Corrigendum: Radical scavenging capacity, antibacterial activity, and quantum chemical aspects of the spectrophotometrically investigated iridium (III) complex with benzopyran derivative
- 1Department of Chemistry, Maharishi Markandeshwar (Deemed to Be University), Mullana, Ambala, India
- 2Department of Biotechnology, Maharishi Markandeshwar (Deemed to Be University), Mullana, Ambala, India
- 3Department of Chemistry, College of Sciences, King Saud University, Riyadh, Saudi Arabia
- 4Department of Chemistry Kurukshetra University, Kurukshetra, India
- 5Department of Chemistry, MCM DAV College, Kangra, Himachal Pradesh, India
- 6Department of Safety Engineering, Dongguk University, Gyeongju, South Korea
- 7Department of Physics, Kazan Federal University, Kazan, Russia
A comprehensive aqueous phase spectrophotometric study concerning the trace level determination of iridium (III) by its reaction with benzopyran-derived chromogenic reagent, 6-chloro-3-hydroxy-7-methyl-2-(2′-thienyl)-4-oxo-4H-1-benzopyran (CHMTB), is performed. The complexing reagent instantly forms a yellow complex with Ir (III) at pH 4.63, where metal is bound to the ligand in a ratio of 1:2 as deduced by Job’s continuous variations, mole ratio, and equilibrium shift methods. The complex absorbs maximally at 413–420 nm retaining its stability for up to 4 days. An optimum set of conditions have been set with respect to the parameters governing the formation of the complex. Under the set optimal conditions, the Ir (III)-CHMTB complex coheres to Beer’s law between 0.0 and 1.5 µg Ir (III) mL−1. The attenuation coefficient and Sandell’s sensitivity are, respectively, 1.18×105 L mol−1 cm−1 and 0.00162 μg cm−2 at 415 nm. The correlation coefficient (r) and standard deviation (SD) were 0.9999 and ± 0.001095, respectively, whereas the detection limit as analyzed was 0.007437 μg ml−1. The interference with respect to analytically important cations and complexing agents has been studied thoroughly. It is found that the majority of the ions/agents do not intervene with the formation of the complex, thus adding to the versatility of the method. The results obtained from the aforesaid studies indicate a simple, fast, convenient, sensitive, and versatile method for microgram analysis of iridium (III) using CHMTB as a binding ligand. Furthermore, the studied complex is subjected to the evaluation of antibacterial and antioxidant capacity by employing the Agar Diffusion assay and DPPH. radical scavenging method, respectively. The results obtained from the mentioned assays reveal that the investigated complex possesses significant potency as an antibacterial and antioxidant agent. Finally, the computational approach through DFT of the formed complex confirmed the associated electronic properties of the studied complex.
Introduction
Owing to the multiplex electronic and stearic properties and, therefore, aptness to undergo ligand exchange with biomolecules, group 9 transition metals and their complexes have attracted considerable attention from inorganic chemists and are thus widely explored (Lu et al., 2015). Iridium is one of the most important rare elements found in the earth’s crust and normally exists in uncombined form or natural alloys such as osmiridium (osmium rich) and iridosmine (iridium rich). Surprisingly, despite its lower reactivity, iridium forms a plethora of compounds with oxidation states ranging from −3 to +9. Iridium, in addition to its unique electronic arrangement, has minimal toxicity and environmental impact, making it a green element. Due to their unique characteristics, iridium and its complexes have a wide range of applications, including industrial, medical, and catalysis (Singh, 2016). Besides this, iridium and its complexes are used in electrical and electrochemical operations, in energy production, as anticancer agents, and for the fixation and conversion of atmospheric carbon dioxide into useful products (Singh, 2016).
Thus, there is an increasing demand for the development of analytical procedures, preferably simple, sensitive, and selective ones to broaden the horizon of the applicability of iridium and its complexes. Sophisticated techniques such as NAA (Miura et al., 2020), spectrofluorimetric (Druskovic et al., 2004), AAS (Ingo et al., 2008), voltammetry (Locatelli, 2013), atomic emission and flame emission spectrometry (Jackson and Qiao, 1992), ICP-OES (Zhang and Tian, 2015), ICP-MS (Yi and Masuda, 1996), sequential flow injection analysis (Khomutova et al., 2013), imprinted polymer-based method (Sid Kalal et al., 2013), and individual catalytic method (Kawamura et al., 2011) have been employed for the purpose. However, the wider application of these techniques is restricted by several factors such as instrumentation costs, technical know-how, and maintenance issues. In order to combat these flaws, a wide range of versatile spectrophotometric methods have been used for the trace level determination of iridium in its complexes (Druskovic and Vojkovic, 2003; Li-Ming et al., 2008; Kuchekar et al., 2014; Borgave and Barhate, 2016; Kuchekar et al., 2019). To date, many spectrophotometric methods, both extractive and non-extractive, have been proposed to study iridium complexes; the aqueous phase non-extractive study, however, is more effective than the extractive ones as the latter involve cumbersome procedures.
