- 1Department of Pharmacy, Faculty of Allied Health Sciences, Daffodil International University, Dhaka, Bangladesh
- 2Department of Pathology, Clinical Medical College and The First Affiliated Hospital of Chengdu Medical College, Chengdu, Sichuan, China
- 3Institutes for Systems Genetics, Frontiers Science Center for Disease-Related Molecular Network, West China Hospital, Sichuan University, Chengdu, Sichuan, China
- 4School of Pharmaceutical Sciences, Lovely Professional University, Phagwara, Punjab, India
- 5Department of Chemistry, University of Swabi, Swabi, Pakistan
- 6Department of Rasa Shastra and Bhaishajya Kalpana, Faculty of Ayurveda, Institute of Medical Sciences, Banaras Hindu University, Varanasi, Uttar Pradesh, India
Natural substances originating from plants have long been used to treat neurodegenerative disorders (NDs). Parkinson’s disease (PD) is a ND. The deterioration and subsequent cognitive impairments of the midbrain nigral dopaminergic neurons distinguish by this characteristic. Various pathogenic mechanisms and critical components have been reported, despite the fact that the origin is unknown, such as protein aggregation, iron buildup, mitochondrial dysfunction, neuroinflammation and oxidative stress. Anti-Parkinson drugs like dopamine (DA) agonists, levodopa, carbidopa, monoamine oxidase type B inhibitors and anticholinergics are used to replace DA in the current treatment model. Surgery is advised in cases where drug therapy is ineffective. Unfortunately, the current conventional treatments for PD have a number of harmful side effects and are expensive. As a result, new therapeutic strategies that control the mechanisms that contribute to neuronal death and dysfunction must be addressed. Natural resources have long been a useful source of possible treatments. PD can be treated with a variety of natural therapies made from medicinal herbs, fruits, and vegetables. In addition to their well-known anti-oxidative and anti-inflammatory capabilities, these natural products also play inhibitory roles in iron buildup, protein misfolding, the maintenance of proteasomal breakdown, mitochondrial homeostasis, and other neuroprotective processes. The goal of this research is to systematically characterize the currently available medications for Parkinson’s and their therapeutic effects, which target diverse pathways. Overall, this analysis looks at the kinds of natural things that could be used in the future to treat PD in new ways or as supplements to existing treatments. We looked at the medicinal plants that can be used to treat PD. The use of natural remedies, especially those derived from plants, to treat PD has been on the rise. This article examines the fundamental characteristics of medicinal plants and the bioactive substances found in them that may be utilized to treat PD.
Highlights
1. Parkinson’s disease (PD) is a chronic progressive neurodegenerative disorder.
2. It can be treated with a variety of natural products and bioactive compounds.
3. Extra care should be provided to patients with PD to minimize the risk of infection.
4. Future perspectives like PD vaccine, cell transplantation, gene therapy, and surgical methods are highlighted.
1 Introduction
James Parkinson wrote the first description of Parkinson’s disease (PD) and published it in 1817. PD, also known as idiopathic paralysis agitans, is a frequent ND of the central nervous system (CNS). PD affects about 1% of those over the age of 65. It seldom occurs before the age of 20 and it generally begins between the ages of 40 and 70. Young-onset Parkinson’s disease is a kind of Parkinson’s disease that appears before the age of 20. Parkinsonian symptoms can be observed in other pathologies such as Wilson’s Disease and Huntington’s Disease (Snyder and Wolozin, 2004; Klein and Westenberger, 2012; Postuma et al., 2015). According to the Global Burden of Disease (GBD) survey, there were 1.02 million new cases of Parkinson’s disease in 2017 (Sengupta et al., 2016). Globally, 6.1 million PD patients were recorded in 2016, and the age-standardized prevalence rate (ASR) rose by 21.7% between 1990 and 2016 (Feigin et al., 2016). Years lived with disability (YLDs) is a measure of both the handicap brought on by that status and the average time it takes for incident instances to recover or pass away. YLDs is a popular metric for measuring the health damage brought on by PD. Age-standardized rates of PD-related young life deaths rose sharply from 1990 to 2007, rising 8.9%, and then increased 1.0% from 2007 to 2017 (Global, 2018). According to studies, the burden of PD would significantly increase in the coming decades (Kowal et al., 2013; Wanneveich et al., 2018). For instance, it was predicted that 4.94 million PD patients, or 50% of all PD patients worldwide, will reside in China by 2030 (Dorsey et al., 2007). However, this has not yet been conclusively demonstrated. Variables including overwork, trauma, exposure to cold, rigid personalities and stress are thought to be the predisposing factors (Rahman et al., 2020). Parkinson’s disease is the world’s second most common neurological condition. The etiology of this disease is unknown, but mutations in some genes described in familial PD could help to better understand the role of some proteins and the mechanisms involved in the development of this disorder. PD is triggered by a complex interplay of genetic, environmental, and epigenetic influences. The corresponding risk of developing PD was 2.0% for men and 1.3% for women (ratio = 1.5) (Elbaz et al., 2002; Schneider et al., 2017; Sharma et al., 2018).
Changes in a significant number of other genes were thought to be PD-causative and were discovered by linkage analysis or a candidate gene approach, in addition to the genes responsible for the six monogenic types of PD. Some of these genes—UCHL1 [PARK5], GYGYF2 [PARK11], OMI/HTRA2 [PARK13], PLA2G6 [PARK14], and FBXO7 [PARK15]—even received the “PARKI” designation. A higher risk of developing PD has been linked to variants in a number of PARK-designated (SNCA, UCHL1, LRRK2, PARK 16, GAK) and a few additional genes (MAPT, GBA, NAT2, INOS2A, GAK, HLA-DRA, and APOE) (Postuma et al., 2015). Parkin, DJ-1, ubiquitin C-terminal hydrolase isozyme L1 (UCH-L1), nuclear receptor-related factor 1, and α-synuclein are genes linked to either Parkinson- or Parkinson-related diseases. Because it rapidly aggregates and forms the majority of Lewy bodies (LBs), α-synuclein is particularly noteworthy. The α-synuclein that has accumulated interacts to the proteasome and effectively suppresses proteasomal action. Proteasomal dysfunction is believed to play a role in the pathogenesis of PD because ubiquitin builds up in LBs and interacts with the proteasomal system through interactions with parkin and UCH-L1. Numerous studies suggest that neurotoxins may interact with α-synuclein or other proteins connected to Parkinson’s disease to influence the etiology of the disease (Klein and Westenberger, 2012).
PD is a complex neurodegenerative disorder (ND). LBs and the loss of dopaminergic neurons in the substantia nigra have long been connected to the motor symptoms of Parkinson’s disease (Rahman et al., 2020; Cassotta et al., 2022; Lv et al., 2022). Although oxidative stress, mitochondrial dysfunction, and aberrant protein aggregation have been associated with Parkinson’s disease, the exact origins of the disease remain unclear. Until recently, dopamine replacement therapy was the primary treatment for Parkinson’s disease. Even though many researchers have tried to find a way to stop the neurodegenerative process of Parkinson’s disease, no medicine has been found to protect neurons or change the course of the disease in clinical PD patients. Surprisingly, a wide range of natural chemicals are being used as alternative medicines to treat Parkinson’s disease (Sharma et al., 2018; Li et al., 2019). The most prevalent symptoms of Parkinson’s disease include dyskinesias, muscle tremors, rigidity and anomalies in body posture and movement. Parkinson’s disease causes dopaminergic neurons in the substantia nigra pars compacta to get worse, and the number of transmitters in dopaminergic neurons in the striatum drops by a lot. As a result, nigrostriatal dopaminergic neuron function declines and cholinergic neuron the function rises, resulting in movement problems (Phani et al., 2012).
However, the pathogenic factors discovered in the majority of Parkinson’s disease patients have yet to be verified. The majority of contemporary opinions believe that fibrillation and aberrant α-synuclein aggregation are the primary components in the PD clinical occurrences. Many factors, including oxidative stress and intermediate oligomer conformation, play important roles in the pathogenesis of Parkinson’s disease in different metabolic pathways. Furthermore, the existence of Lewy bodies (LBs) made up of α-synuclein is a significant pathological marker of PD. As a result, α-synuclein may be linked to PD (Stefanis, 2016; Zhang et al., 2017a; Zhang et al., 2017b; Sharma et al., 2019a; Rahman et al., 2022a). Furthermore, a growing body of evidence suggests that many common molecular signaling pathways are linked to the development and progression of Parkinson’s disease, thanks to recent and updated developments in life sciences technologies, as well as ongoing in-depth research on proteomics and molecular biology. The phosphoinositide 3-kinase/protein kinase B pathway is the most important of them (Nakaso et al., 2008; Quesada et al., 2008), the nuclear factor erythriod2-related factor2 (Williamson, 2012; Wang et al., 2014), the P38 mitogen activated protein kinase (Wilms et al., 2003; Corrêa and Eales, 2012), the glycogen synthase kinase-3b (Wu et al., 2007; Duka et al., 2009; Golpich et al., 2015), the c-jun-N-terminal kinase (Hunot et al., 2004; Kuan et al., 2005), the nuclear transcription factor-κB (NF-κB) (Uberti et al., 2004; Noda et al., 2005), the Want signaling pathway (Berwick and Harvey, 2012; Arenas, 2014), and the autophagy lysosome pathway (Xilouri et al., 2008; Xilouri and Stefanis, 2011). This article discusses newly identified natural compounds having substantial anti-PD capabilities, as well as the current state of research into their medicinal chemistry.
2 Etiology of PD
In the past, the cause was completely random, but now both environmental and genetic factors are thought to play a role (Schapira, 2006; Schapira and Tolosa, 2010; Schapira, 2011), and understanding of etiological elements in Parkinson’s disease has altered substantially. However, age or the aging process is the single most important predictor of the beginning of PD. Despite the certainty of age’s significance, little effort appears to have been made to investigate how age and the aging process interact (Obeso et al., 2010; Sharma and Martins, 2020; Sharma and Prajapati, 2020). The most widespread explanation is that as normal cellular physiological and biochemical processes fail more frequently, dopaminergic neurons become more sensitive to toxic assault. Dopaminergic cells aging linked with L-type calcium channels (Surmeier et al., 2010) is a recent example. In reality, most models of cell death in PD disregard the role of age or aging, and young animals are used to represent the disease process in the great majority of experimental research (Hindle and Ageing, 2010). When looking at the prevalence of PD, the same might be said about men outnumbering women (Shulman, 2007). This must also provide insight into the etiology of the illness, especially given men’s shorter life expectancy. Despite evidence that estrogen plays a role in determining the age at which women develop PD and its effect on dopaminergic neuron function, no explanation for the disparity has emerged. Industrialization, rural areas, well water, plant-derived toxins, bacterial and viral infection, and exposure to organic solvents, carbon monoxide and carbon disulfide are some of the broad environmental factors on the prevalence of PD (Tellier et al., 2022). Despite inconsistent results from various research and the difficulty of identifying specific pesticide compounds that may be connected to an increased risk of PD, pesticide exposure has recently attracted interest (Richardson et al., 2009). Paraquat and rotenone are two specific agrochemicals that have been shown to cause dopaminergic cell death in the nigral area of mice (Berry et al., 2010; Chia et al., 2020). Some of these will be discussed later while discussing pathogenic mechanisms. Factors that reduce the risk of developing Parkinson’s disease can also provide important information about the disease’s progression. Exercise, anti-inflammatories, antihypertensives (mostly calcium antagonists) and antilipidemic all seem to lower the risk, but the role of some, like anti-inflammatories, exercise, antihypertensives (mostly calcium antagonists) and antilipidemic, is still unknown (Ascherio et al., 2001; Warner and Schapira, 2003). Even though there are many different factors that have been linked to the pathogenesis of Parkinson’s disease, it was the discovery of the neurotoxic effects of MPTP that got the most attention and started a new era of research (Burns et al., 1983; Langston et al., 1983; Jenner et al., 1984). When MPTP was found, it led to research into how it works and how it might be related to the cause of Parkinson’s disease. But perhaps more importantly, it gave us the first model of Parkinson’s disease motor deficits in primates and a predicted test bed for therapeutic action in people (Jenner, 2003). However, MPTP-induced parkinsonism differs from typical PD in that it does not develop in humans, there is no LB development and there is no pathology in other parts of the brain that are damaged in human illness. As a result, despite its significance, MPTP may fail to send the critical information required to answer the genesis of the Parkinson’s disease enigma (Noyce et al., 2016).
3 Pathogenesis of PD
PD is a neurological, progressive condition that causes a number of motor and crippling abnormalities, including as bradykinesia, resting tremor, muscle stiffness and imbalance. Dopamine levels in the striatum, tailed nuclei and putamen are reduced as a result of the slow, progressive degeneration of dopaminergic neurons in the SN compacta, which is a pathological hallmark of PD. The most significant pathogenic finding in the brains of PD patients is the progressive loss of dopaminergic neurons in the basal complexes. Dopamine neurotransmitter levels in this region are decreased as a result of the destruction of these neurons. The disease’s symptoms start to show up when 50%–60% of dopaminergic neurons have been destroyed and dopamine levels in the striatum have dropped by about 80%–85%. However, studies have shown that oxidative stress and mitochondrial dysfunction probably play a key role in the pathogenesis of PD; the loss of nigrostriatal dopaminergic neurons and the presence of intracellular cytoplasmic proteins, i.e., Lewy bodies, are also involved. The precise molecular mechanism of the degradation of dopaminergic neurons and the incidence of PD is unknown. The cells are transmitted to the putamen from the nigrostriatal neurons in the SN pars compacta (SNpc). Depigmentation of SNpc results from the absence of these neurons, which normally contain modest levels of melanin (Lees, 2012). PD, the most prevalent form of Multiple Sclerosis (MS), results from the progressive degeneration of dopaminergic neurons along the nigrostriatal pathway (Hacker et al., 2012; Luo et al., 2014; Prodoehl et al., 2014). Magnetic resonance diffusion tensor imaging revealed signs of neurodegeneration in the nigrostriatal circuits (Wang et al., 2015). Early-onset Parkinson’s disease was shown to have frontal and parietal lobe microstructural damage, which was connected to postural and walking issues (Gattellaro et al., 2009; Gu et al., 2014; Auning et al., 2015). Furthermore, radial alterations in nigrostriatal fibers have been associated with the degree of movement impairments in Parkinson’s disease patients (Zhang et al., 2015). PD is caused by a variety of causes that cause neurodegeneration.
3.1 Mitochondrial dysfunction in PD
Recently some researcher found missing of the substantial nigra of the PD patients, investigators also revealed abnormalities of the mitochondria in skeletal muscle, platelets and lymphoblast that basically indicate direct link between mitochondrial dysfunction and PD (Schapira et al., 1989; Schapira et al., 1990; Schapira, 1994). PD patients with mitochondrial dysfunction confirmed by pathologically especially in sporadic PD, against a backdrop of increased oxidative stress and elevated brain iron levels, this helps us to understand how mitochondrial abnormalities interconnected in PD pathogenesis (Dexter et al., 1992; Mann et al., 1994; Olanow et al., 1995; Schapira, 1995; Schapira, 2011). The discovery of specific mutations in genes that cause dopaminergic cell death and familial PD have confirmed the role of mitochondria in the development and pathophysiology of PD. Many instances of PD with PINK1 or LRRK2 mutations, on the other hand, are clinically indistinguishable from spontaneous PD. Several knockouts or expression models of these mutations have been discovered to exhibit mitochondrial function abnormalities (Moon, 2007). Parkin is a ubiquitously transcribed protein that has been linked to the endoplasmic reticulum, synaptic vesicles, Golgi apparatus, and mitochondria in intracellular investigations (Shimura et al., 1999; Kubo et al., 2001; Mouatt-Prigent et al., 2004).
3.2 LBs and microgliosis
The alternative method to investigating PD etiology has been to look at the pathological components of the disease process. The formation of LBs and the existence of a reactive microgliosis, which may contribute to disease progression, are two characteristics of PD. The LB has long been regarded as the distinguishing hallmark of PD, although its significance in the disease process has been debated. It has been alternately described as a pathogenesis marker and a graveyard for dead and dying neurons. In PD α-synuclein mutations is common and in sporadic PD LBs strongly immunoreactive for wild-type α-synuclein with many other protein in their normal or damaged condition (Spillantini et al., 1998). The discovery of parkin and UCH-L1 mutations, as well as their involvement in the ubiquitin-proteasomal system, ushered in a new age of research into changes in protein processing in dopaminergic cells via both proteasomes and lysosomes (Eldeeb et al., 2022; Kumar et al., 2022). The argument has been fanned by postmortem tests and toxicology research. In the substantia nigra, there is a decrease in proteasomal enzyme activity that is unique to PD and does not occur in other parts of the brain (McNaught et al., 2003; Tofaris et al., 2003).
3.3 Neuroinflammation in PD
McGeer et al. discovered that post-mortem brains of people with PD exhibit elevated human leucocyte antigen, which was the first indication of neuroinflammation’s role in PD pathogenesis in 1988. Microglia with DR positivity (McGeer et al., 1988). Furthermore, elevated pro-inflammatory mediators such as TNF, ILβ, IL6, iNOS, and COX two have been found in the striatum and substantia nigra, according to this study (Tansey et al., 2007). Microglia are one of the most important kinds of cells in the central nervous system’s inflammatory response (Saijo et al., 2009). As previously mentioned, PD is linked to aberrant α-synuclein aggregation, which activates microglia even more (Su et al., 2008). Neuroinflammation is characterized by the activation of microglia and reactive astrocytes in the brain, as well as the production of inflammatory mediators such as cytokines (TNF-α, IL-1, and IL-6), chemokines, complement cascade proteins, reactive oxygen species, and reactive nitrogen species (RNS). Several factors have been shown to reduce the permeability of the blood-brain barrier (BBB) (da Fonseca et al., 2014) (Figure 1). The degeneration of the nigral dopaminergic neurons in PD is caused mainly by inflammasome-induced neuroinflammation. Baicalein, a flavonoid derived from the Scutellaria baicalensis Georgi plant used in traditional Chinese medicine, has been shown to have anti-inflammatory and neuroprotective effects in animal models of NDs, including PD. In a PD mice model induced by MPTP, baicalein inhibits the NLRP3/Caspase-1/GSDMD Pathway to decrease neuroinflammation (Rui et al., 2020).
