- 1Guangxi Key Laboratory of Tumor Immunology and Microenvironmental Regulation, Guilin Medical University, Guilin, China
- 2School of Public Health, Guilin Medical University, Guilin, China
- 3State Key Laboratory for Chemistry and Molecular Engineering of Medicinal Resources, Collaborative Innovation Center for Guangxi Ethnic Medicine, School of Chemistry and Pharmaceutical Sciences, Guangxi Normal University, Guilin, China
Metal compounds, especially gold complexes, have recently gained increasing attention as possible lung cancer therapeutics. Some gold complexes display not only excellent activity in cisplatin-sensitive lung cancer but also in cisplatin-resistant lung cancer, revealing promising prospects in the development of novel treatments for lung cancer. This review summarizes examples of anticancer gold(I) and gold (III) complexes for lung cancer treatment, including mechanisms of action and approaches adopted to improve their efficiency. Several excellent examples of gold complexes against lung cancer are highlighted.
Introduction
Cancer, especially lung cancer, ranks as a leading cause of death worldwide (Boshoff et al., 2018; Cheng et al., 2020; Boshoff et al., 2021; Hanna and Miller, 2021; Heist et al., 2021; Tan et al., 2021). The 2020 global cancer statistics covering 185 countries show that lung cancer remained the leading cause of cancer mortality, with an estimated 1.8 million deaths (18% of all cancer) (Bray et al., 2021). Thus, efforts to develop novel anti-lung cancer drugs and strategies are critical for the global control of this disease.
Metal compounds, especially gold complexes, have recently gained increasing attention in the design of lung cancer therapeutics (Grapperhaus et al., 2020; Akbarsha et al., 2021; Zhang et al., 2021a; Chao et al., 2021; Daher et al., 2021). Gold complexes are a class of compounds formed by ligand coordination with gold(I) or gold (III) ions, which contain nitrogen, phosphorus, sulfur, carbon, and other atoms. The geometric configuration of gold complexes is changeable, mostly in compositions of two, three, and four ligands (Che et al., 2017; Bourissou et al., 2020; Gornitzka et al., 2020; Ott et al., 2020; Zou et al., 2020, 2021, 2022). Gold complexes also have unique electronic structures and changeable redox states responsible for their excellent catalytic performance, rich optical properties, and notable biological activities. Therefore, they have broad application prospects as catalysts, optical materials, protein inhibitors, and anticancer drugs (Dou et al., 2010; Pizarro et al., 2018; Arambula et al., 2020; Liu et al., 2020; Dos et al., 2021; Rodriguez-Yoldi et al., 2021). With the discovery of auranofin’s anticancer activity (Figure 1) (Abdalbari and Telleria, 2021), a new chapter exploring gold complexes as anticancer drugs was opened. Researchers have successively modified and improved the ligands bound to gold ions and synthesized many gold complexes with anticancer activity, including gold(I) and gold (III) (Liu et al., 2022).
In recent years, gold complexes have gained increasing attention in the search for lung cancer treatments. Many anti-lung cancer gold(I) and gold (III) complexes have been designed and synthesized for lung cancer treatments (Fregona et al., 2018; Bostancıoğlu et al., 2019; Alonso et al., 2020; Gianneschi et al., 2021; Pawlak et al., 2021). These complexes contain multidentate N-donor, cyclometalating, dithiocarbamate, triazole–peptide, N-heterocyclic carbine (NHC), thiourea, alkynyl, thiolate, phosphine, or other ligands (Eichler et al., 2014; Kühn et al., 2017; Fereidoonnezhad et al., 2020; Marchetti et al., 2022). This review summarizes the development of gold complexes as anti-lung cancer agents, including their mechanisms against lung cancer and the approaches adopted to improve their anti-lung cancer efficiency. In particular, several examples which exhibit excellent anti-lung cancer effects in vivo are highlighted.
Anti-lung cancer gold(I) complexes
Auranofin, a well-known gold(I)–phosphine–thiolate complex, was developed to treat rheumatoid arthritis in a clinical setting (Furst, 1983; Avery et al., 1984; Shea et al., 2000; Kast, 2010; Choi et al., 2021). Recently, auranofin was evaluated as an agent to inhibit the growth of different cancers (Fang et al., 2018; Kawada et al., 2019; Wang et al., 2020; Abdalbari and Telleria, 2021; Kawada et al., 2021), including lung cancer (IC50 < 2 μM, for A549). Research showed that auranofin is an effective selective inhibitor of thioredoxin reductase (TrxR) by forming a linear Scys-AuI-Scys coordination bond with TrxR (Figure 2) (Bellelli et al., 2009; Colotti et al., 2012). It can induce the generation of reactive oxygen species (ROS) and activate p38 mitotic activated protein kinase (p38 MAPK) (Park and Kim, 2005). The latest research found that auranofin may exert its anticancer effect by inhibiting proteasome-associated deubiquitinases (DUBs). Liang and co-workers found that inhibiting TrxR would lead to 36% Cys oxidation of 606 Cys-containing peptides. These studies prove that auranofin can effectively induce oxidation of Cys peptides and inhibit TrxR activity (Jones et al., 2013; Liu et al., 2014). The success of auranofin as a cancer treatment has aroused the interest of pharmaceutical chemists in other gold(I) complexes (Liu et al., 2022).
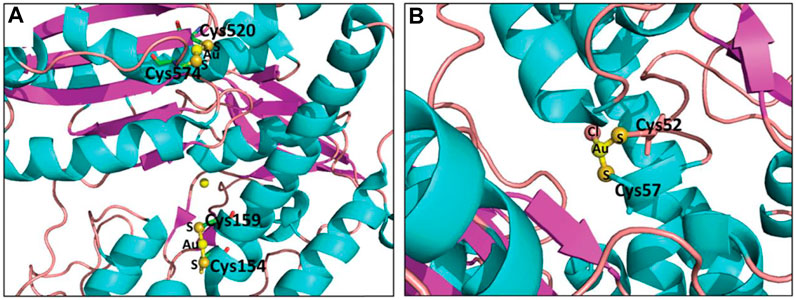
FIGURE 2. Crystal structure of gold(I)-protein conjugates: (A) Au(I)-TGR conjugates (PDB code: 3H4K, gold source: auranofin). (B) Au(I)-TR conjugates (PDB code: 2YAU, gold source: auranofin). Reproduced with permission (Bellelli et al., 2009; Colotti et al., 2012).
Anti-lung cancer gold(I)-NHC complexes
Kühn and co-workers investigated gold(I)-NHC complex 1. After a 48 h treatment, complex one showed high IC50-values in an A549 cell line, which may be due to the low solubility of complex one in aqueous medium (Kühn et al., 2017). Arumugam and co-workers investigated ferrocenylated N-heterocyclic carbene-supported gold(I) complex 2, the cytotoxicity of 2 was found to be tenfold greater than auranofin in the A549 cell line (Arumugam et al., 2016). In addition, Arumugam and co-workers found that ferrocene significantly enhanced the cytotoxicity of gold(I)-NHC complexes in the A549 cell line. Although the in vivo anti-lung cancer activity of complex 2 was not described in this literature, Arumugam and co-workers have confirmed that complex 2 kills A549 cells by a dual-mode of action, inducing ROS generation (Figures 3A,B) and TrxR inhibition (Figure 3C).
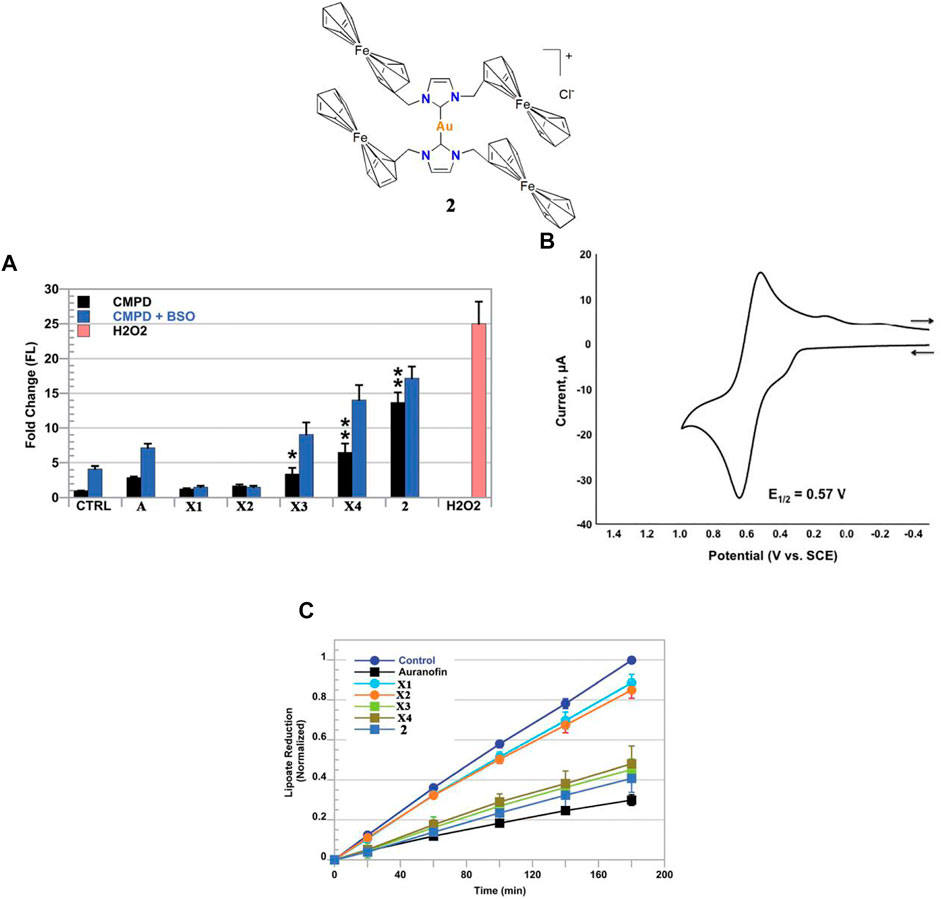
FIGURE 3. (A) ROS detected by fluorescent signal increases DCF via flow cytometric analysis in live A549 cells treated with complex 2. H2O2 was used as a positive control. (B) CV (100 mV s−1 scan rate) and DPV (50 mV pulse amplitude) of complex 2. (C) Time-dependent inhibition of thioredoxin reductase (TrxR) via the reduction of lipoate (*p < 0.05; **p < 0.01). Reproduced with permission (Arumugam et al., 2016).
In 2017 Arambula and co-workers investigated a gold(I)-NHC complex 3 with excellent cytotoxicity in the A549 cell line; the IC50 value was 0.07 μM (Arambula et al., 2017). Zebrafish-A549 embryos as a tumor xenograft model were treated with complex 3 at a concentration of 0.5 μM for 72 h. Tumor inhibition was determined by acridine orange staining. Apoptotic cells show a significant increase in yellow or orange color. At a dose of 0.5 μM, complex 3 displayed low toxicity and excellent tumor inhibition activity (Figure 4A), indicating it is worthwhile to consider it for further study as an anti-lung cancer agent. Like complex 2, the anticancer mechanism of complex 3 is mainly via ROS generation by redox cycling (Figure 4B) and TrxR inhibition.