The current method elucidates the non-extractive aqueous phase spectrophotometric determination of Ir (III) as its coordination complex with 6-chloro-3-hydroxy-7-methyl-2-(2′-thienyl)-4-oxo-4H-1-benzopyran (CHMTB). A keen literature survey reveals that CHMTB, one of the benzopyran derivatives, has so far not been used for the analytical determination of Ir (III). Furthermore, because the suggested approach does not use solvent extraction, the use of organic solvents that have been known to be harmful, polluting, and carcinogenic are avoided, keeping in view the therapeutic properties of iridium complexes (Mukherjee et al., 2014; Lapasam et al., 2020; Mohmad et al., 2022). Ir (III)-CHMTB was investigated for antibacterial and antioxidant properties by following the standard protocols and was found to exhibit the same. Moreover, the complex was found to be a more potent antibacterial and antioxidant agent, as was inferred from MIC and IC50 values, respectively. Insight and comprehension into the electronic structural details and chemical reactivity of the complex were accomplished using DFT, which further reinforced the basis of structural properties of the studied complex. This conforms with the computational studies as already been performed for the analogous metal complexes (Kataria et al., 2017; Dhonchak et al., 2021; Mohmad et al., 2022).
Experimental
Instrumentation
All the absorbance measurements were performed using UV-Vis spectrophotometer (double beam; EI-2375) with 1 cm identical quartz cuvettes. Highly precise electronic weighing balance (EI-101) and sensitive pH meter (alpha-01) with combination electrodes were used, respectively, for quantifying weights and pH observations. An exhaustive contamination control was followed while carrying out the experiments. The calibrated glassware used during the experiments was cleaned by soaking it in dilute HCl for 12 h followed by rinsing with double-distilled water.
Reagents and solutions
High purity chemicals of AR grade and deionized water were used during the test. A 100 ml of the stock solution of iridium with a strength of 1 mg Ir (III) mL−1 was prepared by dissolving a precisely weighed amount (0.155 g) of iridium trichloride (IrCl3) in 6M HCl. Working solutions of the required concentration (100 and 10 μg ml−1) were prepared by appropriate dilution of the stock solution with doubly distilled water. For the regulation of the ambient pH of the reaction medium, 0.5 M H3PO4 was prepared.
Ligand solution
The chromogenic reagent, CHMTB, containing two functional sites, the carbonyl group and the hydroxyl group (Figure 1), was synthesized by applying the methodology as followed in the Algar–Flynn–Oyamada (AFO) reaction (Algar and Flynn, 1934; Oyamada, 1934). 0.1% (w/v) solution of CHMTB was made by dissolving 0.025 g of it in a small amount of ethanol, followed by dilution with the same solvent up to the mark in a 25 ml graduated flask.
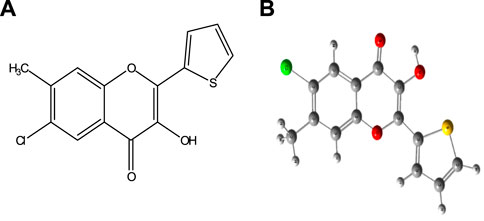
FIGURE 1. Structure of 6-chloro-3-hydroxy-7-methyl-2-(2′-thienyl)-4-oxo-4H-1-benzopyran (CHMTB): (A) 2D structure and (B) optimized structure.
Other required solutions
In order to study the diverse effects caused by most of the analytically important cations and complexing agents, required solutions were prepared from the salts by dissolving in deionized water or the mineral acids.
Suggested procedure for determination
In order to achieve accurate and flawless results, the spectrophotometric inspection was carried out in the most optimized manner. By diluting the stock solution with appropriate amounts of double-deionized water, a working solution (10 μg ml−1) of the metal ion under study was prepared for analysis. For the process of complexation, 1 ml of the working solution was pipetted out in a 10 ml standard volumetric flask, 1 ml of the 0.1% reagent CHMTB solution was added to it to carry out complexation in the presence of 0.3 ml of 0.5 M H3PO4, thereby, adjusting the ambient pH 4.63 of the reaction medium. The remaining volume was made by adding double-distilled water up to the mark. After adding 1 ml of ethanolic solution of 0.1% (w/v) CHMTB, a yellow-colored complex was formed instantaneously. The optical density of the resulting complex at 415 nm was compared to that of a similarly treated reagent blank for absorbance studies. The precise amount of metal ion was calculated using the calibration curve constructed between absorbance and varying iridium concentrations (III).
Computational details
Quantum chemistry (DFT) computations producing molecular configurations of the minimum energies molecular orbitals (HOMO-LUMO) and other properties were performed using the Gaussian 09 (Revision B.04) package. The geometry optimization was done when the system was gaseous. Gauss View 05 program was used to view the optimized molecular structures, including the HOMO and LUMO surfaces (Frisch et al., 2000). The Lee–Yang–Parr expression’s nonlocal correlation; the Vosko–Wilk–Nuair (1980) local correlation functional (III) (B3LYP); and Becke’s three-parameter hybrid exchange functional were all used (Becke, 1993). For carbon and oxygen, the basis set 6-31G (d, p) was used. During the theoretical analysis, the LANL2DZ basis set (Patersson and Al-Latham, 1991) and Hay and Wadt pseudo potentials (Hay and Wadt, 1985; Wadt and Hay, 1985) were employed. The theoretical studies for various quantum chemical parameters were hence carried out employing the Gaussian 09 (Revision B.04) software as previously reported (Dhonchak et al., 2021; Mohmad et al., 2022).
In vitro antibacterial activity
By considering the clinical importance of bacterial pathogens, four bacterial strains were selected to check antibacterial potential of the iridium (III) complex. All the bacterial strains were procured from the Microbial Type Culture Collection (MTCC), Institute of Microbial Technology (IMTECH), Chandigarh, India. The selected strains included two Gram-positive [Staphylococcus aureus (MTCC-96) and Bacillus subtilis (MTCC-121)] and two Gram-negative [Escherichia coli (MTCC 1652) and Pseudomonas aeruginosa (MTCC 741)] bacteria. These strains were further preserved on the slants of Nutrient Agar. Antimicrobial activity has been recorded in the form of the zone of inhibition after the completion of the incubation period.
