- Department of Clinical Pharmacy, The First Affiliated Hospital of Shandong First Medical University & Shandong Provincial Qianfoshan Hospital, Jinan, China
A wound occurs when the epidermis and dermis of the skin are damaged internally and externally. The traditional wound healing method is unsatisfactory, which will prolong the treatment time and increase the treatment cost, which brings economic and psychological burdens to patients. Therefore, there is an urgent need for a new method to accelerate wound healing. As a cell-free therapy, exosome derived from stem cell (EdSC) offers new possibilities for wound healing. EdSC is the smallest extracellular vesicle secreted by stem cells with diameters of 30–150 nm and a lipid bilayer structure. Previous studies have found that EdSC can participate in and promote almost all stages of wound healing, including regulating inflammatory cells; improving activation of fibroblasts, keratinocytes, and endothelial cells; and adjusting the ratio of collagen Ⅰ and Ⅲ. We reviewed the relevant knowledge of wounds; summarized the biogenesis, isolation, and identification of exosomes; and clarified the pharmacological role of exosomes in promoting wound healing. This review provides knowledge support for the pharmacological study of exosomes.
Introduction
The skin is the largest multifunctional organ in the body. It can prevent bacterial invasion and resist chemical and physical assaults by forming a strong barrier between the organism and the environment (Proksch et al., 2008; Wang et al., 2019). A wound is a disruption of normal anatomic structure and function because of internal and external breakage of the epidermis and dermis and occurs when the skin is torn and burned and has pressure ulcer and diabetes ulcer (Reinke and Sorg, 2012; Kanji and Das, 2017; Ayavoo et al., 2021). A prompt and suitable wound healing is necessary for the repair of functional tissues as well as disordered structures after an injury (Gurtner et al., 2008; Ayavoo et al., 2021). Normal wound healing is a sophisticated and dynamic activity that involves many physiological activities, such as inflammation, cell proliferation, and extracellular matrix remodeling (Wang et al., 2018). Chronic wounds fail to heal orderly and timely through normal healing mechanisms (Velnar et al., 2009), which are characterized by long healing time and scar hyperplasia.
According to the statistics, chronic wounds affect more than 6 million people. It is anticipated that the number of people with chronic wounds will increase among our growing elderly and diabetes population (Powers et al., 2016). Chronic nonhealing wounds will make patients experience serious pain and become physically anxious (Järbrink et al., 2017), which would bring strong pressure on society (Järbrink et al., 2017). Therefore, the development of novel technologies and practices in the best practice clinical management of chronic wounds is imperative to diminish the possible burdens on the health and economy of the society and optimize the healthcare management for this prospective silent pandemic.
Conventional wound care methods include wound dressings (Han and Ceilley, 2017), skin substitutes (Dai et al., 2020), and growth factors (Shpichka et al., 2019). However, the drawbacks of these methods such as long healing time, immune rejection, high cost, and easy infection (Goodarzi et al., 2018; Vu et al., 2021) limit their application. In recent years, stem cells for wound healing have become one of their most important tools because of their strong self-renewal and differentiation ability (Nourian Dehkordi et al., 2019). Studies have confirmed that the effect of stem cell therapy has to do with the paracrine effect mediated by stem cell secretory factor exosomes (Yang J. et al., 2020). The exosome is the smallest extracellular vesicle, which is released into the extracellular environment after the fusion of late endosomes with the plasma membrane (Hessvik and Llorente, 2018). Exosome derived from stem cell (EdSC) is secreted by stem cells, which can transfer functional cargos such as proteins, DNA, and RNA from donor cells to the recipient cells (Nikfarjam et al., 2020; Gurung et al., 2021) and mediate intercellular communication to promote the activities of wound healing–related cells, such as fibroblasts and keratinocytes (Arishe et al., 2021). In this review, we reported the biogenesis, isolation, and identification of exosomes; elaborated on the mechanism of exosomes promoting wound healing; and discussed the clinical trials of exosomes in the treatment of wound healing.
Representative therapeutics for wound healing
Wounds are disruption of normal anatomic structure and function because of internal and external breakage of the epidermis and dermis (Reinke and Sorg, 2012; Kanji and Das, 2017; Ayavoo et al., 2021) (Figure 1). Current strategies for wound healing include wound dressings (Han and Ceilley, 2017), skin substitutes (Dai et al., 2020), and growth factors (Shpichka et al., 2019).
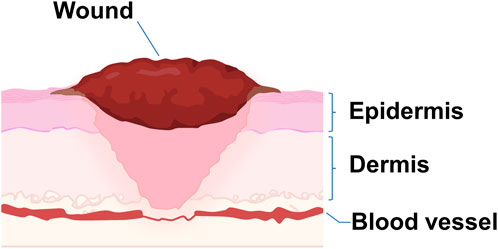
FIGURE 1. Schematical illustration of a wound. A wound occurs when the dermis and epidermis of the skin, as well as blood vessels, are damaged.
Wound dressings
Wound dressing is a sterile pad that is used in direct contact with the injury (Tang et al., 2021), which can keep a local moist environment around the wound, protect the wound from micro-organisms, and sustain good gas transmission (Kamoun et al., 2017). Common wound dressings include cotton gauze, human amniotic membrane, and polysaccharide-based factors (Zeng et al., 2018). However, traditional wound dressings are limited in providing a proper sterile environment for wounds (Farahani and Shafiee, 2021).
Skin substitutes
Skin substitutes are heterogenous biomaterials that can provide a substitute for the extracellular matrix to accelerate the healing process of wounds (Auger et al., 2009; Dai et al., 2020). According to different sources, it can be divided into allografts of human origin and xenografts of animal origin (FerreiraCastropaggiaro et al., 2011). This way provides a physical barrier from bacteria (Wei et al., 2022) and trauma and can keep a moist microenvironment in the wound bed (Dai et al., 2020), but allogenic skin grafts have the risk of immune rejection (Iy and Al-Rubaiy, 2009).
Growth factors
Growth factors can affect the microenvironment in the wound bed when released. (Dolati et al., 2020), such as promoting intercellular communication, including endothelial cells and fibroblasts (Werner and Grose, 2003). Although the direct application of growth factors is beneficial to wound healing, it also has certain limitations. For example, under the action of protein hydrolases, growth factors will degrade quickly (Golchin et al., 2018).
Recent emerging novel tool: exosome derived from stem cell
Stem cells play an important role in wound healing and skin regeneration because of their strong self-renewal and differentiation ability (Mazini et al., 2020; Guillamat-Prats, 2021; Jo et al., 2021). The main tissue sources of stem cells for wound healing and skin regeneration include fat, bone marrow, and umbilical cord. In the process of wound treatment, using stem cells can close the wound early and reduce scar formation (Isakson et al., 2015; Guillamat-Prats, 2021). Of note, many studies have reported the effect of mesenchymal stem cells (MSCs) in wounds and regenerative medicine through their paracrine factors such as exosomes.
The emergence of EdSC offers new possibilities for wound healing. As a cell-free therapy, EdSC overcomes the limitations of stem cells. EdSC therapy is easy to use and time-saving and has low immune rejection (Ha et al., 2020). Studies have found that EdSC can induce benefits in nearly all phases of wound healing. For instance, it can inhibit inflammation, control immune responses, and promote cell proliferation and angiogenesis (He et al., 2019; Chen Md et al., 2021).
Biogenesis of exosome derived from stem cell
Exosomes are a subset of extracellular vesicles that are secreted by the majority of the types of cell-like dendritic cells, T cells, stem cells, and a variety of cancer cells (Isaac et al., 2021). According to the biogenesis and size, extracellular vesicles can be divided into exosomes, microvesicles, and apoptotic bodies (Crescitelli et al., 2013; Thakur et al., 2021). The diameter of exosomes is 30–150 nm, which is the smallest extracellular vesicle (Raposo and Stoorvogel, 2013). The release of exosomes occurs via three major steps (Figure 2): 1) Cell membrane invaginates to form primary endosomes, and the early endosomes are acidified into late endosomes. 2) The late endosomes bud inward to form a multivesicular body (MVB) (Tiwari A. et al., 2021). 3) Exosomes are released into the extracellular environment after the fusion of MVB and plasma membrane by exocytosis (Huotari and Helenius, 2011; Van Niel et al., 2018; Gurunathan et al., 2021). The exosomes secreted by stem cells such as adipose-derived stem cells and umbilical cord MSCs are EdSCs (Yu et al., 2014). EdSC contains many biomolecules of donor stem cells, such as DNA, RNA, nucleic acid, lipids, metabolites, and cytosolic (Kalluri and Lebleu, 2020; Gurung et al., 2021). Surrounded by lipid bilayers, EdSC can regulate cell–cell communication by transferring a lot of functional biomolecules to recipient cells (Tran et al., 2020; An et al., 2021; Arishe et al., 2021). As the main paracrine factors of stem cells, many studies established that EdSCs are also involved in the immune response (Hodge et al., 2021; Dai et al., 2022), cancer prevention and treatment (Jiang et al., 2022; Nik Nabil et al., 2022), antigen presentation (You et al., 2021; Zheng et al., 2022), angiogenesis (Yang et al., 2021), drug delivery (Riau et al., 2019; Ding et al., 2022; Rao et al., 2022), and inflammation (Shen et al., 2021). Multiple pharmacological effects make EdSC attract much attention in enhancing skin wound healing (Las Heras et al., 2020; Al Gailani et al., 2022; Dong et al., 2022; Opoku-Damoah et al., 2022).
