Corrigendum: Compounds purified from edible fungi fight against chronic inflammation through oxidative stress regulation
- 1Department of Hand and Foot Surgery, The First Hospital of Jilin University, Changchun, China
- 2Laboratory Animal Center, College of Animal Science, Jilin University, Changchun, China
Chronic inflammation is associated with various chronic diseases, including cardiovascular disease, neurodegenerative disease, and cancer, which severely affect the health and quality of life of people. Oxidative stress induced by unbalanced production and elimination of reactive oxygen species (ROS) is one of the essential risk factors for chronic inflammation. Recent studies, including the studies of mushrooms, which have received considerable attention, report that the antioxidant effects of natural compounds have more advantages than synthetic antioxidants. Mushrooms have been consumed by humans as precious nourishment for 3,000 years, and so far, more than 350 types have been identified in China. Mushrooms are rich in polysaccharides, peptides, polyphenols, alkaloids, and terpenoids and are associated with several healthy biological functions, especially antioxidant properties. As such, the extracts purified from mushrooms could activate the expression of antioxidant enzymes through the Keap1/Nrf2/ARE pathway to neutralize excessive ROS and inhibit ROS-induced chronic inflammation through the NF-κB pathway. Recently, the antioxidant properties of mushrooms have been successfully applied to treating cardiovascular disease (CAD), neurodegenerative diseases, diabetes mellitus, and cancer. The present review summarizes the antioxidant properties and the mechanism of compounds purified from mushrooms, emphasizing the oxidative stress regulation of mushrooms to fight against chronic inflammation.
Introduction
Mushrooms have been extensively cultivated in China using artificial techniques due to their high yield, desirable taste, and widespread consumption and application as a medicinal resource (Huang and Nie, 2015). Recently, various compounds have been isolated from mushrooms, such as polysaccharides, alkaloids, peptides, terpenoids, and polyphenols (Homer and Sperry, 2017; Zhou et al., 2020; Kuang et al., 2021; Leong et al., 2021; Zhang et al., 2021). Mushroom extracts can resist free radicals, reduce the activities of pro-inflammatory factors, and relieve chronic inflammation, which are valuable natural antioxidants with the advantages of safety, nontoxic, and easy to obtain (Hu et al., 2021). Some studies have also demonstrated the therapeutic potential of mushroom extract for cardiovascular disease (CAD), neurodegenerative diseases, and cancer (Klupp et al., 2015; Jin et al., 2016; Chun et al., 2021). This review discusses the mechanisms underlying the effect of oxidative stress on chronic inflammation and summarizes the antioxidant properties of compounds purified from mushrooms. The anti-inflammatory effects of these compounds on CAD, neurodegenerative diseases, diabetes mellitus, and cancer provide potential treatment measures for chronic inflammation caused by oxidative stress.
Chronic inflammation and oxidative stress
Predisposing factors of oxidative stress
The predisposing factors, including diet, exercise, chemicals, radiation, and drugs, can increase reactive oxygen species (ROS) production and disrupt the antioxidant system, inducing oxidative stress (Klaunig et al., 2011; Quindry et al., 2016; Tan et al., 2018; Tan and Norhaizan, 2019). Similarly, eating habits, including refined carbohydrates, high-fat, and high-animal protein diets, can increase oxidative stress through the nuclear factor-kappa B (NF-κB) signaling pathways (Tan et al., 2018). High sugar intake can produce advanced glycation end products to promote oxidation and activate the protein phosphatase 2 A and NF-κB pathways, inducing oxidative stress in mitochondria (Man et al., 2020). Regular and moderate exercise has also been proven to inhibit ROS production, while high intensity and long-term exercise might induce oxidative stress in the skeletal muscle cells, causing muscle contractile dysfunction (Powers and Jackson, 2008; Thirupathi et al., 2020). A previous study has reported that the exposure of cells to metals and metal oxide nanoparticles induces oxidative stress, thereby damaging the deoxyribonucleic acids (DNAs), proteins, and lipids (Tee et al., 2016). For instance, aluminum accumulation could impair the oxidative function of mitochondria, especially in the brain, which is explicitly sensitive to oxidative stress and is one of the causes of neurodegenerative diseases (Kumar and Gill, 2014). Similarly, continuous exposure to ionizing radiation induces excessive production of hydroxyl radicals through water oxidation or secondary partially ROS formation (Klaunig et al., 2011). Drugs, including gentamicin and bleomycin, could produce free radicals during degeneration and metabolism (Pizzino et al., 2017). Additionally, the potential predisposing factors, including unhealthy living habits, exposure to harmful substances, and certain drugs, could induce oxidative stress and damage the cellular components through different mechanisms.
Mechanism of oxidative stress
In mitochondria, most oxygen is utilized for aerobic respiration and energy release, with ROS being produced as a byproduct (Cadenas and Davies, 2000). Mitochondria convert glucose to adenosine triphosphate (ATP) through the tricarboxylic acid cycle and oxidative phosphorylation by using nicotinamide adenine dinucleotide (NADH) and reduced flavin adenosine dinucleotide (FADH2) produced in the cytoplasmic matrix (Ryoo and Kwak, 2018). In the oxidative phosphorylation process, NADH and FADH2 efficiently reduce oxygen and release energy through the electron transport chain (ETC) (Bhatti et al., 2017). However, a small quantity of oxygen is still reduced prematurely by electrons, producing superoxide anion (O2−), a relatively stable intermediate, which can generate hydrogen peroxide (H2O2) by disproportionation reaction, or hydroxyl radicals (OH) by the Haber-Weiss and Fenton reaction (Figure 1) (Sinha et al., 2013).
Under physiological conditions, ROS can be removed by the antioxidant enzymes, including superoxide dismutase (SOD), catalase (CAT), glutathione peroxidase (GSH-PX), and peroxiredoxin/thioredoxin (Prx/Trx) (Griendling et al., 2021). SOD consists of manganese superoxide dismutase (MnSOD) located in the mitochondrial matrix and cuprum/zinc superoxide dismutase (Cu/ZnSOD) in the membrane gap, which can dismutate the O2—produced by ETC into H2O2 (Peoples et al., 2019). The GSH-PX and Prx/Trx systems of the cytoplasmic matrix could reduce H2O2, while nicotinamide adenine dinucleotide phosphate (NADPH) maintains the reducing power of these two antioxidant systems (Nolfi-Donegan et al., 2020). Meanwhile, NADH/NADPH oxidase serves as an intracellular ROS source, especially in the vascular tissues and cardiomyocytes (Elahi et al., 2009). The persistence of ROS damages the somatic cells, including the cardiac muscle cells and nerve cells, while the antioxidant properties of the compounds in mushrooms neutralize the ROS (Chun et al., 2021).
Mechanism of oxidative stress in chronic inflammation
The persistence of inflammatory factors causing tissue damage, such as trauma, chemical erosion, microbial infection, and autoimmune reaction, significantly contributes to chronic inflammation (Medzhitov, 2008; Leuti et al., 2020). When inflammation occurs, oxygen uptake by the white blood cells and mast cells in the inflammatory area increases, leading to “respiratory burst,” which enhances ROS production and release (Reuter et al., 2010). It is reported that low density lipoprotein (LDL) can be oxidized by ROS and phagocytosed by macrophages, thereby improving the release of pro-inflammatory factors and inducing inflammatory responses mediated by the NF-κB signal pathway (Zuo et al., 2019). The expression of phagocytic NADPH oxidase relying on NF-κB could produce ROS and increase the bactericidal activity of macrophages through the Toll-like receptors, including Toll-like receptor 1 (TLR1), TLR2, and TLR4 (West et al., 2011). However, excessive intracellular ROS stimulate the expression of NF-κB by activating the p38 signaling pathway and regulating the liberation of pro-inflammatory factors, including tumor necrosis factor-α (TNF-α), inducible nitric oxide synthase (iNOS), and cyclooxygenase-2 (COX-2) (Xi et al., 2020). Additionally, ROS could stimulate the expression of TLR4 and trigger an inflammatory response in the lungs by activating the NF-κB pathway (Mishra et al., 2018). Oxidative stress can induce chronic inflammation, while the inflammatory response can improve ROS release, primarily mediated through the NF-κB signal pathway (Figure 2).
Antioxidant effects and mechanisms of compounds purified from edible fungi
Extraction of compounds from edible fungi
Mushrooms are rich in polysaccharides, peptides, polyphenols, and other compounds beneficial to human health. Hence, mushrooms have been widely recognized as functional food resources and are being used for developing drugs and nutrients (Alves et al., 2012). In recent years, mushroom-derived polysaccharides have gained considerable attention due to their antioxidant, antibacterial, anti-tumor, and immunomodulatory effects (Wang et al., 2017). Most of the polysaccharides from mushrooms are water-soluble and can be extracted frequently by water extraction using heating, ultrasound, and microwave techniques (Leong et al., 2021). According to modern technology, water extraction has some disadvantages, such as long extraction time, low efficiency, and risk of biopolymer degradation. As such, the subcritical pressurized hot water extraction technology and deep eutectic solvent extraction technology have emerged as potential approaches to achieving efficient water extraction (Rodrigues Barbosa et al., 2020). Extracting mushroom-derived bioactive peptides is mostly dependent on the proteolytic action of exogenous enzymes and microbial fermentation technology (Zhou et al., 2020). Traditionally, microwave-assisted and ultrasound-assisted methods were used to extract the phenolic compounds (Petrovic et al., 2014). Different extraction methods may influence the antioxidant effects of different compounds in mushrooms, thus helping to select the appropriate extraction method to inhibit chronic inflammation by eliminating ROS.
