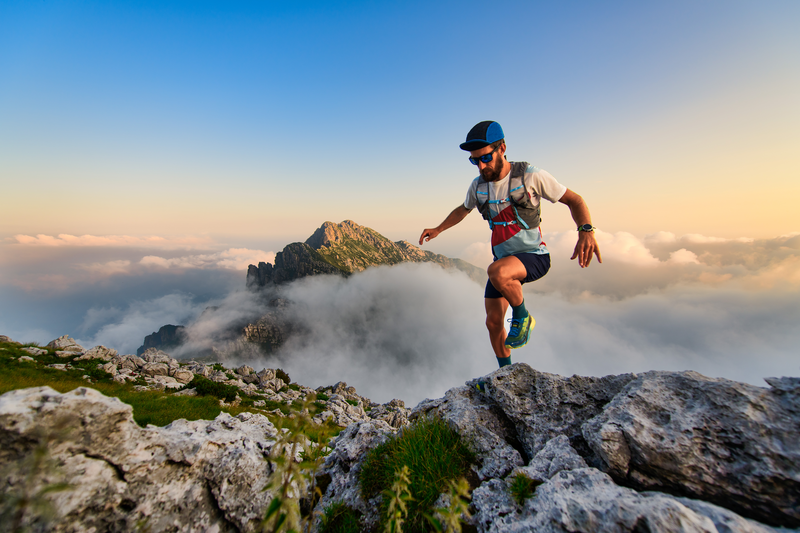
94% of researchers rate our articles as excellent or good
Learn more about the work of our research integrity team to safeguard the quality of each article we publish.
Find out more
REVIEW article
Front. Pharmacol. , 19 January 2023
Sec. Inflammation Pharmacology
Volume 14 - 2023 | https://doi.org/10.3389/fphar.2023.1003658
This article is part of the Research Topic The Role of Natural Bioactive Molecules in Inflammatory Injury View all 8 articles
Sepsis is a common but critical illness in patients admitted to the intensive care unit and is associated with high mortality. Although there are many treatments for sepsis, specific and effective therapies are still lacking. For over 2,000 years, traditional Chinese medicine (TCM) has played a vital role in the treatment of infectious diseases in Eastern countries. Both anecdotal and scientific evidence show that diverse TCM preparations alleviate organ dysfunction caused by sepsis by inhibiting the inflammatory response, reducing oxidative stress, boosting immunity, and maintaining cellular homeostasis. This review reports on the efficacy and mechanism of action of various TCM compounds, herbal monomer extracts, and acupuncture, on the treatment of sepsis and related multi-organ injury. We hope that this information would be helpful to better understand the theoretical basis and empirical support for TCM in the treatment of sepsis.
Sepsis is one of the leading causes of death from critical diseases, affecting each year more than 30 million people worldwide (Fleischmann et al., 2016). Sepsis is defined as a life-threatening organ dysfunction caused by the host’s dysfunctional response to infection, and is diagnosed based on a Sequential [Sepsis-related] Organ Failure Assessment (SOFA) score ≥2 (Singer et al., 2016). This definition highlights the importance of the systemic inflammatory response caused by immune disturbance. Pattern recognition receptors of innate immune cells recognize highly conserved microbial pathogen-associated molecular patterns (PAMPs), which activate signaling pathways, e.g., mitogen activated protein kinase (MAPK) and nuclear factor-κB (NF-κB), thus triggering the production and secretion of pro-inflammatory cytokines such as tumor necrosis factor alpha (TNF-α) and interleukin 1 (IL-1), IL-2, IL-6, and IL-8. These cytokines promote the adhesion between neutrophils and endothelial cells, which is followed by the activation of complement and blood coagulation cascades, and eventually lead to disseminated intravascular coagulation (Boyd et al., 2014; Huang et al., 2019). According to conventional view, after this early hyperinflammatory state, a subsequent hypo-inflammatory state partly from the release of anti-inflammatory cytokines such as IL-4 and IL-10, results in widely immune suppression. However, newer paradigms indicate a phase at which the pro-inflammatory and immunosuppression may occur simultaneously, and the complex interactions between host (genetics and comorbidities) and pathogen (type, virulence, and burden) is the leading factor (Cecconi et al., 2018).
Despite recent advances in anti-infective therapy and advanced life support, the high mortality rate of sepsis remains an urgent clinical challenge. High medical costs and possible sequela such as renal insufficiency and cognitive impairment make sepsis a global public health issue (Singer et al., 2016). In addition, one-sixth of survivors of sepsis frequently suffer from long-term impairments such as physical, cognitive, and organ function (Delano and Ward, 2016; Prescott and Angus, 2018). Accelerated progression of preexisting chronic conditions, residual organ damage, and impaired immune function accounts for the deterioration of health after sepsis (Prescott and Angus, 2018). Since a sustained, uncontrolled inflammatory response is regarded as key factor promoting the onset of sepsis and multiple organ damage, novel anti-inflammatory therapies are eagerly pursued for sepsis treatment. However, clinical trials aimed at blocking cytokine responses, such those testing TNF-α inhibitors and Toll-like receptor 4 (TLR4)/myeloid differentiation factor 2 (MD2) antagonists, failed to decrease the mortality rate of patients with septic (Fisher et al., 1996; Opal et al., 2013). In view of the limited treatment options, complementary and replacement therapies are increasingly investigated to develop new therapeutic measures to treat sepsis and related organ damage.
As an alternative and complementary therapy, TCM is increasingly recognized for its efficacy and safety in the treatment of diseases. For instance, in the last 2 years, TCM has been shown to improve immunity and alleviate fever and other symptoms of COVID-19 (Kang et al., 2022). Indeed, TCM offers unique advantages in the treatment of inflammatory diseases such as sepsis (classified as “exogenous fever disease” in TCM) (Li C et al., 2018; Lu Z.B. et al., 2020). According to TCM tenets, the main principles of sepsis treatment are clearing away heat and detoxifying, clearing the internal organs and expelling heat, promoting blood circulation and removing blood stasis, and strengthening the body and solidifying the detoxification. Many studies, including some clinical trials, have reported the effectiveness of TCM in suppressing inflammatory pathways, regulating the immune response, and inhibiting oxidative stress (Gao et al., 2019; Song Y et al., 2019; Xia et al., 2019; Shang et al., 2020). Evidence supports as well the potential of TCM compounds, herbal extracts, and electroacupuncture in the prevention and treatment of heart-, brain-, lung-, and intestine-related diseases (Kim et al., 2007; Liu et al., 2018; Nabavi et al., 2018; Pang et al., 2018; Zhang W et al., 2019; Zhang X et al., 2019; Liu S et al., 2020). Therefore, this review aims to summarize the efficacy and mechanism of action of TCM compounds, herbal extracts, and electroacupuncture, in the treatment of sepsis and sepsis-related multiple organ damage.
TCM compounds are prescriptions consisting of two or more substances that provide multi-target synergistic effects (Sun et al., 2017). Through mutual compatibility of different chemical substances, components of TCM herbs react with each other, thereby lowering toxicity and adverse side effects and enhancing the therapeutic effects (Zhang R et al., 2019). Several TCM compounds, such as Xuebijing injection, Shenfu injection, Huanglian Jiedu decoction, Dachengqi decoction, and Xijiao Dihuang decoction, demonstrated efficacy in the treatment of sepsis-related organ injury.
Xuebijing injection (XBJI) is an injectable prescription obtained from a combination of carthami flos, paeoniae radix rubra, szechuan lovage rhizome, angelicae sinensis radix, and salviae miltiorrhizae (Li et al., 2021) that shows distinct anti-inflammatory activities in several settings. XBJI decreased the expression of IL-6, TNF-α, IL-1β, and IL-12 in mouse macrophages stimulated by Pam3CSK4 (a synthetic tripalmitoylated lipopeptide mimicking bacterial lipoproteins) (Li T et al., 2020). Its pharmacology targets are the NF-κB and MAPK pathways, and its effects are manifested by inhibition of the phosphorylation of IKKα/β, IκBα, p65 NF-κB, and JNK (Li T et al., 2020). High-dose XBJI increased the number of T-regs, reduced the number of Th-17 T cells, downregulated the expression of inflammatory cytokines such as IL-6 and TNF-α, inhibited the infiltration of neutrophils in lung and kidney tissues, and improved survival in cecal ligation and puncture (CLP) model mice (Chen et al., 2018). In another study addressing also the mouse CLP sepsis model, XBJI administration significantly improved renal microvascular perfusion and oxygenation and inhibited renal expression of IL-1β, IL-6, TNF-α, and high mobility group box 1 (HMGB1) protein, although without affecting the survival rate (Liu J et al., 2021).
Shenfu injection (SFI) is mainly composed of ginsenosides and aconitine alkaloids (Liu et al., 2019). SFI was reported to exert antioxidant, anti-inflammatory, anti-apoptotic, and immunoregulatory effects in a rabbit model of lipopolysaccharide (LPS)-induced septic shock. SFI decreased serum levels of lactate dehydrogenase (LDH) and aminotransferase (AST), improved myocardial metabolism, and protected tissue morphology in the heart, liver, and kidney (Liu et al., 2019). SFI also suppressed inflammatory markers, such as TNF-α and IL-1β, in serum and heart of LPS-treated rats, and disrupted inflammatory signal transduction mediated by the mitogen-activated protein kinase repalmitoylated (MEK) and extracellular regulated protein kinase (ERK) pathways by decreasing p-MEK and p-ERK expression in LPS-stimulated H9C2 cells (Chen et al., 2020). Besides, SFI upregulated the expression of B cell lymphoma-2 (Bcl-2) and lowered the expression of Bid, t-Bid, and caspase-9, thus reducing cardiomyocyte apoptosis and attenuating myocardial injury in septic rats (Xu et al., 2020). In turn, beneficial effects of SFI on patients with septic shock were manifested by increased CD4+ and CD8+ T cells in peripheral blood, upregulated expression of human leukocyte antigen DR (HLA-DR) in monocytes, and enhanced cellular immunity (Zhang N et al., 2017). Importantly, clinical trials on patients with septic showed that SFI combined with conventional treatment led to significant improvement of clinical symptoms and prognosis, without obvious adverse reactions (Wen-Ting et al., 2012; Mo et al., 2014; Zhang Q et al., 2017; Wang X et al., 2019).
Shengmai injection (SMI), consisting of extracts from panax ginseng, ophiopogon japonicas, and schisandra chinensis, is one of the most widely used TCM prescriptions. According to the basic theory of TCM, simple tonic drugs like SMI cannot normally be used to treat infectious disease. However, in animal models of cardiac disease, marked improvement of myocardial metabolism was observed after treatment with SMI (Li et al., 2019; Cao et al., 2020). Specifically, SMI enhanced fatty acid and glucose oxidation, promoted mitochondrial biogenesis, and inhibited apoptosis by activating the AMPK signaling pathway in cardiomyocytes rendered hypertrophic by exposure to angiotensin II (Li et al., 2019). Meanwhile, SMI upregulated the expression of PTEN-induced kinase 1 (Pink1) and Parkin RBR E3 ubiquitin-protein ligase (Parkin), therefore improving myocardial mitophagy, in a mouse model of septic cardiomyopathy (Cao et al., 2020). Furthermore, SMI was shown to improve immune function and prolong survival in mice with CLP-induced peritonitis (Yu et al., 2005).
Huanglian Jiedu decoction (HLJDD) consist of rhizoma coptidis, radix scutellariae, cortex phellodendri, and fructus gardeniae and has been widely used in the treatment of inflammatory diseases (Lu Z et al., 2020). The beneficial regulatory role of HLJDD in lipid homeostasis represents the key mechanism of its anti-inflammatory actions. Upon LPS-induced inflammation in zebrafish, HLJDD ameliorated lipid imbalance mainly through the glycerophospholipid metabolism pathway. By normalizing the production of proinflammatory lipid intermediates, this effect was proposed to underlie TLR4/myeloid differentiation factor 88 (MyD88)/NF-κB pathway inhibition and reduced secretion of IL-6, IL-1β, TNF-α, and IFN-γ (Zhou et al., 2019). Notably, berberine, baicalin, and gardenin, the main components of HLJDD, were shown to play a protective role against sepsis-related multi-organ damage by binding to lipid A to neutralize LPS activity. This resulted in inhibition of IL-6, TNF-α, and IFN-γ secretion, as well as reduced synthesis of pathological lipid markers (Chen G et al., 2017). In a rat model of LPS-induced gingivitis, HLJDD administration suppressed serum inflammatory cytokines, lowered malondialdehyde (MDA) and reactive oxygen species (ROS) production, and upregulated total antioxidant capacity in periodontitis lysates. These effects were correlated with inhibition of AMPK and ERK1/2 expression (Zhang F et al., 2018).
