- 1College of Pharmacy, Shandong University of Traditional Chinese Medicine, Jinan, China
- 2Shandong First Medical University Affiliated Shandong Tumor Hospital and Institute, Shandong Cancer Hospital and Institute, Jinan, China
Atractylodes macrocephala Koidz (AM), traditional Chinese medicine (TCM) with many medicinal values, has a long usage history in China and other oriental countries. The phytochemical investigation revealed the presence of volatile oils, polysaccharides, lactones, flavonoids, and others. The polysaccharides from AM are important medicinal components, mainly composed of glucose (Glc), galactose (Gal), rhamnose (Rha), arabinose (Ara), mannose (Man), galacturonic acid (GalA) and xylose (Xyl). It also showed valuable bioactivities, such as immunomodulatory, antitumour, gastroprotective and intestinal health-promoting, hepatoprotective, hypoglycaemic as well as other activities. At the same time, based on its special structure and pharmacological activity, it can also be used as immune adjuvant, natural plant supplement and vaccine adjuvant. The aim of this review is to summarize and critically analyze up-to-data on the chemical compositions, biological activities and applications of polysaccharide from AM based on scientific literatures in recent years.
Introduction
AM is the dry rhizome of Atractylodes macrocephala Koidz, which was first recorded in the ‘Shennong’s classic of materia medica’ and listed as the top grade. It is one of the common Chinese herbal medicines with high formula frequency in China, and it is used for treating diseases such as spleen hypofunction, loss of appetite, abdominal distension, diarrhoea, dizziness, and heart palpitations (Liu W. et al., 2021). There are more than 4755 Chinese patent medicines containing AM in China, which have been widely used because of their medicinal value and good clinical efficacy. AM (called “Baizhu” in China) is recorded in Chinese Pharmacopoeia (2020 version). AM has been prescribed as a traditional medicine in China, Korea and Japan for thousands of years (Editorial Committee of Flora of China, 2015; Kim et al., 2016; Liu J. et al., 2021). It is traditional Chinese medicine and edible plant having a health-protective effect. In recent years, a large number of related health care products have been listed, such as Jianwei Xiaoshi Tea, Huangqi Baizhu oral liquid, etc. At the same time, it is recorded in ancient Chinese medicine books that AM has the functions of removing blackness, replenishing qi and nourishing blood, whitening and nourishing, so it is fully used in beauty and medicinal food products. In the last few decades, various chemical components have been isolated from AM, and its chemical constituents include volatile oils, polysaccharides, lactones, flavonoids and other substances (Gu et al., 2019; Wang P. et al., 2020; Yang et al., 2021).
Polysaccharides are widely distributed in vegetation, animals, fungi and bacteria, and they have become hot spots in contemporary medical research. Many studies have shown that polysaccharides from AM are important biological active components. It possesses many pharmacological activities, such as enhancing immunity, antitumour, gastroprotective, hepatoprotective, intestinal health-promoting, hypoglycaemic, antioxidant, etc. (Zhu et al., 2018). This review is the first of its kind that gives a comprehensive discussion on the recent findings related to chemical composition, biological activities, pharmacological effects, and applications of polysaccharides from AM. It will construct a new foundation for further study of its mechanism and development of better therapeutic agent and healthy products.
Chemical composition and structure
Polysaccharide is one of the important active components of AM. Most studies have shown that polysaccharide from AM is a heteropolysaccharide composed of glucose, galactose, rhamnose, arabinose, mannose, galacturonic acid, xylose, etc. Among them, the proportion of Glc, Gal and Man is the highest, which is the main monosaccharide of polysaccharides from AM. The molecular weight is between 2.1 and 220 kDa. It is found that 1→3, 1→6, and 1→3.6 bond-type Glc is the main bond type of polysaccharides from AM, followed by 1→3 and 1→6 bond-type Gal. The chemical composition of polysaccharide from AM is shown in Table 1. The molecular weight, composition, molar ratio and sugar chain structure of polysaccharide from AM is different from those obtained from different extraction and purification methods. At the same time, polysaccharides with uncomplicated structures often do not have complex helical structures, and usually exist in the form of random coils in solution, and their conformations can be changed by metal ions, denaturants, and temperature. UAM is a homogeneous polysaccharide composed of glucose, mainly connected by β-D-1→3 and β-D-1→3.6, with simple structure and small molecular weight. Congo red test, viscometry, spectrophotometry, UV absorption and circular dichroism were used to analyze the behavior of UAM solution and the conformation of molecular chain in the solution. It was found that in the DMSO solvent system, UAM was in an irregular chain shape, without fixed form, and was easily affected by the external environment (Wang, 2012). Therefore, it is of great significance to control the extraction and purification methods of polysaccharides to ensure the structure and biological activity of AM. UAM, WAM and inulin-type polysaccharide of AM are three polysaccharides from AM with well-defined structures (Figure 1).
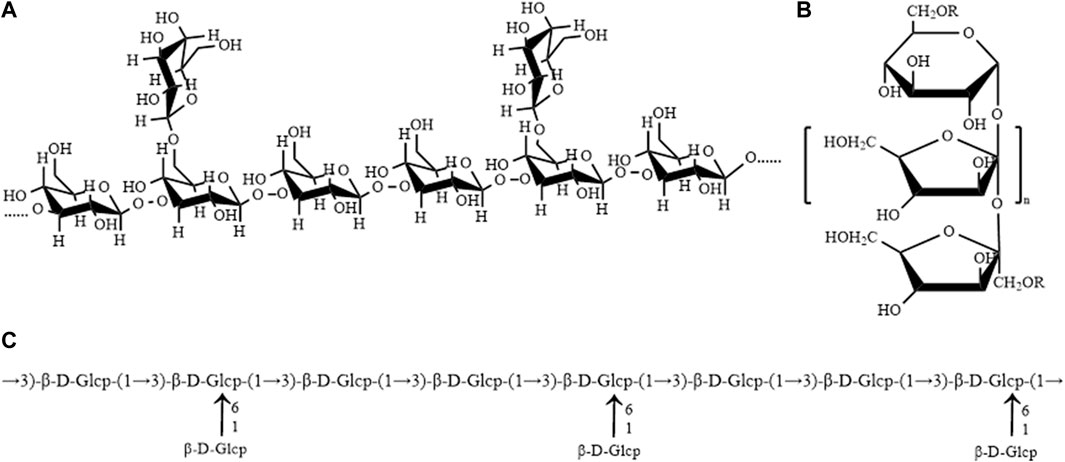
FIGURE 1. (A) Structure of UAM (B) Structure of inulin-type polysaccharide of AM (C) Structure of WAM (Wu et al., 2011; Wang, 2012; Lin et al., 2015).
Biological activities
Immunomodulatory activity
Polysaccharides from AM have an immunomodulatory effect, and it is also known as Biological Response Modifier. The immunomodulatory effect is one of the main research areas of polysaccharides from AM, and its immunomodulatory activity has been demonstrated by a large number of in vitro and in vivo research experiments. (Li R. et al., 2018; Zhu et al., 2018; Yin et al., 2019). The following will introduce the immunomodulatory effects of polysaccharides from AM from four aspects: immune organs, immune cells, immune molecules, other signaling pathways and intracellular messengers (Table 2). The immune organs are composed of central immune organs (including bone marrow, thymus and bursa in poultry) and peripheral immune organs (including spleen, lymph and mucosal immune system etc.). The immune organs are responsible for immunogenesis, differentiation, maturation, settlement, proliferation, and immune response. The thymus is the site of T lymphocytes differentiation and maturation. The spleen is the body’s largest immune organ and produces many lymphocytes, especially B lymphocytes (Jiang et al., 2010; Wang et al., 2012; Xu and Tian, 2015a). Therefore, the developmental status of immune organs can determine the body’s immune regulation level. Thymus index, spleen index and weight are usually used as the initial indicators of immune function. A large number of experiments have proved that polysaccharides from AM can significantly increase the spleen and thymus index and body weight of experimental animals.
Immune cells can be directly or indirectly involved in the body’s immune regulation, thus playing a role in the immune system. Immune cells include macrophages (Mφ), natural killer (NK) cells, mast cells, T cells, B cells, dendritic cells (DC) etc. (Yang J. T. et al., 2018). Mφ can phagocytose and eliminate foreign pathogens. Polysaccharides from AM can bind with macrophage-specific membrane receptors to activate the immune response, which has the effect of directly killing pathogens. In addition, Mφ can also secrete various cytokines to enhance the body’s immune response (Yang et al., 2010; Hou et al., 2017). T cells are the primary effector cells of cellular immunity, including helper T (Th) cells and cytotoxic T (TC) cells (Zhuang et al., 2020). Th cells help B cells differentiate and make antibodies or help Mφ destroy intracellular pathogens. TC cells exert immune regulation mainly through CD8+ T cells, inhibiting T cell proliferation, antibody synthesis and secretion. The stability of the CD4+/CD8+ ratio is an important marker for maintaining an effective immune response (Liu et al., 2021). B cells mainly produce antibodies and enhance humoral immunity. Polysaccharides from AM can directly or indirectly increase the number of T cells or B cells and play an immunomodulatory role. DC mainly migrates and delivers captured antigens to Th cells, promotes T cell polarisation, and participates in regulating B cell function (Kara et al., 2014). The main effect of polysaccharides from AM on DC is to promote the expression of its surface molecules and the secretion of cellular molecules, thus enhancing the immune function of the organism.
The effects of polysaccharides from AM on immune molecules are mainly through affecting cytokines and antibody molecules. Cytokines are multi-functional active protein molecules, often involved in immune regulation, inflammation, hematopoiesis and other biological processes (Butcher and Zhu, 2021). The main families of cytokines include interleukins (ILs), chemokines, colony-stimulating factors (CSF), granulocyte-macrophage colony-stimulating factors (GM-CSF), interferons (IFNs) and tumour necrosis factors (TNF). Among them, IL-2, GM-CSF, TNF, and IFN-γ are secreted by Th1 cells and related to tumour cells’ immune response. IL-4, IL-5, IL-6, and IL-10 are secreted by Th2 cells and related to tumour cells’ immune escape. The secretion of ILs is regulated by forkhead lineage-transcription factor (FoxP3), which can increase the expression of FoxP3 by promoting the phosphorylation of signal transducers and activators of transcription 5 (STAT5) (Chen, 2021; Schmetterer and Pickl, 2017). Therefore, cytokines can act directly or indirectly on immune cells and exert biological effects. The antibody is a kind of immunoglobulin that can specifically bind to the antigen. Its structure can be divided into IgG, IgA, IgM, IgE, and IgD, with a certain immune regulation effect (Wei et al., 2017).
Cyclophosphamide (CTX) is an alkylating agent widely used in immunotherapy and cancer chemotherapy. However, it has many side effects, such as immunosuppression, myelosuppression, leukopenia, oxidative stress, disruption of Th1/Th2 balance and induction of decreased absolute T and B cell counts (Bordbar et al., 2011; Deng et al., 2018; Sun et al., 2018; Yesillik and Gupta, 2020; Boucher et al., 2021). Xiang et al. (Xiang et al., 2020) studied the mechanism of polysaccharides from AM in alleviating the decline of T cell activation induced by CTX. The results showed that polysaccharides from AM could significantly improve spleen indexes, balance Th1/Th2 ratio, alleviate abnormal shape and death of spleen cells, and increase the expression levels of IL-2, IL-6, TNF-α, and IFN-γ mRNA in serum of mice. Li et al. (Li et al., 2021a) also proved that polysaccharides from AM could alleviate the immunosuppressive effect of CTX on the goose. Sun et al. (Sun et al., 2015) proved that RAMPStp could promote the proliferation of T cells, promote the lymphocytes to enter S and G2/M phases, and significantly increase the percentage of CD4+T cells and CD8+T cells.
In addition, polysaccharides from AM can also affect the activities of intracellular messenger substances and related signal pathways. Nitric oxide (NO) can directly act on Mφ as a cytotoxic effector molecule to inhibit cancer cell immune response and improve the body’s immunity (Guzik et al., 2003). Ca2+ acts as the second messenger of the inositol phospholipid metabolic pathway. It is involved in the synthesis of lymphocytes DNA and cells growth, differentiation, and proliferation, thus regulating the body’s immune function.
