- 1College of Pharmacy, Chengdu University of Traditional Chinese Medicine, Chengdu, China
- 2School of Basic Medical Sciences, Chengdu University of Traditional Chinese Medicine, Chengdu, China
- 3Pediatric Department, Ya’an City Hospital of Traditional Chinese Medicine, Ya’an, Sichuan, China
Bolbostemma paniculatum (Maxim.) Franquet is a unique species in China with a long history of medicinal use, which has the effects of detoxifying, dissolving lumps and dispersing swellings. And it is commonly used to treat many diseases, such as carbuncle and sore, acute mastitis, mammary cancer, scrofula and subcutaneous nodule traditionally. Modern clinical studies have found that B. paniculatum and its compounds can be used for the treatment of a variety of cancers, mastitis, hyperplasia of mammary glands, chronic lymphadenitis, cervical lymph tuberculosis and surgical wart skin diseases, and the curative effect is positive. At present, a variety of Chinese patent medicines containing B. paniculatum have been exploited and marketed in China for the treatment of cancers, breast diseases and flat warts. This review article comprehensively discussed the traditional application, botany, chemical components, pharmacological activities, and quality control of B. paniculatum, put forward some noteworthy issues and suggestions in current studies, and briefly discussed the possible development potential of this plant as well as future research perspectives. 96 compounds have been isolated from B. paniculatum, including triterpenoids, sterols, alkaloids and other components, of which triterpenoid saponins are the main bioactive components. The crude extracts and monomer compounds of B. paniculatum have a wide range of pharmacological activities, such as anti-tumor, antiviral, anti-inflammatory, immunoregulatory, and so on. Moreover, its anti-tumor mechanism involves many aspects, including inhibiting cell proliferation, promoting cell apoptosis, blocking the cell cycle, interfering with cell invasion and metastasis, suppressing angiogenesis, and regulating autophagy. While there is a lack of systematic and in-depth research on its anti-tumor active components and mechanism of action at the moment; and a tight connection between the chemical composition and pharmacological activity of B. paniculatum has also not been established. Besides, a systematic quality determination standard for B. paniculatum should also be built, in order to carry out further research.
1 Introduction
Bolbostemma paniculatum (Maxim.) Franquet is an endemic species in China (Editorial Committee of flora of China, 1986), which belongs to the Bolbostemma genus of Cucurbitaceae family; and its traditional medicinal part is dry tuber (Wang et al., 2015). B. Paniculatum has a long history of medication in China, a large number of traditional applications have shown that it is able to eliminate the lumps in many parts of human body and can be used to treat many diseases, such as pyogenic infection and ulceration of skin, acute mastitis, mammary cancer, scrofula and subcutaneous nodule. Modern clinical studies have found that B. paniculatum and its compounds can be used for the treatment of a variety of cancers (Ling, 2015), mastitis, hyperplasia of mammary glands (Wang, 1995), chronic lymphadenitis, cervical lymph tuberculosis and surgical wart skin diseases (Xu et al., 1980); and the definite curative effect has been achieved.
So far, 96 compounds have been isolated from B. paniculatum, including triterpenoids, sterols, alkaloids and other components, of which triterpenoid saponins are the main bioactive components. The compounds isolated from B. paniculatum exhibit various pharmacological activities, such as anti-tumor, antiviral, anti-inflammatory, immunoregulatory, and so on.
B. paniculatum has received increasing attention because of its good medicinal values. After collecting and sorting out the research information of B. paniculatum from scientific journals and reports through library and electronic database (including PubMed, Science Direct, CNKI, Web of Science and Baidu Scholar), we comprehensively reviewed traditional application, the botany, chemical components, pharmacological activities, and quality control of B. paniculatum, in order to provide reference for further study.
2 Traditional applications
B. paniculatum known as “Tu Beimu” is a unique species in China with a long history of medicinal use. However, there were many kinds of drugs used in the name of “Beimu” in ancient China; and according to researches, at least 4 families, 6 genera, and more than 10 species of plants (Shang and Liu, 1995). There is a certain disorder of the basic source, while the common species are mainly three types: Bolbostemma paniculatum (Maxim.) Franquet, Fritillaria cirrhosa D.Don and Fritillaria thunbergii Miq. At present, it is believed that B. paniculatum is the earliest “Beimu” used in China from the pre-Qin Dynasty to the Qin and Han Dynasties (770 BC to 221 BC) (Qiu, 2016). Later, Fritillaria thunbergii Miq. known as “Zhe Beimu” appeared in the Wei and Jin Dynasties; from the Tang Dynasty to the late Ming Dynasty, Fritillaria cirrhosa D.Don known as “Chuan Beimu” and other varieties appeared. Until the Qing Dynasty, the three “Beimu” varieties were officially distinguished and used independently. In order to avoid disputes over the historical efficacy and evolution of B. paniculatum, we did not adopt the ancient documents before the Qing Dynasty in the data collection of this article.
The Qing Dynasty’s “Ben Cao Cong Xin” (1757) (Wu et al., 2013) and “Ben Cao Fen Jing” (1840) (Yao, 2015) recorded that B. paniculatum can treat “phlegm” and “toxin.” Traditional Chinese medicine holds that “phlegm” and “toxin” often interplay and form abnormal masses in the body, which related to various tumors, lymphadenopathy and other diseases in modern medicine (Lou et al., 2020; Xu et al., 2021). There are descriptions of the treatment of “Swelling-abscess of breast” (referring to acute suppurative mastitis) and “Ru-yan” (referring to mammary cancer (Liu L. L. et al., 2013) by B. paniculatum in the medical classics such as “De Pei Ben Cao” (1761) (Yan et al., 1994), “Ben Cao Gang Mu Shi Yi” (1765) (Zhao, 2017), “Xu Ming Yi Lei’an” (1770) (Wei and Huang, 1997), “Cao Cang Zhou Yi’an” (1924) (Cao and Liu, 2005). In “Wu Ju Tong Yi’an” (1798) and “Gu Jin Yi Che” (1908) (Huai, 1958), it was used to treat “Luo-li” (referring to multiple nodules or masses in the neck). Although people knew little about the mechanisms of B. paniculatum at that time, it was fully recognized that it has the effects of detoxifying, dissolving lumps and dispersing swellings, which was an effective drug to eliminate lumps in various parts of human body via a large number of clinical practices, see Table 1. Till modern times, traditional Chinese medical science has adopted B. paniculatum to treat multiple tumors, mastitis, hyperplasia of mammary glands, lymph node tuberculosis, lymphadenitis, skin warts disease (Fu et al., 1984) and so on, all of which have achieved good efficacy clinically. Nowadays, nine Chinese patent medicines containing B. Paniculatum have been developed and marketed in China. Among them, Tianfoshen Koufuye is an anti-tumor agent, which can be used for many malignant tumors, such as lung, esophageal, gastric, liver and breast cancer (Wang et al., 2018). Six Chinese patent medicines such as Rupishu Jiaonang (Zhang, 2001), Xiaoru Sanjie Jiaonang, Rupiqing Jiaonang, Rupiqing Pian (Ji, 2020), Ruzengning Jiaonang (Chinese Pharmacopoeia Commission, 2020), Rupishu Pian (Liu Y. et al., 2019) have been used to treat hyperplasia of mammary glands, mastitis, etc. Besides, Pifubing Xuedu Wan are used for nettle rash, eczema, acne, acne rosacea, ulcerative naevus, scabies, etc. (Xin et al., 2004). Tubeimu Zaogan Zhusheye can be injected intramuscularly or applied externally to treat flat warts (Shi, 2004).
3 Botany
B. paniculatum is a perennial climbing herb belonging to the genus Bolbostemma of the Cucurbitaceae family. The tubers were hypertrophic, fleshy, and ivory; the stem is grassy, glabrous and climbing-like; its branch has prismatic grooves. The petiole is slender, about 1.5–3.5 cm long; the leaf blade is ovate-orbicular with 4–11 cm long and 3–10 cm wide, and is palmately 5-partite, there is 3–5 shallow lobes per lobe, the lateral lobes are ovate-oblong, acute, the central lobe is oblong-lanceolate, acuminate; there is a prominent gland at the tip of each basal lobe, and its leaves are glabrous or pilose only on veins. The cane vine is tendrils filiform, which grows momo or 2-fid. Flower are dioecious. The female and male inflorescences were evacuated panicles, with solitary flowers on it, and inflorescences are shaft filamentous, about 4–10 cm in length, the pedicel is slim, about 1.5–3.5 cm in length; the flowers are yellow-green, its calyx and corolla are similar, the segments are ovate-lanceolate and about 2.5 cm long, with filament tail at the top; there are five stamens which grow free; the filament apex is not enlarged with 0.3–0.5 mm long, the anthers is about 0.5 mm long, while the rostellum is on the back of anther and does not extend beyond the anthers. The ovary is ovoid with insignificant verrucous bulge, and it is 3-locular with two ovules in each locule, and there is three stylus which is 2-fid. The fruit is cylindric, about 1.5–3 cm in length and 1–1.2 cm in width, it cracks from top cap after ripening, the carpostegium is conic and has six seeds. Its seeds are ovate rhomboid and crineous, the surface has embossed bulge and irregular teeth on the edge, about 8–10 mm in length, 5 mm in width, 1.5 mm in depth, there is a long membranous wing at apex with 8–10 mm long. The flowering period is from June to August; the fruiting period is from August to September. The aboveground parts and tubers are shown in Figure 1. B. paniculatum is mainly produced in Hebei, Shandong, Henan, Shanxi, Shaanxi, Gansu, Eastern and southern Sichuan regions, and Northwest Hunan. It was born on the slope of the scrotum; and now has been widely cultivated (Editorial Committee of flora of China, 1986).
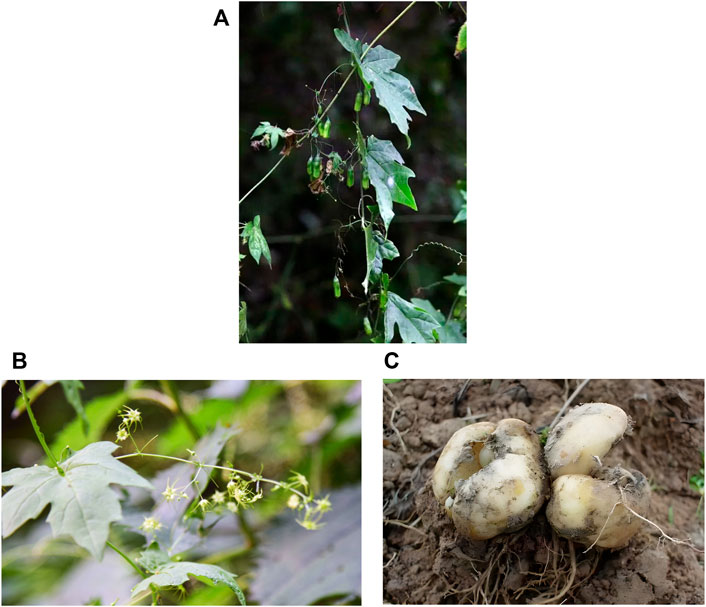
FIGURE 1. Bolbostemma paniculatum (Maxim.) Franquet: (A) Aboveground part, (B) flowers, and (C) stem tubers.