Agar well diffusion Method
The antibacterial potentials of the Ir (III)-CHMTB complex were detected by the agar well diffusion method (Kamal et al., 2015; Kaur et al., 2016; Kamal et al., 2019). The suspensions of selected bacteria were mixed in sterile saline (0.9% NaCl) by taking 16 h preserved cultures and adjusting to 1.5 × 108 cfu ml−1 by referring to the 0.5 McFarland standards. 15–20 ml of Nutrient Agar medium was poured into the Petri plate, which was then swabbed with 100 µL inocula of the bacterial culture and left for 15 min to allow for adsorption over the media. The agar wells with a diameter of 8 mm were created into the seeded agar plates with a sterile cork-borer and loaded with a 100 µL volume of the metal complex with a concentration of 5 mg ml−1. All the plates were incubated at 37°C for 24 h to promote bacterial growth. The antibacterial activity of the complex has been evaluated using a zone reader ((Hi Antibiotic Zone Scale)) to measure the zone of growth inhibition (including the well diameter) against the test organisms. A broad-spectrum antibiotic, ciprofloxacin (5 mg ml−1), was used as a positive control for bacteria growth inhibition. The complex showing significant antibacterial potential was selected further for minimum inhibitory concentration (MIC) studies.
Determination of minimum inhibitory concentration of Ir (III)-CHMTB complex
MIC is the lowest concentration of an antimicrobial compound that will inhibit the visible growth of a microorganism after overnight incubation. MIC of the Ir (III)-CHMTB complex against bacterial strains has been tested using a modified agar well diffusion method as mentioned above (Kamal et al., 2015; Kaur et al., 2016; Kamal et al., 2019; Mohmad et al., 2022). In this method, twofold serial dilutions of the complex were prepared by dissolving the complex in dimethylsulfoxide (DMSO) to achieve decreasing concentration ranges of 250–3.91 µg/100 µL. A 100 µL volume of each dilution was introduced into wells (in triplicate) in the agar plates already seeded with 100 µL of standardized inoculum (106 cfu ml−1) of the bacterial strains. All seeded plates were incubated aerobically at 37°C and observed for the inhibition zones after 24 h. MIC, taken as the lowest concentration of the complex that completely inhibited the growth of the microbe, was indicated in the form of a clear zone of inhibition. It was recorded for the complex against each bacterial strain. Ciprofloxacin was used as a positive control, whereas DMSO was used as a negative control.
Antioxidant activity in vitro
The antioxidant property of complex and the ligand was studied by Radical Scavenging Activity (RSA) toward DPPH, and the reactions were observed by employing a UV-Vis spectrophotometer (Tabrizi et al., 2019; Lewandowski et al., 2020; Lapasam et al., 2021).
Free radical scavenging of the compounds using 2,2-diphenyl-1-picrylhydrazyl analysis
DPPH radical scavenging is the most regularly applied assay to the evaluation of the antioxidant activity of chemical compounds. The DPPH (methanolic solution) proffers vivid purple color giving an intense absorption band at 517 nm; the color of the solution changes from deep purple to yellow on interaction with the compound exhibiting antioxidant potential. The DPPH free radical scavenging mechanism resides in the fact that a methanolic DPPH solution is reduced in the presence of an antioxidant. The stock solution of DPPH with a concentration 1.0 mM was prepared by precisely weighing 0.0394 g of DPPH and dissolving the same in 100 ml of methanol. A total of 1,000 μg ml−1 stock solution of the complex under study was prepared by dissolving 5 mg of the complex in 5 ml of methanol. The solution acting as a negative control was prepared by taking 5 ml of the DPPH solution in a 25 ml volumetric flask from its stock solution and making up the volume with methanol. A varied range of concentrations (500 μg, 250 μg, 125 μµg, 62.5, and 31.25 μg ml−1) of Ir (III)-CHMTB complex were prepared from the stock solution. In an attempt to juxtapose the antioxidant activity of the complex and the ligand (CHMTB), lower dilutions of CHMTB of the same concentrations as those of Ir (III)-CHMTB complex were prepared concomitantly. This was followed by incubating the solutions at 37°C for 30 min in darkness. The same concentrations of the positive control, gallic acid, were prepared simultaneously. Finally, the absorbance of each solution was spectrophotometrically determined at 517 nm against the negative control, and the % free radical scavenging was calculated as per the following equation:
% Free radical scavenging = [(Anegativecontrol-Asample)/Acontrol] ×100,where Anegative control denotes the absorbance of negative control (which contains all reagents except for the test compounds) and Asample denotes absorbance of the antioxidant compounds (gallic acid, Ir (III)-CHMTB, CHMTB).
Results and discussion
Spectrophotometric studies
Absorption spectrum
At a pH of 3.27–4.89, iridium (III) reacted with CHMTB to give an appreciably stable (4 days) yellow-colored compound. The maximum color intensity as exhibited by the complex was in the wavelength range of 413–420 nm when studied against a similarly treated reagent blank, manifesting negligible absorbance in the same visible region (Figure 2). Therefore, all the absorbance measurements were performed at 415 nm.