Cyclic AMP (cAMP) and cyclic GMP (cGMP) are involved in cells activation and lymphocytes differentiation (Hou et al., 2017). Mitogen-activated protein kinase (MAPK) is the main signal pathway of cells oxidative stress and injury, including extracellular signal-regulated kinase (ERK), c-Jun N-terminal kinase (JNK) and p38, which can regulate cell growth, differentiation, and cell pathology (Kumar et al., 2015). CD28+ is an essential second signal in T cell activation by inducing phospholipase Cγ-1 (PLCγ-1) phosphorylation, which causes PLCγ-1 to hydrolyze inositol phospholipids on the membrane to generate inositol 1,4,5-trisphosphate receptor (IP3R) and diacylglycerol (DAG) (Boucher et al., 2021). DAG activates the protein kinase C (PKC) signalling pathway, which activates the phosphorylation of T nuclear factor (NFAT), activating protein 1 (AP-1) and nuclear factor κappa B (NF-κB). After being regulated by apoptosis-related gene B cell lymphoma-2 (Bcl-2), the Caspase family can directly destroy cell structure and induce mitochondrial apoptosis (Häcker, 2018). Related studies have found that Smac/DIABLO and Omi/HtrA2 are present in mitochondria, which can be released from mitochondria into cytoplasm when the cell structure is disrupted, thus serving as a marker of mitochondrial apoptosis (Grosser et al., 2021; Vega-Naredo et al., 2021).
Toll-like receptor 4 (TLR4) signalling is a classical pathway of polysaccharide mediated immunoregulation (Li et al., 2022; Zhao et al., 2022). Myelogenous differentiation factor 88 (MyD88) is the key downstream signal ligand of the TLR4 signalling pathway (Bates et al., 2020), which can induce resting nuclear transcription factor NF-κB to enter the cell nucleus, activate related gene transcription, promote the expression of inducible nitric oxide synthase, NO and cytokines, and activate the T cells to produce an immune response. Li et al. (Li B. X. et al., 2019) explored whether polysaccharides from AM could regulate mouse spleen function through the TLR4 signalling pathway. The results showed that the expressions of TLR4, MyD88, tumour necrosis factor receptor-associated factor 6 (TRAF6), TRAF3, and NF-κB mRNA in the spleen of mice in the polysaccharides of AM group were significantly increased. Therefore, the results suggest that polysaccharides from AM can act on the TLR4-MyD88-NF-κB signalling pathway and play an immune regulatory role.
In conclusion, polysaccharides from AM can increase the weight of the spleen and thymus, reduce the damage to the immune organs, activate the immune cells, promote phagocytic activity, lymphocyte proliferation, associated cytokine secretion, antibody production, and improve the body’s immune system (Guo et al., 2012; Suabjakyong et al., 2015; Zhu et al., 2018). The related mechanisms are shown in Figure 2. These results suggest that polysaccharides from AM have potential as a therapeutic agent for as an immunomodulatory agent.
Antitumour activity
Cancer is one of the major threats to human health. Radiotherapy and chemotherapy are currently widely used in cancer treatment, but most drugs used to treat cancer have serious side effects. Therefore, it is necessary to find new drugs or methods to replace the traditional cancer therapies (Yu et al., 2020). Plant polysaccharides are bioactive macromolecular substances extracted from plants, which have been proved to have antitumour activity with a high safety profile and alleviate the side effects of chemotherapy (Tian et al., 2017; Yu et al., 2018; Chen and Huang, 2019; Liu and Huang, 2019). It has been reported that Astragalus polysaccharide (Li et al., 2020a), Hericium erinaceus polysaccharide (Liu et al., 2020), Angelica sinensis polysaccharide (Yang J. et al., 2018) and Lentinus edodes polysaccharide (Xu et al., 2018) have antitumour activity. The polysaccharides from AM showed extensive antitumour activity on many kinds of tumour cells, such as oesophagal cancer, liver cancer, malignant glioma, colorectal cancer and lung cancer cells (Kou et al., 2017; Zhu et al., 2018). The anticancer activity is mediated via inducing tumour cell apoptosis, inhibiting tumour cell migration, invasion and metastasis, regulating the tumour microenvironment and immune function, and inhibiting tumour cell proliferation (Chen and Huang, 2018) (Table 3).
The apoptosis of tumour cells induced by TCM includes two signalling pathways (Jearawuttanakul et al., 2020; Obeng, 2021): the exogenous pathway, activated by extracellular death signals. The other is the endogenous pathway, also known as the mitochondrial apoptosis pathway, in which mitochondria induce apoptosis in tumour cells. The mitochondrial apoptosis pathway mainly mediates the apoptosis of tumour cells induced by polysaccharides from AM.
Caspase and polyadenosine diphosphate ribose polymerase (PARP) are the key enzymes of apoptosis, which can degrade the proteins in cancer cells and cause the cancer cells to die irreversibly (Okubo et al., 2017; Van Opdenbosch and Lamkanfi, 2019). Cytochrome C (Cyt-C) is present in mitochondria, and when the endogenous mitochondrial signalling pathway is activated, it causes mitochondrial outer membrane permeation (MOMP), and Cyt-C enters the cytoplasm to initiate Caspase cascade reaction and induce apoptosis (Orzalli and Kagan, 2017; Carneiro and El-Deiry, 2020). In addition, Bcl-2-associated X protein (Bax) has a pro-apoptotic effect, while Bcl-2 inhibits apoptosis. Feng et al. (Feng et al., 2019a) conducted structural analysis and in vitro cell experiments on the prepared alcohol-soluble polysaccharide and found that APA at a dose of 2 mg/ml could inhibit the proliferation of Eca109 cells by 74.63%, indicating that APA may reduce MOMP, increase Cyt-C, Caspase-9 and Caspase-3 by changing the expression of Bcl-2/Bax, and induced Eca109 cell apoptosis, as is shown in Figure 3.
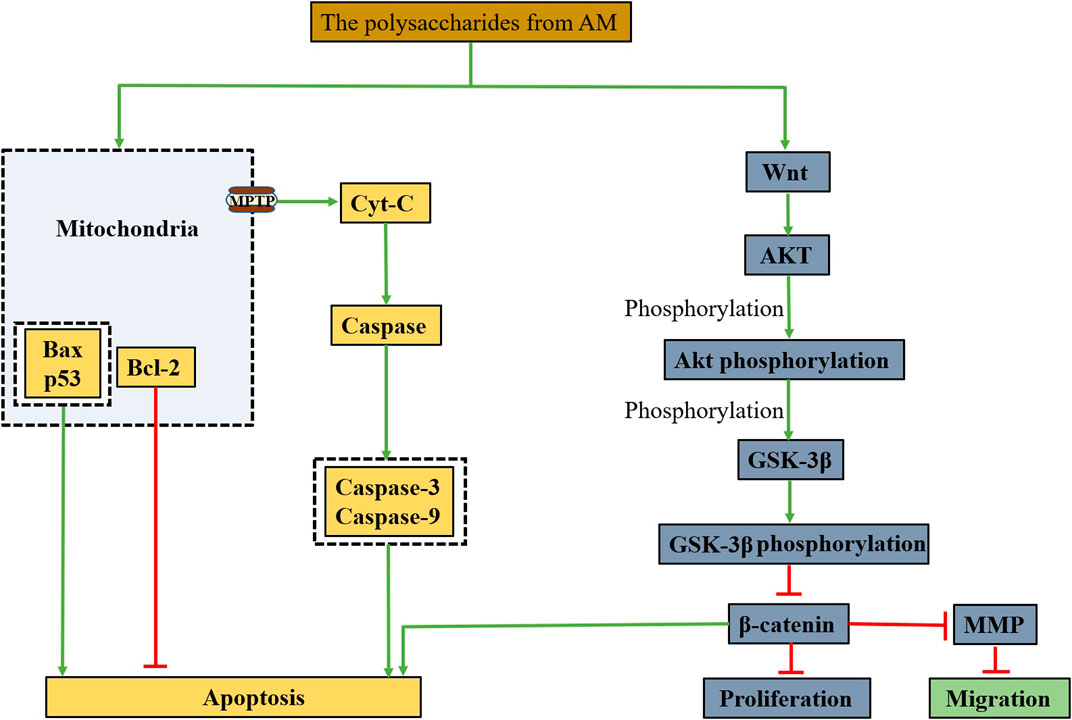
FIGURE 3. A possible mechanism by polysaccharides from AM to promote apoptosis, inhibit proliferation, migration through the mitochondrial apoptotic pathway and Wnt/β-catenin signaling pathway.
In addition, polysaccharides from AM can inhibit the migration, invasion and metastasis of tumour cells by regulating related signal pathways. The secretory glycoprotein (Wnt)/β-catenin signalling pathway is a classic pathway of the Wnt pathway, including the Wnt protein family, glycogen synthesis kinase 3β (GSK-3β), adenomatous polyposis coli (APC), Axin, β-catenin and other key proteins (Pai et al., 2017; Zhang et al., 2017). Among them, β-catenin regulates transcriptional activity in cancer cells is related to the proliferation and invasion of cancer cells (Russell and Monga, 2018). AKT/GSK-3β is the upstream regulator of β-catenin in the Wnt pathway (Jain et al., 2017). When the Wnt signalling pathway is activated, AKT is phosphorylated to form phosphorylated AKT with kinase activity, which can inactivate GSK-3β by phosphorylating GSK-3β to form phosphorylated GSK-3β, causing reduced degradation of intracellular β-catenin to promote apoptosis of cancer cells (Katoh and Katoh, 2017; Zhang et al., 2017). Furthermore, it has been shown that matrix metalloproteinase (MMP) is a downstream target of the Wnt/β-catenin signalling pathway and participates in the migration of tumour cells (Katoh and Katoh, 2017; Zhang et al., 2017), in which MMP-2 is the dominant molecule in the metastasis of hepatoma cells, its overexpression is associated with metastasis of hepatoma cells (Dou et al., 2016). Zhu et al. (Zhu et al., 2019) explored the mechanism of PAM on proliferation and invasion of hepatoma cells in vitro. They found that PAM may inhibit proliferation and invasion of hepatoma cells by regulating the Wnt/β-catenin signal pathway (Figure 3).
Studies have shown that the occurrence of cancer is related to the characteristics of cancer cells themselves and influenced by the internal environment in which they live (Klein-Goldberg et al., 2014). The immune cells are important components of the tumour microenvironment, which can enhance the body’s immune function and inhibit the immune escape of tumour cells (Merlo et al., 2016). Myeloid-derived suppressor cell (MDSC) is one of the most important immunosuppressive cells. It has been found that there are a lot of MDSCs in tumour tissue, which can inhibit the activation of T cells in many ways and promote the adaptive growth of tumour cells (Hawila et al., 2017). Feng et al. (Feng et al., 2019b) suggested that PAM could promote lymphocyte proliferation and stimulate immune cells as an immune enhancer. The mechanism of action was investigated, and it was found that PAM could enhance T cells production of cytokines IL-6, IFN-λ, TNF-α, and NO, increase the CD4+/CD8+ ratio, and reduce the level of MDSCs through TLR4/MyD88 signalling pathway.
The cell growth process is divided into four stages: G1, S, G2, and M (Ye et al., 2020). Anticancer drugs can inhibit the growth of cancer cells by inhibiting their cell cycle. Feng et al. (Feng Z. et al., 2019) obtained the crude polysaccharide of AM by water extraction and alcohol precipitation method and purified by ultrafiltration. Finally, a neutral heteropolysaccharide PAMK with a molecular weight of 4.1 kDa was obtained by HPGPC, FT-IR, GC, and NMR. PAMK arrested the cell cycle in the S phase, prevented the chromosome replication, inhibited their division, and thus inhibited the proliferation of cancer cells.
In conclusion, polysaccharides from AM have good antitumor activity, which is mainly mediated via inducing tumour cell apoptosis, inhibiting tumour cell migration, invasion and metastasis, regulating the tumour microenvironment and immune function, and inhibiting tumour cell proliferation. However, the structure of polysaccharides from AM are complex, and it is difficult to explain its structure-activity relationship. The mechanism involved in the antitumour activity of polysaccharides from AM should be further studied. In addition, the antitumour activity of polysaccharides from AM was mainly focused on in vitro experiments. Thus, more studies should be conducted to evaluate its antitumour activity and safety profile in the relevant in vivo models.
Gastroprotection and intestinal health promotion
AM has a long history of use as a herb with health-protective effects mainly for digestive system diseases such as food intake reduction, bloating and diarrhoea. It has the functions of invigorating the spleen, improving gastrointestinal function, repairing gastrointestinal mucosal damage and regulating intestinal microecology (Wang J. et al., 2019; Yang et al., 2020; Wen et al., 2021). Many papers report that plant polysaccharides (Wang W. et al., 2020; Sun et al., 2020; Yan et al., 2020; Cui et al., 2021) can effectively treat gastrointestinal diseases. The gastroprotective activity of polysaccharides from AM is mediated via the regulation of intestinal flora and related signalling pathways, the synthesis and release of gastrointestinal hormones and neurotransmitters, and oxidative stress response. The details are shown in Table 4.