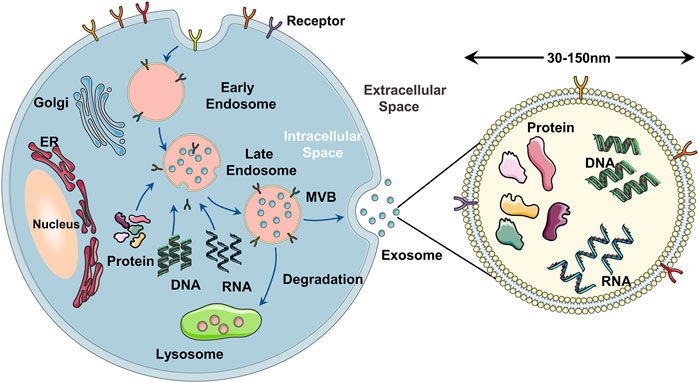
FIGURE 2. Schematical illustration of the exosome biogenesis. (A) the cell membrane forms early endosomes in the form of endocytosis. (B) the early endosomes mature into MVB containing exosomes after further acidification. (C) the MVB fuses with the cell membrane and releases exosomes into the extracellular space in the form of exocytosis.
Technologies for isolating exosome derived from stem cell
The isolation of pure EdSC is critical to understanding its mechanism and application to wound healing. Conventional methods include ultracentrifugation-based techniques, size-based techniques, and immunoaffinity capture-based techniques (Wu et al., 2019).
(1) For ultracentrifugation-based techniques: Ultracentrifugation-based techniques are known as the “gold standard” for EdSC isolation technology (Yang D. et al., 2020), which is according to the difference in size and density of each constituent in mixture solution (Livshits et al., 2015; Saad et al., 2021). Ultracentrifugation can be divided into density gradient ultracentrifugation and differential ultracentrifugation (Konoshenko et al., 2018; Gurunathan et al., 2019). Differential ultracentrifugation is easy to operate and low in cost (Zhao R. et al., 2021). However, compared with differential centrifugation, the purity of EdSC isolated by density gradient centrifugation is higher (Shirejini and Inci, 2021; Tarasov et al., 2021).
(2) For size-based techniques: Size exclusion chromatography is a typical technology for separation based on the size of exosomes. The sample containing exosomes flows through a stationary phase column filled with a porous matrix. The sample molecules smaller than the pore size can diffuse into the matrix and need a longer time to pass through the column, whereas large molecules get eluted faster (Tiwari S. et al., 2021). This method cannot distinguish EdSC and microvesicles of the same size, and the yield of exosomes is low. However, it is quick, easy, and cheap, and the isolated EdSCs are uniform in size and intact biophysically and functionally (Heydari et al., 2021; Purghe et al., 2021; Saad et al., 2021).
(3) For immunoaffinity capture-based techniques: Immunoaffinity-based isolation strategies use antibodies that were embedded with different materials such as magnetic beads (Kandimalla et al., 2021) to target the specific surface antigens of exosomes (Li S. et al., 2021). Then, antibody-recognized exosomes are captured (Alzhrani et al., 2021). This method can evidently increase the purity of EdSC and save time and samples of isolation (Li S. et al., 2021). However, the defect of this method is that it is not suitable for the isolation of large sample volumes (Alzhrani et al., 2021). Moreover, it only works with cell-free samples and isolates EdSC with low yield (Fu et al., 2019).
Tools for identifying exosome derived from stem cell
Once EdSCs are isolated, they need to be further quantified and analyzed (Li S. et al., 2021). According to the International Society of Extracellular Vesicles, the identification techniques of EdSCs can be based on morphology, size, and specific proteins on the surface of exosomes (Thery et al., 2018), such as electron microscope, nanoparticle tracking analysis (NTA), and western blot.
(1) For electron microscope: Electron microscopy techniques have been widely used to detect the morphology and size of EdSC (Alzhrani et al., 2021). It mainly includes a cryo-electron microscope and a transmission electron microscope (TME). In TME, two electron beams pass through the samples and are subsequently collected and magnified (Liu Q. et al., 2021). However, EdSCs show a saucer-like structure under TME (Zhao R. et al., 2021). Many researchers attribute this phenomenon to the collapse of samples caused by drying during sample processing (Cizmar and Yuana, 2017). Unlike TME, EdSCs detected using a cryo-electron microscope are round (Jin et al., 2021). This technique is now widely used since the destruction of the sample is avoided.
(2) For NTA: NTA can identify the dimension as well as the concentration of EdSC (Zara et al., 2020). The Brownian motion of suspended particles and light scattering are the basic principles of NTA (Pelissier Vatter et al., 2020; Zhao R. et al., 2021). By viewing in the mind each very small bit through image observations using either distributed widely light or gave out fluorescence, NTA measures the Brownian motion of person EdSC and connects it to a very small bit size (Carnino et al., 2019; Alzhrani et al., 2021). The advantages of this method include high detection sensitivity, easy sample preparation, fast analysis speed, and suitability for a large number of samples (Zara et al., 2020; Singh et al., 2021). However, this technique is accompanied by the problems of poor sensitivity, low efficiency of sorting targeted EdSC, and poor reproducibility (Jin et al., 2021).
(3) For western blot: Western blot identifies EdSC based on the specific proteins on the surface. Specific proteins (such as CD9, CD81, and CD63) are separated by electrophoresis and then combined with the corresponding antibodies (Zhao et al., 2019; Jalaludin et al., 2021). This identification method has high sensitivity and specificity (Singh et al., 2021). However, workflow is prolonged (Singh et al., 2021).
A promising therapeutics, exosome derived from stem cell, for wound healing
EdSC can be administered to the wound through intravenous injection and subcutaneous injection (Hu et al., 2016). After administration of EdSC to the wound site, it can induce benefits in almost all stages of wound healing, such as inhibiting inflammation by controlling immune cells, accelerating wound closure and angiogenesis by promoting proliferation and migration of cells, and reducing scar formation by regulating the proportion of collagen (Li D. et al., 2018; Wei et al., 2021). This part mainly discusses the delivery systems and mechanisms of EdSC that promote wound healing (Figure 3).
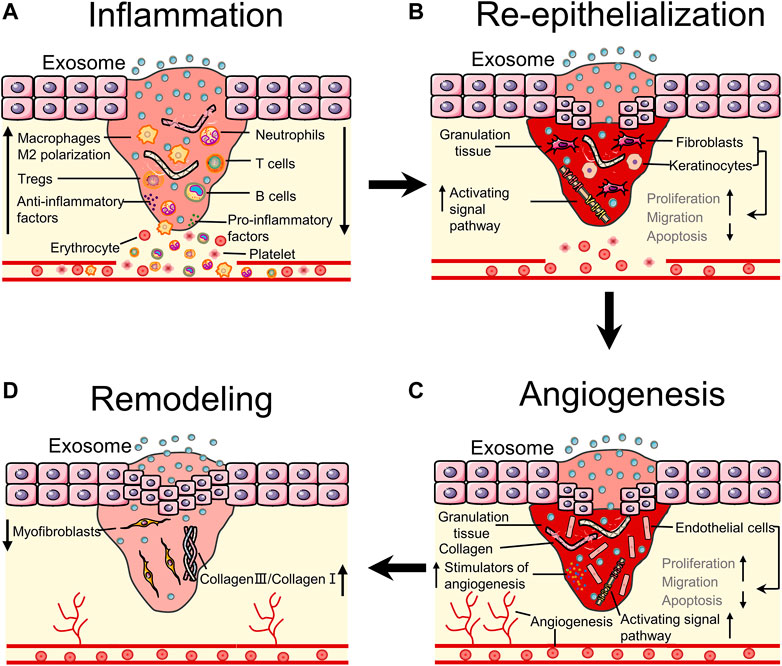
FIGURE 3. Bioeffects of stem cells derived exosomes on wound healing. (A) EdSC can inhibit inflammation by regulating the number of inflammatory cells and the polarization of macrophages. (B) EdSC can promote re-epithelialization by increasing the activity of fibroblasts as well as keratinocytes and activating pathways. (C) EdSC can improve angiogenesis by stimulating the release of angiogenic factors and regulating the activity of endothelial cells. (D) EdSC can improve tissue remodeling by regulating the ratio of collagen and myofibroblast differentiation.
Exosome derived from stem cell delivery systems for wound healing
The most common way of administration of EdSC is intravenous injection. For instance, delivering EdSC to the wound site of mice through intravenous injection can stimulate the activities of fibroblasts, to accelerate wound healing (Hu et al., 2016). Subcutaneous injection is another delivery method of EdSC for wound healing. Liu et al. injected melatonin-stimulated EdSCs subcutaneously into the wound and found that EdSCs enhance diabetic wound healing by regulating the polarization of macrophage M1 phenotype to M2 phenotype through targeting PTEN/AKT pathway (Liu et al., 2020). Although the method of direct injection seems more efficient, it is highly invasive (Kosaka et al., 2012; Akbari et al., 2020). Although injection is simple and effective, it can limit EdSC therapeutic function because the clearance rate of this route is relatively rapid (Takahashi et al., 2013). In recent years, many researchers have combined EdSC with hydrogel to prolong the efficacy time and improve the stability of EdSC, to accelerate wound healing. As a new dressing, a hydrogel is a three-dimensional structure formed by physical or chemical crosslinking between hydrophilic polymer chains (Zhao Y. et al., 2021; Ma and Wu, 2022; Safari et al., 2022). Hydrogels can load EdSC by absorbing a large amount of solution containing EdSC because of the hydrophilic functional groups in polymers (Xu et al., 2018; Golchin et al., 2022). Applying the EdSC-loaded hydrogel dressing to the wound bed, the hydrogel network can control the release concentration and time of EdSC and increase the moisture content of the wound (Kim et al., 2017; Shafei et al., 2020). It maintains a good microenvironment in the wound bed that supports the activities of loaded cells to accelerate wound healing (Kim et al., 2017; Riha et al., 2021). Hence, hydrogels are utilized as desirable therapeutic agents for EdSC on wound healing.
