- 1Department of Anesthesiology, Union Hospital, Tongji Medical College, Huazhong University of Science and Technology, Wuhan, China
- 2Institute of Anesthesia and Critical Care Medicine, Union Hospital, Tongji Medical College, Huazhong University of Science and Technology, Wuhan, China
- 3State Key Laboratory of Pharmaceutical Biotechnology, The University of Hong Kong, Hong Kong, China
- 4Department of Anesthesiology, Affiliated Hospital of Guangdong Medical University, Zhanjiang, China
Mechanisms contributing to the pathogenesis of myocardial ischemia-reperfusion (I/R) injury are complex and multifactorial. Many strategies have been developed to ameliorate myocardial I/R injuries based on these mechanisms. However, the cardioprotective effects of these strategies appear to diminish in diabetic states. Diabetes weakens myocardial responses to therapies by disrupting intracellular signaling pathways which may be responsible for enhancing cellular resistance to damage. Intriguingly, it was found that Dexmedetomidine (DEX), a potent and selective α2-adrenergic agonist, appears to have the property to reverse diabetes-related inhibition of most intervention-mediated myocardial protection and exert a protective effect. Several mechanisms were revealed to be involved in DEX’s protection in diabetic rodent myocardial I/R models, including PI3K/Akt and associated GSK-3β pathway stimulation, endoplasmic reticulum stress (ERS) alleviation, and apoptosis inhibition. In addition, DEX could attenuate diabetic myocardial I/R injury by up-regulating autophagy, reducing ROS production, and inhibiting the inflammatory response through HMGB1 pathways. The regulation of autonomic nervous function also appeared to be involved in the protective mechanisms of DEX. In the present review, the evidence and underlying mechanisms of DEX in ameliorating myocardial I/R injury in diabetes are summarized, and the potential of DEX for the treatment/prevention of myocardial I/R injury in diabetic patients is discussed.
1 Introduction
The prevalence of diabetes has rapidly reached an epidemic level globally, shaping the disease into one of the 21st-century healthcare challenges and posing an incredibly high economic burden to the whole world (Zimmet et al., 2014; Schmidt, 2018; Berezin and Berezin, 2019). Coronary occlusions are more likely to occur and the heart is more sensitive to ischemia-reperfusion (I/R) injury in diabetic patients than in non-diabetic patients (Sasso et al., 2011; Henning, 2018). The incidence of cardiovascular disease in adults with diabetes is two to three times higher than those without diabetes (Sarwar et al., 2010; Henning, 2018; Strain and Paldánius, 2018). One possible reason is that the diabetic patients share several pathological features including inflammation and oxidative stress (Fisher, 1999; Alegria et al., 2007; Marso et al., 2007; Miki et al., 2012; Lejay et al., 2016; Russo et al., 2017; Zhao et al., 2017). Additionally, cardioprotective interventions such as ischemic preconditioning and postconditioning that are effective in nondiabetic subjects, are largely ineffective in diabetes (Keating, 2015; Russell et al., 2019). Therefore, it is extremely important to identify new pharmacological targets for the prevention and treatment of diabetic myocardial I/R injury.
DEX is a potent α-2 adrenergic receptor agonist that has sedative, analgesic, anxiolytic and opioid-sparing properties (Ebert et al., 2000; Venn and Grounds, 2001; Panzer et al., 2009; Keating, 2015). For its low risk of respiration inhibition and unique property of organ protection, the application of DEX is gaining popularity (Belleville et al., 1992; Venn et al., 2000). Plenty of evidence proved that the administration of DEX could protect the intestine (Sun et al., 2015; Zhang et al., 2020a), heart (Zhang et al., 2020b; Wu et al., 2020), kidney (Li et al., 2018), lung (Liang et al., 2019), and liver (Sahin et al., 2013; Lim et al., 2021) against I/R injury through “pharmacological preconditioning” or “pharmacological postconditioning” (Cai et al., 2014). Although most interventions failed to confer protection against I/R injury in diabetic states, it is interesting to note that DEX could ameliorate diabetic I/R injuries of various organs in animal models (Arslan et al., 2012; Kip et al., 2015; Yeda et al., 2017; Liu C. Y. et al., 2018; Castillo et al., 2019; Chen et al., 2019; Hou et al., 2020). Thus, it is of great importance to elucidate the biological function and the associated molecular mechanism of DEX under diabetic conditions, which will be useful for new pharmaceutical target finding and drug development against I/R injury in diabetes. Here, in this paper, the mechanisms of myocardial I/R injury and current strategies are reviewed and the therapeutic value of DEX and its molecular mechanisms in the treatment of diabetic myocardial I/R injury are elaborated.
2 Basic mechanisms of myocardial I/R injury and current strategies of protective interventions
The restoration of blood supply to the ischemic myocardium after myocardial I/R paradoxically leads to more intense cellular damage, with complex, diverse and highly integrated pathogenesis (Frank et al., 2012). During ischemia, hydrogen ions accumulate in large quantities intracellularly (Frank et al., 2012). When myocardial blood supply is restored, intracellular pH rapidly returns to its physiological state, disturbing the ions exchange of sodium and calcium, and causing an increase in mitochondrial calcium ions (Yellon and Hausenloy, 2007; Frank et al., 2012). The calcium ions overload activates calpain pathway and contributes to cell death (Potz et al., 2016; Valikeserlis et al., 2021). The mitochondrial permeability transition pore (mPTP) would open in response to mitochondrial calcium ions overload, oxidative stress, and the restoration of a physiological pH (Zhang M. L. et al., 2021; Valikeserlis et al., 2021). MPTP is a crucial determinant of cellular damage that occurs after ischemic myocardial reperfusion (Heusch et al., 2010). The irreversible opening of this channel leads to mitochondrial collapse, further aggravating ATP depletion and cellular damage (Szabó and Zoratti, 1991; Kim et al., 2003). Myocardial ischemia deteriorates with the ensuing inflammatory response after reperfusion (Frank et al., 2012). However, timely and effective myocardial reperfusion still represents the most effective clinical treatment for myocardial ischemia. To reduce the risk of reperfusion, various strategies have emerged, including ischemic preconditioning (Arriel et al., 2020), ischemic postconditioning (Heusch, 2015), remote ischemic preconditioning, remote ischemic postconditioning (Donato et al., 2017), and therapeutic hypothermia (Sobczyk et al., 2020). These cardioprotective strategies were proven to be effective for the prevention and treatment of myocardial I/R injury in non-diabetic subjects (Mokhtari-Zaer et al., 2018; Li et al., 2022). In general, myocardial I/R injury has been extensively studied, but in patients with comorbidities, especially with diabetes mellitus, it needs to be further investigated.
3 Myocardial I/R injury in diabetes
Unfortunately, most strategies for the protection against myocardial I/R injury appear to be ineffective in diabetic patients (Gao et al., 2016; Lejay et al., 2016). The non-responsiveness seems to be related to the alterations in several signaling pathways (Wang et al., 2013; Gao et al., 2021). Reperfusion injury signaling kinase (RISK) pathway including PI3K/Akt signaling cascade, and survivor activating factor enhancement (SAFE) pathway including JAK2/STAT3 signaling cascade are key for myocardial protection. Defects in these pathways reduce the sensitivity of diabetic myocardium to treatments. Hyperglycemia induces the expression of phosphatase and tensin homolog deleted on chromosome ten (PTEN) and blocks PI3K/Akt pathway (Mocanu and Yellon, 2007), leading to a failure of GSK-3β phosphorylation, which promotes mPTP opening and exacerbates myocardial I/R injury (Lejay et al., 2016). Mitochondrial dysfunction is an essential cause of irreversible myocardial damage. STAT3, an effector in the SAFE pathway, is down-regulated in diabetes, leading to impairment of mitochondrial function (Wang et al., 2013). Persistent hyperglycemia of diabetes can also disrupt mitochondria by increasing dynamin-related protein 1 (DRP1) expression (Ding et al., 2017), blocking the mitochondrial KATP channel (Li et al., 2013), and inactivating hypoxia-inducible factor-1 (HIF-1α) (Riquelme et al., 2016). The above factors are highly integrated and contribute to the desensitization of diabetic myocardium to therapeutic interventions against I/R injury.