4 Chemical components
So far, 96 compounds have been isolated and identified from B. paniculatum, including triterpenoids (1–32), sterols (33–49), alkaloids (50–53) and other compounds (54–96). Among them, triterpenoids and their glycosides are the most important active components of B. paniculatum; the categories, names and corresponding structures of these compounds are summarized in Figures 2–8 and Supplementary Table S1.
4.1 Triterpenoids
Triterpenoids, which are a large group of constituents found in B. paniculatum, are one of the major active constituents. Tubeimoside I, which is the most studied compounds with high content in B. paniculatum, it has curative effects on glioblastoma, pancreatic cancer, cervical carcinoma and condyloma acuminatum, and is one of the anti-tumor and antiviral active ingredients (Li and Wang, 2006). Triterpenoid saponins from B. paniculatum include 13 pentacyclic triterpenoids (1–13), which are Tubeimoside I (1), Tubeimoside II (2), Tubeimoside V (3), 6′-O-palmitoyltubeimoside I (4), Dexylosyltubeimoside III (5), Lobatoside C (6), Tubeimoside III (7), Actinostemmoside E (8), Actinostemmoside F (9), Actinostemmoside H (10), Cucurbitacin B (11), Cucurbitacin E (12), Isocucurbitacin B (13); and 19 tetracyclic triterpenoids (14–32), which are Tubeimoside IV (14), 7β,18,20,26-tetrahydroxy-(20S)-dammar-24E-en-3-O-α-L-(3-acetyl)arabinopyranosyl-(1→2)-β-D-glucopyranoside (15), 7β,18,20,26-tetrahydroxy-(20S)-dammar-24E-en-3-O-α-L-(4-acetyl)arabinopyranosyl-(1→2)-β-D-glucopyranoside (16), 7β,18,20,26-tetrahydroxy-(20S)-dammar-24E-en-3-O-α-L-arabinopyranosyl-(1→2)-β-D-(6-acetyl)-glucopyranoside (17), 7β,20,26-trihydroxy-(20S)-dammar-24E-en-3-O-α-L-arabinopyranosyl-(1→2)-β-D-glucopyranoside (18), 7β,20,26-trihydroxy-(20S)-dammar-24E-en-3-O-α-L-(3-acetyl)arabinopyranosyl-(1→2)-β-D-glucopyranoside (19), 7β,20,26-trihydroxy-(20S)-dammar-24E-en-3-O-α-L-(4-acetyl)arabinopyranosyl-(1→2)-β-D-glucopyranoside (20), 7β,20,26-trihydroxy-8-formyl-(20S)-dammar-24E-en-3-O-α-L-(3-acetyl)arabinopyranosyl-(1→2)-β-D-glucopyranoside (21), 7β,20,26-trihydroxy-8-formyl-(20S)-dammar-24E-en-3-O-α-L-(4-acetyl)arabinopyranosyl-(1→2)-β-D-glucopyranosid (22), 23,24-dihydrocucurbitacin E (23), 23,24-dihydrocucurbitacin B (24), 23,24-dihydroisocucurbitacin B (25), Isocucurbitacin D 25-O-acetate (26), 3-O-α-L-arabinopyranosyl-(1→2)-β-D-glucopyranosyl-bayogenin-28-O-β-D-xylopyranosyl-(1→ 3)-α-L-rhamnopyranosyl-(1→2)-α-L-arabinopyranosyl ester glycosides (27), Lobatoside A (28), Lobatoside B (29), Lobatoside E (30), Lobatoside D (31), Lobatoside F (32). It was found that the cytotoxic activity of the bulbs of B. paniculatum mainly came from cucurbitacine triterpenoid sapogenins (12, 13, 23, 25) and the cyclic bisdesmosides (1, 3, 5, 6); structurally, the macrocyclic structure of cyclic bisdesmosides plays an important role in their potential cytotoxicity and has certain anticancer potential (Tang et al., 2015). These compounds and their corresponding structures are shown in Figures 2–4 and Supplementary Table S1.
4.2 Sterols
Sterols are also one of the major active constituents of B. paniculatum. Liu et al. (2004b) isolated four sterols from the chloroform extract, which are Stigmasta-7,22,25-triene-3-ol (33), Stigmasta-7,22,25-triene-3-O-nonadecanoic acid ester (34), Stigmasta-7,22,25-triene-3-O-β-D-(6′-palmitoyl)glucopyranosid e (35), Stigmasta-7,22,25-triene-3-O-β-D-glucopyranoside (36). Zheng et al. (2005a) used solvent method and various chromatographic methods (silica gel column chromatography, dextran gel column chromatography, HPLC, etc.) to get Stigmasterol (37), Daucosterol palmitate (38), Daucosterol (39), β-sitosterol (40), β-sitosterol palmitate (41); and other studies isolated Stigmasta-7,16,25-triene-3-ol (42), Stigmasta-7,16,25-triene-3-O-β-D-glucopyranoside (43), (3β,22E)-stigmasta-7,22,25-trien-3-yl-β-D-glucopyranoside (44), Uzarigenin-3-β-sophoroside (45), Sileneoside H (46), Integristerone A-25-acetate (47), 24(28)-dehydromakisterone A (48), 3-oxo-androsta-1,4-dien-17a′-spiro-2′-3′-oxo-oxetane (49). Liu Y. G. et al. (2013) isolated and extracted the fat-soluble components of fresh B. paniculatum, which mainly contained sterols, and found that these components have cytotoxic effects on some cancer cell lines and have certain anti-tumor activity. These compounds and their corresponding structures are shown in Figure 5 and Supplementary Table S1.
4.3 Alkaloids
Zeng Y. et al. (2018) used UPLC/LTQ-Orbitrap MSn analysis and multivariate statistical analysis to quickly identify the medicinal material of B. paniculatum, and isolated three alkaloids: 4-(2-formyl-5-methoxymethylpyrrol-1-yl)butyric acid methyl ester (50), (E)-N-hydroxy phenyl ethyl-3-(4-hy-droxy-3-methoxy phenyl) acrylamide (51); in addition, Liu et al. (2003) isolated and structurally identified two new pyrrole alkaloids, which are 2-(2-formyl-5-methoxymethylpyrrol-1-yl)-3-phenylpropionic acid methyl ester (52), α-methyl pyrrole ketone (53). These compounds and their corresponding structures are shown in Figure 6 and Supplementary Table S1.
4.4 Others
There are few sugars in B. paniculatum, mainly maltose and sucrose. The tubers of B. paniculatum contain maltose, after drying, it contain sucrose; the petiole mainly contains reducing sugar, and the leaves mainly contain sucrose (Zhao et al., 2010); including Glucose (54), D-fructose (55), Maltose (56), Sucrose (57), Stachyose (58). Other compounds with important biological activities have also been found in different parts of B. paniculatum, such as glycosides: Isomaltol mannoside (59), α-hydroxyacetone glucoside (60), β-D-glucose 2→1 β-D-glucoside (61), Methyl α-D-fructofuranoside (62), Methyl β-D-fructofuranoside (63), n-Butyl-β-D-fructopyranoside (64); anthraquinones: Emodin (65), Emodinmonomethylether (66); flavonols: Quercitrin (67), 3-O-[β-D-pyranrham-nose-(1-6)-β-D-galactopyranose]-5,7,4′-trihydroxyl flavone (68), 6-C-glucose-5,7,3′,4′-hydroxy flavone (69), Quercetin-3-O-α-L-arabinopyranoside (70); aromatic compounds: Maltol (71), 4-Hydroxybenzoic acid (72), Dibutyl phthalate (73); aldehydes: 5-hydroxymethylfurfural (74); fatty acids: Palmitic acid (75), Hexadecanoic acid (76), 9-octadecenamide (77), D-alanine (78); nucleosides: Uridine (79), Thymidine (80), Adenosine (81), Cytosine (82); alcohols: D-Sorbitol (83), D-Mannitol (84); phenols: Chlorogenic acid (85), Pyrogallol (86), Catechin (87), Epicatechin (88); phenylpropionic acid compounds: Scopoletin (89), 5-O-feruloylquinic acid (90); alkanes: n-Hentriacontane (91), Nonacosane (92), Triacontane (93); heterocyclic compounds: Allantoin (94); amides: (E)-N-(4-hydroxyphenethyl)-3-(4-hydroxy-3-methoxyphenyl)-acrylamide (95) and lignin compounds: (Z)-3-O-caffeoyl-4-O-methylquinic acid methyl ester (96). These compounds and their corresponding structures are shown in Figures 7, 8 and Supplementary Table S1.
5 Pharmacological activities
At present, modern pharmacological studies of B. paniculatum mainly focus on the anti-tumor activities of crude extracts and monomer compounds, and there are few relative studies on other pharmacological effects. In this part, we will comprehensively review the modern pharmacological effects of B. paniculatum, including anti-tumor, antiviral, anti-inflammatory, immuneoregulatory, etc.; detailed pharmacological activities and biological studies are shown in Figure 9 and Supplementary Table S2.
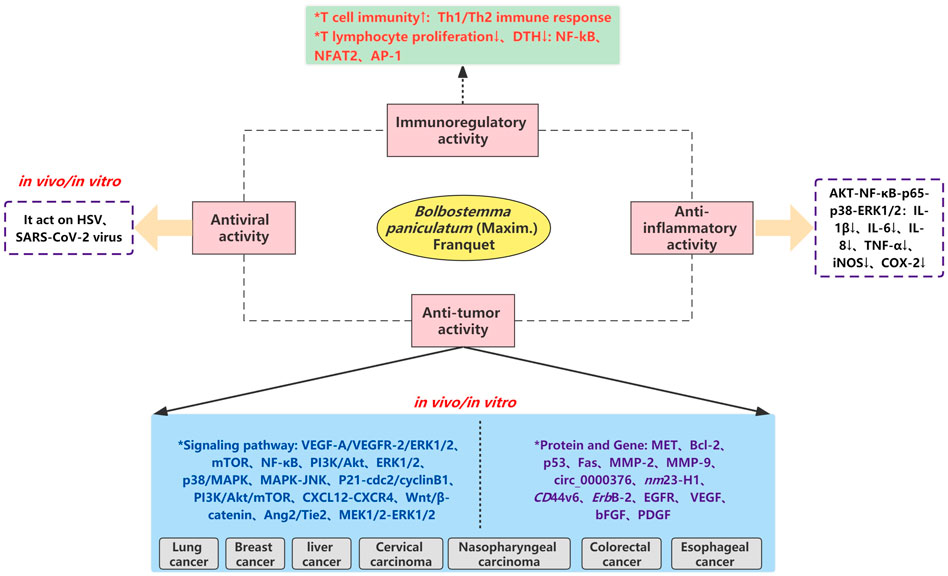
FIGURE 9. The molecular mechanism and pharmacological relationship network of Bolbostemma paniculatum (Maxim.) Franquet.
