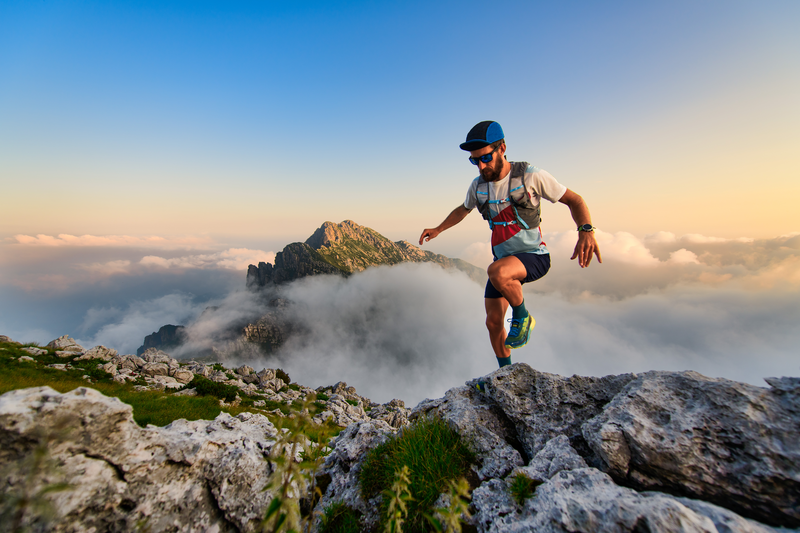
94% of researchers rate our articles as excellent or good
Learn more about the work of our research integrity team to safeguard the quality of each article we publish.
Find out more
SYSTEMATIC REVIEW article
Front. Pharmacol. , 30 August 2022
Sec. Pharmacology of Infectious Diseases
Volume 13 - 2022 | https://doi.org/10.3389/fphar.2022.955050
This article is part of the Research Topic Advances in antimicrobial therapy and combating resistance View all 7 articles
Introduction: Linezolid (LNZ) is an effective antibiotic to treat patients with multidrug-resistant tuberculosis (MDR-TB) treatment failure. M. tuberculosis strains resistant to isoniazid and rifampin are defined as MDR-TB. In recent years, resistance to LNZ among MDR-TB cases has been reported in several different countries. In this study, we performed a systematic review and meta-analysis to investigate the prevalence of LNZ resistance among MDR-TB isolates.
Methods: The databases of Embase, PubMed/Medline, and Web of Science were searched systematically from January 2000 to April 2021. Statistical analyses were performed by using Comprehensive Meta-Analysis software. Heterogeneity was reported by using the t-squared statistic and Q-statistic. Begg’s rank correlation in combination with the funnel plot were used to evaluate any possible publication bias.
Results: In total, 25 studies were selected for meta-analysis from 14 different countries; the majority was from China (n = 5) and Turkey (n = 4). Moreover, 7,366 patients were infected with MDR M. tuberculosis. Among the study population, 98 patients were co-infected with HIV, and 18 patients with hepatitis C virus (HCV). Furthermore, 28 cases had diabetes, and139 cases were alcohol abuser. Overall, 4,956 MDR M. tuberculosis strains were isolated from TB patients. The pooled frequency of LNZ resistance among the clinical isolates of MDR M. tuberculosis was 4.2% (95%). Begg’s (p = 0.72) test showed no evidence of publication bias.
Conclusion: LNZ resistance among MDR M. tuberculosis isolates is increasing. On the other hand, long-term treatment of MDR-TB cases with LNZ alone is associated with several adverse effects. Thus, it is recommended that newer anti-TB drugs, including bedaquiline and delamanid, in combination with linezolid could increase its effectiveness and decrease toxicities. However, more studies should be done in this field.
Mycobacterium tuberculosis (M. tuberculosis) is the leading cause of tuberculosis (TB), a disease with a major threat to the public health and millions of deaths worldwide (Azimi et al., 2017; Azimi et al., 2018). According to the 2020 global TB report by the World Health Organization (WHO), there were 10 million (range: 8.9–11.0 million) new TB cases and 1.2 million (range: 1.1–1.3 million) TB deaths among human immunodeficiency virus (HIV)-negative people in 2019 (WHO, 2020). The emergence of drug-resistant TB poses a danger to TB control throughout the world (Ahmed et al., 2013).
