- 1Department of Clinical Laboratory, The Sixth Affiliated Hospital of Nantong University, Yancheng Third People’s Hospital, Yancheng, Jiangsu, China
- 2Institute of Medical Genetics and Reproductive Immunity, School of Medical Science and Laboratory Medicine, Jiangsu College of Nursing, Huai’an, Jiangsu, China
- 3Department of Clinical Laboratory, Affiliated Hospital of Nanjing University Medical School, Yancheng First People’s Hospital, Yancheng, Jiangsu, China
TRPV1 is a non-selective channel receptor widely expressed in skin tissues, including keratinocytes, peripheral sensory nerve fibers and immune cells. It is activated by a variety of exogenous or endogenous inflammatory mediators, triggering neuropeptide release and neurogenic inflammatory response. Previous studies have shown that TRPV1 is closely related to the occurrence and/or development of skin aging and various chronic inflammatory skin diseases, such as psoriasis, atopic dermatitis, rosacea, herpes zoster, allergic contact dermatitis and prurigo nodularis. This review summarizes the structure of the TRPV1 channel and discusses the expression of TRPV1 in the skin as well as its role of TRPV1 in skin aging and inflammatory skin diseases.
1 Introduction
The skin is the largest organ in the human body and is mainly composed of three layers. The epidermis is most superficial layer and contains nerves and numerous specialized cells such as keratinocytes, melanocytes, Langerhans cells and Merkel cells (Orsmond et al., 2021). The dermis is located below the epidermis and is rich in fibrous extracellular matrix (ECM), containing fibroblasts and mast cells as well as interspersed resident immune cells like T cells, dendritic cells (DCs) and macrophages which participate in the immune response against pathogen infection (Orsmond et al., 2021; Naik, 2022). Moreover, abundant blood vessels and nerves are also found in the dermis. The deepest layer is the hypodermis, which is mostly composed of fatty tissue (Orsmond et al., 2021). In addition, the skin contains several appendages including hairs, hair follicles, sweat glands and sebaceous glands (Umar et al., 2018). These structures constitute a mechanical barrier to restrict water loss and protect the body from external environmental insults through physical and immune system functions.
In addition to its well-characterized mechanical barrier function, the skin is also the largest sensory organ of the human body, which can sense external cold, heat, touch, pressure pain, itch and other stimuli through receptors and primary sensory nerve endings (Middleton et al., 2021). Transient receptor potential (TRP) is a widely distributed protein in the peripheral and central nervous system, which is divided into seven subfamilies: TRPA (Ankyrin), TRPC (Canonical), TRPM (Melastatin), TRPN (NOMPC-like) TRPML (Mucolipin), TRPP (Polycystin) and TRPV (Vanilloid) (Venkatachalam and Montell, 2007; Samanta et al., 2018). TRPV1, the first protein found in TRP channels, is world-famous as its discoverer David Julius was awarded the 2021 Nobel Prize in Physiology or Medicine. Recent studies have revealed that TRPV1 is widely expressed in many cell types, including keratinocytes, sensory neurons and immune/inflammatory cells in the skin, attracting the attention of many researchers (Caterina and Pang, 2016; Bagood and Isseroff, 2021).
This review introduces the basic knowledge about the structure of the TRPV1 channel and discusses the expression of TRPV1 in the skin. Furthermore, the role of TRPV1 in skin aging and inflammatory skin diseases is explored.
2 Overall features of TRPV1 channels
In the early 19th century, researchers pointed out that capsaicin, the main pungent component in “hot” chili pepper, caused a burning sensation and pain in mammalian mucosa or skin (Nagy et al., 2004). In 1997, Caterina et al. successfully cloned and isolated the first capsaicin-sensitive receptor from rat dorsal root ganglion and named it vanilloid receptor subtype 1 (VR1) (Caterina et al., 1997). The human TRPV1 protein composed of 838 amino acids is encoded by the TRPV1 gene with exons located at 17p13.2 (Hayes et al., 2000). The TRPV1 protein is a homo-tetramer, and each of its subunits consists of six-transmembrane domains (S1-S6), a long N-terminal region and a relatively short C-terminal region. Between the fifth and sixth transmembrane domains, a pore-forming hydrophobic span is considered to mediate the passage of ions (Caterina et al., 1997) (Figure 1). The N-terminal domain contains several phosphorylation sites and six ankyrin repeats to bind calmodulin and ATP (Lishko et al., 2007). Upon binding, these molecules modulate the sensitivity and function of TRPV1 (Phelps et al., 2010). On the other hand, the C-terminal domain bears a conserved TRP region, multiple calmodulin binding regions and endogenous substance binding sites, which can bind to protein kinase C (PKC), Ca2+/calmodulin-dependent protein kinaseⅡ(CaMK II), and phosphatidyl-inositol-4,5-bisphosphate (PIP2) (Garcia-Sanz et al., 2004).
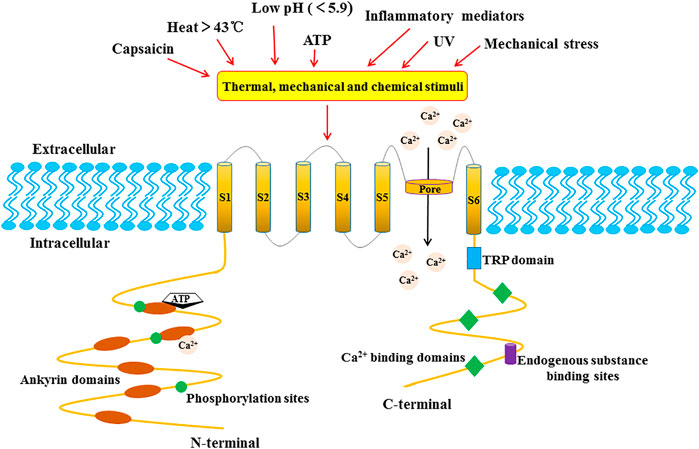
FIGURE 1. The structure of the TRPV1 channel subunit. TRPV1 is a homo-tetramer, and each subunit is composed of six-transmembrane domains (S1-S6) with a pore-forming hydrophobic group. The long N-terminal contains several phosphorylation sites and six ankyrin repeat domains. The C-terminal bears a TRP domain, multiple calmudulain binding domains and endogenous substance binding sites.
TRPV1 is a multimodal nociceptor that is activated and/or allosterically modulated by various thermal, mechanical and chemical stimuli (Holzer, 2008). In addition to capsaicin, the thermal sensitivity of TRPV1 is controlled by other endogenous substances including ATP, bradykinin, nerve growth factor (NGF) (NatureChuang et al., 2001), inflammatory mediators such as arachidonic acid amides (Sinning et al., 2008) and prostaglandin (Zhong et al., 2021). Similarly, TRPV1 also is activated by other exogenous physical or chemical stimuli, such as camphor and resiniferatoxin (RTX), vanillatoxins 1–3 (tarantula), ginger, and ethanol (Holzer, 2008). Moreover, TRPV1 is activated under noxious heat conditions (≥43°C) (Loyd et al., 2012), acidosis (pH ≤ 5.9) (Kaszas et al., 2012), UV irradiation and mechanical stress (Inoue et al., 2009).