4 Dexmedetomidine and its potential protection against myocardial I/R injury
4.1 Dexmedetomidine and its potential preconditioning
4.1.1 Dexmedetomidine preconditioning induced cardioprotection against non-diabetic I/R injury
Rational use of DEX for preconditioning is effective in reducing the damage caused by myocardial I/R. It was verified in numerous animal studies that cardioprotection of DEX preconditioning could be achieved through the administration of DEX (10 nM) intravenously 30 minutes before ischemia (Okada et al., 2007; He et al., 2019). In rats model of myocardial I/R injury, DEX preconditioning could reduce myocardial infarct size, decrease the incidence of arrhythmia and improve left ventricular function (Dong et al., 2017; He et al., 2019; Xiong et al., 2021). Specific mechanisms of its cardioprotective effects were explored. It was revealed that DEX might protect the heart through its direct effects on myocardial signaling cascades, such as activation of the RISK, in particular, the PI3K/Akt signaling pathway (Zhang et al., 2020c).
DEX has been recommended to be used as an auxiliary sedative for cardiac patients. For those who underwent cardiac surgery with cardiopulmonary bypass (CPB), a loading dose of 1 μg/kg DEX with a continued infusion at 0.5 μg/kg/h could reduce intraoperative myocardial injury and attenuate inflammatory responses and oxidative stress, as evidenced by decreases in CK-MB, inflammatory factors and MDA (Bayram et al., 2014; Chen et al., 2021). A retrospective study of 2,068 cardiac surgery patients showed that DEX pretreatment was associated with a better cardiac outcome and a 7% increase in 5-year patient survival (Peng et al., 2021) (Table 1). Elucidating the molecular mechanism underlying the cardioprotection benefits of DEX would be useful for finding new promising pharmacological targets and catalyze the translation of cardioprotection related research into clinical settings.
4.1.2 Dexmedetomidine preconditioning induced cardioprotection against diabetic I/R injury
By stabilizing and maintaining blood lipids and blood glucose levels in patients with type 2 diabetes mellitus, DEX preconditioning is expected to be a new treatment modality for diabetic myocardial I/R injury (Hui Yun and Suk Choi, 2016; Sun et al., 2019). Arslan et al. (2014) proposed that DEX could improve the deformability of erythrocytes in rats model of diabetic myocardial I/R injury. By maintaining the blood glucose level and reducing oxidative stress-induced damage to cell membrane, DEX preconditioning could attenuate the alteration of erythrocyte deformability (Ozer et al., 2018), improve microcirculation and tissue perfusion (Simchon et al., 1987; Mokken et al., 1992), and offer protection against diabetic myocardial I/R injury. Several recent studies demonstrated that DEX preconditioning had cardioprotective effects against I/R injury in both streptozotocin-induced type 1 diabetic rats (Chang et al., 2020) and type 2 diabetic rats (Deng et al., 2019; Li et al., 2019; Guo et al., 2020) as evidenced by significantly reduced myocardial infarct sizes and plasma cTnT levels.
DEX also confers protection against remote organ injury caused by diabetic myocardial I/R insult. Intraperitoneal injection of DEX protected against myocardial I/R induced lung damage in diabetic rats, as evidenced by decreased neutrophil infiltration/aggregation and lung injury scores (Kip et al., 2015). More studies are needed to investigate the effect of DEX on different organ injuries resulting from diabetic myocardial I/R, which may contribute to the understanding of the underlying mechanisms of tissue damage associated with I/R injury in diabetes. The above studies revealed the unique features of DEX in diabetic myocardial I/R injury, which are the ability of DEX to balance blood glucose and lipids at a certain level, minimize the damage to the circulatory system, and mitigate injuries and adverse effects on distal organs.
Generalizing and exploring the dosage of DEX used has important implications for studying its effect on myocardial protection. We summarized the common doses of DEX used in most experimental studies. It seemed that a large dose of DEX usage was more likely to be beneficial than detrimental. The dosage of DEX used in animal models ranges from 1 μg/kg to 100 μg/kg, and 1 µM for mostly cellular models (Tables 2, 3) (Kip et al., 2015; Oh et al., 2019; Zhang et al., 2020b). It is necessary to determine the exact pharmacological dose of DEX that is biologically effective or harmful so that the side effects of DEX such as hypotension and cardiac arrest can be effectively avoided. In humans, DEX is administered as a premedication at a dose range of 0.33–0.67 μg/kg (Afonso and Reis, 2012). The above studies give a reference for an appropriate use of DEX in diabetic myocardial protection. Nevertheless, the cardioprotective effect of DEX preconditioning on I/R injury in diabetic patients has rarely been reported. One possible explanation is that the diabetic myocardium is highly vulnerable to I/R injury and the effect of DEX preconditioning is quite limited. DEX’s potential of lowering blood pressure and causing bradycardia or even sinus arrest might also compromise its cardioprotective effects (Reel and Maani, 2022).
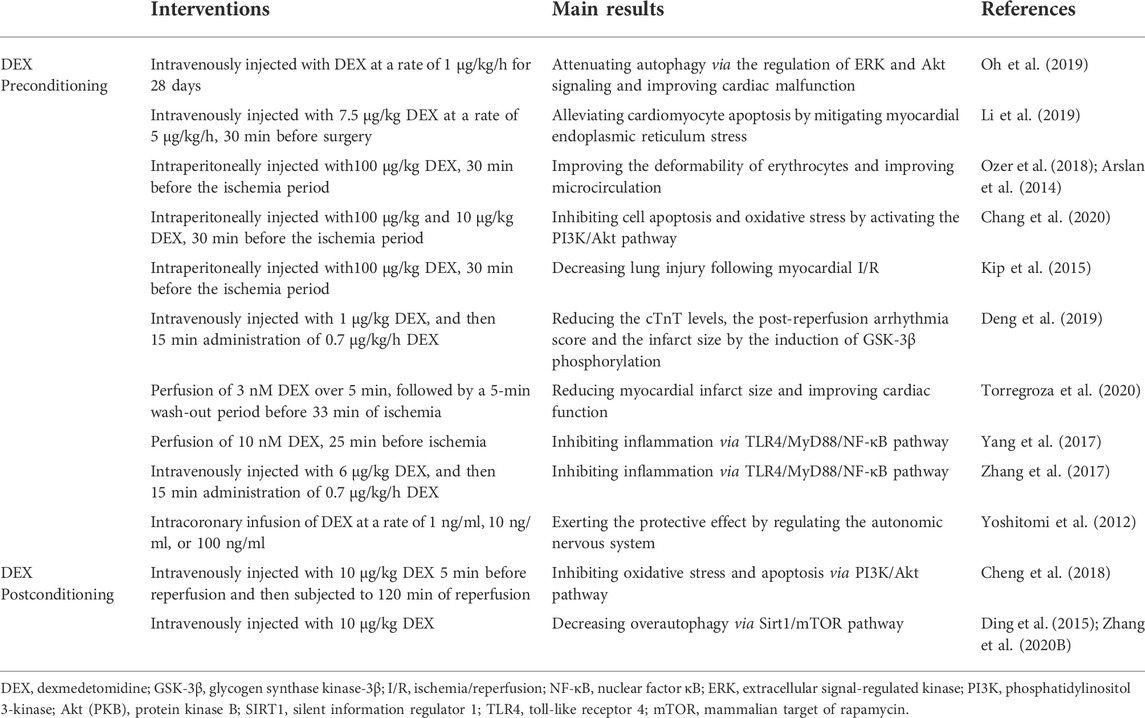
TABLE 2. Molecular mechanisms of DEX in the treatment of diabetic myocardial I/R injury at the animal level.
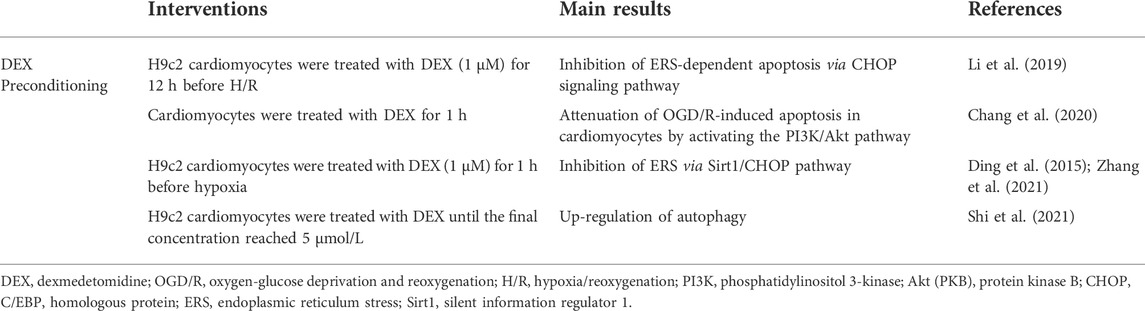
TABLE 3. Molecular mechanisms of DEX in the treatment of diabetic myocardial I/R injury at the cellular level.
