- 1College of Veterinary Medicine, Hunan Agricultural University, Changsha, China
- 2Key Laboratory of Chinese Veterinary Medicine in Hunan Province, Hunan Agricultural University, Changsha, China
- 3College of Veterinary Medicine, China Agricultural University, Beijing, China
- 4College of Veterinary Medicine, Shanxi Agricultural University, Jinzhong, China
- 5Hunan MICOLTA Biological Resources Co., Ltd., Changsha, China
Macleaya cordata extract (MCE) is widely used for its diverse pharmacological actions and beneficial effects on farm animals. Modern pharmacological studies have shown that it has anti-inflammatory, anti-cancer, and anti-bacterial activities, and is gradually becoming a long-term additive veterinary drug used to improve animal intestinal health and growth performance. Although some evidence points to the DNA mutagenic potential of sanguinarine (SAN), a major component of MCE, there is a lack of sufficient basic toxicological information on the oral route, posing a potential safety risk for human consumption of food of animal origin. In this study, we assessed the acute oral toxicity, repeated 90-day oral toxicity and 180-day chronic toxicity of MCE in rats and mice and re-evaluated the genotoxicity of MCE using a standard combined in vivo and ex vivo assay. In the oral acute toxicity test, the LD50 for MCE in rats and mice was 1,564.55 mg/kg (95% confidence interval 1,386.97–1,764.95 mg/kg) and 1,024.33 mg/kg (95% confidence interval 964.27–1,087.30 mg/kg), respectively. The dose range tested had no significant effect on hematology, clinical chemistry, and histopathological findings in rodents in the long-term toxicity assessment. The results of the bacterial reverse mutation, sperm abnormality and micronucleus test showed negative results and lack of mutagenicity and teratogenicity; the results of the rat teratogenicity test showed no significant reproductive or embryotoxicity. The results indicate that MCE was safe in the dose range tested in this preclinical safety assessment. This study provides data to support the further development of maximum residue limits (MRLs) for MCE.
Introduction
The Papaveraceae Juss. has about 38 genera and 700 species worldwide, mainly in the north temperate zone, especially in the Mediterranean region, West Asia, Central Asia to East Asia and southwestern North America (Zhang et al., 2020). Some of these species are used as medicines, especially Corydalis yanhusuo W. T. Wang in the genus Corydalis (Liu et al., 2021; Wu et al., 2021). However, a variety of plants in this family have obvious toxicity, such as Papaver somniferum L., Macleaya cordata (Willd.) R. Br., and Chelidonium majus L. Symptoms of poisoning manifest as depression of the central nervous system, liver damage, circulatory disorders, and gastrointestinal irritation (Moro et al., 2009; Günaydın et al., 2015; Lin et al., 2018). Because of its severe toxicity for human when taken orally, as documented in Tang Dynasty medical texts, M. cordata (Willd.) R. Br. (M. cordata) has been restricted to external use in China (Chen and Shang, 2002).
A variety of isoquinoline alkaloids have been identified in M. cordata (e.g., sanguinarine, dihydrosanguinarine, chelerythrine, dihydrochelerythrine, 6-cyano-dihydrochelerythrine, protopine, allocryptopine, berberine, etc.) (Ye et al., 2009; Qing et al., 2014, Qing et al., 2016a, Qing et al., 2016b; Lin et al., 2020), phenolic acids (ferulic acid, eugenol, p-hydroxybenzoic acid, and p-coumaric acid) and sterols (stigmasterol), etc. (Zou et al., 2016). Among them, alkaloids have the greatest advantage in terms of biological activity (Cai et al., 2020; Liu et al., 2022b).
Sanguinarine (SAN) and chelerythrine (CHE) are the most abundant alkaloids in M. cordata, which are also distributed in other plants of the poppy family, such as Chelidonium majus L., Eomecon chionantha Hance, etc. (Warowicka et al., 2019; Lu et al., 2020). They have a wide range of pharmacological activities, such as anti-inflammatory (Danielewski et al., 2022), anti-cancer (Zhu et al., 2020; Prabhu et al., 2021), antibacterial (Falchi et al., 2021; Lu et al., 2022), antifungal (Anjago et al., 2021), anthelmintic (Li et al., 2022), and immune enhancing (Liu et al., 2022a), etc. The biggest application of M. cordata is in livestock production, where studies have shown that MCE can provide a slight bitter taste in feed to increase animal intake (Chin, 2008; Wang et al., 2018). The main active ingredient of M. cordata, SAN, was included in the Chinese feed additive species list in 1999 (Ministry of Agriculture Announcement No. 105) and classified as a flavoring agent and spice management; SAN was approved as a flavoring agent by the European Union in 2004 and is on the feed additive list (Council of the European Union and European Parliament, 2008). Meanwhile, the standardized MCE product Sangrovit® is approved for use in animal feeds as a plant-derived feed additive as an alternative to feeding growth-promoting antibiotics (AGPs) to promote animal growth and improve feed conversion (Juskiewicz et al., 2011). MCE is well tolerated in animals such as pigs, chickens, sheep and reindeer, and has been shown to significantly improve animal intestinal health, reduce oxidative stress damage, enhance innate immunity and disease resistance, and thus improve growth performance (Juskiewicz et al., 2011; Aguilar-Hernández et al., 2016; Chen et al., 2018; Chen et al., 2020; Bussabong et al., 2021). MCE does not induce the spread of antibiotic resistance genes and has a lower ecological risk (Zhang et al., 2021). In view of the obvious “antibiotic” nature of SAN, the 2008 edition of the “Feed Additive Species List” (Ministry of Agriculture Announcement No. 1126) issued by the Chinese Ministry of Agriculture and Rural Development removed SAN and transferred it to the veterinary medicine department for management. A growing body of research evidence demonstrating the benefits of MCE as a farm input for animal health and antibiotic resistance control has raised public and governmental concerns about the safety of MCE. Although some reports point out the risks associated with SAN, such as intraperitoneal injection of SAN can induce hepatotoxicity through oxidation of the protein thiol (Choy et al., 2008); In a zebrafish model, SAN can trigger cardiotoxicity by inducing extracellular Ca2+ influx and MAPK pathway (Hu et al., 2005; Wang et al., 2022); In addition, studies have shown the damaging effects of SAN on oocytes of mice fertilized in vitro and the increased embryonic resorption and reduced fetal weight after embryo transfer into the body (Chan, 2011, 2015). However, the concerns about toxicity of SAN were mainly attributed to the 1998 outbreak in New Delhi, India, of a disease known collectively as “epidemic dropsy” caused by contamination or adulteration of Argemone mexicana seed oil (AO) (Vaidya et al., 2001; Sharma et al., 2002). Numerous studies have shown evidence of a correlation between AO and genotoxicity (Ansari et al., 2006; Ghosh and Mukherjee, 2016). SAN is considered to be a major contributor to AO toxicity, and in vitro tests have shown that SAN can be embedded in DNA, and in vivo tests have shown the potential of SAN to damage DNA in blood and bone marrow cells in mice (Ansari et al., 2005). Zdarilova et al. (2008) evaluated the use of MCE as a feed additive in rats fed 600 mg per kg of feed for 90 days without any significant risk of organismal damage or genotoxicity (Stiborova et al., 2008). In addition, MCE was declared a veterinary drug for long-term use in China and its safety needs to be re-evaluated due to a change in use, with the EU removing MCE from the list of feed additives in May 2021 (Directorate-General for Health and Food Safety, 2021). Since the predominant route of exposure to exogenous compounds is oral, however, oral safety data for MCE are still vacant. In this study, we systematically evaluated the acute, long-term toxicity (repeated oral toxicity and chronic toxicity) of MCE in rodents. Given the risk of genotoxicity of SAN, we also conducted a series of standardized genetic toxicology tests, including the murine Salmonella revertant mutation (Ames) test, the mouse sperm aberration test, and the mouse bone marrow micronucleus test, to reassess the genotoxicity of MCE. In addition to this, a rat teratogenicity test was carried out to assess the embryotoxicity of MCE. Our findings provide data to support a comprehensive understanding of the potential risks of oral exposure to MCE.
