- 1Department of Pharmacology, Faculty of Medicine, UKM Medical Centre, Universiti Kebangsaan Malaysia, Kuala Lumpur, Malaysia
- 2Department of Pharmacology and Toxicology, Faculty of Medicine, Umm Al-Qura University, Makkah, Saudi Arabia
This study investigated the gastroprotective effect of Piper sarmentosum (PS) on stress-induced gastric ulcers in rats by measuring its effect on oxidative stress, gastric mucosal nitric oxide (NO), and inflammatory biomarkers. Twenty-eight male Wistar rats were randomly divided into four groups; two control groups (non-stress and stress) and two treated groups supplemented with either methanolic PS extract (500 mg/kg body weight) or omeprazole (OMZ; 20 mg/kg) orally. After 28 days of treatment, the stress control, PS, and OMZ groups were subjected to water-immersion restrain stress (WIRS) for 3.5 h. Gastric tissue malondialdehyde (MDA), NO, superoxide dismutase (SOD), inducible NO synthase (iNOS), SOD mRNA, tumor necrosis factor (TNF)-α, interleukin (IL)-1β, and IL-6 levels were measured. WIRS significantly increased gastric MDA, NO, and pro-inflammatory cytokine levels compared to the non-stressed control group. PS and omeprazole supplementation significantly reduced WIRS-exposure-induced gastric ulcers and MDA, iNOS, and IL-1β levels. However, only PS reduced NO, TNF-α, and IL-6 levels, which were upregulated in this ulcer model. In conclusion, the gastroprotection afforded by PS is possibly mediated by gastric mucosal NO normalization through reduced iNOS expression and attenuation of inflammatory cytokines. PS showed a greater protective effect than omeprazole in reducing gastric lesions and NO, TNF-α, and IL-6 levels, and iNOS expression.
1 Introduction
Upper gastrointestinal bleeding is associated with significant morbidity and mortality. Bleeding peptic ulcers remain the most common cause of acute non-variceal upper gastrointestinal bleeding. This condition is often associated with non-steroidal anti-inflammatory drug use (Atchison et al., 2013), Helicobacter pylori infection (Wang et al., 2014), and stress (Nur Azlina et al., 2013; Kudryavtsev et al., 2014). Stress ulcer syndrome can cause mucosal erosions and superficial hemorrhages in critically ill patients or individuals under extreme physiologic stress. The ulcers frequently emerge due to major stressful events, including surgery, trauma, shock, sepsis, and burns. This study used water-immersion restraint stress (WIRS), an established method to induce gastric lesions in rats (Konturek et al., 2008; Kwiecien et al., 2012; Raja Kumar et al., 2019), to create a stress model that mimics stress ulcers in human patients.
Stress is associated with increased oxidative stress due to increased free radical formation. Kwiecien et al. (2012) found that WIRS causes acute inflammatory responses, with interleukin (IL)-1 and tumor necrosis factor-alpha (TNF-α) acting as the primary pro-inflammatory cytokines induced by neutrophil infiltration into the gastric mucosa. Neutrophils produce superoxide anions (O2•-) that react with cellular membrane lipids, resulting in lipid peroxidation. Malondialdehyde (MDA) and 4-hydroxynonenal are examples of lipid peroxidation end-products (Khoubnasabjafari et al., 2015). Stress will also produce high nitric oxide (NO) levels. NO is catalyzed by inducible NO synthase (iNOS) and acts as a potent cytotoxic oxidant (Lanas, 2008; Nimse and Pal, 2015). Stress also decreases superoxide dismutase (SOD) activity in gastric mucosa, impairing its antioxidative defense mechanism (Kwiecień et al., 2002; Nur Azlina et al., 2013; Patlevič et al., 2016).
Piper (P.) sarmentosum Roxb. belongs to the Piperaceae family. It is an about 20 cm tall herb that grows wild in the forest and is commonly found in Southeast Asia (e.g., Malaysia, Cambodia, Philippines, Thailand, and Myanmar). P. sarmentosum is a traditional medicinal plant whose leaves are usually eaten raw as ulam. For centuries, it has been used to treat wind-cold cough, fever, postpartum foot edema, stomachache, toothache, diabetes, and traumatic injury (Sun et al., 2020). P. sarmentosum has anti-inflammatory (Zakaria et al., 2010; Azlina et al., 2019; Salehi et al., 2019) and anti-atherosclerotic (Amran et al., 2010) properties. Methanolic P. sarmentosum extract has been shown to have antioxidative activity due to the natural antioxidant superoxide scavenger, naringenin (Subramaniam et al., 2003; Chanwitheesuk et al., 2005). Therefore, this study investigated the protective effects of methanolic P. sarmentosum extract against gastric mucosa injury as an alternative to other antioxidants. We used omeprazole as a positive control since it is one of the most widely prescribed drugs to treat gastric ulcers (Hajrezaie et al., 2012; Ketuly et al., 2013).
2 Materials and methods
2.1 Plant materials
Fresh P. sarmentosum leaves were collected from the Forest Research Institute Malaysia (FRIM) reserve forest at Kepong, Selangor, Malaysia, and identified by FRIM’s Medicinal Plant Division. A voucher specimen (FRI 45870) was deposited at FRIM’s Medicinal Plant Division.
2.2 Preparation of methanolic P. sarmentosum extract
The methanolic extraction procedure was performed at the FRIM laboratory. The leaves were cleaned with tap water and dried at room temperature before being finely chopped. Next, 250 g of leaves were extracted in 2.5 L of methanol. This mixture was heated to 40°C–60°C using a Soxhlet to evapourate the methanol (Sawangjaroen et al., 2004). The paste material produced was kept at 4°C until required. The percentage yield from the crude dried extract was around 10%. The plant extract was analyzed using liquid chromatography-mass spectrometry at the Universiti Kebangsaan Malaysia’s (UKM) Research and Instrumentation Management Center. The active chemical and purity results have been previously reported (Bactiar and Fahami, 2019). Methanol P. sarmentosum leaf extracts yielded fifteen compounds extracted and characterized by spectroscopic methods, including didymin, naringenin, methyl piperate, quercetin, beta asarone, brachyamide, amurensin, piperitol, guineensine, hesperidin, rutin, malvidin, and difucol (Table 1). The term P. sarmentosum in this study refers to P. sarmentosum methanolic extract.
2.3 Experimental design
Twenty-eight male Sprague Dawley rats (obtained from the Animal Unit, Faculty of Medicine, UKM) were divided into four groups: non-stress control, stress control, P. sarmentosum-treated, and omeprazole-treated. The non-stress and stress control groups were administered vitamin E-free palm oil via oral gavage. Omeprazole and P. sarmentosum were diluted in vitamin E-free palm oil as a vehicle. Omeprazole (20 mg/kg body weight) and P. sarmentosum extract (500 mg/kg body weight) were administered via oral gavage. This P. sarmentosum dose was chosen based on our previous study showing a protective P. sarmentosum methanolic extract effect on stress-induced gastric lesions (Azlina et al., 2019). Throughout the feeding period, all rats were habituated to handling to reduce their stress-related disturbances. After 28 days, the rats in the stress control, omeprazole-treated, and P. sarmentosum-treated groups were exposed to WIRS for 3.5 h. The rats in the non-stress control group were not subjected to any stress. The rats were restrained in a plastic restrainer before being placed individually in a beaker containing room-temperature tap water. The water level was adjusted to the rat’s neck level (Azlina et al., 2015). Then, the rats were sacrificed, and their stomach was isolated to measure gastric lesion index, gastric MDA content, NO level, iNOS mRNA level, SOD activity, SOD mRNA levels, TNF-α, IL-1β, and IL-6 levels. This study was approved by the UKM Animal Ethics Committee (354/2011).
2.4 Parameters measurements
2.4.1 Gastric lesion
Gastric lesions were measured using an image analyzer at ×3 magnification. The lesion length in mm was measured at the lesion’s greatest diameter. Each five petechial was equal to a 1 mm lesion. Lesion length was expressed as the lesion index according to a previously described method (Ibrahim et al., 2012).
2.4.2 Gastric MDA levels
Gastric MDA levels were measured using a previously described method (Ledwoz et al., 1986). Briefly, 2.5 mL of a trichloroacetic acid (1.22 mol/L) and hydrochloric acid (.6 mol/L) solution was added to .5 mL of the sample and incubated at room temperature for 15 min. Next, .05 mL of sodium hydroxide was added, and the sample was incubated at 100°C for 30 min before being cooled to room temperature. Then, 4 mL of n-butanol was added, and the sample was vigorously vortex for 3 min. Finally, the sample was centrifuged at 3,000 rpm for 10 min, and the absorbance of the upper layer at 535 nm was measured using a spectrophotometer.
2.4.3 Gastric SOD levels
SOD levels in gastric tissue were measured using Cayman’s SOD Assay Kit (70600; Ann Arbor, MI, United States). This kit uses a tetrazolium salt to detect O2•- generated by xanthine oxidase.
2.4.4 NO levels
NO levels in gastric tissue were measured using the Quantichrome NO Assay Kit (D2NO-100; BioAssay Systems; Hayward, CA, United States). NO was oxidized to nitrate and nitrite. Total nitrate and nitrites levels were quantified based on the absorbance at 540 nm measured using an ELISA reader.
2.4.5 SOD and iNOS mRNA levels
SOD and iNOS mRNA levels were measured using the QuantiGene Plex Assay Lit (Genospectra; Fremont, CA, United States). Tissue lysate was added to a well containing a gene-specific probe set and then hybridized overnight at 53°C. Next, wells were washed twice with bDNA wash buffer before being incubated at 46°C with an amplifier and then an alkaline phosphatase-linked label probe, with a wash step in between. Finally, streptavidin phycoerythrin was added, producing a luminescent signal proportional to the target RNA amount that was measured using a Luminex machine (Zhang et al., 2005).
2.4.6 TNF-α, IL-1β, and IL-6 protein quantification assays
TNF-α, IL-1β, and IL-6 levels in gastric tissue were measured using Panomics’ Procarta Cytokine Assay Kit (Affymetrix; Santa Clara, CA, United States) and a Luminex 200 analyzer (Luminex Corporation; Darmstadt, Germany). Procarta Protein Assays use xMAP technology (multi-analyte profiling beads) to enable simultaneous quantitation of multiple protein targets. The xMAP system combines a flow cytometer, fluorescent-dyed microspheres (beads), lasers, and digital signal processing to effectively multiplex up to 100 different assays within a single sample.
2.5 Statistical analysis
Statistical analyses were performed using SPSS v.23 (SPSS Inc., Chicago, IL, United States). The normality of each variable’s distribution was assessed using the Shapiro–Wilk test. All results are expressed as the mean ± standard error of the mean (SEM). The significance (p < .05) of differences between groups was assessed with an analysis of variance followed by Tukey’s post hoc test.
3 Results
3.1 Gastric lesions
No lesions were observed in the stomachs of rats in the non-stressed control group. Rats exposed to WIRS for 3.5 h developed gastric lesions at the glandular part of the stomach (Figure 1). Supplementation with P. sarmentosum or omeprazole significantly lowered gastric lesion scores (p = .002 and p = .026, respectively; Figure 2). Moreover, P. sarmentosum reduced stomach lesions significantly more than omeprazole (p = .002; Figure 2).
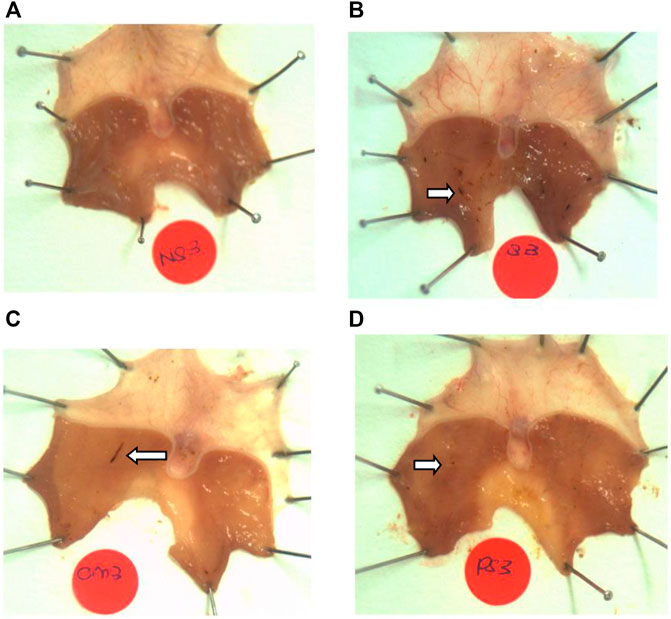
FIGURE 1. Macroscopic observation of gastric lesions in (A) normal rats (no lesions), (B) rats exposed to WIRS for 3.5 h (developed lesions), (C) omeprazole-treated, and (D) P. sarmentosum-treated. Arrows indicate gastric lesions.
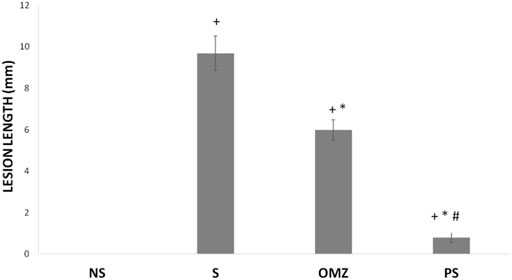
FIGURE 2. Effects of P. sarmentosum (500 mg/kg; PS) and omeprazole (20 mg/kg; OMZ) on gastric lesion length (in mm) in rats exposed to WIRS. Bars represent mean ± SEM (n = 7). Key: +, p < .05 compared to the non-stress (NS) control group; *, p < .05 compared to the stress (S) control group; #, p < .05 compared to the OMZ-treated group.
3.2 Antioxidant levels
Gastric MDA content was significantly higher in stress-exposed rats than in non-stressed rats (p = .001; Table 2). P. sarmentosum and omeprazole supplementation attenuated the stress-induced increase in MDA levels, maintaining them at similar levels to non-stress rats. P. sarmentosum and omeprazole showed comparable effects on WIRS-induced gastric MDA levels. In addition, WIRS exposure significantly reduced gastric SOD activity (p = .002). However, P. sarmentosum and omeprazole supplementation did not attenuate the reduction in SOD activity in stress-exposed rats.

TABLE 2. The effect of P. sarmentosum and omeprazole on MDA and NO levels and SOD activity in rats exposed to WIRS for 3.5 h.
SOD mRNA levels were significantly lower in non-stressed rats than in stress-exposed rats. In addition, SOD mRNA levels were significantly lower in stress-exposed rats treated with P. sarmentosum or omeprazole than in untreated stress-exposed rats (Figure 3).
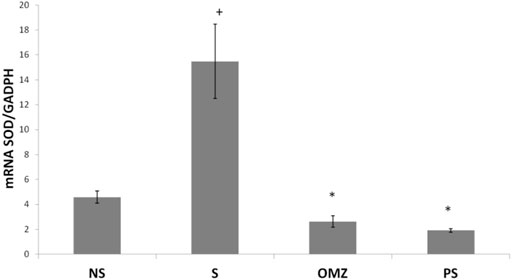
FIGURE 3. Effects of P. sarmentosum (500 mg/kg; PS) and omeprazole (20 mg/kg; OMZ) on gastric Sod mRNA levels in rats exposed to WIRS for 3.5 h. Bars represent mean ± SEM (n = 7). Key: +, p < .05 compared to the non-stress (NS) control group; *, p < .05 compared to the stress (S) control group; #, p < .05 compared to the omeprazole-treated group.
3.3 NO and iNOS mRNA levels
NO levels were significantly higher in stress-exposed rats than in non-stressed rats (p = .036). P. sarmentosum but not omeprazole supplementation significantly attenuated the increase in NO levels (p = .026; Table 2). In addition, iNOS mRNA levels were significantly higher in stress-exposed rats than in non-stressed rats (Figure 4). However, P. sarmentosum and omeprazole supplementation significantly attenuated the stress-induced elevation in gastric iNOS mRNA levels. Moreover, iNOS mRNA levels were significantly lower with P. sarmentosum than with omeprazole supplementation.
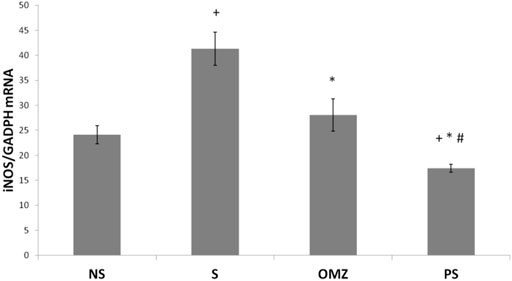
FIGURE 4. Effects of P. sarmentosum (500 mg/kg; PS) and omeprazole (20 mg/kg; OMZ) on gastric iNOS mRNA levels in rats exposed to WIRS for 3.5 h. Bars represent mean ± SEM (n = 7). Key: +, p < .05 compared to the non-stress (NS) control group; *, p < .05 compared to the stress (S) control group; #, p < .05 compared to the omeprazole-treated group.
3.4 Cytokines
Gastric TNF-α levels were significantly higher (∼2-fold) in stress-exposed rats than in non-stressed rats (p = .001; Figure 5A). P. sarmentosum supplementation significantly attenuated the increase in TNF-α levels in stress-exposed rats (p = .026). However, omeprazole supplementation did not affect TNF-α levels.
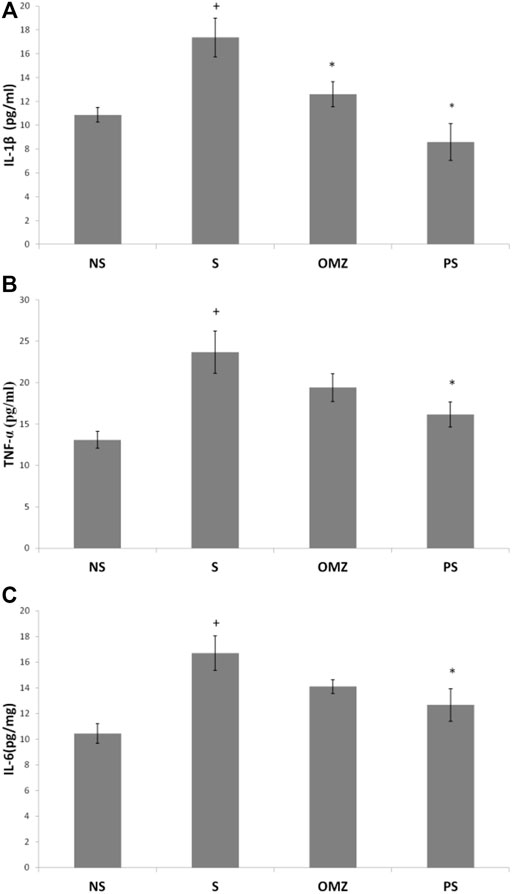
FIGURE 5. Effects of P. sarmentosum (500 mg/kg; PS) and omeprazole (20 mg/kg; OMZ) on gastric (A) TNF-α, (B) IL-1β, and (C) IL-6 protein levels in rats exposed to WIRS for 3.5 h. Bars represent mean ± SEM (n = 7). Key: +, p < .05 compared to the non-stress (NS) control group; *, p < .05 compared to the stress (S) control group; #, p < .05 compared to the omeprazole-treated group.
In addition, gastric IL-1β levels were significantly increased in stress-exposed rats than in non-stressed rats (p = .004; Figure 5B). However, P. sarmentosum (p = 1.0 × 10–4) and omeprazole (p = .043) supplementation significantly attenuated the increase in IL1-β levels in stress-exposed rats. Gastric IL-1β levels did not differ significantly between stress-exposed rats given P. sarmentosum and omeprazole supplements, suggesting they have comparable beneficial effects.
Gastric IL-6 levels were significantly increased in stress-exposed rats than in non-stressed rats (p = .004; Figure 5C). P. sarmentosum supplementation significantly attenuated the increase in gastric IL-6 levels in stress-exposed rats (p = .044). However, omeprazole supplementation did not restore gastric tissue IL-6 levels to their non-stressed values (p = .043).
4 Discussion
Our findings showed that rats exposed to WIRS for 3.5 h developed gastric lesions at the glandular part of the stomach, consistent with previous studies (Konturek et al., 2008; Kwiecien et al., 2012; Azlina et al., 2017). This study showed that P. sarmentosum could protect gastric mucosa against stress-induced injury, supporting this plant’s use as a preventive treatment against gastric mucosa injury. P. sarmentosum supplementation provided better protection against stress-induced gastric ulcers than omeprazole. Omeprazole is an established gastric ulcer treatment used in numerous studies to provide gastroprotective effects (Hajrezaie et al., 2012; Sidahmed et al., 2013). However, this study showed that pretreatment with P. sarmentosum provided better protection in reducing gastric mucosal lesions than omeprazole. Our assessment of gastric lesions was based on measuring their greatest diameter and averaging their total length.
Chan et al. (2019) described P. sarmentosum’s ability to inhibit pro-inflammatory cytokines such IL-1β, TNF-α, and Il-6. Omeprazole affects cytokines differently from P. sarmentosum, decreasing IL-1β levels but increasing IL-6 and TNF-α levels compared to the non-stress control group. While studies in H. pylori-infected humans have shown omeprazole to positively affect pro-inflammatory cytokines (Kountouras et al., 2000), our rat-based study did not show similar effects. In addition, IL-1β significantly increased intercellular adhesion molecule 1 expression (Watanabe et al., 2001) and leucocyte infiltration in the scarred mucosa’s superficial region before ulcer recurrence (Watanabe et al., 1997). They discovered that inhibiting gastric acid sufficiently with omeprazole prevented both ulcer recurrence and responses, indicating that acid may enhance gastric mucosal inflammation in response to IL-1β stimulation, resulting in gastric ulcers. Metabolic pathway analysis indicated that P. sarmentosum exerts anti-inflammatory activity mainly by affecting tryptophan metabolism (Wang et al., 2020). Its metabolic product, melatonin, has anti-inflammatory properties by inhibiting the activation of nuclear factor kappa-light-chain-enhancer of activated B cells (Wang et al., 2020). These findings are consistent with our results showing P. sarmentosum’s anti-inflammatory activity on the inflammatory signaling pathway by downregulating IL-1β, IL-6, and TNF-α.
The enhanced expression and release of IL-1β, IL-6, and TNF-α could also contribute to increased reactive oxygen species production in the gastric mucosa. Lipid peroxidation arises in biological systems due to the oxidation of unsaturated, mostly polyunsaturated, lipids, leading to the formation of free radicals and lipid peroxides that are harmful to viable tissues. Lipid peroxides quickly decompose to produce many compounds. One common byproduct of that process is MDA. MDA is present in serum, plasma, and tissues because of lipid peroxidation. It is the most reported analyte for estimating lipid peroxidation and oxidative stress (Del Rio et al., 2005; Rappaport, 2006). Our results indicate that MDA levels are significantly higher in stress-exposed rats. This study confirms the involvement of free radicals in the pathogenesis of stress-induced gastric injuries. This finding is consistent with other studies showing the importance of lipid peroxidation in causing injuries to the gastric mucosa (Kamisah et al., 2011; Nur Azlina et al., 2013).
Antioxidants have been shown to protect against gastric mucosa injury (Nur Azlina et al., 2009; Ibrahim et al., 2012; Azlina et al., 2015). This study showed that P. sarmentosum significantly reduced MDA levels compared to stress control rats, which probably reduced gastric injury by retarding the lipid peroxidation process. P. sarmentosum has higher antioxidant activity than other traditional plants (Chanwitheesuk et al., 2005). This plant also contains a natural antioxidant (naringenin, didymin, methyl piperate, quercetin, beta asarone, brachyamide, amurensin, piperitol, guineensine, hesperidin, rutin, malvidin, and difucol) (Subramaniam et al., 2003; Bactiar and Fahami, 2019; Raja Kumar et al., 2019), which might contribute to P. sarmentosum’s ability to reduce MDA. Our findings also showed no differences between P. sarmentosum and omeprazole in reducing gastric MDA levels, suggesting they have similar radical scavenging abilities. Omeprazole was previously shown to confer dose-dependent protection against ethanol (Sener et al., 2004) and stress-induced (Azlina et al., 2015) acute gastric mucosal injury by inhibiting lipid peroxidation.
Several reactive oxygen species scavenging systems, including SOD, glutathione peroxidases, and catalases, prevent their destructive action. SOD catalyzes the dismutation of O2-into less noxious hydrogen peroxide, which is further degraded by catalases or glutathione peroxidases. This study found that stress exposure led to lipid peroxide production, indicated by increased gastric tissue MDA levels and SOD mRNA levels. This effect could be due to the normal physiological response upregulating SOD expression due to impaired antioxidative enzymes. Dinu et al. (2009) found reduced SOD activity in the gastric mucosa due to stress, increasing lipid peroxidation. P. sarmentosum and omeprazole supplementation did not increase SOD expression and enzyme activity, suggesting that they do not affect SOD activity. Reduced MDA levels and lesion occurrences in the P. sarmentosum-supplemented group indicate that it acts as an antioxidant (Subramaniam et al., 2003), potentially reflecting its naringenin content, a natural antioxidant superoxide scavenger (Bactiar and Fahami, 2019; Raja Kumar et al., 2019). Antioxidant agents have been shown to be effective in treating gastric ulcers (Kudryavtsev et al., 2014).
NO exerts either protective or destructive effects depending on the extent of its production. While NO produced by endothelial NO synthase plays an important role in gastric ulcer formation and healing, NO produced by iNOS only participates in ulcer formation. This study has shown that iNOS mRNA levels were significantly higher in stress-exposed rats than in non-stressed control rats, with a concomitant increase in NO levels in the stress-exposed rats. This change is mainly due to excessive iNOS NO production in inflammatory cells, inducing oxidative tissue stress and promoting mucosal damage (Cho, 2001; Mittal et al., 2014). Nitrite levels were significantly elevated, potentially due to iNOS stimulation, which reacts with superoxide to form peroxynitrite, a potent cytotoxic oxidant causing gastric tissue damage (Lanas, 2008; Azlina et al., 2015). P. sarmentosum supplementation significantly reduced stress-induced increases in NO levels and iNOS expression. It also reduced iNOS expression to a significantly greater extent than omeprazole. Therefore, its beneficial effects are likely due to its ability to decrease gastric iNOS expression and NO levels, reducing gastric mucosal damage.
5 Conclusion
Our findings provide evidence that oral P. sarmentosum supplementation confers protection against stress-induced gastric lesions, possibly via its antioxidant mechanism, reducing pro-inflammatory cytokines, and its effects on NO through reduced iNOS expression. P. sarmentosum extract showed a better protective effect than omeprazole in reducing gastric lesions; TNF-α, IL-6, NO levels; and iNOS expression. Therefore, it is a potential therapeutic agent for gastric ulcers with similar pathologies.
Data availability statement
The original contributions presented in the study are included in the article/supplementary material, further inquiries can be directed to the corresponding author.
Ethics statement
The animal study was reviewed and approved by the Animal research of Universiti Kebangsaan Malaysia (FAR/PP/2016/AZLINA/28-SEPT./354-OCT.2016-OCT.-2019).
Author contributions
MN and MA had full access to all the data in the study and take responsibility for the integrity of the data and the accuracy of the data analysis. Study concept and design: MN, IA, and MA. MA and MN performed the research and wrote the manuscript. All authors approved the final manuscript.
Funding
This research was funded by Ministry of Education, Malaysia, grant number FGRS/1/2016/SKK08/UKM/02/5 and by Universiti Kebangsaan Malaysia, grant number UKM-DLP-066 and FF-2018-422.
Acknowledgments
We also thank to Mr Muhamad Arizi Aziz, Mrs Nurfarhana Mohd Fozi, Ms Hafizah Abas, Mrs Juliana Abdul Abdul Hamid and Fadhlullah Zuhair Japar Sidik for their technical help.
Conflict of interest
The authors declare that the research was conducted in the absence of any commercial or financial relationships that could be construed as a potential conflict of interest.
Publisher’s note
All claims expressed in this article are solely those of the authors and do not necessarily represent those of their affiliated organizations, or those of the publisher, the editors and the reviewers. Any product that may be evaluated in this article, or claim that may be made by its manufacturer, is not guaranteed or endorsed by the publisher.
Abbreviations
4-HNE, 4-hydroxynonenal; eNOS, endothelial NO synthase; FRIM, Forest Research Institute Malaysia; H2O2, hydrogen peroxide; iNOS, inducible NO synthase; IL-1β, interleukin-1β; MDA, malondialdehyde; NO, nitric oxide; NSAIDS, non-steroidal anti-inflammatory drugs; O2•-, radical anions; OMZ, omeprazole; PS, Piper sarmentosum; SAPE, streptavidin phycoerythrin; SOD, superoxide dismutase; SOD, superoxide dismutase enzyme; TNF-α, tumor necrosis factor-α; UKMAEC, University Kebangsaan Malaysia Animal Ethics Committee; WIRS, water-immersion restraint stress.
References
Amran, A. A., Zakaria, Z., Othman, F., Das, S., Raj, S., and Nordin, N. A. M. M. (2010). Aqueous extract of Piper sarmentosum decreases atherosclerotic lesions in high cholesterolemic experimental rabbits. Lipids Health Dis. 9 (1), 44–46. doi:10.1186/1476-511X-9-44
Atchison, J. W., Herndon, C. M., and Rusie, E. (2013). NSAIDs for musculoskeletal pain management:current perspectives and novel strategies to improve safety. J. Manag. Care Pharm. JMCP 19 (9), 1–19. doi:10.18553/jmcp.2013.19.s9.1
Azlina, M. F. N., Kamisah, Y., Chua, K. H., Ibrahim, I. A. A., and Qodriyah, H. M. S. (2015). Preventive effects of tocotrienol on stress-induced gastric mucosal lesions and its relation to oxidative and inflammatory biomarkers. PLoS ONE 10 (10), 01393488–e139414. doi:10.1371/journal.pone.0139348
Azlina, M. F. N., Qodriyah, H. M. S., Akmal, M. N., Ibrahim, I. A. A., and Kamisah, Y. (2019). In vivo effect of Piper sarmentosum methanolic extract on stress-induced gastric ulcers in rats. Archives Med. Sci. 15 (1), 223–231. doi:10.5114/aoms.2016.63156
Azlina, M. F. N., Qodriyah, H. M. S., Chua, K. H., and Kamisah, Y. (2017). Comparison between tocotrienol and omeprazole on gastric growth factors in stress-exposed rats. World J. Gastroenterology 23 (32), 5887–5894. doi:10.3748/wjg.v23.i32.5887
Bactiar, C. F., and Fahami, N. A. M. (2019). LC-MS analysis of phytocomponents in the methanol extract of piper sarmentosum leaves. Pharmacogn. J. 11 (5), 1071–1076. doi:10.5530/pj.2019.11.167
Chan, E. W. L., Yeo, E. T. Y., Wong, K. W. L., See, M. L., Wong, K. Y., and Gan, S. Y. (2019). Piper sarmentosum Roxb. Root extracts confer neuroprotection by attenuating beta amyloid-induced pro-inflammatory cytokines released from microglial cells. Curr. Alzheimer Res. 16 (3), 251–260. doi:10.2174/1567205016666190228124630
Chanwitheesuk, A., Teerawutgulrag, A., and Rakariyatham, N. (2005). Screening of antioxidant activity and antioxidant compounds of some edible plants of Thailand. Food Chem. 92 (3), 491–497. doi:10.1016/j.foodchem.2004.07.035
Cho, C. H. (2001). Current roles of nitric oxide in gastrointestinal disorders. J. Physiology Paris 95 (1–6), 253–256. doi:10.1016/S0928-4257(01)00034-1
Del Rio, D., Stewart, A. J., and Pellegrini, N. (2005). A review of recent studies on malondialdehyde as toxic molecule and biological marker of oxidative stress. Nutr. Metabolism Cardiovasc. Dis. 15 (4), 316–328. doi:10.1016/j.numecd.2005.05.003
Dinu, C., Diaconescu, C., and Avram, N. (2009). Gastric mucosa injury associated with oxidative stress. Lucr. Stiintife Med. Veterinaara XLII (2), 369–374.
Hajrezaie, M., Golbabapour, S., Hassandarvish, P., Gwaram, N. S., Hadi, A., Mohd Ali, H., et al. (2012). Acute toxicity and gastroprotection studies of a new schiff base derived copper (II) complex against ethanol-induced acute gastric lesions in rats. PLoS ONE 7 (12), e51537. doi:10.1371/journal.pone.0051537
Ibrahim, I. A. A., Kamisah, Y., Nafeeza, M. I., and Nur Azlina, M. F. (2012). The effects of palm vitamin E on stress hormone levels and gastric lesions in stress-induced rats. Archives Med. Sci. 8 (1), 22–29. doi:10.5114/aoms.2012.27276
Kamisah, Y., Ibrahim, A. A. I., Nafeeza, M. I., and Nur-Azlina, M. F. (2011). Palm tocotrienol-rich fraction supplementation suppressed stress-induced gastric oxidative stress in rats. J. Appl. Pharm. Sci. 1 (10), 118–122.
Ketuly, A., Hadi, K. A., Golbabapour, S., Hajrezaie, M., Hassandarvish, P., Ali, H. M., et al. (2013). Acute toxicity and gastroprotection studies with a newly synthesized steroid. PLoS ONE 8 (3), e59296–e59299. doi:10.1371/journal.pone.0059296
Khoubnasabjafari, M., Ansarin, K., and Jouyban, A. (2015). Reliability of malondialdehyde as a biomarker of oxidative stress in psychological disorders. BioImpacts 5 (3), 123–127. doi:10.15171/bi.2015.20
Konturek, S. J., Brzozowski, T., Konturek, P. C., Zwirska-Korczala, K., and Reiter, R. J. (2008). Day/night differences in stress-induced gastric lesions in rats with an intact pineal gland or after pinealectomy. J. Pineal Res. 44 (4), 408–415. doi:10.1111/j.1600-079X.2007.00543.x
Kountouras, J., Boura, P., and Lygidakis, N. (2000). Omeprazole and regulation of cytokine profile in Helicobacter pylori-infected patients with duodenal ulcer disease. Hepatogastroenterology 47 (35), 1301–1304.
Kudryavtsev, K. V., Markevich, A. O., Virchenko, O. V., Falalyeyeva, T. M., Beregova, T. V., Ostapchenko, L. I., et al. (2014). Pharmacological correction of stress-induced gastric ulceration by novel small-molecule agents with antioxidant profile. Sci. World J. 2014, 217039. doi:10.1155/2014/217039
Kwiecień, S., Brzozowski, T., and Konturek, S. J. (2002). Effects of reactive oxygen species action on gastric mucosa in various models of mucosal injury. J. Physiology Pharmacol. 53 (1), 39–50.
Kwiecien, S., Ptak-Belowska, A., Krzysiek-Maczka, G., Targosz, A., Jasnos, K., Magierowski, M., et al. (2012). Asymmetric dimethylarginine, an endogenous inhibitor of nitric oxide synthase, interacts with gastric oxidative metabolism and enhances stress-induced gastric lesions. J. Physiology Pharmacol. 63 (5), 515–524.
Lanas, A. (2008). Role of nitric oxide in the gastrointestinal tract. Arthritis Res. Ther. 10 (2), S4–S7. doi:10.1186/ar2465
Ledwoz, A., Michalak, J., Stȩpień, A., Ka̧dziołka, A., Stepien, A., et al. (1986). The relationship between plasma triglycerides, cholesterol, total lipids and lipid peroxidation products during human atherosclerosis. Clin. Chim. Acta 155 (3), 275–283. doi:10.1016/0009-8981(86)90247-0
Mittal, M., Siddiqui, M. R., Tran, K., Reddy, S. P., and Malik, A. B. (2014). Reactive oxygen species in inflammation and tissue injury. Antioxidants Redox Signal. 20 (7), 1126–1167. doi:10.1089/ars.2012.5149
Nimse, S. B., and Pal, D. (2015). Free radicals, natural antioxidants, and their reaction mechanisms. RSC Adv. 5 (35), 27986–28006. doi:10.1039/c4ra13315c
Nur Azlina, M. F., Kamisah, Y., Chua, K. H., and Qodriyah, H. M. S. (2013). Tocotrienol attenuates stress-induced gastric lesions via activation of prostaglandin and upregulation of COX-1 mRNA. Evidence-Based Complementary Altern. Med. 2013, 804796. doi:10.1155/2013/804796
Nur Azlina, M. F., Rubaizah, K., Siti Muliana, M., and Nafeeza, M. I. (2009). Modulation of restraint induced gastric oxidative changes in rats by tocotrienol and tocopherol. Int. J. Pharmacol. 5, 58–64. doi:10.3923/ijp.2009.58.64
Patlevič, P., Vašková, J., Švorc, P., Vaško, L., and Švorc, P. (2016). Reactive oxygen species and antioxidant defense in human gastrointestinal diseases. Integr. Med. Res. 5 (4), 250–258. doi:10.1016/j.imr.2016.07.004
Raja Kumar, S., Mohd Ramli, E. S., Abdul Nasir, N. A., Ismail, N. H. M., and Mohd Fahami, N. A. (2019). Preventive effect of naringin on metabolic syndrome and its mechanism of action: A systematic review. Evidence-Based Complementary Altern. Med. 2019, 9752826. doi:10.1155/2019/9752826
Rappaport, Z. H. (2006). Robotics and artificial intelligence: Jewish ethical perspectives. Acta Neurochir. Suppl. 98, 9–12. doi:10.1007/978-3-211-33303-7_2
Salehi, B., Zakaria, Z. A., Gyawali, R., Ibrahim, S. A., Rajkovic, J., Shinwari, Z. K., et al. (2019). Piper species: A comprehensive review on their phytochemistry, biological activities and applications. Molecules 24 (7), 1364. doi:10.3390/molecules24071364
Sawangjaroen, N., Sawangjaroen, K., and Poonpanang, P. (2004). Effects of Piper longum fruit, Piper sarmentosum root and Quercus infectoria nut gall on caecal amoebiasis in mice. J. Ethnopharmacol. 91 (2–3), 357–360. doi:10.1016/j.jep.2004.01.014
Sener, G., Paskaloglu, K., and Ayanoglu-Dülger, G. (2004). Protective effect of increasing doses of famotidine, omeprazole, lansoprazole, and melatonin against ethanol-induced gastric damage in rats. Indian J. Pharmacol. 36 (3), 171–174.
Sidahmed, H. M. A., Abdelwahab, S. I., Mohan, S., Abdulla, M. A., Mohamed Elhassan Taha, M., Hashim, N. M., et al. (2013). α -mangostin from cratoxylum arborescens (vahl) blume demonstrates anti-ulcerogenic property: A mechanistic study. Evidence-Based Complementary Altern. Med. 2013, 450840. doi:10.1155/2013/450840
Subramaniam, V., Adenan, M. I., Ahmad, A. R., and Sahdan, R. (2003). Natural antioxidants: Piper sarmentosum (kadok) and Morinda elliptica (mengkudu). Malays. J. Nutr. 9 (1), 41–51.
Sun, X., Chen, W., Dai, W., Xin, H., Rahmand, K., Wang, Y., et al. (2020). Piper sarmentosum Roxb: A review on its botany, traditional uses, phytochemistry, and pharmacological activities. J. Ethnopharmacol. 263, 112897. doi:10.1016/j.jep.2020.112897
Wang, D., Zhou, L., Hanlin, Z., and Hou, G. (2020). Piper sarmentosum extract alleviates inflammatory responses and improves barrier function in porcine intestinal epithelial (IPEC-J2) cells induced by lipopolysaccharide (LPS). J. Ethnopharmacol. doi:10.21203/rs.3.rs-17917/v1
Wang, F., Meng, W., Wang, B., and Qiao, L. (2014). Helicobacter pylori-induced gastric inflammation and gastric cancer. Cancer Lett. 345 (2), 196–202. doi:10.1016/j.canlet.2013.08.016
Watanabe, T., Arakawa, T., Fukuda, T., Higuchi, K., and Kobayashi, K. (1997). Role of neutrophils in a rat model of gastric ulcer recurrence caused by interleukin-1 beta. Am. J. Pathology 150 (3), 971–979.
Watanabe, T., Higuchi, K., Tominaga, K., Fujiwara, Y., and Arakawa, T. (2001). Acid regulates inflammatory response in a rat model of induction of gastric ulcer recurrence by interleukin 1beta. Gut 48 (6), 774–781. doi:10.1136/gut.48.6.774
Zakaria, Z. A., Patahuddin, H., Mohamad, A. S., Israf, D. A., and Sulaiman, M. R. (2010). In vivo anti-nociceptive and anti-inflammatory activities of the aqueous extract of the leaves of Piper sarmentosum. J. Ethnopharmacol. 128 (1), 42–48. doi:10.1016/j.jep.2009.12.021
Keywords: Piper sarmentosum, malondialdehyde, gastric ulcer, nitric oxide, iNOS, IL-1β, TNF-α, IL-6
Citation: Akmal MN, Abdel Aziz I and Nur Azlina MF (2023) Piper sarmentosum Roxb. methanolic extract prevents stress-induced gastric ulcer by modulating oxidative stress and inflammation. Front. Pharmacol. 13:971443. doi: 10.3389/fphar.2022.971443
Received: 17 June 2022; Accepted: 29 December 2022;
Published: 12 January 2023.
Edited by:
Ahmad Nazrun Shuid, MARA University of Technology, MalaysiaReviewed by:
K. M. Sakthivel, PSG College of Arts and Science, IndiaMohamed Aly Morsy, King Faisal University, Saudi Arabia
Pouya Hassandarvish, University of Malaya, Malaysia
Ahmad Naqib Shuid, Universiti Sains Malaysia (USM), Malaysia
Copyright © 2023 Akmal, Abdel Aziz and Nur Azlina. This is an open-access article distributed under the terms of the Creative Commons Attribution License (CC BY). The use, distribution or reproduction in other forums is permitted, provided the original author(s) and the copyright owner(s) are credited and that the original publication in this journal is cited, in accordance with accepted academic practice. No use, distribution or reproduction is permitted which does not comply with these terms.
*Correspondence: Mohd Fahami Nur Azlina, nurazlinamf@ukm.edu.my