- 1Key Laboratory of Drug-Targeting and Drug Delivery System of the Education Ministry and Sichuan Province, Sichuan Engineering Laboratory for Plant-Sourced Drug and Sichuan Research Center for Drug Precision Industrial Technology, West China School of Pharmacy, Sichuan University, Chengdu, China
- 2State Key Laboratory of Southwestern Chinese Medicine Resources, Chengdu University of Traditional Chinese Medicine, Chengdu, China
- 3College of Life Science, Sichuan Normal University, Chengdu, China
Safety has always been an important issue affecting the development of traditional Chinese medicine industry, especially for toxic medicinal materials, the establishment of risk prevention and control measures for toxic herbs is of great significance to improving the use of traditional Chinese medicine in clinical. Fuzi is a kind of traditional Chinese medicine and its toxicity has become the most important obstacle of limit in clinical using. In this paper, network pharmacology and molecular docking technology were used to analyze the main toxic components of Fuzi, the key targets and the mechanism of neurotoxicity. We carried out CCK-8 and WB assays, and detected LDH release and SDH activity. It was verified that aconitine caused neurotoxicity through a variety of pathways, including MAPK signaling pathway, pathways related to Akt protein, destruction of cell membrane integrity, damage of mitochondrial function affecting energy metabolism and apoptosis. What’s more, this study confirmed that aconitine could produce neurotoxicity by promoting apoptosis of hippocampus neuron and decreasing its quantity through Nissl Staining and TUNEL assay. This paper found and confirmed multiple targets and various pathways causing neurotoxicity of Fuzi, in order to provide reference for clinical application and related research.
Introduction
Aconiti Lateralis Radix Praeparata (Fuzi in Chinese), known as Chinese aconite, Chinese wolfsbane and monkshood, is the lateral root of Aconitum Carmichaelii Debeaux (Zhao et al., 2020). Fuzi is a traditional Chinese medicine in China, which was recorded in the Shennong herbal Scripture. In the history of clinical use for thousands of yeas, numerous classical prescriptions such as four inverse soup, zhenwu decoction, and mahuang fuzi xixin decoction have emerged. As for today, modern research have shown that Fuzi has a wide range of pharmacological effects and is extensively used in treatment of cardiovascular diseases, rheumatism arthritis, neuropathic pain and bronchitis (Yang et al., 2018). Although Fuzi has promising therapeutic effects, its toxicities are frequently observed, like cardiac toxicity, neurotoxicity, hepatotoxicity, nephrotoxicity and so on (Chan, 2009). The current research about for cardiac toxicity of Fuzi is relatively wide, but the mechanism of its neurotoxicity still needs further research.
In 2007, the concept of “network pharmacology” was first proposed (Hopkins, 2007), in the same year, Chinese scholars used the biological network to study the traditional Chinese medicine prescriptions. In recent years, the research filed of systems biology has greatly advanced, as a result, the application of network pharmacology in traditional Chinese medicine has been developed and has a series of achievements (Li and Zhang, 2013; Zhang et al., 2019).
In this study, on the basis of related literature reports, we aim to research the mechanism of neurotoxicity of Fuzi, using network pharmacology and molecular docking method, in order to provide reference for clinical application and related research. The workflow of this study is shown in Figure 1.
Materials and methods
Network pharmacology research
Screening the toxic compounds of fuzi and their targets
HERB database (http://herb.ac.cn/) and SymMap database (http://www.symmap.org/) were used to get all active compounds of Fuzi. Then, we retrieved the targets associated with active compounds from CTD database (http://ctdbase.org/), and our filter was set to “Interaction Count >1”. We collected all the targets, deleted duplicates and got candidate toxic compounds of Fuzi and potential target genes.
Predicting the targets of neurotoxicity
We searched potential targets of neurotoxicity by using “toxic encephalopathy” and “nerve toxicity” as the key words in GeneCards database (https://www.genecards.org/) and set “Relevance score ≥10” as a filter. Then the results of the two were combined and duplicated targets were removed in order to get potential targets of neurotoxicity.
Prediction of candidate targets and construction of PPI network
We used Venny 2.1.0 database (https://bioinfogp.cnb.csic.es/tools/venny/) to match the candidate targets of toxic compounds and the potential targets of neurotoxicity, and intersection target genes were the potential targets in the toxic effect of Fuzi on nerve.
All the targets were uploaded to String database (https://cn.string-db.org/) to build the PPI network interaction. Cytoscape 3.9.1 was used to construct and visualize the PPI network. CytoHubba, a network topology analysis plug-in in Cytoscape, was used for analyzing topology parameters of each target. The value of “Degree” was used as a reference for the importance of the core targets.
After that, we put the candidate toxic compounds of Fuzi and the corresponding 10 core targets into Cytoscape 3.9.1 to construct the relationship network between candidate toxic compounds of Fuzi and the corresponding 10 core targets.
GO biological process and KEGG pathway enrichment analysis
Intersected target genes were uploaded to DAVID database (https://david.ncifcrf.gov/) for performing GO biological process and KEGG pathway enrichment analysis (p < 0.05). GO enrichment analysis includes Biological Process (BP), Molecular Function (MF), and Cellular Component (CC) analysis. KEGG is a bioinformatics resource for mining significantly altered metabolic pathways enriched in the gene list. GO and KEGG pathway analysis were visualized using the R programming language.
Molecular docking
PubChem database (https://pubchem.ncbi.nlm.nih.gov/) was used to download the SDF format of key toxic compounds, and OpenBabel software was used to transfer into MOL2 format. The 3D structure of the protein was downloaded in PDB database (http://www.rcsb.org/).
Then, we imported the search results into AutoDock Tool software. The target protein was used as a receptor with the water molecule removed and nonpolar hydrogen added. The key toxic compound was used as a ligand, and the Grid box coordinated and size were set according to the target protein. At last, we selected the binding conformation with the lowest free binding energy by using AutoDock Vina and imported it into PyMol software for visualization.
Experimental validation
Reagents
Aconitine (purity ≥98%), the chemical structure is shown in Figure 2, was obtained from Chengdu Must Bio-Technology Co., Ltd. (Chengdu, China).
