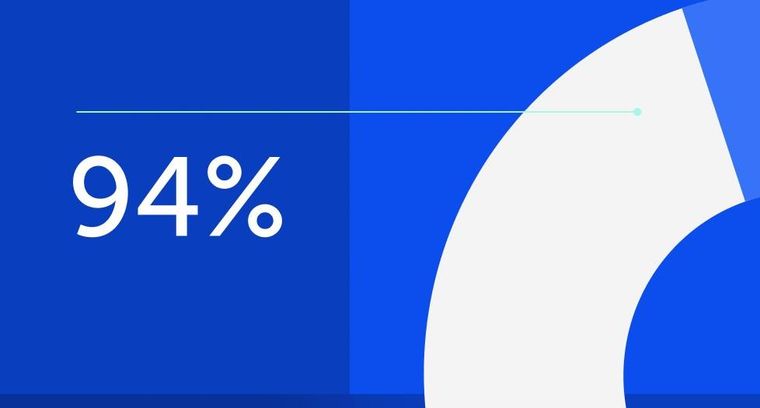
94% of researchers rate our articles as excellent or good
Learn more about the work of our research integrity team to safeguard the quality of each article we publish.
Find out more
REVIEW article
Front. Pharmacol., 07 October 2022
Sec. Translational Pharmacology
Volume 13 - 2022 | https://doi.org/10.3389/fphar.2022.975181
This article is part of the Research TopicWomen in Translational Pharmacology: 2021View all 6 articles
Melatonin is an important endogenous hormone that modulates homeostasis in the microenvironment. Recent studies have indicated that serum melatonin levels are closely associated with the occurrence and development of osteoporosis in postmenopausal women. Exogenous melatonin could also improve bone mass and increase skeletal strength. To determine the underlying mechanisms of melatonin in the prevention and treatment of postmenopausal osteoporosis, we performed this review to analyze the role of melatonin in bone metabolism according to its physiological functions. Serum melatonin is related to bone mass, the measurement of which is a potential method for the diagnosis of osteoporosis. Melatonin has a direct effect on bone remodeling by promoting osteogenesis and suppressing osteoclastogenesis. Melatonin also regulates the biological rhythm of bone tissue, which benefits its osteogenic effect. Additionally, melatonin participates in the modulation of the bone microenvironment. Melatonin attenuates the damage induced by oxidative stress and inflammation on osteoblasts and prevents osteolysis from reactive oxygen species and inflammatory factors. As an alternative drug for osteoporosis, melatonin can improve the gut ecology, remodel microbiota composition, regulate substance absorption and maintain metabolic balance, all of which are beneficial to the health of bone structure. In conclusion, our review systematically demonstrates the effects of melatonin on bone metabolism. Based on the evidence in this review, melatonin will play a more important role in the diagnosis, prevention and treatment of postmenopausal osteoporosis.
Postmenopausal osteoporosis is a common disease of bone metabolism occurring in women after amenorrhea (Farlay et al., 2022). The onset of osteoporosis is insidious without specific symptoms. It is usually diagnosed after the occurrence of serious complications, including pain, spinal deformity and fracture (Chow et al., 1989). Osteoporotic fracture is the main factor threatening the health of patients with osteoporosis. Previous studies have indicated that 20% of women suffer from osteoporosis and 10% of these women have fractures in different sites (Wang et al., 2021). Early diagnosis and treatment are essential to prevent osteoporosis complications. At present, dual energy X-ray absorptiometry (DXA) is the gold standard for the detection of bone mineral density. However, DXA test results have obvious limitations for the diagnosis and treatment of osteoporosis. In terms of localization, DXA generally detects the bone density of the waist and hip, but it cannot determine the distribution and heterogeneity of the bone (Silva et al., 2013; Cataño Jimenez et al., 2020). Additionally, DXA is unable to detect the trabecular bone microstructure and predict the risk of fractures (Nazarian et al., 2009). More importantly, DXA testing equipment is expensive, and it is difficult to apply to the whole population, resulting in the omission of patient screening. The development of complementary assays to assess systemic bone mass and fracture risk is essential.
Postmenopausal women are the main high-risk group for osteoporosis (Liu et al., 2022a). The core pathogenesis of postmenopausal osteoporosis is estrogen deficiency. Hormone replacement therapy has been applied in the treatment of osteoporosis. However, exogenous hormones disrupt endocrine homeostasis and increase the risk of breast cancer, endometrial cancer and cholelithiasis (Zhang et al., 2021a). Drug therapy for osteoporosis is divided into two types: promotion of osteogenesis and inhibition of osteoclasts, with the latter being the mainstay (Zhou et al., 2020a). Due to the limitations of detection methods and the lack of awareness during a physical examination, patients with osteoporosis often undergo drug intervention after serious complications occur. Drugs for inhibiting osteoclasts only prevent further bone loss but do not fundamentally improve bone mass. Osteogenesis drugs such as teriparatide can cause endocrine disturbances, gastrointestinal irritation and central nervous system lesions. The development of mild osteogenic drugs is an effective measure to address the limitations of current drug treatments for osteoporosis. In addition, revealing the pathophysiological changes induced by estrogen decline will contribute to determining the pathogenesis and clinical treatment of postmenopausal osteoporosis.
Melatonin is a hormone secreted by the pineal gland. Its functions include adjusting biological clock rhythm, eliminating free radicals, delaying aging and enhancing immunity (Zhang et al., 2020; Guo et al., 2021a; Li et al., 2021a; Zhang et al., 2021b). Melatonin is used as a component of nutraceuticals to maintain body health due to its limited side effects. At present, melatonin is mainly applied to people with sleep disorders and insomnia (da Silveira Cruz-Machado et al., 2021). Melatonin effectively shortened the time to fall asleep, reduced the time of light sleep, increased the time of deep sleep, improved sleep quality, and helped people stay awake after waking up. Additionally, there is good evidence that melatonin can be used to treat ischemia-reperfusion injury, primary headache, and fibromyalgia and can control blood sugar and blood pressure (Leelaviwat et al., 2022). Melatonin is increasingly valued by patients and clinicians due to its antioxidant and antiaging properties. In our previous study, we determined that serum melatonin levels were obviously decreased in postmenopausal women with osteoporosis (Cao et al., 2022). Changes in serum melatonin were significantly correlated with bone metabolism markers in the development of postmenopausal osteoporosis (Ostrowska et al., 2001a). This evidence indicated the potential role of melatonin in the evaluation of bone mass and strength. Additionally, our previous studies also revealed the positive effect of melatonin on osteoblasts and its therapeutic effect in postmenopausal osteoporosis in animals (Da et al., 2020; Wen et al., 2020). Oral administration of melatonin could increase the serum level of melatonin to improve bone mass (Guan et al., 2022). Melatonin decreased the relative ratio of serum osteoclasts and osteoblasts to improve bone balance (Kotlarczyk et al., 2012; Maria et al., 2018). It is obvious that melatonin plays an important role in bone metabolism. In addition to the direct effect on bone cells, some studies also indicated that melatonin could regulate bone homeostasis in different indirect ways (Xu et al., 2018; Zhou et al., 2019). Melatonin is a mild osteogenic drug with few complications and has the potential and prospect of being an effective drug for the treatment of osteoporosis. Clarification of the role of melatonin in resisting the pathological changes caused by estrogen deficiency and the optimization of the drug formulation of melatonin for the treatment of osteoporosis are promising research directions. Therefore, we aim to review the studies that investigated the role of melatonin in the development of postmenopausal osteoporosis, elucidate the mechanism by which melatonin improves bone metabolism, and provide insights into the application of melatonin in osteoporosis treatment.
The PubMed, Web of Science, Ei Compendex and Wiley databases were used to search the research literature on melatonin and postmenopausal osteoporosis. We searched any identified studies including reviews, articles, early access, editorial materials, and letters. The results comprised papers available from the inception of the database to July 2022. Search terms included Osteoblast, Osteoclast, Melatonin, Osteogenesis, Osteogenic differentiation, Osteoclast differentiation, Biological rhythms, Antioxidant effect, Anti-inflammation, Immuomodulation, Gut, Gut microbiota, and Postmenopausal osteoporosis. The abstract or full text of these studies was reviewed to check whether they matched the corresponding section.
Osteoblasts differentiate from the mesenchymal stem cells in bone marrow (BMSCs) (Sun et al., 2022). Previous studies have indicated that there are two opposite trends of BMSC differentiation that result from the balance between osteogenesis and adipogenesis (Wang et al., 2022a; Suo et al., 2022). Melatonin could increase the expression of osteogenic markers of mesenchymal stem cells and contribute to the mineralization of the bone matrix (Guan et al., 2022). Melatonin promotes the osteogenic differentiation of BMSCs via the Wnt/β-catenin pathway and inhibits the adipogenic differentiation of BMSCs via the PPARγ pathway (Zhang et al., 2010; Han et al., 2021). In pathological conditions, melatonin could also resist cell damage and prevent osteoblast function (Zhao et al., 2020; Gong et al., 2022). As mentioned above, melatonin is a potential osteogenic promoter in bone metabolism. Melatonin combined with the MT2 receptor could induce signaling transduction in osteogenesis and promote ossification (Zhang et al., 2021c). However, MT2 activation also suppresses osteoclastogenesis activity by inactivating the NF-kappaB pathway (Zhou et al., 2020b). In coculture conditions, exogenous melatonin promotes the osteogenesis of mesenchymal stem cells and inhibits the osteoclastogenesis of peripheral blood monocytes via the MT2-mediated MEK1/2 and MEK5 pathways (Maria et al., 2018). Additionally, melatonin decreased the expression of RANKL and further suppressed the activity of osteoclasts via the receptor-independent MARK and NFATc1 pathway (Kim et al., 2017; Kim et al., 2022) (Figure 1).