Polysaccharides from AM can regulate the gene signalling pathway to increase tight junction (TJ) protein expression. Gastrointestinal mucosa is an epithelial barrier formed by intercellular connection and interaction to resist the invasion of foreign harmful substances. TJs, mainly composed of membrane proteins such as occludin, claudins and zona occludens 1 (ZO-1), are important components of the intestinal epithelial barrier and play an important role in maintaining intestinal permeability, tissue differentiation and homeostasis (Ren et al., 2019; Tan et al., 2019). Polyamines regulate cell junctions by influencing cell signalling. TJs are located at the top of the junction complex, and the adhesive junctions below are rich in cadherin. Cadherin is a Ca2+ dependent transmembrane protein. E-cadherin is located in the adherens junctions of the intestinal mucosa and binds to intracellular α, β, and γ connexins (catenin) for the formation and regulation of epithelial barriers (Shafraz et al., 2020). Kv pathway (Fellerhoff-Losch et al., 2016) is the decisive factor to increase Ca2+ level. Long non-coding RNA are non-protein coding transcripts (Noh et al., 2018), used for the regulation of transcriptional genes, and play an important role in describing intestinal barrier function (Batista and Chang, 2013; Quinn and Chang, 2016; Kok and Baker, 2019; Hanning et al., 2021). It protects intestinal barrier function by blocking phosphorylated signal transduction and nuclear translocation of transcriptional activator 2 (STAT2). CDKN1A gene inhibits jejunal epithelial cells apoptosis by regulating the activity of cyclin-dependent kinases (Kreis et al., 2019). AMKP can increase polyamines in cells, enhance the expression and activity of the Kv1.1 pathway, increase the level of Ca2+, stimulate accelerated cell migration, increase the expression of mucosal junction proteins, and accelerate the healing of intestinal injury (Luo et al., 2021). Short-chain fatty acids are intestinal microbial metabolites. Their abnormal changes are a significant feature of ulcerative colitis (UC) at the metabolic level (Feng et al., 2018; Paramsothy et al., 2019).
Polysaccharides from AM can also regulate the synthesis and release of gastrointestinal hormones and neurotransmitters and improve gastrointestinal function. The hormones or peptides produced by the gastrointestinal tract can be divided into three groups: gastrointestinal hormones, gastrointestinal neuropeptides and gastrointestinal growth factors (Hayati et al., 2021). Gastrin (GAS) and vasoactive intestinal peptide (VIP) are two brain-gut peptides. GAS can stimulate gastrointestinal movement; VIP mainly regulates gastrointestinal relaxation and gastrointestinal sphincter relaxation. Somatostatin (SS) is a peptide hormone that inhibits gastric acid secretion. The gastrointestinal growth factor can regulate the process of wound repair caused by intestinal inflammation. AMP can promote the expression of cytokines related to intestinal mucosal repair, such as pro-epidermal growth factor (EGF) and transforming growth factor-β1 (TGF-β1), to alleviate the injury of gastrointestinal mucosa (Shang, 2017).
Polysaccharides from AM can improve the antioxidant capacity of gastric mucosa, alleviate oxidative stress, regulate the expression of apoptosis-related genes, and reduce the damage of free radicals to the gastric mucosa. AMP alleviates exercise stress ulcers in mice by increasing Superoxide dismutase (SOD) activity, up-regulating Bcl-2 expression, down-regulating Bax expression and malondialdehyde (MDA) content (Cao and Bai, 2016).
PAM can further improve the intestinal microenvironment by regulating the growth of intestinal flora and play a protective role in the gastrointestinal tract. Studies have found that it can promote the proliferation of intestinal probiotics, inhibit the growth of harmful bacteria, and regulate the composition of intestinal flora (Wang et al., 2014).
In addition, YY11901, a polysaccharide isolated from AM by Wang (Wang, 2018), can alleviate the injury of the gastrointestinal mucosa, but the specific mechanism needs to be further studied. Thus, these results suggest that the representative compound AMP could be a promising structural template for the development of new gastrointestinal drug candidates.
Hepatoprotective effect
Liver injury (Qu et al., 2020) is an important pathological process in liver diseases, leading to fatty liver, liver ischemia-reperfusion injury (IRI), liver cirrhosis, fibrosis and even liver cancer. Studies have shown that plant polysaccharides (Zhang et al., 2021) have hepatoprotective activity and also have the advantages of wide distribution, low toxicity, and low price. It is considered to be an important natural resource for alleviating liver injury. AM has hepatoprotective activity, and its main chemical constituent polysaccharides from AM are effective in the treatment of IRI, non-alcoholic fatty liver disease (NAFLD) and liver injury. Its mechanism of action mainly includes regulating related anti-inflammatory signaling pathways and relieving oxidative stress. The related mechanism of polysaccharides from AM alleviating liver injury is shown in Figure 4.
Oxidative stress produces large amounts of free radicals, which damage the membranes of liver cells through lipid peroxidation (Li et al., 2021b). Enzymes and proteins such as serum alanine transaminase (ALT), aspartate transaminase (AST), total bilirubin (TBIL) and direct bilirubin (DBIL) escape from hepatocyte membranes after injury so that these components can be used as markers of liver injury. The levels of MDA and SOD reflect the extent of lipid peroxidation damage in hepatocytes (Li et al., 2020b). It has been found that the occurrence and development of fatty liver are often accompanied by an abnormal increase in the content of triglyceride (TG) and free fatty acids (FFA) in liver tissue (Song et al., 2021). Han et al. (Han et al., 2016) isolated a polysaccharide PRAM2 from AM and found it to protect the acute liver injury induced by carbon tetrachloride in mice via alleviating the oxidative stress response. PRAM2 reduced the ALT and AST activity, reduced the nitric oxide synthase, NO and MDA contents, increased the SOD and glutathione peroxidase (GSH-Px) activity.
IRI is often accompanied by oxidative stress and reactive oxygen intermediates. It has been reported that oxidative stress may activate NF-κB to regulate inflammatory factors (Kuboki et al., 2007; Tóbon-Velasco et al., 2014). Jin et al. (Jin et al., 2011) found that polysaccharides from AM inhibited the IRI induced lipid peroxidation, suppressed the elevation of ALT, AST, TBIL, and MDA, decreased the NF-κB expression, and promoted the SOD activity. In addition, Guo et al. (Guo et al., 2021) found that PAMK alleviates lipopolysaccharide-induced hepatitis in mice by regulating the TLR4-MyD88-NF-κB signalling pathway, decreasing the IL-1β, IL-6, and TNF-α levels, increasing the IL-4 level and inhibiting GSH-Px and MDA levels. Qian et al. (Qian et al., 2019) also found that PAMK alleviates CTX-induced liver injury in goslings, which may be related to the regulation of the TLR4 signalling pathway.
NAFLD is a liver disease closely related to insulin resistance, with high incidence in Europe and America. It may induce diseases such as liver cirrhosis and liver cancer in severe cases. Che et al. (Che et al., 2017) found that polysaccharides from AM could effectively prevent and treat NAFLD, alleviate steatosis and vacuolar degeneration of liver cells, and reduce the levels of TG, FFA, ALT, and AST. Studies have shown that polysaccharides from AM are also associated with fatty acid transporter and apolipoprotein B100 in NAFLD treatment (Xia et al., 2017).
Hence, polysaccharides from AM have certain hepatoprotective activity, and pharmacological studies have shown that it can effectively alleviate acute liver injury, IRI, hepatitis and NAFLD in mice. Further studies should be carried out to explore its activity in alleviating alcoholic fatty liver disease.
Hypoglycaemic activity
Diabetes mellitus (Jiang et al., 2020) is a metabolic disease caused by long-term hyperglycemia due to insufficient insulin secretion and insulin dysfunction. TCM has significant hypoglycaemic activity with few side effects. The mechanism of hypoglycaemic activity of polysaccharides may be related to the protection of islet cells, the promotion of insulin secretion and release, the improvement of insulin resistance, the regulation of glucose metabolism, the regulation of hypoglycaemic hormone and glucagon levels, and the promotion of glucose utilisation in peripheral tissues and organs (Dong et al., 2019; Zhong et al., 2021). The mechanisms are shown in Figure 5.
A study found that alloxan selectively destroys islet β cells, making insulin less available and causing blood sugar to rise (Drikvandi et al., 2020). Shan et al. (Shan and Tian, 2003) screened the purified polysaccharide AMP-A and AMP-B from AM for their hypoglycaemic activity. AMP-A had no effect, whereas AMP-B had the effect in alloxan-induced diabetic rats. AMP-B can effectively alleviate the atrophy of the thymus and islet in diabetic rats, which may be through inhibition of islet β cell injury induced by alloxan. Li et al. (Li et al., 2015) found that polysaccharides from AM can reduce fasting and postprandial blood glucose in db/db mice. Its mechanism may be related to improving the sensitivity of peripheral target organs to insulin, reducing plasma insulin levels, increasing insulin sensitivity index and improving insulin resistance.
In summary, the hypoglycaemic activity mechanism of polysaccharides from AM is the same as other plant polysaccharides. Its activity is mainly mediated via protecting islet cells, promoting insulin secretion or release, improving insulin resistance and promoting sugar utilisation by peripheral tissues and organs. In the future, we should continue to study the hypoglycemic mechanism of polysaccharides from AM, and focus on exploring its different action pathways and targets from other polysaccharides, so as to develop it into a specific drug for the treatment of diabetes.
Other activities
In addition to the above-mentioned pharmacological activities, polysaccharides from AM also have antibacterial, neuroprotective, antiaging (Ma et al., 2006), antioxidant and other activities. The antibacterial activity of polysaccharides from AM was mainly manifested in inhibiting the growth of Aeromonas (Fan and Liang, 2017). Polysaccharides from AM also have antioxidant activity. Pu et al. (Pu et al., 2015) used ultrasound-assisted enzymatic extraction to obtain RAMP with antioxidant activity. The antioxidant activity was evaluated using DPPH free radical scavenging method, and the scavenging rate was up to 62.18%. The polysaccharides obtained from AM purified by DEAE column were selenized by Hou et al. (Hou et al., 2019) to obtain sAMP6. It possesses the strongest in vitro and in vivo antioxidant activity mediated via increasing the GSH-Px and SOD activities and reducing the MDA in experimental chickens. In addition, polysaccharides from AM also have a neuroprotective effect (Hu et al., 2014). It can significantly inhibit hypoxia-induced neuronal growth inhibition, mitochondrial injury and apoptosis. The mechanism of polysaccharides from AM is related to the decrease of Bax and Caspase-3, the increase of Bcl-2/Bax ratio, and the inhibition of mitochondrial apoptosis. In addition, the adverse reactions and side effects of polysaccharides from AM have not been found so far. In summary, polysaccharides from AM have numerous pharmacological activities, which provide a strong reference for the clinical application of AM in Chinese medicine.
Structure-activity relationship
At present, the research on the structure-activity relationship of plant polysaccharides is a hotspot of polysaccharides. Plant polysaccharides have complex molecular structures, and differences in monosaccharide composition, molecular weight, spatial structure and chemical modification have important effects on the biological activity of polysaccharides (Lin and Huang, 2022).
The monosaccharide composition of polysaccharides from AM is related to its pharmacological activity. It has been reported that xylose could activate the proliferation of bifidobacteria and improve the intestinal microbial environment (Broekaert et al., 2011) (Wang et al., 2020) (Wang et al., 2014), found that PAM regulates the intestinal flora associated with xylose in its structure, which activates and accelerates the growth of intestinal flora. In addition, galacturonic acid is an important monosaccharide constituting PAMK, and related studies have found that galacturonic acid can activate complement, which may be an important reason for PAMK-mediated immune response (Huang et al., 2022). Studies have shown that spherical polysaccharide molecules containing Man and Gcl are more conducive to binding with receptors to trigger downstream signaling factors (Lombard et al., 1994). RAMP2 and PAM are spherical polysaccharides containing Man and Glc, and their immunomodulatory and anti-tumor effects may be related to the specific binding of monosaccharides Man and Glc to polysaccharide receptors on Mφ. It can be seen that the composition of polysaccharides determines its structural properties, and the structural properties determine its biological activity.
A specific spatial structure is a necessary condition for the biological activity of polysaccharides. The study found that the basis of the biological activity of polysaccharides is the single-stranded helical conformation of β-(1→3)-D-glucan (Saitô et al., 1991). UAM is a homogeneous polysaccharide composed of glucose, mainly connected by β-D-1→3 and β-D-1→3.6, with simple structure and small molecular weight. Its activity of inhibiting the growth of K562 cells may be related to its structure. Meanwhile, the side chain portion of the RG-I region in AMAP-1 and AMAP-2 was found to stimulate immune activity and significantly increase NO release in RAW264.7 cells (Broekaert et al., 2011; Cui et al., 2020). However, the current research on the spatial structure of polysaccharides from AM is still very superficial, and the regular structural pattern has not been summarized, and a large number of experiments are needed for supplementary verification.
The molecular weight is closely related to the activity of polysaccharides from AM. A study found that the immune activity of AMAP-1 and AMAP-2 was significantly higher than that of AMAP-3, which may be related to the higher molecular weight of the former and the lower molecular weight of AMAP-3 (Cui et al., 2020). Sijunzi Decoction consists of Panax ginseng, Atractylodes macrocephala, Poria cocos Wolf and Glycyrrhiza uralensis. A total of seven polysaccharides were isolated through experiments. Among them, BZ-three to one extracted from AM has the highest immune activity, which can significantly enhance the phagocytosis of RAW264.7 cells, which may be related to its highest molecular weight (Ji et al., 2017). It can be seen that polysaccharides with higher molecular weight may have stronger immune activity.
