- 1Department of Acupuncture and Moxibustion, Neuroscience Centre, Integrated Hospital of Traditional Chinese Medicine, Southern Medical University, Guangzhou, China
- 2Beijing Key Laboratory of Pharmacology of Chinese Materia, Institute of Basic Medical Sciences, Xiyuan Hospital of China Academy of Chinese Medical Sciences, Beijing, China
- 3Beijing Increasepharm Safety and Efficacy Co. Ltd., Beijing, China
- 4Shineway Pharmaceutical Group Co. Ltd., Shijiazhuang, China
- 5NICM, Western Sydney University, Penrith, NSW, Australia
- 6Department of Anesthesiology, Punan Hospital, Shanghai, China
- 7School of Pharmaceutical Sciences, Institute for Chinese Materia Medica, Tsinghua University, Beijing, China
Stroke is a major cause of death and disability throughout the world. A combination of Panax Ginseng and Ginkgo biloba extracts (CGGE) is an effective treatment for nervous system diseases, but the neuroprotective mechanism underlying CGGE remains unclear. Both network analysis and experimental research were employed to explore the potential mechanism of CGGE in treating ischemic stroke (IS). Network analysis identified a total number of 133 potential targets for 34 active ingredients and 239 IS-related targets. What’s more, several processes that might involve the regulation of CGGE against IS were identified, including long-term potentiation, cAMP signaling pathway, neurotrophin signaling pathway, and Nod-like receptor signaling pathway. Our studies in animal models suggested that CGGE could reduce inflammatory response by inhibiting the activity of Nod-like receptor, pyrin containing 3 (NLRP3) inflammasome, and maintain the balance of glutamate (Glu)/gamma-aminobutyric acid (GABA) via activating calmodulin-dependent protein kinase type Ⅳ (CAMK4)/cyclic AMP-responsive element-binding protein (CREB) pathway. These findings indicated the neuroprotective effects of CGGE, possibly improving neuroinflammation and excitotoxicity by regulating the NLRP3 inflammasome and CAMK4/CREB pathway.
1 Introduction
Stroke is one of the leading causes of mortality and disability, especially in low-income and middle-income countries (O'Donnell et al., 2016). According to the recent Global Burden of Disease survey, stroke has risen to the third position from the fifth (Collaborators, 2020). Approximately 87% of all strokes are ischemic strokes (Virani et al., 2020).
The underlying mechanism of ischemic stroke (IS) is complex. Excitotoxicity and inflammation play crucial roles in the pathophysiological process of IS. Overactivation of the glutamate receptor mediates excitotoxicity, which has been suggested as a critical event in ischemic brain injury. Glutamate (Glu) is a major excitatory neurotransmitter in the central nervous system and acts on metabotropic and ionotropic receptors (including N-methyl-D-aspartate receptor, NMDAR) to mediate excitatory synapse transmission (Traynelis et al., 2010). While gamma-aminobutyric acid (GABA) mainly works as an inhibitory neurotransmitter in the brain and modulates excitatory neurotransmission (Boddum et al., 2016). Under ischemic conditions, excessive glutamate over-stimulates NMDAR, causing massive Ca2+ influx. The increased intracellular Ca2+ causes mitochondrial dysfunction and overproduction of reactive oxygen species (ROS), which activates inflammatory responses and results in the death of damaged neurons (Zhou et al., 2018). NMDAR activation at a moderate level promotes neuroprotective signaling pathways, including activation of the RAS-mitogen-activated protein kinase (RAS-MAPK) pathway and cyclic AMP-responsive element-binding protein (CREB)-dependent gene transcription. Brain-derived neurotrophic factor (BDNF) plays a key role in neuronal development and synaptic plasticity, which could be promoted by CREB (Park and Poo, 2013). Tat-NR2B9c, a blocker of NMDAR-postsynaptic density protein-95 (PSD95), exerts a neuroprotective effect on stroke by activating Ca2+ dependent pathways and enhancing calmodulin-dependent protein kinase type Ⅳ (CaMKⅣ) and CREB (Bell et al., 2013). Nod-like receptor, pyrin containing 3 (NLRP3)-inflammasome is a member of innate cell sensors, which can produce a variety of proinflammatory cytokines and mediate nerve cell dysfunction, thus leading to cell death after cerebral ischemia (Xu et al., 2021). There is evidence of increased expression and activation of NLRP3 inflammasome in ischemic stroke neurons (Savage et al., 2012; Fann et al., 2018). The NLRP3 inflammasome is composed of three components, a sensor (NLRP3), an adaptor (ASC; apoptosis-associated speck-like protein containing a caspase recruitment domain) and an effector (caspase-1) (Swanson et al., 2019).
Panax Ginseng (P.ginseng C.A.Mey.) is a kind of valuable traditional Chinese medicine (TCM) with a history of thousands of years. The neuroprotective effects of panax ginseng on neurodegeneration diseases have been widely reported in the past decades, including cerebral ischemia, Alzheimer’s disease, and Parkinson’s disease (Kim et al., 2018). The key bioactive components of ginseng include more than 60 ginsenosides, such as ginsenoside Rb1, Rb2, Rb3, Rc, Rd, Re, and Rg1 (Ong et al., 2015). In vitro and in vivo studies have demonstrated that ginsenoside Rd can protect against cerebral ischemia by promoting extracellular glutamate clearance (Zhang et al., 2013). What’s more, the extracts of red ginseng could reduce cerebral infarction and edema through anti-inflammatory and anti-apoptotic pathways (Jin et al., 2022). G. biloba (G. biloba L.) is a natural medicine widely used to treat neurodegenerative diseases. The neuroprotective effects of ginkgo biloba on ischemic brain injury are mainly through the regulation of excitotoxicity, inflammatory pathways and oxidative damage (Feng et al., 2019). It can reduce the formation of cellular edema and neuroinflammatory injury (Zhang et al., 2018b), and regulate the imbalance of excitatory and inhibitory amino acids (Yang et al., 2011; Mdzinarishvili et al., 2012). Previous studies have shown that combining P. ginseng and G. biloba into a single treatment has a synergistic effect in clinical application, and they improve aspects of physiological and cognitive function in humans (Reay et al., 2019). Both G. biloba and P. ginseng could reduce excitotoxic insult-induced neuronal damage in the hippocampus and cortex, and the combination could improve the neuroprotective effects (Landucci et al., 2019). Interestingly, Liang et al. (2020) observed that G. biloba extract could enhance ginsenoside uptake by increasing the permeability of blood-brain barrier in rats. According to our previous work, the combination of P. ginseng and G. biloba extracts (CGGE) showed beneficially neuroprotective effects against neuronal apoptosis (Cong et al., 2011). What’s more, CGGE could increase acetylcholine (ACh) and improve neurodegeneration and memory deficiency (Liu et al., 2004). Besides, both panax ginseng and ginkgo biloba are the essential components of SaiLuoTong (SLT), which is an effective treatment for vascular dementia (Jia et al., 2018) to enhance cognitive and cardiovascular function (GZ et al., 2016). However, the neuroprotective effects of CGGE have not been comprehensively studied.
