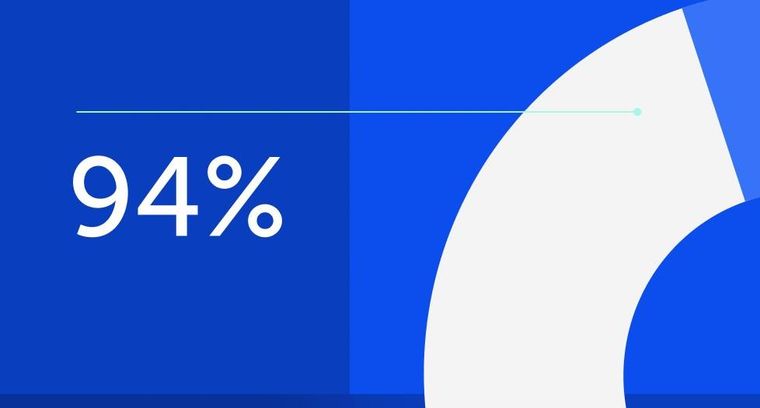
94% of researchers rate our articles as excellent or good
Learn more about the work of our research integrity team to safeguard the quality of each article we publish.
Find out more
REVIEW article
Front. Pharmacol., 16 September 2022
Sec. Respiratory Pharmacology
Volume 13 - 2022 | https://doi.org/10.3389/fphar.2022.978151
This article is part of the Research TopicAdvances on Epigenetics Pharmacological Research for Chest-related DiseaseView all 4 articles
Non-coding RNA is still one of the most popular fields in biology research. In recent years, people paid more attention to the roles of H19 in lung diseases, which expressed abnormally in various pathological process. Therefore, this review focus on the regulatory role of H19 in asthma, pulmonary arterial hypertension (PAH), idiopathic pulmonary fibrosis (IPF), lung injury, pneumonia, lung cancer, etc. And the potential therapeutic agents and molecular treatments of H19 are collected. The aim is to demonstrate its underlying mechanism in pulmonary diseases and to guide the basic research targeting H19 into clinical drug translation.
The central dogma of genetic inheritance states that DNA is transcribed into RNA, which is then translated into protein. However, protein-coding RNAs make up only a proportion of total RNA, leaving more non-coding RNAs. Non-coding RNAs are now known to have many regulatory functions concerning gene expression, and interactions with DNA, RNA, and protein have all been reported (Hangauer et al., 2013). Non-coding RNAs act as inducers, mediators, guides, or signal molecules, influencing many biological processes, including cell proliferation and differentiation (Wang et al., 2021a). For example, with respect to pulmonary dysfunction, the lncRNA TUG1 has been found to sponge miR-222-3p with the result that the expression of the genes encoding CELF1 and p53 are up-regulated (Li et al., 2021).
Non-coding RNAs are broadly divided into two classes based on their length, with long non-coding RNAs (lncRNA) having greater than 200 nucleotides and short non-coding RNAs, predominantly micro-endogenous RNAs having fewer than 200 nucleotides. LncRNAs, in particular, have been extensively studied and are known to have many functions (Li et al., 2019a).
One member of the lncRNA family, H19, is abundant and shows a high degree of conservation. Its involvement in cancer (Muller et al., 2019), diabetes (Li et al., 2020a), cardiovascular (Zhang et al., 2018), and cerebrovascular disease (Feng et al., 2021) has been reported. For example, H19 binds hnRNPA2B1 and induces the epithelial-mesenchymal transformation, and promotes metastasis and invasion of colorectal cancer cells (Zhang et al., 2020a).
In recent years, research on drugs targeting lncRNAs has gradually emerged, and reviews have discussed the progress and challenges encountered in the development of lncRNAs as potential biomarkers and molecular drugs (Winkle et al., 2021). Similarly, H19, a clinical marker with broad application prospects, is more and more concerned in lung diseases. The review aims to summarize the roles of H19 in pulmonary diseases to reveal the therapeutic target and the direction to improve treatment.
H19 is a maternally imprinted gene located on the short arm of chromosome 11 at locus 11P15.5 (Ghafouri-Fard et al., 2020). It is a single-copy gene that shows a high degree of conservation. The 2.5 kb length consists of 5 exons and 4 introns and generates a mature 2.3 kb transcript lacking an open reading frame (Cai and Cullen, 2007). H19 is expressed at a high rate during development in utero but is down-regulated after birth, with heart and skeletal muscle being two of the few tissues that retain residual expression (Martinet et al., 2016). The expression may be detected predominantly in the cytosol, but H19 is also present in the nucleus (Nordin et al., 2014). Transcription is considered to result from the activity of RNA polymerase II and an antisense RNA molecule of H19, 91H, has also been detected, although its function remains unclear (Pachnis et al., 1988; Berteaux et al., 2008).
The current study found that H19 has four regulatory functions (Schoenfelder et al., 2007). ① H19/miR-675 axis: H19 is the precursor for the miR-675 and the sequence of miR-675 is found in the first exon of the H19 sequence. H19/miR-675 axis has many roles in cell growth and proliferation (Keniry et al., 2012). For instance, the H19/miR-675 axis has been implicated in the growth and migration of human squamous cell carcinoma cells (Zhang et al., 2021a). ② Binding to miRNAs: H19 is known to bind a range of microRNAs, including miRNA-138, let-7 and miRNA-200a and separately buffer their individual effects on target genes, such as Vimentin, Integrin β3, and ZEB1/ZEB2 to change the phenotype of the diseases (Liang et al., 2015; Zhao et al., 2019a; He et al., 2019). ③ Recruiting proteins: H19 is also known to bind to a range of proteins, thereby modulating downstream activities. By this mechanism, H19 binds to EZH2 and inhibits E-cadherin expression, promoting the metastasis of bladder cancer cells (Luo et al., 2013; Zhu et al., 2018). ④ Epigenetic effects: epigenetic effects have also been reported for H19 that binding to gene promoter regions, histone modification enzymes and transcription factors to affect gene expression. For instance, H19 is thought to induce p-glycoprotein expression and by regulating the methylation of MDR1 promoter with the effect that drug resistance is promoted in hepatocellular carcinoma cells (Tsang and Kwok, 2007). The physiological effects outlined above confirm that H19 is a biomarker and potential therapeutic target in diseases such as cancers and cardiovascular diseases (Liu et al., 2019a). We have plotted the mechanism of H19 in Figure1.
FIGURE 1. The regulatory roles of H19. (A) H19 can indirectly regulates downstream molecules by its spliceosome miR-675-3p or miR-675-5p. (B) H19 can regulates downstream molecules by acting as a “miRNA sponge”. (C) H19 can regulates downstream molecules by binding to functional proteins. (D) By epigenetic pathways, H19 can regulate modification enzymes, transcription factors and promoters to regulate downstream molecular expression.
Lung cancer, pulmonary arterial hypertension, asthma, and many other diseases are all regarded as systemic disorders with manifestations in the lung. Many such disorders have reported the involvement of H19, among which lung cancer makes frequent appearances in the medical literature. Different effects have been characterized in different cell types. For example, H19 promotes pulmonary fibrosis by inhibiting the expression of miR-140 in human lung fibroblasts (HFL), leading to enhanced deposition of extracellular matrix (Wang et al., 2019). Further specific regulatory roles have been recorded in airway smooth muscle cells (ASMC), myoblasts, cardiomyocytes, small cell lung cancer lines (SCLC), and non-small cell lung cancer lines (NSCLC). Pathways and downstream targets are summarized in Table 1. All the above lung diseases are worthy of further discussion during this review with respect to the roles of H19.
Asthma is characterized by episodic and reversible airway constriction as part of an inflammatory response to environmental allergens, infections and irritants. It is a complex, multifactorial immune-mediated process with multiple clinical types and airway remodeling, promoted by abnormal proliferation and migration of smooth muscle cells (Lambrecht and Hammad, 2012; Patel and Teach, 2019). The work of Chen has shown that H19 regulated the expression of mucoprotein 5AC (Muc5ac) through the PI3K/Akt/NF-κB pathway in an in vitro mouse model of inflammation in which the expression of H19 was low (Chen et al., 2021a). And Low expression of H19 has been proposed as a protective mechanism in mouse models of asthma. The inhibitors of phosphatidylinositol 3-kinase (PI3K) eliminated the promotion of Muc5ac expression by H19 through dephosphorylation of protein kinase B (Akt) and subsequent activation of nuclear factor kappa-B (NF-κB). Muc5ac is a central effector of allergic inflammation involved in airway hyper reactivity (AHR) (Evans et al., 2015). The activity of Muc5ac may lead to mucus blockage and airway inflammation worsening asthma symptoms. Therefore, H19 inhibition may alleviates the development of asthma by downregulating Muc5ac.