Studies have shown that the biological activity of polysaccharides from AM after chemical modification has been significantly improved. The structural modification of polysaccharides from AM mainly focuses on selenization, modification of functional groups and chelation. Selenium is an important trace element with many pharmacological activities such as anti-cancer, enhancing immunity and anti-inflammatory (Huang et al., 2020). Selenium polysaccharide is a compound in which selenium is bonded to active polysaccharide, which can play the dual functions of selenium and polysaccharide (Li J. et al., 2019; Zhou et al., 2020). It has been shown that selenium-modified polysaccharides from PAMK can enhance immune activity significantly promote the proliferation of lymphocytes and the secretion of related cytokines (Xu et al., 2014; Li W. et al., 2018). At the same time, Xu and Tian (Xu and Tian, 2015b) also found that the combination of PAMK and selenium could better improve the immune response of chickens with acute heat stress. And found that selenium can specifically regulate TNF-a and IFN-γ pathways, and PAMK can affect more cytokines. In addition, the modification and types of functional groups in polysaccharides have a significant impact on their pharmacological effects. The introduction of sulfuric acid groups changed both the primary and higher structures of polysaccharides from AM. Sulfation modification had better anticoagulation and B lymphocyte proliferative activities and showed a dose-response relationship. Jiang (Jiang, 2011) investigated the effects of unsulfated PAMK and sulfated PAMK on the proliferation of chicken peripheral blood lymphocytes through experiments, and found that sulfation could significantly improve the lymphocyte proliferation of PAMK in a dose-effect relationship. Wang (Wang, 2012) compared the antioxidant ability of hydroxymethylated AMP and the positive control acarbose on glycosidase, and found that hydroxymethylated AMP has a good inhibitory effect on glycosidase, and can be developed into a hypoglycemic drug in the future. In addition, polysaccharide-metal complexes are also an important modification method to enhance the activity of PAMK. Wu used the prepared PAMK-zinc complex as a feed additive to investigate its effect on the immune regulation function of immunosuppressed rats, and found that low-dose PAMK-zinc complexe could restore part of the immune function of rats, which may be related to the better absorption and utilization in rats. In conclusion, structural modification is an important way to improve the biological activity of polysaccharides from AM. However, due to the limitations of spatial structure testing technology, the research on the relationship between polysaccharides from AM structure and biological activity mechanism and the improvement of its structural activity are relatively rough, and the clinical trials are not sufficient, which limits its development and application.
In addition, there are related reports on the type modification of polysaccharides from AM preparations. Mesoporous silica nanoparticles (MSNs) are a kind of porous non-metallic material-based carriers, which have the advantages of high surface area, stability, targeting, and biocompatibility, and are widely used in drug control delivery. Based on PAMK’s fast metabolism and poor targeting, MSNs were prepared for loading PAMK. It was found that PAMK-MSNS can enhance the phagocytosis of macrophages and has good safety. Nanostructured lipid carriers have high drug loading capacity for lipophilic and hydrophilic drugs, good biocompatibility and high bioavailability. Liu et al. found that PAKM-NLC could promote the maturation and differentiation of bone marrow dendritic cells, and promote the secretion of IL-1β, IL-12, TNF-α, and IFN-γ, which is expected to be a good adjuvant for improving immune efficacy. It can be seen that the formulation modification of PAMK can also significantly improve its pharmacological activity. However, there still have certain limitations, and their stability is easily affected by environmental factors (such as temperature, pH value, etc.). At the same time, there are only a few studies on polysaccharides from AM as a drug carrier. Thus further studies should be carried out to explore the potential of developing polysaccharides from AM as a drug carrier.
It can be seen that there is a significant relationship between the structure of polysaccharides from AM and its pharmacological activity. However, the structure of polysaccharide is complex and different extraction processes may affect its structure and yield, resulting in structural instability. Therefore, in the future research, more emphasis should be placed on the optimization of the extraction process of polysaccharides from AM, and the connection between its primary structure and even its advanced structure and pharmacological activity should be deeply analyzed by using advanced structure identification methods. At the same time, different techniques should be used to modify the structure of polysaccharides from AM to improve its structural properties and enhance its pharmacological activity.
Applications
AM is a commonly used Chinese medicine in clinics and belongs to dietary supplements. It has good therapeutic effects on protecting gastrointestinal mucosa, balancing intestinal flora, relieving cardiovascular disease and regulating immune system function.
Polysaccharides from AM have the name of immune adjuvant, which is the basis of the medicinal effect of AM to exert immune regulation function. It has been proved that polysaccharides from AM have efficacy in regulating the body’s immunity and have a certain alleviating effect on the adverse reactions caused by the immune deficiency. Therefore, polysaccharides from AM can treat chemotherapy-induced adverse reactions such as weight loss, intestinal mucosa injury, and intestinal flora disturbance. It can protect the integrity of the intestinal barrier and maintain the stability of gastrointestinal function to alleviate the side effects caused by chemotherapy (Wang et al., 2018).
Due to their naturalness and versatility, natural plant supplements are safe and do not develop drug resistance. These are in line with the development trend of the modern food industry and are the hot topics of domestic and foreign animal husbandry research (Ali et al., 2021; Redhead et al., 2021). Polysaccharides from AM can significantly improve intestinal health and enhance the immune function of animals, promote the growth of animals, increase feed intake and reduce the feed-meat ratio. In addition, polysaccharides from AM also have good antibacterial, antiviral and antioxidant effects. These effects would improve the resistance capacity of animals to the diseases (Song et al., 2014). The development of polysaccharides from AM as an effective food supplement to animals is of great significance and is in line with the rational and sustainable development of antibiotic-free animal feed. However, it should be noted that the structure of polysaccharide has important implications for the generation of its pharmacological effects. The source and extraction method of AM may affect the structure of polysaccharides, since the composition of polysaccharides from different regions or extracted using different methods may not be the same and may affect the efficacy. At the same time, the dose of polysaccharides from AM used is not the same in all animals, and different developmental stages of animals may require different doses (Gao et al., 2019; Yang et al., 2019). Furthermore, only for extraction conditions, the laboratory studies are not sufficient to translate into its application in animal husbandry industry. Hence, The extraction procedures also should be optimised on an industrial scale.
Polysaccharides from AM can also be used as a vaccine adjuvant to enhance immunomodulatory effects. It has been found that the immunomodulation of polysaccharides from AM can be enhanced by structural modification or the preparation of nanoparticles. Liu et al. (Liu X. et al., 2018) combined PAMK-NLC with PAMK to prepare PAMK-NLC nanoparticles and found that PAMK-NLC could enhance the immune effect of PAMK, so it could be used as an adjuvant of OVA. In addition, oral or subcutaneous injection of AMP was found to enhance the immune response of mice to the foot-and-mouth disease (FMD) vaccine and can be used as an adjuvant of the FMD vaccine (Xie, 2012; Lu et al., 2016). Polysaccharides are chemically stable, readily available and easily modified, and are widely used in the development of targeted drug carriers. However, there are few studies on polysaccharides from AM as a drug carrier. Therefore, the potential of developing polysaccharides from AM as a drug carrier should be explored in depth.
Conclusion and prospect
This review summarizes the research progress in chemical compositions, pharmacological activities and applications of polysaccharides from AM. It is the main chemical component present in AM and possesses significant pharmacological activities. Polysaccharide from AM is a heteropolysaccharide, mainly composed of monosaccharides such as Gal, Rha, Ara, Man, GalA, and Xyl. In addition, it has immunomodulatory, antitumour, gastroprotective, hepatoprotective and hypoglycaemic activities, which has great potential in product development. At present, polysaccharides from AM are mainly used as an immune adjuvant, natural plant food supplement to animals, vaccine adjuvant, etc., and have great development prospects.
Generally, polysaccharides from AM have been extensively studied worldwide but the research is very basic and there are still many unsolved puzzles. Firstly, the influence of polysaccharides from AM structure on biological activity is in infancy as it is shown that polysaccharides from AM structures vary with the source and extraction method. Secondly, the activity of polysaccharides is closely related to monosaccharide composition, molecular weight and its distribution, glycosidic linkage, branching degree and conformation. However, all these components in AM have not yet been elucidated. Many studies reported various biological activities (antitumour, immunomodulatory, hepatoprotective, hypoglycaemic, gastroprotective, etc.) of polysaccharides from AM, but there are few studies on its molecular mechanisms. In addition, there are few reports on the development of products containing polysaccharide of AM. It is necessary to establish accurate and reliable methods to confirm the chemical properties of polysaccharides from AM. Plant polysaccharides have great potential for development as delivery systems, so it is necessary to explore the use of polysaccharides from AM as drug carriers to maximize its medicinal value. It is also necessary to perform more in vivo experiments to confirm its efficacy and establish safety. In conclusion, the research on the structure, quality control, and activity of polysaccharides from AM should be further strengthened to form a scientific basis for producing novel therapeutic agents, functional foods, and adjuvants to prevent or treat different pathological conditions.
Author contributions
Writing, CL, SW, PG, and ZC; Literature search, CL, ZX, TX, and MH; Figures, CL, QX, and HS.
Funding
This work was supported by the Key Technology Research and Development Program of Shandong, China (No.2020CXGC010505-06), Shandong University Youth Innovation Team (Talent Induction Program)—Research Team of New Materials and New Preparations for External Treatment.
Conflict of interest
The authors declare that the research was conducted in the absence of any commercial or financial relationships that could be construed as a potential conflict of interest.
Publisher’s note
All claims expressed in this article are solely those of the authors and do not necessarily represent those of their affiliated organizations, or those of the publisher, the editors and the reviewers. Any product that may be evaluated in this article, or claim that may be made by its manufacturer, is not guaranteed or endorsed by the publisher.
References
Ali, A., Ponnampalam, E. N., Pushpakumara, G., Cottrell, J. J., Suleria, H., and Dunshea, F. R. (2021). Cinnamon: A natural feed additive for poultry health and production-A review. Animals. 11, 2026. doi:10.3390/ani11072026
Bates, M., Spillane, C. D., Gallagher, M. F., McCann, A., Martin, C., Blackshields, G., et al. (2020). The role of the MAD2-TLR4-MyD88 axis in paclitaxel resistance in ovarian cancer. PloS one 15, e0243715. doi:10.1371/journal.pone.0243715
Batista, P. J., and Chang, H. Y. (2013). Long noncoding RNAs: Cellular address codes in development and disease. Cell 152, 1298–1307. doi:10.1016/j.cell.2013.02.012
Bordbar, S., Anwar, F., and Saari, N. (2011). High-value components and bioactives from sea cucumbers for functional foods--a review. Mar. Drugs 9, 1761–1805. doi:10.3390/md9101761
Boucher, J. C., Li, G., Kotani, H., Cabral, M. L., Morrissey, D., Lee, S. B., et al. (2021). CD28 costimulatory domain-targeted mutations enhance chimeric antigen receptor T-cell function. Cancer Immunol. Res. 9, 62–74. doi:10.1158/2326-6066.CIR-20-0253
Broekaert, W. F., Courtin, C. M., Verbeke, K., Van de Wiele, T., Verstraete, W., and Delcour, J. A. (2011). Prebiotic and other health-related effects of cereal-derived arabinoxylans, arabinoxylan-oligosaccharides, and xylooligosaccharides. Crit. Rev. Food Sci. Nutr. 51, 178–194. doi:10.1080/10408390903044768
Butcher, M. J., and Zhu, J. (2021). Recent advances in understanding the Th1/Th2 effector choice. Fac. Rev. 10, 30. doi:10.12703/r/10-30
Cao, Y. X., and Bai, G. B. (2016). Experimental study on atractylodes macrocephala koidz polysaccharides for exercise stress ulcer rats with antioxidant and bcl-2 and Bax expression in gastric mucosa. J. Northwest Univ. Nat. Sci. Ed. 46, 553–557. doi:10.16152/j.cnki.xdxbzr.2016-04-017
Carneiro, B. A., and El-Deiry, W. S. (2020). Targeting apoptosis in cancer therapy. Nat. Rev. Clin. Oncol. 17, 395–417. doi:10.1038/s41571-020-0341-y
Che, C. Y., Li, H. S., Ying, H., and Zhou, F. (2017). Research of prevention and treatment function of atractylodes polysaccharides on non-alcoholic steatohepatitis. Chin. Archives Traditional Chin. Med. 35, 1801–1803+1924. doi:10.13193/j.issn.1673-7717.2017.07.045
Chen, L., and Huang, G. (2019). Antioxidant activities of sulfated pumpkin polysaccharides. Int. J. Biol. Macromol. 126, 743–746. doi:10.1016/j.ijbiomac.2018.12.261
Chen, L., and Huang, G. (2018). Antitumor activity of polysaccharides: An overview. Curr. Drug Targets 19, 89–96. doi:10.2174/1389450118666170704143018
Chen, L. L., Zou, T. D., He, Q., Guo, X. B., Lu, Y. F., Luo, B. W., et al. (2020). Effects of polysaccharide compounds from atractylodes macrocephalaon and poriaccocos on intestinal morphological structure of weaned piglets. Chin. J. Animal Sci. 56, 133–138. doi:10.19556/j.0258-7033.20200212-02
Chi, Y., Li, W., Wen, H., Cui, X., Cai, H., and Bi, X. (2001). [Studies on separation, purification and chemical structure of polysaccharide from Atractylodes macrocephala]. Zhong yao cai = Zhongyaocai = J. Chin. Med. Mater. 24, 647–648. doi:10.13863/j.issn1001-4454.2001.09.013
Chinese Pharmacopoeia Commission (2020). Pharmacopoeia of the people’s Republic of China (beijing. China: China Medical Science and Technology Press, 107–108.