It is more effective for predicting the therapeutic targets and mechanisms of drugs via establishing a network among the compounds, targets, and diseases utilizing various databases and tools (Zhang et al., 2019). Therefore, our present study was designed to use a network analysis approach to explore the therapeutic effects of CGGE on IS. The mechanism of CGGE ameliorating neuroinflammation and excitotoxicity with modulation of NLRP3 inflammasome and CAMK4/CREB pathway were observed in experimental research. The workflow of the study is shown in Figure1.
2 Materials and methods
2.1 Drugs and reagents
Extracts of P. ginseng (NO.090909) and G. biloba (NO.090914) were provided by the Shineway Pharmaceutical Group Co. Ltd. (Hebei, China). Panax ginseng extract was prepared by adding eight times water to the powder of panax ginseng roots and rhizomes, extracting with boiling water for 3 h, then drying with a vacuum rotary evaporator at 80°C (50 g extract from 1 kg P. ginseng). Ultraviolet (UV) spectroscopy or high-performance liquid chromatography (HPLC)-UV method was used to determine the extract’s total active components and main ingredients to control the quality. The quantitative analysis showed that the total ginsenoside content was 77% in ginseng extract, among which ginsenoside Rb1, Rg1, and Re are13.1% (6.55 mg/g), 5.5% (2.75 mg/g), and 3.2% (1.6 mg/g), respectively (Zhang et al., 2018c). Ginkgo biloba extract was obtained by adding six times volume of 70% ethanol to the powder of dry ginkgo biloba leaves, heating and recycling the reflux liquid, then concentration and drying (50 g extract from 1 kg ginkgo biloba leaves). In the extract of ginkgo biloba, total flavonoids accounted for 49%, quercetin, kaempferol, and isorhamnetin occupied 28.7% (14.35 mg/g), ginkgolide A, B, C and bilobalide accounted for 11.6% (5.8 mg/g), and ginkgolide A was 3.3% (1.65 mg/g) (Zhang et al., 2018c). Both ingredient extracts were mixed in a ratio of 1:1. Tat-NR2B9C, 2,3,5-triphenyltetrazolium chloride (TTC), and glutamate assay kits were obtained from Sigma (St Louis, MO, United States). GABA assay kits were purchased from USCN (Wuhan, China). Fluorescent microspheres were purchased from Sheng Yi Yao Technology Development Co., Ltd. (Beijing, China).
2.2 Animals
Adult male SD (Sprague-Dawley) rats (200–220 g) were provided by the Beijing Vital River Laboratory Animal Technology Co., Ltd. (License No. SCXK (Beijing) 2016-0011). All rats were housed at 25 ± 1°C temperature and 65% ± 5% humidity, with a 12 h light/dark cycle (lights on from 7:00 to 19:00). All rats had free access to food and water. The Ethics Review Committee for Animal Experimentation of Xiyuan Hospital, China Academy of Chinese Medical Sciences, approved the experimental protocols described in this study.
2.3 Microsphere-induced cerebral embolism rat model
Fifty rats were randomly assigned into five groups (n = 10): Sham group, MCE group, Tat-NR2B9C group (7.5 mg/kg), CGGE low group (15 mg/kg), and CGGE high group (30 mg/kg). The MCE rat model was established according to a previously described method (Moriyama et al., 2011). In each group, anesthesia was induced with a 2% sodium pentobarbital intraperitoneally injection (60 mg/kg). The right common carotid artery (CCA) and internal carotid artery (ICA) were occluded with vascular clamps temporarily. Immediately, a syringe with 0.2 ml serum (20 mg of the microsphere with 100–200 μm diameter was suspended in 20 ml serum) was injected into the right external carotid, and the microsphere entered the internal carotid artery from the external carotid artery. After injection, the wound was sutured and disinfected with iodophor alcohol. The rats in the sham group received the same surgical procedure without the insertion of microspheres.
CGGE was administrated before cerebral ischemia surgery and 12 h after operation by oral gavage at the dosage of 15 or 30 mg/kg. The tat-NR2B9C peptide was administered 5 min before ischemia surgery via the tail vein (Srejic et al., 2013). Rats in the sham group received the same volume of saline.
2.4 Neurological behavior scores and 2,3,5-triphenyltetrazolium chloride staining
Behavioral changes were assessed at 2 and 24 h after the operation. Neurologic symptoms were scored according to Longa’s five-point scale (Longa et al., 1989). The scales are as follows: 0, with no neurological deficit; 1, unable to fully extend the forepaw; 2, unable to move linearly or spiral to one side; 3, falling to one side; 4, no spontaneous movement. After 24 h, the rats were anesthetized and decapitated. The brains were frozen at −20°C for 5 min, and 2 mm-thick coronal slices were acquired. Then incubated in 2% TTC at 37°C for 10 min and fixed in 4% paraformaldehyde for 30 min.
2.5 Hematoxylin-eosin staining
HE staining was performed 24 h after cerebral ischemia. Rats were sacrificed after deep anesthetization. Brain tissues were fixed in 4% paraformaldehyde at 4°C for 24 h, dehydrated in gradient alcohols, then embedded in paraffin, and cut into 5 µm thick sections (Zhao et al., 2021). The sections were stained with HE and assessed by a light microscope (Olympus FV1200, Tokyo, Japan).
2.6 Network analysis
2.6.1 Active compounds and drug targets screening
The active compounds and potential targets of CGGE for treating IS were investigated based on network analysis. The Traditional Chinese Medicine Systems Pharmacology Database and Analysis Platform (TCMSP, https://old.tcmsp-e.com/tcmsp.php) was used to identify and screen the active compounds of CGGE with a restriction of oral bioavailability (OB) ≥ 30% and drug-likeness (DL) ≥ 0.18 (Ru et al., 2014). The potential targets were predicted with the TCMSP database, and the reliable targets from the DrugBank database (Wishart et al., 2018) were selected for the collection. Then, the potential targets’ gene symbols were searched in the universal protein resource (UniProt, https://www.uniprot.org/).
2.6.2 Screening of disease targets
The differential expressed genes of IS patients were obtained from Gene Expression Omnibus (GEO, https://www.ncbi.nlm.nih.gov/geo/, Series: GSE22255) database. Genes with p value <0.05 and |Fold change| > 1.2 were considered to be of significant differential expression and IS-related targets.
2.6.3 Construction of protein-protein interaction networks
Referring to our previous method (Jiang et al., 2019), PPI networks of CGGE targets and IS targets were constructed by Cytoscape 3.9.0 software plugin BisoGenet, which integrates six kinds of PPI databases. The databases include Database of Interacting Proteins (DIP™), Biomolecular Interaction Network Database (BIND), Biological General Repository for Interaction Datasets (BioGRID), IntAct Molecular Interaction Database (IntAct), Human Protein Reference Database (HPRD), and Molecular INTeraction database (MINT). Then, the two PPI networks were merged with Cytoscape software. CytoNCA plugin was used to obtain the values of Degree, Closeness, Betweenness, Network, Eigenvector, Local Average Connectivity-based method (LAC), and other parameters. The nodes with more than twice the median degree were chosen as the significant targets. Moreover, the topological characteristics were calculated through degree centrality (DC), betweenness centrality (BC), and closeness centrality (CC) to filter the key targets.
2.6.4 Gene ontology and pathway enrichment analysis
The Database for Annotation, Visualization, and Integrated Discovery (DAVID, https://david.ncifcrf.gov/) was used for GO enrichment analysis, and plugin ClueGO of Cytoscape 3.9.0 software was used to perform Kyoto Encyclopedia of Genes and Genomes (KEGG) pathway analysis. The biological process, cellular component, and molecular function analysis were selected for the bar charts. Pathways with significant changes of p < 0.05 were identified for further analysis.