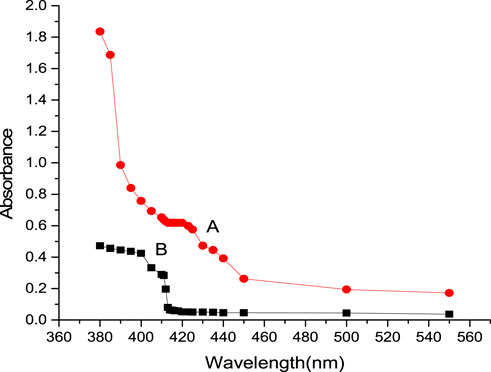
FIGURE 2. Absorption spectra of Ir (III)-CHMTB complex: spectrum A represents the absorbance of complex against reagent blank; spectrum B represents the absorbance of reagent blank against solvent (pure water).
Optimum conditions for Ir (III)-CHMTB complex
None of the solvents, polar or nonpolar, including benzene, toluene, ethyl acetate, cyclohexane, chloroform, methyl isobutyl ketone, isoamyl alcohol, carbon tetrachloride, and dichloromethane, was found effective while evaluating the extraction behavior of Ir (III)-CHMTB complex. In contrast, the complexation tendency of CHMTB under the acidic condition with Ir (III) noticeably attained a maximum in the aqueous phase only and hence was the choice for further investigation.
The formation of the complex was explored in acidic (H2SO4, HCl, HClO4, H3PO4, and CH3COOH) and basic media (NaHCO3, Na2CO3, and NaOH), resulting in maximum and stable absorbance in the phosphoric acid medium as is shown Figure 3. Highly turbid solutions, however, were obtained while using Na2CO3 and NaOH as the reaction media.
While studying absorbance diversification of the complex with changing the concentration of H3PO4, the optical density was observed to be highest at 0.005–0.03M H3PO4 within the pH range of 3.27–4.89 (Table 1); hence, it was selected as the ideal condition for complexation. Complete complexation was further confirmed by variable addition of the ligand, CHMTB solution to the reaction mixture, and being maximum and constant with 0.8–1.2 ml of its ethanol solution (Table 1); thus, it was fixed as the optimum condition for Ir (III)-CHMTB complex.
Interference studies
To evaluate the tolerance level and selectivity of the Ir (III)-CHMTB system, the impact of analytically important anions or complexing agents and cations was explored (Table 2 and Table 3). Under the ideal set of conditions for the system, the addition of foreign ions was done to Ir (III) (10 µg in 10 ml aqueous volume) initially in pretty large amounts, followed by the addition of small amounts in cases where appreciable interference was observed. None of the complexing agents/anions was found to interfere. However, among 32 selected cations, V(V) showed interference even in trace levels, enhancing the color intensity to a much higher value but was made ineffective in the presence of ascorbic acid acting as the masking agent.
Optical and statistical parameters
Under the ambient conditions of the studied system, an illustrious equation for spectrophotometric analysis was obtained from Beer’s law. Ir (III)-CHMTB complex adhered to a linear response between 0.0 and 1.5 µg Ir (III) mL−1 (Figure 4), whereas Ringbom’s plot (Ringbom, 1938) exhibited an optimum range of determination as 0.1725–1.4976 ppm of Ir (III) (Figure 5). The molar attenuation coefficient was enormously high amongst the binary complexes studied so far of the metal with a value of 1.188×105 L mol−1 cm−1 with Sandell’s sensitivity of 0.00162 μg cm−2. The linear relationship between variables of the equation of straight line of y = 0.6158 x + 0.0036, absorbance (dependent), and concentration (independent), respectively, is revealed by the coefficient of the determination of the complex with a value of 0.9999. The various optical and statistical parameters are shown in Table 4.
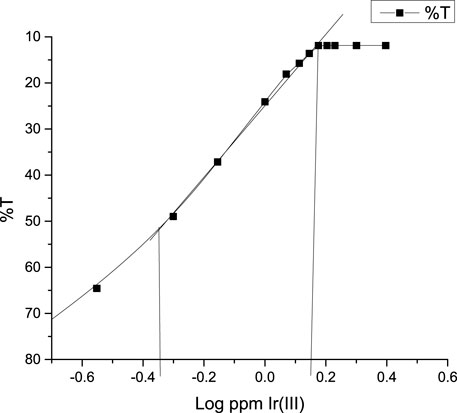
FIGURE 5. Optimum determination range (Ringbom’s range) of the complex at a wavelength of 415 nm Ir (III)-CHMTB complex.
Composition of the absorbing species
For ascertaining the metal-to-ligand ratio and hence the geometry of the formed Ir (III)-CHMTB coordination compound, Job’s continuous variations (Job, 1928; Vosburgh and Cooper, 1941), mole Ratio (Yoe and Jones, 1944), and equilibrium shift methods (Tarasiewicz et al., 1977) were employed (Figure 6). All of these methods deduced and confirmed a metal-to-ligand 1:2 stoichiometry in the studied compound. The slope of 2.12 as obtained from the equilibrium shift method confirms 1:2 M:L composition of the Ir (III)-CHMTB complex.
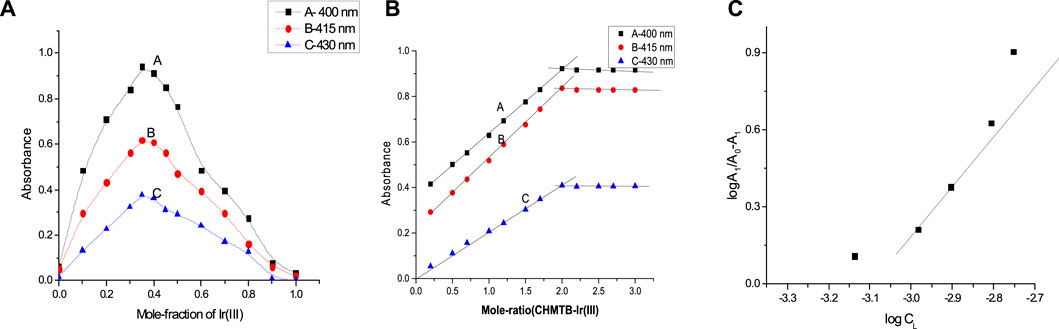
FIGURE 6. Determination of the composition of the complex (A) Job’s continuous variations method, (B) mole-ratio method, and (C) equilibrium shift method.
