- 1Graduate School of Biotechnology, College of Life Sciences, Kyung Hee University, Yongin-si, South Korea
- 2Department of Oriental Medicinal Biotechnology, College of Life Science, Kyung Hee University, Yongin-si, South Korea
- 3Hanbangbio Inc., Yongin-si, South Korea
- 4Daedong Korea Ginseng Co., Ltd., Geumsan-gun, South Korea
- 5Exercise Nutrition & Biochemistry Lab, Kyung Hee University, Yongin-si, South Korea
Ginseng and ginsenosides have been reported to have various pharmacological effects, but their efficacies depend on intestinal absorption. Compound K (CK) is gaining prominence for its biological and pharmaceutical properties. In this study, CK-enriched fermented red ginseng extract (DDK-401) was prepared by enzymatic reactions. To examine its pharmacokinetics, a randomized, single-dose, two-sequence, crossover study was performed with eleven healthy Korean male and female volunteers. The volunteers were assigned to take a single oral dose of one of two extracts, DDK-401 or common red ginseng extract (DDK-204), during the initial period. After a 7-day washout, they received the other extract. The pharmacokinetics of DDK-401 showed that its maximum plasma concentration (Cmax) occurred at 184.8 ± 39.64 ng/mL, Tmax was at 2.4 h, and AUC0–12h was 920.3 ± 194.70 ng h/mL, which were all better than those of DDK-204. The maximum CK absorption in the female volunteers was higher than that in the male volunteers. The differentially expressed genes from the male and female groups were subjected to a KEGG pathway analysis, which showed results in the cell death pathway, such as apoptosis and necroptosis. In cytotoxicity tests, DDK-401 and DDK-204 were not particularly toxic to normal (HaCaT) cells, but at a concentration of 250 μg/mL, DDK-401 had a much higher toxicity to human lung cancer (A549) cells than DDK-204. DDK-401 also showed a stronger antioxidant capacity than DDK-204 in both the DPPH and potassium ferricyanide reducing power assays. DDK-401 reduced the reactive oxygen species production in HaCaT cells with induced oxidative stress and led to apoptosis in the A549 cells. In the mRNA sequence analysis, a signaling pathway with selected marker genes was assessed by RT-PCR. In the HaCaT cells, DDK-401 and DDK-204 did not regulate FOXO3, TLR4, MMP-9, or p38 expression; however, in the A549 cells, DDK-401 downregulated the expressions of MMP9 and TLR4 as well as upregulated the expressions of the p38 and caspase-8 genes compared to DDK-204. These results suggest that DDK-401 could act as a molecular switch for these two cellular processes in response to cell damage signaling and that it could be a potential candidate for further evaluations in health promotion studies.
Introduction
Traditional Chinese medicine (TCM) is the oldest medicinal practice in history, and its basic rule is to incorporate the principles of Yin and Yang in all of its therapeutics. Various other medical systems, particularly oriental medicine rooted in Chinese medicine, are still considered valid (Borman and Kim, 1966). Given that ginseng is mentioned as a medicinal herb in the Classic Herbal of Shennong, which was written around 100 CE, it is apparent that the therapeutic history of ginseng began in the ancient times (Dharmananda, 2002). Ginseng is a common name for plants in the Panax family. In Chinese, “gin” refers to man, and “seng” means essence; it was known as a gift to man from the deity of the mountains in ancient times. It is also known as a plant made of crystals of the essence needed to cure human diseases (Hu, 1976). In addition to historical references, fossil evidence shows that plants from the Araliaceous family existed 65 million years ago, and the Panax species are about 38 million years old (Court, 2000). The book of Shanghan Lun, written in 220 CE, mentions the medical applications of and methods to measure 107 formulas, of which 21 contain ginseng. Even today, most people practicing TCM follow the formulations of Shanghan Lun. Dharmananda (2002) documented the medical history of ginseng from 220 CE to the 20th century. Experts in various fields, such as oncology (Majeed et al., 2018; Nakhjavani et al., 2019; Yu-hang et al., 2019), central nervous system (Radad et al., 2011), energy metabolism (Zhang et al., 2017), stroke (Liu et al., 2019), depression (Jin et al., 2019), infectious diseases (Nguyen and Nguyen, 2019), neurology (Huang et al., 2019), skin disorders (Kim and Kim, 2018), Parkinson’s disease (González-Burgos et al., 2015), autophagy (Wu et al., 2019), inflammation (Ramadhania et al., 2022), diabetes (Zhou et al., 2019), hepatology (Gao et al., 2017), obesity (Li and Ji, 2018), mitochondrial activity (Zhou et al., 2019c), cardiology (Zheng et al., 2012), antimicrobials (Kachur and Suntres, 2016), immune functions (Kang and Min, 2012; Riaz et al., 2019), and molecular signaling pathways (Mohanan et al., 2018), have reviewed the continuous details of ginseng’s efficacy to understand how the ginsenosides disrupt diseases as well as their related mechanisms. Ginseng is generally classified into white, red, and black ginseng according to different stages of processing, and all of these ginseng products are available in the market. Depending on the stage of processing, the therapeutic metabolite content varies widely. The different therapeutic functions attributed to products from different steps have been classified previously (Jin et al., 2015; Shin et al., 2019; Zhu et al., 2019). The dried fresh roots are called white ginseng. The process of obtaining red ginseng begins by washing the fresh ginseng roots in water to remove soil particles; then, they are steamed at 90–98 °C for 1–3 h. This process is repeated once or twice more to achieve appropriate gelatinization of the ginseng starch; the product is then dried until the root has a moisture content of 15–18%. This processing method has been used since 1123 CE, although it has been optimized in various ways (Lee et al., 2015). The value of the resulting formulation depends upon the key chemical ingredient, i.e., tri-terpenoid saponins called ginsenosides, which are the key metabolites of ginseng (Christensen, 2008; Liu, 2012; Boopathi et al., 2020). Ginsenosides are classified into major and minor based on their molecular weights. Naturally biosynthesized ginsenosides in plants are called major ginsenosides, and the converted forms are called minor ginsenosides. The conversion method involves hydrolysis of the glycose molecules in the backbone moiety using physical (heat, microwave, and puffing), chemical (acid and alkali), or enzymatic (various glycosidase enzymes, genetic engineering, lactic acid bacteria) techniques. The bioavailability of the major and minor ginsenosides are the key issue in promoting ginsenosides as drug candidates. Ginsenoside compound K (CK) is one of the major metabolites that reaches systemic circulation, where it has its various pharmacological effects (Sharma and Lee, 2020; Murugesan et al., 2022). Recently, the benefits of fermented functional food products (i.e., probiotics) that enhance human gut health and immunity have gained attention for their potential in treating various chronic diseases. The ginseng functional food industry has also risen to leverage the efficacy of ginseng. Another current trend focuses on the advantages of drug combinations over individual drugs, with primarily enhanced efficacy in slowing or reversing disease progression and reduced side effects (Liu et al., 2020). However, choosing effective combinations through trial and error is both tedious and expensive. Therefore, a principle similar to that long used in TCM is suggested to moderate the various side effects without requiring systematic evaluations of the extract formulations (Posadzki et al., 2013). However, such a system needs to accommodate modern medicine by offering valid evidence when identifying novel drug combinations practically (Shin et al., 2021). Therefore, various studies have been conducted to assess the therapeutic effects of individual ginsenosides as well as crude, red, or fermented red ginseng extracts in animal models and human trials (Lee et al., 2012; Jung et al., 2013; Kim, 2013; Sohn et al., 2013; Choi et al., 2016; Choi et al., 2018; Ban et al., 2021; Panossian et al., 2021), but these datasets are insufficient to conclusively demonstrate the effectiveness of ginseng/ginsenosides at the molecular level. In the present study, we examine a CK-enriched fermented ginseng extract DDK-401 in a human trial with a healthy population to understand its effects on various signaling pathways (Figure 1). Moreover, we elucidate its effects on the functional and therapeutic markers already approved for treating various diseases.
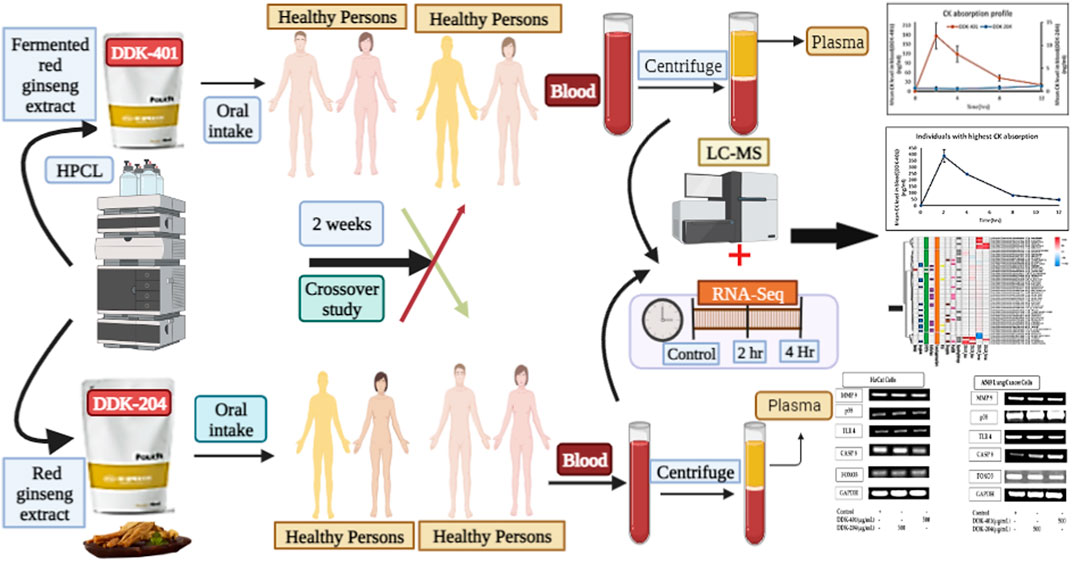
FIGURE 1. Graphical illustration of the fermented red ginseng extract (DDK-401) and red ginseng extract (DDK-204) analyzed by Korean volunteers.