Inflammation
Inflammation is characterized by removing debris and preventing infection through activation and recruitment of resident immune cells, such as neutrophils, mast cells, and eosinophils (Wang et al., 2018). Accumulating evidence suggests that EdSCs inhibit the process of inflammation in various pathways. Studies have confirmed that MSC-exosomes can block the infiltration of neutrophils and reduce the number of neutrophils in wounds to prevent excessive inflammation (Li et al., 2016; Shojaati et al., 2019). In addition, MSC-exosomes from different sources reduce pro-inflammatory factors such as IL-1, IL-6, TNF-ɑ, IFN-γ, IL-17, TNF-ɑ, and IL-1β along with increase anti-inflammatory factors such as IL-10, TSG-6, IL-4, and TGF-β in wounds to accelerate the inflammatory process (Liu et al., 2014; Chen et al., 2016; Nojehdehi et al., 2018; Li K. L. et al., 2021; Shen et al., 2021). In the later stage of inflammatory, pro-inflammatory M1 macrophages are transformed into anti-inflammatory M2 macrophages, which can activate fibroblasts, keratinocytes, and endothelial cells to promote re-epithelialization as well as angiogenic processes (Rani and Ritter, 2016; Sorg et al., 2017). For one thing, Xu et al. found that under LPS stimulation, exosomes from BMSCs resulted in an increase in M2 macrophage polarization and a decrease in M1 macrophage polarization (Xu et al., 2019). For another thing, some researchers have found that stem cell–derived exosomes from different sources can also induce the polarization of macrophage M1 phenotype to M2 phenotype through various biological pathways. For example, exosomes derived from MSCs stimulated by melatonin have been shown to enhance diabetic wound healing by targeting the PTEN/AKT pathway (Liu et al., 2020). Meanwhile, Khare et al. demonstrated that BMSC-derived exosomes promote the inflammation process by decreasing the proliferation and activation of B cells (Khare et al., 2018). In addition, EdSC also can suppress inflammatory T cell proliferation (Blazquez et al., 2014; Di Trapani et al., 2016; Monguio-Tortajada et al., 2017), activate T cells into T regulatory cells (Chen et al., 2016), and increase the number and proliferation of Tregs (Del Fattore et al., 2015; Chen et al., 2016; Du et al., 2018; Nojehdehi et al., 2018; Zhang et al., 2018; Riazifar et al., 2019; Li K. L. et al., 2021). In the immune system, dendritic cells are the cells that present antigens that can enhance T cell proliferation (Xie et al., 2020). Reis et al. found that dendritic cells can be inhibited by exosome treatment, which indirectly inhibits T cell activity (Reis et al., 2018). Therefore, all evidence suggests that SCdEs have anti-inflammatory potential and the ability to prevent excessive inflammation.
Re-epithelialization
In the phase of re-epithelialization, fibroblasts proliferate and migrate in large numbers and produce and deposit ECM to form granulation tissues that replace initial fibrin clots and repair tissue losses (Wilkinson and Hardman, 2020). EdSCs were readily taken up by fibroblasts that stimulate cell activity to promote wound healing (Hu et al., 2016; Chen Md et al., 2021; Tutuianu et al., 2021). As a scaffold, granulation tissue supports migration as well as the proliferation of wound cells and promotes new angiogenesis. Meanwhile, fibroblasts can stimulate keratinocytes by secreting keratinocyte-derived growth factors, which can undergo a partial epithelial–mesenchymal transition (Sorg et al., 2017). Then, keratinocytes proliferate and migrate toward the wound center until contact with reverse cells stops (Han and Ceilley, 2017). EdSC can promote wound healing by regulating keratinocyte and fibroblast characteristics and enhancing re-epithelialization. In addition, ADSC-derived exosomes enhance keratinocyte activities by activating Wnt/β-catenin signaling, AKT/HIF-1alpha signaling, or AKT pathway to promote wound healing (Ma et al., 2019; Zhang et al., 2020). According to Chen et al., highly expressed microRNA-21 in ADSC-exosomes can increase the MMP-9 expression via the PI3K/AKT to promote the activity of the keratinocytes (Yang C. et al., 2020). Moreover, ADSC-exosomes inhibited miR-19b expression via lncRNA H19 (H19) and activated the Wnt/β-catenin pathway using upregulated SPY-related high-mobility group box 9 (SOX9), resulting in enhanced human skin fibroblast function (Miao et al., 2021; Qian et al., 2021). Human ADSC-exosomes contain lncRNA MALAT1, which is capable of increasing fibroblast migration in the dermis (Cooper et al., 2018). Jeffrey et al. suggested that CD63+ exosomes from BMSCs contribute to the transport exterior Wnt3a signal to recipient cells significantly, thereby promoting fibroblast and endothelial functions (Mcbride et al., 2017). In addition, the study also found that umbilical cord–derived MSC (uMSC)-exosomes contain microRNAs such as miR-21, miR-23a, and miR-125b, which can suppress the differentiation of fibroblasts into myofibroblasts formation via inhibiting collagen deposition (Mcbride et al., 2017) to accelerated re-epithelialization (Fang et al., 2016; Li D. et al., 2021). Taken together, the role of exosomes in promoting re-epithelialization is mainly achieved by enhancing the function of keratinocytes and fibroblasts.
Angiogenesis
Angiogenesis is another important process in the proliferative phase. Promoting angiogenesis is the main factor for stem cell–derived exosomes to promote wound healing. Angiogenesis provides oxygen, blood supply, and metabolic pathways for wound healing. Hypoxic environment after injury has induced the release of fibroblast growth factor 2 and vascular endothelial growth factor A. This stimulates vascular endothelial cell proliferation to build new blood vessels. TutuiaNu et al. demonstrated that exosomes stimulated endothelial cell migration via scratch test assay (Tutuianu et al., 2021). In addition, stimulators of angiogenesis such as angiopoietin-2 (Ang-2) and endothelin (ET-1) were found in EdSC (Tutuianu et al., 2021). Meanwhile, exosomes derived from human uMSCs can improve angiogenesis by delivering angiopoietin-2 to promote wound healing (Liu J. et al., 2021). Wounds in the feet of diabetic rats treated with exosomes from ADSCs overexpressing Nrf2 exhibited reduced ulcer area, granulation tissue formation, enhanced growth factor expression, and increased angiogenesis (Li X. et al., 2018). In recent years, it is reported that embryonic stem cell–derived exosomes can activate Nrf2 to improve endothelial senescence (Chen B. et al., 2019). Some studies have found that stem cell–derived exosomes can transfer RNA or protein, such as miR-125a (Liang et al., 2016), miR-31 (Kang et al., 2016), miR-21 (An et al., 2019), and DMBT1 protein (Chen et al., 2018), to promote angiogenesis for wound healing. Ding et al. demonstrated that exosomes from human BMSCs can stimulate angiogenesis by activating the PI3K/AKT signaling pathway in vitro (Ding et al., 2019). Signaling pathways with similar efficacy include AKT/eNOS pathway (Yu et al., 2020) and Wnt4/β-Catenin pathway (Zhang et al., 2015). As mentioned above, stem cell–derived exosomes accelerate wound healing via promoting angiogenesis.
Remodeling
In the remodeling stage, the primary task is to reduce scar formation. Uncontrolled accumulation of myofibroblasts that contract the wound and excessive proportion of collagen Ⅲ in the wound lead to scar formation (Zeng and Liu, 2021). In granulation tissue, collagen Ⅰ replaced collagen Ⅲ gradually to promote scar-free repair. In recent years, some studies observed the effects of exosomes on matrix remodeling. Liu et al. injected exosomes secreted by human ADSCs intravenously into murine incisional wounds and found that ADSC-exosomes can reconstruct ECM in wound bed by regulating the proportion of collagen-like type Ⅲ to type Ⅰ and reduce scar formation by regulating differentiation of fibroblast (Wang et al., 2017; Wang et al., 2021). MiR-192-5p expressing exosomes derived from human ADSCs can mitigate hypertrophic scar fibrosis by modulating the smad pathway. Its performance in wound healing is attenuated collagen deposition, transdifferentiation of fibroblasts to myofibroblasts, and formation of hypertrophic scars (Li Y. et al., 2021). In addition, microRNAs enriched in epidermal stem cell–derived exosomes (EPSC-exos) include miR-16, let-7a, miR-425-5p, and miR-142-3p (Duan et al., 2020). EPSC-exos-specific microRNAs, such as miR-425-5p and miR-142-3p, can reduce the TGF-β1 expression in dermal fibroblasts to inhibit myofibroblast differentiation (Duan et al., 2020). Furthermore, EdSC suppressed scar formation by reducing collagen deposition and regulating inflammation (Jiang et al., 2020). The findings of li et al. indicated that ADSCs-exosomes facilitate scar-free healing by enhancing the characteristics of fibroblasts (Hu et al., 2016; 2020). EdSC increased collagen Ⅰ and Ⅲ production through systemic administration at the initial stage of wound healing, whereas EdSC may inhibit collagen expression to reduce scar formation in the late stage (Hu et al., 2016). Taken together, EdSC can reduce scar formation by regulating the proportion of collagen.
Clinical applications of exosomes in wound healing therapy
The therapeutic potential of stem cells and EdSC for wound treatment have been conducted in various kinds of animal studies. It demonstrated that stem cell injection not only effectively suppresses inflammation but also enhances re-epithelialization and angiogenesis and reduces scar formation, which can promote the repair of skin wounds through secretion of stem cell-like EdSC. However, the structure and physiology of animal skin are different from those of human skin. Therefore, it is important to understand the mechanism of EdSC in human skin wounds.
