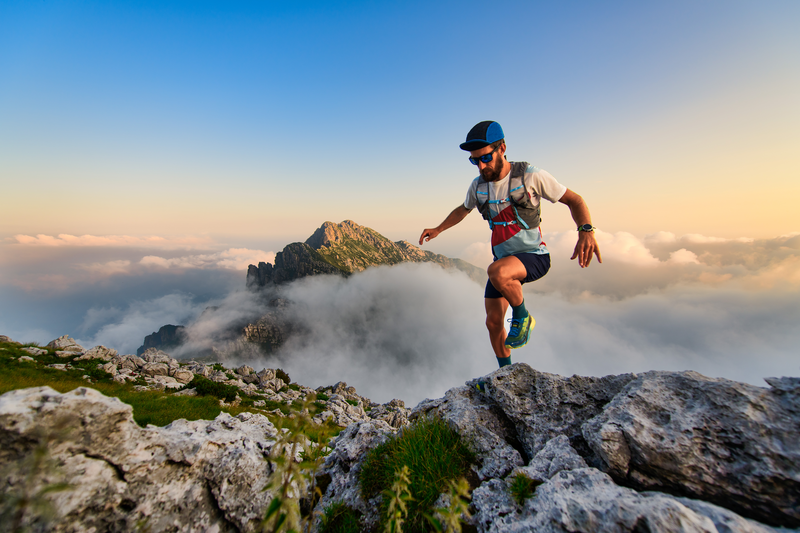
94% of researchers rate our articles as excellent or good
Learn more about the work of our research integrity team to safeguard the quality of each article we publish.
Find out more
ORIGINAL RESEARCH article
Front. Pharmacol. , 25 July 2022
Sec. Experimental Pharmacology and Drug Discovery
Volume 13 - 2022 | https://doi.org/10.3389/fphar.2022.942143
This article is part of the Research Topic The Polysaccharides From Marine Organisms and Fungi: Biological Functions and Molecular Mechanisms View all 6 articles
Porphyran possesses various activities, while the effects of the porphyran from Porphyra haitanensis (PPH) on obesity are rarely reported. In this study, C57BL/6J male mice were fed with HFD combined with PPH gavage (50 mg/kg/d) for 16 weeks, and body weight was measured once a week. After that, serum, adipose, and liver tissues were collected for physiological and biochemical analyses. Our research indicated that PPH treatment alleviated obesity in HFD-fed mice. PPH alleviated fat accumulation in serum, liver, and adipose tissues. In addition, PPH activated the AMPK-HSL/ACC pathway in epididymal adipose tissue to reduce lipid accumulation. Moreover, PPH turned white adipose into brown and activated the PGC 1α-UCP 1-mitochondrial pathway in scapular adipose tissue to generate more heat. Interestingly, PPH regulated colonic microbiota homeostasis in obese mice, including significant elevation of Roseburia and Eubacterium and marked reduction of Helicobacter. Moreover, Spearman’s correlation analysis demonstrated that regulation of gut microbiota can decrease lipid accumulation. In summary, our study illustrated that PPH possesses the potential to be developed as an anti-obesity agent.
Obesity is a growing epidemic worldwide and developed into the most significant contributor to health threats (Ogden et al., 2007). In particular, obesity is closely associated with diabetes, metabolic syndrome, non-alcoholic fatty liver disease (NAFLD), and even cancer (Kopelman, 2000), owing to the toxic effects of ectopic accumulation of lipids (Butler et al., 2006). At present, approaches for obesity treatment include drug modulation, surgery, and diet regulation (Plourde, 2000). However, safe and effective weight loss therapies are still of great need so far.
Recently, dietary polysaccharides from seaweed have been demonstrated to confer positive effects in managing metabolic diseases such as obesity (Ahmadi et al., 2017). Porphyran from Porphyra is a sulfated polysaccharide that comprises cold-water-soluble portion of the cell wall (Zhang et al., 2004). As a functional seaweed polysaccharide, porphyran from various sources exhibits a series of biological activities, including antioxidant (porphyran from Porphyra haitanensis) (Wang et al., 2017), anti-inflammatory (porphyran from Porphyra vietnamensis) (Bhatia et al., 2015), immunomodulatory (porphyran from Porphyra yezoensis) (Wang et al., 2020), anticancer (porphyran from Porphyra haitanensis) (Kwon and Nam, 2007), anti-aging (porphyran from Porphyra haitanensis) (Zhang et al., 2018), hypoglycemic (porphyran from Porphyra haitanensis) (Cao et al., 2016), and hypolipidemic effects (Hoang et al., 2015).
