- 1College of Life Sciences and Medicine, Zhejiang Sci-Tech University, Hangzhou, China
- 2School of Environmental Science and Engineering, Zhejiang Gongshang University, Hangzhou, China
- 3Zhejiang Provincial Key Laboratory of Solid Waste Treatment and Recycling, Hangzhou, China
Puerarin is a flavonoid molecule that widely exists in various plants. Puerarin has been reported to exhibit anti-tumor effects in various cancers. However, its exact underlying pharmacological mechanism is unclear. This study evaluated the anticancer effect of puerarin combined with oxaliplatin (OXA) in vitro and in vivo. Our results indicated that puerarin can reverse platinum-based anti-cancer drug resistance, and enhance the OXA’s anticancer effects on breast cancer. Furthermore, puerarin can inhibit migration and reverse the epithelial-mesenchymal transition (EMT) induced by low-dose OXA. Further studies showed that the carbonic anhydrase (CA) XII is a potential target of puerarin. In conclusion, puerarin is expected to become an adjuvant chemotherapy drug and potentially become one of the medicated foods for breast cancer patients.
Introduction
Chemotherapy is used to treat cancer, prolong the life of cancer patients, and even cure cancer, however, sometimes it can stimulate cancer cells and cause metastasis (Wills et al., 2021; Dai et al., 2022). Therefore, continuous efforts have been made to find and develop new strategies for cancer therapy with lower side effects and better efficacy. For this, various researchers pay great attention to compounds from natural plants and their derivatives, which can be potential in the treatment of cancers (Chen et al., 2009; Shirode et al., 2015). These compounds are found in many diets and can be good options for adjuvant cancer treatment.
Flavonoids are a type of natural small molecules with anti-cancer, anti-inflammatory and antioxidant effects (Maleki et al., 2019; Bisol et al., 2020; Kopustinskiene et al., 2020; Liu et al., 2022). Puerarin is a typical flavonoid molecule and active ingredient extracted from leguminous plants of the genus Pueraria, an important medicinal plant known for its health and beauty benefits. In 1993, puerarin was approved for clinical use and was widely used in treating cardiovascular diseases (Ahmad et al., 2020). In addition, puerarin has two benzene rings (A ring and B ring) linked to each other through the central three carbon structure, and many evidences have proved that this structure has the ability to down-regulate mutant p53 protein, block cell cycle and inhibit Ras protein expression and anti-cancer properties (Lamson and Brignall, 2000; Bisol et al., 2020). Various studies demonstrated that puerarin plays an anti-cancer role in several trials (Wang et al., 2013; Kang et al., 2017; Liu et al., 2017). Puerarin inhibits lymphatic carcinoma cell proliferation and reduces the levels of matrix metalloproteinase through reactive oxygen oxidative stress. In addition, through endogenous and exogenous mitochondrial pathways, puerarin induces tumor cells apoptosis (Chen et al., 2016; Hu et al., 2018). Puerarin selectively reduces tumor cells’ proliferative capacity and extensively inhibits cancer cells signaling pathway transduction (Liu et al., 2017). Liu et al. showed that lipopolysaccharide (LPS) treatment increases the capacity to metastasize of breast cancer cells, while puerarin reduces the metastasis and invasion of LPS-induced breast cancer cells (Liu et al., 2017), suggesting that puerarin can potentially be used for anti-breast cancer.
Epithelial-mesenchymal transition (EMT) refers to how epithelial cells are depolarized and transformed into mesenchymal cells due to certain factors (Jiang et al., 2022). During the metastasis in epithelial tumors, the phenotype of tumor cells changes primarily caused by environmental stimuli that enable tumor cells to adapt to the various microenvironments they encounter (intercellular stroma, humoral components, or blood) (Park et al., 2020; Qiao et al., 2021). EMT regulates these phenotypic transformations. Therefore, to some extent, EMT promotes tumor metastasis (Pastushenko and Blanpain, 2019). Meanwhile, it has been reported that low-concentration chemotherapy drugs not only significantly inhibit tumor proliferation, but also induce EMT of tumor cells, thus promoting tumor metastasis (Middleton et al., 2018).
Therefore, this study aims to detect whether puerarin can enhance the effect of oxaliplatin (OXA), the third generation of platinum chemotherapy drugs, on breast cancer and inhibit metastasis of breast cancer cells.
Materials and methods
Chemicals and cell culture
Puerarin was purchased from Meilunbio (Dalian, China). E-cadherin antibody (ab40772) and vimentin antibody (BF8006) were purchased from Abcam and Affinity, respectively. Apoptosis Detection Kit was purchased from Beyotime (Shanghai, China). Crystal violet was purchased from Sigma-Aldrich Fluka (America). OXA was purchased from Meilunbio (Dalian, China), LTD. Cisplatin (DDP) was purchased from Sigma-Aldrich.
The MCF-7 (human breast cancer cell lines) and MCF-7/DDP (DDP-resistant cell lines), were from KeyGEN BioTECH (Nanjing, China). These cells were grown in DMEM containing penicillin, streptavidin, and 10% bovine serum at 5% CO2, and 37°C.
Cell viability assay
The drug tolerance effects of puerarin were detected using the 3-(4,5-dimethyl-2-thiazolyl)-2,5-diphenyl-2-H-tetrazolium bromide (MTT) assay on MCF-7, MCF-7/DDP. The cells (5,000 cells/well) were added to 96-well plates cultured overnight. All assays were repeated three times. In each flask, MTT solution was added after 48 h of drug treatment. Incubation in dimethyl sulfoxide (DMSO) for 4 h dissolves the formazan crystals. OD590 value was detected with a microplate reader, and detected a 50% inhibitory concentration (IC50) value. The synergistic effect was calculated using CompuSyn software.