Dulbecco’s Modified Eagle’s Medium (DMEM) was purchased from Hylone (Logan, Utah, United States). 10% Fetal Bovine Serum (FBS) was obtained from GIBCO (NY, United States). The Cell Counting Kit-8 (CCK-8) was bought from Sigma-Aldrich (Nevada, United States). Penicillin-Streptomycin Solution was obtained from Allcare Biomedical Development (Qingdao, China). The primary antibodies against p-MAPK, MAPK1, p53, p-Akt, Akt, Caspase-3 and GAPDH were purchased from Cell Signaling Tech. (MA, United States). Trypsin, Tris-base, glutathione (GSH), acrylamide, SDS, AP, THMED and other reagents were obtained from Amresco (United States). Nissl Staining Solution and LDH Assay Kit were bought from Beyotime (Shanghai, China). SDH Assay Kit was obtained from Nanjing Jiancheng Bioengineering Institute (Nanjing, China). The In Situ Cell Death Detection Kit was purchased from Roche (Basel, Switzerland). Alcohol and xylene were obtained from SINOPHARM (Beijing, China). And paraformaldehyde was purchased from Sangon Biotech (Shanghai, China).
Cell culture
SH-SY5Y cells were obtained from cell bank in Shanghai Institutes for Biological Sciences (SIBS, CAS). They were cultured in DMEM medium supplemented with 10% (v/v) FBS and 1% (v/v) penicillin-streptomycin solution, and maintained at 37°C in a humidified chamber with 5% CO2.
Cell viability assay
Cell viability was measured using CCK-8 assay. SH-SY5Y cells were seeded into 96-well plates in the concentration of 5×103 cells per well. After incubating in a cell incubator overnight, SH-SY5Y cells were exposed to aconitine in concentrations of 0, 25, 50, 100, 200, 300, 400 μM for 24 and 48 h. The wells added amount of medium is the blank group. Then, 10 μL of CCK-8 solution was added to each well of the plate. After 1 h of incubating, we measured the optical density (OD) at 450 nm wavelength with a microplate reader. Each experiment was repeated three times. (Cell viability = [(ODExperiment group - ODBlank)/(ODControl group - ODBlank)]×100%)
Western blot assay
SH-SY5Y cells (5×105 cells/well) were seeded into 6-well plates and were exposed to aconitine in concentrations of 0, 100, 200, 400 μM for 24 h. Cell proteins were extracted after treatment with RIPA lysis buffer. The BCA method was used to calculate the content of protein. According to the relative molecular mass of target protein, we prepared 10% sodium dodecyl sulfate polyacrylamide gel electrophoresis (SDS-PAGE). An equal amount of the protein (20 μg per sample) was loaded onto SDS-PAGE for separation and transferred onto polyvinylidene difluoride (PVDF) membranes. Then we used 5% BSA for 1 h to block the membranes and used the primary antibodies including anti-p-MAPK, anti-MAPK1, anti-p53, anti-p-Akt, anti-Akt, anti-Caspase-3 and anti-GAPDH overnight at 4°C. After washed with TBST three times and further incubated with HRP-conjugated secondary antibodies for 1 h at room temperature, the protein bands were detected by the CheniDoc MP Imaging System.
Detection of lactate dehydrogenase release
SH-SY5Y cells were incubated in 96-well plates and exposed to aconitine in concentrations of 0, 25, 50, 100, 200, 300, 400 μM for 24 h. Then, 10 μL of Lysis solution was added to each well of the plate to assess maximum LDH release. We collected supernatants after centrifugation at 400 g for 5 min and transferred 120 μL of the supernatant from each well to a new assay plate. Finally, we added 60 μL of the detection solution to each well and recorded absorbance at 490 nm after 30min of incubating. Experiments were repeated three times.
Detection of succinate dehydrogenase activity
SH-SY5Y cells were incubated in 6-well plates and exposed to aconitine in concentrations of 0, 100, 200, 400 μM for 24 h. Then we collected supernatants after centrifugation. And the SDH activity was measured using SDH Assay Kit according to the manufacturer’s protocol. Experiments were repeated three times.
Animals and treatment
Twenty rats of SPF grade were used in this study. All rats were about six weeks old and divided into two equal groups for different experimental analysis. Each rat in group I (experimental) was given intraperitoneal injection (i.p.) of aconitine (1 mg/kg), while each rat in group II, which served as control, received an equal volume of normal saline.
The treatment was last for one week. Then, the rats were sacrificed. We quickly removed the brain tissue. Tissues for examination were fixed and preserved in paraformaldehyde, processed and trimmed, embedded in paraffin, and sectioned to a thickness of 5 μm.
These studies were conducted in compliance with the “Guide for the Care and Use of Laboratory Animals” (National Research Council (US), 2011).
Nissl staining experiment
The brain sections were dewaxed, rehydrated and washed with distilled water three times. Then we used Nissl Staining solution to stain the sections for 5 min and used distilled water to wash them twice. After being stained, the sections were dehydrated with 95% ethanol for 2 min (twice) and xylene for 5 min (twice), and then fixed using neutral gum. The number of hippocampus neuron was visualized with an optical microscope at 40 × and 100 × magnification. Then we used ImageJ software to count normal nerve cells.
TUNEL assay
Apoptosis cells were measured by using In Situ Cell Death Detection Kit. After being dewaxed, rehydrated and washed with distilled water three times, the sections were stained according to the manufacturer’s instructions and apoptotic cells were stained brown due to the binding of dUTP enzyme to their fragmented DNA. We used an optical microscope at 40× and 100 × magnification to observe apoptosis of hippocampus neuron. Then, we used ImageJ software to calculate the number of apoptotic cells.
Statistical analysis
All statistical analyses were preformed using GraphPad prism 8.0. Differences in multiple groups were analyzed by ANOVA and p < 0.05 was considered statistically significant.
Results
Toxic compounds of fuzi and their targets
We used HERB and SymMap database to predict the toxic compounds of Fuzi, and a total of 177 active compounds were retrieved. All the active compounds were input into CTD database for compound retrieval, and 22 candidate toxic compounds of Fuzi were obtained. After we combined the targets of all toxic compounds and deleted the duplicates, a total of 1810 corresponding targets were obtained. Then toxic compounds with corresponding targets greater than 10 were selected as candidate core toxic compounds (Table 1).