The experimentation done above led to the following (Figure 7) proposed structure of the studied complex along with its optimized form.
The geometrical properties of the optimized octahedral complex as evaluated by the B3LYP/6-31G (d, p) method are shown in Table 5.
Frontier molecular orbital analysis and quantum chemical molecular descriptors
Herein, Egap (HOMO
Figure 8 shows the HOMO/LUMO representations of the ligand, CHMTB, and its metal ion complex. The figure clearly denotes that the highest occupied HOMO and lowest unoccupied LUMO are located over the entire molecule of ligand. However, both of them for the complex are mainly contributed by the ligand.
Analytical, antibacterial, and antioxidant applications
Analytical applications
In order to check the versatility and applicability of the method, it was employed in a large number of synthetic mixtures of varying compositions with highly reproducible and satisfactory results, as is indicated from Table 7, by the values of Ir (III) found in various samples, a result of the triplicate analysis. Additionally, the applicability of the method is strengthened by the adequate analysis of iridium in two of its most important alloys, namely, iridosmine and osmiridium (Table 7).
Evaluation of in vitro antibacterial activity
As discussed in the methodology, two Gram-positive [Bacillus subtilis (MTCC-121) and Staphylococcus aureus (MTCC-96)] and Two Gram-negative [Escherichia coli (MTCC 1652) and Pseudomonas aeruginosa (MTCC 741)] bacterial strains were selected to check the antibacterial potential of the newly synthesized Ir (III)-CHMTB complex by determining their MICs. The tested complex showed a highly significant zone of growth inhibition against all the bacterial strains, as mentioned in Table 8 and Figure 9. It inhibited the bacterial cultures in the range of 42–48 mm (zone of inhibition). Ciprofloxacin has been used as the positive control to compare the results. Ciprofloxacin showed a zone of inhibition in the range of 33–35 mm, which was less in comparison to the tested complex.
To further compare the antibacterial activities of the complex with the standard antibiotic, the MIC values of both were compared as depicted in Table 9 and Figure 10. The antibacterial effects against the tested strains were found more significant for the complex, which showed MIC values up to 3.91 μg/100 μL. As compared to the complex, ciprofloxacin showed MIC values in the range of 7.81–15.62 μg/100 μL. It is noteworthy that the Ir (III)-CHMTB complex was highly active against the tested bacterial strains, and also, the complex showed activity higher than that exhibited by ciprofloxacin.
DPPH radical scavenging activity
The free radicals generated by various metabolic processes or UV radiations cause the deterioration of DNA, proteins, and lipids. Under such circumstances, the administration of antioxidants from outside play a vital role for the protection of biological systems. Antioxidants work by scavenging free radicals and inhibiting chain reactions by giving the free radical an electron or hydrogen. As a result, there has been a surge in interest in creating new, safer antioxidants with fewer adverse effects.
The antioxidant activity at various concentrations of CHMTB and Ir (III) complex was determined by measuring the de-colorization of DPPH.. The RSA increased with the increase in the concentration of the compounds. CHMTB and Ir (III)-CHMTB complex exhibited the highest scavenging activity of 56.1% and 68.06%, respectively, at 500 μg ml−1 concentration. Under experimental conditions, IC50 of Ir (III) complex is 62.5 μg ml−1. The RSA can be compared in the following order: gallic acid > Ir (III)-CHMTB complex > CHMTB (Table 10; Figure 11, Figure 12). Thus, the Ir (III)-CHMTB complex displays greater scavenging activity than the free ligand and, hence, can be used as an effective antioxidant.
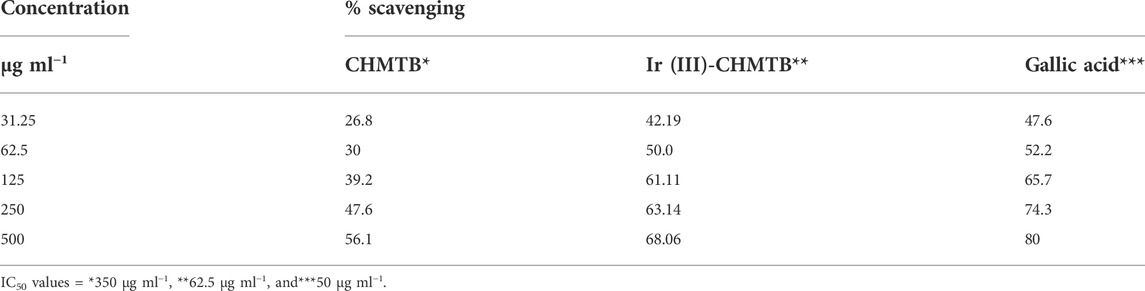
TABLE 10. Antioxidant activity of the studied ligand and its complex in terms of % scavenging activity.