Rh123 efflux assay
Cells (1 × 106) were cultured for 24 h in six-well plates. A variety of levels of puerarin were used for the pretreatment of MCF-7/DDP cells for 24 h [0 μM, 20 μM (L), 40 μM (H)]. After the pretreatment, cells were incubated with Rh123 (5 mg/mL) in a dark room. Then, Rh123-free medium was used to replace the above medium, and drained the remaining efflux intervals every hour. After incubation, PBS was used to wash cells twice. Then, 400 μL lysis buffer was used for lysing cells, and PBS with 10% FBS was used to maintain the cells. The flow cytometry was used to determine the green fluorescence of Rh123.
Wound-healing assay
A wound healing assay was performed to assess changes in cell motility and migration. Cells were grown to confluency at 5 × 105 cells per well in 48-well plates. Scratch the cell monolayer with an apipette tip and then rinse using phosphate buffer saline (PBS). After the treatment [Control, OXA (5 μM), puerarin (40 μM) + OXA (5 μM)], a Nikon microscope was used to take images 0, 24, and 48 h.
Transwell assays
The transwell assay is used to evaluate cell invasiveness. Three different concentrations of medium [Control, OXA (5 μM), puerarin (40 μM) + OXA (5 μM)] were used for the suspension of the cells below. The cells were then inoculated into an 8 μm polyethylene terephthalate filter membrane coated with matrix gel. In the lower chamber, about 500 mL of medium was placed. The cells were fixed for 30 min with paraformaldehyde (4%) and stained for 20 min with crystal violet (0.1%). A hundred-fold magnification inverted microscope was used to image the invaded cells, and the cell numbers were manually counted.
Immunofluorescence assays
Cells were treated [Control, OXA (5 μM), puerarin (40 μM) + OXA (5 μM)] and were cultured overnight. The cells were treated with methanol and Triton X-100. E-cadherin and vimentin (both 1:100) were used in an immunofluorescence experiment. After washing thrice for 20 min, Incubation of the cells with a secondary antibody (1:200) for 30 min. Following 4,6-diamino-2-phenyl indole (DAPI) staining for 10 min, and observed under a laser scanning confocal microscope.
Apoptosis assays
A 96-well plate was used to culture the cells. After drugs treatment, the culture solution was discarded. Then, Annexin V-FITC binding solution, Annexin V-FITC and propyl iodide solution were successively added and gently mixed all the solutions. In a dark room, plates were incubated for 20 min. The red and green fluorescence was detected by a fluorescence microscope.
Animal studies
In this section, BALB/c nude mice (5 weeks) were used. All mice were raised in sterile conditions. All procedures were approved according to the guidelines of the Animal Ethics Committee of the Zhejiang Sci-tech University. The MCF-7 cells were orthotopically implanted into the mice. Cells were grown until the logarithmic growth stage, centrifuged with PBS. 50% Matrigel mixture was resuspended in PBS, resulting in 2 × 107 cells/mL. The right flank of each mouse was injected 0.2 mL cell suspension. After 14 d of tumor transplantation, four groups of mice were divided: control group (saline given orally once daily), puerarin treated group (50 mg/kg), OXA treated group (5 mg/kg), and puerarin and OXA treated group (50 mg/kg + 5 mg/kg). After the tumor was transplanted, daily measurements were taken of its volume and weight. All mice were euthanized after 3 weeks. Tumors were resected and volumes were measured. V = ab2/2 (a = length, b = width) was used to calculate tumor volumes. To measure their survival rates, another 40 mice were distributed into 4 groups (10 per group). The survival time of each mouse was monitored.
RNA sequencing data collection and procession
The RNA sequencing data for cancer tissues and adjacent tissues from patients with breast cancer were obtained from the Gene Expression Omnibus (GEO) database. The differentially expressed gene (DEG) was considered by four sample data, involving GSM2286198, GSM2286199, GSM2286316, and GSM2286317 with two drug treatment samples and two control samples.
Analysis of differentially expressed genes
In the R computing environment, with the Limma package, the corresponding fold change and p value for DEGs between different groups were compared using a volcano plot. Up- and down-regulated genes had p ≤ 0.05, a fold change of more than 2.0, defined as log2 (fold change) > 1 or < - 1 for up- and down-regulated genes, respectively. For gene function enrichment analysis, gene ontology (GO) annotations of genes in R software were used. A maximum gene of 5,000 and a minimum gene of 5 was set (p < 0.05 and FDR <0.25).
Target prediction and molecular docking
To predict puerarin’s targets, the simplified molecular input line entry system (SMILES) format of puerarin from PubChem and the similarity ensemble approach (SEA) website were used (Keiser et al., 2007). Molecular docking was performed with puerarin and carbonic anhydrase (CA) XII. An analysis of the complex between the ligand and protein was carried out by Pymol using the PDB format.
CA XII activity analysis
The CA XII activity in breast cancer cells was determined by extracellular pH analysis. Cells were treated with [20 μM (L) puerarin +500 nM U-104] and [40 μM (H) puerarin +200 nM U-104] for 3 h. The Wilbur-Anderson method was used to calculate CA XII activity (WAU/mg = 2× (T0-T)/T*mg protein). To determine how long it will take to reduce the pH of an isotonic buffer from 8.00 to 6.60, using time (T) (T: catalyzed reaction and T0: unanalyzed reaction).
Data statistics
Data are analyzed as mean ± standard deviation (SD). The independent variance t-test were used to assess the differences between the two groups. The multiple comparisons test were compared using a one-way analysis of variance, followed by the least significance difference (LSD) post-hoc test (SPSS 23.0).
Results
Puerarin enhanced the effects of platinum-based anti-cancer drug and reversed drug-resistance
Figure 1A shows the structural formulas of puerarin. In order to detect the effect of puerarin in reversing drug resistance, OD590 was measured. In our results, drug resistance to DDP and OXA can be reversed by puerarin in MCF-7/DDP cells. In addition, there is a dose-effect relationship in the reverse effect of puerarin on MCF-7/DDP cells (Figure 1B). A study was carried out to evaluate the efflux of Rh123 from MCF-7/DDP cells. Figure 1C indicates that as compared to the other groups, the puerarin groups had a higher fluorescence intensity.