Targets of neurotoxicity
In the GeneCards database, 252 potential targets were searched by using “toxic encephalopathy” as the key word, and 290 potential targets were searched by using “nerve toxicity” as the key word. All the collected target genes were merged and duplicated. Then we obtained 474 potential targets of neurotoxicity.
Prediction of candidate targets and construction of PPI network
Matching the candidate targets of toxic compounds and the potential targets of neurotoxicity, 133 genes were selected as potential targets in the toxic effect of Fuzi on nerve. Then, String database was used to identify the PPI network of the 133 intersection target genes, as shown in Figure 3A.
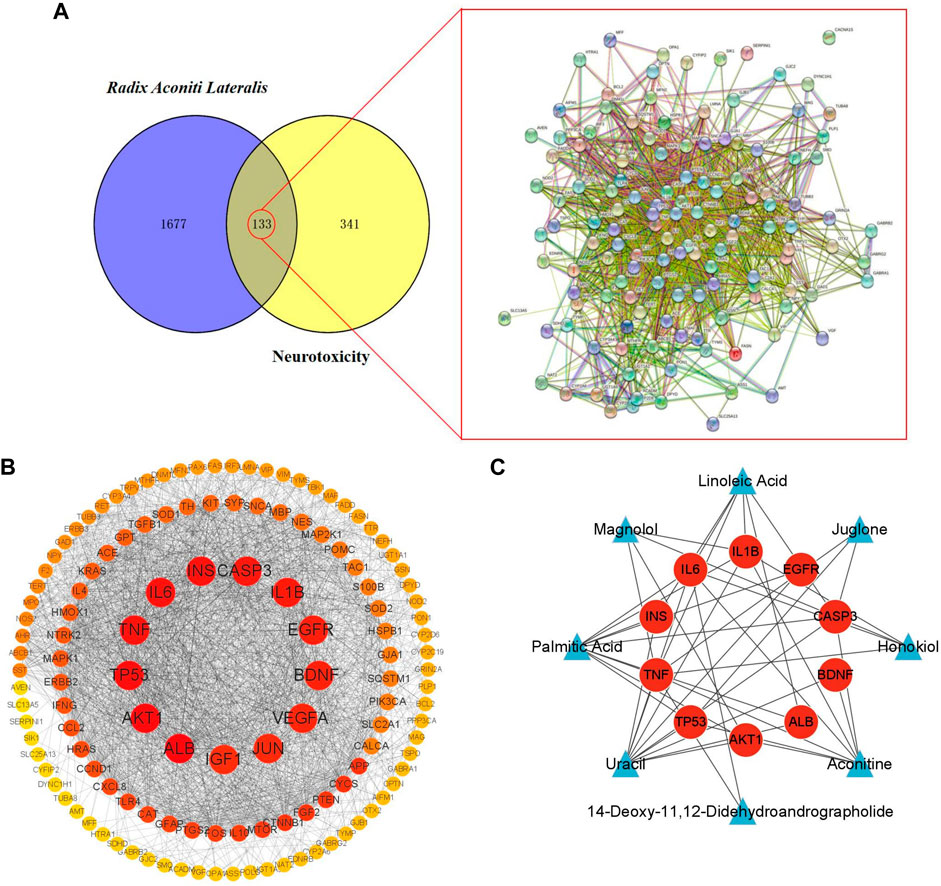
FIGURE 3. Target screening and establishment of PPI network. (A) Potential targets of neurotoxicity caused by Fuzi and PPI network. (B) PPI network of neurotoxicity targets caused by Fuzi. (The color of the node is marked from red to yellow according to the Degree value in descending order.) (C) Relationship network between candidate toxic compounds and core targets of Fuzi. (Blue triangles represent candidate toxic compounds and red circles represent core targets).
We uploaded the PPI network in the figure above to Cytoscape 3.9.1 and used the Network Analyzer function in the software to analyze the topology parameters of each target (Table 2).
The top 10 are selected as the core targets according to the ranking of Degree value, followed by ALB, AKT1, TP53, IL6, TNF, INS, CASP3, IL1B, EGFR and BDNF. The visualization results are shown in Figure 3B.
Subsequently, we used Cytoscape3.9.1 to construct the relationship network between candidate toxic compounds of Fuzi and the corresponding 10 core targets in Figure 3C, and selected uracil, palmitic acid and aconitine as the key toxic compounds of Fuzi for subsequent analysis according to Degree value.
GO biological process and KEGG pathway enrichment analysis
To explore the toxic mechanisms, we imported 133 intersection targets into DAVID database for GO and KEGG pathway enrichment analyses. A total of 184 GO functional items were obtained, including 124 biological processes (BP), which mainly involved response to nutrient levels, gliogenesis, neuron death and aging, etc. There were 18 cellular components (CC), mainly including membrane raft, membrane microdomain, organelle outer membrane and vesicle lumen, etc. It also included 42 molecular function (MF), focusing on signaling receptor activator activity and receptor ligand activity. The first 10 items of enrichment results were visualized according to p-value, as shown in Figure 4A.
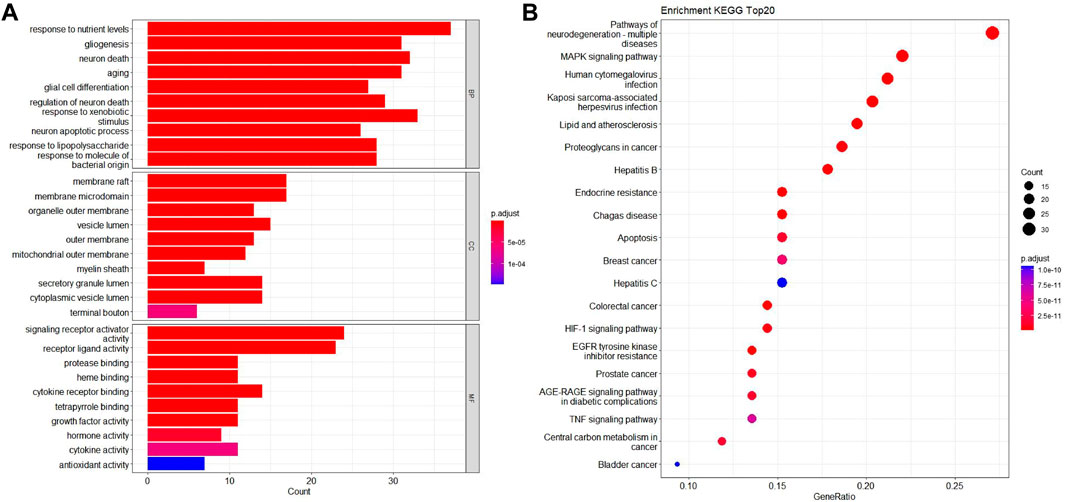
FIGURE 4. GO biological process and KEGG pathway enrichment analysis. (A) The bubble diagram of GO enrichment analysis of 133 intersection targets, including the top 10 significant enrichment terms of BP, CC and MF. (B) The bar plot diagram of KEGG pathway enrichment analysis of 133 intersection targets (top 20).
