- 1Department of Pharmacy, Anhui Provincial Cancer Hospital, The First Affiliated Hospital of USTC, Division of Life Sciences and Medicine, University of Science and Technology of China, Hefei, China
- 2Anhui Provincial Laboratory of Inflammatory and Immunity Disease, Anhui Institute of Innovative Drugs, School of Pharmacy, Anhui Medical University, Hefei, China
It is acknowledged that chronic inflammation is associated with a rise in extracellular proton concentrations. The acid-sensing ion channel 1a (ASIC1a) belongs to the extracellular H+-activated cation channel family. Recently, many studies have been conducted on ASIC1a and inflammatory immune diseases. Here, in this review, we will focus on the role of ASIC1a in several inflammatory immune diseases so as to provide new perspectives for clinical treatment.
Introduction
Acid-sensing ion channels (ASICs) are a subfamily of degenerin/epithelial Na+ channel family of non-voltage gated cation channels, currently classified as ligand-gated ion channels, including ASIC1, ASIC2, ASIC3, and ASIC4, which are widely expressed in both the peripheral and central nervous systems. ASICs regulation may occur through various means: multitudinous classes of drugs including small molecules, individual chemical entities, protein–protein interactions including toxins and venoms, metals, signal transduction pathways including receptor–receptor initiated activity, and even endogenous compounds (Alvarez de la Rosa et al., 2000; Zha et al., 2006; Zeng et al., 2014; Wang et al., 2016). Numerous studies have illustrated that ASICs are directly activated by extracellular protons, are shown to contribute to a variety of pathophysiological condition that involves tissue acidosis (Ziemann et al., 2008; Wang and Xu, 2011; Wang et al., 2016; Salinas Castellanos et al., 2021; Xu et al., 2022). ASIC1a is a kind of cation channel protein complex made up of intracellular amino terminal and carboxyl terminal, two membrane spaning domains and an extracellular loop, which can be activated by extracellular H+, open channel has permeability to Na+,Ca2+ (Figure 1) (Zha et al., 2006; Baconguis et al., 2014; Yoder et al., 2018; Zhu et al., 2020; Wang et al., 2021).
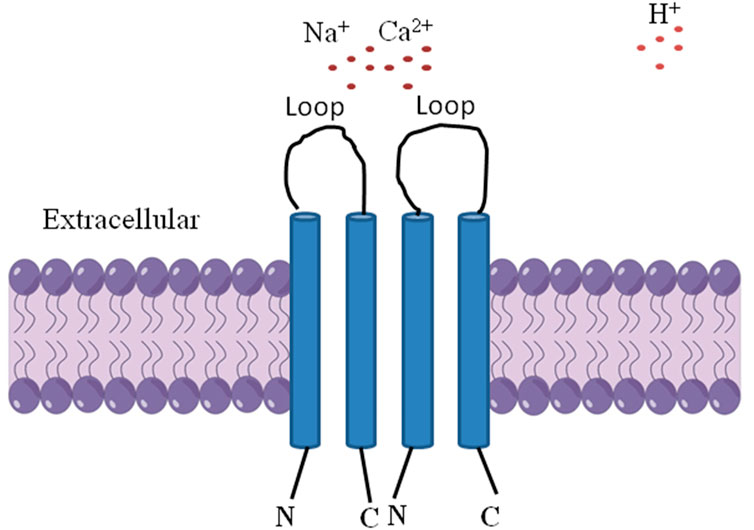
FIGURE 1. The structure of ASIC1a is comprised of three identical 1a subunits, intracellular amino terminal and carboxyl terminal, two membrane spaning domains and an extracellular loop, which can be activated by extracellular H+, open channel has permeability to Na+, Ca2+.
Recently there is increasing evidence that ASIC1a plays an important role in the occurrence and development of diseases involving the central and peripheral nervous systems (Zhang et al., 2020; Kong et al., 2021; Qi et al., 2022). It is acknowledged that chronic inflammation is associated with a rise in extracellular proton concentrations. Recently there is considerable evidence that extracellular acidification is a common phenomenon that is relevant to the physiological and pathological mechanism of inflammation, including infectious diseases, early wound healing and tumorigenesis et al. (Riemann et al., 2015; Riemann et al., 2019). The drop in pH occurs during inflammation as a result of the infiltration of inflammatory cells into the tissue, which causes the tissue to burn more energy and oxygen as well as increase its glucose consumption via glycolysis and thus increased lactic acid secretion (Xu and Chen, 2020a). Meanwhile, researches show that ASIC1a plays a key role in infiltration and activation of inflammatory cells, which has been detected in different kinds of inflammatory cells, including macrophages, dendritic cells, central microglia, Th cells, eosinophils, neutrophils, mast cells, B cells, plasma cells, and T cells (Tong et al., 2011; Yu et al., 2015; Ni et al., 2018; Tang et al., 2021). In addition, studies also have shown that inflammatory cytokines IL-1β, IL-6, and TNF-α are also closely associated with ASIC1a, which can affect the expression of ASIC1a (Zhou et al., 2015; Zhou et al., 2018). These inflammatory cells involved in ASIC1a are highlighted in Table 1.
It is also remarkable that inflammation and trauma can lead to enhanced pain sensitivity, which is closely related to ASICs. Research suggested that ASICs in dorsal horn neurons are involved in central sensory transmission and modulation and may play a role in inflammation-related persistent pain (Wu et al., 2004). In a later study, Ca2+ signaling in spinal dorsal horn neurons is enhanced following peripheral inflammation via upregulation of ASIC1a channels, which contributes to the hypersensitivity of the dorsal horn neurons and inflammatory processes. Blocking Ca2+ permeable ASIC1a channels can result in antinociceptive effects by minimizing or preventing the development of central sensitization induced by inflammation pain (Duan et al., 2007). Further evidence has shown that hyperalgesia is associated with increased mRNA expression of ASIC1a, 1b, and three in the dorsal root ganglions (DRGs) on the ipsilateral side (Nagae et al., 2006; Fu et al., 2016). In this review, we mainly focus on ASIC1a involved in inflammatory immune diseases, in order to provide new perspectives for clinical treatment.
The Central Nervous System
It is acknowledged that microglia are the most important innate immune cells in the brain and play a vital role in CNS homeostasis, which act as agents of surveillance and scavengers of immune defense and inflammatory response. Yu et al. (2015) identified the existence of ASICs in cultured and in situ rat microglia. They found the expression of ASIC1 and ASIC2a in microglia are increased upon stimulation with LPS. Furthermore, ASIC1a blocker amiloride and PcTx1 reduced the expression of inflammatory cytokines, including inducible nitric oxide synthase and cyclooxygenase 2 activated by LPS. Overall, their results suggest that ASICs are involved in the neuroinflammatory response.