From clinicaltrials.gov, we retrieved several clinical trials about wound healing treated with MSCs, but only two have exosomes. Using stem cells to treat burns as early as 2005, this study demonstrates that BM-MSC therapy is safe, which promoted angiogenesis and accelerated granulation tissue formation (Rasulov et al., 2005). Another completed clinical trial, which began in 2019, used stem cell–conditioned medium containing exosomes or microbubbles to treat chronic ulcerative wounds and found that conditioned medium stem cells can improve skin ulcer healing as an additional growth factor for the first time (NCT04134676). In addition, we retrieved a clinical trial initiated by Kumamoto University—the effect of plasma-derived exosomes on skin wound healing. Participants’ wounds were treated with plasma rich in exosomes for 28 days to evaluate the effect of exosomes on skin wound healing (NCT0256526). This study found that compared with normal subjects, patients with chronic wounds such as skin ulcers had significantly lower levels of serum exosomes. In conclusion, the results of existing clinical trials show that exosomes can accelerate skin wound healing.
Similar to wound treatment, we can retrieve 258 clinical trials when the key word is exosome, such as for periodontitis (NCT04270006), melanoma (NCT02310451), chronic low back pain (NCT04849429), knee osteoarthritis (NCT05060107), and COVID-19 (NCT05216562). With the increasing number of clinical trials on the therapeutic effect of exosomes, it is believed that exosomes will come out as a therapeutic drug as soon as possible.
Conclusion
EdSCs are small in size and efficient, have low immune rejection, and have special physiological and biological functions, which have significant advantages for the treatment of wounds. With scientific and technological progress, a deeper understanding of EdSC, and the treatment of related diseases in the medical field, interdisciplinary integration will complement and enhance the application of EdSC in various fields. For example, combining the advantages of exosomes as carriers with advanced design methods of nano-medicine can establish a nano-treatment platform based on EdSC. In addition, understanding the interaction between exosomes and other organelles is helpful to better understand the process of disease (Chen Q. et al., 2019; Chen et al., 2020). The most difficult component of the research of exosomes is the inadequate number of exosomes meeting the application standards. In the near future, advances in the scaling-up technology for GMP-compliant exosome manufacturing will enhance the applications of exosomes for wound healing.
Author contributions
HL investigated the literature. TS wrote the manuscript, and HW and XZ revised the figures. WX guided and revised the overall structure and content of the review.
Funding
Mild photothermal targeted therapy remolds the microenvironment to improve the therapeutic sensitivity of PD-1 breast cancer and its mechanism (ZR2021MH118). Application of multifunctional liposome nanoreactor in tumor therapy (202102065). Study on the synergistic effect of PD-1 on triple-negative breast cancer based on tumor microenvironment remodeling (202019176).
Conflict of interest
The authors declare that the research was conducted in the absence of any commercial or financial relationships that could be construed as a potential conflict of interest.
Publisher’s note
All claims expressed in this article are solely those of the authors and do not necessarily represent those of their affiliated organizations, or those of the publisher, the editors, and the reviewers. Any product that may be evaluated in this article, or claim that may be made by its manufacturer, is not guaranteed or endorsed by the publisher.
References
Akbari, A., Jabbari, N., Sharifi, R., Ahmadi, M., Vahhabi, A., Seyedzadeh, S. J., et al. (2020). Free and hydrogel encapsulated exosome-based therapies in regenerative medicine. Life Sci. 249, 117447. doi:10.1016/j.lfs.2020.117447
Al Gailani, M., Liu, M., and Wen, J. (2022). Ligands for oral delivery of peptides across the blood-brain-barrier. Acta Mater. Medica 1, 1. doi:10.15212/amm-2021-0007
Alzhrani, G. N., Alanazi, S. T., Alsharif, S. Y., Albalawi, A. M., Alsharif, A. A., Abdel-Maksoud, M. S., et al. (2021). Exosomes: Isolation, characterization, and biomedical applications. Cell Biol. Int. 45, 1807–1831. doi:10.1002/cbin.11620
An, T., Chen, Y., Tu, Y., and Lin, P. (2021). Mesenchymal stromal cell-derived extracellular vesicles in the treatment of diabetic foot ulcers: Application and challenges. Stem Cell Rev. Rep. 17, 369–378. doi:10.1007/s12015-020-10014-9
An, Y., Zhao, J., Nie, F., Qin, Z., Xue, H., Wang, G., et al. (2019). Exosomes from adipose-derived stem cells (ADSCs) overexpressing miR-21 promote vascularization of endothelial cells. Sci. Rep. 9, 12861. doi:10.1038/s41598-019-49339-y
Arishe, O. O., Priviero, F., Wilczynski, S. A., and Webb, R. C. (2021). Exosomes as intercellular messengers in hypertension. Int. J. Mol. Sci. 22, 11685. doi:10.3390/ijms222111685
Auger, F. A., Lacroix, D., and Germain, L. (2009). Skin substitutes and wound healing. Skin. Pharmacol. Physiol. 22, 94–102. doi:10.1159/000178868
Ayavoo, T., Murugesan, K., and Gnanasekaran, A. (2021). Roles and mechanisms of stem cell in wound healing. Stem Cell Investig. 8, 4. doi:10.21037/sci-2020-027
Blazquez, R., Sanchez-Margallo, F. M., De La Rosa, O., Dalemans, W., Alvarez, V., Tarazona, R., et al. (2014). Immunomodulatory potential of human adipose mesenchymal stem cells derived exosomes on in vitro stimulated T cells. Front. Immunol. 5, 556. doi:10.3389/fimmu.2014.00556
Carnino, J. M., Lee, H., and Jin, Y. (2019). Isolation and characterization of extracellular vesicles from broncho-alveolar lavage fluid: A review and comparison of different methods. Respir. Res. 20, 240. doi:10.1186/s12931-019-1210-z
Chen, B., Sun, Y., Zhang, J., Zhu, Q., Yang, Y., Niu, X., et al. (2019a). Human embryonic stem cell-derived exosomes promote pressure ulcer healing in aged mice by rejuvenating senescent endothelial cells. Stem Cell Res. Ther. 10, 142. doi:10.1186/s13287-019-1253-6
Chen, C. Y., Rao, S. S., Ren, L., Hu, X. K., Tan, Y. J., Hu, Y., et al. (2018). Exosomal DMBT1 from human urine-derived stem cells facilitates diabetic wound repair by promoting angiogenesis. Theranostics 8, 1607–1623. doi:10.7150/thno.22958
Chen Md, G., Wu Md, Y., Zou Md, L., and Zeng Md, Y. (2021). Effect of MicroRNA-146a modified adipose-derived stem cell exosomes on rat back wound healing. Int. J. Low. Extrem. wounds 1. doi:10.1177/15347346211038092
Chen, Q., Shao, X., Hao, M., Fang, H., Guan, R., Tian, Z., et al. (2020). Quantitative analysis of interactive behavior of mitochondria and lysosomes using structured illumination microscopy. Biomaterials 250, 120059. doi:10.1016/j.biomaterials.2020.120059
Chen, Q., Shao, X., Tian, Z., Chen, Y., Mondal, P., Liu, F., et al. (2019b). Nanoscale monitoring of mitochondria and lysosome interactions for drug screening and discovery. Nano Res. 12, 1009–1015. doi:10.1007/s12274-019-2331-x
Chen, W., Huang, Y., Han, J., Yu, L., Li, Y., Lu, Z., et al. (2016). Immunomodulatory effects of mesenchymal stromal cells-derived exosome. Immunol. Res. 64, 831–840. doi:10.1007/s12026-016-8798-6
Cizmar, P., and Yuana, Y. (2017). Detection and characterization of extracellular vesicles by transmission and cryo-transmission electron microscopy. Methods Mol. Biol. 1660, 221–232. doi:10.1007/978-1-4939-7253-1_18
Cooper, D. R., Wang, C., Patel, R., Trujillo, A., Patel, N. A., Prather, J., et al. (2018). Human adipose-derived stem cell conditioned media and exosomes containing MALAT1 promote human dermal fibroblast migration and ischemic wound healing. Adv. Wound Care 7, 299–308. doi:10.1089/wound.2017.0775
Crescitelli, R., Lässer, C., Szabó, T. G., Kittel, A., Eldh, M., Dianzani, I., et al. (2013). Distinct RNA profiles in subpopulations of extracellular vesicles: Apoptotic bodies, microvesicles and exosomes. J. Extracell. Vesicles 2, 20677. doi:10.3402/jev.v2i0.20677
Dai, C., Shih, S., and Khachemoune, A. (2020). Skin substitutes for acute and chronic wound healing: An updated review. J. Dermatol. Treat. 31, 639–648. doi:10.1080/09546634.2018.1530443
Dai, H., Fan, Q., and Wang, C. (2022). Recent applications of immunomodulatory biomaterials for disease immunotherapy. Hoboken, NJ: Exploration, 20210157. n/a.