Isoniazid and rifampin are two effective and standard anti-TB drugs. M. tuberculosis strains resistant to these two antibiotics are defined as multidrug-resistant tuberculosis (MDR-TB) (Eker et al., 2008; Rey-Jurado et al., 2013; Guglielmetti et al., 2015). Based on the WHO estimates, virtually 3.3% of new TB cases and 17.7% of previously treated TB cases develop MDR-TB globally (WHO, 2020). Treatment of MDR-TB is performed using less efficient and more expensive second-line treatment drugs, with great adverse events. Moreover, unlike the treatment of drug-susceptible cases, that of MDR-TB needs a long period of time (Yang et al., 2018; Zong et al., 2018).
Linezolid (LNZ) is a member of the oxazolidinone class of antimicrobials that acquired the WHO license for clinical use. In 2016, the WHO recommended LNZ as the most important second-line drug for treating MDR-TB (Zong et al., 2018). Several in vitro and in vivo studies have revealed that LNZ has promising bacteriostatic activity against MDR-TB (Alcalá et al., 2003; Dietze et al., 2008; Tsona et al., 2010; Strydom et al., 2019). LNZ binds to domain V of 23S rRNA on the 50S ribosomal subunit, inhibits the formation of a 70S initiation complex, and prevents bacterial protein synthesis. It is presumed that mutation in two ribosomal proteins L3 and L4 and 23S rRNA is the main mechanism used by MDR-TB isolates, conferring resistance to LNZ (Zhang et al., 2014). In recent years, resistance to LNZ among MDR-TB cases has been reported in various countries (Richter et al., 2007; Pang et al., 2017). To this end, we performed a systematic review and meta-analysis to investigate the prevalence of LNZ resistance among MDR-TB isolates across the world.
The present systematic and meta-analysis study was carried out based on the Preferred Reporting Items for Systematic Review and Meta-Analyses (PRISMA) guidelines. To evaluate the prevalence of LNZ resistance among MDR-TB isolates, we conducted a literature search in three main electronic databases, including PubMed/Medline, Web of Science, and Embase, from 1 January 2000 to 12 April 2021. We performed the searching process using the following keywords: “Mycobacteriaceae”, “mycobacteria”, “mycobacterium”, “Mycobacterium tuberculosis”, “tubercle bacillus”, “pulmonary tuberculosis”, “tuberculosis”, “Multidrug Resistance”, “Multi-Drug Resistance”, “Multiple drug resistance”, “MDR”, “multiresistance”, “linezolid”, “linezolid”, “oxazolidinone class antibiotic”, and “protein synthesis inhibitors”. The systematic search process was accomplished based on the medical subject heading (MeSH) terms alone or in combination with “AND” and/or “OR”. The search was limited to the original articles published in English and indicated the prevalence or incidence of LNZ resistance among MDR M. tuberculosis isolates. We also searched the bibliographies for any retrieved articles for additional references.
The inclusion criteria included all the original papers presenting cross-sectional or cohort studies on the prevalence of LNZ resistance among MDR-TB. The selected studies were analyzed in terms of titles, abstracts, and full texts. The entire recorded studies included in our analysis based on the following criteria: original articles that provided sufficient data on LNZ resistance in MDR M. tuberculosis isolates, and those that used standard (conventional and molecular) methods. The conventional methods encompassed niacin accumulation, growth in Lowenstein-Jensen (LJ) media containing thiophene-carboxylic acid hydrazide (TCH), growth at 42 and 44°C, tolerance towards NaCl 5% and urease, pigment production in light and dark, catalase, arylsulfatase activity, Tween hydrolysis, tellurite reduction, and nitrate reduction, and molecular methods embraced PCR-RFLP and sequencing.
The exclusion criteria were comprised of review article, modelling study, commentary, correspondence, duplicate articles for the same investigation, editorial, guideline, news, and studies without enough data on LNZ resistance in MDR M. tuberculosis isolates.
In the first step, we saved all initial records collected during database searching in a Word file, and primary screening was performed based on their topics and/or abstracts. In the next step, the full-texts of potentially eligible records were downloaded. Then the inclusion criteria and final eligibility for the downloaded full texts were evaluated by two trained researchers (TA and MH). The full texts of potentially eligible articles were read objectively and with details by these two researchers. Any disagreement between the two researchers was resolved by the decision of a third trained researcher (MJN). The extraction of necessary data was accomplished by two authors (SK and AA), and all obtained data were rechecked by other authors (TA, MH, and MJN). After extracting from each relevant article, data, including first author, date of investigation, year of publication, country/continent, diagnostic method, number of MDR M. tuberculosis, number and percentage of LNZ resistance, drug susceptibility testing, and source of isolates, were saved in Excel software (Microsoft, Redmond, WA, United States).