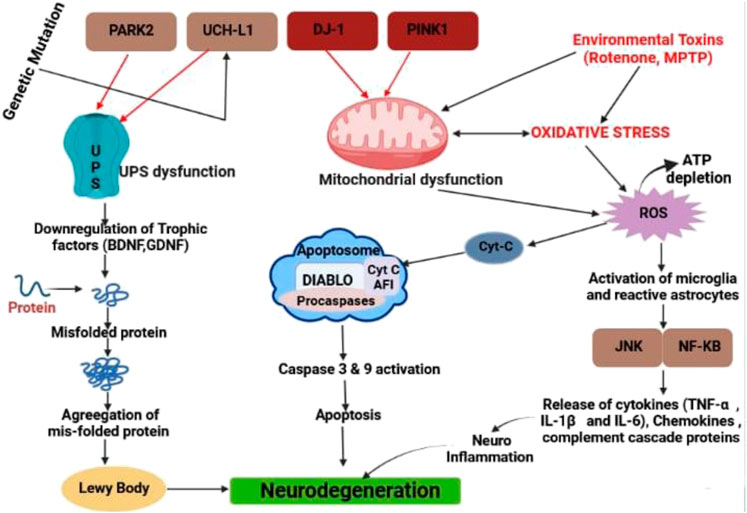
FIGURE 1. Different types of pathway for neurodegeneration in PD by LB, caspase activation and releasing different types of inflammatory cytokine.
4 Role of natural products
4.1 Herbal products
4.1.1 Baicalein
Baicalein is a chemical compound derived from the dried root of the Scutellaria baicalensis plant (Labiatae) (Amro et al., 2018). Baicalein prevented the buildup of ROS, apoptosis, ATP depletion and mitochondrial membrane rupture in PC12 cells when tested for rotenone-induced neurotoxicity (Li et al., 2012). Baicalein treatment prevents Dopamine levels in the basal ganglia from dropping and boosts Dopamine and 5-hydroxytryptamine levels (Cheng et al., 2008; Mu et al., 2011). In Hela and SH-SY5Y cells, Baicalein inhibited the aggregation of α-synuclein and the production of α-synuclein oligomers (Lu et al., 2011).
4.1.2 Erythrina velutina
The ethanol extract of this plant (Fabaceae) has a neuroprotective effect. It has been shown to reduce the neurotoxicity caused by 6-OHDA in SH-SY5Y cells and to get rid of free radicals, which suggests it could be used to treat Parkinson’s disease (Silva et al., 2016).
4.1.3 Resveratrol
Resveratrol is a polyphenolic compound present in a variety of plants, including grapes and berries (Frémont, 2000; Rahman et al., 2021a). Resveratrol has been found to help with motor deficits, oxidative stress, and the loss of TH neurons in animal models of Parkinson Disease (Lu et al., 2008). Resveratrol inhibits mitochondrial enlargement and chromatin condensation while also lowering COX-2 and TNF-α gene expression (Jin et al., 2008).
4.1.4 Peganum harmala
Peganum harmala (Nitrariaceae) made muscles less stiff, stopped oxidation of fats and proteins in the brain and stopped dopaminergic neurons from dying off (Rezaei et al., 2016). It is thought that this herb’s neuroprotective properties come from its ability to reduce the activity of angiotensin II. This reduces oxidative stress and protects dopaminergic neurons (LopezReal et al., 2005).
4.1.5 Curcuma longa
Curcuma longa (Zingiberaceae) has been shown to have anti-inflammatory, chemotherapeutic, anti-oxidant, wound-healing, anti-proliferative and antiparasitic properties. The active component polyphenolic fraction, curcumin, is probably to blame (Gupta et al., 2012). Curcumin protects MPTP-induced loss of TH-positive neurons and DA depletion in the striatum of MPTP-induced mouse models, as well as a reduction in cytokines, total nitrite, and inflammatory markers such inducible nitric oxide synthase (Ojha et al., 2012).
4.1.6 Carthamus tinctorius L. (Safflower)
Safflower (Asteraceae) has been discovered to contain flavonoids and is widely used as a conventional treatment for cerebrovascular disorders in China (Amro et al., 2018). It increased DA transporter and DJ-1 protein expression as well as DA levels (Ren et al., 2016). Overexpression or aggregation of α-synuclein, as well as reactive astrogliosis, may be inhibited by safflower (Ren et al., 2016).
4.1.7 Pueraria lobata
Puerarin (Fabaceae) has been shown to inhibit proteasomal malfunction as well as the buildup of ubiquitin-conjugated proteins and other potentially hazardous proteins (Amro et al., 2018). On the other hand Puerarin, lowers the ratio of bcl-2/bax and caspase-3 activity (Cheng et al., 2009). Puerarin protects tyrosine hydroxylase (TH)-positive neurons from 6-OHDA-mediated injury, recovers DA and its metabolites (Zhu et al., 2010).
4.1.8 Juglandis semen
The neuroprotective effects of aqueous Juglandis semen (walnut) extract have been demonstrated. The walnut extract was reported to reduce reactive oxygen species (ROS) and nitric oxide (NO) formation as well as restrict the depletion of striatal DA and its metabolites, resulting in a considerable improvement in PD movement abnormalities in a mouse model of Parkinson’s disease (Choi et al., 2016). Walnut is thought to have neuroprotective effects because it can block the monoamine oxidase B (MAO-B) enzyme, which increases oxidative stress in people with Parkinson’s disease. Walnut also has antioxidant and mitochondrial protection properties (Essa et al., 2015).
4.1.9 Ginkgo biloba
Ginkgo biloba (Ginkgoaceae) is a Chinese tree that has long been used to treat symptoms related to heart and lung problems. Flavonoids, ginkgolic acid and terpenoids are three of the most common constituents in G. Biloba (DeFeudis and Drieu, 2000). In a PD rat model treated with 1-methyl-4-phenyl-1,2,3,6-tetrahydropyridine (MPTP), long-term use of EGb761 prevented the loss of dopaminergic nerve terminals caused by MPTP (Ramassamy et al., 1990). EGb761 was shown to protect against dopaminergic neurotoxicity caused by MPTP whether it was given before or after the treatment (Sharma et al., 2019b). Also, EGb761 decreased the neurotoxicity of levodopa in the 6-hydroxydopamine (6-OHDA) Parkinson’s disease (PD) model. This suggests that levodopa is neurotoxic and that EGb761 may reduce this toxicity (Fei et al., 2003).
4.1.10 Ginseng
Ginsenosides (Araliaceae) Rb1 and Rg1 are regarded to be the primary molecules responsible for ginseng’s therapeutic properties. A previous study found that the ginsenosides Rb1 and Rg1 both decreased MPTP-induced cell death in SN-K-SH cells (a neuroblastoma cell line) (Rudakewich et al., 2001). Rg1 protects cells against apoptosis caused by MPTP by increasing Bcl-2 and Bcl-xl expression, decreasing Bax and iNOS expression and blocking caspase-3 activation (Chen et al., 2002). Ginsenosides protect by lowering intracellular reactive oxygen species (ROS), boosting antioxidant activity, maintaining complex I activity, and raising intracellular Adenosine triphosphate (ATP) levels, according to research. Mice given 1-methyl-4-phenyl-1,2,3,6-tetrahydropyridine (MPTP) had better motor function and more dopaminergic neurons in the substantia nigra (SN) and striatum when they were given Rg1 (Jiang et al., 2015). In addition, the ginsenoside Rb1 has the ability to disaggregate fibrils and inhibit α-synuclein polymerization (Ardah et al., 2015; Rahman et al., 2021b).
4.1.11 Flavonoids
Flavonoids are a type of natural polyphenol phytochemical that has been used as a medicinal agent for many years. Baicalin, a flavonoid derived from Scutellaria baicalensis, is the major metabolite of baicalein (Lamiaceae). In an in vivo model of Parkinson’s disease with the neurotoxin 6-OHDA, which showed protective effects on dopaminergic dysfunction and lipid peroxidation, the neuroprotective effects of baicalein were shown to be real (Im et al., 2005). Lutein and apigenin, two flavones, protect dopaminergic neurons against inflammatory neurotoxicity. (Chen et al., 2008).
4.1.12 Valeriana officinalis
Valeriana officinalis (Valerian) is a sedative and antispasmodic herb that has long been used for sleeplessness, anxiety, and restlessness. In SH-SY5Y cells, valerian has been shown to inhibit rotenone-induced cell death (Amaral de Brito et al., 2020). Furthermore, valerian extract was effective in reducing rotenone toxicity in Drosophila melanogaster, as evidenced by the normalization of superoxide dismutases-SOD and catalase mRNA expression, implying that valerian’s effects are, at least in part, due to the plant’s antioxidant properties due to its phenolic and flavonoid constituents (Sudati et al., 2013).
4.1.13 Passion flower
Flavonoids, glycosides, alkaloids, and phenolic chemicals are all found in passion flowers, also known as Passiflora incarnata (Passifloraceae). Anxiety, epilepsy, sleeplessness, muscle spasms, and other disorders have all been treated with it (Dhawan et al., 2004). As a result, the biological effects of passion flowers on people with Parkinson’s disease have been investigated. The quantity of jaw movements produced by tacrine, a typical animal model of Parkinson’s disease tremors, was reduced by passionflower extract. The animal’s cognitive abilities improved as well, with haloperidol-induced catalepsy lasting significantly less time. As evidenced by its strong scavenging ability, the passionflower has antioxidant activity (Ingale and Kasture, 2017).
4.1.14 St. John’s Wort
St. John’s wort (Hypericaceae) contains naphthodianthrones, phloroglucinols, flavonoids as well as essential oils, have all been found as active ingredients. As a result, the active ingredients have antioxidative and neuroprotective properties (Barnes et al., 2001). We looked at how two standardized extracts of St. John’s Wort affected neurodegeneration in rats caused by long-term rotenone treatment. St. John’s wort, through lowering Bax levels, decreased neuronal damage and prevented the apoptotic process (Kiasalari et al., 2016). Furthermore, rats with intrastriatal 6-hydroxydopamine (6-OHDA) lesions were given a St. John’s wort extract, which resulted in lower striatal malondialdehyde levels, increased catalase activity, decreased glutathione (GSH) content, normalized Glial fibrillary acidic protein (GFAP) and tumor necrosis factor alpha or TNF-α expression, reduced Deoxyribonucleic acid (DNA) fragmentation, and prevented dopaminergic neuron damage (Kiasalari et al., 2016).
4.1.15 Terpenoids
Centella asiatica is a medicinal herb that appears to help with rheumatoid arthritis, inflammation, and mental and physical weariness. Bornyl acetate, α-pinene, β-pinene and δ-terpinene are all monoterpenes found in the leaves of this plant (Asakawa et al., 1982; Brinkhaus et al., 2000). Acetylcholinesterase (AChE) activity was shown to be inhibited by these monoterpenes. C. paniculatus ethanolic plant extract exhibited sedative and antidepressant properties (in vivo) (Sakina and Dandiya, 1990). Compound 5 reduced Ab formation in neuroblastoma cells transfected with amyloid precursor protein (APP) constructs. Toxiigenin also reduced the activity of b-proteolytic secretase on its substrate. In the same way, a pharmacological study found that P. tenuifolia (BT-11) dried root extract (80% ethanol-water) could help rats with scopolamine-induced amnesia learn and remember better (Jia et al., 2004).
4.1.16 Phenolics
Curcuma longa has been used for anti-aging in Ayurvedic medicine for thousands of years. Curcumin, an antioxidant and anti-inflammatory substance, is found in the rhizomes of Curcuma longa (Arshad et al., 2017; Shah et al., 2021; Memarzia et al., 2022; Salem et al., 2022). The compound also showed neuroprotective properties when it came to ethanol-induced brain damage. Curcumin reduced lipid peroxide levels while increasing glutathione levels when taken orally (Kim et al., 2001). Curcumin, demathoxycurcumin, bis-demethoxycumin, and calebin-A, all extracted from Curcuma longa, have been shown to protect PC12 cells from β-amyloid assault (Maurer et al., 1997; Park and Kim, 2002). The stem bark of Knema laurina was used to isolate compounds 18–22. The most significant Acetylcholinesterase (AChE inhibitory activity was found in compound 22, which had an IC50 of 0.573 mM (Akhtar et al., 2011). In vitro experiments showed that a fruit extract from Styraxagrestis suppressed AChE. Three novel egonoltypebenzofurans were isolated using a bioassay-guided fractionation and isolation method: egonol-9(Z),12(Z) linoleate, 7-demethoxyegonol9(Z),12(Z) linoleate and 7-demethoxyegonol oleate (IC50 = 1.4–3.1 mM) (Liu et al., 2011).
4.1.17 Alkaloids
In vitro and in vivo, the lycopodium alkaloid huperzine A (structurally similar to quinolizidine) obtained from H. serrata is a reversal inhibitor of AChE (Small et al., 1997; Shu, 1998; Wang et al., 2000; Zhou et al., 2001). It stopped acetylcholinesterase from working (IC50 = 0.08 mM) (Xiao et al., 2002). The alkaloid leonurine, derived from Leonurus heterophyllus Sweet, inhibited ROS, protected mitochondrial integrity, and reduced cytochrome c levels in vivo (Kuribara et al., 2000). The rhizomes of Coptis chinensis have yielded numerous alkaloids, including berberine, groenlandicine, palmatine, jateorrhizine, coptisine, and epiberberine. These compounds can stop AChE from doing its job. In addition, the compounds groenlandicine and epiberberine inhibited beta-secretase enzymatic activity (Karakida et al., 2007). The marine Streptomyces sp. Strain LB173 produced a novel phenazine natural product called geranylphenazinediol. With an IC50 value of 2.62mM, compound 91 inhibited the AChE enzyme (Ohlendorf et al., 2012).
4.1.18 Mucuna pruriens
Mucuna pruriens (Mp) has therapeutic qualities in all of its components. They are said to have anti-inflammatory, analgesic, anti-epileptic, anti-neoplastic and anti-microbial properties (Adepoju and Odubena, 2009). They showed that Mp is a better way to treat Parkinson’s disease over the long term than standard L-DOPA therapy, which causes severe dyskinesia when used for a long time. MP’s seed, leaf, and stem all have significant neuroprotective properties. Because seeds have more L-DOPA than other parts of the plant, they are often used as anti-PD drugs (Benfica et al., 2020).
4.1.19 Withania somnifera
Environmental poisons Maneb (MB) and paraquat (PQ) have been used in tests to generate selective damage to dopaminergic neurons, leading to the development of Parkinson’s disease (PD). When an ethanolic root extract of Withania somnifera (Ws) was given to a mouse model of Parkinson’s disease caused by MB-PQ, it was shown to significantly improve classic Parkinson’s disease symptoms like slow movement, less dopamine in the substantia nigra and different types of oxidative damage (Ahmad et al., 2005; Yadav et al., 2012).
4.1.20 Tinospora cordifolia
T. cordifolia is classified as “Rasayana” in Ayurveda and is used to combat infections due to its general adaptogenic and prohost immunomodulatory effect. It has antiandrogenic and anti-inflammatory properties, is effective against throat cancer, stress, and promotes learning and memory. In an acute toxicity investigation, T. cordifolia was shown to be nontoxic. It has recently been intensively researched and shown to have significant antioxidant action. Because antioxidants are known to prevent or protect neurodegeneration, the current study was designed to assess the anti-Parkinson’s efficacy of T. cordifolia ethanol extract (TCEE) (Kosaraju et al., 2014).
4.1.21 Bacopa monnieri
In vitro and in animal models of neurodegenerative illness, B. monnieri’s antiparkinsonian efficacy has been studied in vitro. B. monnieri’s antioxidant and neuroprotective properties confer anti-Parkinsonian action, which is related to decreased-synuclein protein aggregation and the selective death of dopaminergic neurons. In worms, B. monnieri reduced synuclein aggregation, prevented dopaminergic neurodegeneration, and restored lipid content, indicating that this species may have anti-Parkinsonian effects (Hosamani, 2009).
4.2 Marine derived compound
Natural marine-derived chemicals might have several pharmacologic effects and could be extremely beneficial for the creation of novel medicines (Corona, 2018).
4.2.1 Archaea
Many superheats may produce Zwitterionic organic products to avoid thermal denaturation and protein aggregation under severe circumstances like as extreme temps and osmolarity (Empadinhas and da Costa, 2011). In a hot environment, hyper thermophilic bacteria produce mannosylglycerate (MG) as a suitable solute. In a PD yeast model, MG inhibited the development of α-synuclein aggregates. Mannosylglycerate may help α-synuclein fold properly, preventing abnormal aggregation. MG is a promising therapy for PD (Faria et al., 2013).
4.2.2 Bacteria
Ancillary marine compounds provide a rich pharmacological source with new chemical structures and a wide range of biological activity (Nikapitiya, 2012; Monciardini et al., 2014). NP7 is a marine Streptomyces sp. Compound (NP7) is an anti-oxidant and could cross The BBB. H2O2, caused Apoptosis and H2O2 in nerves and Microglia cells NP7 at 5–10 μM might avoid (Koppula et al., 2012). Piloquinones, marine-derived chemicals isolated from Streptomyces sp. Have been found to have inhibitory action of MAO-B (Takeuchi et al., 1973). The Streptomyces sp. CNQ-027 isolated piloquinones A, and B (Nam et al., 2011).