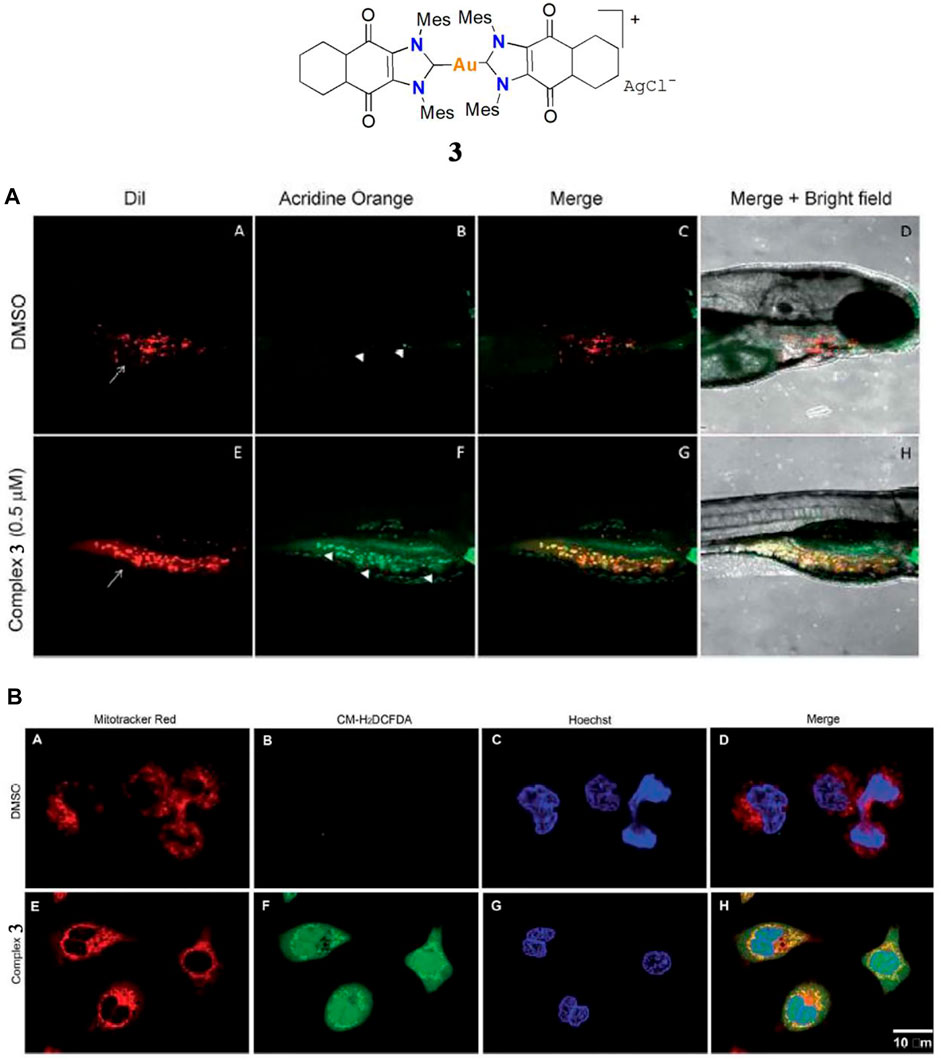
FIGURE 4. (A) Complex 3 against A549 cells in a Zebrafish tumor xenograft model. (B) Confocal microscopy images of mitochondria ROS generation after treatment with 1.25 μM of complex 3 in the A549 cell line. Reproduced with permission (Arambula et al., 2017).
Arambula and co-workers modified a gold(I)-NHC complex 4 in 2021 (Figure 5A), which could also be effectively tracked by confocal microscopy (Figure 5B) (Arambula et al., 2021). Intracellular tracking experiments showed that complex 4 accumulated in the mitochondria, causing oxidative stress and promoting ROS production. In addition, human serum albumin can significantly enhance the solubility of complex 4 in water. Studies focused on the rationally designed gold(I)-NHC complex via non-covalent binding with serum proteins for tumor targeting. The resulting complex 4 retained strong cytotoxicity and a therapeutic effect.
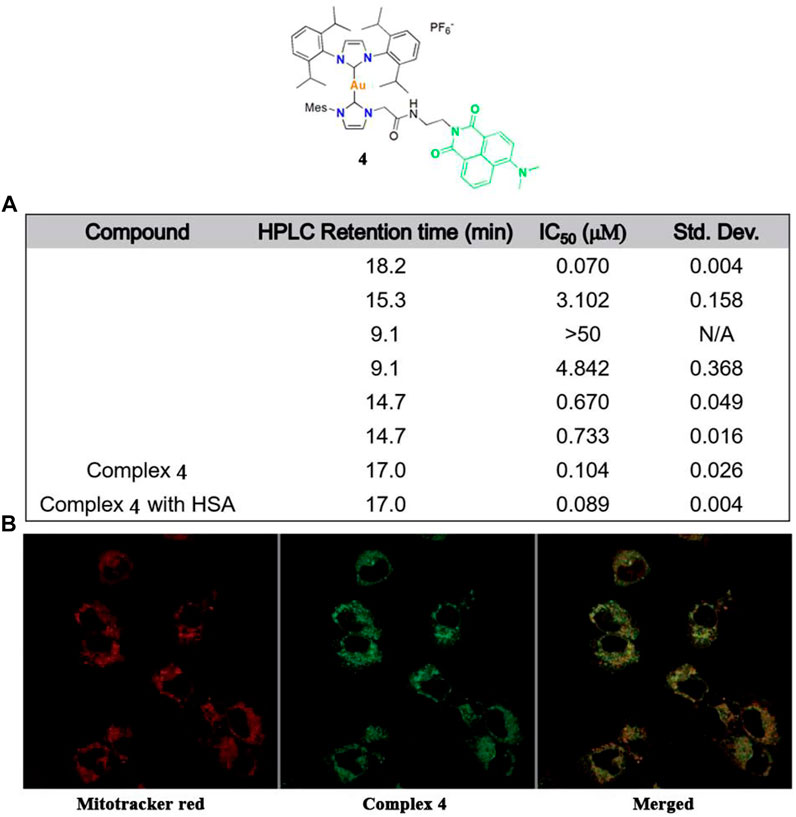
FIGURE 5. (A) Cytotoxicity values (IC50, μM) of complex 4 against A549 cells by MTT assays. (B) Confocal microscopy images obtained after treatment with complex 4 in the A549 cell line. Reproduced with permission (Arambula et al., 2021).
Anti-lung cancer gold(I)-sulfur complexes
Auranofin, gold(I)-sulfur complexes have been studied as anticancer complexes for more than 20 years (Avarvari et al., 2008; Silva et al., 2016; de Almeida et al., 2017; Du et al., 2017; Gimeno et al., 2018; Liu et al., 2020). Recently, these gold(I)-sulfur complexes have been investigated as lung cancer treatments. Fereidoonnezhad and co-workers reported a novel gold(I)-sulfur complex 5 that appeared to have moderate anti-proliferative effects in the A549 cell line (Fereidoonnezhad et al., 2020). The mechanism of action is slightly different from that previously reported in that molecular docking analysis confirmed that complex 5 affected A549 cells by inhibiting TrxR and intercalation of DNA. Dhuna and co-workers reported a gold(I)-sulfur complex 6 (Dhuna et al., 2015); however, the mechanism of action was not reported in this case.
Complex 7, reported by Korashy and co-workers, exhibited much better anti-lung cancer potency than cisplatin (Korashy et al., 2018). They constructed a biological network of miRNA and gene targets for up and downregulated miRNAs to study the effect of gold complex-altered miRNA expression profiles in A549 cells. Their studies show that complex 7 can disrupt the basic cellular mechanisms in A549 cell lines by affecting the micro-RNA network. The importance of developing new gold(I) complexes and their potential as new anti-lung cancer therapeutic agents were clarified.
Gefitinib is an epidermal growth factor receptor tyrosine kinase (EGFR-TK) inhibitor for non-small cell lung cancer treatment (Kirkpatrick et al., 2003; Yoshida et al., 2009; Inoue et al., 2020; Novello et al., 2020; Gibbons et al., 2021; Wu et al., 2021). Bierbach and co-workers utilized thiourea-modified gefitinib to derive linear gold(I)-sulfur complex 8. The cytotoxicity of complex 8 was also studied in lung cancer cells NCI-H460 (IC50 = 1.9 μM) (Bierbach et al., 2015). Compound 8 inhibited EGFR kinase-mediated phosphorylation, and its submicromolar IC50 value was similar to gefitinib observed under the same analytical conditions. Extension of the side chain on carbon 6 of the quinazoline ring to generate 8 led to a pronounced increase in potency. Compound 8 alone showed significantly better activity than gefitinib in a TKI-resistant cancer cell line. This observation suggests that the newly introduced thiourea-containing side chain may enhance the binding affinity of the classical TKI structure with the enzyme’s active site. This new type of metal hybrid agent designed by combining a biomolecular targeting inhibitor and metal ions provides new inspiration for the development of novel anticancer drugs.
Liu and co-workers developed a gold(I)-sulfur-phosphine complex 9 as a deubiquitinase inhibitor to prevent lung cancer A549 tumor growth (Liu et al., 2019). The cytotoxic effects of complex nine in lung cancer cells A549 were determined by MTT assay at different concentrations for 24, 48, or 72 h. The results indicated that complex nine significantly inhibited A549 cell viability after the 72 h treatment. In addition, Liu and co-workers also determined the complex 9-induced A549 cell death mechanism. There was a dose-dependent increase in AnnexinV/PI-positive cells after treatment with complex nine for 24 h. Western blot analysis showed that complex nine significantly increased the cleaved forms of caspase-3, 8, and nine to induce A549 cell apoptosis (Figures 6A,B). Like others gold complexes (1–5), complex nine also increased ROS production in A549 cells. However, the production of ROS induced by complex nine does not play a major role in apoptosis of A549 cells. Liu and co-workers used BALB/c nude mice to create xenograft models and assess the anti-lung cancer tumor action of complex nine in vivo. Treatment with complex nine via intraperitoneal injection (7 mg/kg/day) for 16 days in the A549 xenograft model showed significant inhibition of tumor growth, which led to a reduction in tumor weight of ∼42.5% (Figures 6C–F). Furthermore, the mice did not experience significant body weight loss after injection of complex 9.
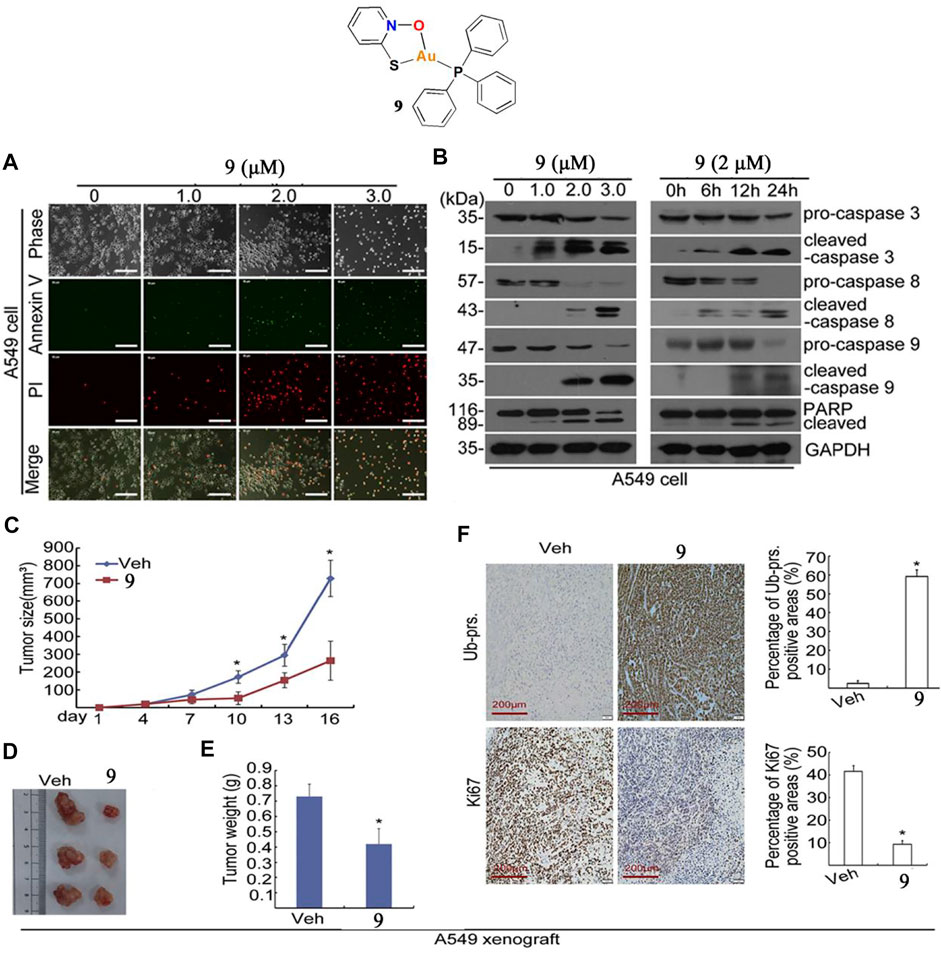
FIGURE 6. (A) Apoptotic cells were detected by Annexin V-FITC/PI staining after treatment with complex 9. (B) Western blot analysis of caspase-3, 8, and nine in A549 cells after treatment with complex 9. (C–F) BALB/c nude mice bearing A549 tumors after treatment with complex 9 (7 mg/kg/day) for 16 days. Reproduced with permission (Liu et al., 2019).