We screened 170 major signaling pathways in KEGG pathway enrichment results. The high ranking enriched pathways involved pathways of neurodegenerative multiple disease, MAPK signaling pathway, HIF-1 signaling pathway, TNF signaling pathway, and various infection and tumor-specific pathways. Other pathways included lipid and atherosclerosis, Hepatitis B and Hepatitis C, endocrine resistance, apoptosis and so on. The most significant enriched 20 pathways in KEGG analysis were shown in Figure 4B.
Among them, MAPK1, TP53, TNF, EGFR, INS, CASP3, IL1B and BDNF in the core targets were all related to MAPK signaling pathway, which is an important mechanism of Fuzi leading to neurotoxicity.
Molecular docking
In order to further verify the molecular mechanism of neurotoxicity of Fuzi, we chose uracil, palmitic acid and aconitine, the key toxic substances of Fuzi, to preliminarily simulate their binding to the core targets (Table 3).
It is generally believed that when the binding energy is less than −5.0 kJ mol−1, this compound has a good binding activity with the core target protein, while when the binding energy is less than −7.0 kJ mol−1, this compound has a strong binding activity with the core target protein (Hsin et al., 2013).
Among the key toxic substances of Fuzi, the binding energy of aconitine with ALB, AKT1 and CASP3 proteins were all less than −7.0 kJ·mol−1, indicating that aconitine was the most stable active ingredient. Figure 5 showed the docking diagram of aconitine with ALB, AKT1 and CASP3 protein molecules.
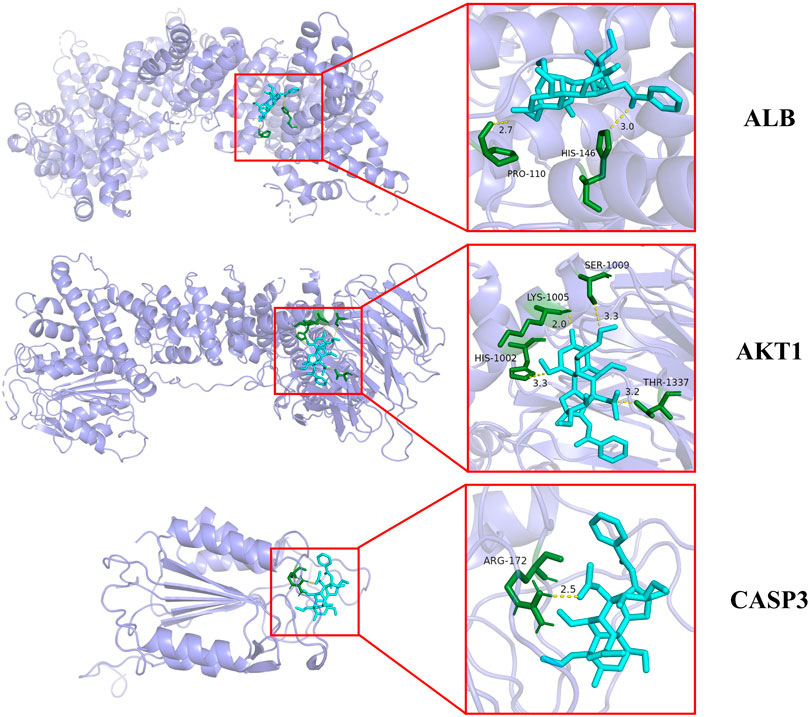
FIGURE 5. Molecular docking patterns of aconitine and core protein molecules. (The yellow lines represent the hydrogen bond interaction force, which is the main force promoting molecule binding with the active site.)
Aconitine inhibits SH-SY5Y cell viability
Network pharmacology analysis showed that aconitine was the main toxic substance causing neurotoxicity of Fuzi, so we further investigated the toxic effect of aconitine on SH-SY5Y cells. The results of CCK-8 assay showed that aconitine at 300 and 400 μM concentrations could decrease SH-SY5Y cell viability following 24 h treatment and aconitine at all the different concentrations (25, 50, 100, 200, 300 and 400 μM) significantly decreased SH-SY5Y cell viability after 48 h treatment, and the cell viability rate gradually decreased with the increase of concentration, indicating that aconitine had significant toxic effect on SH-SY5Y cells (Figure 6). Moreover, IC50 value of the CCK-8 is 271.4226 μM (48 h).
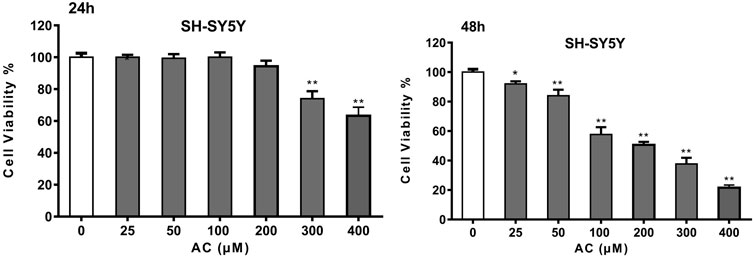
FIGURE 6. Cell Viability of SH-SY5Y cells after 24 and 48 h aconitine treatment. (*p < 0.05, **p < 0.01 versus control group.)