Materials and methods
Test drugs and reagents
MCE, was provided by Hunan MICOLTA Biological Resources Co., Ltd. (Changsha, China). MCE is a standardized extract prepared by a series of processes, including heated sulfuric acid percolation, sodium hydroxide precipitation, filtration, drying, alcohol extraction, salt formation, and crushing. The total content of SAN (Figure 1A) and CHE (Figure 1B) in MCE is greater than 60%, with SAN ≥ 40% and CHE ≥ 20%, and the remaining trace components have been qualitatively characterized (Dong et al., 2021). The samples were kept at the Veterinary Drug Safety Supervision, Inspection and Testing Center of the Ministry of Agriculture and Rural Affairs, China Agricultural University (Beijing, China). Reagents for hematological and serum biochemical assays were purchased from Mindray Biomedical Electronics Co., Ltd. (Shenzhen, China). Other reagents were purchased from Sinopharm Chemical Reagent Co., Ltd. (Shanghai, China).
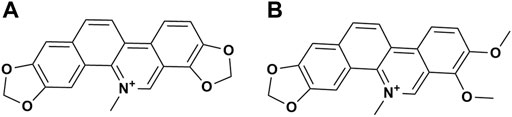
FIGURE 1. Chemical structure formula of the main compounds in MCE. (A) Sanguinarine; (B) Chelerythrine.
Animals
SPF-grade SD rats and ICR mice were purchased from Beijing Vital River Laboratory Animal Technology Co., Ltd. (Beijing, China) and housed in a China Ministry of Agriculture and Rural Affairs certified GLP animal rearing room at the Veterinary Drug Safety Supervision, Inspection and Testing Center, Ministry of Agriculture and Rural Affairs, China Agricultural University, with controlled environmental parameters Conditions were as follows: temperature 25 ± 2°C, relative humidity 50% ± 20%, 12-h light/dark cycle, and ventilation 8–15 times/hour. All animals had free access to maintenance diets (crude protein ≥18%, crude fat ≥4%, crude fiber ≤5%, Beijing Keao Xieli Feed Co., Ltd.) and reverse osmosis water. All animal experiments were approved by the Animal Ethics Committee of China Agricultural University (Experiment approval number: WTPJ20090001-1∼7) and strictly followed the Chinese Guide for Ethical Review of Laboratory Animal Welfare (GB/T 35892, 2018).
Oral acute toxicity in rats and mice
Sixty healthy SD rats (weighing 180–200 g) and ICR mice (18–22 g) were housed adaptively for 5 days. The oral acute toxicity test of MCE was carried out on rats and mice with reference to the half lethal dose method in the Compendium of Technical Guidelines for Veterinary Drug Research and the Guidelines for the acute toxicity (LD50 determination) of veterinary drugs (Ministry of Agriculture and Rural Affairs of PRC, 2009f; Veterinary Drug Assessment Centre of the Ministry of Agriculture of the and People’s Republic of China, 2012). The dose ranges were obtained from 2,500.00 to 879.20 mg/kg (rats) and 1750 to 573.44 mg/kg (mice) by pretesting, and the rats and mice were grouped separately (n = 10, half were males and the other half were females) using the complete randomization method, and the MCE was mixed in 1% CMCC-Na solution and transfused into rodents by oral gavage. The volume of the oral solution was 2 ml/100 g (rats) and 0.2 ml/100 g (mice) according to the protocol in Table 1. The rodents were observed for general behavior, signs of poisoning and mortality after poisoning.
Repeated dose 90-day oral toxicity test in rats
Repeated Dose 90-Day Oral Toxicity test was conducted with reference to the Guidelines for 30-Day and 90-Day Feeding Tests for Veterinary Drugs (Ministry of Agriculture and Rural Affairs of PRC, 2009a). SD rats of 80–100 g (n = 20/group, half males, and the other half females) were divided into four groups and pellets were made at 156.46 (1/10 LD50), 31.49 (1/50 LD50), 6.26 (1/250 LD50) and 0 mg/kg of MCE added to the feed and given by ad libitum feeding for 90 days. At the end of 90 days, all surviving rats were anesthetized, heart blood was collected and executed.
Chronic toxicity test in rats
The 180-day chronic toxicity test was conducted with reference to the Guidelines for Chronic Toxicity and Carcinogenicity Testing of Veterinary Drugs (Ministry of Agriculture and Rural Affairs of PRC, 2009c). Based on the results of 90-day subchronic toxicity, SD rats of 60–80 g (n = 50/group, half males, and the other half females) were divided into four groups and pellets were made at 78.23 (1/20 LD50), 15.65 (1/100 LD50), 3.13 (1/500 LD50) and 0 mg/kg of MCE added to the feed and given by ad libitum feeding for 180 days. At the end of 180 days, all surviving rats were anesthetized, heart blood was collected and executed.
Hematology and serum biochemical tests
Blood from rats taken via the heart was tested separately in 90-day and 180-day long-term toxicity tests. Whole blood stored in vacuum EDTA-K2 anticoagulation tubes was examined on a BC-2800 Vet Animal Blood Cell Analyzer (Mindray Biomedical Electronics Co., Ltd., Shenzhen, China) for hemoglobin (Hg), red blood cell count (RBC), white blood cell count (WBC), and platelet count (PLT). Blood preserved in ordinary vacuum blood collection tubes without additives is separated by centrifugation to obtain serum. The serum alanine aminotransferase (ALT), aspartate aminotransferase (AST), urea nitrogen (BUN), creatinine (CR), glucose (GLU), albumin (ALB), total protein (TP), and total cholesterol (TCH) were measured using a BS-180 automated biochemical analyzer (Mindray Biomedical Electronics Co., Ltd., Shenzhen, China).
Gross autopsy and histopathological examination
The major organs of rats anesthetized and executed in 90-day and 180-day long-term toxicity tests were removed from the surface blood and weighed after visual detection of tissue damage. All organs were preserved in 10% neutral buffered formalin solution for more than 3 days. The fixed tissues were washed with water, dehydrated in gradient ethanol, and treated with xylene before paraffin embedding. The prepared paraffin tissue block was sectioned and stained with hematoxylin & eosin (H&E) and then glued and stored. According to the requirements of veterinary drug declaration, if no toxicity-related histopathological changes are found, the relevant results are not required, so the histopathological picture results are not reflected in this report.
Salmonella typhimurium revert mutation (Ames) test
Ames tests are conducted in accordance with the Guidelines for Ames Tests of Veterinary Drugs (Ministry of Agriculture and Rural Affairs of PRC, 2009b). Four test strains, TA97, TA98, TA100, and TA102, were tested by spot test and plate incorporation method using 100, 20, 4, 0.8, and 0.16 μg/ml of MCE, respectively. Additional solvent control, DMSO and positive control groups were set up. Positive drugs were selected as follows: 2 mg/ml of 2-aminofluorene (2-AF) was used for TA97, TA98, TA100, and TA102 for the point test plus S9. Without S9, TA97, TA98, TA100, and TA102 were all treated with 1 mg/ml of diquat. In the plate incorporation test, TA97, TA98, TA100, and TA102 used 100 μg/ml of 2-AF when S9 was added; TA97 used 50 μg/ml of acriflavine; TA98 used 200 μg/ml of 2,7-diaminofluorene (2,7-AF); TA100 used 15 μg/ml of sodium azide (NaN3) when S9 was not added; TA102 used 5 μg/ml of mitomycin; and TA97, TA98, TA100, and TA102 used 5 μg/ml of NaN3. TA100 used 15 μg/ml of NaN3; TA102 used 5 μg/ml of mitomycin C. The test was repeated twice and the number of revertant colonies formed in each test strain plate was counted after 48 h of incubation at 37°C. The test strains were purchased from China Veterinary Culture Collection Centre and approved for use by the Biosafety Committee of China Agricultural University.