Materials and methods
Ethical committee and study design
A randomized, open-label, single-dose, two-period, two-sequence, crossover study was performed with healthy Korean male and female subjects. This study was performed in accordance with the principles of the Declaration of Helsinki and Korean Good Clinical Practice guidelines. Informed written consent was obtained from each subject in advance. The study was approved by the Institutional Review Board of Kyung Hee University Hospital (KHGIRB-21-419). Eleven healthy male and female Koreans were enrolled in this study, and their age details are shown in Supplementary Table S1. We expected to find large individual variability in the pharmacokinetic profile of CK. To reduce the individual variability in pharmacokinetics caused by sex, we combined and calculated the means of the pharmacokinetic data from the male and female groups separately. The exclusion criteria were any significant clinical illness within 2 weeks before the study, i.e., history of high blood pressure, diabetes, and cardiovascular, hepatic, renal, hematological, gastrointestinal, neurologic, or psychiatric disease; blood donation within 8 weeks before the study; and use of any medications, including prescription and over-the-counter drugs, within 2 weeks before the study. In addition, subjects who previously experienced adverse reactions to ginseng were excluded.
The enrolled subjects were assigned to receive a single oral dose of one of two extracts, DDK-401 (100 mL spout pouch, combination of well-known representative ginsenosides Rg1, Rb1, and Rg3 at 21.51 mg and ginsenoside CK at 31.19 mg) or DDK-204 (100 mL spout pouch, ginsenosides Rg1, Rb1, and Rg3 at 11.29 mg and ginsenoside CK at 0 mg) during the first period. After a 7-day washout, each subject received the other extract. The dose for oral administration was chosen based on the recommended total daily intake of each investigational product.
DDK-401 and DDK-204 extract preparations
CK-enriched fermented ginseng extract (DDK-401) and common red ginseng extract (DDK-204) were supplied by Deadong Korea Ginseng Co., Ltd. (Geumsan, Korea). First, the red ginseng powder was dissolved in a mixture of water and food-grade alcohol and extracted at 75 ± 5 °C. This extraction procedure was repeated 4 times. Then, the supernatant was collected and evaporated at 60 ± 5 °C with 500–760 mmHg vacuum until the sugar content was 65 brix and solid content was ≥60%. Finally, the sample was sterilized at 80–85 °C for 30–40 min and aged at 60 ± 5 °C for 24–75 h; this product was named DDK-204 and used as the control.
Second, the red ginseng concentrate (60 brix) was diluted in water until the solid content was 5%. Then, an enzyme mixture (pectinase and β-glucosidase) was added to the diluted red ginseng concentrate at a concentration of 3% and reacted at 60 ± 2 °C for 114–168 h at 3000 rpm. Thereafter, the enzyme was inactivated at 90 °C for 30 min. Next, the sample was evaporated at 55 ± 5 °C and 500–760 mmHg vacuum until the solid content reached 40%. Then the sample was dissolved in 80% food-grade alcohol and incubated for 1–2 h. Following this, centrifugation was performed at 0.5 m3/h for 5–6 h, and the supernatant was collected. A second evaporation was then performed at 55 ± 5 °C and 500–760 mmHg vacuum until the sugar content was 65 brix and solid content was ≥60%. The concentrate was then fermented with a mixture of Lactobacillus species at 1% concentration and 37 °C for one day. The resulting CK-enriched red ginseng concentrate was named DDK-401 and stored in the refrigerator until it was used for the analysis and bioassays.
Chromatographic conditions for analyzing the ginsenoside profiles of DDK-401 and DDK-204
One g each of DDK-401 and DDK-204 were dissolved in 50 mL of 70% methanol and filtered with a 0.45 μm membrane filter. The samples were then injected into an Ultimate 3000 HPLC system with a PRONTOSIL 120-5-C18 ACE-EPS (250 × 4.6 mm i.d., 5 µm particle size) (Bischoff Chromatography, Leonberg, Germany). The mobile phase consisted of water (solvent A) and acetonitrile (solvent B) in the following gradients: 0–10 min, 20% B; 10–42 min, 29% B; 42–67 min, 41% B; 67–70 min, 47% B; 70–90 min, 71% B; 90–95 min, 71% B. The flow rate of the mobile phase was 1.0 mL/min, and an injection volume of 10 µL was used in the quantitative analysis. The column temperature was maintained constant at 40 °C. The ginsenoside profiles were determined at 203 nm.
Preparation of standard solution for quantitative calibration
A standard stock solution of 10% was prepared by dissolving accurately weighed quantities of the standard for each ginsenoside in high-performance liquid chromatography (HPLC)-grade methanol. These stock solutions were then diluted with HPLC-grade methanol to 200, 100, 50, 25, and 12.5 μg/mL concentrations as working solutions for the quantitative calibrations. The calibration curves and quantitative evaluations were then obtained at 203 nm.
Pharmacokinetic assessment
The quantitative determination of CK concentration in the plasma was achieved using 2 mL of intravenous blood collected from each volunteer before administration and at 2, 4, 8, and 12 h after dosing during each period. The blood samples were centrifuged at 3000 rpm for 10 min, and the supernatant was separated and frozen at -80 °C until analysis. The plasma concentrations of ginsenoside CK were determined by PCAM KOREA Co., Ltd. (Daejeon, Korea) using a HPLC–tandem mass spectrometry system. The chromatographic analysis was performed using a Waters I-class (Waters, USA), with Berberine (Dr. Ehrenstorfer GmbH, Germany) as the internal standard. Chromatographic separation was achieved with an Acquity UPLC BEH C18 column (100 mm × 2.1 mm, 1.7 μm; Waters, USA) maintained at 45 °C. The mobile phase was a gradient of 0.1% formic acid in water and 100% acetonitrile. Mass spectrometry was performed in the positive mode on an API Xevo TQ-XS instrument (Waters, USA) equipped with an electrospray ionization probe. The temperature of the ion source was set to 150 °C, and the voltage of the ion spray was 3 kV. The quantifications were performed by multiple reaction monitoring of the transitions at 645.2–203 nm for ions of ginsenoside CK, with a dwell time of 11.28 min. To validate the quantitative data in terms of linearity, the limit of detection (LOD) and limit of quantification metrics were calculated (Supplementary Table S2).
RNA-sequencing and analysis
The total mRNA was extracted from each blood plasma sample to build the mRNA-seq libraries that were generated using a TruSeq stranded mRNA LT sample prep kit (Illumina, San Diego, CA, USA) following manufacturer protocols and sequenced using a Novaseq 6000 sequencing system (Illumina). The reads were trimmed with Trimmomatic (Bolger et al., 2014) to remove any adapters and low-quality reads, resulting in clean reads for improved paired-end mapping. The trimmed reads were mapped to the Homo sapiens reference genome (GRCm38) transcriptome using Salmon software version 1.3.0 (Patro et al., 2017). Differential gene expressions among the three experimental groups were evaluated using edgeR (version 3.30.3) software (McCarthy et al., 2012). The differentially expressed genes were identified based on a cutoff threshold of p < 0.05 and log-fold change >1 before being subjected to further analyses.
Functional annotations
Functional annotations for each gene were made using the drug discovery protocol. The seven datasets used are included in Supplementary Table S3: DrugBank (Wishart et al., 2018), Human Protein Atlas (Uhlén et al., 2015), STITCH (Szklarczyk et al., 2016), Surfaceome (Bausch-Fluck et al., 2018), Tumor Suppressor Gene Database v2.0 (TSGene) (Zhao et al., 2016), pepBDB (Wen et al., 2019), and Comparative Toxicogenomics Database (Grondin et al., 2021). First, entered the DrugBank ID for each gene to navigate the details of known drugs from the complete database xml file. Second, downloaded the FDA-approved potential drug candidate list from the Human Protein Atlas database. Third, searched STITCH to observe small-molecule drug interactions. Fourth, used Surfaceome to understand the cell surface proteins. Fifth, used TSGene to obtain the cancer therapeutic gene candidates. Sixth, observed the peptide-binding protein interactions.
Cell cytotoxicity assay
Cell cultures
Immortalized human epidermal keratinocyte (HaCaT) and murine macrophage RAW 264.7 cells were cultured in Dulbecco’s modified Eagle’s medium (DMEM) supplemented with 10% fetal bovine serum (FBS) and 1% penicillin-streptomycin. Generally, 89% Roswell Park Memorial Institute (RPMI) 1640 with 10% FBS and 1% penicillin-streptomycin were used to culture the human lung carcinoma cells (A549). All three cell lines were allowed to adhere and develop for 24 h before being treated with different samples in a humidified 37 °C incubator with a 5% CO2 atmosphere.
Cell cytotoxicity assay
We evaluated the cytotoxicities of DDK-401 and DDK-204 on the HaCaT and RAW 264.7 cells using an MTT colorimetric assay, which was performed in 96-well plates (Mathiyalagan et al., 2019; Pu et al., 2021). Seeding was performed at 5 × 104 cells/well (HaCaT) and 1 × 104 cells/well (RAW 264.7), and the 96-well plates were incubated at 37 °C in a humidified atmosphere of 5% CO2 for 24 h (Ramadhania et al., 2022). Subsequently, the cells were treated with various concentrations of DDK-401 or DDK-204 in serum-free-medium at 62.5, 125, 250, and 500 μg/mL for the HaCaT cells and at 25, 50, 100, 250, and 500 μg/mL for the RAW 264.7 and A549 cells, followed by incubation for 24 h. Then, 20 µL of MTT (5 mg/mL, phosphate-buffered saline (PBS), Life Technologies, Eugene, OR, USA) were added to the cells at 37 °C for 4 h. The insoluble formazan was dissolved by placing 100 μL of dimethylsiloxane (DMSO) in each well and absorbance was measured at 570 nm using an enzyme-linked immunosorbent assay (ELISA) microplate reader (Bio-Tek, Instruments, Inc., Winooski, VT, USA).
Antioxidant assay
In vitro DPPH assay
The 2,2-diphenyl-1-picryl-hydrazyl (DPPH) method was used with a slight modification to estimate the free-radical scavenging activities of the samples (Subbiah et al., 2020). DPPH (0.2 mM) was dissolved with ethanol (pro-analysis grade) to obtain a DPPH radical solution. Then, 20 µL of the sample extract and 180 µL of the DPPH solution were added to a 96-well plate and incubated at 25 °C for 30 min in the dark, followed by absorbance measurement at 517 nm. Vitamin C (ascorbic acid) standard curves with concentrations from 0 to 100 μg/mL were used to determine the DPPH radical scavenging activity, which is expressed in milligrams of ascorbic acid equivalent per gram (mg AAE/g) of the extract.