Polysaccharide
Polysaccharide, the most widely studied compound in mushrooms, is primarily composed of more than 10 monosaccharides linked with glycosidic bonds of polymeric sugar polymer carbohydrates (Supplementary Figure.S1) (Leong et al., 2021). The hydrogen ion on the sugar chain of polysaccharides can directly bind to OH and break down into harmless products (Lu et al., 2020). Polysaccharides can inhibit oxidative stress by improving the activities of antioxidant enzymes, including SOD, CAT, and GSH-PX, or chelate with metal ions, essential for ROS production (Huang et al., 2017). The antioxidant effect of polysaccharides is mainly mediated through the activation of the Keap1/Nrf2/ARE pathway to achieve the expression of downstream antioxidant enzymes (Figure 3A) (Chen et al., 2021). Nuclear factor erythroid-2 (Nrf2) is the major regulatory element of the antioxidant system in vivo, which can detach from the binding site of Kelch-like ECH-associated protein 1 (Keap1) in the cytoplasm after being stimulated and transferred to the nucleus to bind with the anti-oxidant response element (ARE) (Liao et al., 2019). Polysaccharides have been reported to regulate oxidative stress by upregulating the expression of phosphatidylinositol 3-kinase (P13K) and phosphorylation of protein kinase B (AKT) (Yun et al., 2020).
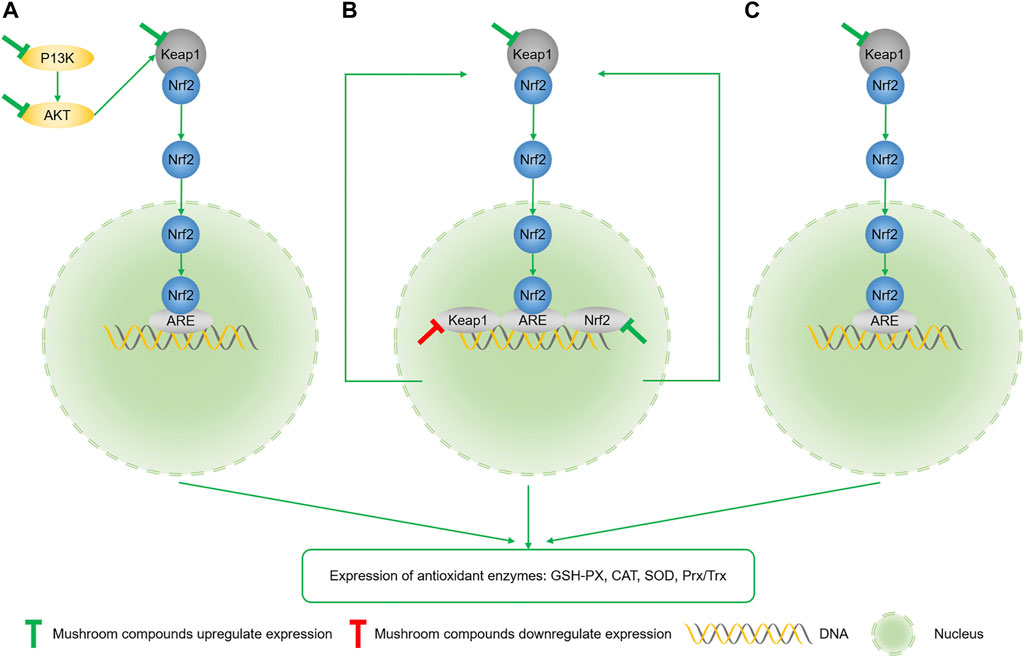
FIGURE 3. (A) Mushroom polysaccharides activate the Keap1/Nrf2/ARE pathway by upregulating the expression of P13K and AKT. (B) Mushroom peptides activate the Nrf2 pathway by downregulating the Keap1 gene and upregulating the Nrf2 gene expression. (C) Polyphenols directly promote the dissociation of Keap1 and Nrf2.
Poria cocos polysaccharide could eliminate O2—and OH, while carboxymethylated pachymaran could promote the expression of SOD to achieve an antioxidant effect (Li et al., 2019). Lepista nuda polysaccharides extracted through water extraction and alcohol precipitation method could chelate iron ions and scavenge O2—and 1,1-diphenyl-2-picrylhydrazyl free (DPPH) radicals and in a concentration-dependent manner (Shu et al., 2019). A water-soluble polysaccharide isolated from the alkaline extract of Entoloma lividoalbum could eliminate OH through the hydrogen atom donation ability of its hydroxyl group (Maity et al., 2014). In a previous study, the ultrasonic-assisted extraction of Flammulina velutipes polysaccharide showed stronger scavenging ability of DPPH, OH, and O2—than hot water extraction, with better inflammation inhibitory ability (Chen et al., 2019). For instance, the polysaccharide purified for the first time from the floral mushrooms cultivated in Huangshan could scavenge 79.46% DPPH free radical and 74.18% OH at 5 mg/ml (Wang et al., 2015). A new polysaccharide AAP-3-1 isolated from the fruit body of Auricularia auricula could inhibit ROS production, reduce malondialdehyde content, and increase the activities of SOD, GSH-PX, and CAT (Qian et al., 2020).
Peptides
Peptides are composed of three or more amino acid molecules linked by peptide bonds, which can be easily absorbed by the intestine and pose excellent physiological characteristics than proteins (Erdmann et al., 2008). The aromatic amino acids and hydrophobic amino acid residues in the peptides could significantly increase the antioxidant activity, thereby inducing hydrogen atoms (Fontoura et al., 2019; Yang et al., 2020). Peptides can activate the Nrf2 pathway by downregulating the Keap1 gene and upregulating the Nrf2 gene expression to achieve antioxidant effects (Figure 3B) (Liu et al., 2021). Mushrooms are the primary sources of natural active peptides with significant potency, high tissue affinity, low toxicity, and high stability (Zhou et al., 2020). An analysis of the GSH levels in various mushroom species showed that the GSH content of Maitake was higher than most fruits and vegetables, indicating that mushrooms could be an indispensable source of GSH in the daily diet (Kalaras et al., 2017). In a previous study, Matsutake derived peptide WFNNAGP significantly inhibited glucan sulfate-induced oxidative stress in mice by scavenging OH and promoting the SOD activity, thereby preventing colon inflammation (Li et al., 2021a). Low molecular weight peptides decomposed from the protein hydrolysates of Agaricus bisporus by enzymatic processes are abundant in negatively charged amino acids, which can be applied to neutralize free radicals to resist oxidative stress (Kimatu et al., 2017).
Polyphenol
Polyphenols consisting of at least one aromatic ring with hydroxyl functional groups, including flavonoids, phenolic acids, stilbenes, and lignans, are the natural antioxidants in plant foods (Kozarski et al., 2015). Polyphenols can provide electrons or hydrogen atoms to neutralize free radicals or chelate with metal ions to reduce the rate of Fenton reaction (Figure 3C) (Cheng et al., 2017). Agaricus brasiliensis mushroom containing phenolic compounds, such as gallic acid, serum acid, and pyrogallol, could inhibit ROS production by tert-butyl hydrogen peroxide stimulated macrophages (Navegantes-Lima et al., 2020). A novel polyphenol isolated from Phellinus linteus hispolon and its derivatives has been reported to have a strong free radical scavenging ability (Sarfraz et al., 2020). In a previous study, the release of phenolic compounds in agaricus bisporus, cantharellus cibarius, and lentinula edodes was determined by the simulating human gastrointestinal digestion method. The results indicated that lentinus edodes released the most phenolic acids, confirming that the phenolic compounds in lentinus edodes might easily improve the antioxidant capacity of the human body through consumption (Kala et al., 2021). Meanwhile, the flavonoids purified from Flammulina velutipes significantly increased the glutathione level, and the SOD activity of PC12 cells efficiently inhibited intracellular ROS accumulation (Hu et al., 2016). The phenolic compounds in the water extract of lentinus edobes and methanol extract of volvariella volvacea showed a higher hydrogen-providing ability to scavenge the DPPH radicals (Stajic et al., 2013).
Proteins, terpenoids, arene, and lipids
Polysaccharides, polypeptides, and polyphenols are the major antioxidant compounds in mushrooms, while other compounds, including proteins, terpenes, arene, and lipids, tend to have antioxidant properties (Kuang et al., 2021). A novel protein from edible fungi could effectively remove OH and DPPH in vitro, while promoting the apoptosis of breast cancer cells through anti-tumor activity (Zhang et al., 2014). Terpenoids isolated and identified from Sanghuangporus sanghuang could scavenge DPPH and 2,2′-Azinobis-(3-ethylbenzthiazoline-6-sulphonate) (ABTS) free radicals; however, their antioxidant activity is significantly inferior to the polysaccharides and phenolic compounds (Zhang et al., 2021). P-terphenyl compounds isolated from mushroom Boletopsis leucomelas through chromatography possess effective DPPH scavenging capacity, which can be enhanced with heating (Sakemi et al., 2021). 2,5-diarylcyclopentenone derivatives from Paxillus involutus possess clearing abilities of DPPH,·OH, and O2—(Lv et al., 2021). Mushrooms are rich in fatty acids, much higher than in beef and pork, which might contribute to their antioxidant ability to fight against mitochondrial dysfunction (Fontes et al., 2019; Sande et al., 2019).