Mice bone marrow cell micronucleus test
Bone marrow cell micronucleus testing in mice according to the Guidelines for Bone Marrow Cell Micronucleus Testing in Veterinary Mice (Ministry of Agriculture and Rural Affairs of PRC, 2009g). ICR mice from 25 to 30 g were housed for 1 week for environmental adaptation. With reference to the results obtained for oral acute toxicity in mice, three MCE groups were set up in the test at concentrations of 512.17, 256.08, and 128.04 mg/kg, a positive control group (40 mg/kg, cyclophosphamide monohydrate, Reagent grade, 97.0%–103.0%, Beyotime Biotechnology Shanghai, China) and negative control group (distilled water, homemade) (n = 20, male and female). The drug was administered by gavage at 0.2 ml/10 g. The drug was administered twice with an interval of 24 h between doses. Mice were euthanized by cervical dislocation 6 h after the second administration, and bone marrow cells were harvested from mouse femurs using FBS, coated on slides, oven-dried, fixed in methanol, stained with Giemsa working solution (Beyotime Biotechnology, Shanghai, China) for 30 min, rinsed in distilled water, and air-dried for examination. The number of polychromatic erythrocytes (PCE), micronucleated polychromatic erythrocytes and mature erythrocytes (RBC) were counted by double-blind light microscopic examination. PCE micronucleus rate and PCE/RBC values were calculated for female and male mice in each test group, and the respective standard deviations were also calculated. The formula is as follows: PCE micronucleus rate = number of PCE containing micronucleus/number of PCE examinations × 100%; PCE/RBC ratio = number of PCE examinations/number of RBCs.
Mice sperm abnormality test
Sperm deformity test for mice was conducted according to the Guidelines for Sperm Deformity Test for Veterinary Mice (Ministry of Agriculture and Rural Affairs of PRC, 2009e). Three MCE dose groups of 204.87 (1/5 LD50), 102.43 (1/10 LD50), and 51.22 mg/kg (1/20 LD50), a negative control group (distilled water, homemade) and a positive control group (40 mg/kg, cyclophosphamide, Reagent grade, 97.0%–103.0%, Beyotime Biotechnology, Shanghai, China) (n = 10, males). The drug was administered by gavage at 0.2 ml/10 g once a day for 5 days at 24 h intervals. On the 35th day after the first poisoning, the mice were executed by cervical dislocation and the epididymis was removed from both sides. Sperm solution was obtained using saline, and synthetic fiber blood mesh bags were filtered, and the filtrate was aspirated to make sperm smears. After air-drying, the smears were fixed with methanol for 10 min, dried and stained with 2% eosin (Solarbio Science & Technology Co., Ltd., Beijing, China) for 1 h, lightly rinsed with water and dried. Normal and abnormal spermatozoa (hookless, banana-shaped, fat-headed, amorphous, tail-folded, double-headed, double-tailed, etc.) were examined and counted under the microscope in the same field of view.
Traditional teratogenicity test in rats
Referring to the Guidelines for Traditional Teratogenicity Tests on Veterinary Drugs in Rats with some modifications, we conducted a traditional teratogenicity test in rats (Ministry of Agriculture and Rural Affairs of PRC, 2009d) and made some modifications. Based on the results of oral acute toxicity tests in rats, 391.15 (1/4 LD50), 97.79 (1/16 LD50), and 24.45 mg/kg (1/64 LD50) and a negative control group (distilled water, homemade) were selected. Due to the instability of positive drug teratogenicity, a positive control group was not established for this test. The number of unmated young female rats in each group was 20 and the number of male rats was 10. Only female rats were used for gavage administration in the experiment, and males were used only for mating behavior. After the sexually mature male and female rats were caged together at 2:1, the females were checked daily to ensure that they had mated, and the mated females were used as the test rats, and the day of conception was taken as day zero. Repeated mating in the same cage was done until the number of rats detected that met the test conditions was sufficient for the test. The drug was administered from the 7th to the 16th day of conception, once/day for 10 consecutive days. The feeding, drinking and weight gain of pregnant rats were examined and recorded during the test period, and the general behavioral performance, poisoning and death of pregnant rats were observed. The pregnant rats were weighed on the 20th day of gestation, decapitated, and the ovaries and uterus were removed by dissection to check the number of corpus luteum, the number of absorbed fetuses, the number of stillbirths, the number of live fetuses, the male to female ratio of live fetuses, and the weight of ovaries and uterus. The live fetal rats were examined for weight, body length, tail length, and cosmetic abnormalities. Half of the live fetuses from each litter were fixed in 95% ethanol for 3 weeks, and the fixed fetuses were rinsed in water for several minutes and then placed in 2% potassium hydroxide solution for 72 h. The fetuses were then stained in alizarin red (sodium 3,4-dihydroxy anthraqinone-2-sulfonate) application solution (Beyotime Biotechnology, Shanghai, China) for 48 h and gently shaken twice a day until the skulls were stained red. The fetal rats were then removed and placed in transparent solution A (glycerol 20 ml, 2% potassium hydroxide solution 3 ml, add distilled water to 100 ml) for 2 days and in transparent solution B (glycerol 50 ml, 2% potassium hydroxide solution 3 ml, add distilled water to 100 ml) for 3 days, and the bones were left to stain red while the soft tissues were basically discolored. The stained fetal mouse specimens were observed under a stereomicroscope using a transmission light source, and then the skeleton was gradually examined for abnormalities. The other half of the fetal rats from each litter were placed in Bouin’s fluid (Solarbio Science & Technology Co., Ltd., Beijing, China) and fixed for 2 weeks for visceral examination.
Statistical analysis
Data generated from the experiments were saved and organized by Microsoft Excel (Microsoft, Redmond, United States). Data were processed for appropriate statistical analysis such as t Test, One-way ANOVA and Chi-Squared Test using SPSS 19.0 (International Business Machines Corporation, Armonk, United States). Data are expressed as Mean ± SD and percentages. Image production was done by GraphPad prism 7.0 (GraphPad Software, San Diego, United States). p-values less than 0.05 and 0.01 were considered statistically significant and highly significant.
Results
Acute toxicity test in rats and mice
Acute oral toxicity tests were performed on SD rats and ICR mice, and the mortality of each dose group is shown in Table 2. The oral LD50 of MCE was calculated using Kärber’s method (Mantel, 1967) to be 1,564.55 mg/kg (95% confidence interval 1,386.97–1764.95 mg/kg) and 1,024.33 mg/kg (95% confidence interval 964.27–1,087.93) for rats and mice, respectively. mg/kg). Rats and mice showed signs of intoxication with erect back hair, arching of the back, and slow movement, and death occurred about 3 h after administration (Table 2). No obvious pathological organ damage was found at necropsy. Referring to the GHS acute toxicity classification criteria, MCE was found to be grade 4 (United Nations, 2015).
Repeated 90-day oral toxicity test in rats
The rats were given MCE at three doses of 156.46, 31.29, and 6.26 mg/kg for 90 days by ad libitum feeding, and the general behavior of the rats was normal during the observation period, with no significant differences in feeding, drinking and weight gain (Figure 2) compared to the blank control group (p > 0.05). There was no poisoning or death in each group. There were no significant differences in hematology (Table 3), serum biochemistry (Table 4), organ coefficients (Table 5), and histopathological examination in each test group compared with the control group (p > 0.05).