As a cation channel, TRPV1 shows similar permeability to monovalent cations such as Na+, K+, Li+, but it possesses moderate selectivity to divalent cations (Caterina et al., 1997). When TRPV1 is activated by capsaicin, the permeability of TRPV1 to ions is Ca2+ >Mg2+>Na+≈K+ (Caterina et al., 1997). The direct consequence of the TRPV1 activation is the inflow of extracellular Ca2+ into the cells and release of the intracellular Ca2+ pool, resulting in an increase in intracellular Ca2+ concentration, which mediates the basic activities of various cells, such as muscle contraction, neuronal activity, transmitter release, cell proliferation and apoptosis (Sappington et al., 2009). Notably, TRPV1 regulates body temperature and pain after activation. Studies have demonstrated that the activated TRPV1 promotes the release of substance P (SP) and calcitonin gene-related peptide (CGRP) (Brain and Grant, 2004). CGRP is a powerful vasodilator that significantly increases the blood flow of skin vessels, while SP is a short-term vasodilator that can significantly promote plasma extravasation. Both molecules can induce dermal vasodilation, local edema and other inflammatory reactions (Brain and Grant, 2004; Aubdool and Brain, 2011). In addition, SP mediated pruritus and influenced neurogenic inflammation via the neurokinin-1 receptor (NK-1R) and Mas-related G protein-coupled receptors (Mrgprs), which are located on both mast cells and on the cells of the dorsal horn of the spinal cord (Azimi et al., 2017). What’s more, the release of inflammatory mediators can mediate pain and hyperalgesia (Cavanaugh et al., 2009).
3 The expression and function of TRPV1 in skin
TRPV1 is considered a primary cellular sensor of thermal and chemical stimulation in the skin (Tominaga et al., 1998). In 2001, Denda et al. discovered the expression of TRPV1 in human epidermal keratinocytes (KC) and human epidermal immortalized keratinocyte line HaCaT cells (Denda et al., 2001). Subsequently, other studies demonstrated that heat, photoaging and natural aging could increase TRPV1 expression in human KC ex vivo and in vivo (Lee et al., 2008; Lee et al., 2009a). Theoretically, the TRPV1 agonist capsaicin evoked an influx of Ca2+ that was inhibited by the TRPV1 antagonist capsazepine or PAC-14028 in normal human keratinocytes (Inoue et al., 2002; Yun et al., 2011a). The dynamic balance of Ca2+ played an essential role in maintaining the stability of KC, and the increase of intracellular Ca2+ concentration promoted KC differentiation and inhibited KC proliferation (Thio et al., 1994). In addition, the activation of TRPV1 promoted the polarization and migration of KC (Li et al., 2012; Miyazaki et al., 2015).
Melanocytes are the cells primarily responsible for protecting against the harmful effects of UV irradiation on the skin. A study evaluated the TRPV1 immunoreactivity in situ in normal human skin, revealing that melanocytes were TRPV1 negative (Bodó et al., 2004). However, the researchers used immunocytochemistry and found positive TRPV1 expression in the cultured primary human melanocytes. Capsaicin could activate TRPV1 and increase intracellular Ca2+, but this did not affect melanogenesis (Choi et al., 2009). The contradictory results may arise from the low TRPV1 expression in vivo, which could not be detected using IHC. In contrast, a genome-wide transcriptome analysis of human epidermal melanocytes found the expression of TRPV1 (Haltaufderhyde and Oancea, 2014).
Moreover, TRPV1 was also found to be expressed in skin fibroblasts (Lan et al., 2013), dermal vascular endothelial cells (Ständer et al., 2004), apocrine and exocrine sweat gland duct cells (Ständer et al., 2004), mast cells (Ständer et al., 2004) and dendritic cells (Basu and Srivastava, 2005) (Figure 2). TRPV1 activation by capsaicin triggered the maturation of immature DCs in vitro and the migration of dermal DCs to lymph nodes in vivo (Basu and Srivastava, 2005). In addition, it has been reported that TRPV1 is widely expressed on skin sensory nerve fibers (Nakagawa and Hiura, 2013). The activation of TRPV1+ sensory nerves with capsaicin produced pain, burning and itching and induced the release of CGRP and SP, mediating neurogenic inflammation, which eventually led to various skin diseases and pruritus (Nakagawa and Hiura, 2013). Another study revealed that TRPV1+ sensory neurons innervating the hair follicle promoted epithelial proliferation and hair follicle stem cell progeny migration after wounding (Martinez-Martinez et al., 2012). In human and mice, the stimulation of TRPV1 with capsaicin increased the insulin-like growth factor-I (IGF-1) levels and significantly promoted hair growth (Harada et al., 2007).
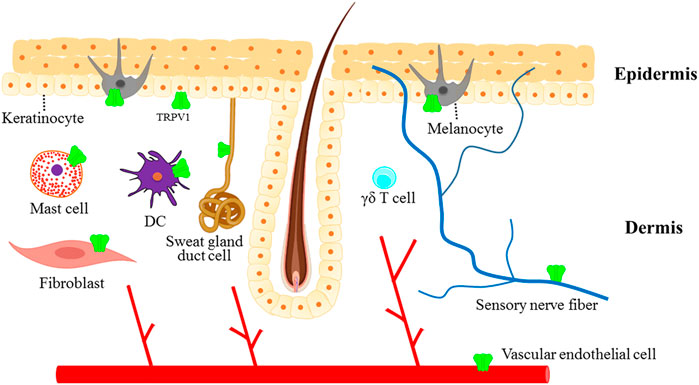
FIGURE 2. The expression of TRPV1 in the skin. TRPV1 channel is not only expressed in sensory nerve fibers innervating the skin but also in different non-neuronal cells, which contribute to maintaining normal skin physiology or play important roles in the pathogenesis of skin diseases.
In summary, these findings improve the understanding of the complexity and plasticity of TRPV1 in the skin.
4 The role of TRPV1 in skin aging
The aging of human skin is a complex process including intrinsic aging and photoaging (Lee et al., 2021). Intrinsic aging refers to chronological damage caused by slow, irreversible tissue degeneration, whereas photoaging is mainly induced by continuous UV exposure (Pourang et al., 2022). Wrinkles are one of the common characteristics of intrinsic aging and photoaging, which is caused by the degeneration of collagen and elastin fibers (Pedić et al., 2020). As well all known, collagen and elastin fibers are responsible for the strength and elasticity of the skin (Losquadro, 2017). Matrix metalloproteinase (MMP)-mediated collagen destruction contributes to wrinkle formation, laxity, and fragility during intrinsic skin aging and UV-induced photoaging (Lee et al., 2021). Some studies reported that TRPV1 and Ca2+ regulate the expression and activation of MMP-1 in UV-induced photoaging (Figure 3). UV irradiation increased TRPV1 mRNA and protein expression and activated TRPV1 to trigger Ca2+ influx, which in turn increased the MMP-1 mRNA and protein expression via Ca2+/calcineurin/NFATc1/GSDMC signaling in HaCaT cells (Lee et al., 2009a; Kusumaningrum et al., 2018). Additionally, MMP-1 expression induced by UV irradiation was mediated by TRPV1/Ca2+/PKC signaling in human keratinocytes (Lee et al., 2009b). A study has revealed that capsaicin boosted collagen synthesis in cultured dermal fibroblasts via extracellular signal-regulated kinases (Erk)/c-Jun-mediated MMP signaling. Moreover, the application of low-dose capsaicin on the shaved mouse dorsal skins alleviated the UV-induced reduction of collagen (Wu et al., 2022). In addition, baicalein suppressed MMP-1 expression by activating the TRPV1-Ca2+-ERK pathway in UVB-irradiated human dermal fibroblasts (Huang et al., 2019). Collectively, the various downstream signaling pathways of TRPV1/Ca2+ are involved in UV-induced skin aging. However, further research is required to determine if such mechanisms are present in chronological aging.