The antioxidant properties of compounds purified from mushrooms are affected by species, the extracted parts, and purification methods. Accumulating researchers have studied the polysaccharides, peptides, and phenolic compounds comprehensively, while studies focusing on protein, terpenoids, arene, and fatty acids are limited. The antioxidant effects of different compounds in mushrooms are presented in Table 1.
Applications of mushroom-derived compounds on chronic inflammatory diseases
Cardiovascular disease
Endothelial cells act as the barrier between the blood and blood vessel wall, mediating the synthesis and release of a series of active substances (Miller, 2020). Oxidative stress associated with increased ROS production and reduced nitric oxide (NO) availability in the blood vessels and myocardium could induce endothelial dysfunction, including impaired vasodilation, proinflammatory, and thrombogenic, a risk factor for cardiovascular disease (Zhao et al., 2021). Endothelial-derived NO is the primary vasodilator and can react with increased ROS to form toxic peroxynitrite (ONOO−) (Meng et al., 2021). Meanwhile, ROS can induce the uncoupling of endothelial NO synthase (eNOS), a vital enzyme for NO production (Wu et al., 2014). The mushroom-derived compounds exert antioxidant effects through the Nrf2 pathway to effectively treat chronic cardiovascular diseases caused by endothelial disorders (Cheng et al., 2017; Shen et al., 2019) (Figure 4A).
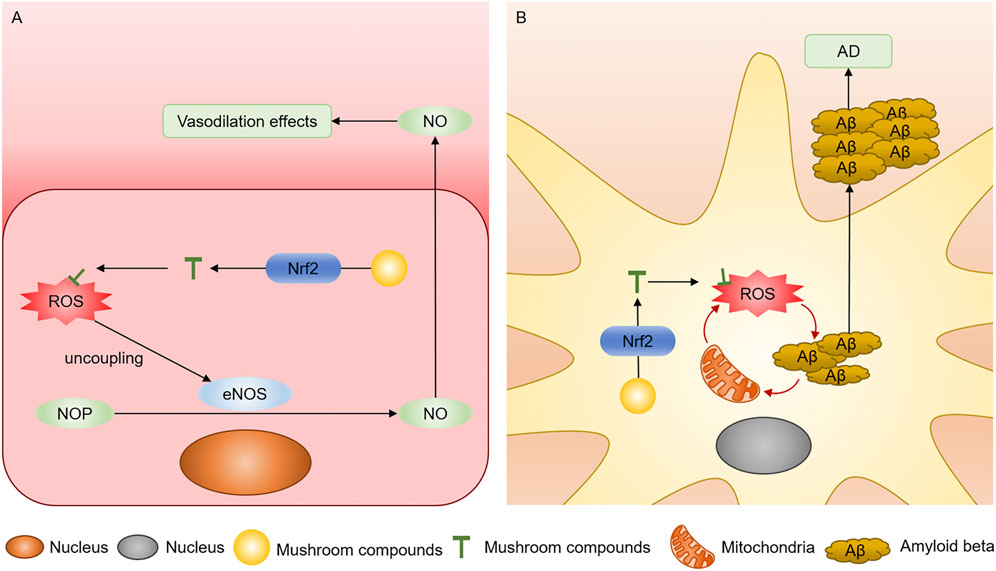
FIGURE 4. (A) Compounds in mushrooms inhibit eNOS uncoupling through the Nrf2 pathway. (B) Compounds in mushrooms neutralize ROS through the Nrf2 pathway and inhibit Aβ accumulation.
Ergothioneine is highly abundant in mushrooms and has the ability to scour ROS and chelate the metal cations. This phenomenon of ergothioneine could effectively regulate endovascular inflammation caused by endothelial dysfunction and reduce the release of pro-inflammatory factors, including interleukin-6 (IL-6), IL-1β, and TNF-α (Lam-Sidun et al., 2021). The water-soluble polysaccharide FC isolated from wild mushroom agaricus bitorquis could inhibit the NADPH oxidase activity, which might be used to treat myocardial infarction, hypertension, and atherosclerosis caused by excessive ROS (Jiao et al., 2019). In a previous study, the vasodilatation of phellinus linteus extracts was found in the rat mesenteric artery, which might induce the antioxidant and anti-inflammatory effects of polysaccharides, peptides, and terpenoids in the mycelium (Cai et al., 2019; Kwon et al., 2020; Zuo et al., 2021).
Neurodegenerative disease
Neurodegenerative diseases are characterized by the degeneration and loss of neurons in the brain and spinal cord, including Parkinson’s disease (PD), Alzheimer’s disease (AD), and Huntington’s disease (Djajadikerta et al., 2020). Lipids in the brain play a vital role in the function of neurons, which are vulnerable to being attacked by ROS and produce lipid peroxidation to form malondialdehyde (MDA), inducing reduced membrane fluidity and neuron degeneration (Singh et al., 2019). Meanwhile, the damaged neurons activate microglia and astrocytes to release the pro-inflammatory cytokines and ROS, further exacerbating neurodegeneration (Fischer and Maier, 2015). ROS produced by oxidative stress could damage and mutate the mitochondrial DNA, increasing oxidative decomposition of dopamine as the pathogenesis of neurodegenerative diseases and the accumulation of abnormal protein, such as amyloid beta (Aβ) in AD (Figure 4B) (Lin and Beal, 2006). Antioxidants in mushrooms could directly neutralize ROS or activate the Nrf2/ARE signaling pathway to induce the competence of antioxidant enzymes (Elfawy and Das, 2019).
Ethanol extracts from hericium erinaceus could alleviate the mitochondrial damage caused by H2O2 and activate antioxidant enzymes to treat hippocampal neurons’ injury in mice (Kushairi et al., 2019). The polysaccharide peptides and vitamins in coriolus versicolor could significantly reduce the proportion of GSH and oxidized glutathione (GSSG) in the plasma to relieve the systemic oxidative stress state and nerve injury of patients (Scuto et al., 2019). Meroterpenoids from Albatrellus yasudae have been found to inhibit Aβ aggregation, which might be a therapeutic and health care product for AD (Masuda et al., 2021). Polyphenols, polysaccharides, and triterpenes in Amauroderma rugosum could reduce oxidative stress and 6-hydroxydopamine-induced mitochondria dysfunction in the PC12 cells (Li et al., 2021b).
Diabetes mellitus
The occurrence of diabetes mellitus is related to the dysfunction of islet β cells and insulin resistance, in which oxidative stress plays a vital role in regulating multiple signaling pathways (Zhang et al., 2020). Excessive ROS can activate the NF-κB, JNK/SAPK, and p38 MAPK pathways by activating TLR and inducing the dysfunction of islet β cells (Yaribeygi et al., 2020). A persistent hyperglycemic environment induces ROS production through the binding of advanced glycation end products (AGE) to peroxisome proliferators activated receptors (PPAR) (Deng et al., 2021). Additionally, excessive production of AGE increases the expression of inflammatory mediators through the NF-κB pathway (Karam et al., 2017). The inflammatory factor TNF-α activates the intracellular signaling factor IKKβ that affects insulin production through the NF-κB signaling pathway (Wellen and Hotamisligil, 2005; Rohm et al., 2022).
Polysaccharides isolated from Inonotus obliquus are the potential to treat type 2 diabetes by directly removing ROS or mediating lipid peroxidation (Lu et al., 2021). Terpenoids from Antrodia camphorata could significantly inhibit the binding of AGE to PPAR and reduce blood glucose levels, thus inhibiting ROS production and promoting insulin secretion (Kuang et al., 2021). Alkali-soluble polysaccharides from Amillariella mellea could improve pancreatic B-cell dysfunction caused by oxidative stress, thereby improving insulin sensitivity and reducing insulin resistance (Yang et al., 2019). As food supplements, P. Ostreatus and L. Subnudus have been shown to increase the activity of antioxidant enzymes and non-enzymatic antioxidants, inducing memory loss in diabetic rats (Agunloye and Oboh, 2021).
Cancer
ROS can induce chronic inflammation, and the continuous inflammatory environment and oxidative stress might damage the adjacent epithelial cells and stromal cells, inducing cancer (Reuter et al., 2010). Additionally, ROS induces the proliferation of cancer cells and promotes tumor growth by activating the MAPK pathway (Moloney and Cotter, 2018). Tumor cell metastasis depends on the epithelial-mesenchymal transformation process, in which ROS activates the proteins, including β -catenin, e-cadherin, and matrix metalloproteinases (MMP), through the Wnt/β -catenin signaling pathway (Sosa et al., 2013).
Termitomyces Clypeatus significantly inhibited the tumor volume and number of ascites carcinoma mice by inhibiting lipid peroxidation and increasing the levels of GSH, SOD, and CAT (Mondal et al., 2016). The extracts from Lactarius deliciosus and Coprinus comatus inhibited the activity of MMP produced by oxidative stress and induced apoptosis in gliomas associated with G1 or G2/M phase cell cycle stagnation (Nowakowski et al., 2021). Natural antioxidants isolated from Thelephora Ganbajun by ultrasonic-assisted technology exhibited anti-proliferation effects on the liver and lung cancer cells (Xu et al., 2016). Antrodia salmonea induces protective autophagy and apoptosis in colon cancer cells through cascades of extracellular signal kinase (ERK) signaling, which has been reported to mediate ROS due to the double-sided effects of antioxidants on cancer cells (Dastmalchi et al., 2020; Yang et al., 2021).
The compounds purified from mushrooms might possess promising applications in preventing and treating cardiovascular diseases, neurodegenerative diseases, and cancer by regulating oxidative stress and chronic inflammation.