Del Fattore, A., Luciano, R., Pascucci, L., Goffredo, B. M., Giorda, E., Scapaticci, M., et al. (2015). Immunoregulatory effects of mesenchymal stem cell-derived extracellular vesicles on T lymphocytes. Cell Transpl. 24, 2615–2627. doi:10.3727/096368915X687543
Di Trapani, M., Bassi, G., Midolo, M., Gatti, A., Kamga, P. T., Cassaro, A., et al. (2016). Differential and transferable modulatory effects of mesenchymal stromal cell-derived extracellular vesicles on T, B and NK cell functions. Sci. Rep. 6, 24120. doi:10.1038/srep24120
Ding, J., Wang, X., Chen, B., Zhang, J., and Xu, J. (2019). Exosomes derived from human bone marrow mesenchymal stem cells stimulated by deferoxamine accelerate cutaneous wound healing by promoting angiogenesis. Biomed. Res. Int. 2019, 9742765. doi:10.1155/2019/9742765
Ding, Y., Wang, Y., and Hu, Q. (2022). Recent advances in overcoming barriers to cell-based delivery systems for cancer immunotherapy. Exploration 2, 20210106. doi:10.1002/exp.20210106
Dolati, S., Yousefi, M., Pishgahi, A., Nourbakhsh, S., Pourabbas, B., Shakouri, S. K., et al. (2020). Prospects for the application of growth factors in wound healing. Growth Factors (Chur, Switz. 38, 25–34. doi:10.1080/08977194.2020.1820499
Dong, N., Lv, H., Liu, C., and Zhang, P. (2022). Research progress in 3D-printed medicinal tablets. Acta Mater. Medica 1, 1. doi:10.15212/amm-2021-0010
Du, Y. M., Zhuansun, Y. X., Chen, R., Lin, L., Lin, Y., Li, J. G., et al. (2018). Mesenchymal stem cell exosomes promote immunosuppression of regulatory T cells in asthma. Exp. Cell Res. 363, 114–120. doi:10.1016/j.yexcr.2017.12.021
Duan, M., Zhang, Y., Zhang, H., Meng, Y., Qian, M., Zhang, G., et al. (2020). Epidermal stem cell-derived exosomes promote skin regeneration by downregulating transforming growth factor-β1 in wound healing. Stem Cell Res. Ther. 11, 452. doi:10.1186/s13287-020-01971-6
Fang, S., Xu, C., Zhang, Y., Xue, C., Yang, C., Bi, H., et al. (2016). Umbilical cord-derived mesenchymal stem cell-derived exosomal MicroRNAs suppress myofibroblast differentiation by inhibiting the transforming growth factor-β/SMAD2 pathway during wound healing. Stem Cells Transl. Med. 5, 1425–1439. doi:10.5966/sctm.2015-0367
Farahani, M., and Shafiee, A. (2021). Wound healing: From passive to smart dressings. Adv. Healthc. Mat. 10, e2100477. doi:10.1002/adhm.202100477
FerreiraCastropaggiaro, M., Oliveiraisaac, A., Neto, C. T., and Dos, G. B. (2011). Substitutos cutâneos: Conceitos atuais e proposta de classificação??o. Rev. Bras. Cir. Plast. 26, 696–702. doi:10.1590/s1983-51752011000400028
Fu, M., Gu, J., Jiang, P., Qian, H., Xu, W., Zhang, X., et al. (2019). Exosomes in gastric cancer: Roles, mechanisms, and applications. Mol. Cancer 18, 41. doi:10.1186/s12943-019-1001-7
Golchin, A., Hosseinzadeh, S., and Roshangar, L. (2018). The role of nanomaterials in cell delivery systems. Med. Mol. Morphol. 51, 1–12. doi:10.1007/s00795-017-0173-8
Golchin, A., Shams, F., Basiri, A., Ranjbarvan, P., Kiani, S., Sarkhosh-Inanlou, R., et al. (2022). Combination therapy of stem cell-derived exosomes and biomaterials in the wound healing. Stem Cell Rev. Rep. 1, 1. doi:10.1007/s12015-021-10309-5
Goodarzi, P., Larijani, B., Alavi-Moghadam, S., Tayanloo-Beik, A., Mohamadi-Jahani, F., Ranjbaran, N., et al. (2018). Mesenchymal stem cells-derived exosomes for wound regeneration. Adv. Exp. Med. Biol. 1119, 119–131. doi:10.1007/5584_2018_251
Guillamat-Prats, R. (2021). The role of MSC in wound healing, scarring and regeneration. Cells 10, 1729. doi:10.3390/cells10071729
Gurtner, G. C., Werner, S., Barrandon, Y., and Longaker, M. T. (2008). Wound repair and regeneration. Nature 453, 314–321. doi:10.1038/nature07039
Gurunathan, S., Kang, M.-H., Jeyaraj, M., Qasim, M., and Kim, J.-H. (2019). Review of the isolation, characterization, biological function, and multifarious therapeutic approaches of exosomes. Cells 8, 307. doi:10.3390/cells8040307
Gurunathan, S., Kang, M. H., and Kim, J. H. (2021). A comprehensive review on factors influences biogenesis, functions, therapeutic and clinical implications of exosomes. Int. J. Nanomedicine 16, 1281–1312. doi:10.2147/IJN.S291956
Gurung, S., Perocheau, D., Touramanidou, L., and Baruteau, J. (2021). The exosome journey: From biogenesis to uptake and intracellular signalling. Cell Commun. Signal. 19, 47. doi:10.1186/s12964-021-00730-1
Ha, D. H., Kim, H.-K., Lee, J., Kwon, H. H., Park, G.-H., Yang, S. H., et al. (2020). Mesenchymal stem/stromal cell-derived exosomes for immunomodulatory therapeutics and skin regeneration. Cells 9, 1157. doi:10.3390/cells9051157
Han, G., and Ceilley, R. (2017). Chronic wound healing: A review of current management and treatments. Adv. Ther. 34, 599–610. doi:10.1007/s12325-017-0478-y
He, X., Dong, Z., Cao, Y., Wang, H., Liu, S., Liao, L., et al. (2019). MSC-derived exosome promotes M2 polarization and enhances cutaneous wound healing. Stem Cells Int. 2019, 7132708. doi:10.1155/2019/7132708
Hessvik, N. P., and Llorente, A. (2018). Current knowledge on exosome biogenesis and release. Cell. Mol. Life Sci. 75, 193–208. doi:10.1007/s00018-017-2595-9
Heydari, R., Abdollahpour-Alitappeh, M., Shekari, F., and Meyfour, A. (2021). Emerging role of extracellular vesicles in biomarking the gastrointestinal diseases. Expert Rev. Mol. diagn. 21, 939–962. doi:10.1080/14737159.2021.1954909
Hodge, A. L., Baxter, A. A., and Poon, I. K. H. (2021). Gift bags from the sentinel cells of the immune system: The diverse role of dendritic cell-derived extracellular vesicles. J. Leukoc. Biol. 111, 903–920. doi:10.1002/JLB.3RU1220-801R
Hu, L., Wang, J., Zhou, X., Xiong, Z., Zhao, J., Yu, R., et al. (2020). Author Correction: Exosomes derived from human adipose mensenchymal stem cells accelerates cutaneous wound healing via optimizing the characteristics of fibroblasts. Sci. Rep. 10, 6693. doi:10.1038/s41598-020-63068-7
Hu, L., Wang, J., Zhou, X., Xiong, Z., Zhao, J., Yu, R., et al. (2016). Exosomes derived from human adipose mensenchymal stem cells accelerates cutaneous wound healing via optimizing the characteristics of fibroblasts. Sci. Rep. 6, 32993. doi:10.1038/srep32993
Huotari, J., and Helenius, A. (2011). Endosome maturation. EMBO J. 30, 3481–3500. doi:10.1038/emboj.2011.286
Isaac, R., Reis, F. C. G., Ying, W., and Olefsky, J. M. (2021). Exosomes as mediators of intercellular crosstalk in metabolism. Cell Metab. 33, 1744–1762. doi:10.1016/j.cmet.2021.08.006
Isakson, M., De Blacam, C., Whelan, D., Mcardle, A., and Clover, A. J. P. (2015). Mesenchymal stem cells and cutaneous wound healing: Current evidence and future potential. Stem Cells Int. 2015, 831095. doi:10.1155/2015/831095
Iy, A. B., and Al-Rubaiy, K. K. (2009). Skin substitutes: A brief review of types and clinical applications. Oman Med. J. 24, 4–6. doi:10.5001/omj.2009.2
Jalaludin, I., Lubman, D. M., and Kim, J. (2021). A guide to mass spectrometric analysis of extracellular vesicle proteins for biomarker discovery. Mass Spectrom. Rev. 8, e21749. doi:10.1002/mas.21749
Järbrink, K., Ni, G., Sönnergren, H., Schmidtchen, A., Pang, C., Bajpai, R., et al. (2017). The humanistic and economic burden of chronic wounds: A protocol for a systematic review. Syst. Rev. 6, 15. doi:10.1186/s13643-016-0400-8
Jiang, J., Mei, J., Ma, Y., Jiang, S., Zhang, J., Yi, S., et al. (2022). Tumor hijacks macrophages and microbiota through extracellular vesicles. Exploration 2, 20210144. doi:10.1002/exp.20210144
Jiang, L., Zhang, Y., Liu, T., Wang, X., Wang, H., Song, H., et al. (2020). Exosomes derived from TSG-6 modified mesenchymal stromal cells attenuate scar formation during wound healing. Biochimie 177, 40–49. doi:10.1016/j.biochi.2020.08.003
Jin, C., Wu, P., Li, L., Xu, W., and Qian, H. (2021). Exosomes: Emerging therapy delivery tools and biomarkers for kidney diseases. Stem Cells Int. 2021, 7844455. doi:10.1155/2021/7844455
Jo, H., Brito, S., Kwak, B. M., Park, S., Lee, M.-G., Bin, B.-H., et al. (2021). Applications of mesenchymal stem cells in skin regeneration and rejuvenation. Int. J. Mol. Sci. 22, 2410. doi:10.3390/ijms22052410