4.1.3 Protection mechanisms of dexmedetomidine preconditioning in diabetic I/R injury
By inhibiting GSK-3β and affecting oxidative stress, apoptosis, calcium overload, and mPTP opening, DEX preconditioning presumably shields the myocardium from diabetes-induced activation of GSK-3β-mediated pathogenic effects (Chang et al., 2020; Guo et al., 2020). Another molecule possibly regulated by DEX is Sirt1, the absence of which is also closely related to the resistance of diabetic myocardium to medical interventions against I/R injury (Ding et al., 2015; Zhang Y. et al., 2021). In addition, the autonomic nervous system may also be involved.
4.1.3.1 Dexmedetomidine preconditioning and oxidative stress, apoptosis
DEX grants an anti-diabetic myocardial I/R injury profile through anti-apoptosis and anti-oxidant stress pathways (Chang et al., 2020). Preconditioning of H9c2 cardiomyocytes with DEX significantly mitigated apoptosis and oxidative stress induced by hyperglycemic hypoxic/reoxygenation injury (Chang et al., 2020). The protective effects of DEX against diabetic I/R injury-induced apoptosis and oxidative stress in cardiomyocytes might be mediated by inhibition of PI3K/Akt pathway (Chang et al., 2020). GSK-3β plays an important role in necrosis and apoptosis of cardiomyocytes as one of the downstream targets of PI3K/Akt pathway (Miura and Miki, 2009). GSK-3β activity is a determinant of the threshold for mPTP’s opening in cardiomyocytes (Yin et al., 2012). Its phosphorylation or inactivation can inhibit the opening of mPTP, which is associated with the upregulation of apoptotic cascade response process and oxidative stress (Yin et al., 2012). In the diabetic state, GSK-3β is activated and its activity is 2-fold higher than in non-diabetic state (Yin et al., 2012). DEX preconditioning could promote the phosphorylation of GSK-3β, inhibit mPTP opening, maintain mitochondrial function, block apoptotic cascade initiation, and enhance myocardial antioxidant defense (Kip et al., 2015; Guo et al., 2020). Intraperitoneal injection of DEX attenuates ischemia-reperfusion injury by reducing the upregulated expression of apoptosis-associated protein (p-BAD, BAX) and oxidative stress-related protein (MAD) in diabetic heart (Chang et al., 2020). Hyperglycemia dramatically induced cardiac dysfunction and ultrastructural disruption following I/R injury. Treatment with DEX remarkably ameliorated these abnormalities. Yohimbine, an α2-adrenergic receptor antagonist, could block the cardioprotective effects induced by DEX by inhibition the phosphorylation of GSK-3β (Deng et al., 2019). The underlying mechanisms that involved in the negative modulation of cardiomyocyte apoptosis and oxidative stress in these studies further proved the protective role of DEX in diabetic I/R-induced cardiac injury. However, in the current study, the molecular mechanism of DEX to attenuate apoptosis and oxidative stress induced by diabetic myocardial I/R is not sufficient, and further studies are needed to be carried out.
4.1.3.2 Dexmedetomidine preconditioning and endoplasmic reticulum stress
The endoplasmic reticulum is the arena where proteins are folded, modified and processed. Its normal function is crucial to the stability of the intracellular environment (Yan et al., 2019). The significant role of endoplasmic reticulum stress (ERS) in the pathogenesis of diabetic myocardial I/R injury has been widely accepted (Li W. et al., 2020). Inhibition of ERS would be an effective strategy to treat diabetic myocardial I/R injury. The myocardial protection of DEX in diabetes is associated with ERS inhibition. Preconditioning H9c2 cardiomyocytes with DEX (1 μM) could decrease the expression of mitochondrial apoptotic proteins and ERS-related proteins, including glucose-regulated protein (GRP78), C/EBP-homologous protein (CHOP), ERO1α, ERO1β and PDI. ERS agonist reversed the effects of DEX on hypoxia/reoxygenation (H/R)-induced apoptosis (Li W. et al., 2020). In diabetic rats model of myocardial I/R injury, DEX preconditioning could inhibit diabetes-exacerbated ERS and significantly reduce myocardial infarct size and improve myocardial ultrastructure damage (Li et al., 2019). The above results suggest that DEX is a novel myocardial protective agent for the treatment of diabetic myocardial I/R injury due to its strong inhibition of ERS-dependent apoptosis pathway.
4.1.3.3 Dexmedetomidine preconditioning and autophagy
Autophagy is a process of self-digestion, which can remove damaged cells and renew dysfunctional organelles and proteins (Huang et al., 2020). During myocardial I/R injury, autophagy plays diverse roles in different stages. At the stage of ischemia, autophagy is beneficial for providing cells with the energy needed and inhibiting apoptosis and necrosis. Nevertheless, at the stage of reperfusion, excessive autophagy has the detrimental effect of destroying cellular components and causing myocardial injury (Shi et al., 2019). Modulation of autophagy has been considered an appropriate therapeutic option for cardioprotection (Liu et al., 2017; Liu R. et al., 2018; Li Y. et al., 2020). Studies revealed that DEX could upregulate autophagy and reduce myocardial H/R injury in isolated cardiomyocytes under high glucose conditions (Shi et al., 2021). It was found that DEX could ameliorate cardiac dysfunction and autophagic impairment in diabetic rats by suppressing the expression of LC3B and autophagy related genes (ATG) and proteins (Oh et al., 2019). Thus it is hypothesized that DEX could exert different effects on autophagy at different stages of I/R. The autophagy regulation of DEX in diabetic myocardial I/R injury and its molecular mechanism are to be further explored in the future.
4.1.3.4 Dexmedetomidine preconditioning and inflammation
Inflammation is one of the most important pathological mechanisms of myocardial I/R injury. DEX reduced the expression of inflammatory factors such as TNF-α and IL-1β, and inhibited the inflammatory response in vivo (Yang et al., 2017; Zhang et al., 2017). TLR4/MyD88/NF-κB signaling pathway plays a key role in the regulation of inflammation. DEX preconditioning could down-regulate HMGB1 mediated the TLR4/MyD88/NF-кB signaling pathway and attenuate myocardial I/R injury (Yang et al., 2017; Zhang et al., 2017). It is believed that the inhibition of HMGB1-mediated TLR4/MyD88/NF-κB signaling pathway may be one of the anti-inflammatory mechanisms of DEX to induce myocardial protection (Yang et al., 2017; Zhang et al., 2017). Interestingly, DEX could also reduce systemic inflammatory response through TLR4/MyD88/NF-κB pathway in lower limb surgery of diabetic patients. However, whether DEX could alleviate myocardial I/R injury in diabetes mellitus by inhibiting HMGB1 mediated inflammation remains unclear.
4.1.3.5 Dexmedetomidine preconditioning and autonomic nervous system
DEX has been found to protect against myocardial I/R injury by regulating the autonomic nervous system (Yoshitomi et al., 2012). Through inhibiting the norepinephrine neuron activity in the locus coeruleus, DEX could suppress sympathetic excitation, reduce catecholamine level in the blood, and decrease cardiac load and myocardial oxygen consumption. Meanwhile, with prolonged time of diastolic perfusion and increased left ventricular coronary blood flow, the release of cardiac lactic acid was reduced and the resistance of myocardial to ischemia and hypoxia was enhanced. In addition, DEX could directly inhibit the release of cardiac norepinephrine and lower the incidence of arrhythmia in high-risk patients. The mechanism may be due to the parasympathetic effect of DEX on calcium ions transport in cardiomyocytes (Yoshitomi et al., 2012; Bao and Tang, 2020). Furthermore, studies have shown that DEX increases vagal nerve tone and triggers its anti-inflammatory effect, which may contribute to the alleviation of myocardial I/R injury (Zi et al., 2019; Ju et al., 2020). Heart rate variability reflects the balance of the patient’s autonomic nervous system. In the diabetic state, DEX affects heart rate variability by regulating autonomic nerve function (Ye et al., 2021). However, the mechanism of how DEX regulates diabetic myocardial I/R injury through autonomic nervous system has not yet been well studied.
Sirt1 is a multifunctional molecule involved in myocardial I/R injury (Tian et al., 2019; Wang et al., 2020; Wang and Hu, 2020). Overexpression of Sirt1 could improve cardiac function and protect the myocardium in diabetic rats (Ding et al., 2015). DEX inhibits oxidative stress, inflammation and apoptosis by up-regulating the expression of Sirt1 and improves myocardial I/R injury in non-diabetic states (Zhang Y. et al., 2021). The effect of DEX on Sirt1 in diabetic myocardial I/R injury is well worth studying, which will provide a new theoretical basis for the treatment of diabetic myocardial I/R injury.