Melatonin also modulated the estrogen receptor on osteoclasts to maintain the inhibitory effect on osteoclast differentiation (Suzuki and Hattori, 2002). In vivo experiments, estrogen-deficient mice were obtained by bilateral ovariectomy (OVX). Melatonin feeding could improve bone mass and relieve bone loss in OVX mice (Gürler et al., 2019). Histological detection of bone tissues indicated that melatonin increases the expression of Collagen I and BMP2 but decreases the expression of PRMT1 and TRAP (Choi et al., 2021; Huang et al., 2021). These data demonstrate that melatonin has positive effects on osteogenesis and negative effects on osteoclastogenesis in animal models, which is consistent with the results at the cellular level. In conclusion, melatonin plays an important role in bone balance through direct regulation.
The direct mechanism of melatonin in regulating bone metabolism is summarized and listed in Table 1.
Biorhythm plays an important role in the development of postmenopausal osteoporosis. A recent study showed that the endochondral bone formation process exhibits a biological rhythm characterized by rapid DNA replication and active cellular mitosis during the day, followed by matrix synthesis at night (Yu et al., 2022). The circadian clock regulates the biological cycle of mammalian physiological functions. Biological regulation maintains energy metabolism in bone tissue, including the metabolism of glucose, amino acid and fat (Luo et al., 2021).
Bone metabolism markers were secreted in a special circadian rhythm (Zheng et al., 2021). Rhythmic regulation of bone metabolism hormones, such as parathyroid hormone and growth hormone, maintains the homeostasis of calcium, phosphate, Collagen I peptide and 1,25-dihydroxyvitamin D in bone tissue (Joseph et al., 2008). Circadian clock disturbance led to the disorder of bone metabolism when clock-related genes were knocked out or sleep restriction occurred (Song et al., 2018). As the circadian clock was disturbed in postmenopausal women, the process of bone formation, energy metabolism and the expression of turnover markers in bone tissue, as well as the secretion of bone metabolic hormones were disordered (Kruijver and Swaab, 2002). Melatonin is a type of rhythmic hormone. Its secretion increases with weak light stimulation at night but decreases in the daytime (Carstensen et al., 2022). The rhythmic regulation of melatonin affected the metabolism of nutrients, including carbohydrates, lipids and proteins, indicating the important role of melatonin in maintaining body functions (Kent et al., 2022; Qian et al., 2022). A previous clinical study demonstrated the protective effect of melatonin in rhythmic secretion on bone metabolism in postmenopausal women (Ostrowska et al., 2001b).
The circadian levels of Collagen I biomarkers were suppressed when melatonin concentrations were altered during the day in postmenopausal women. In addition, serum carboxyterminal telopeptide of type I collagen (ICTP) together with urinary hydroxyproline and Ca was negatively correlated with the serum melatonin level (Ostrowska et al., 2001a). Melatonin also improved bone mass in a rhythmic regulatory method by exogenous supplementation in addition to its relationship in physiological and pathological conditions. Circadian clock genes were closely associated with bone metabolism (Table 2). CLOCK gene mutations inhibit the combination of 1,2,5(OH)2D3 and the PDIA3 receptor, which is involved in bone remodeling (Yuan et al., 2017). Melatonin increased the expression of CLOCK (Wan et al., 2020). As the downstream target of CLOCK, BMAL1 also led to the occurrence of osteoporosis when the factor was defective (Chen et al., 2020a). Inactivation of BMAL1 suppressed the differentiation of osteoblasts and enhanced the function of osteoclasts. Melatonin induced AMPKβ1 phosphorylation to increase BMAL1 expression by activating melatonin receptor 1 (Yu et al., 2022). REV-ERB/ROR is a group of response elements that modulate the expression of BMAL1 (Lee et al., 2016). Melatonin suppressed osteoclastogenesis by increasing the expression of REV-ERB (Tian et al., 2021). As mentioned above, the melatonin level is closely related to the expression of bone metabolism biomarkers. Disruptions in the circadian rhythm of melatonin secretion lead to an imbalance in bone remodeling, and exogenous melatonin plays a positive role in bone homeostasis by modulating circadian clock genes and factors.
Estrogen is a type of antioxidant hormone that regulates the function of mitochondria to maintain the activity of oxidase and the production of oxidants (Miyazaki-Akita et al., 2007; Yung et al., 2011). Disturbances in the antioxidant systems lead to imbalances in bone metabolism (Yang et al., 2022a). Postmenopausal osteoporosis is a disease caused by oxidation-reduction disorders due to estrogen deficiency (Yang et al., 2021). On the one hand, oxidative stress weakens the function of osteogenesis (Lian et al., 2021). On the other hand, reactive oxygen species (ROS) signals could activate RANKL-mediated osteoclastogenesis (Li et al., 2022). Both effects resulted in bone mass loss in bone tissue.
Melatonin is the most powerful endogenous free radical scavenger. Its main antioxidant mechanism acts through its binding to oxidative substances (Lu et al., 2022). Additionally, melatonin binds to its cellular receptor and activates the transduction signals that induce the synthesis of antioxidant enzymes, including superoxide dismutase, catalase, glutathione peroxidase, and glutathione reductase (Bantounou et al., 2022). To prevent oxidative damage, melatonin suppresses the cellular senescence induced by oxidative stress in bone marrow mesenchymal stem cells to maintain the osteogenic differentiation (Chen et al., 2022). Melatonin also increases the expression of SIRT1, which is a deacetylase that is closely involved in mitochondrial biosynthesis to improve oxidative damage in osteoblasts (Chen et al., 2020b; Liu et al., 2022b). In addition, melatonin promotes SIRT3-mediated antioxidase production to hydrolyze free radicals and thereby protect osteoblasts against apoptosis (Zhou et al., 2019; Xiao et al., 2020). For the regulation of osteoclast activity, melatonin could suppress ROS-induced osteoclast differentiation and aging-mediated bone loss (Zhou et al., 2017; Tao et al., 2020). The nuclear factor Nrf2 plays an important role in oxidative-reductive signaling pathway-mediated bone remodeling (Han et al., 2022). Activation of Nrf2 not only maintains the survival of osteoblasts and mesenchymal stem cells but also inhibits osteoclast differentiation (Su et al., 2021; Wang et al., 2022b).
Melatonin increases the expression of Nrf2 to promote osteogenic differentiation via the Wnt/β-catenin pathway and inhibits osteolysis by enhancing the activity of catalase (Vriend and Reiter, 2016; Zhu et al., 2020). Heme oxygenase 1 (HO-1) is an essential downstream target of Nrf2 in the process of regulating the oxidation-reduction response (Ma et al., 2019). HO-1 was regarded as the potential target for postmenopausal osteoporosis based on its physiological function of controlling carbon monoxide and ferritin iron to prevent cell apoptosis in bone tissue (Zhou et al., 2021). Melatonin is an agonist of the Nrf2/HO-1 signaling pathway (Yang et al., 2022b; Zhou et al., 2022). Melatonin also exerted antioxidant functions by activating the Nrf2/HO-1 signal to enhance intracellular antioxidant reactions (Guo et al., 2021b). In conclusion, melatonin plays an important role in the protection of bone metabolism from oxidative damage by enhancing the activity of antioxidase and scavenging free radicals via the Nrf2/HO-1 signaling pathway.
Targets and signaling pathways modulated by melatonin are listed in Table 3 to reveal the antioxidant effect of melatonin in bone balance.
TABLE 3. Targets and signaling pathways of melatonin exerting antioxidant effects in bone metabolism.
Estrogen has potential anti-inflammatory properties. Studies have demonstrated that estrogen receptor participates in the activation and proliferation of T lymphocytes, and estrogen also suppressed the production of proinflammatory cytokines by activating the NF-κB signaling pathway (Mohammad et al., 2018; Harding and Heaton, 2022). Therefore, postmenopausal osteoporosis has been regarded as a type of inflammatory disease (McLean, 2009). Immunological homeostasis also played an important role in the maintenance of bone balance. First, mononuclear macrophages are the precursors of osteoclasts. After combining with estrogen receptor α (ERα), estrogen inhibits the differentiation of mononuclear macrophages into osteoclast by inhibiting the secretion of IL-1β, TNF-α and IL-6 (Riggs, 2000). Estrogen could also reduce the secretion of IL-17 and RANKL, which promote osteoclast differentiation from CD4 T cells (Okamoto et al., 2017). Estrogen deficiency decreased the production of TGF-β, which could suppress osteoclasts with the inhibitory effect of IFNγ and TNF-α in T cells. Additionally, estrogen promoted the production of efficient B lymphopoiesis in bone marrow and reduced osteoclast differentiation by increasing OPG expression (Masuzawa et al., 1994; Fujiwara et al., 2016). The systemic immune-inflammation index is an important index for the prediction and diagnosis of bone mass disorders in postmenopausal women (Du et al., 2021).