Reducing power assay
The reducing capacity of a compound indicates its potential antioxidant activity. To conduct this assay (Akter et al., 2021), 100 µL of various concentrations of the samples were mixed with 250 µL of 0.2 mM phosphate buffer (pH 6.6) and 250 µL of 1% potassium ferricyanide. The mixtures were then incubated at 50 °C for 20 min. After cooling, 250 µL of 10% trichloroacetic acid was added to the mixtures and centrifuged at 3000 rpm for 10 min. Then, 50 µL of the upper layer of each mixed solution was transferred and mixed with 50 µL of distilled water and 250 µL of 0.1% ferric chloride solution in a 96-well plate. The absorbance was then measured at 700 nm using a UV spectrometer microplate reader (Bio-Tek, Instruments, Inc., Winooski, VT, USA). Vitamin C was used as the standard, and a blank solution was prepared by omitting the sample; the results are expressed as mg AAE/g of extract.
Reactive oxygen species generation assays in HaCaT and lung cancer cells
Effects of DDK-401 on reactive oxygen species production in HaCaT cells under oxidative stress
Intracellular reactive oxygen species (ROS) were determined using the 2′,7′-dichlorodihydro-fluorescein diacetate (DCFH-DA) reagent, as described by Pu et al. (2021), with a slight modification. Briefly, HaCaT cells (5 × 104 cells/well) were seeded in a 96-well plate (Nest Inc., Corning, NY, USA) and incubated for 24 h at 37 °C and 5% CO2. To assess the antioxidant activity, the cells were treated with H2O2 (500 μmol/L) for 2 h, and the supernatant was aspirated. The cells were then either treated or not treated with different concentrations of the samples for 24 h. Vitamin C was used as the positive control. After washing the cells twice with PBS, we added 20 μM DCFH-DA in PBS and incubated them for another 20 min. The supernatant was next removed by washing the cells with PBS twice, and a multimodal plate reader was used to measure the fluorescence intensity at an excitation wavelength of 485 nm and emission wavelength of 528 nm.
Effects of DDK-401 on reactive oxygen species production in A549 cells under oxidative stress
To detect the ROS intensity of human lung cancer cells (A549), we used the DCFH-DA reagent with fluorescent image capture technique. We plated the cells at a density of 1 × 104 cells/well in 96-well culture plates, allowed them to adhere, and then placed them in an incubator overnight to achieve 100% confluency. The A549 cells were then treated with various concentrations of DDK-401 or DDK-204 (0, 25, 50, 100, 250, and 500 μg/mL) for 24 h. The next day, the cells were stained by adding 100 μL of DCFH-DA solution (10 μM) to each well and incubated in the dark for 30 min. The old media were discarded, and the cells were washed twice with 1× PBS (100 μL/well). A multimodal plate reader (spectrofluorometer) was used to determine the fluorescence intensity caused by ROS production at an excitation wavelength of 485 nm and emission wavelength of 528 nm.
Inflammation inhibition assay
The detection of nitric oxide (NO) levels has been described previously (Ramadhania et al., 2022). The RAW 264.7 cells (1 × 104) were placed in 24-well culture plates and incubated for 24 h at 37 °C in a humidified environment with 5% CO2. Then, they were treated with different concentrations of DDK-401 or DDK-204 (0, 25, 50, 100, 250, and 500 μg/mL) for 1 h. In the presence of the samples, 1 μg/mL lipopolysaccharide (LPS) was used as the stimulator, and the treated cells were placed in an incubator for one day. The nitrite levels in the cell media were determined using the Griess reagent: 100 μL of the stimulated supernatant was mixed with an equivalent volume of the Griess reagent. A microplate reader was used to compare the absorbance at 540 nm with a standard curve obtained using sodium nitrite (BioTek Instruments, Inc.). L-NMMA (50 μM), a standard inhibitor, was used as the positive control in this experiment. Each assay was repeated three times, and the results are expressed in terms of percentage of NO production.
Reverse transcription polymerase chain reaction (RT-PCR)
The total RNA was extracted using QIAzol lysis reagents (QIAGEN, Germantown, MD, USA), and the reverse transcription reactions were performed using 1 µg of total RNA in 20 µL of the reaction buffer with an amfiRivert reverse transcription kit (GenDepot, Barker, TX, USA), according to manufacturer instructions. The obtained cDNA was amplified with primers, as shown in Table 1. The reaction was cycled 35 times: 30 s at 95 °C, 30 s at 60 °C, and 50 s at 72 °C. Using 1% agarose gels, the amplified RT-PCR products were analyzed, visualized using Safe-Pinky DNA Gel Staining (GenDepot, Barker, TX, USA), and imaged under ultraviolet light.
Statistical analysis
All experiments were performed at least in triplicate (n = 3) unless stated otherwise. The experimental data are reported as mean ± standard error (SEM). Statistical significances between the control and sample groups were evaluated by Student’s t-test with a two-tailed distribution and two-sample equal variances. A greater extent of statistical significance is indicated by an increasing number of asterisks (*p < 0.05, **p < 0.01, and ***p < 0.001) and hash markers (#p < 0.05, ##p < 0.01 and ###p < 0.001). The hash marker (#) indicates significance between the normal and stimulated controls, and the asterisk (*) indicates significant differences between the stimulation groups (DDK-204 or DDK-401).
Results and discussion
Ginsenoside absorption profiling after oral intake
Preparation of DDK-401 and DDK-204
Ginsenosides and ginseng extracts have been reported to have various pharmacological effects, and ginseng has been used as a medicinal herb in TCM for several centuries. However, ginsenosides are mainly absorbed in the gastrointestinal tract after the gut microbes hydrolyze the linear carbohydrates from their backbones. In addition, the minor ginsenosides, which have only one or no glycose moieties, generally reach the systemic circulation. Thus, the absorption and bioavailability of ginsenosides greatly depend on the gastrointestinal bioconversion ability of each individual, and the minor saponins must be enriched by various processing technologies. Bioconversion techniques such as puffing (Pu et al., 2021) and heat treatment (steaming) do not produce ginsenoside CK (Piao et al., 2020), which is one of the active metabolites that reaches systemic circulation and has various pharmacological activities (Sharma and Lee, 2020). Therefore, the pharmacologically active minor saponin CK must be enriched by the edible enzymes in the ginseng extract to maximize its biological activity irrespective of an individual’s gut function. This study, we aimed to increase the total ginsenoside and CK content using pectinase and β-glucosidase enzymes to begin the glucose hydrolysis of major ginsenosides in red ginseng concentrate, such as Rb1, Rd, and Rg3. We performed additional fermentation with Lactobacillus species at 37 °C for 1 day to accelerate the hydrolysis of the glucose molecules from the major ginsenosides to increase CK production (Table 2). The synthesis of CK has been mainly reported from the hydrolysis of glycose molecules of the major ginsenosides, such as Rb1, Rb2, Rd, Rc, compound O, compound Y, compound Mc, Rg3, gypenoside XVII, and F2 (Sharma and Lee, 2020). As a result of the above processes, the fermented red ginseng extract (DDK-401) was enriched, with 10 mg/g of CK and 32.98 mg/g of total ginsenoside content, compared to the control red ginseng extract (DDK-204), which contained 9.72 mg/g of total ginsenosides and without CK. The CK was thus clearly produced by the fermentation process and not the steaming process (Piao et al., 2020). It was previously reported that the bioconversion and fermentation of red ginseng yields CK in Korean ginseng (Choi et al., 2016; Fukami et al., 2018).
Ginsenoside absorption profiling after oral intake of DDK-401
After oral intake, the human volunteers showed greater CK absorption from the fermented DDK-401 extract than from the control red ginseng extract (DDK-204) (Figure 2A). The Tmax was 2.4 h, Cmax was 184.8 ± 39.64 ng/mL, and AUC0–12h was 920.3 ± 194.70 ng∙h/mL for DDK-401, whereas the Tmax was 12 h, Cmax was 2.5 ± 1.09 ng/mL, and AUC0–12h was 11.3 ± 4.66 ng∙h/mL for DDK-204 (Table 3). These pharmacokinetic patterns are similar to those in other reports (Sharma and Lee, 2020). Although various studies have reported enhanced CK absorption after oral administration of fermented red ginseng extract, the concentration of CK in the blood plasma still varies by individual, as shown in Figure 2B (individuals with the highest CK absorption profiles).
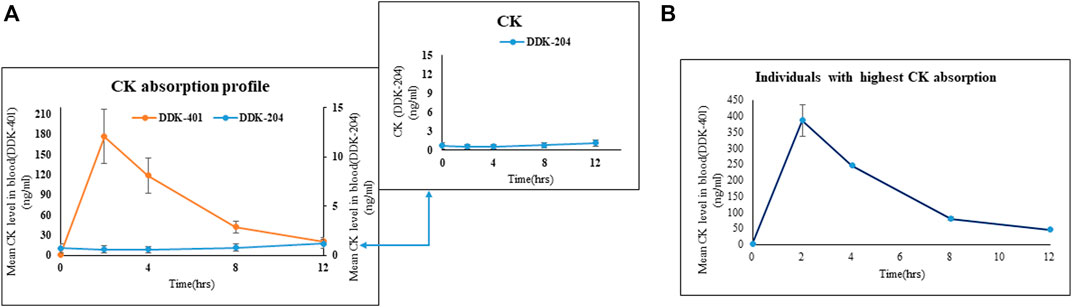
FIGURE 2. Absorption profiles of CK in human blood after oral intake of fermented red ginseng extract (DDK-401) and red ginseng extract (DDK-204). (A) Mean CK level. Inset shows the mean CK absorption profile after DDK-204 intake. (B) Mean CK level from individuals with highest CK absorption in this study.
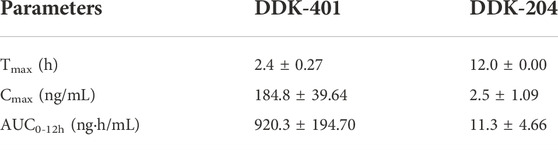
TABLE 3. Pharmacokinetic parameters of CK in human blood after oral intake of fermented ginseng extract (DDK-401) and red ginseng extract (DDK-204).