Taken together, the pathophysiologic process of diabetic myocardial I/R injury is extremely complex and multifactorial. DEX can work on multiple pathways simultaneously to effectively protect diabetic myocardium against I/R damage, which might be an explanation for the effectiveness of DEX for the treatment of diabetic myocardial I/R. Hence, more powerful multi-targets drug development could be a direction for future research, which will make the therapy against diabetic myocardial I/R injury more effective.
4.2 Dexmedetomidine and its potential postconditioning
Pharmacological postconditioning with DEX shows more clinical advantages than invasive ischemic postconditioning (Wu et al., 2021). From the perspective of clinical application value, pharmacological postconditioning would be a more suitable alternative treatment. Results from several experimental studies seemed to be consistent with the findings that postconditioning with DEX could ameliorate myocardial I/R injury (Cheng et al., 2018; Zhang et al., 2020b). The effect of DEX postconditioning was concentration-dependent, in ranges between 0.3 and 3 nM. Increased concentrations of above 3 nM failed to further enhance the effect. The cardioprotective effect is independent of the time point and the length of application in the reperfusion period (Cheng et al., 2016; Bunte et al., 2020).
4.2.1 Dexmedetomidine postconditioning-induced cardioprotection against diabetic I/R injury
The currently available data on the application of DEX postconditioning in diabetic myocardial I/R injury are minimal. A study by Cheng et al. (Cheng et al., 2018) reported that DEX postconditioning exerted the same effect as DEX preconditioning in diabetic myocardial I/R model. The potency of DEX postconditioning mediated cardioprotection was reflected in the reduction of plasma CK-MB, LDH and MDA, and an improvement in myocardial histology. However, an opposite result was found by Torregroza et al. who concluded that DEX postconditioning was unable to maintain its cardioprotective properties with acute hyperglycemia (Torregroza et al., 2020). This discrepancy was probably due to the difference in experimental models. Chen et al. investigated type 2 diabetes, a chronic inflammatory condition, whereas Torregroza et al. studied an acute hyperglycemic model. Due to the unpredictability of preconditioning, postconditioning is of higher value. Diabetes mellitus and acute hyperglycemia ought to be involved in future translational research with DEX postconditioning.
4.2.2 Protection mechanisms of dexmedetomidine postconditioning in diabetic I/R injury
Similar to DEX preconditioning, the protective role of DEX postconditioning in diabetic myocardium is also associated with GSK-3β. DEX postconditioning promotes the phosphorylation of GSK-3β and inhibits its activity via PI3K/Akt pathway (Cheng et al., 2018; Del'Guidice and Beaulieu, 2010). The phosphorylation of GSK-3β could reduce I/R damage and protect the myocardium by adjusting the Bcl-2/Bax ratio and inhibiting the caspase-controlled apoptotic pathway (Deng et al., 2019; Wang et al., 2019; Liu et al., 2020; Sharma et al., 2020). In diabetic rats subjected to myocardial I/R, DEX postconditioning inhibited cardiomyocyte apoptosis and oxidative stress with an elevation of p-PI3K, p-Akt, and a reduction in GSK-3β. And the effects were abrogated by PI3K inhibitors (Cheng et al., 2018). In addition, a study showed that DEX postconditioning could alleviate myocardial I/R injury by activating the Sirt1/mTOR axis (Zhang et al., 2020b). Whether the Sirt1/mTOR axis plays a role in alleviating myocardial I/R injury in diabetes by DEX postconditioning has not been reported. These results suggested that both preconditioning and postconditioning of DEX activate the PI3K/Akt signaling pathway, inducing the phosphorylation of downstream kinases to inhibit several pro-apoptotic factors and the irreversible opening of mPTP, then with increased the expression of p-GSK-3β in myocardial tissue with diabetic I/R injury, effectively inhibiting apoptosis and oxidative stress. However, the degree of activation of related signaling pathway by the two conditioning methods might be different. Study has shown that acute hyperglycemia abolished the protective effect of DEX postconditioning but retained the beneficial effect of DEX preconditioning on I/R injured myocardium (Torregroza et al., 2020). This can be explained that the degree of activation of related signaling pathway by the two conditioning methods might be different. Preconditioning of DEX probably could be more effective in activation of PI3K/Akt than postconditioning of DEX. There is also a possibility that preconditioning could provide more powerful protection effect through some other unknown pathways, which needs to be further investigated.
5 Conclusion and perspective
In this review, the evidence and the possible mechanisms of DEX in reducing myocardial I/R injury in diabetes are discussed (Figure 1). The Activation of PI3K/Akt/GSK-3β, inhibition of CHOP, attenuation of oxidative stress, regulation of autophagy, and protection of mitochondrial function are possible mechanisms that collectively contribute to DEX’s protection of diabetic myocardium. The protection of DEX on I/R insulted myocardium was not entirely dependent on the activation of α2-adrenoceptor (Yin et al., 2020). DEX may also exert protective effects by activating several other receptors (Zhang et al., 2012). This potentially interesting hypothesis needs to be verified in future studies. Other α2-adrenoceptors have not been reported in the study of diabetic myocardial protection, and their effectiveness in attenuating myocardial I/R injury in diabetes is to be confirmed. Although DEX has been widely used in various clinical scenarios, its research on diabetic myocardial I/R injury is quite limited. A better understanding of the mechanisms underlying DEX-related myocardial protection would be helpful for establishing new protective methods and developing more promising cardioprotective agents against diabetic myocardial I/R injury in the future.
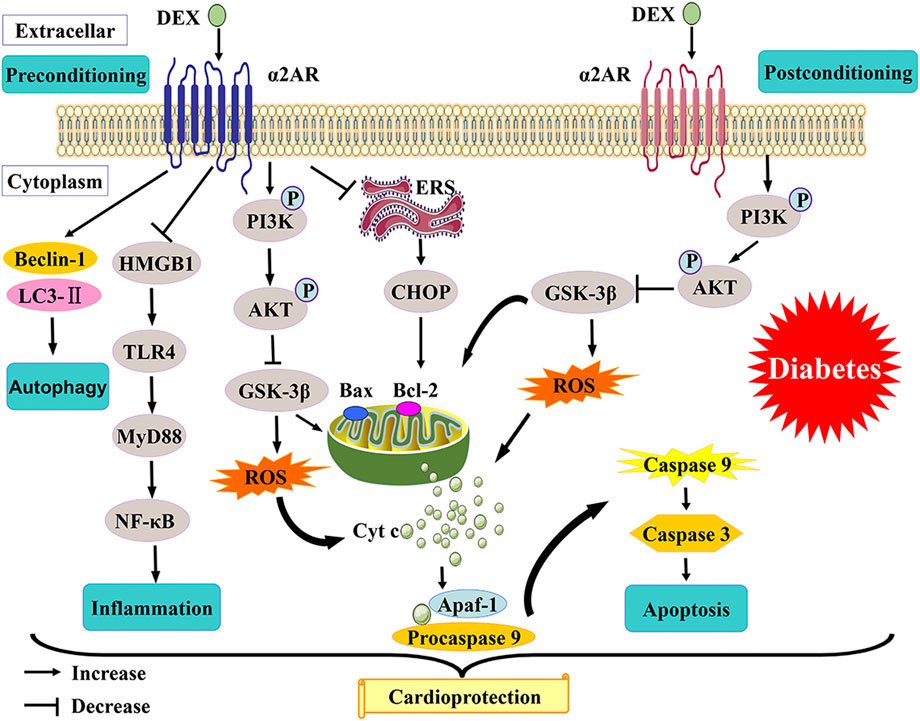
FIGURE 1. A schematic of proposed mechanisms of cardioprotection from DEX preconditioning and postconditioning in diabetes.
Author contributions
MS, RW, RX, ZX, ZW, and TW contributed to the design of the review. MS, RW, and RX drafted the manuscript. MS, RW, ZX, ZW, and TW contributed to discussion and revised the manuscript. All the authors read and approved the final version of the manuscript.
Funding
This work was supported by grants from the National Natural Science Foundation of China (NO. 81770824) and Natural Science Foundation of Hubei Province (NO. 2020CFB797).
Conflict of interest
The authors declare that the research was conducted in the absence of any commercial or financial relationships that could be construed as a potential conflict of interest.
Publisher’s note
All claims expressed in this article are solely those of the authors and do not necessarily represent those of their affiliated organizations, or those of the publisher, the editors and the reviewers. Any product that may be evaluated in this article, or claim that may be made by its manufacturer, is not guaranteed or endorsed by the publisher.