Here, we focus on two common central nervous system diseases and review the relationship with ASIC1a to develop a novel therapeutic approach for the control of neuronal diseases related to inflammation.
Parkinson’s Disease
Parkinson’s Disease (PD), the second-most common neuro-degenerative disease, is characterized by the progressive and selective destruction of dopaminergic neurons located in the substantia nigra pars compacta (SNpc) area of the brain and cytoplasmic inclusions of alpha-synuclein (Mango and Nisticò, 2021; Zhang et al., 2021). Presently available treatments for PD are limited, mostly symptomatic, and associated with decreasing effectiveness and unwanted side effects over time. Treatment strategies targeting the underlying pathogenesis of the disease in order to slow down or halt disease progression, together with reliable and sensitive tests for early detection of the disease, which represents a large unmet medical need (Gelders et al., 2018). The involvement of the innate and adaptive immune systems in neurodegeneration has been documented by a number of postmortem, brain imaging, epidemiological, and animal studies (Chen et al., 2005; Theodore et al., 2008; Sanchez-Guajardo et al., 2010; Harms et al., 2018). Multiple studies have indicated that there were elevated levels of proinflammatory interleukins IL1β, IL2, IL6, TNF-α, and TGFβ1 in the striatum, in addition, concentrations of TNF-α, IL1β, IFNγ, NOS, and ROS were also found to be increased in the substantia nigra of postmortem samples (Mogi et al., 1994; Mogi et al., 1995; Mogi et al., 1996). Furthermore, these findings support the idea that microglia initiate both pro- and anti-inflammatory events, pointing towards that there are multiple phenotypes and distinct roles in PD. As for the association between PD and ASIC1a, Arias et al. (2008) established a model of PD accompanying lactic acidosis by MPTP, they explored the effects of amiloride in the MPTP-treated mouse, and found that amiloride can protect substantia nigra neurons from MPTP-induced degeneration. Moreover, amiloride also preserved dopaminergic cell bodies in the SNc. Parkin encodes a 465 amino acid protein that is expressed in multiple tissues as an E3 Ub-ligase within the ubiquitin (Ub) system, mutations in the parkin gene cause an autosomal recessive form of Parkinson’s disease that develops at a young age (Shimura et al., 2000). Joch et al. (2007) found by knocking out parkin, hippocampal neurons of parkin knockout mice exhibit prominent potentiation of native ASIC currents that are normally suppressed by endogenous parkin in wild-type neurons, which indicated that ASIC1a may be crucially involved in the pathophysiology of PD. Even so, the role of ASIC1a on PD remains unclear.
Multiple Sclerosis
Multiple Sclerosis (MS) is a chronic autoimmune inflammatory disease which can lead to motor deficit by neuronal damage-induced inflammation and demyelination in the central nervous system. Generally, it is considered to be an autoimmune and T cell-mediated disease. Chronic neuropathic pain is the most serious symptom which severely reduces quality of life (Friese et al., 2014). Roles of ASIC1a in MS were explored by many studies. The experimental autoimmune encephalomyelitis (EAE) model is a well-accepted animal model of MS (Friese et al., 2007; Bjelobaba et al., 2018). To explore the effect of ASIC1 on MS, Friese et al. (2007) established EAE model and ASIC1−/− mice were used, results showed clinical defificit and axonal degeneration markedly reduced compared to wild-type mice. Moreover, pH measurements in the spinal cord of EAE mice indicated tissue acidosis could lead to ASIC1 open. Therefore, they consider that ASIC1 blockers could provide neuroprotection in MS. Vergo et al. (2011) found that ASIC1 was upregulated in axons and oligodendrocytes within lesions from mice with acute EAE and from patients with active multiple sclerosis. Furthermore, ASIC1 co-localization with the axonal injury marker beta amyloid precursor protein indicated ASIC1 was closely related to axonal damage, meanwhile, they found there was a significant decrease in disease severity in amiloride-treated EAE mice compared with vehicle-treated EAE mice, blocking ASIC1 with amiloride protected both myelin and neurons from damage in the acute model. They also examined the role of ASIC1 expression in the oligodendrocytes, results showed in longitudinal white matter tissue sections of acute EAE, there was a significantly increased number of ASIC1a mRNA positive and ASIC1+ve oligodendrocytes in EAE. Taken together, their findings indicate that blockade of ASIC1 has the potential to provide both neuro- and myelo-protective benefits in MS. Similarly, Arun et al. (2013) also found ASIC1 was increased in axons and oligodendrocytes in chronic inactive lesions of cases with progressive MS, axons with an injury profile were continually seen to co-express ASIC1, while ASIC1-positive oligodendrocytes were also identified in chronic MS lesions, indicating a molecular signature that may contribute to cellular damage. And they recruited a group of patients with primary progressive MS participating in a longitudinal imaging protocol to assess whether amiloride could impact on surrogate imaging markers of neurodegeneration, results showed there was statistically significant MRI evidence of benefit during the amiloride phase in progressive MS using small numbers of patients. Their results further extend evidence of the role of ASIC1 to neurodegeneration in MS and indicate that amiloride may exert neuro-protective effects in patients with progressive MS. As for mechanisms of ASIC1a involved neurodegeneration and axonal dysfunction in MS, it is acknowledged that Ca2+ plays an important role in this process. Friese et al. (2014) summarized that ASIC1 promotes further Ca2+ influx and potentiates Na+ influx. While reduced ATP levels lower Na+/K+-ATPase activity, leading to reverse operation of NCX and increasing intracellular Ca2+ and Na+ levels. Eventually Ca2+ overload activates degradative enzymes and NOS which can lead to neuronal apoptosis and necrosis.
It is recognized that inflammation is characterized by edema, redness, fever, and pain (Kim et al., 2018; Reis et al., 2019). The inflammatory process of the CNS is accompanied by cerebral edema. Recent researches show Piroxicam may play a neuroprotective role in cerebral ischemia by inhibiting aquaporin 4 (AQP4) and ASIC1a, which may indicate a potential association between AQP4 and ASIC1a (Bhattacharya et al., 2012; Mazumder and Borah, 2015). Here, we also briefly introduce AQP4 in order to provide new understanding about the mechanism of ASIC1a in the process of CNS inflammatory. Aquaporins (AQPs) are plasma membrane channels that aid in the bidirectional transmembrane transport of water, which is an important component of cytotoxic edema. AQP4 is the main member of this family expressed in the CNS, an abundance of which is found in astrocytes, which appears to play different roles in CNS edema development and resolution. Therefore, reversible inhibition of AQP4 function during the acute phase may help prevent CNS edema (Kitchen et al., 2020; Liu et al., 2021; Sylvain et al., 2021). The results of previous studies on a single representative water channel protein suggest narrow channels conduct water, whereas wider channels permit solutes to pass through. Kitchen et al. found that single amino acid substitutions in the selectivity filters of AQP1, AQP4, and AQP3 differentially affect glycerol and urea permeability in an AQP-specific manner. Their data showed that substrate discrimination in water channels relies on a complex interplay between the solute, pore size, and polarity, and that using single water channel proteins as representative models has led to an underestimation of this complexity (Hara-Chikuma and Verkman, 2006; Kitchen et al., 2019). The role of water homeostasis in buffering the ion concentration which is mediated mainly by glial cells through the function of water channels. When inflammation occurs, both AQP4 and ASIC1a may be open at the same time, whether there is a certain association between AQP4 and ASIC1a in inflammatory diseases of CNS, relative research should be studied in the future. Targeting the subcellular relocalization mechanisms of aquaporins and ASIC1a will provide an alternative strategy for developing drugs. The definition of signature motifs and molecular mechanisms of ion transport also will provide new avenues for future drug discovery (Markou et al., 2022; Salman et al., 2022; Wagner et al., 2022).