Dachengqi decoction (DCQD) is composed of extracts from rheum palmatum l, magnolia henryi dunn, citrus aurantium l, and natrii sulfas. In a mouse model of LPS-induced acute lung injury (ALI), DCQD treatment inhibited TLR4/NF-κB signaling and IL-6, IL-8, and TNF-α secretion in lung tissue, and reduced pulmonary edema by upregulating aquaporin (AQP) 1/5 expression (Hu et al., 2019). Another study showed that DQCD attenuated intestinal vascular endothelial injury in rats with severe acute pancreatitis (SAP) induced by cerulein and LPS, and decreased matrix metalloproteinase 9 (MMP-9) and junctional adhesion molecule C (JAM-C) expression, while increasing AQP-1 expression, in TNF-α-treated vascular endothelial cells (Pan et al., 2017). Further in vivo and in vitro research on the SAP rat model indicated that DCQD inhibited the phosphatidylinositol 3-kinase (PI3K)/protein kinase B (AKT) signaling pathway, thereby inhibiting inflammation by decreasing production of IL-1β, IL-6, and TNF-α and promoting apoptosis of pancreatic acinar cells (Sun et al., 2020).
The Xijiao Dihuang decoction (XJDHD) comprises rehmannia, peony, cortex moudan, and cornu bubali and is used in China for the treatment of sepsis (Lu et al., 2020b). XJDHD administration was shown to improve the survival rate of rats subjected to CLP, as well as of cultured macrophages, by inhibiting aerobic glycolysis triggered by the TLR4/hypoxia-inducible factor 1α (HIF-1α)/pyruvate kinase M2 (PKM2) pathway (Lu et al., 2020b). Additional research on the above sepsis models further indicated that prolonged survival correlated with XJDHD-mediated inhibition of HIF-1a and p65 (Lu et al., 2020a).
Tanshinone IIA (TSA) occurs in the dried roots and rhizomes of salvia miltiorrhiza (lamiaceae), and is one of the main pharmacologically active components of hydrophilic tanshinones (Wang N et al., 2020). TSA has anti-inflammatory activity by inhibiting a variety of cytokines. In LPS-stimulated bone marrow-derived macrophages (BMDMs), TSA exposure inhibited succinate dehydrogenase (SDH)-mediated IL-1β and IL-6 production and blocked BMDM polarization towards the M1 phenotype (Liu Q.Y. et al., 2021). In addition, suggesting beneficial effects against neuroinflammatory and neurotoxic insults, TSA pretreatment was shown to attenuate pro-inflammatory cytokine secretion through inhibition of TLR4, MyD88, and TNF receptor associated factor 6 (TRAF6) expression and subsequent repression of signaling through the NF-κB and MAPK pathways in LPS-treated human U87 astrocytoma cells (Jin et al., 2020). TSA treatment also reduced calcium inflow, inhibited transient receptor potential melastatin 7 (TRPM7), and suppressed the release of pro-inflammatory cytokines in pulmonary interstitial macrophages from rats with sepsis-induced ALI (Li J et al., 2018). Meanwhile, incubation with Salvia miltiorrhiza extract decreased LPS-induced phosphorylation of IκB-α and IKK, thus inhibiting NF-κB activity, inhibited MAPK phosphorylation, and disrupted TLR4 dimerization to prevent TLR4-MyD88 complex formation in RAW264.7 cells (Gao et al., 2017).
Astragaloside IV (AS-IV) is a small saponin obtained from the roots of astragalus membranaceus (Xia et al., 2020). AS-IV has anti-inflammatory and neuroprotective activities. Studies in the CLP mouse model showed that AS-IV treatment inhibited the secretion of inflammatory cytokines and downregulated the expression of NLRP3, apoptosis associated speck like protein containing CARD (ASC), and cleaved caspase-1 in both intestinal tissue and BALF, thus reducing multi-organ injury (Huang and Li, 2016; Xie et al., 2020). AS-IV administration was also shown to protect blood-brain barrier integrity in LPS-treated mice by upregulating zonula occludens-1 (ZO-1) and occludin and downregulating vascular cell adhesion molecule 1 (VCAM1) expression in brain micro-vessels; experimental results in cultured bEnd.3 and microglial cells attributed this protective effect to both activation of the Nrf2-dependent antioxidant response and inhibition of the NF-κB/NLRP3 inflammasome signaling pathway (Li H et al., 2018; Yang et al., 2019). Further research showed that AS-IV attenuated LPS-induced neuroinflammation in mice by upregulating the expression of PPARγ and inducing phosphorylation of glycogen synthase kinase-3β (GSK3-β) in the hippocampus. AS-IV-mediated neuroprotection was further evidenced by reduced expression of inflammatory factors, in association with NF-κB signaling suppression (Song et al., 2018). In a mouse model of acute E. coli peritoneal infection, AS-IV treatment alleviated peritonitis symptoms by promoting the influx of neutrophils to the infection site, an effect mediated by inhibition of G protein-coupled receptor kinase-2 (GRK2) expression and subsequent blockade of LPS-induced suppression of CXC motif chemokine receptor 2 (CXCR2) on neutrophils (Huang et al., 2016). Moreover, in LPS-treated rats, AS-IV administration attenuated cardiac dysfunction, reduced myocardial damage, improved mitochondrial energy metabolism, and inhibited cardiomyocyte apoptosis and autophagy by downregulating miRNA-1 expression (Wang et al., 2021).
Glycyrrhizin is a natural triterpene glycoside and the major active component of gan cao (licorice root). Glycyrrhizin treatment was associated with significantly improved survival in a rat model of CLP-induced sepsis. This effect was associated with suppression of HMGB1 expression and inhibition of downstream MAPK/NF-κB pathways both in vivo and in vitro (Zhao et al., 2017). In turn, in LPS-treated mice, a protective role of glycyrrhizin on heart and lung was attributed to the increase in the ratio of myeloid-derived suppressor cells (MDSCs) to CD11b+Gr1 bone marrow cells in the blood, heart, and lungs (Seo et al., 2017). In addition, glycyrrhizin was shown to negatively regulate PI3K/mTOR signaling and inhibit the expression of inflammatory markers such as iNOS, COX-2, HMGB1, TNF-α, IL-1β, and IL-6 in LPS-stimulated human liver macrophages (Shen et al., 2020).
Triptolide is a primary bioactive ingredient of the roots of the Chinese herb tripterygium wilfordii Hook. F. Triptolide exerted vascular anti-inflammatory effects by downregulating proinflammatory cytokine and chemokine secretion, attenuating VCAM-1 and intercellular adhesion molecule-1 (ICAM-1) expression, and inhibiting IκBα phosphorylation and NF-κB p65 DNA binding activity in LPS-stimulated endothelial cells (Song C et al., 2019). ZT01, a triptolide derivative, showed also anti-inflammatory activity via reducing TNF-α and IL-6 levels, blocking the formation of the TGF-β-activated kinase1 (TKA1)/TAK1-binding protein1 (TAB1) complex, and inhibiting the phosphorylation of both mitogen-activated protein kinase kinase 4 (MKK4) and JNK in both in vivo and in vitro sepsis models (Fu et al., 2020).
Artemisinin is a sesquiterpene lactone obtained from the sweet wormwood plant Artemisia annua. Although mainly recognized by its efficacy to treat malaria, there is growing evidence that artemisinin may be useful to treat several health conditions, including sepsis, by exerting potent anti-inflammatory and immunoregulatory effects. Artemisinin treatment was shown to significantly reduce LPS-induced cognitive impairment in mice by attenuating both neuronal damage and microglial activation in the hippocampus (Lin S.P et al., 2021). This study further showed that artemisinin reduced TNF-α, IL-6, IL-1α, IL-1β, and iNOS production and suppressed the migratory ability of LPS-stimulated BV2 microglial cells by activating the AMPKα1 pathway and inhibiting nuclear translocation of NF-κB (Lin S.P et al., 2021). Artesunate is a water-soluble derivative of artemisinin with multiple biological activities. In rats with LPS-induced ALI, artesunate treatment reduced TNF-α and IL-6 levels in BALF, decreased oxidative stress markers (i.e., MDA, SOD, and GSH-Px), and reduced apoptosis of lung cells by activating the mTOR/AKT/PI3K signaling pathway (Zhang E et al., 2020). Notably, artesunate was reported to reverse sepsis-induced immunosuppression in mice by interacting with the vitamin D receptor in an autophagy- and NF-κB-dependent manner (Shang et al., 2020).
Ginsenoside Rg1 (GRg1), a triterpenoid saponin, is the main bioactive component of ginseng (panax ginseng). Administration of GRg1 ameliorated LPS-induced acute myocardial injury by inhibiting the NF-κB pathway and attenuating inflammatory responses, including NLRP3 expression (Luo et al., 2020). GRg1 treatment also alleviated lung injury and extended survival in mice with LPS-induced ALI; experiments in LPS-challenged pulmonary epithelial A549 cells further showed that GRg1 exposure inhibited ROS production, prevented apoptosis, and reduced ER stress and inflammatory cytokine expression by upregulating Sirt1 (Wang Q.L. et al., 2019). In animal models of sepsis-associated ALI and encephalopathy, the protective effect of GRg1 was shown to also depend on autophagy enhancement, via a mechanism related to the activation of Nrf-2 and inhibition of NF-κB signal transduction (Li Y et al., 2017; Ji et al., 2021).
Apigenin, a flavonoid found in abundance in many fruits and vegetables, has shown remarkable efficiency in controlling the inflammatory response. In LPS-treated mice, apigenin administration decreased the levels of cardiac troponin I (cTnI), cardiac myosin light chain-1 (cMLC1), and inflammatory cytokines such as TNF-α, IL-1β, MIP-1α, and MIP-2, an effect attributed to reduced NF-κB nuclear translocation and enhanced peroxisome proliferator-activated receptor gamma (PPARγ) nuclear translocation (Li F et al., 2017; Zhou et al., 2017). In addition, apigenin ameliorated LPS-induced acute liver injury in mice via inhibiting oxidative stress, evidenced by upregulation of the activities of superoxide dismutase (SOD), catalase (CAT), glutathione-S-transferase (GST) and glutathione reductase (GR). This protective role was partly dependent on increased hepatic expression of nuclear factor erythroid 2-related factor 2 (Nrf-2) (Zhou et al., 2017). The potential of apigenin to counteract neuroinflammation was in turn suggested by its ability to downregulate the expression of CD68 (an M1 pro-inflammatory microglia marker), OX42 (a microglia activation marker), IL-6, and glycoprotein 130 in LPS-stimulated neuronal/glial co-cultures (Dourado et al., 2020).
Salidroside is extracted mainly from the root and rhizome tissues of the rose (rhodiola rosea). Many studies reported that salidroside has anti-inflammatory, anti-oxidative, and antibacterial properties. In rats with myocardial or lung injury triggered by LPS-induced endotoxemia, salidroside enhanced antioxidative activity and inhibited iNOS and COX2 expression, as well as NF-κB phosphorylation, in cardiac and lung tissues (Chen L et al., 2017; Jingyan et al., 2017; Zheng et al., 2020). Salidroside also reduced lung inflammation and alleviated ALI symptoms by upregulating Sirt1 expression and inhibiting both NF-κB activity and nucleocytoplasmic translocation of HMGB1 both in vivo and in vitro (Lan et al., 2017; Qi et al., 2017). In LPS-stimulated HUVECs, salidroside exposure increased antioxidant activity, inhibited apoptosis and NLRP3 inflammasome activation, and decreased the expression of NLRP3-related proteins, including ASC and caspase-1 (You et al., 2021).