Numerous studies demonstrated that intestinal microbiota can effectively regulate nutrition and energy metabolism (Sommer and Bäckhed, 2013), which has monumental importance for the regulation of obesity and other metabolic diseases (Kallus and Brandt, 2012). A high-fat diet (HFD) induced microbiome dysbiosis with a decrease in bacterial diversity (Kong et al., 2019). Gut microflora dysbiosis and distinct metabolites may induce obesity (Turnbaugh and Gordon, 2009). Indeed, certain compositions of the gut microbiota may predispose to store excess calories in adipose tissue (Bäckhed et al., 2005). Also, we previously reported that porphyran from Porphyra yezoensis–derived oligosaccharides alleviates NAFLD and reshaped the cecal microbiota (Wang et al., 2021). However, effects of porphyran from Porphyra haitanensis (PPH) on alleviating obesity and its effect on intestinal microecology were not clear until now. In present study, we first investigated the anti-obesity function of PPH and its underlying mechanism.
The animal experiment was designed as shown in Figure 1A. After the 16-weeks feeding trial, body weight of the HFD group was markedly elevated when compared with the NCD-fed group (p < 0.001), while PPH administration markedly reversed this adverse situation (p < 0.01) (Figure 1B). HFD induced much more weight gain than the NCD group (p < 0.001) (Figure 1C), while PPH treatment conspicuously prevented body weight gain (p < 0.01) (Figure 1C). Moreover, PPH notably prevented body mass index (BMI) and adiposity index increasement compared with the HFD group (Figures 1D,E) (p < 0.05). The abovementioned results prove that PPH can effectively relieve obesity and decrease adiposity index, which may be beneficial for relieving HFD-induced obesity.
FIGURE 1. PPH alleviated HFD-induced obesity in mice. Schematic diagram of the animal experiment (A); body weight change (B); body weight gain (C); BMI (D), calculated by body weight/height2; adiposity index (E). *p < 0.05, **p < 0.01, and ***p < 0.001.
It has been demonstrated that obesity can exacerbate lipid accumulation in the liver (Prodanović et al., 2016). Histological analysis of the liver tissue was conducted to evaluate the effects of PPH on alleviating hepatic steatosis. We observed that the hepatic lobule structure was clear and complete in the NCD group (Figure 2A). However, the liver tissue was infiltrated with white spots, confirming injury of hepatic cells in the HFD group. Moreover, large areas of necrosis in the central parts of the hepatic lobules were observed, which were characterized by dissolution and disappearance of the nuclei in the HFD group (Figure 2A). After PPH treatment, the abovementioned state was markedly reversed compared with the HFD group and tended to be normal (Figure 2A). Moreover, Oil Red O staining demonstrated that excess lipid accumulation was observed in the HFD group, while PPH treatment significantly decreased the lipid accumulation (p < 0.01) (Figure 2B), free fat acid (FFA) (p < 0.01) (Figure 2C), and the liver index (p < 0.01) (Figure 2D). In general, the abovementioned results indicate that PPH can suppress lipid accumulation in liver to produce an anti-obesity effect.
FIGURE 2. PPH decreased lipid accumulation in liver. Hematoxylin and eosin (H,E) staining (A); Oil red O staining (B); FFA (C); liver index (D), calculated by liver weight (mg)/body weight. *p < 0.05, **p < 0.01, and ***p < 0.001.
vWhite adipose tissue (WAT) distribution markedly affects metabolic disease, and increased abdominal and visceral WAT is associated with elevated danger of obesity (Cummins et al., 2014). The major role of WAT is to store superfluous cholesterol and triglycerides (TGs), which is closely related to obesity (Vázquez-Vela et al., 2008). As shown in Figure 3A, the size of the fat bubble was significantly decreased in HFD mice treated with PPH. In addition, PPH intake markedly reduced the epididymal and perirenal WAT weights (Figures 3B,C) (p < 0.001) compared with the HFD group. The abovementioned findings indicate that PPH can alleviate obesity by reducing WAT fat accumulation.
FIGURE 3. PPH decreased fat accumulation in WAT. H&E staining of epididymal WAT (A); epididymal (B) and perirenal WAT weights (C). *p < 0.05, **p < 0.01, and ***p < 0.001.
Activated adenosine 5‘-monophosphate-activated protein kinase (AMPK) can limit hepatic triglyceride accumulation (O’Neill et al., 2013). Thus, we further analyzed whether PPH administration could regulate WAT fat metabolism via the AMPK signaling pathway. As shown in Figure 4, the level of pAMPK was markedly reduced in HFD-fed mice compared with the NCD-fed mice (p < 0.001). Meanwhile, PPH gavage administration notably increased the activity of AMPK and regulated its downstream proteins, including increasing the levels of phosphorylated hormone-sensitive lipase (pHSL) and phosphorylated acetyl-CoA carboxylase (pACC) (p < 0.01). Thus, supplementation of PPH in obese mice can effectively decrease lipid accumulation in WAT by activating the AMPK-HSL/ACC signaling pathway.
FIGURE 4. Effects of PPH on activating AMPK signaling pathway in WAT. Western blotting analyses of pAMPK, pHSL, and pACC protein expression in WAT (A); quantification of the pAMPK/AMPK, pHSL/HSL, and pACC/ACC ratios (B). *p < 0.05, **p < 0.01, and ***p < 0.001.