Rheumatoid Arthritis
Rheumatoid arthritis (RA) is a chronic autoimmune disease characterized by synovial cell proliferation, multiple inflammatory cell infiltration, pannus formation, cartilage and bone tissue destruction, which eventually results in joint deformity and loss of function (Gibofsky, 2014). Recently, it has been reported that ASIC1a was closely related to RA, numerous studies have been conducted on ASIC1a and RA. To explore the roles of IL-1β and TNF-α in acid-induced apoptosis of chondrocytes, Zhou et al. (2018) studied rat adjuvant arthritis and primary articular chondrocytes as in vivo and in vitro models, they found that IL-1β and TNF-α simultaneously increased ASIC1a expression in articular chondrocytes on a time- and dose-dependent basis. Their results indicated that these cytokines activated MAPK and NF-κB pathways in chondrocytes, and inhibitors of these pathways suppressed the ASIC1a upregulation caused by IL-1β and TNF-α. Furthermore, results showed IL-1β and TNF-α induced ASIC1a promoter activity in chondrocytes by increasing binding to DNA by NF-κB. Additionally, IL-1β and TNF-α decreased cell viability while enhanced LDH release, intracellular Ca2+ concentration elevation, loss of mitochondrial membrane potential, cleaved PARP and cleaved caspase-3/9 expression, and apoptosis in acid-stimulated chondrocytes. Their results indicated that IL-1β and TNF-α can enhance acidosis-induced cytotoxicity through increasing the expression of ASIC1a in primary articular chondrocytes. Pyroptosis is a type of proinflammatory programmed cell death that involves the activation of caspase-1 and the production of interleukin IL-1β/18. There has been previous evidence that pyroptosis may be related to the development of some autoimmune diseases, such as RA (Choulaki et al., 2015). Wu et al. (2019) found extracellular acidosis significantly increased the expression of ASIC1a, IL-1β, IL-18, ASC, NLRP3, and caspase-1, while PcTX1 inhibited these effects. Simultaneously, in rats with AA, there were higher levels of the proinflammatory cytokines IL-1β and IL-18 than in rats with normal blood, but aspirin and amiloride suppressed these effects. According to these results, they concluded that ASIC1a is required for pyroptosis to occur in chondrocytes from AA rats, which may be related to ASIC1a’s ability to activate Ca2+ inflow, drugs targeting pyroptosis and IL-1β/18 might be developed that can effectively treat RA in the future. Moreover, to identify the role of ASIC1a, calpain, calcineurin, and NLRP3 inflammasome proteins in regulating acid-induced articular chondrocyte pyroptosis, in Zu’s research, chondrocytes from primary rat articular articular cartilage were exposed to different pH, different time, and different treatments including or without ASIC1a, calpain-2, and calcineurin, respectively. They found that extracellular acidosis increased the protein expression of ASIC1a in a pH- and time-dependent manner, and the messenger RNA and protein levels of calpain, calcineurin, NLRP3, and apoptosis-associated speck-like protein were also increased in a time-dependent manner. Interestingly, they also indicated that inhibiting ASIC1a, calpain-2, or calcineurin could decrease the cell death. As a result, they concluded that extracellular acidosis activated ASIC1a, causing pyroptosis of chondrocytes in rat articular cartilage, mechanisms for which may be partly linked to calpain-2/calcineurin activation (Zu et al., 2020). In addition, ASIC1a is also involved in synovial invasion. Niu et al. (2020) investigated the roles of ASIC1a in synovial invasion in vivo as well as the migration and invasion of RA-FLS in vitro. They found that ASIC1a was highly expressed in RA synovial tissues and RA-FLSs, ASIC1a inhibition by PcTX-1 reduced synovial invasion and expression of MMPs2, 9, and p-FAK to prevent cartilage degradation in AA rats. Furthermore, they also showed that ASIC1a-RNAi and PcTX-1 inhibited the acidity-induced invasion and migration of RA-FLSs while overexpression of ASIC1a increased their expression of MMP2, MMP9, and p-FAK. And in this process Ca2+ influx through ASIC1a activated the Ras-related calcium chelating agent Rab1 (Rac1), which was decreased by BAPTA-AM, an intracellular calcium chelating agent. Similarly, Rac1 specific blocker NSC23766 reduced migration and invasion of RA-FLSs as well as MMP2, MMP9 and p-FAK expressions. As a result of their study, they concluded that ASIC1a may be a master regulator of synovial invasion via the Ca2+/Rac1 pathway. Meanwhile, Zhang et al. (2020) indicated that ASIC1a induces synovial inflammation, which leads to the progression of RA, it was found that ASIC1a was significantly expressed in RA synovial tissues and in human primary RASF as well as in the ankle synovium of AA rats in their research. They showed that activation or overexpression of ASIC1a in RASF enhanced inflammatory cytokines RANTES, sTNF RI, MIP-1a, IL-8, sTNF RII, and ICAM-1, with RANTES increasing most prominently. Furthermore, in vivo they found activation of ASIC1a also led to inflammation, synovial hyperplasia, articular cartilage destruction, and bone destruction, resulting in the progression of AA. In summary, these findings offer a rationale for ASIC1a as a potential therapeutic target for RA.