2.7 Glu and gamma-aminobutyric acid assays
The rats were sacrificed 24 h after cerebral embolism, and the whole brain tissues were removed rapidly. Brain tissues were homogenized and centrifuged based on the product instructions. The contents of Glu and GABA in brain tissue were determined by enzyme-linked immunosorbent assay (ELISA) kits. The results were expressed as the means ± standard deviation.
2.8 Western blotting
The protein concentration was calculated by using bicinchoninic acid (BCA) assay kit (KGI Biotechnology Co. Ltd. China). Identical quantities of protein samples were denatured by protein loading buffer, separated by sodium dodecyl sulfate(SDS)-polyacrylamide gel electrophoresis, then transferred to PVDF membranes (Millipore, Billerica, MA, United States). The PVDF membranes were sealed with 5% bovine serum albumin (BSA) at room temperature for one hour. The following antibodies were incubated at 4°C overnight: anti-CAMK4 (1:1,000, A5304, ABclonal), anti-p-CREB (1:1,000, ab119711, Abcam), anti-CREB1 (1:1,000, A0011, ABclonal), anti-NLRP3 (1:500, DF7438, Affinity Biosciences), anti-ASC (1:500, DF6304, Affinity Biosciences), anti-CASP1 (1:500, AF5418, Affinity Biosciences). The stained blots were detected using an ECL reagent (Thermo Fisher Scientific, MA, United States). Each blot was quantified using Bio-Rad Image Lab™ Version 3.0 software. All the experiments reported in this study were performed three times, and the results were reproducible.
2.9 Real-time quantitative polymerase chain reaction
According to the instructions, total RNA was extracted from the brain tissue using Trizol reagent (Thermo Scientific, United States). The concentration and purity of RNA were determined by a NanoDrop 2000 spectrophotometer (Thermo Scientific, United States). The absorbance ratio (A260/280) of all samples ranged from 1.8 to 2.0, and 2 μg of total RNA was reverse transcribed into cDNA using Prime Script RT Master Mix (Takara, Dalian, China). RT-qPCR was determined using the QuantiFast® SYBR® Green PCR Master Mix (Qiagen, Germany) with specific primers and expression of each sample in Light Cycler®480ⅡReal-time PCR Instrument (Roche, Swiss), which internally normalized against Actb. The PCR primers for Camk4, Creb1, and Actb are as follows: Camk4 forward: 5′-CACAGAAATCAGCCTGGTT-3′ and reverse: 5′- ATCTGCTTCACAGCATCGC-3′; Creb1 forward: 5′- TTCTAGTGCCCAGCAACC-3′ and reverse: 5′-GAGGACGCCATAACAACTC-3′; Actb forward: 5′- CCACCATGTACCCAGGCATT-3′ and reverse: 5′- CGGACTCATCGTACTCCTGC-3′. Relative expression fold change was performed by using the 2−△△Ct method. Each experiment was repeated three times.
2.10 Statistical analysis
The results were expressed as the mean ± standard deviation (SD). The statistical analyses were performed using Statistical Package for the Social Sciences (SPSS) software (version 18.0, IBM, Chicago, IL, United States). One-way analysis of variance (ANOVA) was used to compare all groups’ differences. p < 0.05 was considered statistically significant.
3 Results
3.1 Combination of panax ginseng and ginkgo biloba extracts ameliorated MCE-induced neurological deficits
The infarct volume of rats and neurological scores were determined after the acute treatment of the CGGE. As shown in Figures 2A,B, TTC staining results revealed that the infarct volume in the MCE group was larger than that of the sham group. However, the ischemic lesions in the Tat-NR2B9C and CGGE treatment groups were smaller than in the MCE group.
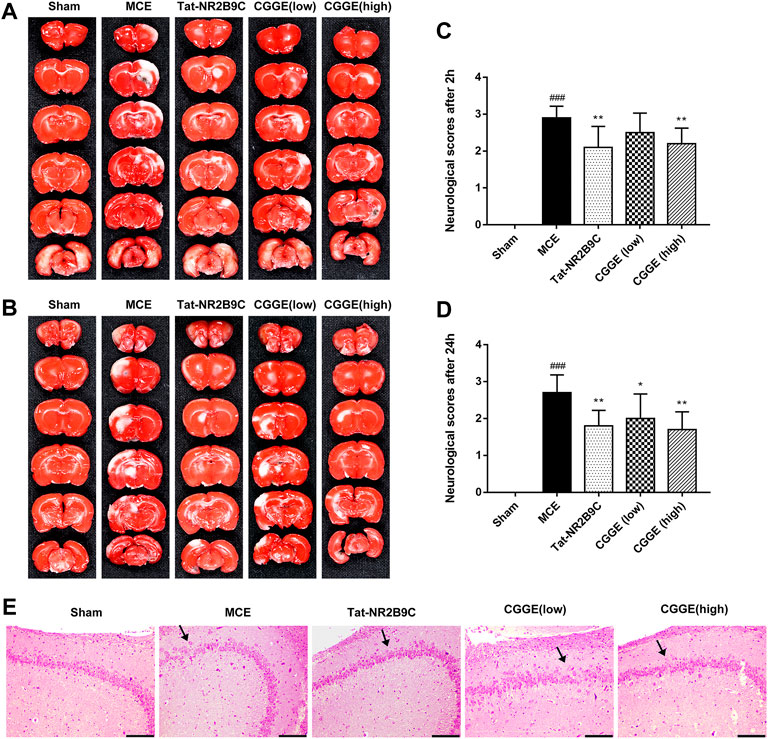
FIGURE 2. CGGE ameliorated MCE-induced neurological deficits. (A,B) TTC staining. The positive side (A) and the negative side (B) of the brain slices. White color indicates “infarcted area.” (C,D) Neurological deficit scores after 2 h (C) and 24 h (D). All data were expressed as mean ± SD, n = 10, ###p < 0.001 vs. Control group, **p < 0.01, *p < 0.05 vs. Model group. (E) HE staining of the hippocampus, scale bar, 200 μm (CA3 × 100).
Figures 2C,D show that the neurological score of the MCE group was significantly higher than that of the sham group. Compared to the MCE group, the Tat-NR2B9C group and the high dosage CGGE group had significantly decreased scores (p < 0.01), while the low dosage of CGGE group could only decrease the scores after 24 h (p < 0.05).
Figure 2Eshows the histological changes of brain neurons with HE staining. HE stained hippocampal area (CA3) showed neuron loss, brain edema, and cell swelling. Several apoptotic neurons, such as nuclear pyknosis, intercellular space, and debris, were observed after the operation. Therefore, these results suggested that CGGE could attenuate brain injury induced by cerebral ischemia with a dose-dependent.
3.2 Compound-target network analysis
The network analysis approach was used to elucidate the active components and mechanisms of the CGGE against IS. The chemical constituents of CGGE were obtained from the TCMSP database (Version: 2.3) with screening parameters of OB ≥ 30% and DL ≥ 0.18. Twenty-two compounds of Panax ginseng and 27 compounds of Ginkgo biloba were obtained. A total of 34 components were characterized after removing the compounds without targets and repetitions (Table 1). The total number of potential targets for 34 compounds was 133. The compound-target network of CGGE is shown in Figure 3.