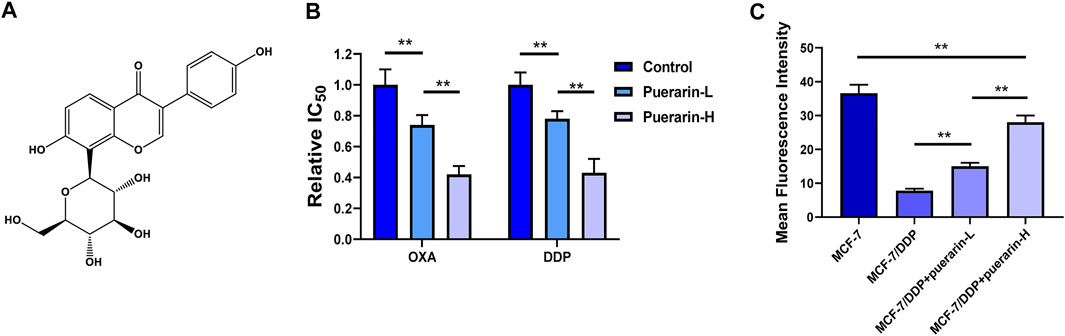
FIGURE 1. Puerarin reversed drug resistance of platinum-based anti-cancer drug. (A) The structural formulas of puerarin (B) The IC50 values of OXA and DDP on MCF-7 were detected. The cancer cells were treated with different concentrations of puerarin (Control: DMSO, puerarin-L: 20 μM, puerarin-H: 40 μM). (C) The fluorescence intensity of Rh123 in MCF-7/DDP was lower than the fluorescence intensity in MCF-7. Puerarin treatment reversed the change in a dose-dependent manner (*p < 0.05, **p < 0.01).
There is a synergistic effect of puerarin and OXA on tumor inhibition
In order to detect the synergistic effects of puerarin and OXA, the MTT assay was performed. As shown in Figures 2A,B, puerarin improves the inhibitory effect of OXA and DDP on cell proliferation. Furthermore, combining puerarin with platinum-based drugs resulted in a combination index (CI) value less than 1, thus indicating synergistic effects. The effect of co-treatment on MCF-7/DDP xenografts in nude mice BALB/c was evaluated. Mice in the combination treatment group gained weight compared to those in the OXA-treated group. Compared to the other groups, the tumor weight of co-treatment group is lowest (Figures 2C–E).
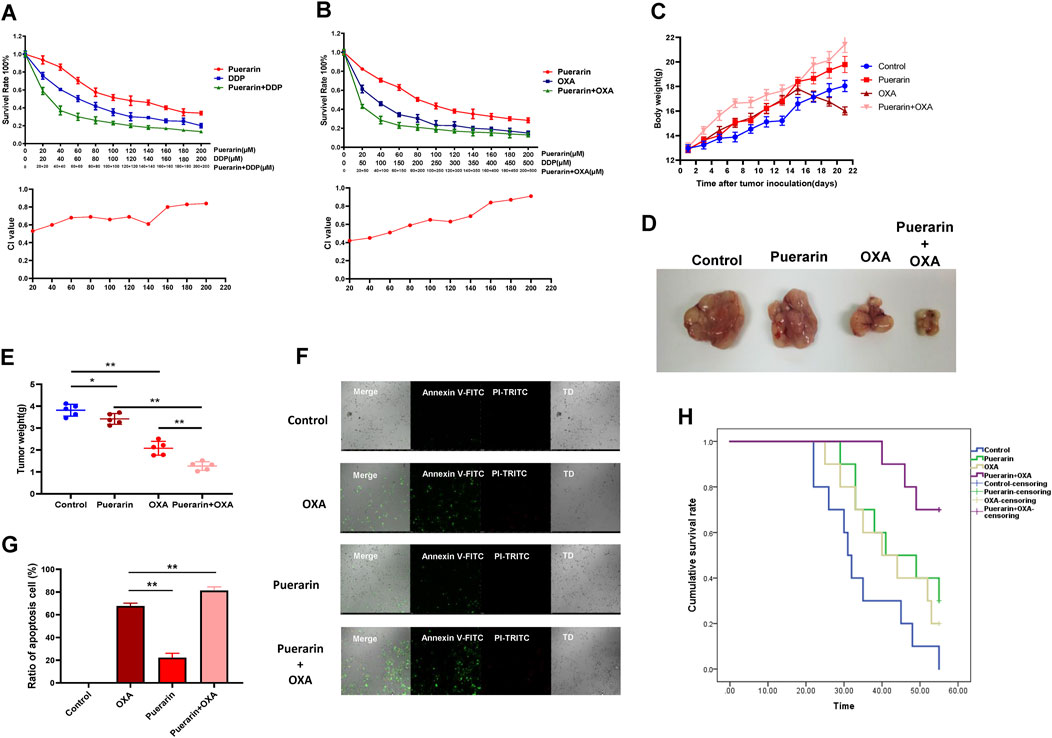
FIGURE 2. Puerarin enhanced the effects of OXA. (A,B) MTT assay was operated to detect the inhibition on MCF-7 cells. Puerarin enhances the inhibitory effect of OXA and DDP on cell proliferation (C–E) Mice in the combination treatment group gained weight compared to those in the OXA-treated group. Compared to the other groups, the tumor weight of co-treatment group is lowest. (F,G) Annexin V-FITC and PI-TRITC were used to detected the apoptosis cells. Puerarin improves the efficacy of OXA and a significant increase in apoptosis cells was observed in the co-treatment group (H) Mice of co-treatment group had a higher survival rate than the mice in other groups (*p < 0.05, **p < 0.01).