Melatonin was revealed to inhibit the inflammatory response in recent studies (Xi et al., 2021; Zakria et al., 2021). Its anti-inflammatory mechanism mainly acts through the inhibition of inflammasome formation and the abolishment of proinflammatory factor expression (Liu et al., 2022c; Xiong et al., 2022). Melatonin promotes osteogenic differentiation via the Wnt/β-catenin pathway and suppresses the inhibitory effect of NF-κB on osteogenesis in an inflammatory environment (Li et al., 2019). Melatonin also overcame the IL-1β-induced weakening of the osteogenic capacity of mesenchymal stem cells and tumor necrosis factor-alpha (TNF-α) by increasing bone resorption of osteoclasts to improve inflammation-related osteoporosis (Liu et al., 2013; Lian et al., 2016).
Immune function is a necessary factor in the regulation of the inflammatory state of the body. Osteoimmunology is becoming the focus of research on bone metabolic diseases (Xu et al., 2021; Fischer and Haffner-Luntzer, 2022). Melatonin plays an important role in immune organs homeostasis and maturation of immunocytes. Melatonin regulated the immune network composition of hematopoietic lineage cells in bone marrow (Maestroni, 1998). The survival of T cells in the thymus and B cells in bone marrow depended on melatonin regulation (Yu et al., 2000). The immune response was also affected by melatonin. Melatonin combined with the membrane receptor MT1 in the spleen to increase immune parameters such as the spleen mass and lymphocyte and leukocyte counts (Vishwas and Haldar, 2013). The most direct effect of melatonin on immune function was to modulate the response of immune cells in peripheral lymph nodes, especially T cells (Álvarez-Sánchez et al., 2015). Melatonin inhibited the immune response of effective T cells and enhanced the function of regulatory T cells. As the precursors of osteoclasts, monocyte-derived macrophages are the direct link between the immune and skeletal systems (Yang and Liu, 2021). Melatonin inhibits the osteoclastogenesis and migration of macrophages to suppress inflammation-mediated bone resorption (Markus et al., 2021; Kim et al., 2022). Melatonin also acted on other immunocytes to modulate bone metabolism indirectly. First, melatonin inhibited the proliferation and differentiation of Th1 and Th17 cells, reducing the secretion of IFNγ, IL-17 and TNF-α to improve bone mass (Srivastava et al., 2018; Huang et al., 2022). Immature B cells were associated with bone mass by increasing the RANKL/OPG ratio, but melatonin could promote B cell activation (Luo et al., 2020; Titanji et al., 2020). The mechanisms of immune cells and inflammatory factors mentioned above are summarized in Table 4. Therefore, clarifying the role of melatonin in the modulation of the immune-bone link could improve the inflammatory state and bone mass loss in postmenopausal women with osteoporosis.
TABLE 4. Mechanism of melatonin-regulated immune cells and inflammatory factors involved in bone metabolism.
The “gut-bone axis” is a new concept that has been proposed in recent studies. If refers to the influence of the gut microbiota on the absorption of nutrients, which alters the blood metabolism factors involved in bone remodeling (Fernández-Murga et al., 2020; Gobron et al., 2020). The gut microbiota play an important role in bone homeostasis (Li et al., 2021b). Detection of the intestinal flora abundance in OVX mice indicated that the ratio of Firmicutes to Bacteroidetes was higher than that of normal female mice (Wen et al., 2020). Disorders in the composition of the gut microbiota led to changes in substance metabolism that resulted in bone imbalances (Greenbaum et al., 2022). In addition, the gut microbiota can also improve bone mass by promoting osteogenesis and inhibiting osteoclasts (Ding et al., 2020). Special microbiota such as Bifidobacterium longum could also enhance the immunomodulatory potential of regulatory B cells to improve bone mass (Sapra et al., 2022).
Melatonin is an essential factor in the regulation of the gut microenvironment. Melatonin primarily protects against gut barrier defects to control substance absorption and improves the microbiome to influence substance metabolism (Jing et al., 2022). In terms of substance absorption, melatonin altered intestinal permeability by regulating the expression of the intestinal tight junction proteins ZO-1, occludin and claudin-1 (Liu et al., 2022d). For substance metabolism, melatonin also had a positive effect on the diversity of the gut microbiome (Zhang et al., 2022). Additionally, melatonin protected the intestinal tract from pathological damage. Melatonin increased the activity of antioxidases, such as SOD, GSH-Px and CAT, and prevented the expression of oxidative damage-induced gastrointestinal apoptosis factors. (Khan et al., 2017). Melatonin also improved intestinal inflammation by increasing the production of anti-inflammatory cytokines (IL-10 and IFN-γ) and reducing the production of proinflammatory cytokines (IL-6 and TNF-α) (Wang et al., 2022c).
It was found that melatonin could modulate the microbiota-dependent butyrate metabolism that prevents bone loss (Wu et al., 2021). Butyrate combined with its receptor GPR109A to alleviate osteolysis. In addition, increasing the absorption of Ca with the stimulation of melatonin directly contributes to the formation of the bone matrix (Areco et al., 2015). Melatonin improves the pathological state of the gut intestine by promoting Ca transportation through the transcellular and paracellular pathway (Diaz de Barboza et al., 2015). Melatonin adjusted gut metabolism and prevented gastric inflammation in OVX rats, which improved drug absorption for the treatment of osteoporosis (Gürler et al., 2019). Based on the evidence mentioned above, melatonin could improve the intestinal microenvironment, remodel the microbiota composition, regulate metabolism and absorption, and maintain the balance of bone tissue.
Osteoporosis is characterized by decreases in bone quality and bone mass loss, which mainly occurs in postmenopausal osteoporosis (Lim et al., 2021). Estrogen deficiency weakens the inhibitory effects on osteoclasts by activating estrogen receptor (Cheng et al., 2022). Bone resorption of osteoclasts is enhanced beyond the bone formation ability of osteoclasts, resulting in the development of osteoporosis (Heubel and Nohe, 2021). To make matters worse, the onset of osteoporosis is insidious and it is usually detected when serious complications occur instead of during routine physical examination (Salamanna et al., 2015). Although many drugs have been designed for the treatment of osteoporosis that mainly targeting osteoclasts, their effect is not satisfactory. In addition, the bone mass of the patient is significantly reduced, and the bone quality has typically been significantly decreased when osteoporosis is diagnosed. Therefore, optimizing diagnostic criteria, improving treatment plans, and developing more effective drugs are of great benefit to the early diagnosis, prevention and treatment of osteoporosis.
Melatonin is an endocrine hormone that is secreted by the pineal gland. With its rhythmic secretion characteristics, melatonin regulates many physiological functions of the body. In recent years, researchers have paid more attention to the regulatory effects of melatonin on bone tissue. Neuropathological changes such as sleep disturbance and depression often appeared and were thought to be highly related to melatonin in women after amenorrhea (Yardimci et al., 2021). Previous studies have indicated that melatonin could act on GnRH neurons to inhibit estrogen synthesis by influencing the PKA, PKC, and MAPK pathways (Roy and Belsham, 2002). Melatonin is also regarded as a selective estrogen enzyme modulator (Gonzalez et al., 2008). Although serum melatonin levels appear to be inversely correlated with estrogen levels under physiological conditions, nocturnal melatonin levels were significantly lower in postmenopausal osteoporosis (Toffol et al., 2014). Additionally, serum melatonin levels also show a close relationship with osteogenic markers (Ostrowska et al., 2002). Imbalances in the circadian clock that are mediated by disturbances in melatonin secretion increase the risk of osteoporotic fractures in postmenopausal women (Feskanich et al., 2009). Therefore, serum melatonin measurements might become a new diagnostic standard for the prediction of the risk of postmenopausal osteoporosis. In fact, they could replace conventional dual energy X-rays, which would reduce economic costs and improve detection efficiency.
In addition to early diagnosis, melatonin also plays a potential role in the treatment of osteoporosis. Melatonin treatment positive affected the increase in bone mineral density and the improvement in body mass index (Treister-Goltzman and Peleg, 2021). However, oral estrogen could inhibit nocturnal melatonin release in postmenopausal women (Okatani et al., 2000). The therapeutic effect of melatonin mainly included a direct effect on bone and an improvement in the pathological state after estrogen deficiency, rather than improvement in estrogen levels. As we demonstrated in this review, melatonin directly promotes osteogenesis and inhibits osteoclastogenesis. Melatonin regulated the differentiation of BMSCs toward osteogenesis but not adipogenesis via the Wnt/β-catenin pathway and PPARγ pathway. In animal experiments, melatonin markedly improved bone mass in ovariectomized mice (Cao et al., 2022). Additionally, melatonin improves the microenvironment of bone tissue in postmenopausal women. As shown in Figure 2, estrogen synthesized by the ovaries intervenes in the expression of OPG and RANKL between osteoblasts and osteoclasts to maintain the balance of bone formation and bone resorption. Estrogen deficiency reduces the ratio of OPG/RANKL and promotes osteoclast proliferation and differentiation. Estrogen deficiency changes the daynight rhythm (Blattner and Mahoney, 2014). Biological rhythm disorder results in pathological states, including oxidative stress and an inflammatory storm (Sehirli et al., 2021; Yoshitane et al., 2022). Circadian disturbances mediate oxidative and inflammatory damage that lead to an imbalance in bone metabolism (Ostrowska et al., 2003; Oršolić and Jazvinšćak Jembrek, 2022). Melatonin could correct the circadian rhythm by regulating CLOCK and BMAL1, prevent oxidative stress by increasing the activity of antioxidant enzymes and reducing ROS production, and inhibit inflammation by reducing the secretion of inflammatory factors from immunocytes in bone tissue (Lee et al., 2018; Ren et al., 2018; Lu et al., 2021; Sadaf et al., 2021). Finally, melatonin remodels the gut ecology to promote the absorption of Ca and osteogenic substances, which also benefits the formation of the bone matrix and the strength of the bone structure. Based on these positive effects on bone metabolism, melatonin is an alternative drug that could be effective for the treatment of osteoporosis.