Alonso and co-workers reported gold(I)-sulfur-phosphine complex 10 as an excellent cytotoxicity gold(I) complex against lung cancer cell line A549 with an IC50 value of 0.03 μM (Alonso et al., 2020). The high cytotoxicity of complex 10 was not due to an increase in ROS content in cells but through inhibition of topoisomerase I. Unfortunately, the in vivo anti-lung cancer activity of complex 10 was not described in the literature.
Anti-lung cancer gold(I)-phosphine complexes
Metal-phosphine complexes have been utilized as cancer therapeutics for decades (Marzano et al., 2010; Bhargava et al., 2018; Awuah et al., 2019; Sitole et al., 2020; Batista et al., 2021; Tavares et al., 2021; Liu et al., 2022). In 2003, Marchetti and co-workers described a mixed phosphine gold(I) complex 11 as a potent anticancer agent (Marchetti et al., 2003), with positive anti-proliferative effects in the A549 cell line. The possibility exists for tuning the antitumor activity of gold-diphosphine cationic compounds between these two extremes: 1) more lipophilic complexes which are more cytotoxic and have increased side effects on mitochondria; 2) more hydrophilic compounds which are more selective, less cytotoxic, and have lower side effects. Unfortunately, the in vitro anti-lung cancer mechanism of complex 11 was not described in the literature. In 2014, Trávníček and co-workers modified a gold(I)-triphenylphosphine complex 12 with a hypoxanthine derivative as an anticancer agent (Trávníček et al., 2014). The IC50 values for the tested complex 12 showed moderate activity in the micromolar range.
In 2018, Bezuidenhout and co-workers identified a gold(I)-phosphine-ferrocenyl substituted 1,2,3-triazol-5-ylidene complex 13 as a potential anticancer agent (Bezuidenhout et al., 2018). The anticancer activity of complex 13 was assessed against two lung cancer cell lines, A549 and H1975, and the IC50 values were 0.89 and 0.23 μM, respectively. This complex 13 exhibited much higher anti-lung cancer activity than cisplatin. Fluorescence microscopy confirmed that gold(I) complex 13 induced H1975 lung cancer cell death by apoptosis but was not necrotic (Figures 7A,B), and preliminary judgment indicated that ROS played a key role in mediating cell death.
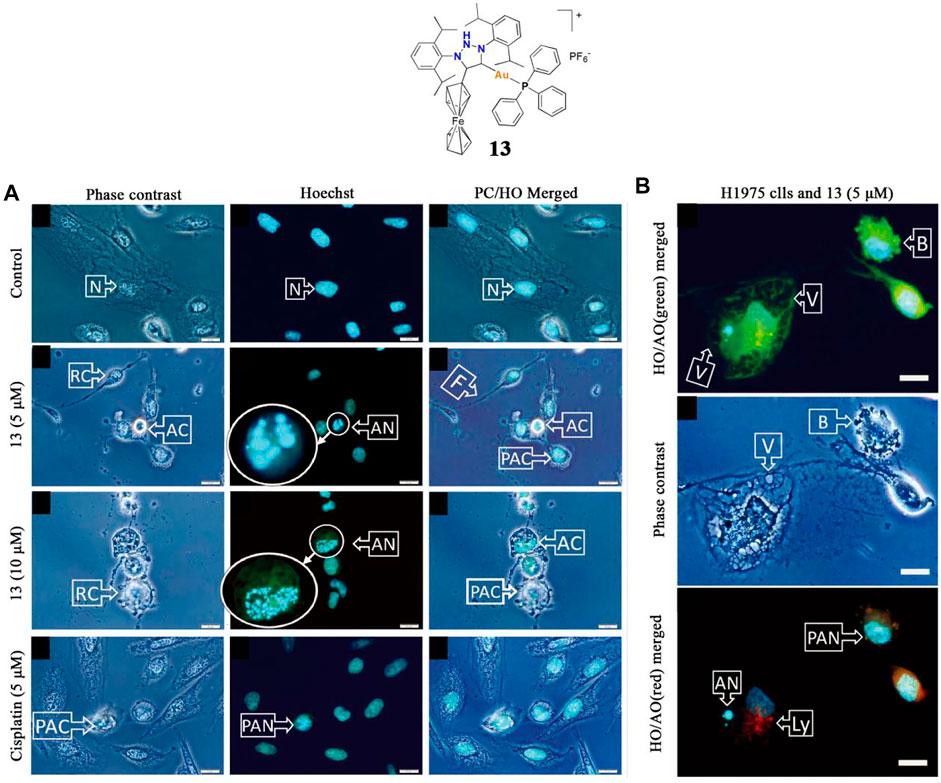
FIGURE 7. (A) Lung cancer cells H1975 undergo apoptosis after being treated with complex 13 at 5/10 μM concentrations for 18 h (B) Microscopic evaluation of H1975 cell morphology after treatment with complex 13 at a 5 μM concentration for 18 h. Reproduced with permission (Bezuidenhout et al., 2018).
In 2019, Bhargava and co-workers reported a gold(I)-phosphine Ph3 containing cinnamide and alkynyl complex 14 (Bhargava et al., 2019). The results clearly indicate that the gold(I) alkynyl fragment enhances the anticancer activity, and the cytotoxicity of the compounds was also influenced by the number of methoxy groups present in the alkyne moiety. They also confirmed that complex 14 has significant antiangiogenic effects. The anticancer mechanism involved is similar to that of most gold(I) complexes in that complex 14 significantly inhibits TrxR and increases ROS accumulation. Sordillo and co-workers discovered that TrxR regulates angiogenesis by increasing endothelial cell-derived vascular endothelial growth factor leading Bhargava and co-workers to consider that complex 14 could be contributing to the inhibition of angiogenesis. Thus, they investigated the angiogenesis-inhibiting properties of complex 14 using a transgenic Tg (fli1a:EGFP) zebrafish model (Figure 8). Their results indicated that complex 14 had significant antiangiogenic effects in zebrafish embryos and that gold(I) complexes may potentially become promising cancer therapeutics.
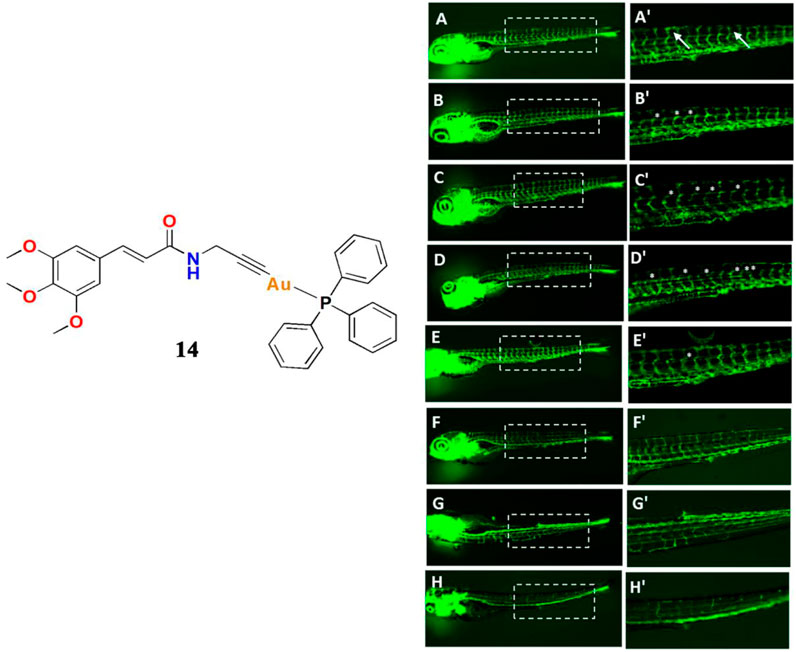
FIGURE 8. Angiogenesis inhibition in transgenic Tg (fli1a:EGFP) zebrafish embryos after treatment with different concentrations of complex 14, with cisplatin and axitinib as positive controls, at 24 hpf. (A, A′) control (B−D, B′−D′) complex 14 at 0.1/0.25/0.5 μM (E,E′) cisplatin (1 μM) (F−H, F′−H′) axitinib at 0.1/0.2/0.5 μM concentrations. Reproduced with permission (Bhargava et al., 2019).
Hussien and co-workers investigated gold(I)-phosphine Ph3 containing Schiff bases and alkynyl complex 15 in 2021 (Hussien et al., 2021) as an anti-proliferative in lung cancer cell line HOP-62. Like most gold(I) complex investigations, Hussien and co-workers also focused on inhibiting the redox enzyme TrxR by complex 15. They used molecular docking to study the gold(I) complexes in the two active sites of the human TrxR enzyme, using auranofin as a benchmark for comparison. They found that introducing the Schiff base phenolic moieties can alter the binding sites of the gold(I) complexes with TrxR, unlike auranofin. Complex 15 seems to be more cytotoxicity against lung cancer when compared to the other complexes, indicating a possible preference for the ortho position for the hydroxy group.
The latest studies indicate that gold(I)-phosphine complexes play an anticancer role other than inhibition of TrxR. In 2022, Marchetti and co-workers described a gold(I)-phosphine Ph3 containing carbamate complex 16 that exhibited positive in vitro cytotoxicity for A549 lung cancer cells (Marchetti et al., 2022). Interestingly, Marchetti and co-workers confirmed that complex 16 could significantly increase intracellular ROS accumulation by mitochondrial membrane depolarization (Figures 9A,B) and that it could also induce necroptosis and block cell cycle arrest in S phase (Figures 9C,D).
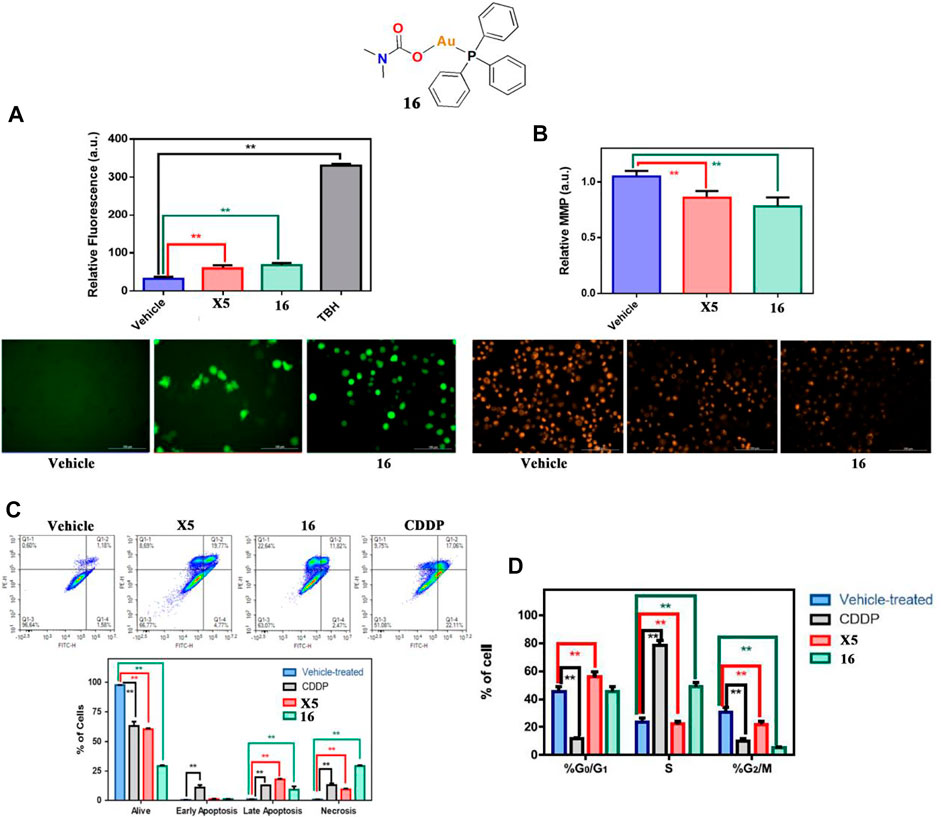
FIGURE 9. (A) ROS production by H2DCFDA in A549 cells after treatment with complex 16 at IC50 values for 4 h. (B) Mitochondrial membrane potential by TMRM in A549 cells after treatment with complex 16 at IC50 values for 1 h. (C) Flow cytometer analysis of A549 cells treated with complex 16 at IC50 values for 24 h. (D) Flow cytometer analysis of A549 cell cycle treated with complex 16 at the IC50 value for 24 h. Reproduced with permission (Marchetti et al., 2022).