Each group of tumor cells was tested for apoptosis using the apoptosis detection kit. Annexin V was marked with green fluorescence indicating apoptotic cells and PI was marked with red fluorescence indicating necrotic cells. As shown in Figures 2F,G, puerarin improves the efficacy of OXA and a significant increase in apoptosis cells was observed in the co-treatment group. Moreover, compared to a single treatment, mice of co-treatment group had a higher survival rate (Figure 2H).
Puerarin inhibited migration and invasion and reversed EMT induced by low dose OXA
Morphological changes in the cancer cells in different treatment groups were observed by an optical microscope. As a result of low dose OXA, cancer cells developed pseudopodia and the co-treatment group displayed signs of apoptosis, such as rounded and shed cells, as shown in Figure 3A. The migration and invasion of co-treated cells was detected by wound-healing assay and transwell assay, and the results as shown in Figures 3B,D, the migration was highly enhanced in the OXA-treated group, whereas, it was significantly inhibited in the co-treatment group. Similarly, A combination of purarin and OXA inhibits the invasion of cancer cells by low-dose OXA (Figures 3C,E). Moreover, the expression of EMT biomarkers was detected using an immunofluorescence assay and it was found that vimentin levels of co-treatment group were lower than that of OXA group, whereas E-cadherin levels were higher, as shown in Figures 3F–H. Based on these results, the OXA-treated group promotes EMT, whereas puerarin inhibits the EMT process caused by OXA.
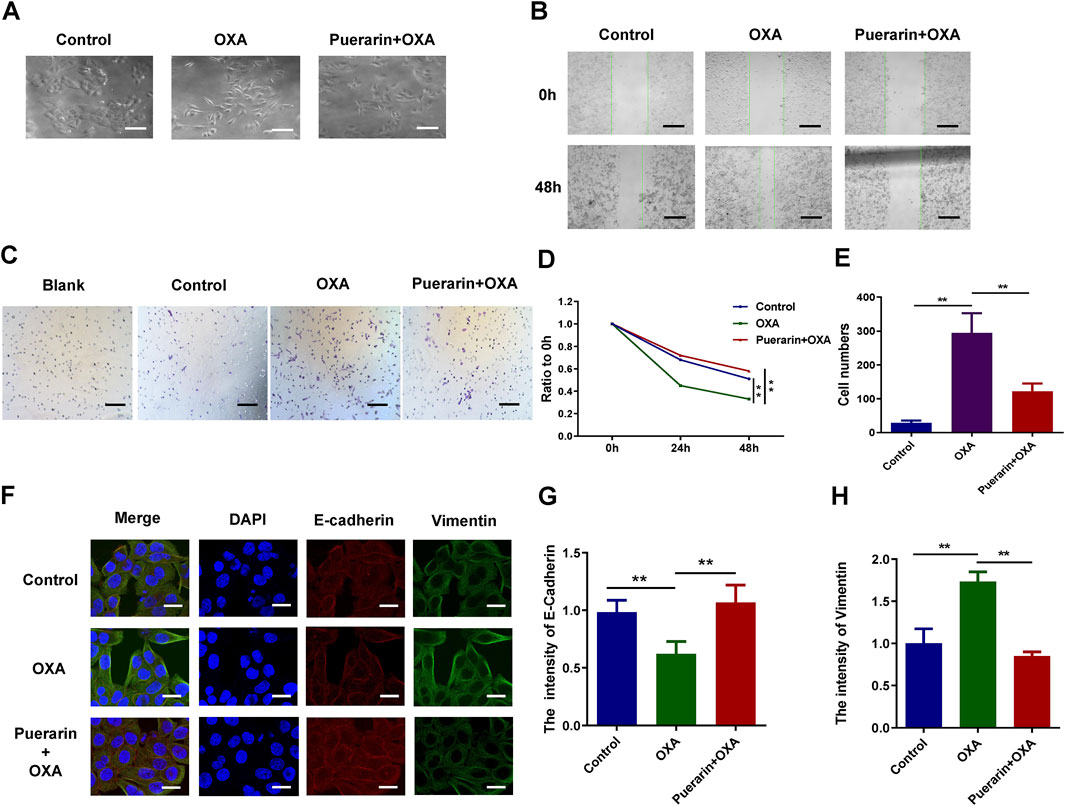
FIGURE 3. Puerarin inhibited migration and invasion and reversed EMT. (A) Morphological changes of the cancer cells in different treatment groups observed by a microscope (B,D) The results of wound-healing assay. (C,E) The results of transwell assay (F–H) The results of immunofluorescence (*p < 0.05, **p < 0.01).
There is a widespread influence of puerarin in cancer cells
The RNA sequencing data (GSE85871) for cancer tissues and adjacent tissues of patients with breast cancer were got from GEO database. The graph was drawn with the log2 ratio and the −log10 (p) of each gene as shown in Figure 4A. Downregulated genes and upregulated genes are indicated by green and red, respectively. The hierarchical clustering was made using the differentially expressed genes, as shown in Figure 4B. The various interactions between the control group and puerarin treatment groups were recorded by the STRING database and visualized in Cytoscape, as shown in Figure 4C. Analysis with GO and the KEGG revealed that differentially expressed genes tended to be enriched for tumor metastasis and energy metabolism, as shown in Figures 4D–G.