Discussion
Excessive ROS increases the release of pro-inflammatory factors, thereby promoting ROS production, which is the primary reason for oxidative stress-induced chronic inflammation. Chronic inflammation can lead to organic diseases in different tissues and organs over time. Chronic inflammation in the blood vessels can affect the vascular endothelial relaxation function and form atherosclerosis, the root cause of coronary atherosclerotic heart disease (Golia et al., 2014). It is reported that chronic inflammatory response persists in the neurodegeneration of AD and is considered an important factor in accelerating the progression (Gasiorowski et al., 2018). In chronic inflammation, ROS can damage DNA and cause biological macromolecule dysfunction, such as proteins and lipids, inducing antioxidant dysfunction and a vicious cycle of oxidative stress, a risk factor for cancer (Murata, 2018). In recent years, antioxidants have attracted much attention due to their oxidative stress combating ability to inhibit chronic inflammation. Natural compounds are more easily recognized and absorbed by the body than synthetic antioxidants, with no toxic side effects (Liu, 2022). Chinese herbs, including Astragalus membranaceus, berberine, and curcumin have also been proven to have antioxidant properties (Kocaadam and Sanlier, 2017; Liu et al., 2017; Song et al., 2020). The separation and purification process of Chinese herbs extract is complicated, implying that purity can affect its application. Meanwhile, improper usage might lead to toxicoses (Hu et al., 2005; Liu et al., 2018). In contrast, mushrooms are widely distributed, easy to process, and can provide various nutrients with simple treatments (Ahmad et al., 2021). Additionally, mushrooms are well absorbed by the human body with no harm as the natural antioxidant (Fontes et al., 2019).
Mushrooms possess many biological and pharmacological characteristics, which are already applied in nutrition, health care, and medical treatment (Venturella et al., 2021). Therefore, studies pay more attention to mushroom-derived polysaccharides than other compounds, as the purification method is mature and the biological activity is stable and diverse (Muszynska et al., 2018). Mushroom polysaccharides mainly contain β-glucan chains, which have been proven to have antioxidant effects (Maity et al., 2021). The hydrogen ions of mushroom polysaccharides could inhibit oxidative stress by neutralizing ROS and enhance antioxidant effects through chemical modification, including carboxymethylation and sulphuration (Huang and Nie, 2015; Lu et al., 2020). The extraction method significantly affected the antioxidant activity of the compound. Water extraction of Lepista nuda polysaccharides showed better scavenging ability of free radicals than ethanol extraction (Shu et al., 2019). The same compound had different scavenging abilities for different free radicals. For instance, Sanghuang could scavenge 68% ·OH and 57% O2—at 1 mg/ml, indicating that the scavenging ability of most compounds in the mushrooms is concentration-dependent (Zuo et al., 2021). Mushrooms are a promising source of natural antioxidants, ergothioneine, and glutathione, with the highest content in the mushroom cap of yellow oyster (Kalaras et al., 2017). However, more research on different species of natural compounds purified from mushrooms should be conducted to broaden their application.
In conclusion, the compounds in mushrooms could inhibit oxidative stress and chronic inflammation by neutralizing excessive ROS or activating antioxidant enzymes through the Nrf2 signaling pathway and have been applied to cardiovascular disease, neurodegenerative disease, and cancer.
Author contributions
YX and DW wrote the manuscript. JL, MC, DW, ZJ, and BL collected the references and prepared figures. All authors reviewed the manuscript.
Funding
This research was financially supported by the National Natural Science Foundation of China (Grant Nos. 52022095, 82071391, 51973216, 51873207, and 51833010), the Provincial Health Special Project of Jilin Province (Grant Nos. JLSCZD 2019-002 and JLSWSRCZX 2020-095), the Science and Technology Development Program of Jilin Province (Grant No. 20200404182YY), the Youth Innovation Promotion Association of Chinese Academy of Sciences (Grant No. 2019230), and Natural science Foundation of the Jilin province (Grant No. 20210101310JC). Project of science and Technology Department of Jilin Province (Grant No. 20220505033ZP).
Conflict of interest
The authors declare that the research was conducted in the absence of any commercial or financial relationships that could be construed as a potential conflict of interest.
Publisher’s note
All claims expressed in this article are solely those of the authors and do not necessarily represent those of their affiliated organizations, or those of the publisher, the editors and the reviewers. Any product that may be evaluated in this article, or claim that may be made by its manufacturer, is not guaranteed or endorsed by the publisher.
Supplementary material
The Supplementary Material for this article can be found online at: https://www.frontiersin.org/articles/10.3389/fphar.2022.974794/full#supplementary-material
SUPPLEMENTARY FIGURE S1Structure of compounds purified from mushrooms, with hydrogen atoms in red as active sites against oxidative stress. N is the number of cycles. (A) Structure of mushroom polysaccharide. (B) Structure of mushroom peptides. (C) Structure of mushroom polyphenols, with flavonoids as an example.
References
Agunloye, O. M., and Oboh, G. (2021). Effect of diet supplemented with P. ostreatus and L. subnudus on memory index and key enzymes linked with Alzheimer's disease in streptozotocin-induced diabetes rats. J. Food Biochem. 45 (3), e13355. doi:10.1111/jfbc.13355
Ahmad, R., Riaz, M., Khan, A., Aljamea, A., Algheryafi, M., Sewaket, D., et al. (2021). Ganoderma lucidum (Reishi) an edible mushroom; a comprehensive and critical review of its nutritional, cosmeceutical, mycochemical, pharmacological, clinical, and toxicological properties. Phytother. Res. 35 (11), 6030–6062. doi:10.1002/ptr.7215
Alves, M. J., Ferreira, I. C., Dias, J., Teixeira, V., Martins, A., and Pintado, M. (2012). A review on antimicrobial activity of mushroom (Basidiomycetes) extracts and isolated compounds. Planta Med. 78 (16), 1707–1718. doi:10.1055/s-0032-1315370
Aprotosoaie, A. C., Zavastin, D. E., Mihai, C. T., Voichita, G., Gherghel, D., Silion, M., et al. (2017). Antioxidant and antigenotoxic potential of Ramaria largentii Marr & D. E. Stuntz, a wild edible mushroom collected from Northeast Romania. Food Chem. Toxicol. 108, 429–437. doi:10.1016/j.fct.2017.02.006
Athanasakis, G., Aligiannis, N., Gonou-Zagou, Z., Skaltsounis, A. L., and Fokialakis, N. (2013). Antioxidant properties of the wild edible mushroom Lactarius salmonicolor. J. Med. Food 16 (8), 760–764. doi:10.1089/jmf.2012.0297
Bao, H. N., Ushio, H., and Ohshima, T. (2008). Antioxidative activity and antidiscoloration efficacy of ergothioneine in mushroom (Flammulina velutipes) extract added to beef and fish meats. J. Agric. Food Chem. 56 (21), 10032–10040. doi:10.1021/jf8017063
Barbosa, J. R., Mm, S. F., Oliveira, L. C., Lh, S. M., Almada-Vilhena, A. O., Oliveira, R. M., et al. (2020). Obtaining extracts rich in antioxidant polysaccharides from the edible mushroom Pleurotus ostreatus using binary system with hot water and supercritical CO2. Food Chem. 330, 127173. doi:10.1016/j.foodchem.2020.127173
Bhatti, J. S., Bhatti, G. K., and Reddy, P. H. (2017). Mitochondrial dysfunction and oxidative stress in metabolic disorders - a step towards mitochondria based therapeutic strategies. Biochim. Biophys. Acta. Mol. Basis Dis. 1863 (5), 1066–1077. doi:10.1016/j.bbadis.2016.11.010
Cadenas, E., and Davies, K. J. (2000). Mitochondrial free radical generation, oxidative stress, and aging. Free Radic. Biol. Med. 29 (3-4), 222–230. doi:10.1016/s0891-5849(00)00317-8