Aconitine affects MAPK signaling pathway
According to the results of the KEGG enrichment analysis, MAPK signaling pathway was an important mechanism of neurotoxicity caused by Fuzi. Therefore, we further evaluated the expression levels of p53, p-MAPK and MAPK1, which are the core target proteins in MAPK signaling pathway. As shown in Figure 7A, aconitine inhibited the expression of p-MAPK, while the expression of MAPK1 remained basically unchanged. Meanwhile, aconitine also promoted the expression of p53. These results suggested that aconitine can inhibit phosphorylation of MAPK and the toxic effect of aconitine on SH-SY5Y cells was related to MAPK signaling pathways.
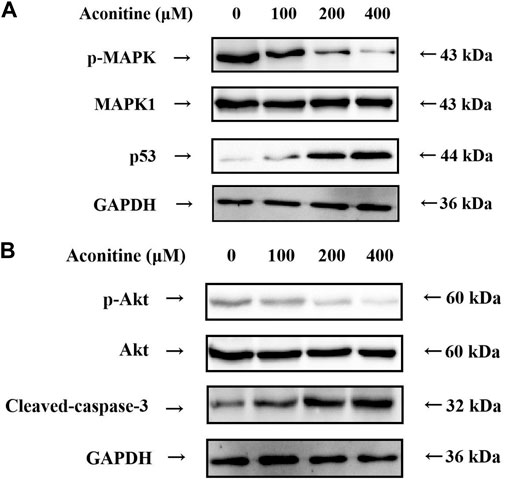
FIGURE 7. Western blot analysis after 48 h aconitine treatment. (A) Effects of aconitine on the protein secretion of MAPK pathway in SH-SY5Y cells. (B) Effects of aconitine on the secretory levels of p-Akt, Akt and Cleaved-caspase-3 in SH-SY5Y cells.
Aconitine affects the expression of the core proteins
Molecular docking results showed that Akt1 and Caspase-3 proteins were the core proteins of neurotoxicity caused by aconitine. Therefore, we assessed the expression level of p-Akt, Akt and Caspase-3 by western blot. As shown in Figure 7B, treatment of SH-SY5Y cells with aconitine (100, 200, 400 μM) led to apparent repression of phosphorylation level of Akt in a dose-dependent manner, with the expression of Akt remaining unchanged. What’s more, aconitine promoted the expression of Caspase-3. The results indicated that the mechanism of neurotoxicity induced by aconitine was associated with inhibition of Akt phosphorylation and promotion of apoptosis.
Aconitine increases the release of LDH
LDH is a stable cytoplasmic enzyme and the release of LDH is a key feature of the plasma membrane damage. Compared with the control group, aconitine at different concentrations (25, 50, 100, 200, 300 and 400 μM) significantly increased the release of LDH in Figure 8A, which suggested that cell membrane damage was an important toxic effect of aconitine and might be a key pathway for other exogenous substances entering the cell.
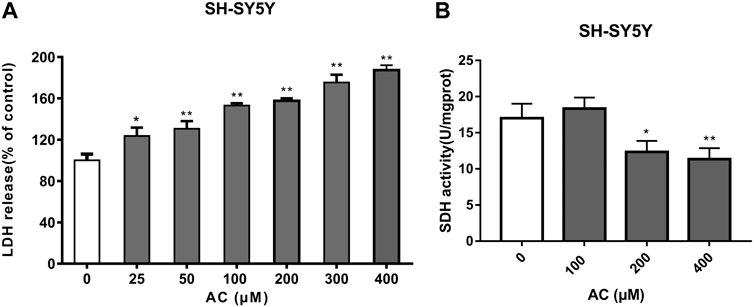
FIGURE 8. Effects of aconitine on mitochondrial function. (A) The release of LDH of SH-SY5Y cells after 24 h aconitine treatment. (*p < 0.05, **p < 0.01 versus control group.) (B) The SDH activity of SH-SY5Y cells after 24 h aconitine treatment. (*p < 0.05, **p < 0.01 versus control group).
Aconitine reduces SDH activity
SDH, belonging to cytochrome oxidase, is the only multi-subunit enzyme integrated on the membrane in the TCA cycle. It can provide electrons for the respiratory chain of mitochondrial oxygen demand and productivity, and is a marker enzyme of mitochondrial. As shown in Figure 8B, aconitine at 200 and 400 μM for 24 h significantly decreased SDH activity. Under the action of aconitine, the activity of SDH in nerve cells decreased at high dose, and the dose-effect relationship was obvious.
Aconitine reduces the number of normal hippocampal neurons
The Nissl Staining result is shown in Figure 9, we can observe that the structure of Nissl’s body in control group is clear, the basophilic granules distribute evenly and the number is more. However, the number of Nissl’s body in experimental group is less than the normal group, and there are a large number of basophilic granules gathering which is caused by numerous Nissl’s bodies dissolving.
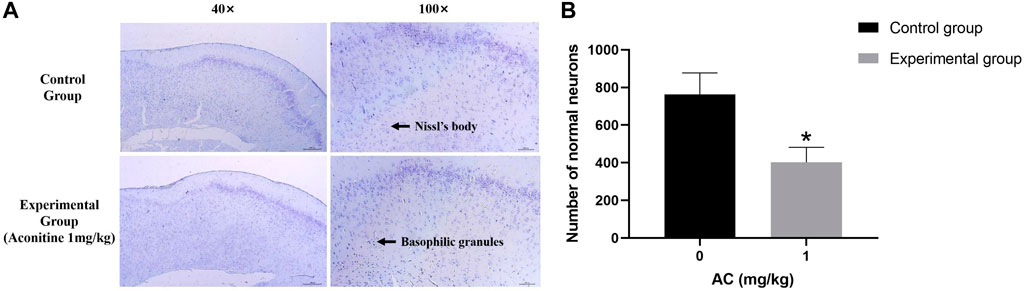
FIGURE 9. Treatment of aconitine for 7 days reduced the activity of hippocampus neurons. (A) Representative images magnified 40 and 100 times in hippocampus. (B) Quantitatively analyzed the number of Nissl’s bodies in control and experimental group (×100). (*p < 0.05 versus control group).
Aconitine induces apoptosis of hippocampus neurons
Compared with the control group, we can see a large number of TUNEL positive cells (brown) in the experimental group obviously. Its expression area is wider and its dyeing is heavier (Figure 10). The result illustrates that aconitine can promote apoptosis of hippocampus neurons.