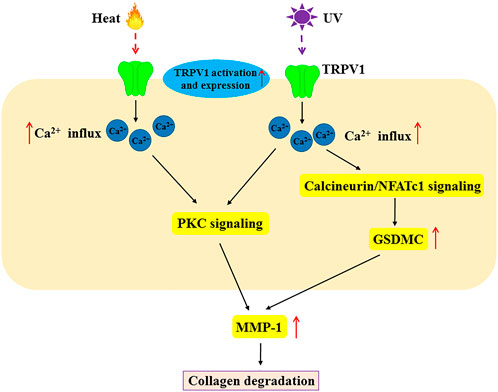
FIGURE 3. The molecular mechanism of TRPV1 in heat and UV-induced MMP-1 expression in skin aging. Heat and UV light not only activate TRPV1 but also increase its expression. Heat and UV induce MMP-1 expression via Ca2+ influx, PKC signaling and calcineurin/NFATc1 signaling. This scheme demonstrates the role of TRPV1 in skin aging by heat and UV. MMP-1, matrix metalloproteinase-1; PKC, protein Kinase C; NFATc1, nuclear factor of activated T-cells, cytoplasmic 1.
Thermal skin aging is caused by increased skin temperature during Sun exposure. Previous research has shown that acute heat shock induced TRPV1 expression (Matsumura and Ananthaswamy, 2004).
Another study reported increased expression of MMP-1 mRNA and protein in HaCaT cells and normal human epidermal keratinocytes following heat shock generated by infrared (IR) irradiation (Cho et al., 2009). Further study showed that heat-shock-induced MMP-1 expression was mediated by TRPV1 (Li et al., 2007). Expectedly, the inhibition of TRPV1 with TRPV1 inhibitors (capsazepine and ruthenium red) or knockdown of TRPV1 using RNA interference effectively suppressed heat shock-induced MMP-1 expression in HaCaT cells (Li et al., 2007). TRPV1-mediated Ca2+ influx and PKC signaling were necessary for heat shock-induced MMP-1 expression in HaCaT cells (Li et al., 2007). These findings suggest that TRPV1/Ca2+ signaling is involved in heat-induced MMP1 expression.
In summary, TRPV1/Ca2+ signaling is critical in skin aging. Therefore, the development of TRPV1-specific, non-toxic inhibitors may be an effective strategy for the prevention of skin aging.
5 TRPV1 and inflammatory skin diseases
The most common forms of skin diseases are inflammatory skin diseases such as psoriasis, atopic dermatitis (AD), rosacea, herpes zoster (HZ), allergic contact dermatitis (ACD) and prurigo nodularis (PN), which are caused by various internal and external infectious and non-infectious factors. Pruritus and erythema are the most common symptoms of skin diseases. Nevertheless, the specific mechanism is not fully understood. Currently, two signal pathways of pruritus have been identified, namely the histamine-dependent (histaminergic) signaling pathway and the protease-activated receptor-2 (PAR-2) signaling pathway (Song et al., 2018). These signaling pathways interact with mediators, such as amines (histamine, serotonin/5-HT, IL-6), peptides (bradykinin, SP, CGRP, neurotrophin) and phospholipid metabolites (cannabinoids, eicosanoids, platelet-activating factor), and result in the occurrence and development of pruritus and erythema (Song et al., 2018).
In recent years, a growing number of studies have investigated the potential role of TRPV1 in the pathogenesis of inflammatory skin diseases (Table 1). The following sections illustrate the mechanism and diverse functions in various inflammatory skin diseases and the potential clinical value of TRPV1.
5.1 TRPV1 and psoriasis
Psoriasis is a chronic, systemic immune-mediated disease characterized by erythematous, indurated, scaly, pruritic and often painful skin plaques that significantly impact patients’ quality of life (Vicic et al., 2021). Studies suggest that TRPV1 may be involved in the development of pruritus in psoriasis. A study found a positive correlation between TRPV1 overexpression and psoriasis itching (Nattkemper et al., 2018). The TrkA kinase inhibitor CT327 reduced pruritus by suppressing the NGF-TrkA-TRPV1 pathway in psoriatic patients (Roblin et al., 2015).
Further research demonstrated a close relationship between TRPV1 and the pathogenesis of psoriasis. Immune cells play a crucial role in the pathogenesis of psoriasis. A study reported increased TRPV1 mRNA expression in peripheral blood mononuclear cells (PBMCs) of patients with psoriasis (Ozcan et al., 2021). In the imiquimod (IMQ)-induced murine model of psoriasis-form dermatitis, TRPV1 gene knockout resulted in a reduction in the infiltration of CD45+ leukocytes, mast cells and CD3+ T, accompanied by a significant decrease in the expression levels of inflammatory cytokines (IL-1β, IL-6, IL-23 and S100A8) in the skin lesions of (61). In addition, upon selective pharmacological or genetic ablation of TRPV1+ sensory fibers, dermal dendritic cells failed to produce IL-23, resulting in decreased IL-17 and IL-22 expression by IL23R+ dermal γδ T cells. Subsequently, reduced recruitment of inflammatory cells to the skin was observed, eventually alleviating IMQ-induced IL-23-dependent psoriasis-like skin inflammation (Riol-Blanco et al., 2014). In preclinical models of psoriasis, resolving D3 (RvD3) derived from ω-3 fatty acids prevented the development of both psoriasis-form itch and skin inflammation with the concomitant decreased expression of CGRP in TRPV1+ neurons (Lee et al., 2020).
The balance between the proliferation and differentiation of the KCs is disrupted in psoriatic lesions, showing increased proliferation and impaired differentiation (Vicic et al., 2021). The concentration gradient of Ca2+ plays an important role in the differentiation of KCs. In the normal epidermis, the Ca2+ concentration is the highest in the granular layer, while that in the basal layer is the lowest (Lee and Lee, 2018). Interestingly, it has been demonstrated that the level of intracellular Ca2+ in the basal layer of psoriatic lesions was higher than extracellular Ca2+, indicating a disruption of the normal Ca2+ concentration gradient in psoriatic lesions (Cubillos and Norgauer, 2016). Furthermore, TRPV1-mediated Ca2+ flow was involved in the differentiation of KCs (Tsutsumi et al., 2011). Capsaicin directly activated TRPV1, leading to Ca2+ influx, thus inhibiting the proliferation of KCs and promoting the differentiation (Thio et al., 1994). Therefore, percutaneous delivery of capsaicin could significantly attenuate the psoriasis-form dermatitis symptom (Chan et al., 2021).