Changes in pulmonary vascular structure or function may lead to increased vascular resistance and arterial pressure culminating in the pathological presentation of pulmonary arterial hypertension (PAH). The condition is defined as a resting mean pulmonary arterial pressure (mPAP), measured by right heart catheterization, in excess of 25 mmHg (Galie et al., 2016). Pulmonary vascular remodeling and right ventricular (RV) hypertrophy or even failure may result from PAH (Hoffmann et al., 2016).
Vascular remodeling results from excessive proliferation of pulmonary artery smooth muscle cells and the dysfunction of arterial endothelial cells (Kim, 2014). There is a great deal of evidence to suggest the involvement of the H19-let-7b axis in vascular remodeling (Sun et al., 2019). H19, in a role as a competing endogenous RNA (ceRNA), binds to let-7b to down-regulate its expression and upregulate the expression of cyclinD1. The result is the proliferation of vascular smooth muscle and vascular remodeling (Sun et al., 2019). It has also been shown that by binding and sequestration of let-7b, H19 can upregulate the expression of the angiotensin I receptor-1 (AT1R) in rat pulmonary artery smooth muscle cells (PASMCs) stimulated by platelet-derived growth factor-BB (PDGF-BB) (Su et al., 2018). The binding of angiotensin II to AT 1 R activates the MAPK signaling pathway to promote the proliferation of blood vessels and smooth muscle cells, contributing to vascular remodeling.
The foregoing results all indicate a pathological role for H19 in PAH. However, there is a contradictory finding. Wang reported elevated H19 in a melatonin-mediated rat model of PAH, in which the H19-miR-200a-PDCD4 axis played a therapeutic role (Wang et al., 2018a). There may be environmental and time exposure factors accounting for the opposite finding.
H19 regulated cardiomyocyte (CM) hypertrophy, promoted CM apoptosis, and stimulated proliferation and fibrosis of cardiac fibroblasts in vitro, all of which are related to the progression of right ventricular failure (Liu et al., 2018; Choong et al., 2019). Omura used CMs from rats with pulmonary hypertension to demonstrate that H19 silencing upregulates the histone methyltransferases, enhancer of Zeste homolog 2 (EZH2) and E2F1 (Omura et al., 2020). Upregulation of EZH2 may contribute to improving CM cross-sectional area. However, any specific regulatory relationships among H19, EZH2, and E2F1 remain unclear. E2F1 is known to be a target of miR-675 and a trans-activator of EZH2 (Ma et al., 2018). Therefore, H19 is likely to inhibit the expression of EZH2 through the miR-675-E2F1-EZH2 axis. EZH2 exerts epigenetic effects to inhibit the expression of sine oculis homeobox homolog 1 (six1) (Gao et al., 2020). Decreased six1 reduced the expression of cardiac sarco-endoplasmic reticulum Ca2+ ATPase marker (SERCA2a) (Meyer et al., 1995). All in all, H19 knockdown may reduce myocardial hypertrophy and heart failure through this cascade.
In addition, H19 inhibits the expression of y-box binding protein 1 (YB1), leading to de-inhibition of type 1 collagenα1 (Col1α1) and a variety of miRNAs, such as miR-29b. The resulting changes in the extracellular matrix may reduce CM survival and promote fibrosis (Choong et al., 2019).
In conclusion, we suggest that H19 may have a central role in the process of vascular smooth muscle cell proliferation and CM extracellular matrix alteration making it a suitable drug target for PAH.
Idiopathic pulmonary fibrosis (IPF) has a high mortality rate, and there is no effective drug treatment (Nathan and Meyer, 2014). Two features characterize IPF: the phenotypic transformation of alveolar epithelial cells and excessive deposition of extracellular matrix collagen (Ley et al., 2011). The involvement of miRNAs has been confirmed in pulmonary fibrosis, and H19 has been shown to bind the 3′UTR and inhibit the expression of the fibrosis regulator, miR-29b (Tang et al., 2016). Moreover, miR-29b inhibits col1a1 via an effect on the PI3K/Akt pathway (Li et al., 2012). Transforming growth factor1 (TGFb1) induces deposition of extracellular collagen to mediate pulmonary fibrosis in vitro. Its role in cell proliferation requires the activity of miR-29, and miR-29 overexpression inhibits TGFb1-induced lung fibroblast proliferation (Tang et al., 2016). Indeed, miR-29 may regulate fibrosis by regulating the TGFb1 signaling pathway (Li et al., 2016). Besides, Lu also confirmed that H19 could promote IPF. As a ceRNA, H19 can compete with miR-196a to positively regulate colla1 and promote the development of IPF (Lu et al., 2018). Similarly, Wang has reported that H19 exerts a promotional effect on pulmonary fibrosis by reducing the expression of miR-140, inhibiting the TGF-b/smad3 pathway (Wang et al., 2019). Optimal levels of TGF-β-smad3 signaling appear to be critical for secondary alveolar septum formation. Over-expression of TGF-β and TGF-β3 receptors may lead to invasive and transient pulmonary fibrosis, respectively (Warburton et al., 2013).
There are many types of lung injury, and acute lung injury (ALI) is the most common one. Inflammation is the main feature of lung injury (Vishnupriya et al., 2020). H19 has been confirmed to be closely associated with various inflammatory genes, regulating the expression of inflammatory genes (Wang et al., 2020a). Wu’s study showed that H19 was significantly expressed in LPS-induced acute lung injury in rats, and overexpression of H19 may be a protective mechanism (Wu et al., 2018). In contrast to the role of H19 in promoting disease progression in most diseases, overexpression of H19 negatively regulated miR-181a, promoting the expression of runt-related transcription factor 2 (Runx2), alleviating LPS-induced cell damage, and ameliorating LPS-induced acute lung injury (Wu et al., 2018). The same experiment confirmed that Runx2 activated the JNK and Notch pathways to promote cell proliferation and survival. In this study, H19 was shown to promote the proliferation of lung fibroblast cells (MRC-5 cells) to attenuate LPS-induced lung injury. However, in IPF, the overexpression of H19 promotes the proliferation of fibroblasts, which will cause the aggravation of IPF. For different diseases, the promotion of lung fibroblast cells proliferation by H19 has the opposite effect on the development of disease.
The incidence of smoke-induced lung injury (SILI) involves inflammatory stimulation (Guo et al., 2019). Ginsenoside Rb3 has been shown to inhibit H19 and play a therapeutic role in SILI. H19 acts as a sponge for miR-29b-3p to regulate its expression negatively. In addition, miR-29b-3p inhibited the expression of toll-like receptor 4 (TLR4) and alleviated lung injury by inhibiting the expression of HMGB1 mRNA (Tan et al., 2021). TLR4 promoted the production of inflammatory mediators (Yang et al., 2020).
Another lung injury associated with inflammation is bronchopulmonary dysplasia (BPD), which is disproportionately common in infants (Gilfillan et al., 2021). Zhang proved that inhibition of H19 could significantly improve alveolar congestion and inflammatory infiltration in BPD mice (Zhang et al., 2020b). H19 has a negative regulatory effect on miR-17, competitively binding to it and upregulating STAT3 to promote inflammation. STAT3 activates inflammatory pathways, including NF-κB in cancer (Yu et al., 2009). Therefore, regulation of the H19/miR-17/STAT3 axis may have therapeutic effects on BPD.
Pneumonia is an acute respiratory tract, including community-acquired pneumonia (CAP) and hospital-acquired pneumonia (Torres et al., 2021). Infection of the lungs and damage to the immune barrier by pathogens are the leading cause of pneumonia (Torres et al., 2021). Sun showed that H19 was significantly increased in LPS-induced pneumonia models and sponging of miR-22-3p positively regulated NLRP3 to promote cell pyroptosis (Sun et al., 2021). The NLRP3 inflammasome promoted the activation and release of protease caspase-1 to induce gasdermin D-mediated pyroptosis (Huang et al., 2021). Another study also showed that inhibiting the expression of H19 can alleviate inflammation by regulating miR-140-5p/TLR4 axis in pneumonia (Yang, 2022). The LPS receptor, TLR4, may promote LPS-mediated endogenic transduction of inflammatory signals (Zamyatina and Heine, 2020). In conclusion, H19 plays a positive promoting role in the pneumonia model. Therefore, H19-targeted therapy may play a role in the treatment of pneumonia.
Lung cancer is the most common cancer-type worldwide (Sung et al., 2021). It can broadly be divided into two types: non-small cell lung cancer (NSCLC) which accounts for 80%–85% of total lung cancer (40% adenocarcinoma, 25%–30% squamous cell carcinoma, and 10%–15% large cell carcinoma) and small cell lung cancer (SCLC) (Schabath and Cote, 2019). H19 may promote the proliferation and migration of tumor cells.