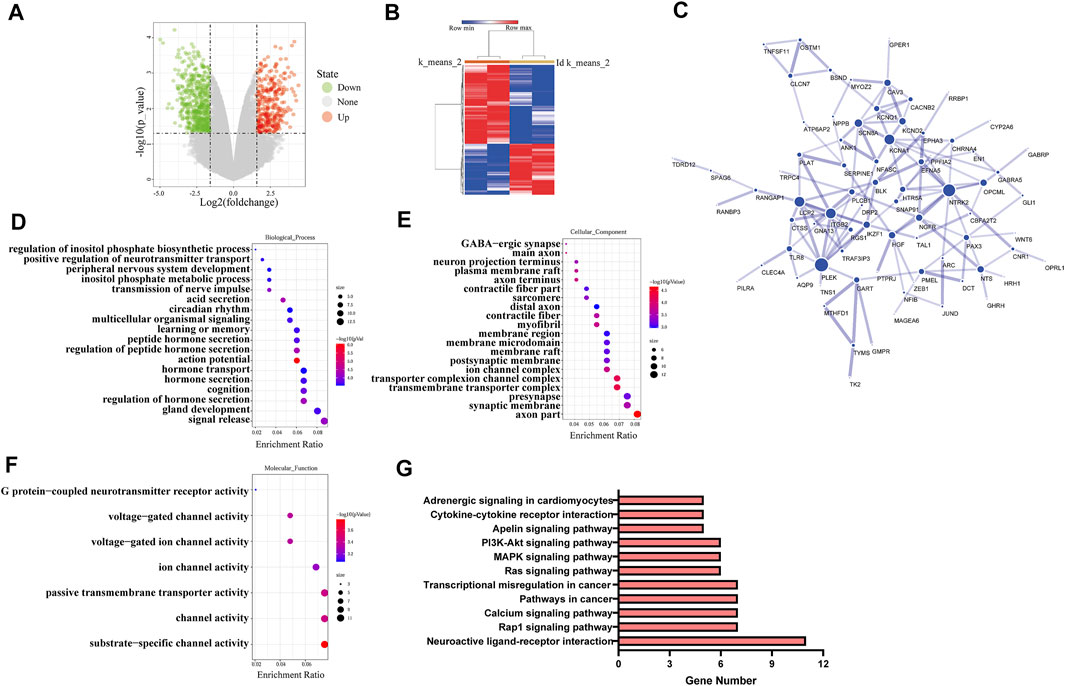
FIGURE 4. Puerarin effected multiple functions and signal pathways of cancer cells. (A) The volcano plot of differentially expressed genes (B) The hierarchical clustering of differentially expressed genes. (C) The protein-protein interaction network of differentially expressed genes (D–F) The GO analysis results of differentially expressed genes, (D) Biological process, (E) Cellular component, (F) Molecular fuction (G) The KEGG analysis results of differentially expressed genes.
CA XII is a potential drug target of puerarin
The SMILES format of puerarin from PubChem and the SEA website was used to predict puerarin’s targets. CA VII and CA XII were the first two potential drug targets with the Max Tanimoto Coefficient (MaxTC) of 1.00, as shown in Figure 5A. The CA XII is more closely associated with cancer cells than with CA VII. The molecular docking results showed that there is a good combination between puerarin and CA XII, with the docking score -5.93, as shown in Figure 5B. CA XII catalyzes the hydration of carbon dioxide to H+ and HCO3−, causing the acidic extracellular pH to decrease. The ability of puerarin to inhibit CA activity was determined by measuring extracellular pH. The results showed that puerarin could inhibit CA activity under a dose-effect relationship (Figure 5C). Low dose OXA treatment increased the extracellular pH compared to the control group, meanwhile, the puerarin inhibited this trend, thereby suggesting that puerarin inhibited CA activity, as shown in Figure 5D.
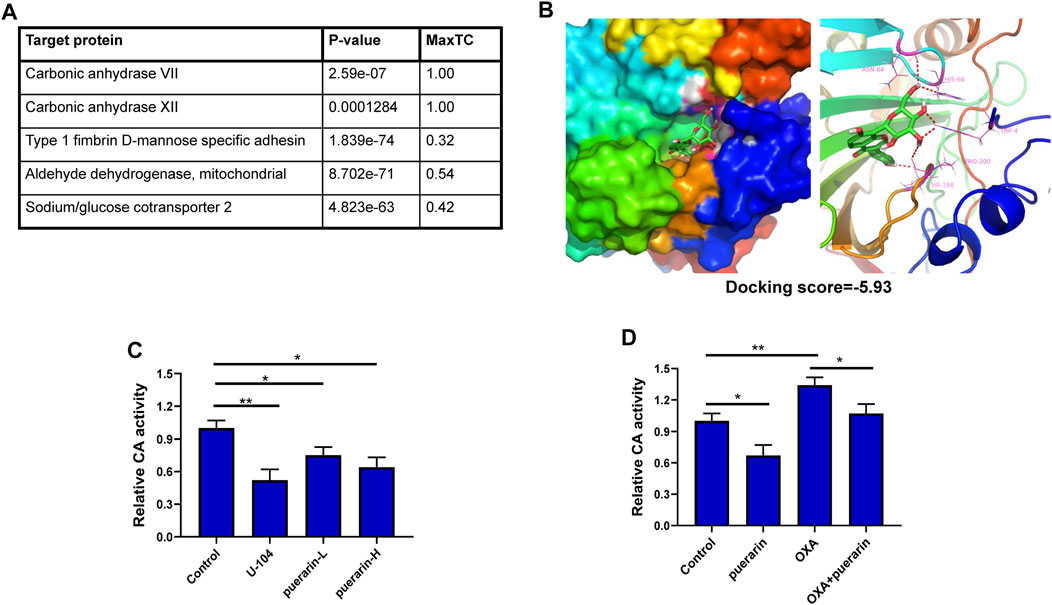
FIGURE 5. CA XII is a potential drug target of puerarin. (A) The predicted targets for puerarin (B) The molecular docking results showed that there is a good combination between puerarin and CA XII, with the docking score -5.93. (C) Puerarin could inhibit CA activity under a dose-effect relationship (D) Low dose OXA treatment increased the extracellular pH level compared with the control group, and puerarin inhibited this trend (*p < 0.05, **p < 0.01).
Discussion
Recently, for breast cancer, while significant progress has been made in diagnosis and treatment, the prognosis remains bleak (Zhao et al., 2017; Chen et al., 2022). It was reported that after OXA treatment, residual cancer cells revealed increased metastasis significantly. Our previous study and other research all showed that low-dose platinum-based anti-cancer drugs could induce EMT of cancer cells (Liu et al., 2015). EMT caused by chemotherapy is a significant determinant of drug resistance to chemotherapy and cancer metastasis.