5.1 Anti-tumor activity
B. paniculatum can be used to treat various cancers in clinic. A large number of experimental studies have shown that the active compounds isolated from B. paniculatum, especially TBMS I, has anti-cancer effect on many kinds of cancer cell lines. TBMS I can exert its anti-tumor activity by inhibiting cell proliferation, directly exerting cytotoxic activity, inducing apoptosis, blocking the cell cycle, interfering with cell invasion and metastasis, repressing angiogenesis and regulating autophagy.
5.1.1 Effect on proliferation
A prominent feature of tumorigenesis is abnormal cell proliferation. Several scholars have found that B. paniculatum exerts a certain inhibitory effect on the proliferation of prostate cancer PC3 cells (Wang et al., 2016), human liver cancer HepG2 cells (Wang et al., 2011), human ovarian cancer A2780/DDP cells (Liu et al., 2011), lung cancer NCI-H1299 cells, and glioblastoma cells. TBMS I can inhibit the proliferation of lung cancer NCI-H1299 cells by depressing the activity of the VEGF-A/VEGFR-2/ERK1/2 signaling pathway (Shi H. B. et al., 2018). In addition, TBMS I also increases the ubiquitination level of protooncogene (MET) to decrease the protein level of MET, thereby repressing the abnormal activation and amplification of MET, and ultimately inhibiting the proliferative activity of glioblastoma (Cao et al., 2019).
5.1.2 Cytotoxic activity
A variety of chemical components of B. paniculatum, such as TBMS I, have direct cytotoxic activity, which can inhibit or kill cancer cells. Liu Y. G. et al. (2013) found that sterols were the main components of the fat-soluble extract of B. paniculatum; and in vitro experiment demonstrated that it have cytotoxic effects on four breast cancer cells (BT-549, MCF-7, MCF-7/ADR-RES, MDA-MB-231/ATCC). Tang et al. (2015) investigated the structure-activity relationship of triterpenoid saponins and their relationship with cytotoxicity in the bulbs of B. paniculatum, the results showed that TBMS I had moderate cytotoxicity against BCG-823, HeLa, HT-29, and MCF-7. Lin et al. (2016) found that the cytotoxic mechanism of TBMS I on NCI-H460 cells involved nucleolar stress-induced p53/murine double minute clone 2 (MDM2), mTOR, and NF-κB signaling pathways. Xu et al. (2009) discovered that the cytotoxic effect of TBMS I on HeLa cells was caused by mitochondrial dysfunction and the ER stress cell death pathway; through further study, they noticed that TBMS I-induced cytotoxicity involves multiple aspects, including molecular changes in ROS production, Ca2+ regulation, and G2/M cell cycle regulatory proteins (Xu et al., 2011).
5.1.3 Effects on apoptosis and autophagy
Apoptosis is a special type of cell death, and tumorigenesis is often associated with the inhibition of apoptosis. Guo et al. (2006) found that embolization of Tubeimosides microcapsules can cause ischemia and hypoxia in tumor tissue of liver cancer, and result in necrosis and apoptosis; medicated serum of B. paniculatum decoction can induce apoptosis of human lung cancer A549 and H1299 cells (Mi et al., 2021). Chao et al. (2019) discovered that peiminine isolated from B. paniculatum can induce apoptosis in human hepatocellular carcinoma HepG2 cells through both extrinsic and intrinsic apoptotic pathways. Modern pharmacological studies have demonstrated that TBMS I shown a powerful ability in inducing apoptosis; and it can induce apoptosis of A549, PC9, SKOV-3, CNE-2Z, BGC823, DU145, EC109, HL-60, SW480, U251, choriocarcinoma cells, etc. According to the studies of several scholars on the apoptotic mechanism of TBMS I, they found that TBMS I-induced apoptosis in tumor cells is a complex event which is polygenic and multichannel. TBMS I can regulate apoptosis of ovarian cancer SKOV-3 cells (Chen et al., 2012), squamous esophageal carcinoma EC109 cells (Xu et al., 2013), and human prostate cancer DU145 cells (Yang et al., 2016) via two important pathways: endoplasmic reticulum stress and mitochondrial dysfunction. One of the major events in apoptosis involving mitochondrial dysfunction is the alteration of apoptotic protein expression; TBMS I promotes apoptosis by regulating the expression of multiple apoptotic proteins, such as Bcl-2 family, p53 protein, Fas protein, etc. It has been found that the mechanism of TBMS I-induced apoptosis of various cells is mainly to downregulate the expression of Bcl-2 and upregulate the expression of Bax, including choriocarcinoma cells (Huang et al., 2011), ovarian cancer SKOV-3 cells (Chen et al., 2012), human nasopharyngeal carcinoma CNE-2Z cells (Weng et al., 2003), lung cancer A549 cells (Zhang X. H. et al., 2011; Hao et al., 2015), gastric cancer BGC823 cells (Zhang et al., 2013), human anterior adenocarcinoma DU145 cells (Yang et al., 2016), human glioma U251 cells (Jia et al., 2015; Cao et al., 2020), etc. The study of Yu et al. (2006) discovered that TBMS I could also downregulate the expression of p53 and upregulate the expression of Fas to induce apoptosis of human rectal cancer SW480 cells. Furthermore, scholars also found that apoptosis refers to a variety of signal pathways, including PI3K/Akt, ERK1/2, p38/MAPK, MAPK-JNK, P21-cdc2/cyclinB1 signal pathways, etc.
As the basic physiological process of cells, the functional status of autophagy directly affects tumorigenesis and its treatment. TBMS I can induce the autophagy of breast cancer MDA-MB-231 cells, and its effect is achieved by regulating the activity of PI3k-Akt-mTOR signaling pathway (Liu F. F. et al., 2019). Similar studies have shown that TBMS I can also activate AMPK signaling pathway to induce autophagy in human hepatoma HepG2 cells (Ruan et al., 2020). On the one hand, it can play its role via AMPK signal pathway; on the other hand, it can also restrain lysosomal cathepsin to block autophagy flux, and act on colorectal cancer CRC cells (Yan et al., 2019), cervical cancer cells (Feng et al., 2018), and lung cancer cells (Wang et al., 2020) according to these two ways. Besides, Du et al. (2020) found that TBMS I could trigger the interruption of partial autophagy flux and cytoprotective autophagy in melanoma A375 cells, which was realized by over-activating MEK1/2-ERK1/2 cascade.
5.1.4 Effect on cell cycle
Han et al. (2009) confirmed that the B. paniculatum preparation can block the transformation from G1 phase cells to S phase cells, which led to a relative increase of cells in G2/M phase; thus the growth of human tongue cancer Tca8113 cells was inhibited. Hu Z. et al. (2003) found that TBMS I blocks the cell cycle of human myeloid leukemia HL-60 cells by reducing the expression level of cyclin B1 related to the cell cycle. Besides, TBMS I can arrest cervical cancer HeLa cells in the G2/M phase, resulting in a significant inhibitory effect on its growth (Zhang et al., 2010). In the process of inhibiting the growth of human hepatoma HepG2 cells by TBMS II, the cells number in the G2/M phase increased; whereas the cells number in the G0/G1 phase decreased significantly (Chao et al., 2012).
5.1.5 Intervention in invasion and metastasis
Invasion and metastasis of tumor cells is one of the significant biological characteristics of malignant tumors, which is the main cause of death for clinical cancer patients. The study of Bao et al. (2017) discovered that the dichloromethane extract of fresh B. paniculatum can inhibit the tumor growth of human triple negative breast cancer MDA-MB-231-GFP nude mice and the occurrence of lung metastasis; and the corresponding in vitro test results also confirmed its anti-metastasis effect (An et al., 2013). Tubeimosides inhibit the activity of PI3K/Akt/mTOR signaling pathway, thus inhibiting the migration ability of breast cancer MDA-MB-231 cells (Dou et al., 2019). TBMS I can also inhibit the invasion and metastasis of breast cancer MDA-MB-231 cells by downregulating the expression of CXCR4 or interrupting CXCL12-CXCR4 cell signal pathway (Peng et al., 2016). Similar studies have mentioned that TBMS I can notably inhibit the metastasis of OSCC cells (Wu et al., 2018). Related pharmacological studies have shown that TBMS I inhibits the invasion and metastasis of tumor cells by different signal pathways. First of all, it can inhibit Wnt/β-catenin signaling pathway to prevent invasion of human colorectal cancer SW480 and HCT-8 cells (Bian et al., 2015); secondly, it can also suppress the migration and invasion of non-small cell lung cancer NCI-H1299 cells by inactivating VEGF-A/VEGFR-2/ERK1/2 signaling pathway (Shi H. et al., 2018). In addition, there is a research found that TBMS I can exert certain inhibitory effects on the adhesion, invasion and migration abilities of human highly metastatic giant cell lung carcinoma PGCL3 cells (Yu et al., 2008a) and human hepatoma HepG2 cells (Zhong et al., 2016) by inhibiting the secretion and activity of MMP-2 and MMP-9. TBMS I represses the migration and invasion of cutaneous squamous cell carcinoma CSCC cells, the mechanism may be that it can downgrades circ_0000376, thereby promoting the expression of miR-203 (Wang et al., 2021). Through further study, it was found that TBMS I significantly inhibited the metastasis of B16 melanoma and Lewis lung cancer in mice; this effect was related to upregulating the expression of metastasis suppressor genes (nm23-H1) and downregulating the expression of metastasis promoting genes (CD44v6, ErbB-2) (Wang et al., 2006). Moreover, TBMS II can reduce the redox related oxidative stress, inhibit the activation of epidermal growth factor receptor (EGFR) and inhibit TGF-β1-induced multiple transfer steps, thereby inhibiting the adhesion, migration of human retinoblastoma cells and invasion (Chen et al., 2021).