Scapular adipose mainly comprises brown adipose tissue (BAT) in the lean population, and the thermogenic capacity of scapular fat makes it an attractive therapeutic target for inducing weight loss through energy expenditure (Cypess and Kahn, 2010). The main role of scapular fat is to convert stored energy into heat, which is closely related to obesity (Himms-Hagen, 1989). As shown in Figure 5A, the fat bubble size was notably increased (p < 0.001), and the scapular adipose tissue turned into WAT in HFD mice, while PPH treatment notably diminished the fat bubble size (p < 0.01) and induced a white-to-brown fat conversion. In addition, PPH intake markedly reduced the scapular fat weight (p < 0.05) compared with the HFD-fed mice (Figure 5B). The abovementioned results indicated that PPH can play an anti-obesity role by effectively conducting white-to-brown fat conversion in HFD-fed mice.
FIGURE 5. PPH alleviated scapular fat turned into WAT conversion in scapula. H&E staining of scapular adipose tissue (A) and scapular adipose tissue weight (B). *p < 0.05, **p < 0.01, and ***p < 0.001.
Scapular adipose tissue is rich in mitochondria, which are the energy factories of cells and can easily burn fat to release heat (Zingaretti et al., 2009). Mitochondria in brown adipose tissue produce more heat. Uncoupling protein 1 (UCP1) is rich in mitochondria and is a key to carry out mitochondrial thermogenesis (Muraoka et al., 2009). As shown in Figure 6A, PPH administration increased the expression of peroxisome proliferator–activated receptor coactivator 1α (PGC 1α) (p < 0.001) and its downstream target protein UCP 1 (p < 0.001) compared with the HFD feeding mice. In addition, PPH treatment increased the expression of mitochondrial respiratory chain complexes I (p < 0.05), II (p < 0.01), III (p < 0.01), and IV (p < 0.001) compared with the HFD one, realizing the effect of increasing heat production. And finally, PPH reduces the accumulation of fat in BAT, which has an effect on weight loss.
FIGURE 6. Effects of PPH on activating the mitochondrion-related signaling pathway in scapular adipose tissue. Western blotting analyses of PGC 1α, UCP 1, and mitochondria complex protein expression (A); Quantification of the PGC 1α/β-Tubulin ratio, UCP 1/β-Tubulin ratio, and mitochondria complex/β-Tubulin ratio (B). CI: complex I; CII: complex II; CIII: complex III; CIV: complex IV; CV: complex V. *p < 0.05, **p < 0.01, and ***p < 0.001.
Obesity can significantly exacerbate the progression of hyperlipidemia (Sullivan et al., 2008), which is a primary health risk factor induced by obesity (Charlton, 2009). HFD can markedly elevated the contents of TG (p < 0.05) and TC (p < 0.01) in serum compared with the NCD group (Figures 7A,B). After PPH treatment, the TG and TC levels in serum were notably lower than those in the HFD group (p < 0.01) (Figures 7A,B). Interestingly, LDL-C decreased, while HDL-C increased in serum after the PPH treatment compared with the HFD one (Figures 7C,D) (p < 0.01). In short, the abovementioned data indicate that PPH attenuates serological lipid accumulation in obese mice.
FIGURE 7. Effects of PPH on serum lipids in obese mice. Serum triglyceride (TG) (A), total cholesterol (TC) (B), low-density lipoprotein cholesterol (LDL-C) (C), and high-density lipoprotein cholesterol (HDL-C) (D) levels. *p < 0.05, **p < 0.01.
Obesity was characterized by gut microbiota disorder (DiBaise et al., 2008). Also, we demonstrated the effects of PPH on colonic microbiota homeostasis in HFD-fed mice. PCoA analysis indicated that HFD-fed one can markedly change the microbiota composition in the colon compared with the NCD-fed one, which was completely separated (Figure 8A). However, PPH treatment markedly altered the colonic microbiota distribution in mice with HFD feeding, which demonstrated that PPH can be remodeling the microbiota community in the colon (Figure 8A). In addition, the PPH treatment significantly altered the microbiota component at the phylum (e.g., increasing the relative abundances of Bacteroidetes, Proteobacteria, and Verrucomicrobia, while decreasing Firmicutes, Figures 8C–F) and genus level (e.g., increasing the relative abundances of Bacteroides and Alistipes, while decreasing Helicobacter, Figures 8H–J) (Figures 8B,C), which were more similar to that of the NCD group. Altogether, these data demonstrate that colonic micro-ecosystem in obese mice can be effectively regulated by PPH treatment.
FIGURE 8. Effects of PPH on regulating colonic microbiota in obese mice. PCoA plot of colonic microbiota based on a weighted UniFrac metric (A); effects of PPH on the relative abundance of gut microbiota at the phylum level (B), such as Bacteroidetes (C), Firmicutes (D), Proteobacteria (E), and Verrucomicrobia (F); Effects of PPH on the relative abundance of gut microbiota at the genus level (G), such as Bacteroides (H), Alistipes (I), and Helicobacter (J). *p < 0.05, **p < 0.01, and ***p < 0.001.