4.2.3 Fungi
Many marine fungus metabolites can have neuroprotective anti-PD actions. Neoechinulin A is an isoprene quinone alkaloid produced by the reddish algae-based fungi Aspergillus sp. and Microsporum sp (Li et al., 2004). Neoechinulin A might protect PC12 cells from the neuronal mortality of MPP+ and peroxynitrite through the reversal of mitochondrial complex I malfunction (Kimoto et al., 2007; Kajimura et al., 2008). The natural substance is Aspergillus ochraceous and Paecilomyces sp, secalonic acid A derived from marine fungal (Kurobane et al., 1987). The suppression of p38 phosphorylation and JNK and reducing Calcium entry and caspase-3 activation have dramatically reduced the induced colchicine apoptosis of cortical nerve cells at 3–10 μM (Zhai et al., 2011).
4.2.4 Algae
Marine algae have a strong antioxidant supply (Meenakshi et al., 2011). Carotenoids, notably astaxanthin, produced by marine micro-organisms, have proved to be a useful adjuvant therapy in preventing and/or delays of NDs, for example, Haematococcus pluvialis and Chlorella zophingiensis (Yuan et al., 2011; Galasso et al., 2017). Astaxanthin may generate mouse anti-PD effects (Grimmig et al., 2017a). Astaxanthin has been proven to decrease microglial activation in the mouse brain (Grimmig et al., 2017b). The marine carotenoid of Fucoxanthin that comes from edible brown seaweeds has demonstrated antioxidant and anti-inflammatory effects (Gammone et al., 2015). By stimulating the PI3-K/Akt cascade, Fukoxanthin might reduce H2O2 neuro substances and block the ERK route (Lin et al., 2017).
4.2.5 Mollusk
A kinase inhibitor called staurosporine (AM-2282) was first discovered in the actinomycete Streptomyces staurosporeus (Karaman et al., 2008). Additionally, flatworm and marine sea squirt contained staurosporine (AM-2282) (Schupp et al., 2001). Staurosporine (AM-2282) at 10 nM might promote DA neurite outgrowth by activating the AMP-activated protein kinase (AMPK)/mammalian target of rapamycin (mTOR) signaling pathway in mesencephalic primary cultures (Wakita et al., 2014). Additionally, staurosporine (AM-2282) may shield neurons from damage brought on by ischemia (Hara et al., 1990). Staurosporine is extremely poisonous, nevertheless. Staurosporine analogues have been generated by structural alteration to reduce toxicity (Bharate et al., 2013).
4.2.6 Sea cucumber
A marine mollusc called the sea cucumber has vital nutrients. In many Eastern nations, sea cucumber is recognized as a tonic and a traditional therapy for neurodegenerative illnesses. Whole body-ethyl acetate (WBEA), whole body-butanol (WBBU), and body wall-ethyl acetate are extracts of the sea cucumber Holothuria scabra (BWEA). These compounds can stop the loss of DA neurons caused by 6-OHDA in Caenorhabditis elegans by a large amount. Additionally, these extracts could restore lipid content and prevent the aberrant aggregation of α-synuclein (Chalorak et al., 2018). SCG-1, SCG-2 and SCG-3 are significant sphingolipids found in Cucumaria frondosa’s sea cucumber extract (Xu et al., 2013). SCG-1, SCG-2 and SCG-3 may promote neurite outgrowth in NGF-induced PC12 cells in a dose-dependent and structure-specific manner, most likely through boosting TrkA phosphorylation and upregulating BDNF expression (Wang et al., 2018). These findings showed that the active components in sea cucumber extracts and their potential anti-PD capabilities.
5 Disorder associated with PD
Some disorders associated with PD are shown in Figure 2.
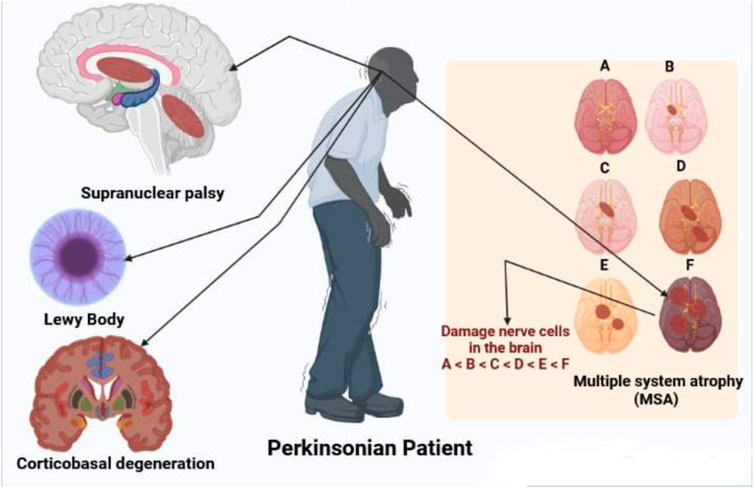
FIGURE 2. Disorders associated with parkinsonian patient like supranuclear palsy, LB multiple system atrophy and corticobasal degeneration.
5.1 Supranuclear palsy
PSP and CBD are the two most frequent tauopathic PD. PSP affects 6.4 persons out of 100,000 (Ikram et al., 2021). Initiating age is an average of 63, males are most affected and survival time is 6–7 years (Wenning et al., 1998). PSP is defined by the early onset of widespread and stiff reverse dips, as well as supranuclear vision paralysis with slow relative direction and difficulty staring down (Litvan et al., 1996a). The NINDS-SPSP clinical diagnosis for probable PSP are based on vertically supranuclear vision paralysis and noticeable poor balance, which occur within the first year of illness start and have the high selectivity (50–62)%, specificity 100%, and positively useful predictions (Litvan et al., 1996b; Litvan et al., 2003).
5.2 Multiple system atrophy
Sporadic progressive adult diseases, with an incidence of around 4.4 instances per 100,000 population, are multiple system atrophies (Schrag et al., 1999; Watanabe et al., 2002). The existence of at least six of the following functions have been consistently identified for MSA: Sporadic adult-onset, self-representation, parkinsonism, cerebral characteristics, pyramidal symptoms, there is a shortage of levodopa, downward ocular paralysis and cognitive dysfunctions (Litvan et al., 1998). Diagnosis of MSA is missed even at tertiary reference centers, in 50%–75% of cases (Litvan et al., 1997). Nigral and putamen degeneration and degeneration in at least one region are part of pathological diagnostic criteria (Ito et al., 1996).
5.3 Dementia with LBs or LB disease
The second most frequent kind of dementia in advanced age is progressive dementia with LBs, typically accompanied by parkinsonism, good visual hallucinations, and oscillations in cognition, alertness, and concentration (McKeith et al., 1996). DLB is dementia that affects the optical, perceptual, and careful functions of the brain (Collerton et al., 2003). The age at which the DLB begins is 60–68, with an average disease duration of 6–7. Men are more impacted than women (Gualtieri, 2004). DLB diagnostic criteria differ substantially in their sensitivity and specificity and improved criteria are needed (Lopez et al., 2000). Cases with more substantial DLB disease have typical symptoms, whereas cases with larger neurofibrillary tangles are likely to show AD (Ballard et al., 2004). Continuing to work memory issues, visual spatial difficulties, psychotic episodes, melancholy, unconcern, and low mood are among early signs of DLB (Simard et al., 2000).
5.4 Corticobasal degeneration or degeneration of corticobasal ganglions
Degeneration of the corticobasal ganglion is gradual, with unilaterally akinesia and rigidity responding badly to apraxia (especially ideomotor apraxia) and levodopa. Myoclonus of the cerebral reflex, limb rigidity, alien limb signs, and cortical sensory loss is all symptoms (Riley et al., 1990; Stover and Watts, 2001). Despite the fact that there are various requirements for diagnoses were submitted, none were validated and their warnings were explored elsewhere (Litvan et al., 2003). The estimated prevalence per 100,000 individuals is 4.9–7.3 cases (Togasaki and Tanner, 2000).
5.5 PD and sleep disorders
5.5.1 Recurrent PD symptoms and sleep fragmentation
Insomnia at night and daytime sleepiness are prevalent among people with PD (Figure 3). 60 to 98 percent of PD patients experience nighttime difficulties (Kales et al., 1971; Lees et al., 1988; Larsen, 2003). 33% of individuals experience moderate to severe sleep difficulties at night (Svensson et al., 2012). The most prevalent complaint of PD patients in nighttime sleep is many nightly awakes or fragmented sleep (Factor et al., 1990). PD fragmentation of sleep A lot of etiology including nightly recurrence of PD symptoms, medicine, coexisting apnea of sleep, and regular limb sleep movements (Comella et al., 2005). Patients with PD may experience nocturnal waking and difficulty sleeping (Factor et al., 1990; Happe et al., 2001). Levodopa medicament can reduce the fragmentation of sleep owing to recurring symptoms and enhance early-morning function (Askenasy and Yahr, 1985; Juncos et al., 1987; Jansen and Meerwaldtt, 1990; Pahwa et al., 1993).
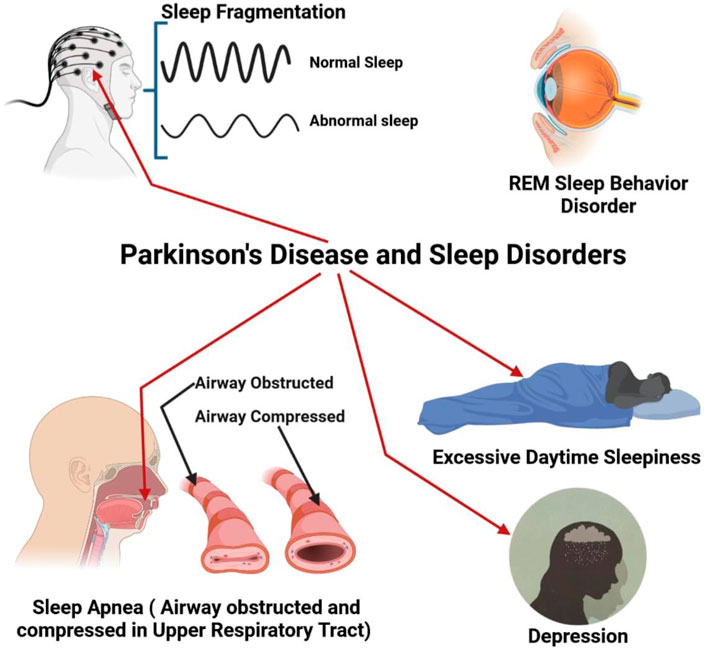
FIGURE 3. PD with different types of sleep disorder like sleep fragmentation, REM (Rapid Eye Movement), Breathing problem during sleep (Apnea), increase daytime sleep and finally depression.
5.5.2 Sleep apnea
Obstructive sleep apnea was once thought to be a minor issue in PD since it was generally associated with a high BMI. Sleep apnea, on the other hand, is more frequent in PD than previously thought. Despite having a normal BMI, 20 to 50 percent of PD patients tested by sleep study had significant apnea, according to descriptive investigations (Ferini-Strambi et al., 1992; Maria et al., 2003; Braga-Neto et al., 2004). People with multiple system atrophy (MSA) have a high prevalence of respiratory problems during sleeping. MSA patients may have difficulty breathing during sleep due to vocal cord abductor paralysis (Isozaki et al., 1996; Braga-Neto et al., 2004).
5.5.3 REM sleep behavior disorder
REM behavioral disorder is a sleep condition characterized by muscular movement in REM sleep with dream activity (Schenck et al., 1986; Schenck et al., 1987; Schenck et al., 2002). REm atony-free sleep (RWA) displays aberrant muscle activation without obvious behavioral behavior during REM sleep (Gagnon et al., 2002). Though RBD’s clinical characteristics seem distinct, sleep apnea can lead to a comparable condition (Iranzo et al., 2005). In patients with Basic RBD, a SPECT scan reveals a reduction in dopaminergic transport which is midway among normal controls and Parkinson patients (without parkinsonism) (Eisensehr et al., 2003).
5.5.4 Excessive daytime sleepiness in PD
The typical complaint of PD-patient patients is excessive daily drowsiness (EDS). This problem has been characterized as sleep daily for PD patients on ropinirole or pramipexole, as well as a result of motor vehicle accidents recorded in 1999 (Hobson et al., 2002). Dementia and the fast advancement of parkinsonism were related to the onset of EDS (Gjerstad et al., 2002). Since its debut in the 1960s, levodopa has been known to produce drowsiness. Levodopa monotherapy produced drowsiness in the first round of 131 PD patients and restricted the dosage of levodopa in 14% of patients (Lesser et al., 1979). Although community studies indicated that almost any dopamine agonist, particularly levodopa, may cause EDS, it was shown that levodopa was the most closely connected to the disease (Hauser et al., 2000; Hobson et al., 2002).
5.6 Depression
Epidemiological studies are regarded as a common non-motor discovery in PD and prevalence calculations range from 2.7 percent to 70 percent. The degree of depression (moderate to serious) and the definition of depression undoubtedly influence these figures. Estimates dispute PD age and depresence, as well as the period of disease, severity of disease, and gender problems (Olanow et al., 20012001; Burn, 2002). Neurochemical and neuroimaging approaches have been appraised in the functions of dopamine, norepinephrine and serotonin, although no definite pathophysiological mechanism is recognized. A function was proposed for an allelic variant in the serotonin transporter (Mössner et al., 2001).
6 How natural product works on PD
6.1 Cellular development and apoptosis
The Bax (BCL2 associated X apoptosis regulator)/Bcl-2 (B-cell lymphoma 2) ratio, as well as caspase-3 activity, are important in apoptosis and cellular development. In this way, Bax may stop apoptosis from happening while Bcl-2 helps it happen and both caspase-3 and caspase-9 can stop Bcl-2 from doing its job. By forming the TP53-HIPK2-AXIN1 complex, HIPK2 (Homeodomain-interacting protein kinase-2) can control the creatine kinase and transcriptional activation of TP53 (Tumor protein 53). This slows down cell growth and speeds up cell death (Li et al., 2019). In cells exposed to MPP (+), Pedicularioside A inhibited the production of the caspase-3 gene as well as the cleavage of poly (ADP-ribose) polymerase (PARP) (Li et al., 2008). Pedicularioside A exerts a protective effect on mesencephalic neurons, increasing their longevity.
6.2 Anti-inflammatory pathway
Pathogenesis of PD is exacerbated by neuroinflammation and oxidative stress (Li et al., 2019). Some transcription factors, for instance MAFG, can promote nNOS by raising NRF1 expression. Nuclear factor erythroid-2-related factor 2 (Nrf2) protein levels and transcriptional activity, as well as overexpression of Nrf2-dependent genes, were shown to rise following therapy. A ligase modulatory component is seen in SH-SY5Y cells (Jing et al., 2016).
6.3 Dopamine transmission
The dopamine transporter (DAT) is in charge of DA re-uptake by altering the PP2A kinase pathway, α-synuclein inhibits VMAT2 but enhances DAT function (Dorsey et al., 2007). NURR1 expression was shown to be considerably lower in Parkinson’s sufferers, according to research, NURR1 has the ability to induce differentiation, maturity, and growth of dopaminergic neurons, protection of dopaminergic neurons, and reduction of inflammation-induced necrosis (Li et al., 2019; Rahman et al., 2022b). Immunohistochemistry results showed that treatment with ME (dried mulberry fruit extract) greatly reduced the overexpression of SNCA (Synuclein Alpha) and ubiquitin, which are two of the most important parts of LBs (Gu et al., 2017).
7 Herb derived Anti-Parkinson compound
The herb derived Anti-Parkinson components may produce very good activity. Some of these components are displayed in Table 1.
8 Clinical trials
8.1 Mucuna pruriens (L.) DC
Numerous laboratories are investigating the remarkable properties of the tropical legume M. pruriens for the treatment of PD. For the first time, a multicentric preclinical trial described the efficacy of the herbal preparation (HP-200) derived from the tropical legume for the treatment of the disease, which was administered to 60 PD patients for 12 weeks (Sengupta et al., 2016). The Hoehn and Yahr (H&Y) scale scores decreased statistically significantly, while the United Parkinson’s Disease Rating Scale (UPDRS) scores improved from the baseline level.
8.2 Hyoscyamus niger L
H. niger has been utilized for a variety of ailments in the traditional medical systems of many cultures, including Indian, Chinese, Roman, and Byzantine. No reference is made in the literature to its independent usage for the treatment of parkinsonism in either human patients or any animal model. However, a clinical research has confirmed its efficacy when combined with three additional Ayurvedic herbs. H. niger contains little L-DOPA (Nagashayana et al., 2000), but it is abundant in tropane alkaloids including hyoscine and hyoscyamine that are well known for their anticholinergic properties (Brown and Taylor, 2006). A relative excess of the neurotransmitter acetylcholine exists in the striatum due to the depletion of DA in PD, which is thought to be one of the causes of the many motor impairments linked to PD. Anticholinergics are therefore used to treat PD, especially tremor (Mirahmadi et al., 2016).
8.3 Nardostachys jatamansi DC
In a 6-OHDA-rat model of PD, an ethanolic extract of the Ayurvedic plant N. jatamansi roots can reduce neuronal damage (Ahmad et al., 2006). The extract effectively decreased the neurotoxin-induced lipid peroxidation, increased GSH content, the activities of GT, GR, GP, SOD, and catalase, attenuated the loss of catecholamines, increased DA-ergic D 2 receptor binding, and increased TH-immunoreactivity in the animals before 6-OHDA lesioning. N. jatamansi extract also dose-dependently reversed the dopaminomimetics-induced rotations and deficits in locomotor activity and muscular coordination brought on by nigrostriatal degeneration.
8.4 Bacopa monnieri L
Ayurveda makes extensive use of this plant as a brain booster. Recent preclinical studies have demonstrated its effectiveness in treating PD. B. monnieri extract was found to have significant preventive action in the paraquat-induced PD model in Drosophila and mice, principally through antioxidant capabilities and restoration of the mitochondrial ETC complexes activities (Ravikumar and Muralidhara, 2010; Hosamani et al., 2016). The ability of the plant’s alcoholic extraction to shield 6-OHDA-lesioned rats from behavioral and biochemical abnormalities were demonstrated to be significantly influenced by similar antioxidant effect (Shobana et al., 2012).