5.2 TRPV1 and atopic dermatitis (AD)
Atopic dermatitis (AD) is a chronic, genetically predisposed, complex inflammatory skin disease that is characterized by recurrent eczematous lesions, inflammation and intense itching (Nakahara et al., 2021). Intense pruritus affects patient behavior (scratching) and is associated with increased stress, anxiety, and other mood disorders, significantly worsening disease prognosis and quality of life (Nakahara et al., 2021). TRPV1 is a critical molecule in pruritus signaling in AD. TRPV1 has been shown to be upregulated in AD lesions, and its activation resulted in the release of mediators that promote inflammation and itching (Steinhoff et al., 2003). TRPV1 is involved in histamine-induced pruritus. Moreover, histamine-induced Ca2+ influx was attenuated by TRPV1 antagonists in rat dorsal root ganglion (DRG) neurons (Kim et al., 2004). A study has reported that human embryonic kidney 293 (HEK293) cells co-expressed TRPV1 and histamine receptors 1 (H1R) (Shim et al., 2007). In the presence of Ca2+, histamine binds to H1R to trigger the phospholipase A2 (PLA-2) and 12-lipoxygenase-dependent activation of TRPV1 on histamine-sensitive C-fibers, mediating itching (Shim et al., 2007). Thus, TRPV1-deficient mice demonstrated impaired scratching behavior in response to histamine injection (Shim et al., 2007; Imamachi et al., 2009). What’s more, a naturally occurring omega-9 fatty reduced itch evoked by histamine and cyclic phosphatidic acid (cPA) by inhibiting TRPV1 in peripheral neurons (Morales-Lázaro et al., 2016). To date, antihistamines have generally offered only marginal therapeutic benefits for AD. Thus, the combination of antihistamines and TPV1 antagonists provides a new strategy for the clinical treatment of AD.
Interleukin-31 (IL-31) is a major inflammatory marker whose level is positively correlated with the severity of AD (Datsi et al., 2021). TRPV1 and IL-31 receptor α (IL-31RA) are co-expressed in mouse and human dorsal root ganglion neurons. Pruritus induced by IL-31 is mediated by directly activating IL-31RA on the cutaneous TRPV1+ sensory nerve (Cevikbas et al., 2014). This evidence indicates a complex between TRPV1 and some mediators.
Furthermore, TRPV1 is involved in epidermal barrier homeostasis. TRPV1 was shown to mediate itch-associated scratching and skin barrier dysfunction by regulating pruritogenic mediators (including TSLP, IL-31 and SP) in a 2,4-dinitrofluorobenzene (DNFB)-induced AD model (Tang et al., 2022). In both mouse and human skin, capsaicin-mediated activation of TRPV1 retarded the skin barrier recovery. In contrast, the TRPV1 antagonists capsazepine and PAC-14028 could accelerate skin barrier function recovery by reducing TEWL (trans-epidermal water loss), promoting the reformation of the neutral lipid layer and reversing the changes in loricrin and filaggrin expression (Denda et al., 2007; Yun et al., 2011a). In addition, PAC-14028 attenuated histamine- and PAR-2-mediated pruritus in murine models of AD (Yun et al., 2011b). Phase II clinical trials to evaluate the efficacy of topical applications of PAC-14028 cream on AD have been completed. However, data from these trials were not available (ClinicalTrials.gov, 2017).
These findings suggest that TRPV1 is a potential therapeutic target for AD. In addition to traditional antipruritic therapy (antihistamines) to control itch symptoms, TRPV1 antagonists may be used as a new antipruritic drug to treat inflammatory skin diseases.
5.3 TRPV1 and rosacea
Rosacea is a chronic inflammatory cutaneous disorder characterized by repeated remission and recurrent episodes of flushing or transient erythema, mainly affecting the cheeks, nose, chin, and forehead (van Zuuren et al., 2021). Although the pathogenesis of rosacea is not fully understood, multiple factors are thought to be involved, such as genetics, immune factors, neurovascular dysregulation, microorganisms, and environmental factors (van Zuuren et al., 2021).
Substantial efforts have been made to elucidate the role of TRPV1 in the inflammatory stage of rosacea. A study has shown significantly increased expression of TRPV1 on non-neuronal cells in rosacea patients with erythema telangiectasia (Sulk et al., 2012). In general, the activation of TRPV1 stimulated the sensory neurons C fibers to release vasoactive peptides, such as CGRP and SP, leading to vasomotor dysfunction, and high neurovascular reactivity manifesting as paroxysmal flushing, persistent erythema and telangiectasia (Aubdool and Brain, 2011). Moreover, TRPV1 plays an essential role in heat sensitive regulation of inflammation. TRPV1-deficient mice demonstrated impaired response to high-temperature injury and acute thermal stimulation (Caterina et al., 2000). Chizh et al. reported that the application of TRPV1 antagonist blocked the erythema and burning caused by external capsaicin cream on human skin (Chizh et al., 2007). Some studies have revealed that TRPV1 antagonists have certain antidepressant and antianxiety effects, which may be beneficial to rosacea patients as they often suffer from psychological stress (de Moura et al., 2014). Therefore, TRPV1 may be a new therapeutic target for rosacea.
5.4 TRPV1 and other inflammatory skin diseases
Herpes zoster (HZ), also known as shingles, is a skin infection disease characterized by debilitating pain caused by the reactivation of varicella-zoster virus (VZV) (Rosamilia, 2020). Elevated protein and mRNA levels of TRPV1 were observed in the skin lesions of HZ patients. Interestingly, the enhanced mRNA and protein expression level of prostaglandin E2 (PGE2) were also detected in skin lesions, suggesting a correlation between TRPV1 and pain in HZ patients. The local pain response may be related to the pro-inflammatory factor PGE2 secreted by TRPV1-mediated keratinocytes (Han et al., 2016).
Allergic contact dermatitis (ACD) is a common inflammatory skin disease caused by exposure to contact allergens (Olusegun and Martincigh, 2021). Banvolgyi et al. successfully depleted the TRPV1-mediated sensory component from the capsaicin-sensitive neurons by systemic resiniferatoxin (RTX) pretreatment or genetic deletion, resulting in an enhanced inflammatory response. This enhancement indicated that capsaicin-sensitive neurons expressing TRPV1 can downregulate hypersensitivity, possibly by influencing the immune state of the skin (Banvolgyi et al., 2005).
Prurigo nodularis (PN) is an extremely severe pruritic skin disease characterized by intensely pruritic, hyperkeratotic nodules (Williams et al., 2021). A significant increase in TRPV1 expression was observed in epidermal KCs and nerve fibers of the pruritic skin of PN, which was normalized after capsaicin application (Ständer et al., 2004). During repeated application of capsaicin therapy, a reduction of neuropeptides (such as SP and CGRP) from sensory nerve fibers was observed due to the desensitization of nerve fibers. After capsaicin treatment was stopped, neuropeptides re-accumulated in cutaneous nerves, which suggests that TRPV1 plays an important role in pruritus and neurogenic inflammation (Ständer et al., 2004).
6 Conclusion and future perspectives
Since TRPV1 was discovered in the skin, it has gradually revealed the important role in the physiological and pathological skin. This review focuses on the structure and biological characteristics of the TRPV1 channel and its expression in various skin cells, such as cutaneous sensory nerve fibers, mast cells, epidermal keratinocytes, immune cells, dermal blood vessels, the inner root sheet and the infundibulum of hair follicles, differentiated sebocytes, sweat gland ducts, and the secretory portion of eccrine sweat glands. Notably, TRPV1 exerts different functions in various cell types. Furthermore, a great deal of studies have demonstrated the involvement of TRPV1 in skin aging and skin inflammation through different mechanisms.