FIGURE 2. The role of melatonin in bone metabolism (Created with BioRender.com).
At present, more than one-third of postmenopausal women are at risk of osteoporosis and its complications, which seriously affect their life and health. Current diagnostic modalities and known treatments have limited effectiveness in improving the situation. The exploration of new diagnosis and treatment options is imminent. As an endogenous hormone, melatonin has been shown to be closely related to the occurrence and development of osteoporosis. In addition, melatonin has considerable prospects in the treatment of osteoporosis because of its limited side effects. Rational use of the advantages of melatonin will contribute to the diagnosis, prevention and treatment of postmenopausal osteoporosis.
KY: Data curation, Formal analysis, Data curation, Methodology, Writing—original draft. XQ: Investigation, Methodology, Software, Validation, LC: Conceptualization, Software, Validation, Writing—review and editing, SQ: Funding acquisition, Project administration, Resources, Writing—review and editing. All authors read and approved the manuscript.
The authors declare that the research was conducted in the absence of any commercial or financial relationships that could be construed as a potential conflict of interest.
All claims expressed in this article are solely those of the authors and do not necessarily represent those of their affiliated organizations, or those of the publisher, the editors and the reviewers. Any product that may be evaluated in this article, or claim that may be made by its manufacturer, is not guaranteed or endorsed by the publisher.
Álvarez-Sánchez, N., Cruz-Chamorro, I., López-González, A., Utrilla, J. C., Fernandez-Santos, J. M., Martinez-Lopez, A., et al. (2015). Melatonin controls experimental autoimmune encephalomyelitis by altering the T effector/regulatory balance. Brain Behav. Immun. 50, 101–114. doi:10.1016/j.bbi.2015.06.021
Areco, V., Rivoira, M. A., Rodriguez, V., Marchionatti, A. M., Carpentieri, A., and Tolosa de Talamoni, N. (2015). Dietary and pharmacological compounds altering intestinal calcium absorption in humans and animals. Nutr. Res. Rev. 28 (2), 83–99. doi:10.1017/S0954422415000050
Bantounou, M., Plascevic, J., and Galley, H. F. (2022). Melatonin and related compounds: Antioxidant and anti-inflammatory actions. Antioxidants (Basel) 11 (3), 532. doi:10.3390/antiox11030532
Blattner, M. S., and Mahoney, M. M. (2014). Estrogen receptor 1 modulates circadian rhythms in adult female mice. Chronobiol. Int. 31 (5), 637–644. doi:10.3109/07420528.2014.885528
Cao, L., Yang, K., Yuan, W., Zhou, S., Zhao, R., and Qiu, S. (2022). Melatonin mediates osteoblast proliferation through the STIM1/ORAI1 pathway. Front. Pharmacol. 13, 851663. doi:10.3389/fphar.2022.851663
Carstensen, M. B., Medvetzky, A., Weinberger, A., Driever, W., Gothilf, Y., and Rath, M. F. (2022). Genetic ablation of the Bsx homeodomain transcription factor in zebrafish: Impact on mature pineal gland morphology and circadian behavior. J. Pineal Res. 72, e12795. doi:10.1111/jpi.12795
Cataño Jimenez, S., Saldarriaga, S., Chaput, C. D., and Giambini, H. (2020). Dual-energy estimates of volumetric bone mineral densities in the lumbar spine using quantitative computed tomography better correlate with fracture properties when compared to single-energy BMD outcomes. Bone 130, 115100. doi:10.1016/j.bone.2019.115100
Chen, G., Tang, Q., Yu, S., Xie, Y., Sun, J., Li, S., et al. (2020). The biological function of BMAL1 in skeleton development and disorders. Life Sci. 253, 117636. doi:10.1016/j.lfs.2020.117636
Chen, W., Chen, X., Chen, A. C., Shi, Q., Pan, G., Pei, M., et al. (2020). Melatonin restores the osteoporosis-impaired osteogenic potential of bone marrow mesenchymal stem cells by preserving SIRT1-mediated intracellular antioxidant properties. Free Radic. Biol. Med. 146, 92–106. doi:10.1016/j.freeradbiomed.2019.10.412
Chen, W., Lv, N., Liu, H., Gu, C., Zhou, X., Qin, W., et al. (2022). Melatonin improves the resistance of oxidative stress-induced cellular senescence in osteoporotic bone marrow mesenchymal stem cells. Oxid. Med. Cell. Longev. 2022, 7420726. doi:10.1155/2022/7420726
Cheng, C. H., Chen, L. R., and Chen, K. H. (2022). Osteoporosis due to hormone imbalance: An overview of the effects of estrogen deficiency and glucocorticoid overuse on bone turnover. Int. J. Mol. Sci. 23 (3), 1376. doi:10.3390/ijms23031376
Choi, J. H., Jang, A. R., Park, M. J., Kim, D. I., and Park, J. H. (2021). Melatonin inhibits osteoclastogenesis and bone loss in ovariectomized mice by regulating PRMT1-mediated signaling. Endocrinology 162 (6), bqab057. doi:10.1210/endocr/bqab057
Chow, R., Harrison, J., and Dornan, J. (1989). Prevention and rehabilitation of osteoporosis program: Exercise and osteoporosis. Int. J. Rehabil. Res. 12 (1), 49–56. doi:10.1097/00004356-198903000-00005
da Silveira Cruz-Machado, S., Guissoni Campos, L. M., Fadini, C. C., Anderson, G., Markus, R. P., and Pinato, L. (2021). Disrupted nocturnal melatonin in autism: Association with tumor necrosis factor and sleep disturbances. J. Pineal Res. 70 (3), e12715. doi:10.1111/jpi.12715
Da, W., Tao, L., Wen, K., Tao, Z., Wang, S., and Zhu, Y. (2020). Protective role of melatonin against postmenopausal bone loss via enhancement of citrate secretion from osteoblasts. Front. Pharmacol. 11, 667. doi:10.3389/fphar.2020.00667
Diaz de Barboza, G., Guizzardi, S., and Tolosa de Talamoni, N. (2015). Molecular aspects of intestinal calcium absorption. World J. Gastroenterol. 21 (23), 7142–7154. doi:10.3748/wjg.v21.i23.7142
Ding, K., Hua, F., and Ding, W. (2020). Gut microbiome and osteoporosis. Aging Dis. 11 (2), 438–447. doi:10.14336/AD.2019.0523
Du, Y. N., Chen, Y. J., Zhang, H. Y., Wang, X., and Zhang, Z. F. (2021). Inverse association between systemic immune-inflammation index and bone mineral density in postmenopausal women. Gynecol. Endocrinol. 37 (7), 650–654. doi:10.1080/09513590.2021.1885642
Farlay, D., Rizzo, S., Dempster, D. W., Huang, S., Chines, A., Brown, J. P., et al. (2022). Bone mineral and organic properties in postmenopausal women treated with denosumab for up to 10 years. J. Bone Min. Res. 37, 856–864. doi:10.1002/jbmr.4538
Fernández-Murga, M. L., Olivares, M., and Sanz, Y. (2020). Bifidobacterium pseudocatenulatum CECT 7765 reverses the adverse effects of diet-induced obesity through the gut-bone axis. Bone 141, 115580. doi:10.1016/j.bone.2020.115580
Feskanich, D., Hankinson, S. E., and Schernhammer, E. S. (2009). Nightshift work and fracture risk: The nurses' health study. Osteoporos. Int. 20 (4), 537–542. doi:10.1007/s00198-008-0729-5
Fischer, V., and Haffner-Luntzer, M. (2022). Interaction between bone and immune cells: Implications for postmenopausal osteoporosis. Semin. Cell Dev. Biol. 123, 14–21. doi:10.1016/j.semcdb.2021.05.014
Fujiwara, Y., Piemontese, M., Liu, Y., Thostenson, J. D., Xiong, J., and O'Brien, C. A. (2016). RANKL (receptor activator of NFκB ligand) produced by osteocytes is required for the increase in B cells and bone loss caused by estrogen deficiency in mice. J. Biol. Chem. 291 (48), 24838–24850. doi:10.1074/jbc.M116.742452