We assessed all reviewed studies based on the quality assessment checklist, which was provided by the Joanna Briggs Institute (Joanna Briggs Institute, 2014).
Meta-analysis was performed using Comprehensive Meta-Analysis (Biostat V2.2) software. The amount of residual heterogeneity was evaluated by the t-squared statistic and the Q-statistic to test the heterogeneity between the inquiries. To assess any possible publication bias, Begg’s rank correlation Egger’s weighted regression methods was used in combination with a funnel plot. The probability level of p < 0.05 was considered as statistically significant publication bias.
Following the initial search of databases, a total of 2,127 articles were retrieved. After screenings the title/abstract and full texts, we selected 25 studies in the final evaluation (Figure 1). Table 1 depicts an overview of the main characteristics of the included studies. All the selected studies reported the prevalence of LNZ resistance among patients infected with MDR M. tuberculosis isolates, and mycobacteria were confirmed by microscopy, culture, and drug susceptibility tests. The location of studies covered all the continents except Africa. These studies were from 14 different countries, with the majority from the China (n = 5), Turkey (n = 4), United States (n = 2), Thailand (n = 2), France (n = 2), and Taiwan (n = 2). Other countries were comprised of Germany, Russia, Korea, Iran, India, Spain, India, and Italy, with one study. A total of 7,366 patients infected with MDR M. tuberculosis were enrolled in the current systematic review and meta-analysis. Of the study population, 98 patients were co-infected with HIV and 18 patients with hepatitis C virus (HCV). In addition, 139 cases were alcohol abuser, and 28 cases had diabetes.
In total, 4,956 MDR M. tuberculosis strains were isolated from the patients with TB. Further analysis showed that 113 strains were LNZ resistant. As illustrated in Figure 2, the pooled frequency of LNZ resistance among the clinical isolates of MDR M. tuberculosis was 4.2% (95% CI: 3.5–5.0).
FIGURE 2. Pooled prevalence of linezolid resistance in multidrug-resistant Mycobacterium tuberculosis isolates. CI: confidence interval.
As presented in Table 1 and based on studies carried out in different countries, Spain (22.2%; 95% CI: 5.6–57.9) and United States (0.2%; 95% CI: 0.0–3.4) had the highest and lowest rate of resistance to LNZ in MDR M. tuberculosis isolates, respectively. We calculated the resistance to LNZ in MDR M. tuberculosis isolates among countries with more than one study and per group of years (Table 2). Our analyses revealed that China with five studies (LNZ resistance: 5.8%) and France with two studies (LNZ resistance: 0%) had the highest and lowest rate of resistance to LNZ, respectively.
As time trend analysis of LNZ resistance in MDR M. tuberculosis is shown in Table 3, there was no specific pattern of LNZ resistance during 2008–2021. However, variation in LNZ resistance during the mentioned time period is considerable. Altogether, the rate of LNZ resistance during 2008–2012 was lower (0%) than that of other years. The highest rate of LNZ resistance was reported in 2013 (7.2%), followed by 2021 (6%).
Figure 3 provides a funnel plot of standard error. From the data in this Figure and according to Begg’s (p = 0.72) test, it can be found that there was not evidence of publication bias.
FIGURE 3. Funnel plot of the meta-analysis of Linezolid resistance in multidrug-resistant Mycobacterium tuberculosis isolates.
Globally, drug resistance among M. tuberculosis is increasing, and surveillance information reveals that MDR-TB has become an emerging and a major public health problem in many countries (Ahmed et al., 2013). Treatment of MDR-TB is exceedingly difficult and requires a prolonged time and multiple second-line drugs. Moreover, the lack of a standard regime for the treatment of MDR-TB infection can lead to the widespread person-to-person spread of drug-resistant organisms (Borisov et al., 2017). In the present systematic and meta-analysis study, the worldwide prevalence of LNZ resistance among MDR-TB isolates was investigated from 2000 to 2021. To the best of our knowledge, the current research is the first comprehensive systematic review on the prevalence of LNZ resistance among MDR-TB isolates in the world. The main goal of this study is to help the reduction of antibiotic resistance and effective control of infectious disease outbreaks.