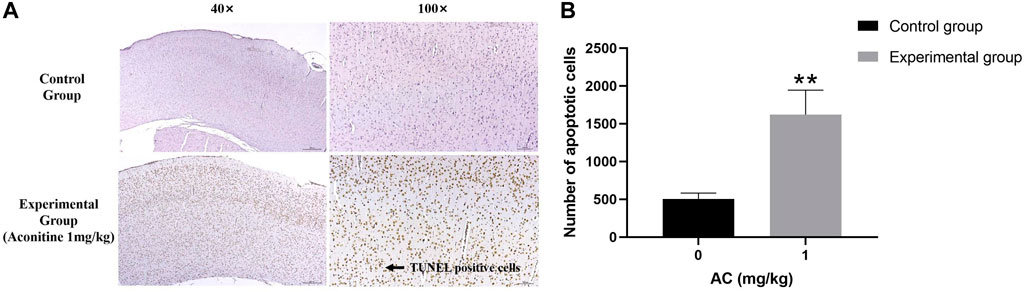
FIGURE 10. Treatment of aconitine for 7 days increased apoptosis of hippocampus neurons. (A) Representative images magnified 40 and 100 times in hippocampus. (B) Quantitatively analyzed the number of apoptotic cells in control and experimental group (×100). (**p < 0.01 versus control group.)
Discussion
Based on network pharmacology, molecular docking and experimental verification, this study systematically analyzed the potential mechanism of neurotoxicity caused by Fuzi, constructed the relationship network between candidate toxic compounds and neurotoxicity targets, and predicted the main toxic substances, potential targets and signaling pathways.
Network pharmacology analysis showed that uracil, palmitic acid and aconitine might play a crucial role in the neurotoxicity of Fuzi. Uracil is an important component in RNA, and fluorouracil, an antitumor drug with similar structure, has long been reported to have obvious neurotoxicity (Pirzada and AliDafer, 2000). Palmitic acid, as a long-chain saturated fatty acid, is an important component of blood lipids. Studies have shown that palmitic acid induces apoptosis by increasing oxidative stress in nerve cells, thereby producing neurotoxicity (Ng and Say, 2018). In addition, aconitine is considered to be the main toxic substance of Fuzi, Chuanwu and Caowu, and its toxic effects have been widely studied. The above existing research results are basically consistent with the prediction results, suggesting that the main toxic substances of Fuzi are closely related to its neurotoxicity.
The result of PPI protein interaction network analysis showed that the potential target genes for neurotoxicity of Aconite were ALB, AKT1, TP53, IL6, TNF, INS, CASP3, IL1B, EGFR and BDNF. AKT1 is the core target of PI3K/AKT signaling pathway. MAPK1, TP53, TNF, EGFR, INS, CASP3, IL1B and BDNF are related to MAPK signaling pathway. GO function and KEGG pathway enrichment analysis also confirmed that MAPK signaling pathway played an important role in the neurotoxicity induced by Fuzi.
Molecular docking results displayed that aconitine, the main toxic substance of Fuzi, exhibited good affinity with key targets ALB, AKT1 and CASP3, and the conformation of the binding site was stable. Based on the above results, the main toxic substance aconitine was selected for subsequent experimental verification, and its potential mechanism was discussed in depth.
The results of WB assay confirmed that aconitine affects the transmission of MAPK signaling pathway by inhibiting the MAPK protein phosphorylation and promoting the expression of p53. In KEGG PATHWAY database (https://www.genome.jp/kegg/pathway.html), we found that MAPK and p53 proteins are mainly involved in the classical MAPK pathway, JNK and p38 MAPK pathwayand p53 signaling pathway, and we suspected they are the main signaling pathways of neurotoxicity caused by Fuzi.
At the same time, the WB results showed that aconitine can inhibit the phosphorylation of the key protein Akt and promote the expression of Cleaved-caspase-3. Akt is the core protein of PI3K-Akt signaling pathway, and can control a variety of downstream signaling pathways, therefore, by adjusting the phosphorylation of Akt, Fuzi affects PI3K-Akt signaling pathway and controls various signaling pathways to cause neurotoxicity. And Cleaved-caspase-3, a kind of cysteine protease, is one of the key enzymes in the cell apoptosis pathways. Fuzi can increase its expression, and then promote the apoptosis of nerve cells in the resulting in neurotoxicity.
Aconitine can also increase LDH release and reduce SDH activity. The increase in LDH release suggests that aconitine can destroy the integrity of neuronal cell membrane, and the decrease in SDH activity suggests that aconitine leads to mitochondrial damage, while the damage of cell membrane and mitochondrial dysfunction are the characteristics of irreversible cell damage (Grimm, 2013). Aconitine may affect mitochondrial energy metabolism by inhibiting ATP production and aerobic respiratory function in nerve cells, and destroy the integrity of the cell membrane, leading to the entry of exogenous substances, thereby causing toxicity to nerve cells.
Mitochondrial dysfunction can lead to energy deficiency, which is an important mechanism for neurotoxicity (Liu et al., 2021). Early studies have found that mitochondrial dysfunction is associated with MAPK signaling pathway, leading to neuronal apoptosis and aggravating brain injury (Kleefstra et al., 2011; Gui et al., 2020; Manikanta et al., 2020). The relationship between mitochondrial function and MAPK signaling pathway needs further study. And appropriate targeted regulation of mitochondria may be a new direction to avoid neurotoxicity of Fuzi.
The hippocampus is the most vulnerable areas of the brain and plays an important role in learning function impairment, memory loss and cognitive dysfunction (Knierim, 2015). Apoptosis is the main cause of hippocampus damage. This research verifies that aconitine has toxicity to the nervous system. As shown by the results of Nissl Staining and TUNEL assay, we can find that aconitine produces neurotoxicity by promoting apoptosis of rat hippocampus neurons and reducing the number of neurons.
In fact, the mechanism of these pathways is not a single work, but the interaction influence causes neurotoxicity of Fuzi. However, these signaling pathways also play an important role in treatment in addition to inducing toxicity (Yang et al., 2020; Chen et al., 2022). We are unable to completely block the pathways to avoid the toxicity of Fuzi, and a more effective approach is to remove the toxic components by processing.