The network contained 169 nodes and 468 edges which indicated the compound-target interactions. The median degree of 34 chemical components is 9, indicating that compounds could interact with multiple targets. The information on the top 10 chemical components and targets is shown in Table 2. The higher the degree, the more important the compound was in the network. According to the results, the important chemical compounds of CGGE included quercetin, kaempferol, beta-stigmasterol, stigmasterol, luteolin, and the crucial targets of CGGE were PTGS2, NCOA2, PTGS1, CALM1, DPP4, and SCN5A.
3.3 Identification of candidate targets for combination of panax ginseng and ginkgo biloba extracts against ischemic stroke
The reliability of the test results can be significantly improved by directly selecting the human chip results and setting relevant screening conditions for analysis. The GEO database identified two hundred and thirty-nine IS-related targets (Series: GSE22255).
CGGE targets PPI network and IS-related targets PPI network were constructed. 6,275 CGGE target proteins and 5,259 IS-related target proteins were obtained (Supplementary Tables S1, S2). In order to reveal the relationship between CGGE and IS, the PPI networks of CGGE targets and IS-related targets were merged to identify the candidate targets for CGGE against IS. The network consists of 3,494 proteins (Supplementary Tables S3). The median degree of all nodes was 35, and the nodes with more than 70° were identified as significant targets. A network of significant targets for CGGE against IS was constructed, containing 848 proteins (Supplementary Table S4). The common targets were the critical goal of CGGE treatment in IS and the vital targets screened for further research. Subsequently, the topological feature values of common targets in the network, including DC, BC, and CC, were analyzed for the important key targets. Finally, 288 nodes were chosen as the candidate targets with DC > 112.5, BC > 6,455.9, and CC > 0.467 (Figure 4).
3.4 Gene ontology and Kyoto Encyclopedia of Genes and Genomes pathway enrichment analysis
DAVID was used to perform GO analysis of the 288 identified candidate targets. GO of candidate targets was analyzed based on the domains “biological process,” “cellular component,” and “molecular function.” The top 10 terms are shown in Figure 5A. The highly enriched GO terms in biological process, cellular component, and molecular function included negative regulation of gene expression, nucleoplasm, nucleus, cytosol, protein binding, and ubiquitin-protein ligase binding.
In order to further analyze the biological mechanisms involved with the 288 candidate targets, the ClueGO was used to perform the KEGG pathway analysis. As shown in Figure 5B, the mechanisms of CGGE against IS might be concerned with long-term potentiation, cAMP signaling pathway, neurotrophin signaling pathway, and NOD-like receptor signaling pathway.
3.5 Combination of panax ginseng and ginkgo biloba extracts modulated the level of Glu and GABA concentration in ipsilateral cortex
The changes in Glu and GABA levels in the ipsilateral cortex were illustrated in Figure 6 (n= 6). It was found that the Glu level was significantly higher in the MCE group than in the sham group (p < 0.01) (Figure 6A). The Tat-NR2B9C and CGGE treatments significantly reduced the Glu level compared to the MCE group (p < 0.01, p < 0.05, and p < 0.01, respectively). However, no significant differences in the GABA level were observed among all groups (Figure 6B). The ratio of Glu/GABA (Figure 6C) was found to be higher in the MCE group than that in the sham group (p < 0.01). Treatment groups significantly reduced the Glu/GABA ratios compared to the MCE group (p < 0.01).
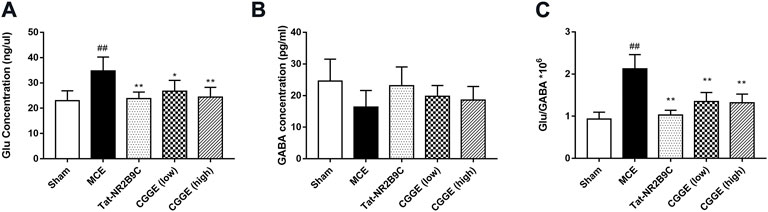
FIGURE 6. CGGE modulated the glutamate and GABA concentration level. (A) The changes in Glu level. (B) The changes in GABA level. (C) Changes in the Glu/GABA ratio. ##p < 0.01 vs. sham group; **p < 0.01, *p < 0.05 vs. MCE group (n = 6).
3.6 Combination of panax ginseng and ginkgo biloba extracts activated CAMK4/ CREB protein in ipsilateral cortex
The effects of CGGE on the CAMK4/CREB are shown in Figure 7A. The protein expression of CAMK4 and p-CREB were decreased following ischemic insult (Figures 7B,C, p < 0.01). CAMK4 and p-CREB expression changes were reversed following the treatments of Tat-NR2B9C and CGGE. The mRNA expression of Camk4 and Creb1 was decreased in the MCE group, whereas it increased with Tat-NR2B9C and the CGGE treatments (Figures 7D,E).
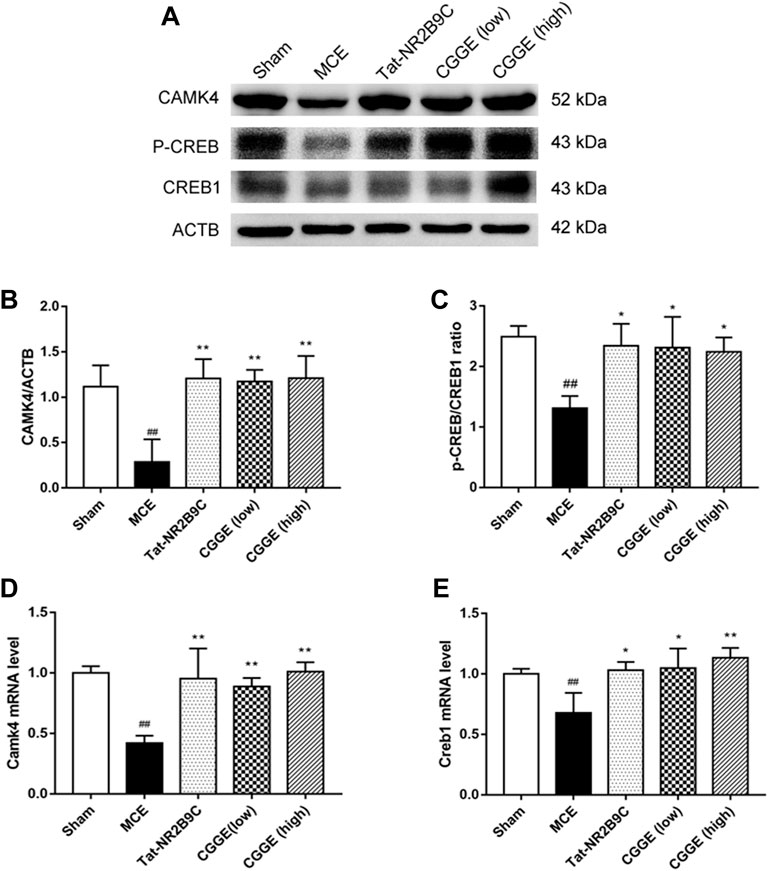
FIGURE 7. CGGE activated CaMKⅣ/CREB in the ipsilateral cortex. (A) Protein expression levels of CAMK4, p-CREB, CREB1. (B,C) The analysis of protein expression of CAMK4, p-CREB/CREB1. (D,E) The mRNA expression of Camk4 and Creb1. (##p < 0.01, vs. sham group; **p < 0.01, *p < 0.05, vs. MCE group) (n = 3).
