- 1Department of Respiratory, The Affiliated Hospital to Changchun University of Chinese Medicine, Changchun, China
- 2State Key Laboratory of Quality Research in Chinese Medicines, Faculty of Chinese Medicine, Macau University of Science and Technology, Macau, China
- 3Jilin Ginseng Academy, Changchun University of Chinese Medicine, Changchun, China
- 4Guangdong-Hong Kong-Macao Joint Laboratory for Contaminants Exposure and Health, Guangzhou, China
Inflammation and oxidative stress lead to various acute or chronic diseases, including pneumonia, liver and kidney injury, cardiovascular and cerebrovascular diseases, metabolic diseases, and cancer. Ginseng is a well-known and widely used ethnic medicine in Asian countries, and ginsenoside Rg3 is a saponin isolated from Panax ginseng C. A. Meyer, Panax notoginseng, or Panax quinquefolius L. This compound has a wide range of pharmacological properties, including antioxidant and anti-inflammatory activities, which have been evaluated in disease models of inflammation and oxidative stress. Rg3 can attenuate lung inflammation, prevent liver and kidney function damage, mitigate neuroinflammation, prevent cerebral and myocardial ischemia–reperfusion injury, and improve hypertension and diabetes symptoms. The multitarget, multipathway mechanisms of action of Rg3 have been gradually deciphered. This review summarizes the existing knowledge on the anti-inflammatory and antioxidant effects and underlying molecular mechanisms of ginsenoside Rg3, suggesting that ginsenoside Rg3 may be a promising candidate drug for the treatment of diseases with inflammatory and oxidative stress conditions.
1 Introduction
Ginseng, the root of Panax ginseng C.A. Meyer, has been used for 2000 years in ethnomedicine in Asia to treat various diseases (Lee et al., 2020a). The roots, leaves, and fruits of ginseng plants have been extensively used as herbal medicines. The antioxidant activity of ginseng is exploited to treat cardiovascular diseases (Hyun et al., 2022). Interestingly, red ginseng has better antioxidant activity and is used to treat neurodegenerative diseases, gastroenteritis, liver damage, diabetes, and aging (Lee et al., 2017a). The Rg3 content of fresh ginseng is relatively low, and the content in ginseng after processing or enzymatic transformation accounts for 0.37–1.13% of the total saponins (Chang et al., 2009; Kim et al., 2013). Red ginseng has undergone steaming treatment. During this process, some ginsenosides with lower polarity are produced. The Rg3 content increases with increasing steaming batches. After nine steam cycles, the Rg3 content in the total saponins was 15–18% (Lee et al., 2012a; Jeong et al., 2017). According to the same process used to make red ginseng roots, Chen et al. steamed ginseng leaves, and Rg3 increased 8.6-fold (Chen et al., 2020). These findings suggest that Rg3 is one of the primary ginsenosides in red ginseng.
This work aimed to understand how Rg3 regulates oxidative stress and inflammation to elicit pharmacological effects and to review the literature on the therapeutic use of Rg3 based on antioxidant and anti-inflammatory effects. We briefly introduced the biological functions of Rg3 in response to inflammation and oxidative stress. We then summarized the effects of Rg3 on neurological disorders, cardiovascular diseases, cancer, lung diseases, diabetes, and digestive system diseases (Figure 1).
2 Methodology
A literature search was performed in PubMed from August 1998 to January 2022, and the last search date was 30 April 2022. The search term was “Ginsenoside Rg3”. A secondary search was conducted by screening the list of articles that met the inclusion criteria. The keywords were inflammation, oxidative stress, antioxidant, and anti-inflammatory. The obtained articles were screened, irrelevant studies were excluded, and eligible articles were sorted and classified. Finally, we organized the tables, wrote the text, and made figures to summarize the application of Rg3 antioxidant and anti-inflammatory effects.
3 Overview of oxidative stress and inflammation
Reactive oxygen species (ROS), including superoxide, hydroxyl radicals, and hydrogen peroxide (H2O2), are necessary for cell growth, differentiation, responses to external stimuli, and other processes. ROS are primarily produced in the mitochondria through the respiratory chain and the nicotinamide adenine dinucleotide phosphate (NADPH) oxidase system. In addition to mitochondria, cytochrome P450 2E1 (CYP2E1), cyclooxygenase-2 (COX-2), and lipoxygenases in the plasma membrane and cytosol produce ROS. ROS are subsequently eliminated by superoxide dismutase (SOD), catalase (CAT), glutathione peroxidase (GSH-px), and nonenzymatic scavenging factors, such as glutathione (GSH) and vitamin C, to maintain redox homeostasis (Ramos-Tovar and Muriel, 2020). In response to ROS accumulation, peroxidase production activates the NF-E2-related factor 2 (Nrf2)/heme oxygenase 1 (HO-1) pathway (Kobayashi and Yamamoto, 2005). Lipid oxidation produces toxic metabolic compounds, hydroperoxides, and hydroxides; they aggravate the formation of atherosclerosis and liver injury. Malondialdehyde (MDA) is an end product of lipid oxidation used as a biomarker for assessing oxidative damage (Ayala et al., 2014; Silva et al., 2022). Nitric oxide synthase (NOS) catalyzes the production of nitric oxide (NO) and citrulline using oxygen and l-arginine as substrates. There are three isoforms of NOS. Endothelial NOS (eNOS) and neuronal NOS (nNOS) are expressed in the normal state. NO produced by eNOS is critical for maintaining vascular homeostasis (Balligand et al., 2009). Inducible NOS (iNOS) is induced after injury (Brown, 2007). iNOS-derived NO reacts with superoxide anions to form peroxynitrite, leading to oxidative stress related to inflammatory neurodegeneration, endothelial dysfunction, and liver and kidney injury (Jaeschke et al., 2002; Landmesser et al., 2003; Heemskerk et al., 2009; Incalza et al., 2018).
Oxidative stress and inflammation occur concomitantly. The accumulation of ROS can induce inflammation. In turn, the inflammatory response triggers oxidative stress. In the inflammatory response, inflammatory factors activate Toll-like receptor (TLR) signaling receptors in neutrophils and macrophages, and inflammatory mediators are released in large quantities. This process leads to oxygen consumption, which is produced through the NADPH pathway, and the accumulation of oxygen free radicals causes oxidative stress (Furman et al., 2019). When cells are damaged by oxidative stress, ROS accumulate in large quantities and activate TLR receptors in Kupffer cells and macrophages, thus increasing proinflammatory mediators such as the release of interleukin-1β (IL-1β) and IL-6 (Yoon et al., 2015; Ramos-Tovar and Muriel, 2020).
4 Antioxidant and anti-inflammatory effects and molecular mechanisms of Rg3
In response to oxidative stress, Rg3 inhibits ROS accumulation and MDA content, improves mitochondrial function, and increases the activity of antioxidant enzymes, including SOD, GSH, GSH-px, and CAT, to promote oxygen free radical metabolism. Rg3 responds to oxidative stress by regulating Nrf2/HO-1 and sirtuin 1 (SIRT1) (Xing et al., 2017; Kee and Hong, 2019; Ren et al., 2021). Rg3 inhibits iNOS and NO release to reduce peroxynitrite formation; interestingly, it promotes eNOS-derived NO to protect vascular endothelial cells (Singh et al., 2018).
In lipopolysaccharide (LPS)-mediated inflammation models, Rg3 decreased the expression of COX-2 and prostaglandin E2 (PGE2) and the secretion of tumor necrosis factor-α (TNF-α), IL-1β, and IL-6 (Shin et al., 2013; Lee et al., 2017b; Kee and Hong, 2019). Inhibitory NLRP3 inflammasome activation and NLRP3-apoptosis-associated speck-like protein (ASC) interaction were observed in an LPS-induced mouse model after treatment with Rg3 (Kang et al., 2018; Shi et al., 2020). The mechanisms of response to inflammation relate to inhibition of the nuclear factor-κB (NF-κB) pathway, including upstream TLR4/myeloid differentiation primary response 88 (MyD88) and downstream COX-2 expression (Kang et al., 2018; Candelario-Jalil et al., 2022). Rg3 upregulates SIRT1 to inhibit the NF-κB pathway, contributing to anti-inflammatory activity (Ren et al., 2021). Downregulation of the phosphatidylinositol-3-kinase (PI3K)/Akt and p38 mitogen-activated protein kinase (MAPK) pathways by Rg3 suppressed inflammation directly or by crosstalk with the NF-κB pathway (Hou et al., 2017; Yang et al., 2018; Kee and Hong, 2019).
4.1 Pulmonary diseases
Inflammation is present in various pulmonary diseases. Persistent inflammation in the airway and lung tissue is involved in the pathogenesis of chronic lung diseases such as chronic obstructive pulmonary disease (COPD), asthma, and pulmonary fibrosis (Racanelli et al., 2018). Acute lung disease is often accompanied by acute inflammation, including acute lung injury (ALI), pneumonia, and acute exacerbation of COPD (AECOPD) (Robb et al., 2016). Inflammation promotes the accumulation of oxidative stress products. These findings suggest that anti-inflammation is a critical step in pulmonary disease treatment (Domej et al., 2014). In animal models of chemical-induced ALI, Rg3 inhibited cytokines and increased the content of antioxidant enzymes (SOD, CAT, and GSH) to reduce pulmonary inflammation and neutrophil infiltration (Wang et al., 2016; Yang et al., 2018). The anti-inflammatory effect of Rg3 was also observed in the AECOPD mouse model, and these effects were related to the inhibition of the PI3K/Akt pathway (Yang et al., 2018; Guan et al., 2020). In an airway inflammation model stimulated by Asian sand dust, upregulation of mucin (MUC)5AC and MUC5B stimulates mucus secretion; significantly, mucus cross-linking exacerbates pulmonary disease (Dunican et al., 2021). Rg3 decreased mucin gene expression through the NF-κB pathway (Shin et al., 2021). Regarding asthma, Rg3 inhibited p65 phosphorylation, and COX-2 inhibited the secretion of chemokine ligands, ILs, and TNF-α. Rg3 exerts its antioxidant effect through the Nrf2/HO-1 pathway to decrease ROS generation. Anti-inflammatory and antioxidant effects have been used to treat asthma models in vitro and in vivo (Lee et al., 2016; Huang et al., 2021). In summary, Rg3 exerts anti-inflammatory effects to alleviate lung damage (Table 1).