To clarify the effects of PPH supplement on the regulation of bacterial taxa in the colon, LEfSe analysis was conducted between the HFD and PPH groups. It indicated that PPH decreased the level of Firmicutes, including Clostridium XIV, Veillonella, and Helicobacter, while notably increasing Bacteroidetes, including Alloprevotella, Roseburia, Corynebacterium, and Alistipes (Figures 9A,B). In addition, PPH treatment increased the relative abundance of Corynebacteriaceae, Porphyromonadaceae, and Rikenellacea, while a reduction in Veillonellaceae and Desulfovibrionaceae was observed. Moreover, obese mice had a conspicuously elevated abundance of Proteobacteria and Deferribacteres at the phylum level compared with the PPH-treated mice (Figure 9 A and B). In addition, Spearman’s correlation analysis was applied to clarify the relationship between physiological index and bacterial abundance (Figure 9C). At the genus level, Roseburia and Eubacterium were positively related to HDL-C levels while negatively related to TC, TG, and LDL-C levels. Meanwhile, the effects of Helicobacter were opposite. More importantly, PPH triggered an increase in the relative abundances of Roseburia and Eubacterium in obese mice, while decreasing the abundances of Helicobacter. The abovementioned results showed that alteration in the gut microbiota composition after PPH gavage brings about a lipid level decrease in obese mice.
FIGURE 9. Effects of PPH treatment on phylotypes of colonic microbiota and its correlation with physiological parameters. LEfSe comparison of colonic microbiota between the HFD and the PPH group: Taxa enriched in the HFD group (dark blue) and PPH group (orange) (A); only those meeting the LDA score threshold >2 are listed (B). Heat map of Spearman’s correlations between colonic bacteria and TC, LDL-C, TG, and HDL-C (C). Correlation between biomarkers and microbial species was visualized by color depth: the darker the color, the higher the relevance. + p < 0.05 and *p < 0.01.
Effects of the water-soluble polysaccharide from Porphyra haitanensis on alleviating obesity were rarely reported. This current study demonstrated that PPH markedly ameliorated HFD-induced obesity, mainly by alleviating lipid accumulation in WAT, scapular adipose tissue, liver, and serum. Moreover, PPH can effectively regulate colonic microbiota dysbiosis, which is related to alleviation of obesity.
AMPK can maintain energy homeostasis, which is regarded as a considerable energy sensor (Horman et al., 2002). Activated AMPK reduces lipid synthesis and accelerates fatty acid oxidation by manipulating its downstream targets such as HMG-CoA reductase and ACC (Lee et al., 2006; Srivastava et al., 2012). In this study, PPH-treatment activated the AMPK-HSL/ACC signaling pathway in WAT compared with the HFD group, thus reducing fat accumulation in WAT.
It has been confirmed that BAT activity is inversely related to BMI and body fat percentage (van Marken Lichtenbelt et al., 2009). BAT is becoming a new target for anti-obesity therapies focusing on increasing thermogenesis (Cypess and Kahn, 2010). PGC 1α and its downstream target UCP-1 in BAT can be activated to modulate mitochondrial and metabolic homeostasis (Golozoubova et al., 2001; Puigserver and Spiegelman, 2003). The impairment of signaling involved in the regulation of PGC 1α causes obesity (Crunkhorn et al., 2007). Also, dietary ingredients have been demonstrated to stimulate BAT function. We confirmed that PPH activated the PGC 1α-UCP 1 signaling pathway and increased the mitochondrial complex activities, which resulted in a dramatic increase in heat production and induced a white-to-brown fat conversion in the scapular fat of obese mice.
Furthermore, gut microbiota served as a vital regulator of lipid metabolism (Sonnenburg and Bäckhed, 2016). Intestinal microecology of the obese population showed decreased Bacteroidetes/Firmicutes ratio, indicating that various gut microbiota compositions can affect energy metabolism (DiBaise et al., 2008). Moreover, fecal microbiota transplantation from obese donors increased body weight gain and BMI (Turnbaugh et al., 2009), which indicated a causal association between microbiota and obesity outcomes (Liou et al., 2013). Interestingly, PPH regulated colonic microbiota homeostasis in obese mice. PPH reduced the level of Firmicutes, including Helicobacte, Veillonella, and Clostridium XIV, while remarkedly elevating Bacteroidetes, such as Alloprevotella, Roseburia, Corynebacterium, and Alistipes. In addition, Spearman’s correlation analysis indicated that colonic microbiota alteration could decrease lipid accumulation. In general, our present study strengthens the linkage between obesity and gut dysbiosis and illustrated that porphyran from Porphyra haitanensis possess the potential to be developed as an anti-obesity agent.
PPH was isolated from cold-water-soluble extracts of Porphyra haitanensis as previously described (Qiu et al., 2021). In brief, the algae were powdered and treated with 50% ethanol solution to remove excess lipid and then water-soluble polysaccharides were extracted with water [1.5% (w/v)] at room temperature for 10 h. After centrifugation, the supernatant was mixed with double volumes of ethanol. After centrifugation, the precipitate was vacuum-dried to get the PPH.