Anti-lung cancer gold (III) complexes
Like many gold(I) complexes, gold (III) complexes can inhibit the activity of mercaptan-containing enzymes (including TrxR) by forming Au-S bonds through ligand exchange reactions (Messori et al., 2009). Nevertheless, there are also many excellent examples of physiologically stable gold (III) complexes, which are well-known to show higher anticancer activity in vitro and in vivo and induce cancer cell death possibly by multi-target mechanisms (Che et al., 2017; Fernandes et al., 2018; Isab et al., 2019; Bhargava et al., 2021; Maia et al., 2021; Fichna et al., 2022). Choosing the appropriate ligand to stabilize Au3+ ions is very important for cancer treatment (Che et al., 2010; Lin et al., 2010). Most of the reported anticancer gold (III) complexes focus on polydentate ligands, such as C ^ N, N ^C ^ N, N ^ N, C ^ N ^C, and phosphine (Figure 10). The Au3+ ions are highly stabilized under physiological conditions and have been reported to display potent anti-lung cancer activity.
Anti-lung cancer gold (III)-(C^N) complexes
Casini and co-workers described a gold (III)-(C^N) complex 17 by modifying auranofin with C^N cyclometalated 2-benzylpyridine in 2015. However, complex 17 did not display a lower IC50 value than auranofin (Casini et al., 2015). In 2018, Liang and co-workers described two similar tetrahydroisoquinoline gold (III)-(C^N) complexes 18 and 19 (Liang et al., 2018), which induce endoplasmic reticulum stress-mediated apoptosis and pro-death autophagy in A549 lung cancer cells. The results suggesting that organometallic complexes in which an Au replaces the first CH2 group of the N-heterocyclic scaffolds of 1,2,3,4-Tetrahydroisoquinoline possess enhanced cytotoxic activities. Among these two gold complexes, complex 19 not only showed strong anti-proliferative ability in lung cancer cell lines, but was also equally potent in cisplatin-resistant lung cancer cell lines (A549 CDDP cells), which is indicative of a different mechanism than cisplatin. Complex 19 also showed strong anti-lung cancer ability in vivo. After intraperitoneal injections with cisplatin (3 mg/kg) or complex 19 (10 mg/kg) for 18 days in A549 xenograft tumor-bearing mice, complex 19 significantly inhibited tumor growth, leading to ∼62.3% reduction of tumor weight (Figure 11A), which was greater than that of cisplatin (∼44.5%). Liang and co-workers confirmed in A549 lung cancer cells that complex 19 could induce mitochondrial damage and causes ATP depletion, mitochondrial membrane depolarization (Figure 11B), elevated ROS levels (Figure 11C), endoplasmic reticulum stress, and ultimately induce apoptosis (Figure 11D) and pro-death autophagy (Figure 11E).
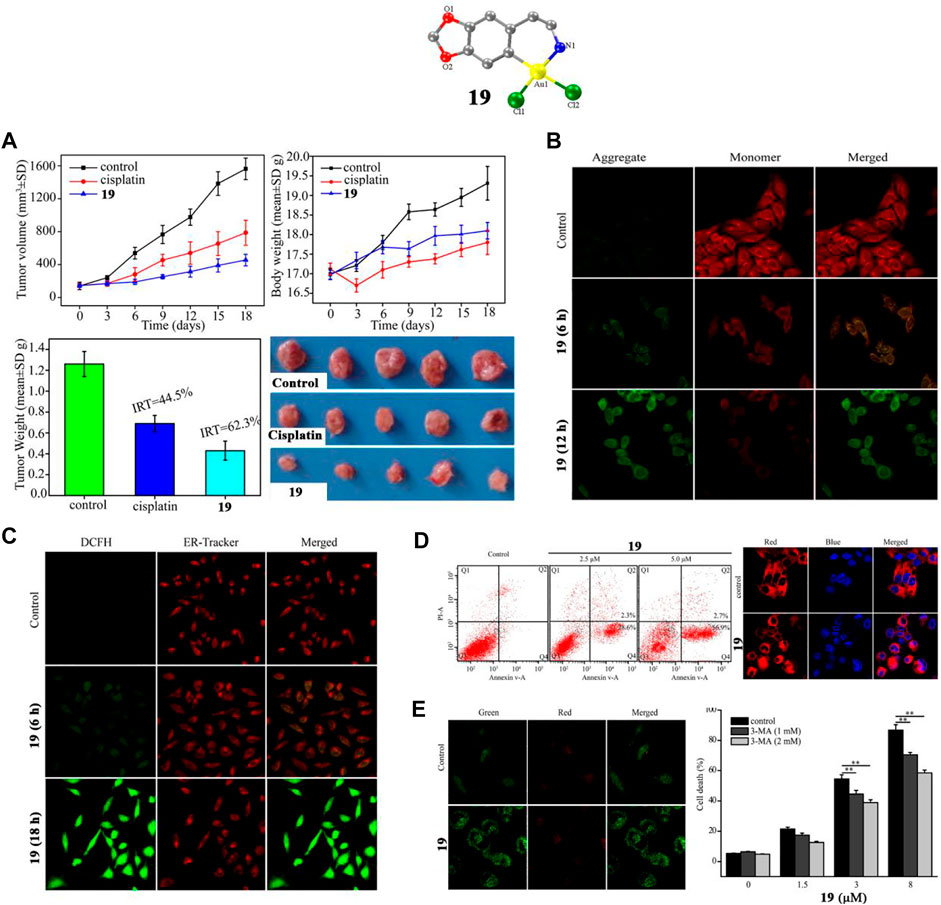
FIGURE 11. (A) BALB/c nude mice bearing A549 tumors after treatment with complex 19 (10 mg/kg/3 days) for 18 days. (B) Mitochondrial membrane potential in A549 cells after treatment with complex 19 at IC50 value for 0.5 h. (C) ROS production by ER-Tracker Red and H2DCFDA in A549 cells after treatment with complex 19 at IC50 value for 6 h. (D) A549 cell apoptosis after treatment with complex 19 at IC50 value measured by flow cytometer and confocal microscopy. (E) Analysis of autophagy in A549 lung cancer cells after treatment with complex 19 at IC50 value. Reproduced with permission (Liang et al., 2018).
Anti-lung cancer gold (III) (C^N^C) complexes
Bochmann and co-workers synthesized and characterized three pyrazine-based cyclometalated (C ^ N ^ C) gold (III) complexes in 2017 (Bochmann et al., 2017b). Of the series, the neutral alkynyl and thiophenolate complexes were nontoxic, whereas the benzimidazolylidene complex 20 showed strong antiproliferative activity, with the cell viability reduced to 22%. In addition, basis of the antiproliferative results, it might be suggested that when PF6 was incorporated into gold complexes, the resulting gold complexes tended to show an enhanced anticancer effect with fewer side effects in vitro. Complex 20 containing the benzimidazole-based NHC ligand had remarkable cytotoxicity, the IC50 values are in the micromolar to the submicromolar range and lower than the other two complexes and cisplatin. With cytotoxicity in the A549 cell line four times more than cisplatin, complex 20 was encouragingly effective. However, complex 20 also had the disadvantage of high cytotoxicity against healthy fibroblast MRC-5 cells. For higher cytotoxicity, 1,3-dimethylbenzimidazol-2-ylidene and pyrazine-based cyclometalated (C^N^C) ligand to stabilize the Au3+ ion is very important. The stable structure of complex 20 contributes to interacting more tightly with human telomerase G-quadruple DNA structures. Inhibition of the MDM2 interaction with p53 by complex 20 was also reported, providing new clues for the possible intracellular targets of this complex. From 2017 to 2018, Bochmann and co-workers tried to modify complex 20 and increase its cytotoxicity in A549 cells, such as complexes 21 and 22 (Bochmann et al., 2017a, 2018). Complex 21 with acridine functionality bound to the NHC ligand, it was more than 3–4 times more toxic than cisplatin against the A549. The complexes with the most lipophilic side-chains turned out to have a reduced activity compared to the other amino ester decorated complexes, while only the adamantyl derivative 22 was also more toxic than cisplatin against lung cancer cells, and the adamantyl compound 22 was over ten times more potent than Au-NHC. Among the series of amino ester derivatives, the glycinebased complex appeared as the least toxic in the series.
Anti-lung cancer gold (III) (N^N) complexes
Many anticancer metal complexes with excellent cytotoxicity have been reported based on phenanthroline bidentate ligands. In 2014, Eichler and co-workers introduced a series of gold (III) (N^N) complexes based on phenanthroline ligands (Eichler et al., 2014). Compounds 23 was centered on the premise that the phenanthroline ligand would impart greater redox stability to the gold (III) center, which in turn would guard this class of metal-based drugs against inactivation/reduction by glutathione. Complex 23 has shown promising anti-lung cancer (A549) activity with a remarkable IC50 value, significantly higher than cisplatin in clinical use. In addition, complex 23 has been reported to effectively inhibit TrxR, even more than the known TrxR inhibitor aurothiomalate; therefore, complex 23 possibly induces lung cancer cell death via a TrxR-mediated mechanism.
As described earlier, gold (III) complexes may induce cancer cell death by multi-target mechanisms. Casini and co-workers have modified several gold (III) (N^N) complexes based on phenanthroline ligands as potential AQP3 inhibitors in 2019, including complexes 24 and 25 (Casini et al., 2019). Aquaporins (AQPs), such as aquaporin-3 (AQP3), which play crucial roles in cell apoptosis, proliferation, and migration, have been proposed as new drug targets for cancer treatment. The two complexes displayed potent cytotoxicity in the A549 cell line, which expresses AQP3, with IC50 values of 0.43 and 0.83 μM for 24 and 25, respectively. Biophysical techniques and computational methods were used to investigate the mechanism of inhibition of AQP3 by gold (III) complexes. Complexes 24 and 25, with EC50 values in the low micromolar range to AQP3, outperformed the benchmark compounds Auphen and Aubipy. This report indicated that in the future, gold (III) complexes based on phenanthroline ligands could be used as potential anticancer agents via AQP inhibition.
However, even though gold (III) complexes based on phenanthroline ligands have displayed potent anti-lung cancer activity in vitro, the in vivo anti-lung cancer efficacy remains in question. Eichler and co-workers demonstrated that those gold (III) complexes could interact with serum albumin proteins, which may cause the gold (III) complex to be destroyed during blood circulation.
Anti-lung cancer gold (III)-phosphine complexes
Gold (III)-phosphine complexes have also shown great potential in anti-lung cancer studies. Bhargava and co-workers developed a stable anti-lung cancer gold (III) complex 26 containing cyclometalated triphenylphosphine sulfide ligands in 2020 (Bhargava et al., 2018). Preliminary in vitro cytotoxicity studies found that complex 26 can inhibit A549 cells. Similarly, in reports by Mostafa and co-workers in 2019, complex 26 had significant antiangiogenic effects and also induced apoptosis by increasing ROS levels. Bhargava and co-workers investigated the angiogenesis-inhibiting properties of complex 26 using WimTube (Figure 12). Quantification revealed all aspects of network formation were significantly affected in complex 26 groups, indicating excellent antiangiogenic properties. Those results suggest cycloaurated gold (III) complexes may become new drug molecules for future cancer therapeutics.