Gobron, B., Bouvard, B., Vyavahare, S., Blom, L. V., Pedersen, K. K., Windelov, J. A., et al. (2020). Enteroendocrine K cells exert complementary effects to control bone quality and mass in mice. J. Bone Min. Res. 35 (7), 1363–1374. doi:10.1002/jbmr.4004
Gong, Z., Da, W., Tian, Y., Zhao, R., Qiu, S., Wu, Q., et al. (2022). Exogenous melatonin prevents type 1 diabetes mellitus-induced bone loss, probably by inhibiting senescence. Osteoporos. Int. 33 (2), 453–466. doi:10.1007/s00198-021-06061-8
Gonzalez, A., Cos, S., Martinez-Campa, C., Alonso-Gonzalez, C., Sanchez-MateoS, S., Mediavilla, M. D., et al. (2008). Selective estrogen enzyme modulator actions of melatonin in human breast cancer cells. J. Pineal Res. 45 (1), 86–92. doi:10.1111/j.1600-079X.2008.00559.x
Greenbaum, J., Lin, X., Su, K. J., Gong, R., Shen, H., Shen, J., et al. (2022). Integration of the human gut microbiome and serum metabolome reveals novel biological factors involved in the regulation of bone mineral density. Front. Cell. Infect. Microbiol. 12, 853499. doi:10.3389/fcimb.2022.853499
Guan, H., Kong, N., Tian, R., Cao, R., Liu, G., Li, Y., et al. (2022). Melatonin increases bone mass in normal, perimenopausal, and postmenopausal osteoporotic rats via the promotion of osteogenesis. J. Transl. Med. 20 (1), 132. doi:10.1186/s12967-022-03341-7
Guo, S., Yang, J., Qin, J., Qazi, I. H., Pan, B., Zang, S., et al. (2021). Melatonin promotes in vitro maturation of vitrified-warmed mouse germinal vesicle oocytes, potentially by reducing oxidative stress through the Nrf2 pathway. Animals. 11 (8), 2324. doi:10.3390/ani11082324
Guo, Y. M., Sun, T. C., Wang, H. P., and Chen, X. (2021). Research progress of melatonin (MT) in improving ovarian function: A review of the current status. Aging (Albany NY) 13 (13), 17930–17947. doi:10.18632/aging.203231
Gürler, E. B., Çilingir-Kaya Ö, T., Peker Eyüboglu, I., Ercan, F., Akkiprik, M., Reiter, R. J., et al. (2019). Melatonin supports alendronate in preserving bone matrix and prevents gastric inflammation in ovariectomized rats. Cell biochem. Funct. 37 (2), 102–112. doi:10.1002/cbf.3379
Han, H., Tian, T., Huang, G., Li, D., and Yang, S. (2021). The lncRNA H19/miR-541-3p/Wnt/β-catenin axis plays a vital role in melatonin-mediated osteogenic differentiation of bone marrow mesenchymal stem cells. Aging (Albany NY) 13 (14), 18257–18273. doi:10.18632/aging.203267
Han, J., Yang, K., An, J., Jiang, N., Fu, S., and Tang, X. (2022). The role of NRF2 in bone metabolism - friend or foe? Front. Endocrinol. 13, 813057. doi:10.3389/fendo.2022.813057
Harding, A. T., and Heaton, N. S. (2022). The impact of estrogens and their receptors on immunity and inflammation during infection. Cancers (Basel) 14 (4), 909. doi:10.3390/cancers14040909
Heubel, B., and Nohe, A. (2021). The role of BMP signaling in osteoclast regulation. J. Dev. Biol. 9 (3), 24. doi:10.3390/jdb9030024
Huang, J., Li, Y., Wang, L., and He, C. (2021). Combined effects of low-frequency pulsed electromagnetic field and melatonin on ovariectomy-induced bone loss in mice. Bioelectromagnetics 42 (8), 616–628. doi:10.1002/bem.22372
Huang, J., Li, Z., Hu, Y., Li, Z., Xie, Y., Huang, H., et al. (2022). Melatonin, an endogenous hormone, modulates Th17 cells via the reactive-oxygen species/TXNIP/HIF-1α axis to alleviate autoimmune uveitis. J. Neuroinflammation 19 (1), 124. doi:10.1186/s12974-022-02477-z
Jing, W., Zhu, M., Wang, F., Zhao, X., Dong, S., Xu, Y., et al. (2022). Hyaluronic acid-melatonin nanoparticles improve the dysregulated intestinal barrier, microbiome and immune response in mice with dextran sodium sulfate-induced colitis. J. Biomed. Nanotechnol. 18 (1), 175–184. doi:10.1166/jbn.2022.3232
Joseph, F., Ahmad, A. M., Ul-Haq, M., Durham, B. H., Whittingham, P., Fraser, W. D., et al. (2008). Effects of growth hormone administration on bone mineral metabolism, PTH sensitivity and PTH secretory rhythm in postmenopausal women with established osteoporosis. J. Bone Min. Res. 23 (5), 721–729. doi:10.1359/jbmr.071117
Kent, B. A., Rahman, S. A., St Hilaire, M. A., Grant, L. K., Ruger, M., Czeisler, C. A., et al. (2022). Publisher Correction: Circadian lipid and hepatic protein rhythms shift with a phase response curve different than melatonin. Nat. Commun. 13 (1), 2241. doi:10.1038/s41467-022-29917-x
Khan, S., Adhikari, J. S., Rizvi, M. A., and Chaudhury, N. K. (2017). Melatonin attenuates (60) Co γ-ray-induced hematopoietic, immunological and gastrointestinal injuries in C57BL/6 male mice. Environ. Toxicol. 32 (2), 501–518. doi:10.1002/tox.22254
Kim, H. J., Kim, H. J., Bae, M. K., and Kim, Y. D. (2017). Suppression of osteoclastogenesis by melatonin: A melatonin receptor-independent action. Int. J. Mol. Sci. 18 (6), E1142. doi:10.3390/ijms18061142
Kim, S. S., Jeong, S. P., Park, B. S., and Kim, I. R. (2022). Melatonin attenuates RANKL-induced osteoclastogenesis via inhibition of Atp6v0d2 and DC-STAMP through MAPK and NFATc1 signaling pathways. Molecules 27 (2), 501. doi:10.3390/molecules27020501
Kotlarczyk, M. P., Lassila, H. C., O'Neil, C. K., D'Amico, F., Enderby, L. T., Witt-Enderby, P. A., et al. (2012). Melatonin osteoporosis prevention study (MOPS): A randomized, double-blind, placebo-controlled study examining the effects of melatonin on bone health and quality of life in perimenopausal women. J. Pineal Res. 52 (4), 414–426. doi:10.1111/j.1600-079X.2011.00956.x
Kruijver, F. P., and Swaab, D. F. (2002). Sex hormone receptors are present in the human suprachiasmatic nucleus. Neuroendocrinology 75 (5), 296–305. doi:10.1159/000057339
Lee, J., Lee, S., Chung, S., Park, N., Son, G. H., An, H., et al. (2016). Identification of a novel circadian clock modulator controlling BMAL1 expression through a ROR/REV-ERB-response element-dependent mechanism. Biochem. Biophys. Res. Commun. 469 (3), 580–586. doi:10.1016/j.bbrc.2015.12.030
Lee, S., Le, N. H., and Kang, D. (2018). Melatonin alleviates oxidative stress-inhibited osteogenesis of human bone marrow-derived mesenchymal stem cells through AMPK activation. Int. J. Med. Sci. 15 (10), 1083–1091. doi:10.7150/ijms.26314
Leelaviwat, N., Mekraksakit, P., Cross, K. M., Landis, D. M., McLain, M., Sehgal, L., et al. (2022). Melatonin: Translation of ongoing studies into possible therapeutic applications outside sleep disorders. Clin. Ther. 44 (5), 783–812. doi:10.1016/j.clinthera.2022.03.008
Li, J., Ho, W. T. P., Liu, C., Chow, S. K. H., Ip, M., Yu, J., et al. (2021). The role of gut microbiota in bone homeostasis. Bone Jt. Res. 10 (1), 51–59. doi:10.1302/2046-3758.101.BJR-2020-0273.R1
Li, J., Liang, J., Wu, L., Xu, Y., Xiao, C., Yang, X., et al. (2022). CYT387, a JAK-specific inhibitor impedes osteoclast activity and oophorectomy-induced osteoporosis via modulating RANKL and ROS signaling pathways. Front. Pharmacol. 13, 829862. doi:10.3389/fphar.2022.829862
Li, M., Hao, B., Zhang, M., Reiter, R. J., Lin, S., Zheng, T., et al. (2021). Melatonin enhances radiofrequency-induced NK antitumor immunity, causing cancer metabolism reprogramming and inhibition of multiple pulmonary tumor development. Signal Transduct. Target. Ther. 6 (1), 330. doi:10.1038/s41392-021-00745-7
Li, X., Li, Z., Wang, J., Li, Z., Cui, H., Dai, G., et al. (2019). Wnt4 signaling mediates protective effects of melatonin on new bone formation in an inflammatory environment. Faseb J. 33 (9), 10126–10139. doi:10.1096/fj.201900093RR