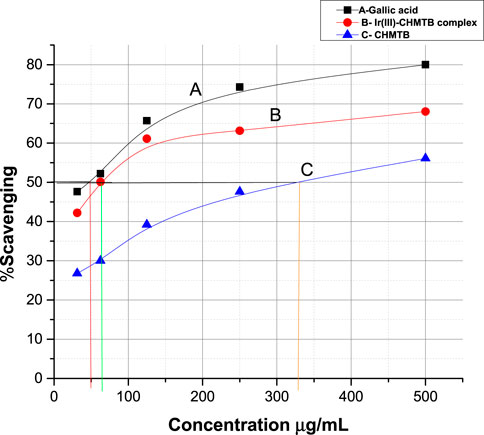
FIGURE 11. Percent radical scavenging activity of (A) gallic acid, (B) Ir (III)-CHMTB complex, and (C) CHMTB.
Conclusion
In summary, in the presented work, for the first time, CHMTB was utilized successfully for the microgram level analysis of iridium (III) employing the spectrophotometric determination technique. Iridium (III) instantaneously reacts with CHMTB in the phosphoric acid medium at an ambient pH of 3.73 to form a stable yellow-colored non-extractable complex cohering to a linear response up to 1.5 µg Ir (III) mL−1. The accuracy and reproducibility of the method are confirmed by the corresponding values of relative standard deviation (RSD) and the coefficient of variance as 0.1774% and 0.9999, respectively. The stoichiometric constitution of the developed complex, as validated by the studied methods, was 1:2 (M:L). Recently, several binary complexes of Ir (III) have been investigated and studied spectrophotometrically. However, in addition to being simple, versatile, and economic, the current method is searched to be highly sensitive in comparison to the previously studied binary complexes of Ir (III) (Kuchekar et al., 2014; Borgave and Barhate, 2016; Kuchekar et al., 2019) as can be inferred from the molar attenuation coefficient of 1.188×105 L mol−1 cm−1. DFT studies were employed to get know-how about structural details and electronic properties of the complex. The DFT simulations not only revealed an energy-optimized structure for CHMTB and its complex with Ir (III), but also confirmed the ligand and complex to have significant nucleophilic and electrophilic properties, respectively. By considering the therapeutic applications of iridium and its complexes, the Ir (III)-CHMTB complex was screened for antioxidant and antibacterial activities following the standard protocols of determination of the same. Successful elucidation of the antioxidant and antibacterial activities made the studied complex serve as a limelight for the formation of antioxidant agents and bactericidal drugs, a novel addition to the iridium-based therapeutic agents. Additionally, with reference to responsiveness, speed, and versatility, the current study aptly contemplates the already reported methods (Druskovic and Vojkovic, 2003; Li-Ming et al., 2008; Kuchekar et al., 2014; Borgave and Barhate, 2016; Kuchekar et al., 2019; Mohmad et al., 2022).
Data availability statement
The original contributions presented in the study are included in the article/Supplementary Material. Further inquiries can be directed to the corresponding authors.
Author contributions
All authors listed have made a substantial, direct, and intellectual contribution to the work and approved it for publication.
Acknowledgments
Sincere thanks go to the authorities, Maharishi Markandeshwar (Deemed to be University), for providing the required facilities to carry out the presented work. The authors are grateful to the Researchers Supporting Project Number (RSP2022R448), King Saud University, Riyadh, Saudi Arabia.
Conflict of interest
The authors declare that the research was conducted in the absence of any commercial or financial relationships that could be construed as a potential conflict of interest.
Publisher’s note
All claims expressed in this article are solely those of the authors and do not necessarily represent those of their affiliated organizations or those of the publisher, the editors, and the reviewers. Any product that may be evaluated in this article, or claim that may be made by its manufacturer, is not guaranteed or endorsed by the publisher.
References
Algar, J., and Flynn, J. P. (1934). A new method for the synthesis of flavones. Proc. R. Ir. Acad. 42B, 1–8.
Becke, A. D. (1993). Density-functional thermochemistry III.The role of exact exchange. J. Chem. Phys. 98, 5648–5652. doi:10.1063/1.464913
Borgave, S. S., and Barhate, V. D. (2016). Extractive and spectrophotometric determination of Iridium (III) using 2-(5-bromo-2-oxoindolin-3-ylidene) hydrazinecarbothioamide as an analytical reagent. J. Chem. Pharm. Res. 8, 584.
Dhonchak, C., Agnihotri, N., and Kumar, A. (2021). Zirconium (IV)-3-hydroxy-2-tolyl-4H-chromen-4-one complex- the analytical and DFT studies. J. Mol. Model. 27, 336. doi:10.1007/s00894-021-04949-0
Druskovic, V., Vojkovic, V., and Miko, S. (2004). Spectrofluorimetric determination of iridium (IV) traces using 4-pyridone derivatives. Talanta 62, 489–495. doi:10.1016/j.talanta.2003.08.031
Druskovic, V., and Vojkovic, V. (2003). Spectrophotometric determination of micro amounts of iridium (IV) with 3-hydroxy- 2-methyl-1-phenyl-4-pyridone or 3-Hydroxy-2-methyl-1-(4-tolyl)-4-pyridone. Croat. Chem. Acta. 76, 49–54.
Frisch, A., Nielson, A. B., and Holder, A. J. (2000). GAUSSVIEW user manual. Pittsburgh.PA: Gaussian Inc.