SCLC only accounts for about 15%–20% of lung cancer cases. However, its propensity for metastasis means that about two-thirds of patients have distant metastatic disease at the initial diagnosis, giving them an abysmal prognosis and low survival rate (Hou et al., 2012). Knockout of H19 has been shown to inhibit the proliferation and migration of SCLC cells. Li demonstrated that H19 regulates the expression of the tumor suppressor gene, miR-140-5p (Li et al., 2020b). The expression of miR-140-5p had an inhibitory action on cell invasion and epithelial-mesenchymal transformation of oral squamous cell carcinoma (Zhao et al., 2019b). The oncogene, fibroblast growth factor (FGF9), is a target of miR-140-5p in SCLC. And miR-140-5p can bind to 3′-UTR of FGF9 and inhibits its expression (Wang et al., 2020b). Therefore, through negative regulation of miR-140-5p, H19 stimulates the tumor-promoting activity of FGF9 in SCLC. The detailed findings expose the possibility that H19 could be a therapeutic target in SCLC and have potential as a biomarker. Additional mechanistic details should be explored.
NSCLC includes adenocarcinoma, squamous cell carcinoma, and large cell carcinoma, among which lung adenocarcinoma accounts for the highest proportion. H19 has been shown to reduce miR-484 expression in A549 cells in vitro (Zhang et al., 2021b). Through its negative regulation of the cytokinesis and proliferation factor, Rho-associated kinase 2 (ROCK2), miR-484 would usually exert a tumor-suppressing activity (Hartmann et al., 2015; Liu and Li, 2020). ROCK2 overexpression has been shown to increase the levels of phosphorylated stress-activated protein kinase (JNK), transcription regulation factors C-Jun, and mesenchymal markers but to decrease levels of epithelial markers. This suggests that ROCK2 regulates the epithelial-mesenchymal transformation (EMT), including loss of cell polarity and increased mobility (Zhang et al., 2021b). EMT promotes invasive metastasis, resistance to anti-tumor drugs, and various stress responses in tumor cells (Nieto, 2017). Therefore, H19 knockout influences proliferation, invasion, and metastasis by upregulating mir-484 and down-regulating ROCK2 to inhibit the EMT in lung cancer cells.
It has been suggested that the activity of H19-induced DNA methyltransferase1 (DNMT1) and DNA methyltransferase3 (DNMT3) promoted methylation and silencing of the Cadherin 1 (CDH1) promoter (Gao et al., 2019). In addition, CDH1-inhibition may promote TGF-β1-induced EMT and tumor invasion in breast cancer (Cano-Gonzalez and Lopez-Rivas, 2016). These findings suggest that inhibition of H19 and CDH1 methylation may inhibit EMT and cell proliferation, promoting apoptosis of lung adenocarcinoma cells. Moreover, H19, as a ceRNA, targets miR-29b-3p to limit its expression (Liu et al., 2019b). The target gene of miR-29b-3p, signal transducer and activator of transcription 3 (STAT3), promotes differentiation of bone-marrow-derived inhibitory cells (MDSCs) and acts as a tumor promoter (Liu et al., 2019b). It may be that STAT3, activated by H19, increases proliferation and decreases apoptosis of lung adenocarcinoma cells and activates the expression of EMT-specific proteins.
H19 has been shown to regulate miR-148b-3p and promote the expression of dimethylarginine dimethylaminohydrolase 1 (DDAH1) through negative feedback in lung adenocarcinoma cells resistant to gefitinib (Huang et al., 2020a). DDAH1 has been shown to inhibit the EMT in gastric cancer, suppressing tumor growth, but is considered a risk factor in breast cancer and cardiovascular systems (Palm et al., 2007; Cano-Gonzalez and Lopez-Rivas, 2016; Hulin et al., 2017). Further research is necessary to explain why DDAH1 shows adverse effects in different disease systems. In addition, H19 may act as a competitive inhibitor of microRNA-107, which usually modulates degradation of the RAS signal transduction pathway negative regulator, NF1 (Qian et al., 2018). Proliferation and migration of NSCLC cells would thus increase in the presence of H19. NF1 is known to regulate metastasis of hepatocellular carcinoma cells (Li et al., 2015). And NF1 is a GTP-activating protein that acts as a Ras protein shutdown signal. Patients who lack NF1 have higher levels of RAS-GTP, which promotes cancer cell growth and differentiation (Brundage et al., 2014). The forkhead box protein F2 (FOXF2) transcription factor has been shown to stimulate H19 transcription, leading to H19-mediated silencing of the tumor suppressor gene, PTEN, by recruitment of EZH2 (Xu et al., 2019). Thus, the progression of NSCLC is exacerbated. Another study showed that Curcumenol could effectively inhibit the expression of H19 and promote the occurrence of ferroptosis in lung cancer (Zhang et al., 2022). H19 acts as the “ceRNA” of miR-19b-3p and promotes the expression of FTH1, which plays a promoting role in lung cancer. FTH1 is an inhibitor of ferroptosis (Tian et al., 2020). Therefore, Curcumenol can target H19 to treat lung cancer.
Finally, we summarized the current studies on H19 in lung diseases (Figure 2) to explain the potential therapeutic value of H19 in lung diseases.
FIGURE 2. The mechanisms and related targets of H19 in lung diseases. H19 acts as a sponge for miR-140, miR-29b, and miR-196a to promote cellular fibrosis. H19 promotes cell hypertrophy by splicing to produce miR-675. H19 acts as a sponge for miR-21 and miR-181a to promote cell proliferation. H19 promotes inflammation by acting as a sponge for miR-17, miR-29b-3p, and miR-140-5p and regulating the PI3K/Akt pathway through epigenetic pathways. H19 regulates cell death by binding to YB1 and acting as a sponge for miR-22-3p and miR-19b-3p. H19 promotes cell carcinogenesis by acting as a sponge for microRNA107 and regulating EZH2 expression through epigenetic pathways. H19 acts as a miR-29b-3p, miR-148b-3p, and miR-484 sponge and regulates the expression of CDH1 promoter through epigenetic pathways to promote cancer cell proliferation and metastasis.
A summary of the drugs targeting H19 from a search of previous publications is shown in Table 2. It is not difficult to see from the table that there are currently three drugs targeting H19 to treat lung diseases, namely melatonin, Curcumenol and Ginsenoside Rb3. Both Curcumenol and Ginsenoside Rb3 inhibit H19 expression through the H19/miR-19b-3p/FTH1 axis and the H19/miR-29b-3p/HGMB1/TLR4 axis to promote ferroptosis in lung cancer cells and reduce inflammation (Tan et al., 2021; Zhang et al., 2022). But Wang showed that melatonin upregulated H19 expression to suppress PAH, in contrast to other reports that H19 promoted this disease (Wang et al., 2018a). In this study, H19 was identified as a positive factor that inhibits the development of the disease.
Currently, we have not found drugs that can target H19 to treat diseases such as pulmonary fibrosis, asthma and pneumonia. Drugs targeting H19 remain a potentially fruitful area of research.
The pathogenesis of asthma and pneumonia are all related to the production of inflammation, and pulmonary fibrosis is related to the production of fibrosis, and H19 is highly expressed in these diseases without exception. Studies by others have found that metformin suppressed inflammation in diabetic nephropathy by inhibiting H19 expression and that dihydroartemisinin inhibited the development of liver fibrosis (Xu et al., 2020; Xia et al., 2021). Therefore, we can also hypothesize whether drugs such as metformin and dihydroartemisinin may target H19 in lung disease. We prospect that future research may demonstrate the applicability of these drugs.
In recent years, monomeric chemical constituents in botanicals have been widely used to target H19 to inhibit disease progression. Using existing online databases, including TCMSP and miRbase, facilitates the bioinformatics-based search for drugs targeting H19.
RNA-based therapeutics, including various antisense oligonucleotides (ASOs), small interfering RNAs (siRNAs), and miRNA sponges, have been approved by the European Medicines Agency (EMA), demonstrating the clinical feasibility of RNA therapeutics (Winkle et al., 2021). However, no effective lncRNA-based therapy has been approved in the clinic, and most are in Phase II or Phase III clinical development. The diverse functions of lncRNAs indicate various opportunities for their therapeutic targeting, and corresponding molecular drugs can be developed by adjusting lncRNA modes of action, such as transcriptional inhibition, post-transcriptional inhibition, or interaction with proteins. We summarize possible future molecular therapies targeting H19.
With the continuous discovery of lncRNA structural information and its functional motifs, the design or discovery of small molecule inhibitors of related targets has become a reality. For example, the methylation inhibitor 5-azathioprine-2′-deoxycytidine (5-aza-dC) inhibited the methylation of the ICR region of the H19 gene and down-regulated the expression of the H19 gene (Zhao et al., 2012).