4.2 Liver and kidney injury
Several studies have demonstrated that Rg3 downregulates abnormally elevated alanine aminotransferase (ALT) and aspartate aminotransferase (AST) to improve liver function in models of drug-induced or inflammation-mediated liver injury (Kang et al., 2007; Zhou et al., 2018). In addition, Rg3 alleviated acetaminophen-mediated liver tissue apoptosis by activating the PI3K/Akt pathway (Zhou et al., 2018). In a mouse model of acetaminophen-mediated/induced liver injury, Rg3 reduced GSH consumption and inhibited CYP2E1 overexpression to combat oxidative stress and hepatotoxicity (Zhou et al., 2018; Gao et al., 2021). Rg3 decreased thiobarbituric acid (TBA) and iNOS to mitigate oxidative damage in the livers and kidneys of LPS-treated rats (Kang et al., 2007). Rg3 also protected against acute liver and kidney function injury caused by chemotherapeutic agents. Chemotherapy containing cis-platinum (DDP) causes liver and kidney damage, and several studies found that Rg3 treatment significantly protected against hepatotoxicity and nephrotoxicity. ALT, AST, blood urea nitrogen (BUN), and creatinine (CRE), which represent liver and kidney function, decreased after treatment with Rg3 in a DDP-induced mouse model (Lee et al., 2012b). Upregulation of GSH, GSH-Px, and SOD enhanced antioxidant capacity and reduced ROS generation and MDA content (Lee et al., 2012b; Zhang et al., 2021). Rg3 downregulates p53 and caspase-3 to inhibit apoptosis of kidney cells (Park et al., 2015; Zhang et al., 2021). For liver fibrosis, Rg3 decreased NF-κB to inhibit hepatic inflammation and fibrosis distribution in a nonalcoholic steatohepatitis mouse model (Lee et al., 2020b). In a thioacetamide (TAA)-induced hepatic fibrosis mouse model, Rg3 reduced inflammation-mediated autophagy and increased SOD, CAT, and GSH levels to improve liver function and attenuate fibrosis (Liu et al., 2020) (Table 2).
4.3 Neurological disorders
When neurons suffer oxidative stress, ROS cooperate with mitochondrial Ca2+ to increase the permeability of the mitochondrial transition pore (MPTP), resulting in mitochondrial swelling. This process leads to cellular inflammation, senescence, and apoptosis (Zhao et al., 2022). In an H2O2-induced mitochondrial suspension from the rat forebrain, 20(S)-Rg3 provided neuroprotection and inhibited mitochondrial swelling through suppression of ROS (Tian et al., 2009). Rg3 inhibited IL-6, IL-8, and monocyte chemotactic protein 1 (MCP-1) and ameliorated cellular senescence via inactivation of NF-κB and p38 in H2O2-treated human astrocyte CRT cells (Hou et al., 2017). The antioxidant effect of Rg3 mitigated neurotoxic effects associated with the prevention and treatment of neurodegeneration. Rg3 protected cortical cells from glutamate-induced nerve damage by enhancing SOD and GSH-px (Kim et al., 1998). Trimethyltin chloride-induced neurotoxicity of ICR mice promoted oxidative stress, inflammation, hippocampal neuron loss, and astrocytic activation in the mouse brain, which generates a clinical syndrome characterized by amnesia and complex seizures. Rg3 and Rh2 improved neurotoxicity via upregulation of PI3K/Akt and suppression of extracellular signal-regulated kinase (ERK) (Hou et al., 2018). In a mouse model of systemic inflammation mediated by LPS, 20(S)-Rg3 suppressed iNOS and COX-2 to attenuate inflammatory activation of the brain (Park et al., 2012).
Cognitive and memory impairments are closely associated with Alzheimer’s disease (AD). Chronic neuroinflammation of the entorhinal cortex and hippocampus leads to memory impairments (Lukiw and Bazan, 2000). The inhibition of COX-2 caused by Rg3 ameliorated LPS-induced learning and memory impairments in rats (Lee et al., 2013). Pathologically, the accumulation of abnormally folded amyloid-β (Aβ) and neuronal fiber aggregation lead to early cognitive impairment in AD. Rg3 reduced the neurotoxicity of Aβ42-treated BV-2 microglial cells. The mechanism suggested that Rg3 inhibited NF-κB p65 nuclear translocation to reduce the release of inflammatory factors and upregulated scavenger receptor type A (SRA) (Joo et al., 2008); this membrane protein recognizes pathological products and activates microglia to clear necrotic neurons (Bairamian et al., 2022). Bairamian et al. reported that Rg3 reduced ROS and Aβ deposition in a Caenorhabditis elegans model of AD (Tangrodchanapong et al., 2020). In addition, an in vitro study confirmed that regulation of inflammatory factors by Rg3 restored M2 activation and promoted Aβ uptake in microglia and neuronal cells (Ahn et al., 2021). In summary, Rg3 increases Aβ uptake to improve memory and cognitive function, which is associated with the inhibition of inflammatory factors through NF-κB and COX-2.
Parkinson’s disease (PD) is a neurodegenerative disorder with a decreased number of nigrostriatal neurons. Loss of nigrostriatal dopamine and α-synuclein aggregation correlates with the severity of PD. In a transgenic Caenorhabditis elegans model of PD, Rg3 suppressed apoptosis and enhanced antioxidant enzymes to prolong lifespan (Chalorak et al., 2021). Another study reported that Rg3 prolonged the latency of rotenone-induced PD mice through evaluation of pole, rotarod, and open field tests. The mice showed improved motor function and dopamine content in the striatum, suggesting that Rg3 enhances the expression of glutamate cysteine ligase (GCL) (Han et al., 2021), which catalyzes GSH synthesis to prevent oxidative damage and ROS generation (Forman et al., 2009). To sum up, Rg3 increases peroxidase activity to promote dopamine secretion and slow PD progression.
Cerebral ischemia is a common disease with severe consequences. The excessive accumulation of cytokines increases the adhesion of leukocytes to blood vessels, leading to endothelial damage and aggravating cerebral ischemia–reperfusion (I/R) injury (Candelario-Jalil et al., 2022). Rg3 attenuated IL-1β, IL-6, TNF-α, and ROS generation in a middle cerebral artery occlusion (MCAO) rat model. The mechanism suggests that Rg3 inhibits TLR4/MyD88 and SIRT1 to protect the brain from ischemic injury (Cheng et al., 2019).
Accelerated chronic inflammation and aging of nucleus pulposus cells (NPCs) lead to intervertebral disk degeneration (IDD) (Heathfield et al., 2008; Li et al., 2017). In TNF-α-treated NPCs, Rg3 improved oxidative stress status and extracellular matrix (ECM) metabolism by inhibiting NF-κB p65 activation in a dose-dependent manner (Chen et al., 2019). Spinal cord injury is a destructive spinal cord lesion that is more severe than IDD. 20(S)-Rg3 promoted the recovery of motor function and attenuated iNOS and COX-2 in a spinal cord injury rat model. (Kim et al., 2017).
In conclusion, Rg3 has a strong neuroprotective effect. When neurons are stimulated by peroxide, Rg3 scavenges free radicals, protects mitochondrial function, inhibits inflammatory factors, suppresses cell senescence. When neurons are exposed to toxic substances, Rg3 increases peroxidase activity to detoxify and activate PI3K/Akt to prevent apoptosis. The development of neurodegenerative and chronic diseases, including AD, PD, and stroke, is often accompanied by oxidative stress and inflammation (Zhao et al., 2022). These studies suggest that Rg3 inhibits cytokines, COX-2, ROS generation, and mitochondrial dysfunction and enhances peroxidase activity and microglial polarization to exert therapeutic effects on neurological disorders (Table 3).
4.4 Cardiovascular diseases
In the early pathological processes of hypertension and atherosclerosis, chronic vascular inflammation and toxic metabolic compounds of lipid oxidation lead to vascular endothelial dysfunction and lipid deposition (Dasagrandhi et al., 2022). This process is accompanied by upregulation of vascular cell adhesion molecule-1 (VCAM-1) and intercellular adhesion molecule-1 (ICAM-1), and oxidized lipids and adhesion molecules mutually promote the plaque formation process of atherosclerosis (Blankenberg et al., 2003; Malekmohammad et al., 2019). In the presence of various inducers (e.g., LPS, NOD1 agonist, or ox-LDL), Rg3 downregulates ICAM-1 and VCAM-1 to inhibit endothelial dysfunction, which is related to anti-inflammatory action by the NF-κB pathway (Cho et al., 2014; Lee et al., 2020c; Geng et al., 2020). Rg3 inhibited THP-1-cell adhesion into human umbilical vein endothelial cells (HUVECs) by suppressing the NF-κB pathway in vitro (Cho et al., 2014). Oral Rg3 restrained the formation of atherosclerosis and inflammation in ApoE−/− mice via the regulation of peroxisome proliferator-activated receptor γ (PPARγ)/focal adhesion kinase (FAK) (Geng et al., 2020).
NO is a significant factor in maintaining vascular homeostasis. Rg3 promoted NO release to increase thoracic aortic relaxation in rats, which had a therapeutic effect on hypertension (Kim et al., 1999; Nagar et al., 2016). The mechanism is related to the activation of eNOS or iNOS. Kim et al. suggested that iNOS was upregulated by NF-κB activation. Low-dose Rg3 promoted macrophage proinflammatory responses, including upregulation of IL-1 and TNF and NO release (Kim et al., 2003). Another study found that the activation and phosphorylation of eNOS mediated Rg3 treatment of hypertension. Rg3 activated estrogen receptor (ER)-dependent PI3K and AMP-activated protein kinase (AMPK) pathways to upregulate eNOS in endothelial cells (Hien et al., 2010). These studies suggest that Rg3 regulates vasodilatation by increasing the release of NO in hypertension.
Ginseng invigorates vitality in ethnic medicine, which is related to the regulation of heart function. Rg3 improved cardiac function and myocardial damage in rats with myocardial I/R surgery (Wang et al., 2015a; Zhang et al., 2016). In the treatment of myocardial injury, Rg3 reduced the secretion of IL-6, IL-1β, and TNF-α, inhibited lactic acid accumulation, improved the oxidative stress state, including upregulation of SOD content and downregulation of ROS accumulation, indicating that Rg3 is a SIRT1 agonist that downregulates the NF-κB pathway (Li et al., 2020a; Tu et al., 2020). Li et al. developed Rg3-loaded ROS-responsive polymeric nanoparticles, which have a lower therapeutic dose (Li et al., 2020a). In addition, Rg3 enhanced B-cell lymphoma-2 (Bcl-2) and inhibited p53, Bcl-2-associated X (Bax) and caspase-3 to resist apoptosis of cardiomyocytes in vitro and in SD rats with myocardial I/R surgery in vivo (Wang et al., 2015a; Zhang et al., 2016; Li et al., 2020a). Upregulation of Akt/eNOS for NO release by Rg3 protected against myocardial injury (Wang et al., 2015a). For hypertrophic cardiomyopathy, Rg3 alleviated oxidative stress, inflammation, and fibrosis via SIRT1/NF-κB in rats undergoing transverse aortic coarctation (TAC) surgery (Ren et al., 2021). Rg3 improved heart function and aortic ring endothelial function in adriamycin (ADM)-treated rats. The mechanism involved the activation of Nrf2/HO-1 to reduce ROS and increase eNOS levels (Wang et al., 2015b). The beneficial effects of Rg3 on cardiovascular disease are summarized in Table 4.