References
Afonso, J., and Reis, F. (2012). Dexmedetomidine: Current role in anesthesia and intensive care. Rev. Bras. Anestesiol. 62, 118–133. doi:10.1016/s0034-7094(12)70110-1
Alegria, J. R., Miller, T. D., Gibbons, R. J., Yi, Q. L., and Yusuf, S. (2007). Infarct size, ejection fraction, and mortality in diabetic patients with acute myocardial infarction treated with thrombolytic therapy. Am. Heart J. 154, 743–750. doi:10.1016/j.ahj.2007.06.020
Arriel, R. A., Meireles, A., Hohl, R., and Marocolo, M. (2020). Ischemic preconditioning improves performance and accelerates the heart rate recovery. J. Sports Med. Phys. Fit. 60, 1209–1215. doi:10.23736/s0022-4707.20.10822-3
Arslan, M., Comu, F. M., Isik, B., Ozturk, L., and Kesimci, E. (2012). Effect of dexmedetomidine on erythrocyte deformability during ischemia-reperfusion injury of liver in diabetic rats. Bratisl. Lek. Listy 113, 687–691. doi:10.4149/bll_2012_156
Arslan, M., Comu, F. M., Kip, G., Alkan, M., Kiraz, H. A., Ozer, A., et al. (2014). Effect of dexmedetomidine on erythrocyte deformability during ischaemia-reperfusion injury of heart in diabetic rats. Bratisl. Lek. Listy 115, 494–497. doi:10.4149/bll_2014_096
Bao, N., and Tang, B. (2020). Organ-protective effects and the underlying mechanism of dexmedetomidine. Mediat. Inflamm. 2020, 6136105. doi:10.1155/2020/6136105
Bayram, A., Ulgey, A., Baykan, A., Narin, N., Narin, F., Esmaoglu, A., et al. (2014). The effects of dexmedetomidine on early stage renal functions in pediatric patients undergoing cardiac angiography using non-ionic contrast media: A double-blind, randomized clinical trial. Paediatr. Anaesth. 24, 426–432. doi:10.1111/pan.12348
Belleville, J. P., Ward, D. S., Bloor, B. C., and Maze, M. (1992). Effects of intravenous dexmedetomidine in humans. I. Sedation, ventilation, and metabolic rate. Anesthesiology 77, 1125–1133. doi:10.1097/00000542-199212000-00013
Berezin, A. E., and Berezin, A. A. (2019). Impaired function of fibroblast growth factor 23/klotho protein Axis in prediabetes and diabetes mellitus: Promising predictor of cardiovascular risk. Diabetes Metab. Syndr. 13, 2549–2556. doi:10.1016/j.dsx.2019.07.018
Bunte, S., Behmenburg, F., Majewski, N., Stroethoff, M., Raupach, A., Mathes, A., et al. (2020). Characteristics of dexmedetomidine postconditioning in the field of myocardial ischemia-reperfusion injury. Anesth. Analg. 130, 90–98. doi:10.1213/ane.0000000000004417
Cai, Y., Xu, H., Yan, J., Zhang, L., and Lu, Y. (2014). Molecular targets and mechanism of action of dexmedetomidine in treatment of ischemia/reperfusion injury. Mol. Med. Rep. 9, 1542–1550. doi:10.3892/mmr.2014.2034
Castillo, R. L., Ibacache, M., Cortínez, I., Carrasco-Pozo, C., Farías, J. G., Carrasco, R. A., et al. (2019). Dexmedetomidine improves cardiovascular and ventilatory outcomes in critically ill patients: Basic and clinical approaches. Front. Pharmacol. 10, 1641. doi:10.3389/fphar.2019.01641
Chang, J. H., Jin, M. M., and Liu, J. T. (2020). Dexmedetomidine pretreatment protects the heart against apoptosis in ischemia/reperfusion injury in diabetic rats by activating pi3k/akt signaling in vivo and in vitro. Biomed. Pharmacother. 127, 110188. doi:10.1016/j.biopha.2020.110188
Chen, L., Cao, J., Cao, D., Wang, M., Xiang, H., Yang, Y., et al. (2019). Protective effect of dexmedetomidine against diabetic hyperglycemia-exacerbated cerebral ischemia/reperfusion injury: An in vivo and in vitro study. Life Sci. 235, 116553. doi:10.1016/j.lfs.2019.116553
Chen, W., Wang, Y., Pan, Z., Chen, X., Luo, D., and Wang, H. (2021). Protective effects of dexmedetomidine on the ischemic myocardium in patients undergoing rheumatic heart valve replacement surgery. Exp. Ther. Med. 21, 427. doi:10.3892/etm.2021.9844
Cheng, X., Hu, J., Wang, Y., Ye, H., Li, X., Gao, Q., et al. (2018). Effects of dexmedetomidine postconditioning on myocardial ischemia/reperfusion injury in diabetic rats: Role of the pi3k/akt-dependent signaling pathway. J. Diabetes Res. 2018, 3071959. doi:10.1155/2018/3071959
Cheng, X. Y., Gu, X. Y., Gao, Q., Zong, Q. F., Li, X. H., and Zhang, Y. (2016). Effects of dexmedetomidine postconditioning on myocardial ischemia and the role of the pi3k/akt-dependent signaling pathway in reperfusion injury. Mol. Med. Rep. 14, 797–803. doi:10.3892/mmr.2016.5345
Del'Guidice, T., and Beaulieu, J. M. (2010). Psychotropic drugs and the involvement of the akt/gsk3 signalling pathway in mental illnesses. Med. Sci. 26, 647–651. doi:10.1051/medsci/2010266-7647
Deng, L., Chen, H., Wei, N., Zhang, Z., and Wang, G. (2019). The cardioprotective effect of dexmedetomidine on regional ischemia/reperfusion injury in type 2 diabetic rat hearts. Microvasc. Res. 123, 1–6. doi:10.1016/j.mvr.2018.08.006
Ding, M., Dong, Q., Liu, Z., Liu, Z., Qu, Y., Li, X., et al. (2017). Inhibition of dynamin-related protein 1 protects against myocardial ischemia-reperfusion injury in diabetic mice. Cardiovasc. Diabetol. 16, 19. doi:10.1186/s12933-017-0501-2
Ding, M., Lei, J., Han, H., Li, W., Qu, Y., Fu, E., et al. (2015). Sirt1 protects against myocardial ischemia-reperfusion injury via activating enos in diabetic rats. Cardiovasc. Diabetol. 14, 143. doi:10.1186/s12933-015-0299-8
Donato, M., Evelson, P., and Gelpi, R. J. (2017). Protecting the heart from ischemia/reperfusion injury: An update on remote ischemic preconditioning and postconditioning. Curr. Opin. Cardiol. 32, 784–790. doi:10.1097/hco.0000000000000447
Dong, J., Guo, X., Yang, S., and Li, L. (2017). The effects of dexmedetomidine preconditioning on aged rat heart of ischaemia reperfusion injury. Res. Vet. Sci. 114, 489–492. doi:10.1016/j.rvsc.2017.09.028
Ebert, T. J., Hall, J. E., Barney, J. A., Uhrich, T. D., and Colinco, M. D. (2000). The effects of increasing plasma concentrations of dexmedetomidine in humans. Anesthesiology 93, 382–394. doi:10.1097/00000542-200008000-00016
Fisher, M. (1999). Diabetes and myocardial infarction. Baillieres Best. Pract. Res. Clin. Endocrinol. Metab. 13, 331–343. doi:10.1053/beem.1999.0024
Frank, A., Bonney, M., Bonney, S., Weitzel, L., Koeppen, M., and Eckle, T. (2012). Myocardial ischemia reperfusion injury: From basic science to clinical bedside. Semin. Cardiothorac. Vasc. Anesth. 16, 123–132. doi:10.1177/1089253211436350
Gao, S., Wang, R., Dong, S., Wu, J., Perek, B., Xia, Z., et al. (2021). Inactivation of topk caused by hyperglycemia blocks diabetic heart sensitivity to sevoflurane postconditioning by impairing the pten/pi3k/akt signaling. Oxid. Med. Cell. Longev. 2021, 6657529. doi:10.1155/2021/6657529
Gao, S., Yang, Z., Shi, R., Xu, D., Li, H., Xia, Z., et al. (2016). Diabetes blocks the cardioprotective effects of sevoflurane postconditioning by impairing nrf2/brg1/Ho-1 signaling. Eur. J. Pharmacol. 779, 111–121. doi:10.1016/j.ejphar.2016.03.018
Guo, Y., Gao, H., Zhao, K., Zhao, G., and Zeng, X. (2020). Therapeutic effect of dexmedetomidine on myocardial ischemia reperfusion injury in type 2 diabetic rat model under P13k/akt pathway. J. King Saud Univ. - Sci. 32, 2553–2560. doi:10.1016/j.jksus.2020.03.045
He, L., Hao, S., Wang, Y., Yang, W., Liu, L., Chen, H., et al. (2019). Dexmedetomidine preconditioning attenuates ischemia/reperfusion injury in isolated rat hearts with endothelial dysfunction. Biomed. Pharmacother. 114, 108837. doi:10.1016/j.biopha.2019.108837
Henning, R. J. (2018). Type-2 diabetes mellitus and cardiovascular disease. Future Cardiol. 14, 491–509. doi:10.2217/fca-2018-0045
Heusch, G., Boengler, K., and Schulz, R. (2010). Inhibition of mitochondrial permeability transition pore opening: The holy grail of cardioprotection. Basic Res. Cardiol. 105, 151–154. doi:10.1007/s00395-009-0080-9
Heusch, G. (2015). Treatment of myocardial ischemia/reperfusion injury by ischemic and pharmacological postconditioning. Compr. Physiol. 5, 1123–1145. doi:10.1002/cphy.c140075