3.7 Combination of panax ginseng and ginkgo biloba extracts inhibited NLRP3 inflammasome formation in MCE rats
To investigate the potential mechanism by which CGGE alleviates neuroinflammation, we examined the expression of NLRP3 inflammasome in the ipsilateral cortex (n = 3). Results are shown in Figure 8. The protein expression of NLRP3, ASC, and CASP1 were significantly increased after cerebral ischemia (p < 0.01). Compared to the MCE group, CGGE significantly decreased the expression of NLRP3, ASC, and CASP1 proteins. In addition, CGGE exhibited concentration-dependent inhibition of the protein expression.
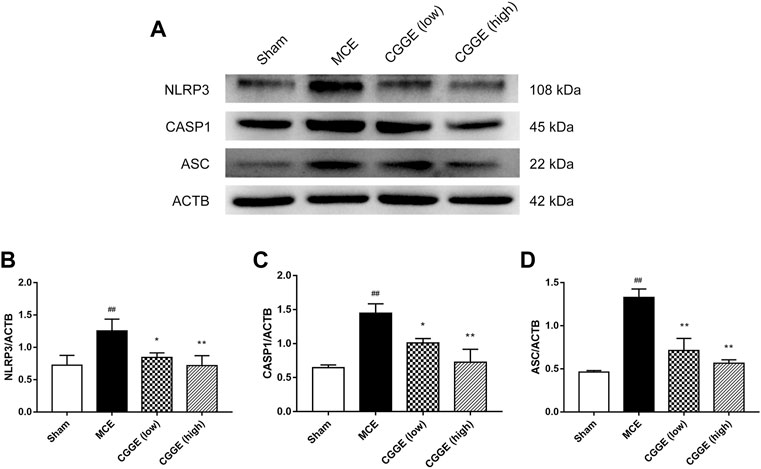
FIGURE 8. CGGE inhibited NLRP3 Inflammasome in ipsilateral cortex. (A) Protein expression of NLRP3, CASP1, and ASC. (B,C,D) The analysis of protein expression of NLRP3, CASP1, and ASC. (##p < 0.01, vs. sham group; **p < 0.01, *p < 0.05, vs. MCE group) (n = 3).
4 Discussion
The present study demonstrated that CGGE could reduce the infarct size and neurological deficits via alleviating excitotoxicity and neuroinflammation in MCE rats. Glu and GABA are the central nervous system’s principal excitatory and inhibitory neurotransmitters. Excessive glutamate overstimulates the ionic glutamate receptor and causes excessive calcium influx, resulting in excitotoxicity to neurons, which is one of the major reasons for neuronal dysfunction and degeneration (Lau and Tymianski, 2010). The results revealed that CGGE could maintain glutamate and GABA balance via activating CAMK4/CREB pathway, which plays a vital role in maintaining normal neural function and reducing secondary brain injury. In addition, CGGE also showed an anti-inflammation effect by inhibiting the activity of NLRP3 inflammasome.
Network pharmacology analysis is a new method that has been applied to predict the pharmacological mechanism of TCM (Li and Zhang, 2013). The potential mechanisms of CGGE against IS-mediated neurotoxicity and neuroinflammation were explored using a network analysis approach. Thirty-four active compounds of CGGE and 133 targets were used to construct a compound-target network. Results suggested that PTGS2, PTGS1, NCOA2, CALM1, DPP4, and SCN5A might be the critical targets of CGGE. CALM1 is the encoding gene of calmodulin (CaM). CaM is related to the calcium signal transduction pathways and is crucial in controlling cellular functions throughout all stages (O'Day et al., 2020). Therefore, CaM might be the key target of CGGE for treating IS. CaM is a Ca2+ multifunctional binding protein. Ca2+/CaM binding modulates various proteins, including CAMKs (Cohen et al., 2016) and cAMP (Lukyanenko et al., 2016). Ca2+ influx triggers phosphorylation of CREB, which binds to a critical Ca2+ response element (CRE) (Tao et al., 1998). CRE could activate the transcription of BDNF. The release of BDNF stimulates TrkB receptors on GABAergic interneurons and increases the input of GABAergic neural precursors, thus stimulating their differentiation and maturation into neurons to balance the excitotoxicity of glutamate (Waterhouse et al., 2012). Ca2+/CaM activates CaM kinases (CaMKs) and CREB kinases from the surface membrane to the nucleus, which is important for synaptic potentiation, learning, and memory (Zhang et al., 2016). In various CaMKs, CaMK4 is thought to phosphorylate CREB in the nuclei (Impey et al., 2002). Interestingly, the results suggested that CGGE may play a neuroprotective role by activating CaMK4 and CREB.
CREB is a crucial transcription factor, and the inactivation of CREB leads to the inhibition of many CREs promoters, including neurotrophic factors and synaptic proteins. The activity of CREB is regulated by phosphorylation and dephosphorylation, and many protein kinases can phosphorylate CREB, such as protein kinase A (PKA) (Dash et al., 1991), mitogen-activated protein kinase (MAPK) (Xing et al., 1996), phosphoinositide-3-kinase (PI3K)-Akt (Zhang W. et al., 2018), and CaMKs (Sheng et al., 1991). CaM is involved in 43 signaling pathways in the KEGG database, while CaMKⅣ is associated with 13 signaling pathways (Figure 9). There were ten common signaling pathways for CaM and CAMK4: long-term potentiation, cAMP signaling pathway, neurotrophin signaling pathway, and calcium signaling pathway. Accordingly, the CGGE targets PPI network and IS-related targets PPI network were structured and merged, and 288 targets were finally identified to elucidate the underlying neuroprotective mechanisms of CGGE. In the KEGG pathway enrichment analysis, long-term potentiation, cAMP signaling pathway, and neurotrophin signaling pathway are closely related to the activation of CREB. The results showed that CGGE might play a role in treating IS by activating CaM and CREB through long-term potentiation, cAMP signaling pathway, and neurotrophin signaling pathway.
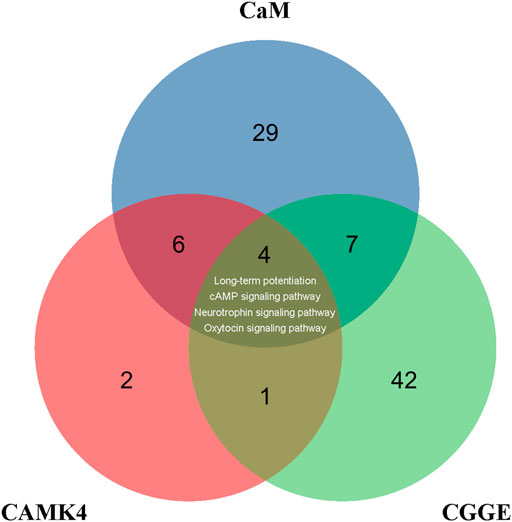
FIGURE 9. Venn diagram depicts the overlap of CaM, CAMK4, and CGGE-involved signaling pathways. Long-term potentiation, cAMP signaling pathway, and neurotrophin signaling pathway are related to the activation of CREB.