The regulatory sequence of H19 has been evaluated as a promising and safe targeted therapy in phase 1 and 2 studies in pancreatic cancer (Hanna et al., 2012). Hochberg et al. constructed a vector named DTA-H19, and it was regulated by the regulatory sequences of H19. The vector can selectively kill H19-positive cells by expressing the diphtheria toxin A chain gene. Anticancer effects have been demonstrated for this treatment in phase I/IIa clinical studies in patients with superficial bladder cancer, unresectable pancreatic cancer, or ovarian cancer (Sidi et al., 2008; Hanna et al., 2012; Gofrit et al., 2014; Lavie et al., 2017). Furtherly, another new DTA vector regulated by double regulatory sequences, H19-DTA-P4-DTA, was subsequently developed, with better anticancer effects than before (Amit and Hochberg, 2010).
The developments of nucleic acid drugs siRNA and antisense oligonucleotides (ASOs) make the development of drugs targeting interfering lncRNAs possible. The development of siRNAs and antisense oligonucleotides (ASOs) have the same effect on degrading the lncRNAs, having pointed out another direction to knock down the expression of lncRNAs (Chen et al., 2021b). For instance, The researchers found that the expression of lncRNA DANCR could be modulated with siRNA nanoparticles for the treatment of non-small cell lung cancer (Nicolescu et al., 2022). Other researchers have constructed an ASO targeting lncRNA MALAT1, which can significantly inhibit the metastasis and invasion of rat lung cancer cells. These third-generation modified ASOs use Au nanoparticles as a carrier, which maintain the stability and good biological activity of ASOs (Gong et al., 2019).
Effective delivery methods may solve the clinical translation of RNA therapy, so we list promising delivery methods for H19-targeting drugs in the future.
Cell-derived exosomes are a good delivery vehicle with minimal antigenicity and cytotoxicity, which achieve cell-to-cell information transfer (Bayraktar et al., 2017). It has been reported that H19 mediated the development of cancer cells such as hepatocellular carcinoma by means of exosomes (Conigliaro et al., 2015). Higher levels of H19 were also found in serum exosomes from bladder cancer (Wang et al., 2018b). The above suggests that delivering H19 through the exosome pathway may be a better drug delivery method in future studies.
Currently, lipid nanoparticles (LNPs) are regarded as the most effective way to deliver siRNA by exogenous carriers due to their easy modification, high biodegradability, and high biocompatibility (Winkle et al., 2021). Ligand-conjugated siRNA therapy based on N-acetylgalactosamine (GalNAc) has shown promising results in hepatocytes (Rajeev et al., 2015). Another delivery mode of metal-based NPs has also attracted attention and may be combined with various compounds for delivery (Khoshnevisan et al., 2018). Therefore, these delivery methods are already undergoing clinical studies and are expected to be used for H19-targeted therapy in the future.
H19 has been extensively studied in cancer, endocrine, and cardiovascular diseases. Given that H19 plays an important regulatory role in lung diseases such as lung cancer, pulmonary fibrosis, and pneumonia, targeting H19 is a promising area of research. Of course, there are still some difficulties in solving the clinical translation of many RNA-based therapies, such as specificity and delivery of molecular drugs. But new chemical modification methods and delivery methods are constantly improving. In addition, drugs targeting H19 with plant-based, low toxicity and few side effects are gotten attention in recent years. Using existing botanical databases and combining with bioinformatics technology, active ingredients targeting H19 can be quickly found to facilitate subsequent research. We prospect that the molecular therapy targeting H19 and related molecular drugs will be applied to clinical therapies.
JX and JZ contributed to write and edit the manuscript. YH and CL helped to collect documents. JZ reviewed and performed critical revision of the manuscript. JX designed the pictures and DS helped to revise them. ZL supervised the manuscript and gave valuable suggestions. All authors have read and agreed to the published version of the manuscript.
This study is supported by a row surface of the National Science Foundation of China (grant number 81974569), the National Natural Science Foundation of China, Joint Fund for Regional Innovation and Development (grant number U20A20398), a row surface of the China Postdoctoral Foundation (grant number 2018M640345), Collaborative Innovation Project of Universities in Anhui Province (grant number GXXT-2021-084), Open project of Key Laboratory of Multimodal cognitive Computing of Anhui Province (Anhui University) (grant number MMC202002).
We thank The Key Laboratory of Traditional Chinese Medicine Prevention and Control on Respiratory Disease. We also would like to express our gratitude to EditSprings (https://www.editsprings.cn) for the expert linguistic services provided.
The authors declare that the research was conducted in the absence of any commercial or financial relationships that could be construed as a potential conflict of interest.
All claims expressed in this article are solely those of the authors and do not necessarily represent those of their affiliated organizations, or those of the publisher, the editors and the reviewers. Any product that may be evaluated in this article, or claim that may be made by its manufacturer, is not guaranteed or endorsed by the publisher.
Amit, D., and Hochberg, A. (2010). Development of targeted therapy for bladder cancer mediated by a double promoter plasmid expressing diphtheria toxin under the control of H19 and IGF2-P4 regulatory sequences. J. Transl. Med. 8, 134. doi:10.1186/1479-5876-8-134
Bayraktar, R., Van Roosbroeck, K., and Calin, G. A. (2017). Cell-to-cell communication: microRNAs as hormones. Mol. Oncol. 11 (12), 1673–1686. doi:10.1002/1878-0261.12144
Berteaux, N., Aptel, N., Cathala, G., Genton, C., Coll, J., Daccache, A., et al. (2008). A novel H19 antisense RNA overexpressed in breast cancer contributes to paternal IGF2 expression. Mol. Cell. Biol. 28 (22), 6731–6745. doi:10.1128/MCB.02103-07
Brundage, M. E., Tandon, P., Eaves, D. W., Williams, J. P., Miller, S. J., Hennigan, R. H., et al. (2014). MAF mediates crosstalk between Ras-MAPK and mTOR signaling in NF1. Oncogene 33 (49), 5626–5636. doi:10.1038/onc.2013.506
Cai, B., Ma, W., Bi, C., Yang, F., Zhang, L., Han, Z., et al. (2016). Long noncoding RNA H19 mediates melatonin inhibition of premature senescence of c-kit(+) cardiac progenitor cells by promoting miR-675. J. Pineal Res. 61 (1), 82–95. doi:10.1111/jpi.12331
Cai, X., and Cullen, B. R. (2007). The imprinted H19 noncoding RNA is a primary microRNA precursor. RNA 13 (3), 313–316. doi:10.1261/rna.351707
Cano-Gonzalez, A., and Lopez-Rivas, A. (2016). Opposing roles of TGF-beta and EGF in the regulation of TRAIL-induced apoptosis in human breast epithelial cells. Biochim. Biophys. Acta 1863 (8), 2104–2114. doi:10.1016/j.bbamcr.2016.05.011