9 Challenges with current synthesis
When several neurotoxic models of Parkinson’s disease are put together, they make a good framework for finding anti-Parkinsonian drugs. Herbal medicines can also be used to make new Parkinson’s disease treatments. But in the future, real-world studies should look into how well plant extracts and their active parts work in PD models (Mercuri and Bernardi, 2005; Diaz and Waters, 2009). Aside from the fact that the gold standard, levodopa, does not operate on the cause, there is an additional restriction in that the symptoms become exceedingly severe after a lengthy period of therapy (Odin et al., 2008). Another significant issue with L-dopa is that it promotes neurodegeneration by causing oxidative stress (Pahwa and Lyons, 2009). While levodopa has all of these side effects, other PD medications might cause sleep difficulties and cognitive issues such disorientation and psychosis, MAO-B inhibitors, COMT inhibitors, and other similar drugs anticholinergic medications (Yuan et al., 2010). Due to great success, DBS (Deep brain stimulation) in the subthalamic nucleus (STN) and globuspallidus internus (GPi) is currently the most popular therapy (Rocchi et al., 2012; Doke et al., 2019). However, DBS has the same drawbacks in that it does not stop the disease from developing and does not prevent it from deteriorating various signs and symptoms (Tsai et al., 2009). Rehabilitative therapy is a type of treatment for PD that involves daily exercises such as stretching and muscle strengthening, exercises to strengthen and improve posture (Tomlinson et al., 2013; Clarke et al., 2016). So overall it can be said that though PD have many treatments but they have also some drawbacks that’s why these treatments are not so ideal. Further research is warranted to explore several folklore or traditional medicinal herbs and their myriad of bioactive phytochemicals to develop new safe and effective anti PD drug agents (Sharma et al., 2022a; Sharma et al., 2022b; Sharma et al., 2022c).
10 Conclusion and future perspectives
We discovered that several organic substances and herbal extracts display varied anti-Parkinsonian properties. When numerous PD neurotoxic models are coupled, they give a good framework for discovering anti-Parkinsonian drugs, and herbal medications can be employed to develop novel PD treatments. But in the future, real-world studies should look into how well plant extracts and their active parts work in PD models. Additionally, there is still a need for more thorough explanations of the constituents and methods of action of herbal extracts. To assure strong reproducibility, to boost therapeutic benefit, and to lessen the possibility of harm, methodological improvements must be made to upcoming clinical studies using natural products for the treatment of PD. The study design should include double blinded trials and the use of placebos. Before beginning clinical trials, protocols must be established to ensure the openness of the study findings.
10.1 PD vaccine
DC vaccination is a cell-based treatment. It uses antigen-loaded or sensitized DCs as the vehicle for vaccination to cause an immune response, and it is very important for getting early immune responses. T-cells become activated when they are exposed to antigen (Steinman, 1991; Boer et al., 2015). Also, peptide-sensitized DC (PSDC) vaccines cause an antigen-specific immune response that lasts longer than traditional vaccinations (Steinman, 2001). In some way, these DCs have become sensitized. They were subsequently used as a vaccination to activate immunological responses in Tg mice, which produce the 140-amino-acid full-length protein α-synuclein (B6; C3-Tg (Prnp-SNCA*A53T) human A53T variant 83Vle/J), which is controlled by the mouse prion protein promoter (Ugen et al., 2015).
10.2 Cell transplantation
Researchers have looked at many different cell sources to make dopaminergic neurons that can be transplanted into Parkinson’s disease patients (Lindvall, 2016). Nevertheless, current experimental and clinical evidence strongly imply that, in order to deliver significant therapeutic effect, the clinical candidate cell must be of human origin and possess the following characteristics: A neuron in the substantia nigra (Lindvall et al., 2012; Rahman et al., 2021c; Bhattacharya et al., 2022). In the first experiment to show that human ES cell-derived dopaminergic neurons can survive intrastriatal implantation and give birth to dopaminergic neurons in a rat Parkinson’s disease model, the behavior of almost all of the people improved (Roy et al., 2006). After intrastriatal transplanting, a large percentage of substantia nigra dopaminergic neurons survived for a long time, there were no tumors seen in the rodents. Furthermore, a large area of the brain was re-innervated thanks to the transplants in a bigger (nonhuman primate) brain, the striatum and behavioral impairments that resemble symptoms have improved in PD patients (Lindvall et al., 2012). Grealish and others found that grafts of human ES cell-derived dopaminergic neurons placed in a mouse were effective, axonal regeneration is possible in the rat model of PD as well as functional growth and long-term survival efficacy more than the human foetal mesencephalic dopaminergic neurons (Grealish et al., 2014). Even if no tumors have been found, safety is still an important consideration when considering human transplantation, Dopaminergic neurons generated from ES cells in patients with PD (Kriks et al., 2011; Kirkeby et al., 2012; Grealish et al., 2014; Rahman et al., 2022c).
10.3 Gene therapy
In 1972, gene therapy was first proposed as a way to replacing bad DNA with good DNA (Friedmann and Roblin, 1972). There are a variety of techniques, but the most common is the use of designed non-replicating viral vectors, primarily recombinant viruses of various serotypes lentivirus or adeno-associated virus (AAV) (Lang et al., 2006). Treatments with non-disease modifying medications aim to relieve the symptoms of PD by attempting to restore aberrant firing of dopaminergic or GABA-producing enzymes in the basal ganglia (Axelsen and Woldbye, 2018). So, we can expect that the gene therapy will be one the most promising therapeutic technique.
10.4 Surgical method
Deep brain stimulation (DBS) or localized treatments may help patients with PD enhance their quality of life and functional independence (Weaver et al., 2009). Surgical lesions and deep brain stimulation (DBS) improve medication and reduce dyskinesia as compared to standard medical therapy (Vitek et al., 2003; Weaver et al., 2009). New surgical and stimulation approaches are reshaping the sector, and technology improvements may enhance possible outcomes. Surgical decision-making is difficult, as it involves determining the best surgical candidates, among other things, such as methodology, complication management, hardware, and code changes, all of which necessitate a multidisciplinary team effort (Mitchell and Ostrem, 2020).
Author contributions
MR: Writing original draft; XW, MI, SA, FS, MM, MH-O-R: Collection of data and analysis; Most. NA, MK, FJ, RS, BS: Editing and proofreading; AR, RS: Supervision and proofreading.
Conflict of interest
The authors declare that the research was conducted in the absence of any commercial or financial relationships that could be construed as a potential conflict of interest.
The handling editor HB declared a past collaboration with the author RS.
Publisher’s note
All claims expressed in this article are solely those of the authors and do not necessarily represent those of their affiliated organizations, or those of the publisher, the editors and the reviewers. Any product that may be evaluated in this article, or claim that may be made by its manufacturer, is not guaranteed or endorsed by the publisher.
References
Adepoju, G., and Odubena, O. (2009). Effect of Mucuna pruriens on some haematological and biochemical parameters. J. Med. Plants Res. 3, 73–76.
Ahmad, M., Saleem, S., Ahmad, A. S., Ansari, M. A., Yousuf, S., Hoda, M. N., et al. (2005). Neuroprotective effects of Withania somnifera on 6-hydroxydopamine induced Parkinsonism in rats. Hum. Exp. Toxicol. 24, 137–147. doi:10.1191/0960327105ht509oa
Ahmad, M., Yousuf, S., Khan, M. B., Hoda, M. N., Ahmad, A. S., Ansari, M. A., et al. (2006). Attenuation by nardostachys jatamansi of 6-hydroxydopamine-induced parkinsonism in rats: Behavioral, neurochemical, and immunohistochemical studies. Pharmacol. Biochem. Behav. 83, 150–160. doi:10.1016/j.pbb.2006.01.005
Akhtar, M. N., Lam, K. W., Abas, F., Ahmad, S., Shah, S. A. A., Choudhary, M. I., et al. (2011). New class of acetylcholinesterase inhibitors from the stem bark of Knema laurina and their structural insights. Bioorg. Med. Chem. Lett. 21, 4097–4103. doi:10.1016/j.bmcl.2011.04.065
Altun, M. L., Yılmaz, B. S., Orhan, I. E., and Citoglu, G. S. J. I. C. (2013). Assessment of cholinesterase and tyrosinase inhibitory and antioxidant effects of Hypericum perforatum L.(St. John's wort). Industrial Crops Prod. 43, 87–92. doi:10.1016/j.indcrop.2012.07.017
Amaral de Brito, A. P., Galvão de Melo, I. M. S., El-Bachá, R. S., and Guedes, R. C. A. J. F. N. (2020). Valeriana officinalis counteracts rotenone effects on spreading depression in the rat brain in vivo and protects against rotenone cytotoxicity toward rat glioma C6 cells in vitro. Front. Neurosci. 23, 759. doi:10.3389/fnins.2020.00759
Amro, M., Teoh, S., Norzana, A., and Srijit, D. J. L. C. (2018). The potential role of herbal products in the treatment of Parkinson’s disease. Clin. Ter. 169, e23–e33. doi:10.7417/T.2018.2050
Ardah, M. T., Paleologou, K. E., Lv, G., Menon, S. A., Khair, S. B. A., Lu, J.-H., et al. (2015). Ginsenoside Rb1 inhibits fibrillation and toxicity of alpha-synuclein and disaggregates preformed fibrils. Neurobiol. Dis. 74, 89–101. doi:10.1016/j.nbd.2014.11.007
Arenas, E. J. (2014). Wnt signaling in midbrain dopaminergic neuron development and regenerative medicine for Parkinson's disease. J. Mol. Cell Biol. 6, 42–53. doi:10.1093/jmcb/mju001
Arshad, L., Haque, M. A., Abbas Bukhari, S. N., and Jantan, I. (2017). An overview of structure–activity relationship studies of curcumin analogs as antioxidant and anti-inflammatory agents. Future Med. Chem. 9, 605–626. doi:10.4155/fmc-2016-0223
Asakawa, Y., Matsuda, R., and Takemoto, T. J. P. (1982). Mono- and sesquiterpenoids from Hydrocotyle and Centella species. Phytochemistry 21, 2590–2592. doi:10.1016/0031-9422(82)85264-3
Ascherio, A., Zhang, S. M., Hernán, M. A., Kawachi, I., Colditz, G. A., Speizer, F. E., et al. (2001). Prospective study of caffeine consumption and risk of Parkinson's disease in men and women. Ann. Neurol. 50, 56–63. doi:10.1002/ana.1052
Askenasy, J., and Yahr, M. J. N. (1985). Reversal of sleep disturbance in Parkinson's disease by antiparkinsonian therapy: A preliminary study. Neurology 35, 527–532. doi:10.1212/wnl.35.4.527
Auning, E., Selnes, P., Grambaite, R., Šaltytė Benth, J., Haram, A., Løvli Stav, A., et al. (2015). Neurobiological correlates of depressive symptoms in people with subjective and mild cognitive impairment. Acta Psychiatr. Scand. 131, 139–147. doi:10.1111/acps.12352
Axelsen, T. M., and Woldbye, D. P. (2018). Gene therapy for Parkinson’s disease, an update. J. Park. Dis. 8, 195–215. doi:10.3233/JPD-181331
Ballard, C. G., Jacoby, R., Del Ser, T., Khan, M. N., Munoz, D. G., Holmes, C., et al. (2004). Neuropathological substrates of psychiatric symptoms in prospectively studied patients with autopsy-confirmed dementia with Lewy bodies. Am. J. Psychiatry 161, 843–849. doi:10.1176/appi.ajp.161.5.843
Barnes, J., Anderson, L. A., Phillipson, J. D. J. J., and St John's wort (Hypericum perforatum, L. (2001). St john's wort (Hypericum perforatum L.): A review of its chemistry, pharmacology and clinical properties. J. Pharm. Pharmacol. 53, 583–600. doi:10.1211/0022357011775910
Benfica, J., Miranda, J. S., Morais, E. S., Freire, M. G., Coutinho, J. A., and de Cássia Superbi de Sousa, R. (2020). Enhanced extraction of levodopa from Mucuna pruriens seeds using aqueous solutions of eutectic solvents. ACS Sustain. Chem. Eng. 8, 6682–6689. doi:10.1021/acssuschemeng.0c00196
Berry, C., La Vecchia, C., and Nicotera, P. (2010). Paraquat and Parkinson's disease. Cell Death Differ. 17, 1115–1125. doi:10.1038/cdd.2009.217
Berwick, D. C., and Harvey, K. J. B. S. T. (2012). The importance of Wnt signalling for neurodegeneration in Parkinson's disease. Biochem. Soc. Trans. 40, 1123–1128. doi:10.1042/BST20120122
Bharate, S. B., Sawant, S. D., Singh, P. P., and Vishwakarma, R. A. J. C. r. (2013). Kinase inhibitors of marine origin. Chem. Rev. 113, 6761–6815. doi:10.1021/cr300410v
Bhattacharya, T., Chopra, H., Rahman, M., Hasan, Z., Swain, S. S., Cavalu, S. J. M., et al. (2022). Applications of phyto-nanotechnology for the treatment of neurodegenerative disorders. Materials 15, 804. doi:10.3390/ma15030804
Boer, M. C., Joosten, S. A., and Ottenhoff, T. H. (2015). Regulatory T-cells at the interface between human host and pathogens in infectious diseases and vaccination. Front. Immunol. 6, 217. doi:10.3389/fimmu.2015.00217
Braga-Neto, P., da Silva-Júnior, F. P., Monte, F. S., de Bruin, P. F., and de Bruin, V. M. J. J. (2004). Snoring and excessive daytime sleepiness in Parkinson's disease. J. Neurol. Sci. 217, 41–45.
Brinkhaus, B., Lindner, M., Schuppan, D., and Hahn, E. J. P. (2000). Chemical, pharmacological and clinical profile of the East Asian medical plant Centella aslatica. Phytomedicine 7, 427–448. doi:10.1016/s0944-7113(00)80065-3
Brown, J. H., and Taylor, P. (2006). “Muscarinic receptor agonists and antagonists,” in Goodman & gilman's pharmacological basis of therapeutics (New York: McGraw-Hill), 183–200.
Burn, D. J. (2002). Beyond the iron mask: Towards better recognition and treatment of depression associated with Parkinson's disease. Mov. Disord. 17, 445–454. doi:10.1002/mds.10114
Burns, R. S., Chiueh, C. C., Markey, S. P., Ebert, M. H., Jacobowitz, D. M., and Kopin, I. J. J. P. (1983). A primate model of parkinsonism: Selective destruction of dopaminergic neurons in the pars compacta of the substantia nigra by N-methyl-4-phenyl-1, 2, 3, 6-tetrahydropyridine. Proc. Natl. Acad. Sci. U. S. A. 80, 4546–4550. doi:10.1073/pnas.80.14.4546
Cassotta, M., Geerts, H., Harbom, L., Outeiro, T. F., Pediaditakis, I., Reiner, O., et al. (2022). The future of Parkinson’s disease research: A new paradigm of human-specific investigation is necessary and possible. ALTEX-Alternatives animal Exp.
Chalorak, P., Jattujan, P., Nobsathian, S., Poomtong, T., Sobhon, P., and Meemon, K. J. N. N. (2018). Holothuria scabra extracts exhibit anti-Parkinson potential in C. elegans: A model for anti-Parkinson testing. Nutr. Neurosci. 21, 427–438. doi:10.1080/1028415X.2017.1299437
Chen, H.-Q., Jin, Z.-Y., Wang, X.-J., Xu, X.-M., Deng, L., and Zhao, J.-W. J. N. L. (2008). Luteolin protects dopaminergic neurons from inflammation-induced injury through inhibition of microglial activation. Neurosci. Lett. 448, 175–179. doi:10.1016/j.neulet.2008.10.046
Chen, X.-C., Chen, Y., Zhu, Y.-G., Fang, F., and Chen, L.-M. J. A. P. S. (2002). Protective effect of ginsenoside Rg1 against MPTP-induced apoptosis in mouse substantia nigra neurons. Acta Pharmacol. Sin. 23, 829–834.