Lian, C., Wu, Z., Gao, B., Peng, Y., Liang, A., Xu, C., et al. (2016). Melatonin reversed tumor necrosis factor-alpha-inhibited osteogenesis of human mesenchymal stem cells by stabilizing SMAD1 protein. J. Pineal Res. 61 (3), 317–327. doi:10.1111/jpi.12349
Lian, W. S., Wu, R. W., Chen, Y. S., Ko, J. Y., Wang, S. Y., Jahr, H., et al. (2021). MicroRNA-29a mitigates osteoblast senescence and counteracts bone loss through oxidation resistance-1 control of FoxO3 methylation. Antioxidants (Basel) 10 (8), 1248. doi:10.3390/antiox10081248
Lim, H. J., Park, E. J., Won, Y. S., Bak, S. G., Cheong, S. H., Lee, S. W., et al. (2021). Anti-osteoporotic effects of n-trans-Hibiscusamide and its derivative alleviate ovariectomy-induced bone loss in mice by regulating RANKL-induced signaling. Molecules 26 (22), 6820. doi:10.3390/molecules26226820
Liu, H. D., Ren, M. X., Li, Y., Zhang, R. T., Ma, N. F., Li, T. L., et al. (2022). Melatonin alleviates hydrogen peroxide induced oxidative damage in MC3T3-E1 cells and promotes osteogenesis by activating SIRT1. Free Radic. Res. 56, 63–76. doi:10.1080/10715762.2022.2037580
Liu, S. C., Tsai, C. H., Wang, Y. H., Su, C. M., Wu, H. C., Fong, Y. C., et al. (2022). Melatonin abolished proinflammatory factor expression and antagonized osteoarthritis progression in vivo. Cell Death Dis. 13 (3), 215. doi:10.1038/s41419-022-04656-5
Liu, S., Kang, W., Mao, X., Ge, L., Du, H., Li, J., et al. (2022). Melatonin mitigates aflatoxin B1-induced liver injury via modulation of gut microbiota/intestinal FXR/liver TLR4 signaling axis in mice. J. Pineal Res. 73, e12812. doi:10.1111/jpi.12812
Liu, X., Gong, Y., Xiong, K., Ye, Y., Xiong, Y., Zhuang, Z., et al. (2013). Melatonin mediates protective effects on inflammatory response induced by interleukin-1 beta in human mesenchymal stem cells. J. Pineal Res. 55 (1), 14–25. doi:10.1111/jpi.12045
Liu, Z., Lee, H. L., Suh, J. S., Deng, P., Lee, C. R., Bezouglaia, O., et al. (2022). The ERα/KDM6B regulatory axis modulates osteogenic differentiation in human mesenchymal stem cells. Bone Res. 10 (1), 3. doi:10.1038/s41413-021-00171-z
Lu, X., Min, W., Shi, Y., Tian, L., Li, P., Ma, T., et al. (2022). Exogenous melatonin alleviates alkaline stress by removing reactive oxygen species and promoting antioxidant defence in rice seedlings. Front. Plant Sci. 13, 849553. doi:10.3389/fpls.2022.849553
Lu, X., Yu, S., Chen, G., Zheng, W., Peng, J., Huang, X., et al. (2021). Insight into the roles of melatonin in bone tissue and bone-related diseases (Review). Int. J. Mol. Med. 47 (5), 82. doi:10.3892/ijmm.2021.4915
Luo, B., Zhou, X., Tang, Q., Yin, Y., Feng, G., Li, S., et al. (2021). Circadian rhythms affect bone reconstruction by regulating bone energy metabolism. J. Transl. Med. 19 (1), 410. doi:10.1186/s12967-021-03068-x
Luo, J., Zhang, Z., Sun, H., Song, J., Chen, X., Huang, J., et al. (2020). Effect of melatonin on T/B cell activation and immune regulation in pinealectomy mice. Life Sci. 242, 117191. doi:10.1016/j.lfs.2019.117191
Ma, A., Hong, J., Shanks, J., Rudebush, T., Yu, L., Hackfort, B. T., et al. (2019). Upregulating Nrf2 in the RVLM ameliorates sympatho-excitation in mice with chronic heart failure. Free Radic. Biol. Med. 141, 84–92. doi:10.1016/j.freeradbiomed.2019.06.002
Maestroni, G. J. (1998). The photoperiod transducer melatonin and the immune-hematopoietic system. J. Photochem. Photobiol. B 43 (3), 186–192. doi:10.1016/s1011-1344(98)00107-9
Maria, S., Samsonraj, R. M., Munmun, F., Glas, J., Silvestros, M., Kotlarczyk, M. P., et al. (2018). Biological effects of melatonin on osteoblast/osteoclast cocultures, bone, and quality of life: Implications of a role for MT2 melatonin receptors, MEK1/2, and MEK5 in melatonin-mediated osteoblastogenesis. J. Pineal Res. 64 (3), e12465. doi:10.1111/jpi.12465
Markus, R. P., Sousa, K. S., da Silveira Cruz-Machado, S., Fernandes, P. A., and Ferreira, Z. S. (2021). Possible role of pineal and extra-pineal melatonin in surveillance, immunity, and first-line defense. Int. J. Mol. Sci. 22 (22), 12143. doi:10.3390/ijms222212143
Masuzawa, T., Miyaura, C., Onoe, Y., Kusano, K., Ohta, H., Nozawa, S., et al. (1994). Estrogen deficiency stimulates B lymphopoiesis in mouse bone marrow. J. Clin. Invest. 94 (3), 1090–1097. doi:10.1172/JCI117424
McLean, R. R. (2009). Proinflammatory cytokines and osteoporosis. Curr. Osteoporos. Rep. 7 (4), 134–139. doi:10.1007/s11914-009-0023-2
Miyazaki-Akita, A., Hayashi, T., Ding, Q. F., Shiraishi, H., Nomura, T., Hattori, Y., et al. (2007). 17beta-estradiol antagonizes the down-regulation of endothelial nitric-oxide synthase and GTP cyclohydrolase I by high glucose: Relevance to postmenopausal diabetic cardiovascular disease. J. Pharmacol. Exp. Ther. 320 (2), 591–598. doi:10.1124/jpet.106.111641
Mohammad, I., Starskaia, I., Nagy, T., Guo, J., Yatkin, E., Vaananen, K., et al. (2018). Estrogen receptor α contributes to T cell-mediated autoimmune inflammation by promoting T cell activation and proliferation. Sci. Signal. 11 (526), eaap9415. doi:10.1126/scisignal.aap9415
Nazarian, A., Cory, E., Müller, R., and Snyder, B. D (2009). Shortcomings of DXA to assess changes in bone tissue density and microstructure induced by metabolic bone diseases in rat models. Osteoporos. Int. 20 (1), 123–132. doi:10.1007/s00198-008-0632-0
Okamoto, K., Nakashima, T., Shinohara, M., Negishi-Koga, T., Komatsu, N., Terashima, A., et al. (2017). Osteoimmunology: The conceptual framework unifying the immune and skeletal systems. Physiol. Rev. 97 (4), 1295–1349. doi:10.1152/physrev.00036.2016
Okatani, Y., Morioka, N., and Wakatsuki, A. (2000). Changes in nocturnal melatonin secretion in perimenopausal women: Correlation with endogenous estrogen concentrations. J. Pineal Res. 28 (2), 111–118. doi:10.1034/j.1600-079x.2001.280207.x
Oršolić, N., and Jazvinšćak Jembrek, M. (2022). Molecular and cellular mechanisms of propolis and its polyphenolic compounds against cancer. Int. J. Mol. Sci. 23 (5), 10479. doi:10.3390/ijms231810479
Ostrowska, Z., Kos-Kudla, B., Marek, B., Kajdaniuk, D., and Ciesielska-Kopacz, N. (2002). The relationship between the daily profile of chosen biochemical markers of bone metabolism and melatonin and other hormone secretion in rats under physiological conditions. Neuro Endocrinol. Lett. 23 (5-6), 417–425.
Ostrowska, Z., Kos-Kudla, B., Marek, B., and KajDaniuk, D. (2003). Influence of lighting conditions on daily rhythm of bone metabolism in rats and possible involvement of melatonin and other hormones in this process. Endocr. Regul. 37 (3), 163–174.
Ostrowska, Z., Kos-Kudla, B., Marek, B., SwiEtochowska, E., and Gorski, J. (2001). Assessment of the relationship between circadian variations of salivary melatonin levels and type I collagen metabolism in postmenopausal obese women. Neuro Endocrinol. Lett. 22 (2), 121–127.
Ostrowska, Z., Kos-Kudla, B., Swietochowska, E., Marek, B., KajDaniuk, D., and Gorski, J. (2001). Assessment of the relationship between dynamic pattern of nighttime levels of melatonin and chosen biochemical markers of bone metabolism in a rat model of postmenopausal osteoporosis. Neuro Endocrinol. Lett. 22 (2), 129–136.