5.1.6 Antiangiogenesis
The growth, invasion and metastasis of solid tumors all depend on the formation of neovascularization; and TBMS I can curb tumor angiogenesis. Hu D. H. et al. (2003) preliminarily used angiogenesis model of chicken embryo chorioallantoic membrane to detect the effect of TBMS I on angiogenesis, and confirmed that it has a strong inhibitory effect on angiogenesis, and the dose-effect relationship is very obvious. The study of Yu et al. (2008b) shows that its antiangiogenesis mechanism is related to inducing apoptosis and inhibiting motility of vascular endothelial cells, and downregulating the expression of VEGF, bFGF, and PDGF. In vitro and vivo studies of Gu et al. (2016), TBMS I depresses angiogenesis based on its stimulating effect on the degradation of proteasomal VEGFR2 and Tie2, and downregulates the AKT/mTOR signaling pathway. Through further study, they found that the inhibitory mechanism of TBMS I against non-small cell lung cancer NCI-H4660 tumor vessel may be that it can significantly restrain the activation of VEGF/VEGFR2 and Ang2/Tie signal transduction pathways (Li T. Y. et al., 2016). Based on the inhibiting effect of TBMS I in HUVECs, Liu et al. (2020) speculated that the antiangiogenic effect may be related to the Piezo1 channel.
5.2 Antiviral activity
In vitro and in vivo tests showed that Tubeimosides had certain inhibitory effects on HSV1 virus and HBV virus (Zhou and Wu, 2005; Zhou et al., 2007; Zhang Y. et al., 2011). Wang et al. (2019) found that TBMS I can simultaneously inhibit the proliferation and genome replication of HSV1 and HSV2 viruses. In recent years, Ju et al. (2021) used high-throughput screening to identify that TBMS I showed obvious antiviral activity against SARS-CoV-2 infection in vitro.
5.3 Anti-inflammatory activity
In vivo, Yu et al. (2016) found that TBMS I can markedly inhibit PPDA-induced dermatitis in guinea pigs; and the study of Ma et al. (1991) shows that it could also significantly restrain TPA and AA-induced ear swelling in mice. In addition, TBMS I has a strikingly anti-inflammatory effect on rheumatoid arthritis (RA); Bao et al. (2018) and Liu et al. (2018) discovered it represses the production of pro-inflammatory cytokines as well as downregulate the expression of MMP-9. Zhang et al. (2018)’s research shows that TBMS I ameliorates PM2.5-induced lung injury in mice; and the mechanism may be that it reduce the level of inflammatory cytokines, such as IL-1β, IL-6, IL-8, TNF-α, so as to improve PM2.5-induced lung injury by inhibiting inflammation. Wu et al. (2013) utilize LPS-induced mice model of lung injury and LPS-stimulated RAW264.7 cells, and they found it remarkably inhibit the generation of pro-inflammatory cytokine (TNF-α, IL-6 and IL-1β) both in vivo and vitro. To some extent, TBMS I also has inhibitory effect on BV-2-mediated neuroinflammation; in tests on LPS-induced PD rat model LPS-exposed BV-2 cells, researchers confirmed that it could inhibit the activation of BV-2 and the reduction of dopaminergic neurons (He et al., 2018) in vivo; moreover, it probably works via inhibiting phosphorylation of AKT, NF-κB p65, p38 and ERK1/2, and then reduces the expression of pro-inflammatory mediators (iNOS, COX-2, TNF-α, IL-1β and IL-6).
Sepsis is a systemic inflammatory response syndrome caused by the invasion of pathogenic microorganisms such as bacteria into the body. Luo et al. (2020) found that the protective effect of TBMS I on sepsis mice was achieved by repressing the TLR4-MyD88-NF-κB-iNOS pathway; while similar pharmacological studies have shown that it may alleviate the pathological factors of sepsis-induced cardiac dysfunction and endothelial dysfunction (such as inflammation, oxidative stress, and apoptosis, etc.), which was raised by SIRT3 (Cheng et al., 2021; Yang et al., 2021).
5.4 Immunoregulatory activity
B. paniculatum is a promising immunoregulator. TBMS I can notably enhance antigen-specific humoral and cellular immunity responses, and lead Th1/Th2 immune responses in mice (Han, 2019). Liu et al. (2021)’s experiment demonstrated that TBMS I can also realize its anti-tumor effect by improving anti-tumor T cell immunity. B. paniculatum has inhibiting effect both on humoral and cellular immunity (Huang et al., 1992); the further test indicated that it markedly inhibit T lymphocyte proliferation in vitro and T cell-mediated delayed type hypersensitivity (DTH) in vivo, and the mechanism may be concerned in depressing the activation of NF-kB, NFAT2 and AP-1 signal transduction pathways (Huang et al., 1992).
5.5 Others
TBMS I has potential development value in the treatment of osteoporosis; it can inhibit NF-κB signaling pathway to reduce the production of osteoclasts, so as to protect against bone damaging diseases such as osteoporosis caused by type 2 diabetes (Yang et al., 2019; Yang et al., 2020). In addition, the extract of B. paniculatum also has certain antibacterial effect, researches confirmed that the 75% ethanol extract of B. paniculatum has obvious inhibitory action on Salmonellae, S. agalactiae and Staphylococcus aureus; while this action becomes inapparent on Bacillus coli (Chen et al., 2009). It has been reported that B. paniculatum possesses definite spermicidal efficacy, which affects the biomembrane system of sperm and damages the plasma membrane, acrosome and mitochondria of sperm; furthermore, its spermicidal active constituent are saponins. Lv et al. (2021) found that TBMS I has certain antioxidant activity and protects myocardial ischemia-reperfusion injury via SIRT3-dependent oxidative stress and apoptosis regulation.
6 Quality control
The current edition of Pharmacopoeia of the People’s Republic of China (Chinese Pharmacopoeia Commission, 2020) stipulates that the index component of B. paniculatum is TBMS I (C63H98O29); its content is determined by high-performance liquid chromatography (General rule 0512), and the content shall not be less than 1.0%; this product is calculated as dry product. Moreover, the identification, inspection and determination of B. paniculatum are also documented; it is required to use ethanol as the solvent and hot-dipped coating which belongs to determination of alcohol soluble extract method (General rule 2201), and shall not be less than 17.0%.
Traditional Chinese medicine (TCM) has the characteristics of multi-component and multi-target, so it is difficult to comprehensively judge the quality of B. paniculatum with only one component as the standard. With the continuous improvement of modern isolation and identification methods, several investigators have used a variety of methods to evaluate the quality of B. paniculatum; among them, HPLC is one of the most commonly used analytical methods, and it is also the main method for the analysis of compounds in B. paniculatum. In Wang et al. (2015)’s research, he used TBMS I and Tubeimosides as detection indicators, and adopt orthogonal test to evaluate and prioritize extracting craft. Wei et al. (2000) measured the content of TBMS I by HPLC, extracted it with water, and determined the content of B. paniculatum from different sources. Except the method for merely determining the content of TBMS I, there is another way to simultaneously measure the content of TBMS I, TBMS II, TBMS III for the first time (Huang et al., 2016). However, in order to describe and evaluate the quality of B. paniculatum as a whole, it is necessary to establish its fingerprints; Li et al. (2007) established its fingerprint via HPLC method, and they found that there are 18 common fingerprint peaks from 15 places of origin. Through further study, investigators built the characteristic maps of saponins in B. paniculatum from different habitats, and they discovered that the main components affecting the quality of the medicinal materials were TBMS I, TBMS II, TBMS III; at the same time, similarity evaluation, principal component analysis and cluster analysis are also applied, which can be used for the quality evaluation of B. paniculatum more comprehensively, accurately and effectively (Zeng Y. L. et al., 2018). Huang et al. (2020) utilize another method to determine its content, QAMS, and established the UPLC fingerprint of B. paniculatum; the results showed that there were four common peaks in the fingerprint of 19 batches of medicinal materials. In addition, Cui et al. (2008) set up a method to measure TBMS I in rat serum; because the amount of TBMS I in rat serum is very low, so the experiment took a solid-phase extraction method to process serum samples, which can rapidly, easily, and accurately determine the content of its preparation and serum. Other researchers used thin-layer chromatography (Wang et al., 1993; Ren and Zhao, 2005) and ultraviolet spectrophotometry (Ma and Yang, 2002) to evaluate the quality of B. paniculatum, but the accuracy was not as good as the above methods.
To sum up, the quality control of B. paniculatum has not been comprehensively and systematically studied. Therefore, it is necessary to further explore and establish a more objective and comprehensive quality standard, so as to achieve reliable, reasonable and systematic quality evaluation and quality control for B. paniculatum.
7 Discussion and prospect
B. paniculatum is a unique species in China with a long history of medicinal use; traditional Chinese medicine science believes that it has the effects of detoxifying, dissolving lumps and dispersing swellings, and can eliminate the lumps in many parts of human body. At present, doctors of TCM use it to treat many diseases such as various cancers, mastitis, breast hyperplasia, chronic lymphadenitis, cervical lymph node tuberculosis and surgical warts skin diseases, and achieve certain curative effects. Over the past 20 years, scholars have done a lot of researches on the chemical components and pharmacological effects of B. paniculatum, and have also obtained some research results; while there is still a long way to reveal its clinical efficacy.
After teasing out the traditional applications, botany, chemical composition, pharmacological activities, quality control, we propose the following considerations and suggestions:
Firstly, it is known that B. paniculatum contains many components such as saponins, sterols, alkaloids, etc.; but the researches on its active components mostly concentrated in the saponins (especially TBMS I), and the activity research of other components have not been involved. Meanwhile, its pharmacological studies are mostly limited to the study of anti-tumor activity; and its anti-tumor effects are mostly conducted in vitro model, but few in vivo experiments. Studies on its material basis of anti-tumor effects are not enough, especially the adequate correspondence between components and anti-tumor effect has not been established.
Secondly, some traditional applications of B. paniculatum, such as treating various wart diseases, have not been thoroughly studied in modern times. And its antiviral activity also has great research value, such as the inhibition of SARS-CoV-2 virus.
Thirdly, TBMS I can play an immunosuppressive role at a small dose, which is also an aspect worthy of attention.
Fourthly, the acute toxicity and long-term toxicity of B. paniculatum and its active components are rarely reported, so it is necessary to promote research in this area to establish its safety.
Fifthly, the 2020 edition of Chinese Pharmacopoeia only selects TBMS I as the quality control index, which is difficult to comprehensively evaluate its quality; hence, its systematic quality control methods need to be further explored.