Hou, X., Xu, F., Zhang, C., Shuai, J., Huang, Z., Liang, Y., et al. (2020). Dexmedetomidine exerts neuroprotective effects during high glucose-induced neural injury by inhibiting mir-125b. Biosci. Rep. 40, BSR20200394. doi:10.1042/bsr20200394
Huang, K. Y., Que, J. Q., Hu, Z. S., Yu, Y. W., Zhou, Y. Y., Wang, L., et al. (2020). Metformin suppresses inflammation and apoptosis of myocardiocytes by inhibiting autophagy in a model of ischemia-reperfusion injury. Int. J. Biol. Sci. 16, 2559–2579. doi:10.7150/ijbs.40823
Hui Yun, S., and Suk Choi, Y. (2016). The effects of dexmedetomidine administration on postoperative blood glucose levels in diabetes mellitus patients undergoing spinal anesthesia: A pilot study. Anesth. Pain Med. 6, e40483. doi:10.5812/aapm.40483
Ju, Y., Xiao, F., Lu, J., Zhou, B., Cai, J., and Chen, S. (2020). Effect of dexmedetomidine and cholinergic anti-inflammatory pathways in myocardial ischemia-reperfusion injury. Pak. J. Pharm. Sci. 33, 1377–1382. doi:10.36721/PJPS.2020.33.3.SP.1377-1382.1
Keating, G. M. (2015). Dexmedetomidine: A review of its use for sedation in the intensive care setting. Drugs 75, 1119–1130. doi:10.1007/s40265-015-0419-5
Kim, J. S., He, L., and Lemasters, J. J. (2003). Mitochondrial permeability transition: A common pathway to necrosis and apoptosis. Biochem. Biophys. Res. Commun. 304, 463–470. doi:10.1016/s0006-291x(03)00618-1
Kip, G., Çelik, A., Bilge, M., Alkan, M., Kiraz, H. A., Özer, A., et al. (2015). Dexmedetomidine protects from post-myocardial ischaemia reperfusion lung damage in diabetic rats. Libyan J. Med. 10, 27828. doi:10.3402/ljm.v10.27828
Lejay, A., Fang, F., John, R., Van, J. A., Barr, M., Thaveau, F., et al. (2016). Ischemia reperfusion injury, ischemic conditioning and diabetes mellitus. J. Mol. Cell. Cardiol. 91, 11–22. doi:10.1016/j.yjmcc.2015.12.020
Li, D., Huang, B., Liu, J., Li, L., and Li, X. (2013). Decreased brain K(atp) channel contributes to exacerbating ischemic brain injury and the failure of neuroprotection by sevoflurane post-conditioning in diabetic rats. PLoS One 8, e73334. doi:10.1371/journal.pone.0073334
Li, J., Chen, Q., He, X., Alam, A., Ning, J., Yi, B., et al. (2018). Dexmedetomidine attenuates lung apoptosis induced by renal ischemia-reperfusion injury through Α(2)Ar/Pi3k/Akt pathway. J. Transl. Med. 16, 78. doi:10.1186/s12967-018-1455-1
Li, J., Zhao, Y., Zhou, N., Li, L., and Li, K. (2019). Dexmedetomidine attenuates myocardial ischemia-reperfusion injury in diabetes mellitus by inhibiting endoplasmic reticulum stress. J. Diabetes Res. 2019, 7869318. doi:10.1155/2019/7869318
Li, T., Tan, Y., Ouyang, S., He, J., and Liu, L. (2022). Resveratrol protects against myocardial ischemia-reperfusion injury via attenuating ferroptosis. Gene 808, 145968. doi:10.1016/j.gene.2021.145968
Li, W., Li, W., Leng, Y., Xiong, Y., and Xia, Z. (2020). Ferroptosis is involved in diabetes myocardial ischemia/reperfusion injury through endoplasmic reticulum stress. DNA Cell. Biol. 39, 210–225. doi:10.1089/dna.2019.5097
Li, Y., Liang, P., Jiang, B., Tang, Y., Liu, X., Liu, M., et al. (2020). Card9 promotes autophagy in cardiomyocytes in myocardial ischemia/reperfusion injury via interacting with rubicon directly. Basic Res. Cardiol. 115, 29. doi:10.1007/s00395-020-0790-6
Liang, S., Wang, Y., and Liu, Y. (2019). Dexmedetomidine alleviates lung ischemia-reperfusion injury in rats by activating pi3k/akt pathway. Eur. Rev. Med. Pharmacol. Sci. 23, 370–377. doi:10.26355/eurrev_201901_16785
Lim, H., Kim, T. Y., Kim, S. Y., Ro, S. J., Koh, S. R., Ryu, S., et al. (2021). The protective effects of dexmedetomidine preconditioning on hepatic ischemia/reperfusion injury in rats. Transpl. Proc. 53, 427–435. doi:10.1016/j.transproceed.2020.10.014
Liu, C. Y., Zhang, Y. H., Li, R. B., Zhou, L. Y., An, T., Zhang, R. C., et al. (2018). Lncrna caif inhibits autophagy and attenuates myocardial infarction by blocking P53-mediated myocardin transcription. Nat. Commun. 9, 29. doi:10.1038/s41467-017-02280-y
Liu, H., Wu, X., Luo, J., Zhao, L., Li, X., Guo, H., et al. (2020). Adiponectin peptide alleviates oxidative stress and Nlrp3 inflammasome activation after cerebral ischemia-reperfusion injury by regulating ampk/gsk-3β. Exp. Neurol. 329, 113302. doi:10.1016/j.expneurol.2020.113302
Liu, L., Jin, X., Hu, C. F., Li, R., Zhou, Z., and Shen, C. X. (2017). Exosomes derived from mesenchymal stem cells rescue myocardial ischaemia/reperfusion injury by inducing cardiomyocyte autophagy via ampk and Akt pathways. Cell. Physiol. biochem. 43, 52–68. doi:10.1159/000480317
Liu, R., Li, X., and Zhang, X. (2018). Dexmedetomidine protects high-glucose induced apoptosis in human retinal pigment epithelial cells through inhibition on P75(ntr). Biomed. Pharmacother. 106, 466–471. doi:10.1016/j.biopha.2018.06.117
Marso, S. P., Miller, T., Rutherford, B. D., Gibbons, R. J., Qureshi, M., Kalynych, A., et al. (2007). Comparison of myocardial reperfusion in patients undergoing percutaneous coronary intervention in st-segment elevation acute myocardial infarction with versus without diabetes mellitus (from the emerald trial). Am. J. Cardiol. 100, 206–210. doi:10.1016/j.amjcard.2007.02.080
Miki, T., Itoh, T., Sunaga, D., and Miura, T. (2012). Effects of diabetes on myocardial infarct size and cardioprotection by preconditioning and postconditioning. Cardiovasc. Diabetol. 11, 67. doi:10.1186/1475-2840-11-67
Miura, T., and Miki, T. (2009). Gsk-3beta, a therapeutic target for cardiomyocyte protection. Circ. J. 73, 1184–1192. doi:10.1253/circj.cj-09-0284
Mocanu, M. M., and Yellon, D. M. (2007). Pten, the achilles' heel of myocardial ischaemia/reperfusion injury? Br. J. Pharmacol. 150, 833–838. doi:10.1038/sj.bjp.0707155
Mokhtari-Zaer, A., Marefati, N., Atkin, S. L., Butler, A. E., and Sahebkar, A. (2018). The protective role of curcumin in myocardial ischemia-reperfusion injury. J. Cell. Physiol. 234, 214–222. doi:10.1002/jcp.26848
Mokken, F. C., Kedaria, M., Henny, C. P., Hardeman, M. R., and Gelb, A. W. (1992). The clinical importance of erythrocyte deformability, a hemorrheological parameter. Ann. Hematol. 64, 113–122. doi:10.1007/bf01697397
Oh, J. E., Jun, J. H., Hwang, H. J., Shin, E. J., Oh, Y. J., and Choi, Y. S. (2019). Dexmedetomidine restores autophagy and cardiac dysfunction in rats with streptozotocin-induced diabetes mellitus. Acta Diabetol. 56, 105–114. doi:10.1007/s00592-018-1225-9
Okada, H., Kurita, T., Mochizuki, T., Morita, K., and Sato, S. (2007). The cardioprotective effect of dexmedetomidine on global ischaemia in isolated rat hearts. Resuscitation 74, 538–545. doi:10.1016/j.resuscitation.2007.01.032
Ozer, A., Comu, F. M., Kucuk, A., Kilic, Y., Alkan, M., Oktar, L., et al. (2018). Effects of dexmedetomidine and thymoquinone on erythrocyte deformability in lower limb ischemia reperfusion injury in streptozotocin-induced diabetic rats. Bratisl. Lek. Listy 119, 642–645. doi:10.4149/bll_2018_115
Panzer, O., Moitra, V., and Sladen, R. N. (2009). Pharmacology of sedative-analgesic agents: Dexmedetomidine, remifentanil, ketamine, volatile anesthetics, and the role of peripheral mu antagonists. Crit. Care Clin. 25, 451–469. vii. doi:10.1016/j.ccc.2009.04.004
Peng, K., Shen, Y. P., Ying, Y. Y., Kiaii, B., Rodriguez, V., Boyd, D., et al. (2021). Perioperative dexmedetomidine and 5-year survival in patients undergoing cardiac surgery. Br. J. Anaesth. 127, 215–223. doi:10.1016/j.bja.2021.03.040
Potz, B. A., Sabe, A. A., Abid, M. R., and Sellke, F. W. (2016). Calpains and coronary vascular disease. Circ. J. 80, 4–10. doi:10.1253/circj.CJ-15-0997
Reel, B., and Maani, C. V. (2022). “Dexmedetomidine. Statpearls,” in StatPearls publishing copyright © 2022 (Treasure Island (FL): StatPearls Publishing LLC.).