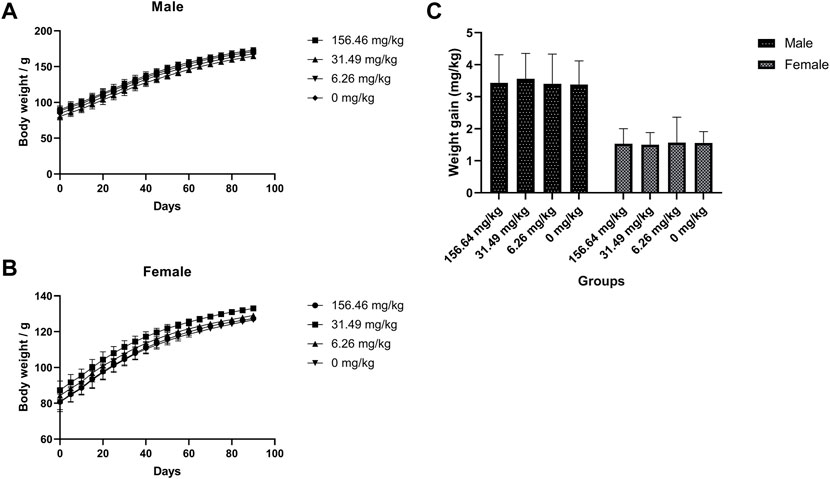
FIGURE 2. No effect of MCE on body weight gain in SD rats during 90 days of feeding. Male and female rats were orally administered 156.46, 31.49, 6.26, and 0 mg/kg (n = 10 rats/gender). (A) Body weight of male rats at 90 days; (B) Body weight of female rats at 90 days; (C) Body weight gain of male and female rats for 90 days.
Chronic toxicity in rats
The potential chronic toxicity risk is of particular concern because MCE has been added to feed for long periods of time for clinical use. The rats were fed at 78.23, 15.65, and 3.13 mg/kg for 180 days and did not exhibit any significant abnormalities or die during the observation period in all dose groups. Similarly, MCE did not produce a significant effect (p > 0.05) on the desire to eat and drink and weight gain of the rats (Figure 3). Further hematological (Table 3), serum biochemical (Table 4), organ coefficients (Table 5) and histopathological examinations also did not observe significant changes compared to the control group (p > 0.05).
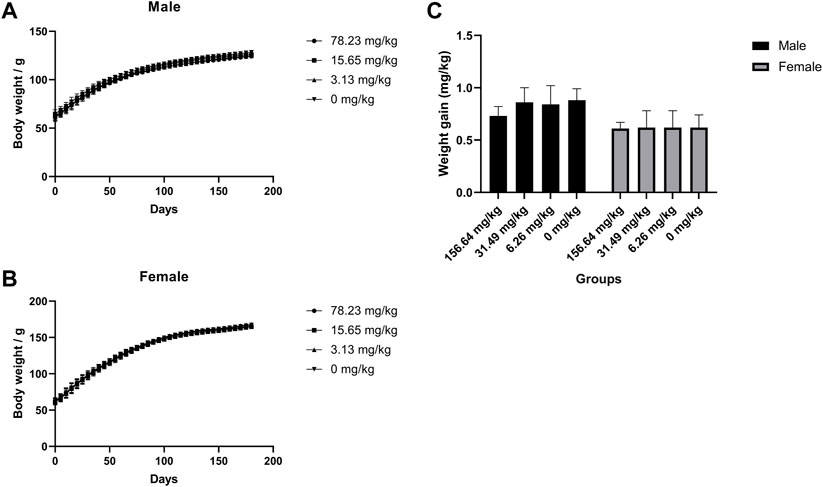
FIGURE 3. No effect of MCE on body weight gain in SD rats during 180 days of feeding. Male and female rats were orally administered 78.23, 15.65, 3.13, and 0 mg/kg (n = 25 rats/gender). (A) Body weight of male rats at 180 days; (B) Body weight of female rats at 180 days; (C) Body weight gain of male and female rats for 180 days.
Ames test
Four test strains of S. typhimurium grew normally, and each strain in the positive control group reacted satisfactorily. MCE was in the range of 100–0.16 μg/dish, and the mean number of revertant colonies per dish for all four test bacteria in the presence or absence of the metabolic activation system (S9) was within twice that of the solvent (sterilized distilled water) control, and no dose-response relationship was seen, and the results of the two tests were consistent. It was shown that MCE was not mutagenic to S. typhimurium for the test. The results of the two replicate tests are shown in Table 6.
Mouse bone marrow cell micronucleus test
Due to the solid evidence of the reported mutagenic effect of SAN on bone marrow cell DNA in vivo, we conducted a mouse bone marrow cell micronucleus assay. The results showed that the positive control group (cyclophosphamide) contained a significantly higher rate of micronucleated PCEs than the negative control group (p < 0.01), in contrast, the values of PCE/RBC were within the normal range in all dose groups. The PCE rates of bone marrow cells in female and male mice in each dose group of MCE were not significantly different from solvent controls (p > 0.05), indicating the lack of mutagenicity of MCE in mice (Table 7).
Mice sperm abnormality test
Subsequently, we tested whether MCE can cause sperm cell aberrations in vivo using a mouse sperm deformation assay. The sperm malformation rate of mice in each dose group of MCE did not show significant differences from the negative control group after five doses of exposure. In contrast to the significant increase in sperm malformations caused by cyclophosphamide-treated group, sperm abnormality rates in mice remained essentially unchanged at any of the MCE doses tested (Table 8), indicating that MCE is not teratogenic in mice.
Traditional teratogenicity test in rats
Because of the previously reported risk of embryotoxicity of SAN (Chan, 2015), we further investigated the potential embryotoxicity of MCE using a conventional teratogenic assay in rats. The results showed that MCE did not produce maternal toxicity in pregnant rats in the dose range of 24.45–391.15 mg/kg, and the pregnant rats showed normal behavior, no poisoning and death, and no significant differences in drinking, feeding and weight gain compared with the negative control group (p > 0.05) (Table 9). There was no significant difference in ovarian weight, number of corpus luteum, number of implantations, uterine weight and number of live fetuses between the dose groups compared to the negative control group (p > 0.05) (Table 9). No teratogenic effect of MCE on fetal rats was also found in the examination of fetal rats (stillbirth, appearance, viscera, and bones) (Tables 10, 11). The test results showed that MCE had no significant reproductive toxicity or embryotoxicity to rats.
Discussion
M. cordata is a commonly used topical drug in traditional Asian ethnomedicine and its use has been restricted due to transoral toxicity. Modern research has revealed that its main component, SAN, has a variety of biological activities and is used extensively in animal husbandry as a farming input to provide animal performance. Due to the long-term use in food animals and the potential toxicity risk of SAN, the safety information of MCE as a plant extract that can be added for a long time is of concern. Previous risk assessments of SAN were mostly based on intraperitoneal injections, whereas the most important route for the risk of ingestion of exogenous substances is via the oral route. Therefore, in this study, we systematically evaluated the safety of MCE by performing acute toxicity and long-term oral toxicity studies (subchronic and chronic toxicity) in rodents, while reassessing genotoxicity and developmental toxicity. This is the first systematic report on the safety of MCE after it was developed as a veterinary drug in China. In this report, MCE lacks long-term oral toxicity in rodents as well as mutagenic potential. In conclusion, our study provides important information for the rational use of MCE and subsequent residual safety assessment through general toxicity in rodents as well as genotoxicity assessment.