Cheng, Y.-F., Zhu, G.-Q., Wang, M., Cheng, H., Zhou, A., Wang, N., et al. (2009). Involvement of ubiquitin proteasome system in protective mechanisms of Puerarin to MPP+-elicited apoptosis. Neurosci. Res. 63, 52–58. doi:10.1016/j.neures.2008.10.009
Cheng, Y., He, G., Mu, X., Zhang, T., Li, X., Hu, J., et al. (2008). Neuroprotective effect of baicalein against MPTP neurotoxicity: Behavioral, biochemical and immunohistochemical profile. Neurosci. Lett. 441, 16–20. doi:10.1016/j.neulet.2008.05.116
Chia, S. J., Tan, E.-K., and Chao, Y.-X. (2020). Historical perspective: Models of Parkinson’s disease. Int. J. Mol. Sci. 21, 2464. doi:10.3390/ijms21072464
Choi, J. G., Park, G., Kim, H. G., Oh, D.-S., Kim, H., and Oh, M. S. J. I. J. M. S. (2016). In vitro and in vivo neuroprotective effects of walnut (Juglandis semen) in models of Parkinson’s disease. Int. J. Mol. Sci. 17, 108. doi:10.3390/ijms17010108
Clarke, C. E., Patel, S., Ives, N., Rick, C. E., Dowling, F., Woolley, R., et al. (2016). Physiotherapy and occupational therapy vs no therapy in mild to moderate Parkinson disease: A randomized clinical trial. JAMA Neurol. 73, 291–299. doi:10.1001/jamaneurol.2015.4452
Collerton, D., Burn, D., McKeith, I., O’Brien, J. J. D., and disorders, G. C. (2003). Systematic review and meta-analysis show that dementia with Lewy bodies is a visual-perceptual and attentional-executive dementia. Dement. Geriatr. Cogn. Disord. 16, 229–237. doi:10.1159/000072807
Comella, C. L., Morrissey, M., and Janko, K. J. N. (2005). Nocturnal activity with nighttime pergolide in Parkinson disease: A controlled study using actigraphy. Neurology 64, 1450–1451. doi:10.1212/01.WNL.0000158652.74601.48
Corona, J. C. J. B. (2018). Natural compounds for the management of Parkinson’s disease and attention-deficit/hyperactivity disorder. Biomed. Res. Int. 2018. doi:10.1155/2018/4067597
Corrêa, S. A., and Eales, K. L. J. J. o. s. t. (2012). The role of p38 MAPK and its substrates in neuronal plasticity and neurodegenerative disease. J. Signal Transduct. 2012.
da Fonseca, A. C. C., Matias, D., Garcia, C., Amaral, R., Geraldo, L. H., Freitas, C., et al. (2014). The impact of microglial activation on blood-brain barrier in brain diseases. Front. Cell. Neurosci. 8, 362. doi:10.3389/fncel.2014.00362
DeFeudis, F., and Drieu, K. J. C. (2000). Ginkgo biloba extract (EGb 761) and CNS functions basic studies and clinical applications. Curr. Drug Targets 1, 25–58. doi:10.2174/1389450003349380
Dexter, D. T., Jenner, P., Schapira, A. H., Marsden, C. D., and Society, C. N. (1992). Alterations in levels of iron, ferritin, and other trace metals in neurodegenerative diseases affecting the basal ganglia. The Royal Kings and Queens Parkinson's Disease Research Group. Ann. Neurol. 32, S94–S100. doi:10.1002/ana.410320716
Dhawan, K., Dhawan, S., and Sharma, A. J. J. (2004). Passiflora: A review update. J. Ethnopharmacol. 94, 1–23. doi:10.1016/j.jep.2004.02.023
Diaz, N. L., and Waters, C. H. J. (2009). Current strategies in the treatment of Parkinson’s disease and a personalized approach to management. Expert Rev. Neurother. 9, 1781–1789. doi:10.1586/ern.09.117
Doke, R. R., Pansare, P. A., Sainani, S. R., Bhalchim, V. M., Rode, K. R., and Desai, S. R. (2019). Natural products: An emerging tool in Parkinson’s disease.
Dorsey, E. a., Constantinescu, R., Thompson, J., Biglan, K., Holloway, R., Kieburtz, K., et al. (2007). Projected number of people with Parkinson disease in the most populous nations, 2005 through 2030. Neurology 68, 384–386. doi:10.1212/01.wnl.0000247740.47667.03
Duka, T., Duka, V., Joyce, J. N., and Sidhu, A. J. T. F. J., "Alpha-Synuclein contributes to GSK-3beta-catalyzed Tau phosphorylation in Parkinson's disease models., " vol. 23, pp. 2820–2830. doi:10.1096/fj.08-1204102009
Eisensehr, I., Linke, R., Tatsch, K., Kharraz, B., Gildehaus, J. F., Wetter, C. T., et al. (2003). Increased muscle activity during rapid eye movement sleep correlates with decrease of striatal presynaptic dopamine transporters. IPT and IBZM SPECT imaging in subclinical and clinically manifest idiopathic REM sleep behavior disorder, Parkinson's disease, and controls. Sleep 26, 507–512. doi:10.1093/sleep/26.5.507
Elbaz, A., Bower, J. H., Maraganore, D. M., McDonnell, S. K., Peterson, B. J., Ahlskog, J. E., et al. (2002). Risk tables for parkinsonism and Parkinson's disease. J. Clin. Epidemiol. 55, 25–31. doi:10.1016/s0895-4356(01)00425-5
Eldeeb, M. A., Thomas, R. A., Ragheb, M. A., Fallahi, A., and Fon, E. A. (2022). Mitochondrial quality control in health and in Parkinson's disease. Physiological Reviews.
Empadinhas, N., and da Costa, M. S. J. E. M. (2011). Diversity, biological roles and biosynthetic pathways for sugar‐glycerate containing compatible solutes in bacteria and archaea. Environ. Microbiol. 13, 2056–2077. doi:10.1111/j.1462-2920.2010.02390.x
Essa, M. M., Subash, S., Dhanalakshmi, C., Manivasagam, T., Al-Adawi, S., Guillemin, G. J., et al. (2015). Dietary supplementation of walnut partially reverses 1-methyl-4-phenyl-1, 2, 3, 6-tetrahydropyridine induced neurodegeneration in a mouse model of Parkinson’s disease. Neurochem. Res. 40, 1283–1293. doi:10.1007/s11064-015-1593-2
Factor, S. A., McAlarney, T., Sanchez‐Ramos, J. R., and Weiner, W. J. (1990). Sleep disorders and sleep effect in Parkinson's disease. Mov. Disord. 5, 280–285. doi:10.1002/mds.870050404
Faria, C., Jorge, C. D., Borges, N., Tenreiro, S., Outeiro, T. F., and Santos, H. J. B. B. B. A.-G. S. (2013). Inhibition of formation of α-synuclein inclusions by mannosylglycerate in a yeast model of Parkinson's disease. Biochim. Biophys. Acta 1830, 4065–4072. doi:10.1016/j.bbagen.2013.04.015
Fei, C., Shenggang, S. J. J. H. U. S., and E-tang, T. (2003). Experimental study on inhibition of neuronal toxical effect of levodopa by ginkgo biloba extract on Parkinson disease in rats. Univ. Sci. Technol. Med. Sci. 23, 151–153. doi:10.1007/BF02859941
Feigin, V. L., Roth, G. A., Naghavi, M., Parmar, P., Krishnamurthi, R., Chugh, S., et al. (2016). Global burden of stroke and risk factors in 188 countries, during 1990–2013: A systematic analysis for the global burden of disease study 2013. Lancet. Neurol. 15, 913–924. doi:10.1016/S1474-4422(16)30073-4
Ferini-Strambi, L., Franceschi, M., Pinto, P., Zucconi, M., and Smirne, S. J. G. (1992). Respiration and heart rate variability during sleep in untreated Parkinson patients. Gerontology 38, 92–98. doi:10.1159/000213312
Frémont, L. J. (2000). Biological effects of resveratrol. Life Sci. 66, 663–673. doi:10.1016/s0024-3205(99)00410-5
Friedmann, T., and Roblin, R. J. S. (1972). Gene therapy for human genetic disease? Science 175, 949–955. doi:10.1126/science.175.4025.949
Gagnon, J.-F., Bédard, M.-A., Fantini, M., Petit, D., Panisset, M., Rompre, S., et al. (2002). REM sleep behavior disorder and REM sleep without atonia in Parkinson’s disease. Neurology 59, 585–589. doi:10.1212/wnl.59.4.585
Galasso, C., Corinaldesi, C., and Sansone, C. J. A. (2017). Carotenoids from marine organisms: Biological functions and industrial applications. Antioxidants 6, 96. doi:10.3390/antiox6040096
Gammone, M. A., Riccioni, G., and D'Orazio, N. J. M. D. (2015). Marine carotenoids against oxidative stress: Effects on human health. Mar. Drugs 13, 6226–6246. doi:10.3390/md13106226
Gattellaro, G., Minati, L., Grisoli, M., Mariani, C., Carella, F., Osio, M., et al. (2009). White matter involvement in idiopathic Parkinson disease: A diffusion tensor imaging study. AJNR. Am. J. Neuroradiol. 30, 1222–1226. doi:10.3174/ajnr.A1556
Gjerstad, M., Aarsland, D., and Larsen, J. J. N. (2002). Development of daytime somnolence over time in Parkinson’s disease. Neurology 58, 1544–1546. doi:10.1212/wnl.58.10.1544
Global, R. (2018). National incidence, prevalence, and years lived with disability for 354 diseases and injuries for 195 countries and territories, 1990–2017: A systematic analysis for the global burden of disease study 2017. Lancet 392, 1789–1858.
Golpich, M., Amini, E., Hemmati, F., Ibrahim, N. M., Rahmani, B., Mohamed, Z., et al. (2015). Glycogen synthase kinase-3 beta (GSK-3β) signaling: Implications for Parkinson's disease. Pharmacol. Res. 97, 16–26. doi:10.1016/j.phrs.2015.03.010
Grealish, S., Diguet, E., Kirkeby, A., Mattsson, B., Heuer, A., Bramoulle, Y., et al. (2014). Human ESC-derived dopamine neurons show similar preclinical efficacy and potency to fetal neurons when grafted in a rat model of Parkinson’s disease. Cell Stem Cell 15, 653–665. doi:10.1016/j.stem.2014.09.017
Grimmig, B., Daly, L., Hudson, C., Nash, K., and Bickford, P. J. F. F. H. (2017). Astaxanthin attenuates neurotoxicity in a mouse model of Parkinson’s disease. Funct. Foods Health Dis. 7, 562–576. doi:10.31989/ffhd.v7i8.352
Grimmig, B., Kim, S.-H., Nash, K., Bickford, P. C., and Douglas Shytle, R. J. G. (2017). Neuroprotective mechanisms of astaxanthin: A potential therapeutic role in preserving cognitive function in age and neurodegeneration. Geroscience 39, 19–32. doi:10.1007/s11357-017-9958-x
Gu, P. S., Moon, M., Choi, J. G., and Oh, M. S. J. T. J. (2017). Mulberry fruit ameliorates Parkinson's-disease-related pathology by reducing α-synuclein and ubiquitin levels in a 1-methyl-4-phenyl-1, 2, 3, 6-tetrahydropyridine/probenecid model. J. Nutr. Biochem. 39, 15–21. doi:10.1016/j.jnutbio.2016.08.014
Gu, Q., Huang, P., Xuan, M., Xu, X., Li, D., Sun, J., et al. (2014). Greater loss of white matter integrity in postural instability and gait difficulty subtype of Parkinson's disease. Can. J. Neurol. Sci. 41, 763–768. doi:10.1017/cjn.2014.34
Gualtieri, C. T. J. J. I. M. (2004). Dementia screening in light of the diversity of the condition. J. Insur. Med. 36, 298–309.
Gupta, S. C., Patchva, S., Koh, W., and Aggarwal, B. B. J. C. (2012). Discovery of curcumin, a component of golden spice, and its miraculous biological activities. Clin. Exp. Pharmacol. Physiol. 39, 283–299. doi:10.1111/j.1440-1681.2011.05648.x
Hacker, C. D., Perlmutter, J. S., Criswell, S. R., Ances, B. M., and Snyder, A. Z. J. B. (2012). Resting state functional connectivity of the striatum in Parkinson’s disease. Brain 135, 3699–3711. doi:10.1093/brain/aws281
Happe, S., Schrödl, B., Faltl, M., Müller, C., Auff, E., and Zeitlhofer, J. J. A. N. S. (2001). Sleep disorders and depression in patients with Parkinson's disease: Results of a study with the sleep disorders questionnaire (SDQ) and the zung depression scale (ZDS). Acta Neurol. Scand. 104, 275–280. doi:10.1034/j.1600-0404.2001.00024.x
Hara, H., Onodera, H., Yoshidomi, M., Matsuda, Y., and Kogure, K. J. J. o. C. B. F. (1990). Staurosporine, a novel protein kinase C inhibitor, prevents postischemic neuronal damage in the gerbil and rat. J. Cereb. Blood Flow. Metab. 10, 646–653. doi:10.1038/jcbfm.1990.117
Hauser, R. A., Gauger, L., Anderson, W. M., and Zesiewicz, T. A. J. (2000). Pramipexole-induced somnolence and episodes of daytime sleep. Mov. Disord. 15, 658–663. doi:10.1002/1531-8257(200007)15:4<658::aid-mds1009>3.0.co;2-n
Hindle, J. V. J. A., and Ageing, (2010). Ageing, neurodegeneration and Parkinson's disease. Age Ageing 39, 156–161. doi:10.1093/ageing/afp223
Hobson, D. E., Lang, A. E., Martin, W. W., Razmy, A., Rivest, J., and Fleming, J. J. J., "Excessive daytime sleepiness and sudden-onset sleep in Parkinson disease: A survey by the Canadian movement disorders group, " vol. 287, pp. 455–463. doi:10.1001/jama.287.4.4552002
Hosamani, R., Krishna, G., and Muralidhara, G. (2016). Standardized Bacopa monnieri extract ameliorates acute paraquat-induced oxidative stress, and neurotoxicity in prepubertal mice brain. Nutr. Neurosci. 19, 434–446. doi:10.1179/1476830514Y.0000000149
Hosamani, R. (2009). Neuroprotective efficacy of Bacopa monnieri against rotenone induced oxidative stress and neurotoxicity in Drosophila melanogaster. Neurotoxicology 30, 977–985. doi:10.1016/j.neuro.2009.08.012
Hunot, S., Vila, M., Teismann, P., Davis, R. J., Hirsch, E. C., Przedborski, S., et al. (2004). JNK-mediated induction of cyclooxygenase 2 is required for neurodegeneration in a mouse model of Parkinson's disease. Proc. Natl. Acad. Sci. U. S. A. 101, 665–670. doi:10.1073/pnas.0307453101
Ikram, S., Parra-Payano, V. D., Kammaripalle, T. K. C., Datta, A., Kaur, M., Bhushan, T., et al. (2021). Progressive supranuclear palsy: A case report and literature review. Arch. Clin. Med. Case Rep. 5, 838–845. doi:10.26502/acmcr.96550428
Im, H.-I., Joo, W. S., Nam, E., Lee, E.-S., Hwang, Y.-j., and Kim, Y. S. J. J. (2005). Baicalein prevents 6-hydroxydopamine-induced dopaminergic dysfunction and lipid peroxidation in mice. J. Pharmacol. Sci. 98, 185–189. doi:10.1254/jphs.sc0050014
Ingale, S. P., and Kasture, S. B. J. A. S. (2017). Protective effect of standardized extract of Passiflora incarnata flower in Parkinson’s and Alzheimer’s disease. Anc. Sci. Life 36, 200. doi:10.4103/asl.ASL_231_16
Iranzo, A., Santamaria, J., Rye, D., Valldeoriola, F., Martí, M., Muñoz, E., et al. (2005). Characteristics of idiopathic REM sleep behavior disorder and that associated with MSA and PD. Neurology 65, 247–252. doi:10.1212/01.wnl.0000168864.97813.e0
Isozaki, E., Naito, A., Horiguchi, S., Kawamura, R., Hayashida, T., Tanabe, H. J. J. N., et al. (1996). Early diagnosis and stage classification of vocal cord abductor paralysis in patients with multiple system atrophy. J. Neurol. Neurosurg. Psychiatry 60, 399–402. doi:10.1136/jnnp.60.4.399
Ito, H., Kusaka, H., Matsumoto, S., and Imai, T. J. N. (1996). Striatal efferent involvement and its correlation to levodopa efficacy in patients with multiple system atrophy. Neurology 47, 1291–1299. doi:10.1212/wnl.47.5.1291
Jansen, E., and Meerwaldtt, J. J. A. (1990). Madopar HBS in nocturnal symptoms of Parkinson's disease. Adv. Neurol. 53, 527–531.