Baicalein is a flavonoid extracted from the roots of the Chinese herb scutellaria baicalensis georgi. Its anti-inflammatory and anti-oxidative qualities have been demonstrated in several experimental settings. Baicalein-mediated protection against neuroinflammation was exemplified by its ability to downregulate LPS-induced NO generation, inhibit the expression of inflammatory cytokines such as IL-6, TNF-α, and COX2, and suppress NF-κB and p65-MAPK signaling in BV2 microglial cells and macrophages (Luo et al., 2017; Yan et al., 2020). Baicalin alleviated LPS-induced liver injury in mice by inhibiting the expression of IL-1α, IL-1β, and gasdermin D (GSDMD) and blocking NLRP3/IL-1β signaling (Xiao et al., 2021). Moreover, baicalin prevented the development of LPS-induced ALI and alleviated colitis symptoms by blocking LPS-induced TLR4/MD-2 complex formation, inhibiting the activation of MAPK and NF-κB signaling pathways, and reducing leukocyte infiltration and production of inflammatory mediators (Luo et al., 2017; Chen H et al., 2019).
Resveratrol is a non-flavonoid polyphenol with potent antioxidant properties, present in the skin of fruits such as grapes and berries. Resveratrol pretreatment protected mice against CLP-induced ALI. Research on LPS stimulated MH-S alveolar macrophages suggested that the underlying mechanism was related to inhibition of NF-κB and MAPK pathways, as well as of LPS-induced autophagy, which depended on enhanced expression of vascular endothelial growth factor B (VEGF-B) (Yang et al., 2018). In primary monocytes, resveratrol inhibited the LPS-stimulated inflammatory response by blocking phospholipase D activity and its downstream signaling molecules SphK1, ERK1/2, and NF-κB, and protected mice against septic shock induced by CLP (Wang B et al., 2020). In rats subjected to CLP, resveratrol improved vasodilation and hemodynamic parameters by upregulating endothelial nitric oxide synthase (eNOS) and downregulating iNOS, Rac family small GTPase 1 (RAC-1), and HIF-1α expression in arterial tissue (Zhang Z.S. et al., 2019). Resveratrol was also shown to ameliorate acute kidney injury (AKI) induced by sepsis by inhibiting renal inflammation triggered by endoplasmic reticulum (ER) stress activated-IRE1/NF-κB pathway activation (Wang et al., 2017). In LPS-treated mice, cardioprotective effects of resveratrol, evidenced by decreased 4-hydroxynonenal and MDA levels in myocardial tissue and improved contractility and Ca2+ homeostasis in cardiomyocytes, were attributed to increased Nrf-2 expression and to phospholamban oligomerization leading to enhanced SERCA2a activity (Bai et al., 2016).
Paeonol, a phenolic compound found in peonies such as paeonia suffruticosa (moutan cortex), shows multiple pharmacological effects, including anti-inflammatory and anti-tumoral activities. Paeonol exposure increased the expression of miR-339-5p and downregulated the expression of inflammatory markers, such as TNF-α, IL-1β, IKK-β, and HMGB1, in LPS-stimulated RAW264.7 cells (Mei et al., 2019). In a rat model of sepsis-induced AKI, paeonol treatment showed protective effects by lowering serum levels of TNF-α and IL-1β and suppressing NF-κB signaling in renal tissue (Mei et al., 2019). In the advanced stage of sepsis, impaired phagocytic activity of macrophages and monocytes contributes to immune dysfunction. Interestingly, the decline in the phagocytic ability of peritoneal macrophages induced by LPS could be reversed by paeonol co-administration, an effect mediated by suppression of HMGB1 nucleocytoplasmic translocation (Miao et al., 2020).
6-gingerol, a major polyphenol extracted from the ginger rhizome (zingiber officinale roscoe), exhibits anti-inflammatory, antioxidant, anticancer, and neuroprotective properties. Treatment with 6-gingerol suppressed systemic IL-1β release and prolonged survival in mice with CLP-induced sepsis. Complementary in vitro studies showed that 6-gingerol pre-treatment blocked MAPK-dependent NLRP3 inflammasome activation in LPS/ATP-treated BMDMs and RAW264.7 cells, which attenuated pyroptosis and therefore decreased the release of mature IL-1β into the medium (Zhang F.L. et al., 2020). In mice with CLP-induced acute liver injury, 6-gingerol administration reduced serum levels of AST, ALT, and IL-1β and elicited antioxidant and anti-apoptotic effects by upregulating hepatic Nrf-2 and HO-1 transcription (Hong et al., 2020). Similarly, renoprotective effects of 6-gingerol, consistent with its antioxidant and anti-inflammatory properties, were reported in a rat model of sepsis-induced AKI (Rodrigues et al., 2018).
Berberine is potent anti-inflammatory alkaloid compound extracted from herbs such as cortex phellodendri and rhizoma coptidis. In animal studies, berberine treatment ameliorated sepsis-related intestinal vascular barrier injury by modulating the Apoliprotein M (ApoM)/Sphingosine-1-Phosphate (S1P) axis and the Wnt/β-catenin pathway (He et al., 2018; Li Y et al., 2020). In LPS-induced acute respiratory distress syndrome (ARDS), berberine treatment prevented endothelial glycocalyx damage and hence reduced pulmonary vascular permeability by inhibiting TNF-α, IL-1β, IL-6, MMP-9, and heparanase expression, attenuating ROS production, and decreasing neutrophil infiltration in BALF (Huang et al., 2018). Further research in the ARDS model indicated that berberine’s protective mechanism resulted from inhibition of TLR4/NF-κB and JAK2/STAT3 signaling pathways (Xu et al., 2021). Cardioprotective effects of berberine, manifested by improved cardiac diastolic function and hemodynamics, were observed in rats with LPS-induced septic cardiomyopathy (Chen et al., 2021). Berberine treatment was also reported to reduce LPS-induced cognitive deficits and restore spatial learning ability in rats. These effects were correlated with increased antioxidant activity, reflected by upregulation of glutathione peroxidase (GPx), SOD, CAT, and glutathione, and decreased acetylcholinesterase (AChE), MDA, carbonyl protein, and caspase-3 activity in the hippocampus (Sadraie et al., 2019). In cultured RAW264.7 macrophages, berberine exposure inhibited LPS-induced synthesis of proinflammatory cytokines (MCP-1, IL-6, and TNF-α). This effect was mediated by reversal of LPS-induced Sirtuin1 (Sirt1) downregulation, which inhibited IκΒα degradation and IKK phosphorylation, effectively suppressing NF-κB signaling (Zhang H et al., 2017).
Cordycepin is the main active component of the fruiting bodies of the ascomycete fungus cordyceps militaris. In a mouse model of LPS-induced ALI, cordycepin administration downregulated the expression of MPO and MDA in lung tissue and reduced TNF-α and IL-1β levels in BALF. The underlying mechanism was found to be related to inhibition of NF-κB activity and stimulation of Nrf-2 and HO-1 expression (Lei et al., 2018; Qing et al., 2018). Cordycepin was also reported to decrease LPS-induced pro-inflammatory cytokine production and COX-2 expression in RAW264.7 and THP-1 cells, effects attributed to the inhibition of the NLRP3 inflammasome and the ERK1/2 signaling pathway (Yang J et al., 2017).
Emodin belongs to the Quinones class of compounds and is mainly extracted from the dry roots and rhizome of rhubarb (Hu et al., 2020). According to TCM precepts, emodin is effective in reducing accumulation, cooling blood, reducing fire, promoting blood circulation, removing blood stasis, and draining the gallbladder to relieve jaundice. Emodin was shown to possess a wide range of pharmacological properties, linked to anticancer, hepatoprotective, anti-inflammatory, antioxidant, and antimicrobial effects (Cui et al., 2020). In rats with LPS-induced ALI, emodin suppressed IKKβ, p-IKKβ, p65, and p-p65 levels, decreased NF-κB DNA binding activity, and inhibited IL-8, IL-1β, TNF-α, and myeloperoxidase (MPO) expression in lung tissues, and increased the proportion of Gr1+/CD11b+ cells in bronchoalveolar lavage fluid (BALF) (Liu B et al., 2020). It was reported that emodin further protected against ALI by downregulating the mechanistic target of rapamycin kinase (mTOR)/HIF-1α/vascular endothelial growth factor (VEGF) signaling pathway (Li X et al., 2020). Granulocytes are the first line of defense against pathogen invasion and play a crucial role in innate immunity. Emodin could upregulate the ability of granulocytes to phagocytize bacteria and generate of neutrophil extracellular trap (NETs), meanwhile, downregulated the production of ROS expression in the LPS-stimulated granulocytes, therefore alleviating lung tissue damage (Mei et al., 2020). Anti-neuroinflammatory effects of emodin were also evidenced by decreased TNF-α, IL-6, nitric oxide (NO), prostaglandin E2 (PGE2), inducible nitric oxide synthase (iNOS), and cyclooxygenase 2 (COX-2) synthesis, inhibition of NF-κB and activation of activator protein-1 (AP-1) signaling pathways, and suppressed phosphorylation of STATs and MAPKs in LPS-stimulated microglial cells (Park et al., 2016; Xie et al., 2019). In a mouse model of LPS-induced acute liver injury, emodin treatment alleviated hepatic inflammation and promoted M2 polarization of liver macrophages. Consistent with these findings, emodin exposure downregulated TLR4, MyD88, Toll/interleukin-1 receptor (TIR) domain-containing adaptor protein (TIRAP), TRAF-6, TIR-domain-containing adapter-inducing interferon-β (TRIF), interferon regulatory factor 3 (IRF-3), and AP-1 protein expression in LPS-activated RAW264.7 macrophages (Ding et al., 2018). In a mouse model of LPS-induced septic cardiomyopathy, emodin treatment inhibited expression of cardiac injury markers, i.e., LDH and creatine kinase-MB (CK-MB), and downregulated the expression of inflammatory cytokines. These protective effects were attributed to inhibition of NOD-like receptor family, pyrin domain containing 3 (NLRP3) inflammasome activation in cardiomyocytes (Dai et al., 2021).
Acupuncture is a non-pharmacological TCM method of treating diseases that acts through mechanical stimulation (usually by needling, but also via heat or pressure) on specific skin sites (acupoints) that lie along passageways through which energy flows throughout the body (meridians). In recent years, evidence that acupuncture on specific acupoints, i. e. Zusanli (ST36) and Tianshu (ST25), can regulate immunity suggested that this procedure represents a promising alternative in clinical anti-inflammatory therapy (Kim et al., 2007; Liu S et al., 2020). As a non-pharmacological therapy, and supported by preclinical studies, acupuncture has increasingly attracted the attention of clinicians (Lai et al., 2020; Liu S et al., 2020). Electroacupuncture treatment was shown to improve sepsis-related damage of brain, heart, kidney, intestines, and other organs in animal models. Its application improved survival rate in CLP model rats, and ameliorated cognitive impairment in rats with sepsis-related encephalopathy by inhibiting hippocampal synaptic damage, neuronal loss, oxidative stress, and release of inflammatory cytokines trough activation of the Nrf-2/HO-1 pathway and hippocampal α7 nicotinic acetylcholine receptors (Han et al., 2018; Li C et al., 2020). In a rat model of sepsis, electroacupuncture pretreatment at ST36 attenuated inflammatory responses, decreased plasma urea, creatinine, and D-lactate levels, and increased intestinal tight junction protein occludin expression and intestinal barrier permeability, thereby reducing acute kidney and intestinal injury (Zhu et al., 2015; Zhang Z et al., 2018; Harpin et al., 2020). In the rat CLP model, electroacupuncture treatment at ST 36 reduced the activity of plasma CK-MB and offered cardioprotection by reducing TNF-α, NO, MPO levels and water content in myocardial tissue by activating the cholinergic anti-inflammatory pathway (Zhang L et al., 2018). In clinical trials, electroacupuncture at both ST36 and Shangjuxu (ST37) significantly reduced procalcitonin (PCT), TNF-α, intestinal fatty acid-binding protein (I-FABP), D-lactate, citrulline, and the TCM quantitative score of intestinal dysfunction in patients with sepsis-related intestinal dysfunction and intestinal obstruction (Meng J.B. et al., 2018). In addition, a prospective randomized controlled trial found that electroacupuncture at both ST 36 and Guanyuan (RN 4) in patients with sepsis played an immunoprotective role by reducing the APACHE II score, increasing CD3+, CD4+, CD8+ expression and the CD4+/CD8+ ratio, and increasing HLA-DR expression in lymphocytes (Yang et al., 2016).
The above systematically summarized the research and mechanism of TCM in the treatment of sepsis (the relevant mechanisms are shown in Figure 1). Tables 1, 2 respectively summarize the relevant research of TCM compounds and monomers in the treatment of sepsis in recent years, which further prove the effectiveness of TCM.