Asthma
Asthma is a chronic obstructive disease of the airways worldwide, characterized by airway inflammation and airway hyperreactivity (AHR) (Alwarith et al., 2020; Aghapour et al., 2022). Treatment of asthma is a complicated problem, current drug treatments mainly include long-term control treatments, such as inhaled corticosteroids, long-acting beta-agonists, and oral medications. The active search for new therapeutic drugs and methods is concerned by scholars (Chen et al., 2015; Vasileiadis et al., 2019). The study of ASIC1a in asthma is now being slowly recognized. Faisy et al. (2007) found the pH-induced relaxation of airway basal tone was inhibited by ASIC1a inhibitor and the initial pH-induced airway relaxation may be independent of sensory nerves, indicating a regulation of airway basal tone mediated by ASICs on smooth muscle. Reznikov et al. (2016) found ASIC1a protein in vagal ganglia neurons and they induced AHR by sensitizing mice to ovalbumin, results showed that ASIC1a−/− mice did not exhibit AHR while accompanied by a strong inflammatory response. They assessed inflammation using quantitative histopathology and found that OVA-sensitization increased bronchovascular inflammation in both wild-type and ASIC1a−/− mice but there was no difference between two genotypes, therefore they concluded that loss of ASIC1a decreases AHR without alleviating the inflammatory response. Interestingly, they found that IL-13 was increased in the bronchoal veolar lavage fluid of OVA-sensitized ASIC1a−/− mice, but the underlying mechanism of IL-13 upregulation is uncertain, it is considered that ASIC1a deficiency may prevent proton-mediated repression of IL-13 release and/or transcription. Moreover, in this project, levels of substance P was decreased in OVA-sensitized ASIC1a−/− mice bronchoalveolar lavage fluid, which is acknowledged to contribute AHR. Collectively, their results suggest that ASIC1a plays an important role in AHR and can support the possibility that inhibiting ASIC1a might be beneficial in asthma.
Chronic Rhinosinusitis
Chronic rhinosinusitis with nasal polyps (CRSwNP) is a chronic inflammatory disease, characterized by nasal congestion, rhinorrhea, and diminished smell, as well as headache (Nguyen et al., 2020). On histologic examination, CRSwNP exhibits infiltration of numerous inflammatory cells within the sinuses. A complex interaction between inflammatory cells and mediators plays an integral role in the pathogenesis of CRSwNP (Terna et al., 2016; Schleimer, 2017). Tang et al. (2021) focused on the pathogenesis of CRSwNP and the role of ASIC1a. First, they measured pH values in nasal secretions from control subjects and CRSwNP patients with and without asthma, as well as ASIC1a expression and co-localization in inflammatory cells located in nasal tissue samples from CRSwNP subjects. Then within dispersed nasal polyp cells’ (DNPCs) cultures in vitro, they quantified ASIC1a, LDH activity, HIF-1α, and inflammatory cytokines levels. Results showed there were lower pH values and increased levels of ASIC1a protein and mRNA in CRSwNP with asthma, ASIC1a protein was also found in various types of inflammatory cells, while amiloride can reverse this effect. Based on these results, they considered that ASIC1a upregulation may play a key role in sensing acidification and triggering inflammatory responses by enhancing HIF-1α expression and LDH activity, which involved activating inflammatory cells in the pathogenesis of CRSwNP, especially in CRSwNP with asthma.
Discussion
ASIC1a desensitization causes a dilemma for neurophysiologists studying ASICs, after rapid desensitization induced by acidification, how can ASIC1a function properly in chronic inflammation? Xu et al. indicated that desensitization of the ASIC1a channel by moderate pH decreases suppressed subsequent activation of the ASIC1a current, but do not prevent acid-induced neuronal death. Their results demonstrated that ASIC1a channels play a vital role in mediating neuronal necroptosis in response to extracellular acidification, however, they discovered the ion-conducting mechanisms of the ASIC1a channels do not seem to be essential in this process. Although it cannot be excluded that ionic fluxes via the channel pore may play a modulatory role, it seems that RIP1 activation and not ionic conduction per se is essential for acid-induced necroptosis (Wang et al., 2015). Furthermore, in subsequent studies, they showed that the N-terminus (NT) of ASIC1a interacts with its CT to form an auto-inhibition that prevents RIPK1 recruitment/activation under resting conditions. This interaction is disrupted by acidosis by the involvement of glutamate residues at the distal NT. In the presence of mutant ASIC1a containing glutamate-to-alanine substitutions at distal NT, constitutive cell death occurs. N-ethylmaleimide-sensitive fusion ATPase (NSF) further disrupts NT-CT interactions, which corelated with ASIC1a-NT under acidosis, facilitating RIPK1 interaction with ASIC1a-CT (Wang et al., 2020). The results by Xu et al. may partly explain the mechanism after desensitization. On the other hand, it is recognized that plasma membrane expression is critical to the function of ASICs, which act as extracellular proton sensors. In part, the number and function of receptors on the plasma membrane depend on protein synthesis and degradation processes as well as dynamic trafficking processes (Waldmann and Lazdunski, 1998; Zeng et al., 2014; Xu and Chen, 2020b). Therefore, we consider that increased ASIC1a expression and membrane transport can occur after desensitization, which may maintain subsequent function of ASIC1a in inflammatory. Of course, further research is necessary to support this claim. In this review, we focused on inflammatory immune diseases including PD, MS, RA, asthma, and chronic rhinosinusitis, summarized the effects and related mechanisms of ASIC1a, however, there are still few studies of ASIC1a in inflammatory immune diseases, we need to pay more attention in the future.
Conclusion
Recent studies have shown that ASIC1a plays a vital role in the occurrence and development of diseases involved in the central and peripheral nervous system. In this review, we concluded that ASIC1a is closely associated with inflammation and immunity, which may be a promising target. A deeper understanding of the role of ASIC1a in inflammatory immune diseases will present the potential of using ASIC1a as a new targeting agent for inflammatory conditions. In addition, in the future, for potential targets identified in research, high-throughput screening and computer-aided drug design can be used for novel drug discovery in the field of pharmaceutics, which can provide a novel insight and support target validation in future studies (Aldewachi et al., 2021; Salman et al., 2021).
Data Availability Statement
The raw data supporting the conclusion of this article will be made available by the authors, without undue reservation.
Author Contributions
YW designed and wrote this manuscript, XH revised the manuscript. YS checked the manuscript. YH designed and checked the manuscript.
Funding
This study is supported by the Fundamental Research Funds for the Central Universities (No. WK9110000113), the hospital youth fund of West Branch of The First Affiliated Hospital of University of Science and Technology of China (No. 2018YJQN016). Anhui Outstanding Youth Fund Project (No.1908085J30).
Conflict of Interest
The authors declare that the research was conducted in the absence of any commercial or financial relationships that could be construed as a potential conflict of interest.
Publisher’s Note
All claims expressed in this article are solely those of the authors and do not necessarily represent those of their affiliated organizations, or those of the publisher, the editors and the reviewers. Any product that may be evaluated in this article, or claim that may be made by its manufacturer, is not guaranteed or endorsed by the publisher.