In acute toxicity studies in SD rats and ICR mice, the LD50 of MCE was 1,564.55 and 1,024.33 mg/kg, respectively, which were classified as low toxic substances in the acute toxicity classification of GHS and WHO compounds. The oral acute toxicity results of MCE in rats were similar to those previously obtained with alkaloid extracts of SAN and Sanguinaria canadensis L. (Becci et al., 1987). In the subsequent repeated 90-day oral toxicity test, the highest dose of 156.46 mg/kg did not affect body weight gain or other physiological factors for 90 days, and no toxicity-related clinical manifestations were observed during this period. No abnormalities associated with MCE were also found in the hematological, serum biochemical, profiling, and histopathological examinations, and the no observed adverse effect level (NOEAL) of the subchronic toxicity test was determined to be 156.46 mg/kg. In a previous 90-day oral toxicity study of a mixture of quaternary benzo[c]phenanthridine alkaloids of M. cordata in rats, 600 mg/kg was considered a safe dose with no significant difference from the negative control (Zdarilova et al., 2008). In addition, in a 90-day feeding trial in pigs, a daily intake of 5 mg/kg of the combined SA/CHE extract was considered safe (Kosina et al., 2004). There may be differences in the proportion of ingredients in the extract as much as possible, but this seems to improve the safety range of MCE. Nevertheless, since the toxicity of a mixture can be influenced by the type and proportion of constituents in it, safety inferences need to be made with caution until the other components of M. cordata alkaloid extracts have been subjected to a definitive risk assessment. Similarly, no signs of toxicity associated with MCE were found in further chronic toxicity studies in rats, and the NOEAL was 78.23 mg/kg.
SAN has been reported to form DNA adducts in vitro and to increase the level of DNA single-strand breaks in mouse erythrocytes and bone marrow cells after intraperitoneal injection (Stiborová et al., 2002; Ansari et al., 2005; Kaminskyy et al., 2008). In the current study of the genotoxicity of MCE, the number of revertant colonies in all tested strains did not increase at the highest dose of 100 μg/dish. showed agreement with the results of a previous SAN trial in the E. coli PQ37 genotoxicity assay (SOS chromosome) (Kevekordes et al., 1999). Similarly, MCE showed a lack of mutagenic potential in the mouse sperm deformation test and micronucleus test. In another 90-day rat study, a mixture of 120 mg SAN and CHE per kg of feed was also shown to lack evidence of genotoxicity (Stiborova et al., 2008). In addition, some studies have shown toxic effects of SAN on oocytes in vitro and developmental risk after embryo transfer (Chan, 2011; Chan, 2015), but in the present study, the results of the conventional teratogenic test in rats were not consistent with this. Within the dose range tested, MCE did not exhibit significant maternal damaging effects or fetal developmental toxicity in pregnant rats. In conclusion, the experimental results in this study contradict the previously reported results, and the reasons for this are related to the nature of the SAN itself. Early risk assessments of SAN used almost exclusively intraperitoneal injections, ignoring the interaction between the gastrointestinal tract and SAN. Previous studies tested the stability of SAN and CHE in an artificially simulated gastrointestinal environment and showed that SAN and CHE were stable in an acidic solution, but the average recoveries of SAN and CHE were only 60.53% and 87.89% in an alkaline environment at pH = 8 (Zhang et al., 2017). The mRNA expression of CYP1A1 and CYP1A2 was increased in porcine intestinal epithelial cells after the administration of 5 and 50 μg/ml SAN (Palócz et al., 2019). Current evidence suggests that CYP1A1 and CYP1A2 play an important role in the conversion of SAN to dihydrosanguinarine (DHSA, the first metabolite of SAN metabolic transformation) (Psotová et al., 2006; Deroussent et al., 2010; Sandor et al., 2018) and this is considered to be a possible detoxification process for SANs (Vrba et al., 2004). Degradation and metabolism in the intestine may be one of the reasons for the low bioavailability of SAN. Although DHSA has also recently been reported to have anti-inflammatory (Xiang et al., 2022) and analgesic (Gaona-Tovar et al., 2022) properties, whether it can regulate intestinal flora still needs to be elucidated. Several pharmacokinetic studies explain that SAN and CHE have low gastrointestinal absorption and bioavailability, and are rapidly metabolized in vivo (Vecera et al., 2007; Hu et al., 2019; Wu et al., 2020; Zhao et al., 2021). Another toxicity test on the alkaloids of Chelidonium majus L. (CAL) yielded similar inferences (Gao et al., 2019). In vitro metabolism studies have shown that SAN may be metabolized in the intestine to DHSA, which has been shown to be much less toxic than SAN (Vecera et al., 2007; Vrublova et al., 2008; Sandor et al., 2016). The accumulation coefficients for SAN and DHSA after multiple oral administrations were 1.21 and 1.11, respectively, which seems to explain the low toxicity exhibited by long-term addition of SAN at reasonable doses (Wu et al., 2020).
Conclusion
We systematically evaluated the general and genetic developmental toxicity of MCE. In this study, MCE was found to exhibit hypotoxic properties in acute exposure to SD rats and ICR mice. The corresponding toxicity risk was also not demonstrated in long-term exposure tests. In addition, the results of systematic mutagenicity and teratogenicity tests showed a lack of evidence of genotoxicity by oral ingestion of MCE at the doses tested. The results of this study provide preliminary and more holistic risk assessment information for MCE in the context of veterinary clinical and human food safety. It is important to note that the results of this study are based on standardized MCE test substances manufactured in GMP production plants. Caution should be exercised when using other M. cordata products that are different from MCE, as the difference in toxicity of the mixture depends mainly on the composition and proportions involved.
Data availability statement
The original contributions presented in the study are included in the article/supplementary material, further inquiries can be directed to the corresponding authors.
Ethics statement
The animal study was reviewed and approved by the Animal Ethics Committee of China Agricultural University.
Author contributions
Conceptualization, ZD and J-GZ; methodology, ZD and S-ST; software, ZD; validation, ZD and Z-HY; formal analysis, ZD and X-LM; resources, C-HL and J-GZ; data curation, ZD; writing—original draft preparation, ZD; writing—review and editing, ZD and S-ST; visualization, ZD; supervision, C-HL and Z-ST; project administration, Z-HY; All authors have read and agreed to the published version of the manuscript.
Funding
This research did not receive any specific grant from funding agencies in the public, commercial, or not-for-profit sectors.
Conflict of interest
C-HL and Z-ST were employed by Hunan MICOLTA Biological Resources Co., Ltd.
The remaining authors declare that the research was conducted in the absence of any commercial or financial relationships that could be construed as a potential conflict of interest.
Publisher’s note
All claims expressed in this article are solely those of the authors and do not necessarily represent those of their affiliated organizations, or those of the publisher, the editors and the reviewers. Any product that may be evaluated in this article, or claim that may be made by its manufacturer, is not guaranteed or endorsed by the publisher.
References
Aguilar-Hernández, J. A., Urías-Estrada, J. D., López-Soto, M. A., Barreras, A., Plascencia, A., Montaño, M., et al. (2016). Evaluation of isoquinoline alkaloid supplementation levels on ruminal fermentation, characteristics of digestion, and microbial protein synthesis in steers fed a high-energy diet. J. Anim. Sci. 94, 267–274. doi:10.2527/jas.2015-9376
Anjago, W. M., Zeng, W., Chen, Y., Wang, Y., Biregeya, J., Li, Y., et al. (2021). The molecular mechanism underlying pathogenicity inhibition by sanguinarine in Magnaporthe oryzae. Pest Manag. Sci. 77, 4669–4679. doi:10.1002/ps.6508
Ansari, K. M., Dhawan, A., Khanna, S. K., and Das, M. (2005). In vivo DNA damaging potential of sanguinarine alkaloid, isolated from argemone oil, using alkaline comet assay in mice. Food Chem. Toxicol. 43, 147–153. doi:10.1016/j.fct.2004.09.005
Ansari, K. M., Dhawan, A., Khanna, S. K., and Das, M. (2006). Protective effect of bioantioxidants on argemone oil/sanguinarine alkaloid induced genotoxicity in mice. Cancer Lett. 244, 109–118. doi:10.1016/j.canlet.2005.12.014
Becci, P. J., Schwartz, H., Barnes, H. H., and Southard, G. L. (1987). Short-term toxicity studies of sanguinarine and of two alkaloid extracts of Sanguinaria canadensis L. J. Toxicol. Environ. Health 20, 199–208. doi:10.1080/15287398709530972
Bussabong, P., Rairat, T., Chuchird, N., Keetanon, A., Phansawat, P., Cherdkeattipol, K., et al. (2021). Effects of isoquinoline alkaloids from macleaya cordata on growth performance, survival, immune response, and resistance to Vibrio parahaemolyticus infection of Pacific white shrimp (Litopenaeus vannamei). PLoS One 16, e0251343. doi:10.1371/journal.pone.0251343
Cai, Q., Meng, J., Ge, Y., Gao, Y., Zeng, Y., Li, H., et al. (2020). Fishing antitumor ingredients by G-quadruplex affinity from herbal extract on a three-phase-laminar-flow microfluidic chip. Talanta 220, 121368. doi:10.1016/j.talanta.2020.121368
Chan, W.-H. (2011). Embryonic toxicity of sanguinarine through apoptotic processes in mouse blastocysts. Toxicol. Lett. 205, 285–292. doi:10.1016/j.toxlet.2011.06.018
Chan, W.-H. (2015). Hazardous effects of sanguinarine on maturation of mouse oocytes, fertilization, and fetal development through apoptotic processes. Environ. Toxicol. 30, 946–955. doi:10.1002/tox.21969
Chen, T., and Shang, Z. (2002). Commentary on supplement to medica. Hefei: Anhui Science and Technology Press.
