- 1School of Pharmacy, Zhejiang Chinese Medical University, Hangzhou, Zhejiang, China
- 2Key Laboratory of Clinical Genetics & Key Disciplines of Clinical Pharmacy, Affiliated Hospital and Clinical Medical College of Chengdu University, Chengdu, Sichuan, China
- 3Department of Nephrology, Shaanxi Traditional Chinese Medicine Hospital, Xi’an, Shaanxi, China
- 4School of Food and Bioengineering, Chengdu University, Chengdu, Sichuan, China
Membranous nephropathy (MN) is a renal-limited non-inflammatory autoimmune disease in the glomerulus, which is the second or third main cause of end-stage kidney diseases in patients with primary glomerulonephritis. Substantial achievements have increased our understanding of the aetiology and pathogenesis of murine and human MN. The identification of nephritogenic autoantibodies against neutral endopeptidase, phospholipase A2 receptor (PLA2R) and thrombospondin type-1 domain-containing 7A (THSD7A) antigens provide more specific concept-driven intervention strategies for treatments by specific B cell-targeting monoclonal antibodies to inhibit antibody production and antibody-antigen immune complex deposition. Furthermore, additional antibody specificities for antigens have been discovered, but their pathogenic effects are uncertain. Although anti-PLA2R and anti-THSD7A antibodies as a diagnostic marker is widely used in MN patients, many questions including autoimmune response development, antigenic epitopes, and podocyte damage signalling pathways remain unresolved. This review describes the current available evidence regarding both established and novel molecular mechanisms based on systems biology approaches (gut microbiota, long non-coding RNAs, metabolite biomarkers and DNA methylation) in MN, with an emphasis on clinical findings. This review further summarizes the applications of traditional Chinese medicines such as Tripterygium wilfordii and Astragalus membranaceus for MN treatment. Lastly, this review considers how the identification of novel antibodies/antigens and unresolved questions and future challenges reveal the pathogenesis of MN.
1 Introduction
Membranous nephropathy (MN) is a major cause of antibody-associated nephrotic syndrome among adults (Gianassi et al., 2019; Ronco et al., 2021). The development of disease is triggered by accumulation of immune complex deposition in the subepithelial region with local complement activation and injury to podocytes and glomerular basement membrane (GBM) thickening (Feng et al., 2020). While 70%–80% of MN is classified as idiopathic or primary MN, the rest of MN is triggered by secondary causes, such as infections, malignancies or autoimmune diseases (Ronco et al., 2021). The two distinct types require different management approaches. In primary MN, approximately 30% of patients can improve by spontaneous remission, while the remainder show persistent proteinuria or progression to end-stage renal disease (Ronco et al., 2021). In the most severe cases, immunosuppressant treatment is required. Secondary MN requires treatment of the underlying diseases. In most patients, MN is an autoimmune disease mediated by autoantibodies directed against phospholipase A2 receptor (PLA2R) or, more unusually, thrombospondin type-1 domain-containing 7A (THSD7A) (Deng et al., 2021). However, THSD7A is not specific for primary MN. When secondary causes are excluded, the disease is called primary MN. To date, the diagnosis of MN can only be determined by renal biopsy. In recent years, MN pathogenesis was investigated by using both patients and animal models to help understand the underlying molecular mechanisms for development of immune complex deposition in GBM (Sinico et al., 2016).
This review considers the underlying molecular pathomechanisms of MN, with a particularly focus on novel pathomechanisms such as dysbiosis of gut microbiota, dysregulation of Non-coding RNAs (long non-coding RNAs, microRNAs), aberrant expression of identified proteins by using proteomics and the disorder of endogenous metabolites by metabolomics, as well as altered DNA methylation. These findings will provide strategies for patient diagnosis and monitoring and the possibility for novel treatments. We summarize the animal models of MN that helped to understand human disease. This review further describes the applications of traditional Chinese medicines such as Tripterygium wilfordii and Astragalus membranaceus for MN treatment.
2 The underlying molecular pathomechanisms of IMN in animal models
Three underlying mechanisms elucidated formation of subepithelial complex deposits in both the animal model and human MN (Jiang et al., 2020; Xu et al., 2020). The first mechanism is associated with circulating immune complexes (CIC) deposition described in chronic serum sickness; the second and third underlying mechanisms are associated with in situ immune complex formation in the capillary wall of glomerular, where the antigen is either an artificially planted exogenous antigen or a native podocyte antigen bound to the podocyte or basement membrane.
2.1 Heymann nephritis rat model
In 1959, Heymann et al. first established a rat MN model, referred to as active Heymann nephritis, which was mediated by immunizing Lewis rats using intrarenal extracts and remind human disease (Heymann et al., 1959). Because subepithelial complex deposits were triggered from intrarenal brush-border membrane rather than glomerular extracts, complex deposits were initially thought to lead to glomerular trapping of CIC containing brush-border-associated antigens and their corresponding antibodies (Sinico et al., 2016; Jiang et al., 2020). However, the rat model of passive Heymann nephritis injected by using the rabbit anti-rat brush-border antibodies argued against a role for CIC (Sinico et al., 2016; Xu et al., 2020). In the passive Heymann nephritis rat model, immune complex deposits occur in minutes after antibody injection, which argues against immune complex formation in circulation followed by their deposition in capillary wall of the glomerulus. Based on ex vivo and isolated perfused renal systems, several earlier seminal publications have highlighted that anti-brush-border antibodies could bind to an antigenic target in podocytes (Sinico et al., 2016; Xu et al., 2020), which demonstrated that MN was mediated by in situ formation of immune complexes.
In the early 1980s, Kerjaschki et al. identified the principal autoantigen megalin (also known as low density lipoprotein receptor-related protein 2), with a molecular weight of ∼600 kDa, in both active and passive Heymann nephritis (Kerjaschki and Farquhar, 1982). Megalin is a large podocyte trans-membrane protein. This polyspecific receptor, as a member of the low-density lipoprotein receptor superfamily, occurs at the sole of podocyte foot processes in the clathrin-coated pits (Figure 1). Immune deposit formation is fuelled by de novo synthesis of megalin. Epitope mapping analysis revealed that although subepithelial immune deposits were mediated by 4 megalin ligand-binding domains, full-blown disease with proteinuria was related to a specific epitope in first ligand-binding domain located in a 60 kDa N-terminal fragment (Larsen et al., 2018), and with intramolecular epitope spreading (Zhang et al., 2021).
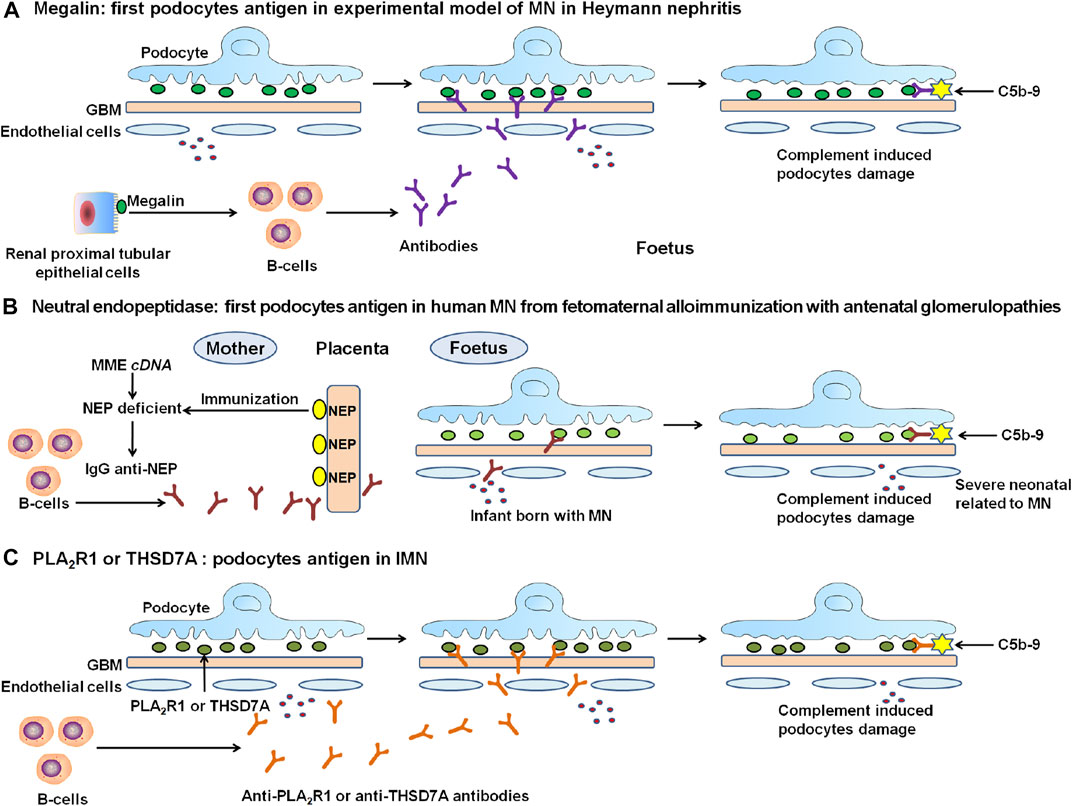
FIGURE 1. The discovery and proposed mechanisms of the representative antigens in the experimental MN and human IMN. (A) In 1982, the polyspecific receptor megalin was identified in Heymann nephritis, but not on human podocytes. (B) In humans, progress in MN started in 2002 with the discovery of target antigen namely neutral endopeptidase (NEP) as a targeting antigen in alloimmune neonatal MN. (C) In 2009, Novel causal antigen PLA2R, which is the first podocyte autoantigen identified in human IMN. Followed by PLA2R, in 2014, second antigen THSD7A was identified in human IMN. PLA2R-related and THSD7A-related MN accounted for about 70% and 1%–5% of IMN patients, respectively. The formation of immune complexes is the circulation of antibodies binding to endogenous antigens in podocytes. Once formed, these complexes are capped then released into the subepithelial region. They attach to GBM, resist degradation, and persist as immune deposits. Immune deposits form by repeated cycles, which could activate complement pathway. The deposits of subepithelial immune complexes and complement activation damage the podocyte and lead to severe proteinuria.
Heymann nephritis-like MN was also demonstrated in mice and rats (Assmann et al., 1985), where the target antigen was dipeptidyl peptidase 4. The animal model of passive Heymann nephritis was mediated in mice injected by using anti-podocyte serum (Meyer-Schwesinger et al., 2011). Although the findings from Heymann nephritis provided an important contribution to our understanding of the MN underlying pathogenetic mechanisms, there is no evidence that megalin was implicated in human MN.
2.2 Cationic bovine serum albumin-Induced IMN rat model
Border and his colleagues established a MN animal model using rabbits immunized with CBSA in 1982 (Border et al., 1982). Their experiments hypothesised that antigen charge was a key mediator for formation of subepithelial deposits, given a negative charge of the capillary wall in the glomeruli. Only rabbits immunized with CBSA showed subepithelial complex deposits of Immunoglobulin G (IgG) and complement C3, whereas those receiving anionic or native (neutral) bovine serum albumin featured mostly mesangial deposits. Those animals immunized with CBSA showed severe proteinuria. The rabbit perfused renal tissues indicated that the complexes were generated in situ after CBSA bound to anionic heparin sulphate proteoglycans in GBM, followed by antibody binding (Adler et al., 1983). bovine serum albumin chemical cationization changed its immunogenicity and caused antibody formation of low avidity and precipitability, thus promoting the complex dissociation and access of free CBSA to rabbit the capillary wall in the glomerulus (Koyama et al., 1986; Bass et al., 1990). This model was also reported in other mammal animals such as mice, rats, and dogs (Jiang et al., 2020; Sun et al., 2020). Except for CBSA, other potential antigens can also implant in the capillary wall from the glomerulus, including enzymatically modified nucleosomes that contain cationic histones and may play a role in lupus-related MN, and lectin or cationized ferritin (Beck and Salant, 2014; Liu et al., 2019).
2.3 Anti-podocyte nephritis mouse model
The CIC deposition in tissues is a well-known characteristic of chronic serum sickness, which is treated by the administration of exogenous antigen at a constant dose. In glomeruli, CIC location is strongly dependent on antibody response magnitude, immune complex size, antigen characteristics including charge, size, hemodynamics, and pharmacological factors (Couser and Salant, 1980; Alexander et al., 2016). If antigen dose is changed based on the circulating antibody response, to keep up a chronic condition of antigen excess, deposits are nearly exclusively subepithelial regardless of the concentration of antibody response (Zhu, 2016). Therefore, it is speculated that small CIC is made up of low-avidity antibody and oligovalent antigen might traverse the capillary wall.