Earlier studies have demonstrated that the toxicity of Fuzi mainly derives from diester diterpenne alkaloids including aconitine (Singhuber et al., 2009), which has been confirmed by molecular docking in this article. In the clinic, we can boil Fuzi in water for a long period of time, transforming hypaconitine into monoester-diterpenoid alkaloids and finally into unesterified compounds, which has no toxicity and no influence on its pharmacological activities (Lu et al., 2010; Zhou et al., 2015).
In our study, some drawbacks should be noted. Our research only contains the toxic components that have been confirmed at present and whether there are other toxic compounds in Fuzi needs to be further studied. In addition, most of the Chinese herbal medicine plays a role in treatment by the form of oral, so its efficacy and toxicity are closely related to metabolites. Unfortunately, our research didn’t predict all the bioactive metabolites of Fuzi in vivo (Feng et al., 2021).
In recent years, gut microbiota has emerged as a new frontier to understand the development and progress of diseases, especially it can influence the development and diseases of the central nervous system along “microbiota-brain-gut axis” (Quigley, 2017; Strandwitz, 2018). Arachic acid, a composition of Fuzi, is reported to be able to modulate the composition of gut microbiota (Zhuang et al., 2017; Sun et al., 2021). And whether Fuzi can cause neurotoxicity by influencing the gut microbiota still needs to be further discussed.
Conclusion
This research analyzed the potential mechanism of neurotoxicity caused by Fuzi through building the relationship network of “compounds - targets - neurotoxicity” using network pharmacology and molecular docking. The results showed that aconitine, the core toxic compound of Fuzi, caused neurotoxicity through multiple targets and multiple ways, including MAPK signaling pathway, pathways related to Akt protein, destroying cell membrane integrity, damaging mitochondrial function and affecting energy metabolism and cell apoptosis. At the same time, aconitine can promote apoptosis of hippocampus neuron and decrease its quantity, thus producing neurotoxicity. Our research provided reference for clinical application and related research.
Data availability statement
The datasets presented in this study can be found in online repositories. The names of the repository/repositories and accession number(s) can be found in the article/Supplementary material.
Ethics statement
The animal study was reviewed and approved by Animal Care and Use Committee of Chengdu University of Tranditional Chinese Medicine. Written informed consent was obtained from the individual(s) for the publication of any potentially identifiable images or data included in this article.
Author contributions
This manuscript was conceptualized by all the authors. JA conceived ideas, completed the network pharmacology and molecular docking experiments and drafted original draft preparation; HF and MH conducted the experimental verification; JX, FP, and CP administrated funding and revised the manuscript. All authors have read and agreed to the published version of the manuscript.
Funding
The study was supported by National Natural Science Foundation of China (no. 82003879 and U19A2010), the Key Project of Science and Technology Department of Sichuan Province (no. 2020YFS0053 and 2021YFS0044), and Youth Talent Promotion Project of China Association for Science and Technology (CACM-2020-QNRC1-01), Project of State Administration of Traditional Chinese Medicine of China (ZYYCXTD-D-202209) and the Open Research Fund of Chengdu University of Traditional Chinese Medicine Key Laboratory of Systematic Research of Distinctive Chinese Medicine Resources in Southwest China.
Conflict of interest
The authors declare that the research was conducted in the absence of any commercial or financial relationships that could be construed as a potential conflict of interest.
Publisher’s note
All claims expressed in this article are solely those of the authors and do not necessarily represent those of their affiliated organizations, or those of the publisher, the editors and the reviewers. Any product that may be evaluated in this article, or claim that may be made by its manufacturer, is not guaranteed or endorsed by the publisher.
References
Chan, T. Y. (2009). Aconite poisoning. Clin. Toxicol. 47 (4), 279–285. doi:10.1080/15563650902904407
Chen, L., Zhang, C., Cao, J., Bei, G., Wang, X., and Miao, Z. (2022). Yiyi fuzi baijiang decoction alleviates ulcerative colitis partly by regulating TLR4-mediated PI3K/Akt and NF-κB pathways. Evid. Based. Complement. Altern. Med. 2022, 8780514. doi:10.1155/2022/8780514
Feng, W., Liu, J., Zhang, D., Tan, Y., Cheng, H., and Peng, C. (2021). Revealing the efficacy-toxicity relationship of Fuzi in treating rheumatoid arthritis by systems pharmacology. Sci. Rep. 11 (1), 23083. Published 2021 Nov 29. doi:10.1038/s41598-021-02167-5
Grimm, S. (2013). Respiratory chain complex II as general sensor for apoptosis. Biochim. Biophys. Acta 1827 (5), 565–572. doi:10.1016/j.bbabio.2012.09.009
Gui, C., Ren, Y., Chen, J., Wu, X., Mao, K., Li, H., et al. (2020). p38 MAPK-DRP1 signaling is involved in mitochondrial dysfunction and cell death in mutant A53T α-synuclein model of Parkinson's disease. Toxicol. Appl. Pharmacol. 388, 114874. doi:10.1016/j.taap.2019.114874
Hopkins, A. L. (2007). Network pharmacology. Nat. Biotechnol. 25 (10), 1110–1111. doi:10.1038/nbt1007-1110
Hsin, K. Y., Ghosh, S., and Kitano, H. (2013). Combining machine learning systems and multiple docking simulation packages to improve docking prediction reliability for network pharmacology. PLoS One 8 (12), e83922. doi:10.1371/journal.pone.0083922
Kleefstra, T., Wortmann, S. B., Rodenburg, R. J., Bongers, E. M. H. F., Hadzsiev, K., Noordam, C., et al. (2011). Mitochondrial dysfunction and organic aciduria in five patients carrying mutations in the Ras-MAPK pathway. Eur. J. Hum. Genet. 19 (2), 138–144. doi:10.1038/ejhg.2010.171
Knierim, J. J. (2015). The hippocampus. Curr. Biol. 25 (23), R1116–R1121. doi:10.1016/j.cub.2015.10.049
Li, S., and Zhang, B. (2013). Traditional Chinese medicine network pharmacology: Theory, methodology and application. Chin. J. Nat. Med. 11 (2), 110–120. doi:10.1016/S1875-5364(13)60037-0
Liu, H., Ho, P. W., Leung, C. T., Pang, S. Y. Y., Chang, E. E. S., Choi, Z. Y. K., et al. (2021). Aberrant mitochondrial morphology and function associated with impaired mitophagy and DNM1L-MAPK/ERK signaling are found in aged mutant Parkinsonian LRRK2R1441G mice. Autophagy 17 (10), 3196–3220. doi:10.1080/15548627.2020.1850008
Lu, G., Dong, Z., Wang, Q., Qian, G., Huang, W., Jiang, Z., et al. (2010). Toxicity assessment of nine types of decoction pieces from the daughter root of Aconitum carmichaeli (Fuzi) based on the chemical analysis of their diester diterpenoid alkaloids. Planta Med. 76 (8), 825–830. doi:10.1055/s-0029-1240688
Manikanta, K., Naveen Kumar, S. K., Hemshekhar, M., Kemparaju, K., and Girish, K. S. (2020). ASK1 inhibition triggers platelet apoptosis via p38-MAPK-mediated mitochondrial dysfunction. Haematologica 105 (8), e419–e423. doi:10.3324/haematol.2019.233908
National Research Council (Us), (2011). Committee for the update of the guide for the Care and use of laboratory animals. Guide for the Care and use of laboratory animals. 8th ed. Washington (DC): National Academies Press US.