Jenner, P. J. P. (2003). The contribution of the MPTP-treated primate model to the development of new treatment strategies for Parkinson's disease. Park. Relat. Disord. 9, 131–137. doi:10.1016/s1353-8020(02)00115-3
Jenner, P., Rupniak, N. M., Rose, S., Kelly, E., Kilpatrick, G., Lees, A., et al. (1984). 1-Methyl-4-phenyl-1, 2, 3, 6-tetrahydropyridine-induced parkinsonism in the common marmoset. Neurosci. Lett. 50, 85–90. doi:10.1016/0304-3940(84)90467-1
Jia, H., Jiang, Y., Ruan, Y., Zhang, Y., Ma, X., Zhang, J., et al. (2004). Tenuigenin treatment decreases secretion of the Alzheimer’s disease amyloid β-protein in cultured cells. Neurosci. Lett. 367, 123–128. doi:10.1016/j.neulet.2004.05.093
Jiang, W., Wang, Z., Jiang, Y., Lu, M., and Li, X. J. P. (2015). Ginsenoside Rg1 ameliorates motor function in an animal model of Parkinson's disease. Pharmacology 96, 25–31. doi:10.1159/000431100
Jin, F., Wu, Q., Lu, Y.-F., Gong, Q.-H., and Shi, J.-S. J. E. (2008). Neuroprotective effect of resveratrol on 6-OHDA-induced Parkinson's disease in rats. Eur. J. Pharmacol. 600, 78–82. doi:10.1016/j.ejphar.2008.10.005
Jing, X., Wei, X., Ren, M., Wang, L., Zhang, X., and Lou, H. J. N. r. (2016). Neuroprotective effects of tanshinone I against 6-OHDA-induced oxidative stress in cellular and mouse model of Parkinson’s disease through upregulating Nrf2. Neurochem. Res. 41, 779–786. doi:10.1007/s11064-015-1751-6
Juncos, J., Fabbrini, G., Mouradian, M., and Chase, T. J. A. (1987). Controlled release levodopa-carbidopa (CR-5) in the management of parkinsonian motor fluctuations. Arch. Neurol. 44, 1010–1012. doi:10.1001/archneur.1987.00520220016008
Kajimura, Y., Aoki, T., Kuramochi, K., Kobayashi, S., Sugawara, F., Watanabe, N., et al. (2008). Neoechinulin A protects PC12 cells against MPP+-induced cytotoxicity. J. Antibiot. 61, 330–333. doi:10.1038/ja.2008.48
Kales, A., Ansel, R. D., Markham, C. H., Scharf, M. B., and Tan, T.-L. J. C. P. (1971). Sleep in patients with Parkinson's disease and normal subiects prior to and following levodopa administration. Clin. Pharmacol. Ther. 12, 397–406. doi:10.1002/cpt1971122part2397
Karakida, F., Ikeya, Y., Tsunakawa, M., Yamaguchi, T., Ikarashi, Y., Takeda, S., et al. (2007). Cerebral protective and cognition-improving effects of sinapic acid in rodents. Biol. Pharm. Bull. 30, 514–519. doi:10.1248/bpb.30.514
Karaman, M. W., Herrgard, S., Treiber, D. K., Gallant, P., Atteridge, C. E., Campbell, B. T., et al. (2008). A quantitative analysis of kinase inhibitor selectivity. Nat. Biotechnol. 26, 127–132. doi:10.1038/nbt1358
Kiasalari, Z., Baluchnejadmojarad, T., Roghani, M. J. C., and Neurobiology, M. (2016). Hypericum perforatum hydroalcoholic extract mitigates motor dysfunction and is neuroprotective in intrastriatal 6-Hydroxydopamine rat model of Parkinson’s disease. Cell. Mol. Neurobiol. 36, 521–530. doi:10.1007/s10571-015-0230-6
Kim, D. S., Park, S.-Y., and Kim, J.-Y. J. N. (2001). Curcuminoids from Curcuma longa L. (Zingiberaceae) that protect PC12 rat pheochromocytoma and normal human umbilical vein endothelial cells from betaA(1-42) insult. Neurosci. Lett. 303, 57–61. doi:10.1016/s0304-3940(01)01677-9
Kimoto, K., Aoki, T., Shibata, Y., Kamisuki, S., Sugawara, F., Kuramochi, K., et al. (2007). Structure-activity relationships of neoechinulin A analogues with cytoprotection against peroxynitrite-induced PC12 cell death. J. Antibiot. 60, 614–621. doi:10.1038/ja.2007.79
Kirkeby, A., Grealish, S., Wolf, D. A., Nelander, J., Wood, J., Lundblad, M., et al. (2012). Generation of regionally specified neural progenitors and functional neurons from human embryonic stem cells under defined conditions. Cell Rep. 1, 703–714. doi:10.1016/j.celrep.2012.04.009
Kiyama, R. J. E. J. (2017). Estrogenic terpenes and terpenoids: Pathways, functions and applications. Eur. J. Pharmacol. 815, 405–415. doi:10.1016/j.ejphar.2017.09.049
Klein, C., and Westenberger, A. (2012). Genetics of Parkinson’s disease. Cold Spring Harb. Perspect. Med. 2, a008888. doi:10.1101/cshperspect.a008888
Koppula, S., Kumar, H., More, S. V., Kim, B. W., Kim, I. S., and Choi, D.-K. J. I. (2012). Recent advances on the neuroprotective potential of antioxidants in experimental models of Parkinson’s disease. Int. J. Mol. Sci. 13, 10608–10629. doi:10.3390/ijms130810608
Kosaraju, J., Chinni, S., Roy, P. D., Kannan, E., Antony, A. S., and Kumar, M. S. (2014). Neuroprotective effect of Tinospora cordifolia ethanol extract on 6-hydroxy dopamine induced Parkinsonism. Indian J. Pharmacol. 46, 176–180. doi:10.4103/0253-7613.129312
Kowal, S. L., Dall, T. M., Chakrabarti, R., Storm, M. V., and Jain, A. (2013). The current and projected economic burden of Parkinson's disease in the United States. Mov. Disord. 28, 311–318. doi:10.1002/mds.25292
Kriks, S., Shim, J.-W., Piao, J., Ganat, Y. M., Wakeman, D. R., Xie, Z., et al. (2011). Dopamine neurons derived from human ES cells efficiently engraft in animal models of Parkinson’s disease. Nature 480, 547–551. doi:10.1038/nature10648
Kuan, C.-Y., Burke, R. E. J. C. D. T.-C., and Disorders, N. (2005). Targeting the JNK signaling pathway for stroke and Parkinson's diseases therapy. Curr. Drug Targets. CNS Neurol. Disord. 4, 63–67. doi:10.2174/1568007053005145
Kubo, S. i., Kitami, T., Noda, S., Shimura, H., Uchiyama, Y., Asakawa, S., et al. (2001). Parkin is associated with cellular vesicles. J. Neurochem. 78, 42–54. doi:10.1046/j.1471-4159.2001.00364.x
Kumar, S., Behl, T., Sehgal, A., Chigurupati, S., Singh, S., Mani, V., et al. (2022). Exploring the focal role of LRRK2 kinase in Parkinson’s disease. Environmental Science and Pollution Research, 1–15.
Kuribara, H., Kishi, E., Hattori, N., Okada, M., and Maruyama, Y. J. J., (2000). The anxiolytic effect of two oriental herbal drugs in Japan attributed to honokiol from magnolia bark. J. Pharm. Pharmacol. 52, 1425–1429. doi:10.1211/0022357001777432
Kurobane, I., Iwahashi, S., Fukuda, A. J. D., research, c., and FukudA, A. (1987). Cytostatic activity of naturally isolated isomers of secalonic acids and their chemically rearranged dimers. Drugs Exp. Clin. Res. 13, 339–344.
Lang, A. E., Gill, S., Patel, N. K., Lozano, A., Nutt, J. G., Penn, R., et al. (2006). Randomized controlled trial of intraputamenal glial cell line–derived neurotrophic factor infusion in Parkinson disease. Ann. Neurol. 59, 459–466. doi:10.1002/ana.20737
Langston, J. W., Ballard, P., Tetrud, J. W., and Irwin, I. J. S. (1983). Chronic Parkinsonism in humans due to a product of meperidine-analog synthesis. Science 219, 979–980. doi:10.1126/science.6823561
Larsen, J. P. J. (2003). Sleep disorders in Parkinson's disease. J. Park. Dis. 91, 329–334. doi:10.3233/JPD-130301
Lees, A. J., Blackburn, N. A., and Campbell, V. L. J. C. (1988). The nighttime problems of Parkinson's disease. Clin. Neuropharmacol. 11, 512–519. doi:10.1097/00002826-198812000-00004
Lees, A. J. J. (2012). Impact commentaries. A modern perspective on the top 100 cited jnnp papers of all time: The relevance of the lewy body to the pathogenesis of idiopathic Parkinson's disease: Accuracy of clinical diagnosis of idiopathic Parkinson's disease. J. Neurol. Neurosurg. Psychiatry 83, 954–955. doi:10.1136/jnnp-2012-302969
Lei, H., Ren, R., Sun, Y., Zhang, K., Zhao, X., Ablat, N., et al. (2020). Neuroprotective effects of safflower flavonoid extract in 6-hydroxydopamine-induced model of Parkinson’s disease may be related to its anti-inflammatory action. Molecules 25, 5206. doi:10.3390/molecules25215206
Lesser, R. P., Fahn, S., Snider, S. R., Cote, L. J., Isgreen, W. P., and Barrett, R. E. J. N. (1979). Analysis of the clinical problems in parkinsonism and the complications of long‐term levodopa therapy. Neurology 29, 1253–1260. doi:10.1212/wnl.29.9_part_1.1253
Li, D., Wilkins, K., McCollum, A. M., Osadebe, L., Kabamba, J., Nguete, B., et al. (2017). Evaluation of the GeneXpert for human monkeypox diagnosis. Am. J. Trop. Med. Hyg. 96, 405–410. doi:10.4269/ajtmh.16-0567
Li, J., Long, X., Hu, J., Bi, J., Zhou, T., Guo, X., et al. (2019). Multiple pathways for natural product treatment of Parkinson's disease: A mini review. Phytomedicine 60, 152954. doi:10.1016/j.phymed.2019.152954
Li, X.-x., He, G.-r., Mu, X., Xu, B., Tian, S., Yu, X., et al. (2012). Protective effects of baicalein against rotenone-induced neurotoxicity in PC12 cells and isolated rat brain mitochondria. Eur. J. Pharmacol. 674, 227–233. doi:10.1016/j.ejphar.2011.09.181
Li, Y.-Y., Lu, J.-H., Li, Q., Zhao, Y.-Y., and Pu, X.-P. J. E. (2008). Pedicularioside A from Buddleia lindleyana inhibits cell death induced by 1-methyl-4-phenylpyridinium ions (MPP+) in primary cultures of rat mesencephalic neurons. Eur. J. Pharmacol. 579, 134–140. doi:10.1016/j.ejphar.2007.10.052
Li, Y., Li, X., Kang, J. S., Choi, H. D., and Son, B. W. J. T. J. A. (2004). New radical scavenging and ultraviolet-A protecting prenylated dioxopiperazine alkaloid related to isoechinulin A from a marine isolate of the fungus Aspergillus. J. Antibiot. 57, 337–340. doi:10.7164/antibiotics.57.337
Lin, J., Yu, J., Zhao, J., Zhang, K., Zheng, J., Wang, J., et al. (2017). Fucoxanthin, a marine carotenoid, attenuates β-amyloid oligomer-induced neurotoxicity possibly via regulating the PI3K/akt and the ERK pathways in SH-SY5Y cells. Oxid. Med. Cell. Longev. 2017, 6792543. doi:10.1155/2017/6792543
Lindvall, O., Barker, R. A., Brüstle, O., Isacson, O., and Svendsen, C. N. J. (2012). Clinical translation of stem cells in neurodegenerative disorders. Cell Stem Cell 10, 151–155. doi:10.1016/j.stem.2012.01.009
Lindvall, O. J. (2016). Clinical translation of stem cell transplantation in Parkinson's disease. J. Intern. Med. 279, 30–40. doi:10.1111/joim.12415
Litvan, I., Agid, Y., Calne, D., Campbell, G., Dubois, B., Duvoisin, R., et al. (1996). Clinical research criteria for the diagnosis of progressive supranuclear palsy (Steele-Richardson-Olszewski syndrome): Report of the NINDS-SPSP international workshop. Neurology 47, 1–9. doi:10.1212/wnl.47.1.1
Litvan, I., Agid, Y., Jankovic, J., Goetz, C., Brandel, J., Lai, E., et al. (1996). Accuracy of clinical criteria for the diagnosis of progressive supranuclear palsy (Steele-Richardson-Olszewski syndrome). Neurology 46, 922–930. doi:10.1212/wnl.46.4.922
Litvan, I., Bhatia, K. P., Burn, D. J., Goetz, C. G., Lang, A. E., McKeith, I., et al. (2003). Movement disorders society scientific issues committee report: SIC task force appraisal of clinical diagnostic criteria for parkinsonian disorders. Mov. Disord. 18, 467–486. doi:10.1002/mds.10459
Litvan, I., Booth, V., Wenning, G., Bartko, J., Goetz, C., McKee, A., et al. (1998). Retrospective application of a set of clinical diagnostic criteria for the diagnosis of multiple system atrophy. J. Neural Transm. 105, 217–227. doi:10.1007/s007020050050
Litvan, I., Goetz, C. G., Jankovic, J., Wenning, G. K., Booth, V., Bartko, J. J., et al. (1997). What is the accuracy of the clinical diagnosis of multiple system atrophy? Arch. Neurol. 54, 937–944. doi:10.1001/archneur.1997.00550200007003
Liu, J., Dumontet, V., Simonin, A.-L., Iorga, B. I., Guerineau, V., Litaudon, M., et al. (2011). Benzofurans from Styrax agrestis as acetylcholinesterase inhibitors: Structure–activity relationships and molecular modeling studies. J. Nat. Prod. 74, 2081–2088. doi:10.1021/np200308j
Liu, Y., Gao, J., Peng, M., Meng, H., Ma, H., Cai, P., et al. (2018). A review on central nervous system effects of gastrodin. Front. Pharmacol. 9, 24. doi:10.3389/fphar.2018.00024
Lopez, O. L., Hamilton, R., Becker, J., Wisniewski, S., Kaufer, D., and DeKosky, S. J. N. (2000). Severity of cognitive impairment and the clinical diagnosis of AD with Lewy bodies. Neurology 54, 1780–1787. doi:10.1212/wnl.54.9.1780
LopezReal, A., Rey, P., SotoOtero, R., MendezAlvarez, E., LabandeiraGarcia, J. J. J., and Lopez-ReAl, A. (2005). Angiotensin‐converting enzyme inhibition reduces oxidative stress and protects dopaminergic neurons in a 6‐hydroxydopamine rat model of Parkinsonism. J. Neurosci. Res. 81, 865–873. doi:10.1002/jnr.20598
Lu, J. H., Ardah, M. T., Durairajan, S. S. K., Liu, L. F., Xie, L. X., Fong, W. F. D., et al. (2011). Baicalein inhibits formation of α‐synuclein oligomers within living cells and prevents aβ peptide fibrillation and oligomerisation. Chembiochem 12, 615–624. doi:10.1002/cbic.201000604
Lu, K.-T., Ko, M.-C., Chen, B.-Y., Huang, J.-C., Hsieh, C.-W., Lee, M.-C., et al. (2008). Neuroprotective effects of resveratrol on MPTP-induced neuron loss mediated by free radical scavenging. J. Agric. Food Chem. 56, 6910–6913. doi:10.1021/jf8007212
Luo, C., Song, W., Chen, Q., Zheng, Z., Chen, K., Cao, B., et al. (2014). Reduced functional connectivity in early-stage drug-naive Parkinson's disease: A resting-state fMRI study. Neurobiol. Aging 35, 431–441. doi:10.1016/j.neurobiolaging.2013.08.018
Lv, Y.-Q., Yuan, L., Sun, Y., Dou, H.-W., Su, J.-H., Hou, Z.-P., et al. (2022). Long-term hyperglycemia aggravates α-synuclein aggregation and dopaminergic neuronal loss in a Parkinson’s disease mouse model. Transl. Neurodegener. 11, 14–16. doi:10.1186/s40035-022-00288-z
Mann, V., Cooper, J., Daniel, S., Srai, K., Jenner, P., Marsden, C., et al. (1994). Complex I, iron, and ferritin in Parkinson's disease substantia nigra. Ann. Neurol. 36, 876–881. doi:10.1002/ana.410360612
Maria, B., Sophia, S., Michalis, M., Charalampos, L., Andreas, P., John, M. E., et al. (2003). Sleep breathing disorders in patients with idiopathic Parkinson's disease. Respir. Med. 97, 1151–1157. doi:10.1016/s0954-6111(03)00188-4
Maurer, K., Ihl, R., Dierks, T., and Frölich, L. J. J. (1997). Clinical efficacy of Ginkgo biloba special extract EGb 761 in dementia of the Alzheimer type. J. Psychiatr. Res. 31, 645–655. doi:10.1016/s0022-3956(97)00022-8
McGeer, P. L., Itagaki, S., Akiyama, H., McGeer, E., and Society, (1988). Rate of cell death in parkinsonism indicates active neuropathological process. Ann. Neurol. 24, 574–576. doi:10.1002/ana.410240415
McKeith, I. G., Galasko, D., Kosaka, K., Perry, E., Dickson, D. W., Hansen, L., et al. (1996). Consensus guidelines for the clinical and pathologic diagnosis of dementia with lewy bodies (DLB): Report of the consortium on DLB international workshop. Neurology 47, 1113–1124. doi:10.1212/wnl.47.5.1113
McNaught, K. S. P., Belizaire, R., Isacson, O., Jenner, P., and Olanow, C. W. J. E. (2003). Altered proteasomal function in sporadic Parkinson's disease. Exp. Neurol. 179, 38–46. doi:10.1006/exnr.2002.8050