Variations in the CK absorption profiles between male and female groups
Although high CK absorption has been reported previously (Sharma and Lee, 2020), differences in the absorption patterns between males and females following oral intake of fermented red ginseng extract have not been explored. Our results indicate that as a group, the female volunteers absorbed more CK (Figure 3B) than the male volunteers (Figure 3A), although this pattern also applied to individual female and male volunteers (Figure 3C). Similarly, the female volunteers were previously reported to absorb higher concentrations of CK than males after oral doses of a high concentration of CK (Chen et al., 2017).
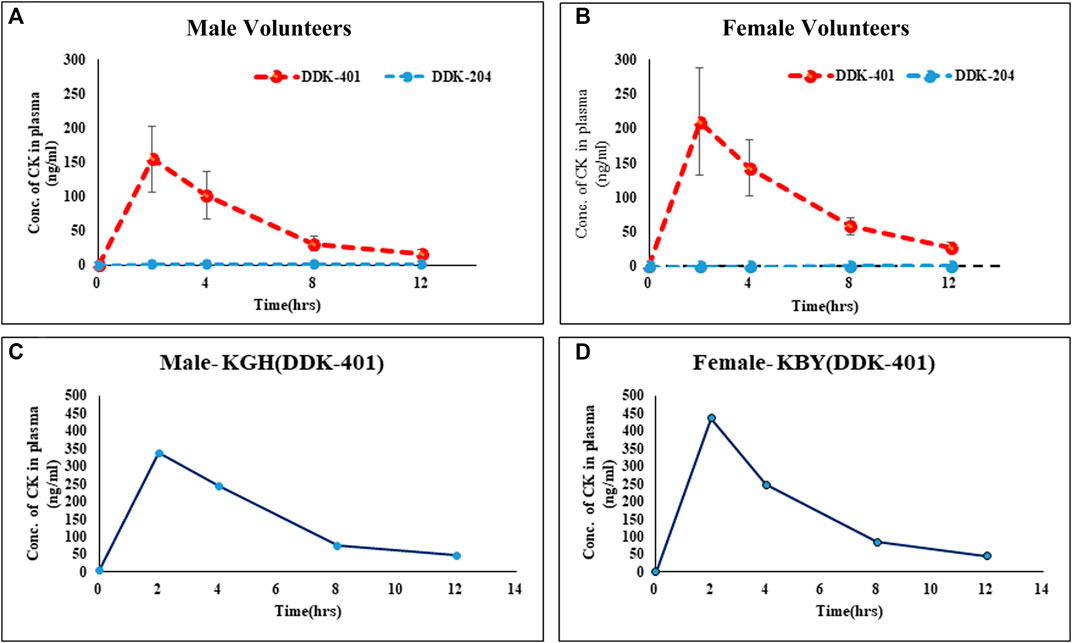
FIGURE 3. Absorption profiles of CK in human blood serum after oral intake of fermented red ginseng extract (DDK-401) and red ginseng extract (DDK-204): (A) male volunteers, (B) female volunteers; maximum CK absorption in individual (C) male and (D) female.
RNA sequence analysis of blood plasma after oral intake of samples
Differential gene expression and KEGG pathway enrichment
As explained in the Pharmacokinetic Assessment section, whole mRNA transcripts were assessed against the human reference genome for genome-wide differential transcript expressions. The samples were grouped into four categories, namely DDK-401 (male and female) and DDK-204 (male and female). Overall, 701 transcripts were found to have differential expressions (Supplementary Table S3), and the transcripts overlapped among the groups, as illustrated in a Venn diagram (Supplementary Figure S1). The transcripts belonging to the tumor suppressor genes category are displayed in a heatmap (Figure 4). Overall, nine annotations were included in this study, as explained earlier. In addition, all differentially expressed genes were subjected to KEGG pathway enrichment in the David online webserver, which showed that the cell death pathways, such as apoptosis and necroptosis, were enriched by the extract treatments (Supplementary Table S4). Finally, we selected gene candidates (FOXO3, cysteine-aspartic protease 8 (caspase-8), toll-like receptor 4 (TLR4), and matrix metallopeptidase 9 (MMP-9)) for the RT-PCR expression analysis because these are known to be involved in the signaling and cell-death pathways as well as tumor suppression.
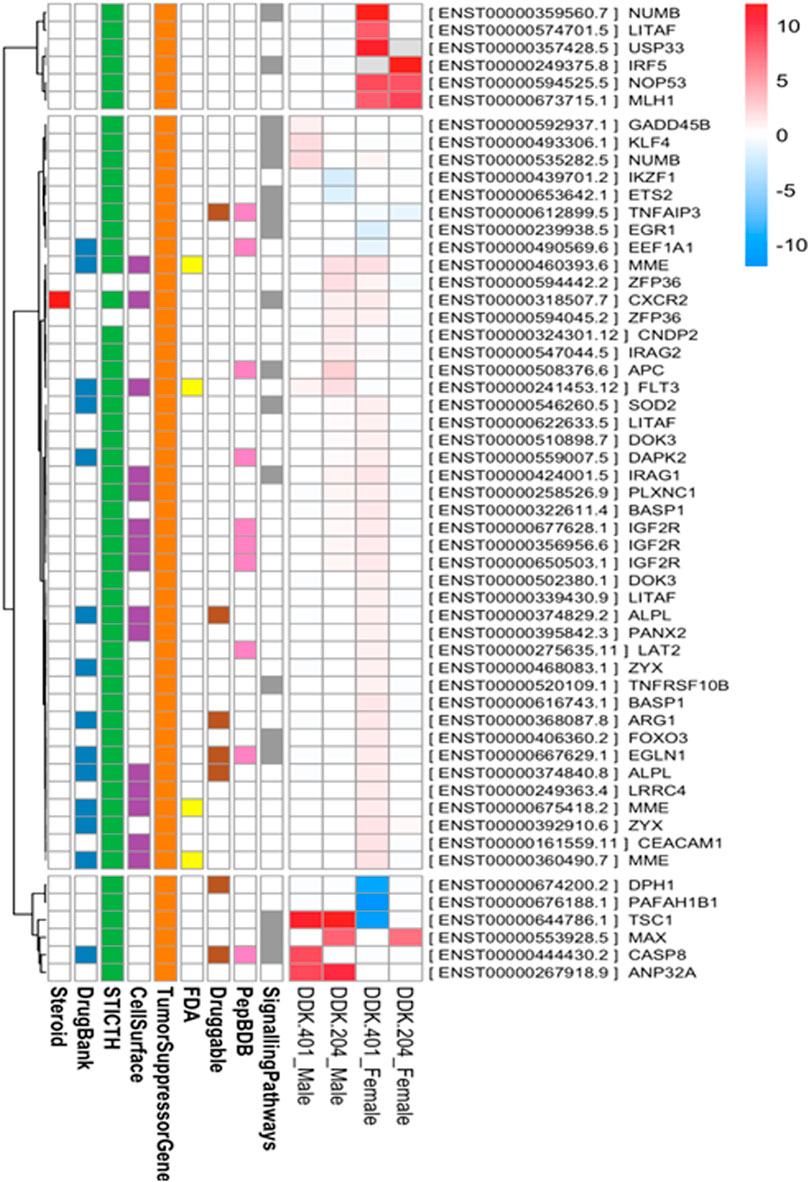
FIGURE 4. Transcripts belonging to the “tumor suppressor genes” category that were differentially expressed between the DDK-401 male and female groups and DDK-204 male and female groups displayed as a heatmap.
Effects of DDK-401 on the viabilities of HaCaT and lung cancer cells
The cytotoxicities of DDK-401 and DDK-204 to HaCaT cells was determined for safety purposes. The HaCaT cells represent normal cell conditions, and lung cancer cells (A549) were used to examine the apoptosis signaling pathway. Each sample was evaluated at various sample concentrations (62.5, 125, 250, and 500 μg/mL in HaCaT and 25, 50, 100, 250, and 500 μg/mL in A549 cells). As shown in Figure 5, at concentrations less than 500 μg/mL, both DDK-401 and DDK-204 were nontoxic to HaCaT cells. In the A549 cells, DDK-401 demonstrated minimal toxicity after 24 h at 250 μg/mL. At a concentration of 500 μg/mL after 24 h, DDK-401 showed significantly decreased cancer cell proliferation than DDK-204. Moreover, A549 cell viability was reduced by DDK-401 in a dose-dependent manner. The cytotoxicity results in this investigation match those in a previous report (Yu et al., 2018).
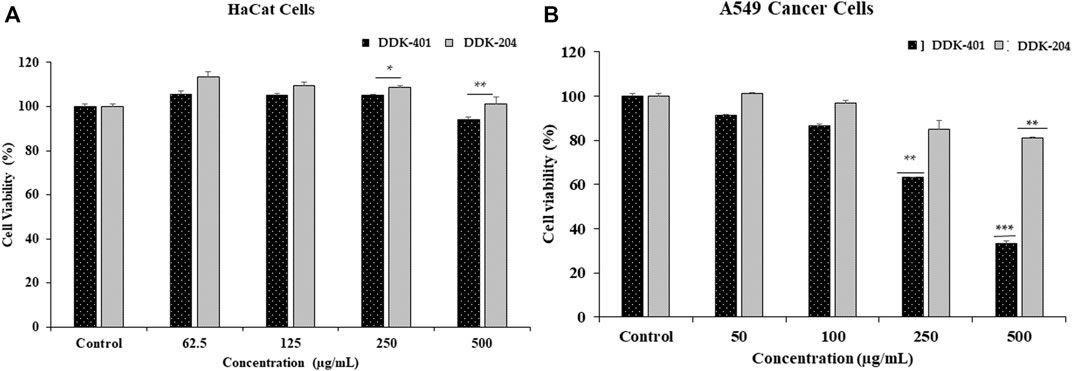
FIGURE 5. In vitro cytotoxicities of DDK-401 and DDK-204 samples in (A) HaCaT and (B) A549 lung cancer cells over 24 h. The graph shows the mean ± SD value of four repetitions. **p < 0.01; ***p < 0.001 indicate significant differences from the control groups.
The results shown in Figure 5 indicate that at 500 μg/mL, DDK-401 and DDK-204 were only mildly toxic, from which it can be concluded that both substances are relatively safe when cell conditions are normal. On the other hand, in lung cancer A549 cells, which represent cell damage and imbalanced conditions, DDK-401 had a much higher toxicity than DDK-204, producing apoptosis of the cancer cells.