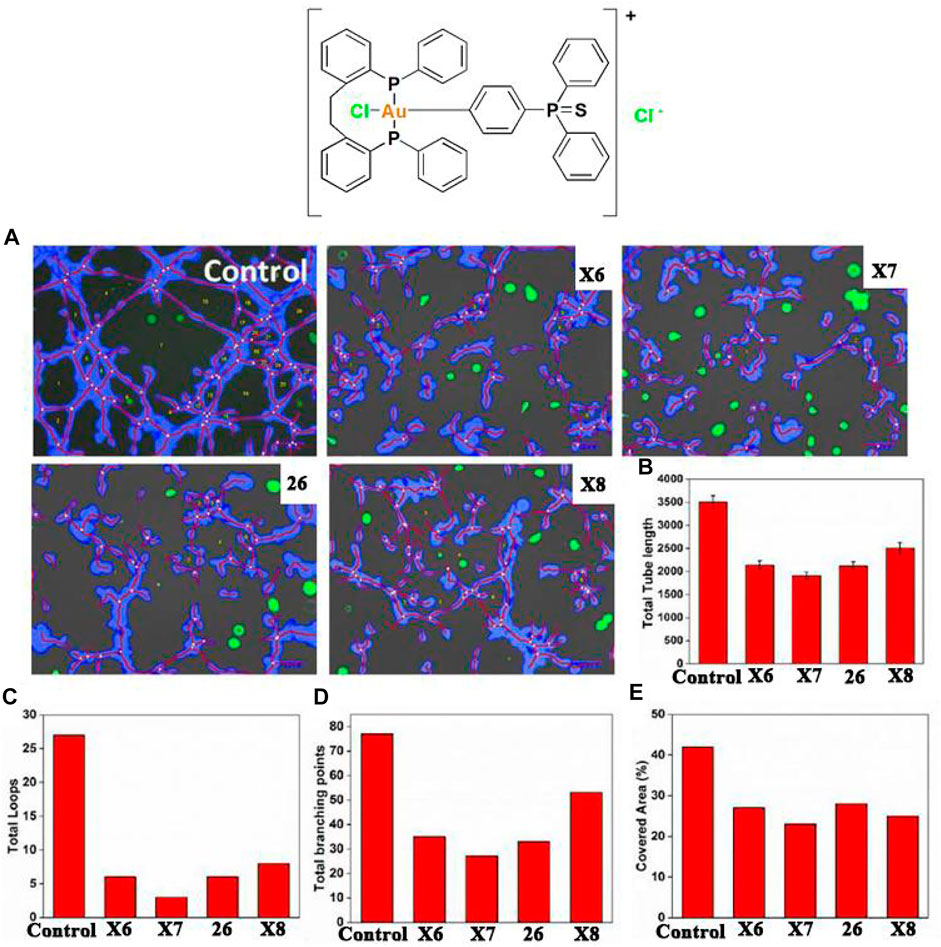
FIGURE 12. Tube formations of human umbilical vascular endothelial (HUVEC) cells. HUVECs cultured on Matrigel-coated plates and treated with gold (III)-phosphine complexes at IC50 concentrations for 16 h. (A) Tube formation observed by fluorescence microscopy. (B–E) The total lengths of the branching points, covered area, tubes, and loops were quantified. Reproduced with permission (Bhargava et al., 2018).
Approaches adopted to improve the efficiency of gold complexes against lung cancer
Although significant achievements have been made in developing anticancer gold complexes showing excellent stability and cytotoxicity in vitro and in vivo, poor bioavailability, selectivity, and serious toxicity have hindered their clinical application. Fortunately, various biocompatible nanoparticle (NP)-based drug-delivery systems, such as hydrogel (Zhang et al., 2019), endogenous protein (Zhang et al., 2020; Zhang et al., 2021b), liposomes (Liu et al., 2020), and silica (Che et al., 2014; Zhang et al., 2021b) have been reported for metal complexes targeting cancer treatment. Furthermore, the use of drug carriers has recently been described to enhance the anti-lung cancer effects of gold complexes.
In 2014, Che and co-workers used low toxicity mesoporous silica nanoparticles (MSNs) to enhance the anticancer efficacy of gold (III) porphyrin complex (AuP) (Figures 13A–C) (Che et al., 2014). The RGD peptide modified on the surface of MSNs effectively enhanced the cellular uptake of AuP and decreased the damage to normal cells. When the cellular uptake of AuP was analyzed by inductively coupled plasma mass spectrometry (ICP-MS), the results showed that intracellular Au content increased in a time-dependent manner in A549 cells but had a lower accumulation in L02 cells (Figure 13D). Encapsulation of AuP to form AuP@MSN(R) significantly improved the cytotoxicity of AuP to A549 cells (Figure 13E) and inhibitory effects on TrxR (Figure 13F). Compared to free AuP, AuP@MSN(R) could induce higher ROS levels and oxidative stress in cancer cells (Figure 13G) and activate ROS-mediated apoptosis signaling pathways.
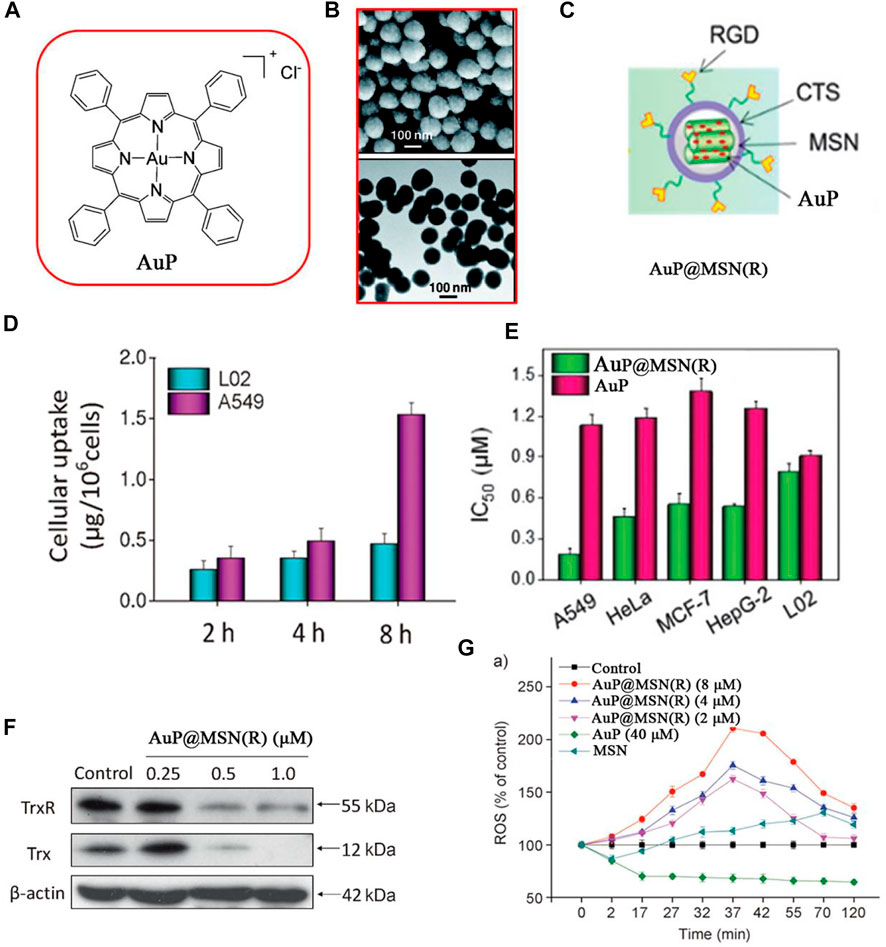
FIGURE 13. (A) Chemical structure of AuP. (B) SEM and TEM of AuP@MSN(R). (C) Structure of AuP@MSN(R). (D) Cytotoxicity of AuP@MSN(R) and AuP against cancer cells. (E) Cellular uptake of AuP@MSN(R) in A549 lung cancer cells and L02 normal cells. (F) Western blot of TrxR in A549 cells after being treated with AuP@MSN(R) for 24 h. (G) Increase intracellular ROS levels treated with AuP@MSN(R), AuP, and MSN. Reproduced with permission (Che et al., 2014).
Another work by Che and co-workers reported the bioavailable and biodegradable hydrogel formulation using an interpenetrating network (IPN) matrix to deliver AuP for lung cancer treatment (Figures 14A–G) (Che et al., 2019, 2020). Xenograft model tests indicated that hydrogel improved the AuP in vivo anticancer efficacy compared to free AuP (Figures 14D–G) and effectively reduced systemic toxicity. The biocompatible hydrogel that can selectively accumulate in tumor tissue and can locally release AuP has promising chemotherapeutic potential. Similar delivery systems used to deliver other gold complexes for the treatment of lung cancer display excellent preclinical applications.
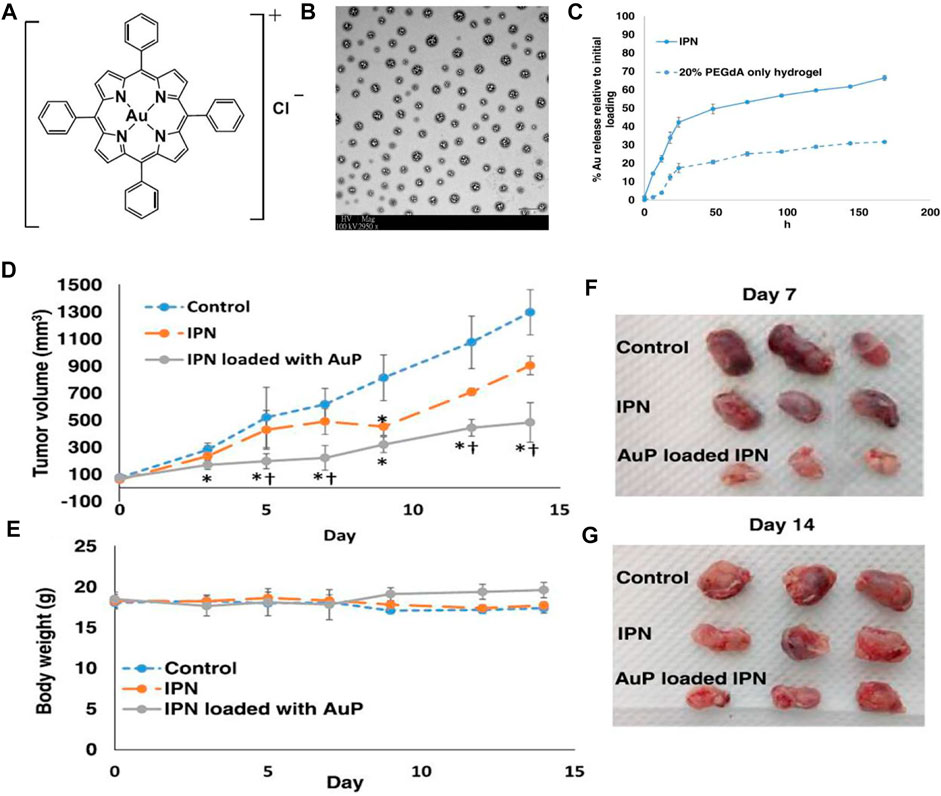
FIGURE 14. (A) Gold (III) porphyrin (AuP). (B) TEM image of hydrogel, scale bar 1 μm. (C) The release kinetics of AuP from hydrogel to 7 days in vitro. (D–G) Tumor growth inhibition in lung cancer xenografted mice for 14 days of treatment. Reproduced with permission (Che et al., 2019, 2020).
Conclusion and prospects
In recent years, gold complexes have been developed as potential anti-lung cancer drugs in the hope of solving the resistance problem of cisplatin. Thiolenzymes, such as thioredoxin reductase (TrxR), are generally considered key targets of anti-lung cancer gold(I) and gold (III) complexes due to the high binding affinity of Au ions with thiols. The X-ray crystal structures and “omics” technologies provide valuable information for gold–protein interactions in the literature, significantly contributing to determining anticancer targets and pathways of gold complexes.