Chen, X., Yang, J., Shen, H., Zhang, X., Wang, H., Wu, G., et al. (2021). Muc5ac production inhibited by decreased lncRNA H19 via PI3K/akt/NF-kB in asthma. J. Asthma Allergy 14, 1033–1043. doi:10.2147/JAA.S316250
Chen, Y., Li, Z., Chen, X., and Zhang, S. (2021). Long non-coding RNAs: From disease code to drug role. Acta Pharm. Sin. B 11 (2), 340–354. doi:10.1016/j.apsb.2020.10.001
Chen, Z., Wei, H., Zhao, X., Xin, X., Peng, L., Ning, Y., et al. (2019). Metformin treatment alleviates polycystic ovary syndrome by decreasing the expression of MMP-2 and MMP-9 via H19/miR-29b-3p and AKT/mTOR/autophagy signaling pathways. J. Cell. Physiol. 234 (11), 19964–19976. doi:10.1002/jcp.28594
Choong, O. K., Chen, C. Y., Zhang, J. H., Lin, J. H., Lin, P. J., Ruan, S. C., et al. (2019). Hypoxia-induced H19/YB-1 cascade modulates cardiac remodeling after infarction. Theranostics 9 (22), 6550–6567. doi:10.7150/thno.35218
Conigliaro, A., Costa, V., Lo Dico, A., Saieva, L., Buccheri, S., Dieli, F., et al. (2015). CD90+ liver cancer cells modulate endothelial cell phenotype through the release of exosomes containing H19 lncRNA. Mol. Cancer 14, 155. doi:10.1186/s12943-015-0426-x
Evans, C. M., Raclawska, D. S., Ttofali, F., Liptzin, D. R., Fletcher, A. A., Harper, D. N., et al. (2015). The polymeric mucin Muc5ac is required for allergic airway hyperreactivity. Nat. Commun. 6, 6281. doi:10.1038/ncomms7281
Feng, M., Zhu, X. F., and Zhuo, C. J. (2021). H19/miR-130a-3p/DAPK1 axis regulates the pathophysiology of neonatal hypoxic-ischemia encephalopathy. Neurosci. Res. 163, 52–62. doi:10.1016/j.neures.2020.03.005
Galie, N., Humbert, M., Vachiery, J. L., Gibbs, S., Lang, I., Torbicki, A., et al. (2016). 2015 ESC/ERS guidelines for the diagnosis and treatment of pulmonary hypertension: The Joint task force for the diagnosis and treatment of pulmonary hypertension of the European society of cardiology (ESC) and the European respiratory society (ERS): Endorsed by: Association for European paediatric and congenital cardiology (AEPC), international society for heart and lung transplantation (ISHLT). Eur. Heart J. 37 (1), 67–119. doi:10.1093/eurheartj/ehv317
Gao, L. M., Xu, S. F., Zheng, Y., Wang, P., Zhang, L., Shi, S. S., et al. (2019). Long non-coding RNA H19 is responsible for the progression of lung adenocarcinoma by mediating methylation-dependent repression of CDH1 promoter. J. Cell. Mol. Med. 23 (9), 6411–6428. doi:10.1111/jcmm.14533
Gao, W., Guo, N., Zhao, S., Chen, Z., Zhang, W., Yan, F., et al. (2020). FBXW7 promotes pathological cardiac hypertrophy by targeting EZH2-SIX1 signaling. Exp. Cell Res. 393 (1), 112059. doi:10.1016/j.yexcr.2020.112059
Ghafouri-Fard, S., Esmaeili, M., and Taheri, M. (2020). H19 lncRNA: Roles in tumorigenesis. Biomed. Pharmacother. 123, 109774. doi:10.1016/j.biopha.2019.109774
Gilfillan, M., Bhandari, A., and Bhandari, V. (2021). Diagnosis and management of bronchopulmonary dysplasia. BMJ 375, n1974. doi:10.1136/bmj.n1974
Gofrit, O. N., Benjamin, S., Halachmi, S., Leibovitch, I., Dotan, Z., Lamm, D. L., et al. (2014). DNA based therapy with diphtheria toxin-A BC-819: A phase 2b marker lesion trial in patients with intermediate risk nonmuscle invasive bladder cancer. J. Urol. 191 (6), 1697–1702. doi:10.1016/j.juro.2013.12.011
Gong, N., Teng, X., Li, J., and Liang, X. J. (2019). Antisense oligonucleotide-conjugated nanostructure-targeting lncRNA MALAT1 inhibits cancer metastasis. ACS Appl. Mat. Interfaces 11 (1), 37–42. doi:10.1021/acsami.8b18288
Guo, B. X., Bai, Y. C., Ma, Y. N., Liu, C., Wang, S., Zhao, R. Z., et al. (2019). Preclinical and clinical studies of smoke-inhalation-induced acute lung injury: Update on both pathogenesis and innovative therapy. Ther. Adv. Respir. Dis. 13, 1753466619847901. doi:10.1177/1753466619847901
Hangauer, M. J., Vaughn, I. W., and McManus, M. T. (2013). Pervasive transcription of the human genome produces thousands of previously unidentified long intergenic noncoding RNAs. PLoS Genet. 9 (6), e1003569. doi:10.1371/journal.pgen.1003569
Hanna, N., Ohana, P., Konikoff, F. M., Leichtmann, G., Hubert, A., Appelbaum, L., et al. (2012). Phase 1/2a, dose-escalation, safety, pharmacokinetic and preliminary efficacy study of intratumoral administration of BC-819 in patients with unresectable pancreatic cancer. Cancer Gene Ther. 19 (6), 374–381. doi:10.1038/cgt.2012.10
Hartmann, S., Ridley, A. J., and Lutz, S. (2015). The function of rho-associated kinases ROCK1 and ROCK2 in the pathogenesis of cardiovascular disease. Front. Pharmacol. 6, 276. doi:10.3389/fphar.2015.00276
He, D. M., Zeng, H., Chen, J. F., Xiao, L., Zhao, Y. H., and Liu, N. H. (2019). H19 regulates trophoblastic spheroid adhesion by competitively binding to let-7. Reproduction 157 (5), 423–430. doi:10.1530/REP-18-0339
Hoffmann, J., Wilhelm, J., Olschewski, A., and Kwapiszewska, G. (2016). Microarray analysis in pulmonary hypertension. Eur. Respir. J. 48 (1), 229–241. doi:10.1183/13993003.02030-2015
Hou, J. M., Krebs, M. G., Lancashire, L., Sloane, R., Backen, A., Swain, R. K., et al. (2012). Clinical significance and molecular characteristics of circulating tumor cells and circulating tumor microemboli in patients with small-cell lung cancer. J. Clin. Oncol. 30 (5), 525–532. doi:10.1200/JCO.2010.33.3716
Huang, P., Wang, L., Li, Q., Tian, X., Xu, J., Xu, J., et al. (2020). Atorvastatin enhances the therapeutic efficacy of mesenchymal stem cells-derived exosomes in acute myocardial infarction via up-regulating long non-coding RNA H19. Cardiovasc. Res. 116 (2), 353–367. doi:10.1093/cvr/cvz139
Huang, Y., Xu, W., and Zhou, R. (2021). NLRP3 inflammasome activation and cell death. Cell. Mol. Immunol. 18 (9), 2114–2127. doi:10.1038/s41423-021-00740-6
Huang, Z. K., Ma, Y. Y., Zhang, P. P., Si, J. H., Xiong, Y., and Yang, Y. (2020). Long non-coding RNA H19 confers resistance to gefitinib via miR-148b-3p/DDAH1 axis in lung adenocarcinoma. Anticancer. Drugs 31 (1), 44–54. doi:10.1097/CAD.0000000000000831
Hulin, J. A., Tommasi, S., Elliot, D., Hu, D. G., Lewis, B. C., and Mangoni, A. A. (2017). MiR-193b regulates breast cancer cell migration and vasculogenic mimicry by targeting dimethylarginine dimethylaminohydrolase 1. Sci. Rep. 7 (1), 13996. doi:10.1038/s41598-017-14454-1
Keniry, A., Oxley, D., Monnier, P., Kyba, M., Dandolo, L., Smits, G., et al. (2012). The H19 lincRNA is a developmental reservoir of miR-675 that suppresses growth and Igf1r. Nat. Cell Biol. 14 (7), 659–665. doi:10.1038/ncb2521
Khoshnevisan, K., Daneshpour, M., Barkhi, M., Gholami, M., Samadian, H., and Maleki, H. (2018). The promising potentials of capped gold nanoparticles for drug delivery systems. J. Drug Target. 26 (7), 525–532. doi:10.1080/1061186X.2017.1387790
Kim, J. (2014). Apelin-APJ signaling: A potential therapeutic target for pulmonary arterial hypertension. Mol. Cells 37 (3), 196–201. doi:10.14348/molcells.2014.2308
Lambrecht, B. N., and Hammad, H. (2012). The airway epithelium in asthma. Nat. Med. 18 (5), 684–692. doi:10.1038/nm.2737