Antioxidant content shown by DPPH assay and ROS generation in HaCaT and cancer cells
Antioxidant capacity: DPPH and reducing power assays
The DPPH scavenging and potassium ferricyanide reducing power assays were used to evaluate the antioxidant capacities of DDK-401 and DDK-204, and the results are shown in Table 4. The most frequently used antioxidant standard for these assays is vitamin C; therefore, the results of the DPPH and potassium ferricyanide reducing power assays are expressed in terms of mg AAE/g of the extract. These assays are widely used to determine the antioxidant properties of compounds as free radical scavengers or hydrogen donors (Warinhomhoun et al., 2021) as well as the ability of the compounds to transform from Fe3+/ferricyanide complex to Fe2+/ferrous forms (Aryal et al., 2019). DDK-401 showed higher antioxidant abilities in both the DPPH and potassium ferricyanide reducing power assays, with values of 0.093 ± 0.02 and 0.340 ± 0.001 mg AAE/g of extract, respectively. In the DPPH assay, the antioxidant capacity of DDK-401 was generally 2 times higher than that of DDK-204, and in the potassium ferricyanide reducing power assay, it was 3 times higher than that of DDK-204. In agreement with a previous study (Jung et al., 2019; Park et al., 2021), we found that CK-enriched ginseng extract (DDK-401) exhibited greater antioxidant activity than the common red ginseng extract (DDK-204). This result could be attributed to CK’s potential for radical scavenging activity in antioxidant assays (Baik et al., 2021). Antioxidants, whether endogenously produced or supplied by external sources, can scavenge ROS and reduce cellular oxidation, thereby alleviating oxidative stress (Liu et al., 2018).
Effect of DDK-401 on ROS production in HaCaT cells with H2O2-induced oxidative stress
We used the DCFH-DA assay to investigate the antioxidant properties of DDK-401 and determine whether it could reduce accumulated intracellular ROS in H2O2-induced HaCaT cells. Commonly, H2O2 is used to induce intracellular ROS and produce imbalance in the cellular oxidant–antioxidant levels. Because the mitochondria are the major sources of ROS, mitochondrial dysfunction caused by excess ROS can lead to apoptosis and DNA damage (Zhang et al., 2020). The mean value of the ROS levels measured in the group treated with 500 μM H2O2 was 260% higher than that in the control group. The trend of decreased cell viability after H2O2 exposure is shown in Figure 6A. Vitamin C was used as the positive control. For H2O2-induced oxidative stress in the HaCaT cells, DDK-401 was stronger than DDK-204 in a dose-dependent manner. At a concentration of 250 μg/mL, DDK-401 and DDK-204 reduced ROS levels by an average of 23% and 7%, respectively, compared with the group treated with only H2O2 (Figure 6A). These results may be attributed to the CK in DDK-401; previous studies have reported that CK activates the NF-κB and JNK pathways, which contribute to the inhibition of TNF-α and anti-inflammatory activity related to ROS inhibition (Choi et al., 2007; Park et al., 2012). Oxidative stress usually activates certain signaling pathways, including the p38, MMP, and caspase pathways.
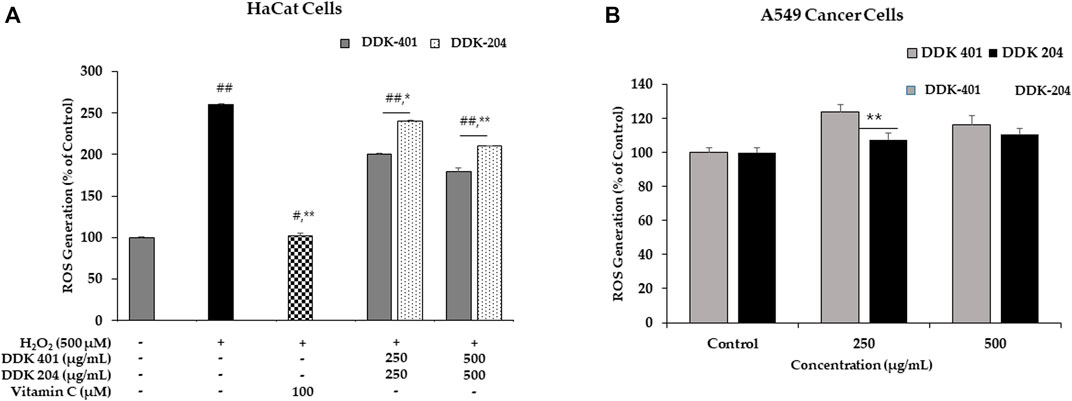
FIGURE 6. (A) Reactive oxygen species (ROS) inhibition by DDK-401 and DDK-204 in HaCaT cell lines treated with H2O2. The graph shows the mean ± SD value of three repetitions. *p < 0.05 and **p < 0.01 indicate significant differences from the control groups; #p < 0.05 and ##p < 0.01 indicate significant differences from the H2O2 stimulation groups. (B) ROS generation by DDK-401 and DDK-204 in A549 cancer cells. The graph shows the mean ± SD value of three repetitions. **p < 0.01 indicates significant difference from the control group.
Effect of DDK-401 on ROS generation to induce apoptosis of cancer cells
In A549 cells, the DCFH-DA reagent was used to measure the intracellular ROS levels with DDK-401 and DDK-204 at various concentrations. Red ginseng extract has been shown to induce cancer cell death by causing DNA damage, stimulating ROS production, and activating numerous pro-apoptotic markers. Furthermore, mitochondrial damage can cause release of ROS because the mitochondria are the largest source of ROS (Brunelle and Chandel, 2002). At 500 g/mL, DDK-401 produced a higher level of ROS than DDK-204, as shown in Figure 6B. Thus, the antiproliferative action of DDK-401 could be assessed by measuring the ROS levels. Intracellular oxidative stress is known to cause cell death in a variety of cell lines; therefore, this assessment was crucial. The data show that DDK-401 could be a potential drug candidate in clinical trials for the treatment of lung cancer.
ROS have been identified as signaling molecules in various pathways that regulate both cell survival and cell death depending on the level (Azad et al., 2008; Chen et al., 2018). Apoptosis and autophagy are important molecular processes that maintain balance in organisms and cells. Apoptosis destroys damaged or unwanted cells, while autophagy maintains cellular homeostasis by recycling specific intracellular organelles and molecules, although autophagy can result in cell death in some cases (Thorburn, 2008; Fan and Zong, 2013). We conclude that DDK-401 reduced ROS production in normal cells (HaCaT) experiencing oxidative stress and also led to apoptosis of lung cancer (A549) cells, suggesting that DDK-401 could act as a molecular switch for these two cellular processes in response to cell damage signaling.
Effect of DDK-401 on gene expression affecting apoptotic and inflammatory responses
Living cells produce ROS as a normal metabolic byproduct. Under excessive stress, the cells generate excess ROS, so living organisms have evolved a series of response mechanisms to adapt to ROS exposure and use ROS as a signaling molecule. ROS molecules cause oxidative stress in a feedback mechanism involving numerous biological processes, including apoptosis, necrosis, and autophagy (He et al., 2017). Apoptosis is a normal process that occurs during development and aging as well as functions as a homeostatic mechanism to maintain cell populations in tissues. Apoptosis can even occur as a defense mechanism during immune responses or when cells are damaged by disease or toxins (Elmore, 2007). In this study, we investigated several gene markers that we selected through a whole-transcriptome search for differential expressions. We found differentially expressed genes related to apoptosis and immune responses to inflammation, such as FOXO3, TLR4, caspase-8, MMP-9, and p38 MAP kinase (p38) (Cuadrado and Nebreda, 2010; van der Vos and Coffer, 2011; Zheng et al., 2021). In addition, we investigated the effects of DDK-401 and DDK-204 without any stimulation (UV-B irradiation) in HaCaT cells to observe whether our samples could trigger inappropriate apoptosis or inflammation under normal conditions showed in (Figure 7). The RT-PCR analysis (Supplementary Figure S3) showed that in HaCaT cells, neither compound regulated FOXO3, TLR4, MMP-9, or p38 expression, indicating that DDK-401 did not trigger inappropriate apoptosis or inflammation under normal conditions. The ability to control cellular living or death has enormous therapeutic potential. However, upregulation of caspase-8 was observed, which is similar to the RNA-seq data indicating differential expression (Figure 4). Although the activation of caspase-8 is mainly associated with death receptor signaling cascades, it is also activated downstream of the mitochondria. The roles of caspase-8 in the shift from autophagy to apoptosis in cisplatin-resistant MCF7 cells and in TRAIL-mediated autophagy in HCT 116 cells have already been studied (de Vries et al., 2006; Hou et al., 2010). In the lung cancer A549 cells, DDK-401 treatment downregulated the expressions of MMP9 and TLR4 while upregulating the expressions of p38 and caspase-8 genes, compared with the cells treated with DDK-204. The MMPs are a group of zinc-dependent metalloenzymes that regulate various cellular processes, including tumor cell proliferation and metastasis (Guo et al., 2019). Several studies have noted that MMPs are overexpressed in malignant tissues than the adjacent normal tissues in a range of tumors, including lung, colon, breast, and pancreatic carcinomas (Radad et al., 2011; Nakhjavani et al., 2019). Previous research has shown that downregulating the expressions of intracellular MMP-9 can increase invasion and metastasis processes several cancers (Zhang et al., 2017, Liu et al., 2019). Furthermore, oxidative stress can induce receptor-dependent apoptosis and damage the mitochondria of normal cells. Mitochondrial dysfunction then further increases ROS accumulation and activates the p38 MAPK pathway. ROS can continuously activate p38 MAPK by activating MAPK kinase and inhibiting MAPK phosphatase. In A549 cells, ROS can regulate the expressions of Bax and Bcl-2 by activating p38 MAPK, which increases the level of cytochrome c in the cytoplasm and triggers the caspase cascade reaction leading to apoptosis (Jin et al., 2019; Nguyen and Nguyen, 2019). The caspases are a family of cysteine-containing proteolytic enzymes that play a central role in the execution phase of cell apoptosis. It has been reported that the effects of the caspase 8 pathway on cancer cells involve inducing apoptosis (Huang et al., 2019). The apoptosis induced by most anticancer drugs occur by the activation of caspases (González-Burgos et al., 2015; Kim and Kim, 2018). Caspase-8 is important in the death receptor-mediated extrinsic pathway, and DDK-401 promotes the activation of caspase-8 in A549 cells in this study, showing that it can cause apoptosis by activating the extrinsic caspase pathway (Wu et al., 2019; Yi, 2019). TLR4 is an important member of the type I transmembrane protein family. Recently, growing evidence has shown TLR4 in various tumors (Gao et al., 2017; Li and Ji, 2018; Zhou et al., 2019), including head and neck, lung, gastrointestinal, liver, pancreatic, skin, breast, ovarian, cervical, and prostate cancers. TLR4-mediated cancer growth is involved in breast tumor progression, and the downregulation of TLR4 prevented breast cancer progression and improved survival (Zhou et al., 2019c). According to our findings, DDK-401 could induce cell apoptosis by upregulating and downregulating various transcriptional factors under cancerous conditions.