In conclusion, B. paniculatum has a long history of application, which has exact efficacy and promising development prospect, so it does deserve in-depth studies from modern investigators. We hope that this paper can provide a valuable reference for those who are interested in the study of B. paniculatum.
Author contributions
YZ, XQ, and SJ conceived and designed the review; JZ, YX, PG, YHX, and LH contributed to the collection of literature and relevant information. YZ wrote this paper with help from JL and WL. All authors have read and agreed to the published version of the manuscript.
Funding
This work is supported by the studio of ZuLun Li (003112011013), a national famous veteran expert in traditional Chinese medicine, and the project of scientific research promotion plan for “Xinglin Scholar” (JSZX2019004).
Acknowledgments
At the end of this article, YZ would like to express her sincere thanks to all those who have helped this article. Each of the authors mentioned in the article has contributed a lot. YZ would like to express her special gratitude to her mentor, XQ, who has provided a lot of writing advice and theoretical guidance.
Conflict of interest
The authors declare that the research was conducted in the absence of any commercial or financial relationships that could be construed as a potential conflict of interest.
Publisher’s note
All claims expressed in this article are solely those of the authors and do not necessarily represent those of their affiliated organizations, or those of the publisher, the editors and the reviewers. Any product that may be evaluated in this article, or claim that may be made by its manufacturer, is not guaranteed or endorsed by the publisher.
Supplementary material
The Supplementary Material for this article can be found online at: https://www.frontiersin.org/articles/10.3389/fphar.2022.974054/full#supplementary-material
References
An, C., Yang, M., Hu, M. X., Fu, Y. L., and Li, Q. W. (2013). Study on anticancer effects of extract of Tubeimu on mouse model of human breast cancer MDA-MB-231-GFP. China J. Tradit. Chin. Med. Pharm. 28, 390–393. doi: CNKI:SUN:BXYY.0.2013-02-029.
Bao, W. Q., Wang, J. Y., An, C., Hu, K. W., Yang, M., and Hoffman, M. R. (2017). Inhibitory effect of fresh tubeimu (rhizoma bolbostemmae) dichloromethane extract on tumor growth and lung metastasis. China J. Tradit. Chin. Med. Pharm. 23, 18–21. doi:10.13862/j.cnki.cn43-1446/r.2017.23.006
Bao, Y. G., Li, H., Li, Q. Y., Li, Y., Li, F., Zhang, C. F., et al. (2018). Therapeutic effects of Smilax glabra and Bolbostemma paniculatum on rheumatoid arthritis using a rat paw edema model. Biomed. Pharmacother. 108, 309–315. doi:10.1016/j.biopha.2018.09.004
Bian, Q., Liu, P., Gu, J., and Song, B. (2015). Tubeimoside-1 inhibits the growth and invasion of colorectal cancer cells through the Wnt/β-catenin signaling pathway. Int. J. Clin. Exp. Pathol. 8, 12517–12524. https://www.ncbi.nlm.nih.gov/pubmed/26722439.
Cao, C. Z., and Liu, X. H. (2005). Cao Cang Zhou yi'an. Shanghai: Shanghai Science and Technology Press, 399–405.
Cao, J. J., Zhao, E. H., Zhu, Q. Z., Ji, J. L., Wei, Z. K., Xu, B., et al. (2019). Tubeimoside-1 inhibits glioblastoma growth, migration, and invasion via inducing ubiquitylation of MET. Cells 8, 774. Cells. doi:10.3390/cells8080774
Cao, L. J., Xie, H. T., Chu, Z. X., Ma, Y., Wang, M. M., and Shi, Z. (2020). Tubeimoside‑1 induces apoptosis in human glioma U251 cells by suppressing PI3K/Akt‑mediated signaling pathways. Mol. Med. Rep. 22, 1527–1535. doi:10.3892/mmr.2020.11224
Chao, X., Wang, G., Tang, Y., Dong, C., Li, H., Wang, B., et al. (2019). The effects and mechanism of peiminine-induced apoptosis in human hepatocellular carcinoma HepG2 cells. PLoS One 14, e0201864. doi:10.1371/journal.pone.0201864
Chao, X., Zhao, Y. Y., Wei, M. H., and Tang, L. (2012). Effects of Tubeimoside Ⅱ on proliferation and cell cycle of human hepatocellular carcinoma cell line HepG2. Jiangsu Med. J. 38, 1732–1742. doi: CNKI:SUN:YIYA.0.2012-15-003.
Chen, W. J., Yu, C., Yang, Z., He, J. L., Yin, J., Liu, H. Z., et al. (2012). Tubeimoside I induces G2/M phase arrest and apoptosis in SKOV-3 cells through increase of intracellular Ca2+ and caspase-dependent signaling pathways. Int. J. Oncol. 40, 535–543. doi:10.3892/ijo.2011.1218
Chen, Y., Wang, T. G., Guo, K. K., and Zhang, W. M. (2009). In vitro bacteriostatic test of extracts of Bolbostemma paniculatum and Angelica dahurica. Heilongjiang J. Anim. Sci. Vet. Med. 2, 109–110. doi:10.3969/j.issn.1004-7034.2009.02.050
Chen, Y., Zhu, X., Wang, K., Zou, W., and Zhou, F. (2021). Tubeimoside II inhibits TGF-β1-induced metastatic progression of human retinoblastoma cells through suppressing redoxosome-dependent EGFR activation. Chem. Biol. Interact. 335, 109367. doi:10.1016/j.cbi.2021.109367
Cheng, G., Zhang, Y., Zhang, X., Tang, H. F., Cao, W. D., Gao, D. K., et al. (2006). Tubeimoside V (1), a new cyclic bisdesmoside from tubers of Bolbostemma paniculatum, functions by inducing apoptosis in human glioblastoma U87MG cells. Bioorg. Med. Chem. Lett. 16, 4575–4580. doi:10.1016/j.bmcl.2006.06.020
Cheng, Z., Lv, D. Y., Luo, M. H., Wang, R. Y., Guo, Y. Z., Yang, X. Y., et al. (2021). Tubeimoside I protects against sepsis-induced cardiac dysfunction via SIRT3. Eur. J. Pharmacol. 905, 174186. doi:10.1016/j.ejphar.2021.174186
Chinese Pharmacopoeia Commission (2020). Chinese pharmacopeia, Part I. Beijing: China Medical Science and Technology Press, 178–179.
Cui, H. M., Chen, H. P., Liu, X. M., and Zhu, X. Y. (2008). Determination of tubeimoside Ⅰ in jiazhongxiao preparation and serum of rat. Chin. J. Inf. Tradit. Chin. Med. 15, 37–38. doi:10.3969/j.issn.1005-5304.2008.11.018
Dou, J. W., Shang, R. G., Lei, X. Q., Li, K. L., Guo, Z. Z., Ye, K., et al. (2019). Total saponins of Bolbostemma paniculatum (maxim.) Franquet exert antitumor activity against MDA-MB-231 human breast cancer cells via inhibiting PI3K/Akt/mTOR pathway. BMC Complement. Altern. Med. 19, 304. doi:10.1186/s12906-019-2708-0
Du, J., Dong, Z., Tan, L., Tan, M., Zhang, F., Zhang, K., et al. (2020). Tubeimoside I inhibits cell proliferation and induces a partly disrupted and cytoprotective autophagy through rapidly hyperactivation of MEK1/2-ERK1/2 cascade via promoting PTP1B in Melanoma. Front. Cell. Dev. Biol. 8, 607757. doi:10.3389/fcell.2020.607757
Editorial Committee of flora of China, C. A. o. S (1986). Flora of China, 73. Beijing: Science Press, 93–94.1
Feng, X. P., Zhou, J., Li, J. Y., Hou, X. Y., Li, L. H., Chen, Y. M., et al. (2018). Tubeimoside I induces accumulation of impaired autophagolysosome against cervical cancer cells by both initiating autophagy and inhibiting lysosomal function. Cell. Death Dis. 9, 1117. doi:10.1038/s41419-018-1151-3
Fu, Z. C., Xu, H. Q., Feng, J., and Liu, R. (1984). Clinical observation on treatment of various warts with Xiaoyouling preparation. Chin. Tradit. Herb. Drugs. 15, 24–25. Available at: https://kns.cnki.net/kcms/detail/detail.aspx?FileName=ZCYO198403009&DbName=CJFQ1984.
Fu, Z. C., Zhou, L. Y., Kong, F. H., Zhu, D. Y., and Xu, R. S. (1987). Study on chemical constituents of Bolbostemma paniculatum (I). Chin. Tradit. Herb. Drugs. 18, 6. Available at: https://kns.cnki.net/kcms/detail/detail.aspx?FileName=ZCYO198704001&DbName=CJFQ1987.
Gu, Y., Korbel, C., Scheuer, C., Nenicu, A., Menger, M. D., and Laschke, M. W. (2016). Tubeimoside-1 suppresses tumor angiogenesis by stimulation of proteasomal VEGFR2 and Tie2 degradation in a non-small cell lung cancer xenograft model. Oncotarget 7, 5258–5272. doi:10.18632/oncotarget.6676
Guo, W. L., Liu, Z. T., Dai, S. J., Zhou, B., and Shi, S. L. (2006). Experimental study of Tubeimoside microcapsule embolization on the expression of VEGF in hepatic carcinoma. J. Interv. Radiol. 12, 738–741. doi:10.3969/j.issn.1008-794X.2006.12.011
Han, C. M., Li, Q. S., Sun, D., and Sun, P. D. (2009). Apoptosis of Tca8113 cells induced by Bolbostemma paniculatum preparation combined with hyperthermia and its effect on cell cycle. Chin. J. Gerontol. 29, 299–301. doi:10.3969/j.issn.1005-9202.2009.03.018
Han, Z. Y. (2019). Study on the adjuvant activity and mechanism of Tubeimoside. Chongqin: Southwest University, 8–17. [master's thesis]Available at: https://kns.cnki.net/kcms/detail/detail.aspx?FileName=1019913988.nh&DbName=CMFD2019.
Hao, W. L., Wang, S., and Zhou, Z. L. (2015). Tubeimoside-1 (TBMS1) inhibits lung cancer cell growth and induces cells apoptosis through activation of MAPK-JNK pathway. Int. J. Clin. Exp. Pathol. 8, 12075–12083.