Lavie, O., Edelman, D., Levy, T., Fishman, A., Hubert, A., Segev, Y., et al. (2017). A phase 1/2a, dose-escalation, safety, pharmacokinetic, and preliminary efficacy study of intraperitoneal administration of BC-819 (H19-DTA) in subjects with recurrent ovarian/peritoneal cancer. Arch. Gynecol. Obstet. 295 (3), 751–761. doi:10.1007/s00404-017-4293-0
Ley, B., Collard, H. R., and King, T. E. (2011). Clinical course and prediction of survival in idiopathic pulmonary fibrosis. Am. J. Respir. Crit. Care Med. 183 (4), 431–440. doi:10.1164/rccm.201006-0894CI
Li, B., Huang, N. N., Wei, S. N., Xv, J., Meng, Q. T., Aschner, M., et al. (2021). lncRNA TUG1 as a ceRNA promotes PM exposure-induced airway hyper-reactivity. J. Hazard. Mat. 416, 125878. doi:10.1016/j.jhazmat.2021.125878
Li, B., Luan, S., Chen, J., Zhou, Y., Wang, T. T., Li, Z. J., et al. (2020). The MSC-derived exosomal lncRNA H19 promotes wound healing in diabetic foot ulcers by upregulating PTEN via MicroRNA-152-3p. Mol. Ther. Nucleic Acids 19, 814–826. doi:10.1016/j.omtn.2019.11.034
Li, C., Wu, X., Zhang, W., Li, J., Liu, H. W., Hao, M., et al. (2015). AEG-1 promotes metastasis through downstream AKR1C2 and NF1 in liver cancer. Oncol. Res. 22 (4), 203–211. doi:10.3727/096504015X14386062091352
Li, N., Cui, J., Duan, X., Chen, H., and Fan, F. (2012). Suppression of type I collagen expression by miR-29b via PI3K, Akt, and Sp1 pathway in human Tenon's fibroblasts. Invest. Ophthalmol. Vis. Sci. 53 (3), 1670–1678. doi:10.1167/iovs.11-8670
Li, P., Tong, L., Song, Y., Sun, J., Shi, J., Wu, Z., et al. (2019). Long noncoding RNA H19 participates in metformin-mediated inhibition of gastric cancer cell invasion. J. Cell. Physiol. 234 (4), 4515–4527. doi:10.1002/jcp.27269
Li, X., Wang, H., Yao, B., Xu, W., Chen, J., and Zhou, X. (2016). lncRNA H19/miR-675 axis regulates cardiomyocyte apoptosis by targeting VDAC1 in diabetic cardiomyopathy. Sci. Rep. 6, 36340. doi:10.1038/srep36340
Li, X. K., Lv, F., Li, F., Du, M. J., Liang, Y. C., Ju, S. L., et al. (2020). Long Noncoding RNA H19 facilitates small cell lung cancer tumorigenesis through miR-140-5p/FGF9 Axis. Onco. Targets. Ther. 13, 3525–3534. doi:10.2147/OTT.S245710
Li, Y., Yin, Z. R., Fan, J. S., Zhang, S. Y., and Yang, W. B. (2019). The roles of exosomal miRNAs and lncRNAs in lung diseases. Signal Transduct. Target. Ther. 4, 47. doi:10.1038/s41392-019-0080-7
Liang, N., Zhang, W., Wang, H., Shi, W., Wang, L., and Ma, L. (2020). Levonorgestrel ameliorates adenomyosis via lncRNA H19/miR-17/TLR4 pathway. Drug Des. devel. Ther. 14, 3449–3460. doi:10.2147/DDDT.S248095
Liang, W. C., Fu, W. M., Wong, C. W., Wang, Y., Wang, W. M., Hu, G. X., et al. (2015). The lncRNA H19 promotes epithelial to mesenchymal transition by functioning as miRNA sponges in colorectal cancer. Oncotarget 6 (26), 22513–22525. doi:10.18632/oncotarget.4154
Liu, J., and Li, S. M. (2020). MiR-484 suppressed proliferation, migration, invasion and induced apoptosis of gastric cancer via targeting CCL-18. Int. J. Exp. Pathol. 101 (6), 203–214. doi:10.1111/iep.12366
Liu, L., Liu, L., and Lu, S. (2019). lncRNA H19 promotes viability and epithelial-mesenchymal transition of lung adenocarcinoma cells by targeting miR-29b-3p and modifying STAT3. Int. J. Oncol. 54 (3), 929–941. doi:10.3892/ijo.2019.4695
Liu, L. T., Song, Y., Li, L. L., Zuo, S., Liu, N., Yang, G., et al. (2018). GW29-e1174 the H19 long noncoding RNA is a novel negative regulator of cardiomyocyte hypertrophy. J. Am. Coll. Cardiol. 72 (16), C36–C. doi:10.1016/j.jacc.2018.08.134
Liu, Y. H., He, A. B., Liu, B. E., Huang, Z. X., and Mei, H. B. (2019). Potential role of lncRNA H19 as a cancer biomarker in human cancers detection and diagnosis: A pooled analysis based on 1585 subjects. Biomed. Res. Int. 2019, 9056458. doi:10.1155/2019/9056458
Lu, Q., Guo, Z., Xie, W., Jin, W., Zhu, D., Chen, S., et al. (2018). The lncRNA H19 mediates pulmonary fibrosis by regulating the miR-196a/col1a1 Axis. Inflammation 41 (3), 896–903. doi:10.1007/s10753-018-0744-4
Luo, H., Wang, J., Liu, D., Zang, S., Ma, N., Zhao, L., et al. (2019). The lncRNA H19/miR-675 axis regulates myocardial ischemic and reperfusion injury by targeting PPARα. Mol. Immunol. 105, 46–54. doi:10.1016/j.molimm.2018.11.011
Luo, M., Li, Z. W., Wang, W., Zeng, Y. G., Liu, Z. H., and Qiu, J. X. (2013). Long non-coding RNA H19 increases bladder cancer metastasis by associating with EZH2 and inhibiting E-cadherin expression. Cancer Lett. 333 (2), 213–221. doi:10.1016/j.canlet.2013.01.033
Lv, X. W., Wang, M. J., Qin, Q. Y., Lu, P., and Qin, G. W. (2021). 6-Gingerol relieves myocardial ischaemia/reperfusion injury by regulating lncRNA H19/miR-143/ATG7 signaling axis-mediated autophagy. Lab. Invest. 101 (7), 865–877. doi:10.1038/s41374-021-00575-9
Ma, L., Tian, X. D., Guo, H. H., Zhang, Z. K., Du, C., Wang, F., et al. (2018). Long noncoding RNA H19 derived miR-675 regulates cell proliferation by down-regulating E2F-1 in human pancreatic ductal adenocarcinoma. J. Cancer 9 (2), 389–399. doi:10.7150/jca.21347
Martinet, C., Monnier, P., Louault, Y., Benard, M., Gabory, A., and Dandolo, L. (2016). H19 controls reactivation of the imprinted gene network during muscle regeneration. Development 143 (6), 962–971. doi:10.1242/dev.131771
Meyer, M., Schillinger, W., Pieske, B., Holubarsch, C., Heilmann, C., Posival, H., et al. (1995). Alterations of sarcoplasmic reticulum proteins in failing human dilated cardiomyopathy. Circulation 92 (4), 778–784. doi:10.1161/01.cir.92.4.778
Muller, V., Oliveira-Ferrer, L., Steinbach, B., Pantel, K., and Schwarzenbach, H. (2019). Interplay of lncRNA H19/miR-675 and lncRNA NEAT1/miR-204 in breast cancer. Mol. Oncol. 13 (5), 1137–1149. doi:10.1002/1878-0261.12472
Nathan, S. D., and Meyer, K. C. (2014). IPF clinical trial design and endpoints. Curr. Opin. Pulm. Med. 20 (5), 463–471. doi:10.1097/MCP.0000000000000091
Nicolescu, C., Vaidya, A., Schilb, A., and Lu, Z. R. (2022). Regulating oncogenic LncRNA DANCR with targeted ECO/siRNA nanoparticles for non-small cell lung cancer therapy. ACS Omega 7 (26), 22743–22753. doi:10.1021/acsomega.2c02260
Nieto, M. A. (2017). Context-specific roles of EMT programmes in cancer cell dissemination. Nat. Cell Biol. 19 (5), 416–418. doi:10.1038/ncb3520
Nordin, M., Bergman, D., Halje, M., Engstrom, W., and Ward, A. (2014). Epigenetic regulation of the Igf2/H19 gene cluster. Cell Prolif. 47 (3), 189–199. doi:10.1111/cpr.12106
Omura, J., Habbout, K., Shimauchi, T., Wu, W.-H., Breuils-Bonnet, S., Tremblay, E., et al. (2020). Identification of long noncoding RNA H19 as a new biomarker and therapeutic target in right ventricular failure in pulmonary arterial hypertension. Circulation 142 (15), 1464–1484. doi:10.1161/CIRCULATIONAHA.120.047626
Pachnis, V., Brannan, C. I., and Tilghman, S. M. (1988). The structure and expression of a novel gene activated in early mouse embryogenesis. EMBO J. 7 (3), 673–681. doi:10.1002/j.1460-2075.1988.tb02862.x
Palm, F., Onozato, M. L., Luo, Z., and Wilcox, C. S. (2007). Dimethylarginine dimethylaminohydrolase (DDAH): Expression, regulation, and function in the cardiovascular and renal systems. Am. J. Physiol. Heart Circ. Physiol. 293 (6), H3227–H3245. doi:10.1152/ajpheart.00998.2007