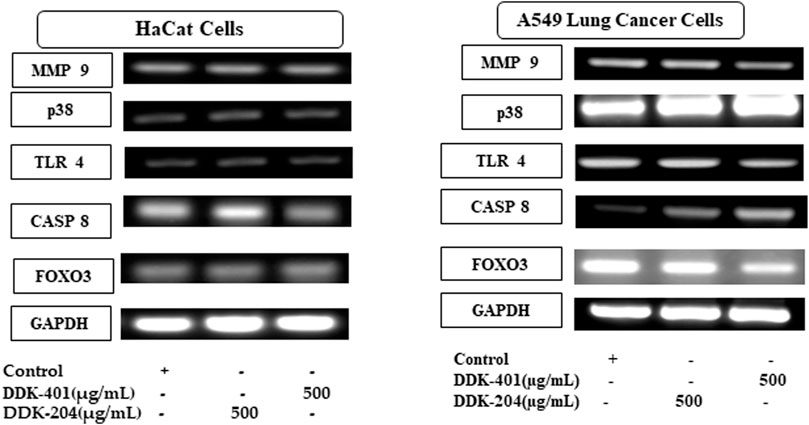
FIGURE 7. In vitro validation of the selected candidate gene expressions in normal (HaCaT) and lung cancer (A549) cell lines by RT-PCR.
Conclusion
Although ginseng and ginsenosides have been reported to have various pharmacological effects, the uptake of ginsenosides into systemic circulation, which is required for their effectiveness, depends on individual factors. Because CK was reported to be a minor saponin that reached systemic circulation, we enriched the total ginsenoside and CK content by fermenting red ginseng extract (DDK-401) via bioconversion and fermentation by edible enzymes. Because clinical trials are a prompt option for evaluating product efficacy, we evaluated DDK-401 in a clinical trial of healthy Korean volunteers. We found higher CK in blood plasma after oral intake of DDK-401 than after the consumption of the control red ginseng formula. Moreover, we identified differences in the CK absorption patterns between female and male volunteers, with higher concentrations of CK being detected in females than in males. We also observed differential expression patterns of various tumor suppressor genes between the female and male groups through RNA-seq analysis. DDK-401 exhibited no cytotoxicity in normal non-diseased HaCaT and RAW 264.7 cells, whereas it showed cytotoxicity in lung cancer cells (A549). Furthermore, DDK-401 inhibited H202-induced ROS production in HaCaT cells and increased ROS production in cancer cells. Finally, the candidate genes responsible for apoptosis and inflammation were validated using RT-PCR (Figure 7). This is a pilot study reporting that fermented red ginseng extract (DDK-401) produces unique, differential absorption and gene regulation patterns compared with red ginseng extract (DDK-204). Thus, DDK-401 could be a potential candidate for further investigations in clinical trials for health promoting activities and anticancer agents; various nanoformulations could also be considered to boost its bioavailability and anticancer properties.
Data availability statement
The datasets presented in this study can be found in online repositories. The names of the repository/repositories and accession number(s) can be found below: ncbi.nlm.nih.gov, PRJNA873242.
Ethics statement
The study was approved by the Institutional Review Board of Kyung Hee University Hospital (KHGIRB-21-419). The patients/participants provided their written informed consent to participate in this study.
Author contributions
Conceptualization, S-KC and C-SL; methodology, S-KC, C-SL, and BK; software, JN, DUY, and VB; validation, DCY, SK, BK, S-KC, C-SL, and D-WL; formal analysis, RM, BK, and ZR; resources, S-KC, C-SL, BK, SK, and DCY; data curation, JA, RM, and VB; writing—original draft preparation, JN, ZR, and JA; writing—review and editing, JA, JN, ZR, S-KC, C-SL, and DUY; supervision, HP, DCY, and SK; project administration, BK, D-WL, S-KC, C-SL, DUY, and SK; funding acquisition, DCY, SK, and BK. All authors have read and agreed to the published version of the manuscript.
Funding
This work was supported by the Korea Institute of Planning and Evaluation for Technology in Food, Agriculture and Forestry (IPET) through the Agri-Food Export Business Model Development Program funded by the Ministry of Agriculture, Food and Rural Affairs (MAFRA) (Project no: 320104-03). This research was also supported by the Basic Science Research Program through the National Research Foundation of Korea (NRF) funded by the Ministry of Education (grant no: NRF-2020R1I1A1A01070867) and Daedong Korea Ginseng Co., Ltd., South Korea.
Acknowledgments
The samples was provided by Daedong Korea Ginseng Co., Ltd., South Korea.
Conflict of interest
D-WL is employed by Hanbangbio Inc. and S-KC is employed by Daedong Korea Ginseng Co., Ltd.
The remaining authors declare that the research was conducted in the absence of any commercial or financial relationships that could be construed as a potential conflict of interest.
Publisher’s note
All claims expressed in this article are solely those of the authors and do not necessarily represent those of their affiliated organizations, or those of the publisher, editors, and reviewers. Any product that may be evaluated in this article or claim that may be made by its manufacturer is not guaranteed or endorsed by the publisher.
Supplementary material
The Supplementary Material for this article can be found online at: https://www.frontiersin.org/articles/10.3389/fphar.2022.999192/full#supplementary-material
References
Akter, R., Kwak, G.-Y., Ahn, J. C., Mathiyalagan, R., Ramadhania, Z. M., Yang, D. C., et al. (2021). Protective effect and potential antioxidant role of kakadu plum extracts on alcohol-induced oxidative damage in HepG2 cells. Appl. Sci. 12, 236. doi:10.3390/app12010236
Aryal, S., Baniya, M. K., Danekhu, K., Kunwar, P., Gurung, R., and Koirala, N. (2019). Total phenolic content, flavonoid content and antioxidant potential of wild vegetables from Western Nepal. Plants 8, 96. doi:10.3390/plants8040096
Azad, M. B., Chen, Y., and Gibson, S. B. (2008). Regulation of autophagy by reactive oxygen species (ROS): Implications for cancer progression and treatment. Antioxid. Redox Signal. 11, 777–790. doi:10.1089/ars.2008.2270
Baik, I.-H., Kim, K.-H., and Lee, K. (2021). Antioxidant, anti-inflammatory and antithrombotic effects of ginsenoside compound K enriched extract derived from ginseng sprouts. Molecules 26, 4102. doi:10.3390/molecules26134102
Ban, M. S., Kim, Y., Lee, S., Han, B., Yu, K.-S., Jang, I.-J., et al. (2021). Pharmacokinetics of ginsenoside compound K from a compound K fermentation product, CK-30, and from red ginseng extract in healthy Korean subjects. Clin. Pharmacol. Drug Dev. 10, 1358–1364. doi:10.1002/cpdd.949
Bausch-Fluck, D., Goldmann, U., Müller, S., Oostrum, M. V., Müller, M., Schubert, O. T., et al. (2018). The in silico human surfaceome. Proc. Natl. Acad. Sci. U. S. A. 115, E10988-E10997–E10997. doi:10.1073/pnas.1808790115
Bolger, A. M., Lohse, M., and Usadel, B. (2014). Trimmomatic: A flexible trimmer for illumina sequence data. Bioinformatics 30, 2114–2120. doi:10.1093/bioinformatics/btu170
Boopathi, V., Subramaniyam, S., Mathiyalagan, R., and Yang, D.-C. (2020). Till 2018: A survey of biomolecular sequences in genus Panax. J. Ginseng Res. 44, 33–43. doi:10.1016/j.jgr.2019.06.004
Borman, N., and Kim, C.-H. J. Y. M. J. (1966). The history of ancient Korean medicine. Yonsei Med. J. 7, 103–118. doi:10.3349/ymj.1966.7.1.103
Brunelle, J., and Chandel, N. J. A. (2002). Oxygen deprivation induced cell death: An update. Apoptosis 7, 475–482. doi:10.1023/a:1020668923852
Chen, L., Zhou, L., Wang, Y., Yang, G., Huang, J., Tan, Z., et al. (2017). Food and sex-related impacts on the pharmacokinetics of a single-dose of ginsenoside compound K in healthy subjects. Front. Pharmacol. 8, 636. doi:10.3389/fphar.2017.00636
Chen, Q., Kang, J., and Fu, C. (2018). The independence of and associations among apoptosis, autophagy, and necrosis. Signal Transduct. Target. Ther. 3, 18. doi:10.1038/s41392-018-0018-5
Choi, I.-D., Ryu, J.-H., Lee, D.-E., Lee, M.-H., Shim, J.-J., Ahn, Y.-T., et al. (2016). Enhanced absorption study of ginsenoside compound K (20-O-β-(D-Glucopyranosyl)-20(S)-protopanaxadiol) after oral administration of fermented red ginseng extract (HYFRG™) in healthy Korean volunteers and rats. Evid. Based. Complement. Altern. Med. 2016, 3908142. doi:10.1155/2016/3908142
Choi, J. H., Jang, M., Nah, S.-Y., Oh, S., and Cho, I.-H. J. J. O. G. R. (2018). Multitarget effects of Korean red ginseng in animal model of Parkinson's disease: Antiapoptosis, antioxidant, antiinflammation, and maintenance of blood–brain barrier integrity. J. Ginseng Res. 42, 379–388. doi:10.1016/j.jgr.2018.01.002
Choi, K., Kim, M., Ryu, J., and Choi, C. (2007). Ginsenosides compound K and Rh(2) inhibit tumor necrosis factor-alpha-induced activation of the NF-kappaB and JNK pathways in human astroglial cells. Neurosci. Lett. 421, 37–41. doi:10.1016/j.neulet.2007.05.017
Christensen, L. P. (2008). Chapter 1 ginsenosides: Chemistry, biosynthesis, analysis, and potential health effects, Advances in food and nutrition research. Cambridge, MA, USA, Academic Press.