Qian, J., Morris, C. J., Phillips, A. J. K., Li, P., Rahman, S. A., Wang, W., et al. (2022). Unanticipated daytime melatonin secretion on a simulated night shift schedule generates a distinctive 24-h melatonin rhythm with antiphasic daytime and nighttime peaks. J. Pineal Res. 72 (3), e12791. doi:10.1111/jpi.12791
Ren, H., Sun, R., and Wang, J. (2018). Relationship of melatonin level, oxidative stress and inflammatory status with osteoporosis in maintenance hemodialysis of chronic renal failure. Exp. Ther. Med. 15 (6), 5183–5188. doi:10.3892/etm.2018.5857
Riggs, B. L. (2000). The mechanisms of estrogen regulation of bone resorption. J. Clin. Invest. 106 (10), 1203–1204. doi:10.1172/JCI11468
Roy, D., and Belsham, D. D. (2002). Melatonin receptor activation regulates GnRH gene expression and secretion in GT1-7 GnRH neurons. Signal transduction mechanisms. J. Biol. Chem. 277 (1), 251–258. doi:10.1074/jbc.M108890200
Sadaf, S., Shameem, M., Siddiqi, S. S., Anwar, S., and Mohd, S. (2021). Effect of obstructive sleep apnea on bone mineral density. Turk. Thorac. J. 22 (4), 301–310. doi:10.5152/TurkThoracJ.2021.20051
Salamanna, F., Maglio, M., Giavaresi, G., Pagani, S., Giardino, R., and Fini, M. (2015). In vitro method for the screening and monitoring of estrogen-deficiency osteoporosis by targeting peripheral circulating monocytes. Age (Dordr) 37 (4), 9819. doi:10.1007/s11357-015-9819-4
Sapra, L., Shokeen, N., Porwal, K., Saini, C., Bhardwaj, A., Mathew, M., et al. (2022). Bifidobacterium longum ameliorates ovariectomy-induced bone loss via enhancing anti-osteoclastogenic and immunomodulatory potential of regulatory B cells (bregs). Front. Immunol. 13, 875788. doi:10.3389/fimmu.2022.875788
Sehirli, A., Chukwunyere, U., Aksoy, U., Sayiner, S., and Abacioglu, N. (2021). The circadian clock gene Bmal1: Role in COVID-19 and periodontitis. Chronobiol. Int. 38 (6), 779–784. doi:10.1080/07420528.2021.1895198
Silva, A. M., Heymsfield, S. B., and Sardinha, L. B. (2013). Assessing body composition in taller or broader individuals using dual-energy X-ray absorptiometry: A systematic review. Eur. J. Clin. Nutr. 67 (10), 1012–1021. doi:10.1038/ejcn.2013.148
Song, C., Wang, J., Kim, B., Lu, C., Zhang, Z., Liu, H., et al. (2018). Insights into the role of circadian rhythms in bone metabolism: A promising intervention target? Biomed. Res. Int. 2018, 9156478. doi:10.1155/2018/9156478
Srivastava, R. K., Dar, H. Y., and Mishra, P. K. (2018). Immunoporosis: Immunology of osteoporosis-role of T cells. Front. Immunol. 9, 657. doi:10.3389/fimmu.2018.00657
Su, X., Guo, W., Yuan, B., Wang, D., Liu, L., Wu, X., et al. (2021). Artesunate attenuates bone erosion in rheumatoid arthritis by suppressing reactive oxygen species via activating p62/Nrf2 signaling. Biomed. Pharmacother. 137, 111382. doi:10.1016/j.biopha.2021.111382
Sun, L., Ma, J., Chen, J., Pan, Z., and Li, L. (2022). Bioinformatics-guided analysis uncovers AOX1 as an osteogenic differentiation-relevant gene of human mesenchymal stem cells. Front. Mol. Biosci. 9, 800288. doi:10.3389/fmolb.2022.800288
Suo, J., Zou, S., Wang, J., Han, Y., Zhang, L., Lv, C., et al. (2022). The RNA-binding protein Musashi2 governs osteoblast-adipocyte lineage commitment by suppressing PPARγ signaling. Bone Res. 10 (1), 31. doi:10.1038/s41413-022-00202-3
Suzuki, N., and Hattori, A. (2002). Melatonin suppresses osteoclastic and osteoblastic activities in the scales of goldfish. J. Pineal Res. 33 (4), 253–258. doi:10.1034/j.1600-079x.2002.02953.x
Tao, Z. S., Lu, H. L., Ma, N. F., Zhang, R. T., Li, Y., Yang, M., et al. (2020). Rapamycin could increase the effects of melatonin against age-dependent bone loss. Z. Gerontol. Geriatr. 53 (7), 671–678. doi:10.1007/s00391-019-01659-4
Tian, Y., Gong, Z., Zhao, R., and Zhu, Y. (2021). Melatonin inhibits RANKL-induced osteoclastogenesis through the miR-882/Rev-erbα axis in Raw264.7 cells. Int. J. Mol. Med. 47 (2), 633–642. doi:10.3892/ijmm.2020.4820
Titanji, K., Ofotokun, I., and Weitzmann, M. N. (2020). Immature/transitional B-cell expansion is associated with bone loss in HIV-infected individuals with severe CD4+ T-cell lymphopenia. Aids 34 (10), 1475–1483. doi:10.1097/QAD.0000000000002563
Toffol, E., Kalleinen, N., Haukka, J., Vakkuri, O., Partonen, T., and Polo-Kantola, P. (2014). Melatonin in perimenopausal and postmenopausal women: Associations with mood, sleep, climacteric symptoms, and quality of life. Menopause 21 (5), 493–500. doi:10.1097/GME.0b013e3182a6c8f3
Treister-Goltzman, Y., and Peleg, R. (2021). Melatonin and the health of menopausal women: A systematic review. J. Pineal Res. 71 (2), e12743. doi:10.1111/jpi.12743
Vishwas, D. K., and Haldar, C. (2013). Photoperiodic induced melatonin regulates immunity and expression pattern of melatonin receptor MT1 in spleen and bone marrow mononuclear cells of male golden hamster. J. Photochem. Photobiol. B 128, 107–114. doi:10.1016/j.jphotobiol.2013.08.018
Vriend, J., and Reiter, R. J. (2016). Melatonin, bone regulation and the ubiquitin-proteasome connection: A review. Life Sci. 145, 152–160. doi:10.1016/j.lfs.2015.12.031
Wan, L., Shi, X. Y., Ge, W. R., Sun, Y. L., Zhang, S., Wang, J., et al. (2020). The instigation of the associations between melatonin, circadian genes, and epileptic spasms in infant rats. Front. Neurol. 11, 497225. doi:10.3389/fneur.2020.497225
Wang, L., Yu, W., Yin, X., Cui, L., Tang, S., Jiang, N., et al. (2021). Prevalence of osteoporosis and fracture in China: The China osteoporosis prevalence study. JAMA Netw. Open 4 (8), e2121106. doi:10.1001/jamanetworkopen.2021.21106
Wang, T., Wang, Z., Cao, J., Dong, Y., and Chen, Y. (2022). Melatonin prevents the dysbiosis of intestinal microbiota in sleep-restricted mice by improving oxidative stress and inhibiting inflammation. Saudi J. Gastroenterol. 28 (3), 209–217. doi:10.4103/sjg.sjg_110_21
Wang, Y. F., Chang, Y. Y., Zhang, X. M., Gao, M. T., Zhang, Q. L., Li, X., et al. (2022). Salidroside protects against osteoporosis in ovariectomized rats by inhibiting oxidative stress and promoting osteogenesis via Nrf2 activation. Phytomedicine. 99, 154020. doi:10.1016/j.phymed.2022.154020
Wang, Z. X., Luo, Z. W., Li, F. X., Cao, J., Rao, S. S., Liu, Y. W., et al. (2022). Aged bone matrix-derived extracellular vesicles as a messenger for calcification paradox. Nat. Commun. 13 (1), 1453. doi:10.1038/s41467-022-29191-x
Wen, K., Tao, L., Tao, Z., Meng, Y., Zhou, S., Chen, J., et al. (2020). Fecal and serum metabolomic signatures and microbial community profiling of postmenopausal osteoporosis mice model. Front. Cell. Infect. Microbiol. 10, 535310. doi:10.3389/fcimb.2020.535310
Wu, Y., He, F., Zhang, C., Zhang, Q., Su, X., Zhu, X., et al. (2021). Melatonin alleviates titanium nanoparticles induced osteolysis via activation of butyrate/GPR109A signaling pathway. J. Nanobiotechnology 19 (1), 170. doi:10.1186/s12951-021-00915-3
Xi, Z., Yuan, X. Q., and Zhang, X. M. (2021). Melatonin reduces inflammation in intestinal cells, organoids and intestinal explants. Inflammopharmacology 29 (5), 1555–1564. doi:10.1007/s10787-021-00869-w
Xiao, L., Lin, J., Chen, R., Huang, Y., Liu, Y., Bai, J., et al. (2020). Sustained release of melatonin from GelMA liposomes reduced osteoblast apoptosis and improved implant osseointegration in osteoporosis. Oxid. Med. Cell. Longev. 2020, 6797154. doi:10.1155/2020/6797154
Xiong, X., Bao, Z., Mi, Y., Wang, X., and Zhu, J. (2022). Melatonin alleviates neonatal necrotizing enterocolitis by repressing the activation of the NLRP3 inflammasome. Gastroenterol. Res. Pract. 2022, 6920577. doi:10.1155/2022/6920577
Xu, H., Cai, L., Li, Z., Zhang, L., Wang, G., Xie, R., et al. (2021). Dual effect of IL-7/IL-7R signalling on the osteoimmunological system: A potential therapeutic target for rheumatoid arthritis. Immunology 164 (1), 161–172. doi:10.1111/imm.13351
Xu, L., Zhang, L., Wang, Z., Li, C., Li, S., Li, L., et al. (2018). Melatonin suppresses estrogen deficiency-induced osteoporosis and promotes osteoblastogenesis by inactivating the NLRP3 inflammasome. Calcif. Tissue Int. 103 (4), 400–410. doi:10.1007/s00223-018-0428-y
Yang, K., Cao, F., Xue, Y., Tao, L., and Zhu, Y. (2022). Three classes of antioxidant defense systems and the development of postmenopausal osteoporosis. Front. Physiol. 13, 840293. doi:10.3389/fphys.2022.840293
Yang, K., Pei, L., Zhou, S., Tao, L., and Zhu, Y. (2021). Metformin attenuates H(2)O(2)-induced osteoblast apoptosis by regulating SIRT3 via the PI3K/AKT pathway. Exp. Ther. Med. 22 (5), 1316. doi:10.3892/etm.2021.10751
Yang, N., and Liu, Y. (2021). The role of the immune microenvironment in bone regeneration. Int. J. Med. Sci. 18 (16), 3697–3707. doi:10.7150/ijms.61080
Yang, W., Zhang, Y., Lu, D., Huang, T., Yan, K., Wang, W., et al. (2022). Ramelteon protects against human pulmonary microvascular endothelial cell injury induced by lipopolysaccharide (LPS) via activating nuclear factor erythroid 2-related factor 2 (Nrf2)/heme oxygenase-1 (HO-1) pathway. Bioengineered 13 (1), 1518–1529. doi:10.1080/21655979.2021.2021065
Yardimci, A., Ozdede, M. R., and Agomelatine, Kelestimur H. (2021). Agomelatine, A potential multi-target treatment alternative for insomnia, depression, and osteoporosis in postmenopausal women: A hypothetical model. Front. Psychiatry 12, 654616. doi:10.3389/fpsyt.2021.654616
Yoshitane, H., Imamura, K., Okubo, T., Otobe, Y., Kawakami, S., Ito, S., et al. (2022). mTOR-AKT signaling in cellular clock resetting triggered by osmotic stress. Bunkyo-ku: Antioxid Redox Signal.