Chen, J., Kang, B., Zhao, Y., Yao, K., and Fu, C. (2018). Effects of natural dietary supplementation with Macleaya cordata extract containing sanguinarine on growth performance and gut health of early-weaned piglets. J. Anim. Physiol. Anim. Nutr. 102, 1666–1674. doi:10.1111/jpn.12976
Chen, K., Liu, Y., Cheng, Y., Yan, Q., Zhou, C., He, Z., et al. (2020). Supplementation of lactobacillus plantarum or Macleaya cordata extract alleviates oxidative damage induced by weaning in the lower gut of young goats. Animals. 10, E548. doi:10.3390/ani10040548
Chin, C.-K. (2008). “Plant cell culture as a source of valuable chemicals,” in Biotechnology in flavor production (Hoboken, United States: John Wiley & Sons), 104–117. doi:10.1002/9781444302493.ch4
Choy, C.-S., Cheah, K.-P., Chiou, H.-Y., Li, J.-S., Liu, Y.-H., Yong, S.-F., et al. (2008). Induction of hepatotoxicity by sanguinarine is associated with oxidation of protein thiols and disturbance of mitochondrial respiration. J. Appl. Toxicol. 28, 945–956. doi:10.1002/jat.1360
Council of the European Union and European Parliament (2008). Regulation (EC) No 882/2004 of the European Parliament and of the Council of 29 April 2004 on official controls performed to ensure the verification of compliance with feed and food law, animal health and animal welfare rules. OJEU L191, 1–52.
Danielewski, M., Zielińska, S., Matuszewska, A., Słupski, W., Włodarczyk, M., Jęśkowiak, I., et al. (2022). Sanguinarine-chelerythrine fraction of coptis chinensis exerts anti-inflammatory activity in carrageenan paw oedema test in rats and reveals reduced gastrotoxicity. Oxid. Med. Cell. Longev. 2022, 1504929. doi:10.1155/2022/1504929
Deroussent, A., Ré, M., Hoellinger, H., and Cresteil, T. (2010). Metabolism of sanguinarine in human and in rat: characterization of oxidative metabolites produced by human CYP1A1 and CYP1A2 and rat liver microsomes using liquid chromatography-tandem mass spectrometry. J. Pharm. Biomed. Anal. 52, 391–397. doi:10.1016/j.jpba.2009.09.014
Directorate-General for Health and Food Safety (2021). European Union register of feed additives pursuant to Regulation (EC) No 1831/2003.Annex I, List of additives (Released date 21.09.2021). LU: Publications Office of the European Union. Available at: https://data.europa.eu/doi/10.2875/434313 (Accessed July 15, 2022).
Dong, Z., Liu, M., Zhong, X., Ou, X., Yun, X., Wang, M., et al. (2021). Identification of the impurities in bopu Powder® and Sangrovit® by LC-MS combined with a screening method. Molecules 26, 3851. doi:10.3390/molecules26133851
Falchi, F. A., Borlotti, G., Ferretti, F., Pellegrino, G., Raneri, M., Schiavoni, M., et al. (2021). Sanguinarine inhibits the 2-ketogluconate pathway of glucose utilization in Pseudomonas aeruginosa. Front. Microbiol. 12, 744458. doi:10.3389/fmicb.2021.744458
Gao, L., Schmitz, H.-J., Merz, K.-H., and Schrenk, D. (2019). Characterization of the cytotoxicity of selected chelidonium alkaloids in rat hepatocytes. Toxicol. Lett. 311, 91–97. doi:10.1016/j.toxlet.2019.04.031
Gaona-Tovar, E., Estrada-Soto, S., González-Trujano, M. E., Martínez-Vargas, D., Hernandez-Leon, A., Narváez-González, F., et al. (2022). Antinociceptive and gastroprotective activities of Bocconia arborea S. Watson and its bioactive metabolite dihydrosanguinarine in murine models. J. Ethnopharmacol. 296, 115492. doi:10.1016/j.jep.2022.115492
Ghosh, I., and Mukherjee, A. (2016). Argemone oil induces genotoxicity in mice. Drug Chem. Toxicol. 39, 407–411. doi:10.3109/01480545.2015.1135339
Günaydın, Y. K., Dündar, Z. D., Çekmen, B., Akıllı, N. B., Köylü, R., and Cander, B. (2015). Intoxication due to papaver rhoeas (corn poppy): five case reports. Case Rep. Med. 2015, 321360. doi:10.1155/2015/321360
Hu, C. M., Cheng, Y. W., Liao, J. W., Cheng, H. W., and Kang, J. J. (2005). Induction of contracture and extracellular Ca2+ influx in cardiac muscle by sanguinarine: a study on cardiotoxicity of sanguinarine. J. Biomed. Sci. 12, 399–407. doi:10.1007/s11373-005-3007-y
Hu, N.-X., Chen, M., Liu, Y.-S., Shi, Q., Yang, B., Zhang, H.-C., et al. (2019). Pharmacokinetics of sanguinarine, chelerythrine, and their metabolites in broiler chickens following oral and intravenous administration. J. Vet. Pharmacol. Ther. 42, 197–206. doi:10.1111/jvp.12729
Juskiewicz, J., Gruzauskas, R., Zdunczyk, Z., Semaskaite, A., Jankowski, J., Totilas, Z., et al. (2011). Effects of dietary addition of macleaya cordata alkaloid extract on growth performance, caecal indices and breast meat fatty acids profile in male broilers. J. Anim. Physiol. Anim. Nutr. 95, 171–178. doi:10.1111/j.1439-0396.2010.01037.x
Kaminskyy, V., Lin, K. W., Filyak, Y., and Stoika, R. (2008). Differential effect of sanguinarine, chelerythrine and chelidonine on DNA damage and cell viability in primary mouse spleen cells and mouse leukemic cells. Cell Biol. Int. 32, 271–277. doi:10.1016/j.cellbi.2007.09.004
Kevekordes, S., Mersch-Sundermann, V., Burghaus, C. M., Spielberger, J., Schmeiser, H. H., Arlt, V. M., et al. (1999). SOS induction of selected naturally occurring substances in escherichia coli (SOS chromotest). Mutat. Res. 445, 81–91. doi:10.1016/s1383-5718(99)00141-2
Kosina, P., Walterová, D., Ulrichová, J., Lichnovský, V., Stiborová, M., Rýdlová, H., et al. (2004). Sanguinarine and chelerythrine: Assessment of safety on pigs in ninety days feeding experiment. Food Chem. Toxicol. 42, 85–91. doi:10.1016/j.fct.2003.08.007
Li, J.-Y., Huang, H.-B., Pan, T.-X., Wang, N., Shi, C.-W., Zhang, B., et al. (2022). Sanguinarine induces apoptosis in eimeria tenella sporozoites via the generation of reactive oxygen species. Poult. Sci. 101, 101771. doi:10.1016/j.psj.2022.101771
Lin, L., Liu, Y.-C., Huang, J.-L., Liu, X.-B., Qing, Z.-X., Zeng, J.-G., et al. (2018). Medicinal plants of the genus macleaya (macleaya cordata, macleaya microcarpa): a review of their phytochemistry, pharmacology, and toxicology. Phytother. Res. 32, 19–48. doi:10.1002/ptr.5952
Lin, L., Li, X.-Y., Liu, S.-S., Qing, Z.-X., Liu, X.-B., Zeng, J.-G., et al. (2020). Systematic identification of compounds in macleaya microcarpa by high-performance liquid chromatography/quadrupole time-of-flight tandem mass spectrometry combined with mass spectral fragmentation behavior of macleaya alkaloids. Rapid Commun. Mass Spectrom. 34, e8715. doi:10.1002/rcm.8715