Inflammation plays a key role in the pathophysiology of ischemic stroke. NLRP3 inflammasome is the best-characterized inflammasome and one of the major contributors to neuroinflammation, which consists of NLRP3, ASC, and Caspase-1 (Alishahi et al., 2019). The KEGG pathway enrichment analysis showed that the NOD-like receptor signaling pathway was the potential mechanism of CGGE against IS. After cerebral ischemia, the changes in the intracellular microenvironment trigger the NLRP3 inflammasome (NLRP3/ASC/CASPASE-1) activation, which leads to the secretion of IL-1β and IL-18 and finally mediated cell death. Western blot analysis showed that CGGE could downregulate the protein expression of NLRP3 in the ipsilateral cortex. The data suggested that CGGE exerted an anti-inflammatory effect after ischemic stroke by inhibiting NLRP3 activation.
Taken as a whole, the present data suggested that the neuroprotective mechanism of CGGE against IS was related to excitotoxicity and neuroinflammation, which mainly through the long-term potentiation, cAMP signaling pathway, neurotrophin signaling pathway, and NOD-like receptor signaling pathway. However, there are still some limitations. Firstly, the rat model of cerebral ischemia was established by microsphere injection, which prevented an accurate assessment of the infarct sizes. Secondly, we only measured the content of amino acids 24 h after ischemic injury, while the change in amino acid level is a continuous process. More sampling points, especially during the early stage of ischemia, would help better understand the dynamic changes of the neurotransmitters and the related mechanisms. Therefore, further studies are expected to explore the deeper mechanism.
5 Conclusion
In conclusion, the underlying mechanisms of CGGE on IS were explored via the integration of network analysis and in vivo experiments. The results showed that CGGE could improve neurological function via alleviating excitotoxicity and neuroinflammation. According to the network analysis, CALM1 might be the critical target of CGGE. Furthermore, the protective mechanisms of CGGE on ischemic stroke might involve long-term potentiation, cAMP signaling pathway, neurotrophin signaling pathway, and NOD-like receptor signaling pathway. The mechanisms by which CGGE exerts anti-inflammatory and anti-excitotoxicity effects involved the inhibition of NLRP3 inflammasome and upregulation of the CAMK4/CREB pathway.
Data availability statement
The datasets presented in this study can be found in online repositories. The names of the repository/repositories and accession number(s) can be found in the article/Supplementary Material.
Ethics statement
The animal study was reviewed and approved by the Ethics Review Committee for Animal Experimentation of Xiyuan Hospital, China Academy of Chinese Medical Sciences.
Author contributions
YJ, JL, and GZ conceived the idea and designed the research. AZ and NL performed the research and drafted the manuscript. GJ prepared the material. LX and MY conducted the main study of the experiment. YZ helped with TTC staining and western blotting. BX and YF were responsible for data analysis. BM and DC assisted in preparing and revising the manuscript. All authors have read and approved the final version.
Funding
This study was finically supported by the National Basic Research Program, China (973 program) (No.2015CB554405) and the National Natural Science Foundation, China (NSFC 82030124, NSFC 81873041).
Conflict of interest
NL was employed by the company Beijing Increasepharm Safety and Efficacy Co., Ltd. GJ was employed by Shineway Pharmaceutical Group Co., Ltd.
The remaining authors declare that the research was conducted in the absence of any commercial or financial relationships that could be construed as a potential conflict of interest.
Publisher’s note
All claims expressed in this article are solely those of the authors and do not necessarily represent those of their affiliated organizations, or those of the publisher, the editors and the reviewers. Any product that may be evaluated in this article, or claim that may be made by its manufacturer, is not guaranteed or endorsed by the publisher.
Supplementary material
The Supplementary Material for this article can be found online at: https://www.frontiersin.org/articles/10.3389/fphar.2022.980449/full#supplementary-material
References
Alishahi, M., Farzaneh, M., Ghaedrahmati, F., Nejabatdoust, A., Sarkaki, A., and Khoshnam, S. E. (2019). NLRP3 inflammasome in ischemic stroke: As possible therapeutic target. Int. J. Stroke 14, 574–591. doi:10.1177/1747493019841242
Bell, K. F., Bent, R. J., Meese-Tamuri, S., Ali, A., Forder, J. P., and Aarts, M. M. (2013). Calmodulin kinase IV-dependent CREB activation is required for neuroprotection via NMDA receptor-PSD95 disruption. J. Neurochem. 126, 274–287. doi:10.1111/jnc.12176
Boddum, K., Jensen, T. P., Magloire, V., Kristiansen, U., Rusakov, D. A., Pavlov, I., et al. (2016). Astrocytic GABA transporter activity modulates excitatory neurotransmission. Nat. Commun. 7, 13572. doi:10.1038/ncomms13572
Cohen, S. M., Ma, H., Kuchibhotla, K. V., Watson, B. O., Buzsáki, G., Froemke, R. C., et al. (2016). Excitation-transcription coupling in parvalbumin-positive interneurons employs a novel CaM kinase-dependent pathway distinct from excitatory neurons. Neuron 90, 292–307. doi:10.1016/j.neuron.2016.03.001
Collaborators, G. D. a. I. (2020). Global burden of 369 diseases and injuries in 204 countries and territories, 1990-2019: A systematic analysis for the global burden of disease study 2019. Lancet 396, 1204–1222. doi:10.1016/S0140-6736(20)30925-9
Cong, W., Sheng, L., Li, Y., Li, P., Lin, C., and Liu, J. (2011). Protective effects of ginseng-ginko extracts combination on rat primary cultured neurons induced by Abeta(1-40). China J. Chin. materia medica 36, 908–911.