The activation or blockade of TRPV1 is a promising therapeutic strategy in the treatment of chronic inflammatory skin diseases. An in-depth understanding of the biological function of TRPV1 and its role in the pathogenesis of chronic inflammatory skin diseases will allow the development of specific TRPV1 agonists or antagonists for the treatment of chronic inflammatory skin diseases.
Author contributions
Writing and original draft preparation, TX and MS; writing and review, CZ; writing, review and editing, JK; funding acquisition, CZ and TX. All authors contributed to the article and approved the submitted version.
Funding
This research was funded by the Huai’an Natural Science Research Program (Grant No. HABL202114), Huai’an “Tianyixing” key Laboratory of Medical Examination (Grant No. HAP202004), the Science and Technology Development Project of Yancheng, China (YK2019108) and the Nantong University Clinical Medicine Special Project, China (2019JZ011).
Conflict of interest
The authors declare that the research was conducted in the absence of any commercial or financial relationships that could be construed as a potential conflict of interest.
Publisher’s note
All claims expressed in this article are solely those of the authors and do not necessarily represent those of their affiliated organizations, or those of the publisher, the editors and the reviewers. Any product that may be evaluated in this article, or claim that may be made by its manufacturer, is not guaranteed or endorsed by the publisher.
References
Aubdool, A. A., and Brain, S. D. (2011). Neurovascular aspects of skin neurogenic inflammation. J. Investig. Dermatol Symp. Proc. 15 (1), 33–39. doi:10.1038/jidsymp.2011.8
Azimi, E., Reddy, V. B., Pereira, P. J. S., Talbot, S., Woolf, C. J., and Lerner, E. A. (2017). Substance P activates Mas-related G protein-coupled receptors to induce itch. J. Allergy Clin. Immunol. 140 (2), 447–453. doi:10.1016/j.jaci.2016.12.980
Bagood, M. D., and Isseroff, R. R. (2021). TRPV1: Role in skin and skin diseases and potential target for improving wound healing. Int. J. Mol. Sci. 22 (11), 6135. doi:10.3390/ijms22116135
Banvolgyi, A., Palinkas, L., Berki, T., Clark, N., Grant, A. D., Helyes, Z., et al. (2005). Evidence for a novel protective role of the vanilloid TRPV1 receptor in a cutaneous contact allergic dermatitis model. J. Neuroimmunol. 169 (1-2), 86–96. doi:10.1016/j.jneuroim.2005.08.012
Basu, S. S. P., and Srivastava, P. (2005). Immunological role of neuronal receptor vanilloid receptor 1 expressed on dendritic cells. Proc. Natl. Acad. Sci. U. S. A. 102 (14), 5120–5125. doi:10.1073/pnas.0407780102
Bodó, E. K. I., Telek, A., Varga, A., Paus, R., Kovács, L., Kovacs, L., et al. (2004). Vanilloid receptor-1 (VR1) is widely expressed on various epithelial and mesenchymal cell types of human skin. J. Invest. Dermatol 123 (2), 410–413. doi:10.1111/j.0022-202X.2004.23209.x
Brain, S. D. G. A., and Grant, A. D. (2004). Vascular actions of calcitonin gene-related peptide and adrenomedullin. Physiol. Rev. 84 (3), 903–934. doi:10.1152/physrev.00037.2003
Caterina, M. J. L. A., Malmberg, A. B., Martin, W. J., Trafton, J., Petersen-Zeitz, K. R., Leffler, A., et al. (2000). Impaired nociception and pain sensation in mice lacking the capsaicin receptor. Science 288 (5464), 306–313. doi:10.1126/science.288.5464.306
Caterina, M. J., and Pang, Z. (2016). TRP channels in skin biology and pathophysiology. Pharm. (Basel) 9 (4), 77. doi:10.3390/ph9040077
Caterina, M. J. S. M., Tominaga, M., Rosen, T. A., Levine, J. D., and Julius, D. (1997). The capsaicin receptor a heat-activated ion channel in the pain pathway. Nature 389 (6653), 816–824. doi:10.1038/39807
Cavanaugh, D. J., Lee, H., Lo, L., Shields, S. D., Zylka, M. J., and Basbaum, A. I., et al. (2009). Distinct subsets of unmyelinated primary sensory fibers mediate behavioral responses to noxious thermal and mechanical stimuli. Proc. Natl. Acad. Sci. U. S. A. 106 (22), 9075–9080. doi:10.1073/pnas.0901507106
Cevikbas, F., Wang, X., Akiyama, T., Kempkes, C., Savinko, T., Antal, A., et al. (2014). A sensory neuron-expressed IL-31 receptor mediates T helper cell-dependent itch: Involvement of TRPV1 and TRPA1. J. Allergy Clin. Immunol. 133 (2), 448–460. doi:10.1016/j.jaci.2013.10.048
Chan, T. C., Lee, M. S., Huang, W. C., Chang, W. Y., Krueger, J. G., and Tsai, T. F. (2021). Capsaicin attenuates imiquimod-induced epidermal hyperplasia and cutaneous inflammation in a murine model of psoriasis. Biomed. Pharmacother. 141, 111950. doi:10.1016/j.biopha.2021.111950
Chizh, B. A., O'Donnell, M. B., Napolitano, A., Wang, J., Brooke, A. C., Aylott, M. C., et al. (2007). The effects of the TRPV1 antagonist SB-705498 on TRPV1 receptor-mediated activity and inflammatory hyperalgesia in humans. Pain 132 (1-2), 132–141. doi:10.1016/j.pain.2007.06.006
Cho, S., Shin, M. H., Kim, Y. K., Seo, J. E., Lee, Y. M., Park, C. H., et al. (2009). Effects of infrared radiation and heat on human skin aging in vivo. J. Investig. Dermatol Symp. Proc. 14 (1), 15–19. doi:10.1038/jidsymp.2009.7
Choi, T. Y., Park, S. Y., Jo, J. Y., Kang, G., Park, J. B., Kim, J. G., et al. (2009). Endogenous expression of TRPV1 channel in cultured human melanocytes. J. Dermatol Sci. 56 (2), 128–130. doi:10.1016/j.jdermsci.2009.07.002
ClinicalTrials.gov (2017). ClinicalTrials.gov. Available at: https://clinicaltrials.gov/ct2/show/NCT02583022. [Accessed 8 28 2017].