New therapeutic options that safely enhance chemotherapy sensitivity significantly improve efficiency in cancer treatment (Luan et al., 2020; Zhang et al., 2020). In our study, the combination of puerarin and OXA can improve the sensitivity of OXA chemotherapy, thus, inhibiting the metastasis of breast cancer. In addition, puerarin can reverse OXA resistance in drug-resistant breast cancer. The combined administration of puerarin can also inhibit low-dose OXA-induced EMT as indicated in the results. Meanwhile, the co-treatment group inhibited tumor weight in vivo compared with the chemotherapy drug group alone. Therefore, puerarin can be used as a complementary medicine for OXA in enhancing the chemotherapy sensitivity and anti-cancer ability of OXA.
Multiple studies have shown that puerarin has good anticancer mechanisms against several cancer cells (Li et al., 2019; Aboushanab et al., 2021). However, the exact molecular mechanism and potential drug target of puerarin remain unknown (Wang et al., 2020). Natural small molecules from plants generally have an extensive range of pharmacological activities (Sun et al., 2017). Our results showed that puerarin has the potential to inhibit various functions and signaling pathways of breast cancer cells. Furthermore, it was found that the CA XII is the puerarin’s potential target and puerarin inhibits the acid secretion mediated by the CA protein. The CA XII exists in various organsand plays a key role in life activities (Karhumaa et al., 2000; Parkkila et al., 2000; Liao et al., 2003). CA XII’s expression can be detected in various types of tumor, including breast cancer and other cancer (Kivela et al., 2005; Hsieh et al., 2010; Ilie et al., 2011). Extensive evidences suggest that CA XII plays a key role in the migration, invasion and metastasis of cancer cells. Hsieh et al. demonstrated that silencing CA XII also reduces the migration and invasion of breast cancer cells, and that CA XII interacts with matrix metalloproteinases in proteolysis of ECM during migration and invasion of cancer cells (Hsieh et al., 2010). As another target protein in this study, CA VII is mainly related to the pathogenesis of neuromuscular disorders and has almost no correlation with cancer (Pastorekova et al., 2004; Viikilä et al., 2016), therefore we chose CA XII as our target protein. Recent studies have indicated that CA XII participates in chemotherapy drug resistance, hence, promoting the further development of tumors (Kobayashi et al., 2012; Kopecka et al., 2015). Our results showed that a low dose of OXA activates the CA XII’s activity in breast cancer cells, which is detrimental to cancer treatment. Puerarin can inhibit the activity of CA XII, which can influence chemotherapy drugs to enhance the anticancer effect of chemotherapy drugs.
There are also some deficiencies in this study. In future research, in-depth studies on the mechanism of puerarin in vivo and systematic studies on the toxicity, side effects of puerarin could be done. In addition, the pharmacological activity of puerarin could be further enhanced by targeted modification of its structure through medicinal chemistry methods.
Our results demonstrated the anticancer effects of puerarin on tumor cells and models of xenograft mice. Puerarin targeted CA XII and affected multiple carcinogenic signaling networks. In addition, puerarin significantly increased platinum sensitivity and inhibited platinum-induced EMT in breast cancer. There are some reasons why puerarin is expected to become an adjuvant chemotherapy drug and has the potential to become one of the medicated foods for breast cancer patients.
Data availability statement
The datasets presented in this study can be found in online repositories. The names of the repository/repositories and accession number(s) can be found in the article/Supplementary Material.
Ethics statement
The animal study was reviewed and approved by the Animal Ethics Committee of the Zhejiang Sci-tech University.
Author contributions
XC and ZZ wrote the main manuscript text and prepared all figures. ZZ and CZ did the data processing. JL, JJ, and BH offered their opinions on the paper. YQ provided the funds and supervised the project.
Funding
Scientific Research Foundation of Zhejiang Sci-Tech University (Project Number: 21042102-Y).
Conflict of interest
The authors declare that the research was conducted in the absence of any commercial or financial relationships that could be construed as a potential conflict of interest.
Publisher’s note
All claims expressed in this article are solely those of the authors and do not necessarily represent those of their affiliated organizations, or those of the publisher, the editors and the reviewers. Any product that may be evaluated in this article, or claim that may be made by its manufacturer, is not guaranteed or endorsed by the publisher.