Qian, B., Wang, D. M., Gu, X. S., Zhou, K., Wu, J., Zhang, C. Y., et al. (2018). LncRNA H19 serves as a ceRNA and participates in non-small cell lung cancer development by regulating microRNA-107. Eur. Rev. Med. Pharmacol. Sci. 22 (18), 5946–5953. doi:10.26355/eurrev_201809_15925
Qu, S. L., Chen, L., Wen, X. S., Zuo, J. P., Wang, X. Y., Lu, Z. J., et al. (2021). Suppression of Th17 cell differentiation via sphingosine-1-phosphate receptor 2 by cinnamaldehyde can ameliorate ulcerative colitis. Biomed. Pharmacother. 134, 111116. doi:10.1016/j.biopha.2020.111116
Rajeev, K. G., Nair, J. K., Jayaraman, M., Charisse, K., Taneja, N., O'Shea, J., et al. (2015). Hepatocyte-specific delivery of siRNAs conjugated to novel non-nucleosidic trivalent N-acetylgalactosamine elicits robust gene silencing in vivo. Chembiochem 16 (6), 903–908. doi:10.1002/cbic.201500023
Sajadpoor, Z., Amini-Farsani, Z., Teimori, H., Shamsara, M., Sangtarash, M. H., Ghasemi-Dehkordi, P., et al. (2018). Valproic acid promotes apoptosis and cisplatin sensitivity through downregulation of H19 noncoding RNA in ovarian A2780 cells. Appl. Biochem. Biotechnol. 185 (4), 1132–1144. doi:10.1007/s12010-017-2684-0
Schabath, M. B., and Cote, M. L. (2019). Cancer progress and priorities: Lung cancer. Cancer Epidemiol. Biomarkers Prev. 28 (10), 1563–1579. doi:10.1158/1055-9965.EPI-19-0221
Schoenfelder, S., Smits, G., Fraser, P., Reik, W., and Paro, R. (2007). Non-coding transcripts in the H19 imprinting control region mediate gene silencing in transgenic Drosophila. EMBO Rep. 8 (11), 1068–1073. doi:10.1038/sj.embor.7401094
Shu, C., Yan, D., Chen, C., Mo, Y., Wu, L., Gu, J., et al. (2019). Metformin exhibits its therapeutic effect in the treatment of pre-eclampsia via modulating the Met/H19/miR-148a-5p/P28 and Met/H19/miR-216-3p/EBI3 signaling pathways. Int. Immunopharmacol. 74, 105693. doi:10.1016/j.intimp.2019.105693
Sidi, A. A., Ohana, P., Benjamin, S., Shalev, M., Ransom, J. H., Lamm, D., et al. (2008). Phase I/II marker lesion study of intravesical BC-819 DNA plasmid in H19 over expressing superficial bladder cancer refractory to bacillus Calmette-Guerin. J. Urol. 180 (6), 2379–2383. doi:10.1016/j.juro.2008.08.006
Song, Z., Wei, D., Chen, Y., Chen, L., Bian, Y., Shen, Y., et al. (2019). Association of astragaloside IV-inhibited autophagy and mineralization in vascular smooth muscle cells with lncRNA H19 and DUSP5-mediated ERK signaling. Toxicol. Appl. Pharmacol. 364, 45–54. doi:10.1016/j.taap.2018.12.002
Su, H., Xu, X., Yan, C., Shi, Y., Hu, Y., Dong, L., et al. (2018). LncRNA H19 promotes the proliferation of pulmonary artery smooth muscle cells through AT1R via sponging let-7b in monocrotaline-induced pulmonary arterial hypertension. Respir. Res. 19 (1), 254. doi:10.1186/s12931-018-0956-z
Sun, J., Mao, S., and Ji, W. (2021). LncRNA H19 activates cell pyroptosis via the miR-22-3p/NLRP3 axis in pneumonia. Am. J. Transl. Res. 13 (10), 11384–11398.
Sun, W., Lv, J., Duan, L., Lin, R., Li, Y., Li, S., et al. (2019). Long noncoding RNA H19 promotes vascular remodeling by sponging let-7a to upregulate the expression of cyclin D1. Biochem. Biophys. Res. Commun. 508 (4), 1038–1042. doi:10.1016/j.bbrc.2018.11.185
Sung, H., Ferlay, J., Siegel, R. L., Laversanne, M., Soerjomataram, I., Jemal, A., et al. (2021). Global cancer statistics 2020: GLOBOCAN estimates of incidence and mortality worldwide for 36 cancers in 185 countries. Ca. Cancer J. Clin. 71 (3), 209–249. doi:10.3322/caac.21660
Tan, Y., Sun, D., Chen, J., Li, R., and Wang, S. (2021). Ginsenoside Rb3 alleviates smoke-induced lung injury via the H19/miR-29b-3p/HGMB1/TLR4 signalling pathway. J. Cell. Mol. Med. 25 (5), 2725–2729. doi:10.1111/jcmm.15844
Tang, Y., He, R., An, J., Deng, P., Huang, L., and Yang, W. (2016). The effect of H19-miR-29b interaction on bleomycin-induced mouse model of idiopathic pulmonary fibrosis. Biochem. Biophys. Res. Commun. 479 (3), 417–423. doi:10.1016/j.bbrc.2016.09.028
Tarnowski, M., Tkacz, M., Czerewaty, M., Poniewierska-Baran, A., Grymula, K., and Ratajczak, M. Z. (2015). 5Azacytidine inhibits human rhabdomyosarcoma cell growth by downregulating insulinlike growth factor 2 expression and reactivating the H19 gene product miR675, which negatively affects insulinlike growth factors and insulin signaling. Int. J. Oncol. 46 (5), 2241–2250. doi:10.3892/ijo.2015.2906
Tian, Y., Lu, J., Hao, X. Q., Li, H., Zhang, G. Y., Liu, X. L., et al. (2020). FTH1 inhibits ferroptosis through ferritinophagy in the 6-OHDA model of Parkinson's disease. Neurotherapeutics 17 (4), 1796–1812. doi:10.1007/s13311-020-00929-z
Torres, A., Cilloniz, C., Niederman, M. S., Menendez, R., Chalmers, J. D., Wunderink, R. G., et al. (2021). Pneumonia. Nat. Rev. Dis. Prim. 7 (1), 25. doi:10.1038/s41572-021-00259-0
Tsang, W. P., and Kwok, T. T. (2007). Riboregulator H19 induction of MDR1-associated drug resistance in human hepatocellular carcinoma cells. Oncogene 26 (33), 4877–4881. doi:10.1038/sj.onc.1210266
Vishnupriya, S., Priya Dharshini, L. C., Sakthivel, K. M., and Rasmi, R. R. (2020). Autophagy markers as mediators of lung injury-implication for therapeutic intervention. Life Sci. 260, 118308. doi:10.1016/j.lfs.2020.118308
Wang, B., Suen, C. W., Ma, H., Wang, Y., Kong, L., Qin, D., et al. (2020). The roles of H19 in regulating inflammation and aging. Front. Immunol. 11, 579687. doi:10.3389/fimmu.2020.579687
Wang, J., Wang, X., Chen, T., Jiang, L., and Yang, Q. (2017). Huaier extract inhibits breast cancer progression through a LncRNA-H19/MiR-675-5p pathway. Cell. Physiol. biochem. 44 (2), 581–593. doi:10.1159/000485093
Wang, J., Yang, K., Yuan, W., and Gao, Z. (2018). Determination of serum exosomal H19 as a noninvasive biomarker for bladder cancer diagnosis and prognosis. Med. Sci. Monit. 24, 9307–9316. doi:10.12659/MSM.912018
Wang, R., Zhou, S., Wu, P., Li, M., Ding, X., Sun, L., et al. (2018). Identifying involvement of H19-miR-675-3p-IGF1R and H19-miR-200a-PDCD4 in treating pulmonary hypertension with melatonin. Mol. Ther. Nucleic Acids 13, 44–54. doi:10.1016/j.omtn.2018.08.015
Wang, W., Min, L., Qiu, X., Wu, X., Liu, C., Ma, J., et al. (2021). Biological function of long non-coding RNA (LncRNA) xist. Front. Cell Dev. Biol. 9, 645647. doi:10.3389/fcell.2021.645647
Wang, X., Cheng, Z., Dai, L., Jiang, T., Jia, L., Jing, X., et al. (2019). Knockdown of long noncoding RNA H19 represses the progress of pulmonary fibrosis through the transforming growth factor β/smad3 pathway by regulating MicroRNA 140. Mol. Cell. Biol. 39 (12), e00143-19. doi:10.1128/MCB.00143-19
Wang, Y., Huang, Q., and Li, F. (2020). miR-140-5p targeted FGF9 and inhibited the cell growth of laryngeal squamous cell carcinoma. Biochem. Cell Biol. 98 (2), 83–89. doi:10.1139/bcb-2018-0351
Wang, Y., Tai, Y. L., Zhao, D., Zhang, Y., Yan, J., Kakiyama, G., et al. (2021). Berberine prevents disease progression of nonalcoholic steatohepatitis through modulating multiple pathways. Cells 10 (2), 210. doi:10.3390/cells10020210