Meenakshi, S., Umayaparvathi, S., Arumugam, M., and Balasubramanian, T. J. A. P. T. B. (2011). In vitro antioxidant properties and FTIR analysis of two seaweeds of Gulf of Mannar. Asian Pac. J. Trop. Biomed. 1, S66–S70. doi:10.1016/S2221-1691(11)60126-3
Memarzia, A., Saadat, S., Behrouz, S., and Boskabady, M. H. (2022). Curcuma longa and curcumin affect respiratory and allergic disorders, experimental and clinical evidence: A comprehensive and updated review. Biofactors 48, 521–551. doi:10.1002/biof.1818
Mercuri, N. B., and Bernardi, G. J. (2005). The ‘magic’of L-dopa: Why is it the gold standard Parkinson's disease therapy? Trends Pharmacol. Sci. 26, 341–344. doi:10.1016/j.tips.2005.05.002
Mirahmadi, M., Rezanejadbardaji, H., Irfan-Maqsood, M., Mokhtari, M. J., and Naderi-Meshkin, H. (2016). Stem cell therapy for neurodegenerative diseases: Strategies for regeneration against degeneration. Cell Ther. Regen. Med. J. 1. doi:10.15562/ctrm.11
Mitchell, K. T., and Ostrem, J. L. J. N. C. (2020). Surgical treatment of Parkinson disease. Neurol. Clin. 38, 293–307. doi:10.1016/j.ncl.2020.01.001
Molina-Jiménez, M. a. F., Sánchez-Reus, M. a. I., Andres, D., Cascales, M. a., and Benedi, J. J. B. R. (2004). Neuroprotective effect of fraxetin and myricetin against rotenone-induced apoptosis in neuroblastoma cells. Brain Res. 1009, 9–16. doi:10.1016/j.brainres.2004.02.065
Monciardini, P., Iorio, M., Maffioli, S., Sosio, M., and Donadio, S. J. M. (2014). Discovering new bioactive molecules from microbial sources. Microb. Biotechnol. 7, 209–220. doi:10.1111/1751-7915.12123
Mössner, R., Henneberg, A., Schmitt, A., Syagailo, Y., Grässle, M., Hennig, T., et al. (2001). Allelic variation of serotonin transporter expression is associated with depression in Parkinson's disease. Mol. Psychiatry 6, 350–352. doi:10.1038/sj.mp.4000849
Mouatt-Prigent, A., Muriel, M.-P., Gu, W.-J., El Hachimi, K., Lücking, C., Brice, A., et al. (2004). Ultrastructural localization of parkin in the rat brainstem, thalamus and basal ganglia. J. Neural Transm. 111, 1209–1218. doi:10.1007/s00702-004-0144-9
Mu, X., He, G.-R., Yuan, X., Li, X.-X., Du, G.-H. J. P. B., and Behavior, (2011). Baicalein protects the brain against neuron impairments induced by MPTP in C57BL/6 mice. Pharmacol. Biochem. Behav. 98, 286–291. doi:10.1016/j.pbb.2011.01.011
Nagashayana, N., Sankarankutty, P., Nampoothiri, M., Mohan, P., and Mohanakumar, K. (2000). Association of L-DOPA with recovery following Ayurveda medication in Parkinson’s disease. J. Neurol. Sci. 176, 124–127. doi:10.1016/s0022-510x(00)00329-4
Nakaso, K., Ito, S., and Nakashima, K. J. N. (2008). Caffeine activates the PI3K/Akt pathway and prevents apoptotic cell death in a Parkinson's disease model of SH-SY5Y cells. Neurosci. Lett. 432, 146–150. doi:10.1016/j.neulet.2007.12.034
Nam, S.-J., Kauffman, C. A., Jensen, P. R., and Fenical, W. J. T. (2011). Isolation and characterization of actinoramides A-C, highly modified peptides from a marine Streptomyces sp. Tetrahedron 67, 6707–6712. doi:10.1016/j.tet.2011.04.051
Nicholatos, J. W., Francisco, A. B., Bender, C. A., Yeh, T., Lugay, F. J., Salazar, J. E., et al. (2018). Nicotine promotes neuron survival and partially protects from Parkinson’s disease by suppressing SIRT6. Acta Neuropathol. Commun. 6, 1–18. doi:10.1186/s40478-018-0625-y
Nikapitiya, C. J. A., (2012). Bioactive secondary metabolites from marine microbes for drug discovery. Adv. Food Nutr. Res. 65, 363–387. doi:10.1016/B978-0-12-416003-3.00024-X
Noda, K., Kitami, T., Gai, W. P., Chegini, F., Jensen, P. H., Fujimura, T., et al. (2005). Phosphorylated IkappaBalpha is a component of Lewy body of Parkinson's disease. Biochem. Biophys. Res. Commun. 331, 309–317. doi:10.1016/j.bbrc.2005.03.167
Noyce, A. J., Lees, A. J., and Schrag, A.-E. J. J. N. (2016). The prediagnostic phase of Parkinson's disease. J. Neurol. Neurosurg. Psychiatry 87, 871–878. doi:10.1136/jnnp-2015-311890
Obeso, J. A., Rodriguez-Oroz, M. C., Goetz, C. G., Marin, C., Kordower, J. H., Rodriguez, M., et al. (2010). Missing pieces in the Parkinson's disease puzzle. Nat. Med. 16, 653–661. doi:10.1038/nm.2165
Odin, P., Wolters, E., and Antonini, A. J. N. S. (2008). Continuous dopaminergic stimulation achieved by duodenal levodopa infusion. Neurol. Sci. 29, 387–388. doi:10.1007/s10072-008-1054-7
Ohlendorf, B., Schulz, D., Erhard, A., Nagel, K., and Imhoff, J. F. J. N. P. (2012). Geranylphenazinediol, an acetylcholinesterase inhibitor produced by a Streptomyces species. J. Nat. Prod. 75, 1400–1404. doi:10.1021/np2009626
Ojha, R. P., Rastogi, M., Devi, B. P., Agrawal, A., and Dubey, G. J. J. N. P. (2012). Neuroprotective effect of curcuminoids against inflammation-mediated dopaminergic neurodegeneration in the MPTP model of Parkinson’s disease. J. Neuroimmune Pharmacol. 7, 609–618. doi:10.1007/s11481-012-9363-2
Olanow, C., Hauser, R., Gauguster, L., Malapira, T., Koller, W., Hubble, J., et al. (1995). The effect of deprenyl and levodopa on the progression of Parkinson's disease. Ann. Neurol. 38, 771–777. doi:10.1002/ana.410380512
Olanow, C. W., Watts, R. L., and Koller, W. C. J. N. (2001). An algorithm (decision tree) for the management of Parkinson's disease (2001): Treatment guidelines. Neurology 56, S1–S88. doi:10.1212/wnl.56.suppl_5.s1
Pahwa, R., Busenbark, K., Huber, S., Michalek, D., Hubble, J., and Koller, W. J. N. (1993). Clinical experience with controlled‐release carbidopa/levodopa in Parkinson's disease. Neurology 43, 677–681. doi:10.1212/wnl.43.4.677
Pahwa, R., and Lyons, K. E. (2009). Levodopa-related wearing-off in Parkinson's disease: Identification and management. Curr. Med. Res. Opin. 25, 841–849. doi:10.1185/03007990902779319
Park, S.-Y., and Kim, D. S. J. J. (2002). Discovery of natural products from Curcuma longa that protect cells from beta-amyloid insult: A drug discovery effort against alzheimer's disease. J. Nat. Prod. 65, 1227–1231. doi:10.1021/np010039x
Phani, S., Loike, J. D., and Przedborski, S. (2012). Neurodegeneration and inflammation in Parkinson's disease. Park. Relat. Disord. 18, S207–S209. doi:10.1016/S1353-8020(11)70064-5
Postuma, R. B., Berg, D., Stern, M., Poewe, W., Olanow, C. W., Oertel, W., et al. (2015). MDS clinical diagnostic criteria for Parkinson's disease. Mov. Disord. 30, 1591–1601. doi:10.1002/mds.26424
Prodoehl, J., Burciu, R. G., and Vaillancourt, D. E. J., (2014). Resting state functional magnetic resonance imaging in Parkinson’s disease. Curr. Neurol. Neurosci. Rep. 14, 448. doi:10.1007/s11910-014-0448-6
Quesada, A., Lee, B. Y., and Micevych, P. E. J. D. (2008). PI3 kinase/Akt activation mediates estrogen and IGF‐1 nigral DA neuronal neuroprotection against a unilateral rat model of Parkinson's disease. Dev. Neurobiol. 68, 632–644. doi:10.1002/dneu.20609
Rahman, M., Islam, F., Parvez, A., Azad, M. A., Ashraf, G. M., Ullah, M. F., et al. (2022). Citrus limon L.(lemon) seed extract shows neuro-modulatory activity in an in vivo thiopental-sodium sleep model by reducing the sleep onset and enhancing the sleep duration. J. Integr. Neurosci. 21, 042. doi:10.31083/j.jin2101042
Rahman, M. M., Ferdous, K. S., and Ahmed, M. (2020). Emerging promise of nanoparticle-based treatment for Parkinson’s disease. Biointerface Res. Appl. Chem. 10, 7135–7151.
Rahman, M. M., Ferdous, K. S., Ahmed, M., Islam, M. T., Khan, M., Perveen, A., et al. (2021). Hutchinson-gilford progeria syndrome: An overview of the molecular mechanism, pathophysiology and therapeutic approach. Curr. Gene Ther. 21, 216–229. doi:10.2174/1566523221666210303100805
Rahman, M. M., Islam, M. R., Islam, M. T., Harun-Or-Rashid, M., Islam, M., Abdullah, S., et al. (2022). Stem cell transplantation therapy and neurological disorders: Current status and future perspectives. Biology 11, 147. doi:10.3390/biology11010147
Rahman, M. M., Islam, M. R., Shohag, S., Ahasan, M. T., Sarkar, N., Khan, H., et al. (2022). Microbiome in cancer: Role in carcinogenesis and impact in therapeutic strategies. Biomed. Pharmacother. 149, 112898.
Rahman, M. M., Rahaman, M. S., Islam, M. R., Rahman, F., Mithi, F. M., Alqahtani, T., et al. (2021). Role of phenolic compounds in human disease: Current knowledge and future prospects. Molecules 27, 233. doi:10.3390/molecules27010233
Rahman, M., Rahaman, M., Islam, M., Hossain, M., Mannan Mithi, F., Ahmed, M., et al. (2021). Multifunctional therapeutic potential of phytocomplexes and natural extracts for antimicrobial properties. Antibiot. (Basel). 10, 1076. doi:10.3390/antibiotics10091076
Ramassamy, C., Clostre, F., Christen, Y., and Costentin, J. J. J. (1990). Prevention by a Ginkgo biloba extract (GBE 761) of the dopaminergic neurotoxicity of MPTP. J. Pharm. Pharmacol. 42, 785–789. doi:10.1111/j.2042-7158.1990.tb07021.x
Ravikumar, H., and Muralidhara, G. (2010). Prophylactic treatment with Bacopa monnieri leaf powder mitigates paraquat-induced oxidative perturbations and lethality in Drosophila melanogaster. Indian J. biochem. Biophys. 47, 75–82.
Ren, R., Shi, C., Cao, J., Sun, Y., Zhao, X., Guo, Y., et al. (2016). Neuroprotective effects of a standardized flavonoid extract of safflower against neurotoxin-induced cellular and animal models of Parkinson’s disease. Sci. Rep. 6, 1–13. doi:10.1038/srep22135
Rezaei, M., Nasri, S., Roughani, M., Niknami, Z., and Ziai, S. A. J. I. I. (2016). Peganum Harmala L. extract reduces oxidative stress and improves symptoms in 6-Hydroxydopamine-induced Parkinson’s disease in rats. Iran. J. Pharm. Res. 15, 275–281.
Richardson, J. R., Shalat, S. L., Buckley, B., Winnik, B., O’Suilleabhain, P., Diaz-Arrastia, R., et al. (2009). Elevated serum pesticide levels and risk of Parkinson disease. Arch. Neurol. 66, 870–875. doi:10.1001/archneurol.2009.89
Riley, D., Lang, A., Lewis, A. e., Resch, L., Ashby, P., Hornykiewicz, O., et al. (1990). Cortical-basal ganglionic degeneration. Neurology 40, 1203–1212. doi:10.1212/wnl.40.8.1203
Rocchi, L., Carlson-Kuhta, P., Chiari, L., Burchiel, K. J., Hogarth, P., and Horak, F. B. J., "Effects of deep brain stimulation in the subthalamic nucleus or globus pallidus internus on step initiation in Parkinson disease, " vol. 117, pp. 1141–1149. doi:10.3171/2012.8.jns1120062012
Roy, N. S., Cleren, C., Singh, S. K., Yang, L., Beal, M. F., and Goldman, S. A. J. N. (2006). Functional engraftment of human ES cell–derived dopaminergic neurons enriched by coculture with telomerase-immortalized midbrain astrocytes. Nat. Med. 12, 1259–1268. doi:10.1038/nm1495
Rudakewich, M., Ba, F., and Benishin, C. G. J. P. M. (2001). Neurotrophic and neuroprotective actions of ginsenosides Rb(1) and Rg(1). Planta Med. 67, 533–537. doi:10.1055/s-2001-16488
Rui, W., Li, S., Xiao, H., Xiao, M., and Shi, J. J. I. J. N. (2020). Baicalein attenuates neuroinflammation by inhibiting NLRP3/caspase-1/GSDMD pathway in MPTP-induced mice model of Parkinson’s disease. Int. J. Neuropsychopharmacol. 23, 762–773. doi:10.1093/ijnp/pyaa060
Saijo, K., Winner, B., Carson, C. T., Collier, J. G., Boyer, L., Rosenfeld, M. G., et al. (2009). A Nurr1/CoREST pathway in microglia and astrocytes protects dopaminergic neurons from inflammation-induced death. Cell 137, 47–59. doi:10.1016/j.cell.2009.01.038
Sakina, M., and Dandiya, P. J. F. (1990). A psycho-neuropharmacological profile of Centella asiatica extract. Fitoterapia 61, 291–296. doi:10.1016/j.fitote.2022.105163
Salem, M. A., El-Shiekh, R. A., Fernie, A. R., Alseekh, S., and Zayed, A. (2022). Metabolomics-based profiling for quality assessment and revealing the impact of drying of Turmeric (Curcuma longa L.). Sci. Rep. 12, 1–11. doi:10.1038/s41598-022-13882-y
Schapira, A., Cooper, J., Dexter, D., Clark, J., Jenner, P., and Marsden, C. J. J. o. n. (1990). Mitochondrial complex I deficiency in Parkinson's disease. J. Neurochem. 54, 823–827. doi:10.1111/j.1471-4159.1990.tb02325.x
Schapira, A., Cooper, J., Dexter, D., Jenner, P., Clark, J., and Marsden, C. J. T. L. (1989). Mitochondrial complex I deficiency in Parkinson's disease. Lancet 333, 1269. doi:10.1016/s0140-6736(89)92366-0
Schapira, A. H. J. N. (2006). Etiology of Parkinson's disease. Neurology 66, S10–S23. doi:10.1212/wnl.66.10_suppl_4.s10
Schapira, A. H., and Tolosa, E. J. N. R. N. (2010). Molecular and clinical prodrome of Parkinson disease: Implications for treatment. Nat. Rev. Neurol. 6, 309–317. doi:10.1038/nrneurol.2010.52
Schapira, A. J. E. (2011). Challenges to the development of disease‐modifying therapies in Parkinson’s disease. Eur. J. Neurol. 18, 16–21. doi:10.1111/j.1468-1331.2010.03324.x
Schapira, A. J. M. D. (1994). Evidence for mitochondrial dysfunction in Parkinson's disease—A critical appraisal. Mov. Disord. 9, 125–138. doi:10.1002/mds.870090202
Schapira, A. J. N.(1995). Oxidative stress in Parkinson's disease. Neuropathol. Appl. Neurobiol. 21, 3–9. doi:10.1111/j.1365-2990.1995.tb01022.x
Schenck, C. H., Bundlie, S. R., Ettinger, M. G., and Mahowald, M. W. J. S. (1986). Chronic behavioral disorders of human REM sleep: A new category of parasomnia. Sleep 9, 293–308. doi:10.1093/sleep/9.2.293
Schenck, C. H., Bundlie, S. R., Patterson, A. L., and Mahowald, M. W. J. J., "Rapid eye movement sleep behavior disorder: A treatable parasomnia affecting older adults, " vol. 257, pp. 1786–1789. doi:10.1001/jama.1987.033901301040381987
Schenck, C. H., Mahowald, M. W. J., and Research, S. D. (2002). REM sleep behavior disorder: Clinical, developmental, and neuroscience perspectives 16 years after its formal identification in SLEEP.
Schneider, R. B., Iourinets, J., and Richard, I. H. J. N. (2017). Parkinson's disease psychosis: Presentation, diagnosis and management. Neurodegener. Dis. Manag. 7, 365–376. doi:10.2217/nmt-2017-0028
Schrag, A., Ben-Shlomo, Y., and Quinn, N. J. T. L. (1999). Prevalence of progressive supranuclear palsy and multiple system atrophy: A cross-sectional study. Lancet 354, 1771–1775. doi:10.1016/s0140-6736(99)04137-9
Schupp, P., Steube, K., Meyer, C., and Proksch, P. J. C. l. (2001). Anti-proliferative effects of new staurosporine derivatives isolated from a marine ascidian and its predatory flatworm. Cancer Lett. 174, 165–172. doi:10.1016/s0304-3835(01)00694-2
Sengupta, T., Vinayagam, J., Singh, R., Jaisankar, P., and Mohanakumar, K. (2016). “Plant-derived natural products for Parkinson’s disease therapy,” in The benefits of natural products for neurodegenerative diseases (Springer), 415–496.