4.5 Diabetes and diabetic complications
Diabetes is a metabolic disease with increasing incidence worldwide. Oxidative stress promotes metabolic dysfunction and complications in diabetes (Bhatti et al., 2022). Rg3 exerts its therapeutic effect in the treatment of diabetes, diabetic nephropathy (DN), and dyslipidemia. In streptozotocin (STZ)-induced diabetes models, Rg3 reduced fasting blood glucose (FBG) and glycosylated protein (Kang et al., 2008; Li et al., 2021). iNOS, COX-2, and NF-кB p65 were decreased after Rg3 intervention (Kang et al., 2008; Kim et al., 2014). iNOS-derived overproduction of NO leads to the development of diabetes (Bahadoran et al., 2020). The NF-κB signaling cascade is the initial event in response to oxidative stress in diabetes (Archuleta et al., 2009). A study showed that Rg3 decreased urine protein associated with DN in a rat model via inhibition of NF-κB p65 (Zhou et al., 2020). Another study found that 20(S)-Rg3 ameliorated renal histopathological injury and dyslipidemia through the MAPK and NF-κB pathways in C57BL/6 mice with an intraperitoneal injection of STZ and a high-fat diet (HFD). At the same time, 20(S)-Rg3 improved oxidative stress status by diminishing the overproduction of MDA and enhancing serum SOD (Zhou et al., 2020). Guo et al. found that 20(S)-Rg3 skewed macrophages to the M2 phenotype and decreased the expression of TNF-α, IL-6, IL-10, and transforming growth factor-β (TGF-β) to mitigate atherosclerosis in diabetic apoE−/− mice (Guo et al., 2018). For diabetic complications, inhibition of the apoptotic signaling pathway can protect renal tissue in DN. Rg3 protected renal tubular epithelial cells from apoptosis in vitro (Zhou et al., 2020). Li et al. suggested that Rg3 reduced Bax and cleaved caspase3 and enhanced the expression of Bcl-2 and Bcl-XL to inhibit apoptosis in the kidneys of DN mice (Li et al., 2021). In addition, Rg3 improved erectile function by upregulating SOD and protected nerve fibers from apoptosis in diabetic rats (Liu et al., 2015). These studies suggest that Rg3 is useful in treating diabetes and complications, attributable to its antioxidant, anti-inflammatory and anti-apoptosis effects (Table 5).
4.6 Cancer
Rg3-rich extracts of ginseng upregulated NO production to activate an immune response in LPS-treated Raw 264.7 cells and inhibited the proliferation of HUVECs (Lee et al., 2009). Interestingly, Kim et al. observed that Rg3 inhibited the differentiation and migration of endothelial progenitor cells through suppression of vascular endothelial growth factor (VEGF)/Akt/eNOS signaling, suggesting an antiangiogenic effect (Kim et al., 2012). However, the study did not detect the release of NO. In addition, a red ginseng preparation with Rg3-fortified facilitated NO release for the proliferation of splenocytes and inhibition of tumor growth in lung cancer tumor-bearing mice (Park et al., 2011). These findings suggest that the promotion of NO release by Rg3 inhibits angiogenesis and tumor proliferation; however, the synthesis pathway of NO production requires further study.
Oxidative stress status and tumor therapy need to be analysed from two aspects. ROS accumulation in tumor tissue induces tumor cell apoptosis (e.g., ferroptosis) (Liang et al., 2019). On the other hand, free radicals derived from tumor cells promote DNA damage and mutation to cause tumorigenesis and tumor metastasis (Prasad et al., 2017). Graphene oxide nanoparticle-loaded Rg3 promoted apoptosis and enhanced ROS generation to enhance photodynamic therapy of osteosarcoma (Lu et al., 2021). Rg3 nanoparticles (Rg3-NPs) promoted apoptosis and improved oxidative stress states against Ehrlich solid tumor growth in mice (El-Banna et al., 2022). Regarding tumorigenesis, abnormal expression of COX-2 and proinflammatory cytokines is associated with skin carcinogenesis (Moon et al., 2020). The topical application of Rg3 inhibited COX-2 and decreased skin carcinogenesis through the downregulation of the transcription factors NF-κB and activator protein-1 (AP-1) (Keum et al., 2003).
4.7 Other oxidative stress-related diseases
The aging process is accompanied by impairment of free radical scavenging and oxidative stress damage (Papaconstantinou, 2019). Rg3 increases mitochondrial biogenesis and enhances antioxidation to reduce ROS against cell senescence (Hong et al., 2020). Reduction of ROS by Rg3 protected keratinocytes from ultraviolet irradiation (Lim et al., 2014). In a d-galactose-induced aging mouse model, Rg3 inhibited CYP2E1 and MDA to protect against aging-related hepatic and renal dysfunction (Li et al., 2020b). Rg3 protected the gastric mucosa and inhibited gastric ulcer formation. Rg3 reduced iNOS-reducing NO release and the accumulation of free radicals in gastric mucosa mediated by alcohol; Rg3 also promoted the expression of epidermal growth factor (EGF) for epithelial proliferation and tissue repair (Zhang et al., 2019). Nanoparticles loaded with Rg3 could increase gastric ulcer treatment efficacy (Cao et al., 2022). Rg3 improved mitochondrial biogenesis and downregulated ROS by activating SIRT1 in TNF-α-treated chondrocytes, which could serve as an adjunct therapy for arthritis (Ma et al., 2021). Rg3 attenuated aluminum-induced osteoporosis by enhancing GSH and SOD activity (Song et al., 2020). Cyclophosphamide is an alkylating agent used to treat hematologic malignancies; however, it is associated with bone marrow toxicity. Rg3 inhibited CP-induced DNA damage by improving oxidative stress states in vivo (Zhang et al., 2008). In TNF-α-treated human chondrocytes, Rg3 inhibited mitochondrial ROS production and enhanced ATP content, which has potential as a therapeutic remedy for muscle weakness and atrophy (Lee et al., 2019). Several studies found that Rg3 inhibited the release of inflammatory factors and downregulated VEGF expression during wound healing, which is a potential treatment for hypertrophic scars (Cheng et al., 2013; Cheng et al., 2014; Ma et al., 2020).
5 Overview of the therapeutic potential of ginsenoside Rg3
Rg3 has potent antioxidant and anti-inflammatory effects, indicating that it might have a potential therapeutic role in acute organ injuries such as acute lung injury, acute liver and kidney injury, etc. Rg3 alleviates inflammatory infiltration of lung tissue and inhibits IL release in lung tissue in mouse and rat models of ALI (Yang et al., 2018). In an OVA-induced asthma mouse model, Rg3 ameliorates goblet cell hyperplasia and eosinophil infiltration (Huang et al., 2021). For liver and kidney injury caused by external factors, Rg3 significantly improves liver function, mainly by inhibiting the abnormal elevation of aminotransferases and overexpression of CYP2E1; meanwhile, Rg3 improves acetaminophen-induced focal centrilobular necrosis and pericentral venous degeneration of liver tissue (Zhou et al., 2018; Gao et al., 2021). In addition, Rg3 inhibits the expression of α-SMA and collagen I during the fibrosis process of nonalcoholic steatohepatitis and hepatic fibrosis (Lee et al., 2020b; Liu et al., 2020). In DDP-mediated nephrotoxicity, Rg3 increases kidney tissue GSH expression, inhibits MDA levels, and downregulates serum BUN and CRE to protect renal function (Lee et al., 2012b; Zhang et al., 2021).
Rg3 can improve cardiac function, reduce markers of cardiac damage, suppress neurocyte aging, and reduce memory impairment in rats (Lee et al., 2013; Hou et al., 2017), indicating that Rg3 might also have the potential to become a promising therapeutic agent for chronic diseases, including cardiovascular diseases, cerebrovascular diseases, diabetes and cancers, etc. Rg3 enhanced Aβ uptake through M2 microglial activation in AD (Ahn et al., 2021). Rg3 prolonged the latency of rotenone-induced PD mice through evaluation of pole, rotarod, and open field tests (Han et al., 2021). The above effects can improve neurological damage, memory deterioration and cognitive impairment, which alleviates the progression of neurodegenerative diseases. In cardiovascular diseases, vascular inflammation and lipid accumulation are reduced after Rg3 intervention (Lee et al., 2020c; Geng et al., 2020). Several studies concluded that Rg3 activated eNOS for vasodilation in the treatment of hypertension (Kim et al., 2003; Hien et al., 2010). Rg3 enhanced left ventricular ejection fraction (EF%) to improve cardiac function in rats with myocardial I/R surgery. Rg3 ameliorated myocardial infarction-related serum markers, including creatine kinase (CK), CK-MB, and lactate dehydrogenase (LDH) (Wang et al., 2015a; Zhang et al., 2016). In metabolic diseases, Rg3 reduces FBG, glycosylated protein and blood lipids in diabetic rats (Kang et al., 2008; Li et al., 2021). Rg3 improves CRE and urine protein elevation in DN mice by increasing antioxidant activity and reducing renal inflammation (Zhou et al., 2020; Li et al., 2021). Rg3 inhibited the proliferation and metastasis of various cancer cells, such as liver cancer cells, intestinal cancer cells, osteosarcoma cells, etc. (Sun et al., 2017). Either Rg3 alone or Rg3 in combination with DDP suppressed the growth of colon tumor in nude mice (Lee et al., 2012b). Treatment with Rg3 decreased the tumor size of Ehrlich solid tumor in mice (El-Banna et al., 2022).
In summary, increasing evidence has shown that Rg3 has therapeutic potential for the treatment of acute organ injuries and chronic diseases, which is worthy of further research.