Cai, C., Ma, J., Han, C., Jin, Y., Zhao, G., and He, X. (2019). Extraction and antioxidant activity of total triterpenoids in the mycelium of a medicinal fungus, Sanghuangporus sanghuang. Sci. Rep. 9 (1), 7418. doi:10.1038/s41598-019-43886-0
Chen, F., Huang, S., and Huang, G. (2021). Preparation, activity, and antioxidant mechanism of rice bran polysaccharide. Food Funct. 12 (2), 834–839. doi:10.1039/d0fo02498h
Chen, X., Fang, D., Zhao, R., Gao, J., Kimatu, B. M., Hu, Q., et al. (2019). Effects of ultrasound-assisted extraction on antioxidant activity and bidirectional immunomodulatory activity of Flammulina velutipes polysaccharide. Int. J. Biol. Macromol. 140, 505–514. doi:10.1016/j.ijbiomac.2019.08.163
Cheng, Y. C., Sheen, J. M., Hu, W. L., and Hung, Y. C. (2017). Polyphenols and oxidative stress in atherosclerosis-related ischemic heart disease and stroke. Oxid. Med. Cell. Longev. 2017, 8526438. doi:10.1155/2017/8526438
Chun, S., Gopal, J., and Muthu, M. (2021). Antioxidant activity of mushroom extracts/polysaccharides-their antiviral properties and plausible AntiCOVID-19 properties. Antioxidants (Basel) 10 (12), 1899. doi:10.3390/antiox10121899
Dastmalchi, N., Baradaran, B., Latifi-Navid, S., Safaralizadeh, R., Khojasteh, S. M. B., Amini, M., et al. (2020). Antioxidants with two faces toward cancer. Life Sci. 258, 118186. doi:10.1016/j.lfs.2020.118186
Deng, L., Du, C., Song, P., Chen, T., Rui, S., Armstrong, D. G., et al. (2021). The role of oxidative stress and antioxidants in diabetic wound healing. Oxid. Med. Cell. Longev. 2021, 8852759. doi:10.1155/2021/8852759
Djajadikerta, A., Keshri, S., Pavel, M., Prestil, R., Ryan, L., and Rubinsztein, D. C. (2020). Autophagy induction as a therapeutic strategy for neurodegenerative diseases. J. Mol. Biol. 432 (8), 2799–2821. doi:10.1016/j.jmb.2019.12.035
Elahi, M. M., Kong, Y. X., and Matata, B. M. (2009). Oxidative stress as a mediator of cardiovascular disease. Oxid. Med. Cell. Longev. 2 (5), 259–269. doi:10.4161/oxim.2.5.9441
Elfawy, H. A., and Das, B. (2019). Crosstalk between mitochondrial dysfunction, oxidative stress, and age related neurodegenerative disease: Etiologies and therapeutic strategies. Life Sci. 218, 165–184. doi:10.1016/j.lfs.2018.12.029
Erdmann, K., Cheung, B. W., and Schroder, H. (2008). The possible roles of food-derived bioactive peptides in reducing the risk of cardiovascular disease. J. Nutr. Biochem. 19 (10), 643–654. doi:10.1016/j.jnutbio.2007.11.010
Feng, Q., Li, Y., Lu, X., Yu, Y., Yuan, G., Sun, J., et al. (2021). Agaricus blazei polypeptide exerts a protective effect on D-galactose-induced aging mice via the Keap1/Nrf2/ARE and P53/Trim32 signaling pathways. J. Food Biochem. 45 (1), e13555. doi:10.1111/jfbc.13555
Fischer, R., and Maier, O. (2015). Interrelation of oxidative stress and inflammation in neurodegenerative disease: Role of TNF. Oxid. Med. Cell. Longev. 2015, 610813. doi:10.1155/2015/610813
Fontes, A., Alemany-Pages, M., Oliveira, P. J., Ramalho-Santos, J., Zischka, H., and Azul, A. M. (2019). Antioxidant versus pro-apoptotic effects of mushroom-enriched diets on mitochondria in liver disease. Int. J. Mol. Sci. 20 (16), E3987. doi:10.3390/ijms20163987
Fogarasi, M., Socaciu, M. I., Salagean, C. D., Ranga, F., Farcas, A. C., Socaci, S. A., et al. (2021). Comparison of different extraction solvents for characterization of antioxidant potential and polyphenolic composition in boletus edulis and cantharellus cibarius mushrooms from Romania. Molecules 26 (24), 7508. doi:10.3390/molecules26247508
Fontoura, R., Daroit, D. J., Correa, A. P. F., Moresco, K. S., Santi, L., Beys-da-Silva, W. O., et al. (2019). Characterization of a novel antioxidant peptide from feather keratin hydrolysates. N. Biotechnol. 49, 71–76. doi:10.1016/j.nbt.2018.09.003
Gasiorowski, K., Brokos, B., Echeverria, V., Barreto, G. E., and Leszek, J. (2018). RAGE-TLR crosstalk sustains chronic inflammation in neurodegeneration. Mol. Neurobiol. 55 (2), 1463–1476. doi:10.1007/s12035-017-0419-4
Golia, E., Limongelli, G., Natale, F., Fimiani, F., Maddaloni, V., Pariggiano, I., et al. (2014). Inflammation and cardiovascular disease: From pathogenesis to therapeutic target. Curr. Atheroscler. Rep. 16 (9), 435. doi:10.1007/s11883-014-0435-z
Griendling, K. K., Camargo, L. L., Rios, F. J., Alves-Lopes, R., Montezano, A. C., and Touyz, R. M. (2021). Oxidative stress and hypertension. Circ. Res. 128 (7), 993–1020. doi:10.1161/CIRCRESAHA.121.318063
Homer, J. A., and Sperry, J. (2017). Mushroom-Derived indole alkaloids. J. Nat. Prod. 80 (7), 2178–2187. doi:10.1021/acs.jnatprod.7b00390
Hu, Q., Yu, J., Yang, W., Kimatu, B. M., Fang, Y., Ma, N., et al. (2016). Identification of flavonoids from Flammulina velutipes and its neuroprotective effect on pheochromocytoma-12 cells. Food Chem. 204, 274–282. doi:10.1016/j.foodchem.2016.02.138
Hu, W., Li, Z., Wang, W., Song, M., Dong, R., Zhou, Y., et al. (2021). Structural characterization of polysaccharide purified from amanita caesarea and its pharmacological basis for application in alzheimer's disease: Endoplasmic reticulum stress. Food Funct. 12 (21), 11009–11023. doi:10.1039/d1fo01963e
Hu, Z., Yang, X., Ho, P. C., Chan, S. Y., Heng, P. W., Chan, E., et al. (2005). Herb-drug interactions: A literature review. Drugs 65 (9), 1239–1282. doi:10.2165/00003495-200565090-00005
Huang, G., Mei, X., and Hu, J. (2017). The antioxidant activities of natural polysaccharides. Curr. Drug Targets 18 (11), 1296–1300. doi:10.2174/1389450118666170123145357
Huang, X., and Nie, S. (2015). The structure of mushroom polysaccharides and their beneficial role in health. Food Funct. 6 (10), 3205–3217. doi:10.1039/c5fo00678c
Jayakumar, T., Thomas, P. A., and Geraldine, P. (2007). Protective effect of an extract of the oyster mushroom, Pleurotus ostreatus, on antioxidants of major organs of aged rats. Exp. Gerontol. 42 (3), 183–191. doi:10.1016/j.exger.2006.10.006
Jiao, Y., Kuang, H., Wu, J., and Chen, Q. (2019). Polysaccharides from the edible mushroom Agaricus bitorquis (quel.) sacc. Chaidam show anti-hypoxia activities in pulmonary artery smooth muscle cells. Int. J. Mol. Sci. 20 (3), E637. doi:10.3390/ijms20030637
Jin, X., Ruiz Beguerie, J., Sze, D. M., and Chan, G. C. (2016). Ganoderma lucidum (Reishi mushroom) for cancer treatment. Cochrane Database Syst. Rev. 4, CD007731. doi:10.1002/14651858.CD007731.pub3
Kala, K., Krakowska, A., Szewczyk, A., Ostachowicz, B., Szczurek, K., Fijalkowska, A., et al. (2021). Determining the amount of potentially bioavailable phenolic compounds and bioelements in edible mushroom mycelia of Agaricus bisporus, Cantharellus cibarius, and Lentinula edodes. Food Chem. 352, 129456. doi:10.1016/j.foodchem.2021.129456
Kalaras, M. D., Richie, J. P., Calcagnotto, A., and Beelman, R. B. (2017). Mushrooms: A rich source of the antioxidants ergothioneine and glutathione. Food Chem. 233, 429–433. doi:10.1016/j.foodchem.2017.04.109
Karam, B. S., Chavez-Moreno, A., Koh, W., Akar, J. G., and Akar, F. G. (2017). Oxidative stress and inflammation as central mediators of atrial fibrillation in obesity and diabetes. Cardiovasc. Diabetol. 16 (1), 120. doi:10.1186/s12933-017-0604-9
Kimatu, B. M., Zhao, L., Biao, Y., Ma, G., Yang, W., Pei, F., et al. (2017). Antioxidant potential of edible mushroom (Agaricus bisporus) protein hydrolysates and their ultrafiltration fractions. Food Chem. 230, 58–67. doi:10.1016/j.foodchem.2017.03.030
Klaunig, J. E., Wang, Z., Pu, X., and Zhou, S. (2011). Oxidative stress and oxidative damage in chemical carcinogenesis. Toxicol. Appl. Pharmacol. 254 (2), 86–99. doi:10.1016/j.taap.2009.11.028
Klupp, N. L., Chang, D., Hawke, F., Kiat, H., Cao, H., Grant, S. J., et al. (2015). Ganoderma lucidum mushroom for the treatment of cardiovascular risk factors. Cochrane Database Syst. Rev. 2021 (2), CD007259. doi:10.1002/14651858.CD007259.pub2