References
Aghapour, M., Ubags, N. D., Bruder, D., Hiemstra, P. S., Sidhaye, V., Rezaee, F., et al. (2022). Role of Air Pollutants in Airway Epithelial Barrier Dysfunction in Asthma and COPD. Eur. Respir. Rev. 31 (163), 210112. doi:10.1183/16000617.0112-2021
Aldewachi, H., Al-Zidan, R. N., Conner, M. T., and Salman, M. M. (2021). High-Throughput Screening Platforms in the Discovery of Novel Drugs for Neurodegenerative Diseases. Bioengineering 8 (2), 30. doi:10.3390/bioengineering8020030
Alvarez de la Rosa, D., Canessa, C. M., Fyfe, G. K., and Zhang, P. (2000). Structure and Regulation of Amiloride-Sensitive Sodium Channels. Annu. Rev. Physiol. 62, 573–594. doi:10.1146/annurev.physiol.62.1.573
Alwarith, J., Kahleova, H., Crosby, L., Brooks, A., Brandon, L., Levin, S. M., et al. (2020). The Role of Nutrition in Asthma Prevention and Treatment. Nutr. Rev. 78 (11), 928–938. doi:10.1093/nutrit/nuaa005
Arias, R. L., Sung, M. L., Vasylyev, D., Zhang, M. Y., Albinson, K., Kubek, K., et al. (2008). Amiloride is Neuroprotective in an MPTP Model of Parkinson's Disease. Neurobiol. Dis. 31 (3), 334–341. doi:10.1016/j.nbd.2008.05.008
Arun, T., Tomassini, V., Sbardella, E., de Ruiter, M. B., Matthews, L., Leite, M. I., et al. (2013). Targeting ASIC1 in Primary Progressive Multiple Sclerosis: Evidence of Neuroprotection with Amiloride. Brain 136 (Pt 1), 106–115. doi:10.1093/brain/aws325
Baconguis, I., Bohlen, C. J., Goehring, A., Julius, D., and Gouaux, E. (2014). X-Ray Structure of Acid-Sensing Ion Channel 1-snake Toxin Complex Reveals Open State of a Na(+)-Selective Channel. Cell 156 (4), 717–729. doi:10.1016/j.cell.2014.01.011
Bhattacharya, P., Pandey, A. K., Paul, S., and Patnaik, R. (2012). Neuroprotective Potential of Piroxicam in Cerebral Ischemia: An In Silico Evaluation of the Hypothesis to Explore its Therapeutic Efficacy by Inhibition of Aquaporin-4 and Acid Sensing Ion Channel1a. Med. Hypotheses 79 (3), 352–357. doi:10.1016/j.mehy.2012.05.033
Bjelobaba, I., Begovic-Kupresanin, V., Pekovic, S., and Lavrnja, I. (2018). Animal Models of Multiple Sclerosis: Focus on Experimental Autoimmune Encephalomyelitis. J. Neurosci. Res. 96 (6), 1021–1042. doi:10.1002/jnr.24224
Chen, H., Jacobs, E., Schwarzschild, M. A., McCullough, M. L., Calle, E. E., Thun, M. J., et al. (2005). Nonsteroidal Antiinflammatory Drug Use and the Risk for Parkinson's Disease. Ann. Neurol. 58 (6), 963–967. doi:10.1002/ana.20682
Chen, X., Kang, Y. B., Wang, L. Q., Li, Y., Luo, Y. W., Zhu, Z., et al. (2015). Addition to Inhaled Corticosteroids of Leukotriene Receptor Antagonists versus Theophylline for Symptomatic Asthma: A Meta-Analysis. J. Thorac. Dis. 7 (4), 644–652. doi:10.3978/j.issn.2072-1439.2015.04.12
Choulaki, C., Papadaki, G., Repa, A., Kampouraki, E., Kambas, K., Ritis, K., et al. (2015). Enhanced Activity of NLRP3 Inflammasome in Peripheral Blood Cells of Patients with Active Rheumatoid Arthritis. Arthritis Res. Ther. 17, 257. doi:10.1186/s13075-015-0775-2
Duan, B., Wu, L. J., Yu, Y. Q., Ding, Y., Jing, L., Xu, L., et al. (2007). Upregulation of Acid-Sensing Ion Channel ASIC1a in Spinal Dorsal Horn Neurons Contributes to Inflammatory Pain Hypersensitivity. J. Neurosci. 27 (41), 11139–11148. doi:10.1523/JNEUROSCI.3364-07.2007
Faisy, C., Planquette, B., Naline, E., Risse, P. A., Frossard, N., Fagon, J. Y., et al. (2007). Acid-induced Modulation of Airway Basal Tone and Contractility: Role of Acid-Sensing Ion Channels (ASICs) and TRPV1 Receptor. Life Sci. 81 (13), 1094–1102. doi:10.1016/j.lfs.2007.08.026
Friese, M. A., Craner, M. J., Etzensperger, R., Vergo, S., Wemmie, J. A., Welsh, M. J., et al. (2007). Acid-sensing Ion Channel-1 Contributes to Axonal Degeneration in Autoimmune Inflammation of the Central Nervous System. Nat. Med. 13 (12), 1483–1489. doi:10.1038/nm1668
Friese, M. A., Schattling, B., and Fugger, L. (2014). Mechanisms of Neurodegeneration and Axonal Dysfunction in Multiple Sclerosis. Nat. Rev. Neurol. 10 (4), 225–238. doi:10.1038/nrneurol.2014.37
Fu, H., Fang, P., Zhou, H. Y., Zhou, J., Yu, X. W., Ni, M., et al. (2016). Acid-sensing Ion Channels in Trigeminal Ganglion Neurons Innervating the Orofacial Region Contribute to Orofacial Inflammatory Pain. Clin. Exp. Pharmacol. Physiol. 43 (2), 193–202. doi:10.1111/1440-1681.12510
Gelders, G., Baekelandt, V., and Van der Perren, A. (2018). Linking Neuroinflammation and Neurodegeneration in Parkinson's Disease. J. Immunol. Res. 2018, 4784268. doi:10.1155/2018/4784268
Gibofsky, A. (2014). Epidemiology, Pathophysiology, and Diagnosis of Rheumatoid Arthritis: A Synopsis. Am. J. Manag. Care 20 (7 Suppl. l), S128–S135. https://pubmed.ncbi.nlm.nih.gov/25180621/
Hara-Chikuma, M., and Verkman, A. S. (2006). Physiological Roles of Glycerol-Transporting Aquaporins: the Aquaglyceroporins. Cell Mol. Life Sci. 63 (12), 1386–1392. doi:10.1007/s00018-006-6028-4
Harms, A. S., Thome, A. D., Yan, Z., Schonhoff, A. M., Williams, G. P., Li, X., et al. (2018). Peripheral Monocyte Entry is Required for Alpha-Synuclein Induced Inflammation and Neurodegeneration in a Model of Parkinson Disease. Exp. Neurol. 300, 179–187. doi:10.1016/j.expneurol.2017.11.010