6 Discussion
The antioxidant and anti-inflammatory effects of Rg3 have been attributed to 1) antioxidant properties, including inhibition of ROS accumulation, promotion of peroxidase activity, and reduction of MDA content; 2) anti-inflammatory properties, inhibition of TNF-α, interleukins, chemokine ligands, suppression of NLRP3 formation and COX-2 expression; 3) regulation of apoptosis: downregulating the expression of p53, caspases and Bax/Bcl-2 ratio to protect tissue injury and apoptosis; and 4) regulation of NOS, inhibition of iNOS to reduce oxidative stress and upregulation of eNOS to protect vascular endothelial function. These antioxidant and anti-inflammatory activities of Rg3 help to maintain or improve organ functions, such as reducing neuroinflammation, attenuating neurotoxicity, protecting myocardial, hepatic and renal functions (Figure 2).
These effects are related to several potential mechanisms of action: (Lee et al., 2020a): inhibition of the NF-κB pathway decreases downstream COX-2 to ameliorate inflammation and oxidative stress; (Hyun et al., 2022); the PI3K/Akt pathway regulates the process of inflammation and apoptosis; and (Lee et al., 2017a) upregulation of the Nrf2/HO-1 pathway is an important pathway to reduce oxidative stress. Therefore, the key mechanism of action of Rg3 is shown in Figure 3. However, the content of these studies tends to be homogeneous, and there is a lack of Rg3 target screening based on high-throughput methods. In the future, we look forward to clinical research to explore the antioxidant activity of Rg3 and in-depth research into the critical targets of Rg3 antioxidant and anti-inflammatory activity.
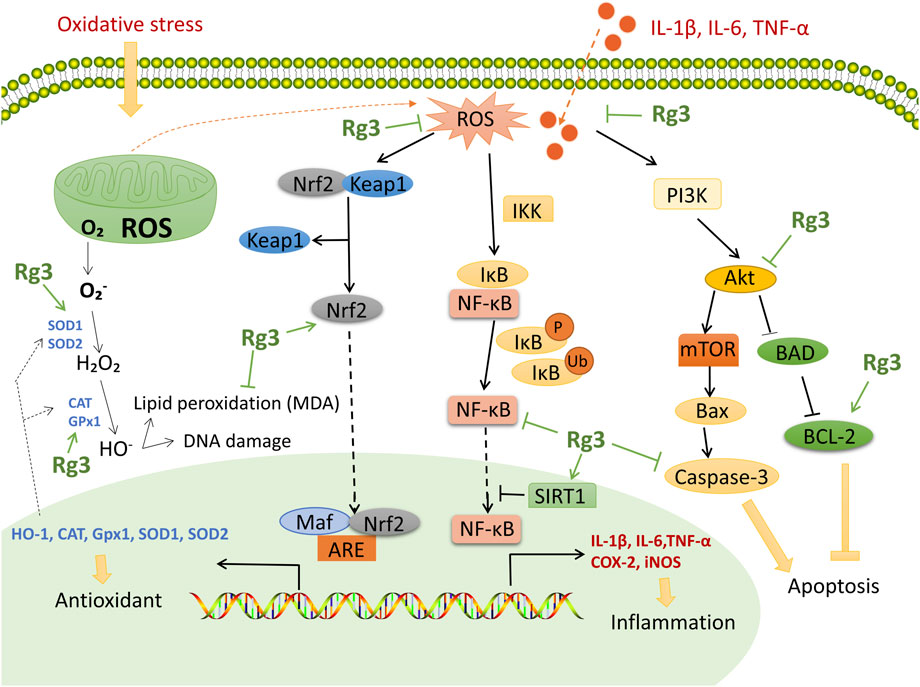
FIGURE 3. Functional mechanisms and targets of Rg3 on antioxidant and anti-inflammatory effects. Rg3 upregulates Nrf2 to enhance antioxidase activity. Rg3 inhibits the NF-κB pathway and downstream COX-2 to ameliorate inflammation and oxidative stress. Rg3 activates SIRT1 to inhibit the NF-κB pathway. Rg3 protects tissue from apoptosis through the PI3K/Akt pathway.
7 Conclusion
Increasing evidence supports that ginsenoside Rg3 has remarkable anti-inflammatory and antioxidant effects on animal and cell models with various diseases, such as pulmonary, neurological, cardiovascular, metabolic diseases, cancer, and liver and kidney injury, and the multitarget and multipathway molecular mechanisms of action of Rg3 have been gradually deciphered. Rg3 may be a promising candidate drug for the treatment of diseases with inflammatory and oxidative stress conditions and is worthy of further research and development.
Author contributions
JW, QW, and TW designed the work. JW, YZ, WQ, ZW, and LT collected and reviewed the references. JW wrote the first draft. JW, LZ, DZ, XL and TW wrote and reviewed the final version of the manuscript. All authors discussed and contributed to the manuscript.
Funding
This study was supported by the National Natural Science Foundation of China (U19A2013), the Education Department of Jilin Province (JJKH20210964KJ), the Science and Technology Development Plan Project of Jilin Province (202002053JC), the Science and Technology Development Fund, Macau SAR (file No.: 0098/2021/A2, 130/2017/A3, and 0099/2018/A3), and the Science and Technology Planning Project of Guangdong Province (2020B1212030008).
Acknowledgments
Figure 1 of the article was drawn by Figdraw (www.figdraw.com).
Conflict of interest
The authors declare that the research was conducted in the absence of any commercial or financial relationships that could be construed as a potential conflict of interest.
Publisher’s note
All claims expressed in this article are solely those of the authors and do not necessarily represent those of their affiliated organizations, or those of the publisher, the editors and the reviewers. Any product that may be evaluated in this article, or claim that may be made by its manufacturer, is not guaranteed or endorsed by the publisher.
References
Ahn, J. W., Jang, S. K., Jo, B. R., Kim, H. S., Park, J. Y., Park, H. Y., et al. (2021). A therapeutic intervention for alzheimer's disease using ginsenoside Rg3: Its role in M2 microglial activation and non-amyloidogenesis. J. Physiol. Pharmacol. 72 (2). Epub 2021/08/11PubMed PMID: 34374655. doi:10.26402/jpp.2021.2.04
Archuleta, T. L., Lemieux, A. M., Saengsirisuwan, V., Teachey, M. K., Lindborg, K. A., Kim, J. S., et al. (2009). Oxidant stress-induced loss of IRS-1 and IRS-2 proteins in rat skeletal muscle: Role of p38 MAPK. Free Radic. Biol. Med. 47 (10), 1486–1493. Epub 2009/08/26PubMed PMID: 19703555; PubMed Central PMCID: PMCPMC2767452. doi:10.1016/j.freeradbiomed.2009.08.014
Ayala, A., Muñoz, M. F., and Argüelles, S. (2014). Lipid peroxidation: Production, metabolism, and signaling mechanisms of malondialdehyde and 4-hydroxy-2-nonenal. Oxidative Med. Cell. Longev. 2014, 360438. Epub 2014/07/08PubMed PMID: 24999379; PubMed Central PMCID: PMCPMC4066722. doi:10.1155/2014/360438
Bahadoran, Z., Mirmiran, P., and Ghasemi, A. (2020). Role of nitric oxide in insulin secretion and glucose metabolism. Trends Endocrinol. Metab. 31 (2), 118–130. Epub 2019/11/07PubMed PMID: 31690508. doi:10.1016/j.tem.2019.10.001
Bairamian, D., Sha, S., Rolhion, N., Sokol, H., Dorothée, G., Lemere, C. A., et al. (2022). Microbiota in neuroinflammation and synaptic dysfunction: A focus on alzheimer's disease. Mol. Neurodegener. 17 (1), 19. Epub 2022/03/07PubMed PMID: 35248147; PubMed Central PMCID: PMCPMC8898063. doi:10.1186/s13024-022-00522-2
Balligand, J. L., Feron, O., and Dessy, C. (2009). eNOS activation by physical forces: from short-term regulation of contraction to chronic remodeling of cardiovascular tissues. Physiol. Rev. 89 (2), 481–534. Epub 2009/04/04PubMed PMID: 19342613. doi:10.1152/physrev.00042.2007
Bhatti, J. S., Sehrawat, A., Mishra, J., Sidhu, I. S., Navik, U., Khullar, N., et al. (2022). Oxidative stress in the pathophysiology of type 2 diabetes and related complications: Current therapeutics strategies and future perspectives. Free Radic. Biol. Med. 184, 114–134. Epub 2022/04/11PubMed PMID: 35398495. doi:10.1016/j.freeradbiomed.2022.03.019
Blankenberg, S., Barbaux, S., and Tiret, L. (2003). Adhesion molecules and atherosclerosis. Atherosclerosis 170 (2), 191–203. Epub 2003/11/13PubMed PMID: 14612198. doi:10.1016/s0021-9150(03)00097-2
Brown, G. C. (2007). Mechanisms of inflammatory neurodegeneration: iNOS and NADPH oxidase. Biochem. Soc. Trans. 35 (5), 1119–1121. Epub 2007/10/25PubMed PMID: 17956292. doi:10.1042/bst0351119
Candelario-Jalil, E., Dijkhuizen, R. M., and Magnus, T. (2022). Neuroinflammation, stroke, blood-brain barrier dysfunction, and imaging modalities. Stroke 53 (5), 1473–1486. Epub 2022/04/08PubMed PMID: 35387495; PubMed Central PMCID: PMCPMC9038693. doi:10.1161/strokeaha.122.036946
Cao, L., Wang, S., Zhang, L., and Li, J. (2022). mPEG-b-P(Glu-co-Phe) nanoparticles increase gastric retention time and gastric ulcer treatment efficacy of 20(S)-ginsenoside Rg3. Biomed. Pharmacother. = Biomedecine Pharmacother. 146, 112608. Epub 2022/01/23PubMed PMID: 35062071. doi:10.1016/j.biopha.2021.112608
Chalorak, P., Sanguanphun, T., Limboonreung, T., and Meemon, K. (2021). Neurorescue effects of frondoside A and ginsenoside Rg3 in C. elegans model of Parkinson's disease. Mol. (Basel, Switz. 26 (16), 4843. Epub 2021/08/28PubMed PMID: 34443430; PubMed Central PMCID: PMCPMC8402114. doi:10.3390/molecules26164843
Chang, K. H., Jee, H. S., Lee, N. K., Park, S. H., Lee, N. W., Paik, H. D., et al. (2009). Optimization of the enzymatic production of 20(S)-ginsenoside Rg(3) from white ginseng extract using response surface methodology. N. Biotechnol. 26 (3-4), 181–186. Epub 2009/09/09PubMed PMID: 19735748. doi:10.1016/j.nbt.2009.08.011
Chen, J., Liu, G. Z., Sun, Q., Zhang, F., Liu, C. Y., Yuan, L., et al. (2019). Protective effects of ginsenoside Rg3 on TNF-α-induced human nucleus pulposus cells through inhibiting NF-κB signaling pathway. Life Sci. 216, 1–9. Epub 2018/11/15PubMed PMID: 30428306. doi:10.1016/j.lfs.2018.11.022
Chen, W., Balan, P., and Popovich, D. G. (2020). Changes of ginsenoside composition in the creation of black ginseng leaf. Mol. (Basel, Switz. 25 (12), E2809. Epub 2020/06/24PubMed PMID: 32570758; PubMed Central PMCID: PMCPMC7355439. doi:10.3390/molecules25122809
Cheng, L., Sun, X., Hu, C., Jin, R., Sun, B., Shi, Y., et al. (2014). In vivo early intervention and the therapeutic effects of 20(s)-ginsenoside rg3 on hypertrophic scar formation. PloS one 9 (12), e113640. Epub 2014/12/17PubMed PMID: 25502572; PubMed Central PMCID: PMCPMC4264739. doi:10.1371/journal.pone.0113640
Cheng, L., Sun, X., Hu, C., Jin, R., Sun, B., Shi, Y., et al. (2013). In vivo inhibition of hypertrophic scars by implantable ginsenoside-Rg3-loaded electrospun fibrous membranes. Acta Biomater. 9 (12), 9461–9473. Epub 2013/08/14PubMed PMID: 23938200. doi:10.1016/j.actbio.2013.07.040
Cheng, Z., Zhang, M., Ling, C., Zhu, Y., Ren, H., Hong, C., et al. (2019). Neuroprotective effects of ginsenosides against cerebral ischemia. Mol. (Basel, Switz. 24 (6), E1102. Epub 2019/03/23PubMed PMID: 30897756; PubMed Central PMCID: PMCPMC6471240. doi:10.3390/molecules24061102
Cho, Y. S., Kim, C. H., Kim, H. N., Ha, T. S., and Ahn, H. Y. (2014). Ginsenoside Rg3 inhibits lipopolysaccharide-induced adhesion molecule expression in human umbilical vein endothelial cell and C57BL/6 mice. Pharmazie 69 (11), 818–822. Epub 2015/05/20. PubMed PMID: 25985577.