Cui, L., Guan, X., Ding, W., Luo, Y., Wang, W., Bu, W., et al. (2021). Scutellaria baicalensis Georgi polysaccharide ameliorates DSS-induced ulcerative colitis by improving intestinal barrier function and modulating gut microbiota. Int. J. Biol. Macromol. 166, 1035–1045. doi:10.1016/j.ijbiomac.2020.10.259
Cui, Y. S., Li, Y. X., Jiang, S. L., Song, A. N., Fu, Z., Dong, C. X., et al. (2020). Isolation, purification, and structural characterization of polysaccharides from Atractylodis Macrocephalae Rhizoma and their immunostimulatory activity in RAW264.7 cells. Int. J. Biol. Macromol. 163, 270–278. doi:10.1016/j.ijbiomac.2020.06.269
Deng, J., Zhong, Y. F., Wu, Y. P., Luo, Z., Sun, Y. M., Wang, G. E., et al. (2018). Carnosine attenuates cyclophosphamide-induced bone marrow suppression by reducing oxidative DNA damage. Redox Biol. 14, 1–6. doi:10.1016/j.redox.2017.08.003
Dong, C. (2021). Cytokine regulation and function in T cells. Annu. Rev. Immunol. 39, 51–76. doi:10.1146/annurev-immunol-061020-053702
Dong, W. N., Li, K. Z., Zhang, W. T., and Wu, H. (2019). Progress in hypoglycemic effect and mechanism of polysaccharides. Chin. J. Exp. Traditional Med. Formulae 25, 219–225. doi:10.13422/j.cnki.syfjx.20191807
Dou, C. Y., Cao, C. J., Wang, Z., Zhang, R. H., Huang, L. L., Lian, J. Y., et al. (2016). EFEMP1 inhibits migration of hepatocellular carcinoma by regulating MMP2 and MMP9 via ERK1/2 activity. Oncol. Rep. 35, 3489–3495. doi:10.3892/or.2016.4733
Drikvandi, P., Bahramikia, S., and Alirezaei, M. (2020). Modulation of the antioxidant defense system in liver, kidney, and pancreas tissues of alloxan-induced diabetic rats by camphor. J. Food Biochem. 44, e13527. doi:10.1111/jfbc.13527
Editorial Committee of Flora of China (2015). Flora of China (beijing. Beijing: Science Press, 40–41.
Fan, M. H., and Liang, R. S. (2017). Effect of polysaccharide from atractylodes macrocephala koidz on bacteriostasis of Aeromonas. Abstr. Chin. animal Husb. veterinary Med. 33, 59+154.
Fan, W., Zhang, S., Hao, P., Zheng, P., Liu, J., and Zhao, X. (2016). Structure characterization of three polysaccharides and a comparative study of their immunomodulatory activities on chicken macrophage. Carbohydr. Polym. 153, 631–640. doi:10.1016/j.carbpol.2016.07.116
Fellerhoff-Losch, B., Korol, S. V., Ganor, Y., Gu, S., Cooper, I., Eilam, R., et al. (2016)., 123. Vienna, Austria, 137–157. doi:10.1007/s00702-015-1446-9Normal human CD4(+) helper T cells express Kv1.1 voltage-gated K(+) channels, and selective Kv1.1 block in T cells induces by itself robust TNFα production and secretion and activation of the NFκB non-canonical pathwayJ. Neural Transm.
Feng, W., Ao, H., and Peng, C. (2018). Gut microbiota, short-chain fatty acids, and herbal medicines. Front. Pharmacol. 9, 1354. doi:10.3389/fphar.2018.01354
Feng, W., Liu, J., Tan, Y., Ao, H., Wang, J., and Peng, C. (2020). Polysaccharides from Atractylodes macrocephala Koidz. Ameliorate ulcerative colitis via extensive modification of gut microbiota and host metabolism. Food Res. Int. 138, 109777. doi:10.1016/j.foodres.2020.109777
Feng, Y. Y., Ji, H. Y., Dong, X. D., and Liu, A. J. (2019a). An alcohol-soluble polysaccharide from Atractylodes macrocephala Koidz induces apoptosis of Eca-109 cells. Carbohydr. Polym. 226, 115136. doi:10.1016/j.carbpol.2019.115136
Feng, Y. Y., Ji, H. Y., Dong, X. D., Yu, J., and Liu, A. J. (2019b). Polysaccharide extracted from Atractylodes macrocephala Koidz (PAMK) induce apoptosis in transplanted H22 cells in mice. Int. J. Biol. Macromol. 137, 604–611. doi:10.1016/j.ijbiomac.2019.06.059
Feng, Z. F., and Yang, R. B. (2021). Effects of Atractylodes Macrocephala polysaccharide on tumor growth and immunoregulation in colon cancer CT26 tumor-bearing mice via TLR4 signaling pathway. Chin. Tradit. Pat. Med., 1–5.
Feng, Z., Yang, R., Wu, L., Tang, S., Wei, B., Guo, L., et al. (2019c). Atractylodes macrocephala polysaccharides regulate the innate immunity of colorectal cancer cells by modulating the TLR4 signaling pathway. Onco. Targets. Ther. 12, 7111–7121. doi:10.2147/OTT.S219623
Gao, Q., Yang, T., Duan, P. N., and Zhao, Y. R. (2019). Physiological function of polysaccharides from dendrobium and its potential application in animal production. Chin. J. Animal Nutr. 31, 4473–4480.
Gisbert-Garzarán, M., Lozano, D., and Vallet-Regí, M. (2020). Mesoporous silica nanoparticles for targeting subcellular organelles. Int. J. Mol. Sci. 21, 9696. doi:10.3390/ijms21249696
Grosser, J. A., Maes, M. E., and Nickells, R. W. (2021). Characteristics of intracellular propagation of mitochondrial BAX recruitment during apoptosis. Apoptosis. 26, 132–145. doi:10.1007/s10495-020-01654-w
Gu, S., Li, L., Huang, H., Wang, B., and Zhang, T. (2019). Antitumor, antiviral, and anti-inflammatory efficacy of essential oils from Atractylodes macrocephala koidz. Prod. Differ. Process. Methods. Mol. (Basel, Switz. 24, 2956. doi:10.3390/molecules24162956
Gu, Y. C., Ren, L. J., and Zhang, L. (1992). Studies on polysaccharide of atractylodes macrocephala koidz I. Separation, purification and properties of AM-1 and AM-2. Chin. Traditional Herb. Drugs 235, 507–508+558.
Guo, L., Sun, Y. L., Wang, A. H., Xu, C. E., and Zhang, M. Y. (2012). Effect of polysaccharides extract of rhizoma atractylodis macrocephalae on thymus, spleen and cardiac indexes, caspase-3 activity ratio, Smac/DIABLO and HtrA2/Omi protein and mRNA expression levels in aged rats. Mol. Biol. Rep. 39, 9285–9290. doi:10.1007/s11033-012-1677-x
Guo, S., Li, W., Chen, F., Yang, S., Huang, Y., Tian, Y., et al. (2021). Polysaccharide of Atractylodes macrocephala Koidz regulates LPS-mediated mouse hepatitis through the TLR4-MyD88-NFκB signaling pathway. Int. Immunopharmacol. 98, 107692. doi:10.1016/j.intimp.2021.107692
Guzik, T. J., Korbut, R., and Adamek-Guzik, T. (2003). Nitric oxide and superoxide in inflammation and immune regulation. J. Physiol. Pharmacol. 54, 469–487. PMID: 14726604.
Häcker, G. (2018). Apoptosis in infection. Microbes Infect. 20, 552–559. doi:10.1016/j.micinf.2017.10.006
Han, B., Gao, Y., Wang, Y., Wang, L., Shang, Z., Wang, S., et al. (2016). Protective effect of a polysaccharide from Rhizoma Atractylodis Macrocephalae on acute liver injury in mice. Int. J. Biol. Macromol. 87, 85–91. doi:10.1016/j.ijbiomac.2016.01.086
Hanning, N., Edwinson, A. L., Ceuleers, H., Peters, S. A., De Man, J. G., Hassett, L. C., et al. (2021). Intestinal barrier dysfunction in irritable bowel syndrome: A systematic review. Ther. Adv. Gastroenterol. 14, 1756284821993586. doi:10.1177/1756284821993586
Hawila, E., Razon, H., Wildbaum, G., Blattner, C., Sapir, Y., Shaked, Y., et al. (2017). CCR5 directs the mobilization of CD11b+Gr1+Ly6Clow polymorphonuclear myeloid cells from the bone marrow to the blood to support tumor development. Cell Rep. 21, 2212–2222. doi:10.1016/j.celrep.2017.10.104
Hayati, F., Abdul Rahim, M. A., and Abdul Rahim, S. (2021). Comment to: Diagnostic value of serum gastrin and epidermal growth factor to the gastric ulcer complicated with upper gastrointestinal hemorrhage. J. Coll. Physicians Surg. Pak. 31, 1522–1523. doi:10.29271/jcpsp.2021.12.1522
Hou, L., Meng, M., Chen, Y., and Wang, C. (2017). A water-soluble polysaccharide from Grifola frondose induced macrophages activation via TLR4-MyD88-IKKβ-NF-κB p65 pathways. Oncotarget 8, 86604–86614. doi:10.18632/oncotarget.21252
Hou, R. R., Li, Q., Liu, J., and Hu, Y. L. (2019). Selenylation modification of Atractylodes macrocephala polysaccharide and evaluation of antioxidant activity. Adv. Polym. Technol. 2019, 1–8. doi:10.1155/2019/8191385
Hu, W. X., Xiang, Q., Wen, Z., He, D., Wu, X. M., and Hu, G. Z. (2014). Neuroprotective effect of Atractylodes macrocephalaon polysaccharides in vitro on neuronal apoptosis induced by hypoxia. Mol. Med. Rep. 9, 2573–2581. doi:10.3892/mmr.2014.2105
Huang, H., Yang, X., Xia, W., and Zhang, W. Q. (2022). Structural analysis and immunomodulatory effects of pectic polysaccharides separated from jasminum sambac flower waste. Sci. Technol. Food Industry, 1–15. doi:10.1016/j.ijbiomac.2020.06.150
Huang, S., Yang, W., and Huang, G. (2020). Preparation and activities of selenium polysaccharide from plant such as Grifola frondosa. Carbohydr. Polym. 242, 116409. doi:10.1016/j.carbpol.2020.116409
Jain, S., Ghanghas, P., Rana, C., and Sanyal, S. N. (2017). Role of GSK-3β in regulation of canonical wnt/β-catenin signaling and PI3-K/akt oncogenic pathway in colon cancer. Cancer Invest. 35, 473–483. doi:10.1080/07357907.2017.1337783
Jearawuttanakul, K., Khumkhrong, P., Suksen, K., Reabroi, S., Munyoo, B., Tuchinda, P., et al. (2020). Cleistanthin A induces apoptosis and suppresses motility of colorectal cancer cells. Eur. J. Pharmacol. 889, 173604. doi:10.1016/j.ejphar.2020.173604
Ji, G. Q., Chen, R. Q., and Zheng, J. X. (2015a). Effect of polysaccharide from atractylodes macrocephala koidz on phenotypic and functional maturation of dendritic cells. Food Sci. 36, 207–211. doi:10.1089/jmf.2011.1575
Ji, G. Q., Chen, R. Q., and Zheng, J. X. (2015b). Macrophage activation by polysaccharides from Atractylodes macrocephala Koidz through the nuclear factor-κB pathway. Pharm. Biol. 53, 512–517. doi:10.3109/13880209.2014.929152
Ji, Y., Wang, R., Peng, Y., Peng, C., and Li, X. (2017). Purification, preliminary characterization, and immunological activity of polysaccharides from crude drugs of sijunzi formula. eCAM 2017, 2170258. Evidence-based complementary and alternative medicine. doi:10.1155/2017/2170258
Jiang, M. H., Zhu, L., and Jiang, J. G. (2010). Immunoregulatory actions of polysaccharides from Chinese herbal medicine. Expert Opin. Ther. Targets 14, 1367–1402. doi:10.1517/14728222.2010.531010
Jiang, T., Wang, L., Ma, A., Wu, Y., Wu, Q., Wu, Q., et al. (2020). The hypoglycemic and renal protective effects of Grifola frondosa polysaccharides in early diabetic nephropathy. J. Food Biochem. 44, e13515. doi:10.1111/jfbc.13515
Jiang, Y. (2011). The antiviral and immune-enhancing activities of atractylodes macroce-phalapolysaccharides and their sulfated derivant. Nanjing: Nanjing Agricultural University.