References
Aboushanab, S. A., Khedr, S. M., Gette, I. F., Danilova, I. G., Kolberg, N. A., Ravishankar, G. A., et al. (2021). Isoflavones derived from plant raw materials: Bioavailability, anti-cancer, anti-aging potentials, and microbiome modulation. Crit. Rev. Food Sci. Nutr. 6, 1–27. doi:10.1080/10408398.2021.1946006
Ahmad, B., Khan, S., Liu, Y., Xue, M., Nabi, G., Kumar, S., et al. (2020). Molecular mechanisms of anticancer activities of puerarin. Cancer Manag. Res. 12, 79–90. doi:10.2147/CMAR.S233567
Bisol, Â., de Campos, P. S., and Lamers, M. L. (2020). Flavonoids as anticancer therapies: A systematic review of clinical trials. Phytother. Res. 34 (3), 568–582. doi:10.1002/ptr.6551
Chen, H. M., Wu, Y. C., Chia, Y. C., Chang, F. R., Hsu, H. K., Hsieh, Y. C., et al. (2009). Gallic acid, a major component of Toona sinensis leaf extracts, contains a ROS-mediated anti-cancer activity in human prostate cancer cells. Cancer Lett. 286 (2), 161–171. doi:10.1016/j.canlet.2009.05.040
Chen, T., Chen, H., Wang, Y., and Zhang, J. (2016). In vitro and in vivo antitumour activities of puerarin 6″-O-xyloside on human lung carcinoma A549 cell line via the induction of the mitochondria-mediated apoptosis pathway. Pharm. Biol. 54 (9), 1793–1799. doi:10.3109/13880209.2015.1127980
Chen, X., Zhou, K., Xiang, Z., Zhou, X., Wang, Y., Hong, J., et al. (2022). Establishment and clinical application of time-resolved immunofluorescence assay of lipoprotein-associated phospholipase A2. Anal. Biochem. 648, 114674. doi:10.1016/j.ab.2022.114674
Dai, C. J., Cao, Y. T., Huang, F., and Wang, Y. G. (2022). Multiple roles of mothers against decapentaplegic homolog 4 in tumorigenesis, stem cells, drug resistance, and cancer therapy. World J. Stem Cells 14 (1), 41–53. doi:10.4252/wjsc.v14.i1.41
Hsieh, M. J., Chen, K. S., Chiou, H. L., and Hsieh, Y. S. (2010). Carbonic anhydrase XII promotes invasion and migration ability of MDA-MB-231 breast cancer cells through the p38 MAPK signaling pathway. Eur. J. Cell Biol. 89 (8), 598–606. doi:10.1016/j.ejcb.2010.03.004
Hu, Y., Li, X., Lin, L., Liang, S., and Yan, J. (2018). Puerarin inhibits non-small cell lung cancer cell growth via the induction of apoptosis. Oncol. Rep. 39 (4), 1731–1738. doi:10.3892/or.2018.6234
Ilie, M. I., Hofman, V., Ortholan, C., Ammadi, R. E., Bonnetaud, C., Havet, K., et al. (2011). Overexpression of carbonic anhydrase XII in tissues from resectable non-small cell lung cancers is a biomarker of good prognosis. Int. J. Cancer 128 (7), 1614–1623. doi:10.1002/ijc.25491
Jiang, J., Li, J., Zhou, X., Zhao, X., Huang, B., and Qin, Y. (2022). Exosomes regulate the epithelial-mesenchymal transition in cancer. Front. Oncol. 12, 864980. doi:10.3389/fonc.2022.864980
Kang, H., Zhang, J., Wang, B., Liu, M., Zhao, J., Yang, M., et al. (2017). Puerarin inhibits M2 polarization and metastasis of tumor-associated macrophages from NSCLC xenograft model via inactivating MEK/ERK 1/2 pathway. Int. J. Oncol. 50 (2), 545–554. doi:10.3892/ijo.2017.3841
Karhumaa, P., Parkkila, S., Tureci, O., Waheed, A., Grubb, J. H., Shah, G., et al. (2000). Identification of carbonic anhydrase XII as the membrane isozyme expressed in the normal human endometrial epithelium. Mol. Hum. Reprod. 6 (1), 68–74. doi:10.1093/molehr/6.1.68
Keiser, M. J., Roth, B. L., Armbruster, B. N., Ernsberger, P., Irwin, J. J., and Shoichet, B. K. (2007). Relating protein pharmacology by ligand chemistry. Nat. Biotechnol. 25 (2), 197–206. doi:10.1038/nbt1284
Kivela, A. J., Parkkila, S., Saarnio, J., Karttunen, T. J., Kivela, J., Parkkila, A. K., et al. (2005). Expression of von Hippel-Lindau tumor suppressor and tumor-associated carbonic anhydrases IX and XII in normal and neoplastic colorectal mucosa. World J. Gastroenterol. 11 (17), 2616–2625. doi:10.3748/wjg.v11.i17.2616
Kobayashi, M., Matsumoto, T., Ryuge, S., Yanagita, K., Nagashio, R., Kawakami, Y., et al. (2012). CAXII Is a sero-diagnostic marker for lung cancer. PLoS One 7 (3), e33952. doi:10.1371/journal.pone.0033952
Kopecka, J., Campia, I., Jacobs, A., Frei, A. P., Ghigo, D., Wollscheid, B., et al. (2015). Carbonic anhydrase XII is a new therapeutic target to overcome chemoresistance in cancer cells. Oncotarget 6 (9), 6776–6793. doi:10.18632/oncotarget.2882
Kopustinskiene, D. M., Jakstas, V., Savickas, A., and Bernatoniene, J. (2020). Flavonoids as anticancer agents. Nutrients 12 (2), E457. doi:10.3390/nu12020457
Lamson, D. W., and Brignall, M. S. (2000). Antioxidants and cancer, part 3: Quercetin. Altern. Med. Rev. 5 (3), 196–208.