Dasagrandhi, D., Muthuswamy, A., and Swaminathan, J. K. (2022). Atherosclerosis: Nexus of vascular dynamics and cellular cross talks. Mol. Cell. Biochem. 477 (2), 571–584. Epub 2021/12/01PubMed PMID: 34845570. doi:10.1007/s11010-021-04307-x
Domej, W., Oettl, K., and Renner, W. (2014). Oxidative stress and free radicals in COPD--implications and relevance for treatment. Int. J. Chron. Obstruct. Pulmon. Dis. 9, 1207–1224. Epub 2014/11/08PubMed PMID: 25378921; PubMed Central PMCID: PMCPMC4207545. doi:10.2147/copd.S51226
Dunican, E. M., Elicker, B. M., Henry, T., Gierada, D. S., Schiebler, M. L., Anderson, W., et al. (2021). Mucus plugs and emphysema in the pathophysiology of airflow obstruction and hypoxemia in smokers. Am. J. Respir. Crit. Care Med. 203 (8), 957–968. Epub 2020/11/13PubMed PMID: 33180550; PubMed Central PMCID: PMCPMC8048745. doi:10.1164/rccm.202006-2248OC
El-Banna, M. A., Hendawy, O. M., El-Nekeety, A. A., and Abdel-Wahhab, M. A. (2022). Efficacy of ginsenoside Rg3 nanoparticles against Ehrlich solid tumor growth in mice. Environ. Sci. Pollut. Res. Int. 29, 43814–43825. Epub 2022/02/05PubMed PMID: 35118592. doi:10.1007/s11356-022-19019-y
Forman, H. J., Zhang, H., and Rinna, A. (2009). Glutathione: Overview of its protective roles, measurement, and biosynthesis. Mol. Asp. Med. 30 (1-2), 1–12. Epub 2008/09/18PubMed PMID: 18796312; PubMed Central PMCID: PMCPMC2696075. doi:10.1016/j.mam.2008.08.006
Furman, D., Campisi, J., Verdin, E., Carrera-Bastos, P., Targ, S., Franceschi, C., et al. (2019). Chronic inflammation in the etiology of disease across the life span. Nat. Med. 25 (12), 1822–1832. Epub 2019/12/07PubMed PMID: 31806905; PubMed Central PMCID: PMCPMC7147972. doi:10.1038/s41591-019-0675-0
Gao, Y., Yan, J., Li, J., Li, X., Yang, S., Chen, N., et al. (2021). Ginsenoside Rg3 ameliorates acetaminophen-induced hepatotoxicity by suppressing inflammation and oxidative stress. J. Pharm. Pharmacol. 73 (3), 322–331. Epub 2021/04/02PubMed PMID: 33793882. doi:10.1093/jpp/rgaa069
Geng, J., Fu, W., Yu, X., Lu, Z., Liu, Y., Sun, M., et al. (2020). Ginsenoside Rg3 alleviates ox-LDL induced endothelial dysfunction and prevents atherosclerosis in ApoE(-/-) mice by regulating PPARγ/FAK signaling pathway. Front. Pharmacol. 11, 500. Epub 2020/05/12PubMed PMID: 32390845; PubMed Central PMCID: PMCPMC7188907. doi:10.3389/fphar.2020.00500
Guan, X., Yuan, Y., Wang, G., Zheng, R., Zhang, J., Dong, B., et al. (2020). Ginsenoside Rg3 ameliorates acute exacerbation of COPD by suppressing neutrophil migration. Int. Immunopharmacol. 83, 106449. Epub 2020/04/12PubMed PMID: 32278128. doi:10.1016/j.intimp.2020.106449
Guo, M., Xiao, J., Sheng, X., Zhang, X., Tie, Y., Wang, L., et al. (2018). Ginsenoside Rg3 mitigates atherosclerosis progression in diabetic apoE-/- mice by skewing macrophages to the M2 phenotype. Front. Pharmacol. 9, 464. Epub 2018/06/06PubMed PMID: 29867472; PubMed Central PMCID: PMCPMC5954105. doi:10.3389/fphar.2018.00464
Han, Y., Wang, T., Li, C., Wang, Z., Zhao, Y., He, J., et al. (2021). Ginsenoside Rg3 exerts a neuroprotective effect in rotenone-induced Parkinson's disease mice via its anti-oxidative properties. Eur. J. Pharmacol. 909, 174413. Epub 2021/08/16PubMed PMID: 34391769. doi:10.1016/j.ejphar.2021.174413
Heathfield, S. K., Le Maitre, C. L., and Hoyland, J. A. (2008). Caveolin-1 expression and stress-induced premature senescence in human intervertebral disc degeneration. Arthritis Res. Ther. 10 (4), R87. Epub 2008/08/07PubMed PMID: 18681962; PubMed Central PMCID: PMCPMC2575636. doi:10.1186/ar2468
Heemskerk, S., Masereeuw, R., Russel, F. G., and Pickkers, P. (2009). Selective iNOS inhibition for the treatment of sepsis-induced acute kidney injury. Nat. Rev. Nephrol. 5 (11), 629–640. Epub 2009/09/30PubMed PMID: 19786992. doi:10.1038/nrneph.2009.155
Hien, T. T., Kim, N. D., Pokharel, Y. R., Oh, S. J., Lee, M. Y., Kang, K. W., et al. (2010). Ginsenoside Rg3 increases nitric oxide production via increases in phosphorylation and expression of endothelial nitric oxide synthase: Essential roles of estrogen receptor-dependent PI3-kinase and AMP-activated protein kinase. Toxicol. Appl. Pharmacol. 246 (3), 171–183. Epub 2010/06/16PubMed PMID: 20546771. doi:10.1016/j.taap.2010.05.008
Hong, T., Kim, M. Y., Da Ly, D., Park, S. J., Eom, Y. W., Park, K. S., et al. (2020). Ca(2+)-activated mitochondrial biogenesis and functions improve stem cell fate in Rg3-treated human mesenchymal stem cells. Stem Cell Res. Ther. 11 (1), 467. Epub 2020/11/06PubMed PMID: 33148318; PubMed Central PMCID: PMCPMC7640456. doi:10.1186/s13287-020-01974-3
Hou, J., Kim, S., Sung, C., and Choi, C. (2017). Ginsenoside Rg3 prevents oxidative stress-induced astrocytic senescence and ameliorates senescence paracrine effects on glioblastoma. Mol. (Basel, Switz. 22 (9), E1516. Epub 2017/09/12PubMed PMID: 28891967; PubMed Central PMCID: PMCPMC6151485. doi:10.3390/molecules22091516
Hou, J., Xue, J., Wang, Z., and Li, W. (2018). Ginsenoside Rg3 and Rh2 protect trimethyltin-induced neurotoxicity via prevention on neuronal apoptosis and neuroinflammation. Phytother. Res. 32 (12), 2531–2540. Epub 2018/10/03PubMed PMID: 30277284. doi:10.1002/ptr.6193
Huang, W. C., Huang, T. H., Yeh, K. W., Chen, Y. L., Shen, S. C., Liou, C. J., et al. (2021). Ginsenoside Rg3 ameliorates allergic airway inflammation and oxidative stress in mice. J. Ginseng Res. 45 (6), 654–664. Epub 2021/11/13PubMed PMID: 34764720; PubMed Central PMCID: PMCPMC8569325. doi:10.1016/j.jgr.2021.03.002
Hyun, S. H., Bhilare, K. D., Park, C. K., and Kim, J. H. (2022). Effects of Panax ginseng and ginsenosides on oxidative stress and cardiovascular diseases: Pharmacological and therapeutic roles. J. Ginseng Res. 46 (1), 33–38. Epub 2022/01/22PubMed PMID: 35058725; PubMed Central PMCID: PMCPMC8753520. doi:10.1016/j.jgr.2021.07.007
Incalza, M. A., D'Oria, R., Natalicchio, A., Perrini, S., Laviola, L., Giorgino, F., et al. (2018). Oxidative stress and reactive oxygen species in endothelial dysfunction associated with cardiovascular and metabolic diseases. Vasc. Pharmacol. 100, 1–19. Epub 2017/06/06PubMed PMID: 28579545. doi:10.1016/j.vph.2017.05.005
Jaeschke, H., Gores, G. J., Cederbaum, A. I., Hinson, J. A., Pessayre, D., Lemasters, J. J., et al. (2002). Mechanisms of hepatotoxicity. Toxicol. Sci. 65 (2), 166–176. Epub 2002/01/29PubMed PMID: 11812920. doi:10.1093/toxsci/65.2.166
Jeong, D., Irfan, M., Kim, S. D., Kim, S., Oh, J. H., Park, C. K., et al. (2017). Ginsenoside Rg3-enriched red ginseng extract inhibits platelet activation and in vivo thrombus formation. J. Ginseng Res. 41 (4), 548–555. Epub 2017/10/13PubMed PMID: 29021703; PubMed Central PMCID: PMCPMC5628340. doi:10.1016/j.jgr.2016.11.003
Joo, S. S., Yoo, Y. M., Ahn, B. W., Nam, S. Y., Kim, Y. B., Hwang, K. W., et al. (2008). Prevention of inflammation-mediated neurotoxicity by Rg3 and its role in microglial activation. Biol. Pharm. Bull. 31 (7), 1392–1396. Epub 2008/07/02PubMed PMID: 18591781. doi:10.1248/bpb.31.1392
Kang, K. S., Kim, H. Y., Yamabe, N., Park, J. H., and Yokozawa, T. (2007). Preventive effect of 20(S)-ginsenoside Rg3 against lipopolysaccharide-induced hepatic and renal injury in rats. Free Radic. Res. 41 (10), 1181–1188. Epub 2007/09/22PubMed PMID: 17886040. doi:10.1080/10715760701581740
Kang, K. S., Yamabe, N., Kim, H. Y., Park, J. H., and Yokozawa, T. (2008). Therapeutic potential of 20(S)-ginsenoside Rg(3) against streptozotocin-induced diabetic renal damage in rats. Eur. J. Pharmacol. 591 (1-3), 266–272. Epub 2008/07/10PubMed PMID: 18611400. doi:10.1016/j.ejphar.2008.06.077