Warburton, D., Shi, W., and Xu, B. (2013). TGF-beta-Smad3 signaling in emphysema and pulmonary fibrosis: An epigenetic aberration of normal development? Am. J. Physiol. Lung Cell. Mol. Physiol. 304 (2), L83–L85. doi:10.1152/ajplung.00258.2012
Winkle, M., El-Daly, S. M., Fabbri, M., and Calin, G. A. (2021). Noncoding RNA therapeutics - challenges and potential solutions. Nat. Rev. Drug Discov. 20 (8), 629–651. doi:10.1038/s41573-021-00219-z
Wu, L., Sun, L., Hua, Y., Yang, C., and Teng, Y. (2018). Overexpression of long non-coding RNA H19 protects lung fibroblasts from LPS-induced injury by targeting miR-181a and Runx2 via activation of Notch and JNK pathways. J. Cell. Biochem. doi:10.1002/jcb.26660
Xia, S., Wang, Z., Chen, L., Zhou, Y., Li, Y., Wang, S., et al. (2021). Dihydroartemisinin regulates lipid droplet metabolism in hepatic stellate cells by inhibiting lncRNA-H19-induced AMPK signal. Biochem. Pharmacol. 192, 114730. doi:10.1016/j.bcp.2021.114730
Xu, J., Xiang, P., Liu, L., Sun, J., and Ye, S. (2020). Metformin inhibits extracellular matrix accumulation, inflammation and proliferation of mesangial cells in diabetic nephropathy by regulating H19/miR-143-3p/TGF-β1 axis. J. Pharm. Pharmacol. 72 (8), 1101–1109. doi:10.1111/jphp.13280
Xu, J. L., Hua, T., Ding, J., Fan, Y., Liu, Z. J., and Lian, J. W. (2019). FOXF2 aggravates the progression of non-small cell lung cancer through targeting lncRNA H19 to downregulate PTEN. Eur. Rev. Med. Pharmacol. Sci. 23 (24), 10796–10802. doi:10.26355/eurrev_201912_19782
Yang, H. (2022). Silencing of long non-coding RNA H19 alleviates lipopolysaccharide (LPS)-induced apoptosis and inflammation injury by regulating miR-140-5p/TLR4 axis in cell models of pneumonia. Curr. Mol. Med. 22. doi:10.2174/1566524022666220407100949
Yang, H., Wang, H., and Andersson, U. (2020). Targeting inflammation driven by HMGB1. Front. Immunol. 11, 484. doi:10.3389/fimmu.2020.00484
Yang, S., Tang, W., He, Y., Wen, L., Sun, B., and Li, S. (2018). Long non-coding RNA and microRNA-675/let-7a mediates the protective effect of melatonin against early brain injury after subarachnoid hemorrhage via targeting TP53 and neural growth factor. Cell Death Dis. 9 (2), 99. doi:10.1038/s41419-017-0155-8
Yu, H., Pardoll, D., and Jove, R. (2009). STATs in cancer inflammation and immunity: A leading role for STAT3. Nat. Rev. Cancer 9 (11), 798–809. doi:10.1038/nrc2734
Yuan, Y., and Zheng, Z. (2019). Geniposide protects PC-12 cells against oxygen and glucose deprivation-induced injury by up-regulation of long-noncoding RNA H19. Life Sci. 216, 176–182. doi:10.1016/j.lfs.2018.11.047
Zamyatina, A., and Heine, H. (2020). Lipopolysaccharide recognition in the crossroads of TLR4 and caspase-4/11 mediated inflammatory pathways. Front. Immunol. 11, 585146. doi:10.3389/fimmu.2020.585146
Zeng, J., Zhu, L., Liu, J., Zhu, T., Xie, Z., Sun, X., et al. (2019). Metformin protects against oxidative stress injury induced by ischemia/reperfusion via regulation of the lncRNA-H19/miR-148a-3p/Rock2 Axis. Oxid. Med. Cell. Longev. 2019, 8768327. doi:10.1155/2019/8768327
Zhang, L., Cheng, H., Yue, Y., Li, S., Zhang, D., and He, R. (2018). H19 knockdown suppresses proliferation and induces apoptosis by regulating miR-148b/WNT/β-catenin in ox-LDL -stimulated vascular smooth muscle cells. J. Biomed. Sci. 25 (1), 11. doi:10.1186/s12929-018-0418-4
Zhang, L., Wang, P., Shen, Y., Huang, T., Hu, X., and Yu, W. (2020). Mechanism of lncRNA H19 in regulating pulmonary injury in hyperoxia-induced bronchopulmonary dysplasia newborn mice. Am. J. Perinatol. 39, 1089–1096. doi:10.1055/s-0040-1721498
Zhang, Q. Q., Li, X. L., Li, X., Li, X. S., and Chen, Z. C. (2021). LncRNA H19 promotes epithelial-mesenchymal transition (EMT) by targeting miR-484 in human lung cancer cells (Retraction of Vol 119, Pg 4447, 2018). J. Cell Biochem. 122 (5), 600.
Zhang, R., Pan, T., Xiang, Y., Zhang, M., Xie, H., Liang, Z., et al. (2022). Curcumenol triggered ferroptosis in lung cancer cells via lncRNA H19/miR-19b-3p/FTH1 axis. Bioact. Mat. 13, 23–36. doi:10.1016/j.bioactmat.2021.11.013
Zhang, W., Zhou, K., Zhang, X., Wu, C., Deng, D., and Yao, Z. (2021). Roles of the H19/microRNA675 axis in the proliferation and epithelialmesenchymal transition of human cutaneous squamous cell carcinoma cells. Oncol. Rep. 45 (4), 39. doi:10.3892/or.2021.7990
Zhang, Y., Li, M., and Han, X. (2020). Icariin affects cell cycle progression and proliferation of human retinal pigment epithelial cells via enhancing expression of H19. PeerJ 8, e8830. doi:10.7717/peerj.8830
Zhang, Y. H., Huang, W. B., Yuan, Y. J., Li, J., Wu, J., Yu, J., et al. (2020). Long non-coding RNA H19 promotes colorectal cancer metastasis via binding to hnRNPA2B1. J. Exp. Clin. Canc Res. 39 (1), 141. doi:10.1186/s13046-020-01619-6
Zhao, J., Bai, X., Feng, C., Shang, X., and Xi, Y. (2019). Long non-coding RNA HCP5 facilitates cell invasion and epithelial-mesenchymal transition in oral squamous cell carcinoma by miR-140-5p/SOX4 Axis. Cancer Manag. Res. 11, 10455–10462. doi:10.2147/CMAR.S230324
Zhao, X. M., Ren, J. J., Du, W. H., Hao, H. S., Wang, D., Liu, Y., et al. (2012). Effect of 5-aza-2'-deoxycytidine on methylation of the putative imprinted control region of H19 during the in vitro development of vitrified bovine two-cell embryos. Fertil. Steril. 98 (1), 222–227. doi:10.1016/j.fertnstert.2012.04.014
Zhao, Y., Feng, C. J., Li, Y. J., Ma, Y. F., and Cai, R. J. (2019). LncRNA H19 promotes lung cancer proliferation and metastasis by inhibiting miR-200a function. Mol. Cell. Biochem. 460 (1-2), 1–8. doi:10.1007/s11010-019-03564-1
Keywords: drugs, lung diseases, mechanisms, competition, long non-coding RNA H19
Citation: Xie J, Hu Y, Sun D, Liu C, Li Z and Zhu J (2022) Targeting non-coding RNA H19: A potential therapeutic approach in pulmonary diseases. Front. Pharmacol. 13:978151. doi: 10.3389/fphar.2022.978151
Received: 25 June 2022; Accepted: 30 August 2022;
Published: 16 September 2022.
Edited by:
Xueping Lei, Guangzhou Medical University, ChinaReviewed by:
Zhijun Jie, Fudan University, ChinaCopyright © 2022 Xie, Hu, Sun, Liu, Li and Zhu. This is an open-access article distributed under the terms of the Creative Commons Attribution License (CC BY). The use, distribution or reproduction in other forums is permitted, provided the original author(s) and the copyright owner(s) are credited and that the original publication in this journal is cited, in accordance with accepted academic practice. No use, distribution or reproduction is permitted which does not comply with these terms.
*Correspondence: Jie Zhu, amFuZXpodXRjbUBmb3htYWlsLmNvbQ==
Disclaimer: All claims expressed in this article are solely those of the authors and do not necessarily represent those of their affiliated organizations, or those of the publisher, the editors and the reviewers. Any product that may be evaluated in this article or claim that may be made by its manufacturer is not guaranteed or endorsed by the publisher.
Research integrity at Frontiers
Learn more about the work of our research integrity team to safeguard the quality of each article we publish.