Kocaadam, B., and Sanlier, N. (2017). Curcumin, an active component of turmeric (Curcuma longa), and its effects on health. Crit. Rev. Food Sci. Nutr. 57 (13), 2889–2895. doi:10.1080/10408398.2015.1077195
Kozarski, M., Klaus, A., Jakovljevic, D., Todorovic, N., Vunduk, J., Petrovic, P., et al. (2015). Antioxidants of edible mushrooms. Molecules 20 (10), 19489–19525. doi:10.3390/molecules201019489
Kuang, Y., Li, B., Wang, Z., Qiao, X., and Ye, M. (2021). Terpenoids from the medicinal mushroom Antrodia camphorata: Chemistry and medicinal potential. Nat. Prod. Rep. 38 (1), 83–102. doi:10.1039/d0np00023j
Kumar, V., and Gill, K. D. (2014). Oxidative stress and mitochondrial dysfunction in aluminium neurotoxicity and its amelioration: A review. Neurotoxicology 41, 154–166. doi:10.1016/j.neuro.2014.02.004
Kumla, J., Suwannarach, N., Tanruean, K., and Lumyong, S. (2021). Comparative evaluation of chemical composition, phenolic compounds, and antioxidant and antimicrobial activities of tropical black bolete mushroom using different preservation methods. Foods 10 (4), 781. doi:10.3390/foods10040781
Kushairi, N., Phan, C. W., Sabaratnam, V., David, P., and Naidu, M. (2019). Lion's mane mushroom, hericium erinaceus (bull.: Fr.) pers. Suppresses H2O2-induced oxidative damage and LPS-induced inflammation in HT22 hippocampal neurons and BV2 microglia. Antioxidants (Basel) 8 (8), E261. doi:10.3390/antiox8080261
Kwon, Y., Haam, C. E., Byeon, S., Choi, S. J., Shin, D. H., Choi, S. K., et al. (2020). Vasodilatory effect of phellinus linteus extract in rat mesenteric arteries. Molecules 25 (14), E3160. doi:10.3390/molecules25143160
Lam-Sidun, D., Peters, K. M., and Borradaile, N. M. (2021). Mushroom-derived medicine? Preclinical studies suggest potential benefits of ergothioneine for cardiometabolic health. Int. J. Mol. Sci. 22 (6), 3246. doi:10.3390/ijms22063246
Lee, I. K., Kim, Y. S., Jang, Y. W., Jung, J. Y., and Yun, B. S. (2007). New antioxidant polyphenols from the medicinal mushroom Inonotus obliquus. Bioorg. Med. Chem. Lett. 17 (24), 6678–6681. doi:10.1016/j.bmcl.2007.10.072
Leong, Y. K., Yang, F. C., and Chang, J. S. (2021). Extraction of polysaccharides from edible mushrooms: Emerging technologies and recent advances. Carbohydr. Polym. 251, 117006. doi:10.1016/j.carbpol.2020.117006
Leuti, A., Fazio, D., Fava, M., Piccoli, A., Oddi, S., and Maccarrone, M. (2020). Bioactive lipids, inflammation and chronic diseases. Adv. Drug Deliv. Rev. 159, 133–169. doi:10.1016/j.addr.2020.06.028
Li, J., Li, R., Wu, X., Hoo, R. L., Lee, S. M., Cheung, T. M., et al. (2021). Amauroderma rugosum protects PC12 cells against 6-OHDA-induced neurotoxicity through antioxidant and antiapoptotic effects. Oxid. Med. Cell. Longev. 2021, 6683270. doi:10.1155/2021/6683270
Li, M., Lv, R., Wang, C., Ge, Q., Du, H., and Lin, S. (2021). Tricholoma matsutake-derived peptide WFNNAGP protects against DSS-induced colitis by ameliorating oxidative stress and intestinal barrier dysfunction. Food Funct. 12 (23), 11883–11897. doi:10.1039/d1fo02806e
Li, W., Lee, S. H., Jang, H. D., Ma, J. Y., and Kim, Y. H. (2017a). Antioxidant and anti-osteoporotic activities of aromatic compounds and sterols from hericium erinaceum. Molecules 22 (1), 108. doi:10.3390/molecules22010108
Li, X., Wang, L., and Wang, Z. (2017b). Structural characterization and antioxidant activity of polysaccharide from Hohenbuehelia serotina. Int. J. Biol. Macromol. 98, 59–66. doi:10.1016/j.ijbiomac.2016.12.089
Li, X., He, Y., Zeng, P., Liu, Y., Zhang, M., Hao, C., et al. (2019). Molecular basis for Poria cocos mushroom polysaccharide used as an antitumour drug in China. J. Cell. Mol. Med. 23 (1), 4–20. doi:10.1111/jcmm.13564
Liao, Z., Zhang, J., Liu, B., Yan, T., Xu, F., Xiao, F., et al. (2019). Polysaccharide from okra (abelmoschus esculentus (L.) moench) improves antioxidant capacity via PI3K/AKT pathways and Nrf2 translocation in a type 2 diabetes model. Molecules 24 (10), E1906. doi:10.3390/molecules24101906
Lin, M. T., and Beal, M. F. (2006). Mitochondrial dysfunction and oxidative stress in neurodegenerative diseases. Nature 443 (7113), 787–795. doi:10.1038/nature05292
Liu, J., Jia, L., Kan, J., and Jin, C. H. (2013a). In vitro and in vivo antioxidant activity of ethanolic extract of white button mushroom (Agaricus bisporus). Food Chem. Toxicol. 51, 310–316. doi:10.1016/j.fct.2012.10.014
Liu, J., Wu, Q., Yang, T., Yang, F., Guo, T., Zhou, Y., et al. (2021). Bioactive peptide F2d isolated from rice residue exerts antioxidant effects via Nrf2 signaling pathway. Oxid. Med. Cell. Longev. 2021, 2637577. doi:10.1155/2021/2637577
Liu, K., Wang, J., Zhao, L., and Wang, Q. (2013b). Anticancer, antioxidant and antibiotic activities of mushroom Ramaria flava. Food Chem. Toxicol. 58, 375–380. doi:10.1016/j.fct.2013.05.001
Liu, P., Zhao, H., and Luo, Y. (2017). Anti-aging implications of Astragalus membranaceus (huangqi): A well-known Chinese tonic. Aging Dis. 8 (6), 868–886. doi:10.14336/AD.2017.0816
Liu, Y., Gao, J., Peng, M., Meng, H., Ma, H., Cai, P., et al. (2018). A review on central nervous system effects of gastrodin. Front. Pharmacol. 9, 24. doi:10.3389/fphar.2018.00024
Liu, Z. Q. (2022). Why natural antioxidants are readily recognized by biological systems? 3D architecture plays a role. Food Chem. 380, 132143. doi:10.1016/j.foodchem.2022.132143
Lu, J., He, R., Sun, P., Zhang, F., Linhardt, R. J., and Zhang, A. (2020). Molecular mechanisms of bioactive polysaccharides from Ganoderma lucidum (Lingzhi), a review. Int. J. Biol. Macromol. 150, 765–774. doi:10.1016/j.ijbiomac.2020.02.035
Lu, Y., Jia, Y., Xue, Z., Li, N., Liu, J., and Chen, H. (2021). Recent developments in Inonotus obliquus (chaga mushroom) polysaccharides: Isolation, structural characteristics, biological activities and application. Polym. (Basel) 13 (9), 1441. doi:10.3390/polym13091441
Lv, J. H., Yao, L., Zhang, J. X., Wang, L. A., Zhang, J., Wang, Y. P., et al. (2021). Novel 2, 5-diarylcyclopentenone derivatives from the wild edible mushroom Paxillus involutus and their antioxidant activities. J. Agric. Food Chem. 69 (17), 5040–5048. doi:10.1021/acs.jafc.1c01160
Maity, P., Samanta, S., Nandi, A. K., Sen, I. K., Paloi, S., Acharya, K., et al. (2014). Structure elucidation and antioxidant properties of a soluble beta-D-glucan from mushroom Entoloma lividoalbum. Int. J. Biol. Macromol. 63, 140–149. doi:10.1016/j.ijbiomac.2013.10.040
Maity, P., Sen, I. K., Chakraborty, I., Mondal, S., Bar, H., Bhanja, S. K., et al. (2021). Biologically active polysaccharide from edible mushrooms: A review. Int. J. Biol. Macromol. 172, 408–417. doi:10.1016/j.ijbiomac.2021.01.081
Maity, P., Sen, I. K., Maji, P. K., Paloi, S., Devi, K. S., Acharya, K., et al. (2015). Structural, immunological, and antioxidant studies of beta-glucan from edible mushroom Entoloma lividoalbum. Carbohydr. Polym. 123, 350–358. doi:10.1016/j.carbpol.2015.01.051
Man, A. W. C., Li, H., and Xia, N. (2020). Impact of lifestyles (diet and exercise) on vascular health: Oxidative stress and endothelial function. Oxid. Med. Cell. Longev. 2020, 1496462. doi:10.1155/2020/1496462
Masuda, Y., Fujihara, K., Hayashi, S., Sasaki, H., Kino, Y., Kamauchi, H., et al. (2021). Inhibition of BACE1 and amyloid-beta aggregation by meroterpenoids from the mushroom Albatrellus yasudae. J. Nat. Prod. 84 (6), 1748–1754. doi:10.1021/acs.jnatprod.0c01329
Medzhitov, R. (2008). Origin and physiological roles of inflammation. Nature 454 (7203), 428–435. doi:10.1038/nature07201
Meng, P., Yang, R., Jiang, F., Guo, J., Lu, X., Yang, T., et al. (2021). Molecular mechanism of astragaloside IV in improving endothelial dysfunction of cardiovascular diseases mediated by oxidative stress. Oxid. Med. Cell. Longev. 2021, 1481236. doi:10.1155/2021/1481236
Miller, M. R. (2020). Oxidative stress and the cardiovascular effects of air pollution. Free Radic. Biol. Med. 151, 69–87. doi:10.1016/j.freeradbiomed.2020.01.004