The current meta-analysis study revealed that the overall estimate of LNZ resistance among clinical isolates of MDR-TB was 4.2%. Likewise, LNZ resistance rate among the clinical isolates of MDR-TB in 14 different countries showed that Spain (22.2%) and United States (0.2%) had the highest and lowest prevalence rate, respectively. Altogether, our analyses suggested that the LNZ resistance rate is 4.2%. In most cases, resistance to LNZ is related to four types of mutation, including T460C in rplC, G2576T, G2447T, and G2061T in 23 S rRNA (Zong et al., 2018). Moreover, the efflux pumps are another factor giving rise to low-level resistance to LNZ among extensively drug-resistant tuberculosis (XDR-TB) isolates (Zhang et al., 2014). In general, LNZ has bacteriostatic activity against M. tuberculosis, and with great penetration into infected tissues, such as lungs and cerebrospinal fluid, this drug can be selected as a promising antibiotic for treating MDR-TB and XDR-TB (Tornheim et al., 2020). However, it has been indicated that the long-term treatment of M. tuberculosis infections with LNZ alone is associated with several adverse effects, particularly painful peripheral neuropathy, optic neuritis, vision-threatening, myelosuppression, and drug interactions (Zong et al., 2018; Gavali et al., 2019). Many different published in vivo and in vitro studies have explored that LNZ in combination with other antimicrobials, e.g. delpazolid, rifampicin, isoniazid, fluoroquinolones, bedaquiline, amikacin, and clofazimine, has effective outcomes and high safety profiles and can improve treatment consequences (Limoncu et al., 2011; Rey-Jurado et al., 2013; Zong et al., 2018). It has been revealed that combination therapy could reduce the length of treatment of active tuberculosis. Furthermore, in asymptomatic patients, combination therapy could destroy the latent M. tuberculosis isolates (Limoncu et al., 2011). The current systematic and meta-analyses review was performed on 25 studies from 14 different countries. Therefore, the main limitation of our study was the lack of further studies from more different countries and regions of the world.
Our analyses uncovered that the LNZ resistance among MDR-TB is 4.2%, and this antimicrobial drug is used as an anti-TB drug in treatment regimes. However, considering several adverse events reported in studies, combination therapy could reduce LNZ toxicities associated with overdose concentrations. We believe that combination therapy, including LNZ plus rifampicin, LNZ plus isoniazid, linezolid-delpazolid, or LNZ with bedaquiline and fluoroquinolones or clofazimine, could have the highest synergistic result against MDR-TB and XDR-TB isolates compared to LNZ alone. However, additional comparative studies are needed to be conducted in different countries to recognize the possible profits of combination therapies in treating MDR-TB and XDR-TB isolates.
The raw data supporting the conclusions of this article will be made available by the authors, without undue reservation.
TA, SK, AA, MH, HM, VHK, MH, and MJN contributed in revising and final approval of the version to be published. All authors agreed and confirmed the manuscript for publication.
The authors declare that the research was conducted in the absence of any commercial or financial relationships that could be construed as a potential conflict of interest.
All claims expressed in this article are solely those of the authors and do not necessarily represent those of their affiliated organizations, or those of the publisher, the editors and the reviewers. Any product that may be evaluated in this article, or claim that may be made by its manufacturer, is not guaranteed or endorsed by the publisher.
Ahmed, I., Jabeen, K., Inayat, R., and Hasan, R. (2013). Susceptibility testing of extensively drug-resistant and pre-extensively drug-resistant Mycobacterium tuberculosis against levofloxacin, linezolid, and amoxicillin-clavulanate. Antimicrob. Agents Chemother. 57 (6), 2522–2525. doi:10.1128/AAC.02020-12
Alcalá, L., Ruiz-Serrano, M. J., Turégano, C. P-F., De Viedma, D. G., Díaz-Infantes, M., Marin-Arriaza, M., et al. (2003). In vitro activities of linezolid against clinical isolates of Mycobacterium tuberculosis that are susceptible or resistant to first-line antituberculous drugs. Antimicrob. Agents Chemother. 47 (1), 416–417. doi:10.1128/aac.47.1.416-417.2003
Azimi, T., Nasiri, M. J., Zamani, S., Hashemi, A., Goudarzi, H., Fooladi, A. A. I., et al. (2018). High genetic diversity among Mycobacterium tuberculosis strains in Tehran, Iran. J. Clin. Tuberc. Other Mycobact. Dis. 11, 1–6. doi:10.1016/j.jctube.2018.01.001
Azimi, T., Shariati, A., Fallah, F., Imani Fooladi, A. A., Hashemi, A., Goudarzi, H., et al. (2017). Mycobacterium tuberculosis genotyping using MIRU-VNTR typing. J. Mazandaran Univ. Med. Sci. 27 (149), 40–48.