Cuadrado, A., and Nebreda, A. R. (2010). Mechanisms and functions of p38 MAPK signalling. Biochem. J. 429, 403–417. doi:10.1042/BJ20100323
De Vries, J. F., Wammes, L. J., Jedema, I., Van Dreunen, L., Nijmeijer, B. A., Heemskerk, M. H. M., et al. (2006). Involvement of caspase-8 in chemotherapy-induced apoptosis of patient derived leukemia cell lines independent of the death receptor pathway and downstream from mitochondria. Apoptosis 12, 181–193. doi:10.1007/s10495-006-0526-6
Dharmananda, S. (2002). The nature of ginseng: Traditional use, modern research, and the question of dosage. HerbalGram 1, 17.
Elmore, S. (2007). Apoptosis: A review of programmed cell death. Toxicol. Pathol. 35, 495–516. doi:10.1080/01926230701320337
Fan, Y. J., and Zong, W. X. (2013). The cellular decision between apoptosis and autophagy. Chin. J. Cancer 32, 121–129. doi:10.5732/cjc.012.10106
Fukami, H., Ueda, T., and Matsuoka, N. (2018). Pharmacokinetic study of compound K in Japanese subjects after ingestion of Panax ginseng fermented by Lactobacillus paracasei A221 reveals significant increase of absorption into blood. J. Med. Food 22, 257–263. doi:10.1089/jmf.2018.4271
Gao, Y., Chu, S., Zhang, Z., and Chen, N. (2017). Hepataprotective effects of ginsenoside Rg1 – a review. J. Ethnopharmacol. 206, 178–183. doi:10.1016/j.jep.2017.04.012
González-Burgos, E., Fernandez-Moriano, C., and Gómez-Serranillos, M. P. (2015). Potential neuroprotective activity of ginseng in Parkinson’s disease: A review. J. Neuroimmune Pharmacol. 10, 14–29. doi:10.1007/s11481-014-9569-6
Grondin, C. J., Davis, A. P., Wiegers, J. A., Wiegers, T. C., Sciaky, D., Johnson, R. J., et al. (2021). Predicting molecular mechanisms, pathways, and health outcomes induced by Juul e-cigarette aerosol chemicals using the Comparative Toxicogenomics Database. Curr. Res. Toxicol. 2, 272–281. doi:10.1016/j.crtox.2021.08.001
Guo, Y.-H., Kuruganti, R., and Gao, Y. (2019). Recent advances in ginsenosides as potential therapeutics against breast cancer. Curr. Top. Med. Chem. 19, 2334–2347. doi:10.2174/1568026619666191018100848
He, L., He, T., Farrar, S., Ji, L., Liu, T., and Ma, X. (2017). Antioxidants maintain cellular redox homeostasis by elimination of reactive oxygen species. Cell. Physiol. biochem. 44, 532–553. doi:10.1159/000485089
Hou, W., Han, J., Lu, C., Goldstein, L. A., and Rabinowich, H. (2010). Autophagic degradation of active caspase-8: A crosstalk mechanism between autophagy and apoptosis. Autophagy 6, 891–900. doi:10.4161/auto.6.7.13038
Hu, S. Y. (1976). The genusPanax (Ginseng) in Chinese medicine. Econ. Bot. 30, 11–28. doi:10.1007/bf02866780
Huang, X., Li, N., Pu, Y., Zhang, T., and Wang, B. (2019). Neuroprotective effects of ginseng phytochemicals: Recent perspectives. Molecules 24, E2939. doi:10.3390/molecules24162939
Jin, Y., Cui, R., Zhao, L., Fan, J., and Li, B. (2019). Mechanisms of Panax ginseng action as an antidepressant. Cell Prolif. 52, e12696. doi:10.1111/cpr.12696
Jin, Y., Kim, Y.-J., Jeon, J.-N., Wang, C., Min, J.-W., Noh, H.-Y., et al. (2015). Effect of white, red and black ginseng on physicochemical properties and ginsenosides. Plant Foods Hum. Nutr. 70, 141–145. doi:10.1007/s11130-015-0470-0
Jung, J. H., Kang, I. G., Kim, D. Y., Hwang, Y. J., and Kim, S. T. J. J. O. G. R. (2013). The effect of Korean red ginseng on allergic inflammation in a murine model of allergic rhinitis. J. Ginseng Res. 37, 167–175. doi:10.5142/jgr.2013.37.167
Jung, J., Jang, H. J., Eom, S. J., Choi, N. S., Lee, N.-K., and Paik, H.-D. (2019). Fermentation of red ginseng extract by the probiotic Lactobacillus plantarum KCCM 11613P: Ginsenoside conversion and antioxidant effects. J. Ginseng Res. 43, 20–26. doi:10.1016/j.jgr.2017.07.004
Kachur, K., and Suntres, Z. E. (2016). The antimicrobial properties of ginseng and ginseng extracts. Expert Rev. anti. Infect. Ther. 14, 81–94. doi:10.1586/14787210.2016.1118345
Kang, S., and Min, H. (2012). Ginseng, the 'immunity boost': The effects of Panax ginseng on immune system. J. Ginseng Res. 36, 354–368. doi:10.5142/jgr.2012.36.4.354
Kim, E. H., and Kim, W. (2018). An insight into ginsenoside metabolite compound K as a potential tool for skin disorder. Evid. Based. Complement. Altern. Med. 2018, 8075870. eCAM. doi:10.1155/2018/8075870
Kim, H. K. (2013). Pharmacokinetics of ginsenoside Rb1 and its metabolite compound K after oral administration of Korean Red Ginseng extract. J. Ginseng Res. 37, 451–456. doi:10.5142/jgr.2013.37.451
Lee, H.-S., Kim, M.-R., Park, Y., Park, H. J., Chang, U. J., Kim, S. Y., et al. (2012). Fermenting red ginseng enhances its safety and efficacy as a novel skin care anti-aging ingredient: In vitro and animal study. J. Med. Food 15, 1015–1023. doi:10.1089/jmf.2012.2187
Lee, S. M., Bae, B.-S., Park, H.-W., Ahn, N.-G., Cho, B.-G., Cho, Y.-L., et al. (2015). Characterization of Korean red ginseng (Panax ginseng meyer): History, preparation method, and chemical composition. J. Ginseng Res. 39, 384–391. doi:10.1016/j.jgr.2015.04.009
Li, Z., and Ji, G. E. (2018). Ginseng and obesity. J. Ginseng Res. 42, 1–8. doi:10.1016/j.jgr.2016.12.005
Liu, H., Zhang, W., Zou, B., Wang, J., Deng, Y., and Deng, L. (2020). DrugCombDB: A comprehensive database of drug combinations toward the discovery of combinatorial therapy. Nucleic Acids Res. 48, D871-D881–D881. doi:10.1093/nar/gkz1007
Liu, L., Anderson, G. A., Fernandez, T. G., and Doré, S. (2019). Efficacy and mechanism of Panax ginseng in experimental stroke. Front. Neurosci. 13, 294. doi:10.3389/fnins.2019.00294
Liu, Z.-Q. (2012). Chemical insights into ginseng as a resource for natural antioxidants. Chem. Rev. 112, 3329–3355. doi:10.1021/cr100174k
Liu, Z., Ren, Z., Zhang, J., Chuang, C.-C., Kandaswamy, E., Zhou, T., et al. (2018). Role of ROS and nutritional antioxidants in human diseases. Front. Physiol. 9, 477. doi:10.3389/fphys.2018.00477
Majeed, F., Malik, F. Z., Ahmed, Z., Afreen, A., Afzal, M. N., and Khalid, N. (2018). Ginseng phytochemicals as therapeutics in oncology: Recent perspectives. Biomed. Pharmacother. 100, 52–63. doi:10.1016/j.biopha.2018.01.155
Mathiyalagan, R., Wang, C., Kim, Y. J., Castro-Aceituno, V., Ahn, S., Subramaniyam, S., et al. (2019). Preparation of polyethylene glycol-ginsenoside Rh1 and Rh2 conjugates and their efficacy against lung cancer and inflammation. Molecules 24, 4367. doi:10.3390/molecules24234367
Mccarthy, D. J., Chen, Y., and Smyth, G. K. (2012). Differential expression analysis of multifactor RNA-Seq experiments with respect to biological variation. Nucleic Acids Res. 40, 4288–4297. doi:10.1093/nar/gks042
Mohanan, P., Subramaniyam, S., Mathiyalagan, R., and Yang, D.-C. (2018). Molecular signaling of ginsenosides Rb1, Rg1, and Rg3 and their mode of actions. J. Ginseng Res. 42, 123–132. doi:10.1016/j.jgr.2017.01.008
Murugesan, M., Mathiyalagan, R., Boopathi, V., Kong, B. M., Choi, S.-K., Lee, C.-S., et al. (2022). Production of minor ginsenoside CK from major ginsenosides by biotransformation and its advances in targeted delivery to tumor tissues using nanoformulations. Nanomaterials 12, 3427. doi:10.3390/nano12193427
Nakhjavani, M., Hardingham, E. J., Palethorpe, M. H., Tomita, Y., Smith, E., Price, J. T., et al. (2019). Ginsenoside Rg3: Potential molecular targets and therapeutic indication in metastatic breast cancer. Medicines 6, E17. doi:10.3390/medicines6010017
Nguyen, N. H., and Nguyen, C. T. (2019). Pharmacological effects of ginseng on infectious diseases. Inflammopharmacology 27, 871–883. doi:10.1007/s10787-019-00630-4
Panossian, A., Abdelfatah, S., and Efferth, T. (2021). Network Pharmacology of ginseng (Part II): The differential effects of red ginseng and ginsenoside Rg5 in cancer and heart diseases as determined by transcriptomics. Pharmaceuticals 14, 1010. doi:10.3390/ph14101010
Park, J.-S., Shin, J. A., Jung, J.-S., Hyun, J.-W., Van Le, T. K., Kim, D.-H., et al. (2012). Anti-inflammatory mechanism of compound K in activated microglia and its neuroprotective effect on experimental stroke in mice. J. Pharmacol. Exp. Ther. 341, 59–67. doi:10.1124/jpet.111.189035
Park, S. K., Hyun, S. H., In, G., Park, C.-K., Kwak, Y.-S., Jang, Y.-J., et al. (2021). The antioxidant activities of Korean red ginseng (Panax ginseng) and ginsenosides: A systemic review through in vivo and clinical trials. J. Ginseng Res. 45, 41–47. doi:10.1016/j.jgr.2020.09.006