Mishra, V., Banga, J., and Silveyra, P. (2018). Oxidative stress and cellular pathways of asthma and inflammation: Therapeutic strategies and pharmacological targets. Pharmacol. Ther. 181, 169–182. doi:10.1016/j.pharmthera.2017.08.011
Mishra, J., Rajput, R., Singh, K., Bansal, A., and Misra, K. (2019). Antioxidant-Rich peptide fractions derived from high-altitude Chinese caterpillar medicinal mushroom ophiocordyceps sinensis (ascomycetes) inhibit bacterial pathogens. Int. J. Med. Mushrooms 21 (2), 155–168. doi:10.1615/IntJMedMushrooms.2019030013
Moloney, J. N., and Cotter, T. G. (2018). ROS signalling in the biology of cancer. Semin. Cell. Dev. Biol. 80, 50–64. doi:10.1016/j.semcdb.2017.05.023
Mondal, A., Banerjee, D., Majumder, R., Maity, T. K., and Khowala, S. (2016). Evaluation of in vitro antioxidant, anticancer and in vivo antitumour activity of Termitomyces clypeatus MTCC 5091. Pharm. Biol. 54 (11), 2536–2546. doi:10.3109/13880209.2016.1168854
Murata, M. (2018). Inflammation and cancer. Environ. Health Prev. Med. 23 (1), 50. doi:10.1186/s12199-018-0740-1
Muszynska, B., Grzywacz-Kisielewska, A., Kala, K., and Gdula-Argasinska, J. (2018). Anti-inflammatory properties of edible mushrooms: A review. Food Chem. 243, 373–381. doi:10.1016/j.foodchem.2017.09.149
Navegantes-Lima, K. C., Monteiro, V. V. S., de Franca Gaspar, S. L., de Brito Oliveira, A. L., de Oliveira, J. P., Reis, J. F., et al. (2020). Agaricus brasiliensis mushroom protects against Sepsis by alleviating oxidative and inflammatory response. Front. Immunol. 11, 1238. doi:10.3389/fimmu.2020.01238
Nolfi-Donegan, D., Braganza, A., and Shiva, S. (2020). Mitochondrial electron transport chain: Oxidative phosphorylation, oxidant production, and methods of measurement. Redox Biol. 37, 101674. doi:10.1016/j.redox.2020.101674
Nowakowski, P., Markiewicz-Zukowska, R., Gromkowska-Kepka, K., Naliwajko, S. K., Moskwa, J., Bielecka, J., et al. (2021). Mushrooms as potential therapeutic agents in the treatment of cancer: Evaluation of anti-glioma effects of Coprinus comatus, Cantharellus cibarius, Lycoperdon perlatum and Lactarius deliciosus extracts. Biomed. Pharmacother. 133, 111090. doi:10.1016/j.biopha.2020.111090
Peoples, J. N., Saraf, A., Ghazal, N., Pham, T. T., and Kwong, J. Q. (2019). Mitochondrial dysfunction and oxidative stress in heart disease. Exp. Mol. Med. 51 (12), 1–13. doi:10.1038/s12276-019-0355-7
Petrovic, J., Papandreou, M., Glamoclija, J., Ciric, A., Baskakis, C., Proestos, C., et al. (2014). Different extraction methodologies and their influence on the bioactivity of the wild edible mushroom Laetiporus sulphureus (Bull.) Murrill. Food Funct. 5 (11), 2948–2960. doi:10.1039/c4fo00727a
Pizzino, G., Irrera, N., Cucinotta, M., Pallio, G., Mannino, F., Arcoraci, V., et al. (2017). Oxidative stress: Harms and benefits for human health. Oxid. Med. Cell. Longev. 2017, 8416763. doi:10.1155/2017/8416763
Powers, S. K., and Jackson, M. J. (2008). Exercise-induced oxidative stress: Cellular mechanisms and impact on muscle force production. Physiol. Rev. 88 (4), 1243–1276. doi:10.1152/physrev.00031.2007
Qian, L., Liu, H., Li, T., Liu, Y., Zhang, Z., and Zhang, Y. (2020). Purification, characterization and in vitro antioxidant activity of a polysaccharide AAP-3-1 from Auricularia auricula. Int. J. Biol. Macromol. 162, 1453–1464. doi:10.1016/j.ijbiomac.2020.07.314
Quindry, J., Dumke, C., Slivka, D., and Ruby, B. (2016). Impact of extreme exercise at high altitude on oxidative stress in humans. J. Physiol. 594 (18), 5093–5104. doi:10.1113/JP270651
Reuter, S., Gupta, S. C., Chaturvedi, M. M., and Aggarwal, B. B. (2010). Oxidative stress, inflammation, and cancer: How are they linked? Free Radic. Biol. Med. 49 (11), 1603–1616. doi:10.1016/j.freeradbiomed.2010.09.006
Rodrigues Barbosa, J., Dos Santos Freitas, M. M., da Silva Martins, L. H., and de Carvalho, R. N. J. (2020). Polysaccharides of mushroom Pleurotus spp.: New extraction techniques, biological activities and development of new technologies. Carbohydr. Polym. 229, 115550. doi:10.1016/j.carbpol.2019.115550
Rohm, T. V., Meier, D. T., Olefsky, J. M., and Donath, M. Y. (2022). Inflammation in obesity, diabetes, and related disorders. Immunity 55 (1), 31–55. doi:10.1016/j.immuni.2021.12.013
Ryoo, I. G., and Kwak, M. K. (2018). Regulatory crosstalk between the oxidative stress-related transcription factor Nfe2l2/Nrf2 and mitochondria. Toxicol. Appl. Pharmacol. 359, 24–33. doi:10.1016/j.taap.2018.09.014
Sakemi, Y., Hagiwara, M., Oikawa, A., Sato, M., Sato, S., Sawa, N., et al. (2021). Antioxidant p-terphenyl compounds in the mushroom Boletopsis leucomelas (PERS.) FAYOD and how they change via cooking. Food Chem. 363, 130281. doi:10.1016/j.foodchem.2021.130281
Sande, D., Oliveira, G. P., Moura, M., Martins, B. A., Lima, M., and Takahashi, J. A. (2019). Edible mushrooms as a ubiquitous source of essential fatty acids. Food Res. Int. 125, 108524. doi:10.1016/j.foodres.2019.108524
Sangtitanu, T., Sangtanoo, P., Srimongkol, P., Saisavoey, T., Reamtong, O., and Karnchanatat, A. (2020). Peptides obtained from edible mushrooms: Hericium erinaceus offers the ability to scavenge free radicals and induce apoptosis in lung cancer cells in humans. Food Funct. 11 (6), 4927–4939. doi:10.1039/d0fo00227e
Sarkozy, A., Kusz, N., Zomborszki, Z. P., Csorba, A., Papp, V., Hohmann, J., et al. (2020). Isolation and characterization of chemical constituents from the poroid medicinal mushroom porodaedalea chrysoloma (agaricomycetes) and their antioxidant activity. Int. J. Med. Mushrooms 22 (2), 125–131. doi:10.1615/IntJMedMushrooms.2020033698
Sarfraz, A., Rasul, A., Sarfraz, I., Shah, M. A., Hussain, G., Shafiq, N., et al. (2020). Hispolon: A natural polyphenol and emerging cancer killer by multiple cellular signaling pathways. Environ. Res. 190, 110017. doi:10.1016/j.envres.2020.110017
Scuto, M., Di Mauro, P., Ontario, M. L., Amato, C., Modafferi, S., Ciavardelli, D., et al. (2019). Nutritional mushroom treatment in meniere's disease with coriolus versicolor: A rationale for therapeutic intervention in neuroinflammation and antineurodegeneration. Int. J. Mol. Sci. 21 (1), E284. doi:10.3390/ijms21010284
Shen, Y., Liu, X., Shi, J., and Wu, X. (2019). Involvement of Nrf2 in myocardial ischemia and reperfusion injury. Int. J. Biol. Macromol. 125, 496–502. doi:10.1016/j.ijbiomac.2018.11.190
Shu, X., Zhang, Y., Jia, J., Ren, X., and Wang, Y. (2019). Extraction, purification and properties of water-soluble polysaccharides from mushroom Lepista nuda. Int. J. Biol. Macromol. 128, 858–869. doi:10.1016/j.ijbiomac.2019.01.214
Singh, A., Kukreti, R., Saso, L., and Kukreti, S. (2019). Oxidative stress: A key modulator in neurodegenerative diseases. Molecules 24 (8), E1583. doi:10.3390/molecules24081583
Sinha, K., Das, J., Pal, P. B., and Sil, P. C. (2013). Oxidative stress: The mitochondria-dependent and mitochondria-independent pathways of apoptosis. Arch. Toxicol. 87 (7), 1157–1180. doi:10.1007/s00204-013-1034-4
Song, D., Hao, J., and Fan, D. (2020). Biological properties and clinical applications of berberine. Front. Med. 14 (5), 564–582. doi:10.1007/s11684-019-0724-6
Sonowal, H., Shukla, K., Kota, S., Saxena, A., and Ramana, K. V. (2018). Vialinin A, an edible mushroom-derived p-terphenyl antioxidant, prevents VEGF-induced neovascularization in vitro and in vivo. Oxid. Med. Cell. Longev. 2018, 1052102. doi:10.1155/2018/1052102
Sosa, V., Moline, T., Somoza, R., Paciucci, R., Kondoh, H., and Me, L. L. (2013). Oxidative stress and cancer: An overview. Ageing Res. Rev. 12 (1), 376–390. doi:10.1016/j.arr.2012.10.004
Stajic, M., Vukojevic, J., Knezevic, A., Lausevic, S. D., and Milovanovic, I. (2013). Antioxidant protective effects of mushroom metabolites. Curr. Top. Med. Chem. 13 (21), 2660–2676. doi:10.2174/15680266113136660192
Sun, Y., Hu, X., and Li, W. (2017). Antioxidant, antitumor and immunostimulatory activities of the polypeptide from Pleurotus eryngii mycelium. Int. J. Biol. Macromol. 97, 323–330. doi:10.1016/j.ijbiomac.2017.01.043