Animal experiments were conducted as shown in Figure 1A. Briefly, 8-week-old SPF C57BL/6J mice were purchased from the Gempharmatech Co., Ltd (Nanjing, China). They were raised in individually ventilated cages, and diet was provided ad libitum. Mice were divided into three groups (10 per group) after 1 week of acclimation. Mice in the NCD group were fed a normal chow diet (D12450B, Research Diets Inc., New Brunswick, NJ, United States), while the HFD and the PPH groups were fed a high-fat diet (HFD) (D12492, Research Diets Inc., New Brunswick, NJ, United States) for 16 weeks to induce obesity. Meanwhile, PPH group received 50 mg/kg/d PPH by gavage, while NCD and HFD groups were given aliquots of phosphate-buffered saline (PBS). At end of the trial, mice were euthanized after starving overnight. Blood was collected and then centrifuged at 2,000 g for 15 m to obtain serum.
Liver tissues, epididymal fat, perirenal fat, and scapular fat tissues were collected and weighed. Tissues were stored for Oil Red O staining and Western blot analysis at −80°C. Partial tissues were fixed in 4% paraformaldehyde solution immediately. After dehydration and embedding, tissues were cut into 4-μm-thick sections and analyzed by H&E or Oil Red O (Wang et al., 2021; Wang et al., 2022). Quantity was analyzed using ImageJ software.
TC, TG, FFA, LDL-C, and HDL-C levels were measured by corresponding kits according to the manufacturer’s instructions (F001-1-1, F002-1-1, A042-2-1, A112-1-1, and A113-1-1; Jiancheng Bioengineering Institute, Nanjing, China).
Proteins of adipose tissues were extracted with the Minute Total Protein Extraction Kit (SD-001, Invent Biotechnologies, Eden Prairie, MN, United States). After denaturation with loading buffer at 100°C for 10 m, 40 μg proteins were loaded on 10% SDS-PAGE and then transferred to PVDF membranes and then incubated with primary antibodies directed against AMPKα (2532, CST, Danvers, MA, United States), pAMPK (2531, CST), ACC (3662, CST), pACC (11818, CST), HSL (18381, CST), pHSL (45804, CST), UCP 1 (72298, CST), PGC 1α (2178, CST), and β-Tubulin (2146, CST) at 4°C overnight, while Total OXPHOS Rodent WB antibody Cocktail (ab110413, Abcam, Cambridge, United Kingdom) was used to analyze the relative levels of OXPHOS complexes. After washing with TBST, membranes were incubated with secondary antibodies at room temperature for another 2 h. The Omni-ECL™Femto Light Chemiluminescence Kit (SQ201, Epizyme Biomedical Technology Co., Ltd., Shanghai, China) was used to visualize protein bands. In addition, membranes were stripped with Minute WB stripping solution (WA-003, Invent Biotechnologies, Eden Prairie, MN, United States). ImageJ software was used for densitometry analysis.
The QIAamp DNA Stool Mini Kit (51604, Qiagen, Hilden, Germany) was used to extract bacterial DNA from colonic contents. Then, universal primers (341F and 806R) were utilized to amplify the bacterial 16S rDNA gene. Then, amplicons were sequenced on an Illumina Miseq PE250 by Biozeron (Biozeron, Shanghai, China), and OTUs were generated by clustering at 3% difference. In addition, microbial taxa differences between various groups were characterized by linear discriminant analysis (LDA) effect size (LEfSe). Heat maps were analyzed using R Cytoscape, which showed the relationship between physiological index and colonic gut microbiota.
Differences between groups were analyzed by GraphPad Prism (GraphPad Software Inc., San Diego, CA, United States). Data are presented as the mean ± standard error of the mean (SEM). Also, one-way ANOVA was applied to analyze multiple comparisons. The difference was considered to be statistically significant while p < 0.05.
The datasets presented in this study can be found in online repositories. The names of the repository/repositories and accession number(s) can be found below: https://www.ncbi.nlm.nih.gov/sra/PRJNA836424.
The animal study was reviewed and approved by Experimental Animal Care and Use Committee of Sun Yat-Sen University.
XW conducted the research and wrote the manuscript. XW, JD, YF, WL, and ML analyzed the data. Xueliang, JD, LX, and WS contributed to manuscript revision. YF, WL, and ML helped with animal experiment. WS and XL designed and directed the project.
This research was funded by Project funded by the China Postdoctoral Science Foundation (2021M703684), Guangdong Basic and Applied Basic Research Foundation (2021A1515110355), and the Special Project of Zhejiang Ocean University (2022C41014).
The authors declare that the research was conducted in the absence of any commercial or financial relationships that could be construed as a potential conflict of interest.
All claims expressed in this article are solely those of the authors and do not necessarily represent those of their affiliated organizations, or those of the publisher, the editors, and the reviewers. Any product that may be evaluated in this article, or claim that may be made by its manufacturer, is not guaranteed or endorsed by the publisher.