Cubillos, S., and Norgauer, J. (2016). Low vitamin D-modulated calcium-regulating proteins in psoriasis vulgaris plaques: S100A7 overexpression depends on joint involvement. Int. J. Mol. Med. 38 (4), 1083–1092. doi:10.3892/ijmm.2016.2718
Datsi, A., Steinhoff, M., Ahmad, F., Alam, M., and Buddenkotte, J. (2021). Interleukin-31: The "itchy" cytokine in inflammation and therapy. Allergy 76 (10), 2982–2997. doi:10.1111/all.14791
de Moura, J. C., Noroes, M. M., Rachetti Vde, P., Soares, B. L., Preti, D., Nassini, R., et al. (2014). The blockade of transient receptor potential ankirin 1 (TRPA1) signalling mediates antidepressant- and anxiolytic-like actions in mice. Br. J. Pharmacol. 171 (18), 4289–4299. doi:10.1111/bph.12786
Denda, M., Fuziwara, S., Inoue, K., Denda, S., Akamatsu, H., Tomitaka, A., et al. (2001). Immunoreactivity of VR1 on epidermal keratinocyte of human skin. Biochem. Biophysical Res. Commun. 285 (5), 1250–1252. doi:10.1006/bbrc.2001.5299
Denda, M., Sokabe, T., Fukumi-Tominaga, T., and Tominaga, M. (2007). Effects of skin surface temperature on epidermal permeability barrier homeostasis. J. Invest. Dermatol 127 (3), 654–659. doi:10.1038/sj.jid.5700590
Garcia-Sanz, N., Fernandez-Carvajal, A., Morenilla-Palao, C., Planells-Cases, R., Fajardo-Sanchez, E., Fernandez-Ballester, G., et al. (2004). Identification of a tetramerization domain in the C terminus of the vanilloid receptor. J. Neurosci. 24 (23), 5307–5314. doi:10.1523/JNEUROSCI.0202-04.2004
Haltaufderhyde, K. D., and Oancea, E. (2014). Genome-wide transcriptome analysis of human epidermal melanocytes. Genomics 104, 482–489. doi:10.1016/j.ygeno.2014.09.010
Han, S. B., Kim, H., Cho, S. H., Lee, J. D., Chung, J. H., and Kim, H. S. (2016). Transient receptor potential vanilloid-1 in epidermal keratinocytes may contribute to acute pain in herpes zoster. Acta Derm. Venereol. 96 (3), 319–322. doi:10.2340/00015555-2247
Harada, N., Okajima, K., Arai, M., Kurihara, H., and Nakagata, N. (2007). Administration of capsaicin and isoflavone promotes hair growth by increasing insulin-like growth factor-I production in mice and in humans with alopecia. Growth Horm. IGF Res. 17 (5), 408–415. doi:10.1016/j.ghir.2007.04.009
Hayes, P. M. H., Gunthorpe, M. J., Harries, M. H., Duckworth, M. D., Cairns, W., Harrison, D. C., et al. (2000). Cloning and functional expression of a human orthologue of rat vanilloid receptor-1. Pain 88 (2), 205–215. doi:10.1016/s0304-3959(00)00353-5
Holzer, P. (2008). The pharmacological challenge to tame the transient receptor potential vanilloid-1 (TRPV1) nocisensor. Br. J. Pharmacol. 155 (8), 1145–1162. doi:10.1038/bjp.2008.351
Huang, K. F., Ma, K. H., Chang, Y. J., Lo, L. C., Jhap, T. Y., Su, Y. H., et al. (2019). Baicalein inhibits matrix metalloproteinase 1 expression via activation of TRPV1-Ca-ERK pathway in ultraviolet B-irradiated human dermal fibroblasts. Exp. Dermatol 28 (5), 568–575. doi:10.1111/exd.13912
Imamachi, N. P. G., Lee, H., Anderson, D. J., Simon, M. I., Basbaum, A. I., Han, S. K., et al. (2009). TRPV1-expressing primary afferents generate behavioral responses to pruritogens via multiple mechanisms. Proc. Natl. Acad. Sci. U. S. A. 106 (27), 11330–11335. doi:10.1073/pnas.0905605106
Inoue, K., Koizumi, S., Fuziwara, S., Denda, S., Inoue, K., and Denda, M. (2002). Functional vanilloid receptors in cultured normal human epidermal keratinocytes. Biochem. Biophys. Res. Commun. 291 (1), 124–129. doi:10.1006/bbrc.2002.6393
Inoue, R., Jian, Z., and Kawarabayashi, Y. (2009). Mechanosensitive TRP channels in cardiovascular pathophysiology. Pharmacol. Ther. 123 (3), 371–385. doi:10.1016/j.pharmthera.2009.05.009
Kaszas, K., Keller, J. M., Coddou, C., Mishra, S. K., Hoon, M. A., Stojilkovic, S., et al. (2012). Small molecule positive allosteric modulation of TRPV1 activation by vanilloids and acidic pH. J. Pharmacol. Exp. Ther. 340 (1), 152–160. doi:10.1124/jpet.111.183053
Kim, B. M., Lee, S. H., Shim, W. S., and Oh, U. (2004). Histamine-induced Ca(2+) influx via the PLA(2)/lipoxygenase/TRPV1 pathway in rat sensory neurons. Neurosci. Lett. 361 (1-3), 159–162. doi:10.1016/j.neulet.2004.01.019
Kusumaningrum, N. L. D., Yoon, H. S., Park, C. H., and Chung, J. H. (2018). Ultraviolet light-induced gasdermin C expression is mediated via TRPV1calciumcalcineurinNFATc1 signaling. Int. J. Mol. Med. 42 (5), 2859–2866. doi:10.3892/ijmm.2018.3839
Lan, C. C., Wu, C. S., and Yu, H. S. (2013). Solar-simulated radiation and heat treatment induced metalloproteinase-1 expression in cultured dermal fibroblasts via distinct pathways: Implications on reduction of sun-associated aging. J. Dermatol Sci. 72 (3), 290–295. doi:10.1016/j.jdermsci.2013.07.015
Lee, H., Hong, Y., and Kim, M. (2021). Structural and functional changes and possible molecular mechanisms in aged skin. Int. J. Mol. Sci. 22 (22), 12489. doi:10.3390/ijms222212489
Lee, S. E., and Lee, S. H. (2018). Skin barrier and calcium. Ann. Dermatol 30 (3), 265–275. doi:10.5021/ad.2018.30.3.265
Lee, S. H., Tonello, R., Im, S. T., Jeon, H., Park, J., Ford, Z., et al. (2020). Resolvin D3 controls mouse and human TRPV1-positive neurons and preclinical progression of psoriasis. Theranostics 10 (26), 12111–12126. doi:10.7150/thno.52135
Lee, Y. M., Kim, Y. K., and Chung, J. H. (2009). Increased expression of TRPV1 channel in intrinsically aged and photoaged human skin in vivo. Exp. Dermatol 18 (5), 431–436. doi:10.1111/j.1600-0625.2008.00806.x
Lee, Y. M., Kim, Y. K., Kim, K. H., Park, S. J., Kim, S. J., and Chung, J. H. (2009). A novel role for the TRPV1 channel in UV-induced matrix metalloproteinase (MMP)-1 expression in HaCaT cells. J. Cell Physiol. 219 (3), 766–775. doi:10.1002/jcp.21729
Lee, Y. M., Li, W. H., Kim, Y. K., Kim, K. H., and Chung, J. H. (2008). Heat-induced MMP-1 expression is mediated by TRPV1 through PKCalpha signaling in HaCaT cells. Exp. Dermatol 17 (10), 864–870. doi:10.1111/j.1600-0625.2008.00738.x