Tan, B. L., and Norhaizan, M. E. (2019). Effect of high-fat diets on oxidative stress, cellular inflammatory response and cognitive function. Nutrients 11 (11), E2579. doi:10.3390/nu11112579
Tan, B. L., Norhaizan, M. E., and Liew, W. P. (2018). Nutrients and oxidative stress: Friend or foe? Oxid. Med. Cell. Longev. 2018, 9719584. doi:10.1155/2018/9719584
Tee, J. K., Ong, C. N., Bay, B. H., Ho, H. K., and Leong, D. T. (2016). Oxidative stress by inorganic nanoparticles. Wiley Interdiscip. Rev. Nanomed. Nanobiotechnol. 8 (3), 414–438. doi:10.1002/wnan.1374
Thirupathi, A., Pinho, R. A., and Chang, Y. Z. (2020). Physical exercise: An inducer of positive oxidative stress in skeletal muscle aging. Life Sci. 252, 117630. doi:10.1016/j.lfs.2020.117630
Udeh, A. S., Ezebialu, C. U., Eze, E. A., and Engwa, G. A. (2021). Antibacterial and antioxidant activity of different extracts of some wild medicinal mushrooms from Nigeria. Int. J. Med. Mushrooms 23 (10), 83–95. doi:10.1615/IntJMedMushrooms.2021040197
Venturella, G., Ferraro, V., Cirlincione, F., and Gargano, M. L. (2021). Medicinal mushrooms: Bioactive compounds, use, and clinical trials. Int. J. Mol. Sci. 22 (2), E634. doi:10.3390/ijms22020634
Wang, J. H., Xu, J. L., Zhang, J. C., Liu, Y., Sun, H. J., and Zha, X. (2015). Physicochemical properties and antioxidant activities of polysaccharide from floral mushroom cultivated in Huangshan Mountain. Carbohydr. Polym. 131, 240–247. doi:10.1016/j.carbpol.2015.05.052
Wang, Q., Wang, F., Xu, Z., and Ding, Z. (2017). Bioactive mushroom polysaccharides: A review on monosaccharide composition, biosynthesis and regulation. Molecules 22 (6), 955. doi:10.3390/molecules22060955
Wellen, K. E., and Hotamisligil, G. S. (2005). Inflammation, stress, and diabetes. J. Clin. Invest. 115 (5), 1111–1119. doi:10.1172/JCI25102
West, A. P., Brodsky, I. E., Rahner, C., Woo, D. K., Erdjument-Bromage, H., Tempst, P., et al. (2011). TLR signalling augments macrophage bactericidal activity through mitochondrial ROS. Nature 472 (7344), 476–480. doi:10.1038/nature09973
Wongaem, A., Reamtong, O., Srimongkol, P., Sangtanoo, P., Saisavoey, T., and Karnchanatat, A. (2021). Antioxidant properties of peptides obtained from the split gill mushroom (Schizophyllum commune). J. Food Sci. Technol. 58 (2), 680–691. doi:10.1007/s13197-020-04582-4
Wu, J., Xia, S., Kalionis, B., Wan, W., and Sun, T. (2014). The role of oxidative stress and inflammation in cardiovascular aging. Biomed. Res. Int. 2014, 615312. doi:10.1155/2014/615312
Xi, X. J., Zeng, J. J., Lu, Y., Chen, S. H., Jiang, Z. W., He, P. J., et al. (2020). Extracellular vesicles enhance oxidative stress through P38/NF-kB pathway in ketamine-induced ulcerative cystitis. J. Cell. Mol. Med. 24 (13), 7609–7624. doi:10.1111/jcmm.15397
Xu, D. P., Zheng, J., Zhou, Y., Li, Y., Li, S., and Li, H. B. (2016). Extraction of natural antioxidants from the Thelephora ganbajun mushroom by an ultrasound-assisted extraction technique and evaluation of antiproliferative activity of the extract against human cancer cells. Int. J. Mol. Sci. 17 (10), E1664. doi:10.3390/ijms17101664
Yang, H. L., Liu, H. W., Shrestha, S., Thiyagarajan, V., Huang, H. C., and Hseu, Y. C. (2021). Antrodia salmonea induces apoptosis and enhances cytoprotective autophagy in colon cancer cells. Aging (Albany NY) 13 (12), 15964–15989. doi:10.18632/aging.203019
Yang, Q., Cai, X., Yan, A., Tian, Y., Du, M., and Wang, S. (2020). A specific antioxidant peptide: Its properties in controlling oxidation and possible action mechanism. Food Chem. 327, 126984. doi:10.1016/j.foodchem.2020.126984
Yang, S., Yan, J., Yang, L., Meng, Y., Wang, N., He, C., et al. (2019). Alkali-soluble polysaccharides from mushroom fruiting bodies improve insulin resistance. Int. J. Biol. Macromol. 126, 466–474. doi:10.1016/j.ijbiomac.2018.12.251
Yaribeygi, H., Sathyapalan, T., Atkin, S. L., and Sahebkar, A. (2020). Molecular mechanisms linking oxidative stress and diabetes mellitus. Oxid. Med. Cell. Longev. 2020, 8609213. doi:10.1155/2020/8609213
Yun, L., Wu, T., Mao, Z., Li, W., Zhang, M., and Sun, X. (2020). A novel wheat germ polysaccharide: Structural characterization, potential antioxidant activities and mechanism. Int. J. Biol. Macromol. 165, 1978–1987. doi:10.1016/j.ijbiomac.2020.10.112
Zhang, J., Zhang, J., Zhao, L., Shui, X., Wang, L. A., and Wu, Y. (2019). Antioxidant and anti-aging activities of ethyl acetate extract of the coral tooth mushroom, hericium coralloides (agaricomycetes). Int. J. Med. Mushrooms 21 (6), 561–570. doi:10.1615/IntJMedMushrooms.2019030840
Zhang, C., Song, X., Cui, W., and Yang, Q. (2021a). Antioxidant and anti-ageing effects of enzymatic polysaccharide from Pleurotus eryngii residue. Int. J. Biol. Macromol. 173, 341–350. doi:10.1016/j.ijbiomac.2021.01.030
Zhang, J. J., Chen, B. S., Dai, H. Q., Ren, J. W., Zhou, L. W., Wu, S. H., et al. (2021b). Sesquiterpenes and polyphenols with glucose-uptake stimulatory and antioxidant activities from the medicinal mushroom Sanghuangporus sanghuang. Chin. J. Nat. Med. 19 (9), 693–699. doi:10.1016/S1875-5364(21)60101-2
Zhang, P., Li, T., Wu, X., Nice, E. C., Huang, C., and Zhang, Y. (2020). Oxidative stress and diabetes: Antioxidative strategies. Front. Med. 14 (5), 583–600. doi:10.1007/s11684-019-0729-1
Zhang, Y., Liu, Z., Ng, T. B., Chen, Z., Qiao, W., and Liu, F. (2014). Purification and characterization of a novel antitumor protein with antioxidant and deoxyribonuclease activity from edible mushroom Pholiota nameko. Biochimie 99, 28–37. doi:10.1016/j.biochi.2013.10.016
Zhao, S., Cheng, C. K., Zhang, C. L., and Huang, Y. (2021). Interplay between oxidative stress, cyclooxygenases, and prostanoids in cardiovascular diseases. Antioxid. Redox Signal. 34 (10), 784–799. doi:10.1089/ars.2020.8105
Zheng, N., Ming, Y., Chu, J., Yang, S., Wu, G., Li, W., et al. (2021). Optimization of extraction process and the antioxidant activity of phenolics from sanghuangporus baumii. Molecules 26 (13), 3850. doi:10.3390/molecules26133850
Zhou, J., Chen, M., Wu, S., Liao, X., Wang, J., Wu, Q., et al. (2020). A review on mushroom-derived bioactive peptides: Preparation and biological activities. Food Res. Int. 134, 109230. doi:10.1016/j.foodres.2020.109230
Zucca, P., Rosa, A., Tuberoso, C. I., Piras, A., Rinaldi, A. C., Sanjust, E., et al. (2013). Evaluation of antioxidant potential of "Maltese mushroom" (Cynomorium coccineum) by means of multiple chemical and biological assays. Nutrients 5 (1), 149–161. doi:10.3390/nu5010149
Zuo, K., Tang, K., Liang, Y., Xu, Y., Sheng, K., Kong, X., et al. (2021). Purification and antioxidant and anti-Inflammatory activity of extracellular polysaccharopeptide from sanghuang mushroom, Sanghuangporus lonicericola. J. Sci. Food Agric. 101 (3), 1009–1020. doi:10.1002/jsfa.10709
Keywords: chronic diseases, natural compounds, edible fungi, antioxidants, molecular mechanisms
Citation: Xia Y, Wang D, Li J, Chen M, Wang D, Jiang Z and Liu B (2022) Compounds purified from edible fungi fight against chronic inflammation through oxidative stress regulation. Front. Pharmacol. 13:974794. doi: 10.3389/fphar.2022.974794
Received: 21 June 2022; Accepted: 17 August 2022;
Published: 09 September 2022.
Edited by:
Li Wu, Nanjing University of Chinese Medicine, ChinaCopyright © 2022 Xia, Wang, Li, Chen, Wang, Jiang and Liu. This is an open-access article distributed under the terms of the Creative Commons Attribution License (CC BY). The use, distribution or reproduction in other forums is permitted, provided the original author(s) and the copyright owner(s) are credited and that the original publication in this journal is cited, in accordance with accepted academic practice. No use, distribution or reproduction is permitted which does not comply with these terms.
*Correspondence: Ziping Jiang, d2F0ZXJqenBAamx1LmVkdS5jbg==; Bin Liu, bF9iaW5Aamx1LmVkdS5jbg==
†These authors have contributed equally to this work