PPH, porphyran from Porphyra haitanensis; PBS, phosphate-buffered saline; HFD, high-fat diet; NAFLD, non-alcoholic fatty liver disease; NCD, normal chow diet; WAT, white adipose tissue; BAT, brown adipose tissue; BMI, body mass index; TG, triglyceride; FFA, free fatty acid; TC, total cholesterol; HDL-C, high-density lipoprotein cholesterol; LDL-C, low-density lipoprotein cholesterol; H&E, hematoxylin, and eosin; AMPK, 5‘-monophosphate-activated protein kinase; HSL, hormone-sensitive lipase; ACC, acetyl-CoA carboxylase; PGC 1α, peroxisome proliferator–activated receptor coactivator 1α; UCP 1, uncoupling protein 1; LDA, linear discriminant analysis; LEfSe, linear discriminant analysis effect size.
Ahmadi, S., Mainali, R., Nagpal, R., Sheikh-Zeinoddin, M., Soleimanian-Zad, S., Wang, S., et al. (2017). Dietary Polysaccharides in the Amelioration of Gut Microbiome Dysbiosis and Metabolic Diseases. Obes. Control Ther. 4 (3). 00140, doi:10.15226/2374-8354/4/2/00140
Bäckhed, F., Ley, R. E., Sonnenburg, J. L., Peterson, D. A., and Gordon, J. I. (2005). Host-bacterial Mutualism in the Human Intestine. science 307 (5717), 1915–1920. doi:10.1126/science.1104816
Bhatia, S., Sharma, K., Sharma, A., Nagpal, K., and Bera, T. (2015). Anti-inflammatory, Analgesic and Antiulcer Properties of Porphyra Vietnamensis. Avicenna J. Phytomed 5 (1), 69–77.
Butler, J., Rodondi, N., Zhu, Y., Figaro, K., Fazio, S., Vaughan, D. E., et al. (2006). Metabolic Syndrome and the Risk of Cardiovascular Disease in Older Adults. J. Am. Coll. Cardiol. 47 (8), 1595–1602. doi:10.1016/j.jacc.2005.12.046
Cao, C., Chen, M., Liang, B., Xu, J., Ye, T., and Xia, Z. (2016). Hypoglycemic Effect of Abandoned Porphyra Haitanensis Polysaccharides in Alloxan-Induced Diabetic Mice. Bioact. carbohydrates Diet. fibre 8 (1), 1–6. doi:10.1016/j.bcdf.2016.05.001
Charlton, M. (2009). Obesity, Hyperlipidemia, and Metabolic Syndrome. Liver Transpl. 15 (S2), S83–S89. doi:10.1002/lt.21914
Crunkhorn, S., Dearie, F., Mantzoros, C., Gami, H., Da Silva, W. S., Espinoza, D., et al. (2007). Peroxisome Proliferator Activator Receptor Gamma Coactivator-1 Expression Is Reduced in Obesity: Potential Pathogenic Role of Saturated Fatty Acids and P38 Mitogen-Activated Protein Kinase Activation. J. Biol. Chem. 282 (21), 15439–15450. doi:10.1074/jbc.M611214200
Cummins, T. D., Holden, C. R., Sansbury, B. E., Gibb, A. A., Shah, J., Zafar, N., et al. (2014). Metabolic Remodeling of White Adipose Tissue in Obesity. Am. J. Physiol. Endocrinol. Metab. 307 (3), E262–E277. doi:10.1152/ajpendo.00271.2013
Cypess, A. M., and Kahn, C. R. (2010). Brown Fat as a Therapy for Obesity and Diabetes. Curr. Opin. Endocrinol. Diabetes Obes. 17 (2), 143–149. doi:10.1097/MED.0b013e328337a81f
DiBaise, J. K., Zhang, H., Crowell, M. D., Krajmalnik-Brown, R., Decker, G. A., and Rittmann, B. E. (2008). Gut Microbiota and its Possible Relationship with Obesity. Mayo Clin. Proc., 83, 460–469. doi:10.4065/83.4.460
Golozoubova, V., Hohtola, E., Matthias, A., Jacobsson, A., Cannon, B., and Nedergaard, J. (2001). Only UCP1 Can Mediate Adaptive Nonshivering Thermogenesis in the Cold. FASEB J. 15 (11), 2048–2050. doi:10.1096/fj.00-0536fje
Himms-Hagen, J. (1989). Brown Adipose Tissue Thermogenesis and Obesity. Prog. Lipid Res. 28 (2), 67–115. doi:10.1016/0163-7827(89)90009-x
Hoang, M. H., Kim, J.-Y., Lee, J. H., You, S., and Lee, S.-J. (2015). Antioxidative, Hypolipidemic, and Anti-inflammatory Activities of Sulfated Polysaccharides from Monostroma Nitidum. Food Sci. Biotechnol. 24 (1), 199–205. doi:10.1007/s10068-015-0027-x
Horman, S., Browne, G., Krause, U., Patel, J., Vertommen, D., Bertrand, L., et al. (2002). Activation of AMP-Activated Protein Kinase Leads to the Phosphorylation of Elongation Factor 2 and an Inhibition of Protein Synthesis. Curr. Biol. 12 (16), 1419–1423. doi:10.1016/s0960-9822(02)01077-1
Kallus, S. J., and Brandt, L. J. (2012). The Intestinal Microbiota and Obesity. J. Clin. Gastroenterol. 46 (1), 16–24. doi:10.1097/MCG.0b013e31823711fd
Kong, C., Gao, R., Yan, X., Huang, L., and Qin, H. (2019). Probiotics Improve Gut Microbiota Dysbiosis in Obese Mice Fed a High-Fat or High-Sucrose Diet. Nutrition 60, 175–184. doi:10.1016/j.nut.2018.10.002
Kopelman, P. G. (2000). Obesity as a Medical Problem. Nature 404 (6778), 635–643. doi:10.1038/35007508
Kwon, M-J., and Nam, T-J. (2007). Chromatographically Purified Porphyran from Porphyra Yezoensis Effectively Inhibits Proliferation of Human Cancer Cells. Food Sci. Biotechnol. 16 (6), 873–878.