Geerlings, P., De Proft, F., and Langenaeker, W. (2003). Conceptual density functional theory. Chem. Rev. 103, 1793–1873. doi:10.1021/cr990029p
Hay, P. J., and Wadt, W. R. (1985). Ab initio effective core potentials for molecular calculations. Potentials for the transition metal atoms Sc to Hg. J. Chem. Phys. 82, 270–283. doi:10.1063/1.448799
Ingo, O., Michael, S., Heike, S., William, S. S., and Gust, R. (2008). Atomic absorption spectrometric determination of iridium content in tumor cells exposed to an iridium metallodrug. J. Pharm. Biomed. Anal. 47, 938.
Jackson, K. W., and Qiao, H. (1992). Atomic absorption, atomic emission and flame emission spectrometry. Anal. Chem. 64, 252R–279R. doi:10.1021/ac00084a012
Kamal, R., Kumar, R., Kumar, V., Kumar, V., Bansal, K. K., and Sharma, P. C. (2019). Synthesis, anthelmintic and antimicrobial evaluation of new 2-arylidene-1-(4-methyl-6-phenylpyrimidin-2-yl) hydrazines. ChemistrySelect 4, 713–717. doi:10.1002/slct.201802822
Kamal, R., Kumar, V., Bhardwaj, V., and Aneja, K. R. (2015). Synthesis, characterization and in vitro antimicrobial evaluation of some novel hydrazone derivatives bearing pyrimidinyl and pyrazolyl moieties as a promising heterocycles. Med. Chem. Res. 24, 2551–2560. doi:10.1007/s0044-014-1313-5
Kataria, R., Patra, R., Sharma, H. K., Singh, G., Kumar, G., and Sharma, S. K. (2017). Spectrophotometric determination of Tin (II) with 6-Chloro-2-(2’furyl)-3-Hydroxy-7-Methyl-4-oxo-4H-1-Benzopyran and its solution state structure study by DFT. Sens. Lett. 15, 25–31. doi:10.1166/sl.2017.3761
Kaur, K., Kumar, K., Beniwal, V., Kumar, V., Kumar, N., Sharma, V., et al. (2016). Synthesis of some novel oxazolidinone-thiazole hybrids as potential antimicrobial, antioxidant and UV mediated DNA damage protecting agents. Med. Chem. Res. 25, 2237–2249. doi:10.1007/s00044-016-1663-2
Kawamura, K., Ikoma, K., Igarashi, S., Hisamoto, H., and Yao, T. (2011). Flow injection analysis combined with a hydrothermal flow reactor: Application to kinetic determination of trace amounts of iridium using a water-soluble porphyrin. Talanta 84, 1318–1322. doi:10.1016/j.talanta.2011.02.038
Khomutova, E. G., Zagorodnikova, V. A., Zagorodnikova, S. A., and Ostanina, O. I. (2013). Individual catalytic determination of iridium and rhodium using the oxidation of sulfarsazene by potassium periodate as an indicator reaction in a flow-injection system. J. Anal. Chem. 68, 611–615. doi:10.1134/S1061934813070058
Kuchekar, S. R., Bhumkar, S., Aher, H., and Han, S. H. (2019). Spectrophotometric determination of Iridium (III) using p-methyl phenyl thiourea as a chelating agent: Sequential Separation of Iridium (III), Ruthenium (III) and Platinum (IV). J. Mat. Environ. 10, 1200.
Kuchekar, S. R., Pulate, S. D., Shelar, Y. S., and Han, S. H. (2014). Spectrophotometric study of interaction of o-methyl phenyl thiourea with iridium (III) and development of a precise determination method from hydrochloric acid media. Indian J. Chem. Tech. 21, 120.
Lapasam, A., Mawnai, I. L., Banothu, B., Kaminsky, W., and Kolliparaa, M. R. (2020). Ruthenium, rhodium and iridium complexes containing pyrimidine based thienyl pyrazoles: Synthesis and antibacterial studies. J. Organomet. Chem. 911, 121155. doi:10.1016/j.jorganchem.2020.121155
Lapasam, A., Shadap, L., Tripathi, D. K., Poluri, K. M., Kaminsky, W., and Kollipara, M. R. (2021). Arene ruthenium, rhodium and iridium complexes containing n?o chelating ligands: Synthesis antibacterial and antioxidant studies. J. Coord. Chem. 74, 2365–2379. doi:10.1080/00958972.2021.1963439
Lewandowski, W., Lewandowska, H., Golonko, A., Świderski, G., Świsłocka, R., and Kalinowska, M. (2020). Correlations between molecular structure and biological activity in "logical series" of dietary chromone derivatives. PLoS One 15, e0229477. doi:10.1371/journal.pone.0229477
Li-Ming, H. E., Zhi-xian, S. U., Hong-fu, W., Su-lan, Z., and Yi, Y. (2008). Catalytic kinetic spectrophotometric determination of trace iridium (IV) in potassium periodate-xylenecyanol FF system. Metall. Anal. 28, 47.