Bektöre, B., Haznedaroglu, T., Baylan, O., Ozyurt, M., Ozkutuk, N., Satana, D., et al. (2013). Investigation of extensive drug resistance in multidrug resistance tuberculosis isolates. Mikrobiyol. Bul. 47 (1), 59–70. doi:10.5578/mb.4229
Borisov, S. E., Dheda, K., Enwerem, M., Leyet, R. R., D'Ambrosio, L., Centis, R., et al. (2017). Effectiveness and safety of bedaquiline-containing regimens in the treatment of MDR-and XDR-TB: A multicentre study. Eur. Respir. J. 49 (5), 1700387. doi:10.1183/13993003.00387-2017
Cambau, E., Viveiros, M., Machado, D., Raskine, L., Ritter, C., Tortoli, E., et al. (2015). Revisiting susceptibility testing in MDR-TB by a standardized quantitative phenotypic assessment in a European multicentre study. J. Antimicrob. Chemother. 70 (3), 686–696. doi:10.1093/jac/dku438
Cavanaugh, J. S., Jou, R., Wu, M-H., Dalton, T., Kurbatova, E., Ershova, J., et al. (2017). Susceptibilities of MDR Mycobacterium tuberculosis isolates to unconventional drugs compared with their reported pharmacokinetic/pharmacodynamic parameters. J. Antimicrob. Chemother. 72 (6), 1678–1687. doi:10.1093/jac/dkx022
Chaiprasert, A., Srimuang, S., Tingtoy, N., Makhao, N., Sirirudeeporn, P., Tomnongdee, N., et al. (2014). Second-line drug susceptibilities of multidrug-resistant tuberculosis strains isolated in Thailand: An update. Int. J. Tuberc. Lung Dis. 18 (8), 961–963. doi:10.5588/ijtld.13.0197
Coban, A. Y., Bilgin, K., Uzun, M., and Durupinar, B. (2009). Effect of linezolid in combination with isoniazid and rifampicin against multidrug resistant Mycobacterium tuberculosis clinical isolates. Mikrobiyol. Bul. 43 (2), 293–297.
Dietze, R., Hadad, D. J., McGee, B., Molino, L. P. D., Maciel, E. L. N., Peloquin, C. A., et al. (2008). Early and extended early bactericidal activity of linezolid in pulmonary tuberculosis. Am. J. Respir. Crit. Care Med. 178 (11), 1180–1185. doi:10.1164/rccm.200806-892OC
Eker, B., Ortmann, J., Migliori, G. B., Sotgiu, G., Muetterlein, R., Centis, R., et al. (2008). Multidrug-and extensively drug-resistant tuberculosis, Germany. Emerg. Infect. Dis. 14 (11), 1700–1706. doi:10.3201/eid1411.080729
Gavali, D., Aring, B., Mullan, S., Nathametha, A. A., and Dave, A. B. (2019). Evaluation of sensitivity and resistance of linezolid in pre extensively drug resistance tuberculosis and extensively drug resistance tuberculosis at a tertiary care hospital, jamnagar, Gujarat, India. J. Clin. Diagnostic Res. 13, 11. doi:10.7860/JCDR/2019/42544.13298
Guglielmetti, L., Le Du, D., Jachym, M., Henry, B., Martin, D., Caumes, E., et al. (2015). Compassionate use of bedaquiline for the treatment of multidrug-resistant and extensively drug-resistant tuberculosis: Interim analysis of a French cohort. Clin. Infect. Dis. 60 (2), 188–194. doi:10.1093/cid/ciu786
Huang, T-S., Liu, Y-C., Sy, C-L., Chen, Y-S., Tu, H-Z., Chen, B-C., et al. (2008). In vitro activities of linezolid against clinical isolates of Mycobacterium tuberculosis complex isolated in Taiwan over 10 years. Antimicrob. Agents Chemother. 52 (6), 2226–2227. doi:10.1128/AAC.00414-07
Joanna Briggs Institute (2014). Joanna Briggs Institute reviewers’ manual: 2014 edition. Australia: The Joanna Briggs Institute.