Lee, W. J., Kim, M., Park, H. S., Kim, H. S., Jeon, M. J., Oh, K. S., et al. (2006). AMPK Activation Increases Fatty Acid Oxidation in Skeletal Muscle by Activating PPARalpha and PGC-1. Biochem. Biophys. Res. Commun. 340 (1), 291–295. doi:10.1016/j.bbrc.2005.12.011
Liou, A. P., Paziuk, M., Luevano, J. M., Machineni, S., Turnbaugh, P. J., and Kaplan, L. M. (2013). Conserved Shifts in the Gut Microbiota Due to Gastric Bypass Reduce Host Weight and Adiposity. Sci. Transl. Med. 5 (178), 178ra41. doi:10.1126/scitranslmed.3005687
Muraoka, M., Fukushima, A., Viengchareun, S., Lombès, M., Kishi, F., Miyauchi, A., et al. (2009). Involvement of SIK2/TORC2 Signaling Cascade in the Regulation of Insulin-Induced PGC-1alpha and UCP-1 Gene Expression in Brown Adipocytes. Am. J. Physiol. Endocrinol. Metab. 296 (6), E1430–E1439. doi:10.1152/ajpendo.00024.2009
Ogden, C. L., Yanovski, S. Z., Carroll, M. D., and Flegal, K. M. (2007). The Epidemiology of Obesity. Gastroenterology 132 (6), 2087–2102. doi:10.1053/j.gastro.2007.03.052
O’Neill, H. M., Holloway, G. P., and Steinberg, G. R. (2013). AMPK Regulation of Fatty Acid Metabolism and Mitochondrial Biogenesis: Implications for Obesity. Mol. Cell. Endocrinol. 366 (2), 135–151. doi:10.1016/j.mce.2012.06.019
Plourde, G. (2000). Treating Obesity. Lost Cause or New Opportunity? Can. Fam. Physician 46 (9), 1806–1813.
Prodanović, R., Korićanac, G., Vujanac, I., Djordjević, A., Pantelić, M., Romić, S., et al. (2016). Obesity-driven Prepartal Hepatic Lipid Accumulation in Dairy Cows Is Associated with Increased CD36 and SREBP-1 Expression. Res. Vet. Sci. 107, 16–19. doi:10.1016/j.rvsc.2016.04.007
Puigserver, P., and Spiegelman, B. M. (2003). Peroxisome Proliferator-Activated Receptor-γ Coactivator 1α (PGC-1α): Transcriptional Coactivator and Metabolic Regulator. Endocr. Rev. 24 (1), 78–90. doi:10.1210/er.2002-0012
Qiu, Y., Jiang, H., Fu, L., Ci, F., and Mao, X. (2021). Porphyran and Oligo-Porphyran Originating from Red Algae Porphyra: Preparation, Biological Activities, and Potential Applications. Food Chem. 349 (5), 129209. doi:10.1016/j.foodchem.2021.129209
Sommer, F., and Bäckhed, F. (2013). The Gut Microbiota-Mmasters of Host Development and Physiology. Nat. Rev. Microbiol. 11 (4), 227–238. doi:10.1038/nrmicro2974
Sonnenburg, J. L., and Bäckhed, F. (2016). Diet-microbiota Interactions as Moderators of Human Metabolism. Nature 535 (7610), 56–64. doi:10.1038/nature18846
Srivastava, R. A., Pinkosky, S. L., Filippov, S., Hanselman, J. C., Cramer, C. T., and Newton, R. S. (2012). AMP-Activated Protein Kinase: an Emerging Drug Target to Regulate Imbalances in Lipid and Carbohydrate Metabolism to Treat Cardio-Metabolic Diseases. J. Lipid Res. 53 (12), 2490–2514. doi:10.1194/jlr.R025882
Sullivan, P. W., Ghushchyan, V. H., and Ben-Joseph, R. (2008). The Impact of Obesity on Diabetes, Hyperlipidemia and Hypertension in the United States. Qual. Life Res. 17 (8), 1063–1071. doi:10.1007/s11136-008-9385-7
Turnbaugh, P. J., and Gordon, J. I. (2009). The Core Gut Microbiome, Energy Balance and Obesity. J. Physiol. 587 (17), 4153–4158. doi:10.1113/jphysiol.2009.174136
Turnbaugh, P. J., Ridaura, V. K., Faith, J. J., Rey, F. E., Knight, R., and Gordon, J. I. (2009). The Effect of Diet on the Human Gut Microbiome: a Metagenomic Analysis in Humanized Gnotobiotic Mice. Sci. Transl. Med. 1 (6), 6ra14–16ra14. doi:10.1126/scitranslmed.3000322