He, D. W., Huang, B. X., Fu, S. P., Li, Y. H., Ran, X., Liu, Y. D., et al. (2018). Tubeimoside I protects dopaminergic neurons against inflammation-mediated damage in Lipopolysaccharide (LPS)-evoked model of Parkinson's disease in rats. Int. J. Mol. Sci. 19, 2242. doi:10.3390/ijms19082242
Hu, D. H., Ma, R. T., and Yu, L. J. (2003a). Inhibition of angiogenesis by Tubeimoside I isolated from Bolbostemma paniculatum. Chin. Pharmacol. Bull. 19, 715–716. doi:10.3321/j.issn:1001-1978.2003.06.033
Hu, Z., Ma, R. T., and Yu, L. J. (2003b). Effects of Tubeimoside I on cell cycle and apoptosis of human myeloblastic leukemia cells (HL-60). Chin. J. Clin. Oncol. 30, 11–14. doi:10.3969/j.issn.1000-8179.2003.03.004
Huang, C. Y., Tang, M., Huang, W. Y., and Li, P. (1992). Effects of Bolbostemma paniculatum and artemisiaannual on the immune function of mice. J. Shandong Colle. Tradit. Chin. Med. 16, 55–78. doi:10.16294/j.cnki.1007-659x.1992.02.023
Huang, J., Zhang, J., and Gu, Z. B. (2016). Determination of tubeimoside Ⅰ, tubeimoside Ⅱ and tubeimoside Ⅲ in Bolbostemma paniculatum by HPLC. China Pharm. 25, 58–60. CNKI:SUN:YYGZ.0.2016-01-019.
Huang, M. Y., Li, Z. Y., Tong, P. Z., Cao, S. Q., Wei, M., Sun, D. M., et al. (2020). [Quality evaluation of Bolbostemmatis Rhizoma by UPLC fingerprint combined with QAMS]. China J. Chin. Mat. Med. 45, 3459–3466. doi:10.19540/j.cnki.cjcmm.20200421.201
Huang, P., Yu, C., Liu, X. Q., Ding, Y. B., Wang, Y. X., and He, J. L. (2011). Cytotoxicity of Tubeimoside I in human choriocarcinoma JEG-3 cells by induction of cytochrome c release and apoptosis via the mitochondrial-related signaling pathway. Int. J. Mol. Med. 28, 579–587. doi:10.3892/ijmm.2011.727
Huang, Y., Ren, M., Xiong, Y., Wang, T., Chen, H., Deng, X., et al. (2015). Tubeimoside-1 inhibits the proliferation and activation of mouse T lymphocytes through signal transduction pathways. Immunopharmacol. Immunotoxicol. 37, 366–374. doi:10.3109/08923973.2015.1063644
Ji, Q. Q. (2020). 164 cases of hyperplasia of mammary gland treated by acupoint application combined with Rupiqing tablet. Chin. J. Tradit. Med. Sci. Technol. 27, 258–260. CNKI:SUN:TJYY.0.2020-02-095.
Jia, G., Wang, Q., Wang, R., Deng, D. N., Xue, L., Shao, N. Y., et al. (2015). Tubeimoside-1 induces glioma apoptosis through regulation of Bax/Bcl-2 and the ROS/Cytochrome C/Caspase-3 pathway. Onco. Targets. Ther. 8, 303–311. doi:10.2147/Ott.S76063
Ju, X. H., Zhu, Y. K., Wang, Y. Y., Li, J. R., Zhang, J. X., Gong, M. L., et al. (2021). A novel cell culture system modeling the SARS-CoV-2 life cycle. PLoS Pathog. 17, e1009439. doi:10.1371/journal.ppat.1009439
Kong, F. H., Zhu, D. Y., Xu, R. S., and Fu, Z. C. (1988a). Studies on the chemical constituents of Bolbostemma paniculatum (IV). Acta. Chim. Sin. 46, 409–411. doi:10.3321/j.issn:1001-5302.2004.10.008
Kong, F. H., Zhu, D. Y., Xu, R. S., Fu, Z. C., and Zhou, L. Y. (1988b). Study on chemical constituents of Bolbostemma paniculatum (Ⅲ). Acta. Chim. Sin. 46, 772–778. https://kns.cnki.net/kcms/detail/detail.aspx?FileName=HXXB198808008&DbName=CJFQ1988.
Li, C. Q., and Wang, J. Z. (2006). Tissue culture and plant regeneration of Bolbostemma paniculatum. J. Chin. Med. Mat. 3, 209–211. doi:10.3321/j.issn:1001-5302.2006.05.004
Li, J., He, F., Yi, X., Hong, Z. G., Liu, X. Q., and Xiang, M. X. (2016a). Study on chemical compound of n-butyl alcohol fraction from Bolbostemma paniculatum. J. Huazhong Norm. Univ. Nat. Sci. 50, 721–725. doi:10.3969/j.issn.1000-1190.2016.05.015
Li, T. Y., Li, H., Zhan, T., and Zhang, F. (2016b). A Study on Tubeimoside I in inhibition of tumor angiogenesis in non-small-cell lung cancer Xenograft R at models. Med. Pharm. J. Chin. People's Lib. Army 28, 56–60. doi:10.3969/j.issn.2095-140X.2016.11.015
Li, X. J., Jiang, L. L., Wu, Z. N., and Zhao, L. H. (2007). Fingerprints of Bolbostemma paniculatum by HPLC. Chin. Tradit. Herb. Drugs. 38, 926–929. doi:10.3321/j.issn:0253-2670.2007.06.051
Lin, Y. Y., Xie, G. B., Xia, J., Su, D., Liu, J., Jiang, F. Q., et al. (2016). TBMS1 exerts its cytotoxicity in NCI-H460 lung cancer cells through nucleolar stress-induced p53/MDM2-dependent mechanism, a quantitative proteomics study. Biochim. Biophys. Acta 1864, 204–210. doi:10.1016/j.bbapap.2015.11.001
Ling, W. J. (2015). Discussion on the clinical application and regularity of common anti-tumor Traditional Chinese medicine. Beijing: Beijing Univ. Chin, 11–18. [master's thesis]. Available at: https://kns.cnki.net/kcms/detail/detail.aspx?FileName=1015385789.nh&DbName=CMFD2015.
Liu, F. F., Zhang, Y. W., Sun, Y. Y., and Chen, J. J. (2019a). Effects of Tubeimoside I on proliferation, apoptosis, and autophagy of breast cancer MDA-MB-231 cells. Shandong Med. J. 59, 14–17. doi:10.3969/j.issn.1002-266X.2019.20.004
Liu, H. Z., Yu, C., Yang, Z., and Wang, Y. X. (2011). Tubeimoside I enhances chemosensitivity of drug-resistant ovarian cancer A2780/DDP cells to Cisplatin. J. Third Mil. Med. Univ. 33, 695–698. doi: CNKI:SUN:DSDX.0.2011-07-017.
Liu, J. Y., Ma, R. T., and Yu, L. J. (2007). Effects of Tubeimoside I on mitogen-activated protein kinase activity in human nasopharyngeal carcinoma epithelial cells. J. Beijing Univ. Tradit. Chin. Med. 26, 119–121. doi:10.3969/j.issn.1674-1307.2007.02.026
Liu, L. L., Xu, H. B., Hu, Z. J., He, J. L., Liu, S., Sun, C. P., et al. (2013a). Treatment of lung metastasis of breast cancer by the transformation of cancer toxin from viscera to sputum. Chin. J. Tradit. Chin. Med. 28, 2331–2334. CNKI:SUN:BXYY.0.2013-08-037.
Liu, S. L., Pan, X. M., Cheng, W. B., Deng, B., He, Y., Zhang, L., et al. (2020). Tubeimoside I antagonizes yoda1-evoked Piezo1 channel activation. Front. Pharmacol. 11, 768–714. doi:10.3389/fphar.2020.00768
Liu, W. Y., Chen, W. G., Zhang, W. D., Chen, H. S., Gu, Z. B., and Li, T. Z. (2004a). Studies on chemical constituents in bulbs of Bolbostemma paniculatum. China J. Chin. Mat. Med. 29, 28–31. doi:10.3321/j.issn:1001-5302.2004.10.008
Liu, W. Y., Zhang, W. D., Chen, H. S., Gu, Z. B., Li, T. Z., and Chen, W. S. (2004b). New triterpenoid saponins from bulbs of Bolbostemma paniculatum. Planta Med. 70, 458–464. doi:10.1055/s-2004-818976
Liu, W. Y., Zhang, W. D., Chen, H. S., Gu, Z. B., Li, T. Z., and Yun, Z. (2003). Pyrrole alkaloids from Bolbostemma paniculatum. J. Asian Nat. Prod. Res. 5, 159–163. doi:10.1080/1028602031000066861
Liu, X. J., Yin, M. X., Dong, J. W., Mao, G. X., Min, W. J., Kuang, Z. A., et al. (2021). Tubeimoside-1 induces TFEB-dependent lysosomal degradation of PD-L1 and promotes antitumor immunity by targeting mTOR. Acta Pharm. Sin. B 11, 3134–3149. doi:10.1016/j.apsb.2021.03.039
Liu, Y. G., Liu, Y., Hu, K. W., and Ni, S. L. (2013b). Study on in vitro anti-breast cancer cell activity of fat-soluble extract from Bolbostemma paniculatum. Liaoning J. Tradit. Chin. Med. 40, 768–769. doi:10.13192/j.ljtcm.2013.04.166.liuyg.059
Liu, Y., Gou, Y., and Peng, X. J. (2019b). Study on quality control of Chinese medicine compound preparation Rupishu Table. J. Chengdu Univ. Chin. Med. 42, 39–43. doi:10.13593/j.cnki.51-1501/r.2019.02.039
Liu, Z. Z., Zhou, L., Ma, X. M., Sun, S. N., Qiu, H. W., Li, H., et al. (2018). Inhibitory effects of Tubeimoside I on synoviocytes and collagen-induced arthritis in rats. J. Cell. Physiol. 233, 8740–8753. doi:10.1002/jcp.26754
Luo, M. H., Luo, S. X., Cheng, Z., Yang, X. Y., Lv, D. Y., Li, X. B., et al. (2020). Tubeimoside I improves survival of mice in sepsis by inhibiting inducible nitric oxide synthase expression. Biomed. Pharmacother. 126, 110083. doi:10.1016/j.biopha.2020.110083
Lv, D. Y., Luo, M. H., Cheng, Z., Wang, R. Y., Yang, X. Y., Guo, Y. Z., et al. (2021). Tubeimoside I ameliorates myocardial ischemia-reperfusion injury through SIRT3-dependent regulation of oxidative stress and apoptosis. Oxid. Med. Cell. Longev., 5577019. doi:10.1155/2021/5577019
Ma, F. C., and Yang, J. (2002). Determination of total saponins in wild and crude Bolbostemma paniculatum. Med. Clin. 17, 57. doi:10.3969/j.issn.1672-8157.2002.04.028
Ma, R. T., Yu, L. J., and Wang, Y. Q. (1991). Strong inhibitory effect of Tubeimoside I from Bolbostemma paniculatum on inflammatory edema of mouse ear and carcinogenic process of mouse skin. Chin. Sci. Bull. 1421-1424. Available at: https://kns.cnki.net/kcms/detail/detail.aspx?FileName=KXTB199118018&DbName=CJFQ1991.