Yu, Q., Miller, S. C., and Osmond, D. G. (2000). Melatonin inhibits apoptosis during early B-cell development in mouse bone marrow. J. Pineal Res. 29 (2), 86–93. doi:10.1034/j.1600-079x.2000.290204.x
Yu, S., Tang, Q., Chen, G., Lu, X., Yin, Y., Xie, M., et al. (2022). Circadian rhythm modulates endochondral bone formation via MTR1/AMPKβ1/BMAL1 signaling axis. Cell Death Differ. 29, 874–887. doi:10.1038/s41418-021-00919-4
Yuan, G., Hua, B., Yang, Y., Xu, L., Cai, T., Sun, N., et al. (2017). The circadian gene clock regulates bone formation via PDIA3. J. Bone Min. Res. 32 (4), 861–871. doi:10.1002/jbmr.3046
Yung, L. M., Wong, W. T., Tian, X. Y., Leung, F. P., Yung, L. H., Chen, Z. Y., et al. (2011). Inhibition of renin-angiotensin system reverses endothelial dysfunction and oxidative stress in estrogen deficient rats. PLoS One 6 (3), e17437. doi:10.1371/journal.pone.0017437
Zakria, M., Ahmad, N., Al Kury, L. T., Alattar, A., Uddin, Z., Siraj, S., et al. (2021). Melatonin rescues the mice brain against cisplatin-induced neurodegeneration, an insight into antioxidant and anti-inflammatory effects. Neurotoxicology 87, 1–10. doi:10.1016/j.neuro.2021.08.010
Zhang, C., Clough, S. J., Adamah-Biassi, E. B., Sveinsson, M. H., Hutchinson, A. J., Miura, I., et al. (2021). Impact of endogenous melatonin on rhythmic behaviors, reproduction, and survival revealed in melatonin-proficient C57BL/6J congenic mice. J. Pineal Res. 71 (2), e12748. doi:10.1111/jpi.12748
Zhang, G. Q., Chen, J. L., Luo, Y., Mathur, M. B., Anagnostis, P., Nurmatov, U., et al. (2021). Menopausal hormone therapy and women's health: An umbrella review. PLoS Med. 18 (8), e1003731. doi:10.1371/journal.pmed.1003731
Zhang, H., Liu, X., Zheng, Y., Zha, X., Elsabagh, M., Zhang, Y., et al. (2022). Effects of the maternal gut microbiome and gut-placental axis on melatonin efficacy in alleviating cadmium-induced fetal growth restriction. Ecotoxicol. Environ. Saf. 237, 113550. doi:10.1016/j.ecoenv.2022.113550
Zhang, J., Tang, J., Liu, J., Yan, B., Yan, B., Huang, M., et al. (2021). Melatonin promotes heterotopic ossification through regulation of endothelial-mesenchymal transition in injured achilles tendons in rats. Front. Cell Dev. Biol. 9, 629274. doi:10.3389/fcell.2021.629274
Zhang, L., Su, P., Xu, C., Chen, C., Liang, A., Du, K., et al. (2010). Melatonin inhibits adipogenesis and enhances osteogenesis of human mesenchymal stem cells by suppressing PPARγ expression and enhancing Runx2 expression. J. Pineal Res. 49 (4), 364–372. doi:10.1111/j.1600-079X.2010.00803.x
Zhang, M., Lu, Y., Chen, Y., Zhang, Y., and Xiong, B. (2020). Insufficiency of melatonin in follicular fluid is a reversible cause for advanced maternal age-related aneuploidy in oocytes. Redox Biol. 28, 101327. doi:10.1016/j.redox.2019.101327
Zhao, R., Tao, L., Qiu, S., Shen, L., Tian, Y., Gong, Z., et al. (2020). Melatonin rescues glucocorticoid-induced inhibition of osteoblast differentiation in MC3T3-E1 cells via the PI3K/AKT and BMP/Smad signalling pathways. Life Sci. 257, 118044. doi:10.1016/j.lfs.2020.118044
Zheng, Q., Kernozek, T., Daoud-Gray, A., and Borer, K. T. (2021). Anabolic bone stimulus requires a pre-exercise meal and 45-minute walking impulse of suprathreshold speed-enhanced momentum to prevent or mitigate postmenopausal osteoporosis within circadian constraints. Nutrients 13 (11), 3727. doi:10.3390/nu13113727
Zhou, L., Chen, X., Yan, J., LiM., , Liu, T., Zhu, C., et al. (2017). Melatonin at pharmacological concentrations suppresses osteoclastogenesis via the attenuation of intracellular ROS. Osteoporos. Int. 28 (12), 3325–3337. doi:10.1007/s00198-017-4127-8
Zhou, S., Huang, G., and Chen, G. (2020). Synthesis and biological activities of drugs for the treatment of osteoporosis. Eur. J. Med. Chem. 197, 112313. doi:10.1016/j.ejmech.2020.112313
Zhou, W., Liu, Y., Shen, J., Yu, B., Bai, J., Lin, J., et al. (2019). Melatonin increases bone mass around the prostheses of OVX rats by ameliorating mitochondrial oxidative stress via the SIRT3/SOD2 signaling pathway. Oxid. Med. Cell. Longev. 2019, 4019619. doi:10.1155/2019/4019619
Zhou, X., Yuan, W., Xiong, X., Zhang, Z., Liu, J., Zheng, Y., et al. (2021). HO-1 in bone biology: Potential therapeutic strategies for osteoporosis. Front. Cell Dev. Biol. 9, 791585. doi:10.3389/fcell.2021.791585
Zhou, X., Zhang, Y., Hou, M., Liu, H., Yang, H., Chen, X., et al. (2022). Melatonin prevents cartilage degradation in early-stage osteoarthritis through activation of miR-146a/NRF2/HO-1 Axis. J. Bone Min. Res. 37, 1056–1072. doi:10.1002/jbmr.4527
Zhou, Y., Wang, C., Si, J., Wang, B., Zhang, D., Ding, D., et al. (2020). Melatonin up-regulates bone marrow mesenchymal stem cells osteogenic action but suppresses their mediated osteoclastogenesis via MT(2) -inactivated NF-κB pathway. Br. J. Pharmacol. 177 (9), 2106–2122. doi:10.1111/bph.14972
Keywords: melatonin, postmenopausal osteoporosis, biological rhythms, antioxidant, anti-inflammation, immuomodulation, gut microbiota
Citation: Yang K, Qiu X, Cao L and Qiu S (2022) The role of melatonin in the development of postmenopausal osteoporosis. Front. Pharmacol. 13:975181. doi: 10.3389/fphar.2022.975181
Received: 22 June 2022; Accepted: 26 September 2022;
Published: 07 October 2022.
Edited by:
Valentina Vengeliene, Vilnius University, LithuaniaReviewed by:
Siyi Zhu, Sichuan University, ChinaCopyright © 2022 Yang, Qiu, Cao and Qiu. This is an open-access article distributed under the terms of the Creative Commons Attribution License (CC BY). The use, distribution or reproduction in other forums is permitted, provided the original author(s) and the copyright owner(s) are credited and that the original publication in this journal is cited, in accordance with accepted academic practice. No use, distribution or reproduction is permitted which does not comply with these terms.
*Correspondence: Lili Cao, Y2FvbGlseTA3MTdAaG90bWFpbC5jb20=; Shui Qiu, cWl1c2h1aWlAaG90bWFpbC5jb20=
Disclaimer: All claims expressed in this article are solely those of the authors and do not necessarily represent those of their affiliated organizations, or those of the publisher, the editors and the reviewers. Any product that may be evaluated in this article or claim that may be made by its manufacturer is not guaranteed or endorsed by the publisher.
Research integrity at Frontiers
Learn more about the work of our research integrity team to safeguard the quality of each article we publish.