Dash, P. K., Karl, K. A., Colicos, M. A., Prywes, R., and Kandel, E. R. (1991). cAMP response element-binding protein is activated by Ca2+/calmodulin- as well as cAMP-dependent protein kinase. Proc. Natl. Acad. Sci. U. S. A. 88, 5061–5065. doi:10.1073/pnas.88.11.5061
Fann, D. Y., Lim, Y. A., Cheng, Y. L., Lok, K. Z., Chunduri, P., Baik, S. H., et al. (2018). Evidence that NF-κB and MAPK signaling promotes NLRP inflammasome activation in neurons following ischemic stroke. Mol. Neurobiol. 55, 1082–1096. doi:10.1007/s12035-017-0394-9
Feng, Z. L., Sun, Q., Chen, W., Bai, Y., Hu, D. H., and Xie, X. (2019). The neuroprotective mechanisms of ginkgolides and bilobalide in cerebral ischemic injury: A literature review. Mol. Med. 25, 57. doi:10.1186/s10020-019-0125-y
Gz, S., Alan, Y., Jian, L., David, C., Frances, B., Andrew, P., et al. (2016). The effect of sailuotong (SLT) on neurocognitive and cardiovascular function in healthy adults: A randomised, double-blind, placebo controlled crossover pilot trial. BMC Complement. Altern. Med. 16, 15. doi:10.1186/s12906-016-0989-0
Impey, S., Fong, A. L., Wang, Y., Cardinaux, J. R., Fass, D. M., Obrietan, K., et al. (2002). Phosphorylation of CBP mediates transcriptional activation by neural activity and CaM kinase IV. Neuron 34, 235–244. doi:10.1016/s0896-6273(02)00654-2
Jia, J., Wei, C., Chen, S., Li, F., Tang, Y., Qin, W., et al. (2018). Efficacy and safety of the compound Chinese medicine SaiLuoTong in vascular dementia: A randomized clinical trial. Alzheimers Dement. 4, 108–117. doi:10.1016/j.trci.2018.02.004
Jiang, Y., Liu, N., Zhu, S., Hu, X., Chang, D., and Liu, J. (2019). Elucidation of the mechanisms and molecular targets of yiqi shexue formula for treatment of primary immune thrombocytopenia based on network pharmacology. Front. Pharmacol. 10, 1136. doi:10.3389/fphar.2019.01136
Jin, M., Kim, K. M., Lim, C., Cho, S., and Kim, Y. K. (2022). Neuroprotective effects of Korean White ginseng and Red ginseng in an ischemic stroke mouse model. J. Ginseng Res. 46, 275–282. doi:10.1016/j.jgr.2021.06.012
Kim, K. H., Lee, D., Lee, H. L., Kim, C. E., Jung, K., and Kang, K. S. (2018). Beneficial effects of panax ginseng for the treatment and prevention of neurodegenerative diseases: Past findings and future directions. J. Ginseng Res. 42, 239–247. doi:10.1016/j.jgr.2017.03.011
Landucci, E., Pellegrini-Giampietro, D. E., Bilia, A. R., and Bergonzi, M. C. (2019). Enhanced neuroprotective effects of panax ginseng G115(®) and ginkgo biloba GK501(®) combinations in vitro models of excitotoxicity. Int. J. Mol. Sci. 20, E5872. doi:10.3390/ijms20235872
Lau, A., and Tymianski, M. (2010). Glutamate receptors, neurotoxicity and neurodegeneration. Pflugers Arch. 460, 525–542. doi:10.1007/s00424-010-0809-1
Li, S., and Zhang, B. (2013). Traditional Chinese medicine network pharmacology: Theory, methodology and application. Chin. J. Nat. Med. 11, 110–120. doi:10.1016/S1875-5364(13)60037-0
Liang, W., Xu, W., Zhu, J., Zhu, Y., Gu, Q., Li, Y., et al. (2020). Ginkgo biloba extract improves brain uptake of ginsenosides by increasing blood-brain barrier permeability via activating A1 adenosine receptor signaling pathway. J. Ethnopharmacol. 246, 112243. doi:10.1016/j.jep.2019.112243
Liu, J. X., Cong, W. H., Xu, L., and Wang, J. N. (2004). Effect of combination of extracts of ginseng and ginkgo biloba on acetylcholine in amyloid beta-protein-treated rats determined by an improved HPLC. Acta Pharmacol. Sin. 25, 1118–1123.
Longa, E. Z., Weinstein, P. R., Carlson, S., and Cummins, R. (1989). Reversible middle cerebral artery occlusion without craniectomy in rats. Stroke 20, 84–91. doi:10.1161/01.str.20.1.84
Lukyanenko, Y. O., Younes, A., Lyashkov, A. E., Tarasov, K. V., Riordon, D. R., Lee, J., et al. (2016). Ca(2+)/calmodulin-activated phosphodiesterase 1A is highly expressed in rabbit cardiac sinoatrial nodal cells and regulates pacemaker function. J. Mol. Cell. Cardiol. 98, 73–82. doi:10.1016/j.yjmcc.2016.06.064
Mdzinarishvili, A., Sumbria, R., Lang, D., and Klein, J. (2012). Ginkgo extract EGb761 confers neuroprotection by reduction of glutamate release in ischemic brain. J. Pharm. Pharm. Sci. 15, 94–102. doi:10.18433/j3ps37
Moriyama, Y., Takagi, N., and Tanonaka, K. (2011). Intravenous injection of neural progenitor cells improved depression-like behavior after cerebral ischemia. Transl. Psychiatry 1, e29. doi:10.1038/tp.2011.32
O'day, D. H., Mathavarajah, S., Myre, M. A., and Huber, R. J. (2020). Calmodulin-mediated events during the life cycle of the amoebozoan Dictyostelium discoideum. Biol. Rev. Camb. Philos. Soc. 95, 472–490. doi:10.1111/brv.12573
O'donnell, M. J., Chin, S. L., Rangarajan, S., Xavier, D., Liu, L., Zhang, H., et al. (2016). Global and regional effects of potentially modifiable risk factors associated with acute stroke in 32 countries (INTERSTROKE): A case-control study. Lancet 388, 761–775. doi:10.1016/S0140-6736(16)30506-2
Ong, W. Y., Farooqui, T., Koh, H. L., Farooqui, A. A., and Ling, E. A. (2015). Protective effects of ginseng on neurological disorders. Front. Aging Neurosci. 7, 129. doi:10.3389/fnagi.2015.00129
Park, H., and Poo, M. M. (2013). Neurotrophin regulation of neural circuit development and function. Nat. Rev. Neurosci. 14, 7–23. doi:10.1038/nrn3379
Reay, J. L., Van Schaik, P., and Wilson, C. J. (2019). A systematic review of research investigating the physiological and psychological effects of combining Ginkgo biloba and Panax ginseng into a single treatment in humans: Implications for research design and analysis. Brain Behav. 9, e01217. doi:10.1002/brb3.1217
Ru, J., Li, P., Wang, J., Zhou, W., Li, B., Huang, C., et al. (2014). Tcmsp: A database of systems pharmacology for drug discovery from herbal medicines. J. Cheminform. 6, 13. doi:10.1186/1758-2946-6-13
Savage, C. D., Lopez-Castejon, G., Denes, A., and Brough, D. (2012). NLRP3-Inflammasome activating DAMPs stimulate an inflammatory response in glia in the absence of priming which contributes to brain inflammation after injury. Front. Immunol. 3, 288. doi:10.3389/fimmu.2012.00288
Sheng, M., Thompson, M. A., and Greenberg, M. E. (1991). Creb: A Ca(2+)-regulated transcription factor phosphorylated by calmodulin-dependent kinases. Science 252, 1427–1430. doi:10.1126/science.1646483
Srejic, L. R., Hutchison, W. D., and Aarts, M. M. (2013). Uncoupling PSD-95 interactions leads to rapid recovery of cortical function after focal stroke. J. Cereb. Blood Flow. Metab. 33, 1937–1943. doi:10.1038/jcbfm.2013.153