Li, J., Guo, C., Lu, X., and Tan, W. (2019). Anti-colorectal cancer biotargets and biological mechanisms of puerarin: Study of molecular networks. Eur. J. Pharmacol. 858, 172483. doi:10.1016/j.ejphar.2019.172483
Liao, S. Y., Ivanov, S., Ivanova, A., Ghosh, S., Cote, M. A., Keefe, K., et al. (2003). Expression of cell surface transmembrane carbonic anhydrase genes CA9 and CA12 in the human eye: Overexpression of CA12 (CAXII) in glaucoma. J. Med. Genet. 40 (4), 257–261. doi:10.1136/jmg.40.4.257
Liu, F., Peng, Y., Qiao, Y., Huang, Y., Song, F., Zhang, M., et al. (2022). Consumption of flavonoids and risk of hormone-related cancers: A systematic review and meta-analysis of observational studies. Nutr. J. 21 (1), 27. doi:10.1186/s12937-022-00778-w
Liu, X., Zhao, W., Wang, W., Lin, S., and Yang, L. (2017). Puerarin suppresses LPS-induced breast cancer cell migration, invasion and adhesion by blockage NF-κB and Erk pathway. Biomed. Pharmacother. 92, 429–436. doi:10.1016/j.biopha.2017.05.102
Liu, Y. Q., Zhang, G. A., Zhang, B. C., Wang, Y., Liu, Z., Jiao, Y. L., et al. (2015). Short low concentration cisplatin treatment leads to an epithelial mesenchymal transition-like response in DU145 prostate cancer cells. Asian pac. J. Cancer Prev. 16 (3), 1025–1028. doi:10.7314/apjcp.2015.16.3.1025
Luan, J., Gao, X., Hu, F., Zhang, Y., and Gou, X. (2020). SLFN11 is a general target for enhancing the sensitivity of cancer to chemotherapy (DNA-damaging agents). J. Drug Target. 28 (1), 33–40. doi:10.1080/1061186X.2019.1616746
Maleki, S. J., Crespo, J. F., and Cabanillas, B. (2019). Anti-inflammatory effects of flavonoids. Food Chem. 299, 125124. doi:10.1016/j.foodchem.2019.125124
Middleton, J. D., Stover, D. G., and Hai, T. (2018). Chemotherapy-exacerbated breast cancer metastasis: A paradox explainable by dysregulated adaptive-response. Int. J. Mol. Sci. 19 (11), E3333. doi:10.3390/ijms19113333
Park, D. D., Phoomak, C., Xu, G., Olney, L. P., Tran, K. A., Park, S. S., et al. (2020). Metastasis of cholangiocarcinoma is promoted by extended high-mannose glycans. Proc. Natl. Acad. Sci. U. S. A. 117 (14), 7633–7644. doi:10.1073/pnas.1916498117
Parkkila, S., Parkkila, A. K., Saarnio, J., Kivela, J., Karttunen, T. J., Kaunisto, K., et al. (2000). Expression of the membrane-associated carbonic anhydrase isozyme XII in the human kidney and renal tumors. J. Histochem. Cytochem. 48 (12), 1601–1608. doi:10.1177/002215540004801203
Pastorekova, S., Parkkila, S., Pastorek, J., and Supuran, C. T. (2004). Carbonic anhydrases: Current state of the art, therapeutic applications and future prospects. J. Enzyme Inhib. Med. Chem. 19 (3), 199–229. doi:10.1080/14756360410001689540
Pastushenko, I., and Blanpain, C. (2019). EMT transition states during tumor progression and metastasis. Trends Cell Biol. 29 (3), 212–226. doi:10.1016/j.tcb.2018.12.001
Qiao, K., Chen, C., Liu, H., Qin, Y., and Liu, H. (2021). Pinin induces epithelial-to-mesenchymal transition in hepatocellular carcinoma by regulating m6A modification. J. Oncol. 2021, 7529164. doi:10.1155/2021/7529164
Shirode, A. B., Bharali, D. J., Nallanthighal, S., Coon, J. K., Mousa, S. A., and Reliene, R. (2015). Nanoencapsulation of pomegranate bioactive compounds for breast cancer chemoprevention. Int. J. Nanomedicine 10, 475–484. doi:10.2147/IJN.S65145
Sun, Y. F., Wang, Z. A., Han, R. L., Lu, H. F., Zhang, J. L., et al. (2017). Isaria cicadae conidia possess antiproliferative and inducing apoptosis properties in gynaecological carcinoma cells. Mycology 8 (4), 327–334. doi:10.1080/21501203.2017.1386243
Viikilä, P., Kivela, A. J., Mustonen, H., Koskensalo, S., Waheed, A., Sly, W. S., et al. (2016). Carbonic anhydrase enzymes II, VII, IX and XII in colorectal carcinomas. World J. Gastroenterol. 22 (36), 8168–8177. doi:10.3748/wjg.v22.i36.8168
Wang, S., Zhang, S., Gao, P., and Dai, L. (2020). A comprehensive review on Pueraria: Insights on its chemistry and medicinal value. Biomed. Pharmacother. 131, 110734. doi:10.1016/j.biopha.2020.110734
Wang, Y., Ma, Y., Zheng, Y., Song, J., Yang, X., Bi, C., et al. (2013). In vitro and in vivo anticancer activity of a novel puerarin nanosuspension against colon cancer, with high efficacy and low toxicity. Int. J. Pharm. 441 (1-2), 728–735. doi:10.1016/j.ijpharm.2012.10.021
Wills, C. A., Liu, X., Chen, L., Zhao, Y., Dower, C. M., Sundstrom, J., et al. (2021). Chemotherapy-induced upregulation of small extracellular vesicle-associated PTX3 accelerates breast cancer metastasis. Cancer Res. 81 (2), 452–463. doi:10.1158/0008-5472.CAN-20-1976
Zhang, X., Wu, L., Xu, Y., Yu, H., Chen, Y., Zhao, H., et al. (2020). Microbiota-derived SSL6 enhances the sensitivity of hepatocellular carcinoma to sorafenib by down-regulating glycolysis. Cancer Lett. 481, 32–44. doi:10.1016/j.canlet.2020.03.027
Keywords: puerarin, oxaliplatin, breast cancer, epithelial-mesenchymal transition, carbonic anhydrase XII
Citation: Chen X, Zhou Z, Zhang Z, Zhao C, Li J, Jiang J, Huang B and Qin Y (2022) Puerarin inhibits EMT induced by oxaliplatin via targeting carbonic anhydrase XII. Front. Pharmacol. 13:969422. doi: 10.3389/fphar.2022.969422
Received: 15 June 2022; Accepted: 25 July 2022;
Published: 25 August 2022.
Edited by:
Weilong Zhong, Tianjin Medical University General Hospital, ChinaReviewed by:
Yifei Gao, Tsinghua University, ChinaZhaowei Xu, Binzhou Medical University, China
Xiaowei Guo, Hunan Normal University, China
Copyright © 2022 Chen, Zhou, Zhang, Zhao, Li, Jiang, Huang and Qin. This is an open-access article distributed under the terms of the Creative Commons Attribution License (CC BY). The use, distribution or reproduction in other forums is permitted, provided the original author(s) and the copyright owner(s) are credited and that the original publication in this journal is cited, in accordance with accepted academic practice. No use, distribution or reproduction is permitted which does not comply with these terms.
*Correspondence: Yuan Qin, cWlueXVhbkB6c3R1LmVkdS5jbg==
†These authors have contributed equally to this work