FIGURE 1. The potential applications of TCM in sepsis. TCM can protect sepsis through TLR4/NF-κB, PI3K/mTOR, NLRP3 inflammasome, MAPK and other signaling pathways.
There are many concepts recognizing the critical state of patients who are survived from early death, such as chronic critical illness (CCI), compensatory anti-inflammatory response syndrome (CARS), and persistent inflammation, immune suppression, and catabolism syndrome (PICS), among which immune suppression are a common state and has been implicated as a predisposing factor for the secondary nosocomial infections and increased mortality, though the identification of these concepts remains to be further clarified (Torres et al., 2022). Immunotherapy such as granulocyte-macrophage colony stimulating factor (GM-CSF) and granulocyte-colony stimulating factor (G-CSF) aiming to promote restoration of normal lymphocyte numbers and function, and/or restore mature functional myeloid populations achieves great improvement in preventing secondary infection, but failed to demonstrate any significant improvements in 28-day mortality and long-term outcomes (Bo et al., 2011; Darden et al., 2021).
According to the basic theory of TCM, there are two strategies for the treatment of infectious diseases and followed critical state—Quxie and Fuzheng. The function of TCM on inhibiting inflammatory response during the early sepsis refers to the Quxie strategy that means blocking of factors leading to disease. In contrast, Fuzheng strategy agree with the therapy enhancing the anti-disease capacity of the body, which focus on the enhancement of anti-infectious immunity. Innate and adaptive immune cells are both the targets of TCM herbs. Dendritic cells are the main professional antigen-presenting cells, whose function drives the activation of macrophage and antigen-specific T-cells (Lin W et al., 2021). Accumulating evidence shows that the extracts or active monomers of TCM herbs, such as cordyceps sinensis, ganoderma lucidum, astragalus mongholicus. etc. can act as the adjuvant to promote the maturation, pro-inflammatory cytokine production and function of DCs, therefore enhancing the immune responses against tumor and infection (Li et al., 2015a). It is worth recalling that astragalus, especially the astragalus polysaccharide may be the representative of TCM herbs for immunity enhancement, whose function varies from humoral to cellular immune responses (Sultan et al., 2014; Deng et al., 2022). Though there is a lack of direct evidence, studies about the immune deficiency diseases and cancer suggested the potential function of immune enhancement herbs on sepsis related immune suppression. Except for the direct inhibition of tumor, immunity-enhancing capacity plays a vital role in the anticancer activity of TCM herbs (Wang S et al., 2020; Wang Y et al., 2020) For example, shenqi fuzheng injection, mainly consist of ginsen and astragalus, could promote NK and T helper cells proliferation and function, therefore enhancing the effects of chemotherapy drugs and decreasing adverse events in cancers (Dong et al., 2010; Li et al., 2015b; Yang Y et al., 2017). Results of randomized clinical trials and real-world data reveals that integrating Fuzheng TCM herbs and anti-retroviral therapy promotes long-term reconstitution of the immune system and significantly improved the survival periods of AIDS patients (Zou et al., 2016; Tao et al., 2021; Jin et al., 2022).
Sepsis is a common severe complication of patients with infection, severe trauma, shock, burns, etc. With the development of public health policy, infection-prevention efforts reduce sepsis incidence. Diversified treatment and multiple organ support therapy contribute to the reduced mortality in past years. However, sepsis remains a major cause of health loss worldwide with high health-related burden, especially in Asia and Africa (Rudd et al., 2020). The problems facing the effective antibiotics-based combination therapies are the ever-growing antibiotic resistance (Reynolds et al., 2022) and the potential risk of drug-induced liver injury, enteric dysbacteriosis, and fungal infection (Kwon et al., 2022), which may further aggravate organ dysfunction (Meng et al., 2017). Therefore, more treatment strategy for sepsis is needed.
TCM established a complete system of diagnosis and treatment for pandemic and endemic diseases, possessing a well-documented history of treating infectious diseases and clinical practice, such as Treatise on Febrile and Miscellaneous Disease (“Shanghan Zabing Lun” in Chinese) and Detailed Analysis of Epidemic Warm Diseases (“Wenbing Tiaobian” in Chinese). Given the complexity of the chemical components of traditional Chinese herbs and the ambiguity of the effective components, research has been devoted to the separation of the active components of prescriptions and single traditional Chinese herb for cell or animal study. This review summarizes the function and mechanism of TCM compounds, active monomers (terpenoids, flavonoids, polyphenols, alkaloids), and acupuncture on sepsis treatment, which varies from anti-inflammation, anti-oxidation, anti-mitochondrial dysfunction, regulating apoptosis and autophagy etc. Because of the lack of clinical evidence, an important issue that is not covered in this review is whether the potential side effects of TCM on related organs would aggravate the multi-organ injury in patients with sepsis. Indeed, a study reveals that TCM herbs and dietary supplements were the leading causes of drug-induced liver injury (DILI) in mainland China (Shen et al., 2019), which attracts much attention. However, researchers pointed that implicated drug categories adopted in this study might seriously influence the reliability of conclusions (Cong et al., 2019). A latest study screened 94,593 DILI reports from 308 medical centers across the China mainland between 2012 and 2016, and found that TCM herbs only accounted for 4.5% of the DILI reports (Wang et al., 2022). The main challenge facing the application of TCM for the treatment of sepsis in drug safety aspect is how to identify the scattered categories of drug that are potentially harmful to the organ function, and the assessment of risk/benefit ratio.
In recent years, conventional molecular biological studies, network pharmacology prediction, molecular docking analysis, and visualization analysis reveal the widely potential targets of TCM compounds and active monomers in infectious diseases (Huang et al., 2021; Zhou et al., 2021; Li et al., 2022), and in this state lack of standardized and large-scale clinical studies counts in limiting clinical translation value. In addition, targeting the activation of the immune system and related imbalance of pro-inflammation and anti-inflammation is the main mechanism that studies of sepsis focus on, while the protection of organs and prevention of sequelae such as sepsis-associated encephalopathy, ICU-acquired weakness, sepsis-induced cardiomyopathy, etc., are partly neglected. Multidisciplinary research is needed to explain the scientific connotation of compound compatibility of anti-sepsis TCM recipes.
YS wrote the manuscript draft. WL revised the manuscript. WZ conceived the review structure.
This work was supported by Wuhan science and technology bureau, Wuhan Applied Basic Research Project (No. 2017060201010177) and the program of Tongji-Rongcheng Center for Biomedicine (HUST).
We are grateful the support from Tongji Hospital, Tongji Medical College, Huazhong University of Science and Technology. The figure was created using BioRender.com.
The authors declare that the research was conducted in the absence of any commercial or financial relationships that could be construed as a potential conflict of interest.
All claims expressed in this article are solely those of the authors and do not necessarily represent those of their affiliated organizations, or those of the publisher, the editors and the reviewers. Any product that may be evaluated in this article, or claim that may be made by its manufacturer, is not guaranteed or endorsed by the publisher.
Bai, T., Hu, X., Zheng, Y., Wang, S., Kong, J., and Cai, L. (2016). Resveratrol protects against lipopolysaccharide-induced cardiac dysfunction by enhancing SERCA2a activity through promoting the phospholamban oligomerization. Am. J. physiology Heart circulatory physiology 311, H1051–H1062. doi:10.1152/ajpheart.00296.2016
Bo, L., Wang, F., Zhu, J., Li, J., and Deng, X. (2011). Granulocyte-colony stimulating factor (G-CSF) and granulocyte-macrophage colony stimulating factor (GM-CSF) for sepsis: A meta-analysis. Crit. care (London, Engl. 15, R58. doi:10.1186/cc10031
Boyd, J. H., Russell, J. A., and Fjell, C. D. (2014). The meta-genome of sepsis: Host genetics, pathogens and the acute immune response. J. innate Immun. 6, 272–283. doi:10.1159/000358835
Cao, Y., Han, X., Pan, H., Jiang, Y., Peng, X., Xiao, W., et al. (2020). Emerging protective roles of shengmai injection in septic cardiomyopathy in mice by inducing myocardial mitochondrial autophagy via caspase-3/Beclin-1 axis. Inflamm. Res. official J. Eur. Histamine Res. Soc. 69, 41–50. doi:10.1007/s00011-019-01292-2
Cecconi, M., Evans, L., Levy, M., and Rhodes, A. (2018). Sepsis and septic shock. Lancet London, Engl. 392, 75–87. doi:10.1016/S0140-6736(18)30696-2
Chen, G., Xu, Y., Jing, J., Mackie, B., Zheng, X., Zhang, X., et al. (2017). The anti-sepsis activity of the components of Huanglian Jiedu Decoction with high lipid A-binding affinity. Int. Immunopharmacol. 46, 87–96. doi:10.1016/j.intimp.2017.02.025
Chen, H., Liu, Q., Liu, X., and Jin, J. (2021). Berberine attenuates septic cardiomyopathy by inhibiting TLR4/NF-κB signalling in rats. Pharm. Biol. 59, 121–128. doi:10.1080/13880209.2021.1877736
Chen, H., Zhang, Y., Zhang, W., Liu, H., Sun, C., Zhang, B., et al. (2019). Inhibition of myeloid differentiation factor 2 by baicalein protects against acute lung injury. Phytomedicine Int. J. phytotherapy Phytopharm. 63, 152997. doi:10.1016/j.phymed.2019.152997
Chen, L., Liu, P., Feng, X., and Ma, C. (2017). Salidroside suppressing LPS-induced myocardial injury by inhibiting ROS-mediated PI3K/Akt/mTOR pathway in vitro and in vivo. J. Cell. Mol. Med. 21, 3178–3189. doi:10.1111/jcmm.12871
Chen, Q., Liu, J., Wang, W., Liu, S., Yang, X., Chen, M., et al. (2019). Sini decoction ameliorates sepsis-induced acute lung injury via regulating ACE2-Ang (1-7)-Mas axis and inhibiting the MAPK signaling pathway. Biomed. Pharmacother. = Biomedecine Pharmacother. 115, 108971. doi:10.1016/j.biopha.2019.108971