Locatelli, C. (2013). Iridium and lead as vehicle emission pollutants: Their sequential voltammetric determination in vegetable environmental bio-monitors. Microchem. J. 106, 282–288. doi:10.1016/j.microc.2012.08.008
Lu, L., Liu, L., Chao, W., Zhong, H., Wang, M., Chen, X., et al. (2015). Identification of an iridium(III) complex with anti-bacterial and anti-cancer activity. Sci. Rep. 5, 14544. doi:10.1038/srep14544
Miura, T., Linuma, Y., and Sekimoto, S. (2020). Precise determination of iridium by neutron activation analysis coupled with internal standard method. J. Radioanal. Nucl. Chem. 324, 1007–1012. doi:10.1007/s10967-020-07152-2
Mohmad, M., Agnihotri, N., Kumar, V., Kumar, R., and Kaviani, S. (2022). Iridium (III)-3-hydroxy-2-(3’-methyl-2’-thienyl)-4-oxo-4H-1-benzopyran complex: The analytical, in-vitro antibacterial and DFT studies. Inorg. Chem. Commun. 139, 109333. doi:10.1016/j.inoche.2022.109333
Mukherjee, T., Manjira, M., Sen, B., Banerjee, S., Hundal, G., and Chattopadhyay, P. (2014). Synthesis, characterization, interactions with DNA and bovine serum albumin (BSA), and antibacterial activity of cyclometalated iridium (III) complexes containing dithiocarbamate derivatives. J. Coord. Chem. 67, 2643–2660. doi:10.1080/00958972.2014.945924
Oyamada, T. (1934). A new general method for the synthesis of flavonol derivatives. J. Chem. Soc. Jpn. 55, 1256–1260. doi:10.1246/bcsj.10.182
Parr, R. G., Szentpály, L. V., and Liu, S. (1999). Electrophilicity index. J. Am. Chem. Soc. 121, 1922–1924. doi:10.1021/ja983494x
Patersson, G. A., and Al-Laham, M. A. (1991). A complete basis set model chemistryII. Open shell systems and the total energies of the first row atoms. J. Chem. Phys. 94, 6081–6090. doi:10.1063/1.460447
Ringbom, A. (1938). On the accuracy of colorimetric analytical methods. Fresenius. Z. F. Anal. Chem. 115, 332–343. doi:10.1007/bf01753937
Sid Kalal, H., Taghiof, M., Hoveidi, H., Pakizvand, N., Vahidi, H., Panahi, H. A., et al. (2013). The pre-concentration and determination of iridium and palladium in environmental water by imprinted polymer-based method. Int. J. Environ. Sci. Technol. (Tehran). 10, 1091–1102. doi:10.1007/s13762-013-0308-y
Singh, S. B. (2016). Iridium chemistry and its catalytic applications: A brief review. gctl. 2, 206–210. doi:10.18510/gctl.2016.247
Tabrizi, L., Nguyen, T., and Quang, Dao D. (2019). Experimental and theoretical investigation of cyclometalated phenylpyridine iridium(iii) complex based on flavonol and ibuprofen ligands as potent antioxidant. RSC Adv. 9, 17220–17237. doi:10.1039/C9RA02726B
Tarasiewicz, H. P., Grudiniewska, A., and Tarasiewicz, M. (1977). An examination of chlorpromazine hydrochloride as indicator and spectrophotometric reagent for the determination of molybdenum (V). Anal. Chim. Acta X. 94, 435–442. doi:10.1016/s0003-2670(01)84546-3
Vosburgh, W. C., and Cooper, G. R. (1941). Complex ions. I. The identification of complex ions in solution by spectrophotometric measurements. J. Am. Chem. Soc. 63, 437–442. doi:10.1021/JA01847A025
Wadt, W. R., and Hay, P. J. (1985). Ab initio effective core potentials for molecular calculations. Potentials for K to Au including the outermost core orbitals. J. Chem. Phys. 82, 299–310. doi:10.1063/1.448975
Yi, Y. V., and Masuda, A. (1996). Simultaneous determination of ruthenium, palladium, iridium and platinum at ultra-trace levels by isotope dilution inductively coupled plasma mass spectrometry in geological samples. Anal. Chem. 68, 1444–1450. doi:10.1021/ac951121y
Yoe, J. H., and Jones, A. L. (1944). Formation and stability of inorganic complexes in solution. Ind. Eng. Chem. 16, 111–115.(Anal.Ed.).
Zhang, G., and Tian, M. (2015). A rapid and practical strategy for the determination of platinum, palladium, ruthenium, rhodium, iridium and gold in large amounts of ultrabasic rock by inductively coupled plasma optical emission spectrometry combined with ultrasound extraction. Opt. Spectrosc. 118, 513–518. doi:10.1134/S0030400X15040049
Keywords: iridium, quantum chemical studies, spectrophotometric determination, antibacterial, antioxidant
Citation: Mohmad M, Agnihotri N, Kumar V, Azam M, Wabaidur SM, Kamal R, Kumar R, Alam M and Kaviani S (2022) Radical scavenging capacity, antibacterial activity, and quantum chemical aspects of the spectrophotometrically investigated iridium (III) complex with benzopyran derivative. Front. Pharmacol. 13:945323. doi: 10.3389/fphar.2022.945323
Received: 16 May 2022; Accepted: 02 August 2022;
Published: 02 September 2022.
Edited by:
Ranjan K. Mohapatra, Government College of Engineering, IndiaReviewed by:
M. Parveen, Aligarh Muslim University, IndiaTran Nguyen Minh An, Industrial University of Ho Chi Minh City, Vietnam
Copyright © 2022 Mohmad, Agnihotri, Kumar, Azam, Wabaidur, Kamal, Kumar, Alam and Kaviani. This is an open-access article distributed under the terms of the Creative Commons Attribution License (CC BY). The use, distribution or reproduction in other forums is permitted, provided the original author(s) and the copyright owner(s) are credited and that the original publication in this journal is cited, in accordance with accepted academic practice. No use, distribution or reproduction is permitted which does not comply with these terms.
*Correspondence: Nivedita Agnihotri, nivagni11@gmail.com; Mohammad Azam, azam_res@yahoo.com; Mahboob Alam, mahboobchem@gmail.com
†ORCID: Nivedita Agnihotri, orcid.org/0000-0002-3588-5852