Li, H-J., Kanazawa, N., Kimura, A., Kaminaka, C., Yonei, N., Yamamoto, Y., et al. (2012). Severe ulceration with impaired induction of growth factors and cytokines in keratinocytes after trichloroacetic acid application on TRPV1-deficient mice. Eur. J. Dermatology 22 (5), 614–621. doi:10.1684/ejd.2012.1788
Li, W. H., Lee, Y. M., Kim, J. Y., Kang, S., Kim, S., Kim, K. H., et al. (2007). Transient receptor potential vanilloid-1 mediates heat-shock-induced matrix metalloproteinase-1 expression in human epidermal keratinocytes. J. Invest. Dermatol 127 (10), 2328–2335. doi:10.1038/sj.jid.5700880
Lishko, P. V., Procko, E., Jin, X., Phelps, C. B., and Gaudet, R. (2007). The ankyrin repeats of TRPV1 bind multiple ligands and modulate channel sensitivity. Neuron 54 (6), 905–918. doi:10.1016/j.neuron.2007.05.027
Losquadro, W. D. (2017). Anatomy of the skin and the pathogenesis of nonmelanoma skin cancer. Facial Plast. Surg. Clin. North Am. 25 (3), 283–289. doi:10.1016/j.fsc.2017.03.001
Loyd, D. R., Chen, P. B., and Hargreaves, K. M. (2012). Anti-hyperalgesic effects of anti-serotonergic compounds on serotonin- and capsaicin-evoked thermal hyperalgesia in the rat. Neuroscience 203, 207–215. doi:10.1016/j.neuroscience.2011.12.019
Martinez-Martinez, E., Galvan-Hernandez, C. I., Toscano-Marquez, B., and Gutierrez-Ospina, G. (2012). Modulatory role of sensory innervation on hair follicle stem cell progeny during wound healing of the rat skin. PLoS One 7 (5), e36421. doi:10.1371/journal.pone.0036421
Matsumura, Y., and Ananthaswamy, H. N. (2004). Toxic effects of ultraviolet radiation on the skin. Toxicol. Appl. Pharmacol. 195 (3), 298–308. doi:10.1016/j.taap.2003.08.019
Middleton, S. J., Perez-Sanchez, J., and Dawes, J. M. (2021). The structure of sensory afferent compartments in health and disease. J. Anat. 241, 1186. doi:10.1111/joa.13544
Miyazaki, A., Ohkubo, T., Hatta, M., Ishikawa, H., and Yamazaki, J. (2015). Integrin α6β4 and TRPV1 channel coordinately regulate directional keratinocyte migration. Biochem. Biophys. Res. Commun. 458 (1), 161–167. doi:10.1016/j.bbrc.2015.01.086
Morales-Lázaro, S. L., Llorente, I., Sierra-Ramírez, F., López-Romero, A. E., Ortíz-Rentería, M., Serrano-Flores, B., et al. (2016). Inhibition of TRPV1 channels by a naturally occurring omega-9 fatty acid reduces pain and itch. Nat. Commun. 7 (1), 13092. doi:10.1038/ncomms13092
Nagy, I., Santha, P., Jancso, G., and Urban, L. (2004). The role of the vanilloid (capsaicin) receptor (TRPV1) in physiology and pathology. Eur. J. Pharmacol. 500 (1-3), 351–369. doi:10.1016/j.ejphar.2004.07.037
Naik, S. (2022). One size does not fit all: Diversifying immune function in the skin. J. Immunol. 208 (2), 227–234. doi:10.4049/jimmunol.2100758
Nakagawa, H. H. A., and Hiura, A. (2013). Four possible itching pathways related to the TRPV1 channel, histamine, PAR-2 and serotonin. Malays J. Med. Sci. 20 (4), 5–12.
Nakahara, T., Kido-Nakahara, M., Tsuji, G., and Furue, M. (2021). Basics and recent advances in the pathophysiology of atopic dermatitis. J. Dermatol 48 (2), 130–139. doi:10.1111/1346-8138.15664
Nattkemper, L. A., Tey, H. L., Valdes-Rodriguez, R., Lee, H., Mollanazar, N. K., Albornoz, C., et al. (2018). The genetics of chronic itch: Gene expression in the skin of patients with atopic dermatitis and psoriasis with severe itch. J. Invest. Dermatol 138 (6), 1311–1317. doi:10.1016/j.jid.2017.12.029
NatureChuang, H. H., Prescott, E. D., Kong, H., Shields, S., Jordt, S. E., Basbaum, A. I., et al. (2001). Bradykinin and nerve growth factor release the capsaicin receptor from PtdIns(4,5)P2-mediated inhibition. Nature 411 (6840), 957–962. doi:10.1038/35082088
Olusegun, O. A., and Martincigh, B. S. (2021). Allergic contact dermatitis: A significant environmental and occupational skin disease. Int. J. Dermatol 60 (9), 1082–1091. doi:10.1111/ijd.15502
Orsmond, A., Bereza-Malcolm, L., Lynch, T., March, L., and Xue, M. (2021). Skin barrier dysregulation in psoriasis. Int. J. Mol. Sci. 22 (19), 10841. doi:10.3390/ijms221910841
Ozcan, S. S., Gurel, G., and Cakir, M. (2021). Gene expression profiles of transient receptor potential (TRP) channels in the peripheral blood mononuclear cells of psoriasis patients. Hum. Exp. Toxicol. 40 (8), 1234–1240. doi:10.1177/0960327121991911
Pedić, L., Pondeljak, N., and Šitum, M. (2020). Recent information on photoaging mechanisms and the preventive role of topical sunscreen products. Acta Dermatovenerologica Alpina Pannonica Adriatica 29 (4), 201–207. doi:10.15570/actaapa.2020.40
Phelps, C. B., Wang, R. R., Choo, S. S., and Gaudet, R. (2010). Differential regulation of TRPV1, TRPV3, and TRPV4 sensitivity through a conserved binding site on the ankyrin repeat domain. J. Biol. Chem. 285 (1), 731–740. doi:10.1074/jbc.M109.052548
Pourang, A., Tisack, A., Ezekwe, N., Torres, A. E., Kohli, I., Hamzavi, I. H., et al. (2022). Effects of visible light on mechanisms of skin photoaging. Photodermatol. Photoimmunol. Photomed. 38 (3), 191–196. doi:10.1111/phpp.12736
Riol-Blanco, L., Ordovas-Montanes, J., Perro, M., Naval, E., Thiriot, A., Alvarez, D., et al. (2014). Nociceptive sensory neurons drive interleukin-23-mediated psoriasiform skin inflammation. Nature 510 (7503), 157–161. doi:10.1038/nature13199
Roblin, D., Yosipovitch, G., Boyce, B., Robinson, J., Sandy, J., Mainero, V., et al. (2015). Topical TrkA kinase inhibitor CT327 is an effective, novel therapy for the treatment of pruritus due to psoriasis: Results from experimental studies, and efficacy and safety of CT327 in a phase 2b clinical trial in patients with psoriasis. Acta Derm. Venereol. 95 (5), 542–548. doi:10.2340/00015555-2047
Rosamilia, L. L. (2020). Herpes zoster presentation, management, and prevention: A modern case-based review. Am. J. Clin. Dermatol 21 (1), 97–107. doi:10.1007/s40257-019-00483-1
Samanta, A., Hughes, T. E. T., and Moiseenkova-Bell, V. Y. (2018). Transient receptor potential (TRP) channels. Subcell. Biochem. 87, 141–165. doi:10.1007/978-981-10-7757-9_6
Sappington, R. M., Sidorova, T., Long, D. J., and Calkins, D. J. (2009). TRPV1: Contribution to retinal ganglion cell apoptosis and increased intracellular Ca2+ with exposure to hydrostatic pressure. Invest. Ophthalmol. Vis. Sci. 50 (2), 717–728. doi:10.1167/iovs.08-2321