Kardan-Yamchi, J., Kazemian, H., Battaglia, S., Abtahi, H., Foroushani, A. R., Hamzelou, G., et al. (2020). Whole genome sequencing results associated with minimum inhibitory concentrations of 14 anti-tuberculosis drugs among rifampicin-resistant isolates of mycobacterium tuberculosis from Iran. J. Clin. Med. 9 (2), 465. doi:10.3390/jcm9020465
Limoncu, M. H., Ermertcan, Ş., Erac, B., and Taşli, H. (2011). An investigation of the antimicrobial impact of drug combinations against Mycobacterium tuberculosis strains. Turkish J. Med. Sci. 41 (4), 719–724. doi:10.3906/sag-1007-934
Lai, C-C., Tan, C-K., Huang, Y-T., Chou, C-H., Hung, C-C., Yang, P-C., et al. (2008). Extensively drug-resistant Mycobacterium tuberculosis during a trend of decreasing drug resistance from 2000 through 2006 at a medical center in Taiwan. Clin. Infect. Dis. 47 (7), e57–e63. doi:10.1086/591702
Pang, Y., Zong, Z., Huo, F., Jing, W., Ma, Y., Dong, L., et al. (2017). In vitro drug susceptibility of bedaquiline, delamanid, linezolid, clofazimine, moxifloxacin, and gatifloxacin against extensively drug-resistant tuberculosis in Beijing, China. Antimicrob. Agents Chemother. 61 (10), e00900–17. doi:10.1128/AAC.00900-17
Pholwat, S., Heysell, S., Stroup, S., Foongladda, S., and Houpt, E. (2011). Rapid first-and second-line drug susceptibility assay for Mycobacterium tuberculosis isolates by use of quantitative PCR. J. Clin. Microbiol. 49 (1), 69–75. doi:10.1128/JCM.01500-10
Rey-Jurado, E., Tudó, G., de la Bellacasa, J. P., Espasa, M., and Gonzalez-Martín, J. (2013). In vitro effect of three-drug combinations of antituberculous agents against multidrug-resistant Mycobacterium tuberculosis isolates. Int. J. Antimicrob. Agents 41 (3), 278–280. doi:10.1016/j.ijantimicag.2012.11.011
Riccardi, N., Alagna, R., Saderi, L., Ferrarese, M., Castellotti, P., Mazzola, E., et al. (2019). Towards tailored regimens in the treatment of drug-resistant tuberculosis: A retrospective study in two Italian reference centres. BMC Infect. Dis. 19 (1), 564. doi:10.1186/s12879-019-4211-0
Richter, E., Rüsch-Gerdes, S., and Hillemann, D. (2007). First linezolid-resistant clinical isolates of Mycobacterium tuberculosis. Antimicrob. Agents Chemother. 51 (4), 1534–1536. doi:10.1128/AAC.01113-06
Şimşek, H., Tarhan, G., and Cesur, S. (2015). Evaluation of second-line antituberculosis drug susceptibilities of multidrug-resistant mycobacterium tuberculosis complex isolates by E-test method. Mikrobiyol. Bul. 49 (1), 47–55. doi:10.5578/mb.8602
Strydom, N., Gupta, S. V., Fox, W. S., Via, L. E., Bang, H., Lee, M., et al. (2019). Tuberculosis drugs’ distribution and emergence of resistance in patient’s lung lesions: A mechanistic model and tool for regimen and dose optimization. PLoS Med. 16 (4), e1002773. doi:10.1371/journal.pmed.1002773
Tornheim, J. A., Intini, E., Gupta, A., and Udwadia, Z. F. (2020). Clinical features associated with linezolid resistance among multidrug resistant tuberculosis patients at a tertiary care hospital in Mumbai, India. J. Clin. Tuberc. Other Mycobact. Dis. 20, 100175. doi:10.1016/j.jctube.2020.100175
Tsona, A., Metallidis, S., Foroglou, N., Selviaridis, P., Chrysanthidis, T., Lazaraki, G., et al. (2010). Linezolid penetration into cerebrospinal fluid and brain tissue. J. Chemother. 22 (1), 17–19. doi:10.1179/joc.2010.22.1.17
Wang, G., Jiang, G., Jing, W., Zong, Z., Yu, X., Chen, S., et al. (2021). Prevalence and molecular characterizations of seven additional drug resistance among multidrug-resistant tuberculosis in China: A subsequent study of a national survey. J. Infect. 82 (3), 371–377. doi:10.1016/j.jinf.2021.02.004