Chen, R. J., Rui, Q. L., Wang, Q., Tian, F., Wu, J., and Kong, X. Q. (2020). Shenfu injection attenuates lipopolysaccharide-induced myocardial inflammation and apoptosis in rats. Chin. J. Nat. Med. 18, 226–233. doi:10.1016/S1875-5364(20)30025-X
Chen, X., Feng, Y., Shen, X., Pan, G., Fan, G., Gao, X., et al. (2018). Anti-sepsis protection of Xuebijing injection is mediated by differential regulation of pro- and anti-inflammatory Th17 and T regulatory cells in a murine model of polymicrobial sepsis. J. Ethnopharmacol. 211, 358–365. doi:10.1016/j.jep.2017.10.001
Cong, W., Xin, Q., and Gao, Y. (2019). RE: Incidence and etiology of drug-induced liver injury in mainland China. Gastroenterology 157, 1438–1439. doi:10.1053/j.gastro.2019.05.076
Cui, Y., Chen, L. J., Huang, T., Ying, J. Q., and Li, J. (2020). The pharmacology, toxicology and therapeutic potential of anthraquinone derivative emodin. Chin. J. Nat. Med. 18, 425–435. doi:10.1016/S1875-5364(20)30050-9
Dai, S., Ye, B., Chen, L., Hong, G., Zhao, G., and Lu, Z. (2021). Emodin alleviates LPS-induced myocardial injury through inhibition of NLRP3 inflammasome activation. Phytotherapy Res. 35 (9), 5203–5213. doi:10.1002/ptr.7191
Darden, D. B., Kelly, L. S., Fenner, B. P., Moldawer, L. L., Mohr, A. M., Efron, P. A., et al. (2021). Chronic critical illness elicits a unique circulating leukocyte transcriptome in sepsis survivors. J. Clin. Med. 10, 3211. doi:10.3390/jcm10153211
Delano, M. J., and Ward, P. A. (2016). The immune system's role in sepsis progression, resolution, and long-term outcome. Immunol. Rev. 274, 330–353. doi:10.1111/imr.12499
Deng, G., Zhou, L., Wang, B., Sun, X., Zhang, Q., Chen, H., et al. (2022). Targeting cathepsin B by cycloastragenol enhances antitumor immunity of CD8 T cells via inhibiting MHC-I degradation. J. Immunother. cancer 10, e004874. doi:10.1136/jitc-2022-004874
Ding, Y., Liu, P., Chen, Z. L., Zhang, S. J., Wang, Y. Q., Cai, X., et al. (2018). Emodin attenuates lipopolysaccharide-induced acute liver injury via inhibiting the TLR4 signaling pathway in vitro and in vivo. Front. Pharmacol. 9, 962. doi:10.3389/fphar.2018.00962
Dong, J., Su, S. Y., Wang, M. Y., and Zhan, Z. (2010). Shenqi fuzheng, an injection concocted from Chinese medicinal herbs, combined with platinum-based chemotherapy for advanced non-small cell lung cancer: A systematic review. J. Exp. Clin. cancer Res. CR 29, 137. doi:10.1186/1756-9966-29-137
Dourado, N. S., Souza, C. D. S., de Almeida, M. M. A., Bispo da Silva, A., Dos Santos, B. L., Silva, V. D. A., et al. (2020). Neuroimmunomodulatory and neuroprotective effects of the flavonoid apigenin in in vitro models of neuroinflammation associated with alzheimer's disease. Front. aging Neurosci. 12, 119. doi:10.3389/fnagi.2020.00119
Fisher, C. J., Agosti, J. M., Opal, S. M., Lowry, S. F., Balk, R. A., Sadoff, J. C., et al. (1996). Treatment of septic shock with the tumor necrosis factor receptor:Fc fusion protein. The Soluble TNF Receptor Sepsis Study Group. N. Engl. J. Med. 334, 1697–1702. doi:10.1056/NEJM199606273342603
Fleischmann, C., Scherag, A., Adhikari, N. K., Hartog, C. S., Tsaganos, T., Schlattmann, P., et al. (2016). Assessment of global incidence and mortality of hospital-treated sepsis. Current estimates and limitations. Am. J. Respir. Crit. care Med. 193, 259–272. doi:10.1164/rccm.201504-0781OC
Fu, J., Zang, Y., Zhou, Y., Chen, C., Shao, S., Hu, M., et al. (2020). A novel triptolide derivative ZT01 exerts anti-inflammatory effects by targeting TAK1 to prevent macrophage polarization into pro-inflammatory phenotype. Biomed. Pharmacother. = Biomedecine Pharmacother. 126, 110084. doi:10.1016/j.biopha.2020.110084
Gao, H., Liu, X., Sun, W., Kang, N., Liu, Y., Yang, S., et al. (2017). Total tanshinones exhibits anti-inflammatory effects through blocking TLR4 dimerization via the MyD88 pathway. Cell death Dis. 8, e3004. doi:10.1038/cddis.2017.389
Gao, S., Li, L., Li, L., Ni, J., Guo, R., Mao, J., et al. (2019). Effects of the combination of tanshinone IIA and puerarin on cardiac function and inflammatory response in myocardial ischemia mice. J. Mol. Cell. Cardiol. 137, 59–70. doi:10.1016/j.yjmcc.2019.09.012
Han, Y. G., Qin, X., Zhang, T., Lei, M., Sun, F. Y., Sun, J. J., et al. (2018). Electroacupuncture prevents cognitive impairment induced by lipopolysaccharide via inhibition of oxidative stress and neuroinflammation. Neurosci. Lett. 683, 190–195. doi:10.1016/j.neulet.2018.06.003
Harpin, D., Simadibrata, C. L., Mihardja, H., and Barasila, A. C. (2020). Effect of electroacupuncture on urea and creatinine levels in the wistar sepsis model. Med. Acupunct. 32, 29–37. doi:10.1089/acu.2019.1369
He, Y., Yuan, X., Zuo, H., Sun, Y., and Feng, A. (2018). Berberine exerts a protective effect on gut-vascular barrier via the modulation of the wnt/beta-catenin signaling pathway during sepsis. Cell. physiology Biochem. Int. J. Exp. Cell. physiology, Biochem. Pharmacol. 49, 1342–1351. doi:10.1159/000493412
Hong, M. K., Hu, L. L., Zhang, Y. X., Xu, Y. L., Liu, X. Y., He, P. K., et al. (2020). 6-Gingerol ameliorates sepsis-induced liver injury through the Nrf2 pathway. Int. Immunopharmacol. 80, 106196. doi:10.1016/j.intimp.2020.106196
Hu, N., Liu, J., Xue, X., and Li, Y. (2020). The effect of emodin on liver disease -- comprehensive advances in molecular mechanisms. Eur. J. Pharmacol. 882, 173269. doi:10.1016/j.ejphar.2020.173269
Hu, X., Liu, S., Zhu, J., and Ni, H. (2019). Dachengqi decoction alleviates acute lung injury and inhibits inflammatory cytokines production through TLR4/NF-κB signaling pathway in vivo and in vitro. J. Cell. Biochem. 120, 8956–8964. doi:10.1002/jcb.27615
Huang, K., Zhang, P., Zhang, Z., Youn, J. Y., Wang, C., Zhang, H., et al. (2021). Traditional Chinese Medicine (TCM) in the treatment of COVID-19 and other viral infections: Efficacies and mechanisms. Pharmacol. Ther. 225, 107843. doi:10.1016/j.pharmthera.2021.107843
Huang, L., Zhang, X., Ma, X., Zhang, D., Li, D., Feng, J., et al. (2018). Berberine alleviates endothelial glycocalyx degradation and promotes glycocalyx restoration in LPS-induced ARDS. Int. Immunopharmacol. 65, 96–107. doi:10.1016/j.intimp.2018.10.001
Huang, M., Cai, S., and Su, J. (2019). The pathogenesis of sepsis and potential therapeutic targets. Int. J. Mol. Sci. 20, 5376. doi:10.3390/ijms20215376
Huang, P., Lu, X., Yuan, B., Liu, T., Dai, L., Liu, Y., et al. (2016). Astragaloside IV alleviates E. coli-caused peritonitis via upregulation of neutrophil influx to the site of infection. Int. Immunopharmacol. 39, 377–382. doi:10.1016/j.intimp.2016.08.011
Huang, R., and Li, M. (2016). Protective effect of Astragaloside IV against sepsis-induced acute lung injury in rats. Saudi Pharm. J. 24, 341–347. doi:10.1016/j.jsps.2016.04.014
Ji, Q., Sun, Z., Yang, Z., Zhang, W., Ren, Y., Chen, W., et al. (2021). Protective effect of ginsenoside Rg1 on LPS-induced apoptosis of lung epithelial cells. Mol. Immunol. 136, 168–174. doi:10.1016/j.molimm.2018.11.003
Jin, H., Peng, X., He, Y., Ruganzu, J. B., and Yang, W. (2020). Tanshinone IIA suppresses lipopolysaccharide-induced neuroinflammatory responses through NF-κB/MAPKs signaling pathways in human U87 astrocytoma cells. Brain Res. Bull. 164, 136–145. doi:10.1016/j.brainresbull.2020.08.019
Jin, Y., Zhang, M., Ma, Y., Sang, F., Li, P., Yang, C., et al. (2022). Effects of Chinese medicine on the survival of AIDS patients administered second-line art in rural areas of China: A retrospective cohort study based on real-world data. Evidence-based complementary Altern. Med. eCAM 2022, 5103768. doi:10.1155/2022/5103768
Jingyan, L., Yujuan, G., Yiming, Y., Lingpeng, Z., Tianhua, Y., and Mingxing, M. (2017). Salidroside attenuates LPS-induced acute lung injury in rats. Inflammation 40, 1520–1531. doi:10.1007/s10753-017-0593-6
Kang, X., Jin, D., Jiang, L., Zhang, Y., Zhang, Y., An, X., et al. (2022). Efficacy and mechanisms of traditional Chinese medicine for COVID-19: A systematic review. Chin. Med. 17, 30. doi:10.1186/s13020-022-00587-7
Kim, H. W., Kang, S. Y., Yoon, S. Y., Roh, D. H., Kwon, Y. B., Han, H. J., et al. (2007). Low-frequency electroacupuncture suppresses zymosan-induced peripheral inflammation via activation of sympathetic post-ganglionic neurons. Brain Res. 1148, 69–75. doi:10.1016/j.brainres.2007.02.030
Kwon, J., Kong, Y., Wade, M., Williams, D. J., Creech, C. B., Evans, S., et al. (2022). Gastrointestinal microbiome disruption and antibiotic-associated diarrhea in children receiving antibiotic therapy for community-acquired pneumonia. J. Infect. Dis. 226, 1109–1119. doi:10.1093/infdis/jiac082
Lai, F., Ren, Y., Lai, C., Chen, R., Yin, X., Tan, C., et al. (2020). Acupuncture at zusanli (ST36) for experimental sepsis: A systematic review. Evidence-based complementary Altern. Med. eCAM 2020, 3620741. doi:10.1155/2020/3620741
Lan, K. C., Chao, S. C., Wu, H. Y., Chiang, C. L., Wang, C. C., Liu, S. H., et al. (2017). Salidroside ameliorates sepsis-induced acute lung injury and mortality via downregulating NF-κB and HMGB1 pathways through the upregulation of SIRT1. Sci. Rep. 7, 12026. doi:10.1038/s41598-017-12285-8
Lei, J., Wei, Y., Song, P., Li, Y., Zhang, T., Feng, Q., et al. (2018). Cordycepin inhibits LPS-induced acute lung injury by inhibiting inflammation and oxidative stress. Eur. J. Pharmacol. 818, 110–114. doi:10.1016/j.ejphar.2017.10.029
Li, C., Wang, P., Li, M., Zheng, R., Chen, S., Liu, S., et al. (2021). The current evidence for the treatment of sepsis with Xuebijing injection: Bioactive constituents, findings of clinical studies and potential mechanisms. J. Ethnopharmacol. 265, 113301. doi:10.1016/j.jep.2020.113301
Li, C., Wang, P., Zhang, L., Li, M., Lei, X., Liu, S., et al. (2018). Efficacy and safety of xuebijing injection (a Chinese patent) for sepsis: A meta-analysis of randomized controlled trials. J. Ethnopharmacol. 224, 512–521. doi:10.1016/j.jep.2018.05.043
Li, C., Yu, T. Y., Zhang, Y., Wei, L. P., Dong, S. A., Shi, J., et al. (2020). Electroacupuncture improves cognition in rats with sepsis-associated encephalopathy. J. Surg. Res. 256, 258–266. doi:10.1016/j.jss.2020.06.056
Li, F., Lang, F., Zhang, H., Xu, L., Wang, Y., Zhai, C., et al. (2017). Apigenin alleviates endotoxin-induced myocardial toxicity by modulating inflammation, oxidative stress, and autophagy. Oxidative Med. Cell. Longev. 2017, 2302896. doi:10.1155/2017/2302896