2.4 PLA2R1-dependent mouse model
PLA2R1 is the main autoantigen in MN patients. Meyer-Schwesinger and colleagues established a novel PLA2R1-dependent mouse model that was produced using a transgenic mouse line that expressed full-length PLA2R1 in murine podocytes (Meyer-Schwesinger et al., 2020). The expression of murine PLA2R1 did not affect any morphological disturbance, showing the intact nephrin with normal foot processes in murine podocytes. Transferring rabbit anti-mPLA2R1 antibodies to these mice led to proteinuria and tissue pathological characteristics of MN. Pathological analyses showed the promotion of staining for murine PLA2R1 but unaffected staining for murine THSD7A, resembling what is demonstrated in PLA2R1-related MN patients. Taken together, this animal model will allow study of underlying PLA2R1-specific pathological mechanisms and help to evaluate antigen-specific disease and treatments.
2.5 THSD7A-induced mouse model
THSD7A expression is observed in murine podocytes. Mice injected with human serum containing anti-THSD7A antibody and human anti-THSD7A autoantibodies could specifically bind to murine THSD7A on podocyte foot processes, which led to proteinuria, and initiated a pathological change that was typical of MN (Tomas et al., 2016). The mice showed severe albuminuria from 3rd day to 70th day. The mice showed granular changes on GBM subepithelial layer, huIgG-mTHSD7A colocalization and CIC deposition. Immunofluorescence analysis showed C3 accumulation within the subepithelial granular pattern. Similarly, pathological analysis by using an electron microscope strongly demonstrated electron-dense deposits in a limited subepithelial localization and serious foot process effacement surrounding local areas. Subsequently, THSD7A-related MN was put forward in a heterologous animal model (Tomas et al., 2017). Tomas and colleagues established rabbit anti-THSD7A antibodies using co-immunization with combined murine THSD7A and human THSD7A cDNAs. Subsequently, mice were injected by using anti-THSD7A IgG purified from rabbit serum. The mice developed serious nephritic syndrome and increased albumin-to-creatinine ratio during the study period. The mice showed similar pathological characteristics as the above-mentioned THSD7A-related animal model, with the only differences being unknown IgG hypotype and undetected C3.
2.6 Anti-dipeptidyl peptidase IV rat model
DPP IV, as a FxlA antigen (gp108) (Natori et al., 1994), is distributed in brush-borders of renal tubules and glomerular capillary loops of renal tissues and gut microvilli. After injecting rabbit anti-DPP IV antibodies into rats, IgG of rabbit was deposited in capillary loops of the glomeruli and generated proteinuria. To identify target antigen, serum DPP IV-depleted rats were used and comparable results were demonstrated. This provided evidence that DPP IV distributed along the capillary wall in the glomeruli had a key effect on proteinuria formation. Compared to the gp330-induced animal model, urinary protein was transient, and here was limited C3 deposition, and faster IgG disappearance (Hunter et al., 1960). Taken together, these studies illuminate the MN pathogenesis model.
3 Clinical pathogenesis of IMN
3.1 PLA2R as a major player in IMN patients
Antibodies are disease specific as they are not observed in other proteinuric nephropathy patients, but are related to MN in several cohorts of patients (Hofstra et al., 2011). Studies have suggested that most MN patients produced an autoimmune response for either PLA2R or THSD7A, but not for two antigens (Larsen et al., 2016; Logt et al., 2021), indicating that both antigens are primary target of specific autoimmunity and verifies that PLA2R-related MN and THSD7A-related MN are separate disease entities. Several publications have demonstrated that anti-PLA2R antibody titre correlate with disease effect and patient outcomes (Brglez et al., 2020; Logt et al., 2021; Nieto-Gañán et al., 2021; Zhang et al., 2021). Low anti-PLA2R autoantibody concentrations were used for the diagnosis prediction of spontaneous remission (Jurubiță et al., 2021; Reinhard et al., 2021; Zhang et al., 2021). In addition, reduced concentrations of anti-PLA2R antibody could also predict proteinuria remission (Radice et al., 2016) and response to the traditional and new CD20-targeted immunosuppressive treatments (Ruggenenti et al., 2015). By contrast, high concentrations of anti-PLA2R antibody at baseline were associated with a reduced probability of spontaneous remission (Jurubiță et al., 2021; Reinhard et al., 2021; Zhang et al., 2021) and were related to progression to nephrotic syndrome in early non-nephrotic proteinuria patients (Gu et al., 2021; Tomas et al., 2021), who showed a high recurrent risk and progressive decline and loss of renal function (Gu et al., 2021; Tomas et al., 2021; Wu et al., 2021; Zhang et al., 2021). Similarly, several previous studies have suggested that anti-THSD7A antibody concentrations could predict disease activity and response to therapy (Hoxha et al., 2017). However, a recent study described a few patients with dual antibody positivity by using renal biopsy staining (Larsen et al., 2016). The remaining IMN patients might either be mediated by autoantibodies against yet unfound antigens or mirror misclassified PLA2R-related MN, THSD7A-related MN, or secondary MN. Therefore, the term IMN might no longer be appropriate.
Disease severity and response to drug intervention of MN patients is affected by circulating anti-PLA2R autoantibody titre. PLA2R1, as a membrane receptor, occurs extracellular region which contains a cystein-rich domain and eight different C-type lectin domains (CTLD1-8) (Xu et al., 2020; Gu et al., 2021). Cystein-rich domain is the dominant epitope of PLA2R1 antigen; while site-directed mutagenesis analyses showed CTLD1 and CTLD7 as additional and distinct PLA2R1 epitopes that are potential targets of anti-PLA2R autoreactivity (Seitz-Polski et al., 2016). Isolated anti-PLA2R reactivity against cystein-rich domain are found in younger in patients, with slight urine protein generation, an increase in spontaneous remission rate, and a decrease in risk to ESRD than patients with antibody reactivity against CTLD1 and/or CTLD7 domains suggesting that epitope profiling changes over time and that epitope distributed to CTLD1 and CTLD7 negatively influence disease (Seitz-Polski et al., 2016). These studies demonstrated that anti-PLA2R reactivity limited to the cysteine-rich domain was related to initial and milder stages of disease development. Therefore, combined anti-PLA2R reactivity and titre could enhance disease activity monitoring and drug therapy response. Whether similar findings could also be useful to THSD7A antigen is unclear.
In the past four decades, two autoantibodies provided the first clear pathological and physiological rationale for MN treatment. Therefore, combined analyses of circulating anti-PLA2R and anti-THSD7A autoantibodies as well as albumin concentrations and proteinuria levels in hypoalbuminaemia patients played an important role in understanding disease activity and forming personalized treatment using the common immunosuppressive approach or B cell-targeting monoclonal antibodies (De Vriese et al., 2017; Dong et al., 2020). Indeed, altered antibody concentrations provide a rapid and accurate indication of immune status and an integrated assessment of serum and proteinuria improve treatment decision-making and address diagnostic uncertainties when the proteinuria concentration is consistent with antibody levels. Specifically, persistent proteinuria levels but low antibody concentrations reflect chronic impairment, whereas high antibody concentrations but few obvious clinical symptoms indicate imminent recurrent disease. This integrated strategy to MN enhances accuracy of diagnosis and prognosis, restricts unnecessary intervention to immunosuppressive treatment, optimizes therapy efficacy, and reduces risk or recurrent disease severity in allotransplants (De Vriese et al., 2017). Currently, these principles are addressed in prospective research designed to detect whether treatment titration for autoantibody titre will improve the probability of remission from nephrotic syndrome and inhibit relapse while reducing the risk of treatment adverse effects. The levels of serum albumin and proteinuria remain the most sensitive markers for the prediction of disease severity and outcome in antibody-negative patients.
3.2 Activation of complement signalling pathway in IMN patients
The complement role in inducing proteinuria in MN animal models is known, and in human, C5b-9 complexes in urine are a biomarker response to disease reactivity. Although C3 and C5b-9 are universal expressions for biopsy specimens of patients with MN, the underlying pathomechanisms of activation of complement and its relative effects on clinical phenotype after activation of autoimmune system is perplexing (Oto et al., 2021). The typical pathway, such as increased C1q deposition, is related to the cases of secondary MN, rather than idiopathic MN. The mannose-binding protein indicated that alternative or lectin pathway was involved in MN pathogenesis (Borza, 2016; Hayashi et al., 2018). Some idiopathic MN patients occur due to genetic and functional deficiency and an alternate pathway is the functionally activated in these cases (Bally et al., 2016). Although in vitro experiments have shown that anti-PLA2R affects podocytes, there is no evidence that anti-PLA2R alone induced proteinuria in vivo. Tomas et al. demonstrated that passive transfer of human anti-THSD7A that expressed THSD7A on podocytes in mice initiated histopathological alternations of MN with proteinuria induction at day 3 despite a lack of activation of complement (Tomas et al., 2016). So far, few findings have revealed how anti-PLA2R and anti-THSD7A autoantibodies interplay with the complement system; this link may play a key role in increasing our understanding of the underlying mechanism driving changing proteinuria levels and clinical consequence in this subgroup of patients.
3.3 The identification of new antigens in IMN patients
From 2009, a causal antigen was found, when an unprecedented study found PLA2R as a target antigen in IMN. This achievement was followed, in 2014, by the discovery of a second antigen, THSD7A in IMN. Since 2014, some studies have reported the identification of several novel minor antigens in the podocytes of MN patients. The first new proteins and antigens were exostosin1/exostosin2 (EXT1/EXT2). EXT1/EXT2 were the most common among the unique proteins in patients with PLA2R-negative MN and are present in patients with secondary MN (Sethi et al., 2019; Salvadori and Tsalouchos., 2022). In patients with EXT1/EXT2-positive antigens compared to patients with EXT1/EXT2-negative antigens; nephrotic proteinuria is found more often in patients with membranous lupus nephritis, than in patients with decreasing glomerulosclerosis and renal fibrosis. Patients EXT1/EXT2-negative reach ESRD quickly and frequently (Ravindran et al., 2021). This was followed by identification of neural EGF-like-1 protein (NELL-1) occurred in 5–10% of patients, which is the second most common MN antigen (Sethi et al., 2020; Wang et al., 2021). NELL-1 was much more common in patients with segmental MN (Kudose et al., 2021). The third new antigen, not common but unique, was semaphorin 3B (Sema3B) which presented in pediatric patients (Sethi et al., 2020). Lastly, the latest study reported that protocadherin 7 (PCDH7), which belongs to both IgG4 subclasses, is another new antigen identified in patients with PLA2R-negative MN (Sethi, 2021), and is the third most common novel protein, after EXT1/EXT2 and NELL-1, in patients with PLA2R-negative MN. Additionally, high temperature recombinant protein A1 (HTRA1), which belongs to both IgG4 subclasses, were identified in some anti-PLA2R-negative IMN patients (Sethi et al., 2021). EXT1/EXT2, NELL-1 and Sema3B belong to the IgG1 subtype, similarly, PCDH7 was an anti-PLA2R antibody of IgG4 subtype and did not activate complement. Subsequent research is needed to verify the prevalence of these new proteins and putative antigens in MN.
In addition, tumor antigens, thyroglobulin, hepatitis B, hepatitis C and Helicobacter pylori antigens and DNA-containing material were identified in subepithelial immune complex deposits in secondary MN patients (Santoro et al., 2017; Tomas et al., 2021). These antigens might be encompassed in GBM due to physico-chemical features, as for cationic albumin. Alternatively, small, circulating, non-precipitating IgG4 complexes including these antigens might deposit in GBM as is found in the model of chronic serum sickness, although there is still no evidence from animal models to demonstrate this hypothesis in humans.
So far, all identified antigens are responsible for up to 90% of IMN patients, therefore, the future search for further minor antigens should not be abandoned (Ahmad and Appel., 2020). Approximately 1% of MN patients have double positivity for anti-THSD7A and anti-PLA2R antibodies (Kaya et al., 2021; Lwezaula et al., 2021; Zhang et al., 2021). In biopsy-proven MN patients, anti-THSD7A antibodies were found in 2.8% patients, eight of which were double positive for two antibodies (Zaghrini et al., 2019; Zhang et al., 2019; Zhang et al., 2021). Different antigens may be implicated in various pathogenesis, mode of histologic injury and various clinical phenotype including response to therapy and outcome (Beck, 2017; Sethi et al., 2021).