Yang, C., Lei, H., Wang, D., Meng, X., He, J., Tong, A., et al. (2012). In vitro activity of linezolid against clinical isolates of Mycobacterium tuberculosis, including multidrug-resistant and extensively drug-resistant strains from Beijing, China. Jpn. J. Infect. Dis. 65 (3), 240–242. doi:10.7883/yoken.65.240
Yang, J. S., Kim, K. J., Choi, H., and Lee, S. H. (2018). Delamanid, bedaquiline, and linezolid minimum inhibitory concentration distributions and resistance-related gene mutations in multidrug-resistant and extensively drug-resistant tuberculosis in Korea. Ann. Lab. Med. 38 (6), 563–568. doi:10.3343/alm.2018.38.6.563
Zhang, Z., Pang, Y., Wang, Y., Liu, C., and Zhao, Y. (2014). Beijing genotype of Mycobacterium tuberculosis is significantly associated with linezolid resistance in multidrug-resistant and extensively drug-resistant tuberculosis in China. Int. J. Antimicrob. Agents 43 (3), 231–235. doi:10.1016/j.ijantimicag.2013.12.007
Zheng, H., He, W., Jiao, W., Xia, H., Sun, L., Wang, S., et al. (2021). Molecular characterization of multidrug-resistant tuberculosis against levofloxacin, moxifloxacin, bedaquiline, linezolid, clofazimine, and delamanid in southwest of China. BMC Infect. Dis. 21 (1), 330. doi:10.1186/s12879-021-06024-8
Zong, Z., Jing, W., Shi, J., Wen, S., Zhang, T., Huo, F., et al. (2018). Comparison of in vitro activity and MIC distributions between the novel oxazolidinone delpazolid and linezolid against multidrug-resistant and extensively drug-resistant Mycobacterium tuberculosis in China. Antimicrob. Agents Chemother. 62 (8), e00165–18. doi:10.1128/AAC.00165-18
Keywords: tuberculosis, meta-analysis, linezolid, TB, MDR-TB, resistance
Citation: Azimi T, Khoshnood S, Asadi A, Heidary M, Mahmoudi H, Kaviar VH, Hallajzadeh M and Nasiri MJ (2022) Linezolid resistance in multidrug-resistant mycobacterium tuberculosis: A systematic review and meta-analysis. Front. Pharmacol. 13:955050. doi: 10.3389/fphar.2022.955050
Received: 28 May 2022; Accepted: 15 July 2022;
Published: 30 August 2022.
Edited by:
Ahmed F. El-Yazbi, Alexandria University, EgyptReviewed by:
Alaa Abouelfetouh, Alexandria university, EgyptCopyright © 2022 Azimi, Khoshnood, Asadi, Heidary, Mahmoudi, Kaviar, Hallajzadeh and Nasiri. This is an open-access article distributed under the terms of the Creative Commons Attribution License (CC BY). The use, distribution or reproduction in other forums is permitted, provided the original author(s) and the copyright owner(s) are credited and that the original publication in this journal is cited, in accordance with accepted academic practice. No use, distribution or reproduction is permitted which does not comply with these terms.
*Correspondence: Mohsen Heidary, bW9oc2VuaGVpZGFyeTQwQGdtYWlsLmNvbQ==; Mohammad Javad Nasiri, bWoubmFzaXJpQGhvdG1haWwuY29t
†These authors have contributed equally to this work and share first authorship
Disclaimer: All claims expressed in this article are solely those of the authors and do not necessarily represent those of their affiliated organizations, or those of the publisher, the editors and the reviewers. Any product that may be evaluated in this article or claim that may be made by its manufacturer is not guaranteed or endorsed by the publisher.
Research integrity at Frontiers
Learn more about the work of our research integrity team to safeguard the quality of each article we publish.