Joch, M., Ase, A. R., Chen, C. X., MacDonald, P. A., Kontogiannea, M., Corera, A. T., et al. (2007). Parkin-mediated Monoubiquitination of the PDZ Protein PICK1 Regulates the Activity of Acid-Sensing Ion Channels. Mol. Biol. Cell 18 (8), 3105–3118. doi:10.1091/mbc.e05-11-1027
Kim, Y. J., Lee, J. Y., Kim, H. J., Kim, D. H., Lee, T. H., Kang, M. S., et al. (2018). Anti-Inflammatory Effects of Angelica Sinensis (Oliv.) Diels Water Extract on RAW 264.7 Induced with Lipopolysaccharide. Nutrients 10 (5), 647. doi:10.3390/nu10050647
Kitchen, P., Salman, M. M., Pickel, S. U., Jennings, J., Törnroth-Horsefield, S., Conner, M. T., et al. (2019). Water Channel Pore Size Determines Exclusion Properties but Not Solute Selectivity. Sci. Rep. 9 (1), 20369. doi:10.1038/s41598-019-56814-z
Kitchen, P., Salman, M. M., Halsey, A. M., Clarke-Bland, C., MacDonald, J. A., Ishida, H., et al. (2020). Targeting Aquaporin-4 Subcellular Localization to Treat Central Nervous System Edema. Cell 181 (4), 784–e19. doi:10.1016/j.cell.2020.03.037
Kong, X., Tang, X., Du, W., Tong, J., Yan, Y., Zheng, F., et al. (2013). Extracellular Acidosis Modulates the Endocytosis and Maturation of Macrophages. Cell Immunol. 281 (1), 44–50. doi:10.1016/j.cellimm.2012.12.009
Kong, L., Huang, H., Luan, S., Liu, H., Ye, M., and Wu, F. (2021). Inhibition of ASIC1a-Mediated ERS Improves the Activation of HSCs and Copper Transport Under Copper Load. Front. Pharmacol. 12, 653272. doi:10.3389/fphar.2021.653272
Liu, X., Wu, G., Tang, N., Li, L., Liu, C., Wang, F., et al. (2021). Glymphatic Drainage Blocking Aggravates Brain Edema, Neuroinflammation via Modulating TNF-α, IL-10, and AQP4 after Intracerebral Hemorrhage in Rats. Front. Cell Neurosci. 15, 784154. doi:10.3389/fncel.2021.784154
Mango, D., and Nisticò, R. (2021). Neurodegenerative Disease: What Potential Therapeutic Role of Acid-Sensing Ion Channels? Front. Cell Neurosci. 15, 730641. doi:10.3389/fncel.2021.730641
Markou, A., Unger, L., Abir-Awan, M., Saadallah, A., Halsey, A., Balklava, Z., et al. (2022). Molecular Mechanisms Governing Aquaporin Relocalisation. Biochim. Biophys. Acta Biomembr. 1864 (4), 183853. doi:10.1016/j.bbamem.2021.183853
Mazumder, M. K., and Borah, A. (2015). Piroxicam Confer Neuroprotection in Cerebral Ischemia by Inhibiting Cyclooxygenases, Acid- Sensing Ion Channel-1a and Aquaporin-4: An In Silico Comparison with Aspirin and Nimesulide. Bioinformation 11 (4), 217–222. doi:10.6026/97320630011217
Mogi, M., Harada, M., Riederer, P., Narabayashi, H., Fujita, K., and Nagatsu, T. (1994). Tumor Necrosis Factor-Alpha (TNF-Alpha) Increases Both in the Brain and in the Cerebrospinal Fluid from Parkinsonian Patients. Neurosci. Lett. 165 (1-2), 208–210. doi:10.1016/0304-3940(94)90746-3
Mogi, M., Harada, M., Kondo, T., Narabayashi, H., Riederer, P., and Nagatsu, T. (1995). Transforming Growth Factor-Beta 1 Levels Are Elevated in the Striatum and in Ventricular Cerebrospinal Fluid in Parkinson's Disease. Neurosci. Lett. 193 (2), 129–132. doi:10.1016/0304-3940(95)11686-q
Mogi, M., Harada, M., Narabayashi, H., Inagaki, H., Minami, M., and Nagatsu, T. (1996). Interleukin (IL)-1 Beta, IL-2, IL-4, IL-6 and Transforming Growth Factor-Alpha Levels Are Elevated in Ventricular Cerebrospinal Fluid in Juvenile Parkinsonism and Parkinson's Disease. Neurosci. Lett. 211 (1), 13–16. doi:10.1016/0304-3940(96)12706-3
Nagae, M., Hiraga, T., Wakabayashi, H., Wang, L., Iwata, K., and Yoneda, T. (2006). Osteoclasts Play a Part in Pain Due to the Inflammation Adjacent to Bone. Bone 39 (5), 1107–1115. doi:10.1016/j.bone.2006.04.033
Nguyen, T. N., Do, B. H., Kitamura, T., Ohkubo, J. I., Wakasugi, T., Ohbuchi, T., et al. (2020). Expression of Cl- Channels/transporters in Nasal Polyps. Eur. Arch. Otorhinolaryngol. 277 (8), 2263–2270. doi:10.1007/s00405-020-05981-1
Ni, L., Fang, P., Hu, Z. L., Zhou, H. Y., Chen, J. G., Wang, F., et al. (2018). Identification and Function of Acid-Sensing Ion Channels in RAW 264.7 Macrophage Cells. Curr. Med. Sci. 38 (3), 436–442. doi:10.1007/s11596-018-1897-y
Niu, R., Hang, X., Feng, Y., Zhang, Y., Qian, X., Song, S., et al. (2020). ASIC1a Promotes Synovial Invasion of Rheumatoid Arthritis via Ca2+/Rac1 Pathway. Int. Immunopharmacol. 79, 106089. doi:10.1016/j.intimp.2019.106089
Qi, X., Lu, J. F., Huang, Z. Y., Liu, Y. J., Cai, L. B., Wen, X. L., et al. (2022). Pharmacological Validation of ASIC1a as a Druggable Target for Neuroprotection in Cerebral Ischemia Using an Intravenously Available Small Molecule Inhibitor. Front. Pharmacol. 13, 849498. doi:10.3389/fphar.2022.849498
Reis, R. F., Fernandes, J. L., Schmal, T. R., Rocha, B. M., Dos Santos, R. W., and Lobosco, M. (2019). A Personalized Computational Model of Edema Formation in Myocarditis Based on Long-axis Biventricular MRI Images. BMC Bioinform. 20 (Suppl. 6), 532. doi:10.1186/s12859-019-3139-0