Li, H., Wang, P., Huang, F., Jin, J., Wu, H., Zhang, B., et al. (2018). Astragaloside IV protects blood-brain barrier integrity from LPS-induced disruption via activating Nrf2 antioxidant signaling pathway in mice. Toxicol. Appl. Pharmacol. 340, 58–66. doi:10.1016/j.taap.2017.12.019
Li, J., Li, J., and Zhang, F. (2015a). The immunoregulatory effects of Chinese herbal medicine on the maturation and function of dendritic cells. J. Ethnopharmacol. 171, 184–195. doi:10.1016/j.jep.2015.05.050
Li, J., Wang, J. C., Ma, B., Gao, W., Chen, P., Sun, R., et al. (2015b). Shenqi fuzheng injection for advanced gastric cancer: A systematic review of randomized controlled trials. Chin. J. Integr. Med. 21, 71–79. doi:10.1007/s11655-014-1768-8
Li, J., Zheng, Y., Li, M. X., Yang, C. W., and Liu, Y. F. (2018). Tanshinone IIA alleviates lipopolysaccharide-induced acute lung injury by downregulating TRPM7 and pro-inflammatory factors. J. Cell. Mol. Med. 22, 646–654. doi:10.1111/jcmm.13350
Li, T., Qian, Y., Miao, Z., Zheng, P., Shi, T., Jiang, X., et al. (2020). Xuebijing injection alleviates Pam3CSK4-induced inflammatory response and protects mice from sepsis caused by methicillin-resistant Staphylococcus aureus. Front. Pharmacol. 11, 104. doi:10.3389/fphar.2020.00104
Li, X., Shan, C., Wu, Z., Yu, H., Yang, A., and Tan, B. (2020). Emodin alleviated pulmonary inflammation in rats with LPS-induced acute lung injury through inhibiting the mTOR/HIF-1α/VEGF signaling pathway. Inflamm. Res. official J. Eur. Histamine Res. Soc. [et al] 69, 365–373. doi:10.1007/s00011-020-01331-3
Li, X., Wei, S., Niu, S., Ma, X., Li, H., Jing, M., et al. (2022). Network pharmacology prediction and molecular docking-based strategy to explore the potential mechanism of Huanglian Jiedu Decoction against sepsis. Computers in biology and medicine 144, 105389. doi:10.1016/j.compbiomed.2022.105389
Li, Y., Ruan, X., Xu, X., Li, C., Qiang, T., Zhou, H., et al. (2019). Shengmai injection suppresses angiotensin II-induced cardiomyocyte hypertrophy and apoptosis via activation of the AMPK signaling pathway through energy-dependent mechanisms. Frontiers in pharmacology 10, 1095. doi:10.3389/fphar.2019.01095
Li, Y., Wang, F., and Luo, Y. (2017). Ginsenoside Rg1 protects against sepsis-associated encephalopathy through beclin 1-independent autophagy in mice. The Journal of surgical research 207, 181–189. doi:10.1016/j.jss.2016.08.080
Li, Y., Zhou, J., Qiu, J., Huang, Z., Wang, W., Wu, P., et al. (2020). Berberine reduces gut-vascular barrier permeability via modulation of ApoM/S1P pathway in a model of polymicrobial sepsis. Life sciences 261, 118460. doi:10.1016/j.lfs.2020.118460
Lin, S. P., Wei, J. X., Hu, J. S., Bu, J. Y., Zhu, L. D., Li, Q., et al. (2021). Artemisinin improves neurocognitive deficits associated with sepsis by activating the AMPK axis in microglia. Acta pharmacologica Sinica 42, 1069–1079. doi:10.1038/s41401-021-00634-3
Lin, W., Shen, P., Song, Y., Huang, Y., and Tu, S. (2021). Reactive oxygen species in autoimmune cells: Function, differentiation, and metabolism. Frontiers in immunology 12, 635021. doi:10.3389/fimmu.2021.635021
Liu, B., Cheng, Y., Wu, Y., Zheng, X., Li, X., Yang, G., et al. (2020). Emodin improves alveolar hypercoagulation and inhibits pulmonary inflammation in LPS-provoked ARDS in mice via NF-κB inactivation. International immunopharmacology 88, 107020. doi:10.1016/j.intimp.2020.107020
Liu, J., Wang, Z., Lin, J., Li, T., Guo, X., Pang, R., et al. (2021). Xuebijing injection in septic rats mitigates kidney injury, reduces cortical microcirculatory disorders, and suppresses activation of local inflammation. Journal of ethnopharmacology 276, 114199. doi:10.1016/j.jep.2021.114199
Liu, Q. Y., Zhuang, Y., Song, X. R., Niu, Q., Sun, Q. S., Li, X. N., et al. (2021). Tanshinone IIA prevents LPS-induced inflammatory responses in mice via inactivation of succinate dehydrogenase in macrophages. Acta pharmacologica Sinica 42, 987–997. doi:10.1038/s41401-020-00535-x
Liu, S., Wang, Z. F., Su, Y. S., Ray, R. S., Jing, X. H., Wang, Y. Q., et al. (2020). Somatotopic organization and intensity dependence in driving distinct NPY-expressing sympathetic pathways by electroacupuncture. Neuron 108, 436–450. e437. doi:10.1016/j.neuron.2020.07.015
Liu, X., Liu, R., Dai, Z., Wu, H., Lin, M., Tian, F., et al. (2019). Effect of Shenfu injection on lipopolysaccharide (LPS)-induced septic shock in rabbits. Journal of ethnopharmacology 234, 36–43. doi:10.1016/j.jep.2019.01.008
Liu, Y., Li, Z., Xue, X., Wang, Y., Zhang, Y., and Wang, J. (2018). Apigenin reverses lung injury and immunotoxicity in paraquat-treated mice. International immunopharmacology 65, 531–538. doi:10.1016/j.intimp.2018.10.046
Lu, J., Yan, J., Yan, J., Zhang, L., Chen, M., Chen, Q., et al. (2020a). Network pharmacology based research into the effect and mechanism of Xijiao Dihuang decoction against sepsis. Biomedicine & pharmacotherapy = Biomedecine & pharmacotherapie 122, 109777. doi:10.1016/j.biopha.2019.109777
Lu, J., Zhang, L., Cheng, L., He, S., Zhang, Y., Yan, J., et al. (2020b). Xijiao Dihuang decoction improves prognosis of sepsis via inhibition of aerobic glycolysis. Biomedicine & pharmacotherapy = Biomedecine & pharmacotherapie 129, 110501. doi:10.1016/j.biopha.2020.110501
Lu, Z. B., Ou, J. Y., Cao, H. H., Liu, J. S., and Yu, L. Z. (2020). Heat-clearing Chinese medicines in lipopolysaccharide-induced inflammation. Chinese journal of integrative medicine 26, 552–559. doi:10.1007/s11655-020-3256-7
Lu, Z., Xiong, W., Xiao, S., Lin, Y., Yu, K., Yue, G., et al. (2020). Huanglian Jiedu Decoction ameliorates DSS-induced colitis in mice via the JAK2/STAT3 signalling pathway. Chinese medicine 15, 45. doi:10.1186/s13020-020-00327-9
Luo, M., Yan, D., Sun, Q., Tao, J., Xu, L., Sun, H., et al. (2020). Ginsenoside Rg1 attenuates cardiomyocyte apoptosis and inflammation via the TLR4/NF-kB/NLRP3 pathway. Journal of cellular biochemistry 121, 2994–3004. doi:10.1002/jcb.29556
Luo, X., Yu, Z., Deng, C., Zhang, J., Ren, G., Sun, A., et al. (2017). Baicalein ameliorates TNBS-induced colitis by suppressing TLR4/MyD88 signaling cascade and NLRP3 inflammasome activation in mice. Scientific reports 7, 16374. doi:10.1038/s41598-017-12562-6
Mei, H., Tao, Y., Zhang, T., and Qi, F. (2020). Emodin alleviates LPS-induced inflammatory response in lung injury rat by affecting the function of granulocytes. Journal of inflammation (London, England) 17, 26. doi:10.1186/s12950-020-00252-6
Mei, L., He, M., Zhang, C., Miao, J., Wen, Q., Liu, X., et al. (2019). Paeonol attenuates inflammation by targeting HMGB1 through upregulating miR-339-5p. Scientific reports 9, 19370. doi:10.1038/s41598-019-55980-4
Meng, F., Lai, H., Luo, Z., Liu, Y., Huang, X., Chen, J., et al. (2018). Effect of xuefu zhuyu decoction pretreatment on myocardium in sepsis rats. eCAM 2018, 2939307. doi:10.1155/2018/2939307
Meng, J. B., Jiao, Y. N., Zhang, G., Xu, X. J., Ji, C. L., Hu, M. H., et al. (2018). Electroacupuncture improves intestinal dysfunction in septic patients: A randomised controlled trial. BioMed research international 2018, 8293594. doi:10.1155/2018/8293594
Meng, M., Klingensmith, N. J., and Coopersmith, C. M. (2017). New insights into the gut as the driver of critical illness and organ failure. Current opinion in critical care 23, 143–148. doi:10.1097/MCC.0000000000000386
Miao, J., Ye, P., Lan, J., Ye, S., Zhong, J., Gresham, A., et al. (2020). Paeonol promotes the phagocytic ability of macrophages through confining HMGB1 to the nucleus. International immunopharmacology 89, 107068. doi:10.1016/j.intimp.2020.107068
Mo, Y., Liu, X., Qin, X., Huang, J., He, Z., Lin, J., et al. (2014). Evidence-based complementary and alternative medicine, 2014, 279853.Shenfu injection for intradialytic hypotension a systematic review and meta-analysiseCAM
Nabavi, S. F., Khan, H., D'Onofrio, G., Šamec, D., Shirooie, S., Dehpour, A. R., et al. (2018). Apigenin as neuroprotective agent: Of mice and men. Pharmacological research 128, 359–365. doi:10.1016/j.phrs.2017.10.008
Opal, S. M., Laterre, P. F., Francois, B., LaRosa, S. P., Angus, D. C., Mira, J. P., et al. (2013). Effect of eritoran, an antagonist of MD2-TLR4, on mortality in patients with severe sepsis: The ACCESS randomized trial. Jama 309, 1154–1162. doi:10.1001/jama.2013.2194
Pan, L. Y., Chen, Y. F., Li, H. C., Bi, L. M., Sun, W. J., Sun, G. F., et al. (2017). Dachengqi decoction attenuates intestinal vascular endothelial injury in severe acute pancreatitis in vitro and in vivo. Cellular physiology and biochemistry international journal of experimental cellular physiology, biochemistry, and pharmacology 44, 2395–2406. doi:10.1159/000486155
Pang, Q., Zhao, Y., Chen, X., Zhao, K., Zhai, Q., and Tu, F. (2018). Apigenin protects the brain against ischemia/reperfusion injury via caveolin-1/VEGF in vitro and in vivo. Oxidative medicine and cellular longevity 2018, 7017204. doi:10.1155/2018/7017204
Park, S. Y., Jin, M. L., Ko, M. J., Park, G., and Choi, Y. W. (2016). Anti-neuroinflammatory effect of emodin in LPS-stimulated microglia: Involvement of AMPK/Nrf2 activation. Neurochemical research 41, 2981–2992. doi:10.1007/s11064-016-2018-6
Prescott, H. C., and Angus, D. C. (2018). Enhancing recovery from sepsis: A review. Jama 319, 62–75. doi:10.1001/jama.2017.17687
Qi, Z., Zhang, Y., Qi, S., Ling, L., Gui, L., Yan, L., et al. (2017). Salidroside inhibits HMGB1 acetylation and release through upregulation of SirT1 during inflammation. Oxidative medicine and cellular longevity 2017, 9821543. doi:10.1155/2017/9821543
Qing, R., Huang, Z., Tang, Y., Xiang, Q., and Yang, F. (2018). Cordycepin alleviates lipopolysaccharide-induced acute lung injury via Nrf2/HO-1 pathway. International immunopharmacology 60, 18–25. doi:10.1016/j.intimp.2018.04.032
Reynolds, D., Burnham, J. P., Vazquez Guillamet, C., McCabe, M., Yuenger, V., Betthauser, K., et al. (2022). The threat of multidrug-resistant/extensively drug-resistant gram-negative respiratory infections: Another pandemic. European respiratory review an official journal of the European Respiratory Society 31, 220068. doi:10.1183/16000617.0068-2022
Rodrigues, F. A. P., Santos, A., de Medeiros, P., Prata, M. M. G., Santos, T. C. S., da Silva, J. A., et al. (2018). Gingerol suppresses sepsis-induced acute kidney injury by modulating methylsulfonylmethane and dimethylamine production. Scientific reports 8, 12154. doi:10.1038/s41598-018-30522-6
