pharm. Bull. 43 (7), 1046–1051. doi:10.1248/bpb.b19-00926
Xiao, Z., and Vijayalakshmi, A. (2022). Protective effect of piperlongumine on inflammation and oxidative stress against ischemia-reperfusion injury in animal kidney. Bratisl. Lek. listy 123 (12), 878–884. doi:10.4149/BLL_2022_140
Xie, Y., Gong, X., Jin, Z., Xu, W., and Zhao, K. (2022). Curcumin encapsulation in self-assembled nanoparticles based on amphiphilic palmitic acid-grafted-quaternized chitosan with enhanced cytotoxic, antimicrobial and antioxidant properties. Int. J. Biol. Macromol. 222, 2855–2867. doi:10.1016/j.ijbiomac.2022.10.064
Xu, H., Nie, B., Liu, L., Zhang, C., Zhang, Z., Xu, M., et al. (2019). Curcumin prevents brain damage and cognitive dysfunction during ischemic-reperfusion through the regulation of miR-7-5p. Curr. neurovascular Res. 16 (5), 441–454. doi:10.2174/1567202616666191029113633
Yamaguchi, A., Botta, E., and Holinstat, M. (2022). Eicosanoids in inflammation in the blood and the vessel. Front. Pharmacol. 13, 997403. doi:10.3389/fphar.2022.997403
Yan, H. F., Tuo, Q. Z., Yin, Q. Z., and Lei, P. (2020). The pathological role of ferroptosis in ischemia/reperfusion-related injury. Zoological Res. 41 (3), 220–230. doi:10.24272/j.issn.2095-8137.2020.042
Yang, K., Xu, C., Li, X., and Jiang, H. (2013). Combination of D942 with curcumin protects cardiomyocytes from ischemic damage through promoting autophagy. J. Cardiovasc. Pharmacol. Ther. 18 (6), 570–581. doi:10.1177/1074248413503495
Ye, B., Chen, X., Dai, S., Han, J., Liang, X., Lin, S., et al. (2019). Emodin alleviates myocardial ischemia/reperfusion injury by inhibiting gasdermin D-mediated pyroptosis in cardiomyocytes. Drug Des. Dev. Ther. 13, 975–990. doi:10.2147/DDDT.S195412
Yin, Y., Wu, X., Peng, B., Zou, H., Li, S., Wang, J., et al. (2020). Curcumin improves necrotising microscopic colitis and cell pyroptosis by activating SIRT1/NRF2 and inhibiting the TLR4 signalling pathway in newborn rats. Innate Immun. 26 (7), 609–617. doi:10.1177/1753425920933656
Yu, R., Wang, K., Luo, W., and Jiang, H. (2022). Knockdown and mutation of Pou4f3 gene mutation promotes pyroptosis of cochleae in cisplatin-induced deafness mice by NLRP3/caspase-3/GSDME pathway. Toxicology 482, 153368. doi:10.1016/j.tox.2022.153368
Yu, W., Qin, X., Zhang, Y., Qiu, P., Wang, L., Zha, W., et al. (2020). Curcumin suppresses doxorubicin-induced cardiomyocyte pyroptosis via a PI3K/Akt/mTOR-dependent manner. Cardiovasc. diagnosis Ther. 10 (4), 752–769. doi:10.21037/cdt-19-707
Zhang, L., Zhang, B., Wang, L., Lou, M., and Shen, Y. (2021). Huanglian-Houpo drug combination ameliorates H1N1-induced mouse pneumonia via cytokines, antioxidant factors and TLR/MyD88/NF-κB signaling pathways. Exp. Ther. Med. 21 (5), 428. doi:10.3892/etm.2021.9845
Zhang, S., Zou, J., Li, P., Zheng, X., and Feng, D. (2018). Curcumin protects against atherosclerosis in apolipoprotein E-knockout mice by inhibiting toll-like receptor 4 expression. J. Agric. food Chem. 66 (2), 449–456. doi:10.1021/acs.jafc.7b04260
Zhang, Y., Zhang, Y., Jin, X. F., Zhou, X. H., Dong, X. H., Yu, W. T., et al. (2019). The role of astragaloside IV against cerebral ischemia/reperfusion injury: Suppression of apoptosis via promotion of P62-LC3-autophagy. Mol. (Basel, Switz. 24 (9), 1838. doi:10.3390/molecules24091838
Zhao, Y., Xin, Z., Li, N., Chang, S., Chen, Y., Geng, L., et al. (2018). Nano-liposomes of lycopene reduces ischemic brain damage in rodents by regulating iron metabolism. Free Radic. Biol. Med. 124, 1–11. doi:10.1016/j.freeradbiomed.2018.05.082
Zhao, Y., Zhou, Y., Ma, X., Liu, X., Zhao, Y., and Liu, X. (2020). DDAH-1 via HIF-1 target genes improves cerebral ischemic tolerance after hypoxic preconditioning and middle cerebral artery occlusion-reperfusion. Nitric oxide Biol. Chem. 95, 17–28. doi:10.1016/j.niox.2019.12.004
Zheng, Y. P., and Liu, C. J. (2019). Effects of total glucosides of paeonia on NLRP3 expression and pyroptososis of myocardial cells of myocardial ischemia reperfusion rats. Traditional Chin. Drug Res. Clin. Pharmacol. 8, 5.
Zhu, J., Qu, J., Fan, Y., Zhang, R., and Wang, X. (2022). Curcumin inhibits invasion and epithelial-mesenchymal transition in hepatocellular carcinoma cells by regulating TET1/wnt/β-catenin signal Axis. Bull. Exp. Biol. Med. 173 (6), 770–774. doi:10.1007/s10517-022-05629-6
Zhu, P., Yang, M., He, H., Kuang, Z., Liang, M., Lin, A., et al. (2019). Curcumin attenuates hypoxia/reoxygenation-induced cardiomyocyte injury by downregulating Notch signaling. Mol. Med. Rep. 20 (2), 1541–1550. doi:10.3892/mmr.2019.10371
Keywords: curcumin, ischemia-reperfusion injury, oxidative stress, inflammation, cell death
Citation: Bi M, Li D and Zhang J (2023) Role of curcumin in ischemia and reperfusion injury. Front. Pharmacol. 14:1057144. doi: 10.3389/fphar.2023.1057144
Received: 30 September 2022; Accepted: 23 February 2023;
Published: 20 March 2023.
Edited by:
Zsuzsanna Helyes, University of Pécs, HungaryReviewed by:
Anikó Görbe, Semmelweis University, HungaryCopyright © 2023 Bi, Li and Zhang. This is an open-access article distributed under the terms of the Creative Commons Attribution License (CC BY). The use, distribution or reproduction in other forums is permitted, provided the original author(s) and the copyright owner(s) are credited and that the original publication in this journal is cited, in accordance with accepted academic practice. No use, distribution or reproduction is permitted which does not comply with these terms.
*Correspondence: Jin Zhang, MTM4OTM0Nzc3MDJAMTYzLmNvbQ==
Disclaimer: All claims expressed in this article are solely those of the authors and do not necessarily represent those of their affiliated organizations, or those of the publisher, the editors and the reviewers. Any product that may be evaluated in this article or claim that may be made by its manufacturer is not guaranteed or endorsed by the publisher.
Research integrity at Frontiers
Learn more about the work of our research integrity team to safeguard the quality of each article we publish.