Ma, T. J., Li, J., Tu, P. F., and Hu, X. S. (2006). Studies on chemical constituents in bulbs of Bolbostemma paniculatum (Ⅱ). Acta Bot. boreali-occident. Sin. 26, 1732–1734. CNKI:SUN:DNYX.0.2006-08-039.
Ma, T. J., Li, J., Tu, P. F., Lu, F. J., and Tai, J. Y. (2005). Studies on chemical constituents in bulbs of Bolbostemma paniculatum. Acta Bot. boreali-occident. Sin. 25, 1163–1165. Available at: https://kns.cnki.net/kcms/detail/detail.aspx?FileName=DNYX200506017&DbName=CJFQ2005.
Mi, W. G., Zhang, W., Liu, J. J., and Zhang, Z. P. (2021). Effects of Tubeimu medicated serum on proliferation and apoptosis of human lung cancer cells. Lab. Med. Clin. 18, 923–928. doi:10.3969/j.issn.1672-9455.2021.07.017
Niu, X. Q. (2012). New utility of pi fu bing xue Du WanCNKI:SUN:JTZY. Fam. Tradit. Chin. Med. 19 (1). 10.2012-01-038.
Peng, Y. J., Zhong, Y., and Li, G. (2016). Tubeimoside-1 suppresses breast cancer metastasis through downregulation of CXCR4 chemokine receptor expression. BMB Rep. 49, 502–507. doi:10.5483/BMBRep.2016.49.9.030
Qiu, L. (2016). [Differential textual research and analysis on the evolution of fritillaria species in successive dynasties]. Zhonghua Yi Shi Za Zhi 46 (06), 323–328. Available at: https://kns.cnki.net/kcms/detail/detail.aspx?FileName=ZHYS201606002&DbName=ZHYX2016.
Ren, Y. Q., Zhao, Y., and Peng, Q. (2005). TLC and UV identification of fresh tubers of Bolbostemma paniculatum and its confusers. Mod. Tradit. Chin. Med. 25, 65–66. doi:10.3969/j.issn.1672-0571.2005.04.047
Ruan, C. X., You, L. J., Qiu, Y. D., Cui, X., and Wu, D. F. (2020). Tubeimoside I induces autophagy in HepG2 cells by activating the AMP-activated protein kinase signaling pathway. Oncol. Lett. 20, 623–630. doi:10.3892/ol.2020.11604
Shang, Z. J., and Liu, X. L. (1995). Investigation on the various species of bulb of Fritillary and its application. Zhonghua Yi Shi Za Zhi (01), 38–42. Available at: https://kns.cnki.net/kcms/detail/detail.aspx?FileName=ZHYS501.009&DbName=CJFQ1995.
Shi, H. B., Bi, H. X., Sun, X. Y., Dong, H. Y., Jiang, Y. F., Mu, H. J., et al. (2018b). Tubeimoside-1 inhibits the proliferation and metastasis by promoting miR-126-5p expression in non-small cell lung cancer cells. Oncol. Lett. 16, 3126–3134. doi:10.3892/ol.2018.9051
Shi, H., Bi, H., Sun, X., Dong, H., Jiang, Y., Mu, H., et al. (2018a). Antitumor effects of Tubeimoside-1 in NCI-H1299 cells are mediated by microRNA-126-5p-induced inactivation of VEGF-A/VEGFR-2/ERK signaling pathway. Mol. Med. Rep. 17, 4327–4336. doi:10.3892/mmr.2018.8459
Shi, X. X. (2004). Treatment of verrucous diseases by Bolbostemma paniculatum and its preparations. Harbin Phar 24, 46.
Su, H., and Guo, R. Y. (1986). Experimental studies on Tubeimosides used as intravaginal spermatocidal. J. J. Xi'an Jiaot. Univ. 7, 225–325. Available at: https://kns.cnki.net/kcms/detail/detail.aspx?FileName=XAYX198603005&DbName=CJFQ1986.
Tang, H. F., Yi, Y. H., Li, L., Sun, P., Wang, Z. Z., and Zhao, Y. P. (2005). Isolation and structural elucidation of bioactive cyclic bisdesmosides from tubers of Bolbostemma paniculatum. Pharm. Care Res. 5, 216–223. doi:10.3969/j.issn.1671-2838.2005.03.003
Tang, H. F., Zhang, S. Y., Yi, Y. H., Weng, A. D., Zhao, Y. P., and Wang, Z. Z. (2006). Isolation and structural elucidation of a bioactive saponin from tubers of Bolbostemma paniculatum. China J. Chin. Mat. Med. 31, 213–217. doi:10.3321/j.issn:1001-5302.2006.03.011
Tang, Y., Cao, J.-Q., Li, W., and Zhao, Y.-Q. (2014). Three New triterpene saponins from Bolbostemma paniculatum. Helv. Chim. Acta 97, 268–277. doi:10.1002/hlca.201300008
Tang, Y., Li, W., Cao, J., Li, W., and Zhao, Y. (2015). Bioassay-guided isolation and identification of cytotoxic compounds from Bolbostemma paniculatum. J. Ethnopharmacol. 169, 18–23. doi:10.1016/j.jep.2015.04.003
Wang, C. X., Ma, R. T., and Yu, L. J. (2006). Inhibitory effects of Tubeimoside I, a cyclic bisdesmoside isolated from Bolbostemma paniculatum‚ on metastases of mouse B16 melanoma and lewis lung carcinoma. Chin. J. Clin. Pharmacol. Ther. 11, 764–770. doi:10.3969/j.issn.1009-2501.2006.07.011
Wang, G. Q. (2014). National compilation of Chinese herbal medicine. Beijing: People's Health Publishing House, 38–39.
Wang, J., Xiao, C. C., Qu, X. Y., and Zhang, C. Y. (2021). Tubeimoside I affects the phenotype of skin squamous cell carcinoma SCL-1 cells by regulating the circ-0000376/miR-203 axis. J. Diagn Ther. Dermato-Venereol. 28, 90–98. Available at: https://kns.cnki.net/kcms/detail/detail.aspx?FileName=LPFZ202102003&DbName=CJFQ2021.
Wang, J., Yang, X., Han, H., Wang, L., Bao, W., Wang, S., et al. (2018). Inhibition of growth and metastasis of triple-negative breast cancer targeted by Traditional Chinese Medicine Tubeimu in orthotopic mice models. Chin. J. Cancer. Res. 30, 112–121. doi:10.21147/j.issn.1000-9604.2018.01.12
Wang, K., Zhan, Y. J., Chen, B. N., Lu, Y. H., Yin, T., Zhou, S. K., et al. (2020). Tubeimoside I-induced lung cancer cell death and the underlying crosstalk between lysosomes and mitochondria. Cell. Death Dis. 11, 708–724. doi:10.1038/s41419-020-02915-x
Wang, Q. L., Yu, L. J., Wang, Y. F., and Ma, R. T. (2019). Inhibitory effects of tubeimoside I on the proliferation and genomic replication of herpes simplex virus type 1 and type 2. Chin. J. Virol. 35, 569–577. doi:10.13242/j.cnki.bingduxuebao.003572
Wang, R. Z. (1995). Treatment of 83 cases of mammary hyperplasia with Ruji Powder. Shanxi Tradit. Chin. Med. 16, 256. CNKI:SUN:SXZY.0.1995-06-015.
Wang, W. F., Li, F., Cui, X. M., Guo, W. B., and Li, F. (2015). Study on extraction technology of Tubeimoside I and total saponins from Bolbostemma panicultum. Shanxi tradit. Chin. Med. 36, 612–614. doi:10.3969/j.issn.1000-7369.2015.05.043
Wang, X. J., Li, C. J., Chui, Z. H., and Yang, S. Y. (2016). Effect of Tubeimoside I on proliferation and morphological of androgen-independent prostate cancer PC3 cells. J. Mode. Oncol. 24, 2509–2511. doi:10.3969/j.issn.1672-4992.2016.16.002
Wang, Y., Deng, L., Zhong, H., Wang, Y., Jiang, X., and Chen, J. (2011). Natural plant extract Tubeimoside I promotes apoptosis-mediated cell death in cultured human hepatoma (HepG2) cells. Biol. Pharm. Bull. 34, 831–838. doi:10.1248/bpb.34.831
Wang, Y. Q., Wang, S. C., Yu, L. J., Ma, R. T., and Guo, W. B. (1993). Method for quantitative analysis of Tubeimoside I. China. J. Chin. Mat. Med. 18, 358–360. https://kns.cnki.net/kcms/detail/detail.aspx?FileName=ZGZY199306021&DbName=CJFQ1993.
Wei, Y. L., Yang, X. M., Xu, C. G., Gao, H., and Sun, W. J. (2000). Determination of tubeimoside I in Bolbostemma paniculatum by HPLC. Northwest Pharm. J. 15, 107–108. doi:10.3969/j.issn.1004-2407.2000.03.006
Weng, X. Y., Ma, R. T., and Yu, L. J. (2003). Apoptosis of human nasopha ryngeal carcinoma CNE-2Z cells induced by tubeimoside I. Chin. J. Cancer. 22, 806–811. Available at: https://kns.cnki.net/kcms/detail/detail.aspx?FileName=AIZH200308004&DbName=CJFQ2003.
Wu, Q., Sun, G., Yuan, X., Soromou, L. W., Chen, N., Xiong, Y., et al. (2013). Tubeimoside-1 attenuates LPS-induced inflammation in RAW 264.7 macrophages and mouse models. Immunopharmacol. Immunotoxicol. 35, 514–523. doi:10.3109/08923973.2013.810643
Wu, Y. L. (2013). New compilation of materia medica. Beijing: China traditional Chinese Medicine Press, 17.
Xiang, M. X., Wu, L., Fan, Y., Yi, X., and Zhang, L. (2017). Studies on the chemical constituents of ethyl acetate extract of Bolbostemma paniculatum. J. South-Cent. Univ. Natl. Nat. Sci. Ed. 36, 56–59. doi:10.3969/j.issn.1672-4321.2017.02.014
Xiu, L. J., Liu, X., Qin, Z. F., and Sun, D. Z. (2012). Professor Wei Pinkang's academic thought of “eliminating phlegm and attacking evil” in treating gastric cancer. Chin. J. Integr. T Rad. West Med. Dig. 20, 264–265. doi:10.3969/j.issn.1671-038X.2012.06.007
Xu, C. Y., Huang, J. B., and Zhang, G. Q. (2021). Discussion on the regularity of tumor occurrence and its characteristics based on sputum toxin theory. China J. Tradit. Chin. Med. Pharm. 36, 5801–5803. Available at: https://kns.cnki.net/kcms/detail/detail.aspx? FileName=BXYY202110021&DbName=CJFQ2021.