Rudd, K. E., Johnson, S. C., Agesa, K. M., Shackelford, K. A., Tsoi, D., Kievlan, D. R., et al. (2020). Global, regional, and national sepsis incidence and mortality, 1990-2017: Analysis for the global burden of disease study. Lancet (London, England) 395, 200–211. doi:10.1016/S0140-6736(19)32989-7
Sadraie, S., Kiasalari, Z., Razavian, M., Azimi, S., Sedighnejad, L., Afshin-Majd, S., et al. (2019). Berberine ameliorates lipopolysaccharide-induced learning and memory deficit in the rat: Insights into underlying molecular mechanisms. Metabolic Brain disease 34, 245–255. doi:10.1007/s11011-018-0349-5
Seo, E. H., Song, G. Y., Kwak, B. O., Oh, C. S., Lee, S. H., and Kim, S. H. (2017). Effects of glycyrrhizin on the differentiation of myeloid cells of the heart and lungs in lipopolysaccharide-induced septic mice. Shock (Augusta, Ga) 48, 371–376. doi:10.1097/SHK.0000000000000850
Shang, S., Wu, J., Li, X., Liu, X., Li, P., Zheng, C., et al. (2020). Artesunate interacts with the vitamin D receptor to reverse sepsis-induced immunosuppression in a mouse model via enhancing autophagy. British journal of pharmacology 177, 4147–4165. doi:10.1111/bph.15158
Shen, C. H., Ma, Z. Y., Li, J. H., Li, R. D., Tao, Y. F., Zhang, Q. B., et al. (2020). Glycyrrhizin improves inflammation and apoptosis via suppressing HMGB1 and PI3K/mTOR pathway in lipopolysaccharide-induced acute liver injury. European review for medical and pharmacological sciences 24, 7122–7130. doi:10.26355/eurrev_202006_21706
Shen, T., Liu, Y., Shang, J., Xie, Q., Li, J., Yan, M., et al. (2019). Incidence and etiology of drug-induced liver injury in mainland China. Gastroenterology 156, 2230–2241. e2211. doi:10.1053/j.gastro.2019.02.002
Singer, M., Deutschman, C. S., Seymour, C. W., Shankar-Hari, M., Annane, D., Bauer, M., et al. (2016). The third international consensus definitions for sepsis and septic shock (Sepsis-3). Jama 315, 801–810. doi:10.1001/jama.2016.0287
Song, C., Wang, Y., Cui, L., Yan, F., and Shen, S. (2019). Triptolide attenuates lipopolysaccharide-induced inflammatory responses in human endothelial cells: Involvement of NF-κB pathway. BMC complementary and alternative medicine 19, 198. doi:10.1186/s12906-019-2616-3
Song, M. T., Ruan, J., Zhang, R. Y., Deng, J., Ma, Z. Q., and Ma, S. P. (2018). Astragaloside IV ameliorates neuroinflammation-induced depressive-like behaviors in mice via the PPARγ/NF-κB/NLRP3 inflammasome axis. Acta pharmacologica Sinica 39, 1559–1570. doi:10.1038/aps.2017.208
Song, Y., Yao, C., Yao, Y., Han, H., Zhao, X., Yu, K., et al. (2019). XueBiJing injection versus placebo for critically ill patients with severe community-acquired pneumonia: A randomized controlled trial. Critical care medicine 47, e735–e743. doi:10.1097/CCM.0000000000003842
Su, Z., Yu, P., Sheng, L., Ye, J., and Qin, Z. (2018). Fangjifuling ameliorates lipopolysaccharide-induced renal injury via inhibition of inflammatory and apoptotic response in mice. Cellular physiology and biochemistry international journal of experimental cellular physiology, biochemistry, and pharmacology 49, 2124–2137. doi:10.1159/000493816
Sultan, M. T., Butt, M. S., Qayyum, M. M., and Suleria, H. A. (2014). Immunity: Plants as effective mediators. Critical reviews in food science and nutrition 54, 1298–1308. doi:10.1080/10408398.2011.633249
Sun, J. N., Sun, W. Y., and Dong, S. F. (2017). Discussion on efficacy evaluation thought and method for innovation medicine of Chinese herbal compound formula based on clinical application characteristics. Zhongguo Zhong yao za zhi = Zhongguo zhongyao zazhi = China journal of Chinese materia medica 42, 852–855. doi:10.19540/j.cnki.cjcmm.20170103.023
Sun, W., Chen, Y., Li, H., Liu, H., Li, J., Chen, J., et al. (2020). Material basis and molecular mechanisms of Dachengqi decoction in the treatment of acute pancreatitis based on network pharmacology. Biomedicine & pharmacotherapy = Biomedecine & pharmacotherapie 121, 109656. doi:10.1016/j.biopha.2019.109656
Tao, Z., Huang, X. J., Liu, Y., Wang, R., Dong, J. P., Liang, B. Y., et al. (2021). Efficacy of integrated traditional Chinese medicine and anti-retroviral therapy on immunological nonresponse in patients with human immunodeficiency virus/acquired immunodeficiency syndrome: A meta-analysis of randomized controlled trial. Journal of traditional Chinese medicine = Chung i tsa chih ying wen pan 41, 669–676. doi:10.19852/j.cnki.jtcm.2021.05.002
Torres, L. K., Pickkers, P., and van der Poll, T. (2022). Sepsis-induced immunosuppression. Annual review of physiology 84, 157–181. doi:10.1146/annurev-physiol-061121-040214
Wang, B., Bellot, G. L., Iskandar, K., Chong, T. W., Goh, F. Y., Tai, J. J., et al. (2020). Resveratrol attenuates TLR-4 mediated inflammation and elicits therapeutic potential in models of sepsis. Scientific reports 10, 18837. doi:10.1038/s41598-020-74578-9
Wang, J., Song, H., Ge, F., Xiong, P., Jing, J., He, T., et al. (2022). Landscape of DILI-related adverse drug reaction in China Mainland. Acta Pharmaceutica Sinica B 12, 4424–4431. doi:10.1016/j.apsb.2022.04.019
Wang, N., Mao, L., Yang, L., Zou, J., Liu, K., Liu, M., et al. (2017). Resveratrol protects against early polymicrobial sepsis-induced acute kidney injury through inhibiting endoplasmic reticulum stress-activated NF-κB pathway. Oncotarget 8, 36449–36461. doi:10.18632/oncotarget.16860
Wang, N., Zhang, X., Ma, Z., Niu, J., Ma, S., Wenjie, W., et al. (2020). Combination of tanshinone IIA and astragaloside IV attenuate atherosclerotic plaque vulnerability in ApoE(-/-) mice by activating PI3K/AKT signaling and suppressing TRL4/NF-κB signaling. Biomedicine & pharmacotherapy = Biomedecine & pharmacotherapie 123, 109729. doi:10.1016/j.biopha.2019.109729
Wang, Q. L., Yang, L., Peng, Y., Gao, M., Yang, M. S., Xing, W., et al. (2019). Ginsenoside Rg1 regulates SIRT1 to ameliorate sepsis-induced lung inflammation and injury via inhibiting endoplasmic reticulum stress and inflammation. Mediators of inflammation 2019, 6453296. doi:10.1155/2019/6453296
Wang, Q., Yang, X., Song, Y., Sun, X., Li, W., Zhang, L., et al. (2021). Astragaloside IV-targeting miRNA-1 attenuates lipopolysaccharide-induced cardiac dysfunction in rats through inhibition of apoptosis and autophagy. Life sciences 275, 119414. doi:10.1016/j.lfs.2021.119414
Wang, S., Long, S., Deng, Z., and Wu, W. (2020). Positive role of Chinese herbal medicine in cancer immune regulation. The American journal of Chinese medicine 48, 1577–1592. doi:10.1142/S0192415X20500780
Wang, X., Zhao, Z., Mao, J., Du, T., Chen, Y., Xu, H., et al. (2019). Randomized, double-blinded, multicenter, placebo-controlled trial of shenfu injection for treatment of patients with chronic heart failure during the acute phase of symptom aggravation (Yang and qi deficiency syndrome). Evidence-based complementary and alternative medicine eCAM 2019, 9297163. doi:10.1155/2019/9297163
Wang, Y., Zhang, Q., Chen, Y., Liang, C. L., Liu, H., Qiu, F., et al. (2020). Antitumor effects of immunity-enhancing traditional Chinese medicine. Biomedicine & pharmacotherapy = Biomedecine & pharmacotherapie 121, 109570. doi:10.1016/j.biopha.2019.109570
Wen-Ting, S., Fa-Feng, C., Li, X., Cheng-Ren, L., and Jian-Xun, L. (2012). Chinese medicine shenfu injection for heart failure: A systematic review and meta-analysis. Evidence-Based complementary and alternative medicine. eCAM 2012, 713149.
Xia, M. L., Xie, X. H., Ding, J. H., Du, R. H., and Hu, G. (2020). Astragaloside IV inhibits astrocyte senescence: Implication in Parkinson's disease. Journal of neuroinflammation 17, 105. doi:10.1186/s12974-020-01791-8
Xia, S., Ni, Y., Zhou, Q., Liu, H., Xiang, H., Sui, H., et al. (2019). Emodin attenuates severe acute pancreatitis via antioxidant and anti-inflammatory activity. Inflammation 42, 2129–2138. doi:10.1007/s10753-019-01077-z
Xiao, T., Cui, Y., Ji, H., Yan, L., Pei, D., and Qu, S. (2021). Baicalein attenuates acute liver injury by blocking NLRP3 inflammasome. Biochemical and biophysical research communications 534, 212–218. doi:10.1016/j.bbrc.2020.11.109
Xie, R., Liu, M., and Li, S. (2019). Emodin weakens liver inflammatory injury triggered by lipopolysaccharide through elevating microRNA-145 in vitro and in vivo. Artificial cells, nanomedicine, and biotechnology 47, 1877–1887. doi:10.1080/21691401.2019.1614015
Xie, S., Yang, T., Wang, Z., Li, M., Ding, L., Hu, X., et al. (2020). Astragaloside IV attenuates sepsis-induced intestinal barrier dysfunction via suppressing RhoA/NLRP3 inflammasome signaling. International immunopharmacology 78, 106066. doi:10.1016/j.intimp.2019.106066
Xu, G., Wan, H., Yi, L., Chen, W., Luo, Y., Huang, Y., et al. (2021). Berberine administrated with different routes attenuates inhaled LPS-induced acute respiratory distress syndrome through TLR4/NF-κB and JAK2/STAT3 inhibition. European journal of pharmacology 908, 174349. doi:10.1016/j.ejphar.2021.174349
Xu, P., Zhang, W. Q., Xie, J., Wen, Y. S., Zhang, G. X., and Lu, S. Q. (2020). Shenfu injection prevents sepsis-induced myocardial injury by inhibiting mitochondrial apoptosis. Journal of ethnopharmacology 261, 113068. doi:10.1016/j.jep.2020.113068
Yan, J. J., Du, G. H., Qin, X. M., and Gao, L. (2020). Baicalein attenuates the neuroinflammation in LPS-activated BV-2 microglial cells through suppression of pro-inflammatory cytokines, COX2/NF-κB expressions and regulation of metabolic abnormality. International immunopharmacology 79, 106092. doi:10.1016/j.intimp.2019.106092
Yang, C., Mo, Y., Xu, E., Wen, H., Wei, R., Li, S., et al. (2019). Astragaloside IV ameliorates motor deficits and dopaminergic neuron degeneration via inhibiting neuroinflammation and oxidative stress in a Parkinson's disease mouse model. International immunopharmacology 75, 105651. doi:10.1016/j.intimp.2019.05.036
Yang, G., Hu, R. Y., Deng, A. J., Huang, Y., and Li, J. (2016). Effects of electro-acupuncture at zusanli, guanyuan for sepsis patients and its mechanism through immune regulation. Chinese journal of integrative medicine 22, 219–224. doi:10.1007/s11655-016-2462-9
Yang, J., Li, Y. Z., Hylemon, P. B., Zhang, L. Y., and Zhou, H. P. (2017). Cordycepin inhibits LPS-induced inflammatory responses by modulating NOD-Like Receptor Protein 3 inflammasome activation. Biomedicine & pharmacotherapy = Biomedecine & pharmacotherapie 95, 1777–1788. doi:10.1016/j.biopha.2017.09.103
Yang, L., Zhang, Z., Zhuo, Y., Cui, L., Li, C., Li, D., et al. (2018). Resveratrol alleviates sepsis-induced acute lung injury by suppressing inflammation and apoptosis of alveolar macrophage cells. American journal of translational research 10, 1961–1975.