Kalluri, R., and Lebleu, V. S. (2020). The biology, function, and biomedical applications of exosomes. Science 367, eaau6977. doi:10.1126/science.aau6977
Kamoun, E. A., Kenawy, E.-R. S., and Chen, X. (2017). A review on polymeric hydrogel membranes for wound dressing applications: PVA-based hydrogel dressings. J. Adv. Res. 8, 217–233. doi:10.1016/j.jare.2017.01.005
Kandimalla, R., Aqil, F., Tyagi, N., and Gupta, R. (2021). Milk exosomes: A biogenic nanocarrier for small molecules and macromolecules to combat cancer. Am. J. Reprod. Immunol. 85, e13349. doi:10.1111/aji.13349
Kang, T., Jones, T. M., Naddell, C., Bacanamwo, M., Calvert, J. W., Thompson, W. E., et al. (2016). Adipose-derived stem cells induce angiogenesis via microvesicle transport of miRNA-31. Stem Cells Transl. Med. 5, 440–450. doi:10.5966/sctm.2015-0177
Kanji, S., and Das, H. (2017). Advances of stem cell therapeutics in cutaneous wound healing and regeneration. Mediat. Inflamm. 2017, 5217967. doi:10.1155/2017/5217967
Khare, D., Or, R., Resnick, I., Barkatz, C., Almogi-Hazan, O., Avni, B., et al. (2018). Mesenchymal stromal cell-derived exosomes affect mRNA expression and function of B-lymphocytes. Front. Immunol. 9, 3053. doi:10.3389/fimmu.2018.03053
Kim, Y.-J., Yoo, S. M., Park, H. H., Lim, H. J., Kim, Y.-L., Lee, S., et al. (2017). Exosomes derived from human umbilical cord blood mesenchymal stem cells stimulates rejuvenation of human skin. Biochem. Biophys. Res. Commun. 493, 1102–1108. doi:10.1016/j.bbrc.2017.09.056
Konoshenko, M. Y., Lekchnov, E. A., Vlassov, A. V., and Laktionov, P. P. (2018). Isolation of extracellular vesicles: General methodologies and latest trends. Biomed. Res. Int. 2018, 8545347. doi:10.1155/2018/8545347
Kosaka, N., Iguchi, H., Yoshioka, Y., Hagiwara, K., Takeshita, F., Ochiya, T., et al. (2012). Competitive interactions of cancer cells and normal cells via secretory microRNAs. J. Biol. Chem. 287, 1397–1405. doi:10.1074/jbc.M111.288662
Las Heras, K., Igartua, M., Santos-Vizcaino, E., and Hernandez, R. M. (2020). Chronic wounds: Current status, available strategies and emerging therapeutic solutions. J. Control. Release 328, 532–550. doi:10.1016/j.jconrel.2020.09.039
Li, D., Zhang, P., Yao, X., Li, H., Shen, H., Li, X., et al. (2018a). Exosomes derived from miR-133b-modified mesenchymal stem cells promote recovery after spinal cord injury. Front. Neurosci. 12, 845. doi:10.3389/fnins.2018.00845
Li, D., Zhao, Y., Zhang, C., Wang, F., Zhou, Y., Jin, S., et al. (2021a). Plasma exosomes at the late phase of remote ischemic pre-conditioning attenuate myocardial ischemia-reperfusion injury through transferring miR-126a-3p. Front. Cardiovasc. Med. 8, 736226. doi:10.3389/fcvm.2021.736226
Li, K. L., Li, J. Y., Xie, G. L., and Ma, X. Y. (2021b). Exosomes released from human bone marrow-derived mesenchymal stem cell attenuate acute graft-versus-host disease after allogeneic hematopoietic stem cell transplantation in mice. Front. Cell Dev. Biol. 9, 617589. doi:10.3389/fcell.2021.617589
Li, S., Yi, M., Dong, B., Tan, X., Luo, S., Wu, K., et al. (2021c). The role of exosomes in liquid biopsy for cancer diagnosis and prognosis prediction. Int. J. Cancer 148, 2640–2651. doi:10.1002/ijc.33386
Li, X., Liu, L., Yang, J., Yu, Y., Chai, J., Wang, L., et al. (2016). Exosome derived from human umbilical cord mesenchymal stem cell mediates MiR-181c attenuating burn-induced excessive inflammation. EBioMedicine 8, 72–82. doi:10.1016/j.ebiom.2016.04.030
Li, X., Xie, X., Lian, W., Shi, R., Han, S., Zhang, H., et al. (2018b). Exosomes from adipose-derived stem cells overexpressing Nrf2 accelerate cutaneous wound healing by promoting vascularization in a diabetic foot ulcer rat model. Exp. Mol. Med. 50, 29. doi:10.1038/s12276-018-0058-5
Li, Y., Zhang, J., Shi, J., Liu, K., Wang, X., Jia, Y., et al. (2021d). Exosomes derived from human adipose mesenchymal stem cells attenuate hypertrophic scar fibrosis by miR-192-5p/IL-17RA/Smad axis. Stem Cell Res. Ther. 12, 221. doi:10.1186/s13287-021-02290-0
Liang, X., Zhang, L., Wang, S., Han, Q., and Zhao, R. C. (2016). Exosomes secreted by mesenchymal stem cells promote endothelial cell angiogenesis by transferring miR-125a. J. Cell Sci. 129, 2182–2189. doi:10.1242/jcs.170373
Liu, J., Yan, Z., Yang, F., Huang, Y., Yu, Y., Zhou, L., et al. (2021a). Exosomes derived from human umbilical cord mesenchymal stem cells accelerate cutaneous wound healing by enhancing angiogenesis through delivering angiopoietin-2. Stem Cell Rev. Rep. 17, 305–317. doi:10.1007/s12015-020-09992-7
Liu, L., Yu, Y., Hou, Y., Chai, J., Duan, H., Chu, W., et al. (2014). Human umbilical cord mesenchymal stem cells transplantation promotes cutaneous wound healing of severe burned rats. PLoS One 9, e88348. doi:10.1371/journal.pone.0088348
Liu, Q., Piao, H., Wang, Y., Zheng, D., and Wang, W. (2021b). Circulating exosomes in cardiovascular disease: Novel carriers of biological information. Biomed. Pharmacother. 135, 111148. doi:10.1016/j.biopha.2020.111148
Liu, W., Yu, M., Xie, D., Wang, L., Ye, C., Zhu, Q., et al. (2020). Melatonin-stimulated MSC-derived exosomes improve diabetic wound healing through regulating macrophage M1 and M2 polarization by targeting the PTEN/AKT pathway. Stem Cell Res. Ther. 11, 259. doi:10.1186/s13287-020-01756-x
Livshits, M. A., Livshts, M. A., Khomyakova, E., Evtushenko, E. G., Lazarev, V. N., Kulemin, N. A., et al. (2015). Isolation of exosomes by differential centrifugation: Theoretical analysis of a commonly used protocol. Sci. Rep. 5, 17319. doi:10.1038/srep17319
Ma, J., and Wu, C. (2022). Bioactive inorganic particles-based biomaterials for skin tissue engineering. Hoboken, NJ: Exploration, 20210083. n/a.
Ma, T., Fu, B., Yang, X., Xiao, Y., and Pan, M. (2019). Adipose mesenchymal stem cell-derived exosomes promote cell proliferation, migration, and inhibit cell apoptosis via Wnt/β-catenin signaling in cutaneous wound healing. J. Cell. Biochem. 120, 10847–10854. doi:10.1002/jcb.28376
Mazini, L., Rochette, L., Admou, B., Amal, S., and Malka, G. (2020). Hopes and limits of adipose-derived stem cells (ADSCs) and mesenchymal stem cells (MSCs) in wound healing. Int. J. Mol. Sci. 21, E1306. doi:10.3390/ijms21041306
Mcbride, J. D., Rodriguez-Menocal, L., Guzman, W., Candanedo, A., Garcia-Contreras, M., Badiavas, E. V., et al. (2017). Bone marrow mesenchymal stem cell-derived CD63(+) exosomes transport Wnt3a exteriorly and enhance dermal fibroblast proliferation, migration, and angiogenesis in vitro. Stem Cells Dev. 26, 1384–1398. doi:10.1089/scd.2017.0087
Miao, C., Zhou, W., Wang, X., and Fang, J. (2021). The research progress of exosomes in osteoarthritis, with particular emphasis on the mediating roles of miRNAs and lncRNAs. Front. Pharmacol. 12, 685623. doi:10.3389/fphar.2021.685623
Monguio-Tortajada, M., Roura, S., Galvez-Monton, C., Pujal, J. M., Aran, G., Sanjurjo, L., et al. (2017). Nanosized UCMSC-derived extracellular vesicles but not conditioned medium exclusively inhibit the inflammatory response of stimulated T cells: Implications for nanomedicine. Theranostics 7, 270–284. doi:10.7150/thno.16154
Nik Nabil, W. N., Xi, Z., Liu, M., Li, Y., Yao, M., Liu, T., et al. (2022). Advances in therapeutic agents targeting quiescent cancer cells. Acta Mater. Medica 1, 1. doi:10.15212/amm-2021-0005
Nikfarjam, S., Rezaie, J., Zolbanin, N. M., and Jafari, R. (2020). Mesenchymal stem cell derived-exosomes: A modern approach in translational medicine. J. Transl. Med. 18, 449. doi:10.1186/s12967-020-02622-3
Nojehdehi, S., Soudi, S., Hesampour, A., Rasouli, S., Soleimani, M., Hashemi, S. M., et al. (2018). Immunomodulatory effects of mesenchymal stem cell-derived exosomes on experimental type-1 autoimmune diabetes. J. Cell. Biochem. 119, 9433–9443. doi:10.1002/jcb.27260
Nourian Dehkordi, A., Mirahmadi Babaheydari, F., Chehelgerdi, M., and Raeisi Dehkordi, S. (2019). Skin tissue engineering: Wound healing based on stem-cell-based therapeutic strategies. Stem Cell Res. Ther. 10, 111. doi:10.1186/s13287-019-1212-2
Opoku-Damoah, Y., Zhang, R., Ta, H. T., and Xu, Z. P. (2022). Therapeutic gas-releasing nanomedicines with controlled release: Advances and perspectives. Hoboken, NJ: Exploration, 20210181. n/a.