Patro, R., Duggal, G., Love, M. I., Irizarry, R. A., and Kingsford, C. (2017). Salmon provides fast and bias-aware quantification of transcript expression. Nat. Methods 14, 417–419. doi:10.1038/nmeth.4197
Piao, X. M., Huo, Y., Kang, J. P., Mathiyalagan, R., Zhang, H., Yang, D. U., et al. (2020). Diversity of ginsenoside profiles produced by various processing Technologies. Molecules 25, E4390. doi:10.3390/molecules25194390
Posadzki, P., Watson, L. K., and Ernst, E. (2013). Adverse effects of herbal medicines: An overview of systematic reviews. Clin. Med. 13, 7–12. doi:10.7861/clinmedicine.13-1-7
Pu, J. Y., Ramadhania, Z. M., Mathiyalagan, R., Huo, Y., Han, Y., Li, J. F., et al. (2021). Ginsenosides conversion and anti-oxidant activities in puffed cultured roots of mountain ginseng. Processes 9, 2271. doi:10.3390/pr9122271
Radad, K., Moldzio, R., and Rausch, W.-D. (2011). Ginsenosides and their CNS targets. CNS Neurosci. Ther. 17, 761–768. doi:10.1111/j.1755-5949.2010.00208.x
Ramadhania, Z. M., Nahar, J., Ahn, J. C., Yang, D. U., Kim, J. H., Lee, D. W., et al. (2022). Terminalia ferdinandiana (kakadu plum)-mediated bio-synthesized ZnO nanoparticles for enhancement of anti-lung cancer and anti-inflammatory activities. Appl. Sci. 12, 3081. doi:10.3390/app12063081
Riaz, M., Rahman, N. U., Zia-Ul-Haq, M., Jaffar, H. Z. E., and Manea, R. (2019). Ginseng: A dietary supplement as immune-modulator in various diseases. Trends Food Sci. Technol. 83, 12–30. doi:10.1016/j.tifs.2018.11.008
Sharma, A., and Lee, H.-J. (2020). Ginsenoside compound K: Insights into recent studies on pharmacokinetics and health-promoting activities. Biomolecules 10, E1028. doi:10.3390/biom10071028
Shin, J. H., Park, Y. J., Kim, W., Kim, D. O., Kim, B. Y., Lee, H., et al. (2019). Change of ginsenoside profiles in processed ginseng by drying, steaming, and puffing. J. Microbiol. Biotechnol. 29, 222–229. doi:10.4014/jmb.1809.09056
Shin, Y., Subramaniyam, S., Chun, J.-M., Jeon, J.-H., Hong, J.-M., Jung, H., et al. (2021). Genome-wide differential methylation profiles from two terpene-rich medicinal plant extracts administered in osteoarthritis rats. Plants 10, 1132. doi:10.3390/plants10061132
Sohn, S.-H., Kim, S.-K., Kim, Y.-O., Kim, H.-D., Shin, Y.-S., Yang, S.-O., et al. (2013). A comparison of antioxidant activity of Korean White and Red Ginsengs on H 2 O 2-induced oxidative stress in HepG2 hepatoma cells. J. Ginseng Res. 37, 442–450. doi:10.5142/jgr.2013.37.442
Subbiah, V., Zhong, B., Nawaz, M. A., Barrow, C. J., Dunshea, F. R., and Suleria, H. A. (2020). Screening of phenolic compounds in Australian grown berries by lc-esi-qtof-ms/ms and determination of their antioxidant potential. Antioxidants 10, 26. doi:10.3390/antiox10010026
Szklarczyk, D., Santos, A., Von Mering, C., Jensen, L. J., Bork, P., and Kuhn, M. (2016). Stitch 5: Augmenting protein-chemical interaction networks with tissue and affinity data. Nucleic Acids Res. 44, D380–D384. doi:10.1093/nar/gkv1277
Thorburn, A. (2008). Apoptosis and autophagy: Regulatory connections between two supposedly different processes. Apoptosis 13, 1–9. doi:10.1007/s10495-007-0154-9
Uhlén, M., Fagerberg, L., Hallström, B. M., Lindskog, C., Oksvold, P., Mardinoglu, A., et al. (2015). Proteomics. Tissue-based map of the human proteome. Science 347, 1260419. doi:10.1126/science.1260419
Van Der Vos, K. E., and Coffer, P. J. (2011). The extending network of FOXO transcriptional target genes. Antioxid. Redox Signal. 14, 579–592. doi:10.1089/ars.2010.3419
Warinhomhoun, S., Muangnoi, C., Buranasudja, V., Mekboonsonglarp, W., Rojsitthisak, P., Likhitwitayawuid, K., et al. (2021). Antioxidant activities and protective effects of dendropachol, a new bisbibenzyl compound from Dendrobium pachyglossum, on hydrogen peroxide-induced oxidative stress in HaCaT keratinocytes. Antioxidants 10, 252. doi:10.3390/antiox10020252
Wen, Z., He, J., Tao, H., and Huang, S. Y. (2019). PepBDB: A comprehensive structural database of biological peptide-protein interactions. Bioinformatics 35, 175–177. doi:10.1093/bioinformatics/bty579
Wishart, D. S., Feunang, Y. D., Guo, A. C., Lo, E. J., Marcu, A., Grant, J. R., et al. (2018). DrugBank 5.0: A major update to the DrugBank database for 2018. Nucleic Acids Res. 46, D1074-D1082–d1082. doi:10.1093/nar/gkx1037
Wu, T., Kwaku, O. R., Li, H.-Z., Yang, C.-R., Ge, L.-J., and Xu, M. (2019). Sense ginsenosides from ginsengs: Structure-activity relationship in autophagy. Nat. Product. Commun. 14, 1934578X1985822. doi:10.1177/1934578x19858223
Yi, Y.-S. (2019). Roles of ginsenosides in inflammasome activation. J. Ginseng Res. 43, 172–178. doi:10.1016/j.jgr.2017.11.005
Yu, J. S., Roh, H.-S., Baek, K.-H., Lee, S., Kim, S., So, H. M., et al. (2018). Bioactivity-guided isolation of ginsenosides from Korean Red Ginseng with cytotoxic activity against human lung adenocarcinoma cells. J. Ginseng Res. 42, 562–570. doi:10.1016/j.jgr.2018.02.004
Yu-Hang, G., Revathimadhubala, K., and Ying, G. (2019). Recent advances in ginsenosides as potential therapeutics against breast cancer. Curr. Top. Med. Chem. 19, 2334–2347. doi:10.2174/1568026619666191018100848
Zhang, H., Wang, D., Ru, W., Qin, Y., and Zhou, X. (2017). “13 - an overview on ginseng and energy metabolism,” in Sustained energy for enhanced human functions and activity. Editor D. BAGCHI (Academic Press).
Zhang, J., Wang, W., and Mao, X. (2020). Chitopentaose protects HaCaT cells against H2O2-induced oxidative damage through modulating MAPKs and Nrf2/ARE signaling pathways. J. Funct. Foods 72, 104086. doi:10.1016/j.jff.2020.104086
Zhao, M., Kim, P., Mitra, R., Zhao, J., and Zhao, Z. (2016). TSGene 2.0: An updated literature-based knowledgebase for tumor suppressor genes. Nucleic Acids Res. 44, D1023–D1031. doi:10.1093/nar/gkv1268
Zheng, S.-D., Wu, H.-J., and Wu, D.-L. (2012). Roles and mechanisms of ginseng in protecting heart. Chin. J. Integr. Med. 18, 548–555. doi:10.1007/s11655-012-1148-1
Zheng, Z., Xiao, Z., He, Y.-L., Tang, Y., Li, L., Zhou, C., et al. (2021). Heptapeptide isolated from isochrysiszhanjiangensis exhibited anti-photoaging potential via MAPK/AP-1/MMP pathway and anti-apoptosis in UVB-irradiated HaCaT cells. Mar. Drugs 19, 626. doi:10.3390/md19110626
Zhou, P., Xie, W., He, S., Sun, Y., Meng, X., Sun, G., et al. (2019). Ginsenoside Rb1 as an anti-diabetic agent and its underlying mechanism analysis. Cells 8, 204. doi:10.3390/cells8030204
Zhou, P., Xie, W., Sun, Y., Dai, Z., Li, G., Sun, G., et al. (2019c). Corrigendum to "ginsenoside Rb1 and mitochondria: A short review of the literature". Mol. Cell. Probes 43, 1–5. doi:10.1016/j.mcp.2020.101626
Keywords: fermented red ginseng, compound K (CK), pharmacokinetics, clinical trial, mRNA sequence, antioxidant, cancer, inflammation
Citation: Ahn JC, Mathiyalagan R, Nahar J, Ramadhania ZM, Kong BM, Lee D-W, Choi SK, Lee CS, Boopathi V, Yang DU, Kim BY, Park H, Yang DC and Kang SC (2022) Transcriptome expression profile of compound-K-enriched red ginseng extract (DDK-401) in Korean volunteers and its apoptotic properties. Front. Pharmacol. 13:999192. doi: 10.3389/fphar.2022.999192
Received: 20 July 2022; Accepted: 31 October 2022;
Published: 01 December 2022.
Edited by:
Guangbo Fu, Huaian No.1 People’s Hospital Nanjing Medical University, ChinaReviewed by:
Dong Bai, Institute of Basic Theory for Chinese Medicine, China Academy of Chinese Medical Science, ChinaNailiang Zhu, Xinyang Agriculture and Forestry University, China
Copyright © 2022 Ahn, Mathiyalagan, Nahar, Ramadhania, Kong, Lee, Choi, Lee, Boopathi, Yang, Kim, Park, Yang and Kang. This is an open-access article distributed under the terms of the Creative Commons Attribution License (CC BY). The use, distribution or reproduction in other forums is permitted, provided the original author(s) and the copyright owner(s) are credited and that the original publication in this journal is cited, in accordance with accepted academic practice. No use, distribution or reproduction is permitted which does not comply with these terms.
*Correspondence: Deok Chun Yang, ZGN5YW5nQGtodS5hYy5rcg==; Se Chan Kang, c2NrYW5nQGtodS5hYy5rcg==
†These authors have contributed equally to this work and share first authorship