Liu, T., Li, T., Chen, X., Zhang, K., Li, M., Yao, W., et al. (2021). A network-based analysis and experimental validation of traditional Chinese medicine Yuanhu Zhitong Formula in treating neuropathic pain. J. Ethnopharmacol. 274, 114037. doi:10.1016/j.jep.2021.114037
Liu, F., Wang, H., Zhu, X., Jiang, N., Pan, F., Song, C., et al. (2022a). Sanguinarine promotes healthspan and innate immunity through a conserved mechanism of ROS-mediated PMK-1/SKN-1 activation. iScience 25, 103874. doi:10.1016/j.isci.2022.103874
Liu, Z.-H., Wang, W.-M., Zhang, Z., Sun, L., and Wu, S.-C. (2022b). Natural antibacterial and antivirulence alkaloids from Macleaya cordata against methicillin-resistant Staphylococcus aureus. Front. Pharmacol. 13, 813172. doi:10.3389/fphar.2022.813172
Lu, M., Li, K., He, H., Cheng, Y., and Yang, P. (2020). Systematic characterization of alkaloids in eomecon chionantha hance using ultrahigh-performance liquid chromatography-tandem quadrupole exactive orbitrap mass spectrometry with a four-step screening strategy. Rapid Commun. Mass Spectrom. 34, e8880. doi:10.1002/rcm.8880
Lu, C., Zhang, N., Kou, S., Gao, L., Peng, B., Dai, Y., et al. (2022). Sanguinarine synergistically potentiates aminoglycoside-mediated bacterial killing. Microb. Biotechnol. 15, 2055–2070. doi:10.1111/1751-7915.14017
Mantel, N. (1967). Adaptation of Kärber–s method for estimating the exponential parameter from quantal data, and its relationship to birth, death, and branching processes. Biometrics 23, 739–746.
Ministry of Agriculture and Rural Affairs of PRC (2009a). Guidelines for 30-day and 90-day feeding trials of veterinary drugs. Notice No. 442 of the Ministry of Agriculture of the people’s Republic of China, 1–6.
Ministry of Agriculture and Rural Affairs of PRC (2009b). Guidelines for Ames testing of veterinary drugs. Notice No. 442 of the Ministry of Agriculture of the people’s Republic of China, 1–17.
Ministry of Agriculture and Rural Affairs of PRC (2009c). Guidelines for chronic toxicity and carcinogenicity testing of veterinary drugs. Notice No. 442 of the Ministry of Agriculture of the people’s Republic of China, 1–6.
Ministry of Agriculture and Rural Affairs of PRC (2009d). Guidelines for classical teratogenicity tests on veterinary drugs in rats, 1–9.
Ministry of Agriculture and Rural Affairs of PRC (2009e). Guidelines for sperm abnormality tests in veterinary mice. Notice No. 442 of the Ministry of Agriculture of the people’s Republic of China, 1–4.
Ministry of Agriculture and Rural Affairs of PRC (2009f). Guidelines for the acute toxicity (LD50 determination) of veterinary drugs. Notice No. 442 of the Ministry of Agriculture of the people’s Republic of China, 1–18.
Ministry of Agriculture and Rural Affairs of PRC (2009g). Guidelines for the veterinary mouse bone marrow cell staining aberration test. Notice No. 442 of the Ministry of Agriculture of the people’s Republic of China, 1–7.
Moro, P. A., Cassetti, F., Giugliano, G., Falce, M. T., Mazzanti, G., Menniti-Ippolito, F., et al. (2009). Hepatitis from greater celandine (chelidonium majus L.): review of literature and report of a new case. J. Ethnopharmacol. 124, 328–332. doi:10.1016/j.jep.2009.04.036
Palócz, O., Szita, G., and Csikó, G. (2019). Alteration in inflammatory responses and cytochrome P450 expression of porcine jejunal cells by drinking water supplements. Mediat. Inflamm. 2019, 5420381. doi:10.1155/2019/5420381
Prabhu, K. S., Bhat, A. A., Siveen, K. S., Kuttikrishnan, S., Raza, S. S., Raheed, T., et al. (2021). Sanguinarine mediated apoptosis in non-small cell lung cancer via generation of reactive oxygen species and suppression of JAK/STAT pathway. Biomed. Pharmacother. 144, 112358. doi:10.1016/j.biopha.2021.112358
Psotová, J., Klejdus, B., Vecera, R., Kosina, P., Kubán, V., Vicar, J., et al. (2006). A liquid chromatographic-mass spectrometric evidence of dihydrosanguinarine as a first metabolite of sanguinarine transformation in rat. J. Chromatogr. B Anal. Technol. Biomed. Life Sci. 830, 165–172. doi:10.1016/j.jchromb.2005.10.030
Qing, Z.-X., Cheng, P., Liu, X.-B., Liu, Y.-S., Zeng, J.-G., and Wang, W. (2014). Structural speculation and identification of alkaloids in macleaya cordata fruits by high-performance liquid chromatography/quadrupole-time-of-flight mass spectrometry combined with a screening procedure. Rapid Commun. Mass Spectrom. 28, 1033–1044. doi:10.1002/rcm.6874
Qing, Z., Xu, Y., Yang, P., Yu, K., Peng, Y., Zuo, Z., et al. (2016a). Study on alkaloids of fruit from macleaya cordata. Zhong Yao Cai 39, 312–314. doi:10.13863/j.issn1001-4454.2016.02.018
Qing, Z.-X., Xu, Y.-Q., Yang, P., Yu, K., Cheng, P., and Zeng, J.-G. (2016b). Mass spectrometry-guided isolation of two new benzoquinoline alkaloids from Macleaya cordata. Nat. Prod. Res. 30, 1030–1035. doi:10.1080/14786419.2015.1101695
Sandor, R., Midlik, A., Sebrlova, K., Dovrtelova, G., Noskova, K., Jurica, J., et al. (2016). Identification of metabolites of selected benzophenanthridine alkaloids and their toxicity evaluation. J. Pharm. Biomed. Anal. 121, 174–180. doi:10.1016/j.jpba.2016.01.024
Sandor, R., Slanina, J., Midlik, A., Sebrlova, K., Novotna, L., Carnecka, M., et al. (2018). Sanguinarine is reduced by NADH through a covalent adduct. Phytochemistry 145, 77–84. doi:10.1016/j.phytochem.2017.10.010
Sharma, B. D., Bhatia, V., Rahtee, M., Kumar, R., and Mukharjee, A. (2002). Epidemic dropsy: observations on pathophysiology and clinical features during the Delhi epidemic of 1998. Trop. Doct. 32, 70–75. doi:10.1177/004947550203200204
Stiborová, M., Simánek, V., Frei, E., Hobza, P., and Ulrichová, J. (2002). DNA adduct formation from quaternary benzo[c]phenanthridine alkaloids sanguinarine and chelerythrine as revealed by the 32P-postlabeling technique. Chem. Biol. Interact. 140, 231–242. doi:10.1016/s0009-2797(02)00038-8
Stiborova, M., Vostalova, J., Zdarilova, A., Ulrichova, J., Hudecek, J., Tschirner, K., et al. (2008). Macleaya cordata extract and sangrovit genotoxicity. Assessment in vivo. Biomed. Pap. Med. Fac. Univ. Palacky. Olomouc Czech. Repub. 152, 35–39. doi:10.5507/bp.2008.005
United Nations (2015). Globally harmonized system of classification and labelling of chemicals (GHS). 6th revised edition. New York: United Nations.