Pelissier Vatter, F. A., Lucotti, S., and Zhang, H. (2020). Recent advances in experimental models of breast cancer exosome secretion, characterization and function. J. Mammary Gland. Biol. Neoplasia 25, 305–317. doi:10.1007/s10911-020-09473-0
Powers, J. G., Higham, C., Broussard, K., and Phillips, T. J. (2016). Wound healing and treating wounds: Chronic wound care and management. J. Am. Acad. Dermatol. 74, 607–625. doi:10.1016/j.jaad.2015.08.070
Proksch, E., Brandner, J. M., and Jensen, J.-M. (2008). The skin: An indispensable barrier. Exp. Dermatol. 17, 1063–1072. doi:10.1111/j.1600-0625.2008.00786.x
Purghe, B., Manfredi, M., Ragnoli, B., Baldanzi, G., and Malerba, M. (2021). Exosomes in chronic respiratory diseases. Biomed. Pharmacother. 144, 112270. doi:10.1016/j.biopha.2021.112270
Qian, L., Pi, L., Fang, B. R., and Meng, X. X. (2021). Adipose mesenchymal stem cell-derived exosomes accelerate skin wound healing via the lncRNA H19/miR-19b/SOX9 axis. Lab. Invest. 101, 1254–1266. doi:10.1038/s41374-021-00611-8
Rani, S., and Ritter, T. (2016). The exosome - a naturally secreted nanoparticle and its application to wound healing. Adv. Mat. 28, 5542–5552. doi:10.1002/adma.201504009
Rao, D., Huang, D., Sang, C., Zhong, T., Zhang, Z., Tang, Z., et al. (2022). Advances in mesenchymal stem cell-derived exosomes as drug delivery vehicles. Front. Bioeng. Biotechnol. 9, 797359. doi:10.3389/fbioe.2021.797359
Raposo, G., and Stoorvogel, W. (2013). Extracellular vesicles: Exosomes, microvesicles, and friends. J. Cell Biol. 200, 373–383. doi:10.1083/jcb.201211138
Rasulov, M. F., Vasilchenkov, A. V., Onishchenko, N. A., Krasheninnikov, M. E., Kravchenko, V. I., Gorshenin, T. L., et al. (2005). First experience of the use bone marrow mesenchymal stem cells for the treatment of a patient with deep skin burns. Bull. Exp. Biol. Med. 139, 141–144. doi:10.1007/s10517-005-0232-3
Reinke, J. M., and Sorg, H. (2012). Wound repair and regeneration. Eur. Surg. Res. 49, 35–43. doi:10.1159/000339613
Reis, M., Mavin, E., Nicholson, L., Green, K., Dickinson, A. M., Wang, X. N., et al. (2018). Mesenchymal stromal cell-derived extracellular vesicles attenuate dendritic cell maturation and function. Front. Immunol. 9, 2538. doi:10.3389/fimmu.2018.02538
Riau, A. K., Ong, H. S., Yam, G. H. F., and Mehta, J. S. (2019). Sustained delivery system for stem cell-derived exosomes. Front. Pharmacol. 10, 1368. doi:10.3389/fphar.2019.01368
Riazifar, M., Mohammadi, M. R., Pone, E. J., Yeri, A., Lasser, C., Segaliny, A. I., et al. (2019). Stem cell-derived exosomes as nanotherapeutics for autoimmune and neurodegenerative disorders. ACS Nano 13, 6670–6688. doi:10.1021/acsnano.9b01004
Riha, S. M., Maarof, M., and Fauzi, M. B. (2021). Synergistic effect of biomaterial and stem cell for skin tissue engineering in cutaneous wound healing: A concise review. Polymers 13, 1546. doi:10.3390/polym13101546
Saad, M. H., Badierah, R., Redwan, E. M., and El-Fakharany, E. M. (2021). A comprehensive insight into the role of exosomes in viral infection: Dual faces bearing different functions. Pharmaceutics 13, 1405. doi:10.3390/pharmaceutics13091405
Safari, B., Aghazadeh, M., Davaran, S., and Roshangar, L. (2022). Exosome-loaded hydrogels: A new cell-free therapeutic approach for skin regeneration. Eur. J. Pharm. Biopharm. 171, 50–59. doi:10.1016/j.ejpb.2021.11.002
Shafei, S., Khanmohammadi, M., Heidari, R., Ghanbari, H., Taghdiri Nooshabadi, V., Farzamfar, S., et al. (2020). Exosome loaded alginate hydrogel promotes tissue regeneration in full-thickness skin wounds: An in vivo study. J. Biomed. Mat. Res. A 108, 545–556. doi:10.1002/jbm.a.36835
Shen, K., Jia, Y., Wang, X., Zhang, J., Liu, K., Wang, J., et al. (2021). Exosomes from adipose-derived stem cells alleviate the inflammation and oxidative stress via regulating Nrf2/HO-1 axis in macrophages. Free Radic. Biol. Med. 165, 54–66. doi:10.1016/j.freeradbiomed.2021.01.023
Shirejini, S. Z., and Inci, F. (2021). The yin and yang of exosome isolation methods: Conventional practice, microfluidics, and commercial kits. Biotechnol. Adv. 54, 107814. doi:10.1016/j.biotechadv.2021.107814
Shojaati, G., Khandaker, I., Funderburgh, M. L., Mann, M. M., Basu, R., Stolz, D. B., et al. (2019). Mesenchymal stem cells reduce corneal fibrosis and inflammation via extracellular vesicle-mediated delivery of miRNA. Stem Cells Transl. Med. 8, 1192–1201. doi:10.1002/sctm.18-0297
Shpichka, A., Butnaru, D., Bezrukov, E. A., Sukhanov, R. B., Atala, A., Burdukovskii, V., et al. (2019). Skin tissue regeneration for burn injury. Stem Cell Res. Ther. 10, 94. doi:10.1186/s13287-019-1203-3
Singh, K., Nalabotala, R., Koo, K. M., Bose, S., Nayak, R., Shiddiky, M. J. A., et al. (2021). Separation of distinct exosome subpopulations: Isolation and characterization approaches and their associated challenges. Analyst 146, 3731–3749. doi:10.1039/d1an00024a
Sorg, H., Tilkorn, D. J., Hager, S., Hauser, J., and Mirastschijski, U. (2017). Skin wound healing: An update on the current knowledge and concepts. Eur. Surg. Res. 58, 81–94. doi:10.1159/000454919
Takahashi, Y., Nishikawa, M., Shinotsuka, H., Matsui, Y., Ohara, S., Imai, T., et al. (2013). Visualization and in vivo tracking of the exosomes of murine melanoma B16-BL6 cells in mice after intravenous injection. J. Biotechnol. 165, 77–84. doi:10.1016/j.jbiotec.2013.03.013
Tang, N., Zheng, Y., Cui, D., and Haick, H. (2021). Multifunctional dressing for wound diagnosis and rehabilitation. Adv. Healthc. Mat. 10, e2101292. doi:10.1002/adhm.202101292
Tarasov, V. V., Svistunov, A. A., Chubarev, V. N., Dostdar, S. A., Sokolov, A. V., Brzecka, A., et al. (2021). Extracellular vesicles in cancer nanomedicine. Semin. Cancer Biol. 69, 212–225. doi:10.1016/j.semcancer.2019.08.017
Thakur, A., Ke, X., Chen, Y. W., Motallebnejad, P., Zhang, K., Lian, Q., et al. (2021). The mini player with diverse functions: Extracellular vesicles in cell biology, disease, and therapeutics. Protein Cell 13, 631–654. doi:10.1007/s13238-021-00863-6
Thery, C., Witwer, K. W., Aikawa, E., Alcaraz, M. J., Anderson, J. D., Andriantsitohaina, R., et al. (2018). Minimal information for studies of extracellular vesicles 2018 (MISEV2018): A position statement of the international society for extracellular vesicles and update of the MISEV2014 guidelines. J. Extracell. Vesicles 7, 1535750. doi:10.1080/20013078.2018.1535750
Tiwari, A., Singh, A., Verma, S., Stephenson, S., Bhowmick, T., Sangwan, V. S., et al. (2021a). Mini review: Current trends and understanding of exosome therapeutic potential in corneal diseases. Front. Pharmacol. 12, 684712. doi:10.3389/fphar.2021.684712
Tiwari, S., Kumar, V., Randhawa, S., and Verma, S. K. (2021b). Preparation and characterization of extracellular vesicles. Am. J. Reprod. Immunol. 85, e13367. doi:10.1111/aji.13367
Tran, P. H. L., Xiang, D., Tran, T. T. D., Yin, W., Zhang, Y., Kong, L., et al. (2020). Exosomes and nanoengineering: A match made for precision therapeutics. Adv. Mat. 32, e1904040. doi:10.1002/adma.201904040
Tutuianu, R., Rosca, A. M., Iacomi, D. M., Simionescu, M., and Titorencu, I. (2021). Human mesenchymal stromal cell-derived exosomes promote in vitro wound healing by modulating the biological properties of skin keratinocytes and fibroblasts and stimulating angiogenesis. Int. J. Mol. Sci. 22, 6239. doi:10.3390/ijms22126239
Van Niel, G., D'angelo, G., and Raposo, G. (2018). Shedding light on the cell biology of extracellular vesicles. Nat. Rev. Mol. Cell Biol. 19, 213–228. doi:10.1038/nrm.2017.125
Velnar, T., Bailey, T., and Smrkolj, V. (2009). The wound healing process: An overview of the cellular and molecular mechanisms. J. Int. Med. Res. 37, 1528–1542. doi:10.1177/147323000903700531