Yang, R., Yang, H., Wei, J., Li, W., Yue, F., Song, Y., et al. (2021). Mechanisms underlying the effects of lianhua qingwen on sepsis-induced acute lung injury: A network pharmacology approach. Frontiers in pharmacology 12, 717652. doi:10.3389/fphar.2021.717652
Yang, Y., Ting, W., Xiao, L., Shufei, F., Wangxiao, T., Xiaoying, W., et al. (2017). Immunoregulation of shenqi fuzheng injection combined with chemotherapy in cancer patients: A systematic review and meta-analysis. eCAM 2017, 5121538. doi:10.1155/2017/5121538
You, L., Zhang, D., Geng, H., Sun, F., and Lei, M. (2021). Salidroside protects endothelial cells against LPS-induced inflammatory injury by inhibiting NLRP3 and enhancing autophagy. BMC complementary medicine and therapies 21, 146. doi:10.1186/s12906-021-03307-0
Yu, Y. H., Cui, N. Q., Fu, Q., and Li, J. (2005). Change of TH1/TH2 cytokine equilibrium in rats with severe sepsis and therapeutic effect of recombinant interleukin-12 and Shenmai injection. Chinese journal of integrative medicine 11, 136–141. doi:10.1007/BF02836471
Zhang, E., Wang, J., Chen, Q., Wang, Z., Li, D., Jiang, N., et al. (2020). Artesunate ameliorates sepsis-induced acute lung injury by activating the mTOR/AKT/PI3K axis. Gene 759, 144969. doi:10.1016/j.gene.2020.144969
Zhang, F., Geng, Y., Zhao, H., Wang, H., Zhang, Y., Li, D., et al. (2018). Effects of huanglian jiedu decoration in rat gingivitis. eCAM 2018, 8249013. doi:10.1155/2018/8249013
Zhang, F. L., Zhou, B. W., Yan, Z. Z., Zhao, J., Zhao, B. C., Liu, W. F., et al. (2020). 6-Gingerol attenuates macrophages pyroptosis via the inhibition of MAPK signaling pathways and predicts a good prognosis in sepsis. Cytokine 125, 154854. doi:10.1016/j.cyto.2019.154854
Zhang, H., Shan, Y., Wu, Y., Xu, C., Yu, X., Zhao, J., et al. (2017). Berberine suppresses LPS-induced inflammation through modulating Sirt1/NF-κB signaling pathway in RAW264.7 cells. International immunopharmacology 52, 93–100. doi:10.1016/j.intimp.2017.08.032
Zhang, L., Huang, Z., Shi, X., Hu, S., Litscher, D., Wang, L., et al. (2018). Protective Effect of Electroacupuncture A. T. Zusanli on Myocardial Injury in Septic RatseCAM. Evidence-based complementary and alternative medicine, 2018, 6509650.
Zhang, N., Liu, J., Qiu, Z., Ye, Y., Zhang, J., and Lou, T. (2017). Shenfu injection for improving cellular immunity and clinical outcome in patients with sepsis or septic shock. The American journal of emergency medicine 35, 1–6. doi:10.1016/j.ajem.2016.09.008
Zhang, Q., Li, C., Shao, F., Zhao, L., Wang, M., and Fang, Y. (2017). Efficacy and safety of combination therapy of shenfu injection and postresuscitation bundle in patients with return of spontaneous circulation after in-hospital cardiac arrest: A randomized, assessor-blinded, controlled trial. Critical care medicine 45, 1587–1595. doi:10.1097/CCM.0000000000002570
Zhang, R., Zhu, X., Bai, H., and Ning, K. (2019). Network pharmacology databases for traditional Chinese medicine: Review and assessment. Frontiers in pharmacology 10, 123. doi:10.3389/fphar.2019.00123
Zhang, W., Xu, J. H., Yu, T., and Chen, Q. K. (2019). Effects of berberine and metformin on intestinal inflammation and gut microbiome composition in db/db mice. Biomedicine & pharmacotherapy = Biomedecine & pharmacotherapie 118, 109131. doi:10.1016/j.biopha.2019.109131
Zhang, X., Wang, Q., Wang, X., Chen, X., Shao, M., Zhang, Q., et al. (2019). Tanshinone IIA protects against heart failure post-myocardial infarction via AMPKs/mTOR-dependent autophagy pathway. Biomedicine & pharmacotherapy = Biomedecine & pharmacotherapie 112, 108599. doi:10.1016/j.biopha.2019.108599
Zhang, Z., Shi, Y., Cai, D., Jin, S., Zhu, C., Shen, Y., et al. (2018). Effect of electroacupuncture at ST36 on the intestinal mucosal mechanical barrier and expression of occludin in a rat model of sepsis. Acupuncture in medicine journal of the British Medical Acupuncture Society 36, 333–338. doi:10.1136/acupmed-2016-011187
Zhang, Z. S., Zhao, H. L., Yang, G. M., Zang, J. T., Zheng, D. Y., Duan, C. Y., et al. (2019). Role of resveratrol in protecting vasodilatation function in septic shock rats and its mechanism. The journal of trauma and acute care surgery 87, 1336–1345. doi:10.1097/TA.0000000000002466
Zhao, F., Fang, Y., Deng, S., Li, X., Zhou, Y., Gong, Y., et al. (2017). Glycyrrhizin protects rats from sepsis by blocking HMGB1 signaling. BioMed research international 2017, 9719647. doi:10.1155/2017/9719647
Zheng, L., Su, J., Zhang, Z., Jiang, L., Wei, J., Xu, X., et al. (2020). Salidroside regulates inflammatory pathway of alveolar macrophages by influencing the secretion of miRNA-146a exosomes by lung epithelial cells. Scientific reports 10, 20750. doi:10.1038/s41598-020-77448-6
Zhou, H., Cao, H., Zheng, Y., Lu, Z., Chen, Y., Liu, D., et al. (2020). Liang-Ge-San, a classic traditional Chinese medicine formula, attenuates acute inflammation in zebrafish and RAW 264.7 cells. Journal of ethnopharmacology 249, 112427. doi:10.1016/j.jep.2019.112427
Zhou, J., Gu, X., Fan, X., Zhou, Y., Wang, H., Si, N., et al. (2019). Anti-inflammatory and regulatory effects of huanglian jiedu decoction on lipid homeostasis and the TLR4/MyD88 signaling pathway in LPS-induced zebrafish. Frontiers in physiology 10, 1241. doi:10.3389/fphys.2019.01241
Zhou, R. J., Ye, H., Wang, F., Wang, J. L., and Xie, M. L. (2017). Apigenin inhibits d-galactosamine/LPS-induced liver injury through upregulation of hepatic Nrf-2 and PPARγ expressions in mice. Biochemical and biophysical research communications 493, 625–630. doi:10.1016/j.bbrc.2017.08.141
Zhou, W., Lai, X., Wang, X., Yao, X., Wang, W., and Li, S. (2021). Network pharmacology to explore the anti-inflammatory mechanism of Xuebijing in the treatment of sepsis. Phytomedicine international journal of phytotherapy and phytopharmacology 85, 153543. doi:10.1016/j.phymed.2021.153543
Zhu, H., Wang, S., Shan, C., Li, X., Tan, B., Chen, Q., et al. (2021). Mechanism of protective effect of xuan-Bai-cheng-qi decoction on LPS-induced acute lung injury based on an integrated network pharmacology and RNA-sequencing approach. Respiratory research 22, 188. doi:10.1186/s12931-021-01781-1
Zhu, M. F., Xing, X., Lei, S., Wu, J. N., Wang, L. C., Huang, L. Q., et al. (2015). Electroacupuncture at bilateral zusanli points (ST36) protects intestinal mucosal immune barrier in sepsis. Evidence-based complementary and alternative medicine eCAM 2015, 639412. doi:10.1155/2015/639412
Zou, W., Wang, J., and Liu, Y. (2016). Effect of traditional Chinese medicine for treating human immunodeficiency virus infections and acquired immune deficiency syndrome: Boosting immune and alleviating symptoms. Chinese journal of integrative medicine 22, 3–8. doi:10.1007/s11655-015-2122-5
AChE Acetylcholinesterase
AKI Acute kidney injury
AKT Protein kinase B
ALI Acute lung injury
AP-1 Activator protein-1
ApoM Apoliprotein M
AQP Aquaporin
ARDS Acute respiratory distress syndrome
ASC Apoptosis associated speck like protein containing CARD
AST Aminotransferase
BALF Bronchoalveolar lavage fluid
Bcl-2 B cell lymphoma-2
BMDMs Bone marrow-derived macrophages
CARS Compensatory anti-inflammatory response syndrome
CAT Catalase
CCI Chronic critical illness
CK-MB Creatine kinase-MB
CLP Cecal ligation and puncture
cMLC1 cardiac myosin light chain-1
CNKI China National Knowledge Infrastructure
COX-2 Cyclooxygenase 2
cTnI cardiac troponin I
CXCR2 CXC motif chemokine receptor 2
DCQD dachengqi decoction
eNOS endothelial nitric oxide synthase
ER endoplasmic reticulum
ERK extracellular regulated protein kinase
GPx glutathione peroxidase
GR glutathione reductase
GRK2 G protein-coupled receptor kinase-2
GSDMD gasdermin D
GSK3-β glycogen synthase kinase-3β
GST glutathione-S-transferase
HIF-1α hypoxia-inducible factor 1α
HLA-DR human leukocyte antigen DR
HLJDD huanglian jiedu decoction
HMGB1 high mobility group box 1
ICAM-1 intercellular adhesion molecule-1
I-FABP intestinal fatty acid-binding protein
IL-1 interleukin 1
iNOS inducible nitric oxide synthase
IRF-3 interferon regulatory factor 3
JAK Janus kinase
JAM-C junctional adhesion molecule C
LDH lactate dehydrogenase
LPS lipopolysaccharide
MAPK mitogen activated protein kinase
MD2 myeloid differentiation factor 2
MDA malondialdehyde
MDSCs myeloid-derived suppressor cells
MEK mitogen-activated protein kinase repalmitoylated
MKK4 mitogen-activated protein kinase kinase 4
MMP-9 matrix metalloproteinase 9
MPO myeloperoxidase
mTOR mechanistic target of rapamycin kinase
MyD88 myeloid differentiation factor 88
NETs neutrophil extracellular trap
NF-κB nuclear factor-κB
NLRP3 NOD-like receptor family pyrin domain containing 3
NO nitric oxide
Nrf-2 nuclear factor erythroid 2-related factor 2
PAMPs pathogen-associated molecular patterns
PCT procalcitonin
PGE2 prostaglandin E2
PI3K phosphatidylinositol 3-kinase
Pink1 PTEN-induced kinase 1
PKM2 pyruvate kinase M2
PPARγ peroxisome proliferator-activated receptor gamma
RAC-1 Rac family small GTPase 1
ROS reactive oxygen species
S1P Sphingosine-1-Phosphate
SAP severe acute pancreatitis
SDH succinate dehydrogenase
SFI Shenfu injection
Sirt1 Sirtuin1
SMI shengmai injection
SOD superoxide dismutase
SOFA sequential organ failure assessment
STAT signal transducer and activator of transcription
TAB1 TAK1-binding protein1
TCM traditional Chinese medicine
TIR interleukin-1 receptor
TIRAP TIR domain-containing adaptor protein
TKA1 TGF-β-activated kinase1
TLR4 Toll-like receptor 4
TNF-α tumor necrosis factor alpha
TRAF6 TNF receptor associated factor 6
TRIF TIR-domain-containing adapter-inducing interferon-β
TRPM7 transient receptor potential melastatin 7
TSA Tanshinone IIA
VCAM1 vascular cell adhesion molecule 1
VEGF vascular endothelial growth factor
VEGF-B vascular endothelial growth factor B
XBJI xuebijing injection
XJDHD xijiao dihuang decoction
ZO-1 zonula occludens-1
Keywords: sepsis, inflammation, traditional Chinese medicine compounds, herbal monomer extracts, acupuncture
Citation: Song Y, Lin W and Zhu W (2023) Traditional Chinese medicine for treatment of sepsis and related multi-organ injury. Front. Pharmacol. 14:1003658. doi: 10.3389/fphar.2023.1003658
Received: 26 July 2022; Accepted: 02 January 2023;
Published: 19 January 2023.
Edited by:
Hanjun Cheng, Institute for Systems Biology (ISB), United StatesReviewed by:
Yuxiang Fei, China Pharmaceutical University, ChinaCopyright © 2023 Song, Lin and Zhu. This is an open-access article distributed under the terms of the Creative Commons Attribution License (CC BY). The use, distribution or reproduction in other forums is permitted, provided the original author(s) and the copyright owner(s) are credited and that the original publication in this journal is cited, in accordance with accepted academic practice. No use, distribution or reproduction is permitted which does not comply with these terms.
*Correspondence: Wei Zhu, dGpqemt6dzUxMkAxNjMuY29t
†These authors have contributed equally to this work
Disclaimer: All claims expressed in this article are solely those of the authors and do not necessarily represent those of their affiliated organizations, or those of the publisher, the editors and the reviewers. Any product that may be evaluated in this article or claim that may be made by its manufacturer is not guaranteed or endorsed by the publisher.
Research integrity at Frontiers
Learn more about the work of our research integrity team to safeguard the quality of each article we publish.