Vaidya, A. D., Pandita, N., Godse, C., and Meshram, M. (2001). Argemone mexicana and epidemic dropsy: phytopharmacology and putative sequelae and therapy. J. Assoc. Physicians India 49, 1041–1042.
Vecera, R., Klejdus, B., Kosina, P., Orolin, J., Stiborová, M., Smrcek, S., et al. (2007). Disposition of sanguinarine in the rat. Xenobiotica. 37, 549–558. doi:10.1080/00498250701230542
Veterinary Drug Assessment Centre of the Ministry of Agriculture of the, and People’s Republic of China (2012). Compilation of technical uidelines for veterinary drug research. Beijing: Chemical Industry Press.
Vrba, J., Kosina, P., Ulrichová, J., and Modrianský, M. (2004). Involvement of cytochrome P450 1A in sanguinarine detoxication. Toxicol. Lett. 151, 375–387. doi:10.1016/j.toxlet.2004.03.005
Vrublova, E., Vostalova, J., Vecera, R., Klejdus, B., Stejskal, D., Kosina, P., et al. (2008). The toxicity and pharmacokinetics of dihydrosanguinarine in rat: a pilot study. Food Chem. Toxicol. 46, 2546–2553. doi:10.1016/j.fct.2008.04.013
Wang, W., Dolan, L. C., von Alvensleben, S., Morlacchini, M., and Fusconi, G. (2018). Safety of standardized macleaya cordata extract in an eighty-four-day dietary study in dairy cows. J. Anim. Physiol. Anim. Nutr. 102, e61–e68. doi:10.1111/jpn.12702
Wang, X., Yang, X., Wang, J., Li, L., Zhang, Y., Jin, M., et al. (2022). Cardiotoxicity of sanguinarine via regulating apoptosis and MAPK pathways in zebrafish and HL1 cardiomyocytes. Comp. Biochem. Physiol. C. Toxicol. Pharmacol. 252, 109228. doi:10.1016/j.cbpc.2021.109228
Warowicka, A., Popenda, Ł., Bartkowiak, G., Musidlak, O., Litowczenko-Cybulska, J., Kuźma, D., et al. (2019). Protoberberine compounds extracted from chelidonium majus L. as novel natural photosensitizers for cancer therapy. Phytomedicine 64, 152919. doi:10.1016/j.phymed.2019.152919
Wu, Y., Zhao, N.-J., Cao, Y., Sun, Z., Wang, Q., Liu, Z.-Y., et al. (2020). Sanguinarine metabolism and pharmacokinetics study in vitro and in vivo. J. Vet. Pharmacol. Ther. 43, 208–214. doi:10.1111/jvp.12835
Wu, L., Yang, Y., Mao, Z., Wu, J., Ren, D., Zhu, B., et al. (2021). Processing and compatibility of corydalis yanhusuo: phytochemistry, pharmacology, pharmacokinetics, and safety. Evid. Based. Complement. Altern. Med. 2021, 1271953. doi:10.1155/2021/1271953
Xiang, Y., Zhang, H., Xu Zhang, Z., Yang Qu, X., and Xia Zhu, F. (2022). Dihydrosanguinarine based RNA-seq approach couple with network pharmacology attenuates LPS-induced inflammation through TNF/IL-17/PI3K/AKT pathways in mice liver. Int. Immunopharmacol. 109, 108779. doi:10.1016/j.intimp.2022.108779
Ye, F., Feng, F., and Liu, W. (2009). Alkaloids from Macleaya cordata. Zhongguo Zhong Yao Za Zhi 34, 1683–1686.
Zdarilova, A., Vrublova, E., Vostalova, J., Klejdus, B., Stejskal, D., Proskova, J., et al. (2008). Natural feed additive of Macleaya cordata: safety assessment in rats a 90-day feeding experiment. Food Chem. Toxicol. 46, 3721–3726. doi:10.1016/j.fct.2008.09.054
Zhang, H., Chen, M., Liu, Y., Tang, Z., Zeng, J., and Liu, Z. (2017). Stability analysis of major alkaloids in macleaya cordata under simulated gastrointestinal environment. Mod. Chin. Med. 19, 1387–1390. doi:10.13313/j.issn.1673-4890.2017.10.007
Zhang, R., Guo, Q., Kennelly, E. J., Long, C., and Chai, X. (2020). Diverse alkaloids and biological activities of fumaria (papaveraceae): an ethnomedicinal group. Fitoterapia 146, 104697. doi:10.1016/j.fitote.2020.104697
Zhang, Q., Zhang, Z., Zhou, S., Jin, M., Lu, T., Cui, L., et al. (2021). Macleaya cordata extract, an antibiotic alternative, does not contribute to antibiotic resistance gene dissemination. J. Hazard. Mat. 412, 125272. doi:10.1016/j.jhazmat.2021.125272
Zhao, N.-J., Wang, L.-L., Liu, Z.-Y., Wang, Q., Liu, L., Sun, Z.-L., et al. (2021). Pharmacokinetics of chelerythrine and its metabolite after oral and intramuscular administrations in pigs. Xenobiotica. 51, 1264–1270. doi:10.1080/00498254.2021.1882714
Zhu, M., Gong, Z., Wu, Q., Shi, X., Su, Q., and Zhang, Y. (2020). Sanguinarine suppresses migration and metastasis in colorectal carcinoma associated with the inversion of EMT through the Wnt/β-catenin signaling. Clin. Transl. Med. 10, 1–12. doi:10.1002/ctm2.1
Keywords: Macleaya cordata, extract, rodent, acute toxicity, long-term toxicity, genotoxicity, teratogenicity
Citation: Dong Z, Tang S-S, Ma X-L, Li C-H, Tang Z-S, Yang Z-H and Zeng J-G (2022) Preclinical safety evaluation of Macleaya Cordata extract: A re-assessment of general toxicity and genotoxicity properties in rodents. Front. Pharmacol. 13:980918. doi: 10.3389/fphar.2022.980918
Received: 29 June 2022; Accepted: 20 July 2022;
Published: 12 August 2022.
Edited by:
Fareeha Anwar, Riphah International University (Lahore), PakistanReviewed by:
Muhamamd Haseeb, Akhtar Saeed Medical and Dental College, PakistanAyesha Shafi, Riphah International University (Lahore), Pakistan
Copyright © 2022 Dong, Tang, Ma, Li, Tang, Yang and Zeng. This is an open-access article distributed under the terms of the Creative Commons Attribution License (CC BY). The use, distribution or reproduction in other forums is permitted, provided the original author(s) and the copyright owner(s) are credited and that the original publication in this journal is cited, in accordance with accepted academic practice. No use, distribution or reproduction is permitted which does not comply with these terms.
*Correspondence: Zi-Hui Yang, eWFuZ3ppaHVpXzIwMDZAMTYzLmNvbQ==; Jian-Guo Zeng, emVuZ2ppYW5ndW9AaHVuYXUuZWR1LmNu