Vu, N. B., Nguyen, H. T., Palumbo, R., Pellicano, R., Fagoonee, S., Pham, P. V., et al. (2021). Stem cell-derived exosomes for wound healing: Current status and promising directions. Minerva Med. 112, 384–400. doi:10.23736/S0026-4806.20.07205-5
Wang, L., Hu, L., Zhou, X., Xiong, Z., Zhang, C., Shehada, H. M. A., et al. (2021). Author Correction: Exosomes secreted by human adipose mesenchymal stem cells promote scarless cutaneous repair by regulating extracellular matrix remodelling. Sci. Rep. 11, 7066. doi:10.1038/s41598-018-24991-y
Wang, L., Hu, L., Zhou, X., Xiong, Z., Zhang, C., Shehada, H. M. A., et al. (2017). Exosomes secreted by human adipose mesenchymal stem cells promote scarless cutaneous repair by regulating extracellular matrix remodelling. Sci. Rep. 7, 13321. doi:10.1038/s41598-017-12919-x
Wang, P. H., Huang, B. S., Horng, H. C., Yeh, C. C., and Chen, Y. J. (2018). Wound healing. J. Chin. Med. Assoc. 81, 94–101. doi:10.1016/j.jcma.2017.11.002
Wang, W. M., Wu, C., and Jin, H. Z. (2019). Exosomes in chronic inflammatory skin diseases and skin tumors. Exp. Dermatol. 28, 213–218. doi:10.1111/exd.13857
Wei, T., Thakur, S. S., Liu, M., and Wen, J. (2022). Oral delivery of glutathione: Antioxidant function, barriers and strategies. Acta Mater. Med. 1, 1. doi:10.15212/amm-2022-0005
Wei, W., Ao, Q., Wang, X., Cao, Y., Liu, Y., Zheng, S. G., et al. (2021). Mesenchymal stem cell–derived exosomes: A promising biological tool in nanomedicine. Front. Pharmacol. 11, 1. doi:10.3389/fphar.2020.590470
Werner, S., and Grose, R. (2003). Regulation of wound healing by growth factors and cytokines. Physiol. Rev. 83, 835–870. doi:10.1152/physrev.2003.83.3.835
Wilkinson, H. N., and Hardman, M. J. (2020). Wound healing: Cellular mechanisms and pathological outcomes. Open Biol. 10, 200223. doi:10.1098/rsob.200223
Wu, X., Showiheen, S. a. A., Sun, A. R., Crawford, R., Xiao, Y., Mao, X., et al. (2019). Exosomes extraction and identification. Methods Mol. Biol. 2054, 81–91. doi:10.1007/978-1-4939-9769-5_4
Xie, M., Xiong, W., She, Z., Wen, Z., Abdirahman, A. S., Wan, W., et al. (2020). Immunoregulatory effects of stem cell-derived extracellular vesicles on immune cells. Front. Immunol. 11, 13. doi:10.3389/fimmu.2020.00013
Xu, N., Wang, L., Guan, J., Tang, C., He, N., Zhang, W., et al. (2018). Wound healing effects of a Curcuma zedoaria polysaccharide with platelet-rich plasma exosomes assembled on chitosan/silk hydrogel sponge in a diabetic rat model. Int. J. Biol. Macromol. 117, 102–107. doi:10.1016/j.ijbiomac.2018.05.066
Xu, R., Zhang, F., Chai, R., Zhou, W., Hu, M., Liu, B., et al. (2019). Exosomes derived from pro-inflammatory bone marrow-derived mesenchymal stem cells reduce inflammation and myocardial injury via mediating macrophage polarization. J. Cell. Mol. Med. 23, 7617–7631. doi:10.1111/jcmm.14635
Yang, C., Luo, L., Bai, X., Shen, K., Liu, K., Wang, J., et al. (2020a). Highly-expressed micoRNA-21 in adipose derived stem cell exosomes can enhance the migration and proliferation of the HaCaT cells by increasing the MMP-9 expression through the PI3K/AKT pathway. Arch. Biochem. Biophys. 681, 108259. doi:10.1016/j.abb.2020.108259
Yang, D., Zhang, W., Zhang, H., Zhang, F., Chen, L., Ma, L., et al. (2020b). Progress, opportunity, and perspective on exosome isolation - Efforts for efficient exosome-based theranostics. Theranostics 10, 3684–3707. doi:10.7150/thno.41580
Yang, J., Chen, Z., Pan, D., Li, H., and Shen, J. (2020c). Umbilical cord-derived mesenchymal stem cell-derived exosomes combined pluronic F127 hydrogel promote chronic diabetic wound healing and complete skin regeneration. Int. J. Nanomedicine 15, 5911–5926. doi:10.2147/IJN.S249129
Yang, Y., Guo, Z., Chen, W., Wang, X., Cao, M., Han, X., et al. (2021). M2 macrophage-derived exosomes promote angiogenesis and growth of pancreatic ductal adenocarcinoma by targeting E2F2. Mol. Ther. 29, 1226–1238. doi:10.1016/j.ymthe.2020.11.024
You, L., Wu, W., Wang, X., Fang, L., Adam, V., Nepovimova, E., et al. (2021). The role of hypoxia-inducible factor 1 in tumor immune evasion. Med. Res. Rev. 41, 1622–1643. doi:10.1002/med.21771
Yu, B., Zhang, X., and Li, X. (2014). Exosomes derived from mesenchymal stem cells. Int. J. Mol. Sci. 15, 4142–4157. doi:10.3390/ijms15034142
Yu, M., Liu, W., Li, J., Lu, J., Lu, H., Jia, W., et al. (2020). Exosomes derived from atorvastatin-pretreated MSC accelerate diabetic wound repair by enhancing angiogenesis via AKT/eNOS pathway. Stem Cell Res. Ther. 11, 350. doi:10.1186/s13287-020-01824-2
Zara, M., Amadio, P., Campodonico, J., Sandrini, L., and Barbieri, S. S. (2020). Exosomes in cardiovascular diseases. Diagn. (Basel) 10, E943. doi:10.3390/diagnostics10110943
Zeng, Q. L., and Liu, D. W. (2021). Mesenchymal stem cell-derived exosomes: An emerging therapeutic strategy for normal and chronic wound healing. World J. Clin. Cases 9, 6218–6233. doi:10.12998/wjcc.v9.i22.6218
Zeng, R., Lin, C., Lin, Z., Chen, H., Lu, W., Lin, C., et al. (2018). Approaches to cutaneous wound healing: Basics and future directions. Cell Tissue Res. 374, 217–232. doi:10.1007/s00441-018-2830-1
Zhang, B., Wu, X., Zhang, X., Sun, Y., Yan, Y., Shi, H., et al. (2015). Human umbilical cord mesenchymal stem cell exosomes enhance angiogenesis through the Wnt4/β-catenin pathway. Stem Cells Transl. Med. 4, 513–522. doi:10.5966/sctm.2014-0267
Zhang, B., Yeo, R. W. Y., Lai, R. C., Sim, E. W. K., Chin, K. C., Lim, S. K., et al. (2018). Mesenchymal stromal cell exosome-enhanced regulatory T-cell production through an antigen-presenting cell-mediated pathway. Cytotherapy 20, 687–696. doi:10.1016/j.jcyt.2018.02.372
Zhang, Y., Han, F., Gu, L., Ji, P., Yang, X., Liu, M., et al. (2020). Adipose mesenchymal stem cell exosomes promote wound healing through accelerated keratinocyte migration and proliferation by activating the AKT/HIF-1α axis. J. Mol. Histol. 51, 375–383. doi:10.1007/s10735-020-09887-4
Zhao, L., Jiang, X., Shi, J., Gao, S., Zhu, Y., Gu, T., et al. (2019). Exosomes derived from bone marrow mesenchymal stem cells overexpressing microRNA-25 protect spinal cords against transient ischemia. J. Thorac. Cardiovasc. Surg. 157, 508–517. doi:10.1016/j.jtcvs.2018.07.095
Zhao, R., Zhao, T., He, Z., Cai, R., and Pang, W. (2021a). Composition, isolation, identification and function of adipose tissue-derived exosomes. Adipocyte 10, 587–604. doi:10.1080/21623945.2021.1983242
Zhao, Y., Zhang, Z., Pan, Z., and Liu, Y. (2021b). Advanced bioactive nanomaterials for biomedical applications. Exploration 1, 20210089. doi:10.1002/exp.20210089
Keywords: exosome, stem cell, wound healing, therapeutics, wound
Citation: Lv H, Liu H, Sun T, Wang H, Zhang X and Xu W (2022) Exosome derived from stem cell: A promising therapeutics for wound healing. Front. Pharmacol. 13:957771. doi: 10.3389/fphar.2022.957771
Received: 31 May 2022; Accepted: 27 June 2022;
Published: 08 August 2022.
Edited by:
Peixue Ling, Shandong University (Qingdao), ChinaReviewed by:
Lei Wang, University of Cincinnati, United StatesXin Wei, University of Texas Health Science Center at Houston, United States
Copyright © 2022 Lv, Liu, Sun, Wang, Zhang and Xu. This is an open-access article distributed under the terms of the Creative Commons Attribution License (CC BY). The use, distribution or reproduction in other forums is permitted, provided the original author(s) and the copyright owner(s) are credited and that the original publication in this journal is cited, in accordance with accepted academic practice. No use, distribution or reproduction is permitted which does not comply with these terms.
*Correspondence: Wei Xu, d2VpeHVAc2R1LmVkdS5jbg==