Riquelme, J. A., Westermeier, F., Hall, A. R., Vicencio, J. M., Pedrozo, Z., Ibacache, M., et al. (2016). Dexmedetomidine protects the heart against ischemia-reperfusion injury by an endothelial enos/No dependent mechanism. Pharmacol. Res. 103, 318–327. doi:10.1016/j.phrs.2015.11.004
Russell, J. S., Griffith, T. A., Helman, T., Du Toit, E. F., Peart, J. N., and Headrick, J. P. (2019). Chronic type 2 but not type 1 diabetes impairs myocardial ischaemic tolerance and preconditioning in C57bl/6 mice. Exp. Physiol. 104, 1868–1880. doi:10.1113/ep088024
Russo, I., Penna, C., Musso, T., Popara, J., Alloatti, G., Cavalot, F., et al. (2017). Platelets, diabetes and myocardial ischemia/reperfusion injury. Cardiovasc. Diabetol. 16, 71. doi:10.1186/s12933-017-0550-6
Sahin, T., Begeç, Z., Toprak, H., Polat, A., Vardi, N., Yücel, A., et al. (2013). The effects of dexmedetomidine on liver ischemia-reperfusion injury in rats. J. Surg. Res. 183, 385–390. doi:10.1016/j.jss.2012.11.034
Sarwar, N., Gao, P., Seshasai, S. R., Gobin, R., Kaptoge, S., Di Angelantonio, E., et al. (2010). Diabetes mellitus, fasting blood glucose concentration, and risk of vascular disease: A collaborative meta-analysis of 102 prospective studies. Lancet 375, 2215–2222. doi:10.1016/s0140-6736(10)60484-9
Sasso, F. C., Furbatto, F., Carbonara, O., Nasti, R., Morra, S., Torella, R., et al. (2011). Prevalence of diabetes in patients with nonacute cad. Acta Diabetol. 48, 247–248. doi:10.1007/s00592-011-0263-3
Schmidt, A. M. (2018). Highlighting diabetes mellitus: The epidemic continues. Arterioscler. Thromb. Vasc. Biol. 38, e1–e8. doi:10.1161/atvbaha.117.310221
Sharma, A. K., Thanikachalam, P. V., and Bhatia, S. (2020). The signaling interplay of gsk-3β in myocardial disorders. Drug Discov. Today 25, 633–641. doi:10.1016/j.drudis.2020.01.017
Shi, B., Ma, M., Zheng, Y., Pan, Y., and Lin, X. (2019). Mtor and Beclin1: Two key autophagy-related molecules and their roles in myocardial ischemia/reperfusion injury. J. Cell. Physiol. 234, 12562–12568. doi:10.1002/jcp.28125
Shi, Z., Xiong, Y., Li, B., and Xia, Z. (2021). Role of autophagy in reduction of high glucose and hypoxia-reoxygenation injury to isolated cardiomyocytes by dexmedetomidine in rats. Chin. J. Anesthesiol. 41 242–245. doi:10.3760/cma.j.cn131073.20200922.00227
Simchon, S., Jan, K. M., and Chien, S. (1987). Influence of reduced red cell deformability on regional blood flow. Am. J. Physiol. 253, H898–H903. doi:10.1152/ajpheart.1987.253.4.H898
Sobczyk, A., Świątkowski, A., Francuz, P., Kowalczyk, J., Kalarus, Z., and Średniawa, B. (2020). Therapeutic hypothermia and postreperfusion myocardial injury in myocardial infarction. Pol. Merkur. Lek. 48, 365–369.
Strain, W. D., and Paldánius, P. M. (2018). Diabetes, cardiovascular disease and the microcirculation. Cardiovasc. Diabetol. 17, 57. doi:10.1186/s12933-018-0703-2
Sun, Y., Gao, Q., Wu, N., Li, S. D., Yao, J. X., and Fan, W. J. (2015). Protective effects of dexmedetomidine on intestinal ischemia-reperfusion injury. Exp. Ther. Med. 10, 647–652. doi:10.3892/etm.2015.2561
Sun, Y., Li, L., Wu, J., Gong, B., and Liu, H. (2019). Germacrone cooperates with dexmedetomidine to alleviate high-fat diet-induced type 2 diabetes mellitus via upregulating Ampkα1 expression. Exp. Ther. Med. 18, 3514–3524. doi:10.3892/etm.2019.7990
Szabó, I., and Zoratti, M. (1991). The giant channel of the inner mitochondrial membrane is inhibited by cyclosporin A. J. Biol. Chem. 266, 3376–3379. doi:10.1016/s0021-9258(19)67802-6
Tian, L., Cao, W., Yue, R., Yuan, Y., Guo, X., Qin, D., et al. (2019). Pretreatment with tilianin improves mitochondrial energy metabolism and oxidative stress in rats with myocardial ischemia/reperfusion injury via ampk/sirt1/pgc-1 alpha signaling pathway. J. Pharmacol. Sci. 139, 352–360. doi:10.1016/j.jphs.2019.02.008
Torregroza, C., Feige, K., Schneider, L., Bunte, S., Stroethoff, M., Heinen, A., et al. (2020). Influence of hyperglycemia on dexmedetomidine-induced cardioprotection in the isolated perfused rat heart. J. Clin. Med. 9, E1445. doi:10.3390/jcm9051445
Valikeserlis, I., Athanasiou, A. A., and Stakos, D. (2021). Cellular mechanisms and pathways in myocardial reperfusion injury. Coron. Artery Dis. 32, 567–577. doi:10.1097/mca.0000000000000997
Venn, R. M., and Grounds, R. M. (2001). Comparison between dexmedetomidine and propofol for sedation in the intensive care unit: Patient and clinician perceptions. Br. J. Anaesth. 87, 684–690. doi:10.1093/bja/87.5.684
Venn, R. M., Hell, J., and Grounds, R. M. (2000). Respiratory effects of dexmedetomidine in the surgical patient requiring intensive care. Crit. Care 4, 302–308. doi:10.1186/cc712
Wang, K., and Hu, W. (2020). Oxypaeoniflorin improves myocardial ischemia/reperfusion injury by activating the sirt1/foxo1 signaling pathway. Acta Biochim. Pol. 67, 239–245. doi:10.18388/abp.2020_5206
Wang, T., Mao, X., Li, H., Qiao, S., Xu, A., Wang, J., et al. (2013). N-acetylcysteine and allopurinol up-regulated the jak/stat3 and pi3k/akt pathways via adiponectin and attenuated myocardial postischemic injury in diabetes. Free Radic. Biol. Med. 63, 291–303. doi:10.1016/j.freeradbiomed.2013.05.043
Wang, Y., Meng, C., Zhang, J., Wu, J., and Zhao, J. (2019). Inhibition of gsk-3β alleviates cerebral ischemia/reperfusion injury in rats by suppressing Nlrp3 inflammasome activation through autophagy. Int. Immunopharmacol. 68, 234–241. doi:10.1016/j.intimp.2018.12.042
Wang, Z. K., Chen, R. R., Li, J. H., Chen, J. Y., Li, W., Niu, X. L., et al. (2020). Puerarin protects against myocardial ischemia/reperfusion injury by inhibiting inflammation and the Nlrp3 inflammasome: The role of the sirt1/nf-κb pathway. Int. Immunopharmacol. 89, 107086. doi:10.1016/j.intimp.2020.107086
Wu, Y., Liu, H., and Wang, X. (2021). Cardioprotection of pharmacological postconditioning on myocardial ischemia/reperfusion injury. Life Sci. 264, 118628. doi:10.1016/j.lfs.2020.118628
Wu, Z. L., Davis, J. R. J., and Zhu, Y. (2020). Dexmedetomidine protects against myocardial ischemia/reperfusion injury by ameliorating oxidative stress and cell apoptosis through the trx1-dependent Akt pathway. Biomed. Res. Int. 2020, 8979270. doi:10.1155/2020/8979270