Jin, C., Zhang, P. J., Bao, C. Q., Gu, Y. L., Xu, B. H., Li, C. W., et al. (2011). Protective effects of Atractylodes macrocephala polysaccharide on liver ischemia-reperfusion injury and its possible mechanism in rats. Am. J. Chin. Med. 39, 489–502. doi:10.1142/S0192415X11008981
Kai, L. X. (2021). Preparation and structural characterization of Atractylodes macrocephala polysaccharide and its regulatory effect on DSS induced intestinal mucosal barrier injury. [Hangzhou (IL)]: Zhejiang University.
Kara, E. E., Comerford, I., Fenix, K. A., Bastow, C. R., Gregor, C. E., McKenzie, D. R., et al. (2014). Tailored immune responses: Novel effector helper T cell subsets in protective immunity. PLoS Pathog. 10, e1003905. doi:10.1371/journal.ppat.1003905
Katoh, M., and Katoh, M. (2017). Molecular genetics and targeted therapy of WNT-related human diseases (Review). Int. J. Mol. Med. 40, 587–606. doi:10.3892/ijmm.2017.3071
Kim, J. H., Doh, E. J., and Lee, G. (2016). Evaluation of medicinal categorization of atractylodes japonica koidz. By using internal transcribed spacer sequencing analysis and HPLC fingerprinting combined with statistical tools. Evid. Based. Complement. Altern. Med. 2016, 2926819. doi:10.1155/2016/2926819
Klein-Goldberg, A., Maman, S., and Witz, I. P. (2014). The role played by the microenvironment in site-specific metastasis. Cancer Lett. 352, 54–58. doi:10.1016/j.canlet.2013.08.029
Kok, F. O., and Baker, A. H. (2019). The function of long non-coding RNAs in vascular biology and disease. Vasc. Pharmacol. 114, 23–30. doi:10.1016/j.vph.2018.06.004
Kou, N., Cho, H., Kim, H. E., Sun, Q., Ahn, K. H., Ji, H., et al. (2017). Anti-cancer effect of Atractylodes macrocephala extract by double induction of apoptotic and autophagic cell death in head and neck cancer cells. Bangladesh J. Pharmacol. 12, 140–146. doi:10.3329/bjp.v12i2.31238
Kreis, N. N., Louwen, F., and Yuan, J. (2019). The multifaceted p21 (Cip1/Waf1/CDKN1A) in cell differentiation, migration and cancer therapy. Cancers 11, 1220. doi:10.3390/cancers11091220
Kuboki, S., Okaya, T., Schuster, R., Blanchard, J., Denenberg, A., Wong, H. R., et al. (2007). Hepatocyte NF-kappaB activation is hepatoprotective during ischemia-reperfusion injury and is augmented by ischemic hypothermia. Am. J. Physiol. Gastrointest. Liver Physiol. 292, G201–G207. doi:10.1152/ajpgi.00186.2006
Kumar, D., Tewari-Singh, N., Agarwal, C., Jain, A. K., Inturi, S., Kant, R., et al. (2015). Nitrogen mustard exposure of murine skin induces DNA damage, oxidative stress and activation of MAPK/Akt-AP1 pathway leading to induction of inflammatory and proteolytic mediators. Toxicol. Lett. 235, 161–171. doi:10.1016/j.toxlet.2015.04.006
Li, B. X., Li, W. Y., Tian, Y. B., Guo, S. X., Huang, Y. M., Xu, D. N., et al. (2019a). Polysaccharide of Atractylodes macrocephala koidz enhances cytokine secretion by stimulating the TLR4-MyD88-NF-κb signaling pathway in the mouse spleen. J. Med. Food 22, 937–943. doi:10.1089/jmf.2018.4393
Li, J., Shen, B., Nie, S., Duan, Z., and Chen, K. (2019b). A combination of selenium and polysaccharides: Promising therapeutic potential. Carbohydr. Polym. 206, 163–173. doi:10.1016/j.carbpol.2018.10.088
Li, R., Li, W., Zhang, Y. Y., Wang, L., and Liu, J. T. (2018a). Effect of Rhizoma Atractylodis macrocephalae and Rhizoma Atractylodis macrocephalae on gastrointestinal function in rats with spleen deficiency. Lishizhen Med. Materia Medica Res. 29, 2626–2628. doi:10.1016/j.jep.2020.113415
Li, S., Zhu, Z., Xue, M., Pan, X., Tong, G., Yi, X., et al. (2021d). The protective effects of fibroblast growth factor 10 against hepatic ischemia-reperfusion injury in mice. Redox Biol. 40, 101859. doi:10.1016/j.redox.2021.101859
Li, W., Guo, S., Xu, D., Li, B., Cao, N., Tian, Y., et al. (2018b). Polysaccharide of Atractylodes macrocephala koidz (PAMK) relieves immunosuppression in cyclophosphamide-treated geese by maintaining a humoral and cellular immune balance. Mol. (Basel, Switz. 23, 932. doi:10.3390/molecules23040932
Li, W., Hu, X., Wang, S., Jiao, Z., Sun, T., Liu, T., et al. (2020a). Characterization and anti-tumor bioactivity of astragalus polysaccharides by immunomodulation. Int. J. Biol. Macromol. 145, 985–997. doi:10.1016/j.ijbiomac.2019.09.189
Li, W., Li, W., Leng, Y., Xiong, Y., and Xia, Z. (2020b). Ferroptosis is involved in diabetes myocardial ischemia/reperfusion injury through endoplasmic reticulum stress. DNA Cell Biol. 39, 210–225. doi:10.1089/dna.2019.5097
Li, W., Xiang, X., Cao, N., Chen, W., Tian, Y., Zhang, X., et al. (2021a). Polysaccharide of atractylodes macrocephala koidz activated T lymphocytes to alleviate cyclophosphamide-induced immunosuppression of geese through novel_mir2/CD28/AP-1 signal pathway. Poult. Sci. 100, 101129. doi:10.1016/j.psj.2021.101129
Li, W., Xiang, X., Li, B., Wang, Y., Qian, L., Tian, Y., et al. (2021b). PAMK relieves LPS-induced enteritis and improves intestinal flora disorder in goslings. Evid. Based. Complement. Altern. Med. 2021, 9721353. doi:10.1155/2021/9721353
Li, X., Liu, F., Li, Z., Ye, N., Huang, C., and Yuan, X. (2014). Atractylodes macrocephala polysaccharides induces mitochondrial-mediated apoptosis in glioma C6 cells. Int. J. Biol. Macromol. 66, 108–112. doi:10.1016/j.ijbiomac.2014.02.019
Li, Y., Chen, S. H., Ji, X., Geng, Z. G., and Lv, G. Y. (2015). Effects of polysaccharide from atractylodes macrocephala on blood glucose and related indicators in spontaneous type 2 diabetes db/db mice. Chin. J. Exp. Traditional Med. Formulae 21, 162–165. doi:10.13422/j.cnki.syfjx.2015100162
Li, Y. H., Liang, Y. Q., Li, C. Y., Li, C. H., Xia, H. Y., and Li, Z. K. (2021c). The effect of plantain aqueous extract on immune function in immunosuppressive mice induced by cyclophosphamide. Chin. J. Ethnomedicine Ethnopharmacy 30, 21–25.
Li, Z., Wang, T., and Yu, Y. (2022). miR-424 inhibits apoptosis and inflammatory responses induced by sevoflurane through TLR4/MyD88/NF-κB pathway. BMC Anesthesiol. 22, 52. doi:10.1186/s12871-022-01590-z
Lin, B., and Huang, G. (2022). Extraction, isolation, purification, derivatization, bioactivity, structure-activity relationship, and application of polysaccharides from White jellyfungus. Biotechnol. Bioeng. 119, 1359–1379. doi:10.1002/bit.28064
Lin, Z., Liu, Y. F., Qu, Y., Shi, L. Y., Dou, D. Q., and Kuang, H. X. (2015). Characterisation of oligosaccharides from Baizhu by HILIC-MS. Nat. Prod. Res. 29, 1194–1200. doi:10.1080/14786419.2014.995652
Liu, J., Chang, H. W., Huang, Z. M., Nakamura, M., Sekhon, S., Ahn, R., et al. (2021b). Single-cell RNA sequencing of psoriatic skin identifies pathogenic Tc17 cell subsets and reveals distinctions between CD8+ T cells in autoimmunity and cancer. J. allergy Clin. Immunol. 147, 2370–2380. doi:10.1016/j.jaci.2020.11.028
Liu, J. Y., Hou, X. X., Li, Z. Y., Shan, S. H., Chang, M. C., Feng, C. P., et al. (2020). Isolation and structural characterization of a novel polysaccharide from Hericium erinaceus fruiting bodies and its arrest of cell cycle at S-phage in colon cancer cells. Int. J. Biol. Macromol. 157, 288–295. doi:10.1016/j.ijbiomac.2020.04.162
Liu, W., Deng, L. H., and Zhao, Y. Q. (2021a). Summary of pharmacological effects of atractylodes macrocephala and its active ingredients. Acta Chin. Med. Pharmacol. 46, 116–119. doi:10.19664/j.cnki.1002-2392.210250
Liu, X., Zhang, Z., Pan, S., Shang, S., and Li, C. (2018a). Interaction between the Wnt/β-catenin signaling pathway and the EMMPRIN/MMP-2, 9 route in periodontitis. J. Periodontal Res. 53, 842–852. doi:10.1111/jre.12574
Liu, Y., and Huang, G. (2019). The antioxidant activities of carboxymethylated cushaw polysaccharide. Int. J. Biol. Macromol. 121, 666–670. doi:10.1016/j.ijbiomac.2018.10.108
Liu, Z., Sun, Y., Zhang, J., Ou, N., Gu, P., Hu, Y., et al. (2018b). Immunopotentiation of Polysaccharides of Atractylodes macrocephala Koidz-loaded nanostructured lipid carriers as an adjuvant. Int. J. Biol. Macromol. 120, 768–774. doi:10.1016/j.ijbiomac.2018.08.108
Lombard, Y., Giaimis, J., Makaya-Kumba, M., Fonteneau, P., and Poindron, P. (1994). A new method for studying the binding and ingestion of zymosan particles by macrophages. J. Immunol. Methods 174, 155–165. doi:10.1016/0022-1759(94)90018-3
Lu, Y. S., Xu, W., Zhang, C. R., Xu, Y., Guan, R., and Hu, S. H. (2016). Enhancing effect of oil emulsion containing Atractylodes Macrocephala polysaccharide on foot-and-mouth disease vaccine. Chin. J. Veterinary Sci. 36, 928–932. doi:10.4142/jvs.2021.22.e30
Luo, M., Wu, T. T., Li, R. L., Zhang, D., Zhu, H. B., Hu, L., et al. (2021). Effect of atractylodes macrocephala polysaccharides on IEC-6 cell barrier and expression of adhesion junction proteins. Traditional Chin. Drug Res. Clin. Pharmacol. 32, 533–539. doi:10.19378/j.issn.1003-9783.2021.04.013
Ma, Q. H., Zhang, P. X., Guo, H. Y., Wei, X. D., and Ou, Q. (2006). Antioxidation effect of Atractylodes Macrocephala polysaccharide on d-galactose induced aging rat neurons. Chin. J. Gerontology 12, 1658–1660.