van Marken Lichtenbelt, W. D., Vanhommerig, J. W., Smulders, N. M., Drossaerts, J. M., Kemerink, G. J., Bouvy, N. D., et al. (2009). Cold-activated Brown Adipose Tissue in Healthy Men. N. Engl. J. Med. 360 (15), 1500–1508. doi:10.1056/NEJMoa0808718
Vázquez-Vela, M. E., Torres, N., and Tovar, A. R. (2008). White Adipose Tissue as Endocrine Organ and its Role in Obesity. Arch. Med. Res. 39 (8), 715–728. doi:10.1016/j.arcmed.2008.09.005
Wang, X., Li, W., Xiao, L., Liu, C., Qi, H., and Zhang, Z. (2017). In Vivo antihyperlipidemic and Antioxidant Activity of Porphyran in Hyperlipidemic Mice. Carbohydr. Polym. 174, 417–420. doi:10.1016/j.carbpol.2017.06.040
Wang, X., Liu, D., Wang, Z., Cai, C., Jiang, H., and Yu, G. (2021). Porphyran-derived Oligosaccharides Alleviate NAFLD and Related Cecal Microbiota Dysbiosis in Mice. FASEB J. 35 (6), e21458. doi:10.1096/fj.202000763RRR
Wang, X., Wang, Z., Liu, D., Jiang, H., Cai, C., Li, G., et al. (2022). Canagliflozin Prevents Lipid Accumulation, Mitochondrial Dysfunction, and Gut Microbiota Dysbiosis in Mice with Diabetic Cardiovascular Disease. Front. Pharmacol. 13, 839640. doi:10.3389/fphar.2022.839640
Wang, Y., Hwang, J., Yadav, D., Oda, T., Lee, P. C., and Jin, J. O. (2020). Inhibitory Effect of Porphyran on Lipopolysaccharide-Induced Activation of Human Immune Cells. Carbohydr. Polym. 232, 115811. doi:10.1016/j.carbpol.2019.115811
Zhang, Q., Li, N., Liu, X., Zhao, Z., Li, Z., and Xu, Z. (2004). The Structure of a Sulfated Galactan from Porphyra Haitanensis and its In Vivo Antioxidant Activity. Carbohydr. Res. 339 (1), 105–111. doi:10.1016/j.carres.2003.09.015
Zhang, Z., Wang, X., Su, H., Pan, Y., Han, J., Zhang, T., et al. (2018). Effect of Sulfated Galactan from Porphyra Haitanensis on H2O2-Induced Premature Senescence in WI-38 Cells. Int. J. Biol. Macromol. 106, 1235–1239. doi:10.1016/j.ijbiomac.2017.08.123
Keywords: signaling pathway, lipid accumulation, colonic microbiota, obesity, porphyran from Porphyra haitanensis
Citation: Wang X, Dong J, Liang W, Fang Y, Liang M, Xu L, Sun W and Li X (2022) Porphyran From Porphyra haitanensis Alleviates Obesity by Reducing Lipid Accumulation and Modulating gut Microbiota Homeostasis. Front. Pharmacol. 13:942143. doi: 10.3389/fphar.2022.942143
Received: 12 May 2022; Accepted: 16 June 2022;
Published: 25 July 2022.
Edited by:
Zhong Liu, Jinan University, ChinaCopyright © 2022 Wang, Dong, Liang, Fang, Liang, Xu, Sun and Li. This is an open-access article distributed under the terms of the Creative Commons Attribution License (CC BY). The use, distribution or reproduction in other forums is permitted, provided the original author(s) and the copyright owner(s) are credited and that the original publication in this journal is cited, in accordance with accepted academic practice. No use, distribution or reproduction is permitted which does not comply with these terms.
*Correspondence: Wuyang Sun, c3Vud3lAempvdS5lZHUuY24=; Xiaoxing Li, bGl4aWFveDIzQG1haWwuc3lzdS5lZHUuY24=
†These authors have contributed equally to this work and share first authorship
Disclaimer: All claims expressed in this article are solely those of the authors and do not necessarily represent those of their affiliated organizations, or those of the publisher, the editors and the reviewers. Any product that may be evaluated in this article or claim that may be made by its manufacturer is not guaranteed or endorsed by the publisher.
Research integrity at Frontiers
Learn more about the work of our research integrity team to safeguard the quality of each article we publish.