Xiong, W., Zhou, R., Qu, Y., Yang, Y., Wang, Z., Song, N., et al. (2021). Dexmedetomidine preconditioning mitigates myocardial ischemia/reperfusion injury via inhibition of mast cell degranulation. Biomed. Pharmacother. 141, 111853. doi:10.1016/j.biopha.2021.111853
Yan, B., Liu, S., Li, X., Zhong, Y., Tong, F., and Yang, S. (2019). Preconditioning with endoplasmic reticulum stress alleviated heart ischemia/reperfusion injury via modulating ire1/atf6/rack1/perk and pgc-1α in diabetes mellitus. Biomed. Pharmacother. 118, 109407. doi:10.1016/j.biopha.2019.109407
Yang, Y. F., Peng, K., Liu, H., Meng, X. W., Zhang, J. J., and Ji, F. H. (2017). Dexmedetomidine preconditioning for myocardial protection in ischaemia-reperfusion injury in rats by downregulation of the high mobility group box 1-toll-like receptor 4-nuclear factor κb signalling pathway. Clin. Exp. Pharmacol. Physiol. 44, 353–361. doi:10.1111/1440-1681.12711
Ye, L., Dai, B., Wu, Z., and Hu, Y. (2021). Dynamics of heart rate variability in patients with type 2 diabetes mellitus during spinal anesthesia using dexmedetomidine. Am. J. Transl. Res. 13, 5395–5403.
Yeda, X., Shaoqing, L., Yayi, H., Bo, Z., Huaxin, W., Hong, C., et al. (2017). Dexmedetomidine protects against renal ischemia and reperfusion injury by inhibiting the P38-mapk/txnip signaling activation in streptozotocin induced diabetic rats. Acta Cir. Bras. 32, 429–439. doi:10.1590/s0102-865020170060000003
Yellon, D. M., and Hausenloy, D. J. (2007). Myocardial reperfusion injury. N. Engl. J. Med. 357, 1121–1135. doi:10.1056/NEJMra071667
Yin, W., Wang, C., Peng, Y., Yuan, W., Zhang, Z., Liu, H., et al. (2020). Dexmedetomidine alleviates H(2)O(2)-induced oxidative stress and cell necroptosis through activating of α2-adrenoceptor in H9c2 cells. Mol. Biol. Rep. 47, 3629–3639. doi:10.1007/s11033-020-05456-w
Yin, X., Zheng, Y., Zhai, X., Zhao, X., and Cai, L. (2012). Diabetic inhibition of preconditioning- and postconditioning-mediated myocardial protection against ischemia/reperfusion injury. Exp. Diabetes Res. 2012, 198048. doi:10.1155/2012/198048
Yoshitomi, O., Cho, S., Hara, T., Shibata, I., Maekawa, T., Ureshino, H., et al. (2012). Direct protective effects of dexmedetomidine against myocardial ischemia-reperfusion injury in anesthetized pigs. Shock 38, 92–97. doi:10.1097/SHK.0b013e318254d3fb
Zhang, F., Ding, T., Yu, L., Zhong, Y., Dai, H., and Yan, M. (2012). Dexmedetomidine protects against oxygen-glucose deprivation-induced injury through the I2 imidazoline receptor-pi3k/akt pathway in rat C6 glioma cells. J. Pharm. Pharmacol. 64, 120–127. doi:10.1111/j.2042-7158.2011.01382.x
Zhang, J. J., Peng, K., Zhang, J., Meng, X. W., and Ji, F. H. (2017). Dexmedetomidine preconditioning may attenuate myocardial ischemia/reperfusion injury by down-regulating the hmgb1-tlr4-myd88-nf-κb signaling pathway. PLoS One 12, e0172006. doi:10.1371/journal.pone.0172006
Zhang, X., Xu, M., Che, X., Cao, X., and Li, X. (2020a). Dexmedetomidine reduces myocardial ischemia-reperfusion injury in rats through pi3k/akt/gsk-3β signaling pathway. Minerva Cardioangiol. 68, 58–59. doi:10.23736/s0026-4725.19.05102-8
Zhang, X., Li, Y., Wang, Y., Zhuang, Y., Ren, X., Yang, K., et al. (2020b). Dexmedetomidine postconditioning suppresses myocardial ischemia/reperfusion injury by activating the sirt1/mtor Axis. Biosci. Rep. 40, BSR20194030. doi:10.1042/bsr20194030
Zhang, X., Zhou, J., Hu, Q., Liu, Z., Chen, Q., Wang, W., et al. (2020c). The role of janus kinase/signal transducer and activator of transcription signalling on preventing intestinal ischemia/reperfusion injury with dexmedetomidine. J. Nanosci. Nanotechnol. 20, 3295–3302. doi:10.1166/jnn.2020.16416
Zhang, M. L., Peng, W., Ni, J. Q., and Chen, G. (2021). Recent advances in the protective role of hydrogen sulfide in myocardial ischemia/reperfusion injury: A narrative review. Med. Gas. Res. 11, 83–87. doi:10.4103/2045-9912.311499
Zhang, Y., Zhao, Q., Li, X., and Ji, F. (2021). Dexmedetomidine reversed hypoxia/reoxygenation injury-induced oxidative stress and endoplasmic reticulum stress-dependent apoptosis of cardiomyocytes via sirt1/chop signaling pathway. Mol. Cell. Biochem. 476, 2803–2812. doi:10.1007/s11010-021-04102-8
Zhao, D., Yang, J., and Yang, L. (2017). Insights for oxidative stress and mtor signaling in myocardial ischemia/reperfusion injury under diabetes. Oxid. Med. Cell. Longev. 2017, 6437467. doi:10.1155/2017/6437467
Zi, S. F., Li, J. H., Liu, L., Deng, C., Ao, X., Chen, D. D., et al. (2019). Dexmedetomidine-mediated protection against septic liver injury depends on tlr4/myd88/nf-κb signaling downregulation partly via cholinergic anti-inflammatory mechanisms. Int. Immunopharmacol. 76, 105898. doi:10.1016/j.intimp.2019.105898
Keywords: cardioprotection, ischemia-reperfusion, oxidative stress, autophagy, inflammation, apoptosis, dexmedetomidine
Citation: Sun M, Wang R, Xia R, Xia Z, Wu Z and Wang T (2022) Amelioration of myocardial ischemia/reperfusion injury in diabetes: A narrative review of the mechanisms and clinical applications of dexmedetomidine. Front. Pharmacol. 13:949754. doi: 10.3389/fphar.2022.949754
Received: 23 May 2022; Accepted: 08 August 2022;
Published: 31 August 2022.
Edited by:
Keliang Xie, Tianjin Medical University, ChinaReviewed by:
Bin Yi, Army Medical University, ChinaGuiling Zhao, University of Maryland, United States
Copyright © 2022 Sun, Wang, Xia, Xia, Wu and Wang. This is an open-access article distributed under the terms of the Creative Commons Attribution License (CC BY). The use, distribution or reproduction in other forums is permitted, provided the original author(s) and the copyright owner(s) are credited and that the original publication in this journal is cited, in accordance with accepted academic practice. No use, distribution or reproduction is permitted which does not comply with these terms.
*Correspondence: Zhilin Wu, 840916@qq.com; Tingting Wang, wangtt201307@163.com
†These authors have contributed equally to this work