Merlo, A., Dalla Santa, S., Dolcetti, R., Zanovello, P., and Rosato, A. (2016). Reverse immunoediting: When immunity is edited by antigen. Immunol. Lett. 175, 16–20. doi:10.1016/j.imlet.2016.04.015
Noh, J. H., Kim, K. M., McClusky, W. G., Abdelmohsen, K., and Gorospe, M. (2018). Cytoplasmic functions of long noncoding RNAs. Wiley Interdiscip. Rev. RNA 9, e1471. doi:10.1002/wrna.1471
Obeng, E. (2021). Apoptosis (programmed cell death) and its signals - a review. Braz. J. Biol. = Revista brasleira de Biol. 81, 1133–1143. doi:10.1590/1519-6984.228437
Okubo, S., Uto, T., Goto, A., Tanaka, H., Nishioku, T., Yamada, K., et al. (2017). Berberine induces apoptotic cell death via activation of caspase-3 and -8 in HL-60 human leukemia cells: Nuclear localization and structure-activity relationships. Am. J. Chin. Med. 45, 1497–1511. doi:10.1142/S0192415X17500811
Orzalli, M. H., and Kagan, J. C. (2017). Apoptosis and necroptosis as host defense strategies to prevent viral infection. Trends Cell Biol. 27, 800–809. doi:10.1016/j.tcb.2017.05.007
Pai, S. G., Carneiro, B. A., Mota, J. M., Costa, R., Leite, C. A., Barroso-Sousa, R., et al. (2017). Wnt/beta-catenin pathway: Modulating anticancer immune response. J. Hematol. Oncol. 10, 101. doi:10.1186/s13045-017-0471-6
Paramsothy, S., Nielsen, S., Kamm, M. A., Deshpande, N. P., Faith, J. J., Clemente, J. C., et al. (2019). Specific bacteria and metabolites associated with response to fecal microbiota transplantation in patients with ulcerative colitis. Gastroenterology 156, 1440–1454.e2. e2. doi:10.1053/j.gastro.2018.12.001
Pu, J. B., Xia, B. H., Hu, Y. J., Zhang, H. J., Chen, J., Zhou, J., et al. (2015). Multi-optimization of ultrasonic-assisted enzymatic extraction of atratylodes macrocephala polysaccharides and antioxidants using response surface methodology and desirability function approach. Mol. (Basel, Switz. 20, 22220–22235. doi:10.3390/molecules201219837
Qian, L., Liu, Y., Li, B. X., Fu, J., Wan, W. Y., Cao, N., et al. (2019). Polysaccharide of atractylodes macrocephala koidz may alleviates liver injury induced by cyclophosphamide via toll-like receptor 4 signaling pathway in goslings. Chin. J. Animal Nutr. 31, 764–774.
Qu, J., Huang, P., Zhang, L., Qiu, Y., Qi, H., Leng, A., et al. (2020). Hepatoprotective effect of plant polysaccharides from natural resources: A review of the mechanisms and structure-activity relationship. Int. J. Biol. Macromol. 161, 24–34. doi:10.1016/j.ijbiomac.2020.05.196
Quinn, J. J., and Chang, H. Y. (2016). Unique features of long non-coding RNA biogenesis and function. Nat. Rev. Genet. 17, 47–62. doi:10.1038/nrg.2015.10
Redhead, A., Noor Azman, F., Nasaruddin, A. I., Vu, T., Santos, F., Malheiros, R., et al. (2021). Peanut skins as a natural antimicrobial feed additive to reduce the transmission of Salmonella in poultry meat produced for human consumption. J. Food Prot. doi:10.4315/JFP-21-205
Ren, M. T., Gu, M. L., Zhou, X. X., Yu, M. S., Pan, H. H., Ji, F., et al. (2019). Sirtuin 1 alleviates endoplasmic reticulum stress-mediated apoptosis of intestinal epithelial cells in ulcerative colitis. World J. Gastroenterol. 25, 5800–5813. doi:10.3748/wjg.v25.i38.5800
Royer, P. J., Henrio, K., Pain, M., Loy, J., Roux, A., Tissot, A., et al. (2017). TLR3 promotes MMP-9 production in primary human airway epithelial cells through Wnt/β-catenin signaling. Respir. Res. 18, 208. doi:10.1186/s12931-017-0690-y
Russell, J. O., and Monga, S. P. (2018). Wnt/β-Catenin signaling in liver development, homeostasis, and pathobiology. Annu. Rev. Pathol. 13, 351–378. doi:10.1146/annurev-pathol-020117-044010
Saitô, H., Yoshioka, Y., Uehara, N., Aketagawa, J., Tanaka, S., and Shibata, Y. (1991). Relationship between conformation and biological response for (1----3)-beta-D-glucans in the activation of coagulation factor G from limulus amebocyte lysate and host-mediated antitumor activity. Demonstration of single-helix conformation as a stimulant. Carbohydr. Res. 217, 181–190. doi:10.1016/0008-6215(91)84128-2
Schmetterer, K. G., and Pickl, W. F. (2017). The IL-10/STAT3 axis: Contributions to immune tolerance by thymus and peripherally derived regulatory T-cells. Eur. J. Immunol. 47, 1256–1265. doi:10.1002/eji.201646710
Shafraz, O., Xie, B., Yamada, S., and Sivasankar, S. (2020). Mapping transmembrane binding partners for E-cadherin ectodomains. Proc. Natl. Acad. Sci. U. S. A. 117, 31157–31165. doi:10.1073/pnas.2010209117
Shan, J. J., and Tian, G. Y. (2003). [Studies on physico-chemical properties and hypoglycemic activity of complex polysaccharide AMP-B from Atractylodes macrocephala Koidz]. Yao xue xue bao = Acta Pharm. Sin. 38, 438–441.
Shang, Q. C. (2017). Study on the effect of Polysaccharide from Atractylodes macrocephala on intestinal mucosal repair in Escherichia coli diarrhea model mice. [Yangzhou (IL)]: Yangzhou University.
Song, H. P., Li, R. L., Chen, X., Wang, Y. Y., Cai, J. Z., Liu, J., et al. (2014). Atractylodes macrocephala Koidz promotes intestinal epithelial restitution via the polyamine--voltage-gated K+ channel pathway. J. Ethnopharmacol. 152, 163–172. doi:10.1016/j.jep.2013.12.049
Song, M., Meng, L., Liu, X., and Yang, Y. (2021). Feprazone prevents free fatty acid (FFA)-Induced endothelial inflammation by mitigating the activation of the TLR4/MyD88/NF-κB pathway. ACS Omega 6, 4850–4856. doi:10.1021/acsomega.0c05826
Suabjakyong, P., Nishimura, K., Toida, T., and Van Griensven, L. J. (2015). Structural characterization and immunomodulatory effects of polysaccharides from Phellinus linteus and Phellinus igniarius on the IL-6/IL-10 cytokine balance of the mouse macrophage cell lines (RAW 264.7). Food Funct. 6, 2834–2844. doi:10.1039/c5fo00491h
Sun, C., Yang, J., Pan, L., Guo, N., Li, B., Yao, J., et al. (2018). Improvement of icaritin on hematopoietic function in cyclophosphamide-induced myelosuppression mice. Immunopharmacol. Immunotoxicol. 40, 25–34. doi:10.1080/08923973.2017.1392564
Sun, Q., Cheng, L., Zeng, X., Zhang, X., Wu, Z., and Weng, P. (2020). The modulatory effect of plant polysaccharides on gut flora and the implication for neurodegenerative diseases from the perspective of the microbiota-gut-brain axis. Int. J. Biol. Macromol. 164, 1484–1492. doi:10.1016/j.ijbiomac.2020.07.208
Sun, W., Meng, K., Qi, C., Yang, X., Wang, Y., Fan, W., et al. (2015). Immune-enhancing activity of polysaccharides isolated from Atractylodis macrocephalae Koidz. Carbohydr. Polym. 126, 91–96. doi:10.1016/j.carbpol.2015.03.034
Tan, M. (2010). Studies on separation, purification and composition of the effective polysaccharides in Atractylodes macrocephala Koidz. Changsha: Hunan Normal University.
Tan, Y., Guan, Y., Sun, Y., and Zheng, C. (2019). Correlation of intestinal mucosal healing and tight junction protein expression in ulcerative colitis patients. Am. J. Med. Sci. 357, 195–204. doi:10.1016/j.amjms.2018.11.011
Tian, S., Hao, C., Xu, G., Yang, J., and Sun, R. (2017). Optimization conditions for extracting polysaccharide from Angelica sinensis and its antioxidant activities. J. Food Drug Anal. 25, 766–775. doi:10.1016/j.jfda.2016.08.012
Tóbon-Velasco, J. C., Cuevas, E., and Torres-Ramos, M. A. (2014). Receptor for AGEs (RAGE) as mediator of NF-kB pathway activation in neuroinflammation and oxidative stress. CNS Neurol. Disord. Drug Targets 13, 1615–1626. doi:10.2174/1871527313666140806144831
Van Opdenbosch, N., and Lamkanfi, M. (2019). Caspases in cell death, inflammation, and disease. Immunity 50, 1352–1364. doi:10.1016/j.immuni.2019.05.020
Vega-Naredo, I., Oliveira, G., Cunha-Oliveira, T., Serafim, T., Sardão, V. A., and Oliveira, P. J. (2021). Analysis of proapoptotic protein trafficking to and from mitochondria. Methods Mol. Biol. 2310, 161–178. doi:10.1007/978-1-0716-1433-4_10
Wang, H., Chen, Y. B., and Zhang, Z. H. (2015a). Effect of Atractylodes Macrocephala polysaccharide on apoptosis of gastric cancer cell SGC-7901. Chin. J. Ethnomedicine Ethnopharmacy 24, 10–11. doi:10.3748/wjg.v13.i48.6575
Wang, J., Feng, W., Zhang, S., Chen, L., Tang, F., Sheng, Y., et al. (2019a). Gut microbial modulation in the treatment of chemotherapy-induced diarrhea with Shenzhu Capsule. BMC Complement. Altern. Med. 19, 126. doi:10.1186/s12906-019-2548-y
Wang, J., Liu, X. P., Liu, M. J., Li, J. G., and Bo, R. N. (2022). Preparation of Polysaccharide mesoporous silica from Atractylodes macrocephala and its effect on phagocytosis of mouse macrophages. Animal Husb. Veterinary Med. 54, 107–112. doi:10.2147/OTT.S219623
Wang, J., Zhang, S. Y., Sheng, Y. C., and Ao, H. (2018). Research progress on pharmacological action of Baizhu in treatment of gastrointestinal diseases. J. Chin. Med. 36, 2854–2858. doi:10.19540/j.cnki.cjcmm.20170121.005
Wang, P., Xie, Z. S., Song, J. Y., Zeng, H. H., Dai, L. P., E, H. C., et al. (2020a). Four new sesquiterpene lactones from Atractylodes macrocephala and their CREB agonistic activities. Fitoterapia 147, 104730. doi:10.1016/j.fitote.2020.104730
Wang, R., Zhou, G., Wang, M., Peng, Y., and Li, X. (2014). The metabolism of polysaccharide from atractylodes macrocephala koidz and its effect on intestinal microflora. Evidence-based complementary Altern. Med. 2014, 926381. eCAM. doi:10.1155/2014/926381
Wang, S. J., Ba, J., Zhang, Y. J., Deng, H., Pu, W. J., and Yang, H. (2019b). Analysis of molecular weight and monosaccharide composition of atractylodes macrocephala polysaccharides. Chin. J. Veterinary Drug 53, 26–32.
Wang, S. L., Wang, K. L., and Chen, D. F. (2012). Progress on immunological regulation effects and mechanisms of edible fungus polysaccharides. Prog. Veterinary Med. 33, 104–108. doi:10.16437/j.cnki.1007-5038.2012.11.013
Wang, W., Xu, A. L., Li, Z. C., Li, Y., Xu, S. F., Sang, H. C., et al. (2020b). Combination of probiotics and Salvia miltiorrhiza polysaccharide alleviates hepatic steatosis via gut microbiota modulation and insulin resistance improvement in high fat-induced NAFLD mice. Diabetes Metab. J. 44, 336–348. doi:10.4093/dmj.2019.0042
Wang, Y. L. (2012). Ultrasound on the structure and biological activity of polysaceharide islated from Atractylodes macrocephala Koidz (Xian: Shanxi Normal University). [master’s thesis].
Wang, Y. Y., Liu, J. W., Si Ma, Z. H., Song, H. P., Li, R. L., Cai, J. Z., et al. (2015b). Isolation, structural characterization and IEC-6 cell migration activities of polysaccharides from atractylodes macrocephala koidz. Chem. J. Chin. Univ. 36, 299–305.
Wang, Y. Y. (2018). Protective Effects of Atractylodes macrocephala polysaccharide on hydrogen peroxide-induced injury of IEC-6 cells. Inn. Mong. J. Traditional Chin. Med. 37, 98–99. doi:10.18632/oncotarget.19709
Wei, X., Zhang, J., Gu, Q., Huang, M., Zhang, W., Guo, J., et al. (2017). Reciprocal expression of IL-35 and IL-10 defines two distinct effector treg subsets that are required for maintenance of immune tolerance. Cell Rep. 21, 1853–1869. doi:10.1016/j.celrep.2017.10.090
Wen, W., Cheng, Z. Q., and Hou, K. (2021). Research Progress of action mechanism of Baizhu powder based on intestinal microecology. Microbiol. China 7, 1–11. doi:10.4103/wjtcm.wjtcm_51_21
Wu, L. Q., Jiang, S. F., and Zhang, J. (2012). Chromatographic analysis and atomic force microscope observation of WAM-1 structure of polysaccharide extracted from atractylodes macrocephala koidz. Nat. Prod. Res. Dev. 24, 631–634+609.