4 Systems biology-Based mechanisms in IMN
Systems biology including genomics, transcriptomics, proteomics, and metabolomics could offer underlying pathomechanisms to illuminate complex and refractory diseases. Genomics, transcriptomics, proteomics, and metabolomics are related to genome (DNA), transcriptome (RNA), proteome (proteins) and metabolome (metabolites), respectively (Zhao and Lin., 2014). High morbidity and mortality of chronic kidney disease (CKD) has emerged as a major public health problem worldwide (Mantovani and Chiara., 2020; Malaki, 2022; Rashid et al., 2022). Multi-omics techniques have been extensive applied to the biomarker discovery, diagnosis and prognosis, drug pharmacological and toxicity evaluation in a variety of diseases including (Cisek et al., 2016; Yang, 2020; Joshi et al., 2021). Although a number of studies of systems biology have been performed on exploring the pathomechanisms of CKD through analyzing DNA, RNA, proteins, metabolites and their molecular and metabolic pathways, the metabolomics in MN is still in its infancy compared with other CKD (Jiang et al., 2013; Cisek et al., 2016; Taherkhani et al., 2019).
4.1 The dysbiosis of gut microbiota in IMN patients
Increasing evidence has demonstrated that the dysbiosis of gut microbiota are implicated in CKD (Chen et al., 2019; Feng et al., 2019; Tsay et al., 2019; Chen et al., 2020; Liu et al., 2021; Zhao., 2022). Recently, several studies suggest that the dysbiosis of gut microbiota also contribute to the pathological mechanisms of MN (Kronbichler et al., 2015). The latest study showed that IMN patients had a significantly different α-diversity and β-diversity compared with CKD patients and healthy controls (Zhang et al., 2020). IMN patients presented with increased Fusobacteria and Proteobacteria, but decreased Firmicutes compared to healthy controls. At genus level, Megasphaera, Megamonas, Akkermansia, Lachnospira, Roseburia and Fusobacterium were higher abundant in healthy controls than CKD and IMN (Zhang et al., 2020). Faecal analysis showed the decrease in propionate and butyrate in IMN than healthy controls. Compared with the healthy controls, Parabacteroides had elevated abundance in CKD and IMN patients. In addition, Oscillospira and Ruminococcus had higher abundance in CKD patients than in IMN patients and healthy controls. At the genus level, 10 bacterial taxa had higher abundance in the healthy controls. Providencia and Myroides had higher abundance in IMN patients (Zhang et al., 2020). Collectively, these findings suggest IMN patients had a significantly different composition and reduced gut microbiota-derived short-chain fatty acids.
Another study showed that Escherichia-Shigella, Streptococcus, Peptostreptococcaceae_incertae_sedis, and Enterobacteriaceae_unclassified were more abundant, while Lachnospira, Lachnospiraceae_unclassified, Clostridium_sensu_stricto_1 and Veillonella were less abundant in patients with kidney biopsy-proven MN (Dong. et al., 2020). Megasphaera and Bilophila were more abundant, whereas Megamonas, Veillonella, Klebsiella and Streptococcus were less abundant in patients with kidney biopsy-proven immunoglobulin A nephropathy (IgAN) than in those with MN patients (Dong, R. et al., 2020). A negative correlation was shown between Escherichia-Shigella and proteinuria, Bacteroides and Klebsiella presented a positive correlation with MN stage (Dong, R. et al., 2020). In addition, Yu et al. reported MN patients exhibited more severe dysbiosis of gut microbiota than patients with diabetic kidney disease (Yu, W. et al., 2020). A higher number of pathogens in MN were major contributors to microbiome changes in MN. Metabolic pathway analysis showed the increase in interconversion of pentose/glucoronate and membrane transport in correlation to adenosine triphosphate-binding cassette transporters and phosphotransferase system in MN (Yu, W. et al., 2020). Faecal microbiota transplantation (FMT) is applied to treatment of patient with MN and chronic diarrhoea, whose mitigated symptoms and improved renal function (Zhou et al., 2021).
4.2 Non-coding RNAs in MN
4.2.1 The dysregulation of long non-coding RNAs in MN
The dysregulation of gene expression through lncRNAs because of epigenetic alternations are increasingly recognized as principal factors in many diseases including kidney diseases (Wang et al., 2021). An earlier publication identified the first dysregulated lncRNAs, X-inactive specific transcript (XIST) and nuclear enriched abundant transcript 1 (NEAT1), whose levels are significantly increased in both tubular epithelial and glomerular cells (Huang et al., 2014). The mouse podocytes treated by lipopolysaccharides led to the stable increase of XIST, but not NEAT1 levels. XIST could be detected in mouse urine, with a highly correlation to disease severity, but not serum in MN. H3K27me3 levels were downregulated in mouse kidney of MN, where also showed decreased H3K27me3 at XIST promoter regions (Huang et al., 2014). The further analysis showed that urinary XIST was significantly increased in the urine of MN patients (Huang et al., 2014). Together, the finding suggests that reduced H3K27me3 at XIST promoter regions resulted in the increased XIST levels in urine. Subsequently, the increase in XIST expression and angiotensin II levels as well as kidney and podocyte damage were observed in kidney tissues of IMN patients (Jin et al., 2019). The upregulated XIST expression induced podocyte apoptosis treated by angiotensin II, while downregulated XIST expression reversed podocyte apoptosis. MiR-217 was negatively regulated by XIST expression. MiR-217 could control Toll-like receptor 4 (TLR4) protein by targeting its 3′-untranslated region. XIST expression modulated TLR4 protein through miR-217 expression and inhibition of XIST expression decreased podocyte apoptosis mediated by angiotensin II via regulating miR-217 (Jin et al., 2019). These findings suggest that the downregulated XIST expression suppressed podocyte apoptosis via miR-217-TLR4 signalling pathway, which could retard IMN. The latest study reported that a total of 327 differentially expressed mRNAs and 48 MN-related immune genes were identified (Li et al., 2021). The mRNAs including FOS and JUN may be implicated in MN through response to immobilization stress and osteoclast differentiation. The mRNA SRY-box transcription factor 4 could contribute to MN through sponging KCNQ1OT1-miR-204-5p interaction (Li et al., 2021). Collectively, these findings suggest that the dysregulation of gene expression of lncRNAs were involved in MN.
4.2.2 The dysregulation of microRNAs in MN
The microRNAs (miRNAs) play key roles in the posttranscriptional gene regulation. Accumulated evidence has demonstrated the dysregulation of miRNAs were involved in CKD (Zhao et al., 2019). Several studies identified the dysregulation of miR-193a and miR-217 in animal models and patients with MN (Li et al., 2019; Zhang et al., 2019). Wilms’ tumor 1 (WT1) was demonstrated as a target gene of miR-193a and WT1 expression were upregulated after miR-193a inhibition (Li et al., 2019). The IMN patients showed the upregulated miR-193a expression and down-regulated WT1 and podocalyxin expression compared to healthy controls (Zhang et al., 2019). Moreover, upregulated miR-193a expression, downregulated WT1 and podocalyxin expression, increased levels of proteinuria/serum creatinine and declined estimated glomerular filtration rate (eGFR) were implicated as prominent markers for poor survival in IMN patients (Zhang et al., 2019). Of note, miR-193a combined with podocalyxin and WT1 presented an optimal effect in differentiating IMN patients from controls and in anticipating survival state. In line with patient’s results, the mRNA level of miR-193a was significantly higher in CBSA-induced MN rats than that in control rats (Li et al., 2019). Inhibition of miR-193a expression ameliorated renal and podocyte injury as well as tissue cell apoptosis in MN rats. Upregulated miR-193a expression was associated with the downregulated expression of podocalyxin, nephrotic syndrome type 1 and Notch1 in MN rats (Li et al., 2019). Inhibition of miR-193a expression by targeting WT1 inhibited kidney function decline and cell apoptosis in renal tissues. In contrast to miR-193a expression, the miR-217 expression was significantly downregulated and tumor necrosis factor ligand superfamily member 11 was significantly upregulated in MN compared with control patients (Rani et al., 2017). The tumor necrosis factor ligand superfamily member 11 was confirmed to be the target gene of miR-217. The upregulated miR-217 expression inhibited tumor necrosis factor ligand superfamily member 11 expression and reduced human podocyte apoptosis (Rani et al., 2017). Similarly, the downregulated miR-217 expression was also observed in kidney tissues of IMN patients compared with normal kidney tissues, and XIST downregulation alleviated podocyte apoptosis via the miR-217-TLR4 pathway in IMN (Jin et al., 2019).
In addition, the miR-186 expression was significantly downregulated while the protein expression of TLR4 and P2×7 was upregulated in renal tissues of MN patients (Sha et al., 2015). In vitro experiments showed that TLR4 siRNA led to the upregulated miR-186 expression and miR-186 inhibitor upregulated mRNA and protein expression of P2×7 as well as cleaved-caspase-3 levels in podocytes induced by angiotensin II. The TUNEL-positive cells and caspase-3 activity of podocytes mediated by angiotensin II were downregulated by miR-186 mimic (Sha et al., 2015). The latest study reported that the expression of miR-19b, miR-106a, and miR-17 was significantly downregulated in IMN patients, whereas phosphatase and tensin homolog (PTEN) protein expression was significantly upregulated compared with controls (Wu et al., 2021). The expression of miR-106a and miR-19b was negatively correlated with serum PTEN protein expression, which were positively correlated with serum creatinine, cystatin C, urea, 24 h urine total protein and negatively correlated with albumin and eGFR (Wu et al., 2021). In addition, downregulated miR-500a-5p was identified in kidney tissues of IMN patients, which participated in the regulation of podocyte apoptosis through Circ_0000524/miR-500a-5p/CXCL16 pathway in MN (Sun et al., 2021). Besides renal tissue samples, 326 miRNAs showed a significant difference in peripheral blood lymphocyte cells between MN patients and healthy controls, which included 286 decreased miRNAs and 40 increased miRNAs (Chen et al., 2014). Of note, six novel miRNAs showed differential expression levels between MN patients and healthy controls (Chen et al., 2014). Another study showed that both peripheral blood and urinary miR-192-3p, miR-195-5p, miR-328-5p, and their target genes RAB1A, PPM1A and BRSK1 may be potential markers for MN by taking part in inflammation (Zhou et al., 2019). Collectively, miRNAs provide a potential progression in the pathogenesis of MN and provide a new marker for diagnosis and intervention of MN.
4.3 The disorder of proteome in MN
Proteomic biomarkers might improve the management of CKD patients by enabling more accurate and earlier prediction of renal function than was possible with serum creatinine and urinary albumin. Earlier studies from Ghiggeri’s research group identified the podocyte neo-expressed intracellular MN antigens by proteomic approach (Murtas and Ghiggeri., 2016). Specific anti-aldose reductase (AR) and anti-manganese superoxide dismutase (SOD2) IgG4 were detected in serum of MN patients and had high titers of anti-AR and anti-SOD2 IgG4 from the glomeruli of MN kidneys but not other glomerulonephritides (Prunotto et al., 2010). The co-localization of anti-AR and anti-SOD2 with IgG4 and C5b-9 were observed in electron-dense podocyte immune deposits. An increase of SOD2 expression was observed on podocyte plasma membrane after treatment with hydrogen peroxide (Prunotto et al., 2010). Another study demonstrated the existence of three immune proteins including α-enolase, elongation factor 2 and glycyl aminoacyl-tRNA synthetase in glomeruli of patients with membranous glomerulonephritis (Bruschi et al., 2011). The co-localization of α-enolase with IgG4 and C5b-9 in immune-deposits was considered an auto-antigen. Anti-α-enolase IgG4 levels were observed in serum of 131 patients with membranous glomerulonephritis and were elevated in 25% of patients (Bruschi et al., 2011). In addition, further study indicated that IgG4 is the prevalent isotype for antibodies against cytoplasmic antigens of podocytes such as AR, SOD2 and α-enolase. Their levels were higher than in other proteinuric glomerulonephritides and normal controls and were correlated with anti-PLA2R levels (Murtas et al., 2012).