Ng, Y. W., and Say, Y. H. (2018). Palmitic acid induces neurotoxicity and gliatoxicity in SH-SY5Y human neuroblastoma and T98G human glioblastoma cells. PeerJ 6, e4696. doi:10.7717/peerj.4696
Pirzada, N. A., Ali, , and Dafer, R. M. (2000). Fluorouracil-induced neurotoxicity. Ann. Pharmacother. 34 (1), 35–38. doi:10.1345/aph.18425
Quigley, E. M. M. (2017). Microbiota-brain-gut Axis and neurodegenerative diseases. Curr. Neurol. Neurosci. Rep. 17 (12), 94. doi:10.1007/s11910-017-0802-6
Singhuber, J., Zhu, M., Prinz, S., and Kopp, B. (2009). Aconitum in traditional Chinese medicine: A valuable drug or an unpredictable risk? J. Ethnopharmacol. 126 (1), 18–30. doi:10.1016/j.jep.2009.07.031
Strandwitz, P. (2018). Neurotransmitter modulation by the gut microbiota. Brain Res. 1693, 128–133. doi:10.1016/j.brainres.2018.03.015
Sun, Y., Wu, D., Zeng, W., Chen, Y., Guo, M., Lu, B., et al. (2021). The role of intestinal dysbacteriosis induced arachidonic acid metabolism disorder in inflammaging in atherosclerosis. Front. Cell. Infect. Microbiol. 11, 618265. Published 2021 Mar 18. doi:10.3389/fcimb.2021.618265
Yang, J., Ma, W., Mei, Q., Song, J., Shu, L., Zhang, S., et al. (2020). Protective effect of fuzi lizhong decoction against non-alcoholic fatty liver disease via anti-inflammatory response through regulating p53 and PPARG signaling. Biol. Pharm. Bull. 43 (11), 1626–1633. doi:10.1248/bpb.b20-00053
Yang, M., Ji, X., and Zuo, Z. (2018). Relationships between the toxicities of Radix aconiti Lateralis preparata (fuzi) and the toxicokinetics of its main diester-diterpenoid alkaloids. Toxins (Basel) 10 (10), 391. doi:10.3390/toxins10100391
Zhang, R., Zhu, X., Bai, H., and Ning, K. (2019). Network pharmacology databases for traditional Chinese medicine: Review and assessment. Front. Pharmacol. 10, 123. doi:10.3389/fphar.2019.00123
Zhao, L., Sun, Z., Yang, L., Cui, R., Yang, W., and Li, B. (2020). Neuropharmacological effects of aconiti Lateralis Radix Praeparata. Clin. Exp. Pharmacol. Physiol. 47 (4), 531–542. doi:10.1111/1440-1681.13228
Zhou, G., Tang, L., Zhou, X., Wang, T., Kou, Z., and Wang, Z. (2015). A review on phytochemistry and pharmacological activities of the processed lateral root of Aconitum carmichaelii Debeaux. J. Ethnopharmacol. 160, 173–193. doi:10.1016/j.jep.2014.11.043
Zhuang, P., Shou, Q., Lu, Y., Wang, G., Qiu, J., Wang, J., et al. (2017). Arachidonic acid sex-dependently affects obesity through linking gut microbiota-driven inflammation to hypothalamus-adipose-liver axis. Biochim. Biophys. Acta. Mol. Basis Dis. 1863 (11), 2715–2726. doi:10.1016/j.bbadis.2017.07.003
Keywords: fuzi, neurotoxicity, network pharmacology, molecular docking, aconitine
Citation: An J, Fan H, Han M, Peng C, Xie J and Peng F (2022) Exploring the mechanisms of neurotoxicity caused by fuzi using network pharmacology and molecular docking. Front. Pharmacol. 13:961012. doi: 10.3389/fphar.2022.961012
Received: 03 June 2022; Accepted: 08 August 2022;
Published: 30 August 2022.
Edited by:
Zhichao Xu, Northeast Forestry University, ChinaReviewed by:
Jinqi Li, Personalized Drug Therapy Key Laboratory of Sichuan Province, ChinaYun Qi, Chinese Academy of Medical Sciences and Peking Union Medical College, China
Copyright © 2022 An, Fan, Han, Peng, Xie and Peng. This is an open-access article distributed under the terms of the Creative Commons Attribution License (CC BY). The use, distribution or reproduction in other forums is permitted, provided the original author(s) and the copyright owner(s) are credited and that the original publication in this journal is cited, in accordance with accepted academic practice. No use, distribution or reproduction is permitted which does not comply with these terms.
*Correspondence: Fu Peng, ZnVqaW5nMTI2QHllYWgubmV0; Cheng Peng, cGVuZ2NoZW5nY2hlbmdkdUAxMjYuY29t; Jie Xie, amlleGllQHNpY251LmVkdS5jbg==