Reznikov, L. R., Meyerholz, D. K., Adam, R. J., Abou Alaiwa, M., Jaffer, O., Michalski, A. S., et al. (2016). Acid-Sensing Ion Channel 1a Contributes to Airway Hyperreactivity in Mice. PloS One 11 (11), e0166089. doi:10.1371/journal.pone.0166089
Riemann, A., Ihling, A., Thomas, J., Schneider, B., Thews, O., and Gekle, M. (2015). Acidic Environment Activates Inflammatory Programs in Fibroblasts via a cAMP-MAPK Pathway. Biochim. Biophys. Acta 1853 (2), 299–307. doi:10.1016/j.bbamcr.2014.11.022
Riemann, A., Rauschner, M., Gießelmann, M., Reime, S., Haupt, V., and Thews, O. (2019). Extracellular Acidosis Modulates the Expression of Epithelial-Mesenchymal Transition (EMT) Markers and Adhesion of Epithelial and Tumor Cells. Neoplasia 21 (5), 450–458. doi:10.1016/j.neo.2019.03.004
Salinas Castellanos, L. C., Uchitel, O. D., and Weissmann, C. (2021). Signaling Pathways in Proton and Non-proton ASIC1a Activation. Front. Cell Neurosci. 15, 735414. doi:10.3389/fncel.2021.735414
Salman, M. M., Al-Obaidi, Z., Kitchen, P., Loreto, A., Bill, R. M., and Wade-Martins, R. (2021). Advances in Applying Computer-Aided Drug Design for Neurodegenerative Diseases. Int. J. Mol. Sci. 22 (9), 4688. doi:10.3390/ijms22094688
Salman, M. M., Kitchen, P., Yool, A. J., and Bill, R. M. (2022). Recent Breakthroughs and Future Directions in Drugging Aquaporins. Trends Pharmacol. Sci. 43 (1), 30–42. doi:10.1016/j.tips.2021.10.009
Sanchez-Guajardo, V., Febbraro, F., Kirik, D., and Romero-Ramos, M. (2010). Microglia Acquire Distinct Activation Profiles Depending on the Degree of Alpha-Synuclein Neuropathology in a rAAV Based Model of Parkinson's Disease. PloS One 5 (1), e8784. doi:10.1371/journal.pone.0008784
Schleimer, R. P. (2017). Immunopathogenesis of Chronic Rhinosinusitis and Nasal Polyposis. Annu. Rev. Pathol. 12, 331–357. doi:10.1146/annurev-pathol-052016-100401
Shimura, H., Hattori, N., Kubo, S., Mizuno, Y., Asakawa, S., Minoshima, S., et al. (2000). Familial Parkinson Disease Gene Product, Parkin, Is a Ubiquitin-Protein Ligase. Nat. Genet. 25 (3), 302–305. doi:10.1038/77060
Sylvain, N. J., Salman, M. M., Pushie, M. J., Hou, H., Meher, V., Herlo, R., et al. (2021). The Effects of Trifluoperazine on Brain Edema, Aquaporin-4 Expression and Metabolic Markers during the Acute Phase of Stroke Using Photothrombotic Mouse Model. Biochim. Biophys. Acta Biomembr. 1863 (5), 183573. doi:10.1016/j.bbamem.2021.183573
Tang, R., Ba, G., Li, M., Li, Z., Ye, H., Lin, H., et al. (2021). Evidence for Role of Acid-Sensing Ion Channel 1a in Chronic Rhinosinusitis with Nasal Polyps. Eur. Arch. Otorhinolaryngol. 278 (7), 2379–2386. doi:10.1007/s00405-020-06521-7
Terna, E., Luukkainen, A., Seppälä, M., Renkonen, R., Huhtala, H., Tommola, S., et al. (2016). The Expression of Cancerous Inhibitor Protein Phosphatase 2A in Chronic Rhinosinusitis with Nasal Polyps. Acta Otolaryngol. 136 (11), 1173–1179. doi:10.1080/00016489.2016.1195918
Theodore, S., Cao, S., McLean, P. J., and Standaert, D. G. (2008). Targeted Overexpression of Human Alpha-Synuclein Triggers Microglial Activation and an Adaptive Immune Response in a Mouse Model of Parkinson Disease. J. Neuropathol. Exp. Neurol. 67 (12), 1149–1158. doi:10.1097/NEN.0b013e31818e5e99
Tong, J., Wu, W. N., Kong, X., Wu, P. F., Tian, L., Du, W., et al. (2011). Acid-sensing Ion Channels Contribute to the Effect of Acidosis on the Function of Dendritic Cells. J. Immunol. 186 (6), 3686–3692. doi:10.4049/jimmunol.1001346
Vasileiadis, I., Alevrakis, E., Ampelioti, S., Vagionas, D., Rovina, N., and Koutsoukou, A. (2019). Acid-Base Disturbances in Patients with Asthma: A Literature Review and Comments on Their Pathophysiology. J. Clin. Med. 8 (4), 563. doi:10.3390/jcm8040563
Vergo, S., Craner, M. J., Etzensperger, R., Attfield, K., Friese, M. A., Newcombe, J., et al. (2011). Acid-sensing Ion Channel 1 Is Involved in Both Axonal Injury and Demyelination in Multiple Sclerosis and its Animal Model. Brain 134 (Pt 2), 571–584. doi:10.1093/brain/awq337
Wagner, K., Unger, L., Salman, M. M., Kitchen, P., Bill, R. M., and Yool, A. J. (2022). Signaling Mechanisms and Pharmacological Modulators Governing Diverse Aquaporin Functions in Human Health and Disease. Int. J. Mol. Sci. 23 (3), 1388. doi:10.3390/ijms23031388
Waldmann, R., and Lazdunski, M. (1998). H(+)-gated Cation Channels: Neuronal Acid Sensors in the NaC/DEG Family of Ion Channels. Curr. Opin. Neurobiol. 8 (3), 418–424. doi:10.1016/s0959-4388(98)80070-6
Wang, Y. Z., and Xu, T. L. (2011). Acidosis, Acid-Sensing Ion Channels, and Neuronal Cell Death. Mol. Neurobiol. 44 (3), 350–358. doi:10.1007/s12035-011-8204-2
Wang, Y. Z., Wang, J. J., Huang, Y., Liu, F., Zeng, W. Z., Li, Y., et al. (2015). Tissue Acidosis Induces Neuronal Necroptosis via ASIC1a Channel Independent of its Ionic Conduction. ELife 4, e05682. doi:10.7554/eLife.05682
Wang, Y., O'Bryant, Z., Wang, H., and Huang, Y. (2016). Regulating Factors in Acid-Sensing Ion Channel 1a Function. Neurochem. Res. 41 (4), 631–645. doi:10.1007/s11064-015-1768-x