Xu, H. Q., Fu, Z. C., Liu, R., Zhang, P. J., and Zou, Y. X. (1980). Clinical study on Bolbostemma panicultum and its preparation —A report of 256 cases of verrucous diseases. J. Tradit. Chin. Med. (02), 35–36.
Xu, Y., Ching, Y. P., Zhou, Y., Chiu, J. F., Chen, F., and He, Q. Y. (2011). Multiple pathways were involved in tubeimoside-1-induced cytotoxicity of HeLa cells. J. Proteomics 75, 491–501. doi:10.1016/j.jprot.2011.08.014
Xu, Y., Chiu, J. F., He, Q. Y., and Chen, F. (2009). Tubeimoside-1 exerts cytotoxicity in HeLa cells through mitochondrial dysfunction and endoplasmic reticulum stress pathways. J. Proteome Res. 8, 1585–1593. doi:10.1021/pr801001j
Xu, Y., Wang, G. H., Chen, Q. C., Lin, T., Zeng, Z. P., Luo, Q., et al. (2013). Intrinsic apoptotic pathway and G2/M cell cycle arrest involved in Tubeimoside I-induced EC109 cell death. Chin. J. Cancer Res. 25, 312–321. doi:10.3978/j.issn.1000-9604.2013.06.03
Yan, J. H., Dou, X. Y., Zhou, J., Xiong, Y. F., Mo, L., Li, L. H., et al. (2019). Tubeimoside I sensitizes colorectal cancer cells to chemotherapy by inducing ROS-mediated impaired autophagolysosomes accumulation. J. Exp. Clin. Cancer Res. 38, 353–366. doi:10.1186/s13046-019-1355-0
Yan, X. T., Shi, Z. Y., and Hong, J. A. (1994). De Pei ben Cao. Shanghai: Shanghai Science and Technology Press, 48.
Yang, J. B., Khan, M., He, Y. Y., Yao, M., Li, Y. M., Gao, H. W., et al. (2016). Tubeimoside-1 induces oxidative stress-mediated apoptosis and G0/G1 phase arrest in human prostate carcinoma cells in vitro. Acta Pharmacol. Sin. 37, 950–962. doi:10.1038/aps.2016.34
Yang, M. L., Xie, J., and Zhou, L. (2019). Effect of tubeimoside I on RANKL induced osteoclast differentiation and its mechanism. Pharmacol. Clin. Chin. Mat. Med. 35, 30–34. doi:10.13412/j.cnki.zyyl.2019.05.007
Yang, M., Xie, J., Lei, X., Song, Z., Gong, Y., Liu, H., et al. (2020). Tubeimoside I suppresses diabetes-induced bone loss in rats, osteoclast formation, and RANKL-induced nuclear factor-κB pathway. Int. Immunopharmacol. 80, 106202–202. doi:10.1016/j.intimp.2020.106202
Yang, X. Y., Li, X. B., Luo, M. H., Li, C., Huang, L. X., Li, X., et al. (2021). Tubeimoside I improves endothelial function in sepsis via activation of SIRT3. Lab. Investig. 101, 897–907. doi:10.1038/s41374-021-00580-y
Yu, J., Qiu, Z. Y., Tang, W. X., and Li, H. Z. (2006). Mechanisms of apoptosis induced by Tubeimosides in human rectal cancer cell line SW 480 in vitro. Chin. Pharmacol. Bull. 22, 880–884. doi:10.3321/j.issn:1001-1978.2006.07.028
Yu, L. J., Hu, D. H., Ma, R. F., and Su, W. M. (2008a). Effects of Tubeimoside I on apoptosis of human umbilical vein endothelial cells and inhibition of tumor-induced angiogenesis. J. Cell. Biol. 30, 747–754. doi:10.3969/j.issn.1674-7666.2008.06.014
Yu, L. J., Ma, R. F., Wang, C. X., Huang, L. Z., and Zhang, X. Y. (2008b). Effects of Tubeimoside I on the adhesion, invasion and migration of PGCL3 cells in human highly metastatic giant cell lung cancer. J. Logist. Univ. Pap. 25, 135–140. Available at: https://kns.cnki.net/kcms/detail/detail.aspx?FileName=ZGTR200802021&DbName=CJFQ2008.
Yu, L. J., Zhang, Y. P., Ma, R. T., and Yu, T. X. (2016). Studies on inhibitory effect of Tubeimosides on delayed contact hypersensitivity induced by p-phenylenediamine in Guinea pigs. Chin. J. Immunol. 32, 1626–1631. doi:10.1038/aps.2016.34
Yu, L., Ma, R., Wang, Y., and Nishino, H. (1994). Potent anti-tumor activity and low toxicity of tubeimoside 1 isolated from Bolbostemma paniculatum. Planta Med. 60, 204–208. doi:10.1055/s-2006-959459
Zeng, Y. L., Tan, L., Gao, Y., and Lu, Y. (2018b). HPLC characteristic spectrum analysis of Bolbostemma paniculatum from different areas and content determination of three saponins. Drug Eval. Res. 41, 1816–1822. doi:10.7501/j.issn.1674-6376.2018.10.012
Zeng, Y., Lu, Y., Chen, Z., Tan, J., Bai, J., Li, P., et al. (2018a). Rapid characterization of components in Bolbostemma paniculatum by UPLC/LTQ-Orbitrap MS(n) analysis and multivariate statistical analysis for herb discrimination. Molecules 23, 1155–1168. doi:10.3390/molecules23051155
Zhang, J. B., Zhang, L., Li, S. Q., Hou, A. H., Liu, W. C., and Dai, L. L. (2018). Tubeimoside I attenuates inflammation and oxidative damage in a mice model of PM2.5-induced pulmonary injury. Exp. Ther. Med. 15, 1602–1607. doi:10.3892/etm.2017.5597
Zhang, X. H., Wang, J. M., and Sun, N. X. (2011a). Experimental research on the efficacy of Tubeimosides on herpes simplex keratitis. Int. Eye Sci. 11, 22–24. doi:10.3969/j.issn.1672-5123.2011.01.008
Zhang, X. L., Qu, Q., Wang, G. X., and Yang, J. (2010). Experimental study of Cisplatin and Tubeimoside I on proliferation and inhibitory effects of human cervical cancer cells. Int. Eye Sci. 18, 1909–1912. doi:10.3969/j.issn.1672-4992.2010.10.09
Zhang, Y., Xu, X., and He, P. (2011b). Tubeimoside-1 inhibits proliferation and induces apoptosis by increasing the Bax to Bcl-2 ratio and decreasing COX-2 expression in lung cancer A549 cells. Mol. Med. Rep. 4, 25–29. doi:10.3892/mmr.2010.379
Zhang, Y., Xu, X. M., Zhang, M., Qu, D., Niu, H. Y., Bai, X., et al. (2013). Effects of tubeimoside-1 on the proliferation and apoptosis of BGC823 gastric cancer cells in vitro. Oncol. Lett. 5, 801–804. doi:10.3892/ol.2013.1117
Zhao, G. P. (2010). Dictionary of traditional Chinese medicine. Second Edition. Shanghai: Shanghai Science and Technology Press, 113–114.
Zhao, X. M. (2017). A supplement to compendium of materia medica. Beijing: Traditional Chinese Medicine Classics Press, 122–126.
Zheng, C. H., Fu, H. W., and Fei, Y. H. (2005b). Isolation and identification of the chemical constituents from Bolbostemma paniculatum. Chin. J. Med. Chem. 15, 291–293. doi:10.3969/j.issn.1005-0108.2005.05.010
Zheng, C. H., Fu, H. W., and Pei, Y. H. (2007). A new cucurbitacin from Bolbostemma paniculatum Franguent. J. Asian Nat. Prod. Res. 9, 187–190. doi:10.1080/10286020500246725
Zheng, C. H. (2005a). Study on the active constituents of Bolbostemma paniculatum. Shenyang: Shenyang pharmaceutical university, 14–39. [doctor's thesis]. Available at: https://d.wanfangdata.com.cn/thesis/ChJUaGVzaXNOZXdTMjAyMjAzMjMSCFkxMDEyMjYyGghsZ3h0eG1xbg%3D%3D.
Zhong, H. Z., Guo, H., Wang, Y. J., and Yang, Y. L. (2016). Inhibitory effects of Tubeimoside I on the migration and invasion of human hepatoma (HepG2) cells. J. Logist. Univ. Pap. 25, 705–708.
Zhou, Y. M., and Wu, Z. M. (2005). The suppressor effect of Tubeimosides on the 2.2.15 cell secreting HBsAg and HBeAg. J. Zunyi Med. Univ. 28, 112–114. doi:10.3969/j.issn.1000-2715.2005.02.003
Keywords: Bolbostemma paniculatum (Maxim.) Franquet, traditional application, botany, chemical components, pharmacological activities, quality control
Citation: Zhou Y, Liu J, Zhang J, Xu Y, Li W, Gao P, Xing Y, Huang L, Qin X and Jin S (2022) Chinese endemic medicinal plant Bolbostemma paniculatum (Maxim.) Franquet: A comprehensive review. Front. Pharmacol. 13:974054. doi: 10.3389/fphar.2022.974054
Received: 20 June 2022; Accepted: 08 August 2022;
Published: 07 September 2022.
Edited by:
Xuelin Zhou, Capital Medical University, ChinaReviewed by:
Zhou Xunian, MD Anderson Cancer Center, United StatesSi-Yuan Song, Department of Neurology, Baylor College of Medicine, United States
Bin Yan, Shandong University of Traditional Chinese Medicine, China
Copyright © 2022 Zhou, Liu, Zhang, Xu, Li, Gao, Xing, Huang, Qin and Jin. This is an open-access article distributed under the terms of the Creative Commons Attribution License (CC BY). The use, distribution or reproduction in other forums is permitted, provided the original author(s) and the copyright owner(s) are credited and that the original publication in this journal is cited, in accordance with accepted academic practice. No use, distribution or reproduction is permitted which does not comply with these terms.
*Correspondence: Xuhua Qin, WHVodWFxaW4wMDI4QDEyNi5jb20=; Shenrui Jin, SjE1MTg0NDM2NTM5QDE2My5jb20=
†These authors have contributed equally to this work and share first authorship