The cytotoxicity and molecular mechanisms of gold(I) complexes against lung cancer cells, which mainly inhibit the activity of thiol enzymes, seem clear. Obviously, gold (III) complexes can also inhibit the activity of thiol enzymes (including TrxR) by forming Au-S bonds similar to most gold(I) complexes. Nevertheless, evidence confirms that physiologically stable gold (III) complexes show higher anti-lung cancer activity in vitro and in vivo by multi-target mechanisms. Such mechanisms include the induction of mitochondrial damage causing ATP depletion, mitochondrial membrane depolarization, increased intracellular ROS levels, endoplasmic reticulum stress, and ultimately apoptosis and autophagy in lung cancer cells. Or they may form adducts with other anticancer molecular targets and interfere with cellular signaling pathways.
In addition, novel drug carriers will improve in vivo delivery efficiency, bioavailability, and targeting, while reducing unexpected side effects. In summary, with appropriately designed ligands to gold (III)/gold(I) complexes and utilization of drug micro-carriers to improve stability and efficacy, it is feasible to develop anti-lung cancer gold complexes with promising chemotherapeutic potential.
Author contributions
JZ, and JC: designed this work of review; JZ, YL, and RF: wrote the manuscript; YW, JJ, and WW: performed the literature search of the databases; JZ and FY: revised the manuscript. All authors approved the paper for publication.
Funding
This work was supported by the Natural Science Foundation of China (No. 81973574, No. 82174082, No. 22077021), and the Natural Science Foundation of Guangxi (No. 2019GXNSFFA245001, 2022GXNSFGA035003).
Conflict of interest
The authors declare that the research was conducted in the absence of any commercial or financial relationships that could be construed as a potential conflict of interest.
Publisher’s note
All claims expressed in this article are solely those of the authors and do not necessarily represent those of their affiliated organizations, or those of the publisher, the editors and the reviewers. Any product that may be evaluated in this article, or claim that may be made by its manufacturer, is not guaranteed or endorsed by the publisher.
References
Abdalbari, F. H., and Telleria, C. M. (2021). The gold complex auranofin: New perspectives for cancer therapy. Discov. Oncol. 12, 42. doi:10.1007/s12672-021-00439-0
Akbarsha, M. A., Gowdhami, B., Ambika, S., Karthiyayini, B., Ramya, V., Kadalmani, B., et al. (2021). Potential application of two cobalt (III) schiff base complexes in cancer chemotherapy: Leads from a study using breast and lung cancer cells. Toxicol. Vitro. 75, 105201. doi:10.1016/j.tiv.2021.105201
Alonso, C., Martín-Encinas, E., Conejo-Rodríguez, V., Miguel, J. A., Martínez-Ilarduya, J. M., Rubiales, G., et al. (2020). Novel phosphine sulphide gold(i) complexes: Topoisomerase I inhibitors and antiproliferative agents. Dalton Trans. 49, 7852–7861. doi:10.1039/d0dt01467b
Arambula, J, F., Sen, S., Perrin, M. W., Sedgwick, A. C., Lynch, V. M., and Sessler, J. L. (2021). Covalent and non-covalent albumin binding of Au(i) Bis-NHCs via post-synthetic amide modification. Chem. Sci. 12, 7547–7553. doi:10.1039/d1sc01055g
Arambula, J. F., McCall, R., Miles, M., Lascuna, P., Burney, B., Patel, Z., et al. (2017). Dual targeting of the cancer antioxidant network with 1, 4-naphthoquinone fused gold(i) N-heterocyclic carbene complexes. Chem. Sci. 8, 5918–5929. doi:10.1039/c7sc02153d
Arambula, J. F., Sen, S., Hufnagel, S., Maier, E. Y., Aguilar, I., Selvakumar, J., et al. (2020). Rationally designed redox-Active Au(I) N-heterocyclic carbene: An immunogenic cell death inducer. J. Am. Chem. Soc. 142, 20536–20541. doi:10.1021/jacs.0c09753
Arumugam, K., Arambula, J. F., McCall, R., Sidoran, K. J., Magda, D., Mitchell, N. A., et al. (2016). Targeting antioxidant pathways with ferrocenylated N-heterocyclic carbene supported gold(I) complexes in A549 lung cancer cells. Chem. Sci. 7, 1245–1256. doi:10.1039/C5SC03519H
Avarvari, N., Guyon, F., Hameau, A., Khatyr, A., Knorr, M., Amrouche, H., et al. (2008). Syntheses, structures, and photophysical properties of mono- and dinuclear sulfur-rich gold(I) complexes. Inorg. Chem. 47, 7483–7492. doi:10.1021/ic7022067
Avery, G. S., Chaffman, M., Brogden, R. N., Heel, R. C., and Speight, T. M. (1984). Auranofin. A preliminary review of its pharmacological properties and therapeutic use in rheumatoid arthritis. Drugs 27, 378–424. doi:10.2165/00003495-198427050-00002
Awuah, S. G., Kim, J. H., Reeder, E., and Parkin, S. (2019). Gold(I/III)-phosphine complexes as potent antiproliferative agents. Sci. Rep. 9, 12335. doi:10.1038/s41598-019-48584-5
Batista, A. A., Oliveira, K. M., Honorato, J., Demidoff, F. C., Schultz, M. S., Netto, C. D., et al. (2021). Lapachol in the design of a new ruthenium(II)-diphosphine complex as a promising anticancer metallodrug. J. Inorg. Biochem. 214, 111289. doi:10.1016/j.jinorgbio.2020.111289
Bellelli, A., Angelucci, F., Sayed, A. A., Williams, D. L., Boumis, G., Brunori, M., et al. (2009). Inhibition of schistosoma mansoni thioredoxin-glutathione reductase by auranofin: structural and kinetic aspects. J. Biol. Chem. 284, 28977–28985. doi:10.1074/jbc.M109.020701
Bezuidenhout, D. I., Aucamp, D., Kumar, S. V., Liles, D. C., Fernandes, M. A., and Harmse, L. (2018). Synthesis of heterobimetallic gold(i) ferrocenyl-substituted 1,2,3-triazol-5-ylidene complexes as potential anticancer agents. Dalton Trans. 47, 16072–16081. doi:10.1039/c8dt03116a
Bhargava, S., Ganga, Reddy. V., Srinivasa, Reddy. T., Privér, S. H., Bai, Y., Mishra, S., et al. (2019). Synthesis of gold(I) complexes containing cinnamide: In vitro evaluation of anticancer activity in 2D and 3D spheroidal models of melanoma and in vivo angiogenesis. Inorg. Chem. 58, 5988–5999. doi:10.1021/acs.inorgchem.9b00281
Bhargava, S. K., Srinivasa, Reddy. T., Privér, S. H., Rao, V. V., and Mirzadeh, N. (2018). Gold(i) and gold(iii) phosphine complexes: synthesis, anticancer activities towards 2D and 3D cancer models, and apoptosis inducing properties. Dalton Trans. 47, 15312–15323. doi:10.1039/c8dt01724g
Bhargava, S., Mirzadeh, N., Telukutla, S. R., Luwor, R., Privér, S., Velma, G. R., et al. (2021). Dinuclear orthometallated gold(I)-gold(III) anticancer complexes with potent in vivo activity through an ROS-dependent mechanism. Metallomics. 13, mfab039. doi:10.1093/mtomcs/mfab039
Bierbach, U., Yang, M., Pickard, A. J., Qiao, X., Gueble, M. J., Day, C. S., et al. (2015). Synthesis, reactivity, and biological activity of gold(I) complexes modified with thiourea-functionalized tyrosine kinase inhibitors. Inorg. Chem. 54, 3316–3324. doi:10.1021/ic502998a
Bochmann, M., Bertrand, B., Fernandez-Cestau, J., Angulo, J., Cominetti, M. M. D., Waller, Z. A. E., et al. (2017a). Cytotoxicity of pyrazine-based cyclometalated (C ^ Npz ^C)Au(III) carbene complexes: Impact of the nature of the ancillary ligand on the biological properties. Inorg. Chem. 56, 5728–5740. doi:10.1021/acs.inorgchem.7b00339
Bochmann, M., Williams, M., Green, A. I., Fernandez-Cestau, J., Hughes, D. L., O’Connell, M. A., et al. (2017b). (C ^ Npz ^C)AuIII complexes of acyclic carbene ligands: synthesis and anticancer properties. Dalton Trans. 46, 13397–13408. doi:10.1039/c7dt02804k
Bochmann, M., Williams, M. R. M., Bertrand, B., Fernandez-Cestau, J., Waller, Z. A. E., O'Connell, M. A., et al. (2018). Acridine-decorated cyclometallated gold(iii) complexes: synthesis and anti-tumour investigations. Dalton Trans. 47, 13523–13534. doi:10.1039/c8dt02507j
Boshoff, C., Herbst, R. S., and Morgensztern, D. (2018). The biology and management of non-small cell lung cancer. Nature 553, 446–454. doi:10.1038/nature25183
Boshoff, C., Wang, M., and Herbst, R. S. (2021). Toward personalized treatment approaches for non-small-cell lung cancer. Nat. Med. 27, 1345–1356. doi:10.1038/s41591-021-01450-2
Bostancıoğlu, R. B., Varol, M., Benkli, K., and Koparal, A. T. (2019). Design and synthesis of novel organometallic complexes using boronated phenylalanine derivatives as potential anticancer agents. Drug Chem. Toxicol. 42, 436–443. doi:10.1080/01480545.2018.1504057
Bourissou, D., Rodriguez, J., Szalóki, G., Sosa, Carrizo. E. D., Saffon-Merceron, N., and Miqueu, K. (2020). Gold(III) π-allyl complexes. Angew. Chem. Int. Ed. Engl. 59, 1511–1515. doi:10.1002/anie.201912314
Bray, F., Sung, H., Ferlay, J., Siegel, R. L., Laversanne, M., Soerjomataram, I., et al. (2021). Global cancer statistics 2020: GLOBOCAN estimates of incidence and mortality worldwide for 36 cancers in 185 countries. Ca. Cancer J. Clin. 71, 209–249. doi:10.3322/caac.21660
Casini, A., Bertrand, B., Spreckelmeyer, S., Bodio, E., Cocco, F., Picquet, M., et al. (2015). Exploring the potential of gold(III) cyclometallated compounds as cytotoxic agents: variations on the C^N theme. Dalton Trans. 44, 11911–11918. doi:10.1039/c5dt01023c
Casini, A., Wenzel, M. N., Mósca, A. F., Graziani, V., Aikman, B., Thomas, S. R., et al. (2019). Insights into the mechanisms of aquaporin-3 inhibition by gold(III) complexes: The importance of non-coordinative adduct formation. Inorg Chem. 58, 2140–2148. doi:10.1021/acs.inorgchem.8b03233
Chao, H., Wang, L., Guan, R., Xie, L., Liao, X., Xiong, K., et al. (2021). An ER-targeting iridium(III) complex that induces immunogenic cell death in non-small-cell lung cancer. Angew. Chem. Int. Ed. Engl. 60, 4657–4665. doi:10.1002/anie.202013987