Swanson, K. V., Deng, M., and Ting, J. P. (2019). The NLRP3 inflammasome: Molecular activation and regulation to therapeutics. Nat. Rev. Immunol. 19, 477–489. doi:10.1038/s41577-019-0165-0
Tao, X., Finkbeiner, S., Arnold, D. B., Shaywitz, A. J., and Greenberg, M. E. (1998). Ca2+ influx regulates BDNF transcription by a CREB family transcription factor-dependent mechanism. Neuron 20, 709–726. doi:10.1016/s0896-6273(00)81010-7
Traynelis, S. F., Wollmuth, L. P., Mcbain, C. J., Menniti, F. S., Vance, K. M., Ogden, K. K., et al. (2010). Glutamate receptor ion channels: Structure, regulation, and function. Pharmacol. Rev. 62, 405–496. doi:10.1124/pr.109.002451
Virani, S. S., Alonso, A., Benjamin, E. J., Bittencourt, M. S., Callaway, C. W., Carson, A. P., et al. (2020). Heart disease and stroke statistics-2020 update: A report from the American heart association. Circulation 141, e139–e596. doi:10.1161/CIR.0000000000000757
Waterhouse, E. G., An, J. J., Orefice, L. L., Baydyuk, M., Liao, G. Y., Zheng, K., et al. (2012). BDNF promotes differentiation and maturation of adult-born neurons through GABAergic transmission. J. Neurosci. 32, 14318–14330. doi:10.1523/JNEUROSCI.0709-12.2012
Wishart, D. S., Feunang, Y. D., Guo, A. C., Lo, E. J., Marcu, A., Grant, J. R., et al. (2018). DrugBank 5.0: A major update to the DrugBank database for 2018. Nucleic Acids Res. 46, D1074–d1082. doi:10.1093/nar/gkx1037
Xing, J., Ginty, D. D., and Greenberg, M. E. (1996). Coupling of the RAS-MAPK pathway to gene activation by RSK2, a growth factor-regulated CREB kinase. Science 273, 959–963. doi:10.1126/science.273.5277.959
Xu, Q., Zhao, B., Ye, Y., Li, Y., Zhang, Y., Xiong, X., et al. (2021). Relevant mediators involved in and therapies targeting the inflammatory response induced by activation of the NLRP3 inflammasome in ischemic stroke. J. Neuroinflammation 18, 123. doi:10.1186/s12974-021-02137-8
Yang, Z. Z., Li, J., Li, S. X., Feng, W., and Wang, H. (2011). Effect of ginkgolide B on striatal extracellular amino acids in middle cerebral artery occluded rats. J. Ethnopharmacol. 136, 117–122. doi:10.1016/j.jep.2011.04.027
Zhang, R., Zhu, X., Bai, H., and Ning, K. (2019). Network pharmacology databases for traditional Chinese medicine: Review and assessment. Front. Pharmacol. 10, 123. doi:10.3389/fphar.2019.00123
Zhang, W., Song, J. K., Yan, R., Li, L., Xiao, Z. Y., Zhou, W. X., et al. (2018a). Diterpene ginkgolides protect against cerebral ischemia/reperfusion damage in rats by activating Nrf2 and CREB through PI3K/Akt signaling. Acta Pharmacol. Sin. 39, 1259–1272. doi:10.1038/aps.2017.149
Zhang, X., Shi, M., Bjoras, M., Wang, W., Zhang, G., Han, J., et al. (2013). Ginsenoside Rd promotes glutamate clearance by up-regulating glial glutamate transporter GLT-1 via PI3K/AKT and ERK1/2 pathways. Front. Pharmacol. 4, 152. doi:10.3389/fphar.2013.00152
Zhang, Y., Lin, R., Tao, J., Wu, Y., Chen, B., Yu, K., et al. (2016). Electroacupuncture improves cognitive ability following cerebral ischemia reperfusion injury via CaM-CaMKIV-CREB signaling in the rat hippocampus. Exp. Ther. Med. 12, 777–782. doi:10.3892/etm.2016.3428
Zhang, Y., Liu, J., Yang, B., Zheng, Y., Yao, M., Sun, M., et al. (2018b). Ginkgo biloba extract inhibits astrocytic lipocalin-2 expression and alleviates neuroinflammatory injury via the JAK2/STAT3 pathway after ischemic brain stroke. Front. Pharmacol. 9, 518. doi:10.3389/fphar.2018.00518
Zhang, Y., Miao, L., Lin, L., Ren, C. Y., Liu, J. X., and Cui, Y. M. (2018c). Repeated administration of Sailuotong, a fixed combination of Panax ginseng, Ginkgo biloba, and Crocus sativus extracts for vascular dementia, alters CYP450 activities in rats. Phytomedicine. 38, 125–134. doi:10.1016/j.phymed.2017.02.007
Zhao, A., Ma, B., Xu, L., Yao, M., Zhang, Y., Xue, B., et al. (2021). Jiedu tongluo granules ameliorates post-stroke depression rat model via regulating NMDAR/BDNF signaling pathway. Front. Pharmacol. 12, 662003. doi:10.3389/fphar.2021.662003
Zhou, Z., Lu, J., Liu, W. W., Manaenko, A., Hou, X., Mei, Q., et al. (2018). Advances in stroke pharmacology. Pharmacol. Ther. 191, 23–42. doi:10.1016/j.pharmthera.2018.05.012
Glossary
CGGE combination of panax ginseng and ginkgo biloba extracts
TCM traditional chinese medicine
IS ischemic stroke
MCE microsphere-induced cerebral embolism
TTC 2,3,5-triphenyltetrazolium chloride
HE hematoxylin-eosin
GABA gamma-aminobutyric acid
Glu glutamate
ELISA enzyme linked immunosorbent assay
RT-qPCR real-time quantitative polymerase chain reaction
BCA bicinchoninic acid
NMDAR N-methyl-D-aspartate receptor
PSD-95 postsynaptic density-95
SDS sodium dodecyl sulfate
MAPK mitogen-activated protein kinase
CaMKs calmodulin-dependent protein kinases
CaMKⅣ calmodulin-dependent protein kinase type Ⅳ
NLRP3 Nod-like receptor, pyrin containing 3
PKA protein kinase A
PI3K phosphoinositide-3-kinase
CREB cyclic AMP-responsive element-binding protein
BDNF brain-derived neurotrophic factor
CCA common carotid artery
ICA internal carotid artery
SD standard deviation
GEO gene expression omnibus
PPI protein-protein interaction
LAC local average connectivity-based method
DC degree centrality
BC betweenness centrality
CC closeness centrality
GO gene ontology
OB oral bioavailability
DL drug-likeness
HPLC high-performance liquid chromatography
UV Ultraviolet
Keywords: ischemic stroke, ginseng extract, ginkgo biloba extract, NLRP3 inflammasome, CAMK4/CREB, network analysis
Citation: Zhao A, Liu N, Jiang G, Xu L, Yao M, Zhang Y, Xue B, Ma B, Chang D, Feng Y, Jiang Y, Liu J and Zhou G (2022) Combination of panax ginseng and ginkgo biloba extracts attenuate cerebral ischemia injury with modulation of NLRP3 inflammasome and CAMK4/CREB pathway. Front. Pharmacol. 13:980449. doi: 10.3389/fphar.2022.980449
Received: 28 June 2022; Accepted: 03 August 2022;
Published: 25 August 2022.
Edited by:
Han-Li Ruan, Huazhong University of Science and Technology, ChinaCopyright © 2022 Zhao, Liu, Jiang, Xu, Yao, Zhang, Xue, Ma, Chang, Feng, Jiang, Liu and Zhou. This is an open-access article distributed under the terms of the Creative Commons Attribution License (CC BY). The use, distribution or reproduction in other forums is permitted, provided the original author(s) and the copyright owner(s) are credited and that the original publication in this journal is cited, in accordance with accepted academic practice. No use, distribution or reproduction is permitted which does not comply with these terms.
*Correspondence: Yunyao Jiang, yyjiang@tsinghua.edu.cn; Jianxun Liu, liujx0324@sina.com; Guoping Zhou, doctorzgp@sina.com
†These authors have contributed equally to this work and share first authorship