Shim, W. S., Tak, M. H., Lee, M. H., Kim, M., Kim, M., Koo, J. Y., et al. (2007). TRPV1 mediates histamine-induced itching via the activation of phospholipase A2 and 12-lipoxygenase. J. Neurosci. 27 (9), 2331–2337. doi:10.1523/JNEUROSCI.4643-06.2007
Sinning, C., Watzer, B., De Petrocellis, L., Di Marzo, V., and Imming, P. (2008). Dopamides, vanillylamides, ethanolamides, and arachidonic acid amides of anti-inflammatory and analgesic drug substances as TRPV1 ligands. ChemMedChem 3 (12), 1956–1964. doi:10.1002/cmdc.200800271
Song, J., Xian, D., Yang, L., Xiong, X., Lai, R., and Pruritus, Z. J. (2018). Pruritus: Progress toward pathogenesis and treatment. Biomed. Res. Int. 2018, 9625936. doi:10.1155/2018/9625936
Ständer, S., Moormann, C., Schumacher, M., Buddenkotte, J., Artuc, M., and Shpacovitch, , et al. (2004). Expression of vanilloid receptor subtype 1 in cutaneous sensory nerve fibers, mast cells, and epithelial cells of appendage structures. Exp. Dermatol 13 (3), 129–139. doi:10.1111/j.0906-6705.2004.0178.x
Steinhoff, M., Neisius, U., Ikoma, A., Fartasch, M., Heyer, G., and Skov, P. S., et al. (2003). Proteinase-activated receptor-2 mediates itch a novel pathway for pruritus in human skin. J. Neurosci. 23 (15), 6176–6180. doi:10.1523/JNEUROSCI.23-15-06176.2003
Sulk, M., Seeliger, S., Aubert, J., Schwab, V. D., Cevikbas, F., Rivier, M., et al. (2012). Distribution and expression of non-neuronal transient receptor potential (TRPV) ion channels in rosacea. J. Invest. Dermatol 132 (4), 1253–1262. doi:10.1038/jid.2011.424
Tang, L., Gao, J., Cao, X., Chen, L., Wang, H., and Ding, H. (2022). TRPV1 mediates itch-associated scratching and skin barrier dysfunction in DNFB-induced atopic dermatitis mice. Exp. Dermatol 31 (3), 398–405. doi:10.1111/exd.14464
Thio, H. B., Zomerdijk, T. P., Oudshoorn, C., Kempenaar, J., Nibbering, P. H., and van der Schroeff, J. G., et al. (1994). Fumaric acid derivatives evoke a transient increase in intracellular free calcium concentration and inhibit the proliferation of human keratinocytes. Br. J. Dermatol 131 (6), 856–861. doi:10.1111/j.1365-2133.1994.tb08589.x
Tominaga, M., Caterina M. J., Malmberg, A. B., Rosen, T. A., Gilbert, H., and Skinner, K., et al. (1998). The cloned capsaicin receptor integrates multiple pain-producing stimuli. Neuron 21 (3), 531–543. doi:10.1016/s0896-6273(00)80564-4
Tsutsumi, M., Kumamoto, J., and Denda, M. (2011). Intracellular calcium response to high temperature is similar in undifferentiated and differentiated cultured human keratinocytes. Exp. Dermatol 20 (10), 839–840. doi:10.1111/j.1600-0625.2011.01318.x
Umar, M., Sastry, K. S., Al Ali, F., Al-Khulaifi, M., Wang, E., and Chouchane, A. I. (2018). Vitamin D and the pathophysiology of inflammatory skin diseases. Skin. Pharmacol. Physiol. 31 (2), 74–86. doi:10.1159/000485132
van Zuuren, E. J., Arents, B. W. M., van der Linden, M. M. D., Vermeulen, S., Fedorowicz, Z., and Tan, J. (2021). Rosacea: New concepts in classification and treatment. Am. J. Clin. Dermatol 22 (4), 457–465. doi:10.1007/s40257-021-00595-7
Venkatachalam, K., and Montell, C. (2007). TRP channels. Annu. Rev. Biochem. 76, 387–417. doi:10.1146/annurev.biochem.75.103004.142819
Vicic, M., Kastelan, M., Brajac, I., Sotosek, V., and Massari, L. P. (2021). Current concepts of psoriasis immunopathogenesis. Int. J. Mol. Sci. 22 (21), 11574. doi:10.3390/ijms222111574
Williams, K. A., Roh, Y. S., Brown, I., Sutaria, N., Bakhshi, P., Choi, J., et al. (2021). Pathophysiology, diagnosis, and pharmacological treatment of prurigo nodularis. Expert Rev. Clin. Pharmacol. 14 (1), 67–77. doi:10.1080/17512433.2021.1852080
Wu, Q., Bai, P., Guo, H., Guo, M. S. S., Xia, Y., Xia, Y., et al. (2022). Capsaicin, a phytochemical from chili pepper, alleviates the ultraviolet irradiation-induced decline of collagen in dermal fibroblast via blocking the generation of reactive oxygen species. Front. Pharmacol. 13, 872912. doi:10.3389/fphar.2022.872912
Yun, J. W., Seo, J. A., Jang, W. H., Koh, H. J., Bae, I. H., Park, Y. H., et al. (2011). Antipruritic effects of TRPV1 antagonist in murine atopic dermatitis and itching models. J. Invest. Dermatol 131 (7), 1576–1579. doi:10.1038/jid.2011.87
Yun, J. W., Seo, J. A., Jeong, Y. S., Bae, I. H., Jang, W. H., Lee, J., et al. (2011). TRPV1 antagonist can suppress the atopic dermatitis-like symptoms by accelerating skin barrier recovery. J. Dermatol Sci. 62 (1), 8–15. doi:10.1016/j.jdermsci.2010.10.014
Zhong, B., Ma, S., and Wang, D. H. (2021). Ablation of TRPV1 abolishes salicylate-induced sympathetic activity suppression and exacerbates salicylate-induced renal dysfunction in diet-induced obesity. Cells 10 (5), 1234. doi:10.3390/cells10051234
Keywords: TRPV1, Ca 2+, skin aging, inflammatory skin diseases, therapeutic strategy
Citation: Xiao T, Sun M, Zhao C and Kang J (2023) TRPV1: A promising therapeutic target for skin aging and inflammatory skin diseases. Front. Pharmacol. 14:1037925. doi: 10.3389/fphar.2023.1037925
Received: 06 September 2022; Accepted: 20 January 2023;
Published: 15 February 2023.
Edited by:
Fan-Rong Wu, Anhui Medical University, ChinaReviewed by:
Christelle Ianotto, Centre Hospitalier Regional Universitaire (CHU) de Brest, FranceMahmoudreza Hadjighassem, Tehran University of Medical Sciences, Iran
Sara L. Morales-Lázaro, National Autonomous University of Mexico, Mexico
Copyright © 2023 Xiao, Sun, Zhao and Kang. This is an open-access article distributed under the terms of the Creative Commons Attribution License (CC BY). The use, distribution or reproduction in other forums is permitted, provided the original author(s) and the copyright owner(s) are credited and that the original publication in this journal is cited, in accordance with accepted academic practice. No use, distribution or reproduction is permitted which does not comply with these terms.
*Correspondence: Jingjing Kang, amluZzAyMDk5OTlAMTYzLmNvbQ==
†These authors have contributed equally to this work and share first authorship