Kang, S., Park, S. J., Lee, A. Y., Huang, J., Chung, H. Y., Im, D. S., et al. (2018). Ginsenoside Rg(3) promotes inflammation resolution through M2 macrophage polarization. J. Ginseng Res. 42 (1), 68–74. Epub 2018/01/20PubMed PMID: 29348724; PubMed Central PMCID: PMCPMC5766702. doi:10.1016/j.jgr.2016.12.012
Kee, J. Y., and Hong, S. H. (2019). Ginsenoside Rg3 suppresses mast cell-mediated allergic inflammation via mitogen-activated protein kinase signaling pathway. J. Ginseng Res. 43 (2), 282–290. Epub 2019/04/13PubMed PMID: 30976166; PubMed Central PMCID: PMCPMC6437450. doi:10.1016/j.jgr.2018.02.008
Keum, Y. S., Han, S. S., Chun, K. S., Park, K. K., Park, J. H., Lee, S. K., et al. (2003). Inhibitory effects of the ginsenoside Rg3 on phorbol ester-induced cyclooxygenase-2 expression, NF-kappaB activation and tumor promotion. Mutat. Res. 523-524, 52375–52485. Epub 2003/03/12PubMed PMID: 12628505. doi:10.1016/s0027-5107(02)00323-8
Kim, D. K., Kweon, K. J., Kim, P., Kim, H. J., Kim, S. S., Sohn, N. W., et al. (2017). Ginsenoside Rg3 improves recovery from spinal cord injury in rats via suppression of neuronal apoptosis, pro-inflammatory mediators, and microglial activation. Mol. (Basel, Switz. 22 (1), E122. Epub 2017/01/14PubMed PMID: 28085110; PubMed Central PMCID: PMCPMC6155773. doi:10.3390/molecules22010122
Kim, J. W., Jung, S. Y., Kwon, Y. H., Lee, S. H., Lee, J. H., Lee, B. Y., et al. (2012). Ginsenoside Rg3 inhibits endothelial progenitor cell differentiation through attenuation of VEGF-dependent Akt/eNOS signaling. Phytother. Res. 26 (9), 1286–1293. Epub 2012/01/19PubMed PMID: 22253055. doi:10.1002/ptr.3722
Kim, N. D., Kang, S. Y., Park, J. H., and Schini-Kerth, V. B. (1999). Ginsenoside Rg3 mediates endothelium-dependent relaxation in response to ginsenosides in rat aorta: Role of K+ channels. Eur. J. Pharmacol. 367 (1), 41–49. Epub 1999/03/19PubMed PMID: 10082263. doi:10.1016/s0014-2999(98)00898-x
Kim, N. D., Kim, E. M., Kang, K. W., Cho, M. K., Choi, S. Y., Kim, S. G., et al. (2003). Ginsenoside Rg3 inhibits phenylephrine-induced vascular contraction through induction of nitric oxide synthase. Br. J. Pharmacol. 140 (4), 661–670. Epub 2003/10/10PubMed PMID: 14534150; PubMed Central PMCID: PMCPMC1574077. doi:10.1038/sj.bjp.0705490
Kim, S. J., Kim, J. D., and Ko, S. K. (2013). Changes in ginsenoside composition of ginseng berry extracts after a microwave and vinegar process. J. Ginseng Res. 37 (3), 269–272. Epub 2013/11/08PubMed PMID: 24198651; PubMed Central PMCID: PMCPMC3818952. doi:10.5142/jgr.2013.37.269
Kim, S. S., Jang, H. J., Oh, M. Y., Eom, D. W., Kang, K. S., Kim, Y. J., et al. (2014). Ginsenoside Rg3 enhances islet cell function and attenuates apoptosis in mouse islets. Transpl. Proc. 46 (4), 1150–1155. Epub 2014/05/13PubMed PMID: 24815149. doi:10.1016/j.transproceed.2013.12.028
Kim, Y. C., Kim, S. R., Markelonis, G. J., and Oh, T. H. (1998). Ginsenosides Rb1 and Rg3 protect cultured rat cortical cells from glutamate-induced neurodegeneration. J. Neurosci. Res. 53 (4), 426–432. Epub 1998/08/26PubMed PMID: 9710262. doi:10.1002/(sici)1097-4547(19980815)53:4<426::Aid-jnr4>3.0.Co;2-8
Kobayashi, M., and Yamamoto, M. (2005). Molecular mechanisms activating the Nrf2-Keap1 pathway of antioxidant gene regulation. Antioxid. Redox Signal. 7 (3-4), 385–394. Epub 2005/02/12PubMed PMID: 15706085. doi:10.1089/ars.2005.7.385
Landmesser, U., Dikalov, S., Price, S. R., McCann, L., Fukai, T., Holland, S. M., et al. (2003). Oxidation of tetrahydrobiopterin leads to uncoupling of endothelial cell nitric oxide synthase in hypertension. J. Clin. Invest. 111 (8), 1201–1209. Epub 2003/04/17PubMed PMID: 12697739; PubMed Central PMCID: PMCPMC152929. doi:10.1172/jci14172
Lee, A., Yun, E., Chang, W., and Kim, J. (2020). Ginsenoside Rg3 protects against iE-DAP-induced endothelial-to-mesenchymal transition by regulating the miR-139-5p-NF-κB axis. J. Ginseng Res. 44 (2), 300–307. Epub 2020/03/10PubMed PMID: 32148412; PubMed Central PMCID: PMCPMC7031736. doi:10.1016/j.jgr.2019.01.003
Lee, B., Sur, B., Park, J., Kim, S. H., Kwon, S., Yeom, M., et al. (2013). Ginsenoside rg3 alleviates lipopolysaccharide-induced learning and memory impairments by anti-inflammatory activity in rats. Biomol. Ther. 21 (5), 381–390. Epub 2013/11/19PubMed PMID: 24244826; PubMed Central PMCID: PMCPMC3825202. doi:10.4062/biomolther.2013.053
Lee, C. K., Park, K. K., Chung, A. S., and Chung, W. Y. (2012). Ginsenoside Rg3 enhances the chemosensitivity of tumors to cisplatin by reducing the basal level of nuclear factor erythroid 2-related factor 2-mediated heme oxygenase-1/NAD(P)H quinone oxidoreductase-1 and prevents normal tissue damage by scavenging cisplatin-induced intracellular reactive oxygen species. Food Chem. Toxicol. 50 (7), 2565–2574. Epub 2012/01/24PubMed PMID: 22266358. doi:10.1016/j.fct.2012.01.005
Lee, H., Kong, G., Tran, Q., Kim, C., Park, J., Park, J., et al. (2020). Relationship between ginsenoside Rg3 and metabolic syndrome. Front. Pharmacol. 11, 130. Epub 2020/03/13PubMed PMID: 32161549; PubMed Central PMCID: PMCPMC7052819. doi:10.3389/fphar.2020.00130
Lee, I. S., Uh, I., Kim, K. S., Kim, K. H., Park, J., Kim, Y., et al. (2016). Anti-inflammatory effects of ginsenoside Rg3 via NF-κB pathway in A549 cells and human asthmatic lung tissue. J. Immunol. Res. 2016, 7521601. Epub 2017/01/25PubMed PMID: 28116321; PubMed Central PMCID: PMCPMC5223042 of this paper. doi:10.1155/2016/7521601
Lee, J. H., Oh, J. Y., Kim, S. H., Oh, I. J., Lee, Y. H., Lee, K. W., et al. (2020). Pharmaceutical efficacy of gypenoside LXXV on non-alcoholic steatohepatitis (NASH). Biomolecules 10 (10), E1426. Epub 2020/10/15PubMed PMID: 33050067; PubMed Central PMCID: PMCPMC7599508. doi:10.3390/biom10101426
Lee, J. J., Kwon, H. K., Jung, I. H., Cho, Y. B., Kim, K. J., Kim, J. L., et al. (2009). Anti-cancer activities of ginseng extract fermented with phellinus linteus. Mycobiology 37 (1), 21–27. Epub 2009/03/01PubMed PMID: 23983502; PubMed Central PMCID: PMCPMC3749450. doi:10.4489/myco.2009.37.1.021
Lee, J. W., Choi, Y. R., Mok, H. J., Seong, H. A., Lee, D. Y., Kim, G. S., et al. (2017). Characterization of the changes in eicosanoid profiles of activated macrophages treated with 20(S)-ginsenoside Rg3. J. Chromatogr. B Anal. Technol. Biomed. Life Sci. 1065-1066, 106514–110669. Epub 2017/09/25PubMed PMID: 28938131. doi:10.1016/j.jchromb.2017.09.002
Lee, S. A., Jo, H. K., Im, B. O., Kim, S., Whang, W. K., Ko, S. K., et al. (2012). Changes in the contents of prosapogenin in the red ginseng (Panax ginseng) depending on steaming batches. J. Ginseng Res. 36 (1), 102–106. Epub 2012/01/01PubMed PMID: 23717110; PubMed Central PMCID: PMCPMC3659570. doi:10.5142/jgr.2012.36.1.102
Lee, S. J., Bae, J. H., Lee, H., Lee, H., Park, J., Kang, J. S., et al. (2019). Ginsenoside Rg3 upregulates myotube formation and mitochondrial function, thereby protecting myotube atrophy induced by tumor necrosis factor-alpha. J. Ethnopharmacol. 242, 112054. Epub 2019/07/05PubMed PMID: 31271820. doi:10.1016/j.jep.2019.112054
Lee, Y. M., Yoon, H., Park, H. M., Song, B. C., and Yeum, K. J. (2017). Implications of red Panax ginseng in oxidative stress associated chronic diseases. J. Ginseng Res. 41 (2), 113–119. Epub 2017/04/18PubMed PMID: 28413314; PubMed Central PMCID: PMCPMC5386131. doi:10.1016/j.jgr.2016.03.003
Li, L., Wang, Y., Guo, R., Li, S., Ni, J., Gao, S., et al. (2020). Ginsenoside Rg3-loaded, reactive oxygen species-responsive polymeric nanoparticles for alleviating myocardial ischemia-reperfusion injury. J. Control. Release 317, 259–272. Epub 2019/11/30PubMed PMID: 31783047; PubMed Central PMCID: PMCPMC7384207. doi:10.1016/j.jconrel.2019.11.032