Shah, D., Gandhi, M., Kumar, A., Cruz-Martins, N., Sharma, R., and Nair, S. (2021). Current insights into epigenetics, noncoding RNA interactome and clinical pharmacokinetics of dietary polyphenols in cancer chemoprevention. Crit. Rev. Food Sci. Nutr., 1–37. doi:10.1080/10408398.2021.1968786
Sharma, R., Bedarkar, P., Timalsina, D., Chaudhary, A., and Prajapati, P. K. (2022). Bhavana, an ayurvedic pharmaceutical method and a versatile drug delivery platform to prepare potentiated micro-nano-sized drugs: Core concept and its current relevance. Bioinorg. Chem. Appl., 2597–2608. doi:10.1155/2022/1685393
Sharma, R., Kabra, A., Rao, M. M., and Prajapati, P. K. (2018). Herbal and holistic solutions for neurodegenerative and depressive disorders: Leads from Ayurveda. Curr. Pharm. Des. 27 (3), 2597–2608. doi:10.2174/1381612824666180821165741
Sharma, R., Kuca, K., Nepovimova, E., Kabra, A., Rao, M. M., and Prajapati, P. K. (2019). Traditional Ayurvedic and herbal remedies for Alzheimer's disease from bench to bedside. Expert Rev. Neurother. 19, 359–374. doi:10.1080/14737175.2019.1596803
Sharma, R., and Martins, N. (2020). Telomeres, DNA damage and ageing: Potential leads from ayurvedic rasayana (Anti-Ageing) drugs. J. Clin. Med. 9 (8), 2544. doi:10.3390/jcm9082544
Sharma, R., and Prajapati, P. K. (2020). Predictive, preventive and personalized medicine: Leads from ayurvedic concept of prakriti (human constitution). Curr. Pharmacol. Rep. 6, 441–450. doi:10.1007/s40495-020-00244-3
Sharma, R., Singla, R. K., Banerjee, S., Sinha, B., Shen, B., and Sharma, R. (2022). Role of Shankhpushpi (Convolvuluspluricaulis) in neurological disorders: An umbrella review covering evidence fromethnopharmacology to clinical studies. Neurosci. Biobehav Rev. 140, 104795. doi:10.1016/j.neubiorev.2022
Sharma, R., Kakodkar, P., Kabra, A., and Prajapati, P. K. (2022). Golden ager Chyawanprash with meager evidential base from human clinical trials. Evidence-Based Complementary Altern. Med., 1–6. doi:10.1155/2022/9106415
Sharma, S., Awasthi, A., and Singh, S. J. N. (2019). Altered gut microbiota and intestinal permeability in Parkinson's disease: Pathological highlight to management. Neurosci. Lett. 712, 134516. doi:10.1016/j.neulet.2019.134516
Shimura, H., Hattori, N., Kubo, S. I., Yoshikawa, M., Kitada, T., Matsumine, H., et al. (1999). Immunohistochemical and subcellular localization of parkin protein: Absence of protein in autosomal recessive juvenile parkinsonism patients. Ann. Neurol. 45, 668–672. doi:10.1002/1531-8249(199905)45:5<668::aid-ana19>3.0.co;2-z
Shobana, C., Ramesh Kumar, R., and Sumathi, T. (2012). Alcoholic extract of bacopa monniera linn. Protects against 6-hydroxydopamine-induced changes in behavioral and biochemical aspects: A pilot study. Cell. Mol. Neurobiol. 32, 1099–1112. doi:10.1007/s10571-012-9833-3
Shu, Y.-Z. J. (1998). Recent natural products based drug development: A pharmaceutical industry perspective. J. Nat. Prod. 61, 1053–1071. doi:10.1021/np9800102
Shulman, L. M. (2007). Gender differences in Parkinson's disease. Gend. Med. 4, 8–18. doi:10.1016/s1550-8579(07)80003-9
Silva, A. H., Fonseca, F. N., Pimenta, A. T., Lima, M. S., Silveira, E. R., Viana, G. S., et al. (2016). Pharmacognostical analysis and protective effect of standardized extract and rizonic acid from Erythrina velutina against 6-hydroxydopamine-induced neurotoxicity in Sh-Sy5Y cells. Pharmacogn. Mag. 12, 307–312. doi:10.4103/0973-1296.192200
Simard, M., van Reekum, R., and Cohen, T. J. T. J. (2000). A review of the cognitive and behavioral symptoms in dementia with Lewy bodies. J. Neuropsychiatry Clin. Neurosci. 12, 425–450. doi:10.1176/jnp.12.4.425
Singh, A. K., Mishra, S. K., Mishra, G., Maurya, A., Awasthi, R., Yadav, M. K., et al. (2020). Inorganic clay nanocomposite system for improved cholinesterase inhibition and brain pharmacokinetics of donepezil. Drug Dev. Ind. Pharm. 46, 8–19. doi:10.1080/03639045.2019.1698594
Small, G. W., Rabins, P. V., Barry, P. P., Buckholtz, N. S., DeKosky, S. T., Ferris, S. H., et al. (1997). Diagnosis and treatment of alzheimer disease and related disorders: Consensus statement of the American association for geriatric psychiatry. Alzheimer's Assoc. Am. Geriatrics Soc. 278, 1363–1371. doi:10.1001/jama.1997.03550160083043
Snyder, H., and Wolozin, B. (2004). Pathological proteins in Parkinson's disease: Focus on the proteasome. J. Mol. Neurosci. 24, 425–442. doi:10.1385/JMN:24:3:425
Spillantini, M. G., Crowther, R. A., Jakes, R., Hasegawa, M., and Goedert, M. J. P. (1998). alpha-Synuclein in filamentous inclusions of Lewy bodies from Parkinson's disease and dementia with lewy bodies. Proc. Natl. Acad. Sci. U. S. A. 95, 6469–6473. doi:10.1073/pnas.95.11.6469
Stefanis, L. J. N. (2016). Alpha-synuclein aggregation and synaptic pathology in Parkinson’s disease and Dementia with Lewy Bodies. Acta Neuropathol. 1, S4.
Steinman, R. M. J. (1991). The dendritic cell system and its role in immunogenicity. Annu. Rev. Immunol. 9, 271–296. doi:10.1146/annurev.iy.09.040191.001415
Steinman, R. M. J. T. (2001). Dendritic cells and the control of immunity: Enhancing the efficiency of antigen presentation. Mt. Sinai J. Med. 68, 160–166.
Stover, N. P., and Watts, R. L. (2001). “Corticobasal degeneration,” in Seminars in neurology, 049–058.
Su, X., Maguire-Zeiss, K. A., Giuliano, R., Prifti, L., Venkatesh, K., and Federoff, H. J. J. N.(2008). Synuclein activates microglia in a model of Parkinson's disease. Neurobiol. Aging 29, 1690–1701. doi:10.1016/j.neurobiolaging.2007.04.006
Sudati, J. H., Vieira, F. A., Pavin, S. S., Dias, G. R. M., Seeger, R. L., Golombieski, R., et al. (2013). Valeriana officinalis attenuates the rotenone-induced toxicity in Drosophila melanogaster. Neurotoxicology 37, 118–126. doi:10.1016/j.neuro.2013.04.006
Sugumar, M., Sevanan, M., and Sekar, S. J. I. J. (2019). Neuroprotective effect of naringenin against MPTP-induced oxidative stress. Int. J. Neurosci. 129, 534–539. doi:10.1080/00207454.2018.1545772
Surmeier, D. J., Guzman, J. N., and Sanchez-Padilla, J. J. C. (2010). Calcium, cellular aging, and selective neuronal vulnerability in Parkinson's disease. Cell Calcium 47, 175–182. doi:10.1016/j.ceca.2009.12.003
Svensson, E., Beiske, A. G., Loge, J. H., Beiske, K. K., and Sivertsen, B. J. B. n. (2012). Sleep problems in Parkinson’s disease: A community-based study in Norway. BMC Neurol. 12, 71–76. doi:10.1186/1471-2377-12-71
Takeuchi, T., Ogawa, K., Iinuma, H., Suda, H., Ukita, K., Nagatsu, T., et al. (1973). Monoamine oxidase inhibitors isolated from fermented broths. J. Antibiot. 26, 162–167. doi:10.7164/antibiotics.26.162
Tansey, M. G., McCoy, M. K., and Frank-Cannon, T. C. J. E. (2007). Neuroinflammatory mechanisms in Parkinson's disease: Potential environmental triggers, pathways, and targets for early therapeutic intervention. Exp. Neurol. 208, 1–25. doi:10.1016/j.expneurol.2007.07.004
Tellier, J. M., Kalejs, N. I., Leonhardt, B. S., Cannon, D., Hӧӧk, T. O., and Collingsworth, P. D. (2022). Widespread prevalence of hypoxia and the classification of hypoxic conditions in the Laurentian Great Lakes. J. Gt. Lakes. Res. 48, 13–23. doi:10.1016/j.jglr.2021.11.004
Tofaris, G. K., Razzaq, A., Ghetti, B., Lilley, K. S., and Spillantini, M. G. J. J. B. C. (2003). Ubiquitination of α-synuclein in Lewy bodies is a pathological event not associated with impairment of proteasome function. J. Biol. Chem. 278, 44405–44411. doi:10.1074/jbc.M308041200
Tomlinson, C. L., Patel, S., Meek, C., Herd, C. P., Clarke, C. E., Stowe, R., et al. (2013). Physiotherapy versus placebo or no intervention in Parkinson's disease.
Tsai, S.-T., Lin, S.-H., Chou, Y.-C., Pan, Y.-H., Hung, H.-Y., Li, C.-W., et al. (2009). Prognostic factors of subthalamic stimulation in Parkinson’s disease: A comparative study between short-and long-term effects. Stereotact. Funct. Neurosurg. 87, 241–248. doi:10.1159/000225977
Uberti, D., Carsana, T., Francisconi, S., Toninelli, G. F., Canonico, P. L., and Memo, M. J. B. (2004). A novel mechanism for pergolide-induced neuroprotection: Inhibition of NF-kappaB nuclear translocation. Biochem. Pharmacol. 67, 1743–1750. doi:10.1016/j.bcp.2004.01.012
Ugen, K. E., Lin, X., Bai, G., Liang, Z., Cai, J., Li, K., et al. (2015). Evaluation of an α synuclein sensitized dendritic cell based vaccine in a transgenic mouse model of Parkinson disease. Hum. Vaccin. Immunother. 11, 922–930. doi:10.1080/21645515.2015.1012033
Vitek, J. L., Bakay, R. A., Freeman, A., Evatt, M., Green, J., McDonald, W., et al. (2003). Randomized trial of pallidotomy versus medical therapy for Parkinson's disease. Ann. Neurol. 53, 558–569. doi:10.1002/ana.10517
Wang, Y.-M., Cui, Q.-F., Zhao, W.-L., Zhang, J.-Y., and Wang, H.-Q. J. B. (2014). "Nrf2/ARE/HO-1 signaling pathway is a new neuroprotective target for Parkinson’s disease, Front. Pharmacol., " 34, 1125.
Wakita, S., Izumi, Y., Nakai, T., Adachi, K., Takada-Takatori, Y., Kume, T., et al. (2014). Staurosporine induces dopaminergic neurite outgrowth through AMP-activated protein kinase/mammalian target of rapamycin signaling pathway. Neuropharmacology 77, 39–48. doi:10.1016/j.neuropharm.2013.09.012
Wang, J., Yang, Q. X., Sun, X., Vesek, J., Mosher, Z., Vasavada, M., et al. (2015). MRI evaluation of asymmetry of nigrostriatal damage in the early stage of early-onset Parkinson's disease. Park. Relat. Disord. 21, 590–596. doi:10.1016/j.parkreldis.2015.03.012
Wang, L. M., Han, Y. F., and Tang, X. C. J. E. (2000). Huperzine A improves cognitive deficits caused by chronic cerebral hypoperfusion in rats. Eur. J. Pharmacol. 398, 65–72. doi:10.1016/s0014-2999(00)00291-0
Wang, X., Cong, P., Liu, Y., Tao, S., Chen, Q., Wang, J., et al. (2018). Neuritogenic effect of sea cucumber glucocerebrosides on NGF-induced PC12 cells via activation of the TrkA/CREB/BDNF signalling pathway. J. Funct. Foods 46, 175–184. doi:10.1016/j.jff.2018.04.035
Wanneveich, M., Moisan, F., Jacqmin‐Gadda, H., Elbaz, A., and Joly, P. (2018). Projections of prevalence, lifetime risk, and life expectancy of Parkinson's disease (2010‐2030) in France. Mov. Disord. 33, 1449–1455. doi:10.1002/mds.27447
Warner, T. T., and Schapira, A. H., (2003). Genetic and environmental factors in the cause of Parkinson's disease. Ann. Neurol. 53, S16–S25. doi:10.1002/ana.10487
Watanabe, H., Saito, Y., Terao, S., Ando, T., Kachi, T., Mukai, E., et al. (2002). Progression and prognosis in multiple system atrophy: An analysis of 230 Japanese patients. Brain 125, 1070–1083. doi:10.1093/brain/awf117
Weaver, F. M., Follett, K., Stern, M., Hur, K., Harris, C., Marks, W. J., et al. "Bilateral deep brain stimulation vs best medical therapy for patients with advanced Parkinson disease: A randomized controlled trial, " vol. 301, pp. 63–73. doi:10.1001/jama.2008.9292009
Wenning, G., Litvan, I., Jankovic, J., Granata, R., Mangone, C., McKee, A., et al. (1998). Natural history and survival of 14 patients with corticobasal degeneration confirmed at postmortem examination. J. Neurol. Neurosurg. Psychiatry 64, 184–189. doi:10.1136/jnnp.64.2.184
Williamson, T. P. (2012). Activation of the Nrf2-ARE pathway as a therapeutic strategy in Parkinson's disease. The University of Wisconsin-Madison.
Wilms, H., Rosenstiel, P., Sievers, J., Deuschl, G., Zecca, L., and Lucius, R. J. T. F. (2003). Activation of microglia by human neuromelanin is NF‐κB‐dependent and involves p38 mitogen‐activated protein kinase: Implications for Parkinson's disease. FASEB J. 17, 1–20. doi:10.1096/fj.02-0314fje
Wu, Y., Shang, Y., Sun, S., Liang, H., and Liu, R. J. A. (2007). Erythropoietin prevents PC12 cells from 1-methyl-4-phenylpyridinium ion-induced apoptosis via the Akt/GSK-3beta/caspase-3 mediated signaling pathway. Apoptosis 12, 1365–1375. doi:10.1007/s10495-007-0065-9
Xiao, X. Q., Zhang, H. Y., and Tang, X. C. J. J. (2002). Huperzine A attenuates amyloid β‐peptide fragment 25‐35‐induced apoptosis in rat cortical neurons via inhibiting reactive oxygen species formation and caspase‐3 activation. J. Neurosci. Res. 67, 30–36. doi:10.1002/jnr.10075
Xilouri, M., and Stefanis, L. J. (2011). Autophagic pathways in Parkinson disease and related disorders. Expert Rev. Mol. Med. 13. doi:10.1017/S1462399411001803
Xilouri, M., Vogiatzi, T., and Stefanis, L. J. A. (2008). alpha-synuclein degradation by autophagic pathways: a potential key to Parkinson’s disease pathogenesis. Autophagy 4, 917–919. doi:10.4161/auto.6685
Xu, J., Guo, S., Du, L., Wang, Y.-M., Sugawara, T., Hirata, T., et al. (2013). Isolation of cytotoxic glucoerebrosides and long-chain bases from sea cucumber Cucumaria frondosa using high speed counter-current chromatography. J. Oleo Sci. 62, 133–142. doi:10.5650/jos.62.133
Yadav, S., Dixit, A., Agrawal, S., Singh, A., Srivastava, G., Singh, A. K., et al. (2012). Rodent models and contemporary molecular techniques: Notable feats yet incomplete explanations of Parkinson’s disease pathogenesis. Mol. Neurobiol. 46, 495–512. doi:10.1007/s12035-012-8291-8
Yuan, H., Zhang, Z.-W., Liang, L.-W., Shen, Q., Wang, X.-D., Ren, S.-M., et al. (2010). Treatment strategies for Parkinson’s disease. Neurosci. Bull. 26, 66–76. doi:10.1007/s12264-010-0302-z
Yuan, J. P., Peng, J., Yin, K., Wang, J. H. J. M., and research, f. (2011). Potential health‐promoting effects of astaxanthin: A high‐value carotenoid mostly from microalgae. Mol. Nutr. Food Res. 55, 150–165. doi:10.1002/mnfr.201000414
Yuan, K., Li, X., Lu, Q., Zhu, Q., Jiang, H., Wang, T., et al. (2019). Application and mechanisms of triptolide in the treatment of inflammatory diseases—A review. Front. Pharmacol. 10, 1469. doi:10.3389/fphar.2019.01469
Zhai, A., Zhang, Y., Zhu, X., Liang, J., Wang, X., Lin, Y., et al. (2011). Secalonic acid A reduced colchicine cytotoxicity through suppression of JNK, p38 MAPKs and calcium influx. Neurochem. Int. 58, 85–91. doi:10.1016/j.neuint.2010.10.016
Zhang, H., Bai, L., He, J., Zhong, L., Duan, X., Ouyang, L., et al. (2017). Recent advances in discovery and development of natural products as source for anti-Parkinson's disease lead compounds. Eur. J. Med. Chem. 141, 257–272. doi:10.1016/j.ejmech.2017.09.068
Zhang, H., Bai, L., He, J., Zhong, L., Duan, X., Ouyang, L., et al. (2017). Recent advances in discovery and development of natural products as source for anti-Parkinson's disease lead compounds. Eur. J. Med. Chem. 141, 257–272. doi:10.1016/j.ejmech.2017.09.068
Zhang, J., Wei, L., Hu, X., Xie, B., Zhang, Y., Wu, G.-R., et al. (2015). Akinetic-rigid and tremor-dominant Parkinson's disease patients show different patterns of intrinsic brain activity. Park. Relat. Disord. 21, 23–30. doi:10.1016/j.parkreldis.2014.10.017
Zhou, J., Fu, Y., and Tang, X. C. J. N. (2001). Huperzine A and donepezil protect rat pheochromocytoma cells against oxygen–glucose deprivation. Neurosci. Lett. 306, 53–56. doi:10.1016/s0304-3940(01)01855-9
Zhu, G., Wang, X., Chen, Y., Yang, S., Cheng, H., Wang, N., et al. (2010). Puerarin protects dopaminergic neurons against 6-hydroxydopamine neurotoxicity via inhibiting apoptosis and upregulating glial cell line-derived neurotrophic factor in a rat model of Parkinson's disease. Planta Med. 76, 1820–1826. doi:10.1055/s-0030-1249976
Keywords: Parkinson’s disease, medicinal plants, natural products, treatment, neurodegeneration, neuroinflammation, pathways
Citation: Rahman MM, Wang X, Islam MR, Akash S, Supti FA, Mitu MI, Harun-Or-Rashid M, Aktar MN, Khatun Kali MS, Jahan FI, Singla RK, Shen B, Rauf A and Sharma R (2022) Multifunctional role of natural products for the treatment of Parkinson’s disease: At a glance. Front. Pharmacol. 13:976385. doi: 10.3389/fphar.2022.976385
Received: 23 June 2022; Accepted: 21 September 2022;
Published: 06 October 2022.
Edited by:
Hareram Birla, The State University of New Jersey, United StatesReviewed by:
Anurag Kumar Singh, Alabama State University, United StatesPrabhat Suman, Central University of Punjab, India
Copyright © 2022 Rahman, Wang, Islam, Akash, Supti, Mitu, Harun-Or-Rashid, Aktar, Khatun Kali, Jahan, Singla, Shen, Rauf and Sharma. This is an open-access article distributed under the terms of the Creative Commons Attribution License (CC BY). The use, distribution or reproduction in other forums is permitted, provided the original author(s) and the copyright owner(s) are credited and that the original publication in this journal is cited, in accordance with accepted academic practice. No use, distribution or reproduction is permitted which does not comply with these terms.
*Correspondence: Rohit Sharma, cm9oaXRzaGFybWFAYmh1LmFjLmlu, ZGhhbnZhbnRhcmk4NkBnbWFpbC5jb20=, Abdur Rauf, bWFzaGFsamNzQHlhaG9vLmNvbQ==
†These authors have contributed equally to this work and share first authorship