Wang, J. J., Liu, F., Yang, F., Wang, Y. Z., Qi, X., Li, Y., et al. (2020). Disruption of Auto-Inhibition Underlies Conformational Signaling of ASIC1a to Induce Neuronal Necroptosis. Nat. Commun. 11 (1), 475. doi:10.1038/s41467-019-13873-0
Wang, Y., Zhang, J., Jiang, P., Li, K., Sun, Y., and Huang, Y. (2021). ASIC1a Promotes Acidic Microenvironment-Induced HCC Cells Migration and Invasion by Inducing Autophagy. Eur. J. Pharmacol. 907, 174252. doi:10.1016/j.ejphar.2021.174252
Wu, L. J., Duan, B., Mei, Y. D., Gao, J., Chen, J. G., Zhuo, M., et al. (2004). Characterization of Acid-Sensing Ion Channels in Dorsal Horn Neurons of Rat Spinal Cord. J. Biol. Chem. 279 (42), 43716–43724. doi:10.1074/jbc.M403557200
Wu, X., Ren, G., Zhou, R., Ge, J., and Chen, F. H. (2019). The Role of Ca2+ in Acid-Sensing Ion Channel 1a-Mediated Chondrocyte Pyroptosis in Rat Adjuvant Arthritis. Lab. Invest. 99 (4), 499–513. doi:10.1038/s41374-018-0135-3
Xu, Y., and Chen, F. (2020a). Acid-Sensing Ion Channel-1a in Articular Chondrocytes and Synovial Fibroblasts: A Novel Therapeutic Target for Rheumatoid Arthritis. Front. Immunol. 11, 580936. doi:10.3389/fimmu.2020.580936
Xu, Y., and Chen, F. (2020b). Factors and Molecular Mechanisms Influencing the Protein Synthesis, Degradation and Membrane Trafficking of ASIC1a. Front. Cell Dev. Biol. 8, 596304. doi:10.3389/fcell.2020.596304
Xu, Y., Zai, Z., Zhang, T., Wang, L., Qian, X., Xu, D., et al. (2022). Nesfatin-1 Exerts Protective Effects on Acidosis-Stimulated Chondrocytes and Rats with Adjuvant-Induced Arthritis by Inhibiting ASIC1a Expression. Lab. Invest. doi:10.1038/s41374-022-00774-y
Yoder, N., Yoshioka, C., and Gouaux, E. (2018). Gating Mechanisms of Acid-Sensing Ion Channels. Nature 555 (7696), 397–401. doi:10.1038/nature25782
Yu, X. W., Hu, Z. L., Ni, M., Fang, P., Zhang, P. W., Shu, Q., et al. (2015). Acid-sensing Ion Channels Promote the Inflammation and Migration of Cultured Rat Microglia. Glia 63 (3), 483–496. doi:10.1002/glia.22766
Zeng, W. Z., Liu, D. S., and Xu, T. L. (2014). Acid-sensing Ion Channels: Trafficking and Pathophysiology. Channels (Austin) 8 (6), 481–487. doi:10.4161/19336950.2014.958382
Zha, X. M., Wemmie, J. A., Green, S. H., and Welsh, M. J. (2006). Acid-sensing Ion Channel 1a is a Postsynaptic Proton Receptor that Affects the Density of Dendritic Spines. Proc. Natl. Acad. Sci. U. S. A. 103 (44), 16556–16561. doi:10.1073/pnas.0608018103
Zhang, Y., Qian, X., Yang, X., Niu, R., Song, S., Zhu, F., et al. (2020). ASIC1a Induces Synovial Inflammation via the Ca2+/NFATc3/RANTES Pathway. Theranostics 10 (1), 247–264. doi:10.7150/thno.37200
Zhang, X., Zhou, J., Gu, Z., Zhang, H., Gong, Q., and Luo, K. (2021). Advances in Nanomedicines for Diagnosis of Central Nervous System Disorders. Biomaterials 269, 120492. doi:10.1016/j.biomaterials.2020.120492
Zhou, R., Wu, X., Wang, Z., Ge, J., and Chen, F. (2015). Interleukin-6 Enhances Acid-Induced Apoptosis via Upregulating Acid-Sensing Ion Channel 1a Expression and Function in Rat Articular Chondrocytes. Int. Immunopharmacol. 29 (2), 748–760. doi:10.1016/j.intimp.2015.08.044
Zhou, R. P., Dai, B. B., Xie, Y. Y., Wu, X. S., Wang, Z. S., Li, Y., et al. (2018). Interleukin-1β and Tumor Necrosis Factor-α Augment Acidosis-Induced Rat Articular Chondrocyte Apoptosis via Nuclear Factor-kappaB-dependent Upregulation of ASIC1a Channel. Biochim. Biophys. Acta Mol. Basis Dis. 1864 (1), 162–177. doi:10.1016/j.bbadis.2017.10.004
Zhu, Y., Pan, X., Du, N., Li, K., Hu, Y., Wang, L., et al. (2020). ASIC1a Regulates miR-350/SPRY2 by N6 -methyladenosine to Promote Liver Fibrosis. FASEB J. 34 (11), 14371–14388. doi:10.1096/fj.202001337R
Ziemann, A. E., Schnizler, M. K., Albert, G. W., Severson, M. A., Howard, M. A., Welsh, M. J., et al. (2008). Seizure Termination by Acidosis Depends on ASIC1a. Nat. Neurosci. 11 (7), 816–822. doi:10.1038/nn.2132
Keywords: ASIC1a, inflammatory, immune, diseases, target
Citation: Wang Y, Hu X, Sun Y and Huang Y (2022) The Role of ASIC1a in Inflammatory Immune Diseases: A Potential Therapeutic Target. Front. Pharmacol. 13:942209. doi: 10.3389/fphar.2022.942209
Received: 12 May 2022; Accepted: 20 June 2022;
Published: 08 July 2022.
Edited by:
Jian Gao, Shanghai Children’s Medical Center, ChinaReviewed by:
Alexander V. Zholos, Taras Shevchenko National University of Kyiv, UkraineMootaz M. Salman, University of Oxford, United Kingdom
Copyright © 2022 Wang, Hu, Sun and Huang. This is an open-access article distributed under the terms of the Creative Commons Attribution License (CC BY). The use, distribution or reproduction in other forums is permitted, provided the original author(s) and the copyright owner(s) are credited and that the original publication in this journal is cited, in accordance with accepted academic practice. No use, distribution or reproduction is permitted which does not comply with these terms.
*Correspondence: Yancai Sun, MTMzNDkyOTMzNTlAMTYzLmNvbQ==; Yan Huang, YXlkaHlAMTI2LmNvbQ==
†These authors have contributed equally to this work