Li, P., Gan, Y., Xu, Y., Wang, L., Ouyang, B., Zhang, C., et al. (2017). 17beta-estradiol attenuates TNF-α-Induced premature senescence of nucleus pulposus cells through regulating the ROS/NF-κB pathway. Int. J. Biol. Sci. 13 (2), 145–156. Epub 2017/03/04PubMed PMID: 28255267; PubMed Central PMCID: PMCPMC5332869. doi:10.7150/ijbs.16770
Li, W., Wang, J. Q., Zhou, Y. D., Hou, J. G., Liu, Y., Wang, Y. P., et al. (2020). Rare ginsenoside 20(R)-Rg3 inhibits D-galactose-induced liver and kidney injury by regulating oxidative stress-induced apoptosis. Am. J. Chin. Med. 48 (5), 1141–1157. Epub 2020/07/17PubMed PMID: 32668974. doi:10.1142/s0192415x20500561
Li, Y., Hou, J. G., Liu, Z., Gong, X. J., Hu, J. N., Wang, Y. P., et al. (2021). Alleviative effects of 20(R)-Rg3 on HFD/STZ-induced diabetic nephropathy via MAPK/NF-κB signaling pathways in C57BL/6 mice. J. Ethnopharmacol. 267, 113500. Epub 2020/10/23PubMed PMID: 33091499. doi:10.1016/j.jep.2020.113500
Liang, C., Zhang, X., Yang, M., and Dong, X. (2019). Recent progress in ferroptosis inducers for cancer therapy. Adv. Mat. 31 (51), e1904197. Epub 2019/10/09PubMed PMID: 31595562. doi:10.1002/adma.201904197
Lim, C. J., Choi, W. Y., and Jung, H. J. (2014). Stereoselective skin anti-photoaging properties of ginsenoside Rg3 in UV-B-irradiated keratinocytes. Biol. Pharm. Bull. 37 (10), 1583–1590. Epub 2014/07/25PubMed PMID: 25056231. doi:10.1248/bpb.b14-00167
Liu, T., Peng, Y. F., Jia, C., Yang, B. H., Tao, X., Li, J., et al. (2015). Ginsenoside Rg3 improves erectile function in streptozotocin-induced diabetic rats. J. Sex. Med. 12 (3), 611–620. Epub 2014/12/03PubMed PMID: 25442300. doi:10.1111/jsm.12779
Liu, X., Mi, X., Wang, Z., Zhang, M., Hou, J., Jiang, S., et al. (2020). Ginsenoside Rg3 promotes regression from hepatic fibrosis through reducing inflammation-mediated autophagy signaling pathway. Cell Death Dis. 11 (6), 454. Epub 2020/06/14PubMed PMID: 32532964; PubMed Central PMCID: PMCPMC7293224. doi:10.1038/s41419-020-2597-7
Lu, S. L., Wang, Y. H., Liu, G. F., Wang, L., Li, Y., Guo, Z. Y., et al. (2021). Graphene oxide nanoparticle-loaded ginsenoside Rg3 improves photodynamic therapy in inhibiting malignant progression and stemness of osteosarcoma. Front. Mol. Biosci. 8, 663089. Epub 2021/05/11PubMed PMID: 33968991; PubMed Central PMCID: PMCPMC8100436. doi:10.3389/fmolb.2021.663089
Lukiw, W. J., and Bazan, N. G. (2000). Neuroinflammatory signaling upregulation in Alzheimer's disease. Neurochem. Res. 25 (9-10), 1173–1184. Epub 2000/11/04PubMed PMID: 11059791. doi:10.1023/a:1007627725251
Ma, C. H., Chou, W. C., Wu, C. H., Jou, I. M., Tu, Y. K., Hsieh, P. L., et al. (2021). Ginsenoside Rg3 attenuates TNF-α-induced damage in chondrocytes through regulating SIRT1-mediated anti-apoptotic and anti-inflammatory mechanisms. Antioxidants (Basel, Switz. 10 (12), 1972. Epub 2021/12/25PubMed PMID: 34943075; PubMed Central PMCID: PMCPMC8750552. doi:10.3390/antiox10121972
Ma, L., Li, L. Y., and Zhao, T. L. (2020). Anti-inflammatory effects of ginsenoside Rg3 on the hypertrophic scar formation via the NF-κB/IκB signaling pathway in rabbit ears. Pharmazie 75 (2), 102–106. Epub 2020/03/28PubMed PMID: 32213242. doi:10.1691/ph.2020.9852
Malekmohammad, K., Sewell, R. D. E., and Rafieian-Kopaei, M. (2019). Antioxidants and atherosclerosis: Mechanistic aspects. Biomolecules 9 (8), E301. Epub 2019/07/28PubMed PMID: 31349600; PubMed Central PMCID: PMCPMC6722928. doi:10.3390/biom9080301
Moon, H., White, A. C., and Borowsky, A. D. (2020). New insights into the functions of Cox-2 in skin and esophageal malignancies. Exp. Mol. Med. 52 (4), 538–547. Epub 2020/04/03PubMed PMID: 32235869; PubMed Central PMCID: PMCPMC7210257. doi:10.1038/s12276-020-0412-2
Nagar, H., Choi, S., Jung, S. B., Jeon, B. H., and Kim, C. S. (2016). Rg3-enriched Korean Red Ginseng enhances blood pressure stability in spontaneously hypertensive rats. Integr. Med. Res. 5 (3), 223–229. Epub 2017/05/04PubMed PMID: 28462122; PubMed Central PMCID: PMCPMC5390432. doi:10.1016/j.imr.2016.05.006
Papaconstantinou, J. (2019). The role of signaling pathways of inflammation and oxidative stress in development of senescence and aging phenotypes in cardiovascular disease. Cells 8 (11), E1383. Epub 2019/11/07PubMed PMID: 31689891; PubMed Central PMCID: PMCPMC6912541. doi:10.3390/cells8111383
Park, D., Bae, D. K., Jeon, J. H., Lee, J., Oh, N., Yang, G., et al. (2011). Immunopotentiation and antitumor effects of a ginsenoside Rg₃-fortified red ginseng preparation in mice bearing H460 lung cancer cells. Environ. Toxicol. Pharmacol. 31 (3), 397–405. Epub 2011/07/27PubMed PMID: 21787710. doi:10.1016/j.etap.2011.01.008
Park, J. Y., Choi, P., Kim, T., Ko, H., Kim, H. K., Kang, K. S., et al. (2015). Protective effects of processed ginseng and its active ginsenosides on cisplatin-induced nephrotoxicity: In vitro and in vivo studies. J. Agric. Food Chem. 63 (25), 5964–5969. Epub 2015/06/09PubMed PMID: 26050847. doi:10.1021/acs.jafc.5b00782
Park, S. M., Choi, M. S., Sohn, N. W., and Shin, J. W. (2012). Ginsenoside Rg3 attenuates microglia activation following systemic lipopolysaccharide treatment in mice. Biol. Pharm. Bull. 35 (9), 1546–1552. Epub 2012/09/15PubMed PMID: 22975507. doi:10.1248/bpb.b12-00393
Prasad, S., Gupta, S. C., and Tyagi, A. K. (2017). Reactive oxygen species (ROS) and cancer: Role of antioxidative nutraceuticals. Cancer Lett. 387, 95–105. Epub 2016/04/03PubMed PMID: 27037062. doi:10.1016/j.canlet.2016.03.042
Racanelli, A. C., Kikkers, S. A., Choi, A. M. K., and Cloonan, S. M. (2018). Autophagy and inflammation in chronic respiratory disease. Autophagy 14 (2), 221–232. Epub 2017/11/14PubMed PMID: 29130366; PubMed Central PMCID: PMCPMC5902194. doi:10.1080/15548627.2017.1389823
Ramos-Tovar, E., and Muriel, P. (2020). Molecular mechanisms that link oxidative stress, inflammation, and fibrosis in the liver. Antioxidants (Basel, Switz. 9 (12), E1279. Epub 2020/12/19PubMed PMID: 33333846; PubMed Central PMCID: PMCPMC7765317. doi:10.3390/antiox9121279
Ren, B., Feng, J., Yang, N., Guo, Y., Chen, C., Qin, Q., et al. (2021). Ginsenoside Rg3 attenuates angiotensin II-induced myocardial hypertrophy through repressing NLRP3 inflammasome and oxidative stress via modulating SIRT1/NF-κB pathway. Int. Immunopharmacol. 98, 107841. Epub 2021/06/22PubMed PMID: 34153662. doi:10.1016/j.intimp.2021.107841
Robb, C. T., Regan, K. H., Dorward, D. A., and Rossi, A. G. (2016). Key mechanisms governing resolution of lung inflammation. Semin. Immunopathol. 38 (4), 425–448. Epub 2016/04/28PubMed PMID: 27116944; PubMed Central PMCID: PMCPMC4896979. doi:10.1007/s00281-016-0560-6
Shi, Y., Wang, H., Zheng, M., Xu, W., Yang, Y., Shi, F., et al. (2020). Ginsenoside Rg3 suppresses the NLRP3 inflammasome activation through inhibition of its assembly. FASEB J. official Publ. Fed. Am. Soc. Exp. Biol. 34 (1), 208–221. Epub 2020/01/10PubMed PMID: 31914640. doi:10.1096/fj.201901537R
Shin, S. H., Ye, M. K., Lee, D. W., Kang, B. J., and Chae, M. H. (2021). Effect of Korean red ginseng and Rg3 on asian sand dust-induced MUC5AC, MUC5B, and MUC8 expression in bronchial epithelial cells. Mol. (Basel, Switz. 26 (7), 2002. Epub 2021/05/01PubMed PMID: 33916022; PubMed Central PMCID: PMCPMC8037637. doi:10.3390/molecules26072002
Shin, Y. M., Jung, H. J., Choi, W. Y., and Lim, C. J. (2013). Antioxidative, anti-inflammatory, and matrix metalloproteinase inhibitory activities of 20(S)-ginsenoside Rg3 in cultured mammalian cell lines. Mol. Biol. Rep. 40 (1), 269–279. Epub 2012/10/12PubMed PMID: 23054007. doi:10.1007/s11033-012-2058-1