Wu, L. Q., Zhang, J., Sun, R. G., Jiang, S. F., Wang, Y. L., and Dai, J. J. (2011). Isolation and structure characterization of polysaccharide from atractylodes macrocephala koidz. Chem. J. Chin. Univ. 32, 2812–2816.
Wu, S. F. (2019). Study on chelation extraction of atractylodes rhizoma polysaccharide and its immunomodulatory activity ([Guangzhou (IL)]: Zhongkai University of Agriculture and Engineering). [master’s thesis].
Xia, J. P., Ying, H., Hu, A. R., Hu, Y. R., and Li, H. S. (2017). Changes of fat transport links in non-alcoholic fatty liver disease and the atractylodes polysaccharides intervention. Chin. J. Health Laboratory Technol. 27, 461–463+476.
Xiang, X., Cao, N., Chen, F., Qian, L., Wang, Y., Huang, Y., et al. (2020). Polysaccharide of Atractylodes macrocephala koidz (PAMK) alleviates cyclophosphamide-induced immunosuppression in mice by upregulating CD28/IP3R/plcγ-1/AP-1/NFAT signal pathway. Front. Pharmacol. 11, 529657. doi:10.3389/fphar.2020.529657
Xie, F. (2012). Study on the immune adjuvant effect of different extracts of Atractylodes Macrocephala Koidz ([Hangzhou (IL)]: Zhejiang University). [master’s thesis].
Xie, H. B., Zou, Y., Liu, L. L., Zhao, Y. S., and He, J. H. (2019). Effects of plant polysaccharides on intestinal mucosal morphology and barrier function in weaned piglets. Chin. J. Veterinary Sci. 39, 150–157+187. doi:10.1016/j.psj.2020.10.021
Xu, D., Li, W., Huang, Y., He, J., and Tian, Y. (2014). The effect of selenium and polysaccharide of Atractylodes macrocephala Koidz. (PAMK) on immune response in chicken spleen under heat stress. Biol. Trace Elem. Res. 160, 232–237. doi:10.1007/s12011-014-0056-y
Xu, D., and Tian, Y. (2015a). Selenium and polysaccharides of atractylodes macrocephala koidz play different roles in improving the immune response induced by heat stress in chickens. Biol. Trace Elem. Res. 168, 235–241. doi:10.1007/s12011-015-0351-2
Xu, H. L., Dai, J. H., Hu, T., and Liao, Y. F. (2018). Lentinan up-regulates microRNA-340 to promote apoptosis and autophagy of human osteosarcoma cells. Int. J. Clin. Exp. Pathol. 11, 3876–3883. PMID: 31949775.
Xu, W., Fang, S., Wang, Y., Chi, X., Ma, X., Zhang, T., et al. (2020a). Receptor and signaling pathway involved in bovine lymphocyte activation by Atractylodis macrocephalae polysaccharides. Carbohydr. Polym. 234, 115906. doi:10.1016/j.carbpol.2020.115906
Xu, W., Fang, S., Wang, Y., Zhang, T., and Hu, S. (2020b). Molecular mechanisms associated with macrophage activation by Rhizoma Atractylodis Macrocephalae polysaccharides. Int. J. Biol. Macromol. 147, 616–628. doi:10.1016/j.ijbiomac.2020.01.081
Xu, W., Guan, R., Shi, F., Du, A., and Hu, S. (2017). Structural analysis and immunomodulatory effect of polysaccharide from Atractylodis macrocephalae Koidz. on bovine lymphocytes. Carbohydr. Polym. 174, 1213–1223. doi:10.1016/j.carbpol.2017.07.041
Xu, D., and Tian, Y. (2015b). Selenium and polysaccharides of atractylodes macrocephala koidz play different roles in improving the immune response induced by heat stress in chickens. Biol. trace Elem. Res. 168, 235–241. doi:10.1007/s12011-015-0351-2
Xue, W., Gao, Y., Li, Q., Lu, Q., Bian, Z., Tang, L., et al. (2020). Immunomodulatory activity-guided isolation and characterization of a novel polysaccharide from Atractylodis macrocephalae Koidz. Int. J. Biol. Macromol. 161, 514–524. doi:10.1016/j.ijbiomac.2020.06.003
Yan, H., Sun, Y., Ma, Y., Ji, B., Hou, X., Yu, Z., et al. (2015). Determination of atractylon in rat plasma by a GC-MS method and its application to a pharmacokinetic study. J. Pharm. Anal. 5, 327–331. doi:10.1016/j.jpha.2015.03.002
Yan, T., Nian, T., Liao, Z., Xiao, F., Wu, B., Bi, K., et al. (2020). Antidepressant effects of a polysaccharide from okra (Abelmoschus esculentus (L) Moench) by anti-inflammation and rebalancing the gut microbiota. Int. J. Biol. Macromol. 144, 427–440. doi:10.1016/j.ijbiomac.2019.12.138
Yang, J., Shao, X., Jiang, J., Sun, Y., Wang, L., and Sun, L. (2018b). Angelica sinensis polysaccharide inhibits proliferation, migration, and invasion by downregulating microRNA-675 in human neuroblastoma cell line SH-SY5Y. Cell Biol. Int. 42, 867–876. doi:10.1002/cbin.10954
Yang, J. T., Cheng, X., Chen, H. W., Wu, J. W., and Zeng, Y. M. (2018a). Advances in the mechanism of polysaccharide immunization in atractylodes macrocephala koidz. Chin. J. Veterinary Drug 52, 80–85.
Yang, L., Hu, R. Z., Xia, S. T., and He, J. H. (2019). Advances in functional studies of Plant polysaccharides and their application in animal production. Chin. J. Animal Nutr. 31, 2534–2543.
Yang, L., Yu, H., Hou, A., Man, W., Wang, S., Zhang, J., et al. (2021). A review of the Ethnopharmacology, phytochemistry, pharmacology, application, quality control, processing, toxicology, and pharmacokinetics of the dried rhizome of Atractylodes macrocephala. Front. Pharmacol. 12, 727154. doi:10.3389/fphar.2021.727154
Yang, S., Zhang, J., Yan, Y., Yang, M., Li, C., Li, J., et al. (2020). Network pharmacology-based strategy to investigate the pharmacologic mechanisms of Atractylodes macrocephala koidz. For the treatment of chronic gastritis. Front. Pharmacol. 10, 1629. doi:10.3389/fphar.2019.01629
Yang, X., Lv, Y., Tian, L., and Zhao, Y. (2010). Composition and systemic immune activity of the polysaccharides from an herbal tea (Lycopus lucidus Turcz). J. Agric. Food Chem. 58, 6075–6080. doi:10.1021/jf101061y
Yang, Y. (2021). Isolation and chemical composition and protective effect on acute alcoholic liver injury of Atractylodes macrocephala polysaccharide ([Nanjing (IL)]: Nanjing University Of Chinese Medicine). [master’s thesis].
Ye, L., Zhang, X., Xu, Q., Cai, Y., Gao, W., and Chen, W. (2020). Anti-tumor activities and mechanism study of α-pinene derivative in vivo and in vitro. Cancer Chemother. Pharmacol. 85, 367–377. doi:10.1007/s00280-019-03997-x
Yesillik, S., and Gupta, S. (2020). Phenotypically defined subpopulations of circulating follicular helper T cells in common variable immunodeficiency. Immun. Inflamm. Dis. 8, 441–446. doi:10.1002/iid3.326
Yin, M., Zhang, Y., and Li, H. (2019). Advances in research on immunoregulation of macrophages by plant polysaccharides. Front. Immunol. 10, 145. doi:10.3389/fimmu.2019.00145
Yin, R., and Wang, J. G. (2019). Regulation and mechanism of Atractylodes Macrocephala L. polysaccharide on immune function in lung cancer model rats. Chin. Med. Biotechnol. 14, 527–533.
Yu, J., Ji, H. Y., and Liu, A. J. (2018). Alcohol-soluble polysaccharide from Astragalus membranaceus: Preparation, characteristics and antitumor activity. Int. J. Biol. Macromol. 118, 2057–2064. doi:10.1016/j.ijbiomac.2018.07.073
Yu, S., Yu, J., Dong, X., Li, S., and Liu, A. (2020). Structural characteristics and anti-tumor/-oxidant activity in vitro of an acidic polysaccharide from Gynostemma pentaphyllum. Int. J. Biol. Macromol. 161, 721–728. doi:10.1016/j.ijbiomac.2020.05.274
Zeng, D., Hu, C., Li, R. L., Lin, C. Q., Cai, J. Z., Wu, T. T., et al. (2018). Polysaccharide extracts of Astragalus membranaceus and Atractylodes macrocephala promote intestinal epithelial cell migration by activating the polyamine-mediated K+ channel. Chin. J. Nat. Med. 16, 674–682. doi:10.1016/S1875-5364(18)30107-9
Zhang, X. X., Li, J. Q., Li, M. S., Chen, Y., He, H. B., Liu, S. P., et al. (2021). Isolation, structure identification and hepatoprotective activity of a polysaccharide from Sabia parviflora. Bioorg. Med. Chem. Lett. 32, 127719. doi:10.1016/j.bmcl.2020.127719
Zhang, Z., Wang, X., Zhang, L., Shi, Y., Wang, J., and Yan, H. (2017). Wnt/β-catenin signaling pathway in trophoblasts and abnormal activation in preeclampsia (Review). Mol. Med. Rep. 16, 1007–1013. doi:10.3892/mmr.2017.6718
Zhao, X., Sun, W., Zhang, S., Meng, G., Qi, C., Fan, W., et al. (2016). The immune adjuvant response of polysaccharides from Atractylodis macrocephalae Koidz in chickens vaccinated against Newcastle disease (ND). Carbohydr. Polym. 141, 190–196. doi:10.1016/j.carbpol.2016.01.013
Zhao, X., Tian, J., Liu, Y., Ye, Z., Xu, M., Huang, R., et al. (2022). TLR4-Myd88 pathway upregulated caveolin-1 expression contributes to coronary artery spasm. Vasc. Pharmacol. 142, 106947. doi:10.1016/j.vph.2021.106947
Zhong, Q. W., Zhou, T. S., Qiu, W. H., Wang, Y. K., Xu, Q. L., Ke, S. Z., et al. (2021). Characterization and hypoglycemic effects of sulfated polysaccharides derived from Brown seaweed Undaria pinnatifida. Food Chem. 341, 128148. doi:10.1016/j.foodchem.2020.128148
Zhou, N., Long, H., Wang, C., Yu, L., Zhao, M., and Liu, X. (2020). Research progress on the biological activities of selenium polysaccharides. Food Funct. 11, 4834–4852. doi:10.1039/c9fo02026h
Zhu, B., Zhang, Q. L., Hua, J. W., Cheng, W. L., and Qin, L. P. (2018). The traditional uses, phytochemistry, and pharmacology of atractylodes macrocephala koidz.: A review. J. Ethnopharmacol. 226, 143–167. doi:10.1016/j.jep.2018.08.023
Zhu, Y., Li, C., Lin, X., Sun, J., and Cheng, Y. (2019). [Effect of Atractylodes macrocephala polysaccharide on proliferation and invasion of hepatocellular carcinoma cells in vitro]. Nan fang yi ke da xue xue bao = J. South. Med. Univ. 39, 1180–1185. doi:10.12122/j.issn.1673-4254.2019.10.08
Zhuang, W., Qu, M., Zhao, D., and Chang, Y. N. (2020). Studies on the structure and immunocompetence of Auricularia Auricula polysaccharides. Food Technol. 45, 205–210. doi:10.13684/j.cnki.spkj.2020.02.034
Keywords: Atractylodes macrocephala Koidz., polysaccharide, chemical composition, biological activity, application
Citation: Liu C, Wang S, Xiang Z, Xu T, He M, Xue Q, Song H, Gao P and Cong Z (2022) The chemistry and efficacy benefits of polysaccharides from Atractylodes macrocephala Koidz. Front. Pharmacol. 13:952061. doi: 10.3389/fphar.2022.952061
Received: 25 May 2022; Accepted: 21 July 2022;
Published: 25 August 2022.
Edited by:
Zhanyong Wang, Shenyang Agricultural University, ChinaReviewed by:
Utoomporn Surayot, Chiang Mai University, ThailandXia Li, Guilin University of Technology, China
Md Tanwir Athar, Buraydah Colleges, Saudi Arabia
Copyright © 2022 Liu, Wang, Xiang, Xu, He, Xue, Song, Gao and Cong. This is an open-access article distributed under the terms of the Creative Commons Attribution License (CC BY). The use, distribution or reproduction in other forums is permitted, provided the original author(s) and the copyright owner(s) are credited and that the original publication in this journal is cited, in accordance with accepted academic practice. No use, distribution or reproduction is permitted which does not comply with these terms.
*Correspondence: Peng Gao, Z2FvcGVuZ0BzZHV0Y20uZWR1LmNu; Zhufeng Cong, Y29uZ3podWZlbmdAMTYzLmNvbQ==
†These authors have contributed equally to this work