Che, C., Fung, S., Zou, T., Cao, B., Lee, P. Y., Fung, Y., et al. (2017). Cyclometalated gold(III) complexes containing N-heterocyclic carbene ligands engage multiple anti-cancer molecular targets. Angew. Chem. Int. Ed. Engl. 56, 3892–3896. doi:10.1002/anie.201612583
Che, C. M., He, L., Chen, T., You, Y., Hu, H., Zheng, W., et al. (2014). A cancer-targeted nanosystem for delivery of gold(III) complexes: enhanced selectivity and apoptosis-inducing efficacy of a gold(III) porphyrin complex. Angew. Chem. Int. Ed. Engl. 53, 12532–12536. doi:10.1002/anie.201407143
Che, C. M., Lee, P., Lok, C. N., Che, C. M., and Kao, W. J. (2019). A Multifunctional Hydrogel Delivers Gold Compound and Inhibits Human Lung Cancer Xenograft. Pharm. Res. 36, 61. doi:10.1007/s11095-019-2581-z
Che, C. M., Lee, P., Lok, C. N., Che, C. M., and Kao, W. J. (2020). Multifunctional Microparticles Incorporating Gold Compound Inhibit Human Lung Cancer Xenograft. Pharm. Res. 37, 220. doi:10.1007/s11095-020-02931-8
Che, C., Sun, R., Li, C., Ma, D., Yan, J., Lok, C., et al. (2010). Stable Anticancer Gold(III)-porphyrin Complexes: Effects of Porphyrin Structure. Chemistry 16, 3097–3113. doi:10.1002/chem.200902741
Cheng, H., Alexander, M., and Kim, S. Y. (2020). Update 2020: Management of Non-small Cell Lung Cancer. Lung 198, 897–907. doi:10.1007/s00408-020-00407-5
Choi, Y. H., Hwangbo, H., Ji, S. Y., Kim, M. Y., Kim, S. Y., Lee, H., et al. (2021). Anti-inflammatory Effect of Auranofin on Palmitic Acid and LPS-Induced Inflammatory Response by Modulating TLR4 and NOX4-Mediated NF-Κb Signaling Pathway in RAW264.7 Macrophages. Int. J. Mol. Sci. 22, 5920. doi:10.3390/ijms22115920
Colotti, G., Ilari, A., Baiocco, P., Messori, L., Fiorillo, A., Boffi, A., et al. (2012). A Gold-Containing Drug against Parasitic Polyamine Metabolism: the X-Ray Structure of Trypanothione Reductase from Leishmania Infantum in Complex with Auranofin Reveals a Dual Mechanism of Enzyme Inhibition. Amino Acids 42, 803–811. doi:10.1007/s00726-011-0997-9
Daher, C. F., Fayad, C., Audi, H., and Khnayzer, R. S. (2021). The Anti-cancer Effect of Series of Strained Photoactivatable Ru(II) Polypyridyl Complexes on Non-small-cell Lung Cancer and Triple Negative Breast Cancer Cells. J. Biol. Inorg. Chem. 26, 43–55. doi:10.1007/s00775-020-01835-7
de Almeida, M. V., Chaves, J. D. S., Tunes, L. G., de J Franco, C. H., Francisco, T. M., Corrêa, C. C., et al. (2017). Novel Gold(I) Complexes with 5-phenyl-1, 3, 4-Oxadiazole-2-Thione and Phosphine as Potential Anticancer and Antileishmanial Agents. Eur. J. Med. Chem. 127, 727–739. doi:10.1016/j.ejmech.2016.10.052
Dhuna, K., Altaf, M., Monim-ul-Mehboob, M., Seliman, A. A., Sohail, M., Wazeer, M. I., et al. (2015). Synthesis, Characterization and Anticancer Activity of Gold(I) Complexes that Contain Tri-tert-butylphosphine and Dialkyl Dithiocarbamate Ligands. Eur. J. Med. Chem. 95, 464–472. doi:10.1016/j.ejmech.2015.03.019
Dos, Santos. H. F., Sánchez, Delgado. G. Y., Arvellos, J. F. A., and Paschoal, D. F. S. (2021). Role of the Enzymatic Environment in the Reactivity of the AuIII-C ^ N ^ C Anticancer Complexes. Inorg. Chem. 60, 3181–3195. doi:10.1021/acs.inorgchem.0c03521
Dou, Q., Zhang, X., Frezza, M., Milacic, V., Ronconi, L., Fan, Y., et al. (2010). Inhibition of Tumor Proteasome Activity by Gold-Dithiocarbamato Complexes via Both Redox-dependent and -independent Processes. J. Cell. Biochem. 109, 162–172. doi:10.1002/jcb.22394
Du, W., Wang, W., Zhao, C., Zhu, D., and Gong, G. (2017). Inhibition of Amyloid Peptide Fibril Formation by Gold-Sulfur Complexes. J. Inorg. Biochem. 171, 1–9. doi:10.1016/j.jinorgbio.2017.02.021
Eichler, J. F., Olsen, P. M., Ruiz, C., Lussier, D., Le, B. K., Angel, N., et al. (2014). Synthesis, Characterization, and Antitumor Activity of Unusual Pseudo Five Coordinate Gold(III) Complexes: Distinct Cytotoxic Mechanism or Expensive Ligand Delivery Systems? J. Inorg. Biochem. 141, 121–131. doi:10.1016/j.jinorgbio.2014.08.014
Fang, B., Hu, J., Zhang, H., Cao, M., Wang, L., and Wu, S. (2018). Auranofin Enhances Ibrutinib's Anticancer Activity in EGFR-Mutant Lung Adenocarcinoma. Mol. Cancer Ther. 17, 2156–2163. doi:10.1158/1535-7163.MCT-17-1173
Fereidoonnezhad, M., Faghih, Z., Yazdani Kachoei, A., Alizadeh, H., Emamdoost, S., and Shirkhan, S. (2020). Synthesis, Molecular Docking and Cytotoxic Activity Evaluation of Organometallic Thiolated Gold(I) Complexes. Iran. J. Pharm. Res. 19, 134–143. doi:10.22037/ijpr.2020.1101118
Fernandes, A. R., Choroba, K., Machura, B., Szlapa-Kula, A., Malecki, J. G., Raposo, L., et al. (2018). Square Planar Au(III), Pt(II) and Cu(II) Complexes with Quinoline-Substituted 2, 2':6', 2″-terpyridine Ligands: From In Vitro to In Vivo Biological Properties. Eur. J. Med. Chem. 218, 113404. doi:10.1016/j.ejmech.2021.113404
Fichna, J., Gurba, A., Taciak, P., Sacharczuk, M., Młynarczuk-Biały, I., and Bujalska-Zadrożny, M. (2022). Gold (III) Derivatives in Colon Cancer Treatment. Int. J. Mol. Sci. 23 (2), 724. doi:10.3390/ijms23020724
Fregona, D., Boscutti, G., Nardon, C., Marchiò, L., Crisma, M., Biondi, B., et al. (2018). Anticancer Gold(III) Peptidomimetics: From Synthesis to In Vitro and Ex Vivo Biological Evaluations. Chem Med Chem 13, 1131–1145. doi:10.1002/cmdc.201800098
Furst, D. E. (1983). Mechanism of Action, Pharmacology, Clinical Efficacy and Side Effects of Auranofin. An Orally Administered Organic Gold Compound for the Treatment of Rheumatoid Arthritis. Pharmacotherapy 3, 284–298. doi:10.1002/j.1875-9114.1983.tb03277.x
Gianneschi, N. C., Proetto, M. T., Alexander, K., Melaimi, M., and Bertrand, G. (2021). Cyclic (Alkyl)(Amino)carbene (CAAC) Gold(I) Complexes as Chemotherapeutic Agents. Chemistry 27, 3772–3778. doi:10.1002/chem.202004317
Gibbons, D. L., Creelan, B. C., Yeh, T. C., Kim, S. W., Nogami, N., Kim, D. W., et al. (2021). A Phase 1 Study of Gefitinib Combined with Durvalumab in EGFR TKI-Naive Patients with EGFR Mutation-Positive Locally Advanced/metastatic Non-small-cell Lung Cancer. Br. J. Cancer 124, 383–390. doi:10.1038/s41416-020-01099-7
Gimeno, M. C., Montanel-Pérez, S., Izaga, A., Laguna, A., and Villacampa, M. D. (2018). Synthesis of Gold(III) Complexes with Bidentate Amino-Thiolate Ligands as Precursors of Novel Bifunctional Acyclic Diaminocarbenes. ACS Omega 3, 13097–13103. doi:10.1021/acsomega.8b01547
Gornitzka, H., Zhang, C., Fortin, P. Y., Barnoin, G., Qin, X., Wang, X., et al. (2020). An artemisinin-derivative-(NHC)Gold(I) Hybrid with Enhanced Cytotoxicity through Inhibition of NRF2 Transcriptional Activity. Angew. Chem. Int. Ed. Engl. 59, 12062–12068. doi:10.1002/anie.202002992
Grapperhaus, C. A., Andres, S. A., Bajaj, K., Vishnosky, N. S., Peterson, M. A., Mashuta, M. S., et al. (2020). Synthesis, Characterization, and Biological Activity of Hybrid Thiosemicarbazone-Alkylthiocarbamate Metal Complexes. Inorg. Chem. 59, 4924–4935. doi:10.1021/acs.inorgchem.0c00182
Heist, R. S., Thai, A. A., Solomon, B. J., Sequist, L. V., and Gainor, J. F. (2021). Lung Cancer. Lancet 398, 535–554. doi:10.1016/S0140-6736(21)00312-3
Hussien, M. A., Babgi, B. A., Alsayari, J., Alenezi, H. M., Abdellatif, M. H., Eltayeb, N. E., et al. (2021). Alteration of Anticancer and Protein-Binding Properties of Gold(I) Alkynyl by Phenolic Schiff Bases Moieties. Pharmaceutics 13, 461. doi:10.3390/pharmaceutics13040461
Inoue, A., Hosomi, Y., Morita, S., Sugawara, S., Kato, T., Fukuhara, T., et al. (2020). Gefitinib Alone versus Gefitinib Plus Chemotherapy for Non-small-cell Lung Cancer with Mutated Epidermal Growth Factor Receptor: NEJ009 Study. J. Clin. Oncol. 38, 115–123. doi:10.1200/JCO.19.01488
Isab, A. A., Altaf, M., Casagrande, N., Mariotto, E., Baig, N., Kawde, A. N., et al. (2019). Potent In Vitro and In Vivo Anticancer Activity of New Bipyridine and Bipyrimidine Gold (III) Dithiocarbamate Derivatives. Cancers 11, 474. doi:10.3390/cancers11040474
Jones, D. P., Go, Y. M., Roede, J. R., Walker, D. I., Duong, D. M., Seyfried, N. T., et al. (2013). Selective Targeting of the Cysteine Proteome by Thioredoxin and Glutathione Redox Systems. Mol. Cell. Proteomics. 12, 3285–3296. doi:10.1074/mcp.M113.030437
Kast, R. E. (2010). Glioblastoma Invasion, Cathepsin B, and the Potential for Both to Be Inhibited by Auranofin, an Old Anti-rheumatoid Arthritis Drug. Cent. Eur. Neurosurg. 71, 139–142. doi:10.1055/s-0029-1242756
Kawada, M., Momose, I., and Onodera, T. (2021). Potential Anticancer Activity of Auranofin. Yakugaku Zasshi. 141, 315–321. doi:10.1248/yakushi.20-00179-2
Kawada, M., Onodera, T., and Momose, I. (2019). Potential Anticancer Activity of Auranofin. Chem. Pharm. Bull. 67, 186–191. doi:10.1248/cpb.c18-00767
Kirkpatrick, P., Muhsin, M., and Graham, J. (2003). Gefitinib. Nat. Rev. Drug Discov. 2, 515–516. doi:10.1038/nrd1136
Korashy, H. M., Alhoshani, A., Alrashdi, A., Alhosaini, K., Alanazi, F. E., Alajez, N. M., et al. (2018). Gold-containing Compound BDG-I Inhibits the Growth of A549 Lung Cancer Cells through the Deregulation of miRNA Expression. Saudi Pharm. J. 26, 1035–1043. doi:10.1016/j.jsps.2018.05.012
Kühn, F. E., Rieb, J., Dominelli, B., Mayer, D., Jandl, C., Drechsel, J., et al. (2017). Influence of Wing-Tip Substituents and Reaction Conditions on the Structure, Properties and Cytotoxicity of Ag(i)- and Au(i)-Bis(NHC) Complexes. Dalton Trans. 46, 2722–2735. doi:10.1039/c6dt04559f
Liang, H., Huang, K. B., Wang, F. Y., Tang, X. M., Feng, H. W., Chen, Z. F., et al. (2018). Organometallic Gold(III) Complexes Similar to Tetrahydroisoquinoline Induce ER-Stress-Mediated Apoptosis and Pro-death Autophagy in A549 Cancer Cells. J. Med. Chem. 61, 3478–3490. doi:10.1021/acs.jmedchem.7b01694
Lin, M., Lum, C., Liu, X., Sun, R., Li, X., Peng, Y., et al. (2010). Gold(III) porphyrin 1a inhibited nasopharyngeal carcinoma metastasis in vivo and inhibited cell migration and invasion in vitro. Cancer Lett. 294, 159–166. doi:10.1016/j.canlet.2010.01.033
Liu, J., Li, X., Huang, Q., Long, H., Zhang, P., and Su, H. (2019). A New Gold(I) Complex-Au(PPh3)PT Is a Deubiquitinase Inhibitor and Inhibits Tumor Growth. EBioMedicine 39, 159–172. doi:10.1016/j.ebiom.2018.11.047
Liu, J., Liu, N., Li, X., Huang, H., Zhao, C., Liao, S., et al. (2014). Clinically Used Antirheumatic Agent Auranofin Is a Proteasomal Deubiquitinase Inhibitor and Inhibits Tumor Growth. Oncotarget 5, 5453–5471. doi:10.18632/oncotarget.2113
Liu, W., Bian, M., Sun, Y., Liu, Y., Xu, Z., Fan, R., et al. (2020). A Gold(I) Complex Containing an Oleanolic Acid Derivative as a Potential Anti-ovarian-cancer Agent by Inhibiting TrxR and Activating ROS-Mediated ERS. Chemistry 26, 7092–7108. doi:10.1002/chem.202000045
Liu, W. K., Lu, Y. L., Chang, X. Y., Liang, Z. L., Lv, L., Shan, M., et al. (2022). Recent Development of Gold(I) and Gold(III) Complexes as Therapeutic Agents for Cancer Diseases. Chem. Soc. Rev. 51, 5518–5556. doi:10.1039/d1cs00933h
Maia, P., Possato, B., Dalmolin, L. F., Pereira, L. M., Alves, J. Q., Silva, R. T. C., et al. (2021). Gold(III) Complexes with Thiosemicarbazonate Ligands as Potential Anticancer Agents: Cytotoxicity and Interactions with Biomolecular Targets. Eur. J. Pharm. Sci. 162, 105834. doi:10.1016/j.ejps.2021.105834
Marchetti, F., Bresciani, G., Busto, N., Ceccherini, V., Bortoluzzi, M., Pampaloni, G., et al. (2022). Screening the Biological Properties of Transition Metal Carbamates Reveals Gold(I) and Silver(I) Complexes as Potent Cytotoxic and Antimicrobial Agents. J. Inorg. Biochem. 227, 111667. doi:10.1016/j.jinorgbio.2021.111667
Marchetti, F., Caruso, F., Rossi, M., Tanski, J., and Pettinari, C. (2003). Antitumor Activity of the Mixed Phosphine Gold Species Chlorotriphenylphosphine-1, 3-bis(diphenylphosphino)Propanegold(I). J. Med. Chem. 46, 1737–1742. doi:10.1021/jm0204690
Marzano, C., Gandin, V., Fernandes, A. P., Rigobello, M. P., Dani, B., Sorrentino, F., et al. (2010). Cancer Cell Death Induced by Phosphine Gold(I) Compounds Targeting Thioredoxin Reductase. Biochem. Pharmacol. 79, 90–101. doi:10.1016/j.bcp.2009.07.023
Messori, L., Bindoli, A., Rigobello, M. P., Scutari, G., Gabbiani, C., and Casini, A. (2009). Thioredoxin Reductase: A Target for Gold Compounds Acting as Potential Anticancer Drugs. Coord. Chem. Rev. 253, 1692–1707. doi:10.1016/j.ccr.2009.02.026
Miller, M., and Hanna, N. (2021). Advances in Systemic Therapy for Non-small Cell Lung Cancer. BMJ 375, n2363. doi:10.1136/bmj.n2363
Novello, S., Leighl, N. B., Karaseva, N., Nakagawa, K., Cho, B. C., Gray, J. E., et al. (2020). Patient-reported Outcomes from FLAURA: Osimertinib versus Erlotinib or Gefitinib in Patients with EGFR-Mutated Advanced Non-small-cell Lung Cancer. Eur. J. Cancer 125, 49–57. doi:10.1016/j.ejca.2019.11.006
Ott, I., Zhang, J. J., Abu, El., Maaty, M. A., Hoffmeister, H., Schmidt, C., et al. (2020). A Multitarget Gold(I) Complex Induces Cytotoxicity Related to Aneuploidy in HCT-116 Colorectal Carcinoma Cells. Angew. Chem. Int. Ed. Engl. 59, 16795–16800. doi:10.1002/anie.202006212
Park, S. J., and Kim, I. S. (2005). The Role of P38 MAPK Activation in Auranofin-Induced Apoptosis of Human Promyelocytic Leukaemia HL-60 Cells. Br. J. Pharmacol. 146, 506–513. doi:10.1038/sj.bjp.0706360
Pawlak, K., Jakubczak, W., and Haczyk-Więcek, M. (2021). Attomole-per Cell Atomic Mass Spectrometry Measurement of Platinum and Gold Drugs in Cultured Lung Cancer Cells. Molecules 26, 7627. doi:10.3390/molecules26247627
Pizarro, A. M., Rodríguez-Fanjul, V., López-Torres, E., and Mendiola, M. A. (2018). Gold(III) Bis(thiosemicarbazonate) Compounds in Breast Cancer Cells: Cytotoxicity and Thioredoxin Reductase Targeting. Eur. J. Med. Chem. 148, 372–383. doi:10.1016/j.ejmech.2018.02.009
Rodriguez-Yoldi, M. J., Quero, J., Ruighi, F., Osada, J., Gimeno, M. C., and Cerrada, E. (2021). Gold(I) Complexes Bearing Alkylated 1, 3, 5-Triaza-7-Phosphaadamantane Ligands as Thermoresponsive Anticancer Agents in Human Colon Cells. Biomedicines 9, 1848. doi:10.3390/biomedicines9121848
Shea, B., Suarez-Almazor, M. E., Spooner, C. H., and Belseck, E. (2000). Auranofin versus Placebo in Rheumatoid Arthritis. Cochrane Database Syst. Rev. 2000, CD002048. doi:10.1002/14651858.CD002048
Silva, H., Garcia, A., Machado, R. C., Grazul, R. M., Lopes, M. T., Corrêa, C. C., et al. (2016). Novel Antitumor Adamantane-Azole Gold(I) Complexes as Potential Inhibitors of Thioredoxin Reductase. J. Biol. Inorg. Chem. 21, 275–292. doi:10.1007/s00775-016-1338-y
Sitole, L., Engelbrecht, Z., Roberts, K. E., Hussan, A., Amenuvor, G., Cronjé, M. J., et al. (2020). Synthesis and Anti-cancer Activity of Bis-Amino-Phosphine Ligand and its Ruthenium(II) Complexes. Bioorg. Med. Chem. Lett. 30, 127492. doi:10.1016/j.bmcl.2020.127492
Tan, D. S. W., Saw, S. P. L., Ong, B. H., Chua, K. L. M., and Takano, A. (2021). Revisiting Neoadjuvant Therapy in Non-small-cell Lung Cancer. Lancet Oncol. 22, 501–516. doi:10.1016/S1470-2045(21)00383-1
Tavares, D. C., Rinaldi-Neto, F., Ribeiro, A. B., Ferreira, N. H., Squarisi, I. S., Oliveira, K. M., et al. (2021). Anti-melanoma Effect of Ruthenium(II)-diphosphine Complexes Containing Naphthoquinone Ligand. J. Inorg. Biochem. 222, 111497. doi:10.1016/j.jinorgbio.2021.111497
Trávníček, Z., Křikavová, R., Hošek, J., Vančo, J., Hutyra, J., and Dvořák, Z. (2014). Gold(I)-triphenylphosphine Complexes with Hypoxanthine-Derived Ligands: In Vitro Evaluations of Anticancer and Anti-inflammatory Activities. PLoS One 9, e107373. doi:10.1371/journal.pone.0107373
Wang, F., Yang, L., Wang, H., Yang, X., Wu, Q., An, P., et al. (2020). Auranofin Mitigates Systemic Iron Overload and Induces Ferroptosis via Distinct Mechanisms. Signal Transduct. Target. Ther. 5, 138. doi:10.1038/s41392-020-00253-0
Wu, Y. L., Zhong, W. Z., Wang, Q., Mao, W. M., Xu, S. T., Wu, L., et al. (2021). Gefitinib versus Vinorelbine Plus Cisplatin as Adjuvant Treatment for Stage II-IIIA (N1-N2) EGFR-Mutant NSCLC: Final Overall Survival Analysis of CTONG1104 Phase III Trial. J. Clin. Oncol. 39, 713–722. doi:10.1200/JCO.20.01820
Yoshida, K., Hida, T., Ogawa, S., Park, J. C., Park, J. Y., Shimizu, J., et al. (2009). Gefitinib for the Treatment of Non-small-cell Lung Cancer. Expert Rev. Anticancer Ther. 9, 17–35. doi:10.1586/14737140.9.1.17
Zhang, J., Liang, H., Zhang, J., Zhang, Z., Jiang, M., Li, S., et al. (2020). Developing a Novel Gold(III) Agent to Treat Glioma Based on the Unique Properties of Apoferritin Nanoparticles: Inducing Lethal Autophagy and Apoptosis. J. Med. Chem. 63, 13695–13708. doi:10.1021/acs.jmedchem.0c01257
Zhang, Q., Sun, Q., Li, Y., Shi, H., Wang, Y., and Zhang, J. (2021b). Ruthenium Complexes as Promising Candidates against Lung Cancer. Molecules 26, 4389. doi:10.3390/molecules26154389
Zhang, Q., Zhang, J., Jiang, M., Li, S., Zhang, Z., Sun, H., et al. (2021a). Developing a Novel Anticancer Gold(III) Agent to Integrate Chemotherapy and Immunotherapy. J. Med. Chem. 64, 6777–6791. doi:10.1021/acs.jmedchem.1c00050
Zhang, Q., Zhao, Z., Hu, R., Shi, H., Wang, Y., Ji, L., et al. (2019). Design of Ruthenium-Albumin Hydrogel for Cancer Therapeutics and Luminescent Imaging. J. Inorg. Biochem. 194, 19–25. doi:10.1016/j.jinorgbio.2019.02.002
Zou, T., Jiang, J., Cao, B., Chen, Y., Luo, H., Xue, J., et al. (2022). Alkylgold(III) Complexes Undergo Unprecedented Photo-Induced β-Hydride Elimination and Reduction for Targeted Cancer Therapy. Angew. Chem. Int. Ed. Engl. 61, e202201103. doi:10.1002/anie.202201103
Zou, T., Long, Y., Cao, B., Xiong, X., Chan, A. S. C., and Sun, R. W. (2021). Bioorthogonal Activation of Dual Catalytic and Anti-cancer Activities of Organogold(I) Complexes in Living Systems. Angew. Chem. Int. Ed. Engl. 60, 4133–4141. doi:10.1002/anie.202013366
Keywords: Gold(I) complexes, gold(III) complexes, anticancer, lung cancer, A549
Citation: Zhang J, Li Y, Fang R, Wei W, Wang Y, Jin J, Yang F and Chen J (2022) Organometallic gold(I) and gold(III) complexes for lung cancer treatment. Front. Pharmacol. 13:979951. doi: 10.3389/fphar.2022.979951
Received: 28 June 2022; Accepted: 01 August 2022;
Published: 13 September 2022.
Edited by:
Li Liu, University of Texas Southwestern Medical Center, United StatesReviewed by:
Wukun Liu, Nanjing University of Chinese Medicine, ChinaRuo Wang, Shanghai Jiao Tong University, China
Copyright © 2022 Zhang, Li, Fang, Wei, Wang, Jin, Yang and Chen. This is an open-access article distributed under the terms of the Creative Commons Attribution License (CC BY). The use, distribution or reproduction in other forums is permitted, provided the original author(s) and the copyright owner(s) are credited and that the original publication in this journal is cited, in accordance with accepted academic practice. No use, distribution or reproduction is permitted which does not comply with these terms.
*Correspondence: Feng Yang, bWFpbHRvOmZ5YW5nQG1haWxib3guZ3hudS5lZHUuY24=, Jian Chen, bWFpbHRvOmNoZW5qaWFuQGdsbWMuZWR1LmNu
†These authors have contributed equally to this work