Silva, D., Baião, D. D. S., Ferreira, V. F., and Paschoalin, V. M. F. (2022). Betanin as a multipath oxidative stress and inflammation modulator: A beetroot pigment with protective effects on cardiovascular disease pathogenesis. Crit. Rev. Food Sci. Nutr. 62 (2), 539–554. Epub 2020/10/01PubMed PMID: 32997545. doi:10.1080/10408398.2020.1822277
Singh, H., Du, J., Singh, P., Mavlonov, G. T., and Yi, T. H. (2018). Development of superparamagnetic iron oxide nanoparticles via direct conjugation with ginsenosides and its in-vitro study. J. Photochem. Photobiol. B 185, 100–110. Epub 2018/06/10PubMed PMID: 29885646. doi:10.1016/j.jphotobiol.2018.05.030
Song, M., Jia, F., Cao, Z., Zhang, H., Liu, M., Gao, L., et al. (2020). Ginsenoside Rg3 attenuates aluminum-induced osteoporosis through regulation of oxidative stress and bone metabolism in rats. Biol. Trace Elem. Res. 198 (2), 557–566. Epub 2020/03/17PubMed PMID: 32173789. doi:10.1007/s12011-020-02089-9
Sun, M., Ye, Y., Xiao, L., Duan, X., Zhang, Y., Zhang, H., et al. (2017). Anticancer effects of ginsenoside Rg3 (review). Int. J. Mol. Med. 39 (3), 507–518. Epub 2017/01/19PubMed PMID: 28098857. doi:10.3892/ijmm.2017.2857
Tangrodchanapong, T., Sobhon, P., and Meemon, K. (2020). Frondoside A attenuates amyloid-β proteotoxicity in transgenic Caenorhabditis elegans by suppressing its formation. Front. Pharmacol. 11, 553579. Epub 2020/10/06PubMed PMID: 33013392; PubMed Central PMCID: PMCPMC7513805. doi:10.3389/fphar.2020.553579
Tian, J., Zhang, S., Li, G., Liu, Z., and Xu, B. (2009). 20(S)-ginsenoside Rg3, a neuroprotective agent, inhibits mitochondrial permeability transition pores in rat brain. Phytother. Res. 23 (4), 486–491. Epub 2008/11/13PubMed PMID: 19003949. doi:10.1002/ptr.2653
Tu, C., Wan, B., and Zeng, Y. (2020). Ginsenoside Rg3 alleviates inflammation in a rat model of myocardial infarction via the SIRT1/NF-κB pathway. Exp. Ther. Med. 20 (6), 238. Epub 2020/11/17PubMed PMID: 33193843; PubMed Central PMCID: PMCPMC7646702. doi:10.3892/etm.2020.9368
Wang, J., Yu, X. F., Zhao, J. J., Shi, S. M., Fu, L., Sui, D. Y., et al. (2016). Ginsenoside Rg3 attenuated omethoate-induced lung injury in rats. Hum. Exp. Toxicol. 35 (6), 677–684. Epub 2015/08/05PubMed PMID: 26240163. doi:10.1177/0960327115597984
Wang, X., Chen, L., Wang, T., Jiang, X., Zhang, H., Li, P., et al. (2015). Ginsenoside Rg3 antagonizes adriamycin-induced cardiotoxicity by improving endothelial dysfunction from oxidative stress via upregulating the Nrf2-ARE pathway through the activation of akt. Phytomedicine 22 (10), 875–884. Epub 2015/09/01PubMed PMID: 26321736. doi:10.1016/j.phymed.2015.06.010
Wang, Y., Hu, Z., Sun, B., Xu, J., Jiang, J., Luo, M., et al. (2015). Ginsenoside Rg3 attenuates myocardial ischemia/reperfusion injury via Akt/endothelial nitric oxide synthase signaling and the Bcell lymphoma/Bcell lymphomaassociated X protein pathway. Mol. Med. Rep. 11 (6), 4518–4524. Epub 2015/02/13PubMed PMID: 25672441. doi:10.3892/mmr.2015.3336
Xing, W., Yang, L., Peng, Y., Wang, Q., Gao, M., Yang, M., et al. (2017). Ginsenoside Rg3 attenuates sepsis-induced injury and mitochondrial dysfunction in liver via AMPK-mediated autophagy flux. Biosci. Rep. 37 (4), BSR20170934. Epub 2017/08/06PubMed PMID: 28779013; PubMed Central PMCID: PMCPMC5577177. doi:10.1042/bsr20170934
Yang, J., Li, S., Wang, L., Du, F., Zhou, X., Song, Q., et al. (2018). Ginsenoside Rg3 attenuates lipopolysaccharide-induced acute lung injury via MerTK-dependent activation of the PI3K/AKT/mTOR pathway. Front. Pharmacol. 9, 850. Epub 2018/08/18PubMed PMID: 30116194; PubMed Central PMCID: PMCPMC6082957. doi:10.3389/fphar.2018.00850
Yoon, S. J., Park, J. Y., Choi, S., Lee, J. B., Jung, H., Kim, T. D., et al. (2015). Ginsenoside Rg3 regulates S-nitrosylation of the NLRP3 inflammasome via suppression of iNOS. Biochem. Biophys. Res. Commun. 463 (4), 1184–1189. Epub 2015/06/19PubMed PMID: 26086107. doi:10.1016/j.bbrc.2015.06.080
Zhang, J. J., Zhou, Y. D., Liu, Y. B., Wang, J. Q., Li, K. K., Gong, X. J., et al. (2021). Protective effect of 20(R)-Ginsenoside Rg3 against cisplatin-induced renal toxicity via PI3K/AKT and NF-[Formula: See text]B signaling pathways based on the premise of ensuring anticancer effect. Am. J. Chin. Med. 49 (7), 1739–1756. Epub 2021/09/01PubMed PMID: 34461812. doi:10.1142/s0192415x21500828
Zhang, K., Liu, Y., Wang, C., Li, J., Xiong, L., Wang, Z., et al. (2019). Evaluation of the gastroprotective effects of 20 (S)-ginsenoside Rg3 on gastric ulcer models in mice. J. Ginseng Res. 43 (4), 550–561. Epub 2019/11/07PubMed PMID: 31695563; PubMed Central PMCID: PMCPMC6823781. doi:10.1016/j.jgr.2018.04.001
Zhang, L. P., Jiang, Y. C., Yu, X. F., Xu, H. L., Li, M., Zhao, X. Z., et al. (2016). Ginsenoside Rg3 improves cardiac function after myocardial ischemia/reperfusion via attenuating apoptosis and inflammation. Evidence-based complementary Altern. Med. 2016, 6967853. Epub 2017/01/21PubMed PMID: 28105061; PubMed Central PMCID: PMCPMC5220470. doi:10.1155/2016/6967853
Zhang, Q. H., Wu, C. F., Duan, L., and Yang, J. Y. (2008). Protective effects of ginsenoside Rg(3) against cyclophosphamide-induced DNA damage and cell apoptosis in mice. Arch. Toxicol. 82 (2), 117–123. Epub 2007/06/29PubMed PMID: 17598087. doi:10.1007/s00204-007-0224-3
Zhao, M., Zhang, B., and Deng, L. (2022). The mechanism of acrylamide-induced neurotoxicity: Current status and future perspectives. Front. Nutr. 9, 859189. PubMed PMID: 35399689. doi:10.3389/fnut.2022.859189
Zhou, T., Sun, L., Yang, S., Lv, Y., Cao, Y., Gang, X., et al. (2020). 20(S)-Ginsenoside Rg3 protects kidney from diabetic kidney disease via renal inflammation depression in diabetic rats. J. Diabetes Res. 2020, 7152176. Epub 2020/04/08PubMed PMID: 32258169; PubMed Central PMCID: PMCPMC7106937 of this article. doi:10.1155/2020/7152176
Zhou, Y. D., Hou, J. G., Liu, W., Ren, S., Wang, Y. P., Zhang, R., et al. (2018). 20(R)-ginsenoside Rg3, a rare saponin from red ginseng, ameliorates acetaminophen-induced hepatotoxicity by suppressing PI3K/AKT pathway-mediated inflammation and apoptosis. Int. Immunopharmacol. 59, 21–30. Epub 2018/04/06PubMed PMID: 29621733. doi:10.1016/j.intimp.2018.03.030
Keywords: ginsenoside Rg3, oxidative stress, inflammation, molecular mechanism, therapeutic effect
Citation: Wang J, Zeng L, Zhang Y, Qi W, Wang Z, Tian L, Zhao D, Wu Q, Li X and Wang T (2022) Pharmacological properties, molecular mechanisms and therapeutic potential of ginsenoside Rg3 as an antioxidant and anti-inflammatory agent. Front. Pharmacol. 13:975784. doi: 10.3389/fphar.2022.975784
Received: 22 June 2022; Accepted: 14 July 2022;
Published: 05 September 2022.
Edited by:
Dongdong Sun, Nanjing University of Chinese Medicine, ChinaReviewed by:
Dayun Sui, Department of Pharmacology, Jilin University, ChinaChanggang Sun, Affiliated Hospital of Weifang Medical University, China
Copyright © 2022 Wang, Zeng, Zhang, Qi, Wang, Tian, Zhao, Wu, Li and Wang. This is an open-access article distributed under the terms of the Creative Commons Attribution License (CC BY). The use, distribution or reproduction in other forums is permitted, provided the original author(s) and the copyright owner(s) are credited and that the original publication in this journal is cited, in accordance with accepted academic practice. No use, distribution or reproduction is permitted which does not comply with these terms.
*Correspondence: Qibiao Wu, qbwu@must.edu.mo; Xiangyan Li, xiangyan_li1981@163.com; Tan Wang, wangtanccutcm@outlook.com
†These authors have contributed equally to this work