Based on the tandem mass spectrometry technique, Sui et al. identified a total of 423 differential proteins in biopsy tissues of MN patients compared to controls. Of these, upregulated 202 proteins and downregulated 221 proteins were associated with immune response activation (Sui et al., 2015). Li et al. identified 249 proteins that were associated with immunization and coagulation and the increased excretion of α-1-antitrypsin and afamin were demonstrated in urine of IMN patients compared with normal controls (Pang et al., 2018). These findings revealed significant role of immunologic mechanism in the development of IMN. Using an animal model of passive Heymann nephritis, Ngai et al. identified Most of altered proteins in urine have functional significance in glomerular trafficking and controlling the glomerular permeability (Ngai et al., 2006).
The deposition of complement is characteristic of MN (Tsai et al., 2019). Traditional renal biopsy assessment is limited to C1q and C3c staining, which showed only limited information and understanding of the pathomechanisms of complement in MN. Ravindran et al. identified high abundance for PLA2R and EXT1/EXT2 in corresponding patients of PLA2R- and EXT1/EXT2-positive MN that had high abundance of complement proteins including C3, C4, C5, C6, C7, C8 and C9 (Ravindran et al., 2020). C1 was observed in low abundance in EXT1/EXT2-related MN. Complement activation regulators were detected in MN including upregulation of factor H, factor H-related (FHR)-1, FHR-5, clusterin and vitronectin expressions as well as downregulation of FHR-3, FHR-4 and CD59 expressions (Ravindran et al., 2020). IgG4 and IgG1 were the most abundant IgG subclasses in PLA2R- and EXT1/EXT2-related MN. These findings conclude that anti-complement agents might be effective in treatment for MN. The identified proteins were related to the pathogenesis of MN and might represent candidate MN markers. These studies underscore the important influence of proteomics in illuminating pathophysiology and biomarker discovery of MN.
4.4 The disorder of metabolites in MN
Metabolomic technique has been extensively applied to various CKD (Zhao., 2013; Chen et al., 2016; Chen et al., 2017; Chen et al., 2019; Feng et al., 2019; Miao et al., 2020). PLA2R was used for the diagnosis and monitoring of MN, whereas the underlying mechanisms of PLA2R-related MN remain unclear. In urine, Kim et al. investigated metabolomic profiles of PLA2R-related MN patients with biopsy-proven with those of patients with minimal alteration disease and to healthy controls (Jo et al., 2021). Fumarate was identified as a significant altered metabolite in PLA2R-related MN compared with minimal change disease. High fumarate levels in urine could indicate composite outcome of MN. Fumarate hydratase, which could hydrolyze fumarate, colocalized with downregulated podocalyxin expression in glomerulus from patients with PLA2R-related MN than in those from healthy controls, patients with non-PLA2R-related MN or minimal change disease. Podocytes stimulated with IgG purified from high serum anti-PLA2R titre reduced fumarate hydratase expression and increased fumarate levels, which were associated with the changes in the expression of the podocytes phenotypic profile including WT1, zonula occluden-1, Snail, and fibronectin, an elevation in albumin flux across podocyte layer and reactive oxygen species production in podocytes. Fumarate hydratase overexpression retarded these alterations, while fumarate hydratase knockdown showed synergistic effects. Therefore, fumarate could promote alterations in the phenotypic profiling of podocytes after PLA2R autoimmunity development. These studies indicated that fumarate could be considered as a promising target for PLA2R-related MN treatment.
Membranous glomerulonephritis is one of causes of nephrotic syndrome in adults. Taherkhani and his colleagues identified a panel of metabolites such as allantoic acid, deoxyuridine, oxalic acid, 2-hydroxyglutaric acid lactone, 3,4-dihydroxymandelic acid, α-hydroxybutyric acid, 5α-cholestanone, nicotinamide, epicoprostanol and palmitic acid that associated with nine impaired pathways such as pyrimidine metabolism and NAD salvage in patients with membranous glomerulonephritis (Taherkhani et al., 2018; Taherkhani et al., 2019). Hao et al. identified increased glucose, dimethylamine and trimethylamine levels as well as decreased pyruvate, valine, hippurate, isoleucine, phenylacetylglycine, citrate, tyrosine, 3-methylhistidine and β-hydroxyisovalerate levels in urine of focal segmental glomerulosclerosis compared with healthy controls (Hao et al., 2013).
Park et al. identified increasing urinary glycine levels were related to lower risk of eGFR decline in IgAN patients. Human kidney tubular epithelial cells treated by glycine mitigated inflammatory signaling mediated by tumour necrosis factor-α. This finding suggests that urinary glycine might be a protective biomarker and has a diagnostic and prognostic value for IgAN. Taken together, these findings suggest that disorder of metabolites were implicated in the pathomechanisms of MN and the metabolites could be considered as promising biomarkers for the prediction of MN.
4.5 DNA methylation in MN
Histone H3K4 trimethylation (H3K4 me3) that was demonstrated in active euchromatic regions played a key role in podocyte function that actin filaments were high in foot processes of glomeruli. H3K4 me3 showed a pattern of nuclear expression in renal podocytes of MN patients. Overlapping H3K4 me3 and cathepsin L expression were increased in MN patients compared with controls. H3K4 me3 was negatively and positively correlated with synaptopodin and proteinuria levels in MN patients, respectively (Fujino and hasebe., 2016). H3K4 me3 levels were increased in podocytes of mice treated by lipopolysaccharide, accompanied by increased podocyte swelling, creatinine in serum and albumin and cathepsin L in urine as well as downregulated synaptopodin expression. H3K4 me3 expression at cathepsin L promoter was increased in lipopolysaccharide-induced mouse kidneys (Fujino and hasebe., 2016). Treatment with shRNA against mixed-lineage leukemia protein 3 to lipopolysaccharide-treated mice and podocytes cocultured with lipopolysaccharide-induced macrophages mitigated podocyte swelling, increased creatinine levels in serum and albumin levels in urine and upregulated expression of histone H3K4 me3 and cathepsin L, and downregulated synaptopodin expression and upregulated in H3K4 me3 expression at the promoter of cathepsin L (Fujino and hasebe., 2016). These findings suggest that H3K4 me3 upregulation was implicated in podocyte injury and MN pathophysiology. Targeting H3K4 me3 followed by regulating the actin dynamics might be an effective strategy to retard MN outcomes. In addition, Sui et al. reported that 108 genes were significantly different expression in peripheral blood mononuclear cells from MN patients compared with healthy controls (Sui et al., 2014). MN patients showed increased activity of 75 H3K9me3 genes and reduced activity of 33 compared with controls. Five positive genes were selected and quantified (Sui et al., 2014). These findings suggest that H3K9me3 might be a target for epigenetic-associated MN therapy.
In addition, mounting studies have demonstrated that chronic kidney diseases were associated with transforming growth factor-β1 (TGF-β1)/Smad, Wnt/β-catenin and Keap1/Nrf2 signaling pathway (Lan, 2021; Li et al., 2021; Luo et al., 2021; Yu et al., 2022). Whether these signaling pathways are involved in the pathogenesis of MN, recent several publications show that these signaling pathways are implicated in MN (Lee, 2011; Sutariya et al., 2016; Kiewisz et al., 2019; Dong et al., 2021). Therefore, these signaling pathways should be further investigated to find the novel therapy for MN.
5 Treatment of traditional Chinese medicine on MN
5.1 Treatment of traditional Chinese medicine on patients with MN
Traditional Chinese medicines have been extensively demonstrated to be an important therapy for the CKD treatment (Wang et al., 2018; Geng et al., 2020; Meng et al., 2020; Fang et al., 2021; Cao et al., 2022; He et al., 2022; Liu et al., 2022; Miao et al., 2022). Increasing publications have showed that traditional Chinese medicines exhibited the excellent efficacy in the treatment of patients with MN (Yang et al., 2021; Wang et al., 2022) (Table 1). A previous study has demonstrated that a 77-year-old woman with IMN was intervened with Astragalus membranaceus and showed remission (Ahmed et al., 2007). In addition, a multicenter randomized controlled clinical study demonstrated that Shenqi particle were safety and exhibited an efficacy effect for patients with IMN and nephrotic syndrome (Chen et al., 2013). Recent several studies demonstrate that traditional Chinese medicines show the beneficial effect on patients with MN. For instance, treatment with Jian pi qu shi formula improve MN patients who fail to immunosuppressive intervention, and indicate that 80% of patients obtain remission, while no obvious adverse effects are demonstrated after 1 year of follow-up (Shi et al., 2018). Similarly, treatment with Shulifenxiao formula exhibits the efficacy effect for steroid and immunosuppressant-resistant refractory IMN patients (Cui et al., 2021). Therefore, these therapies may be an important option for steroid and general immunosuppressant resistant IMN patients.
The latest several studies also show that a number of Chinese herbal injections are superior to treatment of chemical drugs alone in improving primary nephrotic syndrome. For example, Tripterygium wilfordii shows anti-inflammatory and immunosuppressive activities and improve autoimmune and inflammatory diseases including rheumatoid arthritis, kidney disease and systemic lupus erythematosus. Combining multi-glycosides of Tripterygium wilfordii with prednisone is an alternative therapy for IMN. The remission probability is similar for both Tripterygium wilfordii multi-glycosides and tacrolimus (Jin et al., 2020). Mechanistically, the latest publication shows that a total of 153 compound-related genes are associated with 1485 IMN-related genes and 45 core genes are overlapped between two categories (Shi et al., 2022). The protein-protein interaction network and MCODE results show that the targets such as tumor protein p53, mitogen-activated protein kinase 8, signal transducer and activator of transcription 3, intercellular adhesion molecule 1, interleukin-4, transforming growth factor β1 and matrix metalloproteinase-1 play critical roles in the treatment of Tripterygium wilfordii on IMN (Shi et al., 2022). Enrichment analysis indicates that major pathways are advanced glycation end products, interleukin-17, tumor necrosis factor and Toll-like receptor signaling pathways (Shi et al., 2022). In addition, the combination of chemical drugs and Yinxingdamo injection or Danhong injection showed the best Chinese herbal injections compared with total clinical effectiveness, serum albumin and 24-h urinary protein excretion (Yu, H. et al., 2020). These findings demonstrate that traditional Chinese medicines can effectively ameliorate patients with IMN.
5.2 Treatment of traditional Chinese medicine on animal models with MN
The underlying pathogenesis has been investigated and a number of potential molecular mechanisms have been illuminated by using animal models. An earlier study revealed that Astragaloside IV ameliorated complement attack complex-induced podocyte damage through suppressing extracellular regulated protein kinase activity (Zheng et al., 2012). Recent several studies demonstrate that traditional Chinese medicines show a beneficial effect on animal models with MN. For example, treatment with total coumarins of Hydrangea paniculata ameliorates renal damage and dyslipidaemia, which are associated with inhibition of complement activation and phosphoinositide 3-kinase-protein kinase B pathways (Wang, W. et al., 2022). After oral administration, pharmacokinetics indicates that plasma 7-hydoxycoumarin and skimmin are main compound or metabolite. This study further shows that 7-hydoxycoumarin and skimmi may suppress interleukin-10 production through blocking phosphoinositide 3-kinase-protein kinase B and nuclear factor ƙB (NF-ƙB) signaling pathways (Wang et al., 2022). Another study shows that treatment with Sanqi oral solution reduces proteinuria levels, blunts kidney damage, suppresses depositions of C3 and IgG, and maintains protein expressions of podocin and synaptopodin in CBSA-induced MN rats, which are associated with NF-ƙB signaling pathway (Tian et al., 2019). In addition, other study shows that wenyang lishui decoction significantly reduces 24 h urine protein level and kidney histological injury in CBSA-induced rats, similarly, in vitro experiment shows that wenyang lishui decoction inhibits apoptosis rate in mouse podocytes incubated with RPMI-1640 medium containing 10% serum from patients with IMN, which are associated with decreasing p53 in both mRNA and protein expression and increasing Bcl-2 in both mRNA and protein expression (Lu et al., 2020). These findings reveal that traditional Chinese medicines ameliorate MN through regulating phosphoinositide 3-kinase-protein kinase B and NF-ƙB signaling pathways.
6 Future therapies and challenges
Currently, available therapies in MN patients are described in Kidney Disease: Improving Global Outcomes guidelines in 2021. The patients should adopt optimal conservative treatment for at least 6 months or until eGFR decline. Based on proteinuria severity, eGFR change and anti-PLA2R antibody levels, patients are eligible for immunosuppressive treatment. Cyclophosphamide, rituximab or a calcineurin inhibitor may be considered based on expected efficacy and side effects. Over the past decade, achievements in the understanding of the pathomechanisms of MN will establish novel therapy perspectives, which have provided an opportunity for the establishment of more specific regimens that were beneficial for MN patients. The discovery of PLA2R as the predominant antigen in MN fulfilled an unprecedented breakthrough point in the mechanisms of MN, subsequently, the discovery of serum anti-PLA2R antibodies paved the way toward new regimens. Because patients with high serum pathogenic antibodies showed the most severe disease, the removal of antibodies might be beneficial for MN patients. Specific therapies require identification of pathogenic epitopes. Earlier study discovered two mimotopes that mimic conformational epitope recognized by maternal alloantibodies against NEP and immuno-adsorption of maternal serum removed most of the anti-NEP antibodies (Ronco and Debiec., 2017). Currently, a small pilot study of anti-PLA2R antibodies immune adsorption is used for establishment of the feasibility of rendering patients seronegative for anti-PLA2R antibodies in lack of immunosuppression (Hamilton et al., 2018). Removal of serum anti-PLA2R antibodies by immune adsorption ameliorated disease severity, but it could not cure the disease. It will remain necessary to block antibody production. Similarly, the study of THSD7A antigen/antibody is also considered.
If IgG4 antibodies lead to the podocyte damage by binding to PLA2R, independent from complement activation, it would provide feasibility to establish therapies by small peptides that would intervene in the binding of the antibodies to PLA2R antigen. However, substantial evidence showed that complement was activated and C5b–9 assembly caused podocyte damage. The coexistence of IgG1 in immune complex deposits could mediate complement activation. However, most IMN patients showed very low or undetectable levels of complement C1q in immune complex deposits compared with secondary MN patients. Therefore, these findings indicated that lectin signaling pathway might participate in complement activation and C5b–9 complex formation. Indeed, mannose-binding lectin signaling pathway was found in the glomeruli of most IMN patients. The pathomechanisms of C5b–9 complex formation provides an opportunity to develop targeted therapies through complement-specific antibodies.
Author contributions
YYZ designed and wrote the review. HM, YMZ, XY, and LZ revised the manuscript. All authors accepted the final version of the review.
Funding
This study was supported by the National Key Research and Development Project (No. 2019YFC1709405), National Natural Science Foundation of China (Nos. 82074002, 81872985) and Shaanxi Science and Technology Innovation Team (No. 2019TD-016).
Conflict of interest
The authors declare that the research was conducted in the absence of any commercial or financial relationships that could be construed as a potential conflict of interest.
Publisher’s note
All claims expressed in this article are solely those of the authors and do not necessarily represent those of their affiliated organizations, or those of the publisher, the editors and the reviewers. Any product that may be evaluated in this article, or claim that may be made by its manufacturer, is not guaranteed or endorsed by the publisher.
References
Adler, S. G., Wang, H., Ward, H. J., Cohen, A. H., and Border, W. A. (1983). Electrical charge. Its role in the pathogenesis and prevention of experimental membranous nephropathy in the rabbit. J. Clin. Invest. 71 (3), 487–499. doi:10.1172/jci110793
Ahmad, S. B., and Appel, G. B. (2020). Antigens, antibodies, and membranous nephropathy: A decade of progress. Kidney Int. 97 (1), 29–31. doi:10.1016/j.kint.2019.10.009
Ahmed, M. S., Hou, S. H., Battaglia, M. C., Picken, M. M., and Leehey, D. J. (2007). Treatment of idiopathic membranous nephropathy with the herb Astragalus membranaceus. Am. J. Kidney Dis. 50 (6), 1028–1032. doi:10.1053/j.ajkd.2007.07.032
Alexander, J. J., Chaves, L. D., Chang, A., Dighe, S., Jacob, A., and Quigg, R. J. (2016). Abrogation of immune complex glomerulonephritis by native carboxypeptidase and pharmacological antagonism of the C5a receptor. Cell. Mol. Immunol. 13 (5), 651–657. doi:10.1038/cmi.2015.45
Assmann, K. J., Ronco, P., Tangelder, M. M., Lange, W. P., Verroust, P., and Koene, R. A. (1985). Comparison of antigenic targets involved in antibody-mediated membranous glomerulonephritis in the mouse and rat. Am. J. Pathol. 121 (1), 112–122.
Bally, S., Debiec, H., Ponard, D., Dijoud, F., Rendu, J., Fauré, J., et al. (2016). Phospholipase A2 receptor-related membranous nephropathy and mannan-binding lectin deficiency. J. Am. Soc. Nephrol. 27 (12), 3539–3544. doi:10.1681/asn.2015101155
Bass, P. S., Drake, A. F., Wang, Y., Thomas, J. H., and Davies, D. R. (1990). Cationization of bovine serum albumin alters its conformation as well as its charge. Lab. Invest. 62 (2), 185–188.
Beck, L. H. (2017). PLA2R and THSD7A: Disparate paths to the same disease? J. Am. Soc. Nephrol. 28 (9), 2579–2589. doi:10.1681/asn.2017020178
Beck, L. H., and Salant, D. J. (2014). Membranous nephropathy: From models to man. J. Clin. Invest. 124 (6), 2307–2314. doi:10.1172/jci72270
Border, W. A., Ward, H. J., Kamil, E. S., and Cohen, A. H. (1982). Induction of membranous nephropathy in rabbits by administration of an exogenous cationic antigen. J. Clin. Invest. 69 (2), 451–461. doi:10.1172/jci110469
Borza, D. B. (2016). Alternative pathway dysregulation and the conundrum of complement activation by IgG4 immune complexes in membranous nephropathy. Front. Immunol. 7, 157. doi:10.3389/fimmu.2016.00157
Brglez, V., Boyer-Suavet, S., Zorzi, K., Fernandez, C., Fontas, E., Esnault, V., et al. (2020). Personalized medicine for PLA2R1-related membranous nephropathy: A multicenter randomized control trial. Front. Med. 7, 412. doi:10.3389/fmed.2020.00412
Bruschi, M., Carnevali, M. L., Murtas, C., Candiano, G., Petretto, A., Prunotto, M., et al. (2011). Direct characterization of target podocyte antigens and auto-antibodies in human membranous glomerulonephritis: Alfa-enolase and borderline antigens. J. Proteomics 74 (10), 2008–2017. doi:10.1016/j.jprot.2011.05.021
Cao, G., Miao, H., Wang, Y. N., Chen, D. Q., Wu, X. Q., Chen, L., et al. (2022). Intrarenal 1-methoxypyrene, an aryl hydrocarbon receptor agonist, mediates progressive tubulointerstitial fibrosis in mice. Acta Pharmacol. Sin. doi:10.1038/s41401-022-00914-6
Chen, D. Q., Cao, G., Chen, H., Argyopoulos, C. P., Yu, H., Su, W., et al. (2019). Identification of serum metabolites associating with chronic kidney disease progression and anti-fibrotic effect of 5-methoxytryptophan. Nat. Commun. 10 (1), 1476. doi:10.1038/s41467-019-09329-0
Chen, D. Q., Cao, G., Chen, H., Liu, D., Su, W., Yu, X. Y., et al. (2017). Gene and protein expressions and metabolomics exhibit activated redox signaling and Wnt/β-catenin pathway are associated with metabolite dysfunction in patients with chronic kidney disease. Redox Biol. 12, 505–521. doi:10.1016/j.redox.2017.03.017
Chen, H., Cao, G., Chen, D. Q., Wang, M., Vaziri, N. D., Zhang, Z. H., et al. (2016). Metabolomics insights into activated redox signaling and lipid metabolism dysfunction in chronic kidney disease progression. Redox Biol. 10, 168–178. doi:10.1016/j.redox.2016.09.014
Chen, H., Wang, M. C., Chen, Y. Y., Chen, L., Wang, Y. N., Vaziri, N. D., et al. (2020). Alisol B 23-acetate attenuates CKD progression by regulating the renin-angiotensin system and gut-kidney axis. Ther. Adv. Chronic Dis. 11, 2040622320920025. doi:10.1177/2040622320920025
Chen, W., Lin, X., Huang, J., Tan, K., Chen, Y., Peng, W., et al. (2014). Integrated profiling of microRNA expression in membranous nephropathy using high-throughput sequencing technology. Int. J. Mol. Med. 33 (1), 25–34. doi:10.3892/ijmm.2013.1554
Chen, Y., Deng, Y., Ni, Z., Chen, N., Chen, X., Shi, W., et al. (2013). Efficacy and safety of traditional Chinese medicine (Shenqi particle) for patients with idiopathic membranous nephropathy: A multicenter randomized controlled clinical trial. Am. J. Kidney Dis. 62 (6), 1068–1076. doi:10.1053/j.ajkd.2013.05.005
Chen, Y. Y., Chen, D. Q., Chen, L., Liu, J. R., Vaziri, N. D., Guo, Y., et al. (2019). Microbiome-metabolome reveals the contribution of gut-kidney axis on kidney disease. J. Transl. Med. 17 (1), 5. doi:10.1186/s12967-018-1756-4
Cisek, K., Krochmal, M., Klein, J., and Mischak, H. (2016). The application of multi-omics and systems biology to identify therapeutic targets in chronic kidney disease. Nephrol. Dial. Transpl. 31 (12), 2003–2011. doi:10.1093/ndt/gfv364
Couser, W. G., and Salant, D. J. (1980). In situ immune complex formation and glomerular injury. Kidney Int. 17 (1), 1–13. doi:10.1038/ki.1980.1
Cui, H., Fu, F. Q., Liu, B., Liu, W. J., and Liu, Y. N. (2021). Herbal medicine "Shulifenxiao" formula for nephrotic syndrome of refractory idiopathic membranous nephropathy. Front. Pharmacol. 12, 675406. doi:10.3389/fphar.2021.675406
De Vriese, A. S., Glassock, R. J., Nath, K. A., Sethi, S., and Fervenza, F. C. (2017). A proposal for a serology-based approach to membranous nephropathy. J. Am. Soc. Nephrol. 28 (2), 421–430. doi:10.1681/asn.2016070776
Deng, L., Huang, Q., Wang, J., Luo, K., Liu, J., Yan, W., et al. (2021). Efficacy and safety of different immunosuppressive therapies in patients with membranous nephropathy and high PLA2R antibody titer. Front. Pharmacol. 12, 786334. doi:10.3389/fphar.2021.786334
Dong, R., Bai, M., Zhao, J., Wang, D., Ning, X., and Sun, S. (2020). A comparative study of the gut microbiota associated with immunoglobulin a nephropathy and membranous nephropathy. Front. Cell. Infect. Microbiol. 10, 557368. doi:10.3389/fcimb.2020.557368
Dong, Z., Dai, H., Gao, Y., Feng, Z., Liu, W., Liu, F., et al. (2021). Inhibition of the Wnt/β-catenin signaling pathway reduces autophagy levels in complement treated podocytes. Exp. Ther. Med. 22 (1), 737. doi:10.3892/etm.2021.10169
Dong, Z., Liu, Z., Dai, H., Liu, W., Feng, Z., Zhao, Q., et al. (2020). The potential role of regulatory B cells in idiopathic membranous nephropathy. J. Immunol. Res. 2020, 7638365. doi:10.1155/2020/7638365
Fang, C. Y., Lou, D. Y., Zhou, L. Q., Wang, J. C., Yang, B., He, Q. J., et al. (2021). Natural products: Potential treatments for cisplatin-induced nephrotoxicity. Acta Pharmacol. Sin. 42 (12), 1951–1969. doi:10.1038/s41401-021-00620-9
Feng, Y. L., Cao, G., Chen, D. Q., Vaziri, N. D., Chen, L., Zhang, J., et al. (2019). Microbiome-metabolomics reveals gut microbiota associated with glycine-conjugated metabolites and polyamine metabolism in chronic kidney disease. Cell. Mol. Life Sci. 76 (24), 4961–4978. doi:10.1007/s00018-019-03155-9
Feng, Y. L., Chen, H., Chen, D. Q., Vaziri, N. D., Su, W., Ma, S. X., et al. (2019). Activated NF-κB/Nrf2 and Wnt/β-catenin pathways are associated with lipid metabolism in CKD patients with microalbuminuria and macroalbuminuria. Biochim. Biophys. Acta. Mol. Basis Dis. 1865 (9), 2317–2332. doi:10.1016/j.bbadis.2019.05.010
Feng, Z., Liu, W., Jiang, H. X., Dai, H., Gao, C., Dong, Z., et al. (2020). How does herbal medicine treat idiopathic membranous nephropathy? Front. Pharmacol. 11, 994. doi:10.3389/fphar.2020.00994
Fujino, T., and Hasebe, N. (2016). Alteration of histone H3K4 methylation in glomerular podocytes associated with proteinuria in patients with membranous nephropathy. BMC Nephrol. 17 (1), 179. doi:10.1186/s12882-016-0390-8
Geng, X. Q., Ma, A., He, J. Z., Wang, L., Jia, Y. L., Shao, G. Y., et al. (2020). Ganoderic acid hinders renal fibrosis via suppressing the TGF-β/Smad and MAPK signaling pathways. Acta Pharmacol. Sin. 41 (5), 670–677. doi:10.1038/s41401-019-0324-7
Gianassi, I., Allinovi, M., Caroti, L., and Cirami, L. C. (2019). Broad spectrum of interferon-related nephropathies-glomerulonephritis, systemic lupus erythematosus-like syndrome and thrombotic microangiopathy: A case report and review of literature. World J. Nephrol. 8 (7), 109–117. doi:10.5527/wjn.v8.i7.109
Gu, Y., Xu, H., and Tang, D. (2021). Mechanisms of primary membranous nephropathy. Biomolecules 11 (4), 513. doi:10.3390/biom11040513
Hamilton, P., Kanigicherla, D., Hanumapura, P., Walz, L., Kramer, D., Fischer, M., et al. (2018). Peptide GAM immunoadsorption therapy in primary membranous nephropathy (PRISM): Phase II trial investigating the safety and feasibility of peptide GAM immunoadsorption in anti-PLA2R positive primary membranous nephropathy. J. Clin. Apher. 33 (3), 283–290. doi:10.1002/jca.21599
Hao, X., Liu, X., Wang, W., Ren, H., Xie, J., Shen, P., et al. (2013). Distinct metabolic profile of primary focal segmental glomerulosclerosis revealed by NMR-based metabolomics. PLoS One 8 (11), e78531. doi:10.1371/journal.pone.0078531
Hayashi, N., Okada, K., Matsui, Y., Fujimoto, K., Adachi, H., Yamaya, H., et al. (2018). Glomerular mannose-binding lectin deposition in intrinsic antigen-related membranous nephropathy. Nephrol. Dial. Transpl. 33 (5), 832–840. doi:10.1093/ndt/gfx235
He, J. Y., Hong, Q., Chen, B. X., Cui, S. Y., Liu, R., Cai, G. Y., et al. (2022). Ginsenoside Rb1 alleviates diabetic kidney podocyte injury by inhibiting aldose reductase activity. Acta Pharmacol. Sin. 43 (2), 342–353. doi:10.1038/s41401-021-00788-0
Heymann, W., Hackel, D. B., Harwood, S., Wilson, S. G., and Hunter, J. L. (1959). Production of nephrotic syndrome in rats by Freund's adjuvants and rat kidney suspensions. Proc. Soc. Exp. Biol. Med. 100 (4), 660–664. doi:10.3181/00379727-100-24736
Hofstra, J. M., Beck, L. H., Beck, D. M., Wetzels, J. F., and Salant, D. J. (2011). Anti-phospholipase A₂ receptor antibodies correlate with clinical status in idiopathic membranous nephropathy. Clin. J. Am. Soc. Nephrol. 6 (6), 1286–1291. doi:10.2215/cjn.07210810
Hoxha, E., Beck, L. H., Wiech, T., Tomas, N. M., Probst, C., Mindorf, S., et al. (2017). An indirect immunofluorescence method facilitates detection of thrombospondin type 1 domain-containing 7A-specific antibodies in membranous nephropathy. J. Am. Soc. Nephrol. 28 (2), 520–531. doi:10.1681/asn.2016010050
Huang, Y. S., Hsieh, H. Y., Shih, H. M., Sytwu, H. K., and Wu, C. C. (2014). Urinary Xist is a potential biomarker for membranous nephropathy. Biochem. Biophys. Res. Commun. 452 (3), 415–421. doi:10.1016/j.bbrc.2014.08.077
Hunter, J. L., Hackel, D. B., and Heymann, W. (1960). Nephrotic syndrome in rats produced by sensitization to rat kidney proteins: Immunologic studies. J. Immunol. 85, 319–327.
Jiang, H. X., Feng, Z., Zhu, Z. B., Xia, C. H., Zhang, W., Guo, J., et al. (2020). Advances of the experimental models of idiopathic membranous nephropathy (Review). Mol. Med. Rep. 21 (5), 1993–2005. doi:10.3892/mmr.2020.11014
Jiang, S., Chuang, P. Y., Liu, Z. H., and He, J. C. (2013). The primary glomerulonephritides: A systems biology approach. Nat. Rev. Nephrol. 9 (9), 500–512. doi:10.1038/nrneph.2013.129
Jin, L. W., Pan, M., Ye, H. Y., Zheng, Y., Chen, Y., Huang, W. W., et al. (2019). Down-regulation of the long non-coding RNA XIST ameliorates podocyte apoptosis in membranous nephropathy via the miR-217-TLR4 pathway. Exp. Physiol. 104 (2), 220–230. doi:10.1113/ep087190
Jin, Y., Zhang, J., Wang, Y., Xiao, X., and Zhang, Q. (2020). Tripterygium wilfordii multiglycosides combined with prednisone in the treatment of idiopathic membranous nephropathy: A protocol for a systematic review and meta-analysis. Med. Baltim. 99 (5), e18970. doi:10.1097/md.0000000000018970
Jo, H. A., Hyeon, J. S., Yang, S. H., Jung, Y., Ha, H., Jeong, C. W., et al. (2021). Fumarate modulates phospholipase A2 receptor autoimmunity-induced podocyte injury in membranous nephropathy. Kidney Int. 99 (2), 443–455. doi:10.1016/j.kint.2020.06.031
Joshi, A., Rienks, M., Theofilatos, K., and Mayr, M. (2021). Systems biology in cardiovascular disease: A multiomics approach. Nat. Rev. Cardiol. 18 (5), 313–330. doi:10.1038/s41569-020-00477-1
Jurubiță, R., Obrișcă, B., Sorohan, B., Achim, C., Micu, G. E., Mircescu, G., et al. (2021). Clinical phenotypes and predictors of remission in primary membranous nephropathy. J. Clin. Med. 10 (12), 2624. doi:10.3390/jcm10122624
Kaya, B., Paydas, S., Balal, M., Eren Erdogan, K., and Gonlusen, G. (2021). Renal expression of PLA2R, THSD7A, and IgG4 in patients with membranous nephropathy and correlation with clinical findings. Int. J. Clin. Pract. 75 (4), e13855. doi:10.1111/ijcp.13855
Kerjaschki, D., and Farquhar, M. G. (1982). The pathogenic antigen of Heymann nephritis is a membrane glycoprotein of the renal proximal tubule brush border. Proc. Natl. Acad. Sci. U. S. A. 79 (18), 5557–5561. doi:10.1073/pnas.79.18.5557
Kiewisz, J., Skowronska, A., Winiarska, A., Pawlowska, A., Kiezun, J., Rozicka, A., et al. (2019). Wnt4 expression in primary and secondary kidney diseases: Dependence on staging. Kidney Blood Press. Res. 44 (2), 200–210. doi:10.1159/000498989
Koyama, A., Inage, H., Kobayashi, M., Narita, M., and Tojo, S. (1986). Effect of chemical cationization of antigen on glomerular localization of immune complexes in active models of serum sickness nephritis in rabbits. Immunology 58 (4), 529–534.
Kronbichler, A., Kerschbaum, J., and Mayer, G. (2015). The influence and role of microbial factors in autoimmune kidney diseases: A systematic review. J. Immunol. Res. 2015, 858027. doi:10.1155/2015/858027
Kudose, S., Santoriello, D., Debiec, H., Canetta, P. A., Bomback, A. S., Stokes, M. B., et al. (2021). The clinicopathologic spectrum of segmental membranous glomerulopathy. Kidney Int. 99 (1), 247–255. doi:10.1016/j.kint.2020.06.014
Lan, H. Y. (2021). The yin and yang role of transforming growth factor-β in kidney disease. Integr. Med. Nephrol. Androl. 8 (1), 1. doi:10.4103/imna.imna_17_21
Larsen, C. P., Cossey, L. N., and Beck, L. H. (2016). THSD7A staining of membranous glomerulopathy in clinical practice reveals cases with dual autoantibody positivity. Mod. Pathol. 29 (4), 421–426. doi:10.1038/modpathol.2016.32
Larsen, C. P., Trivin-Avillach, C., Coles, P., Collins, A. B., Merchant, M., Ma, H., et al. (2018). LDL receptor-related protein 2 (megalin) as a target antigen in human kidney anti-brush border antibody disease. J. Am. Soc. Nephrol. 29 (2), 644–653. doi:10.1681/asn.2017060664
Lee, H. S. (2011). Pathogenic role of TGF-β in the progression of podocyte diseases. Histol. Histopathol. 26 (1), 107–116. doi:10.14670/hh-26.107
Li, J., Chen, Y., Shen, L., and Deng, Y. (2019). Improvement of membranous nephropathy by inhibition of miR-193a to affect podocytosis via targeting WT1. J. Cell. Biochem. 120 (3), 3438–3446. doi:10.1002/jcb.27616
Li, P., Zhong, X., Zhang, L., Yu, Y., and Niu, J. (2021). Bioinformatic investigation for candidate genes and molecular mechanism in the pathogenesis of membranous nephropathy. Nephrology 26 (3), 262–269. doi:10.1111/nep.13833
Li, S. S., Sun, Q., Hua, M. R., Suo, P., Chen, J. R., Yu, X. Y., et al. (2021). Targeting the Wnt/β-catenin signaling pathway as a potential therapeutic strategy in renal tubulointerstitial fibrosis. Front. Pharmacol. 12, 719880. doi:10.3389/fphar.2021.719880
Liu, J. R., Miao, H., Deng, D. Q., Vaziri, N. D., Li, P., and Zhao, Y. Y. (2021). Gut microbiota-derived tryptophan metabolism mediates renal fibrosis by aryl hydrocarbon receptor signaling activation. Cell. Mol. Life Sci. 78 (3), 909–922. doi:10.1007/s00018-020-03645-1
Liu, X. Q., Jiang, L., Li, Y. Y., Huang, Y. B., Hu, X. R., Zhu, W., et al. (2022). Wogonin protects glomerular podocytes by targeting Bcl-2-mediated autophagy and apoptosis in diabetic kidney disease. Acta Pharmacol. Sin. 43 (1), 96–110. doi:10.1038/s41401-021-00721-5
Liu, Y., Xu, X., Xu, R., and Zhang, S. (2019). Renoprotective effects of isoliquiritin against cationic bovine serum albumin-induced membranous glomerulonephritis in experimental rat model through its anti-oxidative and anti-inflammatory properties. Drug Des. devel. Ther. 13, 3735–3751. doi:10.2147/dddt.s213088
Logt, A. V., Justino, J., Vink, C. H., van den Brand, J., Debiec, H., Lambeau, G., et al. (2021). Anti-PLA2R1 antibodies as prognostic biomarker in membranous nephropathy. Kidney Int. Rep. 6 (6), 1677–1686. doi:10.1016/j.ekir.2021.04.002
Lu, H., Luo, Y., Su, B., Tang, S., Chen, G., Zhang, L., et al. (2020). Wenyang Lishui decoction ameliorates podocyte injury in membranous nephropathy rat and cell models by regulating p53 and Bcl-2. Evid. Based. Complement. Altern. Med. 2020, 6813760. doi:10.1155/2020/6813760
Luo, L. P., Suo, P., Ren, L. L., Liu, H. J., Zhang, Y., and Zhao, Y. Y. (2021). Shenkang injection and its three anthraquinones ameliorates renal fibrosis by simultaneous targeting IƙB/NF-ƙB and Keap1/Nrf2 signaling pathways. Front. Pharmacol. 12, 800522. doi:10.3389/fphar.2021.800522
Lwezaula, B. F., Ameh, O. I., Ekrikpo, U. E., Botha, F. C., Okpechi-Samuel, U. S., Wearne, N., et al. (2021). Diagnostic performance of glomerular PLA2R and THSD7A antibodies in biopsy confirmed primary membranous nephropathy in South Africans. BMC Nephrol. 22 (1), 15. doi:10.1186/s12882-020-02216-7
Malaki, M. (2022). Adenine-rich diet: A potential mechanism for renal fibrosis progression. Explor. Med. 3 (4), 314–316. doi:10.37349/emed.2022.00095
Mantovani, A., and Chiara, Z. (2020). PNPLA3 gene and kidney disease. Explor. Med. 1, 42–50. doi:10.37349/emed.2020.00004
Meng, J., Sai-Zhen, W., He, J. Z., Zhu, S., Huang, B. Y., Wang, S. Y., et al. (2020). Ganoderic acid A is the effective ingredient of Ganoderma triterpenes in retarding renal cyst development in polycystic kidney disease. Acta Pharmacol. Sin. 41 (6), 782–790. doi:10.1038/s41401-019-0329-2
Meyer-Schwesinger, C., Dehde, S., Klug, P., Becker, J. U., Mathey, S., Arefi, K., et al. (2011). Nephrotic syndrome and subepithelial deposits in a mouse model of immune-mediated anti-podocyte glomerulonephritis. J. Immunol. 187 (6), 3218–3229. doi:10.4049/jimmunol.1003451
Meyer-Schwesinger, C., Tomas, N. M., Dehde, S., Seifert, L., Hermans-Borgmeyer, I., Wiech, T., et al. (2020). A novel mouse model of phospholipase A2 receptor 1-associated membranous nephropathy mimics podocyte injury in patients. Kidney Int. 97 (5), 913–919. doi:10.1016/j.kint.2019.10.022
Miao, H., Cao, G., Wu, X. Q., Chen, Y. Y., Chen, D. Q., Chen, L., et al. (2020). Identification of endogenous 1-aminopyrene as a novel mediator of progressive chronic kidney disease via aryl hydrocarbon receptor activation. Br. J. Pharmacol. 177 (15), 3415–3435. doi:10.1111/bph.15062
Miao, H., Wu, X. Q., Wang, Y. N., Chen, D. Q., Chen, L., Vaziri, N. D., et al. (2022). 1-Hydroxypyrene mediates renal fibrosis through aryl hydrocarbon receptor signalling pathway. Br. J. Pharmacol. 179 (1), 103–124. doi:10.1111/bph.15705
Murtas, C., Bruschi, M., Candiano, G., Moroni, G., Magistroni, R., Magnano, A., et al. (2012). Coexistence of different circulating anti-podocyte antibodies in membranous nephropathy. Clin. J. Am. Soc. Nephrol. 7 (9), 1394–1400. doi:10.2215/cjn.02170312
Murtas, C., and Ghiggeri, G. M. (2016). Membranous glomerulonephritis: Histological and serological features to differentiate cancer-related and non-related forms. J. Nephrol. 29 (4), 469–478. doi:10.1007/s40620-016-0268-7
Natori, Y., Shindo, N., and Natori, Y. (1994). Proteinuria induced by anti-dipeptidyl peptidase IV (gp108); role of circulating and glomerular antigen. Clin. Exp. Immunol. 95 (2), 327–332. doi:10.1111/j.1365-2249.1994.tb06532.x
Ngai, H. H., Sit, W. H., Jiang, P. P., Xu, R. J., Wan, J. M., and Thongboonkerd, V. (2006). Serial changes in urinary proteome profile of membranous nephropathy: Implications for pathophysiology and biomarker discovery. J. Proteome Res. 5 (11), 3038–3047. doi:10.1021/pr060122b
Nieto-Gañán, I., Rita, C. G., Villacorta-Pérez, J., Ortego-Pérez, S., Rodríguez-Mendiola, N., and Carrasco-Sayalero, Á. (2021). Comparison of 3 anti-PLA2R inmmunoassaysfor the diagnosis of idiopathic membranous nephropathy in an European population. A pilot study. Clin. Immunol. 227, 108729. doi:10.1016/j.clim.2021.108729
Oto, O. A., Demir, E., Mirioglu, S., Dirim, A. B., Ozluk, Y., Cebeci, E., et al. (2021). Clinical significance of glomerular C3 deposition in primary membranous nephropathy. J. Nephrol. 34 (2), 581–587. doi:10.1007/s40620-020-00915-w
Pang, L., Li, Q., Li, Y., Liu, Y., Duan, N., and Li, H. (2018). Urine proteomics of primary membranous nephropathy using nanoscale liquid chromatography tandem mass spectrometry analysis. Clin. Proteomics 15, 5. doi:10.1186/s12014-018-9183-3
Prunotto, M., Carnevali, M. L., Candiano, G., Murtas, C., Bruschi, M., Corradini, E., et al. (2010). Autoimmunity in membranous nephropathy targets aldose reductase and SOD2. J. Am. Soc. Nephrol. 21 (3), 507–519. doi:10.1681/asn.2008121259
Radice, A., Trezzi, B., Maggiore, U., Pregnolato, F., Stellato, T., Napodano, P., et al. (2016). Clinical usefulness of autoantibodies to M-type phospholipase A2 receptor (PLA2R) for monitoring disease activity in idiopathic membranous nephropathy (IMN). Autoimmun. Rev. 15 (2), 146–154. doi:10.1016/j.autrev.2015.10.004
Rani, A., Ranjan, R., McGee, H. S., Andropolis, K. E., Panchal, D. V., Hajjiri, Z., et al. (2017). Urinary microbiome of kidney transplant patients reveals dysbiosis with potential for antibiotic resistance. Transl. Res. 181, 59–70. doi:10.1016/j.trsl.2016.08.008
Rashid, I., Katravath, P., Tiwari, P., D'Cruz, S., Jaswal, S., and Sahu, G. (2022). Hyperuricemia-a serious complication among patients with chronic kidney disease: A systematic review and meta-analysis. Explor. Med. 3 (3), 249–259. doi:10.37349/emed.2022.00089
Ravindran, A., Casal Moura, M., Fervenza, F. C., Nasr, S. H., Alexander, M. P., Fidler, M. E., et al. (2021). In patients with membranous lupus nephritis, exostosin-positivity and exostosin-negativity represent two different phenotypes. J. Am. Soc. Nephrol. 32 (3), 695–706. doi:10.1681/asn.2020081181
Ravindran, A., Madden, B., Charlesworth, M. C., Sharma, R., Sethi, A., Debiec, H., et al. (2020). Proteomic analysis of complement proteins in membranous nephropathy. Kidney Int. Rep. 5 (5), 618–626. doi:10.1016/j.ekir.2020.01.018
Reinhard, L., Thomas, C., Machalitza, M., Lattwein, E., Weiss, L. S., Vitu, J., et al. (2021). Characterization of THSD7A-antibodies not binding to glomerular THSD7A in a patient with diabetes mellitus but no membranous nephropathy. Sci. Rep. 11 (1), 16188. doi:10.1038/s41598-021-94921-y
Ronco, P., Beck, L., Debiec, H., Fervenza, F. C., Hou, F. F., Jha, V., et al. (2021). Membranous nephropathy. Nat. Rev. Dis. Prim. 7 (1), 69. doi:10.1038/s41572-021-00303-z
Ronco, P., and Debiec, H. (2017). New antigenic epitopes in membranous nephropathy. Bull. l'Academie. Nat. Med. 201 (1-3), 81–94. doi:10.1016/s0001-4079(19)30489-3
Ronco, P., Plaisier, E., and Debiec, H. (2021). Advances in membranous nephropathy. J. Clin. Med. 10 (4), 607. doi:10.3390/jcm10040607
Ruggenenti, P., Debiec, H., Ruggiero, B., Chianca, A., Pellé, T., Gaspari, F., et al. (2015). Anti-phospholipase A2 receptor antibody titer predicts post-rituximab outcome of membranous nephropathy. J. Am. Soc. Nephrol. 26 (10), 2545–2558. doi:10.1681/asn.2014070640
Salvadori, M., and Tsalouchos, A. (2022). New antigens involved in membranous nephropathy beyond phospholipase A2 receptor. World J. Nephrol. 11 (4), 115–126. doi:10.5527/wjn.v11.i4.115
Santoro, D., Vadalà, C., Siligato, R., Buemi, M., and Benvenga, S. (2017). Autoimmune thyroiditis and glomerulopathies. Front. Endocrinol. 8, 119. doi:10.3389/fendo.2017.00119
Seitz-Polski, B., Dolla, G., Payré, C., Girard, C. A., Polidori, J., Zorzi, K., et al. (2016). Epitope spreading of autoantibody response to PLA2R associates with poor prognosis in membranous nephropathy. J. Am. Soc. Nephrol. 27 (5), 1517–1533. doi:10.1681/asn.2014111061
Sethi, S., Debiec, H., Madden, B., Charlesworth, M. C., Morelle, J., Gross, L., et al. (2020). Neural epidermal growth factor-like 1 protein (NELL-1) associated membranous nephropathy. Kidney Int. 97 (1), 163–174. doi:10.1016/j.kint.2019.09.014
Sethi, S., Debiec, H., Madden, B., Vivarelli, M., Charlesworth, M. C., Ravindran, A., et al. (2020). Semaphorin 3B-associated membranous nephropathy is a distinct type of disease predominantly present in pediatric patients. Kidney Int. 98 (5), 1253–1264. doi:10.1016/j.kint.2020.05.030
Sethi, S., Madden, B., Debiec, H., Morelle, J., Charlesworth, M. C., Gross, L., et al. (2021). Protocadherin 7-associated membranous nephropathy. J. Am. Soc. Nephrol. 32 (5), 1249–1261. doi:10.1681/asn.2020081165
Sethi, S., Madden, B. J., Debiec, H., Charlesworth, M. C., Gross, L., Ravindran, A., et al. (2019). Exostosin 1/exostosin 2-associated membranous nephropathy. J. Am. Soc. Nephrol. 30 (6), 1123–1136. doi:10.1681/asn.2018080852
Sethi, S. (2021). New 'antigens' in membranous nephropathy. J. Am. Soc. Nephrol. 32 (2), 268–278. doi:10.1681/asn.2020071082
Sha, W. G., Shen, L., Zhou, L., Xu, D. Y., and Lu, G. Y. (2015). Down-regulation of miR-186 contributes to podocytes apoptosis in membranous nephropathy. Biomed. Pharmacother. 75, 179–184. doi:10.1016/j.biopha.2015.07.021
Shi, B., Zhang, R. R., Liang, Y., Wang, X. H., Lang, R., and Yu, R. H. (2018). Efficacy of traditional Chinese medicine regimen jian pi qu shi formula for refractory patients with idiopathic membranous nephropathy: A retrospective case-series study. Evid. Based. Complement. Altern. Med. 2018, 5854710. doi:10.1155/2018/5854710
Shi, H., Hou, Y., Su, X., Qiao, J., Wang, Q., Guo, X., et al. (2022). Mechanism of action of Tripterygium wilfordii for treatment of idiopathic membranous nephropathy based on network pharmacology. Ren. Fail. 44 (1), 116–125. doi:10.1080/0886022x.2021.2024850
Sinico, R. A., Mezzina, N., Trezzi, B., Ghiggeri, G. M., and Radice, A. (2016). Immunology of membranous nephropathy: From animal models to humans. Clin. Exp. Immunol. 183 (2), 157–165. doi:10.1111/cei.12729
Sui, W. G., He, H. Y., Yan, Q., Chen, J. J., Zhang, R. H., and Dai, Y. (2014). ChIP-seq analysis of histone H3K9 trimethylation in peripheral blood mononuclear cells of membranous nephropathy patients. Braz J. Med. Biol. Res. 47 (1), 42–49. doi:10.1590/1414-431x20132809
Sui, W., Zhang, R., Chen, J., He, H., Cui, Z., Ou, M., et al. (2015). Comparative proteomic analysis of membranous nephropathy biopsy tissues using quantitative proteomics. Exp. Ther. Med. 9 (3), 805–810. doi:10.3892/etm.2015.2197
Sun, P., Feng, S., Guan, Q., Adomat, H., Barbour, S., Gleave, M. E., et al. (2020). Clusterin deficiency predisposes C57BL/6j mice to cationic bovine serum albumin-induced glomerular inflammation. J. Inflamm. Res. 13, 969–983. doi:10.2147/jir.s285985
Sun, Z., Xu, Q., Ma, Y., Yang, S., and Shi, J. (2021). Circ_0000524/miR-500a-5p/CXCL16 axis promotes podocyte apoptosis in membranous nephropathy. Eur. J. Clin. Invest. 51 (3), e13414. doi:10.1111/eci.13414
Sutariya, B., Jhonsa, D., and Saraf, M. N. (2016). TGF-β: The connecting link between nephropathy and fibrosis. Immunopharmacol. Immunotoxicol. 38 (1), 39–49. doi:10.3109/08923973.2015.1127382
Taherkhani, A., Farrokhi Yekta, R., Mohseni, M., Saidijam, M., and Arefi Oskouie, A. (2019). Chronic kidney disease: A review of proteomic and metabolomic approaches to membranous glomerulonephritis, focal segmental glomerulosclerosis, and IgA nephropathy biomarkers. Proteome Sci. 17, 7. doi:10.1186/s12953-019-0155-y
Taherkhani, A., Kalantari, S., Arefi Oskouie, A., Nafar, M., Taghizadeh, M., and Tabar, K. (2018). Network analysis of membranous glomerulonephritis based on metabolomics data. Mol. Med. Rep. 18 (5), 4197–4212. doi:10.3892/mmr.2018.9477
Taherkhani, A., Nafar, M., Arefi-Oskouie, A., Broumandnia, N., Parvin, M., Mahmoudieh, L., et al. (2019). Metabolomic analysis of membranous glomerulonephritis: Identification of a diagnostic panel and pathogenic pathways. Arch. Med. Res. 50 (4), 159–169. doi:10.1016/j.arcmed.2019.08.004
Tian, R., Wang, L., Chen, A., Huang, L., Liang, X., Wang, R., et al. (2019). Sanqi oral solution ameliorates renal damage and restores podocyte injury in experimental membranous nephropathy via suppression of NFκB. Biomed. Pharmacother. 115, 108904. doi:10.1016/j.biopha.2019.108904
Tomas, N. M., Hoxha, E., Reinicke, A. T., Fester, L., Helmchen, U., Gerth, J., et al. (2016). Autoantibodies against thrombospondin type 1 domain-containing 7A induce membranous nephropathy. J. Clin. Invest. 126 (7), 2519–2532. doi:10.1172/jci85265
Tomas, N. M., Huber, T. B., and Hoxha, E. (2021). Perspectives in membranous nephropathy. Cell Tissue Res. 385 (2), 405–422. doi:10.1007/s00441-021-03429-4
Tomas, N. M., Meyer-Schwesinger, C., von Spiegel, H., Kotb, A. M., Zahner, G., Hoxha, E., et al. (2017). A heterologous model of thrombospondin type 1 domain-containing 7A-associated membranous nephropathy. J. Am. Soc. Nephrol. 28 (11), 3262–3277. doi:10.1681/asn.2017010030
Tsai, S. F., Wu, M. J., and Chen, C. H. (2019). Low serum C3 level, high neutrophil-lymphocyte-ratio, and high platelet-lymphocyte-ratio all predicted poor long-term renal survivals in biopsy-confirmed idiopathic membranous nephropathy. Sci. Rep. 9 (1), 6209. doi:10.1038/s41598-019-42689-7
Tsay, T. B., Chen, P. H., and Chen, L. W. (2019). Aryl hydrocarbon receptor ligands enhance lung immunity through intestinal IKKβ pathways. J. Transl. Med. 17 (1), 304. doi:10.1186/s12967-019-2043-8
Wang, G., Sun, L., Dong, H., Wang, Y., Xu, X., Zhao, Z., et al. (2021). Neural epidermal growth factor-like 1 protein-positive membranous nephropathy in Chinese patients. Clin. J. Am. Soc. Nephrol. 16 (5), 727–735. doi:10.2215/cjn.11860720
Wang, M., Chen, D. Q., Chen, L., Cao, G., Zhao, H., Liu, D., et al. (2018). Novel inhibitors of the cellular renin-angiotensin system components, poricoic acids, target Smad3 phosphorylation and Wnt/β-catenin pathway against renal fibrosis. Br. J. Pharmacol. 175 (13), 2689–2708. doi:10.1111/bph.14333
Wang, W., Sheng, L., Chen, Y., Li, Z., Wu, H., Ma, J., et al. (2022). Total coumarin derivates from Hydrangea paniculata attenuate renal injuries in cationized-BSA induced membranous nephropathy by inhibiting complement activation and interleukin 10-mediated interstitial fibrosis. Phytomedicine. 96, 153886. doi:10.1016/j.phymed.2021.153886
Wang, Y. N., Feng, H. Y., Nie, X., Zhang, Y. M., Zou, L., Li, X., et al. (2022). Recent advances in clinical diagnosis and pharmacotherapy options of membranous nephropathy. Front. Pharmacol. 13, 907108. doi:10.3389/fphar.2022.907108
Wang, Y. N., Yang, C. E., Zhang, D. D., Chen, Y. Y., Yu, X. Y., Zhao, Y. Y., et al. (2021). Long non-coding RNAs: A double-edged sword in aging kidney and renal disease. Chem. Biol. Interact. 337, 109396. doi:10.1016/j.cbi.2021.109396
Wu, L., Lai, J., Ling, Y., Weng, Y., Zhou, S., Wu, S., et al. (2021). A review of the current practice of diagnosis and treatment of idiopathic membranous nephropathy in China. Med. Sci. Monit. 27, e930097. doi:10.12659/msm.930097
Wu, L., Zhang, X., Luo, L., Li, X., Liu, Y., and Qin, X. (2021). Altered expression of serum miR-106a, miR-19b, miR-17, and PTEN in patients with idiopathic membranous nephropathy. J. Clin. Lab. Anal. 35 (4), e23737. doi:10.1002/jcla.23737
Xu, Z., Chen, L., Xiang, H., Zhang, C., and Xiong, J. (2020). Advances in pathogenesis of idiopathic membranous nephropathy. Kidney Dis. (Basel). 6 (5), 330–345. doi:10.1159/000507704
Yang, L., Chen, X., Li, C., Xu, P., Mao, W., Liang, X., et al. (2021). Real-world effects of Chinese herbal medicine for idiopathic membranous nephropathy (REACH-MN): Protocol of a registry-based cohort study. Front. Pharmacol. 12, 760482. doi:10.3389/fphar.2021.760482
Yang, X. (2020). Multitissue multiomics systems biology to dissect complex diseases. Trends Mol. Med. 26 (8), 718–728. doi:10.1016/j.molmed.2020.04.006
Yu, H., Han, M., Lin, W., Wang, L., Liu, P., Yang, K., et al. (2020). Efficacy of Chinese herbal injections for the treatment of primary nephrotic syndrome: A bayesian network meta-analysis of randomized controlled trials. Front. Pharmacol. 11, 579241. doi:10.3389/fphar.2020.579241
Yu, W., Shang, J., Guo, R., Zhang, F., Zhang, W., Zhang, Y., et al. (2020). The gut microbiome in differential diagnosis of diabetic kidney disease and membranous nephropathy. Ren. Fail. 42 (1), 1100–1110. doi:10.1080/0886022x.2020.1837869
Yu, X. Y., Sun, Q., Zhang, Y. M., Zou, L., and Zhao, Y. Y. (2022). TGF-β/Smad signaling pathway in tubulointerstitial fibrosis. Front. Pharmacol. 13, 860588. doi:10.3389/fphar.2022.860588
Zaghrini, C., Seitz-Polski, B., Justino, J., Dolla, G., Payré, C., Jourde-Chiche, N., et al. (2019). Novel ELISA for thrombospondin type 1 domain-containing 7A autoantibodies in membranous nephropathy. Kidney Int. 95 (3), 666–679. doi:10.1016/j.kint.2018.10.024
Zhang, C., Zhang, M., Chen, D., Ren, Q., Xu, W., Zeng, C., et al. (2019). Features of phospholipase A2 receptor and thrombospondin type-1 domain-containing 7A in malignancy-associated membranous nephropathy. J. Clin. Pathol. 72 (10), 705–711. doi:10.1136/jclinpath-2019-205852
Zhang, J., Luo, D., Lin, Z., Zhou, W., Rao, J., Li, Y., et al. (2020). Dysbiosis of gut microbiota in adult idiopathic membranous nephropathy with nephrotic syndrome. Microb. Pathog. 147, 104359. doi:10.1016/j.micpath.2020.104359
Zhang, P., Huang, W., Zheng, Q., Tang, J., Dong, Z., Jiang, Y., et al. (2021). A novel insight into the role of PLA2R and THSD7A in membranous nephropathy. J. Immunol. Res. 2021, 8163298. doi:10.1155/2021/8163298
Zhang, W., Ren, Y., and Li, J. (2019). Application of miR-193a/WT1/PODXL axis to estimate risk and prognosis of idiopathic membranous nephropathy. Ren. Fail. 41 (1), 704–717. doi:10.1080/0886022x.2019.1642210
Zhao, H., Ma, S. X., Shang, Y. Q., Zhang, H. Q., and Su, W. (2019). microRNAs in chronic kidney disease. Clin. Chim. Acta. 491, 59–65. doi:10.1016/j.cca.2019.01.008
Zhao, Y. Y., and Lin, R. C. (2014). UPLC-MSE application in disease biomarker discovery: The discoveries in proteomics to metabolomics. Chem. Biol. Interact. 215, 7–16. doi:10.1016/j.cbi.2014.02.014
Zhao, Y. Y. (2013). Metabolomics in chronic kidney disease. Clin. Chim. Acta. 422, 59–69. doi:10.1016/j.cca.2013.03.033
Zhao, Y. Y. (2022). Recent advances of gut microbiota in chronic kidney disease patients. Explor. Med. 3 (3), 260–274. doi:10.37349/emed.2022.00090
Zheng, R., Deng, Y., Chen, Y., Fan, J., Zhang, M., Zhong, Y., et al. (2012). Astragaloside IV attenuates complement membranous attack complex induced podocyte injury through the MAPK pathway. Phytother. Res. 26 (6), 892–898. doi:10.1002/ptr.3656
Zhou, G., Zeng, J., Peng, L., Wang, L., Zheng, W., Di, W., et al. (2021). Fecal microbiota transplantation for membranous nephropathy. Cen. Case Rep. 10 (2), 261–264. doi:10.1007/s13730-020-00560-z
Zhou, G., Zhang, X., Wang, W., Zhang, W., Wang, H., and Xin, G. (2019). Both peripheral blood and urinary miR-195-5p, miR-192-3p, miR-328-5p and their target genes PPM1A, RAB1A and BRSK1 may be potential biomarkers for membranous nephropathy. Med. Sci. Monit. 25, 1903–1916. doi:10.12659/msm.913057
Keywords: membranous nephropathy, anti-PLA 2 R antibody, gut microbiota, long non-coding RNAs, metabolite biomarkers, traditional Chinese medicine
Citation: Miao H, Zhang Y, Yu X, Zou L and Zhao Y (2022) Membranous nephropathy: Systems biology-based novel mechanism and traditional Chinese medicine therapy. Front. Pharmacol. 13:969930. doi: 10.3389/fphar.2022.969930
Received: 15 June 2022; Accepted: 10 August 2022;
Published: 13 September 2022.
Edited by:
Jianping Chen, Shenzhen Traditional Chinese Medicine Hospital, ChinaReviewed by:
Corrado Murtas, Azienda Sanitaria Locale di Viterbo, ItalyHong Liu, Central South University, China
Liqiang Meng, Peking University, China
Copyright © 2022 Miao, Zhang, Yu, Zou and Zhao. This is an open-access article distributed under the terms of the Creative Commons Attribution License (CC BY). The use, distribution or reproduction in other forums is permitted, provided the original author(s) and the copyright owner(s) are credited and that the original publication in this journal is cited, in accordance with accepted academic practice. No use, distribution or reproduction is permitted which does not comply with these terms.
*Correspondence: Xiaoyong Yu, Z3ViNzA3MjVAMTI2LmNvbQ==; Liang Zou, em91bGlhbmdjZHVAMTI2LmNvbQ==; Yingyong Zhao